- State Key Laboratory for Agrobiotechnology and College of Biological Sciences, China Agricultural University, Beijing, China
Nitrogenase in some bacteria and archaea catalyzes conversion of N2 to ammonia. To reconstitute a nitrogenase biosynthetic pathway in a eukaryotic host is still a challenge, since synthesis of nitrogenase requires a large number of nif (nitrogen fixation) genes. Viral 2A peptide mediated “cleavage” of polyprotein is one of strategies for multigene co-expression. Here, we show that cleavage efficiency of NifB-2A-NifH polyprotein linked by four different 2A peptides (P2A, T2A, E2A, and F2A) in Saccharomyces cerevisiae ranges from ~50% to ~90%. The presence of a 2A tail in NifB, NifH, and NifD does not affect their activity. Western blotting shows that 9 Nif proteins (NifB, NifH, NifD, NifK, NifE, NifN, NifX, HesA, and NifV) from Paenibacillus polymyxa that are fused into two polyproteins via 2A peptides are co-expressed in S. cerevisiae. Expressed NifH from Klebsiella oxytoca NifU and NifS and P. polymyxa NifH fusion linked via 2A in S. cerevisiae exhibits Fe protein activity.
Introduction
Although nitrogen is abundant in the atmosphere as N2 on earth, the N2 is not easily available to living organisms, and the bio-available nitrogen (such as ammonia and nitrogen oxides) limits the productivity of major crops (Hoffman et al., 2014). The addition of industrially produced nitrogenous fertilizer can maintain crop productivity, but overuse of chemical nitrogen fertilizers leads to economic costs and environmental pollution (Zhang et al., 2015). One way to reduce use of fertilizers is to transfer biological nitrogen fixation to cereal crops that can fix nitrogen (Beatty and Good, 2011; Curatti and Rubio, 2014; Oldroyd and Dixon, 2014; Vicente and Dean, 2017; Good, 2018; Li and Chen, 2020).
Biological nitrogen fixation, mainly carried out by Mo nitrogenase enzyme in some bacteria and archaea, is a process in which atmospheric N2 is converted to ammonia (Dos et al., 2012; He et al., 2022). Nitrogenase consists of two metalloprotein components: Fe protein and MoFe protein (Georgiadis et al., 1992). Fe protein, also termed as NifH protein, is a homodimer (encoded by nifH) bridged by an intersubunit [4Fe-4S] cluster that serves as the obligate electron donor to the MoFe protein. MoFe protein, also termed as NifDK protein, is a heterotetramer (encoded by nifD and nifK) that contains two metalloclusters: FeMo-cofactor (Mo − 7Fe−9S − C − homocitrate) that serves as the active site of substrate binding and reduction, and the P cluster (8Fe−7S) that shuttles electrons to FeMo-co (Burén et al., 2020). Apart from nifH, nifD and nifK encode the structural subunits of nitrogenase, several genes are required for the biosynthesis of the metalloclusters. nifU and nifS are involved in synthesis of Fe-S cluster (Dos Santos et al., 2007). nifF and nifJ are involved in transport of electron. nifE, nifN, nifX, nifB, nifQ, nifV, nifY, and nifH contribute to the synthesis and insertion of FeMo-co into nitrogenase, and nifM is required for proper folder of nitrogenase Fe protein (Roberts et al., 1978; Gavini et al., 2006; Rubio and Ludden, 2008; Hu and Ribbe, 2011). The nif genes required for synthesis of nitrogenase vary greatly amongst species. For example, in Klebsiella oxytoca, 20 nif genes (JHDKTYENXUSVWZMFLABQ) are co-located within a ∼ 24 kb cluster (Arnold et al., 1988). Whereas, Paenibacillus polymyxa WLY78 has a minimal nif gene cluster composed of nine genes (nifBHDKENXhesAnifV). The nif gene cluster from P. polymyxa WLY78 enabled Escherichia coli to synthesize active nitrogenase (Wang et al., 2013). The four genes (nifS, nifU, nifF and nifJ) from K. oxytoca could enhance the nitrogenase activity of the recombinant Escherichia coli 78–7 carrying the P. polymyxa nif cluster (Li et al., 2016).
Saccharomyces cerevisiae, a eukaryotic organism, is widely used as a host for heterologous expression of metabolite biosynthetic pathway. Recently, several nif genes from different diazotrophic bacteria and archaea have been successfully expressed in S. cerevisiae. The active NifH was generated in S. cerevisiae by expressing the 4 genes nifH, nifU, nifS, and nifM from Azotobacter vinelandii. Of the four genes, nifH and nifM are cloned in one plasmid vector, and nifS and nifU are cloned in another plasmid vector with each gene being under control of a promoter and then the two recombinant plasmids were co-transformed into yeast to express the four Nif proteins (López-Torrejón et al., 2016). The NifDK tetramer with a smaller molecular weight than that of A. vinelandii was formed in S. cerevisiae by expressing the 9 genes (nifH, nifD, nifK, nifU, nifS, nifM, nifE, nifN, and nifB) from A. vinelandii (Buren et al., 2017). To express the nine nif genes in yeast, constraint-based combinatorial design and DNA assembly are used to build libraries of nif gene clusters, and then promoters and terminators were combined to the nine nif genes to generate nine transcript units via Type IIS assembly (Weber et al., 2011). The nine transcription units were assembled into three subclusters that contain homologous sequences to one another and to the S. cerevisiae genome. Finally, nine nif genes (HDKUSMBEN) were integrated into the yeast genome by homologous recombination when three subclusters were transformed into yeast (Buren et al., 2017). An [8Fe-9S-C] cluster, called NifB-co, which constitutes the core of the FeMo-co (Mo − 7Fe−9S − C − homocitrate), was formed in yeast by co-expressing Methanocaldococcus infernus nifB together with nifU, nifS, and fdxN from A. vinelandii (Burén et al., 2019). Our recent study also has shown that the active NifH and the NifDK tetramer were produced in S. cerevisiae by engineering 15 gene (nifB, nifH, nifD, nifK, nifE, nifN, nifX, hesA, nifV, groES and groEL from P. polymyxa WLY78 and 4 genes nifS, nifU, nifF, and nifJ from K. oxytoca (Liu et al., 2019). Each of the 15 genes was fused with a promoter and a terminator of S. cerevisiae to generate 15 transcription units by using overlap extension PCR. The 11 transcription units (nifB, nifH, nifD, nifK, nifE, nifN, nifX, hesA, nifV, groES, and groEL) containing homologous sequences to one another and to the S. cerevisiae genome were integrated into the δ site of S. cerevisiae chromosome in a one-step fashion and the 4 transcription units (nifS, nifU, nifF, and nifJ) were integrated onto the HO site of the S. cerevisiae chromosome (Liu et al., 2019).
Biological nitrogen fixation requires a large number of nif genes. How to engineer large numbers of nif genes into eukaryotic organism is still a challenge. Co-expression of multiple genes at a desired ratio is one strategy to solve the challenge. 2A peptides, the “self-cleaving” small (18–22 amino acids) peptides, are used to express multiple proteins from a single open reading frame (ORF) in eukaryotic cells (Ryan et al., 1999). When two genes are linked via a 2A peptide sequence, two separate proteins are generated from an ORF through a novel “cleavage” event within the 2A peptide sequence (Atkins et al., 2007; Doronina et al., 2008). Cleavage occurs at the end of the 2A peptide sequence through translation by 80S ribosomes (Szymczak-Workman et al., 2012). The cleavage of 2A peptides is not associated with the host cell proteases or viral proteinases, instead, associated with the highly conserved C-terminal of the 2A peptides (-DxExNPGP-) (Palmenberg et al., 1992; Donnelly et al., 2001; Sharma et al., 2012). 2A peptides were first discovered in the foot-and-mouth disease virus (FMDV) (Ryan and Drew, 1994). Since then, many 2A-like sequences have been identified in other viruses (Szymczak and Vignali, 2005; Wang and Marchisio, 2021). Four 2A peptides have been widely used in biomedical research: FMDV 2A (abbreviated herein as F2A); equine rhinitis A virus (ERAV) 2A (E2A); porcine teschovirus-1 2A (P2A) and thosea asigna virus 2A (T2A).
In this study, cleavage efficiency of NifB and NifH proteins linked via four different 2A peptides in S. cerevisiae are investigated by using Western blotting and yeast two-hybrid system analysis. Then, nine Nif proteins (NifB, NifH, NifD, NifK, NifE, NifN, NifX, HesA, and NifV) from P. polymyxa WLY78 are fused into two huge proteins and then they are co-expressed in S. cerevisiae. P. polymyxa NifH and K. oxytoca NifS and NifU are fused into a polyprotein and co-expressed in S. cerevisiae, and the expressed NifH from the huge protein (NifS-2A-NifU-2A-NifH) exhibits Fe activity. This study shows the potential of utilization of 2A peptides to express multicomponent nitrogenase system in S. cerevisiae.
Results and discussion
Cleavage efficiency of NifB and NifH proteins linked via 2A peptide in yeast
To test whether 2A peptide was able of separating bacterial nitrogenase proteins, two Nif proteins (NifB and NifH), which are the first and second proteins in Nif-cluster (NifBHDKENXHesANifV) of P. polymyxa WLY78, were chosen to be tested (Wang et al., 2013). The nifB and nifH genes, codon optimized for S. cerevisiae, were linked into a single open reading frame (ORF) encoding a polyprotein (designated NifB-2A-NifH) by four different 2A peptides (P2A, T2A, E2A and F2A), respectively (Figure 1A). Then, each of a single ORF was cloned into pRS423-GAL1p plasmid under the control of GAL1 promoter (Figure 1B), and each plasmid was transformed into S. cerevisiae YSG50. To determine the cleavage efficiency of the 2A peptide linked NifB and NifH, total protein extracts were prepared from aerobically grown yeast cultures and analyzed by Western blotting with the antibody against the NifH and NifB proteins of P. polymyxa WLY78 which was expressed and purified from E. coli BL21. The calculated molecular weights of NifH and NifB were 31.5 KDa and 55.0 KDa, respectively. The NifH protein was detectable in the recombinant S. cerevisiae strains but not in the wild-type S. cerevisiae YSG50 (Figure 1C). All the four 2A peptides evaluated showed cleavage with different efficiency, ranging from ~50% to ~90%. The best performance was achieved using P2A and T2A, in which the cleaved form represented more than 80% (Figure 1D). Our data are consistent with the reports that order of the work efficiency is P2A, T2A, F2A, and E2A (Szymczak-Workman et al., 2012). The results indicate that P2A and T2A should be preferred for expression of Nif proteins in yeast.
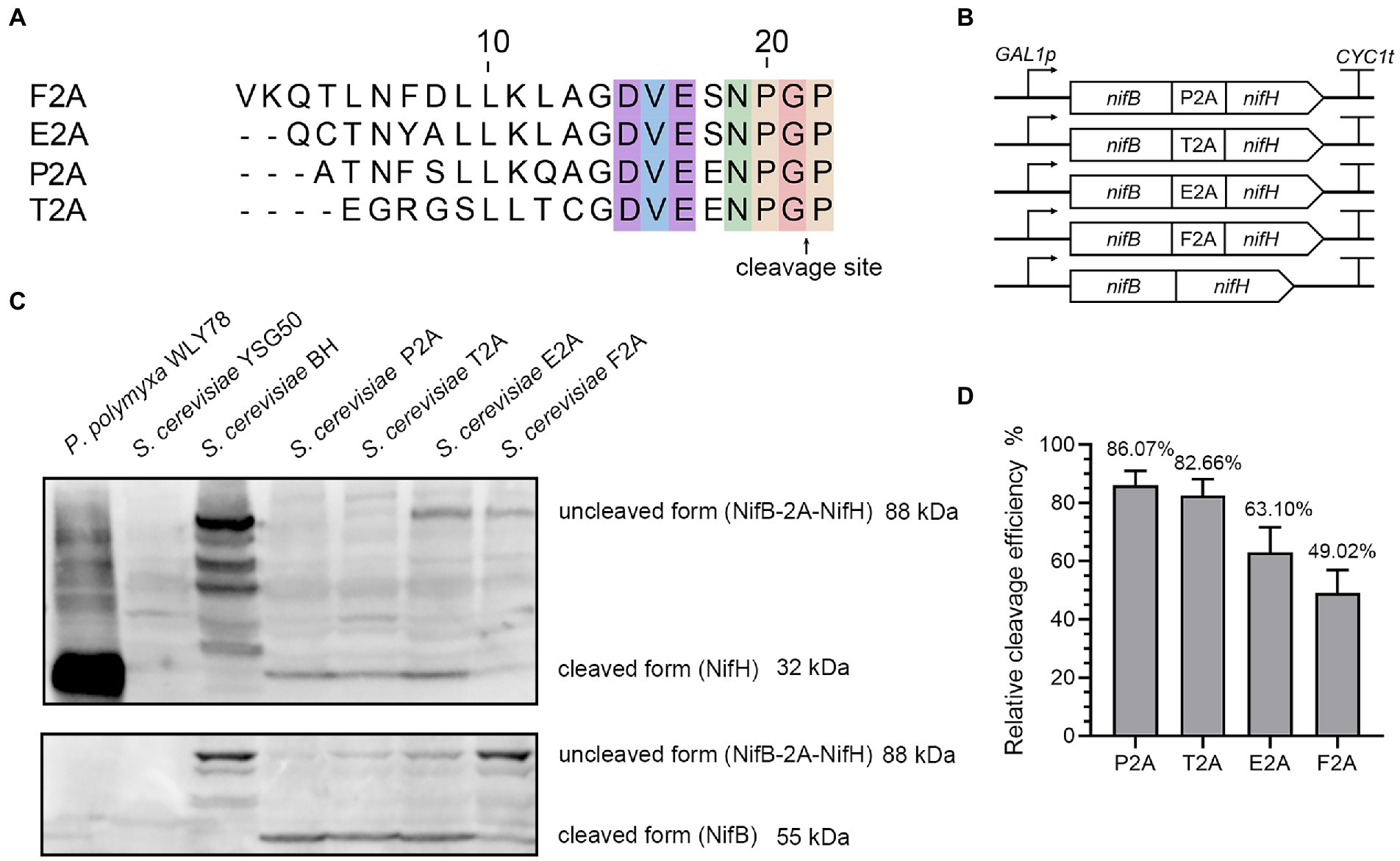
Figure 1. The cleavage efficiency of NifB and NifH linked via four different 2A sequences. (A) Amino acid sequences of the 2A peptides used in this study. (B) Schematic diagrams of the constructs of nifB and nifH linked via 4 different 2A peptides and without 2A peptide in the recombinant vectors. GAL1p indicates promoter and CYC1t indicates terminator. (C) Western blotting analysis of cell homogenates using antibody against NifH and NifB, respectively. P. polymyxa WLY78 was used as a positive control. The recipient S. cerevisiae YSG50 and S. cerevisiae BH carrying NifB-NifH fusion protein without 2A peptide linker are used as negative controls. P2A, T2A, E2A, and F2A indicate that S. cerevisiae strains carrying NifB-NifH fusion protein linked via P2A, T2A, E2A, and F2A, respectively. (D) Quantitation of the cleavage efficiency of NifB and NifH mediated by different 2A sequences. Cleavage efficiency = NifH/ (NifH+NifB-2A-NifH) *100%. The band intensity on the Western blot was measured by ImageJ software. Error bars indicated SEM, n = 3.
In addition, the different cleavage efficiency among the four 2A peptides was verified by yeast two hybrid assay in vivo. Two vectors pGBKT7 carrying GAL4 DNA-binding domain (BD) and pGADT7 carrying a GAL4 activation domain (AD) are usually used in yeast two hybrid (Supplementary Figure S1A). Here, a middle vector pGBKT7-AD that simultaneously carries AD and BD of GAL4 was constructed by cloning the GAL4 activation domain (AD) to vector pGBKT7 as described in Materials and Methods. Then, five nifB and nifH fusions linked with four different 2A peptides or without 2A peptide were individually in frame inserted between BD and AD domains of GAL4 in vector pGBKT7-AD, and then GAL4 BD, nifB, nifH and GLA4 AD were fused into a single ORF under control of ADH1 promoter (Supplementary Figure S1B). These plasmids were individually transformed into S. cerevisiae YSG50, yielding five recombinant strains: S. cerevisiae Y2H-BH carrying NifB-NifH, S. cerevisiae Y2H-BP2AH carrying NifB-P2A-NifH, S. cerevisiae Y2H-BT2AH carrying NifBT2ANifH, S. cerevisiae Y2H-BE2AH carrying NifH-E2A-NifH and S. cerevisiae Y2H-BF2AH carrying NifB-F2A-NifH (Supplementary Table S1). Yeast strain Y2H Gold carrying vector pGBKT7 was used as a negative control. All yeast strains grew well on the SC-Trp plates (Supplementary Figure S1C), indicating that TRP1 carried in vector pGBKT7 was expressed. When grown on SC-Trp-His-Ade plates, S. cerevisiae BH carrying NifB-NifH grew well, suggesting that GAL4 AD-NifB-NifH-GAL4 BD was a polyprotein. Whereas, S. cerevisiae BP2AH carrying NifB-P2A-NifH, S. cerevisiae BT2AH carrying NifBT2ANifH, S. cerevisiae BE2AH carrying NifH-E2A-NifH could not grow on SC-Trp-His-Ade plates, suggesting that NifB and NifH was effectively separated by P2A, T2A and E2A. However, S. cerevisiae BE2AH carrying NifH-F2A-NifH could grow well as S. cerevisiae BH carrying NifB-NifH did on SC-Trp-His-Ade plates, suggesting that E2A could not effectively separate NifB and NifH. The results are consistent with the western blotting data described above.
Tailing-tolerance assay
Since the 2A tail remains attached to the carboxyl terminus of the upstream protein after cleavage, it necessary to investigate influence of the presence of a 2A tail on Nif proteins. Here, the tolerance to the presence of a 2A tail is determined by using Nif protein carrying a 2A tail to complements nitrogenase activity of Δnif mutant of P. polymyxa. As described in Materials and Methods, nifH gene carrying four different 2A peptides (P2A, T2A, E2A and F2A) was used to complement ΔnifH mutant of P. polymyxa, generating four complementation strains P. polymyxa HPtail, P. polymyxa HTtail,P. polymyxa HEtail and P. polymyxa HFtail. P. polymyxa proH carrying nifH without 2A tail was constructed by using nifH gene without 2A tail to complement ΔnifH mutant of P. polymyxa was used as a positive control. Nitrogenase activity analyses showed that strains carrying NifH with different 2A tail exhibited the similar nitrogenase activities as P. polymyxa proH carrying P. polymyxa NifH without 2A tail did (Supplementary Figure S2). The data suggest that the 2A tails did not affect activity of NifH protein.
Further, we selected P2A, an efficient cutter, to verify its influence on the three major nitrogenase proteins NifB, NifD, and NifK. As described in Materials and Methods, complementation strains P. polymyxa Btail, P. polymyxa Dtail and P. polymyxa Ktail were constructed by using nifB, nifD and nifK carrying P2A tail to complement ΔnifB, ΔnifD, ΔnifK mutants of P. polymyxa, respectively. P. polymyxa proB, P. polymyxa proD and P. polymyxa proK that contained, respectively, nifB, nifD, and nifK without P2A were used as positive controls. Nitrogenase activity analyses showed that the residual P2A tail had no effect on the activity of NifB and NifD, while P2A tail made NifK to lose activity (Supplementary Figure S2B). The result is consistent with the reports that ENLYFQ tail on K. oxytoca NifK reduced nitrogenase activity in E. coli (Yang et al., 2018). The way to solve this problem is to put NifK on the C-terminal of fusion protein.
Co-expression of nine Nif proteins (NifH, NifB, NifD, NifK, NifE, NifN, NifX, HesA, and NifV) from Paenibacillus polymyxa WLY78 in yeast
Our previous studies have revealed that P. polymyxa WLY78 possesses a minimal nif gene cluster composed of nine genes (nifBHDKENXhesAnifV). The nine nif genes from P. polymyxa WLY78 enabled E. coli to synthesize active nitrogenase (Wang et al., 2013). Here, we try to investigate whether the nine nif genes from P. polymyxa WLY78 enabled S. cerevisiae to synthesize active nitrogenase. The nine genes (nifBHDKENXhesAnifV) from P. polymyxa WLY78, codon optimized for yeast, were synthesized and linked by 2A peptides (P2A, T2A, E2A, and F2A) into two ORFs: (nifB-P2A-nifH-T2A-nifD-E2A-nifK) and (nifE-F2A-nifN-T2A-nifX-F2A-hesA-P2A-nifV) that were under control of GAL1 promoter (Figure 2A). The recombinant yeast strain carried the nine Nif proteins linked via 2A peptide is called S. cerevisiae Nif.
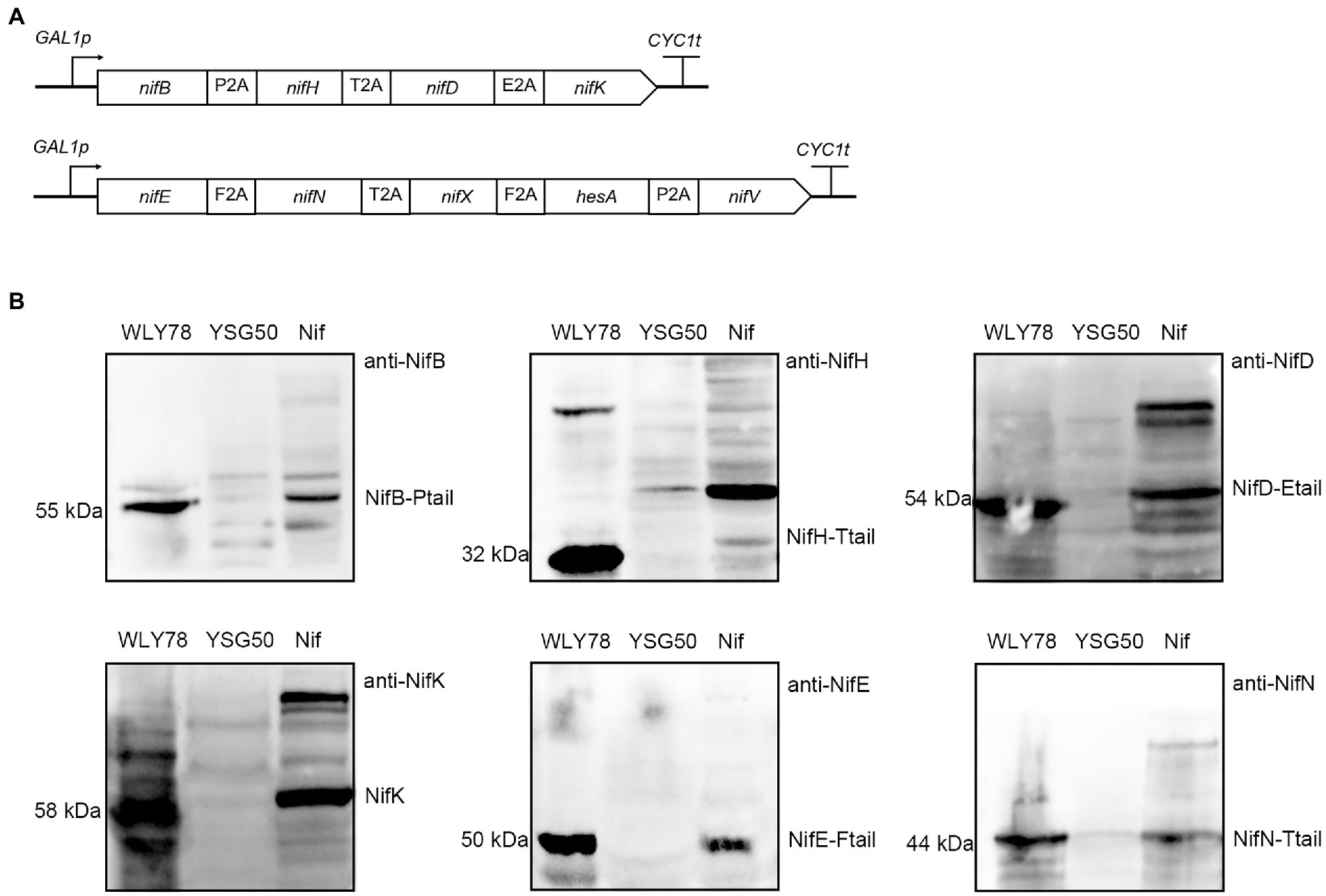
Figure 2. Co-expression of nine Nif proteins in yeast. (A) Schematic diagrams of the constructs of nine genes linked via different 2A peptides in the recombinant vectors. The four genes (nifB, nifH, nifD, and nifK) were linked by P2A, T2A, and E2A peptides, and cloned into pRS423-GAL1p. The five genes (nifE, nifN, nifX, hesA, and nifV) fused by F2A, T2A, F2A, and P2A peptides, and inserted into pRS424-GAL1p. All of the nif genes were under the control of GAL1 promoter. (B) Western blotting analysis of the recombinant S. cerevisiae Nif containing nine Nif proteins with anti-NifB, anti-NifH, anti-NifD, anti-NifK, anti-NifE and anti-NifN. GAL1p indicates promoter and CYC1t indicates terminator. WLY78, P. polymyxa WLY78 as a positive control; YSG50, S. cerevisiae YSG50 as a negative control; Nif, S. cerevisiae Nif.
Total protein extracts were prepared from the recombinant S. cerevisiae Nif to test expression of nine Nif proteins from two large ORFs. Western blotting with the six antibodies against the proteins NifH, NifD, NifK, NifB, NifE, and NifN of P. polymyxa WLY78 showed that all six Nif proteins were detectable in the protein extracts and had similar sizes with those of P. polymyxa WLY78 (Figure 2B). The data suggest that Nif proteins could be correctly co-expressed and cleaved in S. cerevisiae. The bands of the proteins (NifD, NifK, NifB, NifE and NifN) are stronger that of NifH. We deduce that the band strength of NifH appear weaker than other proteins (eg. NifB) may be result from its low antibody titer. Since protein numbers are too high, it is hard to estimate the cleavage efficiency mediated by the 2A peptides. Co-expression of four genes was applied to investigate reprogramming by using three 2A peptides ((P2A, T2A, and E2A) to allow expression of the four reprogramming factors in a single vector (Carey et al., 2009). The protein expression level from the first to fourth position in a quad-cistronic construct was characterized and the protein expression gradually decreased toward the 3′ end in quad-cistronic constructs (Liu et al., 2017). As we know, 5 proteins linked via 2A in this study are the highest number to be co-expressed in yeast. Finally, we measured the nitrogenase activity of the recombinant S. cerevisiae Nif by using acetylene reduction assays (Wang et al., 2013), but the strain did not show activity. As we know, nitrogenase requires both active NifH and NifDK. Since NifS and NifU for synthesis of Fe-S cluster are required for the active NifH. Thus, we will co-express NifU, NifS and NifH in yeast to check the function of NifH in the follow studies.
Co-expression of Paenibacillus polymyxa NifH and Klebsiella oxytoca NifU and NifS in yeast
Function of NifH (Fe protein) is conferred by Fe4-S4 cluster that bridges the NifH subunits in the Fe protein homodimer (Hoffman et al., 2014). NifU and NifS are responsible for synthesis of Fe-S cluster. Most of N2-fixing bacteria, such as Klebsiella oxytoca, have nifS and nifU genes encoding NifU and NifS, but P. polymyxa WLY78 lacks nifU and nifS genes. Also, our previous studies have shown that the K. oxytoca nifU and nifS could enhanced the nitrogenase activity of the recombinant E. coli strain that carried nine nif genes (nifBHDKENXhesAnifV) from P. polymyxa WLY78. Here, K. oxytoca nifU, nifS and P. polymyxa nifH whose 5′-coding region was tagged by 10 histidines, codon optimized for yeast, were fused into a single ORF (nifU-P2A-nifS-P2A-nifH-10 × His) via two P2A peptides or a single ORF (nifU-T2A-nifS-P2A-nifH-10 × His) via a T2A peptide and a P2A peptide. Each of the two ORFs was cloned into pRS423-GAL1p vector to be expressed under the control of GAL1 promoter and then these recombinant plasmids were transformed into S. cerevisiae YSG50, respectively. Thus, strains S. cerevisiae USHp (carrying nifU-P2A-nifS-P2A-nifH-10 × His) and S. cerevisiae USHt (carrying nifU-T2A-nifS-P2A-nifH-10 × His) were generated (Figure 3A).
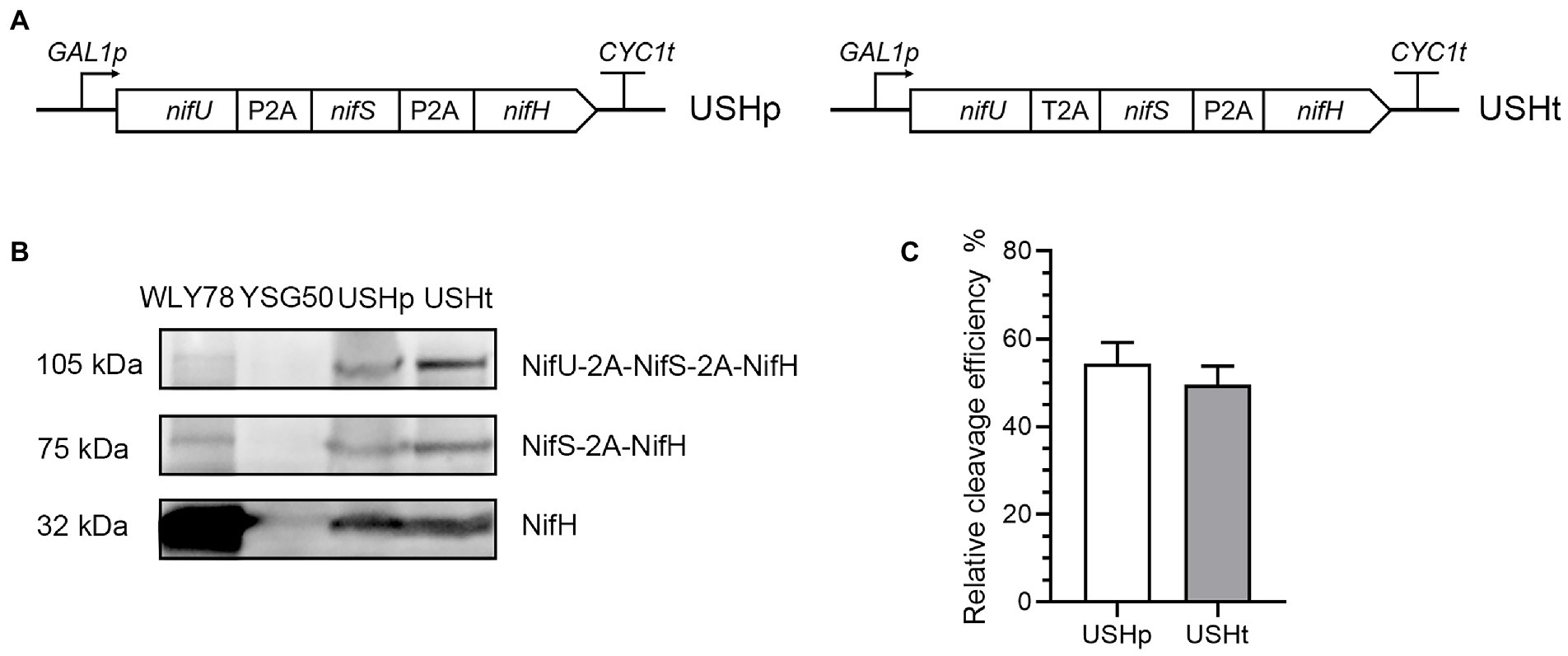
Figure 3. Co-expression of P. polymyxa NifH and K. oxytoca NifU and NifS linked via 2A peptides in yeast. (A) Scheme of nifU-2A-nifS-2A-nifH fusion under control of GAL1 promoter in the constructed recombinant vectors. (B) Western blotting analysis of NifH and its fusions in the recombinant strains S. cerevisiae USHp and USHtm. S. cerevisiae YSG50 was used as negative control and P. polymyxa WLY78 was used as positive control. (C) Quantitation of cleavage efficiency of the second 2A sequence. Cleavage efficiency = NifH/(NifH+NifS-2A-NifH+NifU-2A-NifS-2A-NifH) *100%. The band intensity on the Western blotting measured by ImageJ software. Error bars indicated SEM, n = 3. USHp: S. cerevisiae USHp, USHt: S. cerevisiae USHt.
To investigate whether NifH was expressed in yeast, total protein extracts were prepared from yeast and analyzed by Western blotting with anti-NifH. The cleaved form NifH (32 kDa) and the uncleaved forms NifS-2A-NifH (75 kDa) and NifU-2A-NifS-2A-NifH (105 kDa) were detectable in recombinant S. cerevisiae USHp and S. cerevisiae USHt, while they were not detected in the recipient S. cerevisiae YSG50 (Figure 3B). The cleaved NifH (32 kDa) was the major form in yeast and it had the similar molecular size with that of P. polymyxa NifH.
As shown in Figure 3C, the cleavage efficiency of the P2A in S. cerevisiae USHp and in S. cerevisiae USHt was similar at 49–52%, which is lower than the 80% cleavage efficiency in the NifB-P2A-NifH where NifB and NifH were linked by P2A. Our data are consistent with the reports that the highest protein expression was found at the first position in tri-cistronic constructs (Liu et al., 2017). It is also found in co-expression of cytochrome P450cc, adrenodoxin and adrenodoxin linked by two 2A peptides, the cleavage efficiency of the first 2A ranged 90–96%, while the cleavage efficiency of the second 2A peptides ranged from 11 to 68% (Efimova et al., 2021).
Yeast NifH expressed from the huge protein (NifS-2A-NifU-2A-NifH) exhibits activity of Fe protein
Saccharomyces cerevisiae USHp cells were initially grown in medium containing 0.6% glucose with strong aeration for about 15 h. After glucose was nearly consumed, 2% galactose was added to culture to induce expression of nif genes and also nitrogen gas was used to induce anerobic fermentation for more than 20 h (Figure 4A). Western blotting analysis showed that NifH was produced at 21, 25, 27, 29, and 40 h during the fermentation process (Figure 4B). The cleaved NifH was the major form, and the un-cleaved NifH forms also existed.
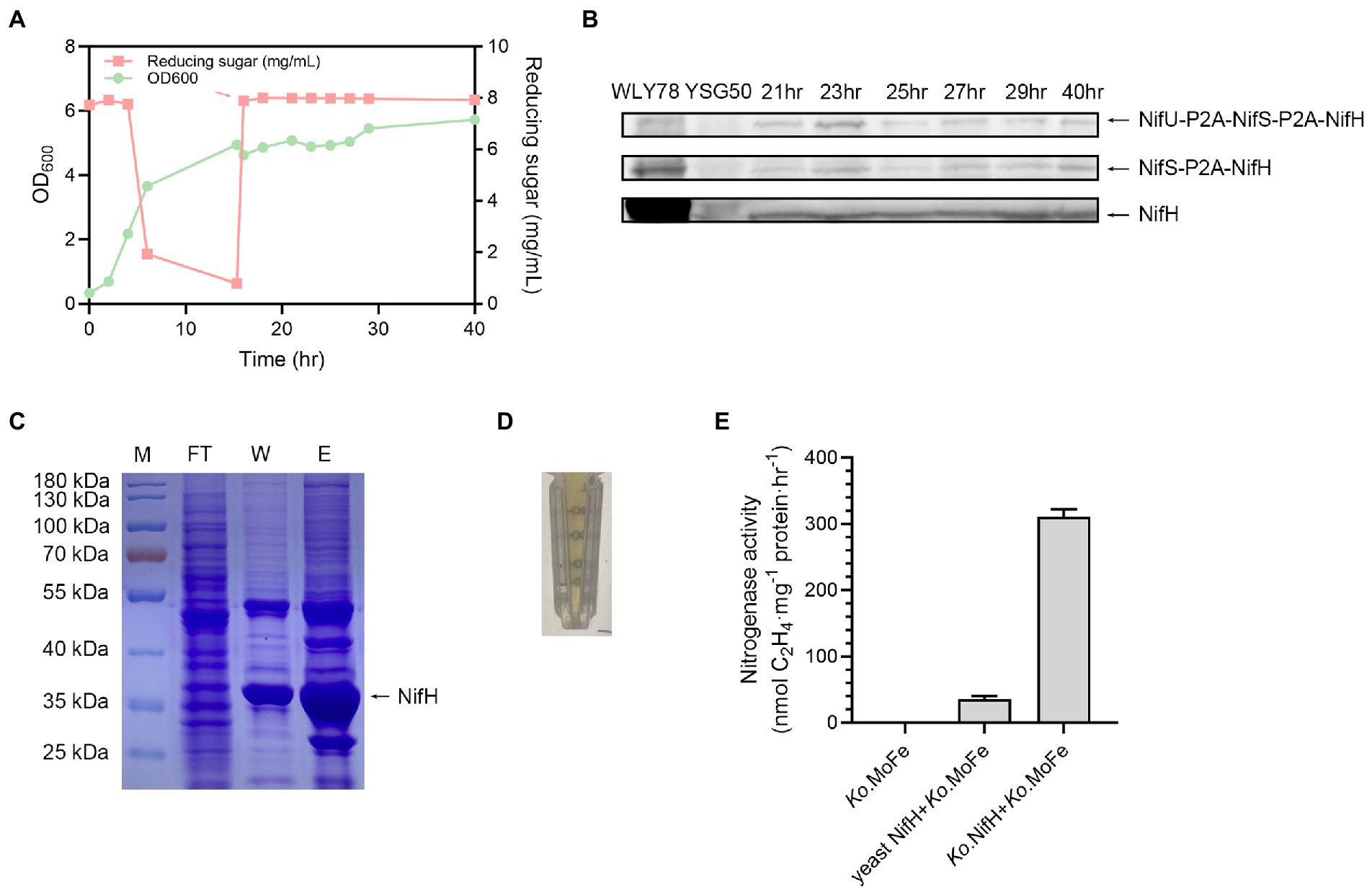
Figure 4. Growth of S. cerevisiae USHp and detection of expression and activity of NifH. (A) Batch fermentation process of S. cerevisiae USHp. OD600 value (green) and concentration of deoxidize sugar (red) were continuously monitored during the process. The red arrow indicates addition time of 2% galactose and trace-metal solution. During the fermentation process, pH was automatically controlled at 5.8 by 5 M KOH, and temperature was controlled at 30°C. (B) Western blotting analysis of NifH expression with anti-NifH during the fermentation process. NifH was cleaved form, while NifS-P2A-NifH and NifU-P2A-NifS-P2A-NifH were fusion protiens. WLY78, P. polymyxa WLY78 as a positive control; YSG50, S. cerevisiae YSG50 as a negative control. (C) Purification of NifH proteins. Ni2+ affinity column was used for the purification of the His-tagged NifH. M, molecular weight marker; FT, flow-through fractions; W, protein fractions eluted after washing with washing buffer; E, protein fractions eluted after washing with elution buffer. Arrow point to purified NifH protein. (D) The characteristic brown color of nitrogenase Fe protein. (E) Activity assays of yeast NifH protein. Fe protein activity was analyzed by assessing the nitrogenase activity (acetylene reduction activity) of the mixtures of NifH protein and the K. oxytoca MoFe protein (Ko. MoFe). Ko. MoFe without K. oxytoca Fe protein (Ko. Fe) as a negative control. Ko. Fe with addition of Ko. MoFe as a positive control. The error bars indicated SEM, n = 3.
His-tagged NifH was purified from S. cerevisiae USHp cells after 40 h of cultivation by using anaerobic Ni2+ affinity chromatography inside a glove box (Figure 4C). The purified NifH exhibited brown color which was the characteristic of nitrogenase Fe protein (Figure 4D). The Fe protein activity of the purified yeast NifH was analyzed by assaying nitrogenase activity in the mixture of yeast NifH and pure K. oxytoca MoFe protein. In absence of yeast NifH, pure K. oxytoca MoFe protein did not exhibit nitrogenase. As observed in positive control of mixtures of pure K. oxytoca Fe and FeMo proteins, yeast NifH showed nitrogenase activity in presence of K. oxytoca MoFe protein. The data indicate that the expressed P. polymyxa NifH in yeast was capable of donating electrons to MoFe protein from K. oxytoca (Figure 4E). The expressed P. polymyxa NifH in yeast did not require NifM to render active, consistent with the finding that there is no nifM gene in the genome of P. polymyxa. In contrast, A. vinelandii NifH requires NifM to render active Fe protein (López-Torrejón et al., 2016). However, the activity of the purified yeast NifH is 5–10% of that of K. oxytoca Fe protein. We deduce that the low activity of the purified yeast NifH results from two reasons. One reason is that K. oxytoca MoFe protein does not match P. polymyxa Fe protein (NifH) very well. The other reason is that the nitrogenase activity in the mixture of K. oxytoca Fe and MoFe protein is much higher than that in the mixture of P. polymyxa Fe and MoFe. Recently, we have revealed that the nitrogenase activity in the mixture of Fe and MoFe purified from Paenibacillus sabinae T27 is much higher than in the mixture of the purified P. sabinae MoFe and K. oxytoca Fe or A. vinelandii Fe. Also, the nitrogenase activity in the mixture of K. oxytoca Fe and MoFe protein is much higher than that in the mixture of P. sabinae Fe and MoFe (Li et al., 2021a). Recently, we have revealed that the sufCDSUB operon, nifS-like and yutI genes were involved in the Fe–S cluster biosynthesis of nitrogenase in P. polymyxa WLY78 (Li et al., 2021b). In the future, we can co-express nifH and these genes involved in Fe–S cluster biosynthesis of P. polymyxa WLY78 in yeast to investigate whether the Fe activity can be enhanced.
In summary, we use 2A peptides to co-express multiple proteins involved in synthesis of the complex nitrogenase system. Our results for the first time demonstrate that Nif proteins linked via 2A peptides can be successfully co-expressed in S. cerevisiae. All the four 2A peptides (P2A, T2A, E2A and F2A) evaluated show cleavage with different efficiency, ranging from ~50% to ~90%. The presence of a 2A tail in NifH, NifB and NifD dose not affect the activity of these Nif proteins, but 2A tail causes a complete loss of NifK activity. Nine Nif proteins (NifH, NifB, NifD, NifK, NifE, NifN, NifX, HesA and NifV) from P. polymyxa WLY78 are successfully co-expressed in yeast. Yeast NifH co-expressed from P. polymyxa NifH and K. oxytoca NifS and NifU exhibits activity of Fe protein.
Materials and methods
Strains and media
Bacterial strains and yeast strains used in this study are shown in Supplementary Table S1. S. cerevisiae YSG50 was used as host for nif gene expression. S. cerevisiae Y2H Gold was used as a host in Yeast two hybrid system for detecting the cleavage efficiency of 2A linkers in vivo. S. cerevisiae YSG50 and Y2H Gold strains were usually grown at 30°C in YPD medium (20 g/l tryptone, 10 g/l yeast extract, 20 g/l glucose). The yeast transformants were screened at 30°C in SC medium without tryptophan (SC-Trp), or without tryptophan, histidine and adenine (SC-Trp-His-Ade). E. coli DH5α was used to routine cloning and plasmid propagation. Luria-Bertani broth. P. polymyxa WLY78 was grown in nitrogen-limited medium (Wang et al., 2013). When appropriate, antibiotics were added at the following concentrations: 100 μg/mL Ampicillin and 5 μg/mL Erythromycin for maintenance of plasmids in bacterial strains. Primers and plasmids used in this study were listed in Supplementary Tables S3, S4, respectively.
The codon-optimized nif gene sequences
The nine genes (nifBHDKENXVhesA) from P. polymyxa WLY78, codon-optimized for yeast, were synthesized together with 2A peptides (P2A, T2A, E2A, and F2A) and they were separated to two large ORFs (GeneScript Biotech Co., China) (Supplementary Table S2). Namely, nifB-P2A-nifH-T2A-nifD-E2A-nifK was synthesized as an ORF that was cloned to vector pUC57, generating the recombinant plasmid pUCE-c1, and nifE-F2A-nifN-T2A-nifX-F2A-hesA-P2A-nifV was synthesized as an ORF that was cloned to vector pUC57, generating the recombinant plasmid pUCE-c2. The nifS and nifU from K. oxytoca were codon-optimized for yeast (Liu et al., 2019).
Construction of plasmids pRS423-c1 and pRS424-c2 for expressing nine nif genes from Paenibacillus polymyxa WLY78 in yeast
Plasmid pRS423-c1 containing nifB-P2A-nifH-T2A-nifD-E2A-nifK fusion was constructed by PCR amplifying a 5,550 bp fragment containing nifB-P2A-nifH-T2A-nifD-E2A-nifK fusion from pUCE-c1 with primers f34/r34 and by ligating the PCR fragment to EcoRI/SalI digested vector pRS423-GAL1p with T4 ligase, pRS424-c2 plasmid containing nifE-F2A-nifN-T2A-nifX-F2A-hesA-P2A-nifV fusion was constructed by PCR amplifying a 5,235 bp fragment containing nifE-F2A-nifN-T2A-nifX-F2A-hesA-P2A-nifV fusion from pUCE-c2 with primers f35/r35 and by ligating the PCR fragment to EcoRI/SalI digensted vector pRS423-GAL1p with T4 ligase. The two plasmids were co-transformed into S. cerevisiae YSG50, and the transformants were selected on SC-His and identified by PCR analysis. A positive yeast transformant carrying 9 nif genes (nifBHDKENX hesA nifV) was designed as S. cerevisiae Nif (Supplementary Table S1).
Construction of plasmids pRS423-USHp and pRS423-USHt for co-expression of Klebsiella oxytoca NifU and NifS and Paenibacillus polymyxa nifH in yeast
Plasmid pRS423-USHp carrying nifU-P2A-nifS-P2A-nifH-His tag was constructed as follows. An 837 bp fragment (nifU-P2A) was PCR amplified from pUCE-U with primers f36/r36, of which primer r36 contained the nifU’-end (without stop codon) and partial P2A sequences. A 1200 bp fragment (P2A-nifS-P2A) was PCR amplified was PCR amplified from pUCE-S with primers f37/r37, of which primer f37 contained partial P2A sequences at its 5′-end and primer r37 contained the nifS’-end (without stop codon) and partial P2A sequences. A 867 bp fragment (P2A-nifH) was PCR amplified from pUCE-c1 with primers f38/r38, of which primer f38 contained partial P2A sequences and primer r38 contained the 3′-end of nifH’ containing stop codon. A fragment (nifU-P2A-nifS-P2A) was obtained by fusing nifU-P2A and P2A-nifS-P2A using overlap extension PCR with primers f36/r37. The nifU-P2A-nifS-P2A fragment was fused to P2A-nifH by using overlap extension PCR with primers f36/r38, generating a 3,018 bp fragment (nifU-P2A-nifS-P2A-nifH). Finally, the fragment (nifU-P2A-nifS-P2A-nifH) was assembled to vector pRS423-GAL1p digested with EcoRI and SalI, generating plasmid pRS423-USH1. Finally, the whole sequences of plasmid pRS423-USH1 was PCR amplified with primers f39/r39 that contained 10xHis sequences (His tag), generating a 9,501 bp fragment (His tag-pRS423-nifU-P2A-nifS-P2A-nifH-His tag). The linear PCR fragment was circled by assembly enzyme, generating plasmid pRS423-USHp.
pRS423-USHt carrying nifU-T2A-nifS-P2A-nifH-His tag was constructed as follows. An 837 bp fragment (nifU-T2A) was PCR amplified from pUCE-U with primers f36/r40, of which primer r40 contained the 3′-end of nifU without stop codon and partial T2A. A 1200 bp fragment (T2A-nifS-P2A) was amplified from pUCE-S with primers f41/r37, of which f41 contained 5′-end of nifS and partial T2A sequences and primer r37 contained 3′-end of nifS without stop codon and partial T2A sequences. A 867 bp fragment (P2A-nifH) was PCR amplified with primers f38/r38, of which primer f38 contained partial P2A sequences and primer r38 contained 3′-end of nifH with a stop codon. The nifU-T2A-nifS-P2A was obtained by fusing nifU-T2A and T2A-nifS-P2A using overlap extension PCR with primers f36/r37. A 3015 bp nifU-T2A-nifS-P2A-nifH fragment was obtained by fusing nifU-T2A-nifS-P2A and P2A-nifH fragments with primers f36/r38. The fragment was assembled to vector pRS423-GAL1p digested by EcoRI and SalI, generating plasmid pRS423-USH2. The whole sequences of plasmid pRS423-USH2 was PCR amplified with primers f39/r39 that contained 10xHis sequences (HiS tag), generating a 9,489 bp fragment (His tag-pRS423-nifU-T2A-nifS-P2A-nifH-His tag). The fragment was circled by assembly enzyme via homologous recombination, yielding plasmid pRS423-USHt.
Plasmids pRS423-USHp and pRS423-USHt were individually transformed into S. cerevisiae YSG50 and transformants were selected on SC-His and identified by PCR analysis. Two positive transformants were S. cerevisiae USHp carrying plasmid pRS423-USHp and S. cerevisiae USHt carrying pRS423-USHt (Supplementary Table S1).
Construction of Paenibacillus polymyxa nif mutants and other recombinant plasmids
Plasmids for assaying cleavage efficiency of of NifB and NifH fusion protein linked via different 2A peptides in yeast and for yeast two hybrid (Y2H) assay were shown in Supplementary Material. Construction of ΔnifB, ΔnifH, ΔnifD, and ΔnifK mutants of P. polymyxa WLY78 and their complement strains were shown in Supplementary Material.
Yeast two-hybrid assay
Saccharomyces cerevisiae Y2H Gold was a host used in yeast two hybrid. The derivatives of S. cerevisiae Y2H Gold carrying the Nif polyprotein linked via 2A peptide were grown on SC medium lacking tryptophan (SC-Trp) and on SC-Trp-His-Ade agar plates.
Tailing-tolerance assay
To determine the tolerance of Nif protein to the C-terminal tail, nif gene carrying 2A peptide sequence (tail) was used to complement the nif deletion mutant of P. polymyxa WLY78, generating complement strains. Nitrogenase activities of wild-type, mutants and complement strains were comparatively determined.
Western blotting
For immunoblot detection of Nif protein expression and 2A self-cleavage efficiency, S. cerevisiae YSG50 (wild-type) and derivatives were grown in 50 ml flasks containing 20 ml of SD medium until glucose was consumed. Then, 2% galactose was added to induce nif genes separated by 2A sequences expression. Cultures were collected immediately after 9 h of incubation with shaking at 30°C and 200 rpm. Cells of P. polymyxa WLY78 cultivated anaerobically in nitrogen-limited medium were collected and then used as a positive control. The cells pellets were suspended in 200 μL 1 × SDS loading buffer and boiled for 10 min, and then, 20 μL of each sample were loaded onto 12% separating gels. Subsequently, proteins on the gels were transferred to NC membranes (Huaxingbio, China). The antibodies (anti-NifH, anti-NifD, anti-NifK, anti-NifB, anti-NifE, and anti-NifN) were used at a dilution of 1: 10000. The secondary antibody goat anti-rabbit IgG-HRP (Huaxingbio, China) was also used at 1: 10000. Immunochemiluminescene was done by using the NcmECL Western Blot Kit (NCM Biotech, China). The band intensity on the Western blots was analyzed using ImageJ. The cleavage efficiency of 2A linkers was calculated as (cleaved form) / (cleaved form + uncleaved form) × 100%.
Growth of yeast strains and purification of yeast NifH
Saccharomyces cerevisiae USHp was used for purification of NifH protein. It was cultivated in 500 ml of SD medium with shaking at 200 rpm at 30°C as described by Liu et al. (2019). The cells were lysed in a high pressure homogenizer (PhD Technology International LLC, United States) at 25,000 lb. per square inch. His-tagged NifH was purified by Ni2+ affinity chromatography (QIAGEN, Germany) under anaerobic conditions inside a glovebox (M. Braun Inertgas systems, Germany). Purified NifH protein was stored in liquid N2.
Determination of Fe protein activity
Fe protein activity of the yeast NifH was analyzed by assaying nitrogenase activity in vitro. Yeast NifH protein was mixed with pure K. oxytoca MoFe protein, and ATP-regeneration mixture 5 mM ATP, 40 mM creatine phosphate, 10 mM MgCl2, 20 mM sodium dithionite, 0.125 mg/mL creatine phosphokinase, 40 mM MOPS-KOH (pH 7.4) (Li and Burris, 1983). Positive control reactions were carried out with pure Fe protein and MoFe protein from K. oxytoca.
Nitrogenase activity assay
Nitrogenase activities of P. polymyxa WLY78 and its derivatives were determined by acetylene reduction assay according to method described by Wang et al. (2018).
Data availability statement
The raw data supporting the conclusions of this article will be made available by the authors, without undue reservation.
Author contributions
SC designed research. MW carried out gene clone and assembly, protein purification, and biochemical studies. XL contributed to gene clone. YS contributed to fermentations. MW and SC analyzed data and wrote the manuscript. All authors contributed to the article and approved the submitted version.
Funding
This work was supported by the National Key Research and Development Program of China Award (No. 2019YFA0904700).
Conflict of interest
The authors declare that the research was conducted in the absence of any commercial or financial relationships that could be construed as a potential conflict of interest.
Publisher’s note
All claims expressed in this article are solely those of the authors and do not necessarily represent those of their affiliated organizations, or those of the publisher, the editors and the reviewers. Any product that may be evaluated in this article, or claim that may be made by its manufacturer, is not guaranteed or endorsed by the publisher.
Supplementary material
The Supplementary material for this article can be found online at: https://www.frontiersin.org/articles/10.3389/fmicb.2023.1137355/full#supplementary-material
References
Arnold, W., Rump, A., Klipp, W., Priefer, U. B., and Pühler, A. (1988). Nucleotide sequence of a 24, 206-base-pair DNA fragment carrying the entire nitrogen fixation gene cluster of Klebsiella pneumoniae. J. Mol. Biol. 203, 715–738. doi: 10.1016/0022-2836(88)90205-7
Atkins, J. F., Wills, N. M., Loughran, G., Wu, C., Parsawar, K., Ryan, M. D., et al. (2007). A case for stop go: reprogramming translation to augment codon meaning of GGN by promoting unconventional termination (stop) after addition of glycine and then allowing continued translation (go). RNA 13, 803–810. doi: 10.1261/rna.487907
Beatty, P. H., and Good, A. G. (2011). Future prospects for cereals that fix nitrogen. Science 333, 416–417. doi: 10.1126/science.1209467
Burén, S., Jiménez-Vicente, E., Echavarri-Erasun, C., and Rubio, L. M. (2020). Biosynthesis of nitrogenase cofactors. Chem. Rev. 120, 4921–4968. doi: 10.1021/acs.chemrev.9b00489
Burén, S., Pratt, K., Jiang, X., Guo, Y., Jimenez-Vicente, E., Echavarri-Erasun, C., et al. (2019). Biosynthesis of the nitrogenase active-site cofactor precursor Nif B-co in Saccharomyces cerevisiae. Proc. Natl. Acad. Sci. U. S. A. 116, 25078–25086. doi: 10.1073/pnas.1904903116
Buren, S., Young, E. M., Sweeny, E. A., Lopez-Torrejon, G., Veldhuizen, M., Voigt, C. A., et al. (2017). Formation of nitrogenase Nif DK tetramers in the mitochondria of Saccharomyces cerevisiae. ACS Synth. Biol. 6, 1043–1055. doi: 10.1021/acssynbio.6b00371
Carey, B. W., Markoulaki, S., Hanna, J., Saha, K., Gao, Q., Mitalipova, M., et al. (2009). Reprogramming of murine and human somatic cells using a single polycistronic vector. Proc. Natl. Acad. Sci. U. S. A. 106, 157–162. doi: 10.1073/pnas.0811426106
Curatti, L., and Rubio, L. M. (2014). Challenges to develop nitrogen-fixing cereals by direct nif-gene transfer. Plant Sci. 225, 130–137. doi: 10.1016/j.plantsci.2014.06.003
Donnelly, M., Hughes, L. E., Luke, G., Mendoza, H., Ten, D. E., Gani, D., et al. (2001). The “cleavage” activities of foot-and-mouth disease virus 2A site-directed mutants and naturally occurring “2A-like” sequences. J. Gen. Virol. 82, 1027–1041. doi: 10.1099/0022-1317-82-5-1027
Doronina, V. A., de Felipe, P., Wu, C., Sharma, P., Sachs, M. S., Ryan, M. D., et al. (2008). Dissection of a co-translational nascent chain separation event. Biochem. Soc. Trans. 36, 712–716. doi: 10.1042/BST0360712
Dos, S. P., Fang, Z., Mason, S. W., Setubal, J. C., and Dixon, R. (2012). Distribution of nitrogen fixation and nitrogenase-like sequences amongst microbial genomes. BMC Genomics 13:162. doi: 10.1186/1471-2164-13-162
Dos Santos, P. C., Johnson, D. C., Ragle, B. E., Unciuleac, M., and Dean, D. R. (2007). Controlled expression of nif and isc iron-sulfur protein maturation components reveals target specificity and limited functional replacement between the two systems. J. Bacteriol. 189, 2854–2862. doi: 10.1128/JB.01734-06
Efimova, V. S., Isaeva, L. V., Orekhov, P. S., Bozdaganyan, M. E., Rubtsov, M. A., and Novikova, L. A. (2021). Using a viral 2A peptide-based strategy to reconstruct the bovine P450scc steroidogenic system in S. cerevisiae. J. Biotechnol. 325, 186–195. doi: 10.1016/j.jbiotec.2020.10.028
Gavini, N., Tungtur, S., and Pulakat, L. (2006). Peptidyl-prolyl cis/trans isomerase - independent functional nifH mutant of Azotobacter vinelandii. J. Bacteriol. 188, 6020–6025. doi: 10.1128/JB.00379-06
Georgiadis, M. M., Komiya, H., Chakrabarti, P., Woo, D., Kornuc, J. J., and Rees, D. C. (1992). Crystallographic structure of the nitrogenase iron protein from Azotobacter vinelandii. Science 257, 1653–1659. doi: 10.1126/science.1529353
He, W., Burén, S., Baysal, C., Jiang, X., Capell, T., Christou, P., et al. (2022). Nitrogenase cofactor maturase NifB isolated from transgenic rice is active in FeMo-co synthesis. ACS Synth. Biol. 11, 3028–3036. doi: 10.1021/acssynbio.2c00194
Hoffman, B. M., Lukoyanov, D., Yang, Z., Dean, D. R., and Seefeldt, L. C. (2014). Mechanism of nitrogen fixation by nitrogenase: the next stage. Chem. Rev. 114, 4041–4062. doi: 10.1021/cr400641x
Hu, Y., and Ribbe, M. W. (2011). Biosynthesis of nitrogenase FeMoco. Coord. Chem. Rev. 255, 1218–1224. doi: 10.1016/j.ccr.2010.11.018
Li, J. L., and Burris, R. H. (1983). Influence of pN2 and pD2 on HD formation by various nitrogenases. Biochemistry 22, 4472–4480. doi: 10.1021/bi00288a019
Li, Q., and Chen, S. (2020). Transfer of nitrogen fixation (nif) genes to non-diazotrophic hosts. Chembiochem 21, 287–288. doi: 10.1002/cbic.201900784
Li, Q., He, X., Liu, P., Zhang, H., Wang, M., and Chen, S. (2021a). Synthesis of nitrogenase by Paenibacillus sabinae T27 in presence of high levels of ammonia during anaerobic fermentation. Appl. Microbiol. Biotechnol. 105, 2889–2899. doi: 10.1007/s00253-021-11231-z
Li, Q., Li, Y., Li, X., and Chen, S. (2021b). Identification of genes involved in Fe-S cluster biosynthesis of nitrogenase in Paenibacillus polymyxa WLY78. Int. J. Mol. Sci. 22:3771. doi: 10.3390/ijms22073771
Li, X. X., Liu, Q., Liu, X. M., Shi, H. W., and Chen, S. F. (2016). Using synthetic biology to increase nitrogenase activity. Microb. Cell Factories 15:43. doi: 10.1186/s12934-016-0442-6
Liu, Z., Chen, O., Wall, J. B. J., Zheng, M., Zhou, Y., Wang, L., et al. (2017). Systematic comparison of 2A peptides for cloning multi-genes in a polycistronic vector. Sci. Rep. 7:2193. doi: 10.1038/s41598-017-02460-2
Liu, X., Wang, M., Song, Y., Li, Y., Liu, P., Shi, H., et al. (2019). Combined assembly and targeted integration of multigene for nitrogenase biosynthetic pathway in Saccharomyces cerevisiae. ACS Synth. Biol. 8, 1766–1775. doi: 10.1021/acssynbio.9b00060
López-Torrejón, G., Jiménez-Vicente, E., Buesa, J. M., Hernandez, J. A., Verma, H. K., and Rubio, L. M. (2016). Expression of a functional oxygen-labile nitrogenase component in the mitochondrial matrix of aerobically grown yeast. Nat. Commun. 7:11426. doi: 10.1038/ncomms11426
Oldroyd, G. E., and Dixon, R. (2014). Biotechnological solutions to the nitrogen problem. Curr. Opin. Biotechnol. 26, 19–24. doi: 10.1016/j.copbio.2013.08.006
Palmenberg, A. C., Parks, G. D. D. J. H., Ingraham, R. H., Seng, T. W., and Pallai, P. V. (1992). Proteolytic processing of the cardioviral P2 region: primary 2A/2B cleavage in clone-derived precursors. Virology 190, 754–762. doi: 10.1016/0042-6822(92)90913-A
Roberts, G. P., MacNeil, T., MacNeil, D., and Brill, W. J. (1978). Regulation and characterization of protein products coded by the nif (nitrogen fixation) genes of Klebsiella pneumoniae. J. Bacteriol. 136, 267–279. doi: 10.1128/jb.136.1.267-279.1978
Rubio, L. M., and Ludden, P. W. (2008). Biosynthesis of the iron-molybdenum cofactor of nitrogenase. Annu. Rev. Microbiol. 62, 93–111. doi: 10.1146/annurev.micro.62.081307.162737
Ryan, M. D., Donnelly, M., Lewis, A., Mehrotra, A. P., Wilkie, J., and Gani, D. (1999). A model for nonstoichiometric, cotranslational protein scission in eukaryotic ribosomes. Bioorg. Chem. 27, 55–79. doi: 10.1006/bioo.1998.1119
Ryan, M. D., and Drew, J. (1994). Foot-and-mouth disease virus 2A oligopeptide mediated cleavage of an artificial polyprotein. EMBO J. 13, 928–933. doi: 10.1002/j.1460-2075.1994.tb06337.x
Sharma, P., Yan, F., Doronina, V. A., Escuin-Ordinas, H., Ryan, M. D., and Brown, J. D. (2012). 2A peptides provide distinct solutions to driving stop-carry on translational recoding. Nucleic Acids Res. 40, 3143–3151. doi: 10.1093/nar/gkr1176
Szymczak, A. L., and Vignali, D. A. (2005). Development of 2A peptide-based strategies in the design of multicistronic vectors. Expert Opin Biol. Thr. 5, 627–638. doi: 10.1517/14712598.5.5.627
Szymczak-Workman, A. L., Vignali, K. M., and Vignali, D. A. A. (2012). Design and construction of 2A peptide-linked multicistronic vectors. Cold Spring Harbor Protoc. 2012, 199–204. doi: 10.1101/pdb.ip067876
Vicente, E. J., and Dean, D. R. (2017). Keeping the nitrogen-fixation dream alive. Proc. Natl. Acad. Sci. U. S. A. 114, 3009–3011. doi: 10.1073/pnas.1701560114
Wang, X., and Marchisio, M. A. (2021). Synthetic polycistronic sequences in eukaryotes. Synth. Syst. Biotechnol. 6, 254–261. doi: 10.1016/j.synbio.2021.09.003
Wang, L., Zhang, L., Liu, Z., Zhao, D., Liu, X., Zhang, B., et al. (2013). A minimal nitrogen fixation gene cluster from Paenibacillus sp. WLY78 enables expression of active nitrogenase in Escherichia coli. PLoS Genet. 9:e1003865. doi: 10.1371/journal.pgen.1003865
Wang, T., Zhao, X., Shi, H., Sun, L., Li, Y., Li, Q., et al. (2018). Positive and negative regulation of transferred nif genes mediated by indigenous GlnR in gram-positive Paenibacillus polymyxa. PLoS Genet. 14:e1007629. doi: 10.1371/journal.pgen.1007629
Weber, E., Engler, C., Gruetzner, R., Werner, S., and Marillonnet, S. (2011). A modular cloning system for standardized assembly of multigene constructs. PLoS One 6:e16765. doi: 10.1371/journal.pone.0016765
Yang, J., Xie, X., Xiang, N., Tian, Z., Dixon, R., and Wang, Y. (2018). Polyprotein strategy for stoichiometric assembly of nitrogen fixation components for synthetic biology. Proc. Natl. Acad. Sci. U. S. A. 115, E8509–E8517. doi: 10.1073/pnas.1804992115
Keywords: nitrogenase, 2A peptide, polyprotein, co-expression, Saccharomyces cerevisiae
Citation: Wang M, Shang Y, Liu X and Chen S (2023) Assembly of nitrogenase biosynthetic pathway in Saccharomyces cerevisiae by using polyprotein strategy. Front. Microbiol. 14:1137355. doi: 10.3389/fmicb.2023.1137355
Edited by:
Mingfeng Cao, Xiamen University, ChinaReviewed by:
Shekhar Mishra, University of Illinois at Urbana-Champaign, United StatesDavid Mulder, National Renewable Energy Laboratory (DOE), United States
Qiang Yan, DSM, United States
Copyright © 2023 Wang, Shang, Liu and Chen. This is an open-access article distributed under the terms of the Creative Commons Attribution License (CC BY). The use, distribution or reproduction in other forums is permitted, provided the original author(s) and the copyright owner(s) are credited and that the original publication in this journal is cited, in accordance with accepted academic practice. No use, distribution or reproduction is permitted which does not comply with these terms.
*Correspondence: Sanfeng Chen, Y2hlbnNmQGNhdS5lZHUuY24=