- 1State Key Laboratory of Biobased Material and Green Papermaking, School of Bioengineering, Qilu University of Technology (Shandong Academy of Sciences), Jinan, China
- 2Institute of Food and Nutrition Development, Ministry of Agriculture and Rural Affairs, Beijing, China
- 3Ultrasound Department of Zhucheng People's Hospital, Weifang, China
- 4Shandong Asia-Pacific Haihua Biotechnology Co., Ltd., Jinan, China
Humic acids (HAs) are natural polymers with diverse functional groups that have been documented and utilized in traditional Chinese medicine. Dextran sulfate sodium (DSS)-induced colitis has been used as a model to study inflammatory bowel disease. In this research, we investigate the effect of HAs on ameliorating DSS-induced colitis in mice. Our aim here was to investigate if HAs could be a remedy against colitis and the mechanisms involved. The results show that HAs facilitated a regain of body weight and restoration of intestinal morphology after DSS-induced colitis. HAs treatment alters the community of gut microbiota with more Lactobacillus and Bifidobacterium. Changes in bacterial community result in lower amounts of lipopolysaccharides in mouse sera, as well as lower levels of inflammatory cytokines through the Toll-like receptor 4 (TLR4)-NF-κB pathway. HAs also promoted the expression of tight junction proteins, which protect the intestinal barrier from DSS damage. Cell experiments show that HAs display an inhibitory effect on DSS growth as well. These results suggest that HAs can alleviate colitis by regulating intestinal microbiota, reducing inflammation, maintaining mucosal barriers, and inhibiting pathogen growth. Thus, HAs offer great potential for the prevention and treatment of colitis.
1. Introduction
Diarrhea and colitis caused by bacterial infection are common diseases in both humans and animals (Hauck et al., 2016; Sun et al., 2022; Jia et al., 2023). Antibiotics have been widely used to treat bacteria-induced colitis. In addition, antibiotics have been exploited to promote animal growth and reduce production costs in livestock breeding. Microbial resistance caused by the misuse of antibiotics has become a serious problem globally (Rivera-Sánchez et al., 2022; Cui et al., 2023). Therefore, it is necessary to explore alternatives to antibiotics for treating colitis.
Humic acids (HAs) are macromolecular organic substances rich in hydroxyl, carboxyl, and other groups (Figure 1A), which are formed from plant and microbial residues through humification (Sarlaki et al., 2019; Xu et al., 2022). In Chinese traditional medicine, HAs have been called “WU-JIN-SAN” (乌金散) since 1786 (Miao et al., 2018). HAs provide molecules with antibacterial, anti-inflammatory, antioxidant, and immunomodulatory functions (Mudronová et al., 2021). Moreover, HAs have been used to treat various diseases, including diarrhea, gastritis, gastric ulcers, and colitis (Rusliandi et al., 2020; Socol, 2023).
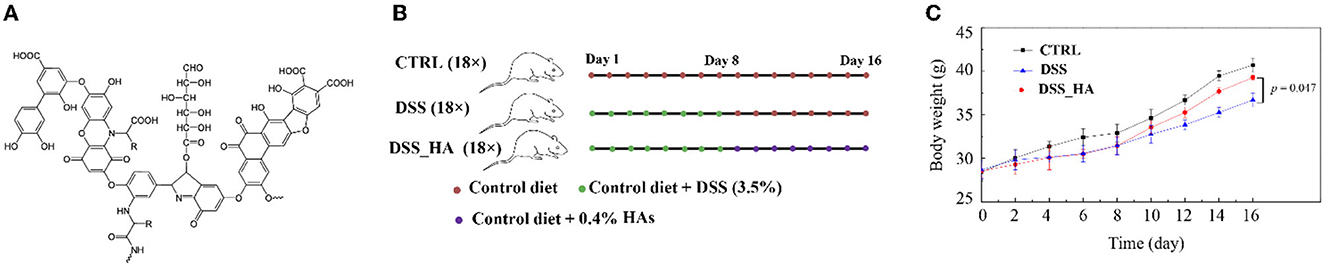
Figure 1. Effect of HAs on colitisin mice. (A) Chemical structure of HAs. (B) Study design for the three mouse cohorts (n = 18 × 3). The three cohorts were fed a control diet (CTRL), a diet containing DSS (DSS), and a diet containing HAs (DSS_HAs) as indicated. (C) Body weight gain in mice of the three cohorts.
Previous studies have shown that adding HAs and lincomycin to the diet can improve the growth performance, nutrient digestibility, blood biochemistry, and intestinal morphology of broiler chickens infected with Clostridium (Saleh et al., 2022). Adding 10% HAs can significantly increase the body weight and feed utilization of finishing pigs (Wang et al., 2008). As far as we know, there are no other studies evaluating the effect of HAs on DSS-induced colitis in mice. The purpose of this study was to evaluate the effects of adding HAs on growth performance, intestinal morphology, intestinal flora, and inflammatory pathway of mice exposed to DSS. The results showed that HAs were helpful in the recovery of body weight and intestinal morphology after colitis. HAs can alleviate colitis by regulating intestinal microflora, reducing inflammation, and maintaining the mucosal barrier. Therefore, HAs have great potential in the prevention and treatment of colitis.
2. Materials and methods
2.1. Ethics statement
All animal experiments followed the protocols approved by the Animal Care and Use Committee of the Qilu University of Technology (Shandong Academy of Sciences).
2.2. Animals
Healthy Kunming mice were obtained from Pengyue Laboratory Animal Breeding Co., Ltd. (Jinan, China; experimental animal use license: SCXK (Lu) 20140007). A total of 54 Kunming mice weighing 28 ± 2 g were randomly divided into three treatment groups, with half male and half female mice in each group. The mice were managed with natural ventilation, ad libitum feeding, and free access to drinking water.
2.3. Experimental design
The CTRL group was administered purified water throughout the whole process; mice in the DSS and DSS_HA groups were fed water containing DSS (3.5%) for 8 days. From day 9, the DSS group was fed distilled water with a normal diet. Mice in the DSS_HA group were fed distilled water containing 0.4% HAs (Shandong Yatai, China) every day (Figure 1B). During the experiment, the body weight was measured every 2 days, and the mental state, diet, water intake, and the final characteristics of the mice were observed and recorded.
2.4. Histological staining
Mice were euthanized by cervical dislocation. The duodenum, the jejunum, the ileum, and the colon (1 cm) were selected, washed with 0.9% normal saline, and then fixed in 4% formaldehyde. The fixed intestines were encapsulated and sliced. After staining with hematoxylin and eosin (H&E), the morphology of the tissues was examined using light microscopy.
2.5. mRNA and protein level detection
The colon tissue was isolated and homogenized. Total RNA was purified and reversely transcribed using PrimeScript® RT reagent kit with gDNA Eraser (Takara, Dalian, China). SYBR® Premix Ex Taq™ (Takara, Dalian, China) was used for fluorescence quantitative PCR, according to the kit method. The GAPDH gene was used as the internal reference. The primers for TLR4 were as follows: GCTTTCACCTCTGCCTTCAC-3′ and 5′-CGAGGCTTTTCCATCCAATA-3′. The primers for ZO-1 were as follows: 5′-GAG-GATGGTCACACCGTGGT-3′ and 5′-GGAGGATGCTGTTGTCTCGG-3′. The primers for occludin were as follows: 5′-CAGGTGCACCCTCCAGATTG-3′ and 5′-TGGACTTTCAA-GAGGCCTGG-3′. The primers for claudin-1 were as follows: 5′-CTGCTTCTCTCTGCCTTCTG-3′ and 5′-GGAAGGCGAAGGTTTTGGAT-3′.
The expression level of NF-κB in the colon tissue was measured by the ELISA kit (Shanghai Ruifan Biotechnology, China), according to the instructions of the manufacturer.
2.6. Measurement of inflammatory factors
On day 16, nine mice were randomly selected from each treatment group (three mice per replicate), blood was collected from the eyeballs, and serum IL-6 was determined with the Mouse IL-6 ELISA kit (Boster Biological Technology, China). IL-1β was determined with the Mouse IL-1β ELISA kit (Shanghai Enzyme-linked Biotechnology, China), while TNF-α was determined with the Mouse TNF-α ELISA kit (Shanghai Yuanmu Biotechnology, China). LPS concentration was detected by the Mouse LPS ELISA kit from Shanghai Shuhua Biotechnology Co., Ltd. The protocols were followed according to the instructions of the manufacturer.
2.7. Microbiota analysis
The gut microbiota was analyzed as in our previous research (Li et al., 2021). The DNeasy PowerSoil kit (QIAGEN, United States) was used to extract the microbial genomic DNA from the cecum contents of mice. A NanoDrop ND-1000 spectrophotometer (Thermo Fisher Scientific, USA) quantified the DNA, and the quality was estimated using 0.8% agarose gel electrophoresis. Using high-quality DNA samples as templates, the bacterial 16S rRNA gene was amplified. The target fragments were extracted using an Axygen gel recovery kit (SelectScience, UK) and quantified on a microplate reader (BioTek, USA). TruSeq Nano DNA LT Library Prep kit (Illumina, USA) was used to construct the library, and the sequences were compared and analyzed using MOTHUR software and sequence clustering software Usearch (version 7.1). Greengenes is used as a comparison database. The data were deposited in NCBI SRA under the accession number PRJNA836642.
2.8. Statistical analysis
Data were analyzed using ANOVA in SPSS 22.0, and multiple comparisons of means were performed using Duncan's test.
3. Results
3.1. HAs treatment alleviates DSS-induced colitis in mice
At the end of the 16 days experiment, the body weight of the mice in the DSS_HA group was significantly higher (p = 0.047) than that of the mice in the DSS group and was not significantly different from that of the mice in the CTRL group (Figure 1C), suggesting that HAs have a positive effect on growth restriction caused by diarrhea.
We performed H&E staining to visualize the intestinal tissue (Figure 2). In the DSS group, crypts in the duodenum were damaged, and inflammatory cells infiltrated the duodenum. In addition, the muscle layer was thinner than that in the CTRL group, and the submucosa was remarkably peeled off in the duodenum. In contrast, the structure of the crypts in the DSS_HA group was similar to that in the CTRL group, suggesting that HAs can prevent and repair crypt damage caused by DSS. In the jejunum of the DSS group, remarkable inflammatory cell infiltration was detected in the crypts with the disappearance of the crypt structure at the inflammatory site. The muscle layer was thinner than that in the control group. However, the degree of inflammatory cell infiltration was reduced in the DSS_HA group with the muscle layer restored. In the ileum, slight submucosal dissection was observed in the DSS group. However, the crypts were deformed, and the shape of the intestinal villi was severely damaged in the DSS_HA group, even though the shape of the intestinal villi was restored to a certain extent. Finally, the mice in the DSS group showed remarkable inflammatory cell infiltration in the colon compared with those in the control group, and the mucosal goblet cells were damaged. In contrast, inflammatory cell infiltration had disappeared in the DSS_HAs group, and the goblet cells were repaired to a certain extent. These results suggest that HAs can decrease the degree of inflammation in the intestinal tract, repair the shape of the intestinal villi and crypts, and ameliorate colitis in mice.
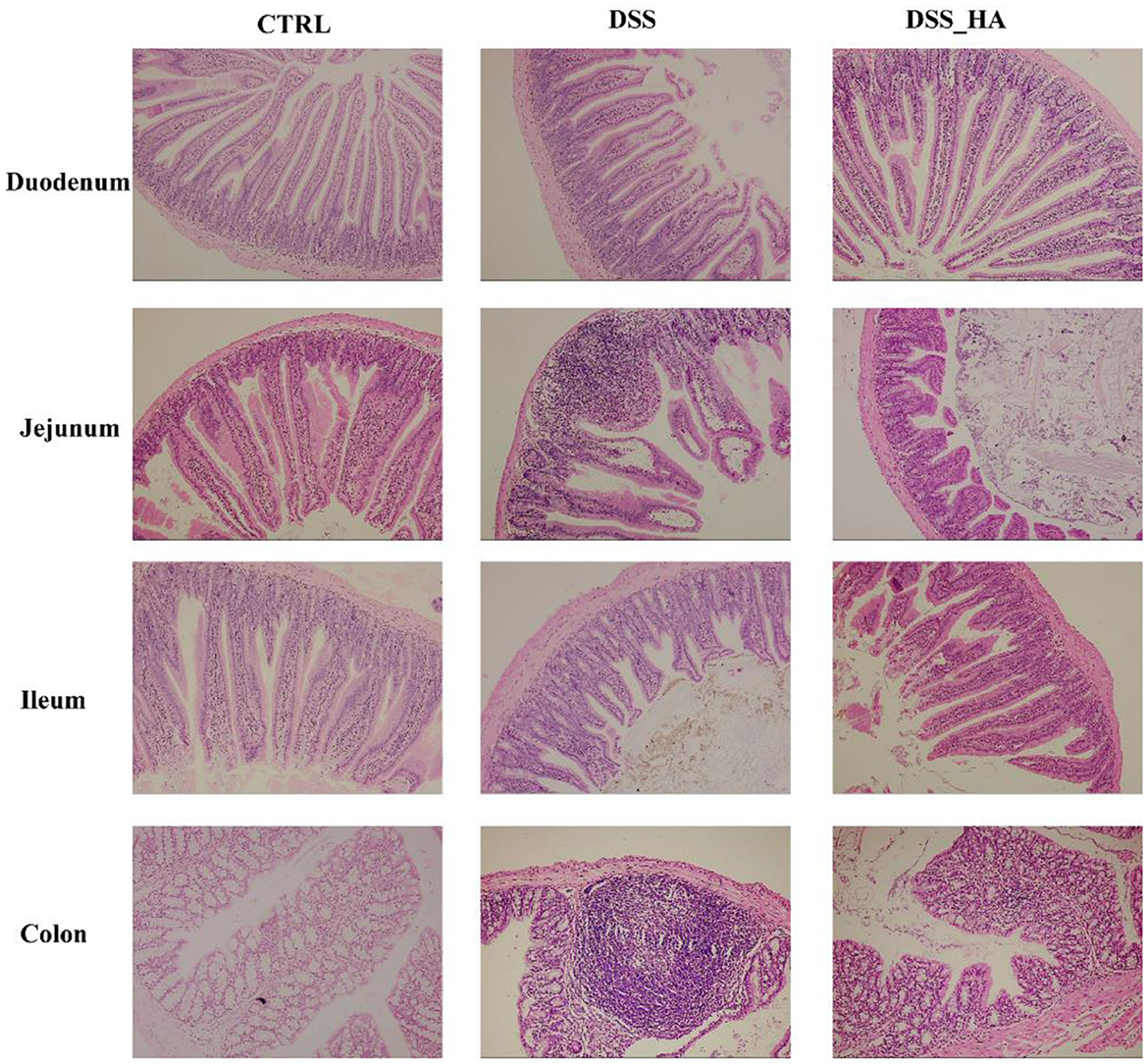
Figure 2. Hematoxylin and eosin staining of the duodenum, jejunum, ileum, and colon in the CTRL, DSS, and DSS_HA groups.
3.2. HAs treatment alters the composition of gut microbiota in mice
After feeding on HAs, Proteobacteria decreased substantially and Firmicutes increased abundantly in the DSS_HA group (Figure 3A). To understand this alteration in detail, the top 50 abundant bacteria were analyzed at the genus level (Figure 3B). The results showed that Streptococcus, Gemella, Blautia, Clostridium, and other genera increased in the DSS group compared with the bacteria in the CTRL group. In contrast, Lactobacillus, Ruminococcus, Collinsella, Pediococcus, and Bifidobacterium increased substantially in the DSS_HA group compared to those in the CTRL and DSS groups. This implies that HAs increased the abundance of profitable bacteria, such as Lactobacillus and Bifidobacterium.
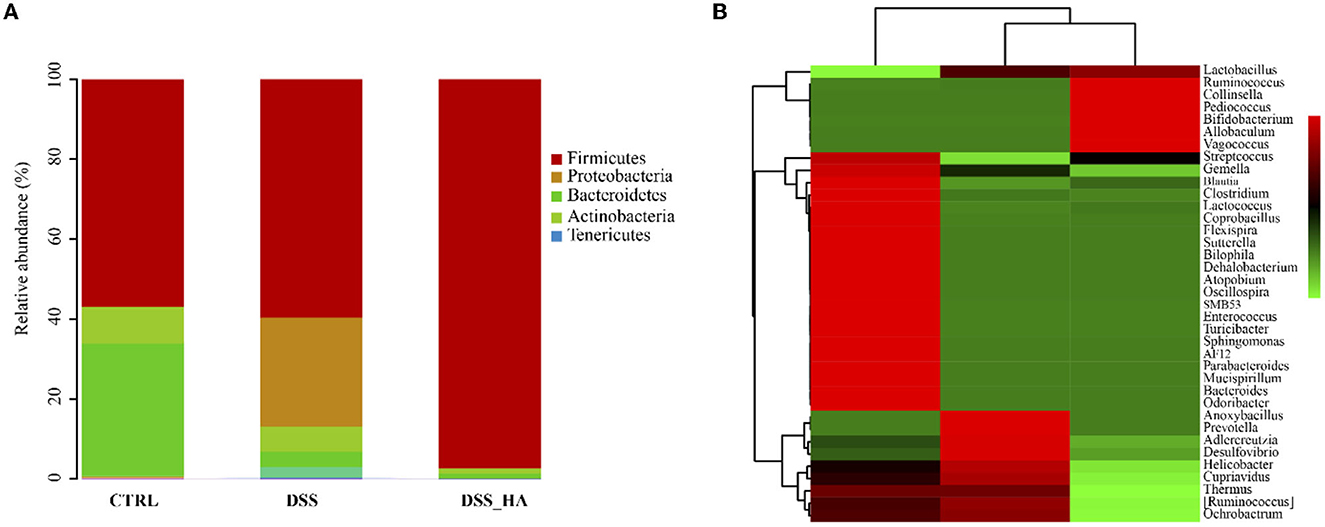
Figure 3. HAs alter the bacterial community of the gut in mice. (A) Taxonomic composition of the microbiota in the CTRL, DSS, and DSS_HA groups at the phylum level. (B) Heatmap of the top 50 dominant genera in each group.
3.3. HAs treatment alleviates colitis through the Toll-like receptor 4-NF-κB pathway
Higher concentrations of LPS were detected in the DSS group, whereas treatment with HAs significantly decreased the LPS concentration (p = 0.029) (Figure 4A). It has been reported that LPS could stimulate the TLR4-NF-κB signaling pathway to induce the release of TNF-α, IL-1β, and IL-6, which are inflammatory cytokines in inflammatory bowel disease. When we analyzed the expression of TLR4 and cytokine concentrations, the results indicated that DSS could induce the expression of TLR4 and NF-κB, whereas HAs could inhibit this expression (Figures 4B, C). In addition, the serum concentrations of the three cytokines were significantly higher in the DSS group than in the control group (p = 0.016, 0.016, and 0.003, respectively) (Figure 4D). However, TNF-α, IL-6, and IL-1β concentrations were significantly lower in the DSS_HA group than in the DSS group (p = 0.047, 0.029, and 0.010, respectively). These results are consistent with those of morphological analysis performed using intestinal HE staining, suggesting that HAs can be used to alleviate inflammation.
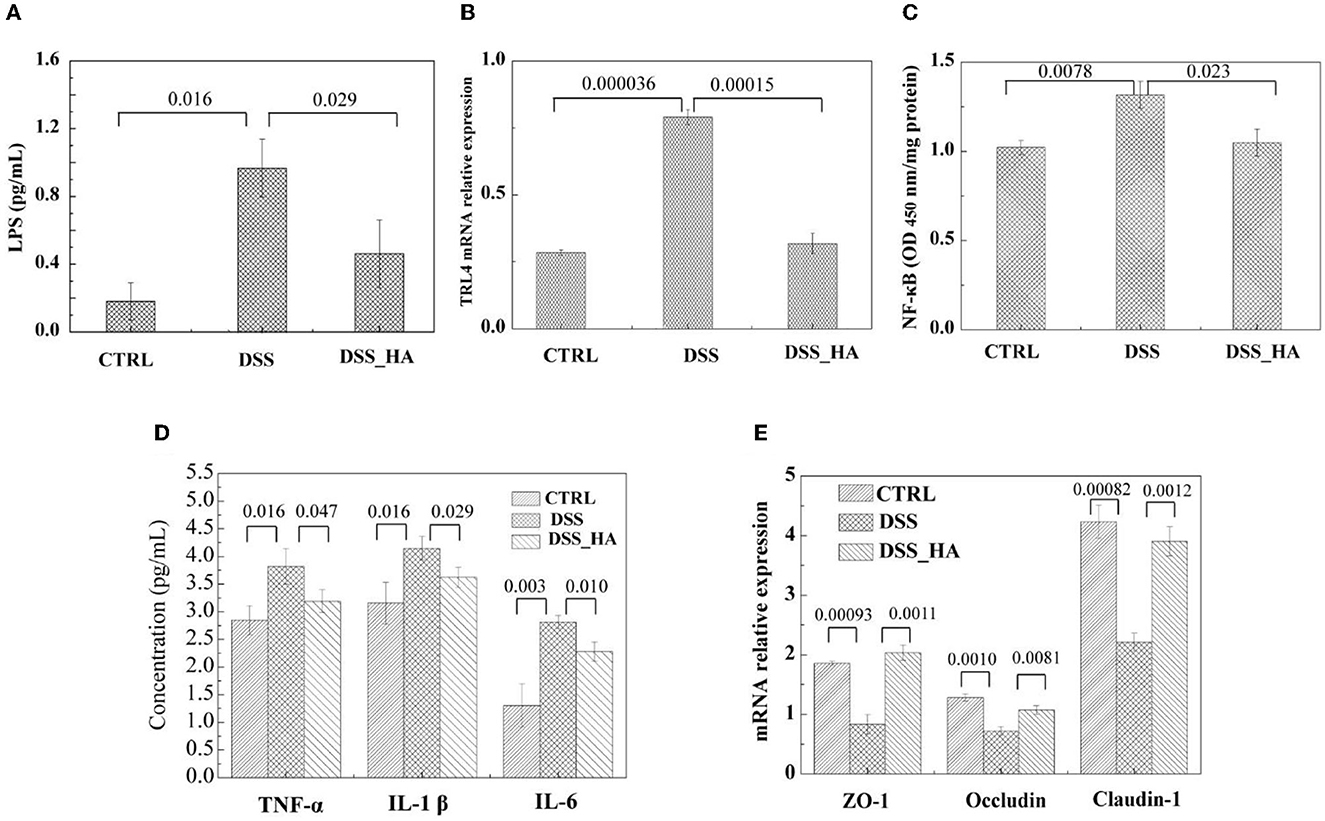
Figure 4. HAs protected mice from inflammatory bowel disease induced by DSS exposure. (A) Lipopolysaccharide (LPS) concentration in the serum of the mice in each group. (B) Relative Toll-like receptor 4 (TLR4) expression level in the colon tissue of each group. (C) NF-κB level in the colon tissue of each group. (D) Comparison of the TNF-α, IL-1β, and IL-6 concentrations in the mice sera of the three groups. (E) Relative expression level of ZO-1, occludin, and claudin-1 in the colon of the three groups. The p-values from (A–E) are shown at the top of the histogram.
Hence, we analyzed the effect of DSS and HAs on the expression of intestinal occludin, ZO-1, and claudin-1, which are critical tight junction proteins. The results showed that DSS decreased the expression of ZO-1, occludin, and claudin-1. In contrast, the mRNA levels of the three genes increased in the DSS-HA group (Figure 4E), suggesting that HAs are beneficial for maintaining the integrity of the intestinal barrier.
4. Discussion
Dextran sulfate sodium (DSS) can induce intestinal inflammation by damaging the epithelial monolayer and disseminating the proinflammatory intestinal contents into underlying tissue. The DSS colitis model is widely accepted and popular in inflammatory bowel disease (IBD) research (Chen et al., 2022; Peng et al., 2022).
We investigated whether HAs could alter the gut microbiota in mice. At the phylum level, adding DSS to the diet markedly increased Proteobacteria in the gut microbiota of the group compared with the CTRL group, which is similar to the previous study (Zhu et al., 2021; Deng et al., 2022; Li W. et al., 2022). The alteration of the gut microbiota motivated us to study the effect of the gut microbiota on mice. Proteus produces lipopolysaccharide (LPS), a pro-inflammatory toxin that induces intestinal inflammation in mice easily (d'Hennezel et al., 2017; Jeong et al., 2019; Jia et al., 2022; Zhong et al., 2022). The results showed that HAs could counteract LPS-induced inflammation, which might be related to the reduction of the relative abundance of Proteobacteria by HAs.
It has been reported that LPS could stimulate the TLR4-NF-κB signaling pathway to induce the release of TNF-α, IL-1β, and IL-6, which are inflammatory cytokines in inflammatory bowel disease (Gerges et al., 2020). This is consistent with our experimental results. We further investigated the potential mechanism of the function of HAs. In healthy mice, normal TLR4-NF-κB signal transduction is an important basic immune mechanism for maintaining the integrity of the epithelial barrier and the tolerance of the microbiota. However, in inflammatory bowel disease, TLR4-NF-κB overexpression and overactive TLR4-NF-κB signal transduction may damage intestinal mucosal homeostasis, leading to tissue damage and inflammation (Cario, 2010). Previous study has found that in DSS-induced colitis, TLR4-NF-κB are overexpressed (Hou et al., 2013). This is consistent with our experimental results. Compared to the DSS group, HAs significantly reduced the relative expression levels of TLR4 and NF-κB.
Alteration of the gut microbiota is related to intestinal permeability (Kang et al., 2022; Koutoukidis et al., 2022; Zhang et al., 2022). The intestinal barrier is key to blocking toxic substances and maintaining normal intestinal homeostasis. Previous studies have shown that DSS-induced colitis is associated with the disruption of intestinal barrier function (Gerges et al., 2020). In this study, HAs can reduce the degree of intestinal inflammation, repair the shape of the intestinal villi and crypts, and improve colitis in mice. At the same time, HAs increased the relative expression of intestinal tight junction protein mRNA. Therefore, we believe that HAs can regulate intestinal barrier function and prevent toxic substances from leaking from the intestine into the circulatory system.
In conclusion (Figure 5), we showed that DSS might have caused colitis in mice by altering the intestinal morphology, whereas HAs restored the morphology and decreased the levels of inflammation. Furthermore, we observed that HAs can alter the community of the gut microbiota damaged by DSS. By increasing the abundance of Firmicutes and decreasing the abundance of Proteobacteria, HAs decreased the expression of TLR4 and further reduced the levels of inflammatory cytokines. HAs also increased intestinal permeability, which was damaged by DSS. These results suggest that HAs have the potential to treat colitis. Despite the valuable findings of our study, it is important to acknowledge its limitations. One major limitation is that the exact mechanism by which HAs alleviate colitis remains unknown. However, our research revealed that the addition of HAs to the diet of aging laying hens led to significant alterations in the alpha and beta diversities of the intestinal microbiome (Li C. et al., 2022). Specifically, we observed an increase in the levels of short-chain fatty acids, including acetic acid, isovaleric acid, and isobutyric acid, in the HA group. These results suggest that the primary benefit of HAs in treating colitis may be through the modulation of the intestinal microbiota and the alteration of microbial metabolites, further reducing inflammation, maintaining mucosal barriers, and inhibiting pathogen growth. Further research is required to elucidate the detailed functions and mechanisms of HAs in the treatment of colitis.
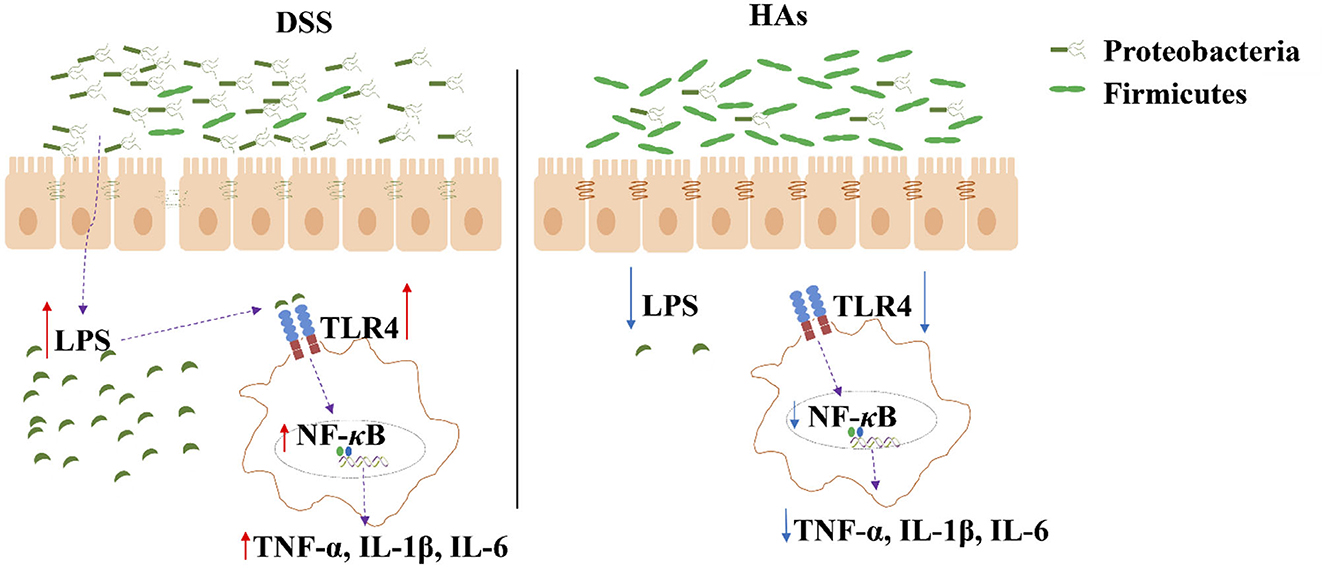
Figure 5. Illustration of the mechanism by which HAs reduce inflammation. HAs decrease the abundance of Proteobacteria but increase that of Firmicutes. The reduced level of Proteobacteria decreases LPS concentration, which further inactivates the expression of TLR4 and the production of inflammatory cytokines (TNF-α, IL-1β, and IL-6).
Data availability statement
The datasets presented in this study can be found in online repositories. The names of the repository/repositories and accession number(s) can be found below: PRJNA836642.
Ethics statement
The animal study was reviewed and approved by the Animal Care and Use Committee of the Qilu University of Technology (Shandong Academy of Sciences).
Author contributions
PX, JH, and JZ designed the research. MS, BW, and CZ performed the experiments and analyzed the data. All authors reviewed the results and approved the final version of the manuscript.
Funding
This study was funded by the Key Innovation Project of Qilu University of Technology (Shandong Academy of Sciences) (2022JBZ01-06), the Natural Science Foundation of Shandong Province (grant no. ZR2021KE038), the Shandong Province Agricultural Major Application Technology Innovation Project (grant no. 20182130106), and the Foundation of Qilu University of Technology of Cultivating Subject for Biology and Biochemistry (grant no. 202119).
Conflict of interest
BW and CZ are employees of Shandong Asia-Pacific Haihua Biotechnology, a company engaged in the production of humic acid.
The remaining authors declare that the research was conducted in the absence of any commercial or financial relationships that could be construed as a potential conflict of interest.
Publisher's note
All claims expressed in this article are solely those of the authors and do not necessarily represent those of their affiliated organizations, or those of the publisher, the editors and the reviewers. Any product that may be evaluated in this article, or claim that may be made by its manufacturer, is not guaranteed or endorsed by the publisher.
References
Cario, E. (2010). Toll-like receptors in inflammatory bowel diseases: a decade later. Inflamm. Bowel Dis. 16, 1583–1597. doi: 10.1002/ibd.21282
Chen, L., Li, R., Wang, Z., Zhang, Z., Wang, J., Qiao, Y., et al. (2022). Lactateutilizing bacteria ameliorates DSS-induced colitis in mice. Life Sci. 288, 120179. doi: 10.1016/j.lfs.2021.120179
Cui, X., Zheng, X., Ren, J., Liu, H., Jia, Y., Wu, A., et al. (2023). Development and validation of two bioanalysis methods for the determination of etimicin in human serum and urine by liquid chromatography-tandem mass spectrometry: applications to a human pharmacokinetic and breakpoint study. Front. Pharmacol. 14, 1076046. doi: 10.3389/fphar.2023.1076046
Deng, L., Guo, H., Wang, S., Liu, X., Lin, Y., Zhang, R., et al. (2022). The attenuation of chronic ulcerative colitis by (R)-salbutamol in repeated DSS-induced mice. Oxid. Med. Cell. Longev. 2022, 9318721. doi: 10.1155/2022/9318721
d'Hennezel, E., Abubucker, S., Murphy, L. O., Cullen, T. W., and Lozupone, C. (2017). Total lipopolysaccharide from the human gut micro-biome silences Toll-like receptor signaling. mSystems 2, e00046–e00017. doi: 10.1128/mSystems.00046-17
Gerges, S. H., Tolba, M. F., Elsherbiny, D. A., and El-Demerdash, E. (2020). The natural flavonoid galangin ameliorates dextran sulphate sodium–induced ulcerative colitis in mice: effect on toll-like receptor 4, inflammation and oxidative stress. Basic Clin. Pharmacol. 127, 10–20. doi: 10.1111/bcpt.13388
Hauck, F., Koletzko, S., Walz, C., Bernuth, H. V., Klenk, A., Schmid, I., et al. (2016). Diagnostic and treatment options for severe IBD in female X-CGD carriers with non-random X-inactivation. J. Crohns Colitis 10, 112–115. doi: 10.1093/ecco-jcc/jjv186
Hou, Y. C., Chu, C. C., Ko, T. L., Yeh, C. L., and Yeh, S. L. (2013). Effects of alanyl-glutamine dipeptide on the expression of colon-inflammatory mediatorsduring the recovery phase of colitis induced by dextran sulfate sodium. Eur. J. Nutr. 52, 1089–1098. doi: 10.1007/s00394-012-0416-3
Jeong, M. Y., Jang, H. M., and Kim, D. H. (2019). High-fat diet causes psychiatric disorders in mice by increasing Proteobacteria population. Neurosci. Lett. 698, 51–57. doi: 10.1016/j.neulet.2019.01.006
Jia, B., Kim, K. H., Ruan, W., Kim, H. M., and Jeon, C. O. (2022). Lantibiotic-encoding Streptococcus in the human microbiome are underlying risk factors for liver diseases. J. Infect. 84, e70–e72. doi: 10.1016/j.jinf.2022.02.020
Jia, B., Zou, Y., Han, X., Bae, J. W., and Jeon, C. O. (2023). Gut microbiome-mediated mechanisms for reducing cholesterol levels: implications for ameliorating cardiovascular disease. Trends Biotechnol. 31, 76–91. doi: 10.1016/j.tim.2022.08.003
Kang, Y., Kang, X., Yang, H., Liu, H., Yang, X., Liu, Q., et al. (2022). Lactobacillus acidophilus ameliorates obesity in mice through modulation of gut microbiota dysbiosis and intestinal permeability. Pharmacol. Res. 175, 106020. doi: 10.1016/j.phrs.2021.106020
Koutoukidis, D. A., Jebb, S. A., Zimmerman, M., Otunla, A., Henry, J. A., Ferrey, A., et al. (2022). The association of weight loss with changes in the gut microbiota diversity, composition, and intestinal permeability: a systematic review and meta-analysis. Gut Microbes 14, 2020068. doi: 10.1080/19490976.2021.2020068
Li, C., Li, X., Li, P., Wei, B., Zhang, C., Zhu, X., et al. (2022). Sodium humate alters the intestinal microbiome, short-chain fatty acids, eggshell ultrastructure, and egg performance of old laying hens. Front. Vet. Sci. 9, 986562. doi: 10.3389/fvets.2022.986562
Li, W., Zhang, L., Xu, Q., Yang, W., Zhao, J., Ren, Y., et al. (2022). Taxifolin alleviates DSS-induced ulcerative colitis by acting on gut microbiome to produce butyric acid. Nutrients 14, 1069. doi: 10.3390/nu14051069
Li, X., Tan, X., Chen, Q., Zhu, X., Zhang, J., Zhang, J., et al. (2021). Prodigiosin of Serratia marcescens ZPG19 alters the gut microbiota composition of kunming mice. Molecules 26, 2156. doi: 10.3390/molecules26082156
Miao, Z. H., Li, K., Liu, P. Y., Li, Z., Yang, H., Zhao, Q., et al. (2018). Natural humic-acid-based phototheranostic agent. Adv. Healthc. Mater. 7, 1701202. doi: 10.1002/adhm.201701202
Mudronová, D., Karaffová, V., Semjon, B., Nad, P., Koščová, J., Bartkovský, M., et al. (2021). Effects of dietary supplementation of humic substances on production parameters, immune status and gut micro-biota of laying hens. Agriculture 11, 744. doi: 10.3390/agriculture11080744
Peng, S. J., Ye, D. T., Zheng, J., Xue, Y. R., Lin, L., Zhao, Y. D., et al. (2022). Synthesis, characterization of low molecular weight chitosan selenium nanoparticles and its effect on dss-induced ulcerative colitis in mice. Int. J. Mol. Sci. 23, 15527. doi: 10.3390/ijms232415527
Rivera-Sánchez, S. P., Rojas-Abadía, J. M., Ríos-Acevedo, J. J., Mejía-Hurtado, A. F., Espinosa-Moya, L. N., and Ocampo-Ibáñez, I. D. (2022). Efficacy of vaporized hydrogen peroxide combined with silver ions against multidrug-resistant gram-negative and gram-positive clinical isolates. Int. J. Mol. Sci. 23, 15826. doi: 10.3390/ijms232415826
Rusliandi, R., Rousdy, D. W., and Mukarlina, M. (2020). The anti-inflammatory activity of humic acid from Borneo peat soil in mice. Maj. Obat. Trad. 25, 22–28. doi: 10.22146/mot.48003
Saleh, A. A., Yassin, M., El-Naggar, K., Alzawqari, M. H., Albogami, S., Mohamed Soliman, M., et al. (2022). Effect of dietary supplementation of humic acid and lincomycin on growth performance, nutrient digestibility, blood biochemistry, and gut morphology in broilers under clostridium infection. J. Appl. Anim. Res. 50, 440–452. doi: 10.1080/09712119.2022.2089674
Sarlaki, E., Paghaleh, A. S., Kianmehr, M. H., and Vakilian, K. A. (2019). Extraction and purification of humic acids from lignite wastes using alkaline treatment and membrane ultrafiltration. J. Clean. Prod. 235, 712–723. doi: 10.1016/j.jclepro.2019.07.028
Socol, D. (2023). Clinical review of humic acid as an antiviral: leadup to translational applications in clinical humeomics. Front. Pharmacol. 13, 1018904. doi: 10.3389/fphar.2022.1018904
Sun, T., Liu, X., Su, Y., Wang, Z., Cheng, B., Dong, N., et al. (2022). The efficacy of anti-proteolytic peptide R7I in intestinal inflammation, function, microbiota, and metabolites by multi-omics analysis in murine bacterial enteritis. Bioeng. Transl. Med. 8, e10446. doi: 10.1002/btm2.10446
Wang, Q., Chen, Y. J., Yoo, J. S., Kim, H. J., Cho, J. H., and Kim, I. H. (2008). Effects of supplemental humic substances on growth performance, blood characteristics and meat quality in finishing pigs. Livest Sci. 117, 270–274. doi: 10.1016/j.livsci.2007.12.024
Xu, P., Zhu, X., Tian, H., Zhao, G., Chi, Y., Jia, B., et al. (2022). The broad application and mechanism of humic acids for treating environmental pollutants: insights from bibliometric analysis. J. Clean. Prod. 337, 130510. doi: 10.1016/j.jclepro.2022.130510
Zhang, Y., Gan, Y., Wang, J., Feng, Z., Zhong, Z., Bao, H., et al. (2022). Dysbiosis of gut microbiota and intestinal barrier dysfunction in pigs with pulmonary inflammation induced by mycoplasma hyorhinis infection. mSystems 7, e00282–e00222. doi: 10.1128/msystems.00282-22
Zhong, W., Wu, K., Long, Z., Zhou, X., Zhong, C., Wang, S., et al. (2022). Gut dysbiosis promotes prostate cancer progression and docetaxel resistance via activating NF-κB-IL6-STAT3 axis. Microbiome 10, 1–19. doi: 10.1186/s40168-022-01289-w
Zhu, H. C., Jia, X. K., Fan, Y., Xu, S. H., Li, X. Y., Huang, M. Q., et al. (2021). Alisol B 23-acetate ameliorates azoxymethane/dextran sodium sulfate-induced male murine colitis-associated colorectal cancer via modulating the composition of gut microbiota and improving intestinal barrier. Front. Cell. Infect. Microbiol. 11, 640225. doi: 10.3389/fcimb.2021.640225
Keywords: humic substances, gut microbiota, inflammation, traditional Chinese medicine, enteritis
Citation: Huang J, Xu P, Shao M, Wei B, Zhang C and Zhang J (2023) Humic acids alleviate dextran sulfate sodium-induced colitis by positively modulating gut microbiota. Front. Microbiol. 14:1147110. doi: 10.3389/fmicb.2023.1147110
Received: 18 January 2023; Accepted: 13 March 2023;
Published: 12 April 2023.
Edited by:
Xiao Han, Fuzhou University, ChinaReviewed by:
Mingyu Wang, Shandong University, ChinaYanxue Yu, Chinese Academy of Inspection and Quarantine (CAIQ), China
Zhengyuan Zhai, China Agricultural University, China
Copyright © 2023 Huang, Xu, Shao, Wei, Zhang and Zhang. This is an open-access article distributed under the terms of the Creative Commons Attribution License (CC BY). The use, distribution or reproduction in other forums is permitted, provided the original author(s) and the copyright owner(s) are credited and that the original publication in this journal is cited, in accordance with accepted academic practice. No use, distribution or reproduction is permitted which does not comply with these terms.
*Correspondence: Jie Zhang, emhhbmdqaWUmI3gwMDA0MDtxbHUuZWR1LmNu
†These authors have contributed equally to this work