- 1State Key Laboratory for Infectious Disease Prevention and Control, National Institute for Communicable Disease Control and Prevention, Chinese Center for Disease Control and Prevention, Beijing, China
- 2Jinzhai County Center for Disease Control and Prevention, Jinzhai, Anhui, China
- 3Anhui Provincial Center for Disease Control and Prevention, Public Health Research Institute of Anhui Province, Hefei, China
- 4Lu'an Municipal Center for Disease Control and Prevention, Lu'an, Anhui, China
- 5Pathogen and Biosecurity, Beijing Institute of Microbiology and Epidemiology, Beijing, China
The order Rickettsiales in the class Alphaproteobacteria comprises vector-borne pathogens of both medical and veterinary importance. Ticks, as a group, are second only to mosquitoes as vectors of pathogens to humans, playing a critical role in the transmission of rickettsiosis. In the present study, 880 ticks collected from Jinzhai County, Lu'an City, Anhui Province, China in 2021–2022 were identified as belonging to five species from three genera. DNA extracted from individual ticks was examined using nested polymerase chain reaction targeting the 16S rRNA gene (rrs), and the gene fragments amplified were sequenced to detect and identify Rickettsiales bacteria in the ticks. For further identification, the rrs-positive tick samples were further amplified by PCR targeting the gltA and groEL gene and sequenced. As a result, 13 Rickettsiales species belonging to the genera Rickettsia, Anaplasma, and Ehrlichia were detected, including three tentative species of Ehrlichia. Our results reveal the extensive diversity of Rickettsiales bacteria in ticks from Jinzhai County, Anhui Province. There, emerging rickettsial species may be pathogenic and cause under-recognized diseases. Detection of several pathogens in ticks that are closely related to human diseases may indicate a potential risk of infection in humans. Therefore, additional studies to assess the potential public health risks of the Rickettsiales pathogens identified in the present study are warranted.
1. Introduction
Rickettsiales are a group of obligate intracellular gram-negative bacteria in the class Alphaproteobacteria, and many of them have been identified as vector-borne pathogens of medical and veterinary importance. The order Rickettsiales comprises three families: Anaplasmataceae, Rickettsiaceae, and Holosporaceae (Salje, 2021). Humans are exposed to and susceptible to many tick-borne pathogens in the order Rickettsiales, including Anaplasma phagocytophilum, which causes human granulocytic anaplasmosis; Ehrlichia canis, E. chaffeensis, and E. ewingii, which cause different types of ehrlichiosis; Rickettsia rickettsii, which causes Rocky Mountain spotted fever (RMSF), and other recently recognized spotted fever group rickettsiae (Nicholson et al., 2010). Recently, globalization, climate warming, and geographical expansion have led to an increase in the incidence and resurgence of human diseases caused by these rickettsial pathogens (Piotrowski and Rymaszewska, 2020; Matos et al., 2022). Rickettsial diseases constitute an emerging public health threat and pose a significant health burden worldwide. However, in past decades, owing to advances in molecular analysis and characterization, an increasing number of novel species/genotypes have been identified, and certain Rickettsiales species previously considered that non-pathogenic have now been linked with human diseases, which facilitates the re-evaluation of the effects of these pathogens on human and animal health (Rar and Golovljova, 2011; Luce-Fedrow et al., 2015; Kim et al., 2022).
Ticks—obligate blood-sucking ectoparasites of vertebrates—are known to carry and transmit various pathogenic microorganisms, including bacterial pathogens in the order Rickettsiales (De La Fuente et al., 2008; Dantas-Torres et al., 2012; Yu et al., 2015). Previous studies suggest that years may elapse between the identification of a potential pathogenic agent in ticks and the detection of associated human infections. This is because the pathogen load and DNA copy number in ticks are much higher than those in human blood (Parola et al., 2013). For example, Rickettsia sibirica subsp. sibirica BJ-90 was first identified in Chinese ticks (Yu et al., 1993), but the associated human infection was not identified until 22 years later (Jia et al., 2013). Similarly, Anaplasma phagocytophilum was first identified in ticks in China in 1997 (Cao et al., 2000), but human granulocytic anaplasmosis was not identified until 10 years later (Zhang et al., 2008). Notably, because of social change and contemporary urbanization, the habitats of humans, animals, and ticks increasingly overlap, which amplifies the risk of human exposure to ticks (Beati et al., 1997; Piotrowski and Rymaszewska, 2020). Therefore, molecular surveillance of pathogens in arthropods is an important aspect of identifying the next rickettsial pathogen that could potentially cause human diseases.
Jinzhai County (Lu'an City) is located on the western border of Anhui Province, at the junction of Anhui, Henan, and Hubei Provinces. This region is ~75% forested and has a humid subtropical monsoon climate, providing suitable habitats for the survival and reproduction of ticks. Previous studies on the molecular detection of tick-borne pathogens in Anhui Province have focused on the detection of Rickettsia, Hepatozoon, Ehrlichia, severe fever with thrombocytopenia syndrome virus in goats (Li et al., 2020), and Anaplasma spp. in healthy small ruminants (goats and sheep) (Yang et al., 2019). Although there are molecular detection strategies for tick-borne pathogens in local areas, these pathogens have not been extensively and systematically studied. Further investigation and molecular characterization of Rickettsiales bacteria in ticks are necessary. Therefore, the present study aimed to investigate the diversity of tick species and the associated rickettsial pathogens in Jinzhai County over a 2-year monitoring period to provide a scientific basis for the prevention and control of tick-borne rickettsial diseases.
2. Materials and methods
2.1. Tick collection and identification
During 2021–2022, adult ticks were collected from Jinzhai County, Lu'an city (115.94°E, 31.73°N) in Anhui Province, China. Ticks were collected directly from domestic animals (cattle, dogs, and goats) and grassland. All ticks were first identified based on morphological differences in their capitulum and body by a trained technician using light microscopy (Azmat et al., 2018); the classification was further confirmed by cytochrome c oxidase subunit 1 (COI) (Cao et al., 2003) gene sequencing. All ticks were stored at −80°C prior to DNA extraction.
2.2. DNA extraction
DNA was extracted from individual adult ticks using a previously described method (Sun et al., 2015). In brief, individual ticks were washed three times with phosphate-buffered saline (PBS) to decontaminate the body surface. The samples were manually homogenized on ice using a 40 mm mortar and pestle (Biosharp, Anhui, China) and then centrifuged for 3 min at 2,500 × g. The supernatant was collected for DNA extraction, and genomic DNA was extracted from individual samples using a QIAamp DNA Mini Kit (Qiagen, Hilden, Germany) according to the manufacturer's instructions and eluted in a final volume of 100 μl. All DNA samples were stored at −20°C until experimental use.
2.3. Polymerase chain reaction
Preliminary screening of Rickettsiales species was performed using nested or semi-nested PCR. For the detection of Rickettsia spp., tick DNA was amplified by nested PCR using primers targeting the 16S ribosomal RNA (16S rRNA) gene (Guo et al., 2016), resulting in the amplification of a 900 bp DNA fragment. To more definitively identify the molecular characteristics of Rickettsia pathogens, the positive samples in the preliminary screening were selected for further amplification and sequencing of the citrate synthase (gltA) gene, 60 kDa chaperonin (groEL) gene, and 16S rRNA (Guo et al., 2016). To identify Anaplasmataceae, molecular screening targeting a fragment of the 16S rRNA gene (Jafar Bekloo et al., 2018) was performed using primers as described previously. To further screen the Ehrlichia-positive samples, two sets of PCR primers were used to amplify the sequences of two overlapping fragments of the 16S rRNA gene. In addition, two semi-nested PCRs targeting partial fragments of the gltA and groEL genes were performed (Lu et al., 2022a), respectively. Subsequently, for Anaplasma-positive samples, species-specific primer pairs for five Anaplasma species were used for PCR amplification, as previously described (Barlough et al., 1996; Loftis et al., 2006; Rar et al., 2015; Yang et al., 2017, 2018; Guo et al., 2018, 2020). The primers used for amplification are listed in Supplementary Table 1.
The amplification products (5 μl) were verified with 1.0% agarose gel electrophoresis, and the size of the DNA fragments was determined by comparison with standard molecular size DNA ladders. The target fragment amplified by each primer pair was purified using a Gel Extraction Kit (Macherey-Nagel, Duren, Germany). The purified DNA was cloned into pMD19-T plasmid (Takara, Dalian, China). Subsequently, each vector was transformed into E. coli cells and its insertion was confirmed by PCR. PCR products with a strong target DNA band were sent for sequencing to Tianyi Huiyuan Biotechnology Company (Beijing, China). DNA fragments shorter than 700 bp were sequenced unidirectionally, whereas fragments longer than 700 bp were sequenced bidirectionally. To avoid cross-contamination, all steps were performed in separate rooms, autoclaved pipettes, and filter tips were used, and the master mix was prepared under a laminar airflow bench (flow rate 0.4 m/s). PCR was performed using Premix Taq Version 2.0 plus dye (Takara, Dalian, China).
2.4. Phylogenetic analysis
The obtained DNA sequences were edited and assembled using the SeqMan program (DNASTAR, Madison, WI) and then compared with previously published sequences deposited in GenBank (http://blast.ncbi.nlm.nih.gov/) using the Basic Local Alignment Search Tool (BLAST). The sequences were aligned and analyzed using the multiple sequence alignment program ClustalW (default parameters) as implemented in MEGA, version 11.0. Phylogenetic trees were constructed using the maximum likelihood method implemented in PhyML version 3 (Guindon et al., 2010). The robustness of the phylogenetic trees was assessed based on bootstrap support values obtained from 1,000 replicates; values more than 70% were considered to indicate significant differences. All phylogenic trees were midpoint rooted for clarity only.
3. Results
3.1. Collection of ticks and detection of Rickettsiales DNA
In 2020–2021, 880 ticks were collected from Jinzhai County, Lu'an City, Anhui Province in China (Figure 1). Based on the morphological examination and analysis of COI sequences, five tick species belonging to three different genera were identified: Haemaphysalis longicornis (62.39%, 549/880), Rhipicephalus microplus (27.95%, 246/880), Haemaphysalis hystricis (6.25%, 55/880), Haemaphysalis flava (2.95%, 26/880), and Amblyomma testudinarium (0.45%, 4/880). The phylogenetic tree constructed based on COI gene sequences is shown in Figure 2; the COI sequences generated in this study were clustered with their respective homologs in five main groups corresponding to five species. The representative COI gene sequences from each group were submitted to GenBank (OQ135123–OQ135128, OQ135142–OQ135169). Table 1 presents the GenBank numbers of the Rickettsiales sequences obtained in this study. The species, numbers, and origins of ticks are shown in Supplementary Table 2. The sequences of three different genes were used to construct supertrees, not only to visualize the evolutionary relationships between species but also to better understand the diversity of species (Supplementary Figures 1–3).
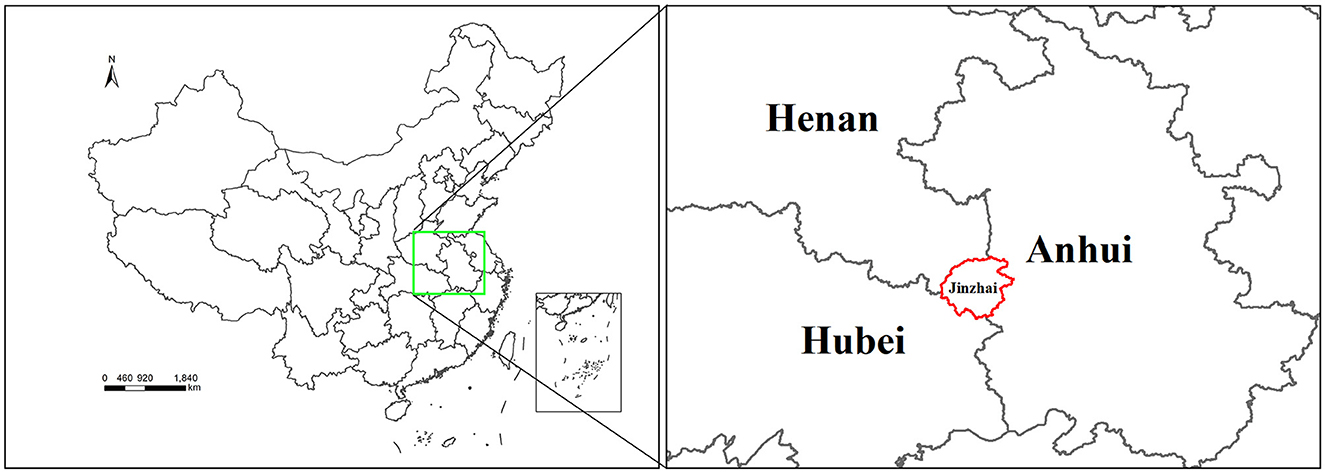
Figure 1. Map showing the location of tick sample collection sites in Jinzhai County, Lu'an City, Anhui Province, China. The region highlighted with a red border indicates the sampling region in this study.
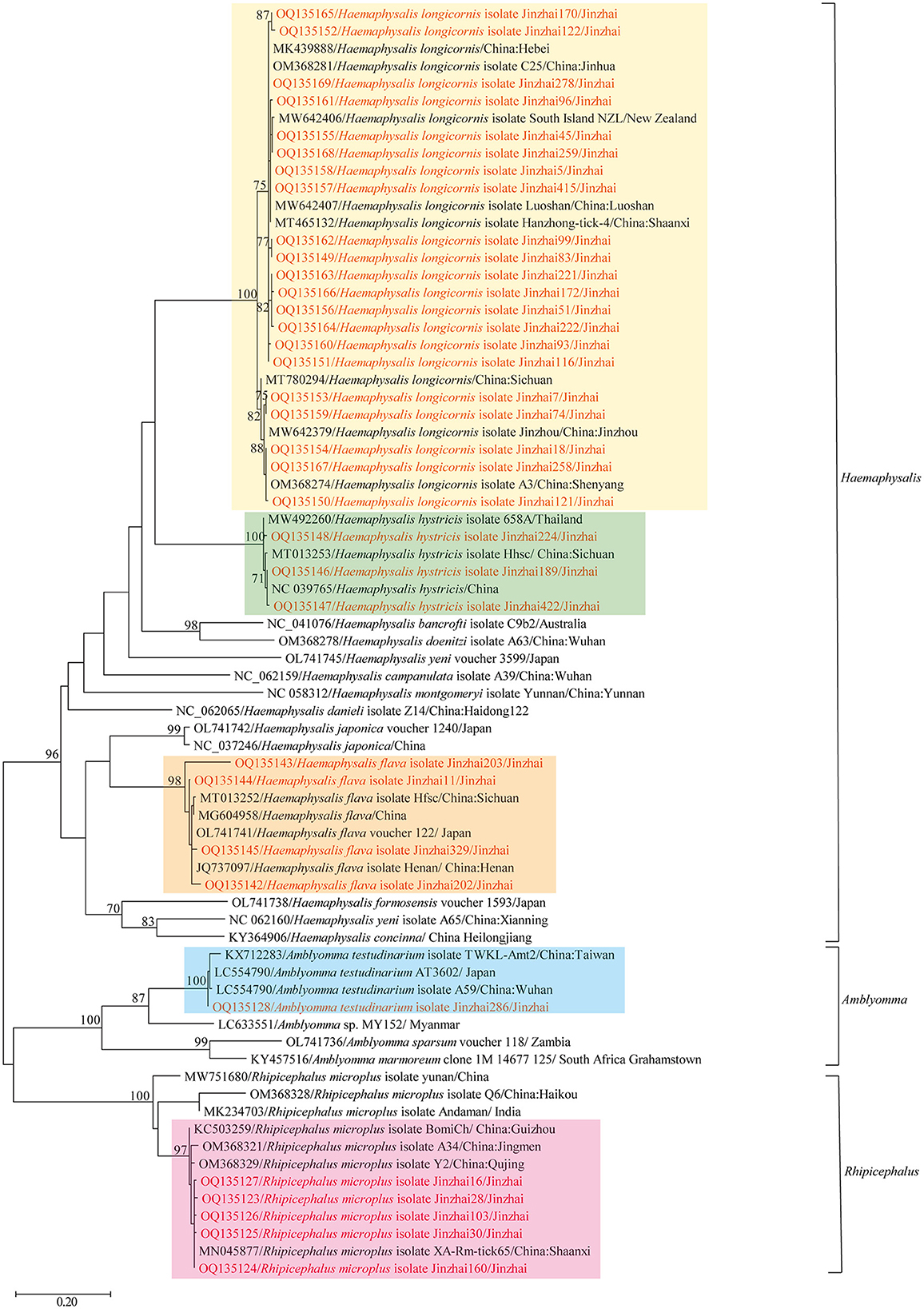
Figure 2. Phylogenetic tree showing the relationship of Haemaphysalis longicornis, Rhipicephalus microplus, Haemaphysalis hystricis, Haemaphysalis flava, and Amblyomma testudinarium with other tick species. Evolutionary analyses were conducted using PhyML 3.0. The COI gene sequences amplified in this study were aligned with other tick COI sequences available on GenBank. Sequences obtained in this study are marked in red.
3.2. Rickettsia bacteria detected in ticks
Bacteria belonging to the genus Rickettsia were detected in H. longicornis and H. flava (Table 2). Sequencing data and phylogenetic analysis of partial fragments of 16S rRNA, gltA, and groEL genes showed the presence of Rickettsia japonica and Rickettsia sp. Specifically, Rickettsia japonica was detected in H. longicornis and H. flava, with positive rates of 1.64% (9/549) and 15.4% (4/26), respectively. The sequences of all R. japonica target gene fragments from different tick samples were 100% identical to each other. The 16S rRNA, gltA, and groEL sequences obtained in this study showed 100%, 99.69%, and 99.81%, respectively, similarity with R. japonica strain LA16/2015 (CP047359) and 99.92%, 99.69%, and 99.52%, respectively, similarity with R. heilongjiangensis 054 (CP002912), suggesting that the R. japonica identified in the present study was closely related to R. japonica strain LA16/2015 and R. heilongjiangensis 054 (Figure 3).
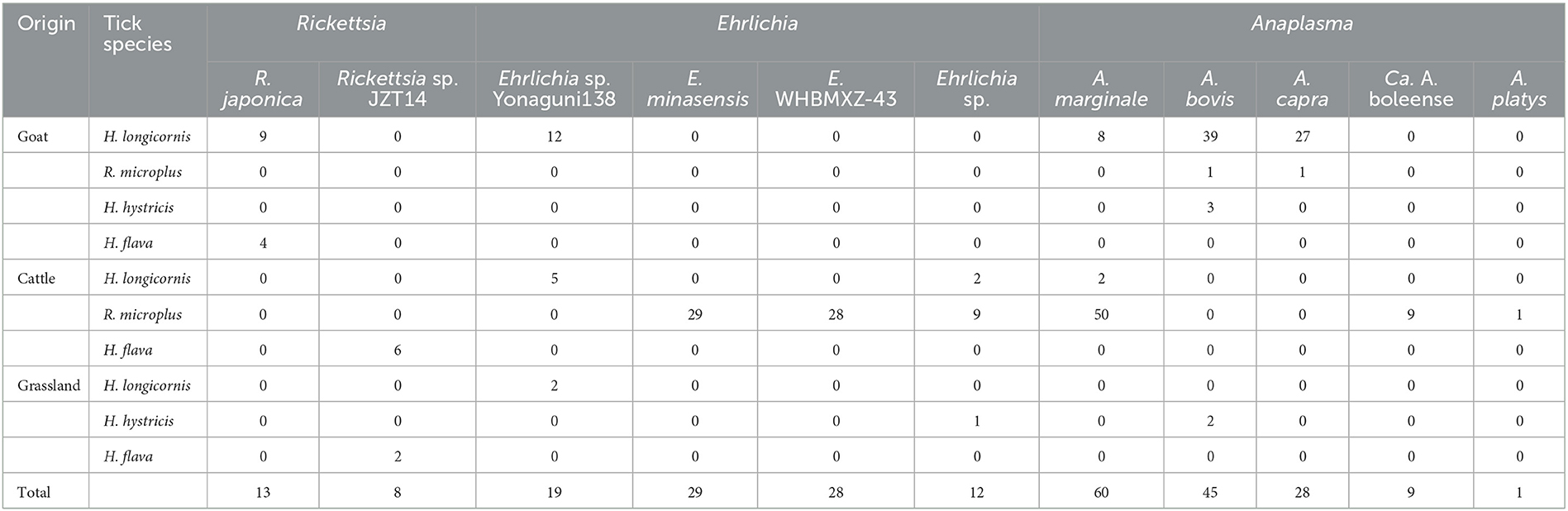
Table 2. Detection of Rickettsiales bacteria in ticks collected from Jinzhai County, China, in 2021–2022.
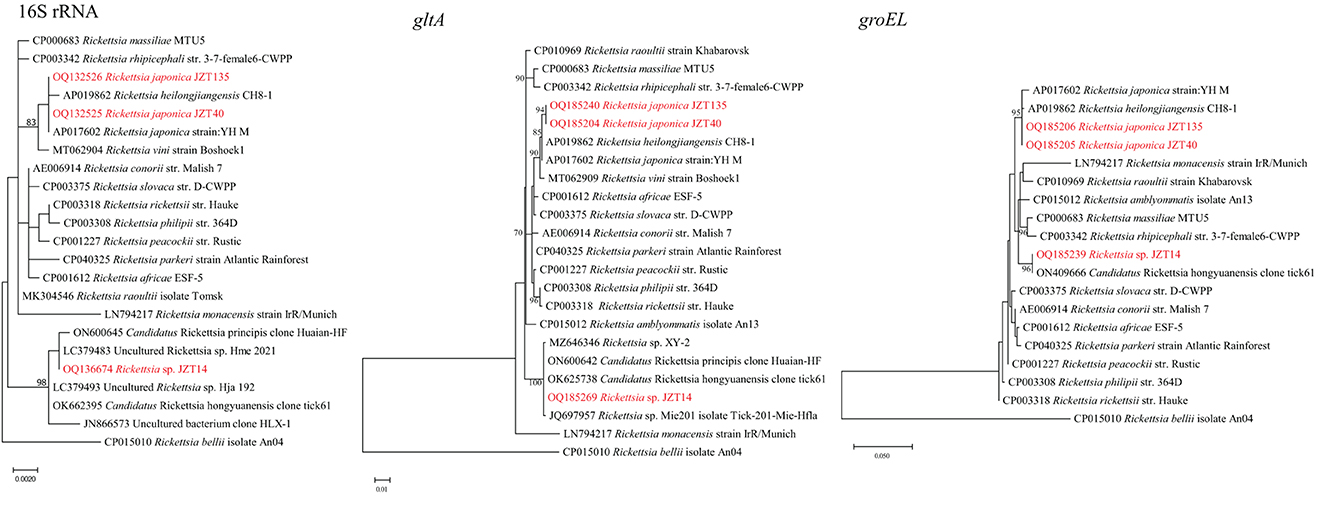
Figure 3. Phylogenetic trees constructed using PhyML 3.0 based on the nucleotide sequences of the 16S rRNA (1213 bp), gltA (985 bp), and groEL (1051 bp) genes of Rickettsia strains. Sequences obtained in this study are marked in red.
Notably, the Rickettsia sp. JZT14 obtained from eight H. flava collected in 2022 constituted another clade; the sequences of all 16S rRNA, gltA, and groEL genes of this pathogen from various tick samples were 100% identical to each other. The 16S rRNA gene sequences obtained in this study shared 100% and 99.84% identity with those of uncultured Rickettsia sp. Hme_2021 (LC379483) and Candidatus Rickettsia hongyuanensis clone tick61 (OK662395), respectively. The gltA gene sequences obtained shared 100% identity with those of Candidatus Rickettsia principis clone Huaian-HF (ON600642) and 100% identity with those of Candidatus Rickettsia hongyuanensis clone tick61 (OK625738). The groEL gene sequences obtained from the ticks shared 98.79% identity with those of Rickettsia peacockii str. Rustic (CP001227) and 100% identity with Candidatus Rickettsia hongyuanensis clone tick61 (ON409666). Rickettsia sp. JZT14 identified in the present study was closely related to Candidatus Rickettsia hongyuanensis clone tick61 (Lu et al., 2022b) and Candidatus Rickettsia principis clone Huaian-HF (Qi et al., 2022).
3.3. Anaplasma bacteria detected in ticks
In the present study, bacteria belonging to the genus Anaplasma were identified in H. longicornis, R. microplus, and H. hystricis (Table 2). Genetic and phylogenetic analyses revealed that the sequences were clustered into five main clades corresponding to five Anaplasma species: Anaplasma marginale, A. bovis, A. capra, A. platys, and Candidatus A. boleense.
Specifically, A. marginale was detected in H. longicornis and R. microplus, with positive rates of 1.8% (10/549) and 20.3% (50/246), respectively. In the 16S rRNA phylogenetic tree (Figure 4), all sequences were clustered together with those of A. marginale strains obtained from cattle (De Andrade et al., 2004) and dairy bovines from Brazil (https://www.ncbi.nlm.nih.gov/bioproject/348690). The topology of the gltA and groEL gene sequence-based phylogenetic trees was similar to that of the 16S rRNA sequence-based phylogenetic tree. The 16S rRNA, gltA, and groEL gene sequences from the strains detected in the present study shared 100%, 99.64–100%, and 99.88–100%, respectively, identity with previously reported A. marginale sequences. Candidatus A. boleense was detected in R. microplus, with a positive rate of 3.7% (9/246). In the 16S rRNA gene sequence phylogenetic tree, the sequences showed a close relationship with the species Ca. A. boleense detected in mosquitoes collected in China (Guo et al., 2016). The 16S rRNA, gltA, and groEL genes from the strains detected in ticks in the present study showed 99.29–99.67%, 98.6–100%, and 99.54–99.77%, respectively, similarity with Ca. A. boleense strains reported in China. Anaplasma capra was detected in H. longicornis and R. microplus, with positive rates of 4.9% (27/549) and 1.0% (1/96). The 16S rRNA gene sequences of A. capra obtained in this study were 100% identical to those of A. capra KWD-23 (LC432114), A. capra isolate AK-Rm-429 (MH762077), and Anaplasma sp. strain WHBMXZ-125 (KX987331) published in GenBank, indicating the same genetic lineage. In the groEL and gltA phylogenetic trees, the sequences identified in the present study showed a close relationship with those of A. capra strains detected in ticks collected in Wuhan (Lu et al., 2017) and Shanxi (Guo et al., 2019) provinces and in goats in Shaanxi province in China (Guo et al., 2018) and in water deer in Korea (Amer et al., 2019). Anaplasma bovis was detected in H. longicornis, R. microplus, and H. hystricis, with positive rates of 7.1% (39/549), 0.4% (1/246), and 9.1% (5/55), respectively. The 16S rRNA, gltA, and groEL genes showed 99.55–99.89%, 92.66–99.66%, and 95.83–99.72%, respectively, similarity with the previously reported sequences A. bovis strains. Anaplasma platys was detected in R. microplus. The 16S rRNA gene sequence of A. platys detected in this study was 100% identical to that of A. platys identified in dogs and mosquitoes in Saint Kitts and Nevis and China and that of Candidatus A. cinensis detected in ticks in China. The gltA and groEL phylogenetic trees showed that the bacterial strains detected in ticks collected in this study are clustered in the same group with A. platys and Candidatus A. cinensis. Anaplasma platys strain S3 formed a neighbor cluster with A. platys JZT2.
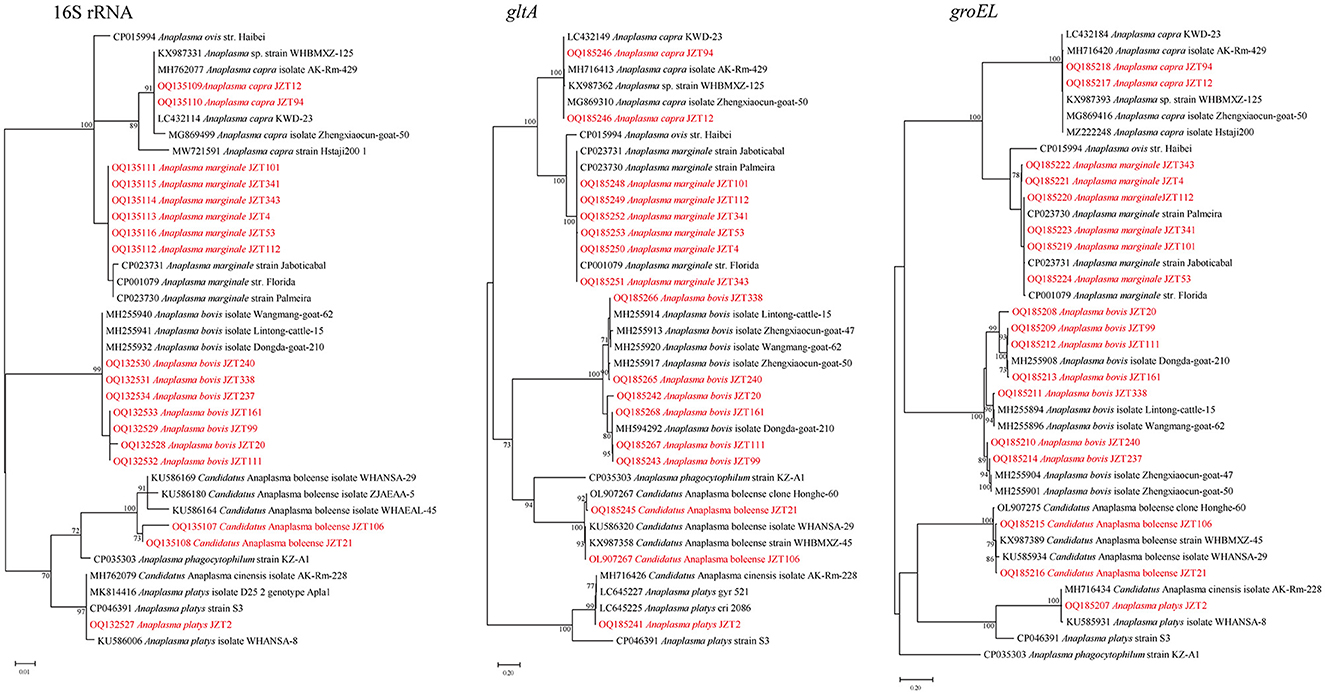
Figure 4. Phylogenetic trees constructed using PhyML 3.0 based on the nucleotide sequences of the 16S rRNA (837–1,234 bp), gltA (711–1,102 bp), and groEL (822–1,371 bp) genes of Anaplasma strains. Sequences obtained in this study are marked in red.
3.4. Ehrlichia bacteria detected in ticks
Bacteria belonging to the genus Ehrlichia were detected in H. longicornis, R. microplus, and H. hystricis (Table 2). The phylogenetic trees of sequences of the target genes amplified by PCR in this study showed that two classes of trees about Ehrlichia bacteria were established, corresponding to three known and three tentative species of Ehrlichia.
Ehrlichia minasensis was detected in R. microplus (11.8%, 29/246). The 16S rRNA gene sequences obtained in this study were 100% similar to that of the E. minasensis strain UFMG-EV published in GenBank, whereas the gltA and groEL sequences were both most closely related to the corresponding sequences of E. minasensis strain UFMG-EV (99.90% similarity for gltA, 99.74–99.91% similarity for groEL). In addition to E. minasensis, some less well-characterized strains were identified. Ehrlichia sp. JZT512, JZT51, and JZT250, which were more closely related to Ehrlichia sp. strain WHBMXZ-43, was identified in R. microplus, with a positive rate of 11.4% (28/246). The obtained 16S rRNA gene sequences were 100% similar to those of Ehrlichia sp. strain WHBMXZ-43, whereas the obtained gltA and groEL sequences showed a high similarity to the corresponding sequences obtained from this strain (100% similarity for gltA and 99.91–100% similarity for groEL). Ehrlichia sp. JZT77, JZT88, JZT234, and JZT305, which were more closely related to Ehrlichia sp. Yonaguni 138/206, were detected in H. longicornis, with a positive rate of 3.5% (19/549), respectively. In the 16S rRNA gene phylogenetic tree (Figure 5), the sequences clustered together with those of Ehrlichia sp. Yonaguni 138 was identified in H. longicornis from Japan (Kawahara et al., 2006). In the groEL gene sequence phylogenetic tree, although the sequences from Ehrlichia sp. JZT88 and JZT305 clustered together with those of Ehrlichia sp. Yonaguni138, the sequences from Ehrlichia sp. JZT77 and JZT234 were more closely related to those of Ehrlichia sp. Yonaguni 206. In the gltA gene sequence phylogenetic tree, the sequences detected in the present study clustered together and showed a relatively close relationship with those of Ehrlichia sp. strain WHHLXZ-117 (Lu et al., 2017) identified in ticks collected in China.
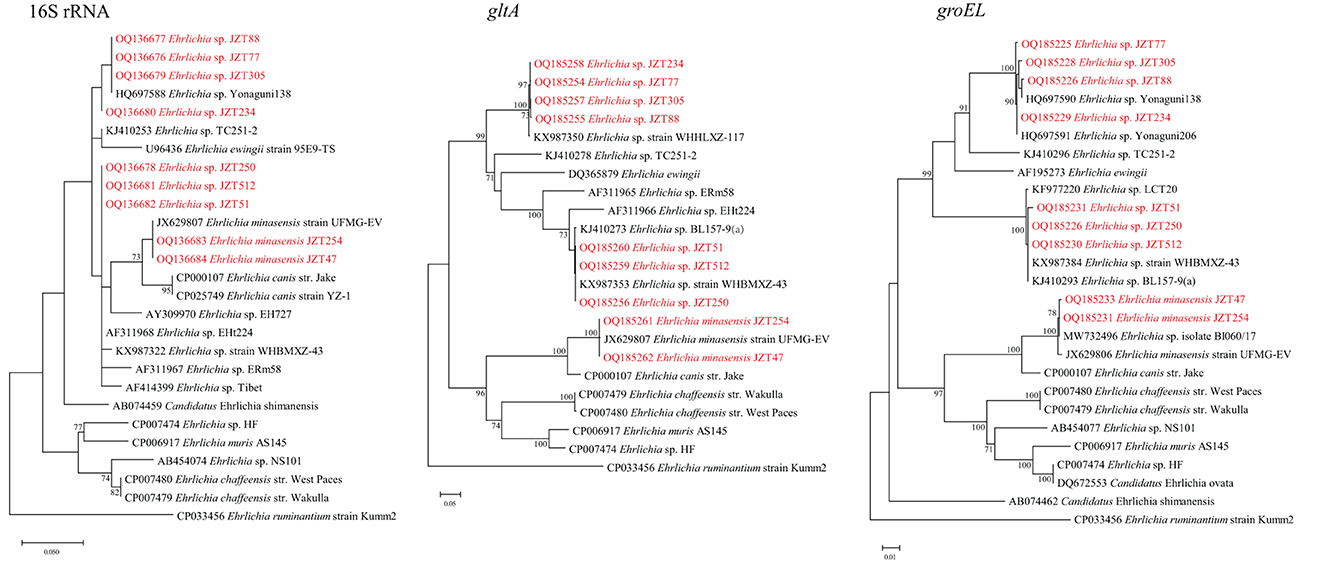
Figure 5. Phylogenetic trees constructed using PhyML 3.0 based on the nucleotide sequences of the 16S rRNA (1,198 bp), gltA (977 bp), and groEL (1,111 bp) genes of Ehrlichia strains. Sequences obtained in this study are marked in red.
Notably, we found that several sequences detected only in the ticks collected in 2022 differed from those deposited in GenBank. To further ascertain the genetic evolutionary relationship and relationship between the Ehrlichia bacteria identified in this study and previously reported Ehrlichia bacteria (based on NCBI sequences), phylogenetic analyses were conducted (Figure 6) based on longer fragments of the 16S rRNA, gltA, and groEL genes. The analysis possibly demonstrated the presence of three potentially novel Ehrlichia variants. Two uncultured Ehrlichia sp. (JZT87 and JZT90) from H. longicornis in all phylogenetic trees were observed to be closely related to Candidatus Ehrlichia shimanensis, with 99.78–99.93% and 93.93–93.97% similarity in 16S rRNA and groEL sequences, respectively. The phylogenetic trees based on the sequences of 16S rRNA, gltA, and groEL showed that Ehrlichia sp. JZT19 detected in H. hystricis was more closely related to species included in clades associated with E. chaffeensis, including Ehrlichia sp. HF (98.46%, 88.32%, and 94.82% similarity for 16S rRNA, gltA, and groEL, respectively), E. muris AS145 (98.67%, 88.95%, and 94.66% similarity for 16S rRNA, gltA, and groEL, respectively), and E. chaffeensis str. West Paces (98.88%, 86.13%, and 95.06% similarity for 16S rRNA, gltA, and groEL, respectively). In addition, the phylogenetic trees based on the sequences of 16S rRNA, gltA, and groEL showed that two uncultured Ehrlichia sp. (JZT22 and JZT43 from R. microplus) formed a unique clade, suggesting that they are closely related to Ehrlichia sp. ERm58, Ehrlichia minasensis strain UFMG-EV, and Ehrlichia ewingii.
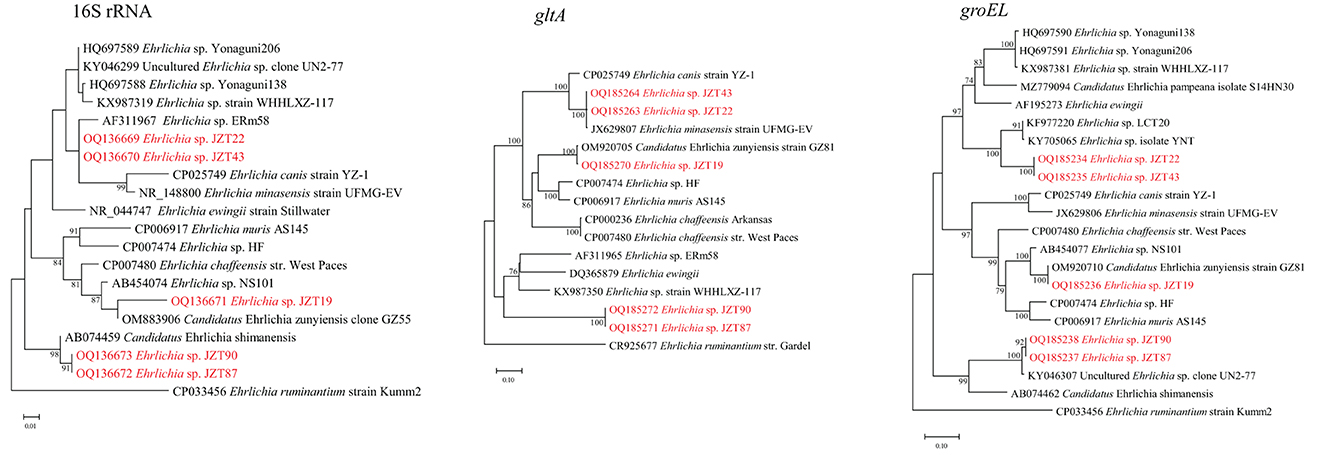
Figure 6. Phylogenetic trees constructed using PhyML 3.0 based on the nucleotide sequences of the 16S rRNA (1,433 bp), gltA (978–1,234 bp), and groEL (1,224 bp) genes of Ehrlichia strains. Sequences obtained in this study are marked in red.
4. Discussion
In the present study, the diversity of ticks collected from Jinzhai County and the rickettsial pathogens they harbored were examined using molecular techniques (PCR, DNA sequencing, and phylogenetic analysis) to improve the understanding of the genetic diversity of local ticks and tick-borne Rickettsiales bacteria. A total of 880 ticks collected from Jinzhai County in 2021–2022 were identified as belonging to H. longicornis, R. microplus, H. hystricis, H. flava, and A. testudinarium. PCR analyses targeting Rickettsiales genes (16S rRNA, gltA, and groEL) identified 13 species/variants belonging to the order Rickettsiales and the genera Rickettsia, Anaplasma, and Ehrlichia, including three tentative species of Ehrlichia. Importantly, many of the detected Rickettsiales species were closely related to known human and animal pathogens. Collectively, our findings suggest that different rickettsial species or genotypes are distributed in the region and reveal extensive diversity of Rickettsiales bacteria in ticks collected from this region.
In the present study, two Rickettsia spp.—including R. japonica and Rickettsia sp. JZT14—were detected in the ticks. R. japonica, the causative agent of Japanese spotted fever (JSF), has become increasingly prevalent in China in recent years (Li et al., 2018, 2019a; Lu et al., 2018; Yin et al., 2018; Li and Liu, 2021). Moreover, the first human death associated with R. japonica infection has recently been reported in Hubei Province in central China (Teng et al., 2023), suggesting that R. japonica poses a potential threat to human health, with serious consequences. In this study, phylogenetic analyses of bacterial sequences obtained from the ticks suggested the presence of R. japonica in the region, revealing a potential risk of JSF for local populations. In addition, we also identified a variant “Rickettsia sp. JZT14.” According to gene sequence-based criteria (Fournier et al., 2003), for a pathogen to be classified as a novel species, it should fulfill five conditions; for example, the sequence similarity of gltA with the species that is validated to be the most homologous should not exceed 99.9%. The similarity of both 16S rRNA and gltA genes in this study was higher than this criterion. Therefore, we concluded that it was not a novel species. However, additional studies are needed to verify this finding, for example, by obtaining the whole-genome sequence of the organism by isolation and culture as a way to accurately classify the rickettsial pathogen. To date, the efforts by our research group to this end have not succeeded in obtaining isolates. Emerging rickettsial bacteria may be pathogenic and cause under-recognized diseases that threaten the health of local populations. Therefore, further studies are warranted to assess the potential public health risks of this pathogen.
Anaplasma species represent globally distributed tick-borne bacteria of veterinary and public health importance. In the present study, five bacterial species belonging to the genus Anaplasma were identified: A. marginale, A. bovis, A. capra, A. platys, and Ca. A. boleense. Anaplasma marginale is the causative agent of tick-transmitted rickettsial diseases of cattle (Kocan et al., 2003). Although this pathogen commonly infects cattle, causing bovine anaplasmosis, it has also been detected in non-bovine species such as sheep (Yousefi et al., 2017) and goats (Barbosa et al., 2021). In the present study, phylogenetic analyses showed that the A. marginale sequences detected in ticks were clustered together in a single clade, suggesting that these sequences are conserved across isolates from different regions and different hosts.
Anaplasma capra, A. bovis, and A. platys detected in this study have been identified as human and animal pathogens (Maggi et al., 2013; Arraga-Alvarado et al., 2014; Li et al., 2015a; Lu et al., 2019). Anaplasma capra is widely distributed in China, Turkey, Kyrgyzstan, and Korea and has been identified in H. longicornis, water buffalo, sheep, goats, and dogs (Lee et al., 2005; Zhang et al., 2017; Han et al., 2019; Shi et al., 2019; Sahin et al., 2022). A. capra was first identified in 2015 as a novel Anaplasma species found to cause human infection in China (Li et al., 2015a). The present study is the first to detect A. capra DNA in ticks collected from goats in Anhui Province, confirming the presence of this pathogen in this region and the potential risk to local residents. In 2017, a case of human anaplasmosis caused by A. bovis, which was previously thought to be a bovine-specific pathogen, was reported in China (Lu et al., 2019). In previous studies, A. bovis infection has been reported in domestic small ruminants in Anhui Province (Yang et al., 2019), suggesting that A. bovis is commonly found in Anhui Province. Anaplasma platys, a widely distributed pathogen, is the causative agent of canine cyclic thrombocytopenia affecting cats (Lima et al., 2010) and some ruminants such as cattle, goats, camels, buffalo, and red deer (Chochlakis et al., 2009; Dahmani et al., 2015; Li et al., 2015b; Lorusso et al., 2016a,b; Machado et al., 2016). In 2013, the first case of A. platys infection in humans was confirmed by DNA sequencing (Maggi et al., 2013). Another study (Arraga-Alvarado et al., 2014) provided additional molecular evidence for A. platys infections in humans, suggesting that A. platys is a potential zoonotic pathogen. A previous study in Wuhu reported the presence of A. platys in stray dogs (Yang et al., 2020). In the present study, we detected A. platys DNA in ticks collected from cattle, indicating a potential risk of zoonotic infections in humans who are in regular contact with their cattle. Three of the five pathogenic bacterial species detected in the present study—A. marginale, A. bovis, and A. capra—were detected in samples collected in both 2021 and 2022, indicating the sustained presence of Anaplasma infection at the study sites. In addition, the transmission intensity of some pathogens belonging to the order Rickettsiales fluctuates throughout the year and from year to year, even in the same region. Long-term surveys with a large sample size are needed to investigate the dynamic changes in pathogen prevalence.
Ehrlichia species are responsible for life-threatening emerging human zoonoses and diseases of veterinary concern worldwide (Esemu et al., 2011). The present study reports the detection of well-known pathogens and unclassified genetic variants in this genus. Ehrlichia minasensis, a new genotype phylogenetically close to Ehrlichia canis (Cruz et al., 2012; Aguiar et al., 2014), has been reported in not only bovines, cervids, and dogs but also several tick species from China, Canada, Brazil, France, Pakistan, Ethiopia, and Israel (Li et al., 2019b). Although the specific species of ticks that transmit this pathogen has not been identified, the detection and transmission of E. minasensis by R. microplus have been documented (Cabezas-Cruz et al., 2016). The present study is the first to report that E. minasensis exists in Anhui Province. The detection of this species suggests that it circulates in cattle in Jinzhai County, and a seroepidemiological investigation of local cattle is needed to understand its prevalence in affected animals. In the present study, uncultured strains belonging to two Ehrlichia spp. (Ehrlichia sp. JZT77, JZT88, JZT234, and JZT305 and Ehrlichia sp. JZT512, JZT51, and JZT250) were detected in this study. As shown in Figure 5, Ehrlichia sp. JZT77, JZT88, JZT234, and JZT305 were clustered together with Ehrlichia sp. Yonaguni 138/206 found in H. longicornis from Japan (Kawahara et al., 2006) and Ehrlichia sp. strain WHHLXZ-117 (Lu et al., 2017) found in ticks from China. In contrast, Ehrlichia sp. JZT512, JZT51, and JZT250 were more closely related to Ehrlichia sp. strain WHBMXZ-43 identified in R. microplus from Wuhan, China. Nevertheless, the pathogenicity of these Ehrlichia strains in humans has not been reported. As previously mentioned, the present study demonstrated three newly identified Ehrlichia members. These tick-borne pathogens may represent a serious public health threat to humans. In addition, many pathogens that were previously considered non-pathogenic are now commonly associated with human diseases (Jia et al., 2013). Thus, even microorganisms of unknown pathogenicity pose a potential and considerable risk of causing emerging diseases. Therefore, isolating new microorganisms from these tick species and examining their potential pathogenicity in humans is of great significance. In the future, our research group aims to isolate the Rickettsiales bacteria identified in the present study to examine their pathogenicity.
It should be noted that our study has several limitations. We cannot conclude whether the entirety of the microbial DNA detected originated from the ticks or whether the results may be influenced by the previous blood meal of the ticks. In addition, the method used for phylogenetic tree construction in this study was a maximum likelihood, which is the optimal algorithm when the evolutionary model is chosen reasonably; however, it is computationally intensive and time-consuming.
5. Conclusion
In the present study, we collected tick specimens for two consecutive years to detect tick-borne Rickettsiales pathogens and recognize the diversity of Rickettsiales in the study area. Early detection and identification of potential rickettsial pathogens are essential for improving response measures and preventing associated zoonotic diseases. The present findings demonstrate the need for constant surveillance of Rickettsiales pathogens in ticks in China to assess the potential risk of transmission of disease-causing pathogens or colonizing species in vector organisms to humans.
Data availability statement
The datasets presented in this study can be found in online repositories. The names of the repository/repositories and accession number(s) can be found below: https://www.ncbi.nlm.nih.gov/genbank/, OQ132525-OQ132534, OQ135107-OQ135116, OQ135123-OQ135128, OQ135142-OQ135169, OQ136669-OQ136674, OQ136676-OQ136684, and OQ185204-OQ185272.
Author contributions
XJ, JL, TQ, and YS provided the idea, designed the study, and drafted the manuscript. QC, JD, HC, and YL performed the sample collection and laboratory work. LY performed the experimental data analysis. TQ, YS, and BW revised the manuscript. All authors contributed to the article and approved the submitted version.
Funding
This work was supported by grants from the National Natural Science Foundation of China (grant number 81671985), the Science Foundation for the State Key Laboratory for Infectious Disease Prevention and Control of China (grant numbers 2022SKLID209 and 2019SKLID403), and the Public Health Service Capability Improvement Project of the National Health Commission of the People's Republic of China (grant number 2100409034).
Conflict of interest
The authors declare that the research was conducted in the absence of any commercial or financial relationships that could be construed as a potential conflict of interest.
Publisher's note
All claims expressed in this article are solely those of the authors and do not necessarily represent those of their affiliated organizations, or those of the publisher, the editors and the reviewers. Any product that may be evaluated in this article, or claim that may be made by its manufacturer, is not guaranteed or endorsed by the publisher.
Supplementary material
The Supplementary Material for this article can be found online at: https://www.frontiersin.org/articles/10.3389/fmicb.2023.1141217/full#supplementary-material
Supplementary Figure 1. Phylogenetic trees based on partial Rickettsiales 16S rRNA sequences using the ML method with PhyML 3.0. Sequences of Rickettsia strains obtained in this study are marked in red; sequences of Ehrlichia strains obtained in this study are marked in blue; and sequences of Anaplasma strains obtained in this study are marked in green.
Supplementary Figure 2. Phylogenetic trees based on partial Rickettsiales gltA sequences using the ML method with PhyML 3.0. Sequences of Rickettsia strains obtained in this study are marked in red; sequences of Ehrlichia strains obtained in this study are marked in blue; and sequences of Anaplasma strains obtained in this study are marked in green.
Supplementary Figure 3. Phylogenetic trees based on partial Rickettsiales groEL sequences using the ML method with PhyML 3.0. Sequences of Rickettsia strains obtained in this study are marked in red; sequences of Ehrlichia strains obtained in this study are marked in blue; and sequences of Anaplasma strains obtained in this study are marked in green.
Supplementary Table 1. The primers used for the amplification of 16S, gltA, and groEL genes by PCR, nested PCR, or semi-nested PCR.
Supplementary Table 2. The species, numbers, and origins of ticks collected from Jinzhai County.
References
Aguiar, D. M., Ziliani, T. F., Zhang, X., Melo, A. L., Braga, I. A., Witter, R., et al. (2014). A novel Ehrlichia genotype strain distinguished by the TRP36 gene naturally infects cattle in Brazil and causes clinical manifestations associated with ehrlichiosis. Ticks Tick Borne Dis. 5, 537–544. doi: 10.1016/j.ttbdis.2014.03.010
Amer, S., Kim, S., Yun, Y., and Na, K. J. (2019). Novel variants of the newly emerged Anaplasma capra from Korean water deer (Hydropotes inermis argyropus) in South Korea. Parasit. Vect. 12, 365. doi: 10.1186/s13071-019-3622-5
Arraga-Alvarado, C. M., Qurollo, B. A., Parra, O. C., Berrueta, M. A., Hegarty, B. C., and Breitschwerdt, E. B. (2014). Case report: molecular evidence of Anaplasma platys infection in two women from Venezuela. Am. J. Trop. Med. Hyg. 91, 1161–1165. doi: 10.4269/ajtmh.14-0372
Azmat, M., Ijaz, M., Farooqi, S. H., Ghaffar, A., Ali, A., Masud, A., et al. (2018). Molecular epidemiology, associated risk factors, and phylogenetic analysis of anaplasmosis in camel. Microb. Pathog. 123, 377–384. doi: 10.1016/j.micpath.2018.07.034
Barbosa, I. C., André, M. R., Amaral, R. B. D., Valente, J. D. M., Vasconcelos, P. C., Oliveira, C. J. B., et al. (2021). Anaplasma marginale in goats from a multispecies grazing system in northeastern Brazil. Ticks Tick Borne Dis. 12, 101592. doi: 10.1016/j.ttbdis.2020.101592
Barlough, J. E., Madigan, J. E., Derock, E., and Bigornia, L. (1996). Nested polymerase chain reaction for detection of Ehrlichia equi genomic DNA in horses and ticks (Ixodes pacificus). Vet. Parasitol. 63, 319–329. doi: 10.1016/0304-4017(95)00904-3
Beati, L., Meskini, M., Thiers, B., and Raoult, D. (1997). Rickettsia aeschlimannii sp. nov., a new spotted fever group rickettsia associated with Hyalomma marginatum ticks. Int. J. Syst. Bacteriol. 47:548–554. doi: 10.1099/00207713-47-2-548
Cabezas-Cruz, A., Zweygarth, E., Vancová, M., Broniszewska, M., Grubhoffer, L., Passos, L. M. F., et al. (2016). Ehrlichia minasensis sp. nov., isolated from the tick Rhipicephalus microplus. Int. J. Syst. Evol. Microbiol. 66, 1426–1430. doi: 10.1099/ijsem.0.000895
Cao, W. C., Zhao, Q. M., Zhang, P. H., Dumler, J. S., Zhang, X. T., Fang, L. Q., et al. (2000). Granulocytic Ehrlichiae in Ixodes persulcatus ticks from an area in China where Lyme disease is endemic. J. Clin. Microbiol. 38, 4208–4210. doi: 10.1128/JCM.38.11.4208-4210.2000
Cao, W. C., Zhao, Q. M., Zhang, P. H., Yang, H., Wu, X. M., Wen, B. H., et al. (2003). Prevalence of Anaplasma phagocytophila and Borrelia burgdorferi in Ixodes persulcatus ticks from northeastern China. Am. J. Trop. Med. Hyg. 68, 547–550. doi: 10.4269/ajtmh.2003.68.547
Chochlakis, D., Ioannou, I., Sharif, L., Kokkini, S., Hristophi, N., Dimitriou, T., et al. (2009). Prevalence of Anaplasma sp. in goats and sheep in Cyprus. Vect. Borne Zoonot. Dis. 9, 457–463. doi: 10.1089/vbz.2008.0019
Cruz, A. C., Zweygarth, E., Ribeiro, M. F., Da Silveira, J. A., De La Fuente, J., Grubhoffer, L., et al. (2012). New species of Ehrlichia isolated from Rhipicephalus (Boophilus) microplus shows an ortholog of the E. canis major immunogenic glycoprotein gp36 with a new sequence of tandem repeats. Parasit. Vect. 5, 291. doi: 10.1186/1756-3305-5-291
Dahmani, M., Davoust, B., Benterki, M. S., Fenollar, F., Raoult, D., and Mediannikov, O. (2015). Development of a new PCR-based assay to detect Anaplasmataceae and the first report of Anaplasma phagocytophilum and Anaplasma platys in cattle from Algeria. Comp. Immunol. Microbiol. Infect. Dis. 39, 39–45. doi: 10.1016/j.cimid.2015.02.002
Dantas-Torres, F., Chomel, B. B., and Otranto, D. (2012). Ticks and tick-borne diseases: a one health perspective. Trends Parasitol. 28, 437–446. doi: 10.1016/j.pt.2012.07.003
De Andrade, G. M., Machado, R. Z., Vidotto, M. C., and Vidotto, O. (2004). Immunization of bovines using a DNA vaccine (pcDNA3.1/MSP1b) prepared from the Jaboticabal strain of Anaplasma marginale. Ann. N. Y. Acad. Sci. 1026, 257–266. doi: 10.1196/annals.1307.040
De La Fuente, J., Estrada-Pena, A., Venzal, J. M., Kocan, K. M., and Sonenshine, D. E. (2008). Overview: ticks as vectors of pathogens that cause disease in humans and animals. Front. Biosci. 13, 6938–6946. doi: 10.2741/3200
Esemu, S. N., Ndip, L. M., and Ndip, R. N. (2011). Ehrlichia species, probable emerging human pathogens in sub-Saharan Africa: environmental exacerbation. Rev. Environ. Health 26, 269–279. doi: 10.1515/REVEH.2011.034
Fournier, P. E., Dumler, J. S., Greub, G., Zhang, J., Wu, Y., and Raoult, D. (2003). Gene sequence-based criteria for identification of new rickettsia isolates and description of Rickettsia heilongjiangensis sp. nov. J. Clin. Microbiol. 41, 5456–5465. doi: 10.1128/JCM.41.12.5456-5465.2003
Guindon, S., Dufayard, J. F., Lefort, V., Anisimova, M., Hordijk, W., and Gascuel, O. (2010). New algorithms and methods to estimate maximum-likelihood phylogenies: assessing the performance of PhyML 3.0. Syst. Biol. 59, 307–321. doi: 10.1093/sysbio/syq010
Guo, W. P., Huang, B., Zhao, Q., Xu, G., Liu, B., Wang, Y. H., et al. (2018). Human-pathogenic Anaplasma spp., and Rickettsia spp. in animals in Xi'an, China. PLoS Negl. Trop. Dis. 12, e0006916. doi: 10.1371/journal.pntd.0006916
Guo, W. P., Tian, J. H., Lin, X. D., Ni, X. B., Chen, X. P., Liao, Y., et al. (2016). Extensive genetic diversity of Rickettsiales bacteria in multiple mosquito species. Sci. Rep. 6, 38770. doi: 10.1038/srep38770
Guo, W. P., Tie, W. F., Meng, S., Li, D., Wang, J. L., Du, L. Y., et al. (2020). Extensive genetic diversity of Anaplasma bovis in ruminants in Xi'an, China. Ticks Tick Borne Dis. 11, 101477. doi: 10.1016/j.ttbdis.2020.101477
Guo, W. P., Zhang, B., Wang, Y. H., Xu, G., Wang, X., Ni, X., et al. (2019). Molecular identification and characterization of Anaplasma capra and Anaplasma platys-like in Rhipicephalus microplus in Ankang, Northwest China. BMC Infect. Dis. 19, 434. doi: 10.1186/s12879-019-4075-3
Han, R., Yang, J. F., Mukhtar, M. U., Chen, Z., Niu, Q. L., Lin, Y. Q., et al. (2019). Molecular detection of Anaplasma infections in ixodid ticks from the Qinghai-Tibet Plateau. Infect. Dis. Poverty 8, 12. doi: 10.1186/s40249-019-0522-z
Jafar Bekloo, A., Ramzgouyan, M. R., Shirian, S., Faghihi, F., Bakhshi, H., Naseri, F., et al. (2018). Molecular characterization and phylogenetic analysis of Anaplasma spp. and Ehrlichia spp. isolated from various ticks in southeastern and northwestern regions of Iran. Vect. Borne Zoonot. Dis. 18, 252–257. doi: 10.1089/vbz.2017.2219
Jia, N., Jiang, J. F., Huo, Q. B., Jiang, B. G., and Cao, W. C. (2013). Rickettsia sibirica subspecies sibirica BJ-90 as a cause of human disease. N. Engl. J. Med. 369, 1176–1178. doi: 10.1056/NEJMc1303625
Kawahara, M., Rikihisa, Y., Lin, Q., Isogai, E., Tahara, K., Itagaki, A., et al. (2006). Novel genetic variants of Anaplasma phagocytophilum, Anaplasma bovis, Anaplasma centrale, and a novel Ehrlichia sp. in wild deer and ticks on two major islands in Japan. Appl. Environ. Microbiol. 72, 1102–1109. doi: 10.1128/AEM.72.2.1102-1109.2006
Kim, Y. J., Seo, J. Y., Kim, S. Y., and Lee, H. I. (2022). Molecular detection of Anaplasma phagocytophilum and Ehrlichia species in ticks removed from humans in the Republic of Korea. Microorganisms 10, 1224. doi: 10.3390/microorganisms10061224
Kocan, K. M., De La Fuente, J., Guglielmone, A. A., and Meléndez, R. D. (2003). Antigens and alternatives for control of Anaplasma marginale infection in cattle. Clin. Microbiol. Rev. 16, 698–712. doi: 10.1128/CMR.16.4.698-712.2003
Lee, S. O., Na, D. K., Kim, C. M., Li, Y. H., Cho, Y. H., Park, J. H., et al. (2005). Identification and prevalence of Ehrlichia chaffeensis infection in Haemaphysalis longicornis ticks from Korea by PCR, sequencing and phylogenetic analysis based on 16S rRNA gene. J. Vet. Sci. 6, 151–155. doi: 10.4142/jvs.2005.6.2.151
Li, H., Zhang, P. H., Du, J., Yang, Z. D., Cui, N., Xing, B., et al. (2019a). Rickettsia japonica Infections in Humans, Xinyang, China, 2014–2017. Emerg. Infect. Dis. 25, 1719–1722. doi: 10.3201/eid2509.171421
Li, H., Zheng, Y. C., Ma, L., Jia, N., Jiang, B. G., Jiang, R. R., et al. (2015a). Human infection with a novel tick-borne Anaplasma species in China: a surveillance study. Lancet Infect. Dis. 15, 663–670. doi: 10.1016/S1473-3099(15)70051-4
Li, J., Hu, W., Wu, T., Li, H. B., Hu, W., Sun, Y., et al. (2018). Japanese Spotted Fever in Eastern China, 2013. Emerg. Infect. Dis. 24, 2107–2109. doi: 10.3201/eid2411.170264
Li, J., Kelly, P., Guo, W., Zhang, J., Yang, Y., Liu, W., et al. (2020). Molecular detection of Rickettsia, Hepatozoon, Ehrlichia and SFTSV in goat ticks. Vet. Parasitol. Reg. Stud. Rep. 20, 100407. doi: 10.1016/j.vprsr.2020.100407
Li, J., Liu, X., Mu, J., Yu, X., Fei, Y., Chang, J., et al. (2019b). Emergence of a novel Ehrlichia minasensis Strain, harboring the major immunogenic glycoprotein trp36 with unique tandem repeat and C-terminal region sequences, in Haemaphysalis hystricis ticks removed from free-ranging sheep in Hainan Province, China. Microorganisms 7, 1559. doi: 10.3390/microorganisms7090369
Li, W., and Liu, S. N. (2021). Rickettsia japonica infections in Huanggang, China, in 2021. IDCases. 26, e01309. doi: 10.1016/j.idcr.2021.e01309
Li, Y., Yang, J., Chen, Z., Qin, G., Li, Y., Li, Q., et al. (2015b). Anaplasma infection of Bactrian camels (Camelus bactrianus) and ticks in Xinjiang, China. Parasit. Vect. 8, 313. doi: 10.1186/s13071-015-0931-1
Lima, M. L., Soares, P. T., Ramos, C. A., Araújo, F. R., Ramos, R. A., Souza, I.i, et al. (2010). Molecular detection of Anaplasma platys in a naturally-infected cat in Brazil. Braz. J. Microbiol. 41, 381–385. doi: 10.1590/S1517-83822010000200019
Loftis, A. D., Reeves, W. K., Spurlock, J. P., Mahan, S. M., Troughton, D. R., Dasch, G. A., et al. (2006). Infection of a goat with a tick-transmitted Ehrlichia from Georgia, U.S.A., that is closely related to Ehrlichia ruminantium. J. Vect. Ecol. 31, 213–223. doi: 10.3376/1081-1710(2006)31213:IOAGWA2.0.CO;2
Lorusso, V., Wijnveld, M., Latrofa, M. S., Fajinmi, A., Majekodunmi, A. O., Dogo, A. G., et al. (2016a). Canine and ovine tick-borne pathogens in camels, Nigeria. Vet. Parasitol. 228, 90–92. doi: 10.1016/j.vetpar.2016.08.014
Lorusso, V., Wijnveld, M., Majekodunmi, A. O., Dongkum, C., Fajinmi, A., Dogo, A. G., et al. (2016b). Tick-borne pathogens of zoonotic and veterinary importance in Nigerian cattle. Parasit. Vect. 9, 217. doi: 10.1186/s13071-016-1504-7
Lu, M., Li, F., Liao, Y., Shen, J. J., Xu, J. M., Chen, Y. Z., et al. (2019). Epidemiology and diversity of rickettsiales bacteria in humans and animals in Jiangsu and Jiangxi provinces, China. Sci. Rep. 9, 13176. doi: 10.1038/s41598-019-49059-3
Lu, M., Meng, C., Gao, X., Sun, Y., Zhang, J., Tang, G., et al. (2022a). Diversity of Rickettsiales in Rhipicephalus microplus ticks collected in domestic ruminants in Guizhou Province, China. Pathogens 11, 1108. doi: 10.3390/pathogens11101108
Lu, M., Tian, J., Zhao, H., Jiang, H., Qin, X., Wang, W., et al. (2022b). Molecular survey of vector-borne pathogens in ticks, sheep keds, and domestic animals from Ngawa, Southwest China. Pathogens 11, 606. doi: 10.3390/pathogens11050606
Lu, M., Tian, J. H., Yu, B., Guo, W. P., Holmes, E. C., and Zhang, Y. Z. (2017). Extensive diversity of rickettsiales bacteria in ticks from Wuhan, China. Ticks Tick Borne Dis. 8, 574–580. doi: 10.1016/j.ttbdis.2017.03.006
Lu, Q., Yu, J., Yu, L., Zhang, Y., Chen, Y., Lin, M., et al. (2018). Rickettsia japonica Infections in Humans, Zhejiang Province, China, 2015. Emerg. Infect. Dis. 24, 2077–2079. doi: 10.3201/eid2411.170044
Luce-Fedrow, A., Mullins, K., Kostik, A. P., St John, H. K., Jiang, J., and Richards, A. L. (2015). Strategies for detecting rickettsiae and diagnosing rickettsial diseases. Fut. Microbiol. 10, 537–564. doi: 10.2217/fmb.14.141
Machado, R. Z., Teixeira, M. M., Rodrigues, A. C., André, M. R., Gonçalves, L. R., Barbosa Da Silva, J., et al. (2016). Molecular diagnosis and genetic diversity of tick-borne Anaplasmataceae agents infecting the African buffalo Syncerus caffer from Marromeu Reserve in Mozambique. Parasit. Vect. 9, 454. doi: 10.1186/s13071-016-1715-y
Maggi, R. G., Mascarelli, P. E., Havenga, L. N., Naidoo, V., and Breitschwerdt, E. B. (2013). Co-infection with Anaplasma platys, Bartonella henselae and Candidatus Mycoplasma haematoparvum in a veterinarian. Parasit. Vect. 6, 103. doi: 10.1186/1756-3305-6-103
Matos, A. L., Curto, P., and Simões, I. (2022). Moonlighting in Rickettsiales: expanding virulence landscape. Trop. Med. Infect. Dis. 7, 32. doi: 10.3390/tropicalmed7020032
Nicholson, W. L., Allen, K. E., Mcquiston, J. H., Breitschwerdt, E. B., and Little, S. E. (2010). The increasing recognition of rickettsial pathogens in dogs and people. Trends Parasitol. 26, 205–212. doi: 10.1016/j.pt.2010.01.007
Parola, P., Paddock, C. D., Socolovschi, C., Labruna, M. B., Mediannikov, O., Kernif, T., et al. (2013). Update on tick-borne rickettsioses around the world: a geographic approach. Clin. Microbiol. Rev. 26, 657–702. doi: 10.1128/CMR.00032-13
Piotrowski, M., and Rymaszewska, A. (2020). Expansion of tick-borne Rickettsioses in the world. Microorganisms 8, 1906. doi: 10.3390/microorganisms8121906
Qi, Y., Ai, L., Jiao, J., Wang, J., Wu, D., Wang, P., et al. (2022). High prevalence of Rickettsia spp. in ticks from wild hedgehogs rather than domestic bovine in Jiangsu province, Eastern China. Front. Cell Infect. Microbiol. 12, 954785. doi: 10.3389/fcimb.2022.954785
Rar, V., and Golovljova, I. (2011). Anaplasma, Ehrlichia, and “Candidatus Neoehrlichia” bacteria: pathogenicity, biodiversity, and molecular genetic characteristics—a review. Infect. Genet. Evol. 11, 1842–1861. doi: 10.1016/j.meegid.2011.09.019
Rar, V. A., Pukhovskaya, N. M., Ryabchikova, E. I., Vysochina, N. P., Bakhmetyeva, S. V., Zdanovskaia, N. I., et al. (2015). Molecular-genetic and ultrastructural characteristics of “Candidatus Ehrlichia khabarensis,” a new member of the Ehrlichia genus. Ticks Tick Borne Dis. 6, 658–667. doi: 10.1016/j.ttbdis.2015.05.012
Sahin, O. F., Erol, U., and Altay, K. (2022). Buffaloes as new hosts for Anaplasma capra: molecular prevalence and phylogeny based on gtlA, groEL, and 16S rRNA genes. Res. Vet. Sci. 152, 458–464. doi: 10.1016/j.rvsc.2022.09.008
Salje, J. (2021). Cells within cells: Rickettsiales and the obligate intracellular bacterial lifestyle. Nat. Rev. Microbiol. 19, 375–390. doi: 10.1038/s41579-020-00507-2
Shi, K., Li, J., Yan, Y., Chen, Q., Wang, K., Zhou, Y., et al. (2019). Dogs as new hosts for the emerging zoonotic pathogen Anaplasma capra in China. Front. Cell Infect. Microbiol. 9, 394. doi: 10.3389/fcimb.2019.00394
Sun, J., Lin, J., Gong, Z., Chang, Y., Ye, X., Gu, S., et al. (2015). Detection of spotted fever group Rickettsiae in ticks from Zhejiang Province, China. Exp. Appl. Acarol. 65, 403–411. doi: 10.1007/s10493-015-9880-9
Teng, Z., Gong, P., Wang, W., Zhao, N., Jin, X., Sun, X., et al. (2023). Clinical forms of Japanese Spotted Fever from case-series study, Zigui County, Hubei Province, China, 2021. Emerg. Infect. Dis. 29, 202–206. doi: 10.3201/eid2901.220639
Yang, B., Sun, E., Wen, Y., Ye, C., Liu, F., Jiang, P., et al. (2019). Molecular evidence of coinfection of Anaplasma species in small ruminants from Anhui Province, China. Parasitol. Int. 71, 143–146. doi: 10.1016/j.parint.2019.04.004
Yang, B., Ye, C., Sun, E., Wen, Y., Qian, D., and Sun, H. (2020). First molecular evidence of Anaplasma spp. co-infection in stray dogs from Anhui, China. Acta Trop. 206, 105453. doi: 10.1016/j.actatropica.2020.105453
Yang, J., Liu, Z., Niu, Q., Liu, J., Han, R., Guan, G., et al. (2017). A novel zoonotic Anaplasma species is prevalent in small ruminants: potential public health implications. Parasit. Vect. 10, 264. doi: 10.1186/s13071-017-2182-9
Yang, Y., Yang, Z., Kelly, P., Li, J., Ren, Y., and Wang, C. (2018). Anaplasma phagocytophilum in the highly endangered Père David's deer Elaphurus davidianus. Parasit. Vect. 11, 25. doi: 10.1186/s13071-017-2599-1
Yin, X., Guo, S., Ding, C., Cao, M., Kawabata, H., Sato, K., et al. (2018). Spotted fever group Rickettsiae in inner Mongolia, China, 2015–2016. Emerg. Infect. Dis. 24, 2105–2107. doi: 10.3201/eid2411.162094
Yousefi, A., Rahbari, S., Shayan, P., Sadeghi-Dehkordi, Z., and Bahonar, A. (2017). Molecular detection of Anaplasma marginale and Anaplasma ovis in sheep and goat in west highland pasture of Iran. Asian Pac. J. Trop. Biomed. 7, 455–459. doi: 10.1016/j.apjtb.2017.01.017
Yu, X., Jin, Y., Fan, M., Xu, G., Liu, Q., and Raoult, D. (1993). Genotypic and antigenic identification of two new strains of spotted fever group rickettsiae isolated from China. J. Clin. Microbiol. 31, 83–88. doi: 10.1128/jcm.31.1.83-88.1993
Yu, Z., Wang, H., Wang, T., Sun, W., Yang, X., and Liu, J. (2015). Tick-borne pathogens and the vector potential of ticks in China. Parasit. Vect. 8, 24. doi: 10.1186/s13071-014-0628-x
Zhang, H., Sun, Y., Jiang, H., and Huo, X. (2017). Prevalence of severe febrile and thrombocytopenic syndrome virus, Anaplasma spp. and Babesia microti in hard ticks (acari: ixodidae) from Jiaodong Peninsula, Shandong Province. Vect. Borne Zoonot. Dis. 17, 134–140. doi: 10.1089/vbz.2016.1978
Keywords: Rickettsiales, ticks, Jinzhai County, Rickettsia, Ehrlichia, Anaplasma
Citation: Jin X, Liao J, Chen Q, Ding J, Chang H, Lyu Y, Yu L, Wen B, Sun Y and Qin T (2023) Diversity of Rickettsiales bacteria in five species of ticks collected from Jinzhai County, Anhui Province, China in 2021–2022. Front. Microbiol. 14:1141217. doi: 10.3389/fmicb.2023.1141217
Received: 10 January 2023; Accepted: 17 March 2023;
Published: 28 April 2023.
Edited by:
Jens Andre Hammerl, Bundesinstitut für Risikobewertung, GermanyReviewed by:
Agustín Estrada-Peña, University of Zaragoza, SpainIoana Adriana Matei, University of Agricultural Sciences and Veterinary Medicine of Cluj-Napoca, Romania
Adrian Patalinghug Ybañez, Cebu Technological University, Philippines
Copyright © 2023 Jin, Liao, Chen, Ding, Chang, Lyu, Yu, Wen, Sun and Qin. This is an open-access article distributed under the terms of the Creative Commons Attribution License (CC BY). The use, distribution or reproduction in other forums is permitted, provided the original author(s) and the copyright owner(s) are credited and that the original publication in this journal is cited, in accordance with accepted academic practice. No use, distribution or reproduction is permitted which does not comply with these terms.
*Correspondence: Tian Qin, cWludGlhbiYjeDAwMDQwO2ljZGMuY24=; Yong Sun, Ymlvc3VueW9uZyYjeDAwMDQwOzE2My5jb20=; Bohai Wen, Ym9oYWl3ZW4mI3gwMDA0MDtzb2h1LmNvbQ==
†These authors have contributed equally to this work and share first authorship