- 1Key Laboratory of Military Stomatology & National Clinical Research Center for Oral Diseases & Shaanxi Key Laboratory of Stomatology, Department of Pediatric Dentistry, School of Stomatology, The Fourth Military Medical University, Xi’an, China
- 2Department of Orthodontics, The Affiliated Hospital of Qingdao University, Qingdao, China
- 3School of Stomatology, Qingdao University, Qingdao, China
- 4Department of Stomatology, General Hospital of Tibet Military Region, Chinese People’s Liberation Army, Lhasa, Tibet, China
- 5Department of Oral Prevention, College of Stomatology, Xi’an Jiaotong University, Xi’an, China
Introduction: Streptococcus mutans is the most prevalent biofilm-forming pathogen in dental caries, while Candida albicans is often detected in the presence of S. mutans.
Methods: We aimed to evaluate the anti-caries effect of stevioside in medium trypticase soy broth (TSB) with or without sucrose supplementation compared with the same sweetness sucrose and xylitol in a dual-species model of S. mutans and C. albicans, based on planktonic growth, crystal violet assay, acid production, biofilm structural imaging, confocal laser scanning microscopy, and RNA sequencing.
Results: Our results showed that compared with sucrose, stevioside significantly inhibited planktonic growth and acid production, changed the structure of the mixed biofilm, and reduced the viability of biofilm and the production of extracellular polysaccharides in dual-species biofilm. Through RNA-seq, Kyoto Encyclopedia of Genes and Genomes (KEGG) pathway impact analysis showed that stevioside decreased sucrose metabolism and increased galactose and intracellular polysaccharide metabolism in S. mutans, and decreased genes related to GPI-modified proteins and secreted aspartyl proteinase (SAP) family in C. albicans. In contrast to xylitol, stevioside also inhibited the transformation of fungal morphology of C. albicans, which did not form mycelia and thus had reduced pathogenicity. Stevioside revealed a superior suppression of dual-species biofilm formation compared to sucrose and a similar anti-caries effect with xylitol. However, sucrose supplementation diminished the suppression of stevioside on S. mutans and C. albicans.
Conclusions: Our study is the first to confirm that stevioside has anticariogenic effects on S. mutans and C. albicans in a dual-species biofilm. As a substitute for sucrose, it may help reduce the risk of developing dental caries.
1. Introduction
Dental caries is one of the most prevalent oral diseases worldwide. It develops when microbial biofilms are formed on the tooth surface due to the interaction between host factors and fermentable carbohydrates over an extended period(Takahashi and Nyvad, 2011; Pitts et al., 2017). Many microorganisms are found on dental plaque, while Streptococcus mutans and Candida albicans are considered the most vital factors of cariogenic infections leading to pathogenic biofilm formation (Falsetta et al., 2014; Sztajer et al., 2014; Ellepola et al., 2017; Xiao et al., 2018b). Many researchers have identified S. mutans as a major bacterial pathogen in dental caries (Takahashi and Nyvad, 2011; Cai et al., 2018). It has the unique ability to ferment dietary sugars, especially sucrose, and generate organic acids as a byproduct that demineralizes tooth surfaces, producing extracellular polysaccharides (EPS) that promote cariogenic biofilm formation act against host defense (Klein et al., 2009; Chan et al., 2020). The interaction between oral bacteria and fungi in caries development has been observed in many studies (Peleg et al., 2010; Azzam et al., 2020; Cui et al., 2021; Tu et al., 2022). Candida albicans has been frequently found when there are high levels of S. mutans in caries, particularly early-childhood caries (ECC; Raja et al., 2010; Xiao et al., 2018a,b; Cui et al., 2021). Experiments have demonstrated a synergistic effect between S. mutans and C. albicans in vitro and in vivo (Harriott and Noverr, 2011; Metwalli et al., 2013; Falsetta et al., 2014; Cui et al., 2021; Garcia et al., 2021; Tu et al., 2022). The presence of C. albicans facilitates S. mutans biofilm formation and accumulation, whereas S. mutans enhances hyphal growth of C. albicans in the presence of sucrose, mediated through sugar-based synergistic interactions, notably between glucosyltransferase B (gtfB) and other factors (Hwang et al., 2015, 2017; Chan et al., 2020).
Sugar substitutes, such as xylitol, erythritol, mannitol, aspartame, and saccharin, have been used for caries prevention (Ferrazzano et al., 2015; Zhu et al., 2021). However, studies in animal models have demonstrated a link between artificial sweeteners and weight gain, cancer, and other health hazards (Gupta et al., 2013; Ferrazzano et al., 2015). Stevioside, the main component of Stevia rebaudiana, is considered one of the best sugar substitutes, being approximately 300 times sweeter than equally weighted sucrose but with lower calories and no notable side effects (Lemus-Mondaca et al., 2012; Lohner et al., 2017). Research results have indicated that stevioside may inhibit growth, plaque formation, and acid synthesis in oral microorganisms (Kishta Derani et al., 2016; Escobar et al., 2020; Ganter et al., 2020).
However, these previous studies concluding that stevioside prevents dental caries have mainly been conducted on a single-species biofilm model, mostly of S. mutans. Interactions between different strains may affect the ultimate anti-carious effect. As previously mentioned, S. mutans–C. albicans associations enhance infection and hypervirulent biofilm formation of S. mutans on tooth surfaces (Falsetta et al., 2014; Kim et al., 2020). Previous studies have not explored the possible effects of stevioside on C. albicans nor in dual-species models. At the same time, most studies have not considered the presence of sugars in the diet, especially sucrose-rich diets, and whether this affects the effects of stevioside.
Therefore, applying a more complex biological membrane model is necessary for further exploring whether stevioside can be effectively used to prevent dental caries. In this study, we aimed to examine the preventive effects of stevioside using S. mutans–C. albicans dual-species biofilm as an in vitro model. In addition, sucrose was included to assess whether it disrupted the preventive effects of stevioside. Finally, due to its higher sweetness, we also discussed the effect of stevioside in comparison with the amount of sucrose corresponding to the same level of sweetness. Thus, the present study investigated the antimicrobial potential of stevioside against the planktonic growth, biofilm formation, acid and EPS production, and other virulence factors attributes of dual species of C. albicans and S. mutans for the application of stevioside in the prevention of caries and other oral diseases.
2. Materials and methods
2.1. Microbial strains and culture conditions
Streptococcus mutans UA159 and Candida albicans SC5314 were purchased from the American Type Culture Collection (ATCC, Manassas, VA, United States). Streptococcus mutans was grown under anaerobic conditions in brain heart infusion (Haibo Biotechnology, Qingdao, China) agar at 37°C. C. albicans was grown under aerobic conditions in Sabouraud dextrose agar (Haibo Biotechnology, Qingdao, China) at 37°C. Single colonies of bacteria and fungi grown on agar plates were cultivated in trypticase soy broth (TSB) (Haibo Biotechnology, Qingdao, China) under microaerobic conditions (8–9% O2, 5% CO2) at 37°C for use in subsequent experiments.
2.2. Preparation of different growth media
The dual-species biofilm was grown in six different media, as shown in Table 1. TSB medium was used as a negative control, and other groups were prepared to a concentration corresponding to the same sweetness as 1% sucrose, using information reported by the Food and Drug Administration (Table 1).
2.3. Planktonic growth of Streptococcus mutans and Candida albicans
Overnight suspensions of S. mutans and C. albicans were standardized to approximately 106 CFU/mL in 5 mL of the corresponding growth medium. The planktonic dual-species growth was grown under microaerobic conditions at 37°C in a rotatory shaker at 180 rpm. Every 2 h until the end of the 24 h culture, 100 μL of cultures from each tube were transferred to a 96-well plate, and the optical density (OD) was measured at 600 nm using a microplate reader (Berten Instruments, Shanghai, China) until the end of the 24 h culture. The experiment was repeated three times with measurements performed on triplicate samples.
2.4. Crystal violet assay
We assessed dual-species biofilm formation using crystal violet (CV) staining assay. The initial concentration of S. mutans and C. albicans overnight suspensions were adjusted to approximately 105 CFU/mL. For dual-species biofilm formation, 200 μL of each strain inoculum was deposited into a 24-well microplate plate containing 1,600 μl of a particular growth medium. After cultivation for 12, 24, and 48 h, the supernatant medium was removed, and the underlying biofilm was washed three times with phosphate-buffered saline (PBS) (Sangon Biotech, Shanghai. China; Guo et al., 2021). Then, the biofilms were fixed in methanol for 15 min and stained with 0.1% (w/v) CV for 10 min. Wells were washed with sterile water and dried for 30 min at 37°C. Images were captured using a light microscope (Olympus SC180, Tokyo, Japan). The biofilms were then treated with 600 μl 33% glacial acetic acid with shaking (80 r/min) at 37°C for 30 min. Then 100 μl of the solution was transferred to a 96-well plate and measured at OD575 nm. The experiment was repeated three times with triplicate samples.
2.5. Acid production
Overnight cultures of S. mutans and C. albicans were standardized to approximately 104 CFU/mL and deposited into a 24-well microplate plate containing various growth media. The culture medium was renewed every 24 h (Guan et al., 2020). The culture supernatants were centrifuged at 5000 × g for 15 min, and the precipitation of bacterial/fungal cells was removed. Then, the pH values of the supernatant after centrifugation were measured using a precise pH meter (Mettler-Toledo, Columbus, OH, United States) at 24, 48, 72, 96, and 120 h after inoculation.
2.6. Scanning electron microscopy
We examined the structure and distribution of dual-species biofilm using scanning electron microscopy (SEM) (Hitachi S-4800, Tokyo, Japan). According to the protocol described above, the biofilms were grown on 14 mm diameter round basic slides (Solarbio Science & Technology, Beijing, China) in 24-well microtiter plates (Krzyściak et al., 2017). Streptococcus mutans and Candida albicans suspensions were inoculated at 104 CFU/mL at a ratio of 1:1 (v/v) in wells each containing a different medium. After 24 h of microaerobic incubation, the biofilms were washed with sterile PBS and fixed with 2.5% glutaraldehyde for 12 h at 4°C in the dark. They were then washed with PBS three times and dehydrated in series of ethanol solutions of increasing concentration (30, 50, 70, 80, 90, and 95% v/v) for 10 min each and dehydrated twice in anhydrous ethanol (Guan et al., 2020). Finally, the samples were dried in hexamethyldisilazane for 30 min and placed in a fume cupboard for 1 day. The samples were adhered to the stage using conductive glue and dusted with gold in a vacuum (Guan et al., 2020). SEM observed the specimens at 2,000× and 5,000× magnifications. Each specimen was observed in three randomly selected areas.
2.7. Confocal laser scanning microscopy
2.7.1. LIVE/DEAD bacteria/fungi staining
The live or dead bacteria and fungi were detected using the LIVE/DEAD BacLight Bacterial Viability Kit (Thermo Fisher, Eugene Oregon, United States), as previously described (Xie et al., 2020; Zhu et al., 2021). Dual-species biofilms were grown using 1:1 (v/v) of each species at approximately 104 CFU/mL on round slides under the same experimental conditions described in CV staining assay. Live bacteria or fungi with intact cell membranes were labeled with SYTO 9, exhibiting green fluorescence (excitation/emission 480/500 nm), whereas dead bacteria or fungi with damaged membranes were stained fluorescent red by propidium iodide (PI) (excitation/emission 490/635 nm; Chan et al., 2020; Xie et al., 2020; Zhu et al., 2021). A 1:1 mixture of SYTO 9 and PI of 2 μM concentration stain solution was added to each well and incubated in the dark for 15 min at 4°C according to the kit manufacturer’s protocol. The imaging was performed using a confocal laser scanning microscope (Olympus FV1000, Tokyo, Japan) equipped with a 40× objective lens; the viability inhibition was quantified using ImageJ software (National Institute of Health, Bethesda, MD, United States).
2.7.2. Bacteria/yeast/extracellular polysaccharide staining
Streptococcus mutans and Candida albicans dual-species biofilms, prepared from 104 CFU/mL suspensions of each, were grown on round slides in sterile 24-well plates under similar conditions in above. As described previously, 1 μM of Alexa Fluor 647-labeled dextran conjugate (10,000 molecular weight, Thermo Fisher, Eugene Oregon, USA) was added to the medium at the beginning of incubation (Zhu et al., 2021). The labeled dextran functions as a primer for the synthesis of glucosyltransferases (Gtfs) and can be simultaneously incorporated during the synthesis of extracellular polysaccharides (EPS) in biofilm formation (Venkitaraman et al., 1995). After 24 h of incubation, the biofilms were washed with sterile PBS; S. mutans was stained by SYTO 9 (excitation/emission 480/500 nm), whereas C. albicans was stained with Fluorescent Brightener 28 (excitation/emission 365/435 nm) (Jinming Biotechnology, Beijing, China) for 15 min at 4°C in the dark (Chan et al., 2020). The glucan was stained with Alexa Fluor 647 (excitation/emission 647/668 nm) for the initial deposit. The biofilm was imaged using a NikonA1plus confocal microscope with a 40× objective lens.
2.8. RNA extraction and sequencing
The initial concentration of the overnight S. mutans and C. albicans suspension were adjusted to approximately 2 × 106 and 2 × 104 CFU/mL in a 24-well microplate under microaerobic conditions. The dual-species biofilm was grown at microaerobic conditions in four different media (Table 1; Group 1 & 2 & 3 & 4). The culture medium was renewed after 18 h and then changed at 24, 48, and 60 h until the end period (72 h; He et al., 2017). Then, the planktonic media were discarded, and the biofilms were washed with sterile PBS. The biofilms were isolated with RNAex Pro Reagent (Accurate Biotechnology, China) at 4°C, and total RNA was extracted using a SteadyPureUniversal RNA Extraction Kit (Accurate Biotechnology, China). The concentration and purity of total RNA were spectrophotometrically determined using a Genova Nano instrument (JENWAY, Staffordshire, United Kingdom). RNA integrity and genomic contamination were detected via agarose gel electrophoresis. The Ribo-off rRNA Depletion Kit (Vazyme Biotech, China) was used for rRNA removal, and the VAHTS™ Stranded mRNA-seq V2 Library Prep Kit for Illumina® (Vazyme Biotech, China) was used for library construction. The library was validated through 8% PAGE electrophoresis and then was performed using Illumina Hiseq™ (Illumina, Inc., San Diego, CA, United States) to obtain Sequenced Reads. Read mapping to the reference genome was performed using Bowtie2 for genomic localization analysis, and the results were statistically compared using the Python script RSeQC (Langmead and Salzberg, 2012; Wang et al., 2012). The reads were mapped to the S. mutans UA159 and C. albicans SC5314 genomes. The R package DESeq2 was used to analyze differentially expressed genes (DEGs) among sample groups of each species (Love et al., 2014). The cutoff value for designating a gene as being significantly differentially expressed was a change in transcript levels of at least a log2 fold change of 1 (|log2FoldChange| ≥ 1) (a two-fold difference) and a Benjamini–Hochberg adjusted p value (FDR) of less than 0.05 (q value ≤0.05). The R package TopGO was used for GO enrichment, clusterProfiler was used for KEGG functional enrichment analysis, and the function was considered significantly enriched when the q value <0.05 (Alexa et al., 2006). Each group was analyzed in triplicate.
2.9. Statistics
All experiments were repeated at least three times. Parametric data of the group mean values were compared by one-way ANOVA and Tukey’s post hoc test, and non-parametric data was analyzed using Kruskal-Wallis with a post hoc Dunn’s test using IBM SPSS 26.0 software (SPSS Inc., Chicago, IL, United States). The graphing of the data was performed using GraphPad Prism 8 (GraphPad Software, San Diego, CA, United States). A 5% significance level was used for all tests.
3. Results
3.1. Stevioside inhibited the growth of Streptococcus mutans and Candida albicans in planktonic cultures
In the experimental groups, 1% stevioside (1% ste) or stevioside (ste) of the same sweetness as 1% sucrose were added, with constant shaking to maintain the planktonic conditions. The 1% ste or ste group significantly inhibited affected planktonic growth compared with the sucrose groups after 10 h of culture (p < 0.001), in which planktonic growth was not affected compared with the xylitol and TSB groups (p > 0.05; Figure 1). The sucrose-added groups displayed significant increase in dual-species planktonic growth compared with other groups after 12 h of culture (p < 0.001; Figure 1).
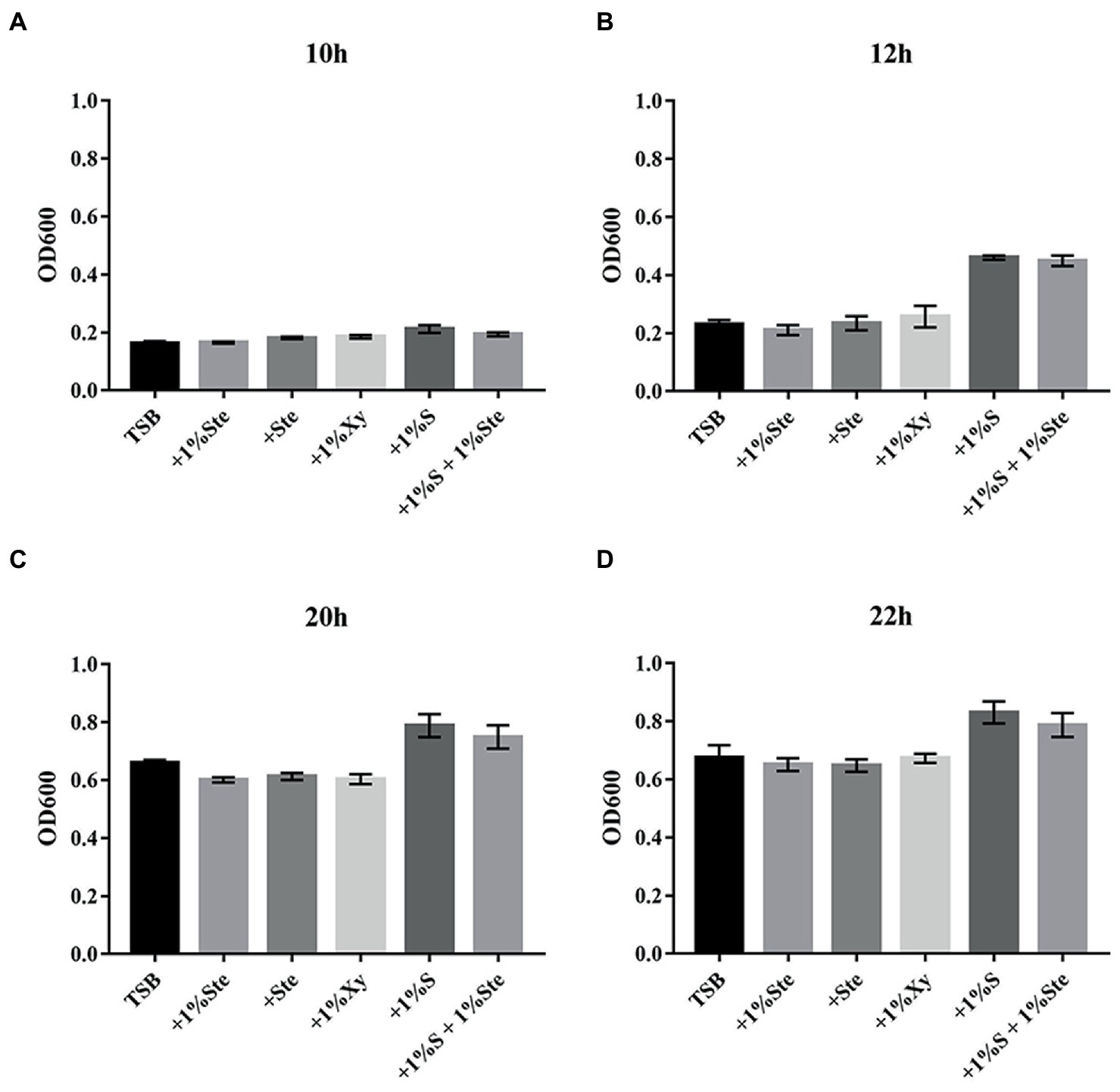
Figure 1. The effects of stevioside treatment on dual species growth of Streptococcus mutans and Candida albicans in planktonic cultures. (A–D) The dual-species planktonic growth at 10, 12, 20, and 22 h was represented by the mean OD 600 nm ± SD for different media. After extending the incubation time, the mean OD values rose significantly, especially in sucrose-added groups. +1% Ste., TSB medium supplemented with 1% stevioside; +Ste., TSB medium supplemented with stevioside of the same sweetness as 1% sucrose; +1%Xy, TSB medium supplemented with 1% xylitol; +1% S, TSB medium supplemented with 1% sucrose; +1% S + 1% Ste., TSB medium supplemented with 1% sucrose and 1% stevioside.
3.2. Stevioside suppressed dual-species biofilm formation, and sucrose supplementation abolished the inhibitory effects of stevioside
There was no significant difference in CV-stained biofilms formation between groups after 12 h of culture, yet the microscopy images showed differences in overall biofilm architecture. After 24 and 48 h of incubation, dual-species biofilm containing stevioside (1% ste or ste) exhibited a significantly lower read at OD575 nm than 1% sucrose and 1% xylitol (p < 0.001; Figure 2). We also observed that compared with 1% stevioside only, 1% sucrose +1% stevioside resulted in significantly increased biofilm growth (p < 0.001), showing that inclusion of stevioside did not inhibit the biofilm from using sucrose for growth, thus abolishing the inhibitory effects of stevioside in dual-species biofilm formation (Figure 2).
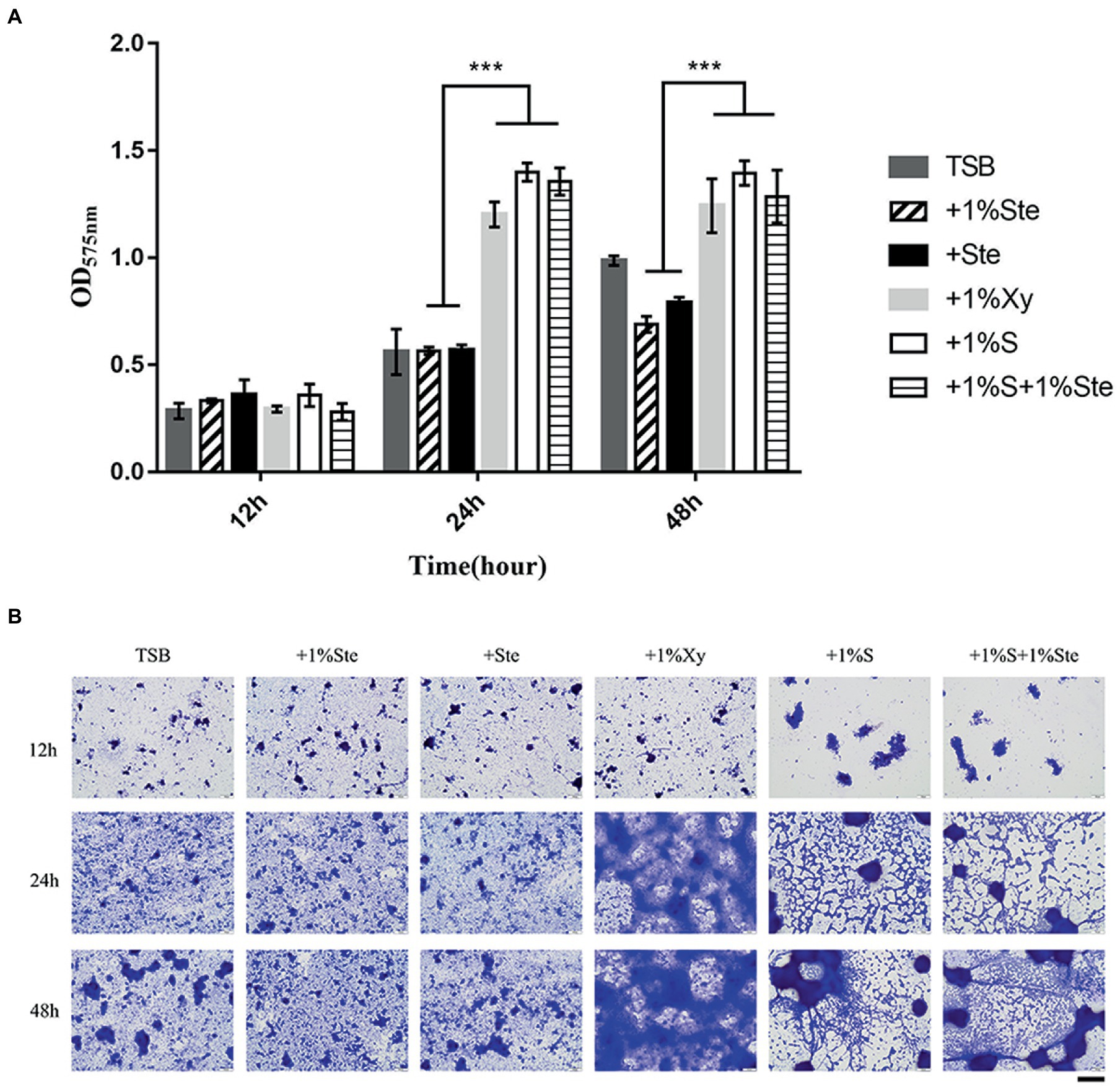
Figure 2. Effects of stevioside on the dual-species biofilm formation of S. mutans and C. albicans by crystal violet assay. (A) OD575nm measurement of dual-species biofilm formation at different times. (B) Representative images of dual-species biofilm after crystal violet staining. Results are presented as mean ± standard deviation (SD). Statistical significance is marked by asterisks: ***, p < 0.001. Scale bar: 100 μm.
3.3. Stevioside inhibited the attachment and damaged the structure of the dual-species biofilm
We observed that stevioside inhibited the biofilm growth and structure of the S. mutans and C. albicans dual-species by SEM at 2000× and 5,000× magnifications (Figure 3). Compared with the sucrose group, stevioside (1% ste or ste) only groups had a particularly sparse biofilm structure and little extracellular matrix. The fungal cells were ovoid in the yeast phase, and the bacterial distribution showed a short-chain, scattered distribution without an intertwined network distribution compared with the xylitol group (Figure 3). In the sucrose added groups, S. mutans and C. albicans had longer-chain and bud mycelium phases. They decomposed and produced large amounts of extracellular components, and the dual species were wrapped in a three-dimensional structure (Figure 3).
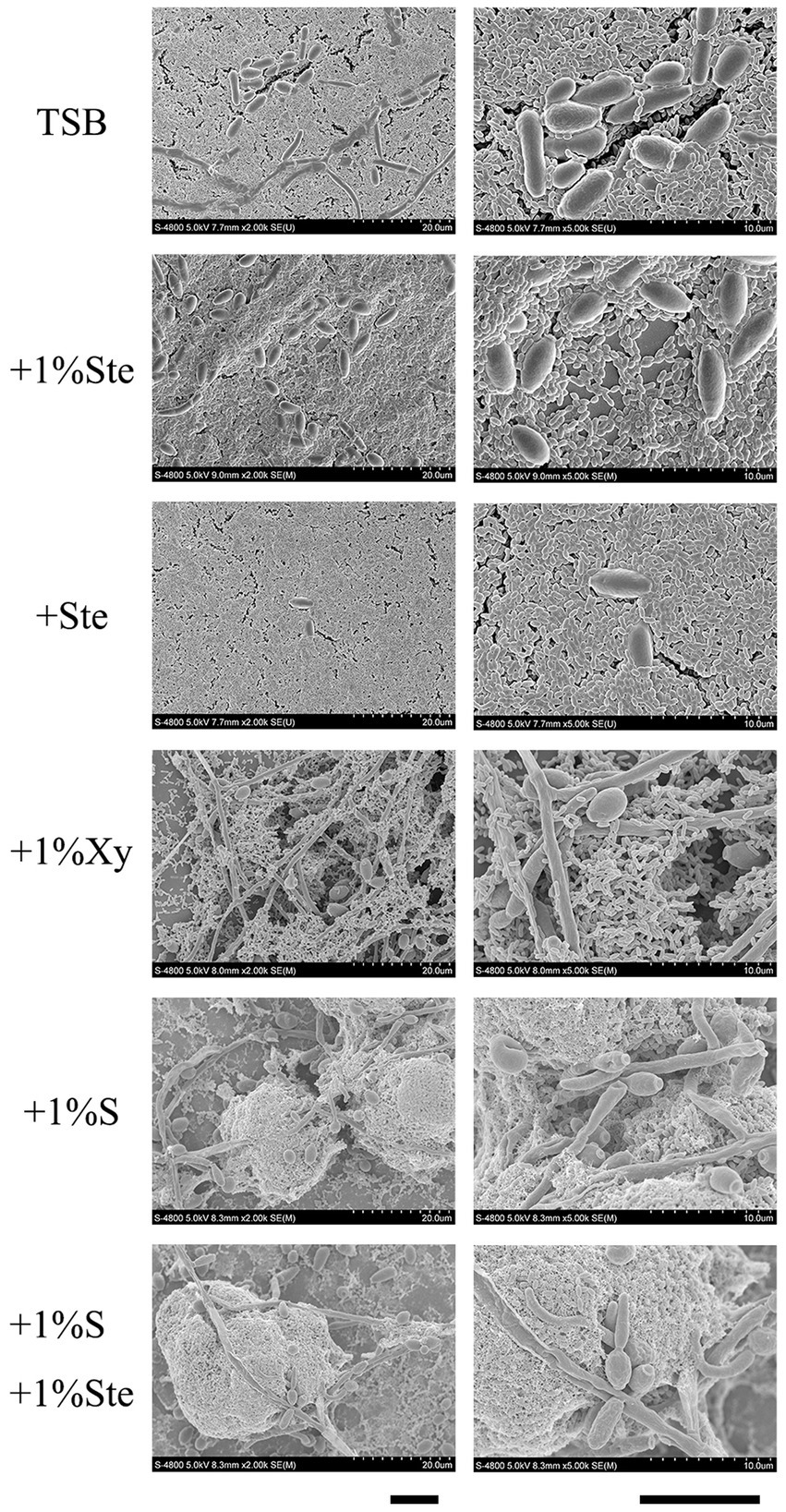
Figure 3. Morphological characteristics effects of stevioside on dual-species biofilm of S. mutans and C. albicans by SEM images. Representative SEM images of dual-species biofilm generated by S. mutans and C. albicans treated with different medium after 24 h of biofilm formation. Scale bar: 100 μm.
3.4. Stevioside inhibited the viability of dual-species biofilms and exerted no effect in the presence of sucrose
The control and stevioside groups had the smallest microcolonies, and the live and dead cells overlapped (Figure 4). The xylitol group had more viable bacteria and fungi observable as larger colonies (Figure 4). The sucrose added groups had the largest microcolonies (Figure 4). The viability inhibition rates were calculated to quantify viability changes in dual-species biofilms and compared by Kruskal–Wallis test with a post hoc Dunn’s test for multiple comparisons (Supplementary Figure S1; Xie et al., 2020). Higher viability inhibition rates were obtained for the 1% stevioside group, which had significantly decreased growth compared with 1% sucrose and 1% xylitol group (p < 0.001). The sample treated with 1% sucrose +1% stevioside had a lower viability inhibition rate compared with 1% stevioside only (p < 0.001), which was not significantly different from that of the 1% sucrose group (p > 0.05).
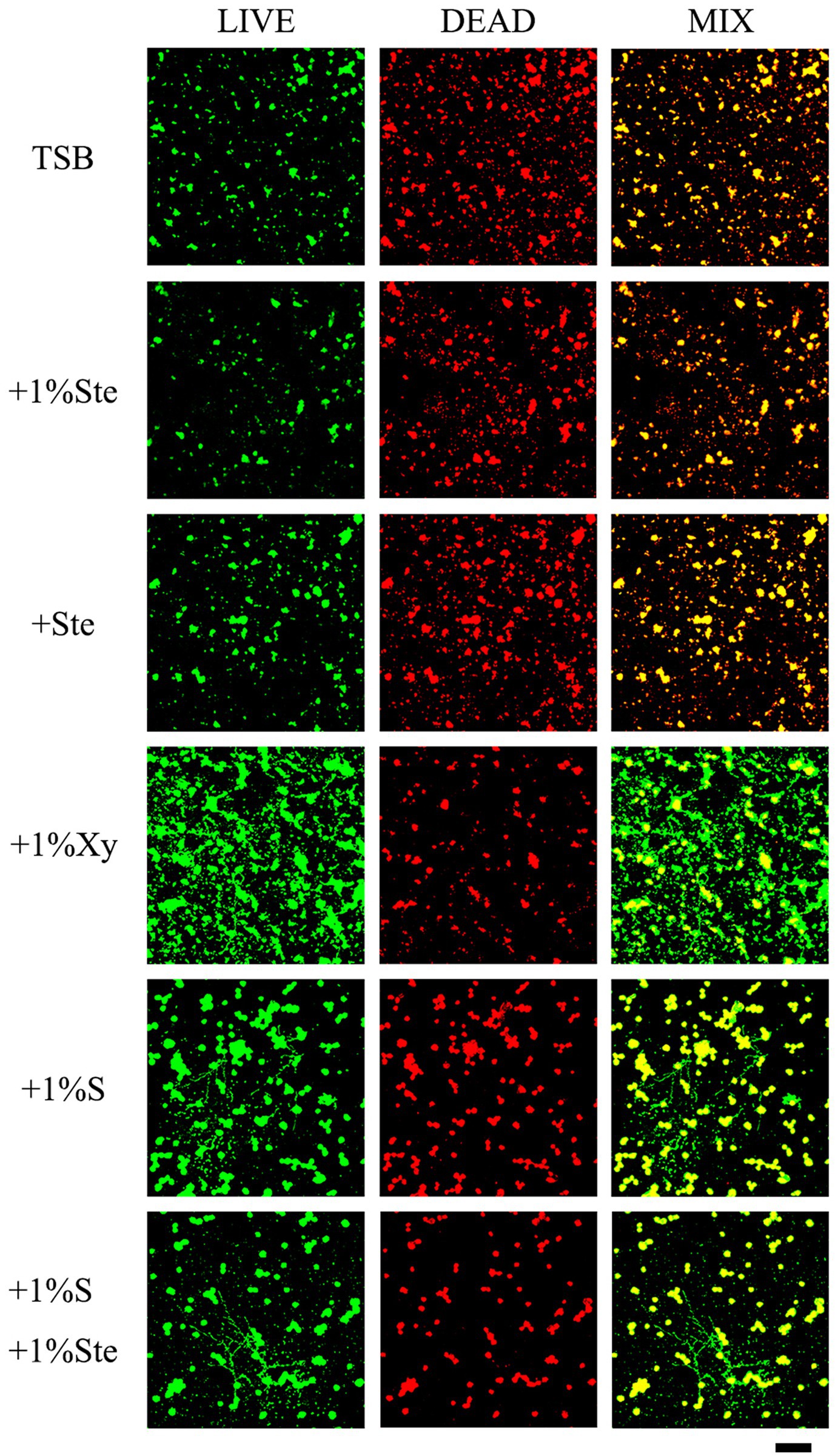
Figure 4. Evaluation of stevioside against dual-species biofilms viability by CLSM. Live bacteria/fungi were stained with fluorescent green. Dead bacteria/fungi were stained with fluorescent red. Scale bar: 200 μm.
3.5. Stevioside inhibited EPS generation in dual-species biofilms and sucrose supplementation abolished the stevioside mediated inhibition
Compared with 1% sucrose, stevioside groups had a particular inhibitory effect on S. mutans–C. albicans biofilms which EPS was significantly reduced in the group containing only stevioside (Figure 5). There was no significant difference between the stevioside groups and the control group (Figure 5). In addition, the sample with 1% xylitol had increased fungal growth with higher amounts of hyphae compared with TSB only (Figure 5). This finding matches previous results showing that addition of xylitol promotes the growth of C. albicans in the bud mycelium phase (Figure 3). However, there were fewer EPS in the xylitol group, which further supported the idea that xylitol improves pH (Figure 6). The sample treated with sucrose alone had the largest microcolonies and significantly increased EPS production (Figure 5). However, the amounts of microorganisms and EPS in 1% sucrose +1% stevioside were significantly more compared to 1% stevioside only, showing that the added sucrose reduced the inhibitory effect of stevioside (Figure 5). This finding is further supported by the increased biofilm formation of dual species in SEM (Figure 3) and CV staining (Figure 2).
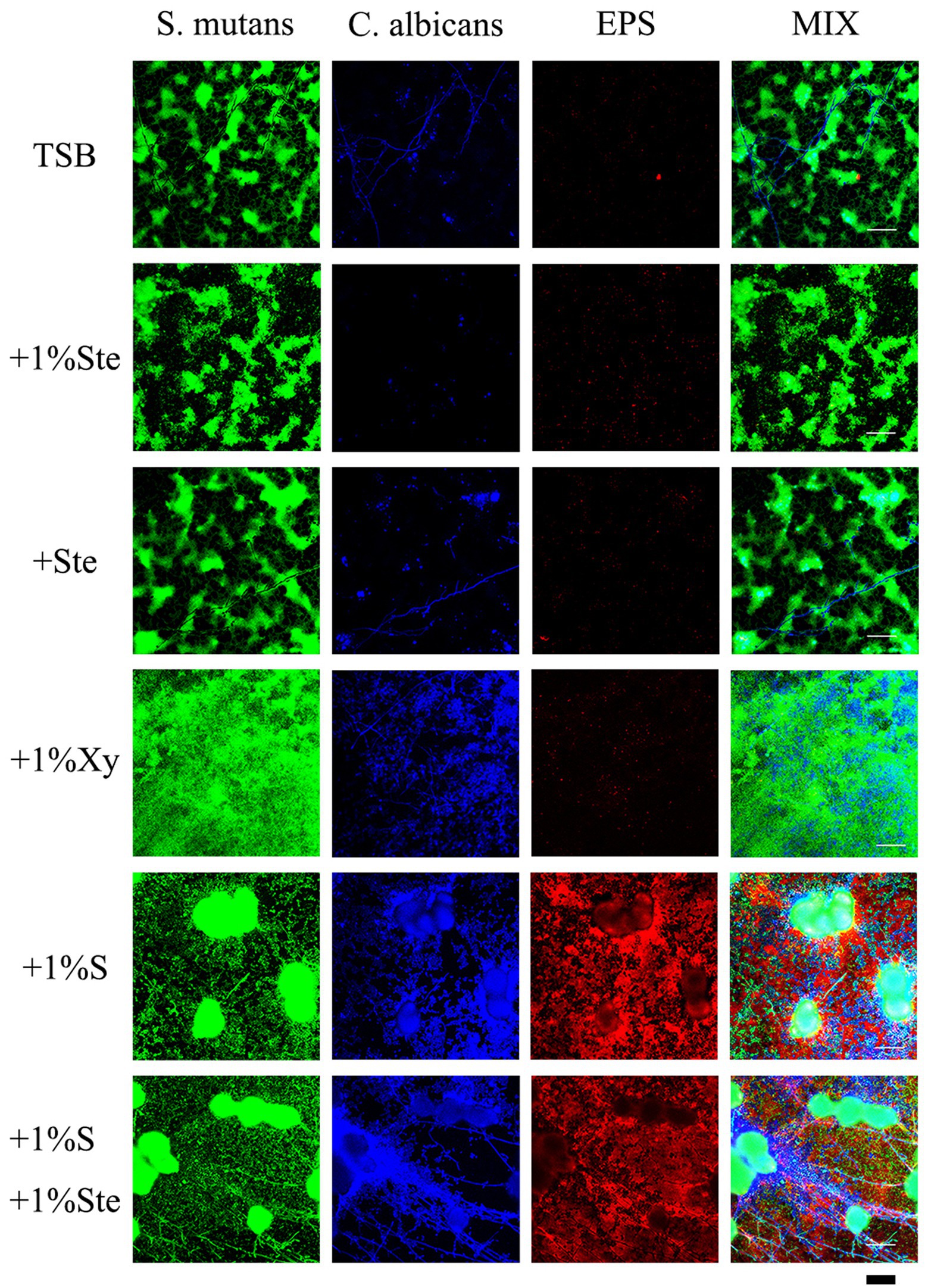
Figure 5. Effects of stevioside on dual-species biofilms and EPS by CLSM. Representative CLSM images of S. mutans–C. albicans and EPS after 24 h of culture in various media. S. mutans was stained with fluorescent green, C. albicans was stained with fluorescent blue, and EPS was stained with fluorescent red. Scale bar: 50 μm.
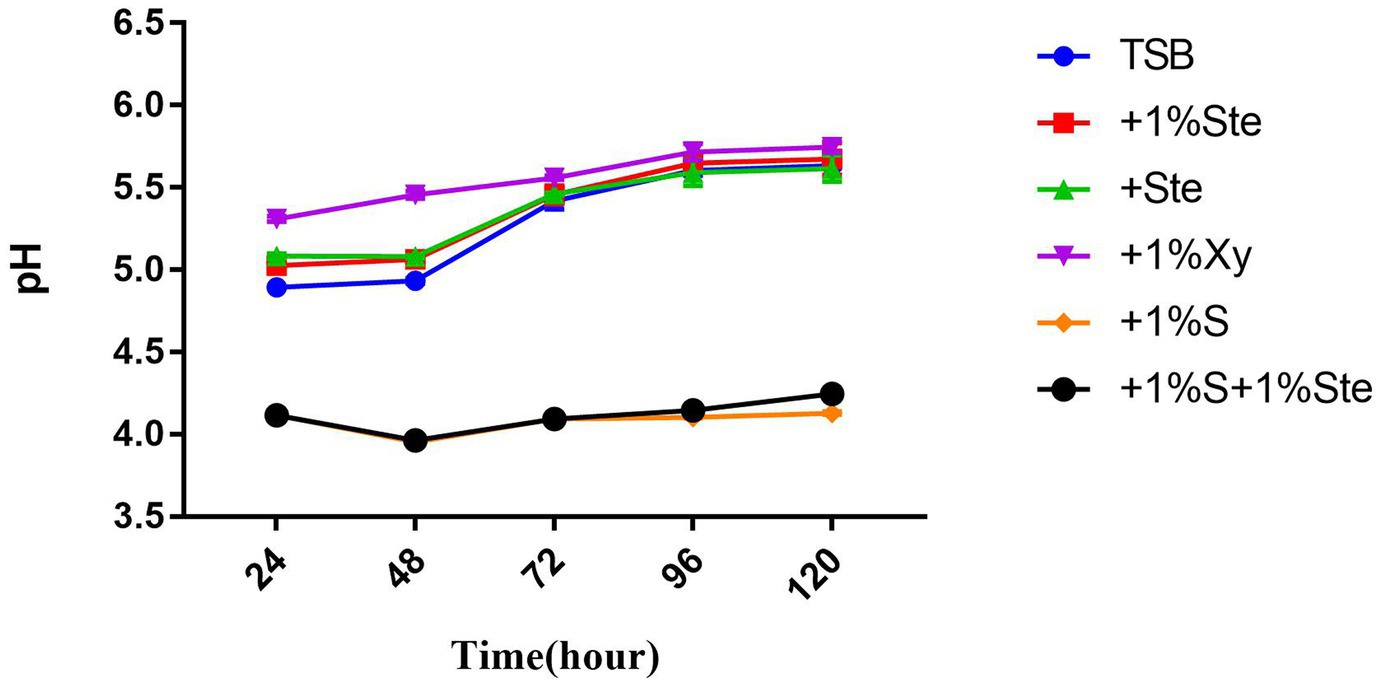
Figure 6. Effects of stevioside on acid production. pH value shows acid production at different times. pH measurement of different groups at 24, 48, 72, 96, and 120 h in various media. The data represent the means ± SD.
3.6. Stevioside decreased pH in dual-species biofilms and exerted no reduction in the presence of sucrose
The pH value was taken as a measure of the metabolic activity of acid production (Figure 6). In this experiment, the initial pH was adjusted to 7.04. There was no significant difference in pH between the control group and stevioside-added groups (p > 0.05), while the pH showed a significant increase between 48 and 72 h (p < 0.05) (Figure 6). The pH of sucrose-added groups was lower than the other groups (p < 0.05), nearly maintaining this level throughout the experiment (Figure 6). Moreover, the pH of the xylitol group was higher than that of other groups at 24 and 48 h (Figure 6), whereas at 72, 96, and 120 h, the xylitol group did not show a significant change compared to the control and stevioside-added groups (p > 0.05) (Figure 6).
3.7. Effects of stevioside on transcriptomic changes in Streptococcus mutans and Candida albicans in mixed-species biofilms
Based on statistical significance (p ≤ 0.05), 512 upregulated genes and 389 downregulated genes were identified in S. mutans (Figure 7A), while 111 upregulated genes and 106 downregulated genes were identified in C. albicans (Figure 7D) in the stevioside group compared with sucrose group. Through KEGG pathway analysis, eight KEGG pathways were significantly affected by stevioside treatment than by sucrose treatment in S. mutans: galactose metabolism, starch and sucrose metabolism, two-component system, phosphotransferase system (PTS), 2-Oxocarboxylic acid metabolism, quorum sensing, ABC transporters, and arginine biosynthesis (p ≤ 0.05; Figure 7B). In C. albicans, five KEGG pathways including carbon metabolism, glycolysis/gluconeogenesis, glyoxylate and dicarboxylate metabolism, pyruvate metabolism, and biosynthesis of amino acids were significantly affected in the stevioside group (p ≤ 0.05; Figure 7E).
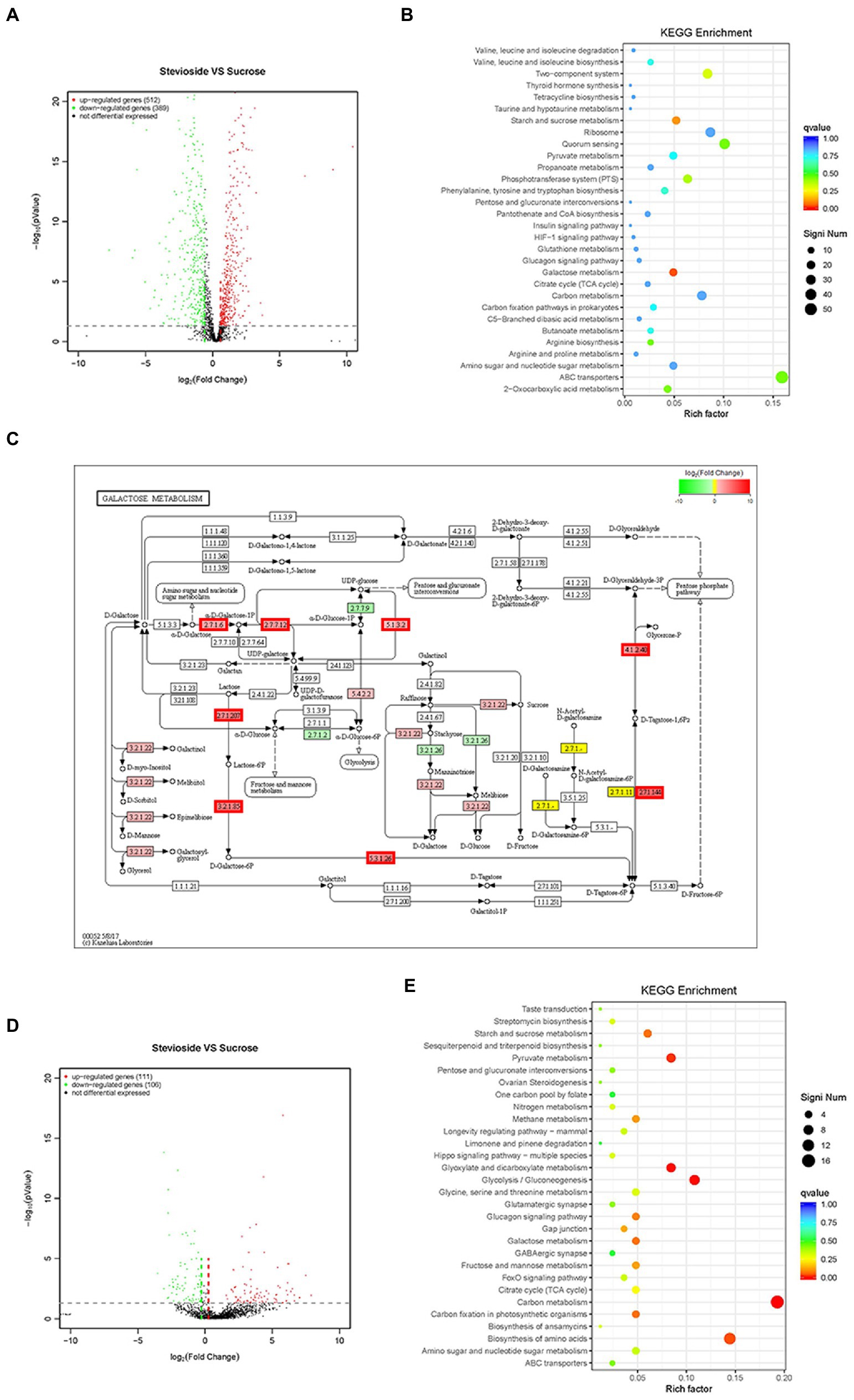
Figure 7. Effects of stevioside through RNA-Seq analysis in S. mutans and C. albicans dual-species biofilms. (A),(D) Volcano plot illustrating genes with significant expression differences of S. mutans and C. albicans in mixed-species biofilms in the stevioside group (versus sucrose group). (B),(E) KEGG pathways analysis in the stevioside group compared with in sucrose groups. (KEGG, Kyoto Encyclopedia of Genes and Genomes) (QValue, a Benjamini–Hochberg adjusted p value). (C) KEGG galactose metabolism map (ko00052) of S. mutans in the stevioside group (versus sucrose group) in dual-species biofilms. The lac operon (lacEF, lacG, lacAB, lacC, and lacD), galK, galT, and galE were upregulated. (The mentioned genes are bordered in red) (A–C) represent S. mutans; (D),(E) represent C. albicans).
In addition, the log2 fold change (|log2FoldChange| ≥ 1) was also used as a filtering technique to identify upregulated or downregulated genes (Ellepola et al., 2019). In S. mutans, scrA, encoding sucrose-specific IIABC component (EIIScr) which internalizes sucrose as sucrose-6-phosphate through PTS system, was significantly downregulated in the stevioside group (versus the sucrose group) (Supplementary Table S1; Kilic et al., 2007). However, genes encoding galactose and intracellular polysaccharide [IPS; glycogen, (glg)] metabolism by S. mutans were significantly upregulated, reflecting the relief of carbohydrate starvation in the stevioside group (versus the sucrose group; Supplementary Table S2). In galactose metabolism, the upregulated genes were lacEF (encoding a lactose-specific enzyme), lacG (encoding 6-phospho-β-galactosidase), lacAB (encoding galactose-6-phosphate isomerase), lacC (encoding tagatose-6-phosphate kinase), and lacD (encoding tagatose-1,6-bisphosphate aldolase) in the tagatose pathway, while galK (encoding galactokinase), galT (encoding hexose1-phosphate uridyltransferase), and galE (encoding UDP-galactose 4-epimerase) in the Leloir pathway in the stevioside group (versus the sucrose group) (Figure 7C; Supplementary Table S1; Abranches et al., 2004). In addition, the glg operon, which includes glgA (encoding glycogen synthase), glgB (encoding branching enzyme), glgC&D (encoding two subunits of the ADP-glucose pyrophosphorylase, ADP-Glc-PP), and glgP (encoding glycogen phosphorylase), was also upregulated that encoded enzymes responsible for IPS synthesis and degradation (Supplementary Table S2; Costa Oliveira et al., 2021).
In C. albicans, downregulated genes included hwp1, pga10, pga23, pga33, and pga54 which encodes cell surface-associated glycoproteins and GPI-modified proteins in stevioside treatment (versus the sucrose group; Supplementary Table S2; Plaine et al., 2008). Their functions can be assigned to cell wall biosynthesis or remodeling and cell–cell adhesion and interactions in C. albicans. Furthermore, sap4, sap5, and sap6 were also downregulated in the stevioside group than in the sucrose group (Supplementary Table S2). They belong to the secreted aspartyl proteinase (SAP) family, which is associated with hyphal formation, tissue damage, and attachment of C. albicans to host surfaces (Naglik et al., 2003).
4. Discussion
Our study showed that stevioside inhibited planktonic growth and biofilm formation. Unlike growth with sucrose, acid and EPS production were unaffected, compared to the no sugar added control when the stevioside sugar was added. These data suggest that stevioside is not used by the microorganisms to generate EPS or acid. Meanwhile, it altered the biofilm structure and decreased the viability of dual-species biofilm. The transcript levels of S. mutans and C. albicans genes are consistent with our findings. When stevioside rather than sucrose was utilized by bacteria, sucrose transport decreased, and galactose and IPS metabolism by S. mutans were upregulated. In C. albicans, downregulated genes have been reported to encode GPI-modified proteins and secreted aspartyl proteinase (SAP) family. However, previous research may not reflect commonly available conditions regarding contact with sucrose. In this study, we demonstrated that sucrose supplementation significantly reduced the inhibitory effects of stevioside. Therefore, if the oral cavity is frequently exposed to sucrose, stevioside cannot prevent bacteria from utilizing sucrose. However, stevioside can be used as an intensely sweet sucrose substitute to reduce sucrose intake.
Sugar substitutes are generally considered to have an inhibitory effect on cariogenic bacteria, such as xylitol and erythritol (Söderling and Pienihäkkinen, 2020). The use of stevioside, as a natural non-carbohydrate sweetener, has also contributed to caries prevention in many studies (Das et al., 1992; Ajagannanavar et al., 2014; Kishta Derani et al., 2016; Escobar et al., 2020; Ganter et al., 2020). It reduces the formation of biofilm by one or more bacteria strains and increases pH (Ajagannanavar et al., 2014; Escobar et al., 2020). Based on artificial caries models, the results suggested less cariogenic effects and enamel demineralization for stevioside, reducing viable cells and EPS formation (Giacaman et al., 2013; Kishta Derani et al., 2016). Animal models have also been used to verify that stevioside is not cariogenic (Das et al., 1992). Stevioside is considered a non-cariogenic sweetener. However, most of the studies have examined a single bacterial biofilm model and did not consider the unavoidable presence of sucrose in the daily diet, which may mitigate or abolish the anti-cariogenic effects of stevioside. Therefore, our study is the first to evaluate the efficacy of stevioside in the presence of sucrose against the dual-species biofilm of S. mutans and C. albicans in vitro. Additionally, since stevioside is about 300 times sweeter than sucrose on an equal weight basis, we evaluated another experiment group in which we used a stevioside concentration with the same sweetness as sucrose, providing a reference for economic application and popularization.
Many studies confirm our results regarding sucrose’s strong cariogenic ability from the perspective of bacterial and fungal planktonic accumulation, biofilm formation, EPS, and acid production (Klein et al., 2009; Forssten et al., 2010; Cai et al., 2018; Cavazana et al., 2018; Guan et al., 2020). From the planktonic growth curve and crystal violet assay, we conclude that stevioside restrains the accumulation of suspension cells and significantly inhibits the growth of hybrid biofilm compared with sucrose. It is also well known that bacteria use carbohydrates to produce an extracellular matrix; then, a multi-species becomes embedded in this matrix (Forssten et al., 2010). EPS are glucosaccharides synthesized by glycosyltransferase and form the biofilm matrix together with extracellular proteins and lipids, and this matrix is involved in bacterial colonization, biofilm formation and maintenance, and pathogenesis (Klein et al., 2015; Cugini et al., 2019; Rainey et al., 2019). SEM showed that stevioside reduced the production of the extracellular matrix via effects on metabolism, and CLSM images showed fewer EPS with a scattered distribution of S. mutans and C. albicans rather than a three-dimensional biofilm. These results are consistent with previous results regarding stevioside’s effects in inhibiting the formation of single-species biofilms (Lemus-Mondaca et al., 2012; Ajagannanavar et al., 2014; Escobar et al., 2020).
The factor initiating caries is the metabolic activity of acid production. When the pH falls below 5.5, demineralization occurs faster than remineralization (Takahashi and Nyvad, 2011; Guan et al., 2020). The lowest pH (far below 5.5) of all treatments was observed when sucrose was added. We also concluded that dual-species biofilm’s ability to produce acid under stevioside treatment was weak and similar to the xylitol and control groups. However, this result is significantly different from those in previous studies on single-species biofilms, in which all groups had a lower pH (Escobar et al., 2020; Ganter et al., 2020). These results may be related to the choice of C. albicans and the significant synergy between S. mutans and C. albicans. Previous in vitro and in vivo studies have revealed that a dual-species S. mutans and C. albicans biofilm maintains low acidic pH values (~4.3; Hwang et al., 2017; Kim et al., 2017; Garcia et al., 2021). The GtfB derived from S. mutans produces abundant extracellular α-glucans capable of binding to C. albicans surfaces and facilitating their acidogenic characteristics (Krzyściak et al., 2017; Huffines and Scoffield, 2020; Kim et al., 2020; Garcia et al., 2021).
In RNA-Seq analysis, we show here that sugar starvation with sugar substitute supplement inhibits sucrose transferase enzymes in PTS, yet promotes galactose and IPS conversion in S. mutans. Streptococcus mutans can survive long periods of nutrient limitation (Costa Oliveira et al., 2021). For instance, S. mutans can metabolize galactose through two distinct pathways under catabolite repression (Abranches et al., 2004). Genes encoding the tagatose 6-phosphate and Leloir pathway in galactose metabolism were significantly upregulated in the stevioside group. Recent studies have also demonstrated that the transcript levels of the glg operon are increased under sucrose-limiting as opposed to sucrose-excess conditions in S. mutans (Costa Oliveira et al., 2021). In our study, the presence of sucrose tends to reduce IPS expression when compared to stevioside (Supplementary Table S2). Furthermore, pyruvate metabolism is important for S. mutans survival and expression of virulence (He et al., 2017). Pyruvate is converted by lactate dehydrogenase (LDH), pyruvate formate lyase (PFL), and pyruvate dehydrogenase complex (PDH; Busuioc et al., 2010). If carbohydrate is limiting, the PDH pathway is activated to convert pyruvate to acetyl-CoA and CO2 with the concomitant reduction of NAD+ to NADH under aerobic conditions (Kim et al., 2015). In the stevioside group, the PDH operons (pdhD, pdhA, pdhB, and pdhC) were all upregulated; ldh and pflA were downregulated (Supplementary Table S2; Busuioc et al., 2010). The activation of the PDH pathway and inactivation of the LDH and PFL pathways may be response to sucrose limitation and mixed-competitor biofilms in S. mutans.
Pathogenic fungi grow in a variety of morphologies, including yeast, pseudohyphae, and hyphae (Sudbery et al., 2004; Kadosh, 2013). These filamentous morphologies, including pseudohyphae and hyphae, comprise elongated cells attached end to end, whereas yeast typically has oval-shaped or round single cells (Sudbery et al., 2004; Kadosh, 2013). The virulence and invasiveness of C. albicans require a transition from yeast to filamentous (Berman, 2006; Kadosh, 2013; Kim et al., 2017). Hyphal formation, surface recognition molecules, phenotypic switching, and extracellular hydrolytic enzyme production have been suggested to be mostly virulence attributes for C. albicans (Naglik et al., 2003). Xylitol may promote C. albicans pathogenicity since the typical mycelium and mature hyphae structure of C. albicans is more pronounced in the treatment of dual-species biofilm with xylitol than with control treatment (Figure 3). Furthermore, xylitol may be able to promote C. albicans to form typical mycelium and cell interactions intertwined with S. mutans, as seen from SEM and CLSM images, indicating that xylitol’s inhibitory effect on C. albicans is weak. Previous research had indicated that xylitol’s inhibitory effects on C. albicans are not significant, and the presence of additional sucrose reduces the inhibitory effects of xylitol on the formation of dual-species S. mutans–C. albicans biofilm, which are consistent with our results (Chan et al., 2020). Until recently, only a few studies have demonstrated the effects of xylitol on biofilm formation and adhesion of C. albicans (Brambilla et al., 2016; Santi et al., 2016; Chan et al., 2020). There is no evidence that xylitol suppresses biofilm formation during prolonged growth (Brambilla et al., 2016; Santi et al., 2016). It may be interesting to address a quantitation of mycelium and mature hyphae over multiple fields of view in independently performed experiments about the effect of xylitol on hypha formation in further studies.
Our experiment also had some limitations. Previous research has shown that the whole oral microecology is more than the simple sum of its parts. The interactions between different microorganism lead to many new physiological functions in oral microecology that cannot be observed for single species (Kuramitsu et al., 2007; Guo et al., 2014; Baker et al., 2017; Miller et al., 2019). We only studied the effects of stevioside on dual-biofilm and not extensive cell–cell interactions, physiological functions, or the synergistic pathogenesis between S. mutans and C. albicans. Moreover, as one of the most complex microbial floras in the human body, oral microecology comprises more than 700 different species (Kuramitsu et al., 2007). The dual-species biofilm model used in our research does not simulate the complex microbial community of dental plaque. Further validation applying more complex multi-microbial biofilms in vitro and caries models in vivo would help supports our findings and conclusions.
As the first study to evaluate the effects of stevioside on a mixed biofilm, our results can serve as a foundation for subsequent studies investigating multi-bacterial biofilms. Furthermore, the results re-emphasize the cariogenicity of sucrose and show that the sugar substitute does not play an inhibitory effect in the presence of sucrose. To prevent caries, it is more important to reduce the intake of sucrose, and in this case, consider stevioside with high sweetness, which can reduce the use of sugar and play a role in inhibiting the cariogenic bacteria.
Data availability statement
The datasets presented in this study can be found in online repositories. The names of the repository/repositories and accession number(s) can be found below: NCBI Sequence Read Archive (SRA) database, PRJNA913472."
Author contributions
MG, KY, YC, ZFZ, PC, RH, and XW conceived the experiments. MG, KY, ZFZ, YC, ZYZ, and PC performed the experiments. MG, KY, ZFZ, YC, RH, and XW analyzed the results and data interpretation. MG, KY, ZFZ, YC, ZYZ, PC, RH, and XW drafted and co-wrote the paper, final approval of the version to be published, and agree to be accountable for all aspects of the work in ensuring that questions related to the accuracy or integrity of any part of the work are appropriately investigated and resolved. XW funded the study. All authors contributed to the article and approved the submitted version.
Funding
This research was funded by Special Project of National Clinical Research Center for Oral Diseases (LCA202010; XW); Independent research project of State Key Laboratory of Military Stomatology (2019ZA06; XW); The Third Affiliated Hospital of Air Force Medical University New Technology and New Business (LX2020-104; XW).
Conflict of interest
The authors declare that the research was conducted in the absence of any commercial or financial relationships that could be construed as a potential conflict of interest.
Publisher’s note
All claims expressed in this article are solely those of the authors and do not necessarily represent those of their affiliated organizations, or those of the publisher, the editors and the reviewers. Any product that may be evaluated in this article, or claim that may be made by its manufacturer, is not guaranteed or endorsed by the publisher.
Supplementary material
The Supplementary material for this article can be found online at: https://www.frontiersin.org/articles/10.3389/fmicb.2023.1128668/full#supplementary-material
References
Abranches, J., Chen, Y. Y., and Burne, R. A. (2004). Galactose metabolism by Streptococcus mutans. Appl. Environ. Microbiol. 70, 6047–6052. doi: 10.1128/aem.70.10.6047-6052.2004
Ajagannanavar, S. L., Shamarao, S., Battur, H., Tikare, S., Al-Kheraif, A. A., and Al Sayed, M. S. (2014). Effect of aqueous and alcoholic stevia (Stevia rebaudiana) extracts against Streptococcus mutans and lactobacillus acidophilus in comparison to chlorhexidine: an in vitro study. J. Int. Soc. Prev. Community Dent. 4, 116–121. doi: 10.4103/2231-0762.146215
Alexa, A., Rahnenführer, J., and Lengauer, T. (2006). Improved scoring of functional groups from gene expression data by decorrelating GO graph structure. Bioinformatics 22, 1600–1607. doi: 10.1093/bioinformatics/btl140
Azzam, S. Z., Cayme, G. J., and Martinez, L. R. (2020). Polymicrobial interactions involving fungi and their importance for the environment and in human disease. Microb. Pathog. 140:103942. doi: 10.1016/j.micpath.2019.103942
Baker, J. L., Bor, B., Agnello, M., Shi, W., and He, X. (2017). Ecology of the Oral microbiome: beyond bacteria. Trends Microbiol. 25, 362–374. doi: 10.1016/j.tim.2016.12.012
Berman, J. (2006). Morphogenesis and cell cycle progression in Candida albicans. Curr. Opin. Microbiol. 9, 595–601. doi: 10.1016/j.mib.2006.10.007
Brambilla, E., Ionescu, A. C., Cazzaniga, G., Ottobelli, M., and Samaranayake, L. P. (2016). Levorotatory carbohydrates and xylitol subdue Streptococcus mutans and Candida albicans adhesion and biofilm formation. J. Basic Microbiol. 56, 480–492. doi: 10.1002/jobm.201500329
Busuioc, M., Buttaro, B. A., and Piggot, P. J. (2010). The pdh operon is expressed in a subpopulation of stationary-phase bacteria and is important for survival of sugar-starved Streptococcus mutans. J. Bacteriol. 192, 4395–4402. doi: 10.1128/jb.00574-10
Cai, J. N., Jung, J. E., Lee, M. H., Choi, H. M., and Jeon, J. G. (2018). Sucrose challenges to Streptococcus mutans biofilms and the curve fitting for the biofilm changes. FEMS Microbiol. Ecol. 94. doi: 10.1093/femsec/fiy091
Cavazana, T. P., Pessan, J. P., Hosida, T. Y., Monteiro, D. R., and Botazzo Delbem, A. C. (2018). pH changes of mixed biofilms of Streptococcus mutans and Candida albicans after exposure to sucrose solutions in vitro. Arch. Oral Biol. 90, 9–12. doi: 10.1016/j.archoralbio.2018.02.019
Chan, A., Ellepola, K., Truong, T., Balan, P., Koo, H., and Seneviratne, C. J. (2020). Inhibitory effects of xylitol and sorbitol on Streptococcus mutans and Candida albicans biofilms are repressed by the presence of sucrose. Arch. Oral Biol. 119:104886. doi: 10.1016/j.archoralbio.2020.104886
Costa Oliveira, B. E., Ricomini Filho, A. P., Burne, R. A., and Zeng, L. (2021). The route of sucrose utilization by Streptococcus mutans affects intracellular polysaccharide metabolism. Front. Microbiol. 12:636684. doi: 10.3389/fmicb.2021.636684
Cugini, C., Shanmugam, M., Landge, N., and Ramasubbu, N. (2019). The role of exopolysaccharides in Oral biofilms. J. Dent. Res. 98, 739–745. doi: 10.1177/0022034519845001
Cui, Y., Wang, Y., Zhang, Y., Pang, L., Zhou, Y., Lin, H., et al. (2021). Oral Mycobiome differences in various spatial niches with and without severe early childhood caries. Front. Pediatr. 9:748656. doi: 10.3389/fped.2021.748656
Das, S., Das, A. K., Murphy, R. A., Punwani, I. C., Nasution, M. P., and Kinghorn, A. D. (1992). Evaluation of the cariogenic potential of the intense natural sweeteners stevioside and rebaudioside a. Caries Res. 26, 363–366. doi: 10.1159/000261469
Ellepola, K., Liu, Y., Cao, T., Koo, H., and Seneviratne, C. J. (2017). Bacterial GtfB augments Candida albicans accumulation in cross-kingdom biofilms. J. Dent. Res. 96, 1129–1135. doi: 10.1177/0022034517714414
Ellepola, K., Truong, T., Liu, Y., Lin, Q., Lim, T. K., Lee, Y. M., et al. (2019). Multi-omics analyses reveal synergistic carbohydrate metabolism in Streptococcus mutans-Candida albicans mixed-species biofilms. Infect. Immun. 87, e00339–e00319. doi: 10.1128/iai.00339-19
Escobar, E., Piedrahita, M., and Gregory, R. L. (2020). Growth and viability of Streptococcus mutans in sucrose with different concentrations of Stevia rebaudiana Bertoni. Clin. Oral Investig. 24, 3237–3242. doi: 10.1007/s00784-020-03197-5
Falsetta, M. L., Klein, M. I., Colonne, P. M., Scott-Anne, K., Gregoire, S., Pai, C. H., et al. (2014). Symbiotic relationship between Streptococcus mutans and Candida albicans synergizes virulence of plaque biofilms in vivo. Infect. Immun. 82, 1968–1981. doi: 10.1128/iai.00087-14
Ferrazzano, G. F., Cantile, T., Alcidi, B., Coda, M., Ingenito, A., Zarrelli, A., et al. (2015). Is Stevia rebaudiana Bertoni a non cariogenic sweetener? A review. Molecules 21:E38. doi: 10.3390/molecules21010038
Forssten, S. D., Björklund, M., and Ouwehand, A. C. (2010). Streptococcus mutans, caries and simulation models. Nutrients 2, 290–298. doi: 10.3390/nu2030290
Ganter, J., Hellwig, E., Doerken, S., and Al-Ahmad, A. (2020). In vitro evaluation of the cariogenic potential of rebaudioside a compared to sucrose and xylitol. Clin. Oral Investig. 24, 113–122. doi: 10.1007/s00784-019-02908-x
Garcia, B. A., Acosta, N. C., Tomar, S. L., Roesch, L. F. W., Lemos, J. A., Mugayar, L. R. F., et al. (2021). Association of Candida albicans and Cbp(+) Streptococcus mutans with early childhood caries recurrence. Sci. Rep. 11:10802. doi: 10.1038/s41598-021-90198-3
Giacaman, R. A., Campos, P., Muñoz-Sandoval, C., and Castro, R. J. (2013). Cariogenic potential of commercial sweeteners in an experimental biofilm caries model on enamel. Arch. Oral Biol. 58, 1116–1122. doi: 10.1016/j.archoralbio.2013.03.005
Guan, C., Che, F., Zhou, H., Li, Y., Li, Y., and Chu, J. (2020). Effect of Rubusoside, a natural sucrose substitute, on Streptococcus mutans biofilm cariogenic potential and virulence gene expression in vitro. Appl. Environ. Microbiol. 86, e01012–e01020. doi: 10.1128/aem.01012-20
Guo, H., Chen, Y., Guo, W., and Chen, J. (2021). Effects of extracellular DNA on dual-species biofilm formed by Streptococcus mutans and Candida albicans. Microb. Pathog. 154:104838. doi: 10.1016/j.micpath.2021.104838
Guo, L., He, X., and Shi, W. (2014). Intercellular communications in multispecies oral microbial communities. Front. Microbiol. 5:328. doi: 10.3389/fmicb.2014.00328
Gupta, P., Gupta, N., Pawar, A. P., Birajdar, S. S., Natt, A. S., and Singh, H. P. (2013). Role of sugar and sugar substitutes in dental caries: a review. ISRN Dent 2013:519421. doi: 10.1155/2013/519421
Harriott, M. M., and Noverr, M. C. (2011). Importance of Candida-bacterial polymicrobial biofilms in disease. Trends Microbiol. 19, 557–563. doi: 10.1016/j.tim.2011.07.004
He, J., Kim, D., Zhou, X., Ahn, S. J., Burne, R. A., Richards, V. P., et al. (2017). RNA-Seq reveals enhanced sugar metabolism in Streptococcus mutans co-cultured with Candida albicans within mixed-species biofilms. Front. Microbiol. 8:1036. doi: 10.3389/fmicb.2017.01036
Huffines, J. T., and Scoffield, J. A. (2020). Disruption of Streptococcus mutans and Candida albicans synergy by a commensal streptococcus. Sci. Rep. 10:19661. doi: 10.1038/s41598-020-76744-5
Hwang, G., Liu, Y., Kim, D., Li, Y., Krysan, D. J., and Koo, H. (2017). Candida albicans mannans mediate Streptococcus mutans exoenzyme GtfB binding to modulate cross-kingdom biofilm development in vivo. PLoS Pathog. 13:e1006407. doi: 10.1371/journal.ppat.1006407
Hwang, G., Marsh, G., Gao, L., Waugh, R., and Koo, H. (2015). Binding force dynamics of Streptococcus mutans-glucosyltransferase B to Candida albicans. J. Dent. Res. 94, 1310–1317. doi: 10.1177/0022034515592859
Kadosh, D. (2013). Shaping up for battle: morphological control mechanisms in human fungal pathogens. PLoS Pathog. 9:e1003795. doi: 10.1371/journal.ppat.1003795
Kilic, A. O., Honeyman, A. L., and Tao, L. (2007). Overlapping substrate specificity for sucrose and maltose of two binding protein-dependent sugar uptake systems in Streptococcus mutans. FEMS Microbiol. Lett. 266, 218–223. doi: 10.1111/j.1574-6968.2006.00522.x
Kim, J. N., Ahn, S. J., and Burne, R. A. (2015). Genetics and physiology of acetate metabolism by the Pta-Ack pathway of Streptococcus mutans. Appl. Environ. Microbiol. 81, 5015–5025. doi: 10.1128/aem.01160-15
Kim, H. E., Liu, Y., Dhall, A., Bawazir, M., Koo, H., and Hwang, G. (2020). Synergism of Streptococcus mutans and Candida albicans reinforces biofilm maturation and Acidogenicity in saliva: an in vitro study. Front. Cell. Infect. Microbiol. 10:623980. doi: 10.3389/fcimb.2020.623980
Kim, D., Sengupta, A., Niepa, T. H., Lee, B. H., Weljie, A., Freitas-Blanco, V. S., et al. (2017). Candida albicans stimulates Streptococcus mutans microcolony development via cross-kingdom biofilm-derived metabolites. Sci. Rep. 7:41332. doi: 10.1038/srep41332
Kishta Derani, M., Neiva, G. F., Boynton, J. R., Kim, Y. E., and Fontana, M. (2016). The antimicrobial potential of stevia in an in vitro microbial caries model. Am. J. Dent. 29, 87–92.
Klein, M. I., Duarte, S., Xiao, J., Mitra, S., Foster, T. H., and Koo, H. (2009). Structural and molecular basis of the role of starch and sucrose in Streptococcus mutans biofilm development. Appl. Environ. Microbiol. 75, 837–841. doi: 10.1128/aem.01299-08
Klein, M. I., Hwang, G., Santos, P. H., Campanella, O. H., and Koo, H. (2015). Streptococcus mutans-derived extracellular matrix in cariogenic oral biofilms. Front. Cell. Infect. Microbiol. 5:10. doi: 10.3389/fcimb.2015.00010
Krzyściak, W., Kościelniak, D., Papież, M., Vyhouskaya, P., Zagórska-Świeży, K., Kołodziej, I., et al. (2017). Effect of a lactobacillus Salivarius probiotic on a double-species Streptococcus mutans and Candida albicans caries biofilm. Nutrients 9:1242. doi: 10.3390/nu9111242
Kuramitsu, H. K., He, X., Lux, R., Anderson, M. H., and Shi, W. (2007). Interspecies interactions within oral microbial communities. Microbiol. Mol. Biol. Rev. 71, 653–670. doi: 10.1128/mmbr.00024-07
Langmead, B., and Salzberg, S. L. (2012). Fast gapped-read alignment with bowtie 2. Nat. Methods 9, 357–359. doi: 10.1038/nmeth.1923
Lemus-Mondaca, R., Vega-Gálvez, A., Zura-Bravo, L., and Ah-Hen, K. (2012). Stevia rebaudiana Bertoni, source of a high-potency natural sweetener: a comprehensive review on the biochemical, nutritional and functional aspects. Food Chem. 132, 1121–1132. doi: 10.1016/j.foodchem.2011.11.140
Lohner, S., Toews, I., and Meerpohl, J. J. (2017). Health outcomes of non-nutritive sweeteners: analysis of the research landscape. Nutr. J. 16:55. doi: 10.1186/s12937-017-0278-x
Love, M. I., Huber, W., and Anders, S. (2014). Moderated estimation of fold change and dispersion for RNA-seq data with DESeq2. Genome Biol. 15:550. doi: 10.1186/s13059-014-0550-8
Metwalli, K. H., Khan, S. A., Krom, B. P., and Jabra-Rizk, M. A. (2013). Streptococcus mutans, Candida albicans, and the human mouth: a sticky situation. PLoS Pathog. 9:e1003616. doi: 10.1371/journal.ppat.1003616
Miller, D. P., Fitzsimonds, Z. R., and Lamont, R. J. (2019). Metabolic signaling and spatial interactions in the Oral Polymicrobial community. J. Dent. Res. 98, 1308–1314. doi: 10.1177/0022034519866440
Naglik, J. R., Challacombe, S. J., and Hube, B. (2003). Candida albicans secreted aspartyl proteinases in virulence and pathogenesis. Microbiol. Mol. Biol. Rev. 67, 400–428, table of contents. doi: 10.1128/mmbr.67.3.400-428.2003
Peleg, A. Y., Hogan, D. A., and Mylonakis, E. (2010). Medically important bacterial-fungal interactions. Nat. Rev. Microbiol. 8, 340–349. doi: 10.1038/nrmicro2313
Pitts, N. B., Zero, D. T., Marsh, P. D., Ekstrand, K., Weintraub, J. A., Ramos-Gomez, F., et al. (2017). Dental caries. Nat. Rev. Dis. Primers. 3:17030. doi: 10.1038/nrdp.2017.30
Plaine, A., Walker, L., Da Costa, G., Mora-Montes, H. M., Mckinnon, A., Gow, N. A., et al. (2008). Functional analysis of Candida albicans GPI-anchored proteins: roles in cell wall integrity and caspofungin sensitivity. Fungal Genet. Biol. 45, 1404–1414. doi: 10.1016/j.fgb.2008.08.003
Rainey, K., Michalek, S. M., Wen, Z. T., and Wu, H. (2019). Glycosyltransferase-mediated biofilm matrix dynamics and virulence of Streptococcus mutans. Appl. Environ. Microbiol. 85, e02247–e0224718. doi: 10.1128/aem.02247-18
Raja, M., Hannan, A., and Ali, K. (2010). Association of oral candidal carriage with dental caries in children. Caries Res. 44, 272–276. doi: 10.1159/000314675
Santi, E., Facchin, G., Faccio, R., Barroso, R. P., Costa-Filho, A. J., Borthagaray, G., et al. (2016). Antimicrobial evaluation of new metallic complexes with xylitol active against P. aeruginosa and C. albicans: MIC determination, post-agent effect and Zn-uptake. J. Inorg. Biochem. 155, 67–75. doi: 10.1016/j.jinorgbio.2015.11.014
Söderling, E., and Pienihäkkinen, K. (2020). Effects of xylitol and erythritol consumption on mutans streptococci and the oral microbiota: a systematic review. Acta Odontol. Scand. 78, 599–608. doi: 10.1080/00016357.2020.1788721
Sudbery, P., Gow, N., and Berman, J. (2004). The distinct morphogenic states of Candida albicans. Trends Microbiol. 12, 317–324. doi: 10.1016/j.tim.2004.05.008
Sztajer, H., Szafranski, S. P., Tomasch, J., Reck, M., Nimtz, M., Rohde, M., et al. (2014). Cross-feeding and interkingdom communication in dual-species biofilms of Streptococcus mutans and Candida albicans. ISME J. 8, 2256–2271. doi: 10.1038/ismej.2014.73
Takahashi, N., and Nyvad, B. (2011). The role of bacteria in the caries process: ecological perspectives. J. Dent. Res. 90, 294–303. doi: 10.1177/0022034510379602
Tu, Y., Zhou, Z., Shu, C., Zhou, Y., and Zhou, X. (2022). The crosstalk between saliva bacteria and fungi in early childhood caries. Front. Cell. Infect. Microbiol. 12:845738. doi: 10.3389/fcimb.2022.845738
Venkitaraman, A. R., Vacca-Smith, A. M., Kopec, L. K., and Bowen, W. H. (1995). Characterization of glucosyltransferaseB, GtfC, and GtfD in solution and on the surface of hydroxyapatite. J. Dent. Res. 74, 1695–1701. doi: 10.1177/00220345950740101101
Wang, L., Wang, S., and Li, W. (2012). RSeQC: quality control of RNA-seq experiments. Bioinformatics 28, 2184–2185. doi: 10.1093/bioinformatics/bts356
Xiao, J., Grier, A., Faustoferri, R. C., Alzoubi, S., Gill, A. L., Feng, C., et al. (2018a). Association between Oral Candida and Bacteriome in children with severe ECC. J. Dent. Res. 97, 1468–1476. doi: 10.1177/0022034518790941
Xiao, J., Huang, X., Alkhers, N., Alzamil, H., Alzoubi, S., Wu, T. T., et al. (2018b). Candida albicans and early childhood caries: a systematic review and meta-analysis. Caries Res. 52, 102–112. doi: 10.1159/000481833
Xie, Y., Liu, X., and Zhou, P. (2020). In vitro antifungal effects of Berberine against Candida spp. in planktonic and biofilm conditions. Drug Des. Devel. Ther. 14, 87–101. doi: 10.2147/dddt.S230857
Keywords: Streptococcus mutans, Candida albicans, stevioside, dental caries, RNA sequencing
Citation: Guo M, Yang K, Zhou Z, Chen Y, Zhou Z, Chen P, Huang R and Wang X (2023) Inhibitory effects of Stevioside on Streptococcus mutans and Candida albicans dual-species biofilm. Front. Microbiol. 14:1128668. doi: 10.3389/fmicb.2023.1128668
Edited by:
Santosh Pandit, Chalmers University of Technology, SwedenReviewed by:
Richard L. Gregory, Purdue University Indianapolis, United StatesPriya Arumugam, The Pennsylvania State University, United States
Copyright © 2023 Guo, Yang, Zhou, Chen, Zhou, Chen, Huang and Wang. This is an open-access article distributed under the terms of the Creative Commons Attribution License (CC BY). The use, distribution or reproduction in other forums is permitted, provided the original author(s) and the copyright owner(s) are credited and that the original publication in this journal is cited, in accordance with accepted academic practice. No use, distribution or reproduction is permitted which does not comply with these terms.
*Correspondence: Ruizhe Huang, huangrzh@xjtu.edu.cn; Xiaojing Wang, 1092487765@qq.com
†These authors have contributed equally to this work and share first authorship