- 1State Key Laboratory for Conservation and Utilization of Bioresources in Yunnan, College of Plant Protection, Yunnan Agricultural University, Kunming, China
- 2Graduate School, Yunnan Agricultural University, Kunming, China
- 3Yunnan Plant Protection and Quarantine Station, Kunming, China
- 4Yunnan Plateau Characteristic Agriculture Industry Research Institute, Kunming, China
Beneficial microorganisms play a pivotal role in the invasion process of exotic plants, including arbuscular mycorrhizal fungi (AMF) and Bacillus. However, limited research exists on the synergistic influence of AMF and Bacillus on the competition between both invasive and native plants. In this study, pot cultures of Ageratina adenophora monoculture, Rabdosia amethystoides monoculture, and A. adenophora and R. amethystoides mixture were used to investigate the effects of dominant AMF (Septoglomus constrictum, SC) and Bacillus cereus (BC), and the co-inoculation of BC and SC on the competitive growth of A. adenophora. The results showed that inoculation with BC, SC, and BC + SC significantly increased the biomass of A. adenophora by 14.77, 112.07, and 197.74%, respectively, in the competitive growth between A. adenophora and R. amethystoides. Additionally, inoculation with BC increased the biomass of R. amethystoides by 185.07%, while inoculation with SC or BC + SC decreased R. amethystoides biomass by 37.31 and 59.70% compared to the uninoculated treatment. Inoculation with BC significantly increased the nutrient contents in the rhizosphere soil of both plants and promoted their growth. Inoculation with SC or SC + BC notably increased the nitrogen and phosphorus contents of A. adenophora, therefore enhancing its competitiveness. Compared with single inoculation, dual inoculation with SC and BC increased AMF colonization rate and Bacillus density, indicating that SC and BC can form a synergistic effect to further enhance the growth and competitiveness of A. adenophora. This study reveals the distinct role of S. constrictum and B. cereus during the invasion of A. adenophora, and provide new clues to the underlying mechanisms of interaction between invasive plant, AMF and Bacillus.
1. Introduction
The invasion of exotic plants has resulted in a rapid decline in global biodiversity (Powell et al., 2013), with severe implications on the functioning of the whole ecosystem function (Richardson and Pyšek, 2012) and incurring significant economic losses. Thus, understanding the invasion mechanisms of these plants has emerged as a pressing and pragmatic concern (Sol et al., 2012; Anna et al., 2020). The spreading capacity in their new habitat is at least partly influenced by their association with symbiotic microorganisms (Fahey and Stephen, 2022). Beneficial symbiotic microorganisms regulate competitive growth between invasive and native plants (Abbott et al., 2015; Dawson et al., 2016). The Enhanced Mutualisms Hypothesis (EMP) suggests that invasive plants facilitate positive soil feedback by enriching their associated beneficial symbiotic microbes, thereby promoting their own growth and expansion (D’Antonio et al., 2017; Zhang et al., 2020). In contrast, the Degraded Mutualisms Hypothesis (DMP) proposes that exotic plants can weaken the symbiotic relationship between beneficial microbes and native plants, creating a competitive disadvantage for the latter and facilitating the successful invasion of invasive plants (Vogelsang and Bever, 2009).
Arbuscular mycorrhizal fungi (AMF) can form a mutualistic symbiosis with most terrestrial plants to adapt better to limited nutrient supply conditions (Smith and Smith, 2011). In most nutrient-poor soils, the low availability of nitrogen and phosphorus is often the main limiting factor affecting plant growth (Xu et al., 2012). AMF is reported to dominate the uptake of phosphate, ammonium and nitrate nitrogen uptake in symbiotic plants (Fan et al., 2020; Wang et al., 2020; Xie et al., 2022). Accumulating evidence suggests that AMF can develop symbiosis with invasive plants, which greatly contributes to the successful invasion of invasive plants (Lekberg et al., 2013; Aslani et al., 2019). AMF enhances the resistance of invasive plants to biotic and abiotic stresses, thus promoting the establishment of invasive plants in their new habitats (Dickie et al., 2017; Qi et al., 2022). The exotic plants affect AMF abundance and richness (Zhang et al., 2017; Kong et al., 2022). When invasive plants grow alongside native plants, the mycorrhizal colonization rate of the invasive plants increases while that of the native plants decreases, giving invasive plants a competitive advantage over native plants (Zhang et al., 2018). AMF forms a common mycorrhizal network (CMN) that allows the plant-to-plant transfer of carbon and mineral nutrients between native plants and alien plants (Weremijewicz et al., 2018; Awaydul et al., 2019). Therefore, AMFs are more beneficial to invasive plants than native species, which may be a key factor in the successful invasion of exotic plants.
Bacillus is an important plant growth-promoting rhizobacteria (PGPR). Most Bacillus have many beneficial effects on plants and can promote plant growth by activating soil nutrients and producing phytohormones (Fan et al., 2018; Saxena et al., 2020), which also protects plants from biotic stresses by directly inhibiting plant pathogens and inducing plants to acquire systemic resistance (Stefany et al., 2021; Kurniawan and Chuang, 2022). Bacillus diversity and abundance differed in the rhizosphere soil of invasive and native plants. Chen et al. (2022) found that Bacillus diversity differed in the rhizosphere soil of the exotic (Flaveria bidentis) and native plants (Setaria viridis). The relative abundance of Brecibacterium frigoritolerans was higher in the F. bidentis rhizosphere than in the S. viridis rhizosphere. Additionally, the dominant Bacillus in the F. bidentis rhizosphere promoted F. bidentis competitive growth by elevating soil nitrogen and phosphorus levels. These studies indicate that Bacillus is also crucial for the successful invasion of exotic plants. As previously evidenced, AMF and PGPR can mutually promote their own growth and development, forming a synergistic effect to further enhance plant growth and promote their tolerance to diverse stresses (Krishnamoorthy et al., 2016; Hidri et al., 2019). However, few studies have examined whether combinations of AMF and Bacillus would contribute to the successful invasion of invasive plants. Therefore, understanding the effects of AMF, Bacillus and their combinations in the rhizosphere soil on the competitiveness of invasive plants with native plants is conducive to exploring the ecological role of two functional rhizosphere microorganisms in plant invasion.
Ageratina adenophora (Spreng.), a perennial herbaceous plant of the Asteraceae family, originates from Mexico and Costa Rica. Due to its strong reproductive and dispersal capabilities, morphological plasticity and stress tolerance, it has invaded many countries across Asia, Africa, and Oceania (Poudel et al., 2019; Tang et al., 2019; Gu et al., 2021). Since its first introduction from Myanmar into the Yunnan Province of China in the 1940s, A. adenophora has spread widely across southwestern and central China, becoming a dominant and invasive plant in China (Wang and Wang, 2006; Gui et al., 2009). A. adenophora’s colonization in southwestern China expanded considerably, with its suitable habitat distribution rapidly advancing eastward and northward (Li W. T. et al., 2022). The invasion of this plant has resulted in the destruction of native biodiversity, alteration of ecological community structures, and posed a severe threat to the development of agriculture, forestry and livestock industries, resulting in enormous economic and ecological losses (Song et al., 2017; Wang et al., 2017; Ren et al., 2021). A. adenophora has been shown to selectively aggregate functional microbes that mediate soil nutrient cycling to form a favorable soil microenvironment in the invasive habitats that facilitate its invasion (Niu et al., 2007; Zhao et al., 2007; Li Q. et al., 2022). In contrast to native plant rhizosphere soil, A. adenophora rhizosphere soil exhibits a remarkably higher abundance of nitrogen-fixing bacteria, phosphorus-solubilizing bacteria, and IAA-producing bacteria (Xu et al., 2012; Fang et al., 2019). Our previous studies demonstrated that Bacillus cereus has a high abundance in the rhizosphere soil of A. adenophora and R. amethystoides and exerted a positive feedback effect on A. adenophora (Sun et al., 2021; Du et al., 2022a). The AM fungus Septoglomus constrictum was also identified in the rhizosphere soil of A. adenophora and R. amethystoides, which improved the growth of A. adenophora and its resistance to A. gossypii feeding (Yu et al., 2012; Li et al., 2016; Du et al., 2022b). However, the relationship between AMF and Bacillus in the rhizosphere of A. adenophora and R. amethystoides and the effects of this relationship on the competitive growth between the two plants remain unknown.
To address this knowledge gap, we hypothesized that a synergistic interaction between B. cereus and S. constrictum might enhance the competitive growth of A. adenophora. To test this hypothesis, we compared the effects of single inoculation with AMF or Bacillus and co-inoculation of two kinds of microorganisms on the AMF colonization rate and Bacillus density of A. adenophora to explore whether both microorganisms form a synergistic effect. To illustrate the impact and reasons of microorganisms on plant growth, we compared their biomass, relative competitiveness, root growth characteristics, and nutrient content, as well as the soil’s available nutrient content.
2. Materials and methods
2.1. Microbial inoculation preparation
The spores of S. constrictum were isolated from the rhizosphere soil of A. adenophora in our previous study (Du et al., 2022b). The mycorrhizal inoculum, consisting of fragments of colonized roots, spores, and hyphae of S. constrictum, was propagated using maize as the host plant. The spore density (20 spores/100 g of soil) was determined based on the quantity of S. constrictum spores in the rhizosphere soil of A. adenophora in the field and used for subsequent analyses.
The B. cereus strain A20 (GenBank accession: OM149794) was isolated from A. adenophora and R. amethystoides rhizosphere soil (Du et al., 2022a). The strain’s organic phosphate-solubilizing ability, inorganic phosphate-solubilizing ability, potassium-solubilizing ability, nitrogen-fixing ability, and IAA-producing ability were 53.66, 92.38, 51.33, 23.67, 61.55 mg/L, respectively. The siderophores-producing ability of the strain was 0.48, according to the methodology of Payne (1994). The strain was separately cultured on nutrient agar plates at 37°C for 8–12 h to obtain single colonies. The colonies of activated Bacillus were selected using an aseptic toothpick and incubated in 1 mL of nutrient liquid medium culture in a 1.5 mL centrifuge tube. After shaking at 180 rpm for 24 h at 37°C, the liquid was transferred into a triangular flask containing 100 mL of nutrient liquid broth medium and shaken at 180 rpm for 24 h at 37°C. The Bacillus suspension was then expanded to a concentration of 108 CFU/mL (Sun et al., 2022).
2.2. Plants and soil preparation
The soils and seeds of the exotic and native plants were purchased from Yunnan Agricultural University (Kunming, China; 25°08′30″ N, 102°45′13″ E, altitude 1964 m). Before sowing, the seeds were surface disinfected for 10 min in a 5% sodium hypochlorite solution. Next, the seeds were washed 5 times using sterile water, followed by 1 min soaking in 75% alcohol and rinsing 5 times in sterile water. The soil was crushed, sieved (2 mm), then mixed with vermiculite (v/v = 1:1) [(Mg, Fe, Al)3[(Si,Al)4O10(OH)2]. 4H2O] (Dounan Plant and Flower Co., Ltd., Kunming, China). The properties of the soil were as follows: pH = 6.25, 15.502 g/kg organic matter, 0.899 g/kg total nitrogen, 0.351 g/kg total phosphorus, 40.03 g/kg total potassium, 20.28 μg/g available nitrogen, 5.089 μg/g available phosphorus (AP), and 32.32 mg/kg available potassium (AK). Lastly, the mixtures were subjected to 2 h heating (121°C) in an autoclave.
2.3. Experiment design
The impact of AMF and Bacillus on competitive A. adenophora and R. amethystoides growth was investigated by conducting a greenhouse experiment at the Yunnan Agricultural University. The experiment considered two factors: (1) Plant type: A. adenophora, R. amethystoides, A. adenophora and R. amethystoides, and (2) inoculum treatments: C (uninoculated treatment), BC (inoculated with B. cereus), SC (inoculated with S. constrictum), and BC + SC (dual-inoculation with B. cereus and S. constrictum). Following the design of Gibson et al. (1999) and Zhang et al. (2018), the planting included a monoculture of A. adenophora and R. amethystoides, and A. adenophora and R. amethystoides mixture, with two plants per pot for monoculture treatment, and one A. adenophora and one R. amethystoides per pot for mixture treatment. Before starting the experiment, 1 kg soil in the pots was used for sowing the seeds of A. adenophora and/or R. amethystoides and inoculums of S. constrictum (20 per/100 g of soil) and/or B. cereus (10 mL 108 CFU/mL). For the non-AMF treatment, we added the same amount of sterilized inoculum and the filtrate (<20 mm) of the AMF inoculum, while for the non-Bacillus treatment, we added 10 mL of the sterilized bacterial suspension. The study was conducted using a randomized design comprising 10 repetitions per treatment (3 planting treatments × 4 inoculation treatments × 10 replicates = 120 pots). The plants were watered with sterile water once every 2 days, and the seeds were grown in a controlled environment in a greenhouse at a temperature of 25°C with 10 h light/14 h dark.
2.4. Measurement
2.4.1. Biomass and corrected index of relative competition intensity
Ageratina adenophora and Rabdosia amethystoides were collected under different treatments following germination for 120 days. The soil in the roots and shoots was collected to measure their biomass. All roots and shoots were dried (80°C, 72 h), and the growth index data were obtained. The total biomass (aboveground and belowground biomass) was measured. The plant competitiveness was quantified using CRCI, calculated following the methodology of Oksanen et al. (2006):
CRCI = arcsin [(X – Y) / max (X, Y)],
where X and Y represent individual plant biomass in intraspecific and interspecific competition, respectively; CRCI value >0 represents the negative effect, whereas CRCI value <0 represents the positive effect of the competition on the target plant.
2.4.2. Root growth characteristics
The roots were washed, cut, and evenly distributed in a scanning tray filled with water. They were scanned using a root scanner (Epson Expression 10000XL; Epson, Long Beach, CA, United States) and analyzed using the WinRhizo software (Regent Instruments Inc., Québec City, QC, Canada) and their root length (RL), root surface area (RS), root diameter (RD), and root volume (RV) were calculated.
2.4.3. Total nitrogen, phosphorus and potassium concentration
The dried plants were ground with a high-throughput Tissuelyser-48 grinder (Shanghai Jingxin Industrial Development Co., Ltd. Shanghai, China). C concentration was determined using 20 mg plant powder via the potassium dichromate-concentrated sulfuric acid oxidation method (K2Cr2O7-H2SO4) (Kong et al., 2022). The plant samples (2 g powder) were digested in a concentrated perchloric and nitric acids mixture (v:v = 1: 6) to measure the N, P, and K concentrations. Nitrogen and phosphorus content was analyzed separately using the micro-Kjeldahl method (Nelson and Sommers, 1973) and inductively coupled plasma spectroscopy (Isaac and Johnson, 1983). Six replicates were set up for each treatment.
2.4.4. Colonization of AMF
After rinsing in 10% KOH, the roots were acidified with 2% HCl and stained with 0.1% acid fuchsin solution. Then, the mycorrhizal root colonization percentage was determined by visually observing fungal colonization (Zhang et al., 2017). The magnified intersections method was used for analyzing AMF colonization in the A. adenophora and R. amethystoides roots (Giovannetti and Mosse, 1980; Biermann and Linderman, 1981). Two hundred root segments for each replicate were analyzed using the Olympus BX43 compound microscope (Olympus, Tokyo, Japan), and six replicates per treatment were conducted. The colonization percentage of each segment was measured by colonization (presence of hyphae, vesicles, or arbuscules) in that region. AMF colonization was calculated by combining the percentage colonization of the 200 root segments.
2.4.5. Density of Bacillus cereus
The density of B. cereus in each soil sample was analyzed to investigate different treatment impacts on their growth on nutrient agar medium plates using the suspension dilution method (Yang et al., 2012; Du et al., 2020; Sun et al., 2022). Briefly, 1 g rhizosphere soil with 9 mL of sterile water was incubated at 200 r/min, heated at 90°C for 10 min, and serially diluted. Then, 0.1 mL of 10−3 supernatant was added to nutrient agar plates and cultured for 18 h at 37°C. Colonies were counted as the colony-forming units/ per gram of dry soil (CFU/g) according to volume dilution. Six replicates were conducted for each treatment.
2.5. Statistical analysis
The variables are expressed as mean ± standard error (n = 6). The SPSS v21.0 (IBM, Armonk, New York) software was used for statistical analyses. The Shapiro–Wilk test was used for testing data normality. All data conformed to normality distribution. A two-way ANOVA (Tuckey test) was conducted to determine inoculum effects on the biomass, root growth characteristics, total N, P, and K concentrations, and soil characteristics in the monoculture and mixture treatment. Differences among different inoculums (C, BC, SC, and BC + SC) in these variables above were determined using multiple comparisons (Tuckey test). A one-way ANOVA (Tuckey test) was conducted to determine inoculum effects on CRCI, AMF colonization rate, and Bacillus density in the monoculture and mixture treatment. Monoculture and the mixture influence on plant growth were evaluated using Student’s t-test. Pearson’s rank correlation coefficient was used to analyze the correlation between AMF colonization and plant growth parameters. All graphics were created by Excel and Origin 2019 (OriginLab, United States).
3. Results
3.1. Impacts of competition and inoculum on Ageratina adenophora and Rabdosia amethystoides biomass
The present study investigates the impact of competition on the biomass of two plant species, A. adenophora and R. amethystoides, and the effect of inoculation treatments on their growth (Figure 1 and Supplementary Table S1). Our results demonstrate that competition has an opposite effect on the two species. A. adenophora exhibited a significantly higher biomass in the presence of other plants [C: F(1,10) = 0.288; BC: F(1,10) = 2.164; SC: F(1,10) = 3.501; BC + SC: F(1,10) = 21.928; all p < 0.001], whereas R. amethystoides showed a higher biomass in monoculture [C: F(1,10) = 1.173; BC: F(1,10) = 5.483; SC: F(1,10) = 2.564; BC + SC: F(1,10) = 1.342; all p < 0.001]. The inoculation treatments significantly increased the biomass of both species, regardless of the presence [F(3,20) = 124.773, p < 0.001] or absence of competition [F(3,20) = 113.963, p < 0.001]. In particular, the BC + SC treatment showed the greatest positive impact, increasing A. adenophora biomass by 197.74 and 116.39% in the mixture and monoculture treatments, respectively. Interestingly, the effect of inoculation treatments varied depending on the presence of competition. The biomass of R. amethystoides in the mixture treatment was significantly reduced by the SC and BC + SC treatments, while the BC treatment led to a remarkable increase. In contrast, all inoculation treatments positively impacted the biomass of R. amethystoides in the monoculture treatment. These results suggest that competition may modulate the response of plants to inoculation treatments.
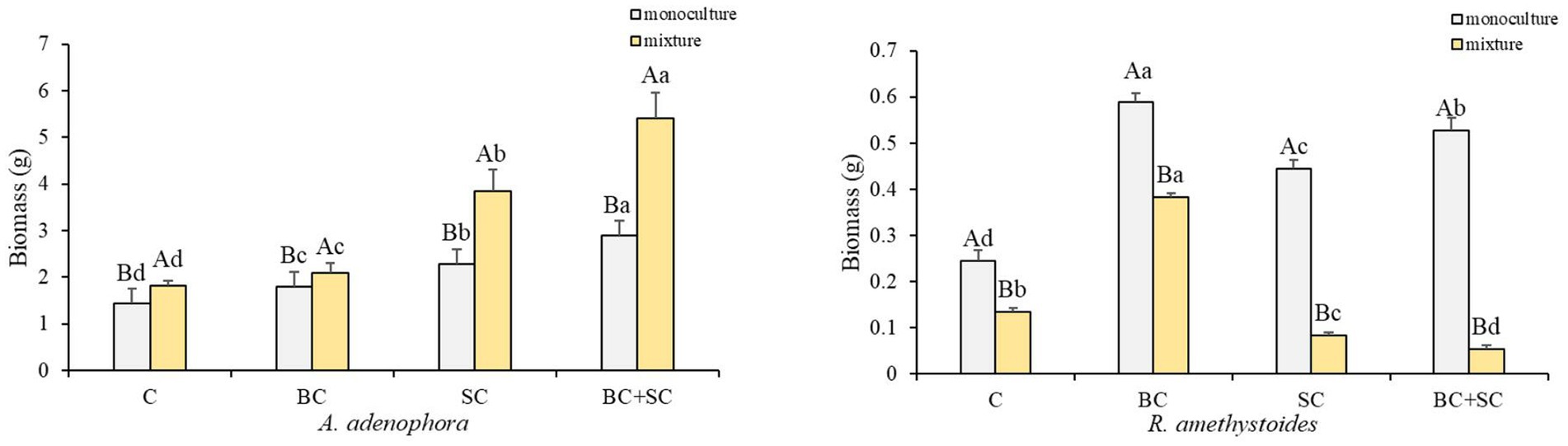
Figure 1. Effect of competition and inoculum on A. adenophora and R. amethystoides biomass. C, control; BC, inoculated with B. cereus; SC, inoculated with S. constrictum; BC + SC, inoculated with B. cereus and S. constrictum. Different lowercase letters in lower case indicate significant differences between the four treatments at p < 0.05. Different uppercase letters indicate significant differences between the monoculture or mixture at p < 0.05. Error bars represent ± SE of mean (n = 6).
3.2. Impacts of inoculum on the corrected index of relative competition intensity of Ageratina adenophora and Rabdosia amethystoides
Our results demonstrate that interspecific competition has a significant positive effect on A. adenophora growth [F(3,20) = 31.547, p < 0.001] and a significant negative effect on R. amethystoides growth [F(3,20) = 492.927, p < 0.001] in both non-inoculation and inoculation treatments (Figure 2). Additionally, inoculation with BC reduced the positive effect of interspecific competition on A. adenophora (p = 0.251), while inoculation with SC and BC + SC enhanced the positive effect on A. adenophora (SC: p = 0.001; SC + BC: p < 0.001). Inoculation of BC treatment alleviated the negative effect on R. amethystoides growth when grown with A. adenophora in comparison with the control (p < 0.001), while inoculation with SC and BC + SC treatments enhanced this negative effect (SC: p < 0.001; SC + BC: p < 0.001).
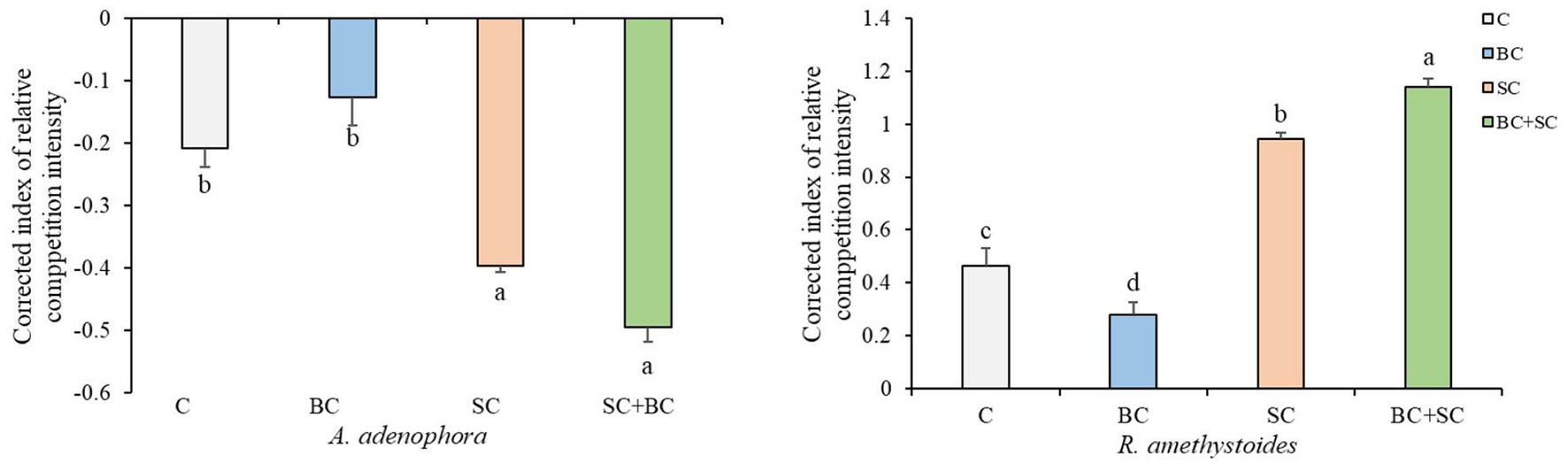
Figure 2. Effect of inoculum on A. adenophora and R. amethystoides CRCI. C, control; BC, inoculated with B. cereus; SC, inoculated with S. constrictum; BC + SC, inoculated with B. cereus and S. constrictum. Different lowercase letters in lower case indicate significant differences between the four treatments at p < 0.05. Error bars represent ± SE of mean (n = 6).
3.3. Impacts of competition and inoculum on root growth characteristics of Ageratina adenophora and Rabdosia amethystoides
The effect of competition on root length, root surface area, root diameter and root volume of A. adenophora and R. amethystoides were consistent with the biomass trend (Figure 3 and Supplementary Table S1). Monoculture treatment of A. adenophora led to significantly lower root growth characteristics than mixture treatment [C: root length: F(1,10) = 3.478; root surface area: F(1,10) = 2.822; root diameter: F(1,10) = 3.451; root volume: F(1,10) = 0.074; BC: root length: F(1,10) = 0.564; root surface area: F(1,10) = 0.277; root diameter: F(1,10) = 5.739; root volume: F(1,10) = 0.542; SC: root length: F(1,10) = 0.645; root surface area: F(1,10) = 0.065; root diameter: F(1,10) = 0.273; all p < 0.001; root volume: F(1,10) = 3.259, p = 0.009; BC + SC: root length: F(1,10) = 1.695, p < 0.001; root surface area: F(1,10) = 6.935, p = 0.001; root diameter: F(1,10) = 1.291, p = 0.002; root volume: F(1,10) = 0.089, p = 0.001], while monoculture treatment of R. amethystoides was associated with significantly higher root growth characteristics compared to mixture treatment [C: root length: F(1,10) = 1.127, p < 0.001; root surface area: F(1,10) = 1.217, p < 0.001; root diameter: F(1,10) = 1.135, p = 0.049; root volume: F(1,10) = 2.646; BC: root length: F(1,10) = 13.136; root surface area: F(1,10) = 11.633; root diameter: F(1,10) = 0.178; root volume: F(1,10) = 4.546; SC: root length: F(1,10) = 1.483; root surface area: F(1,10) = 1.348; root diameter: F(1,10) = 2.248; root volume: F(1,10) = 1.613; BC + SC: root length: F(1,10) = 1.839; root surface area: F(1,10) = 1.938; root diameter: F(1,10) = 2.170; root volume: F(1,10) = 12.570; all p < 0.001]. For A. adenophora, the root growth characteristics were significantly increased by inoculation treatment both in monoculture and mixture treatment, among which the root growth characteristics of BC + SC treatment were significantly higher (p < 0.05). Additionally, inoculation treatment of R. amethystoides in monoculture and mixture treatments with BC exhibited a significant increase in the root growth characteristics (p < 0.05), while inoculation treatments with SC and BC + SC resulted in a significant decrease in the root growth characteristics of R. amethystoides (p < 0.05).
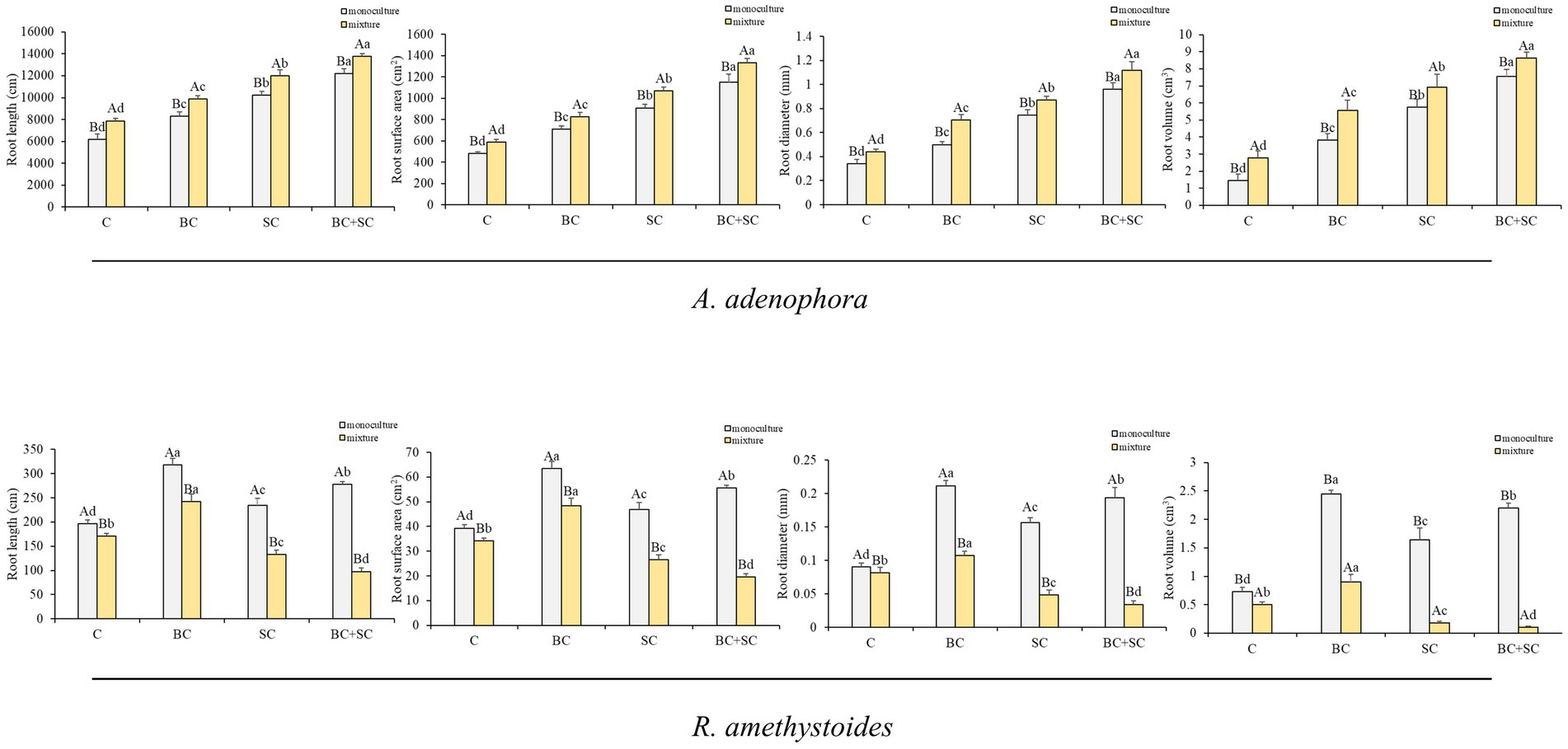
Figure 3. Effect of competition and inoculum on the root growth characteristics of A. adenophora and R. amethystoides. C, control; BC, inoculated with B. cereus; SC, inoculated with S. constrictum; BC + SC, inoculated with B. cereus and S. constrictum. Different lowercase letters significant differences between the four treatments at p < 0.05. Different uppercase letters indicate significant differences between the monoculture or mixture at p < 0.05. Error bars represent ± SE of mean (n = 6).
3.4. Effects of competition and inoculum on Ageratina adenophora and Rabdosia amethystoides nutrient concentrations
Competition effects on total N, P and K concentrations of A. adenophora and R. amethystoides were consistent with the trend in biomass (Figure 4 and Supplementary Table S1). A. adenophora in monoculture treatment showed significantly higher nitrogen, phosphorus and potassium concentrations than in mixture treatment [C: N: F(1,10) = 1.285; P: F(1,10) = 11.400; K: F(1,10) = 1.556; all p < 0.001; BC: N: F(1,10) = 0.010, p = 0.005; P: F(1,10) = 1.560, p < 0.001; K: F(1,10) = 0.890, p < 0.001; SC: N: F(1,10) = 0.109, p = 0.032; P: F(1,10) = 2.822, p < 0.001; K: F(1,10) = 0.632, p < 0.001; BC + SC: N: F(1,10) = 0.509, p = 0.001; P: F(1,10) = 2.822, p < 0.001; K: F(1,10) = 0.068, p < 0.001], while R. amethystoides in monoculture treatment had significantly increased nutrient concentrations than those in mixture treatment [C: N: F(1,10) = 3.491, p < 0.001; P: F(1,10) = 0.872, p = 0.001; K: F(1,10) = 1.101; BC: N: F(1,10) = 1.649; P: F(1,10) = 4.085; K: F(1,10) = 0.039; SC: N: F(1,10) = 1.574; P: F(1,10) = 3.467; K: F(1,10) = 1.337; BC + SC: N: F(1,10) = 0.434; P: F(1,10) = 2.778; K: F(1,10) = 0.448; all p < 0.001]. For A. adenophora, inoculation treatment significantly increased N, P, and K concentrations in monoculture and mixture treatments (p < 0.001). The nutrient contents of BC + SC treatment were significantly increased compared to other treatments (p < 0.001), and the N, P and K concentrations were increased by 41.47, 30.56, and 34.09% in monoculture treatment and 35.97, 43.26, and 28.61% in mixture treatment, respectively. For R. amethystoides, inoculation with BC treatment significantly enhanced N, P, and K concentrations in both monoculture and mixture treatments (p < 0.001), while inoculation of SC and BC + SC significantly increased the nutrient concentrations in monoculture treatment (p < 0.001) but decreased N and P concentrations in mixture treatment (p < 0.001). Inoculation with SC treatment reduced the N and P concentrations by 5.42 and 4.47%, and inoculation with BC + SC treatment reduced the N and P by 10.78 and 14.41% in mixture treatment, respectively.
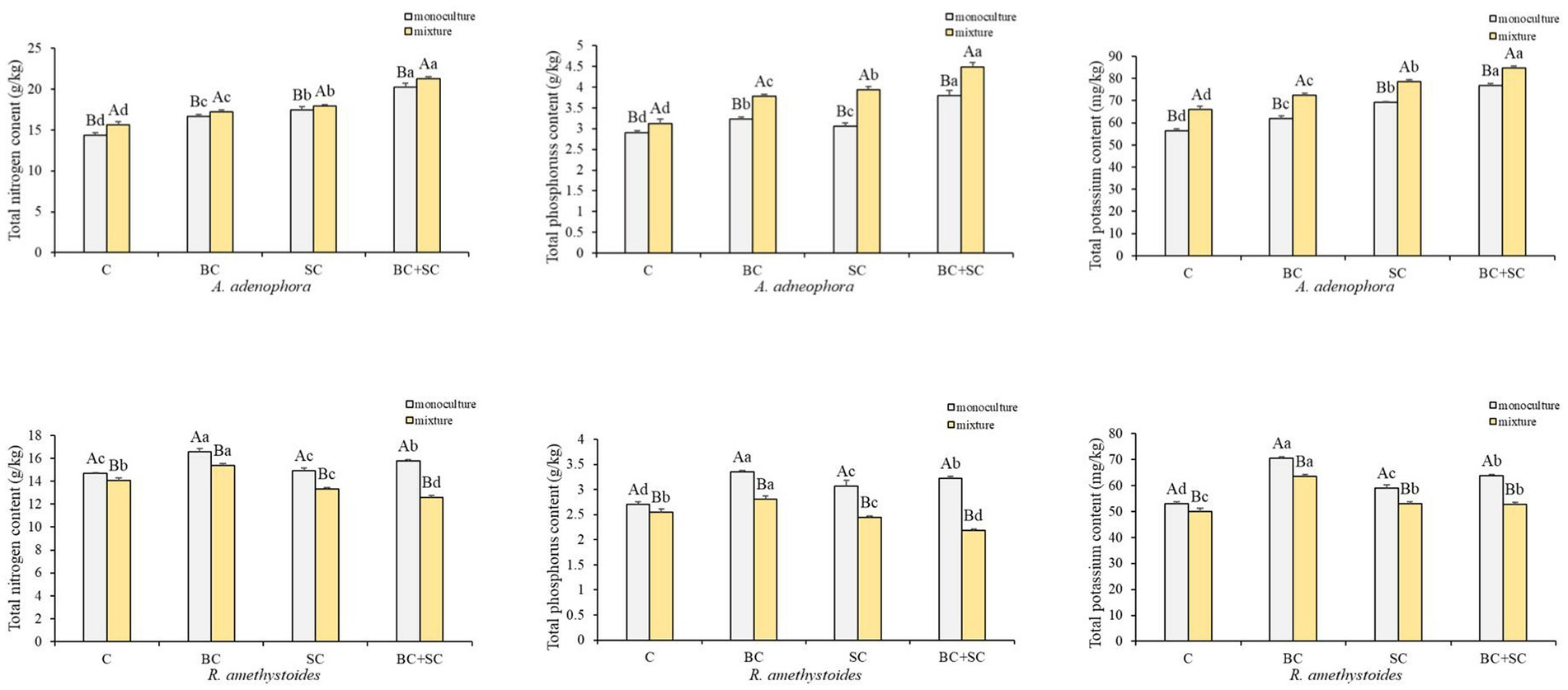
Figure 4. Effect of competition and inoculum on the nutrient concentrations of A. adenophora and R. amethystoides. C, control; BC, inoculated with B. cereus; SC, inoculated with S. constrictum; BC + SC, inoculated with B. cereus and S. constrictum. Different lowercase letters in lower case indicate significant differences between the four treatments at p < 0.05. Different uppercase letters indicate significant differences between the monoculture or mixture at p < 0.05. Error bars represent ± SE of mean (n = 6).
3.5. Effects of competition and inoculum on AMF colonization and Bacillus density of Ageratina adenophora and Rabdosia amethystoides
Ageratina adenophora had a higher colonization rate than R. amethystoides in treatments with SC and BC + SC (Figure 5). Competition differentially affected the AMF colonization of the two plants. A. adenophora in mixture treatment showed a remarkably higher AMF colonization rate than that in monoculture treatment [SC: F(1,10) = 0.003; BC + SC: F(1,10) = 1.104; both p < 0.001], while R. amethystoides in mixture treatment had a significantly lower AMF colonization rate than that in monoculture treatment [SC: F(1,10) = 0.064; BC + SC: F(1,10) = 2.844; both p < 0.001]. A. adenophora inoculated with BC + SC had a significantly higher AMF colonization rate than that inoculated with SC in both monoculture and mixture treatments [monoculture: F(3,20) = 102.150; mixture: F(3,20) = 48.412, both p < 0.001]. However, R. amethystoides inoculated with SC had a significantly higher AMF colonization rate than that inoculated with SC in both monoculture and mixture treatment [monoculture: F(3,20) = 32.194; mixture: F(3,20) = 18.654; both p < 0.001].
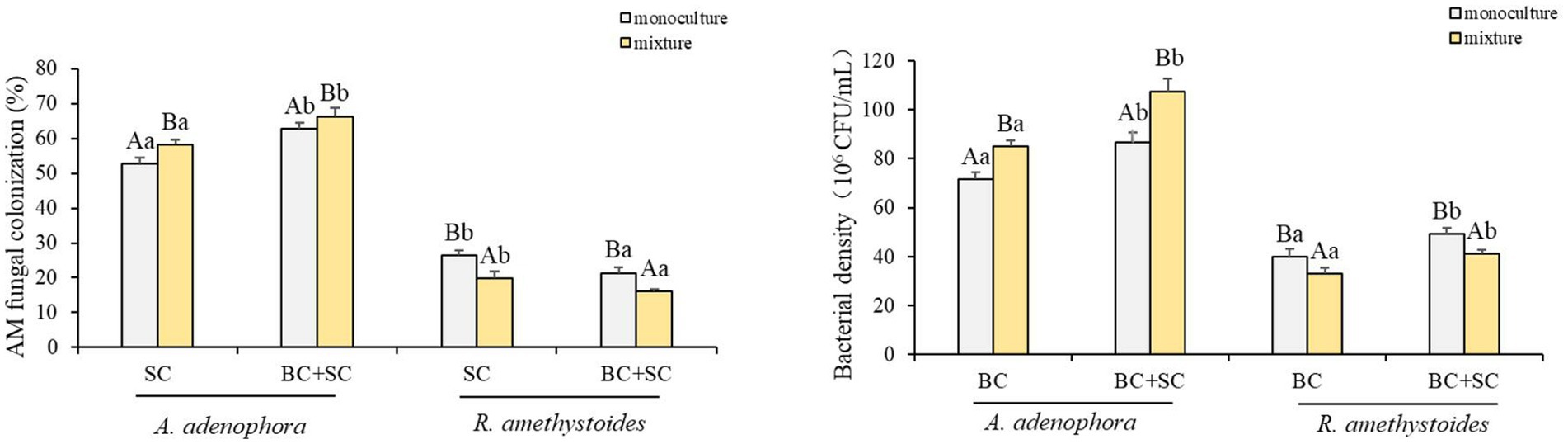
Figure 5. Effect of competition and inoculum on the AMF colonization and Bacterial density of A. adenophora and R. amethystoides. C, control; BC, inoculated with B. cereus; SC, inoculated with S. constrictum; BC + SC, inoculated with B. cereus and S. constrictum. Different lowercase letters in lower case indicate significant differences between the four treatments at p < 0.05. Different uppercase letters indicate significant differences between the monoculture or mixture at p < 0.05. Error bars represent ± SE of mean (n = 6).
Bacillus density in A. adenophora rhizosphere soil was significantly higher than in R. amethystoides rhizosphere soil in all treatments (Figure 5). In addition, the Bacillus density was significantly higher in A. adenophora rhizosphere soil in mixture treatment than that in monoculture treatment [BC: F(1,10) = 0.363; BC + SC: F(1,10) = 0.630; both p < 0.001]. However, Bacillus density was significantly higher in R. amethystoides rhizosphere soil in monoculture treatment than that in mixture treatment [BC: F(1,10) = 1.875; BC + SC: F(1,10) = 0.870; both p < 0.001]. Further, the Bacillus density in rhizosphere soil of A. adenophora and R. amethystoides in BC + SC treatment was significantly increased relative to that with BC treatment [A. adenophora: monoculture: F(3,20) = 58.615; mixture: F(3,20) = 90.504; R. amethystoides: monoculture: F(3,20) = 34.690; mixture: F(3,20) = 38.400; all p < 0.001].
3.6. Impact of competition and inoculum on soil characteristic
For A. adenophora monoculture treatment and A. adenophora and R. amethystoides mixture treatment, inoculation significantly increased nitrate-nitrogen (NO3−-N) and AP content in rhizosphere soil [Am: NO3−-N: F(3,20) = 353.767; AP: F(3,20) = 158.065; A + R: NO3−-N: F(3,20) = 211.638; AP: F(3,20) = 294.625; all p < 0.001, Table 1, and Supplementary Table S1]. Among them, NO3−-N and AP contents in the rhizosphere soil of BC inoculated treatment were significantly increased (p < 0.001). For R. amethystoides monoculture treatment, NO3−-N, AP, ammonium N (NH4+-N) and AK contents were significantly increased by the inoculation treatment [NO3−-N: F(3,20) = 179.125; AP: F(3,20) = 28.439; NH4+-N: F(3,20) = 213.247; AK: F(3,20) = 47.405; all p < 0.001], among which the available nutrient content of the rhizosphere soil of BC inoculation treatment was significantly increased (p < 0.001).
3.7. Correlation of AMF colonization rate and the density of Bacillus with plant growth indicator and soil characteristics
Here, we investigated the impact of monoculture and mixed cropping of A. adenophora with R. amethystoides on the colonization rate of S. constrictum and B. cereus, as well as their correlation with various growth parameters and nutrient concentrations in two different soil types, SC and BC + SC. Our findings indicated that S. constrictum colonization rate was positively correlated with biomass, total N, P and K concentrations, root growth characteristics, NO3−-N, and AP, but a negatively correlated with NH4+-N contents (except in SC inoculated monoculture treatment) and AK contents (Figure 6). In the mixture treatment of A. adenophora with R. amethystoides in SC and BC + SC treatment, S. constrictum colonization rate in R. amethystoides was negatively associated with biomass, total N and P concentrations and root growth characteristics, but positively correlated with total K concentration and soil characteristics (Figure 6).
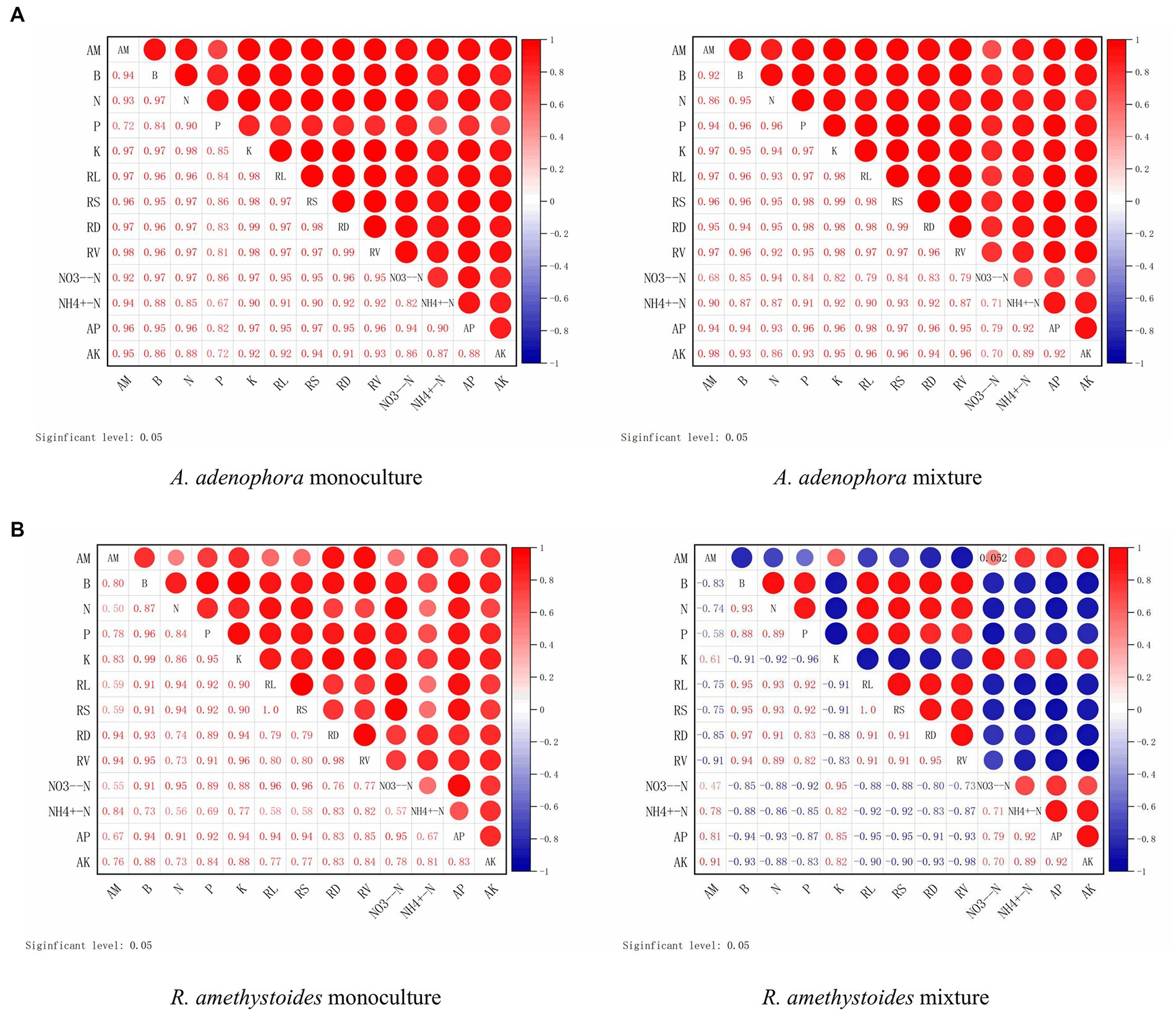
Figure 6. Correlation between root colonization by an arbuscular mycorrhizal fungus, S. constrictum, and plant growth indicators of A. adenophora (A) and R. amethystoides (B). AM, AM colonization; B, biomass; RL, root length; RS, root surface area; RD, root diameter; RV, root volume. Red circles represent a positive correlation between root colonization and plant growth indicators. Larger circles represent a stronger correlation.
In both the monoculture and mixture treatments of A. adenophora with R. amethystoides in BC and BC + SC treatment, the density of B. cereus showed a significantly positive correlation with biomass, total N, P and K concentrations, root growth characteristics, NO3−-N and AP contents, but a negative correlation with NH4+-N and AK contents (Figure 7). In the mixture treatment with A. adenophora in BC + SC treatment, the density of B. cereus showed a significantly negative correlation with biomass, total N and P concentration and root growth characteristics but a positive correlation with total K concentration and soil characteristics (Figure 7).
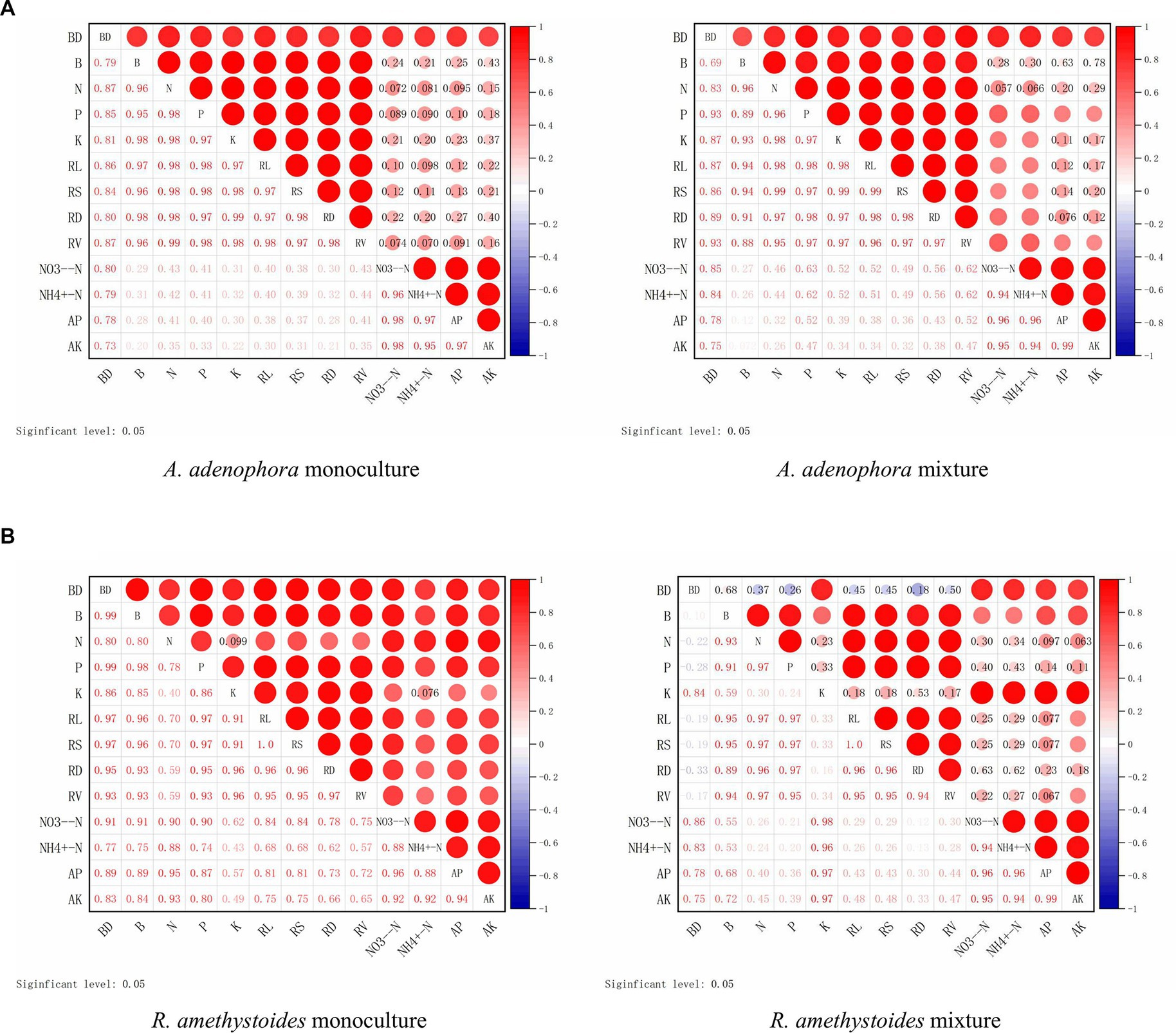
Figure 7. Correlation between the density of B. cereus (BC) and plant growth indicators of A. adenophora (A) and R. amethystoides (B). AM, AM colonization; B, biomass; RL, root length; RS, root surface area; RD, root diameter; RV; root volume. Blue circles represent a negative correlation and red circles represent a positive correlation between root colonization and plant growth indicators. Larger circles represent a stronger correlation.
4. Discussion
When invasive plants establish themselves in a new habitat, they tend to accumulate beneficial microorganisms (mainly comprising AMF and Bacillus) in their rhizosphere to promote their growth and facilitate their invasion in response to abiotic factors (i.e., nutrient deficiencies) and biotic factors (i.e., feeding by generalist insects) (Meisner et al., 2013; Fu et al., 2017; Mohanty et al., 2018). In this present study, we investigated the common AMF and Bacillus present in the rhizosphere soil of both the invasive plant (A. adenophora) and native plant (R. amethystoides) and the explored the effect of single microbes versus co-culture on the competitive growth of the two plants. We found that S. constrictum and B. cereus exerted different effects on the two plants. Compared with the control treatment, inoculation with S. constrictum enhanced A. adenophora’s competitive growth but inhibited that of R. amethystoides, while inoculation with B. cereus inhibited A. adenophora growth but facilitated that of R. amethystoides growth (Figure 2). S. constrictum and B. cereus significantly increased the AM fungal colonization rate in the root and Bacillus density in the rhizosphere of A. adenophora (Figure 5) and further improved its competitiveness (Figure 2). These results supported our hypothesis that S. constrictum and B. cereus can form a synergistic effect that further promotes A. adenophora competitiveness and invasion.
AMF is critical for the successful invasion of some exotic species (Bunn et al., 2015; Reinhart et al., 2017). AMF affects the competition of invasive species with native species by changing nutrient uptake (Zhang et al., 2017, 2018). In this study, the competitive ability of inoculated S. constrictum to A. adenophora was significantly increased, while that of R. amethystoides was significantly decreased compared with the control treatment, indicating that S. constrictum increased A. adenophora competition to R. amethystoides (Figure 2). Relative to the monoculture treatment, the N, P and K concentrations of A. adenophora were significantly increased, and those of R. amethystoides were concomitantly decreased when the two plant species were in interspecific competition (Figure 4). Our results were concordant with Shen et al. (2020), who found that AMF improved the competitive ability of A. adenophora against the native A. annua by significantly enhancing the N and P of the former. Therefore, AMFcan enhance A. adenophora’s competitiveness by providing more nutrients, which may be attributed to their different AMF colonization rates (Waller et al., 2016; Xia et al., 2021). Compared to monoculture treatment, the AMF colonization rate of R. amethystoides was significantly decreased, while the AMF colonization rate of A. adenophora was significantly increased when the two plant species were in interspecific competition. Chen et al. (2020) also found that competition reduced the AM colonization of native plants Sesbania cannabina and Eupatorium chinense by more than half when grown together with the invasive plants Bidens pilosa and Eupatorium catarium. AMF affects the competitiveness of both invasive and native species by altering their capacities for soil nutrient acquisition (Vogelsang and Bever, 2009; Pinzone et al., 2018). The correlation analysis results showed that in the mixture treatment, the increase in biomass, plant N, P, and K concentration and root growth characteristics of A. adenophora, as well as the decrease in biomass, plant N and P concentration and root growth characteristics of R. amethystoides, were significantly associated with AMF colonization rate when grown in the mixture treatment (Figure 6). These indicate that the colonization of roots by AMF can promote plant growth (Kong et al., 2022). Taken together, AMF contributes to A. adenophora out-competing native R. amethystoides through S. constrictum, which provides more nutrients to A. adenophora than to R. amethystoides with higher colonization in A. adenophora.
Bacillus is one of the rhizosphere-promoting bacteria for plants (Gupta et al., 2015; Gouda et al., 2018). It can directly release plant hormones, siderophores and ammonia, which provide soil nutrients through fixing N, and solubilizing P and K, thereby promoting plant growth (Ding et al., 2015; Khan et al., 2019). Moreover, it can also indirectly produce antimicrobial compounds to alleviate the inhibition of diverse pathogens in the plant (Alina et al., 2015; Khan et al., 2019). Our results also revealed that inoculation with B. cereus could significantly increase the nitrate nitrogen and AP contents in A. adenophora and R. amethystoides rhizosphere soil for plant growth (Table 1). Sun et al. (2021) showed that as the density of B. cereus increased, the soil available nutrient contents also increased. Roots directly affect the growth of aboveground parts and overall plant growth and development, with root growth and structure playing a key role in water and nutrient absorption (Comas et al., 2013; Ma et al., 2018). Here, B. cereus inoculation enhanced the root growth and nutrient absorption capacity of A. adenophora (Figure 3), thus increasing the N, P and K concentrations in the plants (Figure 4). A similar trend was also found in R. amethystoides. N and P have a positive synergistic effect, thus increasing the photosynthetic rate and improving the growth of plants (Schleuss et al., 2020). Our study also revealed that inoculation with B. cereus weakened the positive effect of interspecific competition on A. adenophora and alleviated the negative effect on R. amethystoides growth but did not change the competitive relationship between A. adenophora and R. amethhystoides (Figure 2). We also found that B. cereus inoculation led to significantly greater promotion on R. amethystoides biomass than A. adenophora, which may be due to the different effect of root exudates from the two plants on the growth-promoting effect of Bacillus (Sun et al., 2021, 2022). To understand the potential mechanisms, future studies may conduct a comparative analysis of the secondary metabolites of A. adenophora and R. amethystoides and their effect on Bacillus’s ability to solubilize phosphorus and fix nitrogen.
The combination of AMF and Bacillus is not only helpful to plant growth and resistance to various stresses but also benefits each other (Sangwan and Prasanna, 2021). In this present study, co-inoculation with B. cereus and S. constrictum treatments significantly increased the density of B. cereus in A. adenophora and R. amethystoides rhizosphere soil when the two species were grown in monoculture or together. Many Bacillus can be used as mycorrhizal helper bacteria (MHB), which is positively associated with root colonization and hyphal development of AMF. AMF can provide a habitat for bacteria and secrete mycelial secretions to promote the growth and development of PGPR (Zhang et al., 2018; Wang et al., 2022). Zhang et al. (2018) demonstrated that the hyphal exudates of AMF not only served as a carbon source for bacterial growth but also played a signaling role in triggering the bacteria-mediated organic phosphate mineralization process, which stimulated the expression of phosphatase genes in bacteria and released phosphatase. We revealed that inoculation with B. cereus isolated from the soil of mixture treatment of A. adenophora and R. amethystoides could increase soil nutrients in monoculture or mixture treatment, thereby increasing the N and P contents of both plants (Table 1). Several studies have shown that a suitable combination of inoculants with AMF and PGPR significantly affects plant growth compared with inoculations of the two alone (Zhang et al., 2014; Krishnamoorthy et al., 2016; Hidri et al., 2019). We found that the biomass, root growth characteristics, and nutrient contents of two plants with B. cereus and S. constrictum inoculation were significantly higher than those with single inoculation in A. adenophora or R. amethystoides monoculture. AMF mycelium recruits many bacteria, which provide functions that are absent from the AMF, thus further promoting the plant growth (Zhou et al., 2020; Jiang et al., 2021). In comparison with the monoculture, the AMF colonization rate of A. adenophora in the mixture of co-inoculation with B. cereus and S. constrictum treatments was significantly increased, while the AMF colonization rate of R. amethystoides was significantly reduced, which are consistent with the results of changes in nutrient content and growth in their respective plants. B. cereus inoculation increased the AMF colonization rate in A. adenophora rhizosphere soil in the SC + BC treatment and transported more activated nutrients for the plants. Meanwhile, the AMF colonization rate of R. amethystoides decreased when A. adenophora competed with R. amethystoides, which weakened the transport of available nutrients and inhibited the growth of R. amethystoides. Du et al. (2020) reported that AMF and Bacillus co-inoculation provided more N for F. bidentis, which enhanced the competitive advantage of F. bidentis over native E. prostrata. These results indicated that different functional microbial communities are involved in the underlying invasion mechanism, in which AMF may play a major role in the interspecific competition between invasive and native plants, and that B. cereus, as a mycorrhizal helper bacterial, promotes hyphal development and colonization of plant roots, thus helping the alien plant to compete over the native plant. Due to the few microbial species used in this study, the generality of our conclusion should be further tested by including more species. Moreover, it is necessary to continue to test the effects of AMF and Bacillus on the invasion ability of A. adenophora with various environmental factors in the future, such as non-sterile soil and different nutrient concentrations.
5. Conclusion
Our finding revealed that the symbiotic association of AMF and Bacillus with high content in the inter-rhizosphere soil of A. adenophora and R. amethystoides increased the competitive advantage of A. adenophora. However, S. constrictum and B. cereus played different roles in the invasion of A. adenophora. S. constrictum provided competitive advantages with different AM fungal colonization rates in the roots of A. adenophora and R. amethystoides, which resulted in more nutrient supply to invasive plants, while B. cereus may have activated more soil nutrients and promoted the hyphal development and colonization of plant roots. Overall, these findings enhanced our understanding on the role of AMF and bacteria in invasion by A. adenophora.
Data availability statement
The original contributions presented in the study are included in the article/Supplementary material, further inquiries can be directed to the corresponding author.
Author contributions
FG, ED, and YC designed the research. YL and RH collected the samples. ED and YHL performed the experiments. ZS and YHL performed bioinformatic and statistical analyses. ED and YC wrote the first draft. ZS and FG reviewed the manuscript. All authors contributed to the article and approved the submitted version.
Funding
This work was supported by the Yunnan Eco-Friendly Food International Cooperation Research Center (YEFICRC) Project of Yunnan Provincial of Yunnan Provincial Key Program (Grant No. 2019ZG00910), National Key Research and Development Program of China (2021YFD1400200), and Scientific Research Foundation of Education Department of Yunnan Province (2022Y224).
Conflict of interest
The authors declare that the research was conducted in the absence of any commercial or financial relationships that could be construed as a potential conflict of interest.
Publisher’s note
All claims expressed in this article are solely those of the authors and do not necessarily represent those of their affiliated organizations, or those of the publisher, the editors and the reviewers. Any product that may be evaluated in this article, or claim that may be made by its manufacturer, is not guaranteed or endorsed by the publisher.
Supplementary material
The Supplementary material for this article can be found online at: https://www.frontiersin.org/articles/10.3389/fmicb.2023.1131797/full#supplementary-material
References
Abbott, K. C., Karst, J., Biederman, L. A., Borrett, S. R., Hastings, A., Walsh, V., et al. (2015). Spatial heterogeneity in soil microbes alters outcomes of plant competition. PLoS One 10:e0125788. doi: 10.1371/journal.pone.0125788
Alina, S. O., Constantinscu, F., and Petruta, C. C. (2015). Biodiversity of Bacillus subtilis group and beneficial traits of Bacillus species useful in plant protection. Rom. Biotechnol. Lett. 20, 10737–10750.
Anna, A., Pavlína, K., and Zuzana, M. (2020). Plant–soil feedback contributes to predicting plant invasiveness of 68 alien plant species differing in invasive status. Oikos 129, 1257–1270. doi: 10.1111/oik.07186
Aslani, F., Juraimi, A. S., Ahmad-Hamdani, M. S., Alam, M. A., Hasan, M. M., Hashemi, F. S. G., et al. (2019). The role of arbuscular mycorrhizal fungi in plant invasion trajectory. Plant Soil 441, 1–14. doi: 10.1007/s11104-019-04127-5
Awaydul, A., Zhu, W. Y., Yuan, Y. G., Xiao, J., Hu, H., Chen, X., et al. (2019). Common mycorrhizal networks influence the distribution of mineral nutrients between an invasive plant, Solidago canadensis, and a native plant, Kummerowa striata. Mycorrhiza 29, 29–38. doi: 10.1007/s00572-018-0873-5
Biermann, B., and Linderman, R. G. (1981). Quantifying vesicular-arbuscular mycorrhizas: a proposed method towards standardization. New Phytol. 87, 63–67. doi: 10.1111/j.1469-8137.1981.tb01690.x
Bunn, R. A., Ramsey, P. W., and Lekberg, Y. (2015). Do native and invasive plants differ in their interactions with arbuscular mycorrhizal fungi? A meta-analysis. J ecol. 103, 1547–1556. doi: 10.1111/1365-2745.12456
Chen, X., Li, Q., Wang, Y., Chen, F. X., Zhang, X. Y., and Zhang, F. J. (2022). Bacillus promotes invasiveness of exotic Flaveria bidentis by increasing its nitrogen and phosphorus uptake. J. Plant Ecol. 15, 596–609. doi: 10.1093/jpe/rtab046
Chen, E. J., Liao, H. X., Chen, B. M., and Peng, S. L. (2020). Arbuscular mycorrhizal fungi are a double-edged sword in plant invasion controlled by phosphorus concentration. New Phytol. 226, 295–300. doi: 10.1111/nph.16359
Comas, L. H., Becker, S. R., Cruz, V. M. V., Byrne, P. F., and Dierig, D. A. (2013). Root traits contributing to plant productivity under drought. Front. Plant Sci. 4:442. doi: 10.3389/fpls.2013.00442
D’Antonio, C. M., Yelenik, S. G., and Mack, M. C. (2017). Ecosystem vs. community recovery 25 years after grass invasions and fire in a subtropical woodland. J. Ecol. 105, 1462–1474. doi: 10.1111/1365-2745.12855
Dawson, W., Schrama, M., and Austin, A. (2016). Identifying the role of soil microbes in plant invasions. J. Ecol. 104, 1211–1218. doi: 10.1111/1365-2745.12619
Dickie, I. A., Bufford, J. L., Cobb, R. C., Desprez-Loustau, M. L., Grelet, G., Hulme, P. E., et al. (2017). The emerging science of linked plant–fungal invasions. New Phytol. 215, 1314–1332. doi: 10.1111/nph.14657
Ding, X., Peng, X. J., Jin, B. S., Xiao, M., Chen, J. K., Li, B., et al. (2015). Spatial distribution of bacterial communities driven by multiple environmental factors in a beach wetland of the largest freshwater lake in China. Front. Microbiol. 6:129. doi: 10.3389/fmicb.2015.00129
Du, E. W., Chen, X., Li, Q., Chen, F. X., Xu, H. Y., and Zhang, F. J. (2020). Rhizoglomus intraradices and associated Brevibacterium frigoritolerans enhance the competitive growth of Flaveria bidentis. Plant Soil 453, 281–295. doi: 10.1007/s11104-020-04594-1
Du, E. W., Chen, Y. P., Li, Y. H., Sun, Z. X., and Gui, F. R. (2022a). Rhizospheric Bacillus-facilitated effects on the growth and competitive ability of the invasive plant Ageratina adenophora. Front. Plant Sci. 13:882255. doi: 10.3389/fpls.2022.882255
Du, E. W., Chen, Y. P., Li, Y. H., Zhang, F. J., Sun, Z. X., Hao, R. S., et al. (2022b). Effect of arbuscular mycorrhizal fungi on the responses of Ageratina adenophora to Aphis gossypii herbivory. Front. Plant Sci. 13:1015947. doi: 10.3389/fpls.2022.1015947
Fahey, C., and Stephen, L. F. (2022). Soil microbes alter competition between native and invasive plants. J. Ecol. 110, 404–414. doi: 10.1111/1365-2745.13807
Fan, X. N., Che, X. R., Lai, W. Z., Wang, S. J., Hu, W. T., Chen, H., et al. (2020). The auxin-inducible phosphate transporter AsPT5 mediates phosphate transport and is indispensable for arbuscule formation in Chinese milk vetch at moderately high phosphate supply. Environ. Microbiol. 22, 2053–2079. doi: 10.1111/1462-2920.14952
Fan, B., Wang, C., Song, X., Ding, X., Wu, L., Wu, H., et al. (2018). Bacillus velezensis FZB42 in 2018: the gram-positive model strain for plant growth promotion and biocontrol. Front. Microbiol. 9:2491. doi: 10.3389/fmicb.2018.02491
Fang, K., Wang, Y. Z., and Zhang, H. B. (2019). Different effects of plant growth-promoting bacteria on invasive plants. S. Afr. J. Bot. 124, 94–101. doi: 10.1016/j.sajb.2019.04.007
Fu, W., Wang, N., Pang, F., Huang, Y. L., Wu, J., Qi, S. S., et al. (2017). Soil microbiota and plant invasions: current and future. Biodivers. Sci. 25, 1295–1302. doi: 10.17520/biods.2017071
Gibson, D. J., Connolly, J., and Weidenhamer, H. (1999). Designs for greenhouse studies of interactions between plants. J. Ecol. 87, 1–16. doi: 10.1046/j.1365-2745.1999.00321.x
Giovannetti, M., and Mosse, B. (1980). An evaluation of techniques for measuring vesicular arbuscular mycorrhizal infection in roots. New Phytol. 84, 489–500. doi: 10.2307/2432123
Gouda, S., Kerry, R. G., Das, G., Paramithiotis, S., and Patra, J. K. (2018). Revitalization of plant growth promoting rhizobacteria for sustainable development in agriculture. Microbiol. Res. 206, 131–140. doi: 10.1016/j.micres.2017.08.016
Gu, C. J., Tu, Y. L., Liu, L. S., Wei, B., Zhang, Y. L., Yu, H. B., et al. (2021). Predicting the potential global distribution of Ageratina adenophora under current and future climate change scenarios. Ecol. Evol. 11, 12092–12113. doi: 10.1002/ece3.7974
Gui, F. R., Wan, F. H., and Guo, J. Y. (2009). Determination of the population genetic structure of the invasive weed Ageratina adenophora using ISSR-PCR markers. Russ. J. Plant Physiol. 56, 410–416. doi: 10.1134/S1021443709030157
Gupta, G., Parihar, S. S., Ahirwar, N. K., Snehim, S. K., and Singh, V. (2015). Plant growth promoting rhizobacteria (PGPR): current and future prospects for development of sustainable agriculture. J. Microb. Biochem. Technol. 7, 96–102. doi: 10.4172/1948-5948.1000188
Hidri, R., Mahmoud, O. M., Farhat, N., Cordero, I., Pueyo, J. J., Debez, A., et al. (2019). Arbuscular mycorrhizal fungus and rhizobacteria affect the physiology and performance of Sulla coronaria plants subjected to salt stress by mitigation of ionic imbalance. J. Plant Nutr. Soil Sci. 182, 451–462. doi: 10.1002/jpln.201800262
Isaac, R. A., and Johnson, W. C. (1983). High speed analysis of agricultural samples using inductively coupled plasma-atomic emission spectroscopy. Spectrochim. Acta B 38, 277–282. doi: 10.1016/0584-8547(83)80124-4
Jiang, F. Y., Zhang, L., Zhou, J. C., George, T., and Feng, G. (2021). Arbuscular mycorrhizal fungi enhance mineralization of organic P by carrying bacteria along their extraradical hyphae. New Phytol. 230, 304–315. doi: 10.1111/nph.17081
Khan, N., Bano, A., Rahman, M. A., Guo, J., Kang, Z. Y., and Babar, M. A. (2019). Comparative physiological and metabolic analysis reveals a complex mechanism involved in drought tolerance in chickpea (Cicer arietinum L.) induced by PGPR and PGRs. Sci. Rep. 9:2019. doi: 10.1038/s41598-019-38702-8
Kong, L. J., Chen, X., Yerger, E. H., Li, Q., Chen, F. X., Xu, H. Y., et al. (2022). Arbuscular mycorrhizal fungi enhance the growth of the exotic species Ambrosia artemisiifolia. J. Plant Ecol. 15, 581–595. doi: 10.1093/jpe/rtab087
Krishnamoorthy, R., Kim, K., Subramanian, P., Senthilkumar, M., and Anandham, R. (2016). Arbuscular mycorrhizal fungi and associated bacteria isolated from salt-affected soil enhances the tolerance of maize to salinity in coastal reclamation soil. Agric. Ecosyst. Environ. 231, 233–239. doi: 10.1016/j.agee.2016.05.037
Kurniawan, A., and Chuang, H. W. (2022). Rhizobacterial Bacillus mycoides functions in stimulating the antioxidant defence system and multiple phytohormone signalling pathways to regulate plant growth and stress tolerance. J. Appl. Microbiol. 132, 1260–1274. doi: 10.1111/jam.15252
Lekberg, Y., Gibbons, S. M., Rosendahl, S., and Ramsey, P. W. (2013). Severe plant invasions can increase mycorrhizal fungal abundance and diversity. ISME J. 7, 1424–1433. doi: 10.1038/ismej.2013.41
Li, L. Q., Zhang, M. S., Liang, Z. P., Xiao, B., Wan, F. H., and Liu, W. X. (2016). Arbuscular mycorrhizal fungi enhance invasive plant, Ageratina adenophora growth and competition wih native plants. Chin. J, Ecol. 35, 79–86. doi: 10.13292/j.1000-4890.201601.011
Li, Q., Wan, F., and Zhao, M. (2022). Distinct soil microbial communities under Ageratina adenophora invasions. Plant Biol. 24, 430–439. doi: 10.1111/plb.13387
Li, W. T., Zheng, Y. L., Wang, R. F., Wang, Z. Y., Liu, Y. M., Shi, X., et al. (2022). Shifts in chemical and microbiological properties belowground of invader Ageratina adenophora along an altitudinal gradient. J. Plant Ecol. 15, 561–570. doi: 10.1093/jpe/rtac003
Ma, Z. Q., Guo, D. L., Xu, X. I., Lu, M., Bardgett, R. D., Bardgett, R. D., et al. (2018). Evolutionary history resolves global organization of root functional traits. Nat. Int. Weekly J. Sci. 570:E25. doi: 10.1038/s41586-019-1214-3
Meisner, A., De Deyn, G. B., De Boer, W., and Van Der Putten, W. H. (2013). Soil biotic legacy effects of extreme weather events influence plant invasiveness. Proc. Natl. Acad. Sci. U. S. A. 110, 9835–9838. doi: 10.1073/pnas.1300922110
Mohanty, S. K., Arthikala, M. K., Nanjareddy, K., and Lara, M. (2018). Plant-symbiont interactions: the functional role of expansions. Symbiosis 74, 1–10. doi: 10.1007/s13199-017-0501-8
Nelson, D. W., and Sommers, L. E. (1973). Determination of Total nitrogen in plant material. Agron. J. 65, 109–112. doi: 10.2134/agronj1973.00021962006500010033x
Niu, H. B., Liu, W. X., Wan, F. H., and Liu, B. (2007). An invasive aster (Ageratina adenophora) invades and dominates forest understories in China: altered soil microbial communities facilitate the invader and inhibit natives Plant Soil. 294, 73–85. doi: 10.1007/s11104-007-9230-8
Oksanen, L., Sammul, M., and Magi, M. (2006). On the indices of plant-plant competition and their pitfalls. Oikos 112, 149–155. doi: 10.1111/j.0030-1299.2006.13379.x
Payne, S. M. (1994). Detection, isolation, and characterization of siderophores. Methods Enzymol. 235, 329–344. doi: 10.1016/0076-6879(94)35151-1
Pinzone, P., Potts, D., Pettibone, G., and Warren, R. (2018). Do novel weapons that degrade mycorrhizal mutualisms promote species invasion? Plant Ecol. 219, 539–548. doi: 10.1007/s11258-018-0816-4
Poudel, A. S., Jha, P. K., Shrestha, B. B., Muniappan, R., and Novak, S. (2019). Biology and management of the invasive weed Ageratina adenophora (Asteraceae): current state of knowledge and future research needs. Weed Res. 59, 79–92. doi: 10.1111/wre.12351
Powell, K. I., Chase, J. M., and Knight, T. M. (2013). Invasive plants have scale-dependent effects on diversity by altering species-area relationships. Science 339, 316–318. doi: 10.1126/science.1226817
Qi, S. S., Wang, J. H., Wan, L. Y., Dai, Z. C., Da Silva Matos, D. M., Du, D. L., et al. (2022). Arbuscular mycorrhizal fungi contribute to phosphorous uptake and allocation strategies of Solidago canadensis in a phosphorous-deficient environment. Front. Plant Sci. 13:831654. doi: 10.3389/fpls.2022.831654
Reinhart, K. O., Lekberg, Y., Klironomos, J., and Maherali, H. (2017). Does responsiveness to arbuscular mycorrhizal fungi depend on plant invasive status? Ecol. Evol. 7, 6482–6492. doi: 10.1002/ece3.3226
Ren, Z. H., Okyere, S. K., Wen, J., Xie, L., Cui, Y. J., Wang, S., et al. (2021). An overview: the toxicity of Ageratina adenophora on animals and its possible interventions. Int. J. Mol. Sci. 22:11581. doi: 10.3390/ijms222111581
Richardson, D. M., and Pyšek, P. (2012). Naturalization of introduced plants: ecological drivers of biogeographical patterns. New Phytol. 196, 383–396. doi: 10.1111/j.1469-8137.2012.04292.x
Sangwan, S., and Prasanna, R. (2021). Mycorrhizae helper bacteria: unlocking their potential as bioenhancers of plant - arbuscular mycorrhizal fungal associations. Microb. Ecol. 84, 1–10. doi: 10.1007/s00248-021-01831-7
Saxena, A. K., Kumar, M., Chakdar, H., Anuroopa, N., and Bagyaraj, D. J. (2020). Bacillus species in soil as a natural resource for plant health and nutrition. J. Appl. Microbiol. 128, 1583–1594. doi: 10.1111/jam.14506
Schleuss, P. M., Widdig, M., Heintz-Buschart, A., Kirkman, K., and Spohn, M. (2020). Interactions of nitrogen and phosphorus cycling promote P acquisition and explain synergistic plant-growth responses. Ecology 101:e03003. doi: 10.1002/ecy.3003
Shen, K., Cornelissen, J. H. C., Wang, Y., Wu, C., He, Y., Ou, J., et al. (2020). AM fungi alleviate phosphorus limitation and enhance nutrient competitiveness of invasive plants via mycorrhizal networks in karst areas. Front. Ecol. Evol. 8:125. doi: 10.3389/fevo.2020.00125
Smith, S. E., and Smith, F. A. (2011). Roles of arbuscular mycorrhizas in plant nutrition and growth: new paradigms from cellular to ecosystem scales. Annu. Rev. Plant Biol. 62, 227–250. doi: 10.1146/annurev-arplant-042110-103846
Sol, D., Maspons, J., Vall-llosera, M., Bartomeus, I., Garcia-Pea, G. E., Piol, J., et al. (2012). Unraveling the life history of successful invaders. Science 337, 580–583. doi: 10.1126/science.1221523
Song, X. Y., Hogan, J. A., Brown, C., Cao, M., and Yang, J. (2017). Snow damage to the canopy facilitates alien weed invasion in a subtropical montane primary forest in South-Western China. For. Ecol. Manag. 391, 275–281. doi: 10.1016/j.foreco.2017.02.031
Stefany, C., Claudia, P., Giuliana, D., Fabrizio, D. P., Alessio, C., Marco, M., et al. (2021). Plant growth promotion function of Bacillus sp. strains isolated from salt-Pan rhizosphere and their biocontrol potential against Macrophomina phaseolina. Int. J. Mol. Sci. 22:3324. doi: 10.3390/ijms22073324
Sun, C. F., Li, Q., Han, L. L., Chen, X., and Zhang, F. J. (2022). The effects of allelochemicals from root exudates of Flaveria bidentis on two Bacillus species. Front. Plant Sci. 13:1001208. doi: 10.3389/fpls.2022.1001208
Sun, Y. Y., Zhang, Q. X., Zhao, Y. P., Diao, Y. H., Gui, F. R., and Yang, G. Q. (2021). Beneficial rhizobacterium provides positive plant–soil feedback effects to Ageratina adenophora. J. Integr. Agric. 20, 1327–1335. doi: 10.1016/S2095-3119(20)63234-8
Tang, S. C., Pan, Y. M., Wei, C. Q., Li, X. Q., and Lü, S. H. (2019). Testing of an integrated regime for effective and sustainable control of invasive crofton weed (Ageratina adenophora) comprising the use of natural inhibitor species, activated charcoal, and fungicide. Weed Biol. Manage. 19, 9–18. doi: 10.1111/wbm.12171
Vogelsang, K. M., and Bever, J. D. (2009). Mycorrhizal densities decline in association with nonnative plants and contribute to plant invasion. Ecology 90, 399–407. doi: 10.1890/07-2144.1
Waller, L. P., Callaway, R. M., Klironomos, J. N., Ortega, Y. K., Maron, J. L., and Shefferson, R. (2016). Reduced mycorrhizal responsiveness leads to increased competitive tolerance in an invasive exotic plant. J. Ecol. 104, 1599–1607. doi: 10.1111/1365-2745.12641
Wang, S. S., Chen, A. Q., Xie, K., Yang, X. F., Luo, Z. Z., Chen, J. D., et al. (2020). Functional analysis of the OsNPF4.5 nitrate transporter reveals a conserved mycorrhizal pathway of nitrogen acquisition in plants. Proc. Natl. Acad. Sci. U. S. A. 117, 16649–16659. doi: 10.1073/pnas.2000926117
Wang, Y. H., Hou, L. L., Wu, X. Q., Zhu, M. L., Dai, Y., and Zhao, Y. J. (2022). Mycorrhiza helper bacterium Bacillus pumilus HR10 improves growth and nutritional status of Pinus thunbergii by promoting mycorrhizal proliferation. Tree Physiol. 42, 907–918. doi: 10.1093/treephys/tpab139
Wang, C., Lin, H. L., Feng, Q. S., Jin, C. Y., Cao, A. C., and He, L. (2017). A new strategy for the prevention and control of Eupatorium adenophorum under climate change in China. Sustainability 9:11. doi: 10.3390/su9112037
Wang, R., and Wang, Y. Z. (2006). Invasion dynamics and potential spread of the invasive alien plant species Ageratina adenophora (Asteraceae) in China. Divers. Distrib. 12, 397–408. doi: 10.1111/j.1366-9516.2006.00250.x
Weremijewicz, J., Sternberg, L. D. S. L. O., and Janos, D. P. (2018). Arbuscular common mycorrhizal networks mediate intra-and interspecific interactions of two prairie grasses. Mycorrhiza 28, 71–83. doi: 10.1007/s00572-017-0801-0
Xia, Y., Dong, M. H., Yu, L., Kong, L. D., Seviour, R., and Kong, Y. H. (2021). Compositional and functional profiling of the rhizosphere microbiomes of the invasive weed Ageratina adenophora and native plants. PeerJ 9:e10844. doi: 10.7717/peerj.10844
Xie, X. N., Lai, W. Z., Che, X. R., Wang, S. J., Ren, Y., Hu, W. T., et al. (2022). A SPX domain-containing phosphate transporter from Rhizophagus irregularis handles phosphate homeostasis at symbiotic interface of arbuscular mycorrhizas. New Phytol. 234, 650–671. doi: 10.1111/nph.17973
Xu, C. W., Yang, M. Z., Chen, Y. J., Chen, L. M., Zhang, D. Z., Mei, L., et al. (2012). Changes in non-symbiotic nitrogen-fixing bacteria inhabiting rhizosphere soils of an invasive plant Ageratina adenophora. Appl. Soil Ecol. 54, 32–38. doi: 10.1016/j.apsoil.2011.10.021
Yang, X., Zhang, L. H., Zhang, C., Zhang, J. L., Han, J. M., and Dong, J. G. (2012). Effects of Flaveria bidentis invasion on soil microbial communities, enzyme activities and nutrients. Plant Nutr. Fert. Sci. 18, 907–914. doi: 10.11674/zwy.2012.11499
Yu, W. Q., Liu, W. X., Gui, F. R., Liu, W. Z., Wan, F. H., and Zhang, L. L. (2012). Invasion of exotic Ageratina adenophora alters soil physical and chemical characteristics and arbuscular mycorrhizal fungus community. Acta Ecol. Sin. 32, 7027–7035. doi: 10.5846/stxb201110091468
Zhang, L., Fan, J. Q., Ding, X. D., He, X. H., Zhang, F. S., and Feng, G. (2014). Hyphosphere interactions between an arbuscular mycorrhizal fungus and a phosphate solubilizing bacterium promote phytate mineralization in soil. Soil Biol. Biochem. 74, 177–183. doi: 10.1016/j.soilbio.2014.03.004
Zhang, L., Feng, G., and Declerck, S. (2018). Signal beyond nutrient, fructose, exuded by an arbuscular mycorrhizal fungus triggers phytate mineralization by a phosphate solubilizing bacterium. ISME J. 12, 2339–2351. doi: 10.1038/s41396-018-0171-4
Zhang, H. Y., Goncalves, P., Copeland, E., Qi, S. S., Dai, Z. C., Li, G. L., et al. (2020). Invasion by the weed Conyza canadensis alters soil nutrient supply and shifts microbiota structure. Soil Biol. Biochem. 143:107739. doi: 10.1016/j.soilbio.2020.107739
Zhang, F. J., Li, Q., Chen, F. X., Xu, H. Y., Inderjit,, and Wan, F. H. (2017). Arbuscular mycorrhizal fungi facilitate growth and competitive ability of an exotic species Flaveria bidentis. Soil Biol. Biochem. 115, 275–284. doi: 10.1016/j.soilbio.2017.08.019
Zhang, F. J., Li, Q., Yerger, E. H., Chen, X., Shi, Q., and Wan, F. H. (2018). AM fungi facilitate the competitive growth of two invasive plant species, Ambrosia artemisiifolia and Bidens pilosa. Mycorrhiza 28, 703–715. doi: 10.1007/s00572-018-0866-4
Zhao, L., Meng, L., and Li, B. (2007). Effects of fertilization on the relative competitive ability of Eupatorium adenophorum and Lolium perenne at their seedling stage. Chin. J. Ecol. 26, 1743–1747. doi: 10.13292/j.1000-4890.2007
Keywords: Ageratina adenophora , arbuscular mycorrhizal fungi, Bacillus , synergistic inoculation, competitive advantage
Citation: Du E, Chen Y, Li Y, Li Y, Sun Z, Hao R and Gui F (2023) Effects of Septoglomus constrictum and Bacillus cereus on the competitive growth of Ageratina adenophora. Front. Microbiol. 14:1131797. doi: 10.3389/fmicb.2023.1131797
Edited by:
Kai Sun, Nanjing Normal University, ChinaReviewed by:
Xin Qian, Fujian Agriculture and Forestry University, ChinaXianan Xie, South China Agricultural University, China
Jesús Muñoz-Rojas, Meritorious Autonomous University of Puebla, Mexico
Copyright © 2023 Du, Chen, Li, Li, Sun, Hao and Gui. This is an open-access article distributed under the terms of the Creative Commons Attribution License (CC BY). The use, distribution or reproduction in other forums is permitted, provided the original author(s) and the copyright owner(s) are credited and that the original publication in this journal is cited, in accordance with accepted academic practice. No use, distribution or reproduction is permitted which does not comply with these terms.
*Correspondence: Furong Gui, furonggui18@sina.com
†These authors share first authorship