- 1Department of Microbiology, College of Basic Sciences & Humanities, Dr. Rajendra Prasad Central Agricultural University, Samastipur, Bihar, India
- 2Department of Plant Pathology, College of Agriculture, A.N.D University of Agriculture and Technology, Ayodhya, Uttar Pradesh, India
- 3Department of Biological Sciences, College of Basic Sciences and Humanities, G.B. Pant University of Agriculture and Technology, Pantnagar, Uttarakhand, India
- 4Department of Horticulture, PGCA, Dr. Rajendra Prasad Central Agricultural University, Samastipur, Bihar, India
- 5Department of Plant Pathology, PGCA, Dr. Rajendra Prasad Central Agricultural University, Samastipur, Bihar, India
- 6Department of Biotechnology and Synthetic Biology, Center of Innovative and Applied Bioprocessing, Sector 81, Mohali, India
- 7Department of Soil Science, College of Agriculture, G. B. Pant University of Agriculture and Technology, Pantnagar, India
- 8Department of Molecular Biology & Genetic Engineering, College of Basic Sciences and Humanities, GBPUA&; T, Pantnagar, Uttarakhand, India
- 9School of Biotechnology, IFTM University, Moradabad, India
- 10Department of Horticulture, College of Agriculture, G. B. Pant University of Agriculture and Technology, Pantnagar, Uttarakhand, India
- 11Department of Microbiology, Akal College of Basic Sciences, Eternal University, Sirmaur, Himachal Pradesh, India
- 12College of Fisheries, Dholi, Dr. Rajendra Prasad Central Agricultural University, Muzaffarpur, Bihar, India
Modern agriculture is primarily focused on the massive production of cereals and other food-based crops in a sustainable manner in order to fulfill the food demands of an ever-increasing global population. However, intensive agricultural practices, rampant use of agrochemicals, and other environmental factors result in soil fertility degradation, environmental pollution, disruption of soil biodiversity, pest resistance, and a decline in crop yields. Thus, experts are shifting their focus to other eco-friendly and safer methods of fertilization in order to ensure agricultural sustainability. Indeed, the importance of plant growth-promoting microorganisms, also determined as “plant probiotics (PPs),” has gained widespread recognition, and their usage as biofertilizers is being actively promoted as a means of mitigating the harmful effects of agrochemicals. As bio-elicitors, PPs promote plant growth and colonize soil or plant tissues when administered in soil, seeds, or plant surface and are used as an alternative means to avoid heavy use of agrochemicals. In the past few years, the use of nanotechnology has also brought a revolution in agriculture due to the application of various nanomaterials (NMs) or nano-based fertilizers to increase crop productivity. Given the beneficial properties of PPs and NMs, these two can be used in tandem to maximize benefits. However, the use of combinations of NMs and PPs, or their synergistic use, is in its infancy but has exhibited better crop-modulating effects in terms of improvement in crop productivity, mitigation of environmental stress (drought, salinity, etc.), restoration of soil fertility, and strengthening of the bioeconomy. In addition, a proper assessment of nanomaterials is necessary before their application, and a safer dose of NMs should be applicable without showing any toxic impact on the environment and soil microbial communities. The combo of NMs and PPs can also be encapsulated within a suitable carrier, and this method aids in the controlled and targeted delivery of entrapped components and also increases the shelf life of PPs. However, this review highlights the functional annotation of the combined impact of NMs and PPs on sustainable agricultural production in an eco-friendly manner.
1. Introduction
Numerous tactics dealing with the improvement of crop production are essentially required to meet the basic food needs of the rapidly growing human population. The sector of agriculture affected by climate change, where increasing phenomena of abiotic stresses such as drought, salinity, cold, flooding, and biotic stress (attacks by pathogens such as bacteria, fungi, oomycetes, nematodes, and herbivores) negatively affect agricultural production (Shahzad et al., 2021; Upadhayay et al., 2023). In addition, the agrochemicals showed a significant increase in crop yield in the last few decades (Lin et al., 2019), but later harmful effects from the over-application of chemical fertilizers became apparent (Upadhayay et al., 2022a,b). It led to the degradation of soil quality, disturbance of soil microbial ecology, pollution of soil and water bodies, and harmful effects on human health due to residues of pesticides and herbicides (Singh et al., 2020; Tripathi et al., 2020; Boregowda et al., 2022). Moreover, the transition to organic agriculture, particularly the use of biofertilizers, provided an environmentally friendly alternative to chemical-based agriculture, as well as improved crop yield and soil quality (Asghar et al., 2022; Elnahal et al., 2022). The term “plant probiotics (PPs)” can be used to decode a distinct group of microbial strains with all the necessary characteristics to be classified as biofertilizers that influence plant growth through both direct and indirect mechanisms (microbes that show beneficial attributes for plants in terms of growth and yield; Sarbani and Yahaya, 2022; Rai et al., 2023). The rhizosphere and the inner regions of plant tissues each serve as a special hub for their respective microbial communities, the rhizomicrobiome (Jiang G. et al., 2022), and the endophytomicrobiome (Pandey et al., 2022). This microbiome is a rich source of plant probiotics due to the multitude of traits it possesses, such as the solubilization of nutrients (Khan et al., 2022), nitrogen fixation (Abdelkhalek et al., 2022), production of plant hormones [indole-3-acetic acid (IAA); Nazli et al., 2020], ammonia (Upadhayay et al., 2022b), anti-pathogenic compounds (Mathur et al., 2019), hydrogen cyanide (HCN; Kashyap et al., 2021), exopolysaccharides (Latif et al., 2022), siderophore (Mushtaq et al., 2022), and lytic enzymes (Reddy et al., 2022). Plant probiotics enhance nutrient uptake and provide protection for plants from environmental stresses, such as biotic and abiotic stresses, and also improve plant health (Kenawy et al., 2021; Pandey et al., 2022). Plant probiotics with varying plant growth-stimulating capabilities provide advantages such as improved crop productivity and food security (Arif et al., 2020; Ghoghari et al., 2022). In contemporary times, the use of nanotechnology in developing countries is gaining more attention, especially in the field of agriculture (Neme et al., 2021). Due to their greater surface area and solubility, nanomaterials are regarded as superior to conventional agrochemicals when used as nanofertilizers in agriculture (Fen et al., 2022). Nanofertilizers improve the nutrient uptake efficiency of plants, diminish the detrimental effects of environmental stresses, and increase crop productivity (Guleria et al., 2022). It is possible to use a combination of the selective plant probiotics that have been shown to be compatible with the nanoparticles of interest (Khati et al., 2017, 2018; Agri et al., 2021; Chaudhary et al., 2021a,b,c). NMs and PPs together hold a great promise for sustainable agriculture as better alternatives to agrochemicals and are becoming a popular concept in the agricultural sector. This idea of efficient fertilization can be preferred over chemical-based fertilization because of its higher efficacy in resource utilization, sustained and slow release of nutrients, increase in crop productivity with a lesser dose of fertilizer, and least negative impacts on soil. Moreover, the use of NMs and PPs is economically feasible and poses lesser toxicity to the environment. According to the literature, the “cocktail” of NMs and PPs can be considered a “nanobiofertilizer (NBF),” because it has the effectiveness of both components (i.e., NMs and PPs) and aids in the slow and controlled release of nutrients, improves nutrient use efficiency, and results in a significant increase in crop yield (Kumari and Singh, 2020).
The microbial part of this cocktail contributes benefits to the plant system due to its wide array of plant growth-stimulating traits such as the solubilization of nutrients, nitrogen fixation, production of plant hormones, EPS, siderophore, and anti-pathogenic compounds. The improvement in soil fertility, functional enzymatic activities, NPK content, organic carbon content, and soil microbial biomass are reflected under the influence of the effective microbial component. On the contrary, the second and most effective segment, “NMs,” maximize the benefits and contributes to plant growth through the controlled and sustained release of nutrients, a reduction in the fixation of nutrients in the soil, an increase in the bio-availability of nutrients to plants, making plants more tolerant to environmental stress, and the protection of plants from pests. The combination of nanomaterials and plant probiotics can be applied to plants in a variety of ways, including seed treatment, seedling treatment, foliar application, soil application, and other methods. Nanotechnology advancements have also led to the encapsulation of plant probiotic strains within the appropriate nanomaterials (Panichikkal et al., 2019, 2021; Akhtar et al., 2022) or the encapsulation of both NMs and PPs within a suitable carrier (Moradi Pour et al., 2022), depending on the choice of experiments. This concept maintains the efficacy and shelf life of the microbial component (PPs) as well as the controlled and sustained supply of both NMs and PPs. This two-pronged strategy increases nutrient availability directly through the use of nanomaterials, while also stimulating plant growth through effective microbial treatment. The use of such a combination of effective doses of NMs and PPs has the potential to create a big difference in the agricultural sector, which will eventually be fruitful in providing benefits of sustainable agricultural production and as well as food security (Kumari et al., 2021; Agri et al., 2022; Akhtar et al., 2022). The present review illustrates the impact of the combined use of NM and PP, as an effective but two-way strategy, on food crops in terms of increased crop production, reducing the detrimental effects of environmental stress, improving soil fertility, and strengthening the bioeconomy.
2. Compendious outline of nanomaterials
Nanomaterials are naturally or artificially synthesized exceptionally tiny molecules ranging from 1 to 100 nm in size (Rehman and Pandey, 2022). The smaller size and high surface-to-volume ratio of nanomaterials give them distinct and advantageous properties in different scientific fields compared to their bulk analog (Yang et al., 2022). The NM has unique physiochemical properties and flexible scaffolds, making them functional with biomolecules and unique compared to other materials (Muthukumaran et al., 2022). Furthermore, it has been demonstrated that some NMs, such as magnetic (Armenia et al., 2022), gold (Jiang et al., 2017), polymeric (Kamaly et al., 2016), or hybrid NMs (Ferreira Soares et al., 2020), may react to external stimuli, leading to a spatiotemporally regulated release of macromolecules. In the last few decades, synthetic NMs have been efficiently used in pharmacology and medicine, especially for therapeutic or diagnostic applications (Zain et al., 2022). These NMs or nanoparticles are carbon-based, inorganic, or organic. Inorganic nanoparticles have numerous scientific uses and are metallic or metal oxides. Constructive or destructive processes can synthesize these nanomaterials using nearly all metals (Upadhayay et al., 2019). Among the different elements, Cd, Au, Al, Co, Zn, Pb, Fe, Cu, and Ag are frequently used to synthesize nanoparticles (Ali et al., 2022). It was reported that the metal oxide-based nanoparticles alter the nature of their analog metals (Sanzari et al., 2019). For example, iron (Fe) containing nanoparticles (NPs) rapidly oxidized to Fe oxide in the presence of oxygen (O) at room temperature, making them more reactive and efficient compared to their parent iron nanoparticles (Sanzari et al., 2019; Ezealigo et al., 2021). There are different types of commonly manufactured nanoparticles, such as “silicon dioxide (SiO2),” “zinc oxide (ZnO),” “cerium oxide (CeO2),” “aluminium oxide (Al2O3),” “titanium oxide (TiO2),” and “magnetite (Fe3O4)” which contain metal oxides (Fuskele and Sarviya, 2017). Some nanoparticles, viz., liposomes, dendrimers, ferritin, and micelles, are organic nanoparticles and eco-friendly in nature (Sanzari et al., 2019; Upadhayay et al., 2019).
In addition, “carbon-based” refers to another critical group of nanoparticles, further classified into fullerenes, graphene, carbon nanotubes (CNTs), carbon nanofibers, and carbon black (Ramar and Balraj, 2022). Occasionally, the term “activated carbon in nanosize” is also used for carbon-based nanoparticles (Upadhayay et al., 2019). The “bottom-up” strategy and the “top-down” approach have been suggested as two crucial strategies for the synthesis of NPs (Gutiérrez-Cruz et al., 2022). Among them, the bottom-up method is occasionally referred to as the constructive method because it involves the steady construction of a structure that starts from the atomic level and progresses up to the nanoparticle level (Upadhayay et al., 2019). Different methods such as chemical vapor deposition (CVD), biosynthesis, sol–gel, pyrolysis, and spinning are the bottom-up approach for nanoparticle synthesis. The term “biosynthesis” refers to a sustainable process that uses plant, bacterial, and fungal extracts coupled with precursors to create nanoparticles (Sanzari et al., 2019).
On the other hand, the bulk material is broken down into nanometric-sized particles using the “top-down method” or “destructive process (Yin et al., 2021).” Different strategies frequently used for the development of nanoparticles include laser ablation, thermal decomposition, nanolithography, sputtering, and mechanical milling (Sanzari et al., 2019; Upadhayay et al., 2019). Currently, polymeric nanoparticles have sought lots of attention due to their ease of synthesis, biocompatibility, and responsiveness to stimuli (Zu et al., 2021).
However, core or shell nanoparticles are also available in different combinations of materials used, which are organic/organic, inorganic/organic, and inorganic/inorganic materials. The shell of nanoparticles is selected based on ultimate applications and use (Sanzari et al., 2019). For example, it was proposed that polymeric shells enhance nanoparticle biocompatibility (Sharifianjazi et al., 2021). It has also been possible to create NPs with a nanostructured shell. Mesoporous silica nanoparticles (NPs) are nanoparticles with a mesoporous structure and a highly functionalizable surface (Zhang et al., 2022). In nanotechnology, a novel class of NMs known as nanogels (NGs) is gaining more attention due to its colloidal stability, bioconjugation, good physicochemical qualities, and stimuli sensitivity such as temperature and pH (Dalir Abdolahinia et al., 2022). Nanogels are made up of natural or synthetic polymer chains that are nano-sized ionic as well as non-ionic hydrogels. The NGs are highly porous that have high water content, i.e., 70–90% of the whole structure with high load capacity (Sanzari et al., 2019). A few examples of the nanogels are poly (vinyl alcohol), poly (ethyleneimine), chitosan, poly (ethylene oxide), poly (vinylpyrrolidone), alginate, poly (vinylpyrrolidone), and among them, the most frequent NGs is N-isopropyl acrylamide (Pinelli et al., 2022). Hybrid NGs are classified as (i) “nanomaterial–nanogel,” which incorporates nano-sized materials such as “magnetic” or “carbonaceous” NPs and (ii) “polymer–nanogel composites,” which include “interpenetrated networks (IPNs),” “copolymer,” and “core-shell particles” (Eslami et al., 2019; Sanzari et al., 2019).
3. Nanomaterials in agriculture: A way of smart delivery of nano-based fertilizers
The agricultural sector is highly dependent on climatic conditions, but in recent years, climate change has become a major concern of our human civilization (Qin et al., 2020; Kasperson et al., 2022). The adverse effects of climate change manifested as excessive rainfall, drought, extreme cold, heat wave, pest resurgence, and disease outbreaks caused the biological change in the crop life cycle, resulting in reduced grain yield, which directly affects food security on a global scale (Chitara et al., 2017; Liliane and Charles, 2020). Experts are focusing on the development of cutting-edge technologies in the agricultural sector in order to mitigate the detrimental effects of climate change (Shahzad et al., 2021) and emphasizing the synthesis of various nano-based products and the assessment of safer doses of nano-based products prior to their application. Moreover, nano-based fertilizers can provide an economically feasible and ecologically safe option for sustainable crop production under climate change scenarios.
Nanotechnology-based synthetic fertilizer applications in agricultural crop production are becoming popular strategies due to their beneficial role in increasing crop productivity, improving nutrient use efficiency, and reducing the impact of environmental constraints on crops (Beig et al., 2022). There are certain types of NMs viz. inorganic-based NMs, carbon-based NMs, organic-based NMs, and composite-based NMs have been used in agricultural crop production. Using all these NMs, researchers developed site-specific, targeted nanofertilizers, nanoherbicides, nanopesticides, nanofungicides, and nanoinsecticides, which have to prove themselves as highly efficient nano-based agrochemicals (Bana et al., 2020; Qazi and Dar, 2020; Ahmed et al., 2021; Okey-Onyesolu et al., 2021). Targeted application of nanofertilizers to crops improves nutrient use efficiency and prevents nutrient losses, as well as reducing the over-application of fertilizers can also help reduce fertilizer toxicity, which is followed by many farmers (Hofmann et al., 2020; Mejias et al., 2021). In addition to the application of nano fertilizers, nano herbicides are also used in weed control. Weed also hampers the agricultural dry matter accumulation due to their high competitiveness with the main crop for nutrients and space. Thus, with the help of nanotechnology, more competent nano-based herbicides have been developed that give better results compared to commercially available conventional herbicides (Abigail and Chidambaram, 2017; Balah and Pudake, 2019). Conventional herbicides only kill the top of the leaves, resulting in weed regrowth, but in the case of nanoherbicide application, the targets for killing are the root of the weed. After the roots have died, the weed plants are unable to resist regrowth.
Applications of NMs-based nano-pesticides are helpful in the control of a wide variety of pests that affect crops. In general, the conventional application of pesticides to crops increases cultivation costs and causes environmental pollution (Hajji-Hedfi and Chhipa, 2021). Nano-based pesticides have increased retention capacity with high efficacy, durability, good dispersion, and wettability, which makes them a potent pesticide compared to conventional pesticides, as well as their low dose release, which increases effectiveness and reduces environmental losses, soil degradation, and toxicity (Kumar et al., 2019; Vignardi et al., 2020). Some examples of nano-pesticides are Karate® ZEON against soybeans, rice, and cotton pests; and stomach poison for insects sold as Gutbuster. Similarly, nanomaterial-based nanofungicides and antimicrobial compounds are also helpful in plant disease management. Due to its large surface area to volume ratio, it increases their contact with the microbes and easily penetrates into the microbial cell, making excellent contact for nano-fungicides. Applications of nanofungicides provide targeted delivery, improved bioavailability as a result of increased solubility and penetrability, lower dosages, and decreased dose-dependent toxic effects (Ul Haq and Ijaz, 2019). Recently, metal-based NPs such as Ag, Au, Cu, Cd, Al, Se, Zn, Ce, Ti, and Fe synthesized with plant extract have gained in popularity, and they are all effective in the control of phytopathogens (Hernández-Díaz et al., 2021). Many researchers have demonstrated AgNPs as potent nanometal-based pesticides with antibacterial and antifungal activity, successfully used in controlling plant diseases (Khan et al., 2021; Tariq et al., 2022).
The application of NMs such as nanochitosan, nanogypsum, nanourea, carbon nanotubes, and nanophosphorus also showed their important roles in disease suppression, improvement in soil functions and structure, enhancement in photosynthetic efficiency, and crop production. Meloidogyne incognita densities alone or in the presence of TMV were reduced by nano-chitosan by 45.89 to 66.61%, while root gall density was reduced by 10.63 to 67.8% (Khalil et al., 2022). The combined use of nanogypsum and Pseudomonas taiwanensis on maize improves the structure and function of the soil, which has a beneficial influence on plant health without generating toxicity (Chaudhary et al., 2021c). The foliar application of nano-urea to pearl millet plants improved plant growth metrics, dry matter accumulation, chlorophyll content, and NPK content (Sharma S. K. et al., 2022). Carbon nanotubes have potent antibacterial properties as well as induced defense activation after application to tomato crops infested with Alternaria solani (González-García et al., 2021). The administration of the nanophosphorous (nP) via foliar application to plants growing in P-deficient soil increased plant growth and yield attributing metrics, leaf integrity, chlorophyll content, P contents of leaf and seed, and improved anatomical topographies (Abou-Sreea et al., 2022). Table 1 depicts a recent scenario deciphering the beneficial effects of various NMs on plants. In addition, Table 2 shows some of the commercially available fertilizers based on NMs with their ingredients (Elemike et al., 2019; Pirzadah et al., 2020; Avila-Quezada et al., 2022).
4. Molecular insights on NMs for plant growth and development
The advancement of NMs in terms of plant growth is further embellished by illustrating their molecular mechanisms, which are particularly well understood at the level of relative gene expression. Several high-throughput studies have been conducted to investigate the effects of NMs on specific gene expression patterns, whether upregulation or downregulation, for a variety of plant activities such as seed germination, photosynthesis, and abiotic and biotic stress tolerance. Application of ZnO NPs (25 mg/L) recorded the maximum level of photosynthetic pigments due to the higher expression of photosynthesis-related genes (“CHLΙ,” “LHCa/b,” and “RSSU”; Mardi et al., 2022). The effect of silica nanoparticles observed in terms of improvement of wheat growth under filed conditions and upregulation of genes related to plant hormones (“TIR1” for IAA; “PYR/PYL,” “PP2C,” “SnRK2,” and “ABF” for abscisic acid), sugar metabolism (α-glucosidase, SUS, SPC), and chlorophyll (“CHLH,” “CAO,” and “POR”; Li et al., 2023). Foliar application of manganese ferrite NMs (10 mg/L) induced early flowering in tomatoes by upregulating the flowering induction gene SFT. A similar study also reported the upregulation of genes associated with gibberellin biosynthesis (GA20ox2, GA20ox3, and SIGAST; Yue et al., 2022). Among the genes involved in the photosynthetic process in Brassica chinensis L., ferredoxin-NADP reductase (PetH) was highly expressed under various concentrations (0.7, 7, and 70 mg/kg) of CeO2 NPs, while photosystem II lipoprotein (Psb27) was downregulated under varying levels of NPs (7, 70, and 350 mg/kg; Hong et al., 2023). One of the most important mechanisms for plant survival under stress conditions is the expression and regulation of abiotic stress-responsive genes (Sahil et al., 2021). NMs, however, showed a positive impact in terms of improving plant tolerance by upregulating the expression of genes involved in plant survival under stress conditions. Chitosan NPs upregulated drought-responsive genes such as “HsfA1a,” “SlAREB1,” “LeNCED1,” and “LePIP1” in Solanum lycopersicum (Mohamed and Abdel-Hakeem, 2023). The genes involved in drought tolerance, such as “P5CS,” “CAT1,” and “DREB2,” related to “proline biosynthesis,” “catalase activity,” and “dehydration-responsive element-binding proteins,” respectively, were highly expressed in wheat by the application of zinc oxide NPs to mitigate the drastic effect of drought in plants (Raeisi Sadati et al., 2022). Recently, Subotić et al. (2022) reported higher expression of aquaporin genes (PIP1;3, PIP1;5, and PIP2;4) related to water and solute transportation across the plant membrane in tomatoes by exposing them to a nanosubstance, i.e., hyper-harmonized hydroxyl-modified fullerene (3HFWC). Phytochemicals, such as alkaloids, have defensible importance in plants under stress conditions, and their biosynthesis is increased under drought conditions (Amirifar et al., 2022). The further addition of nanomaterials can enhance the level of biosynthesis of phytochemicals in plants. The study of Ali et al. (2021) observed the upregulation of key genes such as STR (strictosidine synthase), PRX1 (peroxidase 1), GS (geissoschizine synthase), and DAT (deacetylvindoline-4-O-acetyltransferase) involved in the biosynthesis of alkaloids under the response of chitosan NMs in drought stress. In tomato plants, Rahmatizadeh et al. (2021) showed the effect of nano-SiO2 (50 mg/L) as a possible mediator, stimulating the expression of “LeNRAMP3” and “LeFER.” The overexpression of these genes might enhance the nutritional status of Cd-stressed tomato plants, indicating that the LeFER transporter plays a vital role in alleviating the impact of Cd stress. Furthermore, the resultant upregulation of the several genes associated with various functions under the response of NMs is illustrated in Table 3.
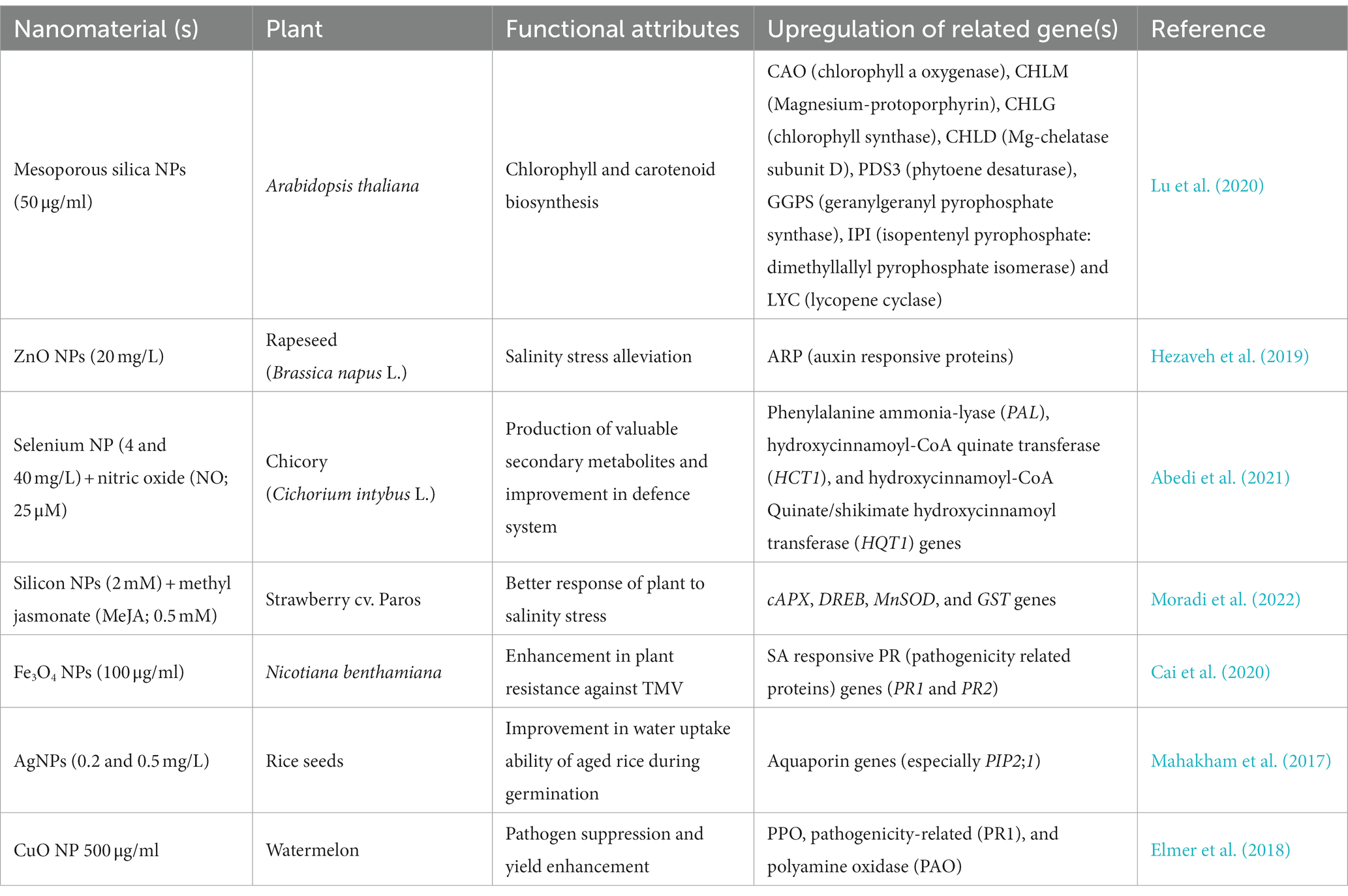
Table 3. Upregulation of genes associated with functional attributes in plants under the influence of nanomaterials.
5. Plant probiotics: Unraveling a long story in a nutshell
Current agricultural production cannot guarantee a consistent food supply for the rapidly expanding global population over the next 50 years. In addition, changes in dietary preferences and the increasing demand for the production of a wide variety of crop-based food products, etc., are imposing massive pressure on the production of crops at a huge scale. In recent decades, excessive amounts of chemical-based fertilizers and pesticides have been used to improve agricultural output on a vast scale; this was also a necessary step in order to solve the food crisis. Indeed, agrochemicals have changed the scenario of the agricultural world in terms of accessing multiple crop yields even under environmental stress conditions, but they have also left negative environmental footprints (Mitra et al., 2021; Tazunoki et al., 2022). Soil quality degradation, disturbance of local soil microbial ecology, health hazards from chemical residues of agrochemicals, and contamination of local water bodies are the adverse consequences of heavy reliance on agro-based chemicals (Mandal et al., 2020; Meena et al., 2020; Tazunoki et al., 2022). In the contemporary world, due to the tremendous awareness of the negative impacts of agrochemicals on organic farming and other chemical-free practices, people’s interest is shifting to reducing dependence on chemical-based products (Nithya et al., 2022). Fortunately, the concept of using plant growth-promoting microbes as biofertilizers/biopesticides is favorable as a green technology for sustainable agriculture (Khan et al., 2019; Elnahal et al., 2022). Plant growth-promoting microbes are actually effective or beneficial microorganisms that confer beneficial attributes to the host plants (Massa et al., 2022; Chaudhary et al., 2022c). Like human probiotics, a specialized set of microbial strains responsible for gut health, the term “plant probiotics” has recently become trendy to denote beneficial microorganisms that are necessary for the wellbeing of host plants (Carro and Nouioui, 2017; Menéndez and Paço, 2020; Sarbani and Yahaya, 2022). Therefore, plant probiotics and plant growth-promoting microorganisms (bacteria, fungi, etc.) are somewhat synonymous with each other and are part of a complex microbial community that either colonizes the rhizosphere (rhizomicrobiome; Ravichandran et al., 2022) or diffuse in or localizes in plant tissues (endophytes; Pandey et al., 2022; Rai et al., 2023) and contribution to beneficial functional traits in favor of plants (Gosal et al., 2017). These beneficial traits include enhancement in plant growth and productivity (Gavelienė et al., 2021), amelioration of abiotic and biotic stresses in plants (Santoyo et al., 2021), lowering the challenges of climate changes effects (Fiodor et al., 2021), and biofortification benefits via improving micronutrients levels in crop edibles (Upadhayay et al., 2018, 2021, 2022a,b,c). Plant probiotics must contain some PGP traits such as the solubilization of elements (P, K, and Zn; Singh et al., 2022), nitrogen fixation (Pandey et al., 2022), production of phytohormones (Kurniawan and Chuang, 2022) aminocyclopropane-1-carboxylate (ACC) deaminase (Santos et al., 2022; Singh et al., 2022), siderophore (Upadhayay et al., 2022a,b), and ammonia (Santos et al., 2022). Production of compounds showing importance in killing pathogens such antibiotics, secretion of enzymes (chitinase, protease/elastase, cellulase, catalase, and β-(1,3)-glucanas; Duhan et al., 2022), volatile compounds (HCN; Vaghela and Gohel, 2022), and also induce systematic resistant in plants against pathogen is the important contribution of plant probiotics (Yin et al., 2022; Chaudhary et al., 2022a). In addition, the ability of plant probiotics to produce exopolysaccharides and biofilms has multiple benefits, including protection from abiotic stress (Banerjee et al., 2019) and desiccation (Mandal et al., 2022), effective root colonization (Naseem et al., 2018), and improved soil aggregation and stabilization (Jhuma et al., 2021). Numerous microbial strains have been identified to possess plant probiotic properties that stimulate plants’ growth and improve crop yield (Das et al., 2022; Pantigoso et al., 2022; Khan et al., 2023). Therefore, such microorganisms can be utilized effectively as bioinoculants for eco-friendly agriculture (Daniel et al., 2022). Plant probiotics are effective “bioelicitors” or “biofertilizers” (Chen et al., 2022) because they improve crop yield-related traits, such as length of shoot and root, biomass of plants, photosynthetic pigments, grain yield, and biological output (Khan et al., 2022; Upadhayay et al., 2022a,b). A remarkable increase in yield-attributed traits was determined for rice, wheat, and maize in response to plant probiotics such as Bacillus (Abd El-Mageed et al., 2022), Azospirillum brasilense (Zaheer et al., 2019), and Pseudomonas stutzeri (Jiang S. et al., 2022), respectively. Plant probiotics such as Burkholderia cepacia and Pantoea rodasii having zinc solubilizing potential improved the overall growth of rice plants and provided biofortification benefits by increasing considerable Zn concentration in grains (Upadhayay et al., 2022b). Plant probiotics also ameliorate abiotic stress effects in plants via enhancing stress tolerance of plants which can be glimpsed by osmolyte accumulation (Tahiri et al., 2022), activation of antioxidant enzymes (Shultana et al., 2022), reduction in MDA content, reduction in electrolyte leakage, and improving in the activity of photosynthetic pigments (Zarei, 2022). Figure 1 shows schematic and beneficial outcomes that can result from using PPs as a green approach. Considering the productive effects of plant probiotics on crop wellbeing, systematic research is needed to identify and characterize a novel microbial strain or microbial consortium having multifarious plant growth-promoting effects. Deeper studies are required to reveal the interaction between plants and microbes at the molecular level and the microbial effects on plants in terms of enhancing physiological phenomena. In addition, a comprehensive analysis is required to illustrate how the inoculation of plant probiotics has a significant impact on the local soil microbiota in addition to their soil-healing properties.
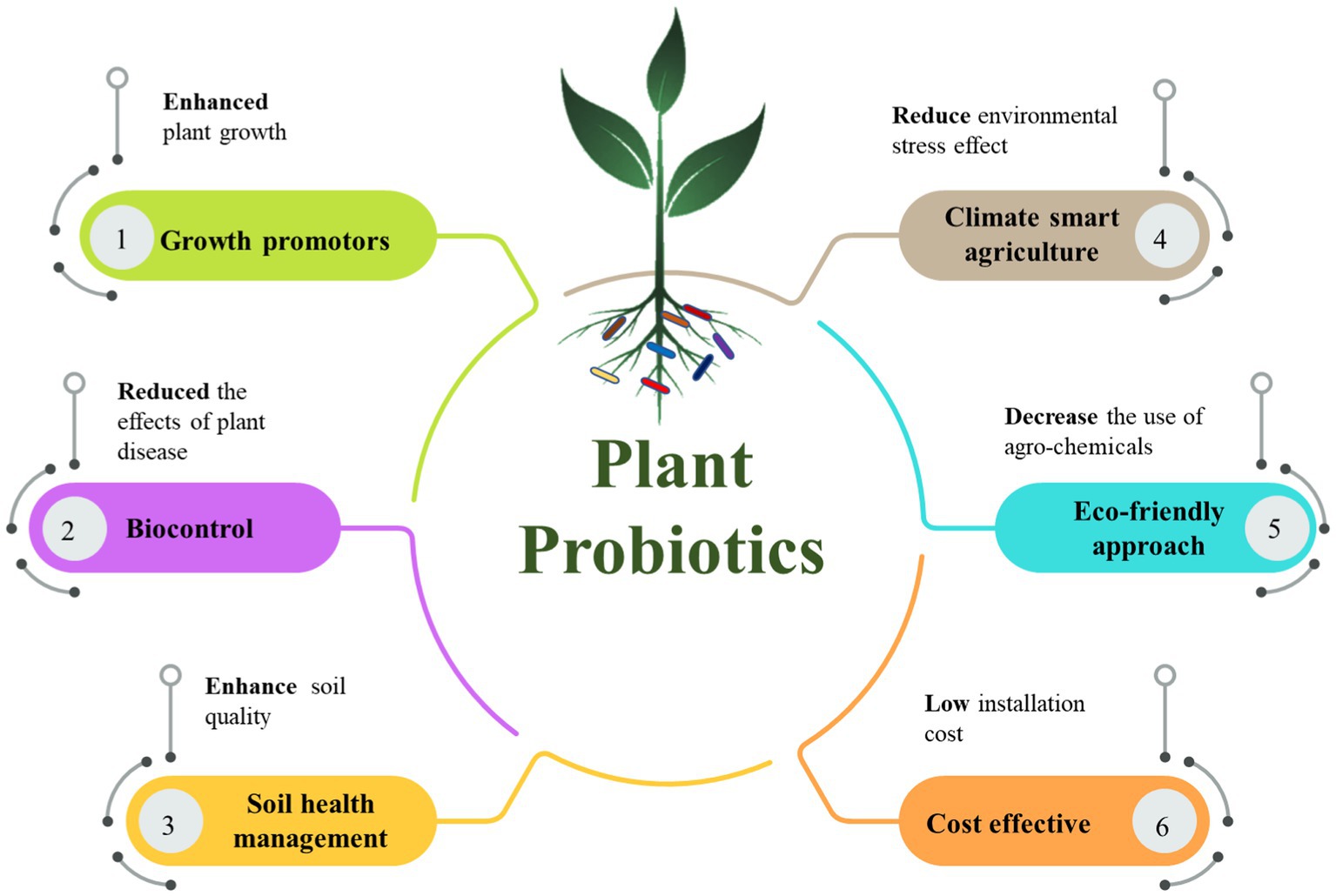
Figure 1. Schematic flow chart representing the prolific attributes of using plant probiotics (PPs).
6. The synergy of nanomaterials and plant probiotics: A green solution for sustainable agriculture
The harmful effects of agrochemicals are well known and occur as a result of the indiscriminate use of various agrochemicals. In addition, the application of PPs as bioinoculant faces several challenges, including a decline in number, a slow rate of action, a lack of suitable carrier materials, susceptibility to certain stress conditions, such as desiccation and salinity, and a loss of effectiveness in field conditions (Nagpal et al., 2021; Walia et al., 2021). Therefore, to overcome these problems, the potential alternative is a cocktail of suitable nanomaterial and PP strain. Using a combination of PPs and NMs can provide the benefits of both biofertilizers and nanofertilizers (Chaudhary et al., 2021a,b,c). Application of the cocktail of NMs and PPs to agricultural crops is viewed as an alternative eco-friendly method to reduce the use of chemical or synthetic fertilizers in crop management (Kumari and Singh, 2020; Akhtar et al., 2022), due to the risk posed by the excessive use of chemical-based fertilizer and pesticides (Chitara et al., 2022). The slow-release ability of nanobiofertilizers makes them highly efficient, resulting in the accessibility of the nutrients for a longer period of time and increased nutrient use efficiency or vice versa, which reduces nutrient losses and supports agricultural development through increased crop growth and yield (Fazelian and Yousefzadi, 2022).
The microbial components of nanobiofertilizers include nitrogen-fixing microorganisms such as free-living Azotobacter, symbiotic Rhizobium, and associative Azospirillium, phosphorous solubilizing microorganisms such as Pseudomonas striata, Penicillium spp., Bacillus sp., and Aspergillus sp., and phosphorous mobilizers microorganism. On the contrary, nanomaterials such as nanosilicon dioxide (Kukreti et al., 2020), AgNPs (Nawaz and Bano, 2020), nano-iron oxide (Babaei et al., 2017), ZnO-NPs (Azmat et al., 2022), nanozeolite (Khati et al., 2019a,b), nanochitosan (Kumari et al., 2020), and nanogypsum (Kumar et al., 2019) have been employed as nano-constituents of “NMS-PPs cocktail.” This association of microorganisms and nanoparticles exhibits a synergistic effect in soil by improving soil nutrient status through nitrogen fixation, iron chelation through siderophore production, phosphorus solubilization, phytohormone production, induces systemic resistance (ISR), systemically acquired resistance (SAR), and gives plants vigor against pests (Chitara et al., 2021; Chaudhary et al., 2021a,b,c,d,e, 2022b; Mahawer et al., 2022).
However, before using a combination of NMs and PPs, the impact of NMs on PPs should be assessed. The NMs should not be detrimental to the microbial component; rather, they must support microbial growth and activity. In previous studies, NM such as nanozeolite and nanogypsum showed positive impacts on the growth of plant growth-promoting bacteria isolated from NM-infested soil (Chaudhary and Sharma, 2019; Khati et al., 2019a). The synergistic effect of the NMs and PPs could be visualized in the form of enhanced physiological and morphological development through an increased rate of photosynthetic translocation in the aerial plant parts, resulting in improved grain quality and increased yield (Khati et al., 2018; Kukreti et al., 2020; Vedamurthy et al., 2021). Application of the chitosan–iron nanobiofertilizer against bacterial leaf blight of rice caused by Xanthomonas oryzae pv. oryzae (Xoo) under in vitro and in vivo. Under in vitro assay against bacteria, nanobiofertilizer significantly inhibit the biological function such as growth, mobility, and biofilm formation of the bacteria and under in vivo condition foliar spray of the nanobiofertilizer reduced the disease incidence as well as modulate the enzyme system of the plants and improved the photosynthesis by increasing chlorophyll content and carotenoid (Ahmed et al., 2022). Under drought, the application of the nano-Zn chelate and nano-biofertilizer effectively alleviate the impact of the drought stress and significantly augmented the plant biomass and grain yield (Farnia et al., 2015). In maize crops, under water scarcity, the application of the nanobiofertilzer improved water use efficiency and enhanced crop productivity (Janmohammadi et al., 2016). Similarly, the NMs influence the dynamics of PPs as the report of Fetsiukh et al. (2021) showed that silica NPs triggered P. polymyxa A26 for producing EPS and increased water-holding capacity and osmotic pressure of biofilm and such reprogrammed bacterium enhanced plant biomass under drought stress. The application of a combo of nanogypsum and P. taiwanensis improved plant growth and soil health, and the metagenomic study revealed the dominance of beneficial microbial groups such as Acidobacteria, Bacteriodetes, Nitrospirae, Proteobacteria, and Planctomycetes in soil (Chaudhary et al., 2021c). The optimized concentration of TiO2 NPs with bacterial treatment increased maize plant growth, germination percentage, leaf area, and chlorophyll content (Kumari et al., 2021). Moreover, algal-based biofertilizers with mineral nanofertilizers can also be a game changer in agricultural productivity (Mahapatra et al., 2022).
Recent studies to determine the combined effect of NMs and PPs on plant growth and development are presented in Table 4. In addition, Figure 2 shows the advantageousness of using a cocktail of NMs and PPs to reap the benefits of agricultural production.
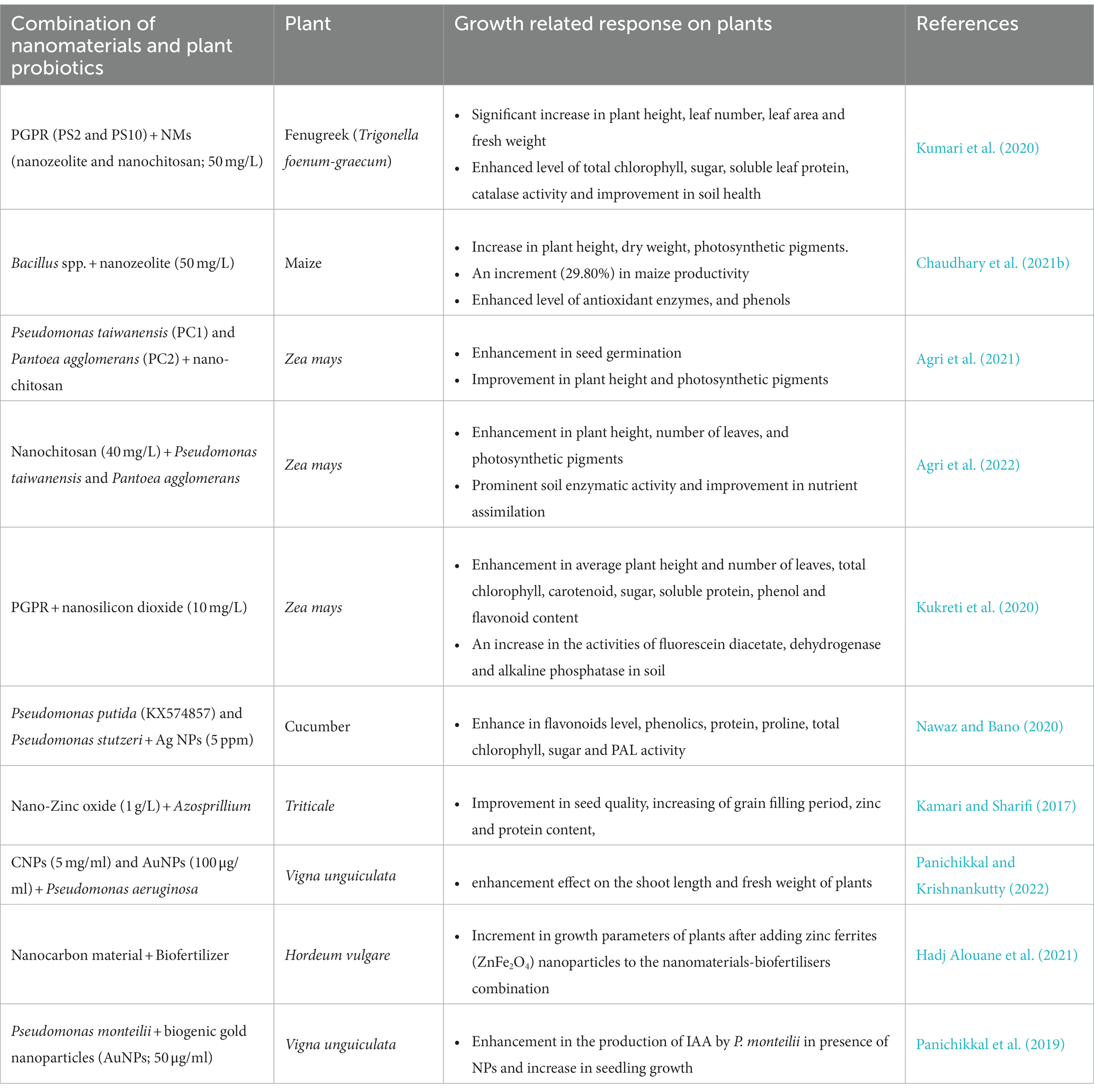
Table 4. Role of combined effects of nanomaterials and plant probiotics in plant growth and development.
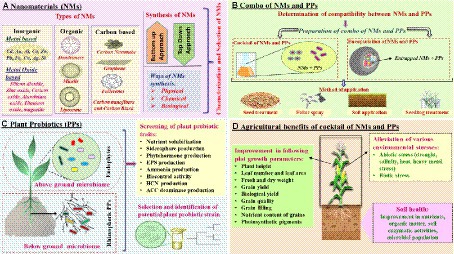
Figure 2. (A) Representation of NMs categorized into the following: inorganic-based (metal-based and metal oxide-based), organic NMs (dendrimers, micells, and liposomes), carbon-based NMs (carbon nanotubes, graphene, fullerenes, carbon nanofibers, and carbon black), and their approaches of synthesis (bottom-up and top-down) with their three physical, chemical, and biological ways of synthesis. (B) The schematization of PPs, especially endophytes and rhizospheric PPs, and their screening on various traits such as nutrient solubilization, production of siderophore, phytohormone, EPS, ammonia, HCN, ACC deaminase, and biocontrol activity. (C) Determination of compatibility between NMs and PPs, and preparation of their combo, either a cocktail of NMs and PPs or the encapsulation of NMs and PPs. Such a combo can be applied by following suitable methods such as seed treatment, foliar spraying, soil application, and seedling treatment. (D) Illustration of the agricultural benefits resulting from the application of a cocktail of NMs and PPs in terms of improvement in plant growth parameters, alleviation of environmental stresses, and prolific effects on soil health.
7. Combo of nanomaterials and plant probiotics in the mitigation of environmental stress
The agriculture sector shows its essentiality in food security as a human population relies on particular crop-based foods for basic diets. However, in the current climate change scenario, crop productivity is experiencing environmental stresses in form of either abiotic or biotic stresses (Xiong et al., 2022). The common instances of abiotic stresses include drought, salinity, heat stress, flood, cold stress, and heavy metal stress (Shikari et al., 2022). On the contrary, pathogens such as bacteria, fungi, and viruses that attack plants are categorized as biotic stressors (Barna, 2022). These categories of stress drastically affect crops in terms of reduction in yield (Anzano et al., 2022). In the coming decades, if the issue of global warming is not solved, the measured portion of arable land might be affected due to various types of abiotic stresses (Shahzad et al., 2021). Therefore, a concept of climate-smart agriculture is in fashion to adopt the strategy to ameliorate the effect of various stresses on crops. The use of agrochemicals to combat the drastic effects of environmental stresses is a leading factor in the contaminating environment and posing a big threat to human health (Omran and Baek, 2022). From the microbial perspective view, the use of plant probiotics can provide an alternative solution for redressing the effects of abiotic and biotic stresses in plants (Mishra et al., 2022). Pant probiotics are a smart player that not only protects the plant from abiotic stress but also reduce the risk of biotic stress by modulating their natural defense (Bhat et al., 2022). Logically, plant probiotics must already be tolerant to various stresses, after which they may only mitigate the effect of various stresses. These special characteristics of tolerance to different types of stress are in fact conferred by the production of exopolysaccharides, the accumulation of osmoprotectants and the production of ACC deaminase, and the activation of different stress-responsive genes (Fadiji et al., 2022). Moreover, when plant probiotics are used as a bioinoculant in plants, they improve the stress-tolerant behavior of host plants by enhancing photosynthetic pigments, accumulating osmolytes, accumulating high phenols, activating antioxidant enzymes, activating stress-responsive genes, and reducting in levels of malondialdehyde and electrolyte leakage (Gamalero and Glick, 2022). Furthermore, plant probiotics mitigate the biotic stress via several mechanisms such as the production of antimicrobial compounds (antibiotics, antifungal, etc.), synthesis of siderophore, volatile compounds (HCN), secretion of enzymes having the capacity to disintegrate pathogen cell wall, and induction of systematic resistance in plants (Boro et al., 2022). Second, the nonfertilizer application is another admirable approach for the fertilizer industry, as they are highly efficient in the context of controlled release of nutrients (Jakhar et al., 2022). However, to combat the negative impact of environmental stresses a systematic application of various nanomaterials such as nanochitosan (Hassan et al., 2021), ZnO NPs (Chanu Thounaojam et al., 2021), nano-selenium (Shalaby et al., 2021), AgNPs (Alabdallah and Hasan, 2021), and carbon nanotubes (CNTs; Faizan et al., 2021) have shown appreciable contribution in improving crop endurance under abiotic stress conditions. Recently, Adil et al. (2022) demonstrated that the application of nano-ZnO (0.12 g/pot) significantly increased photosynthetic pigments (chlorophyll a and b) contents plant height, shoot and spike lengths, root fresh and dry weights, and wheat grain yield under salt stress. Under drought stress conditions, nano-vermicompost application resulted in enhancement in growth, mineral uptake, and activation of antioxidant enzymes in tomatoes (Ahanger et al., 2021). The foliar spray of nanosilicon restored the growth and yield of essential oils of the medicinally important plant feverfew (Tanacetum parthenium) under drought conditions (Esmaili et al., 2022). Nanoparticles exhibit distinctive qualities in plants due to their charge-to-size ratio, such as an improvement in total antioxidant status, which lowers levels of harmful chemicals such as reactive oxygen species (Abdal Dayem et al., 2017). This, in turn, modulates different biochemical and molecular signal transducing pathways, resulting in improved signal perception and, as a result, increased growth and yield potential (Bhatt et al., 2020). However, recent evidence suggests that the coupling effect of plant probiotics (PPs) and NMs may play an excellent role in managing abiotic stress (Azmat et al., 2022; Muhammad et al., 2022; Alharbi et al., 2022a,b). The combo effect of NMs and PPs exhibits various stress ameliorating effects by improving levels of photosynthetic pigments, activities of antioxidant enzymes, total soluble sugars, and reducing stress markers such as MDA content and electrolytic leakage in plants under salt stress (Yasmin et al., 2021; Alharbi et al., 2022a) and drought stress (Akhtar et al., 2021; Azmat et al., 2022). Recently Etesami et al. (2022) deciphered how the combination of nanosilicon and arbuscular mycorrhiza can be a prolific tactic to mitigate environmental stresses in crops and achieve sustainable plant productivity. Table 5 illustrates the combined effect of NMs and PPs in alleviating environmental stresses (salinity, drought, and heavy metal pollution) in plants by demonstrating different mechanisms.
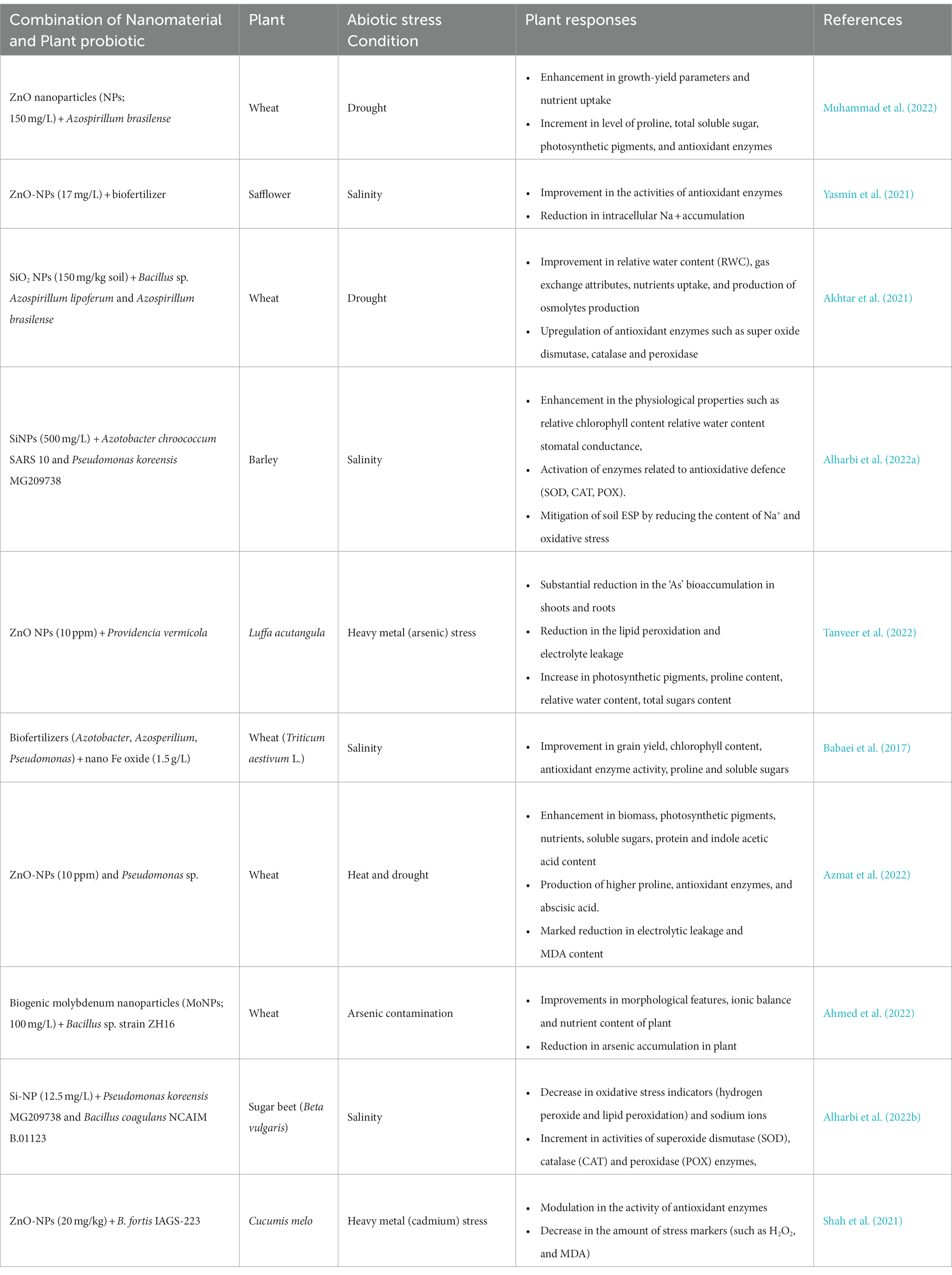
Table 5. Prolific effects of a cocktail of nanomaterials and plant probiotics in alleviating various abiotic stresses in plants.
Combined integration of NPs and PPs to help plants deal with heavy metals and their basic mechanisms involved in the process of phytoremediation and soil remediation. Collective use of Staphylococcus aureus and ZnO NPs detoxifies the effects of chromium on wheat plants and increases its growth, showing a positive impact on plant physiological activities and defense system (Ahmad et al., 2022). Similarly, the joint effect of TiO2 NPs and plant probiotics increased T. repens growth in cadmium-contaminated soil and also improved the accumulation and uptake of this metal by plant (Daryabeigi Zand et al., 2020a). Furthermore, the simultaneous application of nanoscale zero-valent iron (nZVI) and PPs contributed to promoting the phytoremediation of Sb (antimony)-contaminated soils and significantly increased the accumulation capacity of Trifolium repens for Sb (Daryabeigi Zand et al., 2020b). B. subtilis in combination with NMs (ZnO and TiO2) controlled powdery mildew disease in cucumber plants (Hafez et al., 2020). Moreover, nanoencapsulated B. subtilis (Vru1) ameliorated biotic stress by controlling the pathogenic fungus R. solani and decreased the severity of the disease by 75% (Saberi-Rise and Moradi-Pour, 2020). The nanocomposite biofertilizer, which consisted of inclusion complexes of acylated homoserine lactone (AHL)-coated Fe–carbon nanofibers and endospores of P. polymyxa adsorbed in activated carbon beads, demonstrated a good ability to ameliorating effect of biotic stress by preventing Fusarium wilt of chickpea and root rot of wheat (Gahoi et al., 2021).
8. Soil health management through the cocktail of nanomaterials and plant probiotics
Soil is an absolute medium that supports the life of a range of flora and fauna, and provides a better milieu for various microbial activities. The belowground region of soil especially contains rhizospheric and non-rhizospheric environments (Orozco-Mosqueda et al., 2022). Rhizosphere, on the other hand, can be described as a particularly vibrant region due to plant-microbial activities that take part in nutrient cycling (Kumawat et al., 2022). Rhizospheric soil harbors to a variety of beneficial microbiomes that support plants by displaying a range of traits including the solubilization of mineral elements, N2 fixation, siderophore production, and phytohormone synthesis (Mahmud et al., 2021). In addition to this, microbes keep the soil’s nutrient levels balanced through processes such as nitrogen fixation, solubilization of complex inorganic compounds, and mineralization of organic materials (Kaviya et al., 2019). As a result, the soil has a sufficient amount of NPK to support both microbial and plant life. The synthesis of extracellular enzymes by soil microorganisms, such as dehydrogenase, fluorescein diacetate, alkaline phosphatase, and β-glucosidase, contributes to the smooth functioning of the soil environment. These enzymes also serve as a reflection of the microbial activity that takes place in the soil (Kleinert et al., 2018). In addition, the generation of EPS by microorganisms is advantageous in terms of improving soil structure and soil stability (Costa et al., 2018). Due to their extensive roles in soil formation, soil health management, and the remediation of contaminated soil, microorganisms are referred to as “soil probiotics.” In the current scenario, the application of NMs and PPs deciphered a positive impact on soil. Kumari et al. (2020) observed that the application of a combination of NMs (nanozeolite and nanochitosan) and PPs increased soil enzymatic activities such as FDA, dehydrogenase, and alkaline phosphatase and, therefore, showed a growth-stimulating effect on the fenugreek plant. Khati et al. (2017) reported that combining two strains of Bacillus sp. with nanochitosan enhanced the organic carbon content, potassium content, and ammoniacal nitrogen in maize-grown soil. Enzymes that indicate the health of the soil, such as dehydrogenase and alkaline phosphatase, showed a 2- to 3-fold increase after the application of this combination. The study by Kumari et al. (2021) showed that the combination of 10 ppm NPs (TiO2) and bacterial inoculants improved the enzymatic activities (fluorescein diacetate hydrolysis, dehydrogenase, and alkaline phosphatase) of the soil under maize cultivation. In addition, the combination of nanosilicon dioxide and PPs (Pseudomonas taiwanensis and Pantoea agglomerans) improved the pattern in the organic carbon, phosphorus, and potassium content of the cultivated soil and indicated a 1.5- to 2-fold increase in the activities of soil enzymes (dehydrogenase, fuorescein diacetate, and alkaline phosphatase; Kukreti et al., 2020). As the extensive use of agrochemicals has led to a decline in soil quality, an alternative nanobiofertilizers-based strategy can restore soil quality and increase the population of beneficial microbiota. Recent research by Chaudhary et al. (2022b) demonstrated an increase in the microbial population in soil treated with NMs (nanozeolite and nanochitosan) and Bacillus sp. Application of NM should maintain adequate soil microbial population as microbial diversity maintains the elegance of soil fertility level. Through a high-throughput sequencing approach, Khati et al. (2019b) determined the positive impact of nanozeolite on the survival of bacterial populations associated with nutrient cycling and residue degradation.
Although microbial use is usually environmentally acceptable, the combined use of effective microorganisms and nanomaterials is beneficial for improving agricultural production. However, nanoconjugates have not yet been fully determined in the context of environmental concerns. Nanobioferilizers are comparatively less toxic than traditional fertilizers, and very few studies have been reported to decipher the risk associated with the nanomaterial portion of nanobiofertilizer disturbing soil structure and soil microbial activities. Following the nanobiofertilizer application, the nanocomponents are released into the environment and can reach or drain into the soil depending on the type of soil and its properties (Sambangi et al., 2022). NMs, in soil, may show toxic effects on plant growth-promoting microbes, especially nitrogen-fixing bacteria and mineral-solubilizing bacteria, and thus a consequent shift in the bacterial community can affect the functioning of the local soil ecosystem (Chavan et al., 2020). Most NPs based on metal and metal oxide can show the highest degradative effect against microorganisms due to their toxic effects by affecting cell membrane architecture, enzymatic and metabolic activities, and nutrient availability, which ultimately results in microbial death (Kumar et al., 2018; Upadhayay et al., 2019). Recent studies have illustrated the destructive effects of NMs on soil microorganisms involved in various important activities. Ma et al. (2023) showed that the application of elevated levels of CO2 (590 μmol mol−1) and titanium dioxide NPs disrupted soil bacterial activities involved in the nitrogen and carbon cycles. The beneficial contribution of NMs glimpses as their application allows slow and sustained release of nutrients, supporting plant growth while conserving the diversity of the beneficial microbiome. Their toxicity can be attributed to their physical properties, and the ambiguous dose and structure of the exposed microbial community (Chhipa, 2021). Table 6 depicts the negative consequences of using metal and metal oxide-based NMs. An increasing number of researchers are focusing on this problem. Acquired results show contradiction; some authors illustrated evidence of safer use of NMs, while some researchers reported significant risk (Kolesnikov et al., 2019). However, the following points can be considered for the safer use of NMs with lesser toxic effects on the environment, such as (a) an eco-nanotechnological study for massively producing NMs due to nanotechnological advancement; (b) a proper and adequate characterization of NMs on physical and chemical basis and evaluation of environmentally safe exposure doses of NMs before their widespread applications; (c) proper monitoring and risk assessment of NMs use; (d) a comprehensive assessment to decipher the impact of NM as soil pollutants and their potential destructive behavior on soil microbial diversity and their functions.
9. Nanoencapsulation of plant probiotics: How can it shape crop growth?
Nanoencapsulation research is being increased in the last few years in response to the rising need for PPs (Nayana et al., 2020; Saberi Riseh et al., 2022b). Such kind of formulation can address the issues of free-form formulations of PPs (Bala, 2022). Nanoencapsulation can improve the efficacy of PPs by extending their shelf life and providing a controlled release of bio-component (Pour et al., 2019). After inoculation, several factors affect the competency of PPs in the natural environment in terms of ineffective colonization of plant roots by applied microbial inoculant, lesser microbial activity in the rhizospheric milieu, and decline in microbial population (Ahmad et al., 2011; Khare and Arora, 2015). Since a minimum number of inoculant cells (106 and 107) is a critical factor in deciding the positive impact on plants (de Moraes et al., 2021). Thus, PPs need suitable physical protection for an extended period. As a novel approach, the nanoencapsulated PPs is providing a better platform for enhancing crop growth and amelioration of abiotic and biotic stresses (Ravichandran et al., 2022; Saberi Riseh et al., 2022a). The nanoencapsulation provides stability and reproducibility of entrapped PPs by enhancing their resistance to UV radiation, heat, and desiccation (Balla et al., 2022). Encapsulating nanoparticles with biofertilizer is a step in the production of nanobiofertilizer. The encapsulation of biofertilizers and biocontrol agents works well in biopolymer-based nanocomposites (Akhtar et al., 2022). In addition, nanoencapsulation prevents bacterial strains from mechanical stress and lowers nutrient release, which further increases the efficacy of this product (Kumari et al., 2020). Biofertilizer cells are incorporated into the nanomaterial capsule by a process called encapsulation, and this involves the application of non-hazardous, biodegradable materials such as starch and calcium alginate (Vejan et al., 2019; Akhtar et al., 2022). Three crucial steps are involved in the production of nanobiofertilizers: (1) the growth of culture for biofertilizer, (2) the encapsulation of culture with nanoparticles, and (3) the assessment of its efficacy, quality, purity, and shelf life (Akhtar et al., 2022). Microcapsules can also be used to make nanobiofertilizer. Its production includes mixing PGPR suspension in a 2:1 ratio with a solution of 1.5% sodium alginate, 3% starch, and 4% bentonite (Akhtar et al., 2022; Pour et al., 2022). After washing the microcapsules in sterile distilled water, the mixture is covered with the crosslinking calcium chloride solution (Adjuik et al., 2022).
Salicylic acid and nanoparticles have also been combined to form a nanobiofertilizer (Gupta et al., 2019). This technique involves mixing the biofertilizer with sodium alginate (2%), ZnO NPs (1 g/ml), and salicylic acid (1.5 mM). Then, 1-mm beads are prepared, shaped, and air-dried in the solution before incubating at 4°C with calcium chloride (3% solution; Panichikkal et al., 2019; Akhtar et al., 2022).
Pseudomonas sp. (DN18) entrapped in the alginate beads along with the salicylic acid and the ZnO NPs demonstrated antifungal activity against Sclerotium rolfsii and showed superior plant growth-promoting activity on Oryza sativa seedlings compared to the free-living bacterial strain (Panichikkal et al., 2021). Nanoencapsulation of P. fluorescens (VUPF5) and B. subtilis (VRU1; using silica nanoparticles and carbon nanotubes) and their metabolites improved pistachio micropropagation via a significant enhancement in the root length and proliferation (Pour et al., 2019). Nanoencapsulated Bacillus subtilis (VRU1) prepared with sodium alginate, starch, and bentonite have shown effectiveness in controlling the proliferation of Rhizoctonia solani and increased the bean vegetative growth parameters (Saberi-Rise and Moradi-Pour, 2020). “Sodium alginate–gelatin microcapsules” containing nanomaterials (SiO2 and carbon nanotubes) and PPs Bacillus velezensis demonstrated synergistic suppression of pathogens (Phytophthora drechsleri) in Pistacia vera L. (pistachio; Moradi Pour et al., 2022). The study by De Gregorio et al. (2017) exhibited the nanofiber-immobilized rhizobacteria (P. agglomerans and B. caribensis) prepared by electrospinning and observed its efficiency as seed bioinoculant in terms of improving the length of root, dry weight of root and shoot, leaf, and the number of soybeans. The bacteria (Pseudomonas stutzeri) encapsulated in the coating composed of N-hydroxysuccinimide (NHS)-modified poly γ-PGA and Ca ions exhibited remarkable resistance against harsh conditions and showed better plant growth potential (Yang et al., 2021).
10. Cocktail of nanomaterials and plant probiotics: Understanding in the context of the bioeconomy
The concept of the bioeconomy is well described in the context of biofuel production (Zilberman et al., 2018), but the role of agriculture is also justified in strengthening the bioeconomy (Upadhayay et al., 2022c). In the context of agriculture, the bioeconomy can be described as improving crop productivity through the use of various resources. Indeed, innovations in life sciences, agriculture, biotechnology, and the evolving wisdom in these sectors provide the ultimate ground for sustainable production and sustain a stable bioeconomy. The lack of essential nutrients in the soil poses significant problems for farmers due to several factors including intensive and poor farming practices (Elbasiouny et al., 2022). In addition to these factors, soil types and different agroclimatic conditions at different altitudes are common features that contribute to declines in crop growth and production, and adversely affect the socioeconomics of farmers (Sivakumar, 2021; Upadhayay et al., 2022c). Various ways of soil nutrient management are used, such as the use of chemical fertilizers, which reduces soil fertility, have a detrimental impact on local soil microbial ecology, and cause health problems for consumers. The production of agrochemicals by various manufacturers around the world is effective and beneficial in increasing crop productivity, but it is more expensive and not ideal for underprivileged farmers. However, a variety of techniques (agronomic, breeding, and genetic modifications) are used to improve the nutrient content and yield of plants (Ahmar et al., 2020). On the other hand, in areas with a predominantly rural population, these methods are seen as both lucrative and undesirable. In addition, these crop yield-increasing techniques are not consumable as they require more effort and technical skill. In addition, the quality of the harvested commodities must be high so that farmers may sell them for a reasonable price. However, the use of nanotechnology has advanced agriculture, and nano-based fertilizers, insecticides, and herbicides are being used to protect and produce crops in a prodigious manner (Chand Mali et al., 2020). An increase in gain yield has the potential to play a significant job in the improvement of the bioeconomy. Babaei et al. (2017) reported a 17.40% increment in the grain yield of wheat by the application of nano-Zn–Fe oxide in comparison to the control. The nano-urea treatment (3 g/kg) exhibited maximum biological yield (332.7 g/bag) and economic yields (283.1 g/bag) at the third flush (Naim et al., 2020). On the other hand, PPs as potential biostimulators showed the highest grain yield in various crops such as wheat (between 9.6 and 29.29%) by Bacillus sp., (Öksel et al., 2022), rice (3.35 t/ha) by B. subtilis and B. megatherium strain (Abd El-Mageed et al., 2022), and maize (5,880 kg/ha) by P. putida (Mubeen et al., 2021). However, in recent years, the combined application of NM and PP has led to a breakthrough in the agricultural sector, especially in terms of increasing crop yield (Akhtar et al., 2022). Combined application of plant probiotics (Azotobacter) and nano-Zn–Fe oxide showed an 88% increase in wheat grain yield compared to water-restricted conditions (Seyed Sharifi et al., 2020). Hafez et al. (2021) observed that the synergy of rhizobacteria and 500 mg SiNPs per liter showed an increase in maize yield (6325.4 kg/ha) and also improved nutrient uptake such as NPK in plants. This synergistic strategy of utilizing microbes and nanomaterials is described in this article as an ecologically sound solution to optimize plant growth and yield. The use of agrochemicals is reduced in this way, and the combined use of NM and PP will significantly increase crop yield. Thus, the detection, characterization, and competence of PPs as prospective bioinoculants and as a synergistic partner of suitable NMs for improving yield appears to be promising goals in order to (i) in vitro evaluation of PPs from rhizospheric soils of plants and selection of cultivable microorganisms on the basis of multifarious plant growth-promoting traits, (ii) determination of the compatibility of a prospective PPs strain with suitable nanocompounds, (iii) improvement in the overall productivity of crops under the application of a cocktail of PPs and NMs, (iv) evaluating uptake and density of nutrients in different plant parts to illustrate the quality of crop harvest, (v) analyzing the soil health and dynamics of the inoculated bacterial population from field plots and conserving proficient microbial pools for future use, and (vi) ultimately reducing reliance on agrochemicals showing harmful impacts.
11. Future prospects for nano-biofertilizers: A roadmap
Sustainable agricultural practice can be represented as the coordinated action of abiotic and biotic factors to maintain the stability of agricultural production and soil nutrient balance. Nonetheless, the benevolent effect of PPs supports plant growth in a very harmless way and, hence, it is included as a main choice for use in agricultural applications. The incorporation of nanotechnology is both modernizing agriculture and winning consumer acceptance as nano-based fertilizers (Xin et al., 2020). However, the coming decade is eagerly waiting to further design the technology of combined application of NMs and PPs in the agricultural sector. The encapsulation of both PPs and NMs has the unique property of showing productiveness in the context of a smart farming system to improve crop yield, plant-derived food quality, and nutritional value of plant-based products.
The core agricultural sector needs attention in the future and may require the following ways to effectively apply the combination of NMs and PPs.
• The properties of NMs such as size, surface chemistry, structure, dose, and toxicity should be carefully monitored.
• Novel analytical methods are needed to develop NMs with unique properties, their detection, validation, effects under field conditions, and associated toxicity.
• Establish guidelines for the responsible use of NM in agriculture and a roadmap to reduce the risk of using nano-based products.
• The compatibility of PPs with target NMs must be established when NMs are used as a synergistic component of PPs.
• As a variety of environmental factors affect the microbial population in the soil, a PPs strain with the ability to survive under diverse stress conditions should be selected for subsequent application.
• The combined application of NMs and PPs should preserve the local microbial community and must not be detrimental to the soil ecology. The technology for the development of nanobiofertilizers has a significant impact on agricultural yields; therefore, the knowledge related to the effective application of nanobiofertilizer should be communicated from researchers to authorities and industrial sectors.
• A new venue for discussion needs to be established, and it should be used to discuss the significant impact that nanobiofertilizers have on agriculture, the economy, and human life.
• The performance of novel nano-based materials or products should be compared to that of previously formulated products.
• Multiple field studies should be conducted at diverse sites to evaluate the performance of created nanobioformulations in terms of their efficacy and environmental impact.
12. Conclusion
Improving food-based crop production is the primary need for a rapidly growing world population. This goal can be achieved through strategies that use agriculturally important microbe and nanomaterial-based fertilizers without relying heavily on agrochemicals. The abundant scientific literature supports the effectiveness of using NMs and microorganisms as PPs in improving plant growth, ameliorating environmental stresses, and improving soil health. In recent years, however, scientists have been keenly interested in investigating the synergistic effects of NMs and PPs in agriculture to maximize crop yields and maintain soil health. In this cocktail of NMs and PPs, nanomaterials serve as effective sources of nutrients for plants, while PPs stimulate plant growth, therefore serving as natural crop vitalizers. According to the recent literature, the synergistic effect of NMs and PPs has played a promising role in achieving the following target: (a) maximization of crop productivity and crop quality, (b) assurance of food security for the rapidly escalating global population, (c) amelioration of the drastic effects of various environmental stresses such as drought, salinity, and cold, as well as biotic stresses, (d) maintenance of soil health, (e) reduction of the massive reliance on chemical-based fertilizers, and (f) strengthening of the bioeconomy by improving grain yield, grain quality, and biomass in a sustainable way without showing negative impact on the environment. The breakthroughs in nanotechnology have also facilitated the inclusion of plant probiotic strains within the ideal nanomaterials or the entrapment of both NMs and PPs within a suitable carrier. In addition to the controlled and consistent supply of both NMs and PPs, this strategy retains the effectiveness and longevity of the PPs and exhibits a positive impact on crop productivity. In addition, the safe dose of NMs must be determined from an environmental perspective, and a risk assessment must be conducted to ensure that NMs are not hazardous to local soil microbial populations. In conclusion, the application of NMs and PPs in a synergistic manner is demonstrated as an efficient way of improving the quality and production of food-based crops and strengthening the bioeconomy. Furthermore, detailed investigations are also required to develop a customized cocktail of NMs and PPs, understanding their controlled and targeted delivery as well as their molecular mechanisms in plants, to pave the way for sustainable agriculture.
Author contributions
VU and MC: writing of original draft of the manuscript. All authors contributed to the article and approved the submitted version.
Acknowledgments
The authors gratefully acknowledge the Department of Microbiology, College of Basic Sciences and Humanities, Dr. Rajendra Prasad Central Agricultural University, Pusa, Samastipur - 848 125, Bihar, India.
Conflict of interest
The authors declare that the study was conducted in the absence of any commercial or financial relationships that could be construed as a potential conflict of interest.
Publisher’s note
All claims expressed in this article are solely those of the authors and do not necessarily represent those of their affiliated organizations, or those of the publisher, the editors and the reviewers. Any product that may be evaluated in this article, or claim that may be made by its manufacturer, is not guaranteed or endorsed by the publisher.
References
Abd El-Mageed, T. A., Abd El-Mageed, S. A., El-Saadony, M. T., Abdelaziz, S., and Abdou, N. M. (2022). Plant growth-promoting rhizobacteria improve growth, morph-physiological responses, water productivity, and yield of rice plants under full and deficit drip irrigation. Rice 15:16. doi: 10.1186/s12284-022-00564-6
Abdal Dayem, A., Hossain, M., Lee, S., Kim, K., Saha, S., Yang, G.-M., et al. (2017). The role of reactive oxygen species (ROS) in the biological activities of metallic nanoparticles. Int. J. Mol. Sci. 18:120. doi: 10.3390/ijms18010120
Abdelkhalek, A., El-Gendi, H., Al-Askar, A. A., Maresca, V., Moawad, H., Elsharkawy, M. M., et al. (2022). Enhancing systemic resistance in faba bean (Vicia faba L.) to bean yellow mosaic virus via soil application and foliar spray of nitrogen-fixing Rhizobium leguminosarum bv. viciae strain 33504-Alex1. Front. Plant Sci. 13:933498. doi: 10.3389/fpls.2022.933498
Abedi, S., Iranbakhsh, A., Oraghi Ardebili, Z., and Ebadi, M. (2021). Nitric oxide and selenium nanoparticles confer changes in growth, metabolism, antioxidant machinery, gene expression, and flowering in chicory (Cichorium intybus L.): potential benefits and risk assessment. Environ. Sci. Pollut. Res. Int. 28, 3136–3148. doi: 10.1007/s11356-020-10706-2
Abigail, E. A., and Chidambaram, R. (2017). “Nanotechnology in herbicide resistance” in Nanostructured materials - fabrication to applications, ed. M. S. Seehra (InTech), 207–212.
Abou-Sreea, A. I. B., Kamal, M., El Sowfy, D. M., Rady, M. M., Mohamed, G. F., Al-Dhumri, S. A., et al. (2022). Small-sized nanophosphorus has a positive impact on the performance of fenugreek plants under soil-water deficit stress: a case study under field conditions. Biology 11:115. doi: 10.3390/biology11010115
Adil, M., Bashir, S., Bashir, S., Aslam, Z., Ahmad, N., Younas, T., et al. (2022). Zinc oxide nanoparticles improved chlorophyll contents, physical parameters, and wheat yield under salt stress. Front. Plant Sci. 13:932861. doi: 10.3389/fpls.2022.932861
Adjuik, T. A., Nokes, S. E., and Montross, M. D. (2022). Evaluating the feasibility of using lignin–alginate beads with starch additive for entrapping and releasing Rhizobium spp. J. Appl. Polym. Sci. 139:e53181. doi: 10.1002/app.53181
Agri, U., Chaudhary, P., and Sharma, A. (2021). In vitro compatibility evaluation of agriusable nanochitosan on beneficial plant growth-promoting rhizobacteria and maize plant. Natl. Acad. Sci. Lett. 44, 555–559. doi: 10.1007/s40009-021-01047-w
Agri, U., Chaudhary, P., Sharma, A., and Kukreti, B. (2022). Physiological response of maize plants and its rhizospheric microbiome under the influence of potential bioinoculants and nanochitosan. Plant Soil 474, 451–468. doi: 10.1007/s11104-022-05351-2
Ahanger, M. A., Qi, M., Huang, Z., Xu, X., Begum, N., Qin, C., et al. (2021). Improving growth and photosynthetic performance of drought stressed tomato by application of nano-organic fertilizer involves up-regulation of nitrogen, antioxidant and osmolyte metabolism. Ecotoxicol. Environ. Saf. 216:112195. doi: 10.1016/j.ecoenv.2021.112195
Ahmad, F., Husain, F. M., and Ahmad, I. (2011). “Rhizosphere and root colonization by bacterial inoculants and their monitoring methods: a critical area in PGPR research” in Microbes and microbial technology eds. I. Ahmad, F. Ahmad, and J. Pichtel (Springer, New York), 363–391.
Ahmad, S., Mfarrej, M. F. B., El-Esawi, M. A., Waseem, M., Alatawi, A., Nafees, M., et al. (2022). Chromium-resistant Staphylococcus aureus alleviates chromium toxicity by developing synergistic relationships with zinc oxide nanoparticles in wheat. Ecotoxicol. Environ. Saf. 230:113142. doi: 10.1016/j.ecoenv.2021.113142
Ahmar, S., Gill, R. A., Jung, K.-H., Faheem, A., Qasim, M. U., Mubeen, M., et al. (2020). Conventional and molecular techniques from simple breeding to speed breeding in crop plants: recent advances and future outlook. Int. J. Mol. Sci. 21:2590. doi: 10.3390/ijms21072590
Ahmed, T., Noman, M., Luo, J., Muhammad, S., Shahid, M., Ali, M. A., et al. (2021). Bioengineered chitosan-magnesium nanocomposite: a novel agricultural antimicrobial agent against Acidovorax oryzae and Rhizoctonia solani for sustainable rice production. Int. J. Biol. Macromol. 168, 834–845. doi: 10.1016/j.ijbiomac.2020.11.148
Ahmed, T., Noman, M., Rizwan, M., Ali, S., Ijaz, U., Nazir, M. M., et al. (2022). Green molybdenum nanoparticles-mediated bio-stimulation of Bacillus sp. strain ZH16 improved the wheat growth by managing in planta nutrients supply, ionic homeostasis and arsenic accumulation. J. Hazard. Mater. 423:127024. doi: 10.1016/j.jhazmat.2021.127024
Akhtar, N., Ilyas, N., Mashwani, Z.-U.-R., Hayat, R., Yasmin, H., Noureldeen, A., et al. (2021). Synergistic effects of plant growth promoting rhizobacteria and silicon dioxide nano-particles for amelioration of drought stress in wheat. Plant Physiol. Biochem. 166, 160–176. doi: 10.1016/j.plaphy.2021.05.039
Akhtar, N., Ilyas, N., Meraj, T. A., Pour-Aboughadareh, A., Sayyed, R. Z., Mashwani, Z. U., et al. (2022). Improvement of plant responses by nanobiofertilizer: a step towards sustainable agriculture. Nano 12:965. doi: 10.3390/nano12060965
Alabdallah, N. M., and Hasan, M. M. (2021). Plant-based green synthesis of silver nanoparticles and its effective role in abiotic stress tolerance in crop plants. Saudi J. Biol. Sci. 28, 5631–5639. doi: 10.1016/j.sjbs.2021.05.081
Alharbi, K., Hafez, E., Omara, A. E.-D., Awadalla, A., and Nehela, Y. (2022b). Plant growth promoting rhizobacteria and silica nanoparticles stimulate sugar beet resilience to irrigation with saline water in salt-affected soils. Plan. Theory 11:3117. doi: 10.3390/plants11223117
Alharbi, K., Rashwan, E., Mohamed, H. H., Awadalla, A., Omara, A. E.-D., Hafez, E. M., et al. (2022a). Application of silica nanoparticles in combination with two bacterial strains improves the growth, antioxidant capacity and production of barley irrigated with saline water in salt-affected soil. Plan. Theory 11:2026. doi: 10.3390/plants11152026
Ali, E. F., El-Shehawi, A. M., Ibrahim, O. H. M., Abdul-Hafeez, E. Y., Moussa, M. M., and Hassan, F. A. S. (2021). A vital role of chitosan nanoparticles in improvisation the drought stress tolerance in Catharanthus roseus (L.) through biochemical and gene expression modulation. Plant Physiol. Biochem. 161, 166–175. doi: 10.1016/j.plaphy.2021.02.008
Ali, Z., Ullah, R., Tuzen, M., Ullah, S., Rahim, A., and Saleh, T. A. (2022). Colorimetric sensing of heavy metals on metal doped metal oxide nanocomposites: a review. Tren. Environ. Anal. Chem. 37:e00187. doi: 10.1016/j.teac.2022.e00187
Amirifar, A., Hemati, A., Asgari Lajayer, B., Pandey, J., and Astatkie, T. (2022). “Impact of various environmental factors on the biosynthesis of alkaloids in medicinal plants” in Environmental challenges and solutions, ed. T. Aftab (Springer Cham), 229–248.
Anzano, A., Bonanomi, G., Mazzoleni, S., and Lanzotti, V. (2022). Plant metabolomics in biotic and abiotic stress: a critical overview. Phytochem. Rev. 21, 503–524. doi: 10.1007/s11101-021-09786-w
Arif, I., Batool, M., and Schenk, P. M. (2020). Plant microbiome engineering: expected benefits for improved crop growth and resilience. Trends Biotechnol. 38, 1385–1396. doi: 10.1016/j.tibtech.2020.04.015
Armenia, I., Cuestas Ayllón, C., Torres Herrero, B., Bussolari, F., Alfranca, G., Grazú, V., et al. (2022). Photonic and magnetic materials for on-demand local drug delivery. Adv. Drug Deliv. Rev. 191:114584. doi: 10.1016/j.addr.2022.114584
Asghar, W., Akça, M. O., Akça, H., Tarf, O. J., Kataoka, R., and Turgay, O. C. (2022). “Alternative strategies to synthetic chemical fertilizers: revitalization of soil quality for sustainable agriculture using organic-based approaches” in New and Future Developments in Microbial Biotechnology and Bioengineering, eds. H. B. Singh, and A. Vaishnav (Elsevier), 1–30.
Avila-Quezada, G. D., Ingle, A. P., Golińska, P., and Rai, M. (2022). Strategic applications of nano-fertilizers for sustainable agriculture: benefits and bottlenecks. Nanotechnol. Rev. 11, 2123–2140. doi: 10.1515/ntrev-2022-0126
Azam, M., Bhatti, H. N., Khan, A., Zafar, L., and Iqbal, M. (2022). Zinc oxide nano-fertilizer application (foliar and soil) effect on the growth, photosynthetic pigments and antioxidant system of maize cultivar. Biocatal. Agric. Biotechnol. 42:102343. doi: 10.1016/j.bcab.2022.102343
Azmat, A., Tanveer, Y., Yasmin, H., Hassan, M. N., Shahzad, A., Reddy, M., et al. (2022). Coactive role of zinc oxide nanoparticles and plant growth promoting rhizobacteria for mitigation of synchronized effects of heat and drought stress in wheat plants. Chemosphere 297:133982. doi: 10.1016/j.chemosphere.2022.133982
Babaei, K., Seyed Sharifi, R., Pirzad, A., and Khalilzadeh, R. (2017). Effects of bio fertilizer and nano Zn-Fe oxide on physiological traits, antioxidant enzymes activity and yield of wheat (Triticum aestivum L.) under salinity stress. J. Plant Interact. 12, 381–389. doi: 10.1080/17429145.2017.1371798
Bala, K. (2022). “Microbial fertilizer as an alternative to chemical fertilizer in modern agriculture” in Beneficial microorganisms in agriculture, eds. R. Prasad and S. H. Zhang (Springer, Singapore), 111–130.
Balah, M. A., and Pudake, R. N. (2019). “Use nanotools for weed control and exploration of weed plants in nanotechnology” in Nanoscience for Sustainable Agriculture, eds. R. Pudake, N. Chauhan, and C. Kole (Springer, Cham), 207–231.
Balla, A., Silini, A., Cherif-Silini, H., Chenari Bouket, A., Alenezi, F. N., and Belbahri, L. (2022). Recent advances in encapsulation techniques of plant growth-promoting microorganisms and their prospects in the sustainable agriculture. Appl. Sci. 12:9020. doi: 10.3390/app12189020
Bana, R. C., Yadav, S. S., Shivran, A. C., Singh, P., and Kudi, V. K. (2020). Site-specific nutrient management for enhancing crop productivity. Int. Res. J. Pure Appl. Chem. 21, 17–25. doi: 10.9734/irjpac/2020/v21i1530249
Banerjee, A., Sarkar, S., Cuadros-Orellana, S., and Bandopadhyay, R. (2019). “Exopolysaccharides and biofilms in mitigating salinity stress: the biotechnological potential of halophilic and soil-inhabiting PGPR microorganisms” in Soil biology, eds. B. Giri & A. Varma (Springer, Cham), 133–153.
Barna, B. (2022). Manipulation of senescence of plants to improve biotic stress resistance. Life 12:1496. doi: 10.3390/life12101496
Beig, B., Niazi, M. B. K., Sher, F., Jahan, Z., Malik, U. S., Khan, M. D., et al. (2022). Nanotechnology-based controlled release of sustainable fertilizers. A review. Environ. Chem. Lett. 20, 2709–2726. doi: 10.1007/s10311-022-01409-w
Bhat, B. A., Tariq, L., Nissar, S., Islam, S. T., Islam, S. U., Mangral, Z., et al. (2022). The role of plant-associated rhizobacteria in plant growth, biocontrol and abiotic stress management. J. Appl. Microbiol. 133, 2717–2741. doi: 10.1111/jam.15796
Bhatt, D., Bhatt, M. D., Nath, M., Dudhat, R., Sharma, M., and Bisht, D. S. (2020). “Application of nanoparticles in overcoming different environmental stresses” in Protective chemical agents in the amelioration of plant abiotic stress. eds. A. Roychoudhury and D. K. Tripathi (John Wiley & Sons Ltd) 635–654. doi: 10.1002/9781119552154.ch32
Boregowda, N., Jogigowda, S. C., Bhavya, G., Sunilkumar, C. R., Geetha, N., Udikeri, S. S., et al. (2022). Recent advances in nanoremediation: carving sustainable solution to clean-up polluted agriculture soils. Environ. Pollut. 297:118728. doi: 10.1016/j.envpol.2021.118728
Boro, M., Sannyasi, S., Chettri, D., and Verma, A. K. (2022). Microorganisms in biological control strategies to manage microbial plant pathogens: a review. Arch. Microbiol. 204:666. doi: 10.1007/s00203-022-03279-w
Cai, L., Cai, L., Jia, H., Liu, C., Wang, D., and Sun, X. (2020). Foliar exposure of Fe3O4 nanoparticles on Nicotiana benthamiana: evidence for nanoparticles uptake, plant growth promoter and defense response elicitor against plant virus. J. Hazard. Mater. 393:122415. doi: 10.1016/j.jhazmat.2020.122415
Carro, L., and Nouioui, I. (2017). Taxonomy and systematics of plant probiotic bacteria in the genomic era. AIMS Microbiol. 3, 383–412. doi: 10.3934/microbiol.2017.3.383
Chand Mali, S., Raj, S., and Trivedi, R. (2020). Nanotechnology a novel approach to enhance crop productivity. Biochem. Biophys. Rep. 24:100821. doi: 10.1016/j.bbrep.2020.100821
Chanu Thounaojam, T., Thounaojam, T. M., and Upadhyaya, H. (2021). “Role of zinc oxide nanoparticles in mediating abiotic stress responses in plant” in Zinc-Based Nanostructures for Environmental and Agricultural Applications, eds. A. Kamel, and Abd-Elsalam (Elsevier), 323–337.
Chaudhary, P., Agri, U., Chaudhary, A., Kumar, A., and Kumar, G. (2022a). Endophytes and their potential in biotic stress management and crop production. Front. Microbiol. 13:933017. doi: 10.3389/fmicb.2022.933017
Chaudhary, P., Chaudhary, A., Bhatt, P., Kumar, G., Khatoon, H., Rani, A., et al. (2022b). Assessment of soil health indicators under the influence of nanocompounds and Bacillus spp. in field condition. Front. Environ. Sci. 9:769871. doi: 10.3389/fenvs.2021.769871
Chaudhary, P., Chaudhary, A., Parveen, H., Rani, A., Kumar, G., Kumar, R., et al. (2021a). Impact of nanophos in agriculture to improve functional bacterial community and crop productivity. BMC Plant Biol. 21:519. doi: 10.1186/s12870-021-03298-7
Chaudhary, P., Khati, P., Chaudhary, A., Gangola, S., Kumar, R., and Sharma, A. (2021b). Bioinoculation using indigenous bacillus spp. improves growth and yield of Zea mays under the influence of nanozeolite. Biotech : 11. doi: 10.1007/s13205-020-02561-2
Chaudhary, P., Khati, P., Chaudhary, A., Maithani, D., Kumar, G., and Sharma, A. (2021c). Cultivable and metagenomic approach to study the combined impact of nanogypsum and Pseudomonas taiwanensis on maize plant health and its rhizospheric microbiome. PLoS One 16:e0250574. doi: 10.1371/journal.pone.0250574
Chaudhary, P., Khati, P., Gangola, S., Kumar, A., Kumar, R., and Sharma, A. (2021d). Impact of nanochitosan and bacillus spp. on health, productivity and defence response in Zea mays under field condition. Biotech 11:237. doi: 10.1007/s13205-021-02790-z
Chaudhary, P., and Sharma, A. (2019). Response of nanogypsum on the performance of plant growth promotory bacteria recovered from nanocompound infested agriculture field. Environ. Ecol. 37, 363–372.
Chaudhary, P., Sharma, A., Chaudhary, A., Khati, P., Gangola, S., and Maithani, D. (2021e). Illumina based high throughput analysis of microbial diversity of maize rhizosphere treated with nanocompounds and bacillus sp. Appl. Soil Ecol. 159:103836. doi: 10.1016/j.apsoil.2020.103836
Chaudhary, P., Singh, S., Chaudhary, A., Sharma, A., and Kumar, G. (2022c). Overview of biofertilizers in crop production and stress management for sustainable agriculture. Front. Plant Sci. 13:930340. doi: 10.3389/fpls.2022.930340
Chavan, S., Sarangdhar, V., and Nadanathangam, V. (2020). Toxicological effects of TiO2 nanoparticles on plant growth promoting soil bacteria. Emerg. Contam. 6, 87–92. doi: 10.1016/j.emcon.2020.01.003
Chen, H., Chen, J., Qi, Y., Chu, S., Ma, Y., Xu, L., et al. (2022). Endophytic fungus Cladosporium tenuissimum DF11, an efficient inducer of tanshinone biosynthesis in Salvia miltiorrhiza roots. Phytochemistry 194:113021. doi: 10.1016/j.phytochem.2021.113021
Chen, C., Guo, L., Chen, Y., Qin, P., and Wei, G. (2023). Pristine and sulfidized zinc oxide nanoparticles alter bacterial communities and metabolite profiles in soybean rhizocompartments. Sci. Total Environ. 855:158697. doi: 10.1016/j.scitotenv.2022.158697
Chhipa, H. (2021). “Nano-toxicity to microbes: potential implications of nanomaterials on microbial activity” in Environmental chemistry for a sustainable world, eds. V. Kumar, P. Guleria, S. Ranjan, N. Dasgupta, and E. Lichtfouse (Springer, Cham), 99–123.
Chitara, M. K., Chauhan, S., and Singh, R. P. (2021). “Bioremediation of polluted soil by using plant growth–promoting rhizobacteria” in Microbial rejuvenation of polluted environment, eds. Panpatte, D.G., Jhala, Y.K. (Springer, Singapore), 203–226.
Chitara, M. K., Keswani, C., Bisen, K., Singh, V., Singh, S. P., Sarma, B. K., et al. (2017). “Improving crop performance under heat stress using thermotolerant agriculturally important microorganisms” in Advances in PGPR research, eds. H. B. Singh, B. K. Sarma, C. Keswani (Wallingford UK: CABI), 296–305.
Chitara, M. K., Singh, R. P., Gupta, P. K., Mishra, D., Jatav, S. S., Sharma, S., et al. (2022). “The Risk Associated with Crop Ecosystem Management and Pesticides Pollution” in Ecosystem Services, ed. : H. S. Jatav (Nova Science Publishers, Inc.), 151–163.
Costa, O. Y. A., Raaijmakers, J. M., and Kuramae, E. E. (2018). Microbial extracellular polymeric substances: ecological function and impact on soil aggregation. Front. Microbiol. 9:1636. doi: 10.3389/fmicb.2018.01636
Dalir Abdolahinia, E., Barati, G., Ranjbar-Navazi, Z., Kadkhoda, J., Islami, M., Hashemzadeh, N., et al. (2022). Application of nanogels as drug delivery systems in multicellular spheroid tumor model. J. Drug Deliv. Sci. Technol. 68:103109. doi: 10.1016/j.jddst.2022.103109
Daniel, A. I., Fadaka, A. O., Gokul, A., Bakare, O. O., Aina, O., Fisher, S., et al. (2022). Biofertilizer: the future of food security and food safety. Microorganisms 10:1220. doi: 10.3390/microorganisms10061220
Daryabeigi Zand, A., Tabrizi, A. M., and Heir, A. V. (2020a). Application of titanium dioxide nanoparticles to promote phytoremediation of Cd-polluted soil: contribution of PGPR inoculation. Biorem. J. 24, 171–189. doi: 10.1080/10889868.2020.1799929
Daryabeigi Zand, A., Tabrizi, A. M., and Heir, A. V. (2020b). The influence of association of plant growth-promoting rhizobacteria and zero-valent iron nanoparticles on removal of antimony from soil by Trifolium repens. Environ. Sci. Pollut. Res. Int. 27, 42815–42829. doi: 10.1007/s11356-020-10252-x
Das, P. P., Singh, K. R., Nagpure, G., Mansoori, A., Singh, R. P., Ghazi, I. A., et al. (2022). Plant-soil-microbes: a tripartite interaction for nutrient acquisition and better plant growth for sustainable agricultural practices. Environ. Res. 214:113821. doi: 10.1016/j.envres.2022.113821
De Gregorio, P. R., Michavila, G., Ricciardi Muller, L., de Souza Borges, C., Pomares, M. F., Saccol de Sá, E. L., et al. (2017). Beneficial rhizobacteria immobilized in nanofibers for potential application as soybean seed bioinoculants. PLoS One 12:e0176930. doi: 10.1371/journal.pone.0176930
de Moraes, A. C. P., da Ribeiro, L. S., de Camargo, E. R., and Lacava, P. T. (2021). The potential of nanomaterials associated with plant growth-promoting bacteria in agriculture. Biotech 11:318. doi: 10.1007/s13205-021-02870-0
Duhan, L., Kumari, D., Verma, R., and Pasrija, R. (2022). “Fungal hydrolytic enzymes produced by plant growth-promoting rhizobacteria (PGPR)” in Secondary metabolites and volatiles of PGPR in plant-growth promotion, eds. R. Z. Sayyed and V. G. Uarrota (Springer, Cham), 313–333.
Elbasiouny, H., El-Ramady, H., Elbehiry, F., Rajput, V. D., Minkina, T., and Mandzhieva, S. (2022). Plant nutrition under climate change and soil carbon sequestration. Sustainability 14:914. doi: 10.3390/su14020914
Elemike, E., Uzoh, I., Onwudiwe, D., and Babalola, O. (2019). The role of nanotechnology in the fortification of plant nutrients and improvement of crop production. Appl. Sci. 9:499. doi: 10.3390/app9030499
Elmer, W., De La Torre-Roche, R., Pagano, L., Majumdar, S., Zuverza-Mena, N., Dimkpa, C., et al. (2018). Effect of metalloid and metal oxide nanoparticles on Fusarium wilt of watermelon. Plant Dis. 102, 1394–1401. doi: 10.1094/PDIS-10-17-1621-RE
Elnahal, A. S. M., El-Saadony, M. T., Saad, A. M., Desoky, E.-S. M., El-Tahan, A. M., Rady, M. M., et al. (2022). The use of microbial inoculants for biological control, plant growth promotion, and sustainable agriculture: a review. Eur. J. Plant Pathol. 162, 759–792. doi: 10.1007/s10658-021-02393-7
Eslami, P., Rossi, F., and Fedeli, S. (2019). Hybrid nanogels: stealth and biocompatible structures for drug delivery applications. Pharmaceutics 11:71. doi: 10.3390/pharmaceutics11020071
Esmaili, S., Tavallali, V., Amiri, B., Bazrafshan, F., and Sharafzadeh, S. (2022). Foliar application of nano-silicon complexes on growth, oxidative damage and bioactive compounds of feverfew under drought stress. Silicon 14, 10245–10256. doi: 10.1007/s12633-022-01754-z
Etesami, H., Shokri, E., and Jeong, B. R. (2022). “The combined use of silicon/nanosilicon and arbuscular mycorrhiza for effective management of stressed agriculture: action mechanisms and future prospects” in Silicon and Nano-silicon in Environmental Stress Management and Crop Quality Improvement, eds. H. Etesami, A. H. Al Saeedi, H. El-Ramady, M. Fujita, M. Pessarakli, and M. A. Hossain (Academic Press), 241–264.
Ezealigo, U. S., Ezealigo, B. N., Aisida, S. O., and Ezema, F. I. (2021). Iron oxide nanoparticles in biological systems: antibacterial and toxicology perspective. JCIS Open 4:100027. doi: 10.1016/j.jciso.2021.100027
Fadiji, A. E., Santoyo, G., Yadav, A. N., and Babalola, O. O. (2022). Efforts towards overcoming drought stress in crops: revisiting the mechanisms employed by plant growth-promoting bacteria. Front. Microbiol. 13:962427. doi: 10.3389/fmicb.2022.962427
Faizan, M., Hussain, A., Mir, A. R., Rajput, V. D., Minkina, T., and Hayat, S. (2021). “Effect of carbon nanotubes on abiotic stress response in plants: an overview” in Sustainable agriculture reviews, eds. M. Faizan, S. Hayat, and F. Yu (Springer, Cham), 217–229.
Farnia, A., Omidii, M. M., and Farnia, A. (2015). Effect of nano-zinc chelate and nano-biofertilizer on yield and yield components of maize (Zea mays L.), under water stress condition. Indian J Nat Sci, 5, 4614–4624.
Fazelian, N., and Yousefzadi, M. (2022). “Nano-biofertilizers for enhanced nutrient use efficiency” in Nano-enabled Agrochemicals in Agriculture, eds. M. Ghorbanpour, and M. A. Shahid (Academic Press), 145–158.
Fen, L. B., Rashid, A. H. A., Nordin, N. I., Hossain, M. A. M., Uddin, S. M. K., Johan, M. R., et al. (2022). “Applications of nanomaterials in agriculture and their safety aspect” in Biogenic nanomaterials, eds. D. Thangadurai, S. Islam, J. Sangeetha, & N. Cruz-Martins (Boca Raton: Apple Academic Press), 243–299.
Ferreira Soares, D. C., Domingues, S. C., Viana, D. B., and Tebaldi, M. L. (2020). Polymer-hybrid nanoparticles: current advances in biomedical applications. Biomed. Pharmacother. 131:110695. doi: 10.1016/j.biopha.2020.110695
Fetsiukh, A., Conrad, J., Bergquist, J., and Timmusk, S. (2021). Silica particles trigger the exopolysaccharide production of harsh environment isolates of growth-promoting rhizobacteria and increase their ability to enhance wheat biomass in drought-stressed soils. Int. J. Mol. Sci. 22:6201. doi: 10.3390/ijms22126201
Fiodor, A., Singh, S., and Pranaw, K. (2021). The contrivance of plant growth promoting microbes to mitigate climate change impact in agriculture. Microorganisms 9:1841. doi: 10.3390/microorganisms9091841
Fuskele, V., and Sarviya, R. M. (2017). Recent developments in nanoparticles synthesis, preparation and stability of nanofluids. Mater. Today 4, 4049–4060. doi: 10.1016/j.matpr.2017.02.307
Gahoi, P., Omar, R. A., Verma, N., and Gupta, G. S. (2021). Rhizobacteria and acylated homoserine lactone-based nanobiofertilizer to improve growth and pathogen defense in Cicer arietinum and Triticum aestivum plants. ACS Agric. Sci. Technol. 1, 240–252. doi: 10.1021/acsagscitech.1c00039
Gamalero, E., and Glick, B. R. (2022). Recent advances in bacterial amelioration of plant drought and salt stress. Biology 11:437. doi: 10.3390/biology11030437
Gavelienė, V., Šocik, B., Jankovska-Bortkevič, E., and Jurkonienė, S. (2021). Plant microbial biostimulants as a promising tool to enhance the productivity and quality of carrot root crops. Microorganisms 9:1850. doi: 10.3390/microorganisms9091850
Ghoghari, N., Bharwad, K., Champaneria, A., and Rajkumar, S. (2022). “Microbial consortia for augmentation of plant growth–revisiting the promising approach towards sustainable agriculture” in New and Future Developments in Microbial Biotechnology and Bioengineering, eds. H. Singh, and A. Vaishnav (Elsevier), 231–256.
González-García, Y., Cadenas-Pliego, G., Alpuche-Solís, Á. G., Cabrera, R. I., and Juárez-Maldonado, A. (2021). Carbon nanotubes decrease the negative impact of Alternaria solani in tomato crop. Nano 11, 1080. doi: 10.3390/nano11051080
Gosal, S. K., Kaur, J., and Kaur, J. (2017). “Plant growth-promoting rhizobacteria: a probiotic for plant health and productivity” in Probiotics and plant health, eds. V. Kumar, M. Kumar, S. Sharma and R. Prasad (Springer, Singapore), 589–600.
Guleria, G., Thakur, S., Shandilya, M., Sharma, S., Thakur, S., and Kalia, S. (2022). Nanotechnology for sustainable agro-food systems: the need and role of nanoparticles in protecting plants and improving crop productivity. Plant Physiol. Biochem. 194, 533–549. doi: 10.1016/j.plaphy.2022.12.004
Gupta, G. S., Kumar, A., and Verma, N. (2019). Bacterial homoserine lactones as a nanocomposite fertilizer and defense regulator for chickpeas. Environ. Sci. Nano 6, 1246–1258. doi: 10.1039/c9en00199a
Gutiérrez-Cruz, A., Ruiz-Hernández, A. R., Vega-Clemente, J. F., Luna-Gazcón, D. G., and Campos-Delgado, J. (2022). A review of top-down and bottom-up synthesis methods for the production of graphene, graphene oxide and reduced graphene oxide. J. Mater. Sci. 57, 14543–14578. doi: 10.1007/s10853-022-07514-z
Hadj Alouane, M. H., Ahmed, F., Hussein El Semary, N. A., Aldayel, M. F., Alhaweti, F. H., and Nasr, O. (2021). Application of optimised snanocarbon materials and biofertilisers as a potent superfertiliser: towards sustainable agriculture production. Sci. Adv. Mater. 13, 812–819. doi: 10.1166/sam.2021.3948
Hafez, Y. M., Attia, K. A., Kamel, S., Alamery, S. F., El-Gendy, S., Al-Doss, A. A., et al. (2020). Bacillus subtilis as a bio-agent combined with nano molecules can control powdery mildew disease through histochemical and physiobiochemical changes in cucumber plants. Physiol. Mol. Plant Pathol. 111:101489. doi: 10.1016/j.pmpp.2020.101489
Hafez, E. M., Osman, H. S., Gowayed, S. M., Okasha, S. A., Omara, A. E.-D., Sami, R., et al. (2021). Minimizing the adversely impacts of water deficit and soil salinity on maize growth and productivity in response to the application of plant growth-promoting rhizobacteria and silica nanoparticles. Agronomy 11:676. doi: 10.3390/agronomy11040676
Hajji-Hedfi, L., and Chhipa, H. (2021). Nano-based pesticides: challenges for pest and disease management. Euro-Mediterr. J. Environ. Integr. 6:69. doi: 10.1007/s41207-021-00279-y
Hassan, F. A. S., Ali, E., Gaber, A., Fetouh, M. I., and Mazrou, R. (2021). Chitosan nanoparticles effectively combat salinity stress by enhancing antioxidant activity and alkaloid biosynthesis in Catharanthus roseus (L.) G. Don. Plant Physiol. Biochem. 162, 291–300. doi: 10.1016/j.plaphy.2021.03.004
Hernández-Díaz, J. A., Garza-García, J. J., Zamudio-Ojeda, A., León-Morales, J. M., López-Velázquez, J. C., and García-Morales, S. (2021). Plant-mediated synthesis of nanoparticles and their antimicrobial activity against phytopathogens. J. Sci. Food Agric. 101, 1270–1287. doi: 10.1002/jsfa.10767
Hezaveh, T. A., Pourakbar, L., Rahmani, F., and Alipour, H. (2019). Interactive effects of salinity and ZnO nanoparticles on physiological and molecular parameters of rapeseed (Brassica napus L.). Commun. Soil Sci. Plant Anal. 50, 698–715. doi: 10.1080/00103624.2019.1589481
Hofmann, T., Lowry, G. V., Ghoshal, S., Tufenkji, N., Brambilla, D., Dutcher, J. R., et al. (2020). Technology readiness and overcoming barriers to sustainably implement nanotechnology-enabled plant agriculture. Nat. Food. 1, 416–425. doi: 10.1038/s43016-020-0110-1
Hong, J., Jia, S., Wang, C., Li, Y., He, F., and Gardea-Torresdey, J. L. (2023). Transcriptome reveals the exposure effects of CeO2 nanoparticles on pakchoi (Brassica chinensis L.) photosynthesis. J. Hazard. Mater. 444:130427. doi: 10.1016/j.jhazmat.2022.130427
Hosseinpour, A., Ilhan, E., Özkan, G., Öztürk, H. İ., Haliloglu, K., and Cinisli, K. T. (2022). Plant growth-promoting bacteria (PGPBs) and copper (II) oxide (CuO) nanoparticle ameliorates DNA damage and DNA methylation in wheat (Triticum aestivum L.) exposed to NaCl stress. J. Plant Biochem. Biotechnol. 31, 751–764. doi: 10.1007/s13562-021-00713-w
Janmohammadi, M., Navid, A., Segherloo, A. E., and Sabaghnia, N. (2016). Impact of nano-chelated micronutrients and biological fertilizers on growth performance and grain yield of maize under deficit irrigation condition. Biologija (Vilnius) 62:143–147. doi: 10.6001/biologija.v62i2.3339
Jakhar, A. M., Aziz, I., Kaleri, A. R., Hasnain, M., Haider, G., Ma, J., et al. (2022). Nano-fertilizers: a sustainable technology for improving crop nutrition and food security. NanoImpact 27:100411. doi: 10.1016/j.impact.2022.100411
Jhuma, T. A., Rafeya, J., Sultana, S., Rahman, M. T., and Karim, M. M. (2021). Isolation of endophytic salt-tolerant plant growth-promoting rhizobacteria from Oryza sativa and evaluation of their plant growth-promoting traits under salinity stress condition. Front. Sustain. Food Syst. 5:687531. doi: 10.3389/fsufs.2021.687531
Jiang, S., Li, J., Wang, Q., Yin, C., Zhan, Y., Yan, Y., et al. (2022). Maize growth promotion by inoculation with an engineered ammonium-excreting strain of nitrogen-fixing Pseudomonas stutzeri. Microorganisms 10:1986. doi: 10.3390/microorganisms10101986
Jiang, Q., Liu, Q., Shi, Y., Wang, Z.-G., Zhan, P., Liu, J., et al. (2017). Stimulus-responsive plasmonic chiral signals of gold nanorods organized on DNA origami. Nano Lett. 17, 7125–7130. doi: 10.1021/acs.nanolett.7b03946
Jiang, G., Zhang, Y., Gan, G., Li, W., Wan, W., Jiang, Y., et al. (2022). Exploring rhizo-microbiome transplants as a tool for protective plant-microbiome manipulation. ISME Commun. 2, 10. doi: 10.1038/s43705-022-00094-8
Kamaly, N., Yameen, B., Wu, J., and Farokhzad, O. C. (2016). Degradable controlled-release polymers and polymeric nanoparticles: mechanisms of controlling drug release. Chem. Rev. 116, 2602–2663. doi: 10.1021/acs.chemrev.5b00346
Kamari, H., and Sharifi, R. (2017). Effects of nano-zinc oxide and plant growth promoting rhizobacteria on zinc and protein content, phosphatase activity and related traits to grain growth of triticale. Iran. J. Seed Sci. Technol. 6, 151–163. doi: 10.22034/ijsst.2017.113549
Kashyap, A. S., Manzar, N., Rajawat, M. V. S., Kesharwani, A. K., Singh, R. P., Dubey, S. C., et al. (2021). Screening and biocontrol potential of rhizobacteria native to Gangetic plains and hilly regions to induce systemic resistance and promote plant growth in Chilli against bacterial wilt disease. Plan. Theory 10:2125. doi: 10.3390/plants10102125
Kasperson, J. X., Kasperson, R. E., Turner, B. L., Hsieh, W., and Schiller, A. (2022). “Vulnerability to global environmental change” in The social contours of risk, eds. R. E. Kasperson, and J. Kasperson (Routledge), 245–285.
Kaviya, N., Upadhayay, V. K., Singh, J., Khan, A., Panwar, M., and Singh, A. V. (2019). “Role of microorganisms in soil genesis and functions” in Mycorrhizosphere and Pedogenesis, eds. A. Varma, D. Choudhary (Singapore: Springer, Singapore), 25–52.
Kenawy, A., Abo-Zaid, G., El-Gendi, H., Hegazy, G., Ho, T., and El Enshasy, H. (2021). “Probiotics applications in agriculture” in Probiotics, the natural microbiota in living organisms, eds. H. A. El-Enshasy, S. Yang (Boca Raton: CRC Press), 251–291.
Khairy, A. M., Tohamy, M. R. A., Zayed, M. A., Mahmoud, S. F., El-Tahan, A. M., El-Saadony, M. T., et al. (2022). Eco-friendly application of nano-chitosan for controlling potato and tomato bacterial wilt. Saudi J. Biol. Sci. 29, 2199–2209. doi: 10.1016/j.sjbs.2021.11.041
Khalil, M. S., Abd El-Aziz, M. H., and Selim, R. E.-S. (2022). Physiological and morphological response of tomato plants to nano-chitosan used against bio-stress induced by root-knot nematode (Meloidogyne incognita) and tobacco mosaic tobamovirus (TMV). Eur. J. Plant Pathol. 163, 799–812. doi: 10.1007/s10658-022-02516-8
Khan, M., Khan, A. U., Bogdanchikova, N., and Garibo, D. (2021). Antibacterial and antifungal studies of biosynthesized silver nanoparticles against plant parasitic nematode Meloidogyne incognita, plant pathogens Ralstonia solanacearum and Fusarium oxysporum. Molecules 26:2462. doi: 10.3390/molecules26092462
Khan, A., Panthari, D., Sharma, R. S., Punetha, A., Singh, A. V., and Upadhayay, V. K. (2023). “Biofertilizers: a microbial-assisted strategy to improve plant growth and soil health” in Advanced microbial techniques in agriculture, environment, and health management, eds. S. C. Pandey, V. Pande, D. Sati, andM. Samant (Academic Press), 97–118.
Khan, A., Singh, A. V., Upadhayay, V. K., Ballabh, A., and Prasad, B. (2022). Influence of PGPR on growth and yield of oat (Avena sativa L.) under field conditions. Indian. J. Ecol. 49, 1351–1356. doi: 10.55362/ije/2022/3670
Khan, A., Singh, J., Upadhayay, V. K., Singh, A. V., and Shah, S. (2019). “Microbial biofortification: a green technology through plant growth promoting microorganisms” in Sustainable green Technologies for Environmental Management, eds. S. Shah, V. Venkatramanan, and R. Prasad (Springer, Singapore), 255–269.
Khare, E., and Arora, N. K. (2015). “Effects of soil environment on field efficacy of microbial inoculants” in Plant microbes symbiosis: Applied facets, ed. N. Arora (Springer, New Delhi), 353–381.
Khati, P., Chaudhary, P., Gangola, S., Bhatt, P., and Sharma, A. (2017). Nanochitosan supports growth of Zea mays and also maintains soil health following growth. Biotech 7:81. doi: 10.1007/s13205-017-0668-y
Khati, P., Chaudhary, P., Gangola, S., Bhatt, P., and Sharma, A. (2019a). Influence of nanozeolite on plant growth promotory bacterial isolates recovered from nanocompound infested agriculture field. Environ. Ecol. 37, 521–527.
Khati, P., Parul Bhatt, P., Nisha Kumar, R., and Sharma, A. (2018). Effect of nanozeolite and plant growth promoting rhizobacteria on maize. Biotech 8. doi: 10.1007/s13205-018-1142-1
Khati, P., Sharma, A., Chaudhary, P., Singh, A. K., Gangola, S., and Kumar, R. (2019b). High-throughput sequencing approach to access the impact of nanozeolite treatment on species richness and evenness of soil metagenome. Biocatal. Agric. Biotechnol. 20:101249. doi: 10.1016/j.bcab.2019.101249
Kleinert, A., Benedito, V. A., Morcillo, R. J. L., Dames, J., Cornejo-Rivas, P., Zuniga-Feest, A., et al. (2018). “Morphological and symbiotic root modifications for mineral acquisition from nutrient-poor soils” in Soil biology, eds. B. Giri, R. Prasad, and A. Varma (Springer, Cham), 85–142.
Kolesnikov, S. I., Timoshenko, A. N., Kazeev, K. S., Akimenko, Y. V., and Myasnikova, M. A. (2019). Ecotoxicity of copper, nickel, and zinc nanoparticles assessment on the basis of biological indicators of chernozems. Eurasian Soil Sci. 52, 982–987. doi: 10.1134/s106422931908009x
Kukreti, B., Sharma, A., Chaudhary, P., Agri, U., and Maithani, D. (2020). Influence of nanosilicon dioxide along with bioinoculants on Zea mays and its rhizospheric soil. Biotech 10:345. doi: 10.1007/s13205-020-02329-8
Kumar, P., Burman, U., and Kaul, R. K. (2018). “Ecological risks of nanoparticles” in Nanomaterials in plants, algae, and microorganisms, eds. D. K. Tripathi, P. Ahmad, S. Sharma, D. K. Chauhan, and N. K. Dubey (Academic Press), 429–452.
Kumar, S., Nehra, M., Dilbaghi, N., Marrazza, G., Hassan, A. A., and Kim, K.-H. (2019). Nano-based smart pesticide formulations: emerging opportunities for agriculture. J. Control. Release 294, 131–153. doi: 10.1016/j.jconrel.2018.12.012
Kumari, H., Khati, P., Gangola, S., Chaudhary, P., and Sharma, A. (2021). Performance of plant growth promotory rhizobacteria on maize and soil characteristics under the influence of TiO2 nanoparticles. Pantnagar J. Res. 19, 28–39.
Kumari, S., Sharma, A., Chaudhary, P., and Khati, P. (2020). Management of plant vigor and soil health using two agriusable nanocompounds and plant growth promotory rhizobacteria in Fenugreek. Biotech 10:461. doi: 10.1007/s13205-020-02448-2
Kumari, R., and Singh, D. P. (2020). Nano-biofertilizer: an emerging eco-friendly approach for sustainable agriculture. Proc. Natl. Acad. Sci. India Sect. B Biol. Sci. 90, 733–741. doi: 10.1007/s40011-019-01133-6
Kumawat, K. C., Razdan, N., and Saharan, K. (2022). Rhizospheric microbiome: bio-based emerging strategies for sustainable agriculture development and future perspectives. Microbiol. Res. 254:126901. doi: 10.1016/j.micres.2021.126901
Kurniawan, A., and Chuang, H.-W. (2022). Rhizobacterial Bacillus mycoides functions in stimulating the antioxidant defence system and multiple phytohormone signalling pathways to regulate plant growth and stress tolerance. J. Appl. Microbiol. 132, 1260–1274. doi: 10.1111/jam.15252
Latif, M., Bukhari, S. A. H., Alrajhi, A. A., Alotaibi, F. S., Ahmad, M., Shahzad, A. N., et al. (2022). Inducing drought tolerance in wheat through exopolysaccharide-producing rhizobacteria. Agronomy 12:1140. doi: 10.3390/agronomy12051140
Li, Y., Xi, K., Liu, X., Han, S., Han, X., Li, G., et al. (2023). Silica nanoparticles promote wheat growth by mediating hormones and sugar metabolism. J. Nanobiotechnol. 21:2. doi: 10.1186/s12951-022-01753-7
Liliane, T. N., and Charles, M. S. (2020). “Factors affecting yield of crops” in Agronomy - climate change & food security, ed. Amanullah (IntechOpen), 1–16.
Lin, W., Lin, M., Zhou, H., Wu, H., Li, Z., and Lin, W. (2019). The effects of chemical and organic fertilizer usage on rhizosphere soil in tea orchards. PLoS One 14:e0217018. doi: 10.1371/journal.pone.0217018
Lu, X., Sun, D., Zhang, X., Hu, H., Kong, L., Rookes, J. E., et al. (2020). Stimulation of photosynthesis and enhancement of growth and yield in Arabidopsis thaliana treated with amine-functionalized mesoporous silica nanoparticles. Plant Physiol. Biochem. 156, 566–577. doi: 10.1016/j.plaphy.2020.09.036
Ma, W., Du, W., Gu, K., Xu, M., Yin, Y., Sun, Y., et al. (2023). Elevated CO2 exacerbates effects of TiO2 nanoparticles on rice (Oryza sativa L.) leaf transcriptome and soil bacteria. Sci. Total Environ. 857:159689. doi: 10.1016/j.scitotenv.2022.159689
Mahakham, W., Sarmah, A. K., Maensiri, S., and Theerakulpisut, P. (2017). Nanopriming technology for enhancing germination and starch metabolism of aged rice seeds using phytosynthesized silver nanoparticles. Sci. Rep. 7:8263. doi: 10.1038/s41598-017-08669-5
Mahapatra, D. M., Satapathy, K. C., and Panda, B. (2022). Biofertilizers and nanofertilizers for sustainable agriculture: phycoprospects and challenges. Sci. Total Environ. 803:149990. doi: 10.1016/j.scitotenv.2021.149990
Mahawer, S. K., Arya, S., Kabdal, T., Kumar, R., Prakash, O., Chitara, M. K., and Koli, P. (2022). Plant defense systems: mechanism of self-protection by plants against pathogens. Plant Protection: From Chemicals to Biologicals, 115.
Mahmud, K., Missaoui, A., Lee, K., Ghimire, B., Presley, H. W., and Makaju, S. (2021). Rhizosphere microbiome manipulation for sustainable crop production. Curr. Plant Biol. 27:100210. doi: 10.1016/j.cpb.2021.100210
Mandal, M., Chatterjee, S., and Majumdar, S. (2022). “Outside the cell surface: encoding the role of exopolysaccharide producing rhizobacteria to boost the drought tolerance in plants” in Plant stress: challenges and management in the New Decade, eds. S. Roy, P. Mathur, A. P. Chakraborty, & S. P. Saha (Springer, Cham), 295–310.
Mandal, A., Sarkar, B., Mandal, S., Vithanage, M., Patra, A. K., and Manna, M. C. (2020). “Impact of agrochemicals on soil health” in Agrochemicals detection, treatment and remediation, ed. M. N. V. Prasad (Butterworth-Heinemann), 161–187.
Mardi, A., Mohajjel Shoja, H., and Mohajel Kazemi, E. (2022). Comparative study of growth responses, photosynthetic pigment content, and gene expression pattern in tobacco plants treated with ZnO nano and ZnO bulk particles. J. Nanopart. Res. 24:208. doi: 10.1007/s11051-022-05583-4
Massa, F., Defez, R., and Bianco, C. (2022). Exploitation of plant growth promoting bacteria for sustainable agriculture: hierarchical approach to link laboratory and field experiments. Microorganisms 10:865. doi: 10.3390/microorganisms10050865
Mathur, A., Koul, A., and Hattewar, J. (2019). “Plant growth-promoting rhizobacteria (PGPRs): significant revolutionary tools for achieving long-term sustainability and combating the biotic stress caused by the attack of pathogens affecting crops in agriculture” in Plant growth promoting Rhizobacteria for sustainable stress management, ed. R. Sayyed (Springer, Singapore), 379–388.
Meena, R., Kumar, S., Datta, R., Lal, R., Vijayakumar, V., Brtnicky, M., et al. (2020). Impact of agrochemicals on soil microbiota and management: a review. Land 9:34. doi: 10.3390/land9020034
Mejias, J. H., Salazar, F., Pérez Amaro, L., Hube, S., Rodriguez, M., and Alfaro, M. (2021). Nanofertilizers: a cutting-edge approach to increase nitrogen use efficiency in grasslands. Front. Environ. Sci. 9:635114. doi: 10.3389/fenvs.2021.635114
Menéndez, E., and Paço, A. (2020). Is the application of plant probiotic bacterial consortia always beneficial for plants? Exploring synergies between rhizobial and non-rhizobial bacteria and their effects on agro-economically valuable crops. Life 10:24. doi: 10.3390/life10030024
Mishra, R. K., Rathore, U. S., Pandey, S., Mishra, M., Sharma, N., Kumar, S., et al. (2022). “Plant–rhizospheric microbe interactions: enhancing plant growth and improving soil biota” in Re-visiting the Rhizosphere eco-system for agricultural sustainability, eds. U. B. Singh, J. P. Rai, and A. K. Sharma (Springer, Singapore), 445–459.
Mitra, B., Chowdhury, A. R., Dey, P., Hazra, K. K., Sinha, A. K., Hossain, A., et al. (2021). “Use of agrochemicals in agriculture: alarming issues and solutions” in Input use efficiency for food and environmental security, eds. R. Bhatt, R. S. Meena, and A. Hossain (Springer, Singapore), 85–122.
Mohamed, N. G., and Abdel-Hakeem, M. A. (2023). Chitosan nanoparticles enhance drought tolerance in tomatoes (Solanum lycopersicum L) via gene expression modulation. Plant Gene 34:100406. doi: 10.1016/j.plgene.2023.100406
Moradi Pour, M., Saberi Riseh, R., and Skorik, Y. A. (2022). Sodium alginate-gelatin nanoformulations for encapsulation of Bacillus velezensis and their use for biological control of pistachio gummosis. Materials 15:2114. doi: 10.3390/ma15062114
Moradi, P., Vafaee, Y., Mozafari, A. A., and Tahir, N. A.-R. (2022). Silicon nanoparticles and methyl jasmonate improve physiological response and increase expression of stress-related genes in strawberry cv. Paros under salinity stress. Silicon 14, 10559–10569. doi: 10.1007/s12633-022-01791-8
Mubeen, M., Bano, A., Ali, B., Islam, Z. U., Ahmad, A., Hussain, S., et al. (2021). Effect of plant growth promoting bacteria and drought on spring maize (Zea mays L.). Pak. J. Bot. 53, 1–10. doi: 10.30848/pjb2021-2(38)
Muhammad, F., Raza, M. A. S., Iqbal, R., Zulfiqar, F., Aslam, M. U., Yong, J. W. H., et al. (2022). Ameliorating drought effects in wheat using an exclusive or co-applied rhizobacteria and ZnO nanoparticles. Biology 11:1564. doi: 10.3390/biology11111564
Mushtaq, Z., Nazir, A., Asghar, H. N., and Zahir, Z. A. (2022). Interactive effect of siderophore-producing bacteria and l-tryptophan on physiology, tuber characteristics, yield, and iron concentration of potato. Potato Res. 65, 1015–1027. doi: 10.1007/s11540-022-09565-w
Muthukumaran, P., Suresh Babu, P., Shyamalagowri, S., Aravind, J., Kamaraj, M., and Govarthanan, M. (2022). Polymeric biomolecules based nanomaterials: production strategies and pollutant mitigation as an emerging tool for environmental application. Chemosphere 307:136008. doi: 10.1016/j.chemosphere.2022.136008
Nagpal, S., Sharma, P., and Kumawat, K. C. (2021). “Microbial bioformulations: revisiting role in sustainable agriculture” in Biofertilizers, eds. A. Rakshit, V. S. Meena, M. Parihar, H.B. Singh, and A.K. Singh (Woodhead Publishing), 329–346.
Naim, L., Alsanad, M. A., El Sebaaly, Z., Shaban, N., Fayssal, S. A., and Sassine, Y. N. (2020). Variation of Pleurotus ostreatus (Jacq. Ex Fr.) P. Kumm. (1871) performance subjected to different doses and timings of nano-urea. Saudi J. Biol. Sci. 27, 1573–1579. doi: 10.1016/j.sjbs.2020.03.019
Naseem, H., Ahsan, M., Shahid, M. A., and Khan, N. (2018). Exopolysaccharides producing rhizobacteria and their role in plant growth and drought tolerance. J. Basic Microbiol. 58, 1009–1022. doi: 10.1002/jobm.201800309
Nawaz, S., and Bano, A. (2020). Effects of PGPR (Pseudomonas sp.) and ag-nanoparticles on enzymatic activity and physiology of cucumber. Recent Pat. Food Nutr. Agric. 11, 124–136. doi: 10.2174/2212798410666190716162340
Nayana, A. R., Joseph, B. J., Jose, A., and Radhakrishnan, E. K. (2020). “Nanotechnological advances with PGPR applications” in Sustainable agriculture reviews 41, eds. S. Hayat, J. Pichtel, M. Faizan, and Q. Fariduddin (Springer, Cham), 163–180.
Nazli, F., Mustafa, A., Ahmad, M., Hussain, A., Jamil, M., Wang, X., et al. (2020). A review on practical application and potentials of phytohormone-producing plant growth-promoting rhizobacteria for inducing heavy metal tolerance in crops. Sustainability 12:9056. doi: 10.3390/su12219056
Neme, K., Nafady, A., Uddin, S., and Tola, Y. B. (2021). Application of nanotechnology in agriculture, postharvest loss reduction and food processing: food security implication and challenges. Heliyon 7:e08539. doi: 10.1016/j.heliyon.2021.e08539
Nithya, N., Kiruthika, R., and Dhanaprakhash, S. (2022). Shift in the mindset: increasing preference towards organic food products in Indian context. Org. Agric. 12, 213–228. doi: 10.1007/s13165-021-00370-2
Okey-Onyesolu, C. F., Hassanisaadi, M., Bilal, M., Barani, M., Rahdar, A., Iqbal, J., et al. (2021). Nanomaterials as nanofertilizers and nanopesticides: an overview. ChemistrySelect 6, 8645–8663. doi: 10.1002/slct.202102379
Öksel, C., Balkan, A., and Bi̇lgi̇n, O., and Mi̇ri̇k, M., (2022). Investigation of the effect of pgpr on yield and some yield components in winter wheat. Turk. J. Field Crop. 27, 127–133. doi: 10.17557/tjfc.1019160
Omran, B. A., and Baek, K.-H. (2022). Control of phytopathogens using sustainable biogenic nanomaterials: recent perspectives, ecological safety, and challenging gaps. J. Clean. Prod. 372:133729. doi: 10.1016/j.jclepro.2022.133729
Orozco-Mosqueda, M. D. C., Fadiji, A. E., Babalola, O. O., Glick, B. R., and Santoyo, G. (2022). Rhizobiome engineering: unveiling complex rhizosphere interactions to enhance plant growth and health. Microbiol. Res. 263:127137. doi: 10.1016/j.micres.2022.127137
Pandey, S. S., Jain, R., Bhardwaj, P., Thakur, A., Kumari, M., Bhushan, S., et al. (2022). Plant probiotics - Endophytes pivotal to plant health. Microbiol. Res. 263:127148. doi: 10.1016/j.micres.2022.127148
Panichikkal, J., and Krishnankutty, R. E. (2022). Chitosan and gold nanoparticles supplementation for augmentation of indole-3-acetic acid production by rhizospheric Pseudomonas aeruginosa and plant growth enhancement. Curr. Microbiol. 79:185. doi: 10.1007/s00284-022-02850-4
Panichikkal, J., Prathap, G., Nair, R. A., and Krishnankutty, R. E. (2021). Evaluation of plant probiotic performance of Pseudomonas sp. encapsulated in alginate supplemented with salicylic acid and zinc oxide nanoparticles. Int. J. Biol. Macromol. 166, 138–143. doi: 10.1016/j.ijbiomac.2020.10.110
Panichikkal, J., Thomas, R., John, J. C., and Radhakrishnan, E. K. (2019). Biogenic gold nanoparticle supplementation to plant beneficial Pseudomonas monteilii was found to enhance its plant probiotic effect. Curr. Microbiol. 76, 503–509. doi: 10.1007/s00284-019-01649-0
Pantigoso, H. A., Newberger, D., and Vivanco, J. M. (2022). The rhizosphere microbiome: plant–microbial interactions for resource acquisition. J. Appl. Microbiol. 133, 2864–2876. doi: 10.1111/jam.15686
Pinelli, F., Saadati, M., Zare, E. N., Makvandi, P., Masi, M., Sacchetti, A., et al. (2022). A perspective on the applications of functionalized nanogels: promises and challenges. Int. Mater. Rev., 68, 1–25. doi: 10.1080/09506608.2022.2026864
Pirzadah, B., Pirzadah, T. B., Jan, A., and Hakeem, K. R. (2020). “Nanofertilizers: a way forward for green economy” in Nanotechnology in the life sciences, eds. K. Hakeem, & T. Pirzadah, 99–112.
Pour, M. M., Riseh, R. S., Ranjbar-Karimi, R., Hassanisaadi, M., Rahdar, A., and Baino, F. (2022). Microencapsulation of Bacillus velezensis using alginate-gum polymers enriched with TiO2 and SiO2 nanoparticles. Micromachines 13:1423. doi: 10.3390/mi13091423
Pour, M. M., Saberi-Riseh, R., Mohammadinejad, R., and Hosseini, A. (2019). Nano-encapsulation of plant growth-promoting rhizobacteria and their metabolites using alginate-silica nanoparticles and carbon nanotube improves UCB1 pistachio micropropagation. J. Microbiol. Biotechnol. 29, 1096–1103. doi: 10.4014/jmb.1903.03022
Qazi, G., and Dar, F. A. (2020). “Nano-agrochemicals: economic potential and future trends” in Nanotechnology in the life sciences, eds. K. Hakeem, and T. Pirzadah (Springer, Cham), 185–193.
Qin, Y., Abatzoglou, J. T., Siebert, S., Huning, L. S., AghaKouchak, A., Mankin, J. S., et al. (2020). Agricultural risks from changing snowmelt. Nat. Clim. Chang. 10, 459–465. doi: 10.1038/s41558-020-0746-8
Raeisi Sadati, S. Y., Jahanbakhsh Godehkahriz, S., Ebadi, A., and Sedghi, M. (2022). Zinc oxide nanoparticles enhance drought tolerance in wheat via physio-biochemical changes and stress genes expression. Iran. J. Biotechnol. 20:e3027. doi: 10.30498/ijb.2021.280711.3027
Rahmatizadeh, R., Jamei, R., Arvin, M. J., and Rezanejad, F. (2021). Upregulation of LeNRAMP3 and LeFER genes in Solanum lycopersicum confers its cadmium tolerance. Russ. J. Plant Physiol. 68, S92–S102. doi: 10.1134/s1021443721070104
Rai, S., Solanki, M. K., Solanki, A. C., and Samal, S. (2023). “Microbial endophytes as probiotics for the plant health: an overview” in Microbial endophytes and plant growth, eds. M. K. Solanki, M. K. Yadav, B. P. Singh, and V. K. Gupta (Academic Press), 269–281.
Ramar, V., and Balraj, A. (2022). Critical review on carbon-based nanomaterial for carbon capture: technical challenges, opportunities, and future perspectives. Energy Fuel 36, 13479–13505. doi: 10.1021/acs.energyfuels.2c02585
Rashid, M. I., Shahzad, T., Shahid, M., Ismail, I. M. I., Shah, G. M., and Almeelbi, T. (2017). Zinc oxide nanoparticles affect carbon and nitrogen mineralization of Phoenix dactylifera leaf litter in a sandy soil. J. Hazard. Mater. 324, 298–305. doi: 10.1016/j.jhazmat.2016.10.063
Ravichandran, M., Samiappan, S. C., Rangaraj, S., Murugan, K., Al-Dhabi, N. A., and Karuppiah, P. (2022). “Nanoemulsion formulations with plant growth promoting rhizobacteria (PGPR) for sustainable agriculture” in Bio-based nanoemulsions for agri-food applications, eds. K. A. Abd-Elsalam, and K. Murugan (Elsevier), 207–223.
Reddy, E. C., Reddy, G. S., Goudar, V., Sriramula, A., Swarnalatha, G. V., Al Tawaha, A. R. M., et al. (2022). “Hydrolytic enzyme producing plant growth-promoting rhizobacteria (PGPR) in plant growth promotion and biocontrol” in Secondary metabolites and volatiles of PGPR in plant-growth promotion, eds. R. Z. Sayyed, and V. G. Uarrota (Springer, Cham), 303–312.
Rehman, N., and Pandey, A. (2022). “Introduction to nanotherapeutics: a synthetic preview” in Nanotherapeutics in cancer, ed. H. S. Tuli (New York: Jenny Stanford Publishing), 1–22.
Saberi Riseh, R., Hassanisaadi, M., Vatankhah, M., Soroush, F., and Varma, R. S. (2022a). Nano/microencapsulation of plant biocontrol agents by chitosan, alginate, and other important biopolymers as a novel strategy for alleviating plant biotic stresses. Int. J. Biol. Macromol. 222, 1589–1604. doi: 10.1016/j.ijbiomac.2022.09.278
Saberi Riseh, R., Tamanadar, E., Hajabdollahi, N., Vatankhah, M., Thakur, V. K., and Skorik, Y. A. (2022b). Chitosan microencapsulation of rhizobacteria for biological control of plant pests and diseases: recent advances and applications. Rhizosphere 23:100565. doi: 10.1016/j.rhisph.2022.100565
Saberi-Rise, R., and Moradi-Pour, M. (2020). The effect of Bacillus subtilis Vru1 encapsulated in alginate - bentonite coating enriched with titanium nanoparticles against Rhizoctonia solani on bean. Int. J. Biol. Macromol. 152, 1089–1097. doi: 10.1016/j.ijbiomac.2019.10.197
Saffan, M. M., Koriem, M. A., El-Henawy, A., El-Mahdy, S., El-Ramady, H., Elbehiry, F., et al. (2022). Sustainable production of tomato plants (Solanum lycopersicum L.) under low-quality irrigation water as affected by bio-nanofertilizers of selenium and copper. Sustainability 14:3236. doi: 10.3390/su14063236
Sahil, K., Sahil Keshan, R., Patra, A., Mehta, S., Abdelmotelb, K. F., Lavale, S. A., et al. (2021). “Expression and regulation of stress-responsive genes in plants under harsh environmental conditions” in Harsh environment and plant resilience, ed. A. Husen (Springer, Cham), 25–44.
Salama, A. M., Abd El-Halim, A. E.-H. A., Ibrahim, M. M., Aiad, M. A., and El-Shal, R. M. (2022). Amendment with nanoparticulate gypsum enhances spinach growth in saline-sodic soil. J. Soil Sci. Plant Nutr. 22, 3377–3385. doi: 10.1007/s42729-022-00893-x
Sambangi, P., Gopalakrishnan, S., Pebam, M., and Rengan, A.K. (2022). Nano-biofertilizers on soil health, chemistry, and microbial community: benefits and risks. Proc.Indian Natl. Sci. Acad. 88, 357–368. doi: 10.1007/s43538-022-00094-1
Santos, A. P., Belfiore, C., Úrbez, C., Ferrando, A., Blázquez, M. A., and Farías, M. E. (2022). Extremophiles as plant probiotics to promote germination and alleviate salt stress in soybean. J. Plant Growth Regul. 42, 946–959. doi: 10.1007/s00344-022-10605-5
Santoyo, G., Gamalero, E., and Glick, B. R. (2021). Mycorrhizal-bacterial amelioration of plant abiotic and biotic stress. Front. Sustain. Food Syst. 5:672881. doi: 10.3389/fsufs.2021.672881
Sanzari, I., Leone, A., and Ambrosone, A. (2019). Nanotechnology in plant science: to make a long story short. Front. Bioeng. Biotechnol. 7:120. doi: 10.3389/fbioe.2019.00120
Sarbani, N. M. M., and Yahaya, N. (2022). Advanced development of bio-fertilizer formulations using microorganisms as inoculant for sustainable agriculture and environment – a review. MJoSHT 8, 92–101. doi: 10.33102/mjosht.v8i1.228
Seyed Sharifi, R., Khalilzadeh, R., Pirzad, A., and Anwar, S. (2020). Effects of biofertilizers and nano zinc-iron oxide on yield and physicochemical properties of wheat under water deficit conditions. Commun. Soil Sci. Plant Anal. 51, 2511–2524. doi: 10.1080/00103624.2020.1845350
Shah, A. A., Aslam, S., Akbar, M., Ahmad, A., Khan, W. U., Yasin, N. A., et al. (2021). Combined effect of Bacillus fortis IAGS 223 and zinc oxide nanoparticles to alleviate cadmium phytotoxicity in Cucumis melo. Plant Physiol. Biochem. 158, 1–12. doi: 10.1016/j.plaphy.2020.11.011
Shahzad, A., Ullah, S., Dar, A. A., Sardar, M. F., Mehmood, T., Tufail, M. A., et al. (2021). Nexus on climate change: agriculture and possible solution to cope future climate change stresses. Environ. Sci. Pollut. Res. Int. 28, 14211–14232. doi: 10.1007/s11356-021-12649-8
Shalaby, T. A., Abd-Alkarim, E., El-Aidy, F., Hamed, E.-S., Sharaf-Eldin, M., Taha, N., et al. (2021). Nano-selenium, silicon and H2O2 boost growth and productivity of cucumber under combined salinity and heat stress. Ecotoxicol. Environ. Saf. 212:111962. doi: 10.1016/j.ecoenv.2021.111962
Shalaby, T. A., El-Bialy, S. M., El-Mahrouk, M. E., Omara, A. E.-D., El-Beltagi, H. S., and El-Ramady, H. (2022). Acclimatization of in vitro banana seedlings using root-applied bio-nanofertilizer of copper and selenium. Agronomy 12:539. doi: 10.3390/agronomy12020539
Sharifianjazi, F., Irani, M., Esmaeilkhanian, A., Bazli, L., Asl, M. S., Jang, H. W., et al. (2021). Polymer incorporated magnetic nanoparticles: applications for magnetoresponsive targeted drug delivery. Mater. Sci. Eng. B Solid State Mater. Adv. Technol. 272:115358. doi: 10.1016/j.mseb.2021.115358
Sharma, B., Afonso, L. O. B., Singh, M. P., Soni, U., and Cahill, D. M. (2022). Zinc- and magnesium-doped hydroxyapatite-urea nanohybrids enhance wheat growth and nitrogen uptake. Sci. Rep. 12:19506. doi: 10.1038/s41598-022-20772-w
Sharma, A., Kumar, S., and Singh, R. (2022). Synthesis and characterization of a novel slow-release nanourea/chitosan nanocomposite and its effect on Vigna radiata L. Environ. Sci. Nano 9, 4177–4189. doi: 10.1039/d2en00297c
Sharma, S. K., Sharma, P. K., Mandeewal, R. L., Sharma, V., Chaudhary, R., Pandey, R., et al. (2022). Effect of foliar application of nano-urea under different nitrogen levels on growth and nutrient content of pearl millet (Pennisetum glaucum L.). Int. J. Plant Soil Sci. 34, 149–155. doi: 10.9734/IJPSS/2022/v34i2031138
Shikari, A. B., Dikilitas, M., Simsek, E., Guldur, M. E., Simsek, U., Karakas, S., et al. (2022). “Nanotechnology and its role in cereal crops under abiotic stress” in Sustainable remedies for abiotic stress in cereals, ed. A. A. S. Abdel Latef (Springer, Singapore), 675–687.
Shultana, R., Zuan, A. T. K., Naher, U. A., Islam, A. K. M. M., Rana, M. M., Rashid, M. H., et al. (2022). The PGPR mechanisms of salt stress adaptation and plant growth promotion. Agronomy 12:2266. doi: 10.3390/agronomy12102266
Singh, D., Singh, S. K., Modi, A., Singh, P. K., Yeka Zhimo, V., and Kumar, A. (2020). “Impacts of agrochemicals on soil microbiology and food quality” in Agrochemicals detection, treatment and remediation, ed. M. N. V. Prasad (Butterworth-Heinemann), 101–116.
Singh, J., Singh, A. V., Upadhayay, V. K., Khan, A., and Chandra, R. (2022). Prolific contribution of Pseudomonas protegens in Zn biofortification of wheat by modulating multifaceted physiological response under saline and non-saline conditions. World J. Microbiol. Biotechnol. 38:227. doi: 10.1007/s11274-022-03411-4
Sivakumar, M. (2021). “Climate change, agriculture adaptation, and sustainability” in Climate resilience and environmental sustainability approaches, eds. A. Kaushik, C. P. Kaushik, and S. D. Attri (Springer, Singapore), 87–109.
Subotić, A., Jevremović, S., Milošević, S., Trifunović-Momčilov, M., Đurić, M., and Koruga, Đ. (2022). Physiological response, oxidative stress assessment and aquaporin genes expression of cherry tomato (Solanum lycopersicum L.) exposed to hyper-harmonized fullerene water complex. Plan. Theory 11:2810. doi: 10.3390/plants11212810
Sun, H., Peng, Q., Guo, J., Zhang, H., Bai, J., and Mao, H. (2022). Effects of short-term soil exposure of different doses of ZnO nanoparticles on the soil environment and the growth and nitrogen fixation of alfalfa. Environ. Pollut. 309:119817. doi: 10.1016/j.envpol.2022.119817
Tahiri, A.-I., Meddich, A., Raklami, A., Alahmad, A., Bechtaoui, N., Anli, M., et al. (2022). Assessing the potential role of compost, PGPR, and AMF in improving tomato plant growth, yield, fruit quality, and water stress tolerance. J. Soil Sci. Plant Nutr. 22, 743–764. doi: 10.1007/s42729-021-00684-w
Tanveer, Y., Yasmin, H., Nosheen, A., Ali, S., and Ahmad, A. (2022). Ameliorative effects of plant growth promoting bacteria, zinc oxide nanoparticles and oxalic acid on Luffa acutangula grown on arsenic enriched soil. Environ. Pollut. 300:118889. doi: 10.1016/j.envpol.2022.118889
Tariq, M., Mohammad, K. N., Ahmed, B., Siddiqui, M. A., and Lee, J. (2022). Biological synthesis of silver nanoparticles and prospects in plant disease management. Molecules 27:4754. doi: 10.3390/molecules27154754
Tazunoki, Y., Tokuda, M., Sakuma, A., Nishimuta, K., Oba, Y., Kadokami, K., et al. (2022). Comprehensive analyses of agrochemicals affecting aquatic ecosystems: a case study of Odonata communities and macrophytes in Saga Plain, northern Kyushu. Japan. Environ. Pollut. 292:118334. doi: 10.1016/j.envpol.2021.118334
Tripathi, S., Srivastava, P., Devi, R. S., and Bhadouria, R. (2020). “Influence of synthetic fertilizers and pesticides on soil health and soil microbiology” in Agrochemicals detection, treatment and remediation, ed. M. N. V. Prasad (Butterworth-Heinemann), 25–54.
Ul Haq, I., and Ijaz, S. (2019). “Use of metallic nanoparticles and nanoformulations as nanofungicides for sustainable disease management in plants” in Nanobiotechnology in bioformulations, eds. R. Prasad, V. Kumar, M. Kumar, & D. Choudhary (Springer, Cham), 289–316.
Upadhayay, V. K., Khan, A., Singh, J., and Singh, A. V. (2019). “Splendid role of nanoparticles as antimicrobial agents in wastewater treatment” in Microorganisms for sustainability, ed. R. Bharagava (Springer, Singapore), 119–136.
Upadhayay, V. K., Maithani, D., Dasila, H., Taj, G., and Singh, A. V. (2023). “Microbial services for mitigation of biotic and abiotic stresses in plants” in Advanced microbial techniques in agriculture, environment, and health management, eds. S. C. Pandey, V. Pande, D. Sati, & M. Samant (Academic Press), 67–81.
Upadhayay, V. K., Singh, A. V., and Khan, A. (2022a). Cross talk between zinc-solubilizing bacteria and plants: a short tale of bacterial-assisted zinc biofortification. Front. Soil Sci. 1:788170. doi: 10.3389/fsoil.2021.788170
Upadhayay, V. K., Singh, A. V., Khan, A., and Pareek, N. (2021). Influence of zinc solubilizing bacterial co-inoculation with zinc oxide supplement on rice plant growth and Zn uptake. Pharm. Innov. 10, 113–116.
Upadhayay, V. K., Singh, A. V., Khan, A., and Sharma, A. (2022c). Contemplating the role of zinc-solubilizing bacteria in crop biofortification: an approach for sustainable bioeconomy. Front. Agron. 4:903321. doi: 10.3389/fagro.2022.903321
Upadhayay, V. K., Singh, A. V., Khan, A., Singh, J., Pareek, N., and Raghav, A. (2022b). FE-SEM/EDX based zinc mobilization analysis of Burkholderia cepacia and Pantoea rodasii and their functional annotation in crop productivity, soil quality, and zinc biofortification of paddy. Front. Microbiol. 13:852192. doi: 10.3389/fmicb.2022.852192
Upadhayay, V. K., Singh, A. V., and Pareek, N. (2018). An insight in decoding the multifarious and splendid role of microorganisms in crop biofortification. Int. J. Curr. Microbiol. App. Sci. 7, 2407–2418. doi: 10.20546/ijcmas.2018.706.286
Vaghela, N., and Gohel, S. (2022). Medicinal plant-associated rhizobacteria enhance the production of pharmaceutically important bioactive compounds under abiotic stress conditions. J. Basic Microbiol. 63, 308–325. doi: 10.1002/jobm.202200361
Van Nguyen, D., Nguyen, H. M., Le, N. T., Nguyen, K. H., Nguyen, H. T., Le, H. M., et al. (2022). Copper nanoparticle application enhances plant growth and grain yield in maize under drought stress conditions. J. Plant Growth Regul. 41, 364–375. doi: 10.1007/s00344-021-10301-w
Vedamurthy, A. B., Bhattacharya, S., Das, A., and Shruthi, S. D. (2021). “Exploring nanomaterials with rhizobacteria in current agricultural scenario” in Advances in nano-fertilizers and nano-pesticides in agriculture, eds. S. Jogaiah, H. B. Singh, L. F. Fraceto, & R. de Lima (Woodhead Publishing), 487–503.
Vejan, P., Khadiran, T., Abdullah, R., Ismail, S., and Dadrasnia, A. (2019). Encapsulation of plant growth promoting rhizobacteria—prospects and potential in agricultural sector: a review. J. Plant Nutr. 42, 2600–2623. doi: 10.1080/01904167.2019.1659330
Vignardi, C. P., Muller, E. B., Tran, K., Couture, J. L., Means, J. C., Murray, J. L. S., et al. (2020). Conventional and nano-copper pesticides are equally toxic to the estuarine amphipod Leptocheirus plumulosus. Aquat. Toxicol. 224:105481. doi: 10.1016/j.aquatox.2020.105481
Walia, A., Putatunda, C., Bhatia, R. K., Solanki, P., and Pathania, S. (2021). “Techniques for improving microbial inoculants as a tool for sustainable development” in Microbiological activity for soil and plant health management, eds. R. Soni, D. C. Suyal, P. Bhargava, and R. Goel (Springer, Singapore), 599–627.
Xin, X., Judy, J. D., Sumerlin, B. B., and He, Z. (2020). Nano-enabled agriculture: from nanoparticles to smart nanodelivery systems. Environ. Chem. 17:413. doi: 10.1071/en19254
Xiong, W., Reynolds, M., and Xu, Y. (2022). Climate change challenges plant breeding. Curr. Opin. Plant Biol. 70:102308. doi: 10.1016/j.pbi.2022.102308
Xu, C., Peng, C., Sun, L., Zhang, S., Huang, H., Chen, Y., et al. (2015). Distinctive effects of TiO2 and CuO nanoparticles on soil microbes and their community structures in flooded paddy soil. Soil Biol. Biochem. 86, 24–33. doi: 10.1016/j.soilbio.2015.03.011
Yadav, A. S., and Srivastava, D. S. (2015). Application of nano-technology in weed management: a review. Res. Rev. J Crop Sci. Technol. 4, 21–23.
Yang, R. X., McCandler, C. A., Andriuc, O., Siron, M., Woods-Robinson, R., Horton, M. K., et al. (2022). Big data in a nano world: a review on computational, data-driven design of nanomaterials structures, properties, and synthesis. ACS Nano, 16, 19873–19871. doi: 10.1021/acsnano.2c08411
Yang, K., Wang, Q., Wang, Y., Li, S., Gu, Y., Gao, N., et al. (2021). Poly (γ-glutamic acid) nanocoating to enhance the viability of Pseudomonas stutzeri NRCB010 through cell surface engineering. ACS Appl. Mater. Interfaces 13, 39957–39966. doi: 10.1021/acsami.1c12538
Yasmin, H., Mazher, J., Azmat, A., Nosheen, A., Naz, R., Hassan, M. N., et al. (2021). Combined application of zinc oxide nanoparticles and biofertilizer to induce salt resistance in safflower by regulating ion homeostasis and antioxidant defence responses. Ecotoxicol. Environ. Saf. 218:112262. doi: 10.1016/j.ecoenv.2021.112262
Yin, S., Duvigneau, J., and Vancso, G. J. (2021). Fluorescent polyethylene by in situ facile synthesis of carbon quantum dots facilitated by silica nanoparticle agglomerates. ACS Appl. Polym. Mater. 3, 5517–5526. doi: 10.1021/acsapm.1c00821
Yin, J., Zhang, Z., Guo, Y., Chen, Y., Xu, Y., Chen, W., et al. (2022). Precision probiotics in agroecosystems: multiple strategies of native soil microbiotas for conquering the competitor Ralstonia solanacearum. mSystems 7:e0115921. doi: 10.1128/msystems.01159-21
Yue, L., Feng, Y., Ma, C., Wang, C., Chen, F., Cao, X., et al. (2022). Molecular mechanisms of early flowering in tomatoes induced by manganese ferrite (MnFe2O4) nanomaterials. ACS Nano 16, 5636–5646. doi: 10.1021/acsnano.1c10602
Zaheer, M. S., Raza, M. A. S., Saleem, M. F., Khan, I. H., Ahmad, S., Iqbal, R., et al. (2019). Investigating the effect of Azospirillum brasilense and Rhizobium pisi on agronomic traits of wheat (Triticum aestivum L.). Arch. Acker Pflanzenbau Bodenkd. 65, 1554–1564. doi: 10.1080/03650340.2019.1566954
Zain, M., Yasmeen, H., Yadav, S. S., Amir, S., Bilal, M., Shahid, A., et al. (2022). “Applications of nanotechnology in biological systems and medicine” in Nanotechnology for hematology, blood transfusion, and artificial blood, eds. A. Denizli, T. A. Nguyen, M. Rajan, M. F. Alam, & K. Rahman (Elsevier), 215–235.
Zarei, T. (2022). Balancing water deficit stress with plant growth-promoting rhizobacteria: a case study in maize. Rhizosphere 24:100621. doi: 10.1016/j.rhisph.2022.100621
Zhang, Y., Qian, C., Shi, R., Li, S., Shi, F., Hu, J., et al. (2022). In-situ synthesis of metal-organic framework embedded in ordered mesoporous silica functionalized with carboxyl groups for 4-nitrophenol to 4-aminophenol. Appl. Surf. Sci. 597:153720. doi: 10.1016/j.apsusc.2022.153720
Zhao, S., Su, X., Wang, Y., Yang, X., Bi, M., He, Q., et al. (2020). Copper oxide nanoparticles inhibited denitrifying enzymes and electron transport system activities to influence soil denitrification and N2O emission. Chemosphere 245:125394. doi: 10.1016/j.chemosphere.2019.125394
Zilberman, D., Gordon, B., Hochman, G., and Wesseler, J. (2018). Economics of sustainable development and the bioeconomy. Appl. Econ. Perspect. Policy 40, 22–37. doi: 10.1093/aepp/ppx051
Keywords: nanomaterials, plant probiotics, sustainable agriculture, soil fertility, bioeconomy
Citation: Upadhayay VK, Chitara MK, Mishra D, Jha MN, Jaiswal A, Kumari G, Ghosh S, Patel VK, Naitam MG, Singh AK, Pareek N, Taj G, Maithani D, Kumar A, Dasila H and Sharma A (2023) Synergistic impact of nanomaterials and plant probiotics in agriculture: A tale of two-way strategy for long-term sustainability. Front. Microbiol. 14:1133968. doi: 10.3389/fmicb.2023.1133968
Edited by:
Parul Chaudhary, National Dairy Research Institute (ICAR), IndiaReviewed by:
Bartholomew Saanu Adeleke, Olusegun Agagu University of Science and Technology, NigeriaAnuj Chaudhary, Shobhit University, India
Geeta Bhandari, Swami Rama Himalayan University, India
Copyright © 2023 Upadhayay, Chitara, Mishra, Jha, Jaiswal, Kumari, Ghosh, Patel, Naitam, Singh, Pareek, Taj, Maithani, Kumar, Dasila and Sharma. This is an open-access article distributed under the terms of the Creative Commons Attribution License (CC BY). The use, distribution or reproduction in other forums is permitted, provided the original author(s) and the copyright owner(s) are credited and that the original publication in this journal is cited, in accordance with accepted academic practice. No use, distribution or reproduction is permitted which does not comply with these terms.
*Correspondence: Viabhav Kumar Upadhayay, viabhav.amu@gmail.com; Manoj Kumar Chitara, manojchitara01@gmail.com
†These authors have contributed equally to this work and share first authorship