- 1Department of Biotechnology, The University of Tokyo, Tokyo, Japan
- 2Collaborative Research Institute for Innovative Microbiology, The University of Tokyo, Tokyo, Japan
The filamentous fungus Aspergillus oryzae, in which sexual reproduction remains to be discovered, proliferates mainly via asexual spores (conidia). Therefore, despite its industrial importance in food fermentation and recombinant protein production, breeding beneficial strains by genetic crosses is difficult. In Aspergillus flavus, which is genetically close to A. oryzae, structures known as sclerotia are formed asexually, but they are also related to sexual development. Sclerotia are observed in some A. oryzae strains, although no sclerotia formation has been reported in most strains. A better understanding of the regulatory mechanisms underlying sclerotia formation in A. oryzae may contribute to discover its sexual development. Some factors involved in sclerotia formation have been previously identified, but their regulatory mechanisms have not been well studied in A. oryzae. In this study, we found that copper strongly inhibited sclerotia formation and induced conidiation. Deletion of AobrlA encoding a core regulator of conidiation and ecdR involved in transcriptional induction of AobrlA suppressed the copper-mediated inhibition of sclerotia formation, suggesting that AobrlA induction in response to copper leads not only to conidiation but also to inhibition of sclerotia formation. In addition, deletion of the copper-dependent superoxide dismutase (SOD) gene and its copper chaperone gene partially suppressed such copper-mediated induction of conidiation and inhibition of sclerotia formation, indicating that copper regulates asexual development via the copper-dependent SOD. Taken together, our results demonstrate that copper regulates asexual development, such as sclerotia formation and conidiation, via the copper-dependent SOD and transcriptional induction of AobrlA in A. oryzae.
1. Introduction
Aspergillus oryzae is a filamentous fungus, and numerous strains of A. oryzae are industrially used for the fermentation of traditional Japanese foods, such as sake, miso, and soy sauce. A. oryzae is also used industrially to produce recombinant proteins (Ward, 2012) and recently expected as a host for the production of heterologous secondary metabolites (Alberti et al., 2017). Owing to its industrial importance, the establishment of crossbreeding techniques via sexual development is expected to be useful in generating beneficial strains. However, sexual reproduction remains to be discovered in A. oryzae (Wada et al., 2012), and it proliferates through asexual cycle, in which genetically identical individuals are generated. Therefore, the discovery of sexual reproduction in A. oryzae, which generates genetically diverse strains, would greatly enhance its potential industrial applications.
Asexual filamentous fungi, such as A. oryzae, mainly proliferate via asexual spores called conida. The regulation of conidiation has been well established in Aspergillus nidulans. Conidiation is initiated by the transcriptional induction of the gene for the transcription factor brlA, sequentially activating the downstream genes for transcription factors abaA and then wetA, which are required for proper conidiation (Park and Yu, 2012). These components of the conidiation regulatory pathway are conserved and are also required for conidiation in A. oryzae (Ogawa et al., 2010). In addition to conidiation, several filamentous fungal species belonging to Ascomycota and Basidiomycota asexually develop a hardened mycelial aggregation called the sclerotium, which survives for long periods under unfavorable environmental conditions (Georgiou et al., 2006; Dyer and O’Gorman, 2012). In some Aspergillus species, the sclerotium is closely related to the formation of sexual reproductive structures (Kwon-Chung and Sugui, 2009), and functions as a repository for sexual reproductive structures in Aspergillus flavus, which is genetically close to A. oryzae (Horn et al., 2009; Gibbons et al., 2012). Therefore, the sclerotium would be expected to play a crucial role in sexual reproduction of A. oryzae, if it exists. However, no sclerotia formation has been reported in most A. oryzae industrial strains (Murakami, 1971), and understanding of its regulatory mechanisms will be required to enhance sclerotia formation, which probably contributes to the discovery of sexual reproduction of A. oryzae.
Unlike conidiation, the knowledges about the regulatory mechanism of sclerotia formation are limited. However, several environmental factors have been shown to be involved in sclerotia formation, such as temperature, light, oxygen availability, humidity, pH, and medium composition (Dyer and O’Gorman, 2012). Oxidative stress caused by reactive oxygen species (ROS) plays a crucial role in sclerotia formation (Georgiou et al., 2006). Sclerotia formation in A. oryzae is also known to be affected by environmental conditions; malt extract medium has been used to induce sclerotia formation and potato dextrose medium has been used to induce conidiation (Nakamura et al., 2016). In addition, AoRim15 which is homologous to Rim15, a stress-responsive kinase in Saccharomyces cerevisiae, is involved in sclerotia formation in A. oryzae (Nakamura et al., 2016), suggesting that certain environmental stresses are involved in sclerotia formation. Although the involvement of oxidative stress-responsive pathways in sclerotia formation has been well studied (Georgiou et al., 2006), little is known about the genetic basis underlying the regulation of sclerotia formation. In A. oryzae, two transcription factors, EcdR and SclR, are involved in sclerotia formation (Jin et al., 2011a,b). Deletion of ecdR stimulates sclerotia formation and inhibits conidiation (Jin et al., 2011a). In contrast, deletion of sclR leads to loss of the ability to from sclerotia and dense conidial formation (Jin et al., 2009, 2011b). However, the pathways regulating sclerotia formation that these transcription factors act upon remain unclear. In addition, these phenotypes caused by deletion of ecdR and sclR suggest the relationship between the regulatory mechanisms of sclerotia formation and conidiation.
Copper functions as a cofactor for some enzymes and participates in quite diverse cellular processes, including respiration (cytochrome c oxidase), detoxification of ROS (superoxide dismutase (SOD); (Ruiz et al., 2021), nitrogen utilization (nitrite reductase; Long et al., 2015), iron uptake (ferroxidase; Schrettl et al., 2004), and biosynthesis of secondary metabolites (laccase; Upadhyay et al., 2013). In some Aspergillus species, copper is known to be required for conidial pigmentation (Chang et al., 2019). Moreover, genetic evidence for the involvement of copper in asexual development such as conidiation and sclerotia formation has been established in filamentous fungi. Deletion of Afmac1, encoding a copper-binding transcription factor, results in a conidiation defect in Aspergillus fumigatus (Kusuya et al., 2017). The copper transporter BcCcc2 is required for sclerotia formation in Botrytis cinerea (Saitoh et al., 2010). In addition to this genetic evidence, the effects of copper treatment on sclerotia development have also been reported. Copper has been suggested to inhibit sclerotia formation in the A. oryzae G15 strain (Long et al., 2017) and in an Aspergillus strain (Rogers and Li, 1985). In contrast, copper induces sclerotia formation in Penicillium thomii (Zhang et al., 2014; Zhao et al., 2014). These findings support the conclusion that copper is involved in the asexual development of filamentous fungi. However, high concentrations of copper (> 80 μM) used in these studies possibly cause toxic effects such as oxidative stress (Zhang et al., 2014; Zhao et al., 2014), and physiological effects of copper on asexual development have not been investigated.
In this study, we found that trace amounts of copper (1.6 μM) strongly induced conidiation and inhibited sclerotia formation in A. oryzae, and demonstrated that sclerotia formation is inhibited by AoBrlA, which is critical for conidiation. In addition, the copper-dependent SOD AoSod1 functions in induction of copper-mediated conidiation and in inhibiting of sclerotia formation. These results suggest that activation of SOD by copper leads to the stimulation of AobrlA, resulting in the induction of conidiation and inhibition of sclerotia formation.
2. Materials and methods
2.1. Strains and growth conditions
The A. oryzae, Aspergillus sojae, and Aspergillus luchuensis strains used in this study are listed in Supplementary Table S1. Yeast extract-glucose (YG) medium (5 g/L yeast extract and 10 g/L glucose) and malt extract (ME) medium [20 g/L malt extract (ORIENTAL YEAST Co., Ltd., Tokyo, Japan), 20 g/L glucose, and 1 g/L HIPOLYPEPTON (FUJIFILM Wako Pure Chemical Co., Osaka, Japan)] were used for the growth of A. oryzae. To investigate the effects of metal ions, 0.1% trace elements solution (30.56 mM ZnSO4·7H2O, 1.60 mM CuSO4·5H2O, 0.36 mM FeSO4·7H2O, 0.67 mM MnSO4·4H2O, 0.26 mM Na2B4O7·10H2O, and 0.04 mM (NH4)6Mo7O24·4H2O; Rowlands and Turner, 1973) and each component of the trace elements solution were added. To investigate the effect of copper, 0.1% CuCl2 solution (1.60 mM) was added. To test growth, conidiation, and sclerotia formation, conidial suspensions (1 × 104/5 μL) were spotted onto the agar medium and incubated in the dark at 30°C. The ΔecdR, ΔAobrlA, ΔAoabaA, and ΔAowetA mutants hardly formed conidia, and the mycelial mass instead of the conidial suspension was inoculated onto the agar medium.
2.2. Transformation of Aspergillus oryzae
Classical transformation and transformation using genome editing of A. oryzae were performed as previously described (Maruyama and Kitamoto, 2011; Katayama et al., 2019). Dextrin-peptone-yeast extract (DPY) medium (20 g/L dextrin, 10 g/L polypeptone, 5 g/L yeast extract, 5 g/L KH2PO4, and 0.5 g/L MgSO4·7H2O) or dextrin-peptone (DP) medium (20 g/L dextrin, 10 g/L polypeptone, 5 g/L KH2PO4, and 0.5 g/L MgSO4·7H2O) were used for pre-culture. Czapek-dox (CD) medium (3 g/L NaNO3, 2 g/L KCl, 1 g/L KH2PO4, 0.5 g/L MgSO4·7H2O, 0.002 g/L FeSO4·7H2O, and 20 g/L glucose [pH 5.5]) was used to select the transformants. For selection using the pyrithiamine-resistant ptrA marker, 0.1 μg/ml pyrithiamine was added. To remove the genome-editing plasmid from the transformants, dextrin was added to CD medium instead of glucose. To positively select sC and niaD mutants, the selenate medium (3 g/L NaNO3, 2 g/L KCl, 1 g/L KH2PO4, 0.5 g/L MgCl2, 20 g/L glucose, 30 mg/L D-methionine, and 9.5 mg/L sodium selenate [pH 5.5]) and the chlorate medium (1.31 g/L leucine, 2 g/L KCl, 1 g/L KH2PO4, 0.5 g/L MgSO4·7H2O, 0.002 g/L FeSO4·7H2O, 20 g/L glucose, 0.015 g/L methionine, and 57.6 g/L KClO3 [pH 5.5]) were used, respectively. For the growth of niaD mutants, 3 g/L NH4Cl was added to CD medium instead of NaNO3. For the growth of sC mutants, 0.015 g/L methionine was added to CD medium. For the growth of pyroA mutants, 0.5 mg/L pyridoxine hydrochloride was added.
2.3. DNA manipulation
Escherichia coli DH5α strain was used for DNA manipulation. Polymerase chain reaction (PCR) for plasmid construction was performed using KOD-Plus-Neo (TOYOBO, Osaka, Japan). The In-Fusion HD Cloning kit (TaKaRa Bio, Ohtsu, Japan) and the seamless ligation cloning extract (SLiCE) method (Okegawa and Motohashi, 2015a,b) were used for plasmid construction. Genomic PCR was performed using KOD FX Neo (TOYOBO). Primers used in this study are listed in Supplementary Table S2.
2.4. Total DNA extraction from Aspergillus oryzae strains and southern blot analysis
Total DNA extraction from A. oryzae strains and Southern blot analysis were performed as previously described (Maruyama and Kitamoto, 2011).
2.5. Construction of plasmids generating control strains for genetic modification
The U6 promoter with a target sequence for ku70 deletion was amplified from pRGE-gwAup (Katayama et al., 2019) using SmaIIF1-PU6-F2nd/gku70-PU6R primers and ligated with SmaI-digested pRGE-gRT6 (Katayama et al., 2019), yielding a genome-editing plasmid pRGE-gku70. The upstream and downstream flanking regions of ku70 were amplified from RIB40 genomic DNA using 19IF-ku70-5F/ku70-5R and ku70-3F/19IF-ku70-3R primer sets, respectively. These fragments were ligated with BamHI-digested pUC19 (TaKaRa Bio), yielding the donor plasmid pΔku70. The RIB40Δku5–2 strain was constructed by co-introducing pRGE-gku70 and pΔku70 into RIB40. Deletion of ku70 and no unexpected integration of the donor plasmid were confirmed by Southern blot analysis (Supplementary Figure S1A). The genome-editing plasmid was then removed from the Δku70 strain by subculturing on the CD medium containing dextrin instead of glucose.
The R40KS strain was constructed from RIB40Δku5–2 by introducing NotI-digested psCΔ5 (Katayama et al., 2021). The sC− mutants were selected on the selenate medium as sC− mutants exhibit selenate resistance (Yamada et al., 1997), and the deletion of the upstream and 5′ regions of sC was confirmed by genomic PCR (Supplementary Figure S1B).
The R40KSN strain was constructed from the R40KS strain by introducing a DNA fragment amplified from CDK1 (Katayama et al., 2021) genomic DNA using DniaD-F/DniaD-R primers. The niaD− mutants were selected on chlorate medium as niaD− mutants exhibited chlorate resistance (Ishi et al., 2005), and the deletion of the 3′ and downstream regions of niaD was confirmed by genomic PCR (Supplementary Figure S1C).
The U6 promoter with a target sequence for pyroA deletion was amplified from pRGE-gwAup using SmaIIF1-PU6-F2nd/gpyroA-PU6R primers and ligated with SmaI-digested pRGE-gRT6, yielding the genome-editing plasmid pRGE-gpyroA. The upstream and downstream flanking regions of pyroA and the pyrG marker were amplified from RIB40 genomic DNA using 19IF-pyroA5F/pyrG-pyroA5R, pyrG-pyroA3F/19IF-pyroA3R, and pyrGF/pyrGR primer sets, respectively. These DNA fragments were ligated into BamHI-digested pUC19, yielding pΔpyroA-pyrG. To remove the pyrG marker from pΔpyroA-pyrG, the plasmid DNA was digested with XhoI and self-ligated to yield pΔpyroA. Strain R40KSNP was constructed by co-introducing pRGE-gpyroA and pΔpyroA into the R40KSN strain. The pyroA deletion was confirmed using genomic PCR (Supplementary Figure S1D). The genome-editing plasmid was then removed from the transformant by subculturing on the CD medium containing dextrin instead of glucose.
2.6. Construction of gene deletion strains
For gene deletion, the approximate 1-kb flanking regions of the target gene were amplified from RIB40 genomic DNA using the primers listed in Supplementary Table S2. These fragments were then integrated into BamHI-digested pUC19 together with the pyroA marker amplified from RIB40 genomic DNA using AopyroAF/R primers. The DNA fragment for gene deletion was amplified from the resulting plasmid and introduced into the R40KSNP strain. Deletion of the target gene was confirmed by genomic PCR (Supplementary Figure S2).
2.7. Construction of complemented strains
To reintroduce AobrlA into the AobrlA deletion mutant, AobrlA gene was integrated into its native locus, where its ORF was replaced with the pyroA marker. For plasmid construction, the upstream region and ORF of AobrlA were amplified from RIB40 genomic DNA using brlA-1/sC-brlA-R primers. The sC marker was amplified from RIB40 genomic DNA using sC-F/pyroA-sC-R primers. The 5′ region of the pyroA marker was amplified from RIB40 genomic DNA using AopyroAF/19IF-pyroAmid-R primers. These fragments were ligated with BamHI-digested pUC19. The DNA fragment amplified from the yielding plasmid using brlA-1/19IF-pyroAmid-R primers was introduced into the AobrlA deletion mutant. Integration of the introduced DNA fragment into the AobrlA locus was confirmed using genomic PCR (Supplementary Figure S3A).
For complementation of sC, NotI-digested pisC (Mamun et al., 2020) was introduced into the sC− strains, and the integration of the introduced DNA fragment into the sC locus was confirmed by genomic PCR.
To reintroduce Aosod1 and AoccsA into the corresponding deletion mutants, their promoter, ORF, and terminator regions were amplified from RIB40 genomic DNA using the primers listed in Supplementary Table S2, and then ligated with XhoI-digested pUXN (Mori et al., 2019). The resulting plasmids were digested with NotI and introduced into the corresponding deletion mutants. Integration of the introduced DNA fragment into the niaD locus was confirmed using genomic PCR (Supplementary Figures S3B,C).
For complementation of niaD, NotI-digested pUXN was introduced into the niaD− strains, and integration of the introduced DNA fragment into the niaD locus was confirmed by genomic PCR.
2.8. Transcriptional analysis
Mycelia were collected from agar plates and homogenized using a multibead shocker (Yasui Kikai, Osaka, Japan). Total RNA was extracted from the homogenized mycelia, and cDNA synthesis and qRT-PCR were performed as described previously (Katayama et al., 2019). Primers used for qRT-PCR are listed in Supplementary Table S2.
2.9. Stereomicroscopy
Stereomicroscopy was performed using a stereomicroscope (VB-G25/VB-7010; Keyence Co., Ltd., Osaka, Japan).
2.10. Statistical analysis
The results of three independent experiments are shown as mean values, and error bars represent standard deviation (SD), as indicated in the figure legends. Statistical significance was tested using a two-sample Student’s t test in Microsoft Excel, and the results are indicated as *p < 0.05, or **p < 0.01. Tukey-Cramer multiple comparison was performed using software “R” version 4. 0. 3, with a significance level (p < 0.05).
3. Results
3.1. Trace copper induces conidiation and suppresses sclerotia formation
To explore the conditions under which sclerotia formation is induced, we incubated the sclerotiogenic A. oryzae RIB40 strain (Murakami, 1971) on various agar media and found that numerous sclerotia were formed on the YG agar medium (Figure 1A). Stereomicroscopic observation also showed sclerotia formation and sparsely formed conidiophores on the YG agar medium (Figure 1B). In contrast, sclerotia formation was hardly detected, and dense conidiophore formation was observed on YG agar medium supplemented with the trace elements solution, which are often used to prepare media for Aspergillus nidulans (Fukuda et al., 2009; Figures 1A,B). Supplementation with trace elements solution significantly increased the conidiation efficiency without affecting growth (p < 0.01; Figures 1C,D). These results suggest the involvement of a component(s) of the trace elements solution in asexual development of A. oryzae. When the components of the trace elements solution were separately added to the YG medium, supplementation with 1.6 μM CuSO4·5H2O was found to strongly inhibit sclerotia formation and induce conidiation without affecting the growth, similar to what was observed upon supplementation with the trace elements solution (Figures 1C–E). Supplementation with 1.6 μM CuCl2 had indistinguishable effects on development (Figure 1F). These results indicate that trace copper affects asexual development on YG agar medium. To investigate the effects of copper on media other than YG medium, CuSO4·5H2O was added to ME agar medium, on which sclerotia formation is induced (Nakamura et al., 2016). Sclerotia formation was also inhibited on this medium, and conidiation was induced by supplementing CuSO4·5H2O to the ME agar medium as well as YG agar medium (Supplementary Figure S4). Taken together, these results indicate that trace copper induces conidiation and inhibits sclerotia formation in A. oryzae.
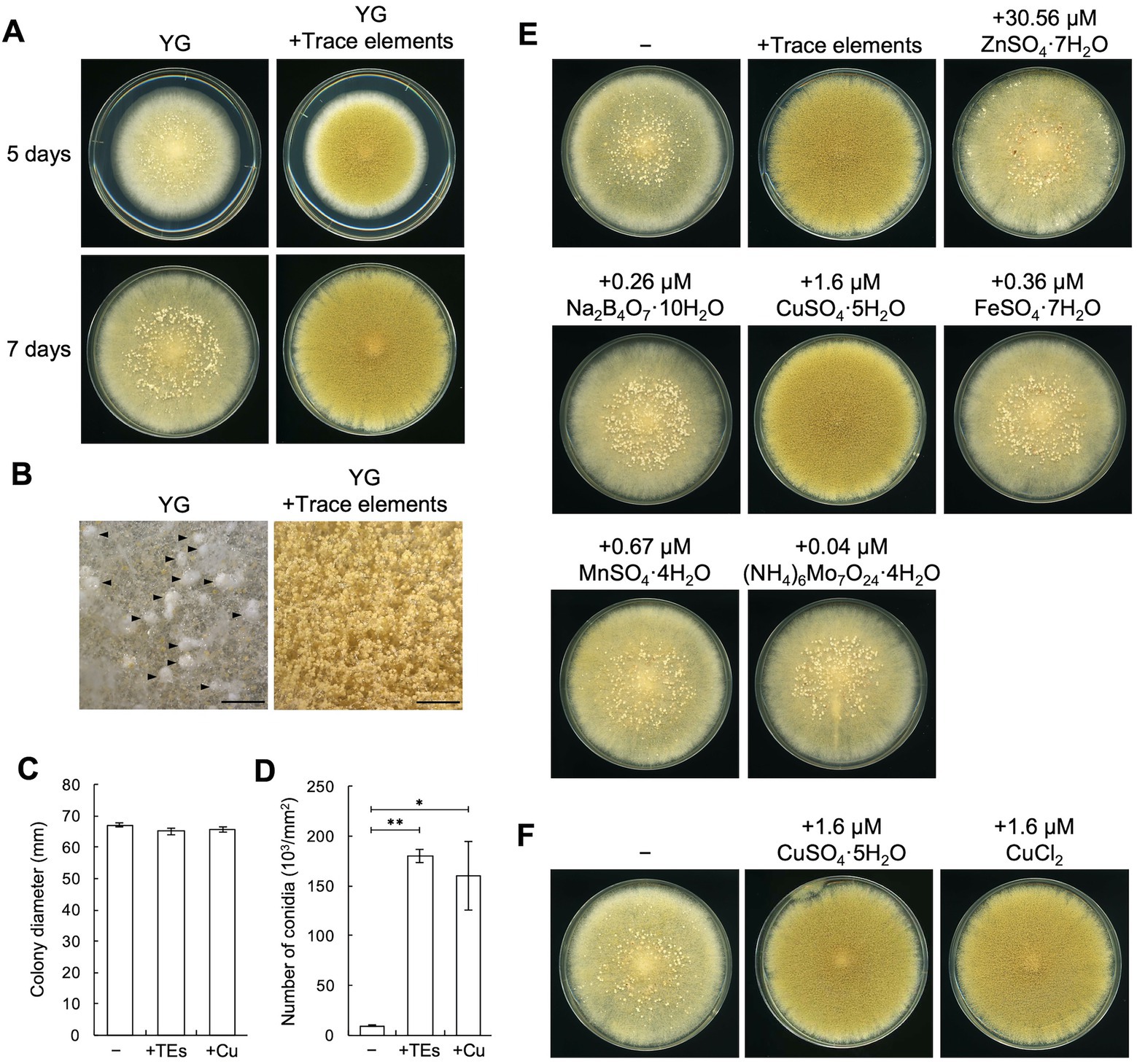
Figure 1. Copper induces conidiation and suppresses sclerotia formation. (A) Conidial suspensions (1 × 104/5 μL) of the RIB40 strain were inoculated onto YG agar media with or without trace elements solution and incubated in dark at 30°C for 5 or 7 days. Sclerotia were observed as the white mass structures around the center of the colonies. (B) Stereomicroscopic images of the colonies shown as 5 days in panel A. Arrowheads indicate sclerotia. Bars: 2 mm. (C,D) Effect of the trace elements solution and CuSO4·5H2O on the growth and conidiation efficiency. Conidial suspensions (1 × 104/5 μL) of the RIB40 strain were inoculated onto YG (−), YG containing trace elements solution (+TEs), and YG containing 1.6 μM CuSO4·5H2O (+Cu), and then incubated in dark at 30°C for 5 days. Colony diameter (C) and number of conidia (D) were measured. Data are shown as means ± SD of three independent experiments. *p < 0.05, **p < 0.01 by Student’s t-test. (E) Conidial suspensions (1 × 104/5 μL) of the RIB40 strain were inoculated onto YG agar media containing the indicated metals and incubated in dark at 30°C for 7 days. (F) Conidial suspensions (1 × 104/5 μL) of the RIB40 strain were inoculated onto YG agar media containing the indicated metals and incubated in dark at 30°C for 7 days.
3.2. Copper affects asexual development in Aspergillus oryzae industrial strains, Aspergillus sojae, and Aspergillus luchuensis
To investigate the generality of the copper-mediated effects on asexual development, A. oryzae industrial strains and other Aspergillus species, such as Aspergillus sojae and Aspergillus luchuensis were incubated on YG agar media with or without copper. In the sclerotiogenic A. oryzae TK-32 and TK-38 strains, numerous sclerotia were formed on the YG agar medium, whereas copper supplementation inhibited sclerotia formation and strongly induced conidiation, as observed in the RIB40 strain (Supplementary Figures S5A,B). The A. oryzae TK-41, A. sojae NBRC4239, and A. luchuensis NBRC4314 strains did not form sclerotia on the tested media, whereas their conidiation was also induced by copper (Supplementary Figures S5A,B). These results indicate that copper affects asexual development in A. oryzae industrial strains, A. sojae, and A. luchuensis.
3.3. Copper affects the expression of asexual development-related genes
Considering our observation that trace copper drastically changes asexual development, such as conidiation and sclerotia formation, we hypothesized that supplementation with copper might affect the expression of asexual development-related genes. In several Aspergillus species, activation of the central regulatory pathway, composed sequentially of brlA, abaA, and wetA, plays critical roles in conidiation (Park and Yu, 2012). AobrlA, AoabaA, and AowetA homologous to these genes are also required for proper conidiation in A. oryzae (Ogawa et al., 2010). The sspA gene, which is homologous to ssp1 that encodes a major protein present in the mature sclerotia of Sclerotinia sclerotium (Li and Rollins, 2009), was upregulated in A. oryzae mutants that form an increased number of sclerotia (Jin et al., 2011a,b). In concordance with the developmental changes caused by copper, such as the induction of conidiation and inhibition of sclerotia formation, the mRNA levels of AobrlA, AoabaA, and AowetA were strongly upregulated, and that of sspA was downregulated by copper supplementation (Figure 2). These results suggest that trace copper affects the expression of the asexual development-related genes, resulting in induction of conidiation and inhibition of sclerotia formation.
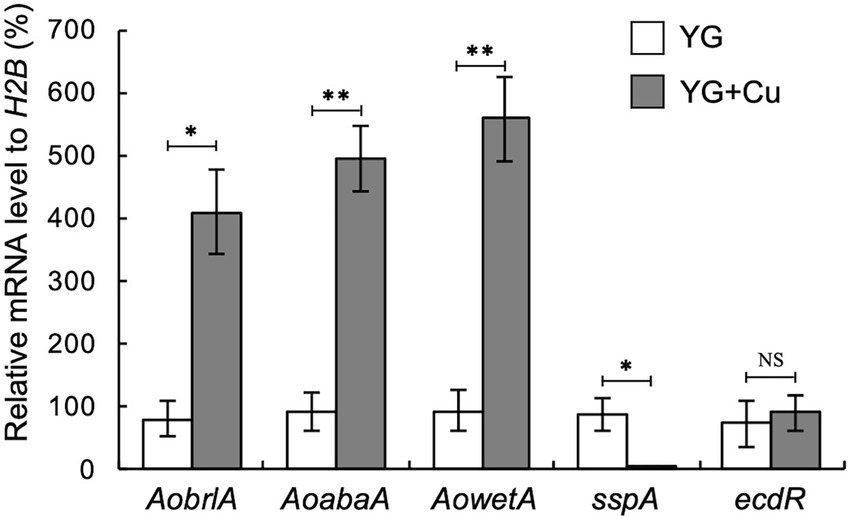
Figure 2. Copper stimulates expression of AobrlA, AoabaA, and AowetA and suppresses sspA. Conidial suspension (1 × 104/5 μL) of the RIB40 strain was spotted onto YG and YG containing 1.6 μM CuSO4·5H2O (YG + Cu) and incubated in dark at 30°C for 5 days. Total RNA was extracted from collected mycelia, and then qRT-PCR for the indicated genes was performed. Data are shown as means ± SD of three independent experiments. *p < 0.05, **p < 0.01 by Student’s t-test. NS: Not significant.
3.4. AoBrlA has an inhibitory function in sclerotia formation
Conidiation was induced and sclerotia formation was inhibited in the presence of copper (Figures 1D–F). In contrast, conidiation was reduced when sclerotia formation was induced (Figure 1D). Such phenotypes suggest a mutually exclusive relationship between conidiation and sclerotia formation. Additionally, the expression of AobrlA, AoabaA, and AowetA was downregulated when sclerotia formation was induced (Figure 2). Therefore, the expression of these genes might be involved in sclerotia formation. To investigate this possibility, sclerotia formation was examined in the deletion mutants of AobrlA, AoabaA, and AowetA. As speculated, the AobrlA and AoabaA deletion mutants showed increased numbers of sclerotia in the presence of copper, although induction of sclerotia formation was not detected in the AowetA deletion mutant (Figures 3A,B). According to this observation, the sspA gene was upregulated in the AobrlA and AoabaA deletion mutants (Figure 3C). However, as the increased level of sspA expression in the AoabaA deletion mutant was lower than that in the AobrlA deletion mutant (Figure 3C), AoBrlA but not AoAbaA functions as the main factor regulating the copper-mediated inhibition of sclerotia formation. Increased sclerotia formation in the AobrlA deletion mutant was suppressed by reintroducing the AobrlA gene (Supplementary Figure S6A). These results indicated that AoBrlA inhibit sclerotia formation.
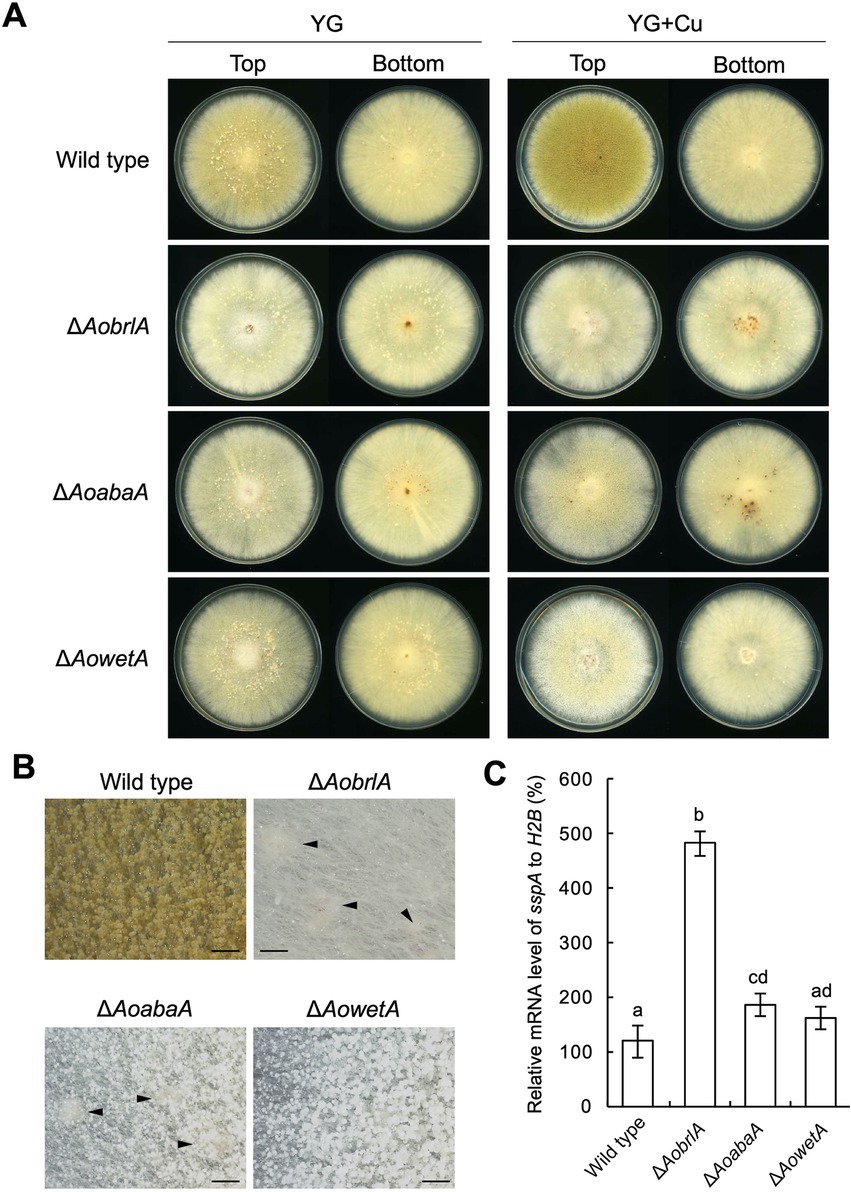
Figure 3. AoBrlA is required for the copper-mediated inhibition of sclerotia formation. (A) Conidial suspension (1 × 104/5 μL) of the wild-type strain was spotted, and the mycelial mass of the indicated deletion strains was put onto YG and YG containing 1.6 μM CuSO4·5H2O (YG + Cu). They were incubated in dark at 30°C for 7 days. The top and bottom side pictures of the plates are shown as “Top” and “Bottom,” respectively. Sclerotia were detected as white or pigmented dots when observed from the bottom side of the plates. (B) Stereomicroscopic images of the colonies grown on YG containing 1.6 μM CuSO4·5H2O shown in panel A. Arrowheads indicate sclerotia. Bars: 1 mm. (C) The relative mRNA level of the sspA gene to that of H2B.The indicated strains were inoculated onto the YG agar media supplemented with 1.6 μM CuSO4·5H2O as described in panel A and incubated in dark at 30°C for 5 days. Data are shown as means ± SD of three independent experiments. Data are analyzed using Tukey’s multiple comparison test, and means sharing the same letter are not significantly different (p > 0.05).
3.5. EcdR is required for the developmental response caused by copper
The transcription factor EcdR was previously suggested to be involved in the induction of AobrlA, and its deletion results in little conidiation and enhanced sclerotia formation (Jin et al., 2011a). Considering the inhibitory function of AoBrlA in sclerotia formation, EcdR may be involved in the inhibition of sclerotia formation by copper. As expected, the ecdR deletion mutant displayed numerous sclerotia and hardly formed conidia on the YG agar medium containing copper (Figure 4A). According to such developmental phenotypes, AobrlA, AoabaA, and AowetA were not induced, and sspA was not repressed in the ecdR deletion mutant grown on media supplemented with copper (Figure 4B). These results indicate that EcdR regulates the expression of AobrlA to inhibit sclerotia formation and induce conidiation in response to copper.
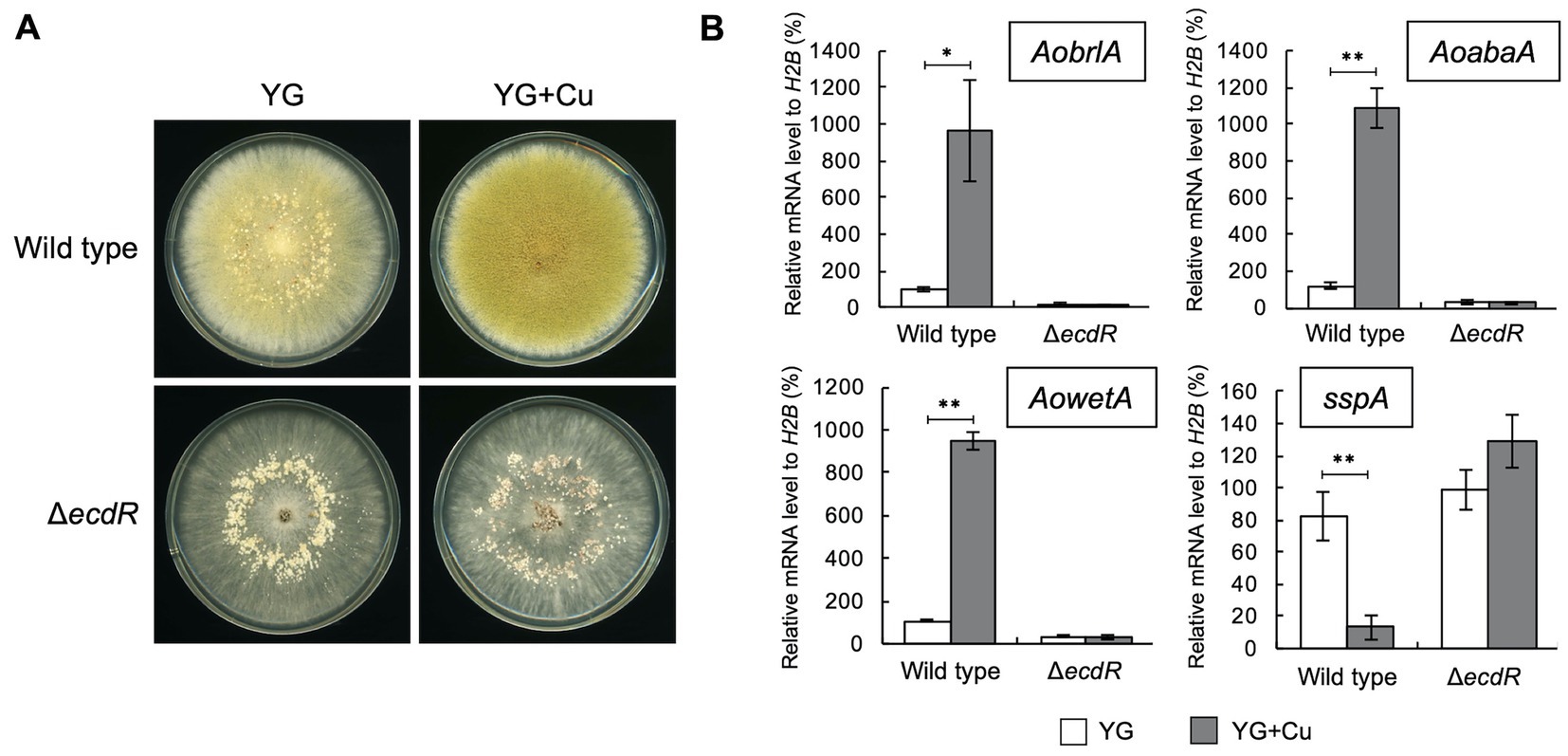
Figure 4. EcdR is required for the increased conidiation and decreased sclerotia formation in response to copper. (A) Conidial suspension (1 × 104/5 μL) of the wild type strain was spotted and the mycelial mass of the ecdR deletion strain was put onto YG and YG containing 1.6 μM CuSO4·5H2O (YG + Cu). They were incubated in dark at 30°C for 7 days. (B) The relative mRNA levels of the indicated genes to that of H2B. Conidial suspension (1 × 104/5 μL) of the wild type strain was spotted and the mycelial mass of the ecdR deletion strain was put onto YG and YG containing 1.6 μM CuSO4·5H2O (YG + Cu) and incubated in dark at 30°C for 5 days. Data are shown as means ± SD of three independent experiments. *p < 0.05, **p < 0.01 by Student’s t-test.
3.6. Copper-dependent superoxide dismutase is involved in the copper-mediated developmental regulation
As the presence of trace copper affects conidiation and sclerotia formation, we hypothesized that proteins related to copper homeostasis are involved in developmental regulation. As the copper-binding proteins with roles in copper homeostasis in A. fumigatus and yeasts have been previously listed (Wiemann et al., 2017), potential homologs to these copper-binding proteins were identified in the proteins encoded in the genome sequence of A. oryzae. In this study, copper transporters were not examined, but rather the focus was on determining the intracellular response to copper. The predicted copper-binding transcription factors AO090003000161 and AO090701001154 and a predicted Cu/Zn superoxide dismutase (SOD) AO090020000521 were identified by BLASTp analyses, and they were designated as AoAceA, AoMacA, and AoSod1, respectively (Table 1). Although proteins homologous to the copper-binding transcription factor CufA and the copper metallothionein CmtA of A. fumigatus were not found by BLASTp analysis, nucleotide sequences predicted to encode these proteins were found by tBLASTn analysis. The cufA homologous gene was predicted at Chr5:1,300,449-1,301,358 with two introns, and the cmtA homologous gene was predicted at Chr7:2,741,077-2,741,532 with two introns. Therefore, we designated these genes as AocufA and AocmtA, respectively (Table 1).
To investigate the involvement of such copper-binding proteins in the copper-mediated regulation of asexual development, deletion mutants of the genes encoding them were constructed and incubated on YG agar medium with or without copper. As the Aomac1 deletion mutant exhibited growth defects, its relationship with the developmental regulation was not investigated (Supplementary Figure S7). In the presence of copper, the Aosod1 deletion mutant formed numerous sclerotia (Figure 5A), whereas the other deletion mutants did not form sclerotia (Supplementary Figure S7), suggesting that AoSod1 inhibits sclerotia formation. In S. cerevisiae and A. fumigatus, activation of Sod1 requires the copper chaperone Ccs1/CcsA, which introduces copper ions into Sod1 (Skopp et al., 2019; Du et al., 2021). Therefore, a copper chaperone for AoSod1 might be involved in the inhibition of sclerotia formation in A. oryzae. BLASTp analysis using the CcsA sequence of A. fumigatus showed AO090011000670 as its homologous protein in A. oryzae, which was designated as AoCcsA. The AoccsA deletion mutant formed numerous sclerotia in the presence of copper, as did the Aosod1 deletion mutant (Figure 5A). In addition, the conidiation efficiencies of the Aosod1 and AoccsA deletion mutants were reduced compared with that of the wild-type strain in the presence of copper (Figure 5B). Enhanced sclerotia formation and decreased conidiation of the Aosod1 and AoccsA deletion mutants were suppressed by reintroducing these genes (Supplementary Figures S6B,C). These results indicate that AoSod1 and AoCcsA are involved in inhibition of sclerotia formation and induction of conidiation in response to copper. On the other hand, since conidiation was still induced by supplementation with copper in the Aosod1 and AoccsA deletion mutants (Figure 5B), factors other than AoSod1 and AoCcsA may be involved in the induction of conidiation by copper.
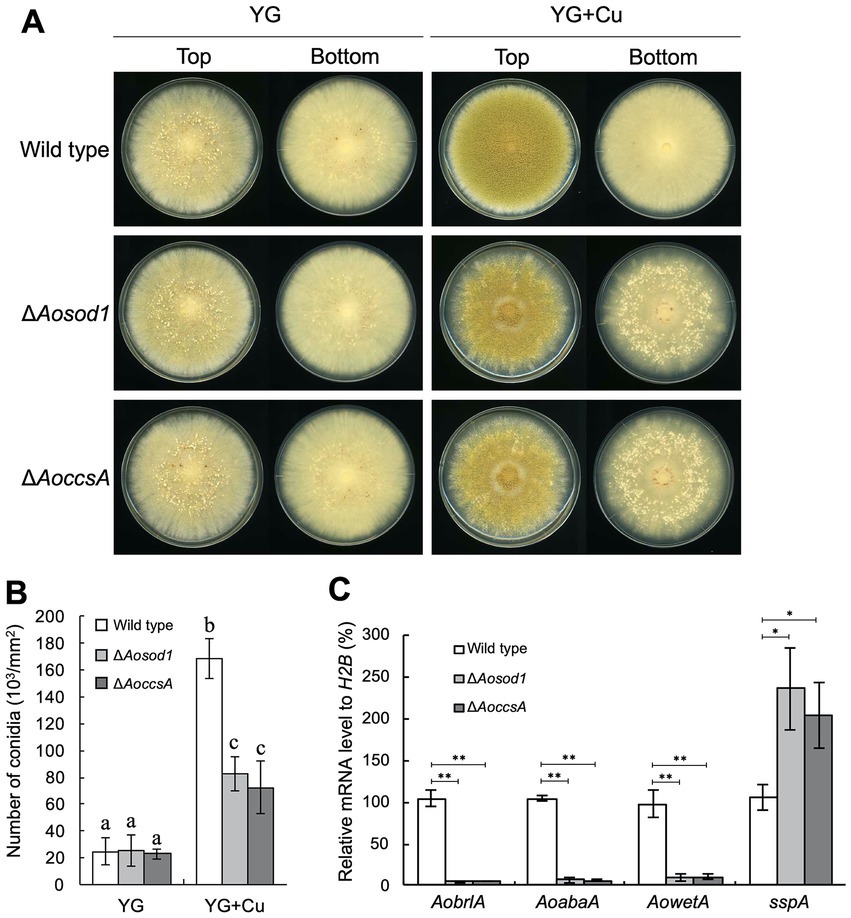
Figure 5. AoSod1 and AoCcsA are involved in copper-mediated developmental regulation. (A) Conidial suspension (1 × 104/5 μL) of the indicated strains was spotted onto YG and YG containing 1.6 μM CuSO4·5H2O (YG + Cu) and incubated in dark at 30°C for 7 days. Sclerotia were detected as white or pigmented particles when observed from the bottom side of the plates. (B) Conidiation efficiencies of the Aosod1 and AoccsA deletion mutants. Conidial suspensions (1 × 104/5 μL) of the indicated strains were inoculated onto YG and YG containing 1.6 μM CuSO4·5H2O (YG + Cu), and then incubated in dark at 30°C for 7 days. Data are shown as means ± SD of three independent experiments. Data are analyzed using Tukey’s multiple comparison test, and means sharing the same letter are not significantly different (p > 0.05). (C) The relative mRNA levels of the indicated genes to that of H2B. Conidial suspension (1 × 104/5 μL) of the indicated strains was spotted onto YG agar medium with 1.6 μM CuSO4·5H2O and incubated in dark at 30°C for 7 days. Data are shown as means ± SD of three independent experiments. *p < 0.05, **p < 0.01 by Student’s t-test.
To investigate how AoSod1 and AoCcsA mediate conidiation and sclerotia formation, a transcriptional analysis of AobrlA, AoabaA, AowetA, and sspA was performed under the condition of being supplemented with copper. In the Aosod1 and AoccsA deletion mutants, the mRNA levels of AobrlA, AoabaA, and AowetA were downregulated (p < 0.01), and those of sspA were significantly induced (p < 0.05; Figure 5C). These results indicate that AoSod1 and AoCcsA affect the expression of genes involved in asexual development to induce conidiation and inhibit sclerotia formation in the presence of copper.
4. Discussion
In the present study, we found that trace copper regulates asexual development in A. oryzae. Induction of conidiation by copper was observed in the A. oryzae strains, as well as in A. sojae and A. luchuensis strains (Figure 1 and Supplementary Figure S5). Conidiation defects have been reported in deletion mutants of Afmac1, which encodes a copper-responsive transcription factor in A. fumigatus (Kusuya et al., 2017) and Bcccc2, which encodes a copper-transporting ATPase in B. cinerea (Saitoh et al., 2010). These findings suggest that copper induces conidiation in filamentous fungi. In contrast, copper strongly inhibited the sclerotia formation in A. oryzae (Figure 1). High concentrations of copper have also been reported to inhibit sclerotia formation in A. oryzae G15 strain (Long et al., 2017) and an Aspergillus strain (Rogers and Li, 1985). These findings indicate the inhibitory effects of copper on sclerotia formation in A. oryzae. In contrast to the inhibitory effects of copper on sclerotia formation, copper has been reported to induce sclerotia formation in P. thomii (Zhang et al., 2014; Zhao et al., 2014), and the Bcccc2 deletion abolished sclerotia formation in B. cinerea (Saitoh et al., 2010). Therefore, the effects of copper on sclerotia formation vary in filamentous fungi.
The deletion mutant of AobrlA, which plays a critical role in conidiation, showed enhanced sclerotia formation and increased expression of sspA in the presence of copper (Figure 3), indicating that AoBrlA has inhibitory functions in sclerotia formation. The enhanced sclerotia formation in the AobrlA deletion mutant was suppressed by the reintroduction of AobrlA, supporting the inhibitory functions of AoBrlA in sclerotia formation. Additionally, in A. flavus which is genetically close to A. oryzae, deletion of fluG results in delayed induction of brlA and increased sclerotia formation (Chang et al., 2012), and brlA is downregulated under the sclerotial state (Wu et al., 2014). These findings also support the interpretation that AoBrlA has inhibitory functions in sclerotia formation in A. oryzae. However, it was previously reported that deletion of AobrlA did not enhance sclerotia formation under the condition where sclerotia formation was induced (Ogawa et al., 2010). Considering these findings, AoBrlA is thought to function as an inhibitor of sclerotia formation in the presence of trace copper but not in the condition inducing sclerotia formation (Figure 6). Moreover, the expression of AobrlA was induced in response to copper (Figure 2), suggesting that copper stimulates the expression of AobrlA to induce conidiation and inhibit sclerotia formation (Figure 6).

Figure 6. The predicted model of the asexual developmental regulation in response to copper in A. oryzae. The copper chaperone AoCcsA introduces copper into the superoxide dismutase AoSod1. AoSod1 activated by copper then scavenges intracellular ROS, which is predicted to interfere the AobrlA induction by EcdR as shown with the dashed line, resulting in induction of conidiation and inhibition of sclerotia formation.
The ecdR deletion mutant formed a large number of sclerotia, even in the presence of copper (Figure 4A), indicating that EcdR is required for the copper-mediated inhibition of sclerotia formation. As EcdR was suggested to be an inducer of AobrlA at the early stage of conidiation (Jin et al., 2011a), it is suggested that copper stimulates transcriptional induction of AobrlA by EcdR to induce conidiation and inhibit sclerotia formation (Figure 6). However, it is unclear how copper affects induction of AobrlA by EcdR. As copper supplementation did not affect ecdR expression (Figure 2), copper-mediated developmental regulation does not seem to be dependent on a transcriptional change of ecdR.
The absence of cupper-dependent SOD AoSod1 and its copper chaperone AoCcsA inhibited conidiation and downregulated the expression of conidiation-related genes, such as AobrlA, AoabaA, and AowetA (Figure 5). In addition, deletion of Aosod1 and AoccsA resulted in enhanced sclerotia formation in the presence of copper (Figure 5A), which is thought to be caused by the downregulation of AobrlA. SOD scavenges ROS (Staerck et al., 2017), and deletion of sodA and ccsA leads to increased ROS accumulation in A. fumigatus (Du et al., 2021). Therefore, it is suggested that copper may stimulate AoSod1 to scavenge intracellular ROS, and that intracellular ROS accumulation in the absence of AoSod1 or AoCcsA may inhibit AobrlA induction in A. oryzae, resulting in inhibited conidiation and an induction of sclerotia formation (Figure 6). The possible induction of sclerotia formation by ROS accumulation led to the speculation that oxidative stress affects sclerotia formation. However, treatment with oxidative stress-inducing agents did not stimulate sclerotia formation in the presence of copper (Supplementary Figure S8). This result is consistent with a previous report that treatment with the oxidative stress agent did not activate sclerotia formation (Papapostolou et al., 2014). Although the effects of copper and Aosod1 deletion on ROS accumulation remain unclear, complex mechanisms besides ROS may be involved in the copper-mediated inhibition of sclerotia formation.
To date, the molecular mechanisms underlying changes in asexual development caused by environmental cues have not been well elucidated in A. oryzae. The present study demonstrating that trace copper mediates regulation of asexual development by inducing SOD and AobrlA will be greatly helpful in understanding such mechanisms in A. oryzae. As the involvements of these factors in sclerotia formation have not been reported, this study provides new insights into the regulatory mechanism of sclerotia formation. Therefore, our findings are expected to contribute to the enhancement of sclerotia formation and the ongoing search for sexual reproduction in A. oryzae.
Data availability statement
The original contributions presented in the study are included in the article/Supplementary material, further inquiries can be directed to the corresponding author.
Author contributions
TK and J-iM conceived and designed the research and wrote the manuscript. TK performed the experiments. All authors contributed to the article and approved the submitted version.
Funding
This research was funded by Japan Society for the Promotion of Science (JSPS) KAKENHI grant number 17 K15242, Grant-in-Aid for Young Scientists (B), 20 K15429, Grant-in-Aid for Early-Career Scientists, 18H02123, Grant-in-Aid for Scientific Research (B), and 21H02098, Grant-in-Aid for Scientific Research (B).
Conflict of interest
The authors declare that the research was conducted in the absence of any commercial or financial relationships that could be construed as a potential conflict of interest.
Publisher’s note
All claims expressed in this article are solely those of the authors and do not necessarily represent those of their affiliated organizations, or those of the publisher, the editors and the reviewers. Any product that may be evaluated in this article, or claim that may be made by its manufacturer, is not guaranteed or endorsed by the publisher.
Supplementary material
The Supplementary material for this article can be found online at: https://www.frontiersin.org/articles/10.3389/fmicb.2023.1135012/full#supplementary-material
References
Alberti, F., Foster, G. D., and Bailey, A. M. (2017). Natural products from filamentous fungi and production by heterologous expression. Appl. Microbiol. Biotechnol. 101, 493–500. doi: 10.1007/s00253-016-8034-2
Chang, P. K., Scharfenstein, L. L., Mack, B., and Ehrlich, K. C. (2012). Deletion of the Aspergillus flavus orthologue of A. nidulans flu G reduces conidiatin and promotes production of sclerotia but does not abolish aflatoxin biosynthesis. Appl. Environ. Microbiol. 78, 7557–7563. doi: 10.1128/AEM.01241-12
Chang, P. K., Scharfenstein, L. L., Mack, B., Wei, Q., Gilbert, M., Lebar, M., et al. (2019). Identification of a copper-transporting ATPase involved in biosynthesis of A. flavus conidial pigment. Appl. Microbiol. Biotechnol. 103, 4889–4897. doi: 10.1007/s00253-019-09820-0
Du, W., Zhai, P., Liu, S., Zhang, Y., and Lu, L. (2021). The copper chaperone ccs a, coupled with superoxide dismutase sod a, mediates the oxidative stress response in Aspergillus fumigatus. Appl. Environ. Microbiol. 87:e0101321. doi: 10.1128/AEM.01013-21
Dyer, P. S., and O’Gorman, C. M. (2012). Sexual development and cryptic sexuality in fungi: insights from Aspergillus species. FEMS Microbiol. Rev. 36, 165–192. doi: 10.1111/j.1574-6976.2011.00308.x
Fukuda, K., Yamada, K., Deoka, K., Yamashita, S., Ohta, A., and Horiuchi, H. (2009). Class III chitin synthase Chs B of Aspergillus nidulans localizes at the sites of polarized cell wall synthesis and is required for conidial development. Eukaryot. Cell 8, 945–956. doi: 10.1128/EC.00326-08
Georgiou, C., Patsoukis, N., Papapostolou, I., and Zervoudakis, G. (2006). Sclerotial metamorphosis in filamentous fungi is induced by oxidative stress. Integr. Comp. Biol. 46, 691–712. doi: 10.1093/icb/icj034
Gibbons, J. G., Salichos, L., Slot, J. C., Rinker, D. C., McGary, K. L., King, J. G., et al. (2012). The evolutionary imprint of domestication on genome variation and function of the filamentous fungus Aspergillus oryzae. Curr. Biol. 22, 1403–1409. doi: 10.1016/j.cub.2012.05.033
Horn, B. W., Moore, G. G., and Carbone, I. (2009). Sexual reproduction in Aspergillus flavus. Mycologia 101, 423–429. doi: 10.3852/09-011
Ishi, K., Watanabe, T., Juvvadi, P. R., Maruyama, J., and Kitamoto, K. (2005). Development of a modified positive selection medium that allows to isolate Aspergillus oryzae strains cured of the integrated nia D-based plasmid. Biosci. Biotechnol. Biochem. 69, 2463–2465. doi: 10.1271/bbb.69.2463
Jin, F. J., Nishida, M., Hara, S., and Koyama, Y. (2011a). Identification and characterization of a putative basic helix-loop-helix transcription factor involved in the early stage of conidiophore development in Aspergillus oryzae. Fungal Genet. Biol. 48, 1108–1115. doi: 10.1016/j.fgb.2011.10.001
Jin, F. J., Takahashi, T., Machida, M., and Koyama, Y. (2009). Identification of a basic helix-loop-helix-type transcription factor gene in Aspergillus oryzae by systematically deleting large chromosomal segments. Appl. Environ. Microbiol. 75, 5943–5951. doi: 10.1128/AEM.00975-09
Jin, F. J., Takahashi, T., Matsushima, K., Hara, S., Shinohara, T., Maruyama, J., et al. (2011b). SclR, a basic helix-loop-helix transcription factor, regulates hyphal morphology and promotes sclerotial formation in Aspergillus oryzae. Eularyot. Cell 10, 945–955. doi: 10.1128/EC.00013-11
Katayama, T., Bayram, Ö., Mo, T., Karahoda, B., Valerius, O., Takemoto, D., et al. (2021). Novel Fus3-and Ste12-interacting protein FsiA activates cell fusion-related genes in both Ste12-dependent and-independent manners in ascomycete filamentous fungi. Mol. Microbiol. 115, 723–738. doi: 10.1111/mmi.14639
Katayama, T., Nakamura, H., Zhang, Y., Pascal, A., Fujii, W., and Maruyama, J. (2019). Forced recycling of AMA1-based genome-editing plasmid allows for efficient multiple gene deletion/integration in the industrial filamentous fungus Aspergillus oryzae. Appl. Environ. Microbiol. 85, e01896–e01818. doi: 10.1128/AEM.01896-18
Kusuya, Y., Hagiwara, D., Sakai, K., Yaguchi, T., Gonoi, T., and Takahashi, H. (2017). Transcription factor Afmac1 controls copper import machinery in Aspergillus fumigatus. Curr. Genet. 63, 777–789. doi: 10.1007/s00294-017-0681-z
Kwon-Chung, K. J., and Sugui, J. A. (2009). Sexual reproduction in Aspergillus species of medical or economical importance: why so fastidious? Trends Microbiol. 17, 481–487. doi: 10.1016/j.tim.2009.08.004
Li, M., and Rollins, J. A. (2009). The development-specific protein (Ssp1) from Sclerotinia scletotiorum is encoded by a novel gene expressed exclusively in sclerotium tissues. Mycologia 101, 34–43. doi: 10.3852/08-114
Long, D. D., Fu, R. R., and Han, J. R. (2017). Tolerance and stress response of sclerotiogenic Aspergillus oryzae G15 to copper and lead. Folia Microbiol. 62, 295–304. doi: 10.1007/s12223-017-0494-y
Long, A., Song, B., Fridey, K., and Silva, A. (2015). Detection and diversity of copper containing nitrite reductase genes (NirK) in prokaryotic and fungal communities of agricultural soils. FEMS Microbiol. 91, 1–9. doi: 10.1093/femsec/fiu004
Mamun, M. A. A., Katayama, T., Cao, W., Nakamura, S., and Maruyama, J. (2020). A novel pezizomycotina-specific protein with gelsolin domains regulates contractile actin ring assembly and constriction in perforated septum formation. Mol. Microbiol. 113, 964–982. doi: 10.1111/mmi.14463
Maruyama, J., and Kitamoto, K. (2011). Targeted gene disruption in koji mold Aspergillus oryzae. Methods in Mol. Biol. 765, 447–456. doi: 10.1007/978-1-61779-197-0_27
Mori, N., Katayama, T., Saito, R., Iwashita, K., and Maruyama, J. (2019). Inter-strain expression of sequence-diverse HET domain genes severely inhibits growth of Aspergillus oryzae. Biosci. Biotechnol. Biochem. 83, 1557–1569. doi: 10.1080/09168451.2019.1580138
Murakami, H. (1971). Classification of the koji mold. J. Gen. Appl. Microbiol. 17, 281–309. doi: 10.2323/jgam.17.281
Nakamura, H., Kikuma, T., Jin, F. J., Maruyama, J., and Kitamoto, K. (2016). AoRim15 is involved in conidial stress tolerance, conidiation and sclerotia formation in the filamentous fungus Aspergillus oryzae. J. Biosci. Bioeng. 121, 365–371. doi: 10.1016/j.jbiosc.2015.08.011
Ogawa, M., Tokuoka, M., Jin, F. J., Takahashi, T., and Koyama, Y. (2010). Genetic analysis of conidiation regulatory pathway in koji-mold Aspergillus oryzae. Fungal Genet. Biol. 47, 10–18. doi: 10.1016/j.fgb.2009.10.004
Okegawa, Y., and Motohashi, K. (2015a). Evaluation of seamless ligation cloning extract (SLiCE) preparation methods from an Escherichia coli laboratory strain. Anal. Biochem. 486, 51–53. doi: 10.1016/j.ab.2015.06.031
Okegawa, Y., and Motohashi, K. (2015b). A simple and ultra-low cost homemade seamless ligation cloning extract (SLiCE) as an alternative to a commercially available seamless DNA cloning kit. Biochem. Biophys. Rep. 4, 148–151. doi: 10.1016/j.bbrep.2015.09.005
Papapostolou, I., Sideri, M., and Georgiou, C. D. (2014). Cell proliferating and differentiating role of H2O2 in Sclerotium rolfsii and Aclerotinia sclerotiorum. Microbiol. Res. 169, 527–532. doi: 10.1016/j.micres.2013.12.002
Park, H. S., and Yu, J. H. (2012). Genetic control of asexual sporulation in filamentous fungi. Curr. Opin. Microbiol. 15, 669–677. doi: 10.1016/j.mib.2012.09.006
Rogers, J. E., and Li, S. W. (1985). Simple detection of potential pollutant effects on fungal growth, sclerotia formation and germination. Bull. Environ. Contam. Toxicol. 34, 684–690. doi: 10.1007/BF01609793
Rowlands, R. T., and Turner, G. (1973). Nuclear and extranuclear inheritance of oligomycin resistance in Aspergillus nidulans. Mol. Gen. Genet. 126, 201–216. doi: 10.1007/BF00267531
Ruiz, L. M., Libedinsky, A., and Elorza, A. A. (2021). Role of copper on mitochondrial function and metabolism. Front. Mol. Biosci. 8:711227. doi: 10.3389/fmolb.2021.711227
Saitoh, Y., Izumitsu, K., Morita, A., and Tanaka, C. (2010). A copper-transporting ATPase BcCCC2 is necessary for pathogenicity of Botrytis cinerea. Mol. Gen. Genomics. 284, 33–43. doi: 10.1007/s00438-010-0545-4
Schrettl, M., Bignell, E., Kragl, C., Joechl, C., Rogers, T., Arst, H. N., et al. (2004). Siderophore biosynthesis but not reductive iron assimilation is essential for Aspergillus fumigatus virulence. J. Exp. Med. 200, 1213–1219. doi: 10.1084/jem.20041242
Skopp, A., Boyd, S. D., Ullrich, M. S., Liu, L., and Winkler, D. D. (2019). Copper-zinc superoxide dismutase (Sod1) activation terminates interaction between its copper chaperone (ccs) and the cytosolic metal-binding domain of the copper importer Ctr1. Biometals 32, 695–705. doi: 10.1007/s10534-019-00206-3
Staerck, C., Gastebois, A., Vandeputte, P., Calenda, A., Larcher, G., Gillmann, L., et al. (2017). Microbial antioxidant defense enzymes. Microb. Pathog. 110, 56–65. doi: 10.1016/j.micpath.2017.06.015
Upadhyay, S., Torres, G., and Lin, X. (2013). Laccases involved in 1,8-dihydroxynaphthalene melanin biosynthesis in Aspergillus fumigatus are regulated by developmental factors and copper homeostasis. Eukaryot. Cell 12, 1641–1652. doi: 10.1128/EC.00217-13
Wada, R., Maruyama, J., Yamaguchi, H., Yamamoto, N., Wagu, Y., Paoletti, P., et al. (2012). Presence and functionality of mating type genes in the supposedly asexual filamentous fungus Aspergillus oryzae. Apl. Environ. Microbiol. 78, 2819–2829. doi: 10.1128/AEM.07034-11
Ward, O. P. (2012). Production of recombinant proteins by filamentous fungi. Biotechnol. Adv. 30, 1119–1139. doi: 10.1016/j.biotechadv.2011.09.012
Wiemann, P., Perevitsky, A., Lim, F. Y., Shadkchan, Y., Knox, B. P., Figueora, J. A. L., et al. (2017). Host copper-mediated oxidative antimicrobial offense is countered by Aspergillus fumigatus copper export machinery and reactive oxygen intermediate defense. Cell Rep. 19, 1008–1021. doi: 10.1016/j.celrep.2017.04.019
Wu, X., Zhou, B., Yin, C., Guo, Y., Lin, Y., Pan, L., et al. (2014). Characterization of natural antisense transcript, sclerotia development and secondary metabolism by strand-specific RNA sequencing of Aspergillus flavus. PLoS One 9:e97814. doi: 10.1371/journal.pone.0097814
Yamada, O., Lee, B. R., and Gomi, K. (1997). Transformation system for Aspergillus oryzae with double auxotrophic mutations, niaD and sC. Biosci. Biotechnol. Biochem. 61, 1367–1369. doi: 10.1271/bbb.61.1367
Zhang, Z. Q., Zhao, W. J., Long, D. D., Niu, L. R., and Han, J. R. (2014). Effect of copper-induced oxidative stress on sclerotial differentiation and antioxidant properties of Penicillium thomii PT95 strain. World J. Microbiol. Biotechnol. 30, 1519–1525. doi: 10.1007/s11274-013-1572-1
Keywords: Filamentous fungi, Aspergillus oryzae, Copper, Conidiation, Sclerotia formation, Superoxide dismutase, AobrlA
Citation: Katayama T and Maruyama J-i (2023) Trace copper-mediated asexual development via a superoxide dismutase and induction of AobrlA in Aspergillus oryzae. Front. Microbiol. 14:1135012. doi: 10.3389/fmicb.2023.1135012
Edited by:
Masashi Kato, Meijo University, JapanReviewed by:
Hokyoung Son, Seoul National University, Republic of KoreaYonghong Meng, Shaanxi Normal University, China
Copyright © 2023 Katayama and Maruyama. This is an open-access article distributed under the terms of the Creative Commons Attribution License (CC BY). The use, distribution or reproduction in other forums is permitted, provided the original author(s) and the copyright owner(s) are credited and that the original publication in this journal is cited, in accordance with accepted academic practice. No use, distribution or reproduction is permitted which does not comply with these terms.
*Correspondence: Jun-ichi Maruyama, amarujun@g.ecc.u-tokyo.ac.jp