- 1Department of Parasitology, Faculty of Medicine, Universiti Malaya, Kuala Lumpur, Malaysia
- 2Department of Medical Microbiology, Faculty of Medicine, Universiti Malaya, Kuala Lumpur, Malaysia
- 3Department of Medical Microbiology, University Malaya Medical Centre (UMMC), Kuala Lumpur, Malaysia
- 4A*STAR Infectious Diseases Labs (A*STAR ID Labs), Agency for Science, Technology and Research (A*STAR), Singapore, Singapore
The complex transmission profiles of vector-borne zoonoses (VZB) and vector-borne infections with animal reservoirs (VBIAR) complicate efforts to break the transmission circuit of these infections. To control and eliminate VZB and VBIAR, insecticide application may not be conducted easily in all circumstances, particularly for infections with sylvatic transmission cycle. As a result, alternative approaches have been considered in the vector management against these infections. In this review, we highlighted differences among the environmental, chemical, and biological control approaches in vector management, from the perspectives of VZB and VBIAR. Concerns and knowledge gaps pertaining to the available control approaches were discussed to better understand the prospects of integrating these vector control approaches to synergistically break the transmission of VZB and VBIAR in humans, in line with the integrated vector management (IVM) developed by the World Health Organization (WHO) since 2004.
Introduction
Zoonoses are infections transmitted from animals to humans (Murphy, 1998). In fact, “zoonosis” is a relatively new word coined by German scientist Rudolf Virchow in the late 19th century, which combines Greek words “zoon” (animal) and “noson” (disease) (Chomel, 2009). Due to increased overlap of habitats by humans and wildlife, climate change, certain economic, cultural and dietary practices, as well as invasion of alien species and convenient international travels, the healthcare and economic burden exerted by zoonoses has increased significantly (Woolhouse and Gowtage-Sequeria, 2005; Jones et al., 2008; Grace et al., 2012; Karesh et al., 2012; Kulkarni et al., 2015). Zoonoses are caused by a variety of pathogens encompassing viruses, bacteria, parasites, fungi, and prions (Taylor et al., 2001; Woolhouse and Gowtage-Sequeria, 2005; Jones et al., 2008). Theoretically, the transmission of a zoonosis can be prevented by segregating humans and the animals that serve as natural hosts of the pathogen (Chomel, 2009; Karesh et al., 2012). However, control and prevention strategies may face additional challenges when the zoonosis is vector-borne, as reflected by knowlesi malaria, a potentially fatal zoonosis transmitted by simio-anthropophilic anopheline mosquitoes (Tan et al., 2008; Jiram et al., 2012; Vythilingam et al., 2014; Wong et al., 2015; Lau et al., 2016). Similar obstacles happen with vector-borne infections possessing animal reservoirs, such as the tsetse fly-transmitted Trypanosoma brucei, the sand fly-transmitted leishmaniasis, and the mosquito-borne Sindbis virus (SINV), Zika virus (ZIKV), and yellow fever virus (Balfour, 1914; Njiokou et al., 2006; Singh et al., 2013; Vorou, 2016; Steyn et al., 2020; Kushwaha et al., 2022). Since the involving animals cannot be culled just to break the transmission circuit to humans (Lee et al., 2022), vector control is a critical component of breaking the transmission of vector-borne zoonoses (VBZ) and vector-borne infections with animal reservoirs (VBIAR). Vector control programs aim at either reducing the population of the vectors, or avoiding, if not reducing the exposure of the targeted vectors to humans (Wilson et al., 2020). Notably, a wide variety of arthropods and arachnids with different biological behaviors have been verified as medically important disease vectors (Table 1). The diverse array of vectors, animal reservoirs, and activities engaged by humans in the vicinity contribute different challenges to the control and elimination of these diseases. Here, we discussed the main vector control strategies in the current scenario, highlighted the strengths, limitations, and concerns arising from these approaches, knowledge gaps that deserve to be filled, and possibility of integrating multiple approaches of vector management into the control and elimination of VBZ and VBIAR.
The trilogy of vector control strategies
In general, vector control strategies can be classified into chemical, biological and environmental management approaches (Bos, 1991). Each of these approaches gained research and public attention at different time points and comes with its own advantages and disadvantages. These approaches are inter-related, where simultaneous application of multiple approaches can produce either synergistic effect against the propagation of vectors, or antagonistic effect that disputes the vector control program. Therefore, a thorough understanding on each vector control approach is crucial for a successful vector control that can lead to the eradication of respective vector-borne diseases (WHO, 2012). This is particularly crucial for the management of VBZ and VBIAR, as the transmission profiles of these infections are usually more complex, involving more organisms. In fact, some of these diseases have multiple transmission cycles. For example, Trypanosoma cruzi has an urban transmission cycle involving humans, and sylvatic cycle involving wildlife (Orozco et al., 2013), whereas yellow fever virus has sylvatic, intermediate/savannah and urban transmission cycles (Valentine et al., 2019; Cunha et al., 2020; Gabiane et al., 2022). Of note, each transmission cycle may involve different vectors with distinct biological properties and behaviors that further complicate transmission blocking via vector control program. Worse still, many of these infections have incompletely deciphered transmission risk factors (Swei et al., 2020). Due to such complexity, a well-designed multi-pronged strategy that integrates multiple approaches may be more suitable to control the transmission of VBZ and VBIAR.
Environment management approach
The environment management approach was the predominant vector control method prior to World War II (WWII). During this period, comprehensive understanding on local vector behavior and ecology dynamics, along with specifically tailored environmental management plans were the prerequisites toward a successful vector control (Quiroz-Martinez and Rodriguez-Castro, 2007; Wilson et al., 2020). The environment approach revolves around behavioral manipulation and landscape modification (Figure 1). Behavioral manipulation can be directed at humans, animals or the vectors involved (Ault, 1994). For example, community members can be trained to practice good sanitary measures around their housing compound, set up barrier proofing against mosquitoes (such as usage of bed net and mosquito screens), and employs personal protection when exploring places with high vector density (Demers et al., 2018; Wilson et al., 2020). Zooprophylaxis can be employed to distract vectors from biting humans (or animals that serve as natural reservoirs of the targeted pathogen), by introducing another animal with similar or better feeding attractiveness to the targeted vectors (Charlwood et al., 1985; Sousa et al., 2001). In this context, the mosquito behavior is manipulated. On the other hand, landscape modification revolves around temporary and permanent strategies of water management, with the goal of removing suitable breeding grounds for the vectors (Watsons, 1921). Vector control via environment management has been employed against the transmission of malaria (Le Prince and Orenstein, 1916; Watsons, 1921; Utzinger et al., 2001; Lindsay et al., 2002; Ferroni et al., 2012), lymphatic filariasis (van den Berg et al., 2013; Davis et al., 2021), yellow fever (Le Prince and Orenstein, 1916; Soper and Wilson, 1943), African trypanosomiasis (Jackson, 1941; Jackson, 1943; Jackson, 1948; Scott, 1966; Hargrove, 2003; Headrick, 2014), and leishmaniasis (Busvine, 1993; Steverding, 2017) in different parts of the world. However, this approach does not work in a “one size fits all” manner. For example, the zooprophylaxis approach reported promising results in Papua New Guinea and São Tomé (Charlwood et al., 1985; Sousa et al., 2001). However, this approach experienced failure in places such as Ethiopia, the Gambia, and Pakistan (Bouma and Rowland, 1995; Ghebreyesus et al., 2000; Bøgh et al., 2001). Such contradicting outcomes were due to various factors, including the types of vectors targeted in these studies. Indeed, the success of zooprophylaxis relies on the prerequisites that the involving vectors must be zoophilic and exophilic (outdoor feeders), in addition to the adequate segregation between the human and animal living spaces (Asale et al., 2017).
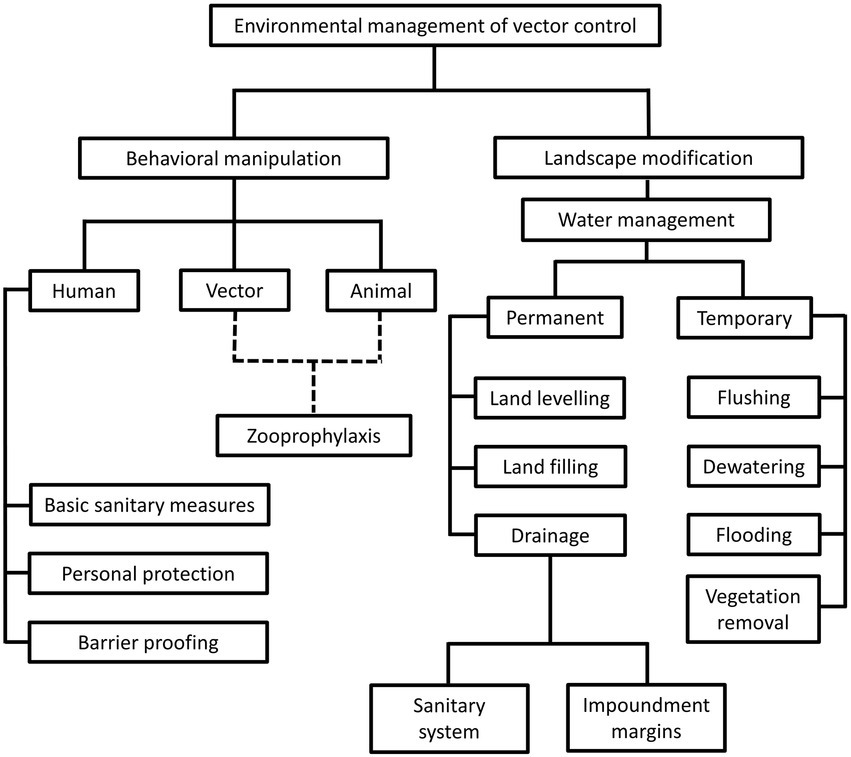
Figure 1. Different strategies under the environmental management of vector control. This approach revolves around behavioral alteration of humans, animals and vectors, as well as landscape modification, to create barriers between humans and vectors. Of note, the “zooprophylaxis” under “behavioral manipulation” involves the introduction of animals that are not pathogen reservoirs, to distract the blood-seeking vectors from humans and animals that serve as natural reservoirs of pathogens. This method involves behavioral alteration of animals and vectors, as indicated by the dotted lines in the diagram. On the other hand, landscape modification consists of permanent and temporary water management strategies to change the breeding environment of vectors.
A thorough evaluation and understanding on the stakeholders and targeted areas, along with long-term engagement (commitment) by the government and community members are needed to ensure a higher success rate of vector control via environment management. However, these can only be achieved with adequate time, financial support, and sustainable manpower. In addition, the benefits brought by this approach may be shadowed by unpredictable and potentially irreversible negative impact cast upon the environment, as exemplified by the bush clearing effort in parts of Africa during the 1950s and 1960s to control the population of tsetse flies (Scott, 1966; Hargrove, 2003; Pilossof, 2016). Hence, this vector control approach may not be an ideal solution for all diseases. Nevertheless, this approach is still a valuable tool for a sustained elimination of the targeted vector-borne diseases, provided that the approach is designed carefully by taking all biological, environmental, legal and socio-economic factors into consideration.
Chemical vector control
Chemical vector control strategies have gained popularity, especially after the WWII, due to the rapid and potent effect of these methods. The development, marketing and application of various insecticides has been the mainstream of chemical vector control strategy. Attempts to employ chemicals for pest control were recorded as early as the 1840s (Table 2). However, the discovery of dichloro-diphenyl-trichloroethane (DDT) revolutionized the approach to control vector population. The insecticidal properties of DDT were discovered in 1939 (Mellanby, 1992; Davies et al., 2007). Following the halted supply of chrysanthemum-derived pyrethrum from Japan due to WWII, DDT became the mainstream chemical player in vector control (Wilson et al., 2020), especially after its involvement in the successful control of typhus outbreak in Europe (Wheeler, 1946). Following this much publicized success against lice, DDT was proven to be potent against many other vectors such as the mosquito, tsetse fly, sandfly and blackfly (Ismail et al., 1975; Loyola et al., 1990, 1991; Roberts and Alecrim, 1991; Casas et al., 1998; Hargrove, 2003; Dias, 2007; Rijal et al., 2019). Nevertheless, the negative impacts brought by DDT to non-targeted organisms and environment were discovered after years of mass application. As a result, the application of this powerful chemical was discontinued abruptly in the 1970s (Davies et al., 2007). Subsequently, other insecticide groups such as organophosphates, carbamates and synthetic pyrethroid gained popularity in many vector control programs. This has stimulated various chemical-oriented vector combating strategies, such as the long-lasting insecticidal net (LLIN), indoor residual spraying (IRS), as well as outdoor residual spraying (ORS; Bonsall and Goose, 1986; Bhatt et al., 2015; Rohani et al., 2020; Tangena et al., 2020; Chaumeau et al., 2022).
Various chemicals have been developed and marketed as readily available larvicides and adulticides. The high availability and instantaneous killing effect of these products have created a dogma that the chemicals are the best way forward in vector management (Casida and Quistad, 1998; Thomas, 2018). Nevertheless, the biology of arthropods plays a critical role in determining the success rate of insecticide-mediated vector control programs. For instance, IRS and LLIN are not suitable for exophagic and exophilic mosquitoes with peak biting time in the early evening (Dolan et al., 1993; Rohani et al., 1999; Smithuis et al., 2013; Wong et al., 2015). Besides, behavioral adaptation of endophilic mosquitoes toward avoidance of insecticide-treated houses or rapid exit from the insecticide-treated buildings will minimize the exposure of these vectors to the insecticides, compromising the efficacy of the applied insecticide (Killeen, 2014). Importantly, the rampant usage of these chemicals has fuelled insecticide resistance in arthropods (Kleinschmidt et al., 2018; Tangena et al., 2020). Moreover, these chemicals may cast negative impacts to the ecosystem, although of lower toxicity than DDT. For example, synthetic pyrethroids are harmful to aquatic environment (Thatheyus and Selvam, 2013; Prusty et al., 2015), whereas organophosphates poisoning remains prevalent among communities involved in agricultural industry, despite being classified as non-persistent pesticides (Jaipieam et al., 2009; Kaushal et al., 2021). Due to these disadvantages, the chemical approach must be considered carefully in vector control programs against VBZ and VBIAR, particularly those with sylvatic transmission cycle.
Despite the non-specific harm to the environment due to their toxicity, the rapid and potent effect of insecticides against different vectors grants them the high popularity in pest and vector control. Many researchers have investigated ways of accelerating the degradation of these chemicals to minimize their adverse effects to the environment, while retaining their potency against the pests (Zhang and Qiao, 2002; Kaushal et al., 2021; Zhao et al., 2022). Meanwhile, the discovery of pyrethrum from chrysanthemum plant continues to inspire scientists to find novel compounds that can serve as bio-insecticides. For example, bioactive metabolites of Streptomyces have been reported to demonstrate good potential of becoming bio-insecticide candidates (Amelia-Yap et al., 2022). Such discovery has been driven by the need of novel, environment-friendly insecticide compounds, following rapid development of insecticide resistance and concerns over environment harm cast by chemical-based insecticides.
Vector biocontrol approach
Among the vector control strategies, biocontrol approaches have received increasing attention and popularity over the past two decades. Therefore, various organisms and strategies have been put forward as potential vector biocontrol candidates. In general, biocontrol approach explores the potential of using organisms and microorganisms to control the vector population (van den Bosch et al., 1982; Kamareddine, 2012; Okamoto and Amarasekare, 2012; Benelli et al., 2016; Huang et al., 2017; Kwenti, 2017; Thomas, 2018), based on the natural predation, pathobiological or parasitism relationship between the candidates and the targeted vectors (Table 3). Biological manipulation targeting certain vital functions of the vectors have been explored as a new approach in vector biocontrol (Gillette, 1988; Iturbe-Ormaetxe et al., 2011; Benelli et al., 2016). Theoretically, the biocontrol approach is more target-specific, thus of lower risk of imparting off-target effects to the environment. Prior to the new millennium, biocontrol approach was not as widely applied as its chemical and environmental counterparts, due to the relative ease of implementing the other two approaches (Quiroz-Martinez and Rodriguez-Castro, 2007; Shaalan and Canyon, 2009; Vershini and Kanagappan, 2014; Vinogradov et al., 2022). Nevertheless, biocontrol approach has received increasing attention following encouraging results obtained from the mass-application of Wolbachia-infected Aedes aegypti, genetically modified mosquitoes, sterile male triatomine bugs and tsetse flies. In fact, with the increased prevalence of VBZ and VBIAR, vector biocontrol approach may offer novel and sustainable strategies to control the transmission of these infections. Biological control approach can be categorized based on the natural relationship between the biocontrol agents and the respective vectors (Figure 2), as elaborated in the next few paragraphs of this review.
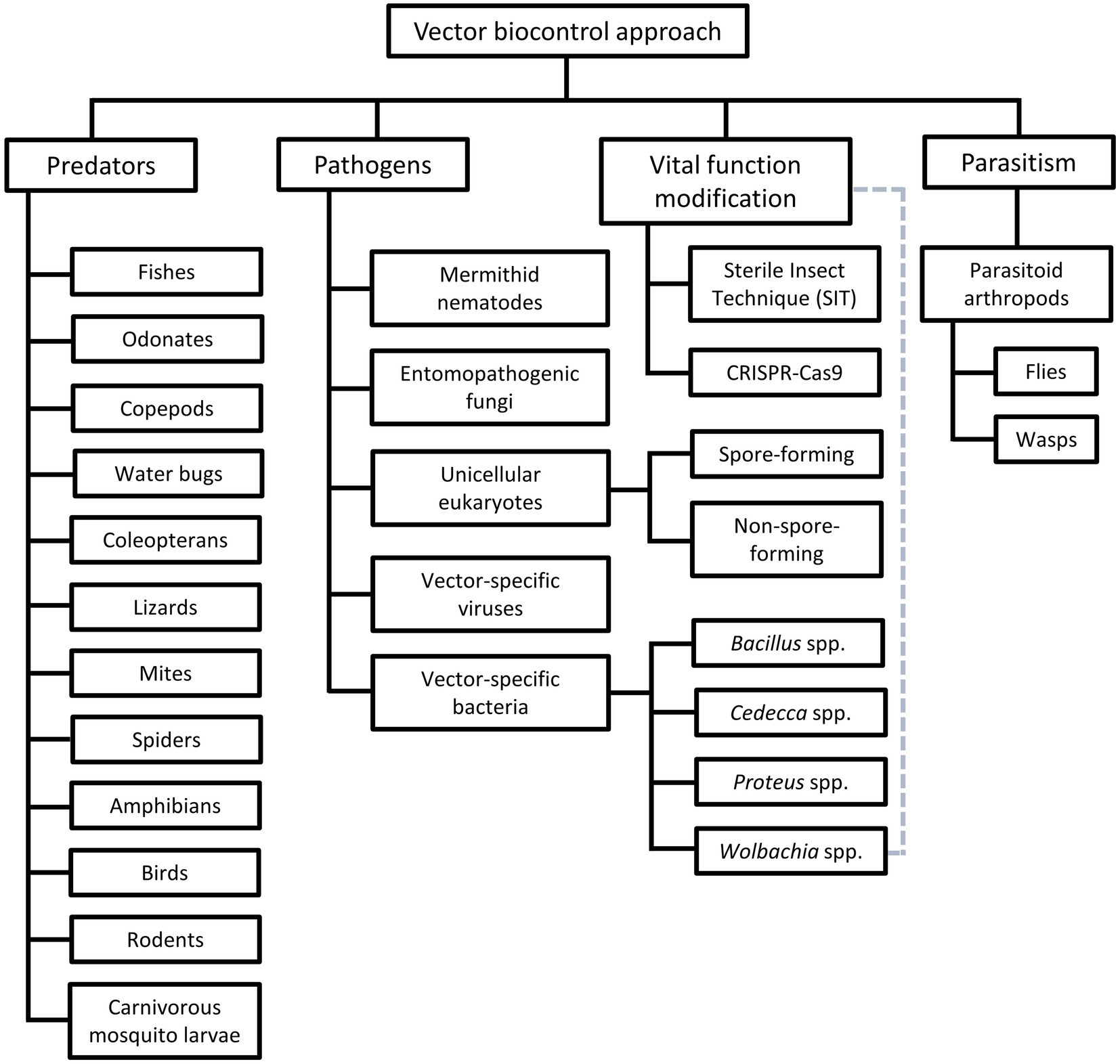
Figure 2. Different groups of vector biocontrol approach. Gray dotted lines reflect the characteristics of the Wolbachia method that combines the features of biocontrol approaches mediated by pathogens and vital function modification.
Biocontrol via predators
The potential of prey–predator relationship in vector control was explored before the era of mass insecticide application. For example, attempts to reduce the larval population of Stegomyia calopus (vector of yellow fever) in Ecuador with freshwater fish were initiated as early as the 1910s (Connor, 1922). Various aquatic and amphibian animals were put forward as potential candidates to control mosquito population, based on their predatory nature to the targeted pests. In this review, emphasis is given to medically relevant examples. Of note, most of these predator-driven strategies target the aquatic stages of mosquitoes because the mosquito larvae share a relatively confined living space with the predators. Thus, the aquatic prey–predator encounter does not rely as much on the overlapping active hours of the prey and predator, as compared to the flying adults. In addition, efficient and persistent predation on the vector offspring will inevitably control the vector population, and hence disease transmission (Kumar and Hwang, 2006; Walker and Lynch, 2007; Louca et al., 2009; Griffin and Knight, 2012).
Among the predators, larvivorous fishes have a prolific history as a biocontrol agent against pests, particularly mosquitoes. Larvivorous fishes were introduced into over 60 countries in 20th century to control vector populations (Gerberich and Laird, 1985). Their popularity was attributed mainly to their adaptability to a wide variety of natural and man-made water bodies that serve as mosquito breeding grounds, as well as their rapid reproduction rates (Hadjinicolaou and Betzios, 1973; Motabar, 1978; Chandra et al., 2008a). Numerous field trials with these predators demonstrated between 70 and 97% reduction of mosquito larvae (Connor, 1922; Menon and Rajagopalan, 1978; Fletcher et al., 1992; Kumar et al., 1998; Chandra et al., 2008a; Louca et al., 2009; Griffin and Knight, 2012). For instance, Aphanius dispar (Arabian toothcarp) managed to suppress the population of Anopheles arabiensis and Anopheles gambiae in wells, cisterns and barrels in Djibouti (Louis and Albert, 1988). However, the application of larvivorous fish has raised several concerns. The effect of an alien species to the native fauna and flora needs to be considered and monitored carefully. For example, the continuous introduction of Gambusia affinis (Western mosquitofish) into Greece from 1927 to 1937 resulted in the decline of an endemic species Valencia letourneuxi (Corfu toothcarp), due to living resource competition between the two species (Economidis, 1995; Economidis et al., 2000). Similar adverse effects associated with G. affinis have been reported from Australia and United States (Motabar, 1978; Arthington, 1991; Walton, 2007).
Similarly, odonates (particularly the larvae) are ferocious and imperative predators of many insects. Members of the order Odonata include various dragonflies and damselflies (Shaalan and Canyon, 2009; Vatandoost, 2021). Given their high predation capacity, relatively long aquatic life cycle (usually 1–2 years), and shared aquatic larval habitat with mosquito juveniles, odonates are potential vector biocontrol candidates. Indeed, field trials demonstrated significant reduction of mosquito larvae in water reservoirs by dragonfly nymphs (Sebastian et al., 1980, 1990; Chatterjee et al., 2007; Mandal et al., 2008). For example, a trial release of dragonfly nymphs in Myanmar reported a significant decrease of Ae. aegypti population in 2–3 weeks, and the effect persisted till the end of the 4-month-long trial (Sebastian et al., 1990). Similar findings were reported from India (Mandal et al., 2008). The odonate adults are agile aerial predators that prey on many insects (Vatandoost, 2021). Nevertheless, diet analyses of wild-caught dragonfly adults inferred that mosquitoes are rarely taken in large numbers by odonate adults (Pritchard, 1964; Sukhacheva, 1996; Pfitzner et al., 2015). In addition, the active hours (feeding time) of odonate adults (most species are diurnal) do not overlap with the active hours of many medically important vectors (Pfitzner et al., 2015; Vatandoost, 2021). Furthermore, the lifespan of odonate adults is relatively short (1–8 weeks). Hence, the potential of odonate adults as vector biocontrol agents is not as attractive as their juveniles.
The population of many mosquito vectors can be controlled by another mosquito via predation. Larvae of mosquitoes from 13 genera prey upon larvae of other arthropods (Harbach, 2007). All members of genera Toxorhynchites, Lutzia and Psorophora (subgenus Psorophora) are obligate predators of other arthropod larvae (Steffan and Evenhuis, 1981; Annis et al., 1990; Rawlins et al., 1991; Collins and Blackwell, 2000; Aditya et al., 2006; Wilkerson et al., 2021; Hancock et al., 2022), whereas larvae of Sabethes, several species of Culex and Anopheles are facultative predators (Lounibos, 1980; Mogi and Chan, 1996; Shaalan and Canyon, 2009; Hancock et al., 2022). Of these, Toxorhynchites has received relatively high research attention, mainly because the adult is non-hematophagous (blood feeding), hence not imposing risk as pest or disease vector (Shaalan and Canyon, 2009). Previously, the release of Toxorhynchites amboinensis larvae led to a 45% reduction of Ae. aegypti population in urban areas of New Orleans (Focks et al., 1985). Similar success was reported with T. splendens (Annis et al., 1989; Aditya et al., 2006) and T. moctezuma (Rawlins et al., 1991). Apart from their direct effect via ferocious predation, the presence of Toxorhynchites larvae can delay the prey’s developmental time and increase the prey’s mortality. This is probably due to the stress experienced by the prey in the presence of the predator, or the predator-derived kairomones (Andrade, 2015; Zuharah et al., 2015). Nevertheless, the mechanism behind this effect has yet to be completely deciphered. Despite the earlier reported success, the application of Toxorhynchites as biocontrol agent has been hindered by several factors. Firstly, sylvatic species such as T. rutilus are not well adapted to urban environment, which restricts its application despite the good predation capacity (Focks et al., 1983). Nevertheless, a more recent surveillance demonstrated the presence of T. rutilus in urban areas, albeit of low numbers (Wilke et al., 2019). Indeed, this discovery has reignited the hope of applying Toxorhynchites as a vector biocontrol agent in urban areas (Schiller et al., 2019). Besides, the slow population expansion of Toxorhynchites is another challenge that needs to be overcome. Under the natural settings, Toxorhynchites produces few offspring, which limits their efficacy and capacity in vector biocontrol. This is further aggravated by the cannibalistic nature of Toxorhynchites immatures, especially under food-restricted conditions (Donald et al., 2020).
Copepods of genera Megacyclops, Mesocyclops, and Macrocyclops are crustaceans that feed primarily on the first instar of mosquito larvae. Copepods can adapt to a large variety of water bodies and micro aquatic habitats such as phytotelmata (structures of terrestrial plants that allow formation of water pockets). Such high adaptability allows copepods to be explored as vector biocontrol agents in different settings (Vinogradov et al., 2022). In fact, the discovery of copepod’s potential in vector biocontrol was rather accidental, following an observed reduction of Ae. aegypti and Ae. polynesiensis larvae from ovitraps set in a study site at Tahiti, after unintentional introduction of copepods to the ovitraps (Riviere and Thirel, 1981). Subsequently, field trials from different regions confirmed the effectiveness of copepods in various water bodies (including drains and land crab burrows) against larvae of medically important mosquitoes, particularly of genera Aedes and Ochlerotatus (Lardeux et al., 1992; Kay et al., 2002). Importantly, the introduced copepods can adapt and colonize nearby water bodies, allowing sustained effort of mosquito larval control (Kay et al., 2002). Although being used mainly against Aedes spp., copepods have been used against other vectors such as Anopheles spp. and Culex spp. (Riviere and Thirel, 1981; Marten et al., 1989; Lardeux, 1992; Lardeux et al., 1992; Vu et al., 1998; Schaper, 1999; Marten et al., 2000; Kay et al., 2002; Zoppi de Roa et al., 2002; Soumare and Cilek, 2011). Despite their ability to adapt to different sizes of water bodies, copepods are particularly sensitive to temperature changes, chlorine content, low oxygen levels, and presence of toxin within the water (Brown, 1996; Vinogradov et al., 2022). Moreover, it is important to highlight that several species of copepods serve as the intermediate hosts of medically important parasites such as Drancunculus medinensis (guinea-worm) and Dibothriocephalus latus/ Diphyllobothrium latum (fish tape worm; Marten and Reid, 2007; Vinogradov et al., 2022). Therefore, careful consideration and planning must be done prior to application of this method. For example, non-vector copepod species can still be considered as biocontrol agents in certain parts of Africa that are endemic for dracunculiasis (Marten and Reid, 2007).
Water bugs, such as the backswimmers (family: Notonectidae), giant water bugs (family: Belostomatidae) and waterboatmen (family: Corixidae) are important predaceous insects under the order Hemiptera (Shaalan and Canyon, 2009). The potential of Anisops assimilis (common backswimmer) to control mosquito population was reported officially for the first time in 1939, following the observation that the backswimmer-harboring water containers were void of mosquito larvae, in contrast to the surrounding backswimmer-free water bodies that were infested with active mosquito larvae (Graham, 1939). Although field and laboratory trials using water bugs to control mosquito larvae exhibited promising results, they are hardly utilized as biocontrol agents due to the high cost and difficulty of mass rearing, as well as logistical challenges (Bay, 1974; Murdock et al., 1984; Venkatesan and Jeyachandra, 1985; Sankaralingam and Venkatesan, 1989; Aditya et al., 2004, 2005, 2006; Selvarajan and Kakkassery, 2019).
Predatory coleopterans from the families Dytiscidae (diving beetle) and Hydrophilidae (water scavenger beetle) are commonly found in ground pools, permanent and temporary ponds (Shaalan and Canyon, 2009). Despite the lower research interest, several studies on the predatory effect of beetles on mosquito reported promising results (Nilsson and Soderstrom, 1988; Juliano and Lawton, 1990; Nilsson and Savensson, 1994; Aditya et al., 2006; Chandra et al., 2008b). However, the efficacy of coleopterans as vector biocontrol agents may be compromised by their diet preference (when mosquitoes are not the only insects presented), species emigration and cannibalism (Juliano and Lawton, 1990; Lundkvist et al., 2003).
Currently, the potential of predators discussed above has not been thoroughly explored, and most of the reported studies focused on mosquitoes (Kim and Merritt, 1987; Werner and Pont, 2003). Notably, several natural predator-based biocontrol strategies have been attempted against non-mosquito vectors, notably the parasitic VBIAR. For example, Tarentola mautitanica, an insectivorous lizard, has been proposed as a candidate to control the population of Triatoma infestans (kissing bug) that spreads Chagas disease (Castello and Gil Rivas, 1980). Mites and spiders have been suggested as biocontrol agents of Phlebotomus spp. (sand fly) that transmits leishmaniasis (Dinesh et al., 2014).
Pathogenesis-mediated vector biocontrol
Besides predatory animals, pathogens have been proposed as biocontrol agents against vectors. In fact, a number of these pathogens have been applied in the field. These candidates vary in sizes and behavior, encompassing both prokaryotic and eukaryotic organisms. The nematodes are probably the largest candidates on the list. The mermithids are members of an endoparasitic nematode family. These nematodes are highlighted as potential vector biocontrol candidates, due to their parasitic relationship with various arthropods and several arachnids (Stabler, 1952; Chapman, 1974). The hatched pre-parasitic juveniles of mermithid nematodes aggressively infect mosquito larvae (usually the early instars) by paralyzing the targeted hosts, followed by penetration of cuticular wound to establish the infection (Sanad et al., 2017). Once infected, the mermithid parasites take over the cellular function regulatory authority of their hosts. If infection occurs during the early larvae instar, the parasitized mosquito larvae are halted from pupating as the infecting parasites develop within (Stabler, 1952; Allahverdipour et al., 2019). When the nutrient resource supplied by the infected host is exhausted, the nematode, now at its third-stage juvenile post-parasite stage, emerges out of the host, which results in the death of the host (Stabler, 1952). The emerged post-parasite stage then molts into the free-living adult to reproduce and lay eggs. Multiple mermithids may repeatedly infect an already infected larva, giving rise to a phenomenon called superparasitism (Sanad et al., 2017). Different research groups have demonstrated the mosquito larvicidal effect of several mermithids such as Romanonermis iyengari (against Ae. aegypti, Ae. albopictus, An. gambiae, Anopheles culicifacies, Anopheles stephensi, Anopheles subpictus, Armigeres subalbatus, Culex pipiens, Culex quinquefasciatus, Culex sitiens, Culex tritaeniorhynchus, and Mansonia annulifera), Diximermis peterseni (against Anopheles crucians, Anopheles quadrimaculatus, and Anopheles punctipennis) and Strelkovimermis spiculatus (against Aedes albifasciatus and Cx. pipiens; Petersen and Willis, 1974; Levy and Miller, 1977; Poinar and Camino, 1986; Santamarina Mijares and Perez Pacheco, 1997; Paily and Balaraman, 2000; Sanad et al., 2013, 2017; Abagli and Alavo, 2019; Abagli et al., 2019). However, the lack of culturable mermithids hinders mass application of this nematode as a biocontrol agent (Kendie, 2020).
Entomopathogenic fungi are another group of insect pathogens that have been explored as a potential vector biocontrol agent (Chapman, 1974). Fungi of genera Beauveria and Metarhizium have been shown to exert high mortality to medically important mosquitoes of genera Anopheles, Culex and Aedes (Blanford et al., 2011; Accoti et al., 2021). The fungal infection exhausts the mosquitoes due to increased metabolic rate and reduces their frequency of taking blood meals. As a result, the lifespan, oviposition rate, as well as the chance of infected mosquitoes to acquire and transmit medically important pathogens reduces greatly (Blanford et al., 2011). Interestingly, the fungi have been reported to affect both the larval and adult stages of mosquitoes (Blanford, 2005; Blanford et al., 2011). However, the virulence of fungi is influenced by various factors (Scholte et al., 2007; Paula et al., 2011b; Alkhaibari et al., 2017). For example, most fungi may lose their potency after a few months (Scholte et al., 2007). Besides, the lethality of entomopathogenic fungi is influenced by the nutritional state of the targeted vector (Paula et al., 2011b). Furthermore, different forms of fungi may demonstrate different potency against the mosquitoes. For instance, Ae. aegypti is more susceptible to the blastospores of Metarhizium, whereas Cx. quinquefasciatus is more susceptible to the conidia forms. On the other hand, An. stephensi is susceptible to both forms of Metarhizium (Alkhaibari et al., 2017). Notably, it is difficult to culture and mass produce fungi (Accoti et al., 2021). More importantly, these entomopathogenic fungi have been reported to cause symptomatic infections in immuno-compromised humans, raising safety concerns regarding this vector biocontrol agent (Henke et al., 2002; Tucker et al., 2004; Lara Oya et al., 2016; Goodman et al., 2018). These drawbacks render fungi a less attractive vector biocontrol option.
Bacillus thuringiensis var. israelis (Bti) is a bacterium commonly used as a household larvicide. This bacterium produces delta endotoxins (known as the “Cry” or “Cyt” toxins) during its sporulation, which are potent insecticide proteins (Tabashnik, 1992; Wu et al., 1994; Ben-Dov et al., 1995). The toxin has been demonstrated to kill larvae of Ae. aegypti and Ae. albopictus effectively, by disrupting the osmotic balance of the midgut epithelial cells upon ingestion (Chapman, 1974; Promdonkoy and Ellar, 2003; Lacey, 2007). Importantly, Bti does not pose direct ecological or health threats as it does not affect any off-target organisms including fishes, birds, mammals, and many other insects (Fayolle et al., 2015; Poulin and Lefebvre, 2018; Poulin et al., 2022). Nevertheless, research is underway to evaluate the indirect impact of Bti application, particularly its impacts on local ecological systems (Novak et al., 1986; Arredondo-Jimenez et al., 1990; Kumar et al., 1998; Ritchie et al., 2010; Fayolle et al., 2015; Poulin and Lefebvre, 2018; Poulin et al., 2022). Resistance against Cry toxin has yet to be reported. Nevertheless, development of tolerance toward some of the Cry toxins (Cry4Aa and Cry11Aa) was reported in a population of Ae. sticticus (Tetreau et al., 2013).
Viruses, such as the mosquito-specific densoviruses (MDV) may be used against the vectors too (Chapman, 1974). MDVs are highly infectious to its targets due to its capability of establishing vertical and horizontal transmission (Johnson and Rasgon, 2018). Upon infection, MDV causes a plethora of pathogeneses on their targets, which lead to apoptosis of infected larvae (Roekring and Smith, 2010), and shortening of adult lifespan (Suchman et al., 2006). Interestingly, MDV has been shown to reduce the viral load of type II DENV in Ae. albopictus (Wei et al., 2006). Besides, MDV can be genetically modified to cater for different conditions of vector control. For instance, a recombinant Ae. aegypti densovirus (AeDNV) expressing BmK IT1(an insect-specific toxin) was demonstrated to exert higher pathogenicity to Ae. albopictus (Gu et al., 2010). Despite these advantages, large-scale implementation of MDV-mediated vector biocontrol strategy may not be easy due to the relatively low stability of viral particles outside the hosts (Johnson and Rasgon, 2018). Nevertheless, advancement of technology may make this method more feasible for mass application in the future.
The potential vector biocontrol candidates above share a drawback that need to be overcome for mass application. It remains uncertain how sustainable these biocontrol agents can exist in the environment for a long-lasting controlling effect against the vector population. This is especially crucial for VBZ and VBIAR with complex and sporadic transmission profiles. Besides, candidates with healthcare risk concerns should not be employed until all doubts are scientifically cleared. Nevertheless, biocontrol candidates such as Bti and entomopathogenic fungi have been commercialized recently (Akutse et al., 2020).
Manipulation of vital biological functions
Alternative approaches that revolve around the manipulation of vector’s biology have been explored to develop a strategy that preserves the relatively target-specific nature of most pathogenesis-mediated biocontrol approaches while overcoming the drawbacks faced by these strategies. Hence, genetic manipulation of vector’s vital functions has gained increasing research attention. Sterile Insect Technique (SIT) is one of the successful examples of such approach (Baumhover et al., 1955; Lofgren et al., 1974; Patterson et al., 1977). In the SIT approach, the male vector is made infertile via radiation exposure or chemosterilization (Serebrovsky, 1940; Baxter, 2016). Subsequently, when these sterile males are released into the wild and mate with females, non-viable offspring are produced. As a result, the targeted vector population is reduced. This technique was successfully employed to control the infestation of the New World screwworm fly (Cochliomyia hominivorax) in the United States, whose maggots are capable of causing myiasis with severe tissue damages (Baumhover et al., 1955). SIT worked well against C. hominivorax because each female fly mates only once. As SIT-modified insects do not produce any offspring, the success of this technique depends on the persistent release of sterile male specimens to compete with the fertile wild type (WT) males for mating. Subsequently, this technique was attempted against mosquitoes in the 1970s, which yielded encouraging results. The population of Anopheles albimanus in El Salvador was reduced by 99% after implementing this technique for 5 months (Lofgren et al., 1974). Several mosquito-targeting field trials were performed in Burkina Faso, France, India, Myanmar, and United States. The experimented mosquitoes were Ae. aegypti, An. gambiae, An. quadrimaculatus, Cx pipiens, and Cx quinquefasciatus (Weidhaas et al., 1962; Dame et al., 1964; Laven, 1967; Davidson et al., 1970; Patterson et al., 1970; Curtis, 1976; Grover et al., 1976; Patterson et al., 1977; Curtis et al., 1982). These field trials yielded mixed results. For example, in India, the population of targeted mosquitoes was not effectively controlled with this approach, due to the immigration of mated WT females from the locations adjacent to the trial sites. In addition, political turmoil significantly affected the execution of this approach, which confounded the success of this strategy (Curtis, 1976; Curtis et al., 1982).
Despite the reported success, SIT is accompanied with several drawbacks. Firstly, there are concerns among the public members regarding the off-target effect of chemosterilizing agents to the environment (Bartumeus et al., 2019). Laboratory bioassays on non-target predators such as the common house spider (Achaeranea tepidariorum) revealed the significant reduction in fertility among the spiders that consumed the chemosterilized mosquitoes (Bracken and Dondale, 1972). Nevertheless, this issue can be overcome via simple bulk detoxification using acid and alkaline, which eliminates residues of chemosterilizing agents without compromising the efficacy of this method (Sharma, 1976). Secondly, the difficulty to precisely segregate male and female specimens in the insect colony implies the possibility of sterilizing female specimens by mistake (Sharma et al., 1976; McInnis et al., 1994; Parker, 2005). Accidental release of these mistakenly treated females will result in mating competition with the fertile WT females. As a result, the dispersal of sterile males will be compromised. In addition, radiation used in sterilization will significantly shorten the lifespan of these irradiated insects, which compromises the success of this technique in the field (Alphey and Andreasen, 2002). To overcome this issue, the concept of homozygous female-specific lethal genes has been applied, giving rise to techniques such as Genetic Sexing Strain (GSS) and Release of Insects carrying a Dominant Lethal Gene (RIDL; Franz, 2005; Fu et al., 2010; Harris et al., 2011; Carvalho et al., 2015; O’Leary and Adelman, 2020). RIDL enables selection of the developmental stage corresponding to the manifestation of engineered lethal traits. The insertion of a repressible dominant lethal transgene into the mosquito genome confers conditional fatality (such as tetracycline-dependent survival) to its late juvenile stage. In this approach, the engineered male mosquitoes are released to mate with the WT females. Instead of completing metamorphosis, the produced juveniles that carry a copy of the engineered gene will die in the absence of tetracycline (Phuc et al., 2007). Indeed, field trials of Ae. aegypti OX513A in Cayman Islands and Brazil demonstrated strong suppression of the targeted mosquito population (Harris et al., 2012; Carvalho et al., 2015). In addition, female-specific flightless phenotype and DENV-susceptible phenotype that are genetically engineered in Ae. aegypti have improved the gender segregation and impeded vector competence to DENV, respectively (de Valdez et al., 2011; Buchman et al., 2020). These techniques minimize the “leakage” of “accidentally treated females” into the wild (Franz, 2002; Calkins and Parker, 2005; Franz, 2005; Koskinioti et al., 2021). In general, the attempts to overcome the shortcomings of SIT revolve around gene editing, which was highly challenging decades ago. However, the discovery and establishment of CRISPR/Cas9 system allows gene editing to be performed much more easily (Gupta et al., 2019). This molecular advancement facilitates the application of SIT against different vectors.
Besides facilitating SIT in vector biocontrol approach, CRISPR/Cas9 can be applied to genetically design arthropod vectors that are not receptive to pathogens transmitted by them under normal circumstances. For example, the knock-out of FREP1 gene has been shown to reduce the susceptibility of An. gambiae to Plasmodium spp. (Dong et al., 2018). Gene drive is another genetic engineering concept that has enjoyed a great push in vector control research following the establishment of CRISPR/Cas9 technology. The CRISPR/Cas9- integrated gene drive method allows the targeted genes to be propagated and inherited much more rapidly than the Mendelian rates, resulting in fast replacement or displacement of the targeted traits in a population (Leung et al., 2022). Recently, this technology has been applied on An. gambiae, resulting in a successful halting of Plasmodium development within the genetically modified mosquitoes, as well as compromising the survival of the homozygous transgenic females (Hoermann et al., 2022). In addition, other gene editing methods, such as the application of homing endonuclease genes (HEG) have been explored to control the malaria vectors (Windbichler et al., 2007; Deredec et al., 2011). Nevertheless, such genetically engineered mosquitoes suffered compromised fitness that hindered their sustainable establishment in the wild. This drawback is in fact a major concern, as modification of one gene may lead to unexpected outcomes on the experimented organism (Resnik, 2014, 2017). If the mutants with unexpected and undesirable traits (following gene editing) thrive in the wild, the ecosystem may be threatened in an unprecedented manner. Nevertheless, the successful application of vital function modification to control a myiasis causative agent with wild and domestic animals as reservoirs reflects the great potential of this approach to control VBZ and VBIAR. Importantly, techniques stemming from this approach should be tested, evaluated, and validated thoroughly before mass application.
Wolbachia as a novel vector biocontrol approach
As elaborated earlier, the pathogenesis-mediated biocontrol agents are arthropod pathogens that shorten the lifespan of vectors, whereas genetic manipulation of arthropod vital functions works by halting the vectors’ population expansion. The application of Wolbachia in vector biocontrol is a unique approach that combines the characteristics of both approaches. Wolbachia are maternally inherited, gram-negative, obligate intracellular endosymbiotic bacteria found in many arthropods such as mites, spiders, scorpions and isopods (Werren et al., 2008). Wolbachia are found in various organs and tissues within the infected arthropod (Werren, 1997; Werren et al., 2008). Besides, medically important filarial nematodes carry Wolbachia as well (Lau et al., 2015).
Approximately 60% of the insects are positive for Wolbachia. Interestingly, Ae. aegypti is Wolbachia-free under normal condition (Kittayapong et al., 2000; Rasgon and Scott, 2004). In the early 2000s, Xi et al. (2005) successfully performed an experimental infection on Ae. aegypti with Wolbachia wAlbB strain (henceforth wAlbB) derived from Ae. albopictus. Subsequently, this finding was explored further, with trial release of wAlbB-infected Ae. aegypti in several locations reported increased resistance of the vector to DENV, ZIKV, and CHIKV (Bian et al., 2010; Aliota et al., 2016a,b; Chouin-Carneiro et al., 2019; Nazni et al., 2019). Meanwhile, the infection of Ae. aegypti by another more virulent strain of Wolbachia (wMelPop strain) has been shown to reduce the number of Ae. aegypti significantly (Rasgon et al., 2003; Ritchie et al., 2015). These findings highlight the potential of Wolbachia as a tool in vector control program. Therefore, the mechanisms behind the effects cast by Wolbachia on the infected mosquitoes have received increasing research attention over the past two decades.
Wolbachia have evolved and developed various mechanisms to manipulate the host’s cellular biology toward their survival advantage, namely cytoplasmic incompatibility (CI), parthenogenesis, feminization, and male killing (O'Neill et al., 1997; Werren, 1997; Werren et al., 2008). CI happens when a Wolbachia-infected male mates with either a Wolbachia-negative female or a female infected with a different strain of Wolbachia, resulting in non-viable progeny (Werren, 1997). This principle forms the basis of “Incompatible Insect Technique (IIT)” that drives many Wolbachia-mediated biocontrol programs against Ae. aegypti in China, the United States, and Singapore (Ritchie et al., 2015; Mains et al., 2019; Zheng et al., 2019; Soh et al., 2021). Parthenogenesis refers to the development of eggs into progenies without fertilization, whereas feminization involves development of genetic male into female. Wolbachia has been shown to induce feminization in several crustaceans and insects (Werren, 1997; Cordaux et al., 2001; Kageyama et al., 2002; Negri et al., 2006; Werren et al., 2008; Asgharian et al., 2014; Scola et al., 2015). Meanwhile, male killing happens when the affected males experience a significantly shorter lifespan than the affected females. CI, parthenogenesis, feminization, and male killing trigger disruption of gender ratio in the affected population toward female dominance. Using these strategies, Wolbachia manipulates the population structure of the infected arthropods, which facilitates the spread and establishment of Wolbachia in the wild (Hoffman and Turelli, 1997; Werren, 1997; Werren et al., 2008). Coupled with the reported resistance to virus infection by the Wolbachia-infected mosquitoes, the establishment of Wolbachia in the vector population may suppress the transmission of these pathogens to humans. In fact, countries such as Malaysia, Indonesia, Laos, Vietnam, Sri Lanka, Australia, Fiji, Vanuatu, Brazil, Colombia, and Mexico have released Wolbachia -infected female Ae. aegypti to establish a stable Wolbachia-infected mosquito colony in the wild (Nazni et al., 2019; World Mosquito Program, 2022). Besides mosquitoes, Wolbachia has been explored for the control of black flies and sand flies. However, difficulties in colony maintenance of black flies and sand flies, coupled with the relatively low Wolbachia load post-infection in these insects giving rise to the undetectable CI among these insects. This implied the unsuitability of Wolbachia for the control of these non-mosquito vectors. Therefore, the versatility of the Wolbachia biocontrol approach remains to be validated (Crainey et al., 2010; Bordbar et al., 2014).
Despite the promising advantages of Wolbachia-mediated vector biocontrol approach, this method has several shortcomings and concerns. Similar to SIT, the Wolbachia method faces the issue of accidental “female leakage” that may compromise the efficacy of IIT-driven vector control strategy. For instance, IIT that incorporates Wolbachia can be dampened by mass production. Accidental release of Wolbachia-infected females during field trial could affect the population suppression goal. Nevertheless, this may not be considered as an absolute disadvantage, as Wolbachia-infected mosquitoes have been claimed to be less susceptible to the medically important pathogens that they carry (Bian et al., 2010; Nazni et al., 2019). To date, the long-term impact of Wolbachia on the targeted mosquitoes has not been well studied, partly due to the relatively short discovery history of this vector biocontrol candidate. Furthermore, the interaction dynamics among the arthropod host, Wolbachia, and the medically important pathogens carried by the arthropod remains to be fully deciphered. Nevertheless, few studies on this topic revealed interesting findings. For example, a previous study demonstrated that the Wolbachia-infected Culex tarsalis became more susceptible to West Nile Virus, with much higher viral load post-infection, as compared to the Wolbachia-free specimens (Dodson et al., 2014). Since Wolbachia has been shown to interfere with interactions between the arthropod host and the medically important pathogens that it carries, it is of utmost importance to consistently assess the efficacy and impact of Wolbachia deployed in vector control programs. Besides, the wMelPop-related strains have been demonstrated to be temperature-sensitive, raising doubts about the sustainability of this approach in areas with higher temperature (Ulrich et al., 2016; Ross et al., 2017). Succinctly, these concerns deserve more research attention despite the higher research difficulty, where longitudinal study covering adequately long duration is needed.
Besides, concerns have been raised regarding the possibility of Wolbachia to cause pathology to humans. Although Wolbachia can be found in mosquito salivary glands, the bacteria are not available in saliva, as backed by polymerase chain reaction (PCR) screenings (Wu et al., 2004; Moreira et al., 2009b). In addition, Wolbachia are larger than the mosquito salivary duct (Moreira et al., 2009b). Hence, it is relatively unlikely for the bacteria to be transmitted to humans via mosquito bites. Moreover, human volunteers exposed to Wolbachia-infected mosquitoes over extended period of time revealed absence of antibody specific to Wolbachia in their blood (Popovici et al., 2010). Of note, responses to Wolbachia or Wolbachia-derived antigens by other key players in human immune system remained unclear. Based on currently available information, Wolbachia application has been considered as a relatively safe vector biocontrol approach. There are environmental concerns regarding this approach as well. In fact, the major environmental concern is extrapolated from the public health concern, where Wolbachia may spread to other organisms across the mosquito-related food chain in the ecosystem. This may disrupt the ecosystem dynamics, hence threatening the biodiversity in the affected environment. Fortunately, Wolbachia has been proven to be unable to establish itself throughout the mosquito-associated food chain (Popovici et al., 2010). Notably, natural cross-species infestation of Wolbachia is extremely rare (Werren et al., 1995; Werren, 1997), let alone a sustained establishment that allows vertical transmission (Hoffman and Turelli, 1997; Turelli, 2010).
Importantly, Wolbachia vector control approach may work well against a disease transmission that involves only one species of arthropod as vector. The effects of Wolbachia infection varies with different species of vectors. Hence, infections with multiple vectors, or those with incomplete list of vectors cannot employ this method as vector control program. The clearance of one vector by the bacteria may allow other vectors to thrive, rendering the disease control futile. Knowlesi malaria is an example of VBZ with multiple vectors, and the list of knowlesi malaria vectors is expanding for the moment (Ang et al., 2020; Jeyaprakasam et al., 2020; Pramasivan et al., 2021; Vythilingam et al., 2021). Apart from this, the actual efficacy of Wolbachia approach to reduce disease transmission has been questioned. Over the past few years, an increasing number of countries have participated in the release of Wolbachia-infected Ae. aegypti. Nevertheless, many of these countries still experience increased burden of dengue transmission after persistent release of these mosquitoes (Kementerian Kesihatan Malaysia, 2022). The difficulty to establish stable Wolbachia colony within the environment, stability and sustainability of this method in the field, and relative attractiveness of Wolbachia-infected mosquitoes during mating may contribute to the challenges faced by this approach to secure a more obvious disease transmission chain breakage in these countries. Obviously, more investigations are needed to better understand this approach, and its practicality, as well as its sustainability in the field.
Prospects and challenges of vector control against VBZ and VBIAR
The control and eradication of VBZ and VBIAR hardly rely on a single approach of vector management, due to the complexity of their transmission circuit. Hence, the application of integrated vector management (IVM) that incorporates multiple vector control approaches may increase the success rate of breaking the transmission circuit of these infections (WHO, 2012). To implement a successful and sustainable IVM, the components of IVM triads (biological, environmental, and chemical) should be covered during the designing of the vector control plan (Figure 3). Adaptation and customization of vector control strategies according to the targeted locations are required to ensure high success. For example, the landscape of a targeted location can be modified to facilitate the implementation of vector biocontrol strategies. At the same time, environment-friendly chemicals that can promote biocontrol strategy (such as predator attractants and pheromone-like substances) can be applied. IVM is a multi-prong approach against the vectors, where the selected strategies may complement each other to bring down the vector population. Moreover, IVM may minimize the risk of complete failure faced by a vector control program, as other components in the IVM may continue to work normally when one component is breaking down. For instance, ORS (chemical approach) may be completely stopped during the total lockdown of sudden onset (as exemplified by the COVID-19 pandemic-triggered lockdown in many countries). If the affected area has a well-constructed and maintained drainage system that hampers oviposition by the vectors (environmental management approach), the vector population in that area may not increase after ORS is brought to an abrupt halt. In short, multiple components should be explored to synergize the vector control effort.
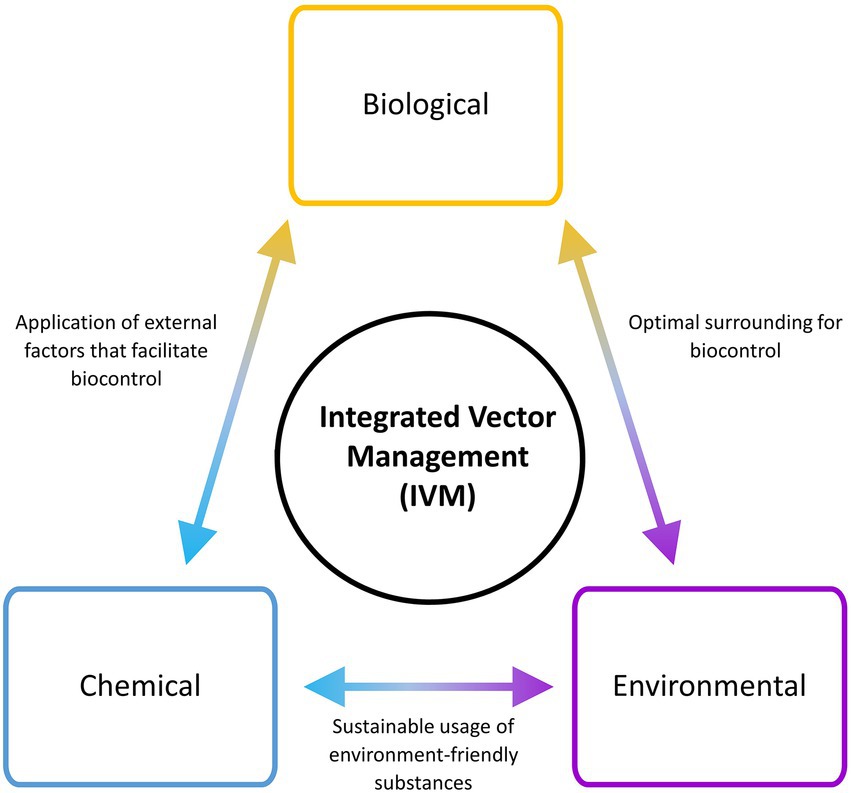
Figure 3. Integrated Vector Management (IVM) involving different components in planning and implementation. Multiple components are factored into an IVM strategy to optimize the output of vector control.
While promoting IVM against VBZ and VBIAR, we should not overlook other co-existing factors in the surrounding that may confound the outcomes of the vector control strategy. For example, knowlesi malaria is one of the most prevalent VBZ in Southeast Asia, with Malaysia serving as the epicenter of transmission. Unlike P. falciparum that has developed resistance strategies against artemisinin (the current first line anti-malarial treatment; Fairhurst and Dondorp, 2016; Lee et al., 2021), P. knowlesi remains susceptible to artemisinin and other anti-malarials in the market (Fatih et al., 2013; van Schalkwyk et al., 2017). Nevertheless, this zoonotic parasite can cause hyperparasitaemia and life-threatening pathogenesis in humans (Lee et al., 2013; Singh and Daneshvar, 2013). Hence, various strategies have been considered to control and eliminate this infection, including the vector management. The potential of different chemical-based approaches has been investigated (Rohani et al., 2020), and IVM against knowlesi malaria transmission has been proposed (Lee et al., 2022). Currently, it is challenging to implement an all-rounded IVM against knowlesi malaria in many hyperendemic areas as the vector profile of this VBZ has yet to be completely deciphered. As mentioned earlier, environment management demands a thorough evaluation of vector profile, transmission dynamics and socio-economic activities in the targeted area. Landscape modification that aims against the incriminated vector may effectively clear the targeted vector’s population. However, the altered landscape may become a conducive breeding ground for another species of anophelines capable of transmitting P. knowlesi. Besides, many places affected by knowlesi malaria are endemic to other vector-borne diseases such as dengue and filariasis (Murphy et al., 2020; Zakaria and Avoi, 2022). Therefore, strategies aimed at reducing the transmission of knowlesi malaria should not facilitate the expansion of vectors responsible for other vector-borne diseases. Given the complexity of disease transmission dynamics in many areas endemic to knowlesi malaria, it is not surprising that chemical-based vector control approaches are preferred over other approaches as chemicals are effective against broader range of vectors, despite the potential harmful effects to the environment. Nevertheless, biocontrol strategies with lower target specificity (such as the predator–prey approaches) deserve more attention. Interestingly, edible fishes can be explored as biocontrol candidates, as demonstrated in western Kenya (Howard et al., 2007). The Nile tilapia used in this earlier study is a commonly farmed and eaten fish. In this study, the Nile tilapia significantly reduced the population of An. gambiae s.l., An. funestus and culicine mosquitoes (Howard et al., 2007). Such integration of vector biocontrol and socioeconomic activity can ensure better sustainability of the implemented vector control efforts. Chemical-based approaches, such as IRS and ORS may be considered and implemented with caution. In addition, environment management via human behavioral changes should be emphasized, particularly for VBZ like knowlesi malaria, in which the transmission is associated with socioeconomic activities near or within forested areas, such as tourism, logging, and subsistence cropping (Singh and Daneshvar, 2013; Müller and Schlagenhauf, 2014; Lee et al., 2022). The challenges faced in the control and prevention of knowlesi malaria in Malaysia are applicable to other VBZ and VBIAR. Obviously, there are numerous knowledge gaps that need to be filled with properly designed studies to put forward better vector control programs. Notably, the long-term safety, efficacy, and sustainability of all proposed methods should be investigated thoroughly prior to mass application. Such information is needed to convince the public members and secure their support and compliance to a proposed vector control program, which is crucial to many IVMs. In short, various factors must be taken into consideration when designing a control strategy against VBZ and VBIAR, particularly in areas endemic to multiple vector-borne diseases.
Conclusion
Vector control has always been a crucial component of breaking the transmission circuit of vector borne diseases. The increased prevalence of vector-borne diseases, including several VBZ and VBIAR in different parts of the world implies a more important role of vector control in healthcare sector. Indeed, there is no “silver bullet” for outbreak management, even more so for the management of the more complex VBZ and VBIAR. Careful integration of multiple vector control approaches in the vector management program may increase the success of disease control and prevention. While battling these pathogens with large investment in the research and development for treatments and vaccines, continuous efforts of discovering novel vector control approaches should be made concurrently, to reduce the prevalence of these infections without compromising the wellbeing of the environment, humans, and the animals involved in the transmission circuit.
Author contributions
W-CL, MLW, and ZZ conceptualized the review. W-CL, MLW, and ZZ performed literature review and information interpretation. W-CL, MLW, ZZ, IV, YL, MYF, and I-CS involved in manuscript preparation. All authors contributed to the article and approved the submitted version.
Funding
We would like to thank the Ministry of Higher Education, Malaysia, for the Fundamental Research Grant Scheme (FRGS/1/2022/SKK12/UM/02/9) awarded to W-CL. MLW and MYF were supported by the Long-Term Research Grant Scheme (LRGS) by the Ministry of Higher Education, Malaysia (LRGS/1/2018/UM/01/1/1). IV was supported by another LRGS of the Ministry of Higher Education, Malaysia (LRGS/1/2018/UM/01/1/3). YL was supported by another LRGS of the Ministry of Higher Education, Malaysia (LRGS/1/2018/UM/01/1/4).
Conflict of interest
The authors declare that the research was conducted in the absence of any commercial or financial relationships that could be construed as a potential conflict of interest.
W-CL is an editorial board member of Frontiers in Microbiology, and one of the guest associate editors for the research topic “Zoonoses – a rising threat to healthcare system.” The authors agree to abide by the rules, ethical standards and practices of Frontiers; and this does not alter the authors’ adherence to all the Frontiers policies on publishing and sharing of information.
Publisher’s note
All claims expressed in this article are solely those of the authors and do not necessarily represent those of their affiliated organizations, or those of the publisher, the editors and the reviewers. Any product that may be evaluated in this article, or claim that may be made by its manufacturer, is not guaranteed or endorsed by the publisher.
References
Aagaard, K., Luna, R. A., and Versalovic, J. (2015). “The human microbiome of local body sites and their unique biology” in Mandell, Douglas, and Bennett's principles and practice of infectious diseases. eds. J. E. Bennett, R. Dolin, and M. J. Blaser, vol. 2. 8th ed (Philadelphia, PA: Elsevier/Saunders), 12–21.
Abagli, A. Z., and Alavo, T. B. C. (2019). Biocontrol of Culex quinquefasciatus using the insect parasitic nematode, Romanomermis iyengari (Nematoda: Mermithidae). Trop. Biomed. 36, 1003–1013.
Abagli, A. Z., Alavo, T. B. C., Perez-Pacheco, R., and Platzer, E. G. (2019). Efficacy of the mermithid nematode, Romanomermis iyengari, for the biocontrol of Anopheles gambiae, the major malaria vector in sub-Saharan Africa. Parasit. Vectors 12:253. doi: 10.1186/s13071-019-3508-6
Accoti, A., Engdahl, C. S., and Dimopoulos, G. (2021). Discovery of novel entomopathogenic fungi for mosquito-borne disease control. Front. Fungal Biol. 2:637234. doi: 10.3389/ffunb.2021.637234
Aditya, G., Ash, A., and Saha, G. K. (2006). Predatory activity of Rhantus sikkimensis and larvae of Toxorhynchites splendens on mosquito larvae in Darjeeling, India. J. Vector Borne Dis. 43, 66–72.
Aditya, G., Bhattacharyya, S., Kundu, N., and Saha, G. K. (2005). Frequency-dependent prey-selection of predacious water bugs on Armigeres subalbatus immatures. J. Vector Borne Dis. 42, 9–14.
Aditya, G., Bhattacharyya, S., Kundu, N., Saha, G. K., and Raut, S. K. (2004). Predatory efficiency of the water bug Sphaerodema annulatum on mosquito larvae (Culex quinquefasciatus) and its effect of the adult emergence. Bioresour. Technol. 95, 169–172. doi: 10.1016/j.biortech.2004.02.007
Ahantarig, A., and Kittayapong, P. (2011). Endosymbiotic Wolbachia bacteria as biological control tools of disease vectors and pests. J. Appl. Entomol. 135, 470–486. doi: 10.1111/j.14390418.2011.01641.x
Ahantarig, A., Trinachartvanit, W., Baimai, V., and Grubhoffer, L. (2013). Hard ticks and their bacteria endosymbionts (or would be pathogens). Folia Microbiol. 58, 419–428. doi: 10.1007/sl2223-013-0222-1
Ahmad, N. A., Vythilingam, I., Lim, Y. A. L., Zabari, N. Z. A. M., and Lee, H. L. (2017). Detection of Wolbachia in Aedes albopictus and their effects on chikungunya virus. Am. J. Trop. Med. Hyg. 96, 148–156. doi: 10.4269/ajtmh.16-0516
Akutse, K. S., Subramaniam, S., Maniania, N., Dubois, T., and Ekesi, S. (2020). Biopesticide research and product development in Africa for sustainable agriculture and food security-experiences from the International Centre of Insect Physiology and Ecology (ICIPE). Front. Sustain. Food Syst. 4:563016. doi: 10.3389/fsufs.2020.563016
Albicócco, A. P., and Vezzani, D. (2009). Further study on Ascogregarina culicis in temperate Argentina: prevalence and intensity in Aedes aegypti larvae and pupae. J. Invertebr. Pathol. 101, 210–214. doi: 10.1016/j.jip.2009.05.003
Aliota, M. T., Peinado, S. A., Velez, I. D., and Osorio, J. E. (2016a). The wMel strain of Wolbachia reduces transmission of Zika virus by Aedes aegypti. Sci. Rep. 6:28792. doi: 10.1038/srep28792
Aliota, M. T., Walker, E. C., Uribe Yepes, A., Dario Velez, I., Christensen, B. M., and Osorio, J. E. (2016b). The wMel strain of Wolbachia reduces transmission of Chikungunya virus in Aedes aegypti. PLoS Negl. Trop. Dis. 10:e0004677. doi: 10.1371/journal.pntd.0004677
Alkhaibari, A. M., Carolino, A. T., Bull, J. C., Samuels, R. I., and Butt, T. M. (2017). Differential pathogenicity of Metarhizium blastospores and conidia against larvae of three mosquito species. J. Med. Entomol. 54, 696–704. doi: 10.1093/jme/tjw223
Allahverdipour, H. H., Talaei-Hassanloui, R., Karimi, J., Wang, Y., Rochlin, I., and Gaugler, R. (2019). Behavior manipulation of mosquitoes by a mermithid nematode. J. Invertebr. Pathol. 168:107273. doi: 10.1016/j.jip.2019.107273
Alphey, L., and Andreasen, M. (2002). Dominant lethality and insect population control. Mol. Biochem. Parasitol. 121, 173–178. doi: 10.1016/S0166-6851(02)00040-3
Amalraj, D. D., and Das, P. K. (1998). Estimation of predation by the larvae of Toxorhynchites splendens on the aquatic stages of Aedes aegypti. Southeast Asian J. Trop. Med. Public Health 29, 177–183.
Amelia-Yap, Z. H., Azman, A. S., AbuBakar, S., and Low, V. L. (2022). Streptomyces derivatives as an insecticide: current perspectives, challenges and future research needs for mosquito control. Acta Trop. 229:106381. doi: 10.1016/j.actatropica.2022.106381
Anderson, J. F. (1968). Microsporidia parasitizing mosquitoes collected in Connecticut. J. Invertebr. Pathol. 11, 440–455. doi: 10.1016/0022-2011(68)90192-4
Anderson, J. F. (1970). An iridescent virus infecting the mosquito Aedes stimulans. J. Invertebr. Pathol. 15, 219–224. doi: 10.1016/0022-2011(70)90238-7
Anderson, J. F., and Ringo, S. L. (1969). Entomophthora aquatica sp. n. Infecting larvae and pupae of floodwater mosquitoes. J. Invertebr. Pathol. 13, 386–393. doi: 10.1016/0022-2011(69)90191-8
Anderson, B. E., Sims, K. G., Olson, J. G., Childs, J. E., Piesman, J. F., Happ, C. M., et al. (1993). Amblyomma americanum: a potential vector of human ehrlichiosis. Am. J. Trop. Med. Hyg. 49, 239–244. doi: 10.4269/ajtmh.1993.49.239
Andrade, M. R. (2015). Container-dwelling mosquitoes: habitat size, direct and indirect effects of predation. Minas Gerais, Brazil: Universidade Federal de Viçosa.
Ang, J. X. D., Kadir, K. A., Mohamad, D. S. A., Matusop, A., Divis, P. C. S., Yaman, K., et al. (2020). New vectors in northern Sarawak, Malaysian Borneo, for the zoonotic malaria parasite, Plasmodium knowlesi. Parasit. Vectors 13:472. doi: 10.1186/s13071-020-04345-2
Annis, B., Krisnowardojo, S., Atmosoedjono, S., and Supardi, P. (1989). Suppression of larval Aedes aegypti populations in household water storage containers in Jakarta, Indonesia through releases of first-instar Toxorhynchites splendens larvae. J. Am. Mosq. Control Assoc. 5, 235–238.
Annis, B., Nalim, S., Hadisuwasono, W., and Boewono, D. T. (1990). Toxorhynchites amboinensis larvae released in domestic containers fail to control dengue vectos in a rural village in Central Java. J. Am. Mosq. Control Assoc. 6, 75–78.
Armengol, G., Hernandez, J., Velez, J. G., and Orduz, S. (2006). Long-lasting effects of a bacillus thuringiensis serovar israelensis experimental tablet formulation for Aedes aegypti (Diptera: Culicidae) control. J. Econ. Entomol. 99, 1590–1595. doi: 10.1603/0022-0493-99.5.1590
Arredondo-Jimenez, J. I., Lopez, T., Rodriguez, M. H., and Bown, D. N. (1990). Small scale field trials of Bacillus sphaericus (strain 2362) against anopheline and culicine mosquito larvae in southern Mexico. J. Am. Mosq. Control Assoc. 6, 300–305.
Arthington, A. H. (1991). Ecological and genetic impacts of introduced and translocated freshwater fishes in Australia. Can. J. Fish. Aquat. Sci. 48, 33–43. doi: 10.1139/f91-302
Asale, A., Duchateau, L., Devleesschauwer, B., Huisman, G., and Yewhalaw, D. (2017). Zooprophylaxis as a control strategy for malaria caused by the vector anopheles arabiensis (Diptera: Culicidae): a systematic review. Infect. Dis. Poverty 6:160. doi: 10.1186/s40249-017-0366-3
Asgharian, H., Chang, P. L., Mazzoglio, P. J., and Negri, I. (2014). Wolbachia is not all about sex: male-feminizing Wolbachia alters the leafhopper Zyginidia pullula transcriptome in a mainly sex-independent manner. Front. Microbiol. 5:430. doi: 10.3389/fmicb.2014.00430
Ault, S. K. (1994). Environmental management: a re-emerging vector control strategy. Am. J. Trop. Med. Hyg. 50, 35–49. doi: 10.4269/ajtmh.1994.50.35
Bacot, A. W. (1915). LXXXI. Further notes on the mechanism of the transmission of plague by fleas. J. Hyg. 14, 774–776.3.
Bacot, A. W., and Martin, C. J. (1914). LXVII. Observations on the mechanism of the transmission of plague by fleas. J. Hyg. 13, 423–439.
Badakhshan, M., Sadraei, J., and Moin-Vaziri, V. (2013). The first report of Eustigmaeus johnstoni (Acari: Stigmaeidae) parasitic mite of Phlebotominae sand flies from Iran. J. Arthropod. Borne Dis. 7, 94–98.
Bakken, J. S., and Dumler, J. S. (2015). Human granulocytic anaplasmosis. Infect. Dis. Clin. N. Am. 29, 341–355. doi: 10.1016/j.idc.2015.02.2007
Balfour, A. (1914). The wild monkey as a reservoir for the virus of yellow fever. Lancet 183, 1176–1178. doi: 10.1016/S0140-6736(01)56899-3
Barker, S. C., and Walker, A. R. (2014). Ticks of Australia: the species that infect domestic animals and humans. Zootaxa 3816, 1–144. doi: 10.11646/zootaxa.3816.1.1
Barrett, W. L. J. (1968). The damage caused by Lankesteria culicis (Ross) to Aedes aegypti (L.). Mosq. News 28, 441–444.
Barrett, A. D. T., and Higgs, S. (2007). Yellow fever: a disease that has yet to be conquered. Annu. Rev. Entomol. 52, 209–229. doi: 10.1146/annurev.ento.52.110405.091454
Bartumeus, F., Costa, G. B., Eritja, R., Kelly, A. H., Finda, M., Lezaun, J., et al. (2019). Sustainable innovation in vector control requires strong partnerships with communities. PLoS Negl. Trop. Dis. 13:e0007204. doi: 10.1371/journal.pntd.0007204
Baumhover, A. H., Graham, A. J., Bitter, B. A., Hopkins, D. E., New, W. D., Dudley, F. H., et al. (1955). Screw-worm control through release of sterilized flies. J. Econ. Entomol. 48, 462–466. doi: 10.1093/JEE/48.4.462
Baxter, R. H. G. (2016). Chemosterilants for control of insects and insect vectors of disease. Chimia (Arau) 70, 715–720. doi: 10.2533/chimia.2016.715
Bay, E. C. (1974). Predatory-prey relationships among aquatic insects. Annu. Rev. Entomol. 19, 441–453. doi: 10.1146/annurev.en.19.010174.002301
Ben-Dov, E., Boussiba, S., and Zaritsky, A. (1995). Mosquito larvicidal activity of Escherichia coli with combinations of genes from bacillus thuringiensis subsp. Israelensis. J. Bacteriol. 177, 2851–2857. doi: 10.1128/jb.177.10.2851-2857.1995
Benelli, G., Jeffries, C. L., and Walker, T. (2016). Biological control of mosquito vectors: past, present, and future. Insects 7:52. doi: 10.3390/insects7040052
Bezuidenhout, J. D., and Stutterheim, C. J. (1980). A critical evaluation of the role played by the red-billed oxpecker Buphagus erythrorhynchus in the biological control of ticks. Onderstepoort J. Vet. Res. 47, 51–75.
Bhatt, S., Weiss, D. J., Cameron, E., Bisanzio, D., Mappin, B., Darymple, U., et al. (2015). The effect of malaria control on plasmodium falciparum in Africa between 2000 and 2015. Nature 526, 207–211. doi: 10.1038/nature15535
Bian, G., Joshi, D., Dong, Y., Lu, P., Zhou, G., Pan, X., et al. (2013). Wolbachia invades Anopheles stephensi populations and induces refractoriness to plasmodium infection. Science 340, 748–751. doi: 10.1126/science.1236192
Bian, G., Xu, Y., Lu, P., Xie, Y., and Xi, Z. (2010). The endosymbiotic bacterium Wolbachia induces resistance to dengue virus in Aedes aegypti. PLoS Pathog. 6:e1000833. doi: 10.1371/journal.ppat.1000833
Biernat, B., Karbowiak, G., Werszko, J., and Stańczak, J. (2014). Prevalence of tick-borne encephalitis virus (TBEV) RNA in Dermacentor reticulatus ticks from natura and urban environment, Poland. Exp. Appl. Acarol. 64, 543–551. doi: 10.1007/s10493-014-9836-5
Blanford, S. (2005). Fungal pathogen reduces potential for malaria transmission. Science 308, 1638–1641. doi: 10.1126/science.1108423
Blanford, S., Shi, W., Christian, R., Marden, J. H., Koekemoer, L. L., Brooke, B. D., et al. (2011). Lethal and pre-lethal effects of a fungal biopesticide contribute to substantial and rapid control of malaria vectors. PLoS One 6:e23591. doi: 10.1371/journal.pone.0023591
Bøgh, C., Clarke, S. E., Pinder, M., Sanyang, F., and Lindsay, S. W. (2001). Effect of passive zooprophylaxis on malaria transmission in the Gambia. J. Med. Entomol. 38, 822–828. doi: 10.1603/0022-2585-38.6.822
Bonnet, S., Brisseau, N., Hermouet, A., Jouglin, M., and Chauvin, A. (2009). Experimental in vitro transmission of Babesia sp. (EU1) by Ixodes ricinus. Vet. Res. 40:21. doi: 10.1051/vetres/2009004
Bonsall, J. L., and Goose, J. (1986). The safety evaluation of bendiocab, a residual insecticide for vector control. Toxicol. Lett. 33, 45–59. doi: 10.1016/0378-4274(86)90070-6
Bordbar, A., Soleimani, S., Fardid, F., Zolfaghari, M. R., and Parvizi, P. (2014). Three strains of Wolbachia pipientis and high rates of injection in Iranian sandfly species. Bull. Entomol. Res. 104, 195–202. doi: 10.1017/S0007485313000631
Bos, R. (1991). Cost-effectiveness of environmental management for vector control in resource development projects. Ann. Soc. Belg. Med. Trop. 71, 243–255.
Bouma, M., and Rowland, M. (1995). Failure of passive zooprophylaxis: cattle ownership in Pakistan is associated with a higher prevalence of malaria. Trans. R. Soc. Trop. Med. Hyg. 89, 351–353. doi: 10.1016/0035-9203(95)90004-7
Boussinesq, M. (2006). Loiasis. Ann. Trop. Med. Parasitol. 100, 715–731. doi: 10.1179/136485906X112194
Bowatte, G., Perera, P., Senevirathne, G., Meegaskumbura, S., and Meegaskumbura, M. (2013). Tadpoles as dengue mosquito (Aedes aegypti) egg predators. Biol. Control 67, 469–474. doi: 10.1016/j.biocontrol.2013.10.005
Bracken, G. K., and Dondale, C. D. (1972). Fertility and survival of Achaeranea tepidariorum (Araneida: Therididae) on a diet of chemosterilized mosquitoes. Can. Entomol. 104, 1709–1712. doi: 10.4039/ent104709-11
Brelsfoard, C. L., and Dobson, S. L. (2009). Wolbachia-based strategies to control insect pests and disease vectors. Asia-Pac. J. Mol. Biol. Biotechnol. 17, 55–63.
Brown, M. D. (1996). Evaluation of Mesocyclops aspericornis (Cyclopoida: Cyclopidae) and Toxorhynchite speciosus as integrated predators of mosquitoes in tire habitats in Queensland. J. Am. Mosq. Control Assoc. 12, 414–420.
Bruce, D. (1895). Preliminary report on the tsetse fly disease or nagana in Zululand. Durban: Bennett & Davis.
Bruce, D. (1915). The Croonian lectures on trypanosomes causing disease in man and domestic animals in Central Africa: delivered before the Royal College of Physicians of London. BMJ 2, 5–10. doi: 10.1136/bmj.2.284.5
Buchman, A., Gamez, S., Li, M., Antoshechkin, I., Li, H.-H., Wang, H.-W., et al. (2020). Broad dengue neutralization in mosquitoes expressing an engineered antibody. PLoS Pathog. 16:e1008103. doi: 10.1371/journal.ppat.1008103
Buczek, A., Buczek, W., Bartosik, K., Kulisz, J., and Stanko, M. (2021). Ixodiphagus hookeri wasps (hymenoptera: Encyrtidae) in two sympatric tick species Ixodes ricinus and Haemaphysalis concinna (Ixodida: Ixodidae) in the Sloavak karst (Slovakia): ecological and biological considerations. Sci. Rep. 11:11310. doi: 10.1038/s41598-021-90871-7
Burroughs, A. L. (1947). Sylvatic plague studies: the vector efficiency of nine species of fleas compared with Xenopsylla cheopis. J. Hyg. 45, 371–396. doi: 10.1017/s0022172400014042
Busvine, J. R. (1993). Disease transmission by insects: Its discovery and 90 years of effort to prevent it. New York/Berlin: Springer.
Calkins, C. O., and Parker, A. G. (2005). “Sterile insect quality” in Sterile insect technique: Principles and practice in area-wide integrated pest management. eds. V. A. Dyck, J. Hendrichs, and A. S. Robinson (The Netherlands: Springer)
Canyon, D. V., and Hii, J. L. (1997). The gecko: an environmentally friendly biological agent for mosquito control. Med. Vet. Entomol. 11, 319–323. doi: 10.1111/j.1365-2915.1997.tb00416.x
Carvalho, D. O., McKemey, A. R., Garziera, L., Lacroix, R., Donnelly, C. A., Alphey, L., et al. (2015). Suppression of a field population of Aedes aegypti in Brazil by sustained release of transgenic male mosquitoes. PLoS Negl. Trop. Dis. 9:e0003864. doi: 10.1371/journal.pntd.0003864
Casas, M., Torres, J. L., Bown, D. N., Rodriguez, M. H., and Arredondo-Jimenez, J. I. (1998). Selective and conventional house-spraying of DDT and bendiocarb against Anopheles pseudopunctipennis in southern Mexico. J. Am. Mosq. Control Assoc. 14, 410–420.
Casida, J. E., and Quistad, G. B. (1998). Golden age of insecticide research: past, present, or future? Annu. Rev. Entomol. 43, 1–16. doi: 10.1146/annurev.ento.43.1.1
Castello, J. A., and Gil Rivas, M. J. (1980). Proposal for a predator for the destruction of Triatoma infestans: Tarentola mauritanica. Medicina (B Aires) 40, 673–677.
Chagas, C. (1909). Nova tripanozomiaze humana: estudos sobre a morfolojia e o ciclo evolutivo do Schizotrypanum cruzi n. gen., n. sp., ajente etiolojico de nova entidade morbida do homem. Mem. Inst. Oswaldo Cruz 1, 159–218. doi: 10.1590/s0074-0276190900020008
Chan, Y. C., Chan, K. L., and Ho, B. C. (1971). Aedes aegypti (L.) and Aedes albopictus (Skuse) in Singapore city: distribution and density. Bull. World Health Organ. 44, 617–627.
Chandra, G., Bhattacharjee, I., Chatterjee, S. N., and Ghosh, A. (2008a). Mosquito control by larvivorous fish. Indian J. Med. Res. 127, 13–27.
Chandra, G., Mandal, S. K., Ghosh, A. K., Das, D., Banerjee, S. S., and Chakraborty, S. (2008b). Biocontrol of larval mosquitoes by Acilius sulcatus (Coleoptera: Dytiscidae). BMC Infect. Dis. 8:138. doi: 10.1186/1471-2334-8-138
Chapman, H. C. (1974). Biological control of mosquito larvae. Annu. Rev. Entomol. 19, 33–59. doi: 10.1146/annurev.en.19.010174.000341
Chapman, H. C., Woodard, D. B., and Petersen, J. J. (1967). Pathogens and parasites of Louisiana Culicidae and Chaoboridae. Proc. Annu. Meet. N. J. Mosq. Exterm. Assoc. 54, 54–60.
Charlwood, J. D., Dagoro, H., and Paru, R. (1985). Blood-feeding and resting behaviour in the Anopheles punctulatus Donitz complex (Diptera: Culicidae) from coastal Papua New Guinea. Bull. Entomol. Res. 75, 463–476. doi: 10.1017/S0007485300014577
Chatterjee, S. N., Ghosh, A., and Chandra, G. (2007). Eco-friendly control of mosquito larvae by Brachytron pratense nymph. J. Environ. Health 69, 44–48.
Chaumeau, V., Kajeechiwa, L., Kulabkeeree, T., Sawasdichai, S., Haohankhunnatham, W., Inta, A., et al. (2022). Outdoor residual spraying for malaria vector-control in Myanmar: a cluster randomized controlled trial. PLoS One 17:e0274320. doi: 10.1371/journal.pone.0274320
Chen, S.-M., Dumler, J. S., Bakken, J. S., and Walker, D. H. (1994). Identification of a granulocytotropic Ehrlichia species as the etiologic agent of human diseases. J. Clin. Microbiol. 32, 589–595. doi: 10.1128/jcm.32.3.589-595.1994
Cheong, W. H., Mak, J. W., Naidu, S., and Mahadevan, S. (1981). Armigeres subalbatus incriminated as an important vector of the dog heartworm Dirofilaria immitis and the bird Cardiofilaria in urban Kuala Lumpur. Southeast Asian J. Trop. Med. Public Health 12, 611–612.
Chevalier, V., Mondet, B., Diaite, A., Lancelot, R., Fall, A., and Ponçon, N. (2004). Exposure of sheep to mosquito bites: possible consequences for the transmission risk of Rift Valley fever in Senegal. Med. Vet. Entomol. 18, 247–255. doi: 10.1111/j.0269-283X.2004.00511.x
Chiang, G. L., Cheong, W. H., Samarawickrema, W. A., Mak, J. W., and Kan, S. K. (1984). Filariasis in Bengkoka peninsula, Sabah, Malaysia: vector studies in relation to the transmission of filariasis. Southeast Asian J. Trop. Med. Public Health 15, 179–189.
Chomel, B. B. (2009). “Zoonoses” in Encyclopedia of microbiology. ed. M. Schaechter. 3rd ed (Davis, CA: University of California, Elsevier Inc.), 820–829.
Chouin-Carneiro, T., Ant, T. H., Herd, C., Louis, F., Failloux, A. B., and Sinkins, S. P. (2019). Wolbachia strain wAlbA blocks Zika virus transmission in Aedes aegypti. Med. Vet. Entomol. 34, 116–119. doi: 10.1111/mve.12384
Clark, T. B., Kellen, W. R., Fukuda, T., and Lindegren, J. E. (1968). Field and laboratory studies on the pathogenicity of the fungus Beauveria bassiana to three genera of mosquitoes. J. Invertebr. Pathol. 11, 1–7. doi: 10.1016/0022-2011(68)90047-5
Clark, T. B., Kellen, W. R., Lindergren, J. E., and Sanders, R. D. (1966). Phytium sp. pathogenic to mosquito larvae. J. Invertebr. Pathol. 8, 351–354. doi: 10.1016/0022-2011(66)90049-8
Collins, L. E., and Blackwell, A. (2000). The biology of Toxorhynchites mosquitoes and their potential as biocontrol agents. Biocontrol News Information 21, 105–116.
Connal, A. (1921). Observations on filaria in Chrysops from West Africa. Trans. R. Soc. Trop. Med. Hyg. 14, 108–109. doi: 10.1016/S0035-9203(21)90176-7
Connor, M. E. (1922). Notes on the use of fresh water fish as consumers of mosquito larvae in containers used in the home. Am. J. Public Health (NY) 12, 193–194. doi: 10.2105/ajph.12.3.193
Cordaux, R., Michel-Salzat, A., and Bouchon, D. (2001). Wolbachia infection in crustaceans: novel hosts and potential routes for horizontal transmission. J. Evol. Biol. 14, 237–243. doi: 10.1046/j.1420-9101.2001.00279.x
Corliss, J. O. (1954). The literature on Tetrehymena: its history, growth, and recent trends. J. Protozool. 1, 156–169. doi: 10.1111/j.1550-7408.1954.tb00809.x
Corliss, J. O. (1960). Tetrahymena chironomini sp. nov., a ciliate from midge larvae and the current status of facultative parasitism in the genus Tetrahymena. Parasitology 50, 111–153. doi: 10.1017/s0031182000025245
Couper, L. I., Yang, Y. Y., Yang, F. X. F., and Swei, A. (2020). Comparative vector competence of north American Lyme disease vectors. Parasit. Vectors 13:29. doi: 10.1186/s13071-020-3893-x
Crainey, J. L., Wilson, M. D., and Post, R. J. (2010). Phylogenetically distinct Wolbachia gene and pseudogene sequences obtained from the African onchocerciasis vector Simulium squamosum. Int. J. Parasitol. 40, 569–578. doi: 10.1016/j.ijpara.2009.10.017
Cunha, M. S., Tubaki, R. M., de Menezes, R. M. T., Pereira, M., Caleiro, G. S., Coelho, E., et al. (2020). Possible non-sylvatic transmission of yellow fever between non-human primates in São Paulo city, Brazil, 2017-2018. Sci. Rep. 10:15751. doi: 10.1038/s41598-020-72794-x
Curtis, C. F. (1976). “Testing systems for the genetic control of mosquitoes,” in: XV International Congress of Entomology. 106–116.
Curtis, C. F., Brookes, G. D., Grover, K. K., Krishnamurthy, B. S., Laven, H., Rajagopalan, L., et al. (1982). A field trial on genetic control of Culex p. fatigans by release of integrated strain IS-31B. Entomol. Exp. Appl. 31, 181–190. doi: 10.1111/j.1570-7458.1982.tb03133.x
da Rocha, J. A., de Oliveira, S. B., Povoa, M. M., Moreira, L. A., and Krettli, A. U. (2008). Malaria vectors in areas of plasmodium falciparum epidemic transmission in the Amazon region, Brazil. Am. J. Trop. Med. Hyg. 78, 872–877. doi: 10.4269/ajtmh.2008.78.872
Dame, D. A., Woodward, D. B., Ford, H. R., and Weidhaas, D. E. (1964). Field behavior of sexually sterile Anopheles quadrimaculatus males. Mosq. News 24, 6–16.
Das, B. P., Sharma, S. N. L. K., Lal, S., and Saxena, V. K. (2005). First time detection of Japanese encephalitis virus antigen in dry and unpreserved mosquito Culex tritaeniorhynchus Giles, 1901, from Karnal district of Hayana state of India. J. Commun. Dis. 37, 131–133.
Davidson, G., Odetoyinbo, J. A., Colussa, B., and Coz, J. (1970). A field attempt to assess the mating competitiveness of sterile males produced by crossing two member species of the Anopheles gambiae complex. Bull. World Health Organ. 42, 55–67.
Davies, F. (1975). Observations on the epidemiology of Rift Valley fever in Kenya. J. Hyg. (Lond.) 75, 219–230. doi: 10.1017/s0022172400047252
Davies, T. G. E., Field, L. M., Usherwood, P. N. R., and Williamson, M. S. (2007). DDT, pyrethrins, pyrethroids and insect sodium channels. IUBMB Life 59, 151–162. doi: 10.1080/15216540701352042
Davies, F. G., and Highton, R. B. (1980). Possible vectors of Rift Valley fever in Kenya. Trans. R. Soc. Trop. Med. Hyg. 74, 815–816. doi: 10.1016/0035-9203(80)90213-8
Davis, E. L., Prada, J., Reimer, L. J., and Hollingsworth, T. D. (2021). Modelling the impact of vector control on lymphatic filariasis programs: current approaches and limitations. Clin. Infect. Dis. 72, S152–S157. doi: 10.1093/cid/ciab191
de Valdez, M. R. W., Nimmo, D., Betz, J., Gong, H., James, A. A., Alphey, L., et al. (2011). Genetic elimination of dengue vector mosquitoes. Proc. Natl. Acad. Sci. U. S. A. 108, 4772–4775. doi: 10.1073/pnas.1019295108
de Wispelaere, M., Despres, P., and Choumet, V. (2017). European Aedes albopictus and Culex pipiens are competent vectors for Japanese encephalitis virus. PLoS Negl. Trop. Dis. 11:e0005294. doi: 10.1371/journal.pntd.0005294
Demers, J., Bewick, S., Calabrese, J., and Fagan, W. F. (2018). Dynamic modelling of personal protection control strategies for vector-borne disease limits the role of diversity amplification. J. R. Soc. Interface 15:20180166. doi: 10.1098/rsif.2018.0166
Deredec, A., Godfray, H. C. J., and Burt, A. (2011). Requirements for effective malaria control with homing endonuclease genes. Proc. Natl. Acad. Sci. U. S. A. 108, E874–E880. doi: 10.1073/pnas.1110717108
Dias, J. C. (2007). Southern cone initiative for the elimination of domestic populations of Triatoma infestans and the interruption of transfusional Chagas disease: historical aspects, present situation, and perspectives. Mem. Inst. Oswaldo Cruz 102, 11–18. doi: 10.1590/S0074-02762007005000092
Dick, G. W. A. (1952). Zika virus. II. Pathogenicity and physical properties. Trans. R. Soc. Trop. Med. Hyg. 46, 521–534. doi: 10.1016/0035-9203(52)900436
Dinesh, D. S., Kumar, V., Kesari, S., and Das, P. (2014). Mites & spiders act as biological control agent to sand flies. Asian Pac. J. Trop. Dis. 4, S463–S466. doi: 10.1016/S2222-1808(14)60491-6
Dodson, B. L., Hughes, G. L., Paul, O., Matacchiero, A. C., Kramer, L. D., and Rasgon, J. L. (2014). Wolbachia enhances West Nile virus (WNV) infection in the mosquito Culex tarsalis. PLoS Negl. Trop. Dis. 8:e2965. doi: 10.1371/journal.pntd.0002965
Doherty, R. L., Carley, J. G., Mackerras, M. J., and Marks, E. N. (1963). Studies of arthropod-borne virus infections in Queensland. III. Isolation and characterization of virus strains from wild-caught mosquitoes in North Queensland. Aus. J. Exp. Biol. Med. Sci. 41, 17–39. doi: 10.1038/icb.1963.2
Dolan, G., ter Kuile, F. O., Jacoutot, V., White, N. J., Luxemburger, C., Malankirii, L., et al. (1993). Bed nets for the prevention of malaria and anaemia in pregnancy. Trans. R. Soc. Trop. Med. Hyg. 87, 620–626. doi: 10.1016/0035-9203(93)90262-o
Domrow, R., and Derrick, E. H. (1964). Ixodes holocyclus, the man-biting tick in S.E. Queensland. Aus. J. Sci. 27, 234–236.
Donald, C. L., Siriyasatien, P., and Kohl, A. (2020). Toxorhynchites species: a review of current knowledge. Insects 11:747. doi: 10.3390/insects11110747
Dong, Y., Simões, M. L., Marois, E., and Dimopoulos, G. (2018). CRISPR/Cas9-mediated gene knockout of Anopheles gambiae FREP1 suppresses malaria parasite infection. PLoS Pathog. 14:e1006898. doi: 10.1371/journal.ppat.1006898
Donnelly, J., and Peirce, M. A. (1975). Experiments on the transmission of Babesia divergens to cattle by the tick Ixodes ricinus. Int. J. Parasitol. 5, 363–367. doi: 10.1016/0020-7519(75)90085-5
Dorta, D. M., Vasuki, V., and Rajavel, A. (1993). Evaluation of organophosphorus and synthetic pyrethroid insecticides against six vector mosquito species. Rev. Saúde Públ. 27, 391–397. doi: 10.1590/s0034-89101993000600001
Durden, L. A. (2019). “Lice (Phthiraptera)” in Medical and veterinary entomology. eds. G. R. Mullen and L. A. Durden (GA, United States: Academic Press), 79–106.
Dutra, H. L. C., Rocha, M. N., Dias, F. B. S., Mansur, S. B., Caragata, E. P., and Moreira, L. A. (2016). Wolbachia blocks currently circulating Zika virus isolates in Brazillian Aedes aegypti mosquitoes. Cell Host Microbe 19, 771–774. doi: 10.1016/j.chom.2016.04.021
Economidis, P. S. (1995). Endangered freshwater fishes of Greece. Biol. Conserv. 72, 201–211. doi: 10.1016/0006-3207(94)00083-3
Economidis, P. S., Dimitriou, E., Pagoni, R., Michaloudi, E., and Natsis, L. (2000). Introduced and translocated fish species in the inland waters of Greece. Fish. Manag. Ecol. 7, 239–250. doi: 10.1046/j.1365-2400.2000.00197.x
Edeson, J. F. B., and Wilson, T. (1964). The epidemiology of filariasis due to Wuchereria bancrofti and Brugia malayi. Annu. Rev. Entomol. 9, 245–268. doi: 10.1146/annurev.en.09.010164.001333
Emmons, R. W. (1988). “Reoviridae: the Orbiviruses (Colorado tick fever)” in Laboratory diagnosis of infectious diseases principle and practice. eds. K. Araki, A. Galloway, and K. Taguchi (New York: Springer New York), 375–383.
Fairhurst, R. M., and Dondorp, A. M. (2016). Artemisinin-resistant Plasmodium falciparum malaria. Microbiol. Spectr. 4, 409–429. doi: 10.1128/microbiolspec.EI10-0013-2016
Fatih, F. A., Staines, H. M., Siner, A., Ahmed, M. A., Woon, L. C., Pasini, E. M., et al. (2013). Susceptibility of human plasmodium knowlesi infections to anti-malarials. Malar. J. 12:425. doi: 10.1186/1475-2875-12-425
Fayolle, S., Bertrand, C., Logez, M., and Franquet, É. (2015). Does mosquito control by Bti spraying affect the phytoplankton community? A 5-year study in Camargue temporary wetlands (France). Ann. Limnol. – Int. J. Limnol. 51, 189–198. doi: 10.1051/limn/2015013
Fenner, F. (1946). The epidemiology of North Queensland tick typhus: natural mammalian hosts. Med. J. Aus. 2, 666–668. doi: 10.5694/j.1326-5377.1946.tb34592.x
Ferroni, E., Jefferson, T., and Gachelin, G. (2012). Angello celli and research on the prevention of malaria in Italy a century ago. J. R. Soc. Med. 105, 35–40. doi: 10.1258/jrsm.2011.11k049
Fischer, P., Bamuhiiga, J., and Büttner, D. W. (1997). Occurrence and diagnosis of Mansonella streptocerca in Uganda. Acta Trop. 63, 43–55. doi: 10.1016/s0001-706x(96)00607-9
Fischhoff, I. R., Burtis, J. C., Keesing, F., and Ostfeld, R. S. (2018). Tritophic interactions between a fungal pathogen, a spider predator, and the blacklegged tick. Ecol. Evol. 8, 7824–7834. doi: 10.1002/ece3.4271
Fletcher, M., Teklehaimanot, A., and Yemane, G. (1992). Control of mosquito larvae in the port city of Assam by an indigenous larvivorous fish, Aphanius dispar. Acta Trop. 52, 155–166. doi: 10.1016/0001-706x(92)90032-s
Floridis, J., McGuinness, S. L., Kurucz, N., Burrow, J. N., Baird, R., and Francis, J. R. (2018). Murray Valley encephalitis virus: an ongoing cause of encephalitis in Australia's north. Trop. Med. Infect. Dis. 3:49. doi: 10.3390/tropicalmed3020049
Florio, L., and Miller, M. S. (1948). Epidemiology of Colorado tick fever. Am. J. Public Health Nations Health 38, 211–213. doi: 10.2105/ajph.38.2.211
Florio, L., Miller, M. S., and Mugrage, E. R. (1950). Colorado tick fever: isolation of the virus from Dermacentor andersoni in nature and laboratory study of the transmission of the virus in the tick. J. Immunol. 64, 257–263. doi: 10.4049/jimmunol.64.4.257
Florio, L., Stewart, M. O., and Mugrage, E. R. (1944). The experimental transmission of Colorado tick fever. J. Exp. Med. 80, 165–188. doi: 10.1084/jem.80.3.165
Focks, D. A., Sackett, S. R., Dame, D. A., and Bailey, D. L. (1983). Toxorhynchites rutilus rutilus (Diptera: Culicidae): field studies on dispersal and oviposition in the context of the biocontrol of urban container-breeding mosquitoes. J. Med. Entomol. 20, 383–390. doi: 10.1093/jmedent/20.4.383
Focks, D. A., Sackett, S. R., Dame, D. A., and Bailey, D. L. (1985). Effect of weekly releases of Toxorhynchites amboinensis (Doleschall) on Aedes aegypti (L.) (Diptera: Culicidae) in New Orleans, Louisiana. J. Econ. Entomol. 78, 622–626. doi: 10.1093/jee/78.3.622
Franz, G. (2002). Recombination between homologous autosomes in medfly (Ceratitis capitata) males: type-1 recombination and the implications for the stability of genetic sexing strains. Genetica 116, 73–84. doi: 10.1023/a:1020911725724
Franz, G. (2005). “Genetic sexing strains in Mediterranean fruit fly, an example for other species amenable to large-scale rearing for the sterile insect technique” in Sterile insect technique: Principles and practice in area-wide integrated pest management. eds. V. A. Dyck, J. Hendrichs, and A. S. Robinson (The Netherlands: Springer)
Fu, G., Lees, R. S., Nimmo, D., Aw, D., Jin, L., Gray, P., et al. (2010). Female-specific flightless phenotype for mosquito control. Proc. Natl. Acad. Sci. U. S. A. 107, 4550–4554. doi: 10.1073/pnas.1000251107
Gabiane, G., Yen, P., and Failoux, A. (2022). Aedes mosquitoes in the emerging threat of urban yellow fever transmission. Rev. Med. Virol. 32:e2333. doi: 10.1002/rmv.2333
Ganguly, S., and Mukhopadhayay, S. K. (2008). Tick-borne ehrlichiosis infection in human beings. J. Vector Borne Dis. 45, 273–280.
Gerberich, J. B., and Laird, M. (1985). “Larvivorous fish in the biocontrol of mosquitoes, with a selected bibliography of recent literature” in Integrated mosquito control methodologies. eds. M. Laird and J. W. Miles, vol. 2. Biocontrol and other innovative components and future directions, (London: Academic Press), 47–76.
Ghebreyesus, T. A., Haile, M., Witten, K. H., Getachew, A., Yohannes, M., Lindsay, S. W., et al. (2000). Household risk factors for malaria among children in the Ethiopian highlands. Trans. R. Soc. Trop. Med. Hyg. 94, 17–21. doi: 10.1016/s0035-9203(00)90424-3
Gillette, B. (1988). Controlling mosquitoes biologically. Bioscience 38, 80–83. doi: 10.2307/1310610
Ginsberg, H. S., Lebrun, R. A., Heyer, K., and Zhioua, E. (2002). Potential nontarget effects of Metarhizium anisopliae (Deuteromycetes) used for biological control of ticks (Acari: Ixodidae). Environ. Entomol. 31, 1191–1196. doi: 10.1603/0046-225X-31.6.1191
Glaser, L. C. (1999) in "organophosphates and carbamate pesticides," in Field manual of wildlife diseases: general field procedures and diseases of birds. eds. M. Friend and J. C. Franson (Reston, VA: US Geological Survey), 287–294.
Goodman, A. L., Lockhart, S. R., Lysen, C. B., Westblade, L. F., Burnham, C. A., and Burd, E. M. (2018). Two cases of fungal keratitis caused by Metarhizium anisopliae. Med. Mycol. Case Rep. 21, 8–11. doi: 10.1016/j.mmcr.2018.03.003
Grace, D., Mutua, F., Ochungo, P., Kruska, R., Jones, K., Brierley, L., et al. (2012). “Mapping of poverty and likely zoonoses hotspots” in Zoonoses project 4. Report to Department for International Development (Nairobi, Kenya: ILRI).
Graham, D. H. (1939). Mosquito life in the Auckland district. Report of Auckland mosquito research committee on an investigation made by David H. Graham. Trans. R. Soc. N. Z 69, 210–244.
Gray, J. S., von Stedingk, L.-V., Gurtelschmid, M., and Granstrom, M. (2002). Transmission studies on Babesia microti in Ixodes ricinus ticks and gerbils. J. Clin. Microbiol. 40, 1259–1263. doi: 10.1128/JCM.40.4.1259-1263.2002
Griffin, L. F., and Knight, J. M. (2012). A review of the role of fish as biological control agents of disease vector mosquitoes in mangrove forests: reducing human health risks while reducing environmental risk. Wetl. Ecol. Manag. 20, 243–252. doi: 10.1007/s11273-012-9248-4
Grover, K. K., Suguna, S. G., Uppal, D. K., Singh, K. R. P., Ansari, M. A., Curtis, C. F., et al. (1976). Field experiments on the competitiveness of males of three genetic control systems of Aedes aegypti. Entomol. Exp. Appl. 20, 8–18. doi: 10.1111/j.1570-7458.1976.tb02611.x
Gu, J.-B., Dong, Y.-Q., Peng, H.-J., and Chen, X.-G. (2010). A recombinant AeDNA containing the insect-specific toxin, BmK IT1, displayed an increasing pathogenicity on Aedes albopictus. Am. J. Trop. Med. Hyg. 83, 614–623. doi: 10.4269/ajtmh.2010.10-0074
Gubler, D. J. (1988). “Dengue” in Epidemiology of arthropod-borne viral diseases. ed. T. P. Monath (Boca Raton: CRC Press Inc.), 223–260.
Gubler, D. J., Kuno, G., and Markoff, L. (2007). “Flaviviruses” in Fields Virology. eds. D. M. Knipe and P. M. Howley. 5th ed (Philadelphia: Lippincott, Williams and Wilkins), 1153–1252.
Guerbouj, S., Chemkhi, J., and Kaabi, B. (2007). Natural infection of Phlebotomus (Larroussius) langeroni (Diptera: Psychodidae) with Leishmania infantum in Tunisia. Trans. R. Soc. Trop. Med. Hyg. 101, 372–377. doi: 10.1016/j.trstmh.2006.07.007
Gupta, D., Bhattacharjee, O., Mandal, D., Sen, M. K., Dey, D., Dasgupta, A., et al. (2019). CRISPR-Cas9 system: a new-fangled dawn in gene editing. Life Sci. 232:116636. doi: 10.1016/j.lfs.2019.116636
Gurycová, D. (1998). First isolation of Francisella tularensis subsp. tularensis in Europe. Eur. J. Epidemiol. 14, 797–802. doi: 10.1023/a:1007537405242
Haddow, A. J. (1969). The natural history of yellow fever in Africa. Proc. R. Soc. Edinb. B. Biol. Sci. 70, 191–227. doi: 10.1017/S0080455X00001338
Hadjinicolaou, J., and Betzios, B. (1973). Gambusia fish as a means of mosquito control of anopheles sacharovi in Greece. Geneva, Switzerland: World Health Organization.
Hadole, P., and Vankhede, G. (2013). Spiders for eradication of vector-borne diseases. Indian Soc. Arachnol. 1, 59–62.
Hall, R. A., Broom, A. K., Smith, D. W., and Mackenzie, J. S. (2002). “The ecology and epidemiology of Kunjin virus” in Japanese encephalitis and West Nile viruses. eds. J. S. Mackenzie, A. D. T. Barrett, and V. Deubel (Berlin, Heidelberg: Springer), 253–269.
Hall, R. A., Scherret, J. H., and Mackenzie, J. S. (2006). Kunjin virus: an Australian variant of West Nile? Ann. N. Y. Acad. Sci. 951, 153–160. doi: 10.1111/j.1749-6632.2001.tb02693
Hancock, R. G., Boyd, T., MacFadden, S., Sowders, A., Foster, W. A., and Lounibos, L. P. (2022). Mosquitoes eating mosquitoes: how Toxorhynchites amboinensis, Psorophora ciliata, and Sabethes cyaneus (Diptera: Culicidae) capture prey? Ann. Entomol. Soc. Am. 115, 461–471. doi: 10.1093/aesa/saac017
Harbach, R. E. (2007). The Culicidae (Diptera): a review of taxonomy, classification and phylogeny. Zootaxa 1668, 591–638. doi: 10.11646/zootaxa.1668.1.28
Hargrove, J. W. (2003). Tsetse eradication: Sufficiency, necessity and desirability. University of Edinburgh, UK: DFID Animal Health Programme, Centre for Tropical Veterinary Medicine.
Harris, A. F., McKemey, A. R., Nimmo, D., Curtis, Z., Black, I., Morgan, S. A., et al. (2012). Successful suppression of a field mosquito population by sustained release of engineered male mosquitoes. Nat. Biotechnol. 30, 828–830. doi: 10.1038/nbt.2350
Harris, A. F., Nimmo, D., McKemey, A. R., Kelly, N., Scaife, S., Donnelly, C. A., et al. (2011). Field performace of engineered male mosquitoes. Nat. Biotechnol. 29, 1034–1037. doi: 10.1038/nbt.2019
Hassan, S. M., Dipeolu, O. O., Amoo, A. O., and Odhiambo, T. R. (1991). Predation on livestock ticks by chickens. Vet. Parasitol. 38, 199–204. doi: 10.1016/0304-4017(91)90129-j
Hassanain, M. A., Garhy, M. F. E., Abdel-Ghaffar, F. A., El-Sharaby, A., and Abdel Megeed, K. N. (1997). Biological control studies of soft and hard ticks in Egypt. Parasitol. Res. 83, 209–213. doi: 10.1007/s004360050235
Headrick, D. R. (2014). Sleeping sickness epidemics and colonial responses in east and Central Africa, 1900-1940. PLoS Negl. Trop. Dis. 8:e2772-e. doi: 10.1371/journal.pntd.0002772
Hedges, L. M., Brownlie, J. C., O'Neill, S. L., and Johnson, K. N. (2008). Wolbachia and virus protection in insects. Science 322:702. doi: 10.1126/science.1162418
Hendy, A., Krüger, A., Pfarr, K., De Witte, J., Kibweja, A., Mwingira, U., et al. (2018). The blackfly vectors and transmission of Onchocerca volvulus in Mahenge, south eastern Tanzania. Acta Trop. 181, 50–59. doi: 10.1016/j.actatropica.2018.01.009
Henke, M. O., de Hoog, G. S., Gross, U., Zimmermann, G., Kraemer, D., and Weig, M. (2002). Human deep tissue infection with an entomopathogenic Beauveria species. J. Clin. Microbiol. 40, 2698–2702. doi: 10.1128/JCM.40.7.2698-2702.2002
Higa, Y. (2011). Dengue vectors and their spatial distribution. Trop. Med. Health 39, 17–27. doi: 10.2149/tmh.2011-S04
Hii, J. L. K., Kan, S., Foh, C. K., and Chan, M. K. C. (1984). Anopheles (Cellia) balabacensis Baisas is a vector of Wuchereria bancrofti in Sabah, Malaysia. Trans. R. Soc. Trop. Med. Hyg. 78, 281–282. doi: 10.1016/0035-9203(84)90302-x
Hill, E. F. (1995). “Organophosphorus and carbamate pesticides” in Handbook of ecotoxicology. eds. D. H. Hoffman, B. A. Rattner, G. A. J. Burton, and J. J. Cairns (Boca Raton, Florida: Lewis Publishers), 243–274.
Hoermann, A., Habtewold, T., Selvaraj, P., Del Corsano, G., Capriotti, P., Inghilterra, M. G., et al. (2022). Gene drive mosquitoes can aid malaria elimination by retarding plasmodium sporogonic development. Sci. Adv. 8:eabo1733. doi: 10.1126/sciadv.abo1733
Hoffman, A., and Turelli, M. (1997). “Cytoplasmic incompatibility in insects” in Influential passengers. Inherited microorganisms and arthropod reproduction. eds. S. L. O'Neill, A. Hoffman, and J. H. Werren (Oxford: Oxford University Press), 42–80.
Hoffmann, A. A., Montgomery, B. L., Popovici, J., Iturbe-Ormaetxe, I., Johnson, P. H., Muzzi, F., et al. (2011). Successful establishment of Wolbachia in Aedes populations to suppress dengue transmission. Nature 476, 454–457. doi: 10.1038/nature10356
Hotta, S. (1998). Dengue vector mosquitoes in Japan: the role of Aedes albopictus and Aedes aegypti in the 1942-1944 dengue epidemics of Japanese main islands. Med. Entomol. Zool. 49, 267–274. doi: 10.7601/mez.49.267
Howard, A. F. V., Zhou, G., and Omlin, F. X. (2007). Malaria mosquito control using edible fish in western Kenya: preliminary findings of a controlled study. BMC Public Health 7:199. doi: 10.1186/1471-2458-7-199
Huang, Y.-J. S., Higgs, S., and Vanlandingham, D. L. (2017). Biological control strategies for mosquito vectors of arboviruses. Insects 8:21. doi: 10.3390/insects8010021
Isenhart, F. R., and DeSante, D. F. (1985). Observations of scrub jays cleaning ectoparasites from black-tailed deer. Condor 87, 145–147. doi: 10.2307/1367147
Ismail, I., Notananda, V., and Schepens, J., Organization, W.H (1975). Studies on malaria and responses of Anopheles balabacensis balabacensis and Anopheles minimus to DDT residual spraying in Thailand. Acta Trop. 32, 206–231.
Iturbe-Ormaetxe, I., Walker, E. C., and O'Neill, S. L. (2011). Wolbachia and the biological control of mosquito-borne diseases. EMBO Rep. 12, 508–518. doi: 10.1038/embor.2011.84
Jackson, C. H. N. (1941). The analysis of a tsetse fly population. Ann. Eugenics 10, 332–369. doi: 10.1111/j.1469-1809.1940.tb02259.x
Jackson, C. H. N. (1943). The analysis of a tsetse fly population II. Ann. Eugenics 12, 176–205. doi: 10.1111/j.1469-1809.1943.tb02322.x
Jackson, C. H. N. (1948). The analysis of a tsetse fly population III. Ann. Eugenics 14, 91–108. doi: 10.1111/j.1469-1809.1947.tb02381.x
Jaipieam, S., Visuthismajarn, P., Sutheravut, P., Siriwong, W., Thoumsang, S., Borjan, M., et al. (2009). Organophosphate pesticide residues in drinking water from artesian wells and health risk assessment of agricultural communities, Thailand. Hum. Ecol. Risk. Assess. 15, 1304–1316. doi: 10.1080/10807030903306984
Jeffries, C. L., and Walker, T. (2016). Wolbachia biocontrol strategies for arboviral diseases and the potential influences of resident Wolbachia strains in mosquitoes. Curr. Trop. Med. Rep. 3, 20–25. doi: 10.1007/s40475-016-0066-2
Jehle, J. A., Blissard, G. W., Bonning, B. C., Cory, J. S., Herniou, E. A., Rohrmann, G. F., et al. (2006). On the classification and nomenclature of baculoviruses: a proposal for revision. Arch. Virol. 151, 1257–1266. doi: 10.1007/s00705-006-0763-6
Jeyaprakasam, N. K., Liew, J. W. K., Low, V. L., Wan Sulaiman, W. Y., and Vythilingam, I. (2020). Plasmodium knowlesi infecting humans in Southeast Asia: What's next? PLoS Negl. Trop. Dis. 14:e0008900. doi: 10.1371/journal.pntd.0008900
Jiram, A. I., Vythilingam, I., NoorAzian, Y. M., Yusof, Y. M., Azahari, A. H., and Fong, M.-Y. (2012). Entomologic investigation of plasmodium knowlesi vectors in Kuala Lipis, Pahang, Malaysia. Malar. J. 11, 213–222. doi: 10.1186/1475-2875-11-213
Johnson, B. K. (1988). “O'nyong nyong virus disease” in The arboviruses: epidemiology and ecology. ed. T. P. Monath, vol. III (Florida: CRC Press), 217–223.
Johnson, R. M., and Rasgon, J. L. (2018). Densonucleosis viruses ('densoviruses') for mosquito and pathogen control. Curr. Opin. Insect Sci. 28, 90–97. doi: 10.1016/j.cois.2018.05.009
Jones, K. E., Patel, N. G., Levy, M. A., Storeygard, A., Balk, D., Gittleman, J. L., et al. (2008). Global trends in emerging infectious diseases. Nature 451, 990–993. doi: 10.1038/nature06536
Juliano, S. A., and Lawton, J. H. (1990). The relationship between competition and morphology II: experiments on co-occurring Dytiscid beetles. J. Anim. Ecol. 59, 831–848. doi: 10.2307/5017
Jurberg, J., and Galvão, C. (2006). “Biology, ecology, and systematics of Triatominae (Heteroptera, Reduviidae), vectors of Chagas disease, and implications for human health” in Hug the bug, for love of true bugs. ed. W. Rabitsch (Austria: OÖ Landes-Kultur GmbH), 1096–1116.
Kageyama, D., Nishimura, G., Hoshizaki, S., and Ishikawa, Y. (2002). Feminizing Wolbachia in an insect, Ostrinia furnacalis (Lepidoptera: Crambidae). Heredity (Edinb.) 88, 444–449. doi: 10.1038/sj.hdy.6800077
Kamareddine, L. (2012). The biological control of the malaria vector. Toxins(Basel) 4, 748–767. doi: 10.3390/toxins4090748
Kambris, Z., Cook, P. E., Phuc, H. K., and Sinkins, S. P. (2009). Immune activation by life-shortening Wolbachia and reduced filarial competence in mosquitoes. Science 326, 134–136. doi: 10.1126/science.1177531
Kanjanopas, K., Choochote, W., Jitpakdi, A., Suvannadabba, S., Loymak, S., Chungpivat, S., et al. (2001). Brugia malayi in a naturally infected cat from Narathiwat province, southern Thailand. Southeast Asian J. Trop. Med. Public Health 32, 585–587.
Karesh, W. B., Dobson, A., Lloyd-Smith, J. O., Lubroth, J., Dixon, M. A., Bennett, M., et al. (2012). Ecology of zoonoses: natural and unnatural histories. Lancet 380, 1936–1945. doi: 10.1016/S0140-6736(12)61678-X
Katargina, O., Geller, J., Alekseev, A., Dubinina, H., Efremova, G., Mishaeva, N., et al. (2012). Identification of Anaplasma phagocytophilum in tick populations in Estonia, the European part of Russia and Belarus. Clin. Microbiol. Infect. 18, 40–46. doi: 10.1111/j.1469-0691.2010.03457.x
Kaushal, J., Khatri, M., and Arya, S. K. (2021). A treatise on organophosphate pesticide pollution: current strategies and advancements in their environmental degradation and elimination. Ecotoxicol. Environ. Saf. 207:111483. doi: 10.1016/j.ecoenv.2020.111483
Kay, B. H., Fanning, I. D., and Carley, J. G. (1984). The vector competence of Australian Culex annulirostris with Murray Valley encephalitis and Kunjin viruses. Aus. J. Exp. Biol. Med. Sci. 62, 641–650. doi: 10.1038/icb.1984.61
Kay, B. H., Lyons, S. A., Holt, J. S., Holynska, M., and Russell, B. M. (2002). Point source inoculation of Mesocyclops (Copepoda: Cyclopidae) gives widespread control of Ochlerotatus and Aedes (Diptera: Culicidae) immatures in service manholes and pits in North Queensland, Australia. J. Med. Entomol. 39, 469–474. doi: 10.1603/0022-2585-39.3.469
Kelly-Hope, L., Paulo, R., Thomas, B., Brito, M., Unnasch, T. R., and Molyneux, D. (2017). Loa loa vectors Chrysops spp.: perspectives on research, distribution, bionomics, and implications for elimination of lymphatic filariasis and onchocerciasis. Parasit. Vectors 10:172. doi: 10.1186/s13071-017-2103-y
Kementerian Kesihatan Malaysia (2022). Kenyataan media Ketua Pengarah Kesihatan-situasi semasa demam denggi di Malaysia Minggu Epidemiologi 49/2022 (4-10 Disember 2022). Available at: https://www. moh.gov.my/index.php/database_stores/store_view_page/17/2244 (Accessed December 14, 2022).
Kendie, F. A. (2020). Potential biological control agents against mosquito vector in the case of larvae stage: a review. World News Nat. Sci. 28, 34–50.
Killeen, G. F. (2014). Characterizing, controlling and eliminating residual malaria transmission. Malar. J. 13:330. doi: 10.1186/1475-2875-13-330
Kim, K. C., and Merritt, R. W. (1987). Black flies: Ecology, population management, and annonated world list. Pennsylvania, USA: The Pennsylvania State University.
Kittayapong, P., Baisley, K. J., Baimai, V., and O’Neill, S. L. (2000). Distribution and diversity of Wolbachia infections in southeast Asian mosquitoes (Diptera: Culicidae). J. Med. Entomol. 37, 340–345. doi: 10.1093/jmedent/37.3.340
Kleine, F. K. (1915). Die Übertragung von Filarien durch Chrysops. Z. Hyg. Infekt. 80, 345–349. doi: 10.1007/BF02174495
Kleinschmidt, I., Bradley, J., Knox, T. B., Mnzava, A. P., Kafy, H. T., Mbogo, C. M., et al. (2018). Implications of insecticide resistance for malaria vector control with long-lasting insecticidal nets: a WHO-coordinated, prospective, international, observational cohort study. Lancet Infect. Dis. 18, 640–649. doi: 10.1016/S1473-3099(18)30172-5
Kohls, G. M. (1947). Vectors of Rickettsial diseases. Ann. Intern. Med. 26:713. doi: 10.7326/0003-4819-26-5-713
Koskinioti, P., Augustinos, A. A., Carvalho, D. O., Misbah-ul-Haq, M., Pillwax, G., de la Fuente, L. D., et al. (2021). Genetic sexing strains for the population suppression of the mosquito vector Aedes aegypti. Philos. Trans. R. Soc. B. Biol. Sci. 376:20190808. doi: 10.1098/rstb.2019.0808
Kozuch, O., and Nosek, J. (1971). Transmission of tick-borne encephalitis (TBE) virus by Dermacentor marginatus and D. reticulatus ticks. Acta Virol. 15:334.
Kugeler, K. J., Mead, P. S., Janusz, A. M., Staples, J. E., Kubota, K. A., Chalcraft, L. G., et al. (2009). Molecular epidemiology of Francisella tularensis in the United States. Clin. Infect. Dis. 48, 863–870. doi: 10.1086/597261
Kulkarni, M. A., Berrang-Ford, L., Buck, P. A., Drebot, M. A., Lindsay, L. R., and Ogden, N. H. (2015). Major emerging vector-borne zoonotic diseases of public health importance in Canada. Emerg. Microbes Infect. 4:e33, 1–7. doi: 10.1038/emi.2015.33
Kumar, R., and Hwang, J.-S. (2006). Larvicidal efficiency of aquatic predators: a perspective for mosquito biocontrol. Zool. Stud. 45, 447–466.
Kumar, N. P., Sabesan, S., Krishnamoorthy, K., and Jambulingam, P. (2012). Detection of Chikungunya virus in wild populations of Aedes albopictus in Kerala state, India. Vector Borne Zoonotic Dis. 12, 907–911. doi: 10.1089/vbz.2012.0969
Kumar, A., Sharma, V. P., Sumodan, P. K., and Thavaselvam, D. (1998). Field trials of biolarvicide bacillus thuringiensis var. israelensis strain 164 and the larvivorous fish Aplocheilus blocki against Anopheles stephensi for malaria control in Goa, India. J. Am. Mosq. Control Assoc. 14, 457–462.
Kurucz, N., Whelan, P. I., Jacups, S. P., Broom, A. K., and Melville, L. F. (2005). Rainfall, mosquito vector numbers and seroconversions in sentinel chickens to Murray Valley encephalitis virus in the Northern Territory. Arbovirus Res. Aus. 9, 188–192.
Kushwaha, A. K., Scorza, B. M., Singh, O. P., Rowton, E., Lawyer, P., Sundar, S., et al. (2022). Domestic mammals as reservoirs for Leishmania donovani on the Indian subcontinent: possibility and consequences on elimination. Transbound. Emerg. Dis. 69, 268–277. doi: 10.1111/tbed.14061
Kwenti, T. E. (2017). “Biological control of parasites” in Natural remedies in the fight against parasites. eds. H. Khater, M. Govindarajan, and G. Benelli (London: IntechOpen)
Labuda, M., and Randolph, S. E. (1999). Survival strategy of tick-borne encephalitis virus: cellular basis and environmental determinants. Zent. bl. Bakteriol. 289, 513–524. doi: 10.1016/s09348840(99)80005-x
Lacey, L. A. (2007). Bacillus thuringiensis serovariety israelensis and Bacillus sphaericus for mosquito control. J. Am. Mosq. Control Assoc. 23, 133–163.
Lacey, L. A., and Inman, A. (1985). Efficacy of granular formulations of bacillus thuringiensis (H-14) for the control of anopheles larvae in rice fields. J. Am. Mosq. Control Assoc. 1, 38–42.
Laine, M., Luukainen, R., and Toivanen, A. (2004). Sindbis virus and other alphaviruses as a cause of human arthritic disease. J. Intern. Med. 256, 457–471. doi: 10.1111/j.1365-2796.2004.01413.x
Lambrechts, L., Scott, T. W., and Gubler, D. J. (2010). Consequences of the expanding global distribution of Aedes albopictus for dengue virus transmission. PLoS Negl. Trop. Dis. 4:e646. doi: 10.1371/journal.pntd.0000646
Lantova, L., Svobodova, M., and Volf, P. (2011). Effects of Psychodiella sergenti (Apicomplexa, Eugregarinorida) on its natural host Phlebotomus sergenti (Diptera, Psychodidae). J. Med. Entomol. 48, 985–990. doi: 10.1603/me11018
Lantova, L., and Volf, P. (2012). The development of Psychodiella sergenti (Apicomplexa: Eugregarinorida) in Phlebotomus sergenti (Diptera: Psychodidae). Parasitology 139, 726–734. doi: 10.1017/S0031182011002411
Lantova, L., and Volf, P. (2014). Mosquito and sand fly gregarines of the genus Ascogregarina and Psychodiella (Apicomplexa: Eugregarinorida, Aseptatorina) – overview of their taxonomy, life cycle, host specificity, and pathogenicity. Infect. Genet. Evol. 28, 616–627. doi: 10.1016/j.meegid.2014.04.021
Lara Oya, A., Medialdea Hurtado, M. E., Rojo Martín, M. D., Pérez, A. A., Alastruey-Izquierdo, A., Casas, C. M., et al. (2016). Fungal keratitis due to Beauveria bassiana in a contact lenses wearer and review of published reports. Mycopathologia 181, 745–752. doi: 10.1007/s11046-016-0027-2
Lardeux, F. (1992). Biological control of Culicidae with the copepod Mesocyclops asperconis and larvivorous fish (Poeciliidae) in a village of French Polynesia. Med. Vet. Entomol. 6, 9–15. doi: 10.1111/j.1365-2915.1992.tb00028.x
Lardeux, F., Riviere, F., Sechan, Y., and Kay, B. H. (1992). Release of Mesocyclops aspericornis (Copepoda) for control of larval Aedes polynesiensis (Diptera: Culicidae) in land crab burrows on an atoll of French Polynesia. J. Med. Entomol. 29, 571–576. doi: 10.1093/jmedent/29.4.571
Lau, Y. L., Lee, W. C., Chen, J. H., Zhong, Z., Jian, J. B., Amir, A., et al. (2016). Draft genomes of Anopheles cracens and Anopheles maculatus: comparison of simian malaria and human malaria vectors in peninsular Malaysia. PLoS One 11:e0157893. doi: 10.1371/journal.pone.0157893
Lau, Y. L., Lee, W. C., Xia, J., Zhang, G., Razali, R., Anwar, A., et al. (2015). Draft genome of Brugia pahangi: high similarity between B. pahangi and B. malayi. Parasit. Vectors 8:451. doi: 10.1186/s13071-015-1064-2
Laven, H. (1967). Eradication of Culex pipiens fatigans through cytoplasmic incompatibility. Nature 216, 383–384. doi: 10.1038/216383a0
Lawrence, P. O. (2004). “Simulium spp., vectors of Onchocerca volvulus: life cycle and control” in Encyclopedia of entomology. ed. J. L. Capinera, vol. 2010 (Dordrecht: Springer)
Le Prince, J. A., and Orenstein, A. J. (1916). Mosquito control in Panama: the eradication of malaria and yellow fever in Cuba and Panama. New York/London: Putnam.
Lee, W. C., Cheong, F. W., Amir, A., Lai, M. Y., Tan, J. H., Phang, W. K., et al. (2022). Plasmodium knowlesi: the game changer for malaria eradication. Malar. J. 21:140. doi: 10.1186/s12936-022-04131-8
Lee, W. C., Chin, P. W., Lau, Y. L., Chin, L. C., Fong, M. Y., Yap, C. J., et al. (2013). Hyperparasitaemic human plasmodium knowlesi infection with atypical morphology in peninsular Malaysia. Malar. J. 12:88. doi: 10.1186/1475-2875-12-88
Lee, W. C., Russell, B., Lee, B., Chu, C. S., Phyo, A. P., Sriprawat, K., et al. (2021). Plasmodium falciparum rosetting protects schizonts against artemisinin. EBioMedicine 73:103680. doi: 10.1016/j.ebiom.2021.103680
Lerdthusnee, K., Khuntirat, B., Leepitakrat, W., Tanskul, P., Monkanna, T., Khlaimanee, N., et al. (2003). Scrub typhus: vector competence of Leptotrombidium chiangraiensis chiggers and transmission efficacy and isolation of Orientia tsutsugamushi. Ann. N. Y. Acad. Sci. 990, 25–35. doi: 10.1111/j.1749-6632-2003.tb07333.x
Leung, S., Windbichler, N., Wenger, E. A., Bever, C. A., and Selvaraj, P. (2022). Population replacement gene drive characteristics for malaria elimination in a range of seasonal transmission settings: a modelling study. Malar. J. 21:226. doi: 10.1186/s12936-022-04242-2
Levy, R., and Miller, T. W. Jr. (1977). Experimental release of Romanomermis culicivorax (Mermithidae: Nematoda) to control mosquitoes breeding in Southwest Florida. Mosq. News 37, 483–486.
Lewis, D., and Young, E. R. (1980). The transmission of a human strain of Babesia divergens by Ixodes ricinus ticks. J. Parasitol. 66, 359–360. doi: 10.2307/3280841
Liew, J. W. K., Mohd Bukhari, F. D., Jeyaprakasam, N. K., Phang, W. K., Vythilingam, I., and Lau, Y. L. (2021). Natural plasmodium inui infections in humans and Anopheles cracens mosquito, Malaysia. Emerg. Infect. Dis. 27, 2700–2703. doi: 10.3201/eid2710.210412
Lindsay, S. W., Emerson, P. M., and Charlwood, J. D. (2002). Reducing malaria by mosquito-proofing houses. Trends Parasitol. 18, 510–514. doi: 10.1016/s1471-4922(02)02382-6
Lo Re, V. R., Occi, J. L., and MacGregor, R. R. (2004). Identifying the vector of Lyme disease. Am. Fam. Physician 69, 1935–1937.
Lockhart, J. M., Davidson, W. R., Stallknecht, D. E., Dawson, J. E., and Little, S. E. (1997). Natural history of Ehrlichia chafeensis (Rickettsiales: Ehrlichieae) in the piedmont physiographic province of Georgia. J. Parasitol. 83, 887–894. doi: 10.2307/3284284
Lofgren, C. S., Dame, D. A., Breeland, S. G., Weidhaas, D. E., Jeffery, G., Kaiser, R., et al. (1974). Release of chemosterilized males for the control of Anopheles albimanus in El Salvador: III. Field methods and population control. Am. J. Trop. Med. Hyg. 23, 288–297. doi: 10.4269/AJTMH.1974.23.288
Louca, V., Lucas, M. C., Green, C., Majambere, S., Fillinger, U., and Lindsay, S. W. (2009). Role of fish as predators of mosquito larvae on the floodplain of the Gambia river. J. Med. Entomol. 46, 546–556. doi: 10.1603/033.046.0320
Louis, J. P., and Albert, J. P. (1988). Malaria in the Republic of Djibouti. Strategy for control using a biological antilarval campaign: indigenous larvivorous fishes (Aphanius dispar) and bacterial toxins. Med. Trop. 48, 127–131.
Lounibos, L. P. (1980). The bionomics of three sympatric Eretmapodites (Diptera: Culicidae) at the Kenya coast. Bull. Entomol. Res. 70, 309–320. doi: 10.1017/S0007485300007598
Loyola, E. G., Rodriguez, M. H., Gonzalez, L., Arredondo, J. I., Bown, D. N., and Vaca, M. A. (1990). Effect of indoor residual spraying of DDT and bendiocarb on the feeding patterns of Anopheles pseudopunctipennis in Mexico. J. Am. Mosq. Control Assoc. 6, 635–640.
Loyola, E. G., Vaca, M. A., Bown, D. N., Perez, E., and Rodriguez, M. H. (1991). Comparative use of bendiocarb and DDT to control Anopheles pseudopunctipennis in a malarious area of Mexico. Med. Vet. Entomol. 5, 233–242. doi: 10.1111/j.1365-2915.1991.tb00546.x
Lundkvist, E., Landin, J., Jackson, M., and Svensson, C. (2003). Diving beetles (Dytiscidae) as predators of mosquito larvae (Culicidae) in field experiments and in laboratory tests of prey preference. Bull. Entomol. Res. 93, 219–226. doi: 10.1079/BER2003237
Macfie, J. W. S., and Corson, J. F. (1922). A new species of filarial larva found in the skin of natives in the Gold Coast. Ann. Trop. Med. Parasitol. 16, 465–471. doi: 10.1080/00034983.1922.11684341
Mackenzie, J. S., Lindsay, M. D., Coelen, R. J., Broom, A. K., Hall, R. A., and Smith, D. W. (1994). Arboviruses causing human disease in the Australasian zoogeographic region. Arch. Virol. 136, 447–467. doi: 10.1007/BF01321074
Mahesh Kumar, P., Murugan, K., Kovendan, K., Panneerselvam, C., Prasanna Kumar, K., Amerasan, D., et al. (2012). Mosquitocidal activity Solanum xanthocarpum fruit extract and copepod Mesocyclops thermocyclopoides for the control of dengue vector Aedes aegypti. Parasitol. Res. 111, 609–618. doi: 10.1007/s00436-012-2876-z
Mains, J. W., Kelly, P. H., Dobson, K. L., Petrie, W. D., and Dobson, S. L. (2019). Localized control of Aedes aegypti (Diptera: Culicidae) in Miami, FL, via inundative releases of Wolbachia-infected male mosquitoes. J. Med. Entomol. 56, 1296–1303. doi: 10.1093/jme/tjz051
Mandal, S., Ghosh, A., Bhattacharjee, I., and Chandra, G. (2008). Biocontrol efficacy of odonate nymphs against larvae of the mosquito, Culex quinquefasciatus say, 1823. Acta Trop. 106, 109–114. doi: 10.1016/j.actatropica.2008.02.002
Männikkö, N. (2011). Etymologia: Francisella tularensis. Emerg. Infect. Dis. 17:799. doi: 10.3201/eid1705.ET1705
Manrique-Saide, P., Ibanez-Bernal, S., Delfin-Gonzalez, H., and Parra Tabla, V. (1998). Mesocyclops longisetus effects on survivorship of Aedes aegypti immature stages in car tyres. Med. Vet. Entomol. 12, 386–390. doi: 10.1046/j.1365-2915.1998.00126.x
Manson, P. (1891). The filaria sanguinis hominis major and minor, two new species of haematozoa. Lancet 137, 4–8. doi: 10.1016/S0140-6736(02)15666-2
Marchette, N. J., Garcia, R., and Rudnick, A. (1969). Isolation of Zika virus from Aedes aegypti mosquitoes in Malaysia. Am. J. Trop. Med. Hyg. 18, 411–415. doi: 10.4269/ajtmh.1969.18.411
Marshall, I. D. (1988). “Murray Valley and Kunjin encephalitis” in The arboviruses: epidemiology and ecology. ed. T. P. Monath, vol. III (Florida: CRC Press), 151–190.
Marten, G. G., Astaiza, R., Suarez, M. F., Monje, C., and Reid, J. W. (1989). Natural control of larval Anopheles albimanus (Diptera: Culicidae) by the predator Mesocyclops (Copepoda: Cyclopoida). J. Med. Entomol. 26, 624–627. doi: 10.1093/jmedent/26.6.624
Marten, G. G., Nguyen, M., and Ngo, G. (2000). Copepod predation on Anopheles quadrimaculatus larvae in rice fields. J. Vector Ecol. 25, 1–6.
Marten, G. G., and Reid, J. W. (2007). Cyclopoid copepods. J. Am. Mosq. Control Assoc. 23, 65–92. doi: 10.2987/8756971X(2007)23[65,CC]2.0.CO;2
Martinez-Sanchez, A., Camacho, A. D., Quintero-Martinez, M. T., and Alejandre-Aguilar, R. (2007). Effect of ectoparasitic Pimeliaphilus plumifer mites (Acari: Pterygosomatidae) on Meccus pallidipennis (Hemiptera: Reduviidae) and several other Chagas' disease vectors under laboratory conditions. Exp. Appl. Acarol. 42, 139–149. doi: 10.1007/s10493-007-9079-9
Mather, T. N., Piesman, J., and Spielman, A. (1987). Absence of spirochaetes (Borrelia burgdorgferi) and piroplasms (Babesia microti) in deer ticks (Ixodes dammini) parasitized by chalcid wasps (Hunterellus hookeri). Med. Vet. Entomol. 1, 3–8. doi: 10.1111/j.1365-2915.1987.tb00317.x
Matsuo, N. (2019). Discovery and development of pyrethroid insecticides. Proc. Jpn. Acad. B. Phys. Biol. Sci. 95, 378–400. doi: 10.2183/pjab.95.027
Maurin, M., Pelloux, I., Brion, J. P., Del Banõ, J.-N., and Picard, A. (2011). Human tularemia in France, 2006-2010. Clin. Infect. Dis. 53, e133–e141. doi: 10.1093/cid/cir612
McCray, E. M. Jr., Fay, R. W., and Schoof, H. F. (1970). The bionomics of Lankesteria culicis and Aedes aegypti. J. Invertebr. Pathol. 16, 42–53. doi: 10.1016/0022-2011(70)90203-X
McDade, J. E., and Newhouse, V. F. (1986). Natural history of rickettsia rickettsii. Annu. Rev. Microbiol. 40, 287–309. doi: 10.1146/annurev.mi.40.100186.001443
McDade, J. E., Shepard, C. C., Redus, M. A., Newhouse, V. F., and Smith, J. D. (1980). Evidence of rickettsia prowazekii infection in the United States. Am. J. Trop. Med. Hyg. 29, 277–284. doi: 10.4269/ajtmh.1980.29.277
McInnis, D., Tam, S., Grace, C., and Miyashita, D. (1994). Populations suppression and sterility rates induced by variable sex-ratio, terile insect releases of Ceratitis capitata (Diptera, Tephritidae) in Hawaii. Ann. Entomol. Soc. Am. 87, 231–240. doi: 10.1093/aesa/87.2.231
Menon, P. K., and Rajagopalan, P. K. (1978). Control of mosquito breeding in wells by using Gambusia affinis and Aplocheilus blochii in Pondicherry town. Indian J. Med. Res. 68, 927–933.
Mitchell, C. J., Chen, P. S., and Chapman, H. C. (1974). Exploratory trials utilizing a mermithid nematode as a control agent for Culex mosquitoes in Taiwan (China). Taiwan Yi Xue Hui Za Zhi 73, 241–254.
Mogi, M., and Chan, K. L. (1996). Predatory habits of dipteran larvae inhabiting nepenthes pinchers. Raffles Bull. Zool. 44, 233–245.
Molloy, D. P., and Jamnback, H. (1977). A larval black fly control field trial using mermithid parasites and its cost implications. Mosq. News 37, 104–108.
Moore, C. G., and Mitchell, C. J. (1997). Aedes albopictus in the United States: ten-year presence and public health implications. Emerg. Infect. Dis. 3, 329–334. doi: 10.3201/eid0303.970309
Mooring, M. S., and Mundy, P. J. (1996). Factors influencing host selection by yellow-billed oxpeckers at Matoba national park, Zimbabwe. Afr. J. Ecol. 34, 177–188. doi: 10.1111/j.1365-2028.1996.008-89008.x
Moreau, R. (1933). The food of the red-billed oxpecker, Buphagus erythrorhynchus (Stanley). Bull. Entomol. Res. 24, 325–335. doi: 10.1017/S000748530003162X
Moreira, L. A., Iturbe-Ormaetxe, I., Jeffery, J. A., Lu, G., Pyke, A. T., Hedges, L. M., et al. (2009a). A Wolbachia symbiont in Aedes aegypti limits infection with dengue, chikungunya and plasmodium. Cells 139, 1268–1278. doi: 10.1016/j.cell.2009.11.042
Moreira, L. A., Saig, E., Turley, A. P., Ribeiro, J. M., O'Neill, S. L., and McGraw, E. A. (2009b). Human probing behavior of Aedes aegypti when infected with a life-shortening strain of Wolbachia. PLoS Negl. Trop. Dis. 3:e568. doi: 10.1371/journal.pntd.0000568
Motabar, M. (1978). Larvivorous fish, Gambusia affinis: a review. Geneva: World Health Organization.
Mourya, D. T., Singh, D. K., Yadav, P., Gokhale, M. D., Barde, P. V., Narayan, N. B., et al. (2003). Role of gregarine parasite Ascogregarina culicis (Apicomplexa: Lecudinicae) in the maintenance of Chikungunya virus in vector mosquito. J. Eukaryot. Microbiol. 50, 379–382. doi: 10.1111/j.1550-7408.2003.tb00153.x
Mousson, L., Zouache, K., Arias-Goeta, C., Raquin, V., Mavingui, P., and Failloux, A. B. (2012). The native Wolbachia symbionts limit transmission of dengue virus in Aedes albopictus. PLoS Negl. Trop. Dis. 6:e1989. doi: 10.1371/journal.pntd.0001989
Mukhopadhyay, J., Ghosh, K., and Braigh, H. R. (2000). Identification of cutaneous leishmaniasis vectors, Phlebotomus papatasi and P. duboscqi using random amplified polymorphic DNA. Acta Trop. 76, 277–283. doi: 10.1016/s0001-706x(00)00130-3
Müller, M., and Schlagenhauf, P. (2014). Plasmodium knowlesi in travellers, update 2014. Int. J. Infect. Dis. 22, 55–64. doi: 10.1016/j/ijid.2013.12.016
Mulyaningsih, B., Umniyati, S. R., Hadisusanto, S., and Edyansyah, E. (2019). Study on vector mosquito of zoonotic Brugia malayi in Musi Rawas, south Sumatera, Indonesia. Vet. World 12, 1729–1734. doi: 10.14202/vetworld.2019.1729-1734
Mundy, P. J., and Cook, A. W. (1975). Observation of the yellow-billed oxpecker Buphagus africanus in northern Nigeria. Ibis 117, 504–506. doi: 10.1111/j/1474-919X.1975.tb04243.x
Murdock, W. W., Scott, M. A., and Ebsworth, P. (1984). Effects of the general predator, Notonecta (Hemiptera) upon a fresh water community. J. Anim. Ecol. 53, 791–808. doi: 10.2307/4660
Murphy, F. A. (1998). Emerging zoonoses. Emerg. Infect. Dis. 4, 429–435. doi: 10.3201/eid0403.980324
Murphy, A., Rajahram, G. S., Jilip, J., Maluda, M., William, T., Hu, W., et al. (2020). Incidence and epidemiological features of dengue in Sabah, Malaysia. PLoS Negl. Trop. Dis. 14:e0007504. doi: 10.1371/journal.pntd.0007504
Muslim, A., Fong, M. Y., Mahmud, R., Lau, Y. L., and Sivanandam, S. (2013). Armigeres subalbatus incriminated as a vector of zoonotic Brugia pahangi filariasis in suburban Kuala Lumpur, Peninsular Malaysia. Parasit. Vectors 6:219. doi: 10.1186/1756-3305-6-219
Mylonakis, E. (2001). When to suspect and how to monitor babesiosis. Am. Fam. Physician 63, 1969–1974.
Najera, J. A., Gonzalez-Silva, M., and Alonso, P. (2011). Some lessons for the future from the global malaria eradication Programme (1955-1969). PLoS Med. 8:e1000412. doi: 10.1371/journal.pmed.1000412
Nazni, W. A., Hoffmann, A. A., NoorAfizah, A., Cheong, Y. L., Mancini, M. V., Golding, N., et al. (2019). Establishment of Wolbachia strain wAlbB in Malaysian populations of Aedes aegypti for dengue control. Curr. Biol. 29, 4241–4248.e5. doi: 10.1016/j.cub.2019.11.007
Ndava, J., Llera, S. D., and Manyanga, P. (2018). The future of mosquito control: the role of spiders as biological control agents: a review. Int. J. Mosq. Res. 5, 6–11.
Negri, I., Pellecchia, M., Mazzoglio, P. J., Patetta, A., and Alma, A. (2006). Feminizing Wolbachia in Zyginidia pullula (Insecta, Hemiptera), a leafhopper with an XX/XO sex-determination system. Proc. R. Soc. B Biol. Sci. 273, 2409–2416. doi: 10.1098/rspb.2006.3592
Nilsson, A. N., and Savensson, B. W. (1994). Dytiscid predators and culicid prey in two boreal snowmelt pools differing in temperature and duration. Ann. Zool. Fenn. 31, 365–376.
Nilsson, A. N., and Soderstrom, O. (1988). Larval consumption rates, interspecific predation and local guild composition of egg-overwintering Agabus (Coleoptera: Dytiscidae) species in vernal ponds. Oecologia 76, 131–137. doi: 10.1107/BF00379611
Nitatpattana, N., Apiwathnasorn, C., Barbazan, P., Leemingsawat, S., Yoksan, S., and Gonzales, J.-P. (2005). First isolation of Japanese encephalitis from Culex quinquefasciatus in Thailand. Southeast Asian J. Trop. Med. Public Health 36, 875–878.
Niyas, K., Abraham, R., Unnikrishnan, R., Mathew, T., Nair, S., Manakkadan, A., et al. (2010). Molecular characterization of Chikungunya virus isolates from clinical samples and adult Aedes albopictus mosquitoes emerged from larvae from Kerala, South India. Virol. J. 7:189. doi: 10.1186/1743-422X-7-189
Njiokou, F., Laveissière, C., Simo, G., Nkinin, S., Grébaut, P., Cuny, G., et al. (2006). Wild fauna as a probable animal reservoir for Trypanosoma brucei gambiense in Cameroon. Infect. Genet. Evol. 6, 147–153. doi: 10.1016/j.meegid.2005.04.003
Novak, R. J., Gubler, D., and Underwood, D. (1986). Evaluation of slow-release formulations of temephos (abate) and bacillus thuringiensis var. israelensis for the control of Aedes aegypti in Puerto Rico. J. Am. Mosq. Control Assoc. 1, 449–453.
Nunthanid, P., Roongruanchai, K., Wongkamchai, S., and Sarasombath, P. T. (2020). Case report: periorbital filariasis caused by Brugia malayi. Am. J. Trop. Med. Hyg. 103, 2336–2338. doi: 10.4269/ajtmh.20-0853
O’Leary, S., and Adelman, Z. N. (2020). CRISPR/Cas9 knockout of female-biased genes AeAct-4 or myo-fem in ae. Aegypti results in a flightless phenotype in female, but not male mosquitoes. PLoS Negl. Trop. Dis. 14:e0008971. doi: 10.1371/journal.pntd.0008971
Ohashi, N., Inayoshi, M., Kitamura, K., Kawamori, F., Kawaguchi, D., Nishimura, Y., et al. (2005). Anaplasma phagocytophilum-infected ticks, Japan. Emerg. Infect. Dis. 11, 1780–1783. doi: 10.3201/eid1111.050407
Okamoto, K. W., and Amarasekare, P. (2012). The biological control of disease vectors. J. Theor. Biol. 309, 47–57. doi: 10.1016/j.jtbi.2012.05.020
O'Neill, S. L., Hoffman, A., and Werren, J. H. (1997). Influential passengers: inherited microorganisms and arthropod reproduction. Oxford: Oxford University Press.
Orozco, M. M., Enriquez, G. F., Alvarado-Otegui, J. A., Cardinal, M. V., Schijman, A. G., Kitron, U., et al. (2013). New sylvatic hosts of Trypanosoma cruzi and their reservoir competence in the humid Chaco of Argentina: a longitudinal study. Am. J. Trop. Med. Hyg. 88, 872–882. doi: 10.4269/ajtmh.12-0519
Paily, K. P., and Balaraman, K. (2000). Susceptibility of ten species of mosquito larvae to the parasitic nematode Romanonermis iyengari and its development. Med. Vet. Entomol. 14, 426–429. doi: 10.1046/j.1365-2915.2000.00263.x
Panteleev, D., Goriacheva, I. I., Andrianov, B. V., Reznik, N. L., Lazebnyi, O. E., and Kulikov, A. M. (2007). The endosymbiotic bacterium Wolbachia enhances the nonspecific resistance to insect pathogens and alters behavior of Drosophila melanogaster. Genetika 43, 1277–1280.
Parker, A. G. (2005). “Mass-rearing for sterile insect release” in Sterile insect technique: Principles and practice in area-wide integrated pest management. eds. V. A. Dyck, J. Hendrichs, and A. S. Robinson (The Netherlands: Springer)
Parker, R. R., Spencer, R. R., and Francis, E. (1924). Tularemia: XI. Tularemia infection in ticks of the species Dermacentor andersoni stiles in the Bitterrot Valley. Mont. Public Health Rep. 39, 1057–1073. doi: 10.2307/4577151
Patterson, R. S., Lowe, R. E., Smittle, B. J., Dame, D. A., Boston, M. D., and Cameron, A. L. (1977). Release of radiosterilized males to control Culex pipiens quinquefasciatus (Diptera: Culicidae). J. Med. Entomol. 14, 299–304. doi: 10.1093/jmedent/14.3.299
Patterson, R. S., Weidhaas, D. E., Ford, H. R., and Lofgren, C. S. (1970). Suppression and elimination of an island population of Culex pipiens quinquefasciatus with sterile males. Science 168, 1368–1370. doi: 10.1126/science.168.3937.1368
Paula, A. R., Carolino, A. T., Paula, C. O., and Samuels, R. I. (2011a). The combination of the entomopathogenic fungus Metarhizium anisopliae with the insecticide imidacloprid increases virulence against the dengue vector Aedes aegypti (Diptera: Culicidae). Parasit. Vectors 4:8. doi: 10.1186/1756-3305-4-8
Paula, A. R., Carolino, A. T., Silva, C. P., and Samuels, R. I. (2011b). Susceptibility of adult female Aedes aegypti (Diptera: Culicidae) to the entomopathogenic fungus Metarhizium anisopliae is modified following blood feeding. Parasit. Vectors 4:91. doi: 10.1186/1756-3305-4-91
Peng, Y. F., Song, J. Z., Tian, G. Z., Xue, Q. L., Ge, F. X., Yang, J. L., et al. (1998). Field evaluations of Romanomermis yunanensis (Nematoda: Mermithidae) for control of Culicinae mosquitoes in China. Fundam. Appl. Nematol. 21, 227–232.
Petersen, J. J., Hoy, J. B., and O'Berg, A. G. (1972). Preliminary field tests with Reesimermis nielseni against mosquito larvae in California rice fields. Calif. Vector Views 19, 47–50.
Petersen, J. J., and Willis, O. R. (1972). Results of preliminary field applications of Reesimermis nielseni (Mermithidae: Nematoda) to control mosquito larvae. Mosq. News 32, 312–316.
Petersen, J. J., and Willis, O. R. (1974). Diximermis peterseni (Nematoda: Mermithidae): a potential biocontrol agent of Anopheles mosquito larvae. J. Invertebr. Pathol. 24, 20–23. doi: 10.1016/0022-2011(74)90158-X
Pfitzner, W. P., Beck, M., Weitzel, T., and Becker, N. (2015). The role of mosquitoes in the diet of adult dragon and damselflies (Odonata). J. Am. Mosq. Control Assoc. 31, 187–189. doi: 10.2987/14-6476R
Pham, X. D., Otsuka, Y., Suzuki, H., and Takaoka, H. (2001). Detection of Orientia tsutsugamushi (Rickettsiales: Rickettsiaceae) in unengorged chiggers (Acari: Trombiculidae) from Oita prefecture, Japan by nested polymerase chain reaction. J. Med. Entomol. 38, 308–311. doi: 10.1603/0022-2585-38.2.308
Phuc, H. K., Andreasen, M. H., Burton, R. S., Vass, C., Epton, M. J., Pape, G., et al. (2007). Late-acting dominant lethal genetic systems and mosquito control. BMC Biol. 5:11. doi: 10.1186/1741-7007-5-11
Pilossof, R. (2016). A brief history of tsetse control methods in Zimbabwe and possible effects of climate change on their distribution. Int. J. Afr. Dev. 4, 88–101.
Plantan, T., Howitt, M., Kotze, A., and Gaines, M. (2012). Feeding preferences of the red-billed oxpecker, Buphagus erythrorhynchus: a parasitic mutualist? Afr. J. Ecol. 51, 325–336. doi: 10.1111/aje.12042
Poinar, G. O., and Camino, N. B. (1986). Strelkovimermis spiculatus n. sp. (Mermithidae: Nematoda) parasitizing Aedes albifasciatus mac. (Culicidae: Diptera) in Argentina. J. Nematol. 18, 317–319.
Popovici, J., Moreira, L. A., Poinsignon, A., Iturbe-Ormaetxe, I., McNaughton, D., and O'Neill, S. L. (2010). Assessing key safety concerns of a Wolbachia-based strategy to control dengue transmission by Aedes mosquitoes. Mem. Inst. Oswaldo Cruz 105, 957–964. doi: 10.1590/s0074-02762010000800002
Poulin, B., and Lefebvre, G. (2018). Perturbation and delayed recovery of the reed invertebrate assemblage in Camargue marshes sprayed with bacillus thuringiensis israelensis. Insect Sci. 25, 542–548. doi: 10.1111/1744-7917.12416
Poulin, B., Tétrel, C., and Lefebvre, G. (2022). Impact of mosquito control operations on waterbirds in a Camargue nature reserve. Wetl. Ecol. Manag. 30, 1049–1064. doi: 10.1007/s11273-021-09834-4
Pramasivan, S., Ngui, R., Jeyaprakasam, N. K., Liew, J. W. K., Low, V. L., Mohamed Hassan, N., et al. (2021). Spatial distribution of plasmodium knowlesi cases and their vectors in Johor, Malaysia: in light of human malaria elimination. Malar. J. 20:426. doi: 10.1186/s12936-021-03963-0
Pritchard, G. (1964). The prey of adult dragonflies in northern Alberta. Can. Entomol. 96, 821–825. doi: 10.4039/Ent96821-6
Promdonkoy, B., and Ellar, D. J. (2003). Investigation of the pore-forming mechanism of a cytolytic delta-endotoxin from bacillus thuringiensis. Biochem. J. 374, 255–259. doi: 10.1042/BJ20030437
Prusty, A. K., Meena, D. K., Mohapatra, S., Panikkar, P., Das, P., Gupta, S. K., et al. (2015). Synthetic pyrethroids (type II) and freshwater fish culture: perils and mitigations. Int. Aquat. Res. 7, 163–191. doi: 10.1007/s40071-015-0106-x
Quiroz-Martinez, H., and Rodriguez-Castro, A. (2007). Aquatic insects as predators of mosquito larvae. J. Am. Mosq. Control Assoc. 23, 110–117. doi: 10.2987/8756-971X(2007)23[110:AIAPOM]2.0.CO;2
Raghavendra, K., Sharma, P., and Dash, A. P. (2008). Biological control of mosquito populations through frogs: opportunities & constraints. Indian J. Med. Res. 128, 22–25.
Ramlee, S., Rashid, N., and Azirun, M. S. (2022). Odonata nymphs as potential biocontrol agent of mosquito larvae in Malaysia. Southeast Asian J. Trop. Med. Public Health 53, 426–435.
Rasgon, J. L., and Scott, T. W. (2004). An initial survey for Wolbachia (Rickettsiales: Rickettsiaceae) infections in selected California mosquitoes (Diptera: Culicidae). J. Med. Entomol. 41, 255–257. doi: 10.1603/0022-2585-41.2.255
Rasgon, J. L., Styer, L. M., and Scott, T. W. (2003). Wolbachia-induced mortality as a mechanism to modulate pathogen transmission by vector arthropods. J. Med. Entomol. 40, 125–132. doi: 10.1603/0022-2585-40.2.125
Rassi, A. Jr., Rassi, A., and Marin-Neto, J. A. (2010). Chagas disease. Lancet 375, 1388–1402. doi: 10.1016/S0140-6736(10)60061-X
Rawlins, S. C., Clark, G. G., and Martinez, R. (1991). Effects of single introduction of Toxorhynchites moctezuma upon Aedes aegypti on a Caribbean Island. J. Am. Mosq. Control Assoc. 7, 7–10.
Resnik, D. B. (2014). Ethical issues in field trials of genetically modified disease-resistant mosquitoes. Dev. World Bioeth. 14, 37–46. doi: 10.1111/dewb.12011
Resnik, D. B. (2017). Field trials of genetically modified mosquitoes and public health ethics. Am. J. Bioeth. 17, 24–26. doi: 10.1080/15265161.2017.1353170
Reynolds, D. G. (1972). Experimental introduction of a microsporidian into awild population of Culex pipiens fatigans Wied. Bull. World Health Organ. 46, 807–812.
Rigau-Perez, J. G., and Gubler, D. J. (1997). “Surveillance for dengue and dengue hemorrhagic fever” in Dengue and dengue hemorrhagic fever. eds. D. J. Gubler and G. Kono (London, United Kingdom: CAB International), 405–423.
Rijal, S., Sundar, S., Mondal, D., Das, P., Alvar, J., and Boelaert, M. (2019). Eliminating visceral leishmaniasis in South Asia: the road ahead. BMJ 364:k5224. doi: 10.1136/bmj.k5224
Ritchie, S. A., Rapley, L. P., and Benjamin, S. (2010). Bacillus thuringiensis var. israelensis (Bti) provides residual control of Aedes aegypti in small containers. Am. J. Trop. Med. Hyg. 82, 1053–1059. doi: 10.4269/ajtmh.2010.09-0603
Ritchie, S. A., Townsend, M., Paton, C. J., Callahan, A. G., and Hoffman, A. A. (2015). Application of wMelPop Wolbachia strain to crash local populations of Aedes aegypti. PLoS Negl. Trop. Dis. 9:e0003930. doi: 10.1371/journal.pntd.0003930
Riviere, F., and Thirel, R. (1981). Predation of the copepod Mesocyclops leuckatipilosa (Crustacea) on the larvae of Aedes (Stegomyia) aegypti and ae. (St.) polynesiensis (Diptera: Culicidae): preliminary trials of its use as a biological agent. Entomophaga 26, 427–439. doi: 10.1007/BF02374717
Robert, L. I., Perich, M. J., Schlein, Y., Jacobson, R. L., Wirtz, R. A., Lawyer, P. G., et al. (1997). Phlebotomine sand fly control using bait-fed adults to carry the larvicide Bacillus sphaericus to the larval habitat. J. Am. Mosq. Control Assoc. 13, 140–144.
Roberts, D. R., and Alecrim, W. D. (1991). Behavioral response of Anopheles darlingi to DDT-sprayed house walls in Amazonia. Bull. Pan Am. Health Organ. 25, 210–217.
Roekring, S., and Smith, D. R. (2010). Induction of apoptosis in densovirus infected Aedes aegypti mosquitoes. J. Invertebr. Pathol. 104, 239–241. doi: 10.1016/j.jip.2010.04.002
Rohani, A., Fakhriy, H. A., Suzilah, I., Zurainee, M. N., Wan Najdah, W. M. A., Ariffin, M. M., et al. (2020). Indoor and outdoor residual spraying of a novel formulation of deltamethrin K-Othrine® (Polyzone) for the control of simian malaria in Sabah, Malaysia. PLoS ONE. 15:e0230860. doi: 10.1371/journal.pone.0230860
Rohani, A., Lokman, H. S., Hassan, A. R., Chan, S. T., Ong, Y. F., Abdullah, A. G., et al. (1999). Bionomics of Anopheles balabacensis Baisas, the principal malaria vectors in Ranau. Sabah. Trop. Biomed. 16, 1–8.
Rosen, L., and Gubler, D. J. (1974). The use of mosquitoes to detect and propagate dengue virus. Am. J. Trop. Med. Hyg. 23, 1153–1160. doi: 10.4269/ajtmh.1974.23.1153
Ross, P. A., Wiwatanaratanabutr, I., Axford, J. K., White, V. L., Endersby-Harshman, N. M., and Hoffman, A. A. (2017). Wolbachia infections in Aedes aegypti differ markedly in their response to cyclical heat stress. PLoS Pathog. 13:e1006006. doi: 10.1371/journal.ppat.1006006
Rupp, H. R. (1996). Adverse assessments of Gambusia affinis: an alternate view for mosquito control practitioners. J. Am. Mosq. Control Assoc. 12, 155–166.
Samish, M., and Glazer, I. (2001). Entomopathogenic nematodes for the biocontrol of ticks. Trends Parasitol. 17, 368–371. doi: 10.1016/s1471-4922(01)01985-7
Sanad, M. M., Shamseldean, M. S. M., Elgindi, A.-E. Y., and Gaugler, R. (2013). Host penetration and emergence patterns of the mosquito-parasitic mermithids Romanonermis iyengari and Strelkovimermis spiculatus (Nematoda: Mermithidae). J. Nematol. 45, 30–38.
Sanad, M. M., Sun, J. S., Shamseldean, M. S. M., Wang, Y., and Gaugler, R. (2017). Superparasitism and population regulation of the mosquito-parasitic mermithid nematodes Romanomermis iyengari and Strelkovimermis spiculatus. J. Nematol. 49, 168–176. doi: 10.21307/jofnem-2017-078
Sang, R., Arum, S., Chepkorir, E., Mosomtai, G., Tigoi, C., Sigei, F., et al. (2017). Distribution and abundance of key vectors of Rift Valley fever and other arboviruses in two ecologically distinct counties in Kenya. PLoS Negl. Trop. Dis. 11:e0005341. doi: 10.1371/journal.pntd.0005341
Sankaralingam, A., and Venkatesan, P. (1989). Larvicidal properties of water bug Diplonychus indicus Venkatesan & Rao and its use in mosquito control. Indian J. Exp. Biol. 27, 174–176.
Santamarina Mijares, A., and Perez Pacheco, R. (1997). Reduction of mosquito larval densities in natural sites after introduction of Romanomermis culicivorax (Nematoda: Mermithidae) in Cuba. J. Med. Entomol. 34, 1–4. doi: 10.1093/jmedent/34.1.1
Schaper, S. (1999). Evaluation of Costa Rican copepods (Crustacea: Eudecapoda) for larval Aedes aegypti control with special reference to Mesocyclops thermocyclopoides. J. Am. Mosq. Control Assoc. 15, 510–519.
Schiller, A., Allen, M., Coffey, J., Fike, A., and Carballo, F. (2019). Updated methods for the production of Toxorhynchites rutilus septentrionalis (Diptera, Culicidae) for use as biocontrol agent against container breeding pest mosquitoes in Harris County, Texas. J. Insect Sci. 19:8. doi: 10.1093/jisesa/iez011
Scholte, E. J., Knols, B. G. J., Samson, R. A., and Takken, W. (2004). Entomopathogenic fungi for mosquito control: a review. J. Insect Sci. 4:19. doi: 10.1093/jis/4.1.19
Scholte, E.-J., Takken, W., and Knols, B. G. J. (2007). Infection of adult Aedes aegypti and ae. Albopictus mosquitoes with the entomopathogenic fungus Metarhizium anisopliae. Acta Trop. 102, 151–158. doi: 10.1016/j.actatropica.2007.04.011
Scola, B. L., Bandi, C., and Raoult, D. (2015). “Wolbachia” in Bergey's manual of systematics of archaea and bacteria. eds. W. B. Whitman, F. Rainey, P. Kampfer, M. Trujillo, J. Chun, and P. DeVoset al (Hoboken, NJ: John Wiley & Sons, Inc), 1–12.
Scott, W. N. (1966). Tsetse control by game destruction. Oryx 8, 244–248. doi: 10.1017/S0030605300004816
Scott, T. W., Naksathit, A., Day, J. F., Kittayapong, P., and Edman, J. D. (1997). A fitness advantage for Aedes aegypti and the viruses it transmits when females feed only on human blood. Am. J. Trop. Med. Hyg. 57, 235–239. doi: 10.4269/ajtmh.1997.57.235
Sebastian, A., Sein, M. M., Thu, M. M., and Corbet, P. S. (1990). Suppression of Aedes aegypti (Diptera: Culicidae) using augmentative release of dragonfly larvae (Odonata: Libellulidae) with community participation in Yangon, Myanmar. Bull. Entomol. Res. 80, 223–232. doi: 10.1017/S0007485300013468
Sebastian, A., Thu, M. M., Kyaw, M., and Sein, M. M. (1980). The use of dragonfly nymphs in the control of Aedes aegypti. Southeast Asian J. Trop. Med. Public Health 11, 104–107.
Secundio, N. F. C., Araujo, M. S. S., Oliveira, G. H. B., Massara, C. L., Carvalho, O. S., Lanfredi, R. M., et al. (2002). Preliminary description of a new entomoparasitic nematode infecting Lutzomyia longipalpis sand fly, the vector of visceral leishmaniasis in the New World. J. Invertebr. Pathol. 80, 35–40. doi: 10.1016/s0022-2011(02)00046-0
Selvarajan, R., and Kakkassery, F. K. (2019). Predatory potential of water bugs against the filarial vector Culex quinquefasciatus say. Indian J. Entomol. 81:40. doi: 10.5958/0974-8172.2019.00035.X
Seo, H. J., Kim, H. C., Klein, T. A., Ramey, A. M., Lee, J. H., Kyung, S. G., et al. (2013). Molecular detection and genotyping of Japanese encephalitis virus in mosquitoes during a 2010 outbreak in the Republic of Korea. PLoS One 8:e55165. doi: 10.1371/journal.pone.0055165
Serebrovsky, A. S. (1940). On the possibility of a new method for the control of insect pests. Zool. Zhurnal 19, 618–630.
Sexton, D. J., Dwyer, B., Kemp, R., and Graves, S. (1991). Spotted fever group rickettsial infections in Australia. Rev. Infect. Dis. 13, 876–886. doi: 10.1093/clinids/13.5.876
Shaalan, E. A.-S., and Canyon, D. V. (2009). Aquatic insect predators and mosquito control. Trop. Biomed. 26, 223–261.
Shaalan, E. A.-S., Canyon, D. V., Muller, R., Younes, M. W. F., Abdel-Wahab, H., and Mansour, A.-H. (2007). A mosquito predator survey in Townsville, Australia, and an assessment of Diplonychyus sp. and Anisops sp. predatorial capacity against Culex annulirostris mosquito immatures. J. Vector Ecol. 32, 16–21. doi: 10.3376/1081-1710(2007)32[16:ampsit]2.0.co;2
Sharma, V. P. (1976). Elimination of aziridine residues from chemosterilized mosquitoes. Nature 261:135. doi: 10.1038/261135a0
Sharma, V. P., Patterson, R. S., LaBrecque, G. C., and Singh, K. R. P. (1976). Three field releases with chemosterilized Culex pipiens fatigans Wied in Delhi villages. J. Commun. Dis. 8, 18–27.
Shirai, A., Tanskul, P. L., and Andre, R. G. (1981). Rickettsia tsutsugamushi strains found in chiggers collected in Thailand. Southeast Asian J. Trop. Med. Public Health 12, 1–6.
Shirato, K., Miyoshi, H., Kariwa, H., and Takashima, I. (2005). Detection of West Nile virus and Japanese encephalitis virus using real-time PCR with a probe common to both viruses. J. Virol. Methods 126, 119–125. doi: 10.1016/j.jviromet.2005.02.001
Shore, H. (1961). O'nyong-nyong fever: an epidemic virus disease in East Africa. III. Some clinical and epidemiological observations in the northern province of Uganda. Trans. R. Soc. Trop. Med. Hyg. 55, 361–373. doi: 10.1016/0035-9203(61)90106-7
Short, N. J., and Norval, R. A. I. (1982). Tick predation by shrews in Zimbabwe. J. Parasitol. 68:1052. doi: 10.2307/3281090
Siler, J. F., Hall, M. W., and Hitchens, A. (1926). Dengue: its history, epidemiology, mechanism of transmission, etiology, clinical manifestations, immunity, and prevention. Philipp. J. Sci. 29, 1–304.
Singh, B., and Daneshvar, C. (2013). Human infections and detection of plasmodium knowlesi. Clin. Microbiol. Rev. 26, 165–184. doi: 10.1128/CMR.00079-12
Singh, R. K., Dhiman, R. C., and Singh, S. P. (2003). Laboratory studies on the predatory potential of dragon-fly nymphs on mosquito larvae. J. Commun. Dis. 35, 96–101.
Singh, N., Mishra, J., Singh, R., and Singh, S. (2013). Animal reservoirs of visceral leishmaniasis in India. J. Parasitol. 99, 64–67. doi: 10.1645/GE-3085.1
Sinka, M. E., Bangs, M. J., Manguin, S., Chareonviriyaphap, T., Patil, A. P., Temperley, W. H., et al. (2011). The dominant anopheles vectors of human malaria in the Asia-Pacific region: occurrence data, distribution maps and bionomic précis. Parasit. Vectors 4:89. doi: 10.1186/1756-3305-4-89
Sinka, M. E., Bangs, M. J., Manguin, S., Rubio-Palis, Y., Chareonviriyaphap, T., Coetzee, M., et al. (2012). A global map of dominant malaria vectors. Parasit. Vectors 5:69. doi: 10.1186/1756-3305-5-69
Sinka, M. E., Rubio-Palis, Y., Manguin, S., Patil, A. P., Temperley, W. H., Gething, P. W., et al. (2010). The dominant anopheles vectors of human malaria in the Americas: occurrence data, distribution maps and bionomic précis. Parasit. Vectors 3:72. doi: 10.1186/1756-3305-3-72
Sjöstedt, A. (2007). Tularemia: history, epidemiology, pathogen physiology, and clinical manifestations. Ann. N. Y. Acad. Sci. 1105, 1–29. doi: 10.1196/annals.1409.009
Smithuis, F. M., Kyaw, M. K., Phe, U. O., van der Broek, I., Katterman, N., Rogers, C., et al. (2013). Entomological determinants of insecticide-treated bed net effectiveness in Western Myanmar. Malar. J. 12:364. doi: 10.1186/1475-2875-12-364
Soh, S., Ho, S. H., Seah, A., Ong, J., Dickens, B. S., Tan, K. W., et al. (2021). Economic impact of dengue in Singapore from 2010 to 2020 and the cost-effectiveness of Wolbachia interventions. PLoS Glob. Public Health 1:e0000024. doi: 10.1371/journal.pgph.0000024
Soper, F. L., and Wilson, D. B. (1943). Anopheles gambiae in Brazil 1930 to 1940. New York: Rockfeller Foundation.
Soumare, M. K. F., and Cilek, J. E. (2011). The effectiveness of Mesocyclops longisetus (Copepoda) for the control of container-inhabiting mosquitoes in residential environments. J. Am. Mosq. Control Assoc. 27, 376–383. doi: 10.2987/11-6129.1
Sousa, C. A., Pinto, J., Almeida, P. G., Ferreira, C., Do Rosário, V. E., and Charlwood, J. D. (2001). Dogs as a fovored host choice of Anopheles gambiae sensu stricto (Diptera: Culicidae) of São Tomé, West Africa. J. Med. Entomol. 38, 122–125. doi: 10.1603/0022-2585-38.1.122
Spielman, A. (1976). Human babesiosis on Nantucket Island: transmission by nymphal Ixodes ticks. Am. J. Trop. Med. Hyg. 25, 784–787. doi: 10.4269/ajtmh.1976.25.784
Stabler, R. M. (1952). Parasitism of mosquito larvae by mermithids (Nematoda). J. Parasitol. 38, 130–132. doi: 10.2307/3273830
Steffan, W. A., and Evenhuis, N. L. (1981). Biology of Toxorhynchites. Annu. Rev. Entomol. 26, 159–181. doi: 10.1146/annurev.en.26.010181.001111
Steverding, D. (2017). The history of leishmaniasis. Parasit. Vectors 10:82. doi: 10.1186/s13071-017-2028-5
Steyn, J., Fourie, I., Steyl, J., Williams, J., Stivaktas, V., Botha, E., et al. (2020). Zoonotic alphaviruses in fatal and neurologic infections in wildlife and nonequine domestic animals, South Africa. Emerg. Infect. Dis. 26, 1182–1191. doi: 10.3201/eid2606.191179
Stouthamer, R., Breeuwer, J. A., and Hurst, G. D. (1999). Wolbachia pipientis: microbial manipulator of arthropod reproduction. Annu. Rev. Microbiol. 53, 71–102. doi: 10.1146/annurev.micro.53.1.71
Subramaniam, J., Murugan, K., Panneerselvam, C., Kovendan, K., Madhiyazhagan, P., Kumar, P. M., et al. (2015). Eco-friendly control of malaria and arbovirus vectors using the mosquito fish Gambusia affinis and ultra-low dosages of Mimusops elengi-synthesized silver nanoparticles: towards an integrative approach. Environ. Sci. Pollut. Res. 22, 20067–20083. doi: 10.1007/s11356-015-5253-5
Sucharit, S., Surathin, K., and Shrestha, S. R. (1989). Vectors of Japanese encephalitis virus (JEV): species complexes of the vectors. Southeast Asian J. Trop. Med. Public Health 20, 611–621.
Suchman, E. L., Kononko, A., Plake, E., Doehling, M., Kleker, B., Black, W. C., et al. (2006). Effects of AeDNV infection on Aedes aegypti lifespan and reproduction. Biol. Control 39, 465–473. doi: 10.1016/j.biocontrol.2006.05.001
Sukhacheva, G. A. (1996). Study of the natural diet of adult dragonflies using an immunological method. Odonatologica 25, 397–403.
Sulaiman, I. (1992). Infectivity and pathogenicity of Ascogregarina culicinis (Eugregarinida: Lecudinidae) to Aedes aegypti (Diptera: Culicidae). J. Med. Entomol. 29, 1–4. doi: 10.1093/jmedent/29.1.1
Swaminath, C. S., Short, H. E., and Anderson, L. A. P. (1942). Transmission of Indian kala-azar to man by the bite of P. argentipes. Indian J. Med. Res. 123, 473–477.
Swei, A., Couper, L. I., Coffey, L. L., Kapan, D., and Bennett, S. (2020). Patterns, drivers, and challenges of vector-borne disease emergence. Vector Borne Zoonotic Dis. 20, 159–170. doi: 10.1089/vbz.2018.2432
Szewczyk, B., de Souza, M. L., de Castro, M. E. B., Moscardi, M. L., and Moscardi, F. (2011). “Baculovirus biopesticides” in Pesticides – formulations, effects, fate. ed. M. Stoytcheva (Rijeka, Croatia: IntechOpen), 25–36.
Szewczyk, B., Rabalski, L., Krol, E., Sihler, W., and de Souza, M. L. (2009). Baculovirus biopesticides-a safe alternative to chemical protection of plants. J. Biopest. 2, 209–216.
Tabashnik, B. E. (1992). Evaluation of synergism among bacillus thuringiensis toxins. Appl. Environ. Microbiol. 58, 3343–3346. doi: 10.1128/aem.58.10.3343-3346.1992
Tan, C. H., Vythilingam, I., Matusop, A., Chan, S. T., and Singh, B. (2008). Bionomics of Anopheles latens in Kapit, Sarawak, Malaysian Borneo in relation to the transmission of zoonotic simian malaria parasite plasmodium knowlesi. Malar. J. 7:52. doi: 10.1186/1475-2875-7-52
Tangena, J.-A. A., Hendriks, C. M. J., Devine, M., Tammaro, M., Trett, A. E., Williams, I., et al. (2020). Indoor residual spraying for malaria control in sub-saharan Africa 1997 to 2017: an adjusted retrospective analysis. Malar. J. 19:150. doi: 10.1186/s12936-020-03216-6
Taylor, R. M., and Hurlbut, H. S. (1953). Isolation of West Nile virus from Culex mosquitoes. J. Egypt. Med. Assoc. 36, 199–208.
Taylor, L. H., Latham, S. M., and Woolhouse, M. E. (2001). Risk factors for human disease emergence. Philos. Trans. R. Soc. B. Biol. Sci. 356, 983–989. doi: 10.1098/rstb.2001.0888
Tetreau, G., Stalinski, R., David, J.-P., and Després, L. (2013). Monitoring resistance to bacillus thuringiensis subsp. israelensis in the field by performing bioassays with each cry toxin separately. Mem. Inst. Oswaldo Cruz 108, 894–900. doi: 10.1590/0074-0276130155
Thatheyus, A. J., and Selvam, A. D. G. (2013). Synthetic pyrethroids: toxicity and biodegradation. Appl. Ecol. Environ. Sci. 1, 33–36. doi: 10.12691/aees-1-3-2
Thomas, M. B. (2018). Biological control of human disease vectors: a perspective on challenger and opportunities. Biocontrol(Dordr) 63, 61–69. doi: 10.1007/s10526-017-9815-y
Tijsse-Klasen, E., Braks, M., Scholte, E. J., and Sprong, H. (2011). Parasites of vectors-Ixodiphagus hookeri and its Wolbachia symbionts in ticks in the Netherlands. Parasit. Vectors 4, 228–234. doi: 10.1186/1756-3305-4-228
Troha, K., Urbancic, N. B., Korva, M., Avsic-Zupanc, T., Battelino, S., and Vozel, D. (2022). Vector-borne tularemia: a re-emerging cause of cervical lymphadenopathy. Trop. Med. Infect. Dis. 7:189. doi: 10.3390/tropicalmed7080189
Tsuda, Y., Kobayashi, J., Nambanya, S., Miyagi, I., Toma, T., Phompida, S., et al. (2002). An ecological survey of dengue vector mosquitos in central Lao PDR. Southeast Asian J. Trop. Med. Public Health 33, 63–67.
Tucker, D. L., Beresford, C. H., Sigler, L., and Rogers, K. (2004). Disseminated Beauveria bassiana infection in a patient with acute lymphoblastic leukemia. J. Clin. Microbiol. 42, 5412–5414. doi: 10.1128/JCM.42.11.5412-5414.2004
Turelli, M. (2010). Cytoplasmic incompatibility in populations with overlapping generations. Evolution 64, 232–241. doi: 10.1111/j.1558-5646.2009.00822.x
Ujváry, I. (2010). “Pest control agents from natural products” in Hayes' handbook of pesticide toxicology. ed. R. Krieger. 3rd ed (United States: Academic Press), 119–229.
Ulrich, J. N., Beier, J. C., Devine, G. J., and Hugo, L. E. (2016). Heat sensitivity of wMel Wolbachia during Aedes aegypti development. PLoS Negl. Trop. Dis. 10:e0004873. doi: 10.1371/journal/pntd.0004873
Utzinger, J., Tozan, Y., and Singer, B. H. (2001). Efficacy and cost-effectiveness of environmental management for malaria control. Tropical Med. Int. Health 6, 677–687. doi: 10.1046/j.1365-3156.2001.00769.x
Valentine, M. J., Murdock, C. C., and Kelly, P. J. (2019). Sylvatic cycles of arboviruses in non-human primates. Parasit. Vectors 12:463. doi: 10.1186/s13071-019-3732-0
van den Berg, H., da Silva Bezerra, H. S., Al-Eryani, S., Chanda, E., Nagpal, B. N., Knox, T. B., et al. (2021a). Recent trends in global insecticide use for disease vector control and potential implications for resistance management. Sci. Rep. 11:23867. doi: 10.1038/s41598-021-03367-9
van den Berg, H., Kelly-Hope, L. A., and Lindsay, S. W. (2013). Malaria and lymphatic filariasis: the case for integrated vector management. Lancet Infect. Dis. 13, 89–94. doi: 10.1016/S1473-3099(12)70148-2
van den Berg, H., Velayudhan, R., and Yadav, R. S. (2021b). Management of insecticides for use in disease vector control: lessons from six countried in Asia and the Middle East. PLoS Negl. Trop. Dis. 15:e0009358. doi: 10.1371/journal.pntd.0009358
van den Berg, H., Zaim, M., Yadav, R. S., Soares, A., Ameneshewa, B., Mnzava, A. P., et al. (2012). Global trends in the use of insecticides to control vector-borne diseases. Environ. Health Perspect. 120, 577–582. doi: 10.1289/ehp.1104340
van den Bosch, R., Messenger, P. S., and Gutierrez, A. P. (1982). “The history and development of biological control” in An introduction to biological control. eds. R. Bosch, P. S. Messenger, and A. P. Gutierrez (US: Springer), 21–36.
van den Hurk, A. F., Hall-Mendelin, S., Pyke, A. T., Frentiu, F. D., McElroy, K., Day, A., et al. (2012). Impact of Wolbachia on infection with chikungunya and yellow fever viruses in the mosquito vector Aedes aegypti. PLoS Negl. Trop. Dis. 6:e1892. doi: 10.1371/journal.pntd.0001892
van den Hurk, A. F., Montgomery, B. L., Northill, J. A., Smith, I. L., Zborowski, P., Ritchie, S. A., et al. (2006). Short report: the first isolation of Japanese encephalitis virus from mosquitoes collected from mainland Australia. Am. J. Trop. Med. Hyg. 75, 21–25. doi: 10.4269/ajtmh.2006.75.21
van Schalkwyk, D. A., Moon, R. W., Blasco, B., and Sutherland, C. J. (2017). Comparison of the susceptibility of plasmodium knowlesi and plasmodium falciparum to antimalarial agents. J. Antimicrob. Chemother. 72, 3051–3058. doi: 10.1093/jac/dkx279
van Someren, V. D. (1951). The red-billed oxpecker and its relation to stock in Kenya. East Afr. Agric. J. 17, 1–11. doi: 10.1080/03670074.1951.11664775
Vatandoost, H. (2021). Dragonflies as an important aquatic predator insect and their potential for control of vectors for different diseases. J. Mar. Sci. 3, 13–20. doi: 10.30564/jms.v3i3.3121
Venkatesan, P., and Jeyachandra, C. M. (1985). Estimation of mosquito predation by the water bug Diplonychus indicus Venkatesan & Rao. Indian J. Exp. Biol. 23, 227–229.
Vershini, R. A., and Kanagappan, M. (2014). Effect of quantity of water on the feeding efficiency of dragonfly nymph – Bradynopyga geminate (Rambur). J. Entomol. Zool. Stud. 2, 249–252.
Vinogradov, D. D., Sinev, A. Y., and Tiunov, A. V. (2022). Predators as control agents of mosquito larvae in micro-reservoirs (review). Inland Water Biol. 15, 39–53. doi: 10.1134/S1995082922010138
Vorou, R. (2016). Zika virus, vectors, reservoirs, amplifying hosts, and their potential to spread worldwide: what we know and what we should investigate urgently. Int. J. Infect. Dis. 48, 85–90. doi: 10.1016/j.ijid.2016.05.014
Vreysen, M. J. B., Saleh, K., Mramba, F., Parker, A., Feldmann, U., Dyck, V. A., et al. (2014). Sterile insects to enhance agricultural development: the case of sustainable tsetse eradication on Unguja Island, Zanzibar, using an area-wide integrated pest management approach. PLoS Negl. Trop. Dis. 8:e2857. doi: 10.1371/journal.pntd.0002857
Vu, S. N., Nguyen, T. Y., Kay, B. H., Marten, G. G., and Reid, J. W. (1998). Eradication of Aedes aegypti from a village in Vietnam, using copepods and community participation. Am. J. Trop. Med. Hyg. 59, 657–660. doi: 10.4269/ajtmh.1998.59.657
Vu, S. N., Nguyen, T. Y., Tran, V. P., Truong, U. N., Le, Q. M., Le, V. L., et al. (2005). Elimination of dengue by community programs using Mesocyclops (Copepoda) against Aedes aegypti in Central Vietnam. Am. J. Trop. Med. Hyg. 72, 67–73. doi: 10.4269/ajtmh.2005.72.67
Vythilingam, I. (2012). Plasmodium knowlesi and Wuchereria bancrofti: their vectors and challenges for the future. Front. Physiol. 3:115. doi: 10.3389/fphys.2012.00115
Vythilingam, I., Chua, T. H., Liew, J. W. K., O'Mannin, B., and Ferguson, H. M. (2021). The vectors of plasmodium knowlesi and other simian malarias Southeast Asia: challenges in malaria elimination. Adv. Parasitol. 113, 131–189. doi: 10.1016/bs.apar.2021.08.005
Vythilingam, I., and Hii, J. (2013). “Simian malaria parasites: special emphasis on plasmodium knowlesi and their anopheles vectors in Southeast Asia” in Anopheles mosquitoes-New insights into malaria vectors. ed. S. Manguin (London: Intech Open), 487–510.
Vythilingam, I., Keokenchan, K., Phommakot, S., Nambanya, S., and Inthakone, S. (2001). Preliminary studies of anopheles mosquitos in eight provinces in Lao PDR. Southeast Asian J. Trop. Med. Public Health 32, 83–87.
Vythilingam, I., Lim, Y. A. L., Venugopalan, B., Ngui, R., Leong, C. S., Wong, M. L., et al. (2014). Plasmodium knowlesi malaria an emerging public health problem in Hulu Selangor, Selangor Malaysia (2009-2013): epidemiologic and entomologic analysis. Parasit. Vectors 7, 436–449. doi: 10.1186/1756-3305-7-436
Vythilingam, I., Oda, K., Chew, T. K., Mahadevan, S., Vijayamalar, B., Morita, K., et al. (1995). Isolation of Japanese encephalitis virus from mosquitoes collected in Sabak Bernam, Selangor, Malaysia in 1992. J. Am. Mosq. Control Assoc. 11, 94–98.
Vythilingam, I., Oda, K., Mahadevan, S., Abdullah, G., Thim, C. S., Hong, C. C., et al. (1997). Abundance, parity, and Japanese encephalitis virus infection of mosquitoes (Diptera: Culicidae) in Sepang district, Malaysia. J. Med. Entomol. 34, 257–262. doi: 10.1093/jmedent/34.3.257
Vythilingam, I., Oda, K., Tsuchie, H., Mahadevan, S., and Vijayamalar, B. (1994). Isolation of Japanese encephalitis virus from Culex sitiens mosquitoes in Selangor, Malaysia. J. Am. Mosq. Control Assoc. 10, 228–229.
Vythilingam, I., Sidavong, B., Chan, S., Phonemixay, T., Vanisaveth, V., Sisoulad, P., et al. (2005). Epidemiology of malaria in Attapeu Province, Lao PDR in relation to entomological parameters. Trans. R. Soc. Trop. Med. Hyg. 99, 833–839. doi: 10.1016/j.trstmh.2005.06.012
Vythilingam, I., Tan, C., Asmad, M., Chan, S., Lee, K., and Singh, B. (2006). Natural transmission of plasmodium knowlesi to humans by Anopheles latens in Sarawak, Malaysia. Trans. R. Soc. Trop. Med. Hyg. 100, 1087–1088. doi: 10.1016/j.trstmh.2006.02.006
Vythilingam, I., Wan-Sulaiman, W. Y., and Jeffery, J. (2013). “Vectors of malaria and Filariasis in Southeast Asia: a changing scenario” in Parasites and their vectors-a special focus on Southeast Asia. eds. Y. A. Lim and I. Vythilingam (Vienna: Springer), 57–75.
Walker, T., Johnson, P. H., Moreira, L. A., Iturbe-Ormaetxe, I., Frentiu, F. D., McMeniman, C. J., et al. (2011). The wMel Wolbachia strain blocks dengue and invades caged Aedes aegypti populations. Nature 476, 450–453. doi: 10.1038/nature10355
Walker, K., and Lynch, M. (2007). Contributions of anopheles larval control to malaria suppression in tropical Africa: review of achievements and potential. Med. Vet. Entomol. 21, 2–21. doi: 10.1111/j.1365-2915.2007.00674.x
Walter, G., and Weber, G. (1981). A study on the transmission (transstadial, transovarial) of Babesia microti, strain Hannover i, in its tick vector, Ixodes ricinus. Tropenmed Parasitol 32, 228–230.
Walton, W. E. (2007). Larvivorous fish including Gambusia. J. Am. Mosq. Control Assoc. 23, 184–220. doi: 10.2987/8756-971X(2007)23[184:LFIG]2.0.CO;2
Wang, Z. Y., He, K. L., Zhang, F., Lu, X. S., and Babendreier, D. (2014). Mass rearing and release of Trichogramma for biological control of insect pests of corn in China. Biol. Control 68, 136–144. doi: 10.1016/j.biocontrol.2013.06.015
Wang, Z. Z., Liu, Y. Q., Shi, M., Huang, J. H., and Chen, X. X. (2019). Parasitoid wasps as effective biological control agents. J. Integr. Agric. 18, 705–715. doi: 10.1016/S2095-3119(18)62078-7
Warburg, A., and Pimenta, P. F. (1995). A cytoplasmic polyhedrosis virus in the phlebotomine sand fly Lutzomyia longipalpis. Med. Vet. Entomol. 9, 211–213. doi: 10.1111/j.1365-2915.1995.tb00182.x
Warren, M., and Wharton, R. (1963). The vectors of simian malaria: identity, biology, and geographical distribution. J. Parasitol. 49, 892–904. doi: 10.2307/3275715
Watsons, M. (1921). The prevention of malaria in the federated Malay states. Liverpool: The Liverpool School of Tropical Medicine
Weeks, P. (1999). Interaction between red-billed oxpeckers, Buphagus erythrorhynchus and domestic cattle, Bos taurus, in Zimbabwe. Anim. Behav. 58, 1253–1259. doi: 10.1006/anbe.1999.1265
Wei, W., Shao, D., Huang, X., Li, J., Chen, H., Zhang, Q., et al. (2006). The pathogenicity of mosquito densovirus (C6/36DNV) and its interaction with dengue virus type II in Aedes albopictus. Am. J. Trop. Med. Hyg. 75, 1118–1126. doi: 10.4269/ajtmh.2006.75.1118
Weidhaas, D. E., Schidmt, C. H., and Seabrook, E. L. (1962). Field studies on the release of sterile males for the control of Anopheles quadrimaculatus. Mosq. News 22, 283–291.
Weitzel, T., Silva-de la Fuente, M. C., Martínez-Valdebenito, C., Stekolnikov, A. A., Pérez, C., Pérez, R., et al. (2022). Novel vector of scrub typhus in sub-Antarctic Chile: evidence from human exposure. Clin. Infect. Dis. 74, 1862–1865. doi: 10.1093/cid/ciab748
Weng, M. H., Lien, J. C., Wang, Y. M., Lin, C. C., Lin, H. C., and Chin, C. (1999). Isolation of Japanese encephalitis virus from mosquitoes collected in northern Taiwan between 1995 and 1996. J. Microbiol. Immunol. Infect. 32, 9–13.
Werner, D., and Pont, A. C. (2003). Dipteran predators of Simuliid blackflies: a worldwide review. Med. Vet. Entomol. 17, 115–132. doi: 10.1046/j.1365-2915.2003.00431.x
Werren, J. H. (1997). Biology of Wolbachia. Annu. Rev. Entomol. 42, 587–609. doi: 10.1146/annurev.ento.42.1.587
Werren, J. H., Baldo, L., and Clark, M. E. (2008). Wolbachia: master manipulators of invertebrate biology. Nat. Rev. Microbiol. 6, 741–751. doi: 10.1038/nrmicro1969
Werren, J. H., Wan, Z., and Li, R. G. (1995). Evolution and phylogeny of Wolbachia: reproductive parasites of arthropods. Proc. R. Soc. Lond. B Biol. Sci. 261, 55–63. doi: 10.1098/rspb.1995.0117
Wheeler, C. M. (1946). Control of typhus in Italy 1943-1944 by use of DDT. Am. J. Public Health 36, 119–129. doi: 10.2105/ajph.36.2.119
WHO (2012). Handbook for integrated vector management. Geneva, Switzerland: World Health Organization.
WHO (2013). Lymphatic filariasis: A handbook of practical entomology for national lymphatic filariasis elimination programs. Italy: WHO.
WHO (2015). Chagas disease in Latin America: an epidemiological update based on 2010 estimates. World Health Organ. Weekly Epidemiol. Record 90, 33–44.
Wilke, A. B. B., Chase, C., Vasquez, C., Carvajal, A., Medina, J., Petrie, W. D., et al. (2019). Urbanization creates diverse aquatic habitats for immature mosquitoes in urban areas. Sci. Rep. 9:15335. doi: 10.1038/s41598-019-51787-5
Wilkerson, R. C., Linton, Y. M., and Strickman, D. (2021). Mosquitoes of the world, Vol 1. Baltimore: John Hopkins University Press.
Wilson, A. L., Courtenay, O., Kelly-Hope, L. A., Scott, T. W., Takken, W., Torr, S. J., et al. (2020). The importance of vector control for the control and elimination of vector-borne diseases. PLoS Negl. Trop. Dis. 14:e0007831. doi: 10.1371/journal.pntd.0007831
Wilson, M. L., Levine, J. F., and Spielman, A. (1985). Mice as reservoirs of the Lyme disease spirochete. Am. J. Trop. Med. Hyg. 34, 355–360. doi: 10.4269/ajtmh.1985.34.355
Windbichler, N., Papathanos, P. A., Catteruccia, F., Ranson, H., Burt, A., and Crisanti, A. (2007). Homing endonuclease mediated gene targeting in Anopheles gambiae cells and embryos. Nucleic Acid Res. 35, 5922–5933. doi: 10.1093/nar/gkm632
Wiwatanaratanabutr, I., and Kittayapong, P. (2009). Effects of crowding and temperature on Wolbachia infection density among life cycle stages of Aedes albopictus. J. Invertebr. Pathol. 102, 220–224. doi: 10.1016/j.jip.2009.08.009
Wong, M. L., Chua, T. H., Leong, C. S., Khaw, L. T., Fornace, K., Wan Yusoff, W. S., et al. (2015). Seasonal and spatial dynamics of the primary vector of plasmodium knowlesi within a major transmission focus in Sabah, Malaysia. PLoS Negl. Trop. Dis. 9:e0004135. doi: 10.1371/journal.pntd.0004135
Woolhouse, M. E., and Gowtage-Sequeria, S. (2005). Host range and emerging and reemerging pathogens. Emerg. Infect. Dis. 11, 1842–1847. doi: 10.3201/eid1112.050997
World Mosquito Program (2022). Global Progress [online]. Available at: https://www.worldmosquito program.org/en/global-progress (Accessed November 13, 2022).
Wu, D., Johnson, J. J., and Federici, B. A. (1994). Synergism of mosquitocidal toxicity between CytA and CryIBD proteins using inclusions produced from cloned genes of bacillus thuringiensis. Mol. Microbiol. 13, 965–972. doi: 10.1111/j.1365-2958.1994.tb00488.x
Wu, M., Sun, L. V., Vamathevan, J., Riegler, M., Deboy, R., Brownlie, J. C., et al. (2004). Phylogenomics of the reproductive parasite Wolbachia pipientis wMel: a streamlined genome overrun by mobile genetic elements. PLoS Biol. 2:e69:E69. doi: 10.1371/journal.pbio.0020069
Wu, W. K., and Tesh, R. B. (1989). Experimental infection of old and New World phlebotomine sandflies (Diptera: Psychodidae) with Ascogregarina chagasi (Eugregarinida: Lecudinidae). J. Med. Entomol. 26, 237–242. doi: 10.1093/jmedent/26.4.237
Xi, Z., Khoo, C. C. H., and Dobson, S. L. (2005). Wolbachia establishment and invasion in an Aedes aegypti laboratory population. Science 310, 326–328. doi: 10.1126/science.1117607
Ximena, J. N., Robert, R. J., and Godfrey, S. (2005). Use of anopheles-specific prey-capture behaviour by the small juveniles of Evarcha culicivora, a mosquito-eating jumping spider. J. Arachnol. 33, 541–548. doi: 10.1636/05-3.1
Yeni, D. K., Büyük, F., Ashraf, A., and Shah, M. S. U. D. (2021). Tularemia: a re-emerging tick-borne infectious disease. Folia Microbiol. 66, 1–14. doi: 10.1007/s12223-020-00827-z
Young, P. R., Ng, L. F. P., Hall, R. A., Smith, D. W., and Johansen, C. A. (2014). “Arbovirus infections” in Manson's tropical infectious diseases. eds. J. Farrar, T. Junghanss, D. Lalloo, P. J. Hotez, G. Kang, and N. J. White. 23rd ed (Philadelphia: Elsevier/Saunders Ltd), 129–161.
Zahedi, M., and White, G. B. (1994). Filaria vector competence of some anopheles species. Trop. Med. Parasitol. 45, 27–32.
Zakaria, N. D., and Avoi, R. (2022). Prevalence and risk factors for positive lymphatic filariasis antibody in Sabah, Malaysia: a cross-sectional study. Trans. R. Soc. Trop. Med. Hyg. 116, 369–374. doi: 10.1093/trstmh/trab132
Zhang, J. L., and Qiao, C. L. (2002). Novel approaches for remediation of pesticide pollutants. Int. J. Environ. Pollut. 18, 423–433. doi: 10.1504/IJEP.2002.002337
Zhao, J., Song, R., Li, H., Zheng, Q., Li, S., Liu, L., et al. (2022). New formulation to accelerate the degradation of pesticide residues: composite nanoparticles of imidacloprid and 24-epibrassinolide. ACS Omega. 7, 29027–29037. doi: 10.1021/acsomega.2c02820
Zheng, X., Zhang, D., Li, Y., Yang, C., Wu, Y., Liang, X., et al. (2019). Incompatible and sterile insect techniques combined eliminate mosquitoes. Nature 572, 56–61. doi: 10.1038/s41586-019-1407-9
Zhioua, E., Lebrun, R. A., Ginsberg, H. S., and Aeschlimann, A. (1995). Pathogeneticity of Steinertma carpocapsae and S. glaseri (Nematoda: Steinernematidae) to Ixodes scapularis (Acari: Ixodidae). J. Med. Entomol. 32, 900–905. doi: 10.1093/jmedent/32.6.900
Zoppi de Roa, E., Gordon, E., Montiel, E., Delgado, L., Berti, J., and Ramos, S. (2002). Association of cyclopoid copepods with the habitat of the malaria vector Anopheles aquasalis in the peninsula of Paria, Venezuela. J. Am. Mosq. Control Assoc. 18, 47–51.
Keywords: zoonoses, vector-borne, vector management, control, prevention
Citation: Wong ML, Zulzahrin Z, Vythilingam I, Lau YL, Sam I-C, Fong MY and Lee W-C (2023) Perspectives of vector management in the control and elimination of vector-borne zoonoses. Front. Microbiol. 14:1135977. doi: 10.3389/fmicb.2023.1135977
Edited by:
Jose G. Estrada-Franco, National Polytechnic Institute, MexicoReviewed by:
Sheng-Qun Deng, Anhui Medical University, ChinaDevojit Kumar Sarma, ICMR-National Institute for Research in Environmental Health, India
Copyright © 2023 Wong, Zulzahrin, Vythilingam, Lau, Sam, Fong and Lee. This is an open-access article distributed under the terms of the Creative Commons Attribution License (CC BY). The use, distribution or reproduction in other forums is permitted, provided the original author(s) and the copyright owner(s) are credited and that the original publication in this journal is cited, in accordance with accepted academic practice. No use, distribution or reproduction is permitted which does not comply with these terms.
*Correspondence: Wenn-Chyau Lee, bGVld2NfODhAaG90bWFpbC5jb20=
†These authors have contributed equally to this work and share first authorship