- 1Qingdao Key Laboratory of Specialty Plant Germplasm Innovation and Utilization in Saline Soils of Coastal Beach, Qingdao Agricultural University, Qingdao, China
- 2Key Laboratory of National Forestry and Grassland Administration on Grassland Resources and Ecology in the Yellow River Delta, Qingdao Agricultural University, Qingdao, China
- 3College of Animal Science and Technology, Southwest University, Chongqing, China
- 4College of Grassland Science, Qingdao Agricultural University, Qingdao, China
- 5Chongqing Jiangxiaobai Farm Co., Ltd., Chongqing, China
Introduction: Cyanogenic glycosides (CNglcs) are bioactive plant products involving in plant defense against herbivores by virtue of their abilities to release toxic hydrogen cyanide (HCN). Aspergillus niger has been shown to be effective in producing β-glucosidase, which could degrade CNglcs. However, whether A. niger could remove CNglcs under ensiling conditions is still unknown.
Methods: In this study, we first investigated the HCN contents in ratooning sorghums for two years, then the sorghums were ensiled with or without the addition of A. niger.
Results: Two years’ investigation indicated that the contents of HCN in fresh ratooning sorghum were larger than 801 mg/kg FW (fresh weight), which could not be reduced by silage fermentation under safety threshold (200 mg/kg FW). A. niger could produce β-glucosidase over a range of pH and temperature, which degraded the CNglcs and removed the hydrogen cyanide (HCN) at early days of ratooning sorghum fermentation. The addition of A. niger (2.56 × 107 CFU/ml) altered the microbial community, increased bacterial diversity, improved the nutritive qualities, and reduced the HCN contents in ensiled ratooning sorghum lower than 100 mg/kg FW after 60 days of fermentation. Overall, the addition of 150 ml A. niger + 50 ml sterile water per 3 kg silage could efficiently remove CNglcs from ratooning sorghum silage.
Conclusion: In conclusion, A. niger could produce β-glucosidase which degraded the CNglcs during the early days of fermentation, benefiting the ensiling process and improving the utilization of ratooning sorghum.
1. Introduction
Cyanogenic glycosides (CNglcs) are bioactive plant products derived from amino acids (Cowan et al., 2021), which involve in plant defense against herbivores by virtue of their abilities to release toxic hydrogen cyanide (HCN) upon tissue disruption (Picmanova et al., 2015). These compounds are widely distributed in plants such as sorghum, cassava, lima beans, bamboo shoots, spinach, and fruits such as drupes, pears and pip (Codex Committee on Contaminants in Foods, 2008). Though CNglcs are not toxic to plant itself, its release of HCN may lead to the risk of acute cyanide poisoning in humans or livestock (Moller, 2010). Overall, 200 mg/kg fresh weight (FW) of HCN in forage is regarded as the safety threshold for livestock (Kumari et al., 2021), and up to 50% of the ingested CNglcs may result in human cyanide exposure (Carlsson et al., 1999). Therefore, release of HCN from CNglcs before they are delivered into animal gut will greatly reduce toxic risk.
Structurally, CNglcs includes a core carbon attached to a CN moiety and two substituent groups attached to a sugar by a glycosidic bond. Due to the difference of the substituent groups, the CNglcs differ among plant species, such as Duhrrin in sorghum, Linamarin in cassava, Prunasin in ferns, and Taxiphyllin in bamboo shoots (Cressey and Reeve, 2019). Irrespective of CNglcs structures, release of HCN from CNglcs can occur through enzymatic hydrolysis by β-glycosidase, where the glycoside is enzymatically converted to the corresponding cyanohydrin, followed by spontaneously decomposing to form HCN (Wanasundara et al., 1993). Though β-glycosidases are widely distributed in seeds and microbes, their activities are depending upon the concentrations of substrates and the environmental conditions (Briante et al., 2001; Michlmayr et al., 2012). Therefore, suitable food process conditions might benefit the activities of β-glycosidases, improving the release of HCN.
Ensiling is one of the important methods widely used in forage production (Yang et al., 2019). Previous study indicated that ensiling ground pods of Acacia sieberiana for 45 days removed 86.1% of CNglcs (reaching the non-toxic levels for livestock) (Ngwa et al., 2004). However, in another study, although the HCN contents of cassava roots was reduced from 506.8 mg/kg (FW) to 295.8 mg/kg (FW) by silage, the final HCN contents in silage was still above the safety threshold (200 mg/kg FW) (Chander et al., 2004). This implied that ensiling benefited the release of HCN but the activities of β-glycosidases might not be high enough to remove the CNglcs under safety level. The ensiling is processed under anaerobic conditions, with the pH value decreasing with the extension of fermentation, which might limit the enzymatic activity of β-glycosidase as well as the activity of microorganisms producing β-glycosidases. Therefore, detailed exploration of the enzymatic activity of β-glycosidase under various environments is needed.
Aspergillus niger is a haploid filamentous fungus widely distributed in various environments (Yu et al., 2021). As an aerobic microorganism, A. niger is the source of several bioactive compounds and industrial enzymes, as well as additives for waste management and biotransformations (Hasanuzzaman et al., 2014). Interestingly, inoculation of A. niger strain had positive effect on the removal of CNglcs from rubber seed meal by fermentation (Liu et al., 2010). This implied that A. niger might be used in silage fermentation to degrade the CNglcs. However, whether the low pH levels and anaerobic conditions of silage will benefit CNglcs removal and how the A. niger remove the CNglcs are still not clear.
To fill the gap between the removal rate of CNglcs and the addition of A. niger in silage production, we evaluated the changes of HCN contents in ensiled ratooning sorghum with the addition of different concentrations of A. niger. Ratooning sorghums regrow from the dormant axillary buds on the sorghum stem nodes and tillers from the stem base nodes, or sprouts branches from the upper part of the plant. For some sorghum varieties, when the straws are harvested, the sorghum will regrow, and even can be harvested for cereal during late season when the environmental conditions are suitable. However, under most conditions, the temperature is not suitable for growth, or the seeding date of the following crop cannot guarantee the maturation of sorghum. Therefore, the ratooning sorghums have to be harvested in their vegetative stages or in their blossom or filling stages, which has the potential to be used as fodder or silage. Currently, these regrown sorghums are directly returned to soils (Seglah et al., 2020), or used as cooking fuel. Due to the risk of HCN poison, the ratooning sorghum are seldomly used as feeds. For some cultivars of sweet sorghum (Mule 8,000), the HCN contents reached 482 mg/kg at early growing stages (Shehab et al., 2020). Drought increased the foliar HCN contents in sorghum from 709 mg/kg in well-watered plants to 1,155 mg/kg in drought-stressed plants (Gleadow et al., 2016). Therefore, reducing the HCN contents in sorghum silage might improve their utilizations. We hypothesized that adding A. niger to silage could remove the CNglcs to safety threshold. The following three research aspects were mainly addressed, (1) to prove the efficiency of A. niger addition in removing CNglcs; (2) to elucidate the mechanism of A. niger in removing CNglcs; and (3) to evaluate the potential utilization of ratooning sorghum as safe feeds.
2. Materials and methods
2.1. Experimental site and materials
The experiment was conducted at Jiangxiaobai Red Sticky Sorghum Planting Demonstration Base (29°07′N, 106°16′E), located in Chongqing, China. The area has a mean temperature of 17.9°C, and a mean annual rainfall of 1,100 mm during the last 30 years (Chongqing Meteorological Bureau). The soil was a neutral yellow soil with a pH 7.5, alkali-dispelled nitrogen 96.15 mg/kg, available phosphorus (Olsen-P) 17.83 mg/kg, and available potassium 90.15 mg/kg.
The red sticky Sorghum bicolor cv. Chuannuoliang 2 is the principal variety planting in this base for Baijiu (distilled liquor) production. Sorghum-Canola rotation system is used in this base. Generally, the sorghum will be sown in May after the canola is harvested, and be harvested in August, then the canola will be sown in October. From August to October, there is about 2 months’ fallow period, which is suitable for the regrowth of the harvested sorghum, called ratooning sorghum. Currently, the ratooning sorghum is chopped and returned to soils or discarded. In this study, on 2th October, 2020 and 10th October, 2021, the ratooning sorghum at their heading and flowering stage were harvested for whole plant silage (above 5 cm from the ground). These ratooning sorghums have the potential to be used as fodder or silage.
A. niger was bought from Beijing Beina Chuanglian Biotechnology Research Institute. Before used as additives, the A. niger was cultured on Potato Dextrose Agar medium (PDA) at 28°C for 72 h, then, the activated A. niger was inoculated into Potato Dextrose Broph liquid medium (PDB) at 32°C for 3 days at 170 ~ 180 r/min (Wang et al., 2007). When the concentration of A. niger solution reached 2.56 × 107 CFU/mL, it was evenly sprayed on the silage as additives.
2.2. Determination of agronomic characters
One day before the harvest of the ratooning sorghum, ten sorghum plants were randomly sampled from the fields to measure the agronomic characters, including the plant height, stem diameter, stem leaf ratio, leaf number, and fresh plant yields.
2.3. Experimental design
In experimental one, the harvested ratooning sorghums (2020) were kept under room temperature for 12 h until the water contents reached ca. 78%. Then, the whole plants were chopped into ca. 2 cm length, thoroughly mixed, and ensiled without additives (Control), with adding 1 g/kg of cellulase, and with adding 1 g/kg of xylanase. The samples were packed into polyethylene plastic bags (18 cm × 26 cm), vacuum sealed, and stored at room temperature (~25°C) for 7, 15, 30 and 60 days. Each silage bag contained 500 g samples and each treatment replicated three times.
In experimental two, the harvested ratooning sorghums (2021) were used. The whole plants were chopped and ensiled with 200 ml sterile water / 3 kg (Control), with 50 ml A. niger + 150 ml sterile water / 3 kg (AN50), with 100 ml A. niger + 100 ml sterile water / 3 kg (AN100), with 150 ml A. niger + 50 ml sterile water / 3 kg (AN150), and with 200 ml A. niger/3 kg (AN200). The silage bags were placed at room temperature (~ 25°C) for 48 h before sealing, to provide oxygen for A. niger propagation (Zhou et al., 2019). The starting time of the fermentation was calculated at the sealing. The other ensiling procedures were same as the experimental one.
In experimental three, the activated A. niger was inoculated into PDB at 32°C for 3 days at 170 ~ 180 r/min. When the concentration of A. niger solution reached 2.56 × 107 CFU/mL, the A. niger was added into fermentation medium. A single-factor experiment was designed with different inoculation concentrations of A. niger (50, 100, 150, and 200 mL of A. niger per 200 mL sterile water), pH levels (3.0–9.0), and temperatures (20, 25, 30, 35 and 40°C). Each treatment replicated four times.
2.4. HCN content determination in fresh ratooning sorghum and silage
Ten-gram dry samples were taken and homogenized in 500 mL distilling bottle with 200 mL distilled water, 20 mL of zinc acetate and 2 g of tartaric acid. The lower end of the condenser tube was inserted into a 100 mL conical flask with 20 g/ml sodium hydroxide solution, to collect the released HCN gas. This was done for three times until the volume of distillate reached ca. 250 mL. Then, 10 mL distillate were taken and 1 mL of 10 g/mL sodium hydroxide solution and a drop of phenolphthalein indicator were added, and adjusted with acetic acid solution until the red color fades. Next, 5 mL phosphoric buffer were added and bathed at 37°C for 10 min, and then 0.25 mL chloramine T solutions were added and stand for 5 min. Finally, 5 mL isonicotinic-pyrazolone solution were added to a final volume of 25 mL, water bathed at 37°C for 40 min, and then the OD value was measured at 638 nm (Dominguez et al., 2002).
2.5. Activities of β-glucosidase produced by A. niger
After 0, 12, 24, 48, 72, and 96 h of cultivation, the fermentation medium was centrifuged at 3000 r/min for 10 min to obtain the supernatant crude enzyme solution. Then, 0.1 mL of the enzyme solution and 0.9 mL of 0.2 mol/L Na2HPO4–0.1 mol/L citric acid buffer solution with pH 4.5 were added, and water bathed at 50°C for 10 min. Next, 1 mL of 5 mmol/L P-NPG solution preheated for 10 min was added. Subsequently, 1 mL of 1 mol/L Na2CO3 solution was added to stop the reaction. Finally, the extract was allowed to stand for 5 min to measure the OD value at 400 nm (Li et al., 1998). The activity of β-glucosidase was calculated as follows:
Note: U is enzyme activity (u/mL); 10 is reaction time; N is dilution multiple of original enzyme solution; 0.1 is take 0.1 ml of enzyme solution for reaction; C is corresponded to the value on the p-nitrophenol optical density curve.
2.6. Chemical composition and fermentation characteristics analysis
To analyze fermentation characteristics of silages, 20 g wet samples were taken and homogenized in 180 mL sterile water at 4°C for 24 h (Arshad et al., 2020). The mixture was then filtered through rapid qualitative filter paper (Fushun Civil Affairs Filter Paper Factory, Liaoning, China) and centrifuged at 3000 r/min for 10 min. The supernatant was collected for the following analysis. The pH was measured by pH meter (pHS-3C, Shanghai Instrument & Electronics Science Instrument Co., Ltd., Shanghai, China). The ammonia nitrogen (AN, g/kg TN) content was determined by using the phenol-hypochlorite colorimetric method (Xia et al., 2018). The contents of lactic acid (LA), acetic acid (AA), propionic acid (PA) and butylic acid (BA) were analyzed on gas chromatography (Fuli 9790II, Zhejiang Fuli Analytical Instrument Co., Ltd., Wenzhou, China.) (Ding et al., 2019).
The rest silage samples were dried at 105°C for 30 min and then at 65°C for 48 h to measure the DM content. Prior to the chemical analysis, the dried samples were ground into powder with grinder through a 2 mm screen. Specifically, the water-soluble carbohydrate (WSC) was extracted by using anhydrous ethanol and analyzed using the anthrone method. Total nitrogen (TN), ether extract (EE) and crude ash (Ash) were determined according to the procedure described by Fountoulakis et al. (2017), and the CP was calculated as TN × 6.25. The contents of NDF (Neutral Detergent Fiber) and ADF (Acid Detergent Fiber) were determined by the methods of Van Soest (Chen et al., 2021).
2.7. Microbial community of silages
Total genomic DNA of surface bacteria of fresh and ensiled sorghum fermented for 0, 7, 15, 30, and 60 days were extracted using a DNA isolation kit. The DNA quality and quantity were assessed by the NanoDrop2000. Afterwards, DNA samples were stored at-80°C until used. The V3-V4 region of the bacterial 16S rRNA gene was amplified with the common primer pair (forward primer 799F, 5′-AACMGGATTAGATACCCKG-3′ and reverse primer 1193R, 5′-ACGTCATCCCCACCTTCC-3′) combined with adapter and barcode sequences. The PCR amplification was performed as follows: 4 μL buffer, 0.4 μL Q5 High-Fidelity DNA Polymerase, 10 μL High GC Enhancer, 2 μL dNTP, 0.8 μM of each primer, 10 ng genome DNA, and sterilized deionized water were mixed to make the total volume to 20 μL. The fungus ITS gene was amplified with the common primer pair (forward primer ITS1F, 5′-CTTGGTCATTTAGAGGAA GTAA-3′ and reverse primer ITS2R, 5′-GCTGCGTTCTTCATCGATGC-3′) combined with adapter and barcode sequences. The PCR amplification was performed as follows: 10 μL Taq, 0.8 μM of each primer, 10 ng/μL Template DNA, and sterilized deionized water were mixed to make the total volume to 20 μL. Thermal cycling conditions included: an initial denaturation at 95°C for 3 min, followed by 27 cycles (95°C for 30 s, 50°C for 30 s and 72°C for 45 s) and a final extension at 72°C for 10 min. The original sequences were processed by Meiji Bio Cloud Platform. The RNA-Seq reads were deposited and available at the database Sequence Read Archive at NCBI (PRJNA921848).
2.8. Data analysis
The data were expressed as “mean ± standard deviation.” One-way ANOVA was applied to analyze the influence of additives on HCN contents and the parameters of silage quality using SPSS 23.0 (SPSS Inc., Chicago, IL, USA). Significance was tested using Tukey’s multiple range test at p < 0.05. The figures were made using origin 2021 (Northampton, MA, USA). After comparison with the NCBI 16S rRNA database (classified at a bootstrap threshold of 0.8) using RDP classifier software, sample ordination based on beta diversity was examined using principal coordinate analysis (PCoA) with phylogeny-based (UniFrac) unweighted and weighted distance. Heat map analysis was used to confirm the relationship between the microbial species, fermentation quality and HCN contents in ratooning sorghum silage. The high throughput sequencing data were analyzed on the online platform of Majorbio Cloud Platform.1
3. Results
3.1. Agronomic characters, nutritional quality and HCN content of ratooning sorghum
After two months’ regrowth, the ratooning sorghums were in their heading and flowering stages, with plant height reaching 157.56 cm, stem diameter 1.77 cm, and stem/leaf ratio 4.45 in year 2020 (Table 1). Similar results were also observed in year 2021. The yield on DM base reached 13.5 t/ha in 2020 and 12.78 t/ha in 2021 for ratooning sorghum. The contents of CP reached 6.27% in 2020 and 7.0% in 2021, and a higher content of WSC was also observed in ratooning sorghum. However, the contents of HCN in ratooning sorghum reached 892 mg/kg FW in 2020 and 801 mg/kg FW in 2021.
3.2. Effects of silage on HCN content of ratooning sorghum
In order to evaluate the effects of ensiling on the removal of CNglcs, the ratooning sorghum was ensiled with cellulase or xylanase. The results showed that the contents of HCN decreased significantly with the prolongation of fermentation (p < 0.05), and the lowest was observed 60 days after ensiling (Figure 1A). Overall, the contents of HCN reduced from 892 mg/kg FW at the beginning to 407 mg/kg FW 60 days after ensiling. Interestingly, lower contents of HCN were observed for silage ensiled with the additions of cellulase or xylanase (Figure 1B). However, the HCN contents in those silages were still above 200 mg/kg FW 60 days after ensiling, higher than the safety level. An increase of HCN removal rate was also observed with the prolongation of fermentation; however, the removal rate became slower 30 days after ensiling.
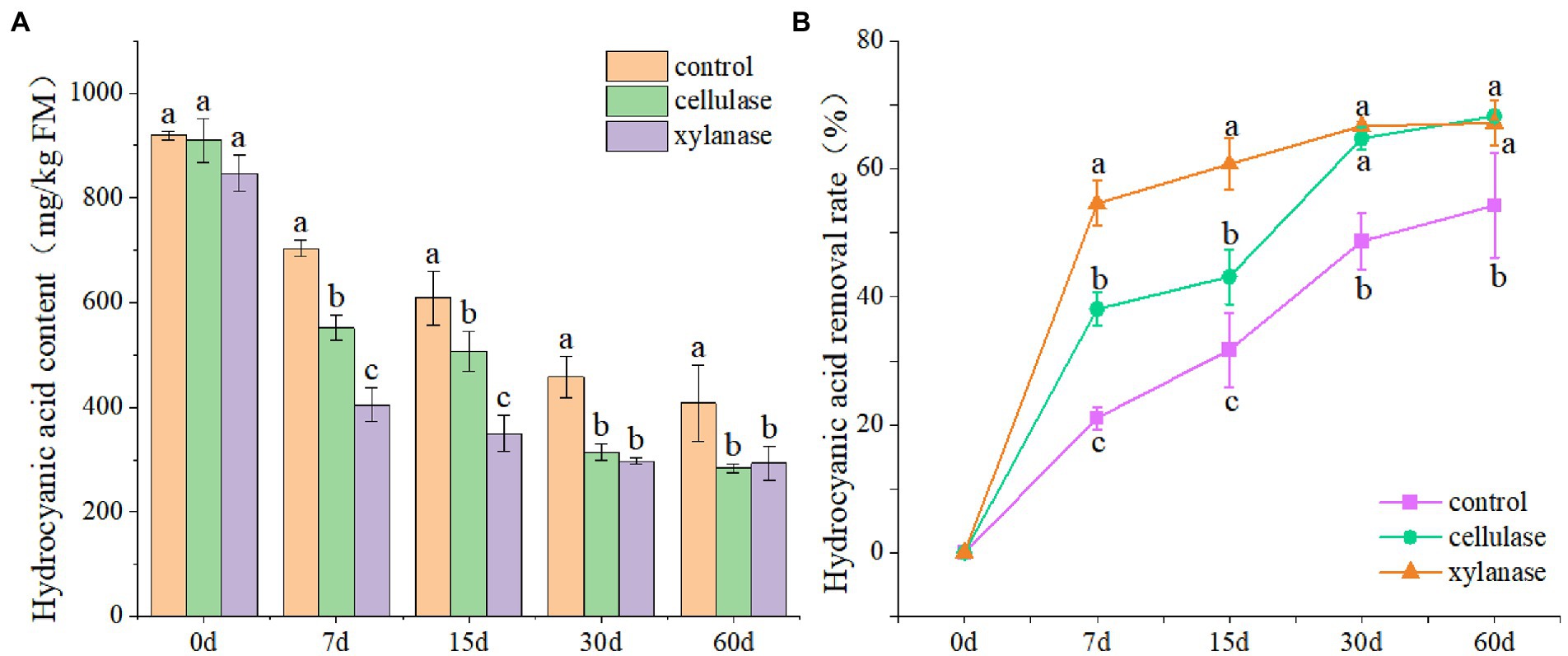
Figure 1. Hydrogen cyanide contents (A) and removal rate (B) of ratooning sorghum ensiled with different additives. CK, control; C, cellulase; X, xylanase. Different lowercase letter above the data bar represented significance between concentrations of A. niger within same fermentation time at p < 0.05.
3.3. Effects of A. niger on HCN remover rate
In this study, the addition of A. niger significantly reduced the contents of HCN in ensiled ratooning sorghum (Figure 2A). The removal rate of HCN 60 days after ensiling reached 87.83–93.63% for silages ensiled with different concentrations of A. niger. However, for silage without A. niger, the removal rate was only 75.83% (Figure 2B). After 60 days of ensiling, the HCN contents in silage ensiled with different concentrations of A. niger were all lower than 120 mg/kg.
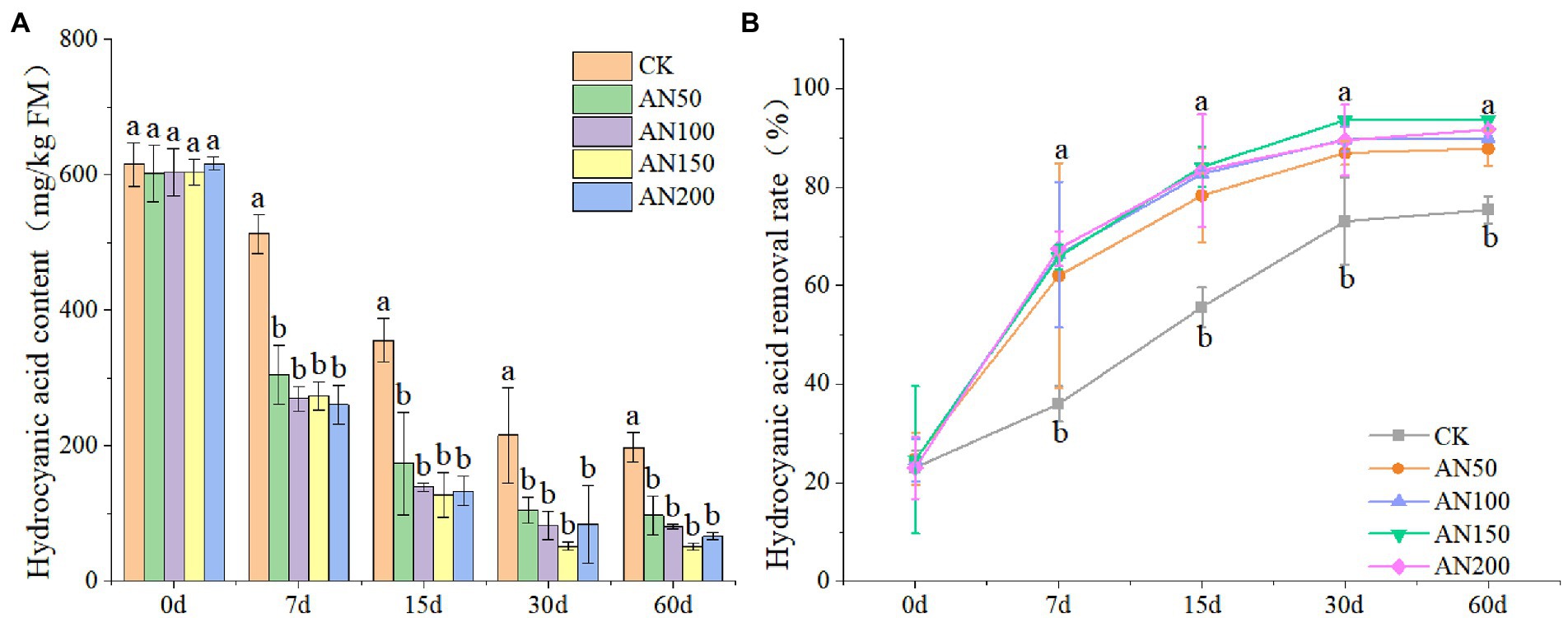
Figure 2. Hydrogen cyanide contents (A) and removal rates (B) of ratooning sorghum ensiled with different concentrations of A. niger. CK: 200 mL of sterile water per 3 kg of silage; AN50: 50 mL A. niger + 150 mL sterile water per 3 kg of silage; AN100:100 mL A. niger + 100 mL sterile water per 3 kg of silage; AN150: 150 mL A. niger + 50 ml sterile water per 3 kg of silage; AN200: 200 mL A. niger per 3 kg of silage. Different lowercase letter above the data bar represented significance between concentrations of A. niger within same fermentation time at p < 0.05.
Though the HCN removal rate increased with the increase of fermentation time, the highest HCN amount removed from silage was observed at Day 7 for silages added with A. niger. For example, the HCN removal rates were all larger than 64% for silages added with A. niger, which were significantly higher than that of silage without A. niger (35.99%). The HCN removal rate did not increase with the increase of the concentrations of A. niger. Among the concentrations of A. niger, 150 ml A. niger + 50 ml sterile water per 3 kg was the optimal concentration for ratooning sorghum, with HCN removal rate reaching 93.63%.
3.4. Mechanisms of A. niger in degrading CNglcs
In order to prove whether A. niger could produce β-glucosidase under lower pH levels in ensiled ratooning sorghum, the β-glucosidase production characteristics of A. niger were measured under different inoculation amounts, pH levels and temperatures (Figure 3). The results indicated that β-glucosidase production increased with the increased inoculation amounts (Figure 3B). With the prolongation of culturing, β-glucosidase production increased first and reached the highest at 12 h, followed by a decrease at 24 h, and then kept stable from 24 to 96 h. A. niger could produce β-glucosidase at all tested pH levels from 3 to 9, suggesting that A. niger had the ability to produce β-glucosidase under the conditions of low silage pH levels (Figure 3A). However, the efficiency of β-glucosidase production depended upon pH levels, with relatively higher productions were obtained at 7.0. Interestingly, A. niger were more adaptable to acidic environments, and the enzyme production significantly decreased in alkaline environments, with the lowest productions were observed when the pH level was larger than 8. Considering the temperatures affecting silage fermentation, the β-glucosidase productions were measured under temperatures ranging from 20 to 40°C (Figure 3C). However, no significant difference in enzyme production could be observed at different temperatures.
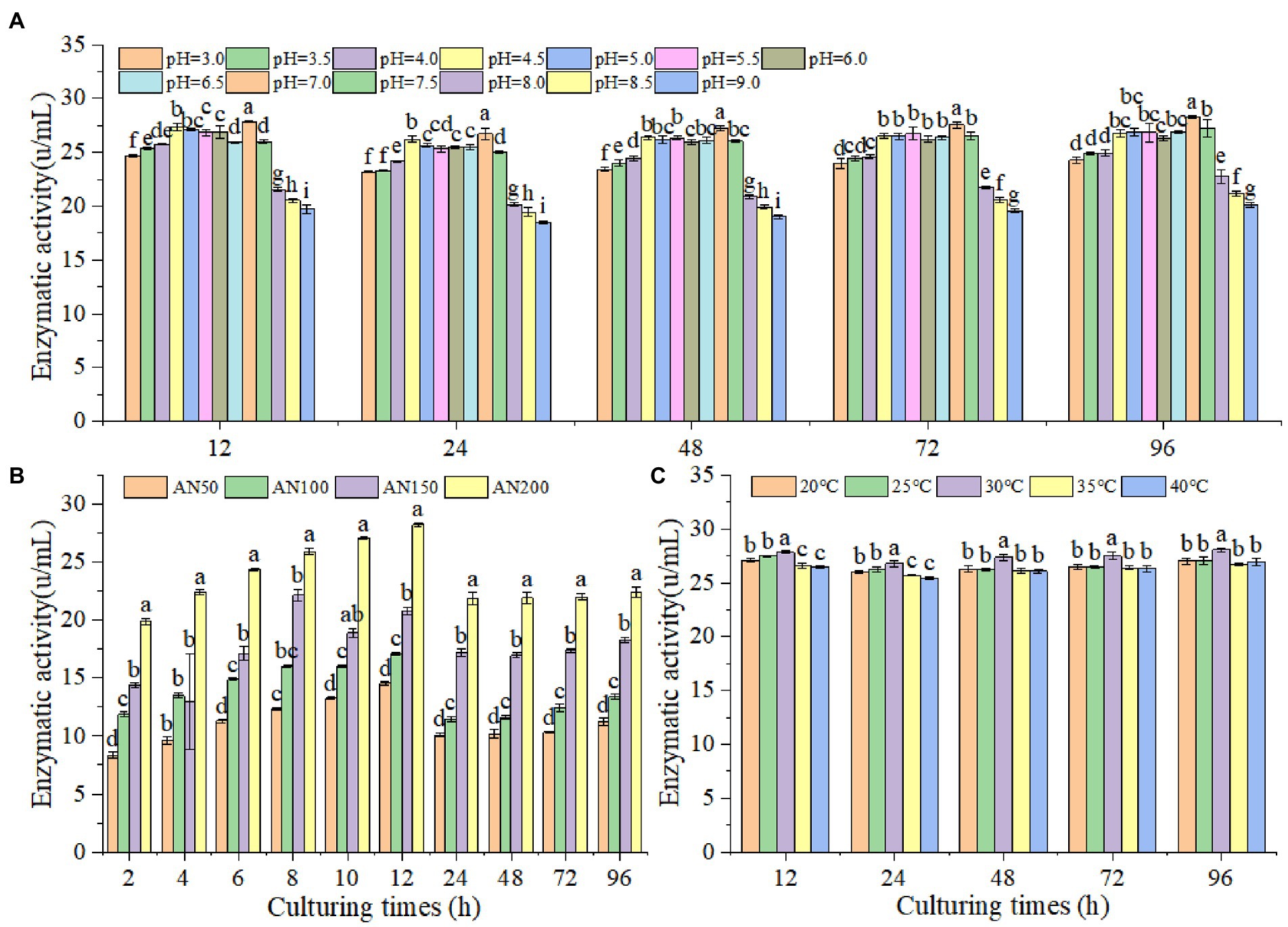
Figure 3. Activities of β-glucosidase produced by A. niger at different pH level (A), inoculation amounts (B) and temperature (C). Different lowercase letter above the data bar represented significance between pH values (concentrations or temperatures) within same culturing time at p < 0.05.
3.5. Nutrient and fermentation qualities of ensiled ratooning sorghum
To check whether A. niger addition for CNglcs degradation was also efficient in obtaining higher quality silages, the nutrient and fermentation qualities of ensiled ratooning sorghum were also evaluated (Figures 4, 5). Compared with silage without A. niger, the CP contents in silage with A. niger increased significantly (p < 0.05) (Figure 4B), whereas the contents of DM and NDF showed a decreased trend (p > 0.05) (Figures 4A,D), with the variations of other nutrients depending upon fermentation time and A. niger concentrations (Figure 4). For example, EE contents reduced when the A. niger concentrations increased above 150 A. niger + 50 ml sterile water per 3 kg after15 days of fermentation (p < 0.05) (Figure 4C). Overall, the DM, EE and NDF decreased, whereas the CP increased with the prolongation of fermentation. Among the different concentrations of A. niger, higher CP content and lower NDF and ADF contents were observed for silage ensiled with AN100 and AN150. Meanwhile, the pH and AN/TN in silage with A. niger was lower whereas the LA concentration was higher than those without adding A. niger (p < 0.05) (Figure 5). No significant difference was observed for the concentrations of AA and PA in silages.
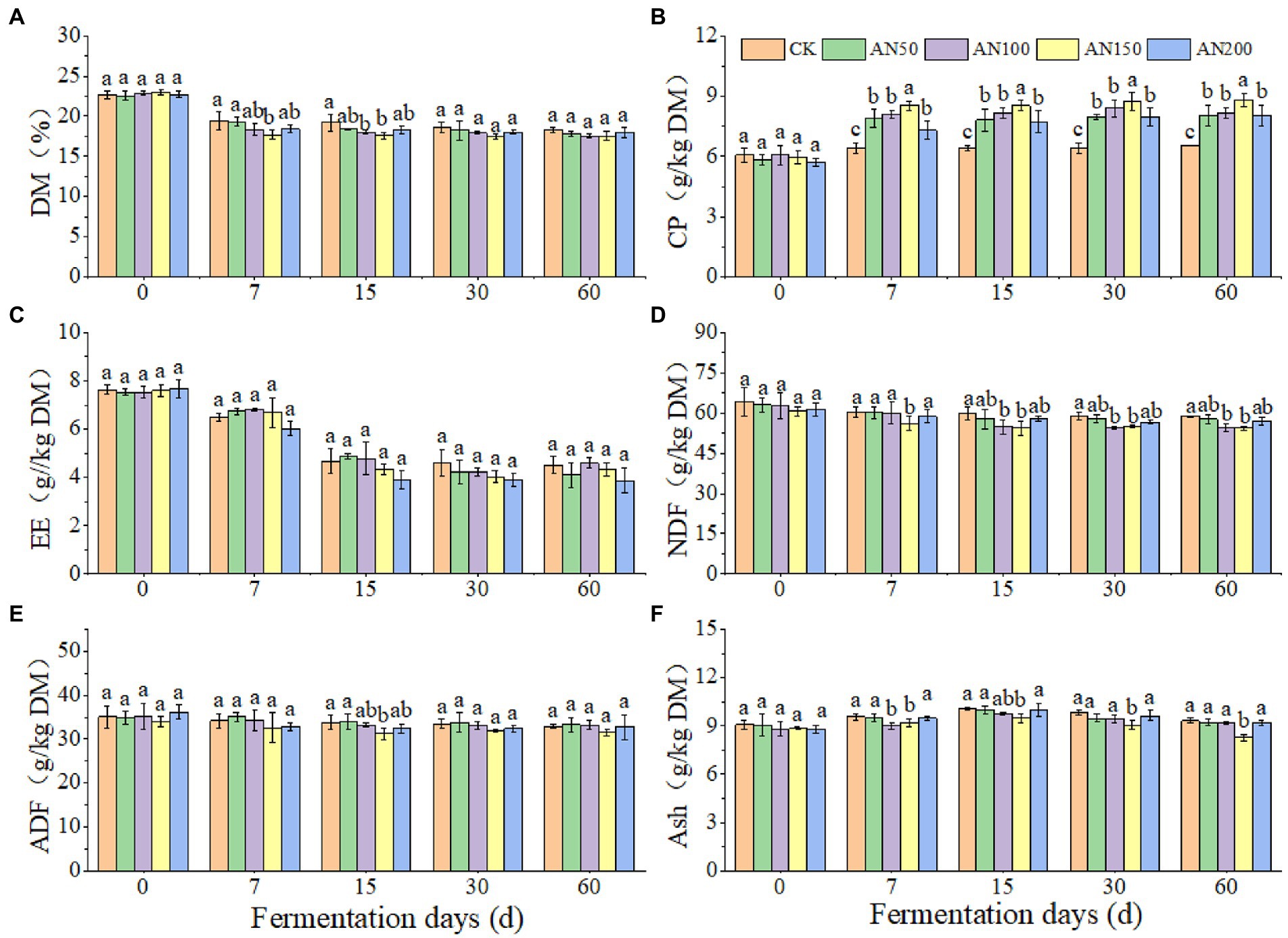
Figure 4. Nutrients of ratooning sorghum ensiled with different concentrations of A. niger. (A) dry matter (DM); (B), crude protein (CP); (C), ether extract (EE); (D), neutral detergent fiber (NDF); (E), acid detergent fiber (ADF); (F), ash. CK: 200 mL of sterile water per 3 kg of silage; AN50: 50 mL A. niger. + 150 mL sterile water per 3 kg of silage; AN100:100 mL A. niger. + 100 mL sterile water per 3 kg of silage; AN150: 150 mL A. niger. + 50 mL sterile water per 3 kg of silage; AN200: 200 mL A. niger. per 3 kg of silage. Different lowercase letter above the data bar represented significance between concentrations of A. niger. within same fermentation time at p < 0.05.
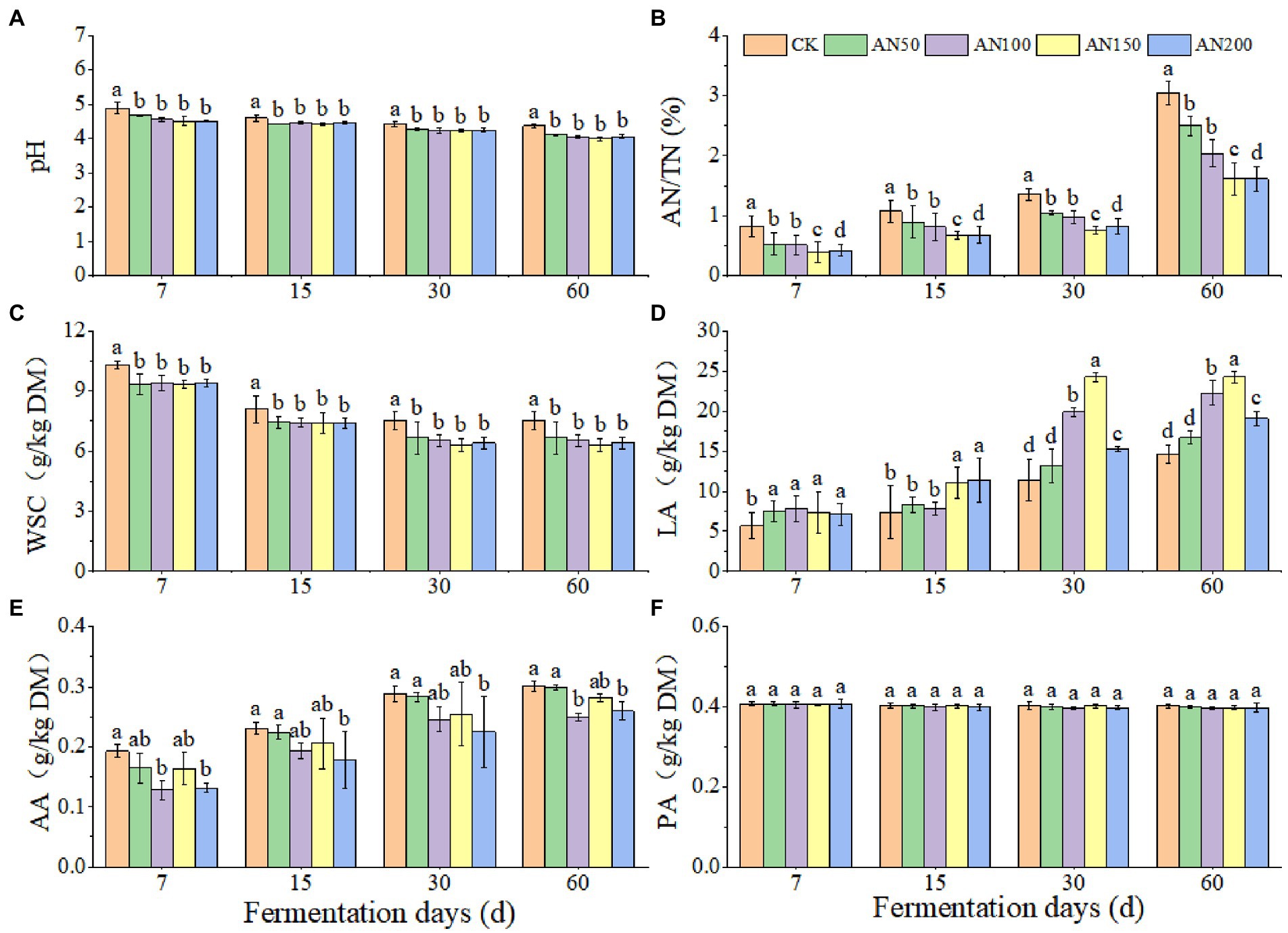
Figure 5. Silage quality of ratooning sorghum ensiled with different concentrations of A. niger. (A), pH; (B), ammonia nitrogen (AN); (C), water soluble carbohydrate (WSC); (D), lactic acid (LA); (E), acetic acids (AA); (F), propionic acid (PA). CK: 200 mL of sterile water per 3 kg of silage; AN50: 50 mL A. niger. + 150 mL sterile water per 3 kg of silage; AN100:100 mL A. niger. + 100 mL sterile water per 3 kg of silage; AN150: 150 mL A. niger. + 50 mL sterile water per 3 kg of silage; AN200: 200 mL A. niger. per 3 kg of silage. Different lowercase letter above the data bar represented significance between concentrations of A. niger. within same fermentation time at p < 0.05.
3.6. Bacterial community diversity and compositions in ratooning sorghum silage
Using high-throughput sequencing, the bacterial community was analyzed. The coverage values of all samples were above 99%, indicating that most bacteria were detected. With the prolongation of fermentation, the number of OUT, index of Shannon, ace and chao reduced, while Simpson index increased (Table 2), resulting in a decrease of the bacterial biodiversity in ratooning sorghum silage. The silage ensiled with A. niger decreased the alpha diversity before ensiling (48 h was given for propagation under oxygen before sealing).
Based on the community dissimilarities calculated by unweighted and weighted UniFrac distances, principal coordinates analysis (PCoA) indicated that the bacterial species had a significant succession with the prolongation of fermentation time (Figures 6A,B). However, the bacterial communities between silages with and without A. niger showed no obvious difference in succession. In PCoA analysis, the microbial diversities were grouped into three clusters, 0 day, 7 and 15 days, and 30 and 60 days. Overall, the microbial diversity of silage at 0 day was obviously separated from other silages.
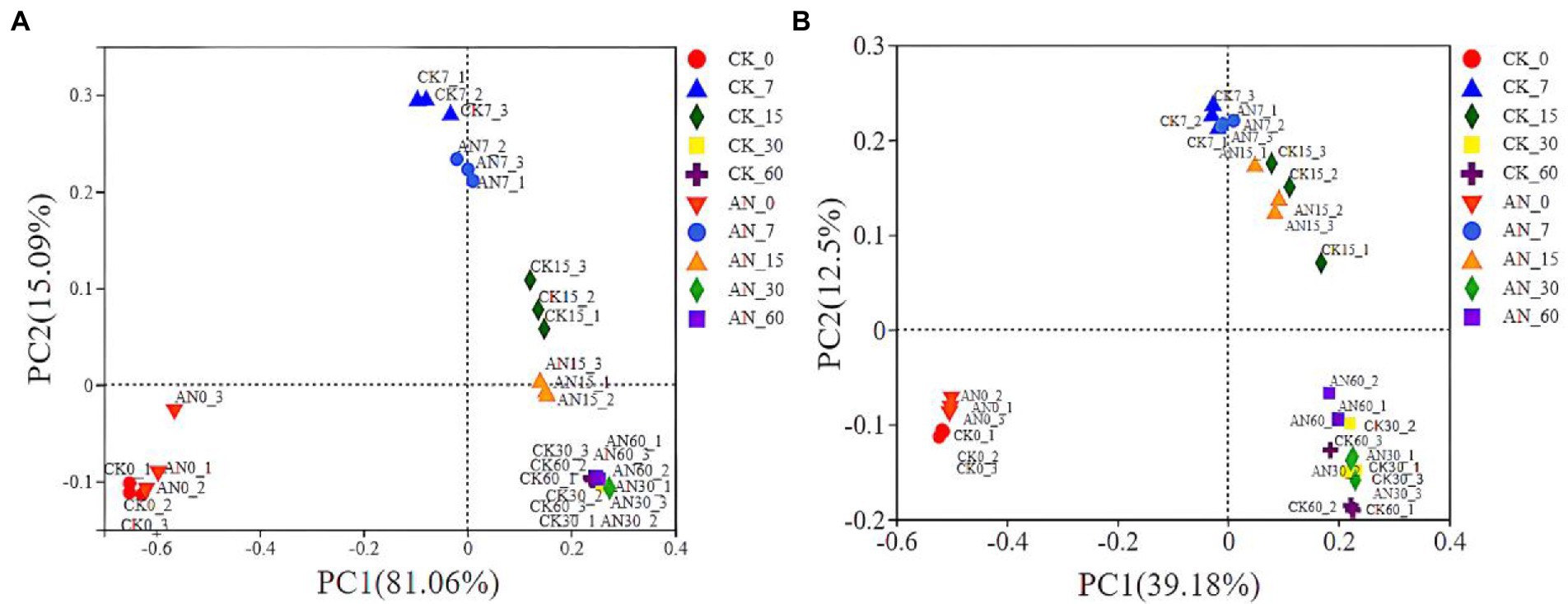
Figure 6. The community dissimilarities calculated by unweighted (A) and weighted (B) UniFrac distances. CK, control; AN, with A. niger.
The silage at day 7 was dominated by Lactobacillus, Lactococcus, Weisseria and Raoultella (Figure 7A). The dominating species were L. plantarum, Lactococcus_lactis, Weissella_paramesenteroides and unclassified_Raoultella (Figure 7B). Higher relative abundance of Lactococcus (39.11–51.67%) and lower relative abundance of Lactobacillus (28.75–30.06%) were observed during early fermentation period. However, with the fermentation continued, the pH declined, Lactobacillus (48.08%) became the dominant bacteria at day 15, which became more active when the pH decreased. From 30 to 60 days of fermentation, Lactobacillus dominated the whole silage process; and the dominant species were L. plantarum, L. brevis, L. lactis, L. unclassified and L. dextrinicus (Figure 7B). Among these LAB species, L. plantarum and L. brevis dominated the microbial community after 60 days of fermentation.
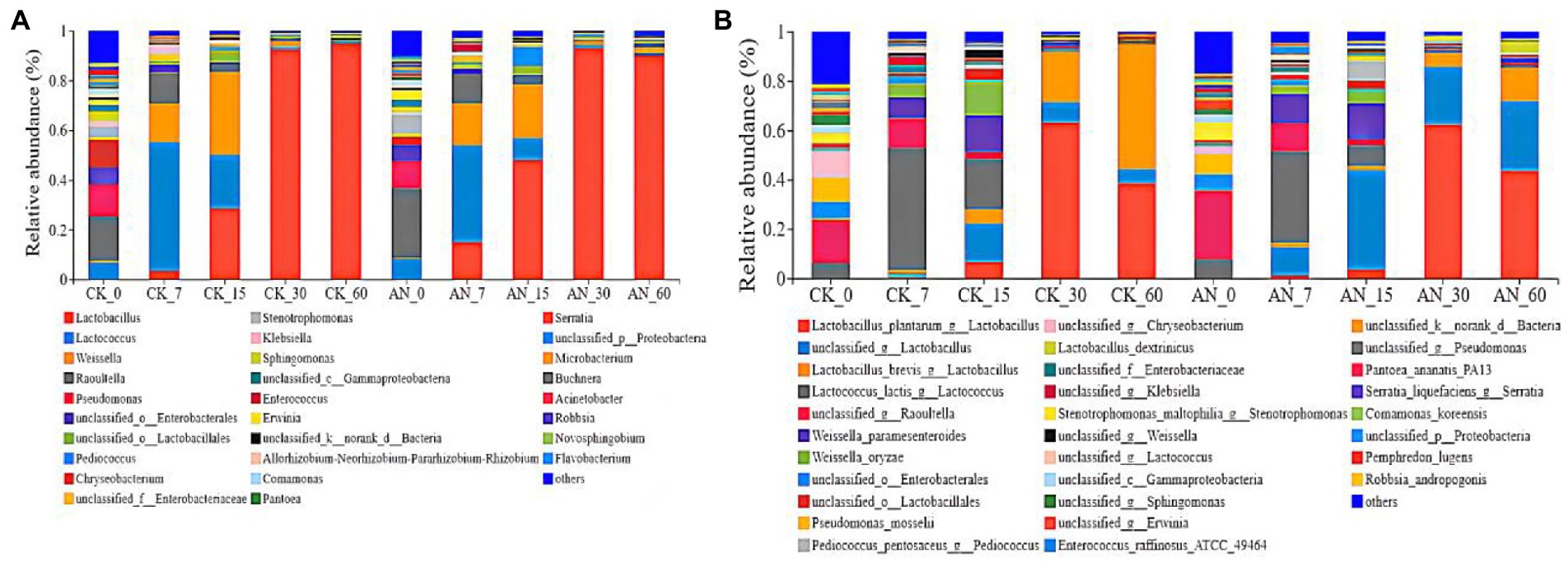
Figure 7. Relative abundances of ratooning sorghum silage bacterial based on genus (A) and species (B). CK, control; AN, with A. niger.
Compared with the control, the silage added with A. niger increased the abundance of L. unclassified while decreased the abundance of L. lactis, Lactococcus and Weissella-oryzae from 7 to 15 days of fermentation. However, the abundance of L.unclassified and Lamellae increased while the abundance of L. brevis and L. dextrinicus decreased from 30 to 60 days of fermentation.
3.7. Correlation analysis between microorganism and fermentation characteristics
The addition of A. niger significantly influenced the correlations between microbe abundance and the silage qualities (Figure 8). For example, the relative abundances of Lactococcus (r = 0.65), Enterobacter (r = 0.58), Enterococcus (r = 0.82), Weigella (r = 0.82) and Lactobacillus (r = 0.81) in silage with A. niger were positively correlated with the pH value (Figure 8A). However, for silage without A. niger, only the relative abundance of Lactococcus (r = 0.68) and Weigella (r = 0.83) were positively correlated with pH value (Figure 8B). For both silages with and without A. niger, AN/TN ratio was negatively correlated with the abundance of Lactococcus (r = −0.65). The HCN contents in silage added with A. niger (r = −0.67) was negatively correlated with the abundance of A. niger (Figure 8D), and the abundance of Lactobacillus and Pediococcus (Figures 8A,B).
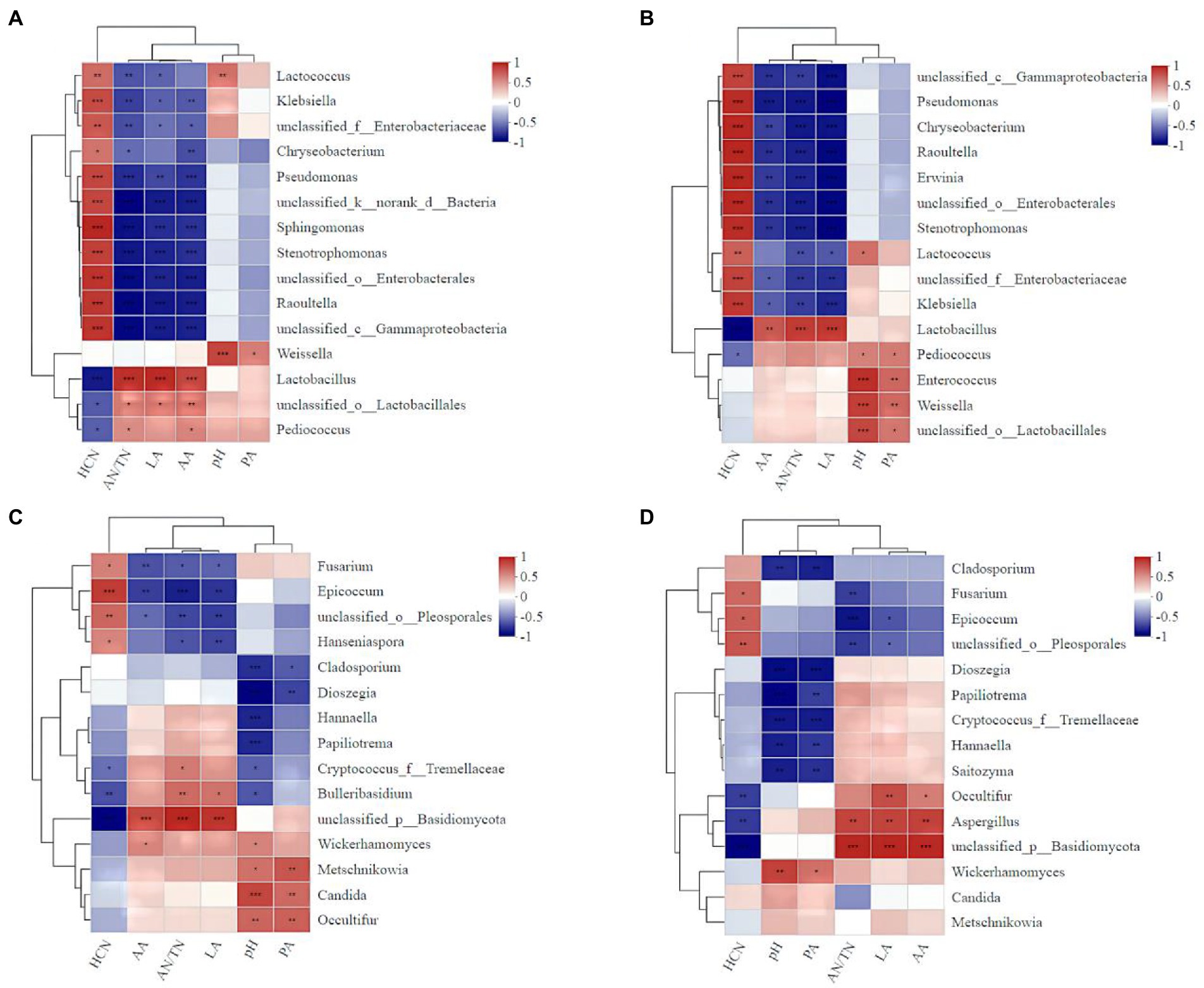
Figure 8. Heat map of the correlations between bacterial (fungal) species and the fermentation characteristics and the HCN content of ratooning sorghum silage. Fermentation characteristics and HCN content are displayed horizontally and the top 15 bacterial species were displayed vertically. The corresponding value of the middle heat map is the Spearman correlation coefficient r, the legend on the right is the color distinction of different r values; r < 0 indicates a negative correlation (blue), r > 0 indicates a negative correlation (red), and ‘*’, ‘**’, and ‘***’ represent p < 0.05, p < 0.01, and p < 0.001, respectively. (A), bacterial of control; (B), bacterial with A. niger addition; (C), fungal of control; (D), fungal with A. niger addition.
4. Discussions
When facing a forage shortage, increasing the forage resources can meet the requirement of increasing demand. Our results indicated that the ratooning sorghum has the potential to be used as forages. When compared with other annual forages, the yield on DM base reached 13.5 t/ha in 2020 and 12.78 t/ha in 2021 for ratooning sorghum, which was lower than that of forage sorghum (Yang and Lan, 2022) and silage corn (Na et al., 2022), but was higher than that of ryegrass and oat (Van Die and Entz, 2022). However, when compared with the growing period of other annual forages, the ratooning sorghum could provide forage without preparing the soil and seeding and without occupying principal food production season. This greatly reduced the cost of forage production and maximized the output from limited crop land, contributing to food safety (Xu et al., 2020). The chemical analysis further indicated that the contents of CP and WSC was similar with that of silage corn (Liu et al., 2021) and forage sorghum (Yang and Lan, 2022), implying that the ratooning sorghum might be a good alternative for fodder.
Generally, sorghum plants contain certain amounts of CNglcs, with its contents depending upon cultivars, soil water conditions, and growing stages (Sun et al., 2018). In this study, the contents of HCN in ratooning sorghum were greater than 800 mg/kg FW, significantly higher than the safety threshold (200 mg/kg FW). As one of the anti-nutritive, the CNglcs in ratooning sorghum increased its risk of poisoning when used as livestock feeds (Cressey et al., 2013). Therefore, the removal of CNglcs is the prerequisites for ratooning sorghum to be used as livestock feeds.
Ensiling could not reduce the HCN to safety level for ratooning sorghum. Interestingly, lower contents of HCN were observed for silage ensiled with the additions of cellulase or xylanase, suggesting that cellulase or xylanase addition would obtain higher HCN removal rate. Such positive effects of these silage additives were also reported on silage qualities (Thomas et al., 2013). However, the HCN contents in those silages 60 days after ensiling were still above 200 mg/kg FW, higher than the safety level. This suggested that these common silage additives could not completely remove HCN from ensiled ratooning sorghum. With the prolongation of fermentation, an increase of HCN removal rate was also observed, with the removal rate became slower 30 days after ensiling. Though no evidence has reported that the pH level might influence the HCN removal rate of silage, it seems that HCN removal rate reduced with the decrease of silage pH levels. Therefore, selecting silage additive that can degrade CNglcs at lower pH level might be a way to remove the HCN further.
A. niger, the most abundant mold found in the environment, has been shown to be effective in improving the silage qualities of rice straw and Napier grass (Bureenok et al., 2019; Li et al., 2022). And the safety and efficacy of the feed additives containing cellulase and endo-1,3(4)-β-glucanase produced by A. niger were also evaluated, proving their potential to be used as silage material (Rychen et al., 2018). A study by Liu et al. (2010) reported that inoculation of A. niger had a notable role on the removal of cyanoside of rubber seed meal. The HCN contents in silage ensiled with different concentrations of A. niger were all lower than 120 mg/kg after 60 days, suggesting that the addition of A. niger was effective in degrading CNglcs, with the remaining HCN in silage meeting the requirements of livestock safety. The highest HCN amount removed from silage was observed at Day 7 for silages added with A. niger, indicating that the A. niger might mainly degrade CNglcs at early days of silage fermentation. For an aerobic microbe, A. niger cannot adapt to the anaerobic condition of fermentation process (Meijer et al., 2007). Therefore, the positive effect of A. niger on HCN removal might mainly rely on the oxygen remained in silage during the early days of fermentation. During the late fermentation period, the A. niger would die, reducing their abilities to produce enzymes to degrade CNglcs. Overall, for ratooning sorghum, 150 ml A. niger + 50 ml sterile water per 3 kg was the optimal concentration, with HCN removal rate reaching 93.63%. This suggested that suitable concentration of A. niger should be screened to efficiently degrading CNglcs in silages.
Generally, CNglcs are degraded by enzyme to produce HCN (Cressey and Reeve, 2019). β-glucosidase, enzyme from the source plant material, has been shown to be involved in catalyzing the sequential breakdown of CNglcs in cyanogenic plants (Bhalla et al., 2017). A. niger had the ability to produce β-glucosidase under the various pH levels (3 to 9) and temperatures (20°C to 40°C), contributing to the degradation of CNglcs. A study on Prunus armeniaca has also shown that the β-glucosidase exhibited higher activities under low pH levels (4 to 6), and the optimum temperature for the activity of this enzyme was 35°C (Bhalla et al., 2017). Oriente et al. (2015) also reported that the optimum pH for β-glucosidase activity was 4.0 although it was stable in the range of 4.0–7.0. Therefore, the environmental conditions of the ensiling ratooning sorghum, lower pH levels in silage and the suitable temperature of fall, might be helpful to remove the CNglcs, and finally resulting in a safe HCN content for livestock. Overall, the addition of A. niger increased the contents of CP and LA, and decrease the contents of DM、NDF、pH and AN/TN in ratooning sorghum silage, thus decreasing the forage quality. The silage ensiled with 150 mL of A. niger + 50 mL of sterile water had the lowest pH, AN/TN and highest LA concentration. There are varieties of factors influencing the hydrolysis of plant protein during ensiling process (Xie et al., 2021). A. niger might influence the AN/TN through altering the distributions of microbes and thus the enzymes involved in protein hydrolysis (Guo et al., 2015). These results indicated that the addition of A. niger would increase the contents of CP and LA, and decrease the contents pH and AN/TN in ratooning sorghum silage, thus increasing the forage qualities. Li et al. (2022) also reported that the quality of mixed straw silage was depended upon concentrations of A. niger, and 100 mL of A. niger + 100 mL of sterile water was recommended to ensile rice straw. Higher concentrations of A. niger might induce competition between A. niger populations for oxygen and other nutrients for proliferation (Tian et al., 2018), reducing their efficiency to produce enzymes. Therefore, suitable A. niger concentration was also important to obtain higher quality silages.
Alpha diversity is mainly used to reflect species richness, evenness and sequencing depth (Caporaso et al., 2012). An decrease of the bacterial biodiversity in ratooning sorghum silage indicated that low pH level and low oxygen contents in silage might be not suitable for most phyllosphere microbes (Bashir et al., 2022), resulting in the propagation of microbes suitable under silage anaerobic conditions (Jung et al., 2022), like lactic bacterial. Adding A. niger decreased the alpha diversity before ensiling. This might be attributed to the quick increase of A. niger population under aerobic condition, which relatively reduced the bacterial diversity. However, with the extension of fermentation, the silages with A. niger showed higher bacterial diversity than that without A. niger. On one hand, most A. niger might die when the oxygen was depleted. On the other hand, the removal of CNglcs might benefit the growth of bacteria suitable under lower pH environments, increasing the bacterial diversity. PCoA analysis indicated that the bacterial species had a significant succession with the prolongation of fermentation time but showed no obvious difference in succession between silages with and without A. niger, suggesting that the addition of A. niger, overall, had no significant influence on ensiling microbe community.
Most of the bacteria involved in silage fermentation belong to Lactobacillus, Laminaria, Weisseria and Leuconostoc (Ni et al., 2018). A survey by Hasanuzzaman et al. (2014) also reported that in the early stage of alfalfa fermentation, P. pentosus was the dominant lactic acid bacteria (LAB), whereas Lactobacillus was dominant 7 days after silage. In this study, we also found that the dominant LAB species changes with the fermentation, and L. plantarum and L. brevis dominated the microbial community after 60 days of fermentation. These results indicated that the predominant microbes in silage of ratooning sorghum were similar with those of other sorghum silages (Tang et al., 2020; Wang et al., 2022; Zhang et al., 2022), suggesting that adding A. niger was feasible in silage fermentation for ratooning sorghum. However, some LAB species such as L. lactis, Lactococcus and Weissella-oryzae from 7 to 15 days of fermentation and L. brevis and L. dextrinicus from 30 to 60 days of fermentation, were negatively influenced by adding A. niger. The pH level and oxygen amount reduced with the prolongation of silage fermentation, which would significantly influence the propagation and enzyme productions of A. niger (Dong et al., 2014; Kanizai Saric et al., 2021), finally altering its influence of silage fermentation as well as the activities of other microbes.
The positive correlation between the silage pH value and the relative abundances of Lactococcus, Enterobacter, Enterococcu, Weigella and Lactobacillus in silage with A. niger suggested that the addition of A. niger altered the abundance of certain microbe species during fermentation, and thus the silage qualities. It is well known that Lactococcus (Weisseria, Laminaria and Enterococcus) starts LA fermentation at the early stage of silage (Jung et al., 2022). However, these strains are sensitive to low pH and cannot survive in an acidic environment (Ogunade et al., 2018). Therefore, Lactobacillus plays an important role when pH reduces at the later stage of silage, in ensuring good fermentation quality of silage (Cai et al., 1998). Interestingly, the abundance of Lactobacillus and Pediococcus was also negatively correlated with HCN contents, indicating that in the fermentation process of ratooning sorghum, these microbes might also have the abilities to remove CNglcs. For example, reduction of cyanide (97.17%) in cassava leaf was achieved under Lactobacillus plantarum fermentation (Terefe et al., 2022). Though these microbes exist in sorghum silages, lower removal rate of CNglcs in silage without adding A. niger suggested that their efficiency in removing CNglcs was less than that of A. niger.
5. Conclusion
Our results indicate that the ratooning sorghum which contain higher amount of CNglcs can be used as safe animal feeds though ensiling with A. niger. The addition of A. niger reduced the HCN contents of ratooning sorghum silage during the early days of fermentation, which improved the nutritive qualities of ratooning sorghum silage and reduced the HCN contents under safety threshold. The addition of exogenous A. niger altered the microbial community and increased bacterial diversity during silage fermentation process. The β-glucosidase can be produced by A. niger in a wide pH and temperature ranges, showing its abilities to remove CNglcs under silage conditions. These results also provide the potential to utilize plants with high CNglcs as animal feeds in the future.
Data availability statement
The datasets presented in this study can be found in online repositories. The names of the repository/repositories and accession number(s) can be found below: BioProject, PRJNA921848.
Author contributions
JZ: investigation, software, data curation, formal analysis, and writing. BW: software, data curation, formal analysis, and writing. YS: investigation, methodology, visualization, and validation. JY, TW, and WZ: investigation, methodology, visualization, and validation. JZ: investigation, methodology, and visualization. CQ: investigation, methodology, and visualization. YG: conceptualization, data curation, funding acquisition, project administration, supervision, validation, and writing – review and editing. All authors contributed to the article and approved the submitted version.
Funding
This work was funded by Chongqing Technology Innovation and Application Development Project (cstc2021jscx-gksbX0014), “First Class Grassland Science Discipline” program in Shandong Province, China, and Special Fund Project of Provincial Science and technology innovation development in agricultural high district of Yellow River Delta (2022SZX32).
Conflict of interest
JZ was employed by Chongqing Jiangxiaobai Farm Co., Ltd.
The remaining authors declare that the research was conducted in the absence of any commercial or financial relationships that could be construed as a potential conflict of interest.
Publisher’s note
All claims expressed in this article are solely those of the authors and do not necessarily represent those of their affiliated organizations, or those of the publisher, the editors and the reviewers. Any product that may be evaluated in this article, or claim that may be made by its manufacturer, is not guaranteed or endorsed by the publisher.
Abbreviations
AA, Acetic acid; ADF, Acid Detergent Fiber; AN, Ammonia nitrogen; Ash, Crude ash; BA, Butylic acid; CNglcs, Cyanogenic glycosides; EE, Ether extract; HCN, Hydrogen cyanide; LA, Lactic acid; LAB, Lactic acid bacteria; NDF, Neutral Detergent Fiber; PA, Propionic acid; PCoA, Principal coordinates analysis; PDA, Potato Dextrose Agar medium; PDB, Potato Dextrose Broph liquid medium; TN, Total nitrogen; WSC, Water-soluble carbohydrate.
Footnotes
References
Arshad, M. A., Bhatti, S. A., Hassan, I., Rahman, M. A., and Rehman, M. S. (2020). Effects of bile acids and lipase supplementation in low-energy diets on growth performance, fat digestibility and meat quality in broiler chickens. Braz. J. Poult. Sci. 22, 104–112. doi: 10.1590/1806-9061-2020-1258
Bashir, I., War, A. F., Rafiq, I., Reshi, Z. A., Rashid, I., and Shouche, Y. S. (2022). Phyllosphere microbiome: diversity and functions. Microbiol. Res. 254:126888. doi: 10.1016/j.micres.2021.126888
Bhalla, T. C., Asif, M., and Smita, K. (2017). Purification and characterization of cyanogenic beta-glucosidase from wild apricot (Prunus armeniaca L.). Process Biochem. 58, 320–325. doi: 10.1016/j.procbio.2017.04.023
Briante, R., La Cara, F., Tonziello, M. P., Febbraio, F., and Nucci, R. (2001). Antioxidant activity of the main bioactive derivatives from oleuropein hydrolysis by hyperthermophilic beta-glycosidase. J. Agric. Food Chem. 49, 3198–3203. doi: 10.1021/jf001342r
Bureenok, S., Langsoumechai, S., Pitiwittayakul, N., Yuangklang, C., Vasupen, K., Saenmahayak, B., et al. (2019). Effects of fibrolytic enzymes and lactic acid bacteria on fermentation quality and in vitro digestibility of Napier grass silage. Ital. J. Anim. Sci. 18, 1438–1444. doi: 10.1080/1828051x.2019.1681910
Cai, Y. M., Benno, Y., Ogawa, M., Ohmomo, S., Kumai, S., and Nakase, T. (1998). Influence of Lactobacillus spp. from an inoculant and of Weissella and Leuconostoc spp. from forage crops on silage fermentation. Appl. Environ. Microb. 64, 2982–2987. doi: 10.3390/aj13105484
Caporaso, J. G., Lauber, C. L., Walters, W. A., Berg-Lyons, D., Huntley, J., Fierer, N., et al. (2012). Ultra-high-throughput microbial community analysis on the Illumina HiSeq and MiSeq platforms. ISME J. 6, 1621–1624. doi: 10.1038/ismej.2012.8
Carlsson, L., Mlingi, N., Juma, A., Ronquist, G., and Rosling, H. (1999). Metabolic fates in humans of linamarin in cassava flour ingested as stiff porridge. Food Chem. Toxicol. 37, 307–312. doi: 10.1016/S0278-6915(99)00015-0
Chander, S., Sharma, K. C., and Toor, G. S. (2004). Effect of cutting schedules and varieties on growth, yield and quality of perennial pasture grasses grown in hot arid region. Indian J. Agron. 100, 567–575. doi: 10.1080/03067319.2019.1637430
Chen, D., Zhong, G., Su, H. E. W., Mhmazr, R., Chen, K., Tang, J., et al. (2021). Physiological variation in ruminal microbiota under altered energy levels in starter ration of suckling angus calves. Pak. Vet. J. 41, 409–413. doi: 10.29261/pakvetj/2021.018
Codex Committee on Contaminants in Foods. (2008) Discussion Paper on Cyanogenic Glycosides. FAO/WHO, Rome
Cowan, M. F., Blomstedt, C. K., Moller, B. L., Henry, R. J., and Gleadow, R. M. (2021). Variation in production of cyanogenic glucosides during early plant development: a comparison of wild and domesticated sorghum. Phytochemistry 184:112645. doi: 10.1016/j.phytochem.2020.112645
Cressey, P., and Reeve, J. (2019). Metabolism of cyanogenic glycosides: a review. Food Chem. Toxicol. 125, 225–232. doi: 10.1016/j.fct.2019.01.002
Cressey, P., Saunders, D., and Goodman, J. (2013). Cyanogenic glycosides in plant-based foods available in New Zealand. Food Addit. Contam. Part A Chem. Anal. Control Expo. Risk Assess. 30, 1946–1953. doi: 10.1080/19440049.2013.825819
Ding, Z. T., Xu, D. M., Bai, J., Li, F. H., Adesogan, A. T., Zhang, P., et al. (2019). Characterization and identification of ferulic acid esterase-producing lactobacillus species isolated from elymus nutans silage and their application in ensiled alfalfa. J. Appl. Microbiol. 127, 985–995. doi: 10.1111/jam.14374
Dominguez, P., Jacobson, A. G., and Jefferies, R. P. S. (2002). Paired gill slits in a fossil with a calcite skeleton. Nature 417, 841–844. doi: 10.1038/nature00805
Dong, Y. M., Fan, Q. Q., Yan, X. F., Guo, M. J., and Lu, F. (2014). Development of a hybridmodel for sodium gluconate fermentation by Aspergillus niger. J. Chem. Technol. Biot. 89, 1875–1882. doi: 10.1002/jctb.4270
Fountoulakis, M. S., Sabathianakis, G., Kritsotakis, I., Kabourakis, E. M., and Manios, T. (2017). Halophytes as vertical-flow constructed wetland vegetation for domestic wastewater treatment. Sci. Total Environ. 583, 432–439. doi: 10.1016/j.scitotenv.2017.01.090
Gleadow, R. M., Ottman, M. J., Kimball, B. A., Wall, G. W., Pinter, P. J., LaMorte, R. L., et al. (2016). Drought-induced changes in nitrogen partitioning between cyanide and nitrate in leaves and sterns of sorghum grown at elevated CO2 are age dependent. Field Crops Res. 185, 97–102. doi: 10.1016/j.fcr.2015.10.010
Guo, G., Yuan, X., Wen, A., Liu, Q., Zhang, S., and Shao, T. (2015). Silage fermentation characteristics of napiergrass harvested at various times on a sunny day. Crop Sci. 55, 458–464. doi: 10.2135/cropsci2014.03.0172
Hasanuzzaman, M., Nahar, K., Alam, M. M., Bhowmik, P. C., Hossain, M. A., Rahman, M. M., et al. (2014). Potential use of halophytes to remediate saline soils. Biomed. Res. Int. 2014:589341. doi: 10.1155/2014/589341
Jung, J. S., Ravindran, B., Soundharrajan, I., Awasthi, M. K., and Choi, K. C. (2022). Improved performance and microbial community dynamics in anaerobic fermentation of triticale silages at different stages. Bioresour. Technol. 345, 126485–121884. doi: 10.1016/j.biortech.2021.126485
Kanizai Saric, G., Rapcan, I., Potocnik, I., Sarajlic, A., and Majic, I. (2021). Potential of lactic acid bacteria in biocontrol of Aspergillus niger, Penicillium chrysogenum and Fusarium graminearum in culture media and natural substrate. Zemdirbyste 108, 153–158. doi: 10.13080/z-a.2021.108.020
Kumari, A., Goyal, M., Kumar, R., and Sohu, R. S. (2021). Morphophysiological and biochemical attributes influence intra-genotypic preference of shoot fly Atherigona soccata (Rondani) among sorghum genotypes. Protoplasma 258, 87–102. doi: 10.1007/s00709-020-01554-5
Li, P., Wan, X. C., Ding, X. L., and Tao, W. X. (1998). Determine and characteristics of β-glucosidase from Aspergillus niger. J. Anhui Agr. Univer. 25, 304–309. doi: 10.13610/j.cnki.1672-352x.1998.03.020
Li, X., Yi, K. L., Lei, H., Zhang, B. Z., Song, W., Li, H. B., et al. (2022). Effects of adding different concentrations of aspergillus Niger inoculant on the quality of mixed rice straw silage. Pak. J. Agr. Sci. 59, 357–364. doi: 10.21162/pakjas/22.8947
Liu, Y., Wang, G., Wu, H., Meng, Q., Khan, M. Z., and Zhou, Z. (2021). Effect of hybrid type on fermentation and nutritional parameters of whole plant corn silage. Animals 11:1587. doi: 10.3390/ani11061587
Liu, X. Q., Zhang, Z. Y., Guo, R. C., and Bai, X. P. (2010). The preliminary study of removing the cyanogen glucoside in rubber seed meal by microorganism fermentation. J. Anhui Agri. Sci. 38, 12941–12942. doi: 10.13989/j.cnki.0517-6611.2010.24.007
Meijer, S., Panagiotou, G., Olsson, L., and Nielsen, J. (2007). Physiological characterization of xylose metabolism in Aspergillus niger under oxygen-limited conditions. Biotechnol. Bioeng. 98, 462–475. doi: 10.1002/bit.21397
Michlmayr, H., Eder, R., Kulbe, K. D., and Del Hierro, A. (2012). Beta-glycosidase activities of Oenococcus oeni: current state of research and future challenges. Mitteilungen Klosterneuburg 62, 87–96.
Moller, B. L. (2010). Functional diversifications of cyanogenic glucosides. Curr. Opin. Plant Biol. 13, 337–346. doi: 10.1016/j.pbi.2010.01.009
Na, R. S., Xiang, F. K., Liang, W. Q., Pan, L. X., Yang, F. X., Li, Q. Q., et al. (2022). Comparison of production performance and feed quality of eight silage maize varieties. Feed Res. 45, 105–110. doi: 10.13557/j.cnki.issn1002-2813.2022.13.024
Ngwa, T. A., Nsahlai, I. V., and Iji, P. A. (2004). Ensilage as a means of reducing the concentration of cyanogenic glycosides in the pods of Acacia sieberiana and the effect of additives on silage quality. J. Sci. Food Agr. 84, 521–529. doi: 10.1002/jsfa.1650
Ni, K. K., Zhao, J. Y., Zhu, B. G., Su, R. N., Pan, Y., Ma, J. K., et al. (2018). Assessing the fermentation quality and microbial community of the mixed silage of forage soybean with crop corn or sorghum. Bioresour. Technol. 265, 563–567. doi: 10.1016/j.biortech.2018.05.097
Ogunade, I. M., Jiang, Y., Pech Cervantes, A. A., Kim, D. H., Oliveira, A. S., Vyas, D., et al. (2018). Bacterial diversity and composition of alfalfa silage as analyzed by Illumina MiSeq sequencing: effects of Escherichia coli O157:H7 and silage additives. J. Dairy Sci. 101, 2048–2059. doi: 10.3168/jds.2017-12876
Oriente, A., Tramontina, R., de Andrades, D., Henn, C., Silva, J., Simão, R., et al. (2015). Characterization of a novel Aspergillus niger beta-glucosidase tolerant to saccharification of lignocellulosic biomass products and fermentation inhibitors. Chem. Pap. 69, 1050–1057. doi: 10.1515/chempap-2015-0111
Picmanova, M., Neilson, E. H., Motawia, M. S., Olsen, C. E., Agerbirk, N., Gray, C. J., et al. (2015). A recycling pathway for cyanogenic glycosides evidenced by the comparative metabolic profiling in three cyanogenic plant species. Biochem. J. 469, 375–389. doi: 10.1042/bj20150390
Rychen, G., Aquilina, G., Azimonti, G., Bampidis, V., Bastos, M. L., Bories, G., et al. (2018). Safety and efficacy of alpha-amylase from Bacillus amyloliquefaciens DSM 9553, Bacillus amyloliquefaciens NCIMB 30251, Aspergillus oryzae CBS 585.94 and Aspergillus oryzae ATTC SD-5374, endo-1,4-beta-glucanase from Trichoderma reesei ATCC PTA-10001, Trichoderma reesei ATCC SD-6331 and Aspergillus niger CBS 120604, endo-1,4-beta-xylanase from Trichoderma koningii MUCL 39203 and Trichoderma citrinoviride CBS 614.94 and endo-1,3(4)-beta-glucanase from Aspergillus tubingensis MUCL 39199 as silage additives for all animal species. EFSA J. 16:e05224. doi: 10.2903/j.efsa.2018.5224
Seglah, P. A., Wang, Y. J., Wang, H. Y., Bi, Y. Y., Zhou, K., Wang, Y., et al. (2020). Crop straw utilization and field burning in Northern region of Ghana. J. Clean. Prod. 261, 121191–121357. doi: 10.1016/j.jclepro.2020.121191
Shehab, A. A., Yao, L. H., Wei, L. L., Wang, D. K., Li, Y., and Zhang, X. F. (2020). The increased hydrocyanic acid in drought-stressed sorghums could be alleviated by plant growth regulators. Crop Pasture Sci. 71, 459–468. doi: 10.1071/cp20057
Sun, Z. M., Zhang, K. X., Chen, C., Wu, Y. M., Tang, Y. X., Georgiev, M. I., et al. (2018). Biosynthesis and regulation of cyanogenic glycoside production in forage plants. Appl. Microbiol. Biot. 102, 9–16. doi: 10.1007/s00253-017-8559-z
Tang, Y., Wang, L. M., Xie, Y. D., Yu, X. N., Lin, L. J., Li, H. X., et al. (2020). Effects of intercropping accumulator plants and applying their straw on the growth and cadmium accumulation of Brassica chinensis L. Environ. Sci. Pollur. R. 27, 39094–39104. doi: 10.1007/s11356-020-09148-7
Terefe, Z. K., Omwamba, M., and Nduko, J. M. (2022). Effect of microbial fermentation on nutritional and antinutritional contents of cassava leaf. J. Food Saf. 42, 1745–1757. doi: 10.1111/jfs.12969
Thomas, M. E., Foster, J. L., McCuistion, K. C., Redmon, L. A., and Jessup, R. W. (2013). Nutritive value, fermentation characteristics, and in situ disappearance kinetics of sorghum silage treated with inoculants. J. Dairy Sci. 96, 7120–7131. doi: 10.3168/jds.2013-6635
Tian, X. W., Shen, Y. T., Zhuang, Y. P., Zhao, W., Hang, H. F., and Chu, J. (2018). Kinetic analysis of sodium gluconate production by Aspergillus niger with different inlet oxygen concentrations. Bioprocess Biosyst. Eng. 41, 1697–1706. doi: 10.1007/s00449-018-1993-1
Van Die, M., and Entz, M. H. (2022). Mid-summer annual forage performance in organic, grass-fed production. Can. J. Plant Sci. 102, 566–574.
Wanasundara, P. K. J. P. D., Amarowicz, R., Kara, M. T., and Shahidi, F. (1993). Removal of cyanogenic glycosides of flaxseed meal. Food Chem. 48, 263–266. doi: 10.1016/0308-8146(93)90138-6
Wang, J., Zhang, Z. D., Liu, H. H., Xu, J. F., Liu, T., Wang, C. L., et al. (2022). Evaluation of gas production, fermentation parameters, and nutrient degradability in different proportions of sorghum straw and ammoniated wheat straw. Fermentation 8, 2311–2325. doi: 10.3390/fermentation8080415
Wang, B., Zhang, J. M., Zhu, G. X., and Chang, X. D. (2007). Selection and bio-desulfurization of a sulfide oxidation bacteria. Basic Sci. J. Textile Univ. 20, 418–420. doi: 10.13338/j.issn.1006-8341.2007.04.022
Xia, C. Q., Liang, Y. X., Bai, S., He, Y., Muhammad, A. U. R., Su, H. W., et al. (2018). Effects of harvest time and added molasses on nutritional content, ensiling characteristics and in vitro degradation of whole crop wheat. Asian-Australas J. Anim. Sci. 31, 354–362. doi: 10.5713/ajas.17.0542
Xie, Y., Guo, J., Li, W., Wu, Z., and Yu, Z. (2021). Effects of ferulic acid esterase-producing lactic acid bacteria and storage temperature on the fermentation quality, invitro digestibility and phenolic acid extraction yields of sorghum (Sorghum bicolor L.) silage. Microorganisms 9:114. doi: 10.3390/microorganisms9010114
Xu, J. L., Zhang, Z. H., Zhang, X., Ishfaq, M., Zhong, J. H., Li, W., et al. (2020). Green food development in China: experiences and challenges. Agriculture 10:614. doi: 10.3390/agriculture10120614
Yang, Y., Ferreira, G., Corl, B. A., and Campbell, B. T. (2019). Production performance, nutrient digestibility, and milk fatty acid profile of lactating dairy cows fed corn silage-or sorghum silage-based diets with and without xylanase supplementation. J. Dairy Sci. 102, 2266–2274. doi: 10.3168/jds.2018-15801
Yang, F., and Lan, J. (2022). Study on adaptability of forage sorghum in semi-arid area of Ningxia. Pratacult. Sci. 4, 42–49. doi: 10.3969/j.issn.2096-3971.2022.04.008
Yu, R. L., Liu, J., Wang, Y., Wang, H., and Zhang, H. W. (2021). Aspergillus niger as a secondary metabolite factory. Front. Chem. 9, 246–269. doi: 10.3389/fchem.2021.701022
Zhang, Y. W., Tao, X. Y., Liu, Q. S., Zhang, Y. J., Xu, J. B., Zhang, W. Y., et al. (2022). Succession changes of fermentation parameters, nutrient components and bacterial community of sorghum stalk silage. Front. Microbiol. 13:982489. doi: 10.3389/fmicb.2022.982489
Keywords: Aspergillus niger, cyanogenic glycosides, hydrocyanic acid, ratooning sorghum, silage
Citation: Zhai J, Wang B, Sun Y, Yang J, Zhou J, Wang T, Zhang W, Qi C and Guo Y (2023) Effects of Aspergillus niger on cyanogenic glycosides removal and fermentation qualities of ratooning sorghum. Front. Microbiol. 14:1128057. doi: 10.3389/fmicb.2023.1128057
Edited by:
Salam A. Ibrahim, North Carolina Agricultural and Technical State University, United StatesReviewed by:
Yi Xu, Hefei University of Technology, ChinaQisen Xiang, Zhengzhou University of Light Industry, China
Copyright © 2023 Zhai, Wang, Sun, Yang, Zhou, Wang, Zhang, Qi and Guo. This is an open-access article distributed under the terms of the Creative Commons Attribution License (CC BY). The use, distribution or reproduction in other forums is permitted, provided the original author(s) and the copyright owner(s) are credited and that the original publication in this journal is cited, in accordance with accepted academic practice. No use, distribution or reproduction is permitted which does not comply with these terms.
*Correspondence: Yanjun Guo, ✉ cWhneWpAcWF1LmVkdS5jbg==