- Laboratory of Animal Pathogenic Microbiology, College of Animal Science, Yangtze University, Jingzhou, China
Introduction: Glutathione peroxidase is abundant in eukaryotes as an important antioxidant enzyme. However, prokaryotic glutathione peroxidase has not been thoroughly studied. Listeria monocytogenes is a facultative intracellular pathogen that is capable of causing listeriosis in animals as well as humans. Despite the fact that L. monocytogenes encodes a putative glutathione peroxidase, GSH-Px (encoded by lmo0983)), the functions of the enzyme are still unknown. Here we revealed the unusual roles of L. monocytogenes GSH-Px in bacterial antioxidants and pathogenicity.
Methods: L. monocytogenes Lm850658 was taken as the parental strain to construct the gsh-px deletion strain and related complement strain. The effect of the gsh-px gene on the resistance of L. monocytogenes to oxidative stress was determined by measuring the concentrations of glutathione and assaying the stress survival rates under different oxidative conditions. In addition, the pathogenicity of L. monocytogenes was determined by cellular adhesion and invasion assays and mice virulence tests, and the expression of virulence factors was determined by Western blot.
Results: Deficiency of GSH-Px not only increased glutathione concentrations in L. monocytogenes but also enhanced its resistance to oxidative stress when exposed to copper and iron ions. In addition, the absence of gsh-px significantly improved the adhesion and invasion efficiency of L. monocytogenes to Caco-2 cells. More importantly, L. monocytogenes lacking GSH-Px could colonize and proliferate more efficiently in mice livers and spleens, enhancing the pathogenicity of L. monocytogenes by increasing the expression of virulence factors like InlA, InlB, and LLO.
Discussion: Taken together, we confirmed that GSH-Px of L. monocytogenes has a counter-intuitive effect on the antioxidant capacity and pathogenicity.
1. Introduction
Listeria monocytogenes is found in plenty of environments and can easily transition from saprophytic organism to intracellular pathogen (Freitag et al., 2009; Xayarath and Freitag, 2012). Humans and animals may develop severe illnesses when they consume contaminated foods containing L. monocytogenes (Hedberg, 2011). L. monocytogenes faces a variety of stresses to survive in different niches, the most prevalent of which is oxidative stress. The oxidative conditions L. monocytogenes confronted include reactive oxygen species (ROS), reactive nitrogen species (RNS), and reactive chlorine species (RCS). Cellular components including DNA, proteins and membrane lipids are damaged when cells exposed to ROS, RNS, and RCS (Chakraborty et al., 1992; Ezraty et al., 2017). Consequently, for L. monocytogenes to thrive in oxidative environments, it needs to use a sophisticated system to ward off oxidative stress as well as prevent oxidative damage (Mates et al., 1999; Mates, 2000). To combat oxidative stress, L. monocytogenes encodes multiple metabolic enzymes like superoxide dismutase (SOD), catalase (CAT), thioredoxin (TRx), glutathione reductase (GR), and glutathione S-transferase (GST; Mains et al., 2021).
Glutathione peroxidases (GSH-Pxs) are a group of antioxidant enzymes found in eukaryotic organisms. In addition to being a vital component of the antioxidant systems, they may be involved in immune defense against pathogen invasion (Bathige et al., 2015). For example, inflammatory signaling cascades are closely linked to GSH-Px1 in mammals (Duong et al., 2010; Brigelius-Flohe and Maiorino, 2013). However, prokaryotic GSH-Px research is lacking. Numerous prokaryotes, including Staphylococcus aureus, Bacillus subtilis, Lactococcus lactis, and L. monocytogenes, have been found to possess genes homologous to gsh-px (Brenot et al., 2004). To date, there have been no investigations into the biological mechanisms of GSH-Px in bacteria in response to oxidative stress and host infection. GSH-Px reduces the organic hydroperoxides and hydrogen peroxide to the corresponding alcohols (water in the case of hydrogen peroxide) in eukaryotic organisms by utilizing glutathione as an electron donor (Ursini et al., 1995; Kutlu and Susuz, 2004). Whether GSH-Px in prokaryotes is closely linked to glutathione and affects oxidative resistance and pathogenicity, what specific biological functions of GSH-Px in prokaryotes are prompting us to understand the effects of GSH-Px on L. monocytogenes.
Glutathione (GSH), one of the glutaredoxin (Grx) systems, is necessary for preserving intracellular redox homeostasis (Masip et al., 2006). L. monocytogenes is one of the Gram-positive bacteria capable of synthesizing glutathione (Newton et al., 1996). GSH binds to PrfA at the broad tunnel between the N-terminal and C-terminal domains to make PrfA change conformation and become constitutive activation (Hall et al., 2016). PrfA is a global virulence regulator, which belongs to the Crp/Fnr transcriptional factor family (Scortti et al., 2007). GSH allosterically binds to PrfA results in activation of PrfA and exhibits high affinity to the promoters of PrfA regulated virulent genes (Chakraborty et al., 1992; Freitag et al., 2009; De Las Heras et al., 2011). Therefore, GSH improves the pathogenicity of L. monocytogenes by increasing the expression levels of PrfA regulated genes (Reniere et al., 2015; Hall et al., 2016). Moreover, it has been demonstrated that L. monocytogenes controlled its biphasic life cycle and transitioned from saprophytic to pathogenic by regulating the concentrations of GSH (Reniere et al., 2015). Hence GSH is indispensable for L. monocytogenes not only for its allosteric binding to PrfA to promote expression of the virulence factors but for the ability to resist oxidative stress to produce a reductive environment for life activity (Masip et al., 2006; Ku and Gan, 2019).
Listeria monocytogenes lmo0983 has been identified as a putative glutathione peroxidase in the GenBank database. The purpose of the research is to confirm whether GSH-Px in L. monocytogenes is involved in the biological process of bacterial infection and the related molecular mechanisms. As shown by our findings that GSH-Px played unanticipated functions in the antioxidant and pathogenicity of L. monocytogenes. The resistance of L. monocytogenes to the oxidative condition induced by metal ions was significantly enhanced when the gsh-px gene has been deleted, and the virulence of the pathogen in cells and mice was also increased. The findings of this research provide valuable evidence for elucidating the multiple physiological roles of glutathione peroxidase in foodborne pathogens to survive in the external environment and, more importantly, in the successful establishment of infection in the host.
2. Materials and methods
2.1. Bacterial strains and culture conditions
Listeria monocytogenes Lm850658 was taken as a wild-type strain, and the other strains were derived from it. All L. monocytogenes strains were incubated in brain-heart infusion (BHI) medium (Oxoid). E. coli DH5α was utilized as a plasmid host strain and cultured in Luria-Bertani broth (LB) (Oxoid). Agar was added to solid media at 1% (w/v). All bacteria were incubated at 37°C unless otherwise requested. The following concentrations were used for the antibiotics: ampicillin (100 μg/ml), erythromycin (10 μg/ml), kanamycin (50 μg/ml), or chloramphenicol (10 μg/ml) were added appropriately to the medium. Sangon Biotech supplied the highest purity of all chemicals. Table 1 displays the primers utilized in the research.
2.2. In-frame deletion and complementation of Listeria monocytogenes
Construction of gene deletion strain utilizing a homologous recombination strategy (Camilli et al., 1993), and temperature-sensitive plasmid pKSV7 was employed as a shuttle vector. Briefly, PCR amplification yielded the upstream and downstream homologous arms of lmo0983. A knock-out plasmid was constructed by connecting the homologous arms to shuttle vector. To promote chromosomal integration, electroporation of the plasmid into Lm850658, and transformants were grown in BHI broth containing 10 μg/ml chloramphenicol at a non-permissive temperature of 42°C. In order to make plasmid excision and curing, the recombinants were continuously passaged without chloramphenicol at a permissive temperature of 30°C. Finally, the chloramphenicol-sensitive recombinant colonies were identified using PCR. The integrative plasmid pIMK2 was utilized to complement the gsh-px gene of L. monocytogenes (Monk et al., 2008). Briefly, the gsh-px gene and its endogenous promoter were obtained using the primer pairs shown in Table 1, which were cloned into the pIMK2 plasmid to create the complementation plasmid. Electroporation of complementary plasmid into L. monocytogenes Δgsh-px. The constructed complement strain was named CΔgsh-px.
2.3. Measurement of growth curves
All strains were streaked in advance on BHI plates and individual colonies of each strain were picked into 3 ml broth. All L. monocytogenes strains were incubated for an entire night at 37°C in BHI broth. Cultures were gathered by centrifugation at 12,000 rpm for 2 min and washed once in phosphate-buffered saline (PBS, 10 mM, pH 7.4). The optical density of the bacteria was standardized to 1.0. Bacterial cultures were diluted at a ratio of 1:100 with normal BHI broth or with H2O2 at sub-lethal concentrations of 10 mM. The diluted bacterial solution was added to 96-well plates at 200 μl per well, 3 parallel wells for each sample, and blank reference using BHI medium. All bacteria were cultivated at 37°C for 12 h. The kinetic growth was conducted and bacterial OD600 nm was recorded at 1-h interval by a microplate reader.
2.4. Oxidative stress tests
H2O2 acted as a direct oxidant in oxidative stress, and the metal ions copper (Cu2+) and iron ions (Fe3+) acted as redox active stress (Birben et al., 2012). The overnight cultured L. monocytogenes was harvested by centrifuging at 12000 rpm for 2 min and washed once with PBS, then diluted to OD600 nm of 1.0 (~109 CFU/ml). The bacterial suspension was serially diluted after being resuspended in sterile PBS, and 100 μl bacterial solution was taken into 900 μl BHI broth containing 10 mM H2O2, 10 mM FeCl3, and 5 mM CuSO4 for 1 h, respectively. After incubating at 37°C for 1 h, the mixtures were serially diluted and placed on BHI agar plates. Spot plate counts were used to count the number of bacteria after incubation at 37°C for 24 h.
2.5. Determination of glutathione
GSH (reduced glutathione) concentrations were detected under different oxidative conditions. Beyotime Biotechnology provided the commercial measurement kits, which were used in accordance with the manufacturer’s instructions. In short, dilution of 10 mM GSSG (oxidized glutathione) stock solution with protein removal reagent M to 15, 10, 5, 2, 1, 0.5 μM sequentially, then the 6 points were taken to make a standard curve. Determination of bacterial samples needed to be performed on the basis of the fresh cells. Bacteria growing to the exponential stage were obtained by centrifugation at 12000 rpm for 5 min, which was weighed and treated with BHI broth containing 10 mM H2O2, 10 mM FeCl3, and 5 mM CuSO4 for 3 h, respectively. An equal amount of bacterial pellet was collected from the control group. All samples were treated with lysozyme at 37°C for 30 min, then added with protein removal reagent M 3 times the volume of the cell pellet and mixed thoroughly. The samples were rapidly freeze-thawed twice using liquid nitrogen and a 37°C water bath. The supernatant was gathered by centrifuging at 4°C and 12,000 rpm for 10 min, then the total glutathione concentrations could be determined. Some samples of total glutathione contents to be measured were taken. Adding diluted GSH removal solution proportionally with vortex immediately, then adding GSH scavenging reagent working solution. Immediately vortexed and reacted at 25°C for 60 min. The above reaction could remove GSH from the sample and be used to determine GSSG. The amount of GSH could be calculated by subtracting the amount of GSSG from the total glutathione (GSSG + GSH). GSH concentrations of the parental and mutant strains were measured as described above (Anaya-Sanchez et al., 2021). A 96-well plate was used for measurement, and the standards and samples were added in sequence. 150 μl total glutathione detection working solution was added to the wells, and 50 μl of 0.5 mg/ml NADPH solution was added after incubation at 25°C for 5 min. The absorbance of 412 nm was determined with a plate reader, measured every 10 min for 1 h.
2.6. Adhesion and invasion tests
The human intestinal epithelial Caco-2 cells were used for adhesion and invasion assays (Cheng et al., 2017b). In brief, the Caco-2 cells were cultured in Dulbecco’s modified Eagle’s medium (DMEM) supplemented with 10% fetal bovine serum (FBS) at 37°C with 5% CO2. Overnight grown L. monocytogenes was washed and resuspended with PBS. A single layer cells infected by bacteria for 30 min at a MOI of 10. For the adhesion assays, cells were lysed after two washes with PBS. As for the invasion assays, the prepared bacterial solution was added to the cells at 37°C with 5% CO2 for 1 h. To kill extracellular bacteria, DMEM comprising 200 mg/ml gentamicin was used for an additional 1 h after being washed twice with PBS. The cells were lysed after washing twice with PBS. Counting viable bacteria on BHI agar plates after the solution was 10-fold diluted. The adhesion or invasion efficiency was determined by dividing the number of colony-forming units (CFUs) of adherent or invasive cells by the total number of infected bacteria.
2.7. Virulence experiments
The virulence of L. monocytogenes parental and mutant strains was detected by the method of bacterial load in mice organs (livers, spleens, and brains; Cheng et al., 2017b). For the bacterial load, overnight-grown L. monocytogenes was diluted to concentrations of 1 × 107 CFU/ml after washing once with PBS. The female Kunming mice were divided into 3 groups and 5 mice in each group. 106 CFU of each strain were injected intraperitoneally into mice. The mice were euthanized 48 h post infection (pi), and samples of their brains, livers, and spleens were taken and homogenized in 1 ml PBS under sterile condition. In order to count the number of bacteria, after being serially diluted, the homogenate was placed on BHI agar plates and incubated overnight.
2.8. Western blot
Listeria monocytogenes uses a collection of virulence factors to increase its pathogenicity and establish an infection successfully, allosteric binding of GSH to the transcription regulator PrfA is necessary for the expression of the PrfA regulated virulence factors (Ku and Gan, 2019). Therefore, we conjectured that the deletion of gsh-px would affect the expression of virulence factors in L. monocytogenes. Specifically, the bacteria colonies of the parental strain and mutant strain were picked into 50 ml medium, respectively. Incubation in a shaker at 37°C for one night. For lysates of whole cells (Lenz and Portnoy, 2002), the bacteria was gathered by centrifugation at 12000 rpm for 10 min, bacterial pellet was resuspended with 500 μl extraction solution (2% SDS, 4% triton, 10 mM PBS). The mixture was freeze-thawed twice at −20°C for 1 h and 37°C for 15 min, the supernatant was obtained by centrifugation at 12000 rpm for 10 min as the whole cell extract. After being boiled for 10 min, the protein samples were isolated by SDS-PAGE and incubated with α-InlA, InlB, LLO, and α-GAPDH antisera (prepared in this study). GAPDH served as an internal reference for proteins extracted from whole cells. Referring to the former study, all primary antibodies were produced in our laboratory (Cheng et al., 2017a). Rabbits were injected subcutaneously with 500 μg protein, including InlA, InlB, LLO, and GAPDH separately, with an equivalent volume of complete adjuvant for primary immunization. After 14 days, each rabbit was injected subcutaneously with 250 μg of incomplete adjuvant and an equal volume of protein, the immunization was given three times, once every 2 weeks. Blood was obtained from rabbits 7 days after the last immunization. Primary antibodies were diluted at 1:5,00 – 5,000, including rabbit polyclonal antibodies against InlA, InlB, LLO, and GAPDH, and following the manufacturer’s instructions when using the appropriate secondary antibody. The enhanced chemiluminescence detection system was used to visualize all immunoreactions.
2.9. Ethics statement
Animal experiments had approved by the Laboratory Animal Management Committee of Yangtze University (Approval No. 20210301).
2.10. Statistical analysis
All experiments were performed three times with biological replicates. Data was expressed as mean ± standard deviation and statistical analysis was performed with GraphPad Prism 6 (version 6, GraphPad, United States). Unpaired t-tests were used to analyze the statistical significance. p-values <0.05 or 0.01 were considered statistically significant difference and marked with “*” and “**”; p-values >0.05 were considered as statistically, no significant difference and marked with “ns”.
3. Results
3.1. In vitro growth of Listeria monocytogenes was unaffected by the deletion of gsh-px
GSH-Px is a vital component of the antioxidant systems in eukaryotes (Margis et al., 2008). To learn more about the specific biological roles of GSH-Px in L. monocytogenes, in-frame deletion mutant strain of the gsh-px gene was constructed. To understand whether the deletion of gsh-px was relevant for the growth of L. monocytogenes, the growth capacity of parental strain Lm850658 and the deletion strain Δgsh-px in BHI with or without 10 mM H2O2 was determined. After incubating all strains at 37°C for 12 h, it was found that the growth ability of the parental and deletion strains was comparable under the normal condition (Figure 1A). When treated with 10 mM H2O2, this phenomenon remained unchanged (Figure 1B), indicating that the absence of gsh-px had no effect on H2O2. This observation demonstrated that the deletion of gsh-px had no influence on the growth of bacteria in vitro.
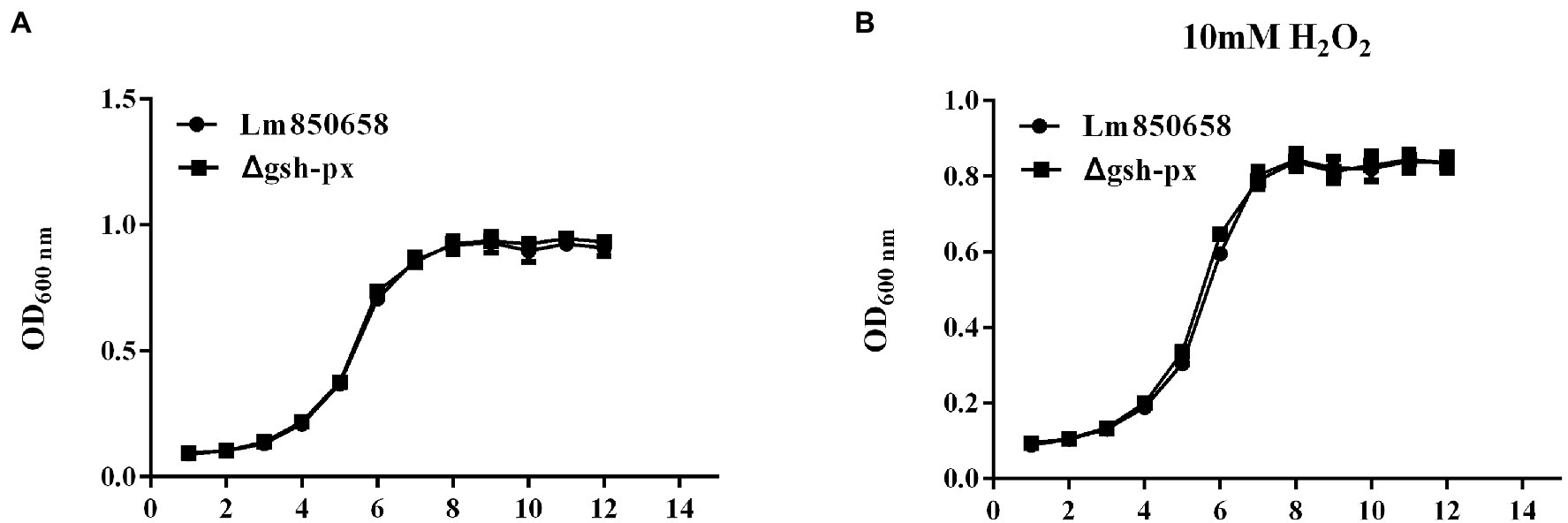
Figure 1. Deletion of gsh-px did no effect on the growth of L. monocytogenes in vitro. Overnight-grown bacteria were washed and diluted (1:100) in fresh BHI broth with (B) or without H2O2 (A), incubated at 37°C for 12 h. Data were expressed as mean ± SD.
3.2. Deficiency of gsh-px dramatically increased tolerance to the copper and iron but not to the hydrogen peroxide
Since it has been shown that GSH-Px is closely related to the antioxidant systems of eukaryotes, further to identify whether GSH-Px of L. monocytogenes is correlated with bacterial oxidative resistance, the Lm850658 and gsh-px mutant strains (Δgsh-px and CΔgsh-px) were treated with diverse oxidizing media. By comparing the survival rates of the parental strain and deletional strain under the stress of the H2O2, the results showed that the survival rates of the parental strain and the mutant were comparable at 10 mM sub-lethal concentrations (Figure 2A). However, when exposed to the same concentrations of Fe3+, the survival rate of the deletion strain was significantly higher than that of the parental strain (Figure 2B), while no viable bacteria were detected in all strains at the same concentrations of Cu2+, so the concentrations of Cu2+ were reduced to 5 mM. The deletion of gsh-px significantly enhanced the resistance of L. monocytogenes to 5 mM Cu2+ (Figure 2C). This phenotype could be restored in the complementation strain CΔgsh-px. These findings indicated that GSH-Px played an exceptional role in the antioxidant process of L. monocytogenes to metal ions, which was different from the classical fact that GSH-Px plays a role in enhancing antioxidant processes in eukaryotes.
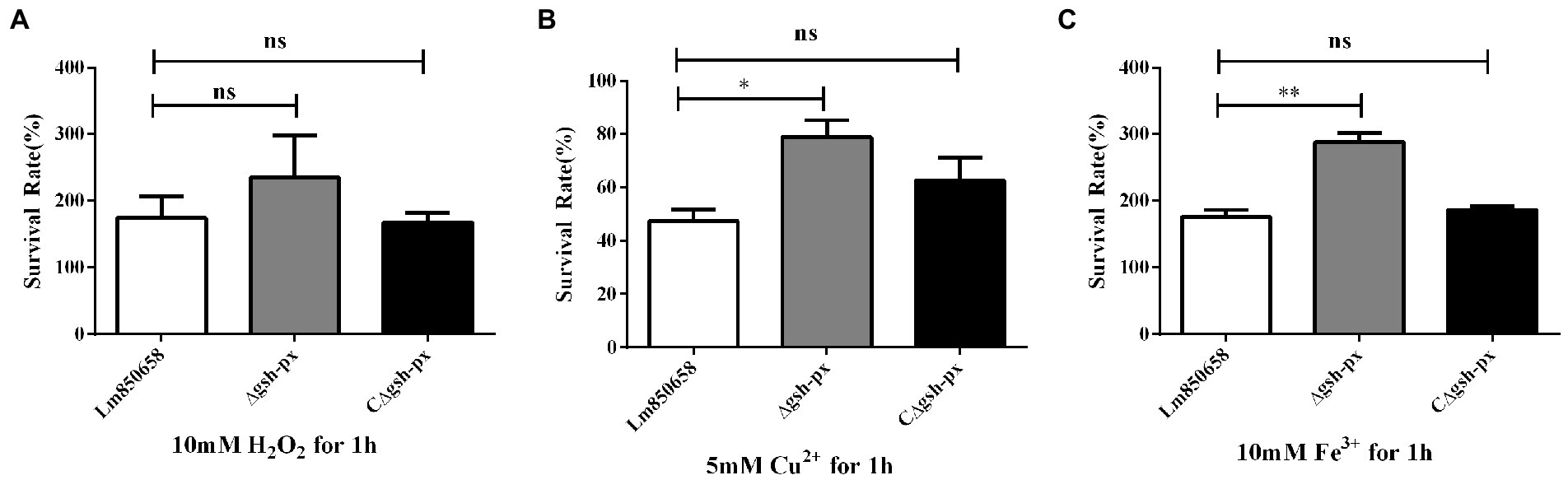
Figure 2. Deletion gsh-px of L. monocytogenes increased the tolerance to copper and iron, but not to hydrogen peroxide. Bacteria were treated with 10 mM H2O2 (A), 5 mM Cu2+ (B) and 10 mM Fe3+ (C) for 1 h, and alive bacteria were counted by plate counting. The experiments were conducted for triplicate and data were expressed as mean ± SD. ns, no significance; *, p < 0.05; **, p < 0.01.
3.3. The GSH concentrations of Listeria monocytogenes were significantly increased under the stimulation of metal ions
To understand whether the unusual effects of GSH-Px in L. monocytogenes are related to GSH, the concentrations of GSH under different oxidative conditions were measured. According to the measurement results, we found that in contrast to the normal BHI control group, the GSH of L. monocytogenes treated with metal ions for 3 h was significantly increased (Figure 3). However, after treating the bacteria with H2O2 for 3 h, GSH concentrations were essentially the same between the experimental and control groups (Figure 3). Unlike the role of GSH-Px in mammals, GSH concentrations in L. monocytogenes did not change significantly when H2O2 was used as a stressor, which was consistent with the results of oxidative stress tests.
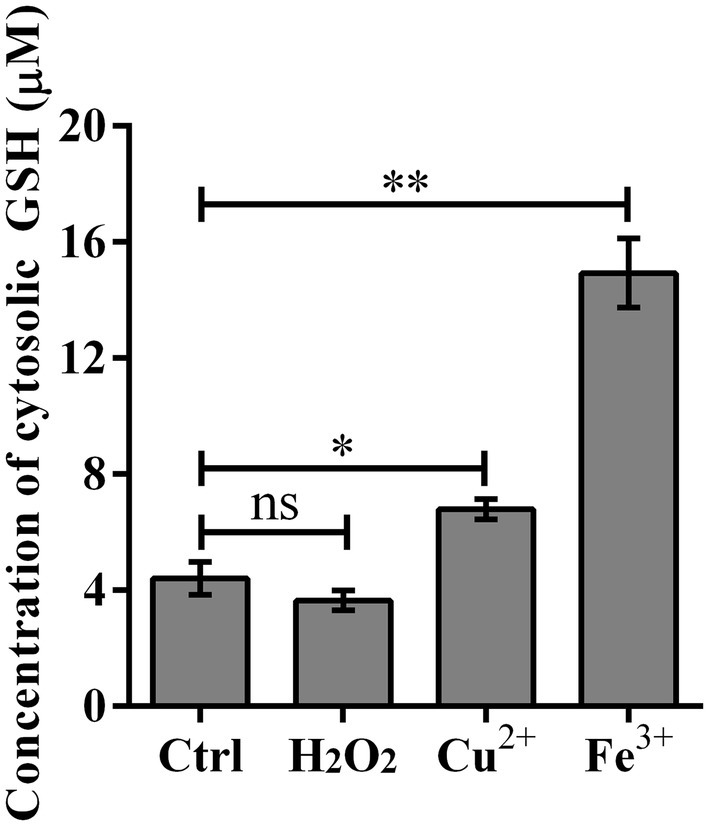
Figure 3. Cytosolic GSH concentration was significantly improved upon copper and iron treatment. Wild type strain Lm850658 was treated with BHI containing 10 mM hydrogen peroxide, 5 mM copper or 10 mM iron for 3 h and then bacteria were collected and lysed with lysozyme for 30 min. The concentrations of GSH were measured with commercial kit supplied by Beyotime Biotechnology as manufacturer’s instruction. The experiments were conducted for triplicate and data were expressed as mean ± SD. ns, no significance; *, p < 0.05; **, p < 0.01.
3.4. Intracellular adhesion and invasion efficiency of Listeria monocytogenes was enhanced by the gsh-px mutation
Due to the unusual effect of GSH-Px on resistance to oxidative stress in L. monocytogenes, we set out to learn more about the role that GSH-Px plays in bacterial intracellular infection. Epithelial cell Caco-2 was used to compare the adhesion and invasion of wild-type and mutant strains. Surprisingly, the Δgsh-px mutant strain was able to adhere and invade cells more effectively than parental strain (Figure 4), suggesting that the gsh-px gene was associated with the invasive ability of L. monocytogenes and may be redundant in helping the bacteria to invade the host.
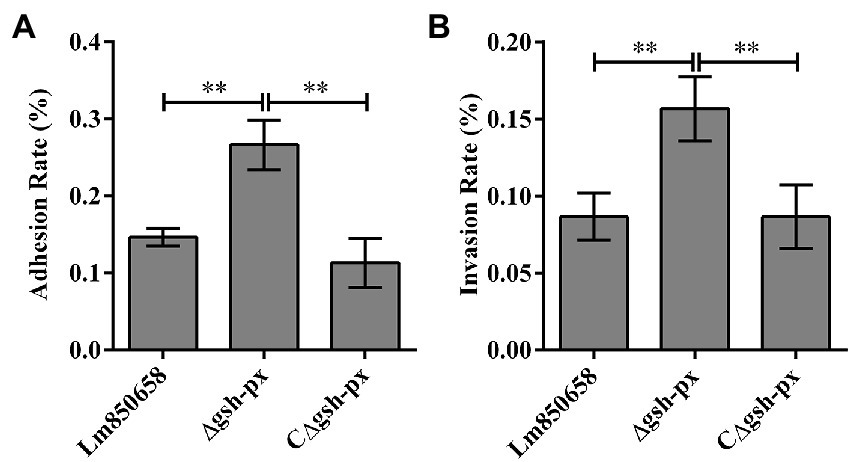
Figure 4. Mutation of gsh-px increased the adhesion (A) and invasion (B) ability of L. monocytogenes to Caco-2 cells. Epithelial cells Caco-2 were incubated with Lm850658, Δgsh-px and CΔgsh-px for 1 h, the adhesive bacteria were collected and counted by plate counting and the invasive bacteria collected after killing the extracellular bacteria with gentamycin. Finally, the adhesion and adhesion rates were calculated using the adherent and invasive bacteria divided by the initial bacteria. The experiments were conducted for triplicate and data were expressed as mean ± SD. ns, no significance; *, p < 0.05; **, p < 0.01.
3.5. Absence of gsh-px upgraded the harmfulness of Listeria monocytogenes to mice
The increased cell adhesion and invasion rates of the gsh-px mutant strain prompted us to explore more about how the GSH-Px affects virulence of L. monocytogenes in mammals. Therefore, we verified the connection between GSH-Px and virulence by measuring the bacterial load in the organs of mice. The results indicated that the Δgsh-px strain recovered more CFU from the spleens, livers and brains of infected mice compared to the wild-type strain (Figure 5). According to the findings, enhanced virulence of the Δgsh-px mutant strain in mice, which was consistent with the consequence of cellular assays. The remarkable role of GSH-Px in the pathogenicity of L. monocytogenes was demonstrated by the discovery of the gsh-px mutant that increased virulence.
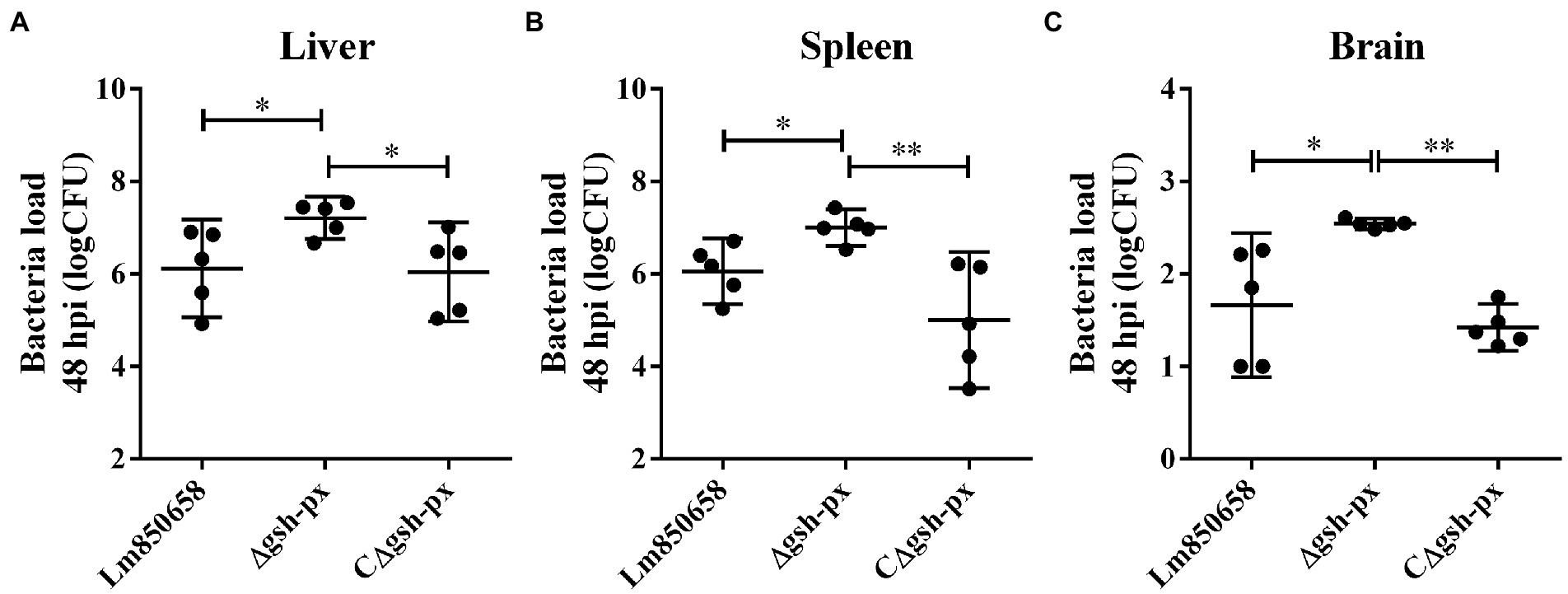
Figure 5. Deletion of gsh-px enhanced the virulence of L. monocytogenes to mice. Mice were infected with 107 CFU by intraperitoneal injection with Lm850658, Δgsh-px and CΔgsh-px. Bacteria load in the livers (A), spleens (B) and brains (C) were counted by plate counting after mice were euthanasia at 48 h post infection. Data were expressed as mean ± SD. ns, no significance; *, p < 0.05; **, p < 0.01.
3.6. Deletion of gsh-px upregulated the expression of major virulence factors in Listeria monocytogenes
The InlA and InlB are vital members of the virulence factors and the adhesion and invasion of L. monocytogenes into cells are crucially influenced by the InlA and InlB. By rupturing phagocytic vesicles, LLO makes it possible for bacteria to enter the cytoplasm. Western blotting was used to examine the expression of the major virulence factors in order to expand our understanding of how GSH-Px affects these factors. We discovered that L. monocytogenes was capable of invading the host more effectively in the absence of gsh-px in cellular experiments and virulence assays on mice. Based on this, we hypothesized that the increased expression of virulence factors might result from the deletion of gsh-px. The blotting validation results indicated that the expression of InlA, InlB and LLO in L. monocytogenes was remarkably increased when gsh-px was lacking, further to confirm the regulatory role of gsh-px on virulence factors in L. monocytogenes (Figure 6). This also supported our hypothesis that the deletion of gsh-px enhanced the adhesion and invasion ability of L. monocytogenes by enhancing the expression of virulence factors.
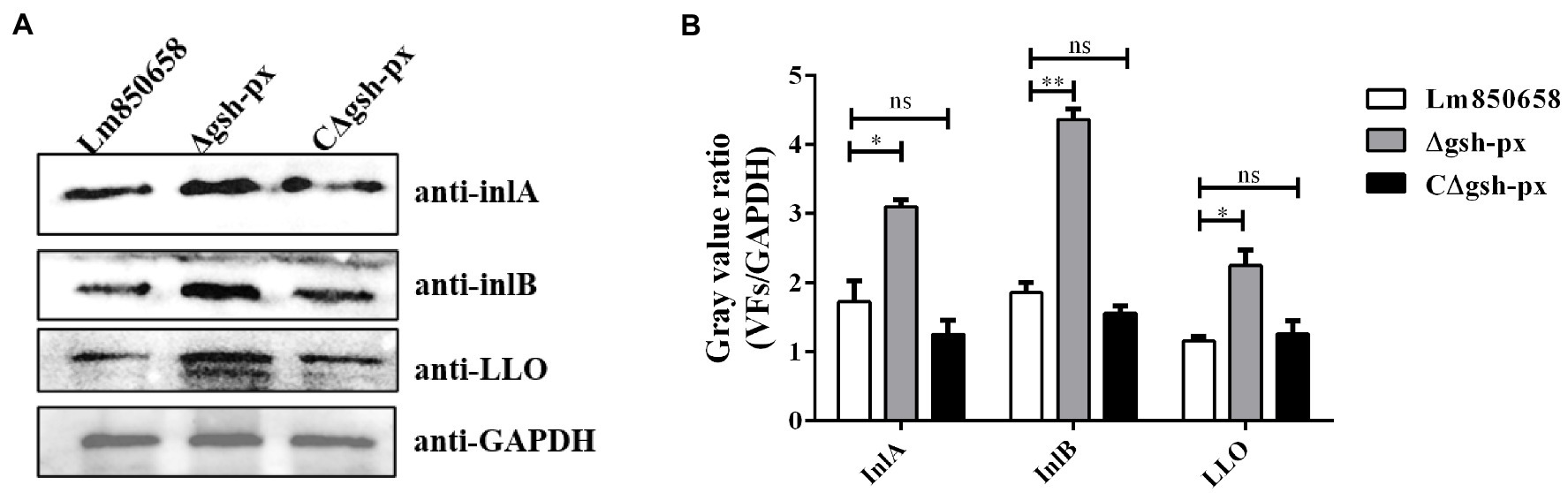
Figure 6. Deletion of gsh-px upregulated the expression of major virulence factors in L. monocytogenes. Overnight cultured bacteria were collected and the expression levels of the major virulence factor InlA, InlB and LLO were measured by western blot (A). The gray value ratio was calculated by the band gray value of virulence factor relative to that of GAPDH (B). Data were expressed as mean ± SD. ns, no significance; *, p < 0.05; **, p < 0.01.
3.7. Deletion of gsh-px increased the GSH levels in Listeria monocytogenes
Due to the fact that intracellular GSH levels are required for PrfA activation, it is necessary to maintain high intracellular GSH concentrations for L. monocytogenes. We hypothesized that the effective intracellular GSH levels of the gsh-px deficient mutant were higher than that of the parental strain, resulting in enhanced virulence of the deletion strain. To validate the assumption, we tested the levels of GSH in the wild-type, Δgsh-px and CΔgsh-px strains. The results showed that the GSH concentrations of deletion strain were higher than that of parental strain, and this phenotype could be restored in the complementation strain CΔgsh-px (Figure 7), suggesting that L. monocytogenes no longer consumed GSH or the rates of GSH consumption slowed down when the gsh-px was deleted, leading to GSH accumulation and enhanced virulence of L. monocytogenes.
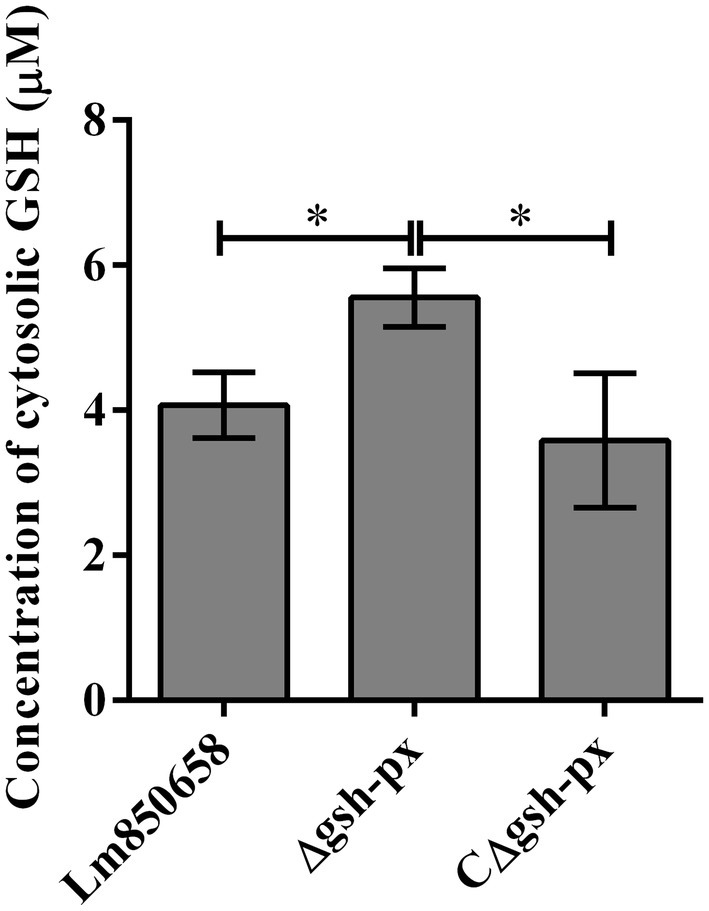
Figure 7. Deletion of gsh-px increased the glutathione levels in L. monocytogenes. Lm850658, Δgsh-px and CΔgsh-px were collected and lysed with lysozyme for 30 min. The concentrations of GSH were measured with commercial kit supplied by Beyotime Biotechnology as manufacturer’s instruction. The experiments were conducted for triplicate and data were expressed as mean ± SD. ns, no significance; *, p < 0.05; **, p < 0.01.
4. Discussion
In normal circumstances, intracellular pro-oxidant agents and antioxidant stressors are in a dynamic equilibrium. However, when the host is infected with bacteria, host cells promote the synthesis of hydrogen peroxide, superoxide, and hydroxyl radicals to prevent bacterial invasion (Mates, 2000; van der Oost et al., 2003). The accumulation of pro-oxidant agents that cause oxidative damage in activated cells turns the cytoplasm into a risky location. Protein damage, oxidative degradation of lipids, DNA denaturation, apoptosis and necrosis are all outcomes of oxidative damage (Limon-Pacheco and Gonsebatt, 2009), because specialized phagocytes like macrophages can prevent pathogens from becoming viable through an oxidative burst of NADPH oxidase complexes assembled on phagocytic vesicles. Furthermore, some metals can also mediate the production of oxidative stimuli. In order to safeguard themselves from oxidative stressors and prevent essential virulent proteins from damaging, bacteria have naturally developed numerous antioxidant defense mechanisms, both enzymatic and non-enzymatic (Mates et al., 1999; Mates, 2000). As a foodborne pathogen, L. monocytogenes also contains several antioxidant enzymes to combat the strict conditions from both outside and inside environments of host. Superoxide dismutase (SOD) easily breaks down superoxide into peroxides, which indirectly causes the accumulation of H2O2 within the cell. Peroxides can be broken down by catalase (CAT) and peroxidase (PER), or the Fenton reaction with metal ions (Fe2+ and Cu2+) can turn them into hydroxyl radicals (Reichmann et al., 2018). However, the products of the Fenton reaction can also damage cells, through DNA or protein repair mechanisms, this can be reduced by removing or synthesizing new substances to replace the damaged cellular components.
By using GSH as an electron donor and catalyzing the conversion of organic hydroperoxides and hydrogen peroxide to the corresponding alcohols, GSH-Pxs in eukaryotes play a crucial role in mediating antioxidant defense (Arthur, 2000). However, prokaryotic GSH-Px is poorly understood, in contrast to the extensive research on eukaryotic GSH-Px. A previous report on the gsh-px gene in prokaryotes, Neisseria meningitidis, was published (Moore and Sparling, 1995, 1996). The susceptibility of N. meningitidis to oxidative stress caused by the redox cyclist paraquat was increased when the gpxA gene was inactivated (Moore and Sparling, 1996). Eukaryotic cells lacking GSH-Px activity experienced a similar phenomenon (Arthur, 2000). With the increased knowledge of prokaryotic GSH-Px, the btuE gene from E. coli and the gpoA gene from Streptococcus pyogenes are genes encoding various bacterial GSH-Px (Aho and Kelly, 1995; Moore and Sparling, 1996; King et al., 2000; Brenot et al., 2004). In E. coli, GSH-Px encoded by btuE suggests that this enzyme reduces cell oxidative stress and prevents cells from being damaged by a variety of oxidants (Arenas et al., 2010). Increased susceptibility of eukaryotic cells to paraquat is indeed present in N. meningitidis gpoA deletion mutant (Brenot et al., 2005), indicating that GSH-Px does aid in oxidative stress resistance. Surprisingly, in present research the tolerance of L. monocytogenes to metal ion-induced oxidative stress was significantly improved when gsh-px was not present, suggesting that GSH-Px has a remarkable effect in L. monocytogenes, contrary to the classical finding that the existence of GSH-Px in E. coli and N. meningitidis contributes to the antioxidant processes. However, the gsh-px deletion strain in L. monocytogenes was not affected by H2O2-induced oxidative stress. This may be due to additional antioxidant enzymes like CAT and SOD are present, which are so powerful and sensitive to H2O2-induced oxidative stress that GSH-Px did not respond significantly to it.
Audrey found that knock out gsh-px resulted in significantly impaired virulence of S. pyogenes in mice subcutaneous infection model, which provided the first concrete evidence that GSH-Px contributed to the pathogenicity of bacteria (Brenot et al., 2004, 2007). In the present work, we found enhanced virulence of L. monocytogenes when lacking the gsh-px gene, together with its adhesion and invasion efficiency, which could be accomplished by binding GSH to PrfA allosterically (Wang et al., 2017). GSH is a necessary small-molecule cofactor for PrfA, which is directly charged with the transcription of 10 essential virulence factors and has an indirect impact on the expression of over 140 other genes in L. monocytogenes. As a result, PrfA is regarded as the regulator of the major virulence factors, including Listeria pathogenic island 1 (LIPI-1) and LIPI-2, which contain plcA-prfA-hly-mpl-actA-plcB gene cluster and inlA-inlB gene cluster, respectively (De Las Heras et al., 2011). Due to the possibility of ROS, RNS and RCS being present in the phagocytic vesicles, glutathione dimers to form oxidized glutathione disulfide (GSSG), which does not bind to PrfA. However, since the host cytoplasm is a highly reduced state, once L. monocytogenes escape into the cytoplasm, all thiols are present in reduced form and the GSH is able to bind PrfA and trigger transcription of PrfA regulatory genes (PRG). Therefore, GSH acts as an irreplaceable element in the metabolic activation of PrfA (Reniere et al., 2015; Hall et al., 2016). Deletion of gsh-px gene increased concentrations of GSH in the mutant, which improved the efficiency of allosteric binding of PrfA to GSH and enhanced the regulation of virulence proteins like InlA, InlB and LLO (Kim et al., 2005), increasing pathogenicity of the gsh-px deletion strain. Moreover, transcriptome data analysis in a study revealed that the lmo0983 (gsh-px) gene was apparently down-regulated in Δgrx strains of L. monocytogenes (Sun et al., 2019). The absence of grx contributed to improve survival of L. monocytogenes when exposed to copper and cadmium ions (Sun et al., 2019), it is speculated that the resistance to oxidative stress in L. monocytogenes should be up-regulated when gsh-px was absent, which was consistent with our experimental results.
The resistance of GSH-Px to oxidative stress is linked to virulence and other bacterial species as previously mentioned. We hypothesized that increased oxidative stress resistance might be the cause of an unexpected change in the pathogenicity of gsh-px mutants. This prompts us to consider how GSH-Px of L. monocytogenes perceives and reacts to the presence of metal ions. Perhaps conformation of GSH-Px is altered or the activity of GSH-Px is changed after stimulation by metal ions, which affects the expression of GSH-Px. Figuring out the mechanisms involved is the goal of our subsequent research. In conclusion, we provided worthwhile insight into a role of GSH-Px in bacterial infections by demonstrating the L. monocytogenes GSH-Px acts a counter-intuitive agent in bacterial oxidative tolerance and intracellular infection.
Data availability statement
The original contributions presented in the study are included in the article/Supplementary material, further inquiries can be directed to the corresponding author.
Ethics statement
The animal study was reviewed and approved by Animal Ethics Committee of Yangtze University.
Author contributions
YZ and CF planned the experimental protocol and finished the manuscript. YZ and QG accomplished the main part of the study. XF, MY, and WH involved in the cell assay and animal assay. XL and JL participated in the gathering of experimental data. CF and YY participated in revising the manuscript. CF funded and oversaw the entire project. All authors contributed to the article and approved the submitted version.
Funding
The work was sponsored by the National Natural Science Foundation of China (31802208) and the Science and Technology Research Project of Education Department of Hubei Province (Q20221302).
Conflict of interest
The authors declare that the research was conducted in the absence of any commercial or financial relationships that could be construed as a potential conflict of interest.
Publisher’s note
All claims expressed in this article are solely those of the authors and do not necessarily represent those of their affiliated organizations, or those of the publisher, the editors and the reviewers. Any product that may be evaluated in this article, or claim that may be made by its manufacturer, is not guaranteed or endorsed by the publisher.
References
Aho, E. L., and Kelly, L. P. (1995). Identification of a glutathione peroxidase homolog in Neisseria meningitidis. DNA Seq. 6, 55–60. doi: 10.3109/10425179509074701
Anaya-Sanchez, A., Feng, Y., Berude, J. C., and Portnoy, D. A. (2021). Detoxification of methylglyoxal by the glyoxalase system is required for glutathione availability and virulence activation in listeria monocytogenes. PLoS Pathog. 17:e1009819. doi: 10.1371/journal.ppat.1009819
Arenas, F. A., Diaz, W. A., Leal, C. A., Perez-Donoso, J. M., Imlay, J. A., and Vasquez, C. C. (2010). The Escherichia coli btuE gene, encodes a glutathione peroxidase that is induced under oxidative stress conditions. Biochem. Biophys. Res. Commun. 398, 690–694. doi: 10.1016/j.bbrc.2010.07.002
Arthur, J. R. (2000). The glutathione peroxidases. Cell. Mol. Life Sci. 57, 1825–1835. doi: 10.1007/pl00000664
Bathige, S. D., Umasuthan, N., Godahewa, G. I., Thulasitha, W. S., Whang, I., Won, S. H., et al. (2015). Two variants of selenium-dependent glutathione peroxidase from the disk abalone Haliotis discus discus: molecular characterization and immune responses to bacterial and viral stresses. Fish Shellfish Immunol. 45, 648–655. doi: 10.1016/j.fsi.2015.05.028
Birben, E., Sahiner, U. M., Sackesen, C., Erzurum, S., and Kalayci, O. (2012). Oxidative stress and antioxidant defense. World Allergy Organ. J. 5, 9–19. doi: 10.1097/WOX.0b013e3182439613
Brenot, A., King, K. Y., and Caparon, M. G. (2005). The PerR regulon in peroxide resistance and virulence of streptococcus pyogenes. Mol. Microbiol. 55, 221–234. doi: 10.1111/j.1365-2958.2004.04370.x
Brenot, A., King, K. Y., Janowiak, B., Griffith, O., and Caparon, M. G. (2004). Contribution of glutathione peroxidase to the virulence of streptococcus pyogenes. Infect. Immun. 72, 408–413. doi: 10.1128/IAI.72.1.408-413.2004
Brenot, A., Weston, B. F., and Caparon, M. G. (2007). A PerR-regulated metal transporter (PmtA) is an interface between oxidative stress and metal homeostasis in streptococcus pyogenes. Mol. Microbiol. 63, 1185–1196. doi: 10.1111/j.1365-2958.2006.05577.x
Brigelius-Flohe, R., and Maiorino, M. (2013). Glutathione peroxidases. Biochim. Biophys. Acta 1830, 3289–3303. doi: 10.1016/j.bbagen.2012.11.020
Camilli, A., Tilney, L. G., and Portnoy, D. A. (1993). Dual roles of plcA in listeria monocytogenes pathogenesis. Mol. Microbiol. 8, 143–157. doi: 10.1111/j.1365-2958.1993.tb01211.x
Chakraborty, T., Leimeister-Wächter, M., Domann, E., Hartl, M., Goebel, W., Nichterlein, T., et al. (1992). Coordinate regulation of virulence genes in listeria monocytogenes requires the product of the prfA gene. J. Bacteriol. 174, 568–574. doi: 10.1128/jb.174.2.568-574.1992
Cheng, C., Dong, Z., Han, X., Wang, H., Jiang, L., Sun, J., et al. (2017a). Thioredoxin a is essential for motility and contributes to host infection of listeria monocytogenes via redox interactions. Front. Cell. Infect. Microbiol. 7:287. doi: 10.3389/fcimb.2017.00287
Cheng, C., Jiang, L., Ma, T., Wang, H., Han, X., Sun, J., et al. (2017b). Carboxyl-terminal residues N478 and V479 required for the Cytolytic activity of Listeriolysin O play a critical role in listeria monocytogenes pathogenicity. Front. Immunol. 8:1439. doi: 10.3389/fimmu.2017.01439
De Las Heras, A., Cain, R. J., Bielecka, M. K., and And Vazquez-Boland, J. A. (2011). Regulation of listeria virulence: PrfA master and commander. Curr. Opin. Microbiol. 14, 118–127. doi: 10.1016/j.mib.2011.01.005
Duong, C., Seow, H. J., Bozinovski, S., Crack, P. J., Anderson, G. P., and Vlahos, R. (2010). Glutathione peroxidase-1 protects against cigarette smoke-induced lung inflammation in mice. Am. J. Physiol. Lung Cell. Mol. Physiol. 299, L425–L433. doi: 10.1152/ajplung.00038.2010
Ezraty, B., Gennaris, A., Barras, F., and Collet, J. F. (2017). Oxidative stress, protein damage and repair in bacteria. Nat. Rev. Microbiol. 15, 385–396. doi: 10.1038/nrmicro.2017.26
Freitag, N. E., Port, G. C., and Miner, M. D. (2009). Listeria monocytogenes - from saprophyte to intracellular pathogen. Nat. Rev. Microbiol. 7, 623–628. doi: 10.1038/nrmicro2171
Hall, M., Grundstrom, C., Begum, A., Lindberg, M. J., Sauer, U. H., Almqvist, F., et al. (2016). Structural basis for glutathione-mediated activation of the virulence regulatory protein PrfA in listeria. Proc. Natl. Acad. Sci. U. S. A. 113, 14733–14738. doi: 10.1073/pnas.1614028114
Hedberg, C. W. (2011). Foodborne illness acquired in the United States. Emerg. Infect. Dis. 17:1338. doi: 10.3201/eid1707.110019
Kim, H., Marquis, H., and Boor, K. J. (2005). SigmaB contributes to listeria monocytogenes invasion by controlling expression of inlA and inlB. Microbiology (Reading) 151, 3215–3222. doi: 10.1099/mic.0.28070-0
King, K. Y., Horenstein, J. A., and Caparon, M. G. (2000). Aerotolerance and peroxide resistance in peroxidase and PerR mutants of streptococcus pyogenes. J. Bacteriol. 182, 5290–5299. doi: 10.1128/JB.182.19.5290-5299.2000
Ku, J. W., and Gan, Y. H. (2019). Modulation of bacterial virulence and fitness by host glutathione. Curr. Opin. Microbiol. 47, 8–13. doi: 10.1016/j.mib.2018.10.004
Kutlu, M., and Susuz, F. (2004). Biochemical properties of glutathione peroxidase in Gammarus pulex. Bull. Environ. Contam. Toxicol. 73, 432–436. doi: 10.1007/s00128-004-0447-4
Lenz, L. L., and Portnoy, D. A. (2002). Identification of a second listeria secA gene associated with protein secretion and the rough phenotype. Mol. Microbiol. 45, 1043–1056. doi: 10.1046/j.1365-2958.2002.03072.x
Limon-Pacheco, J., and Gonsebatt, M. E. (2009). The role of antioxidants and antioxidant-related enzymes in protective responses to environmentally induced oxidative stress. Mutat. Res. 674, 137–147. doi: 10.1016/j.mrgentox.2008.09.015
Mains, D. R., Eallonardo, S. J., and Freitag, N. E. (2021). Identification of listeria monocytogenes genes contributing to oxidative stress resistance under conditions relevant to host infection. Infect. Immun. 89:e00700. doi: 10.1128/IAI.00700-20
Margis, R., Dunand, C., Teixeira, F. K., and Margis-Pinheiro, M. (2008). Glutathione peroxidase family-an evolutionary overview. FEBS J. 275, 3959–3970. doi: 10.1111/j.1742-4658.2008.06542.x
Masip, L., Veeravalli, K., and Georgiou, G. (2006). The many faces of glutathione in bacteria. Antioxid. Redox Signal. 8, 753–762. doi: 10.1089/ars.2006.8.753
Mates, J. M. (2000). Effects of antioxidant enzymes in the molecular control of reactive oxygen species toxicology. Toxicology 153, 83–104. doi: 10.1016/s0300-483x(00)00306-1
Mates, J. M., Perez-Gomez, C., and Nunez de Castro, I. (1999). Antioxidant enzymes and human diseases. Clin. Biochem. 32, 595–603. doi: 10.1016/s0009-9120(99)00075-2
Monk, I. R., Gahan, C. G., and Hill, C. (2008). Tools for functional postgenomic analysis of listeria monocytogenes. Appl. Environ. Microbiol. 74, 3921–3934. doi: 10.1128/AEM.00314-08
Moore, T. D., and Sparling, P. F. (1995). Isolation and identification of a glutathione peroxidase homolog gene, gpxA, present in Neisseria meningitidis but absent in Neisseria gonorrhoeae. Infect. Immun. 63, 1603–1607. doi: 10.1128/iai.63.4.1603-1607.1995
Moore, T. D., and Sparling, P. F. (1996). Interruption of the gpxA gene increases the sensitivity of Neisseria meningitidis to paraquat. J. Bacteriol. 178, 4301–4305. doi: 10.1128/jb.178.14.4301-4305.1996
Newton, G. L., Arnold, K., Price, M. S., Sherrill, C., Delcardayre, S. B., Aharonowitz, Y., et al. (1996). Distribution of thiols in microorganisms: mycothiol is a major thiol in most actinomycetes. J. Bacteriol. 178, 1990–1995. doi: 10.1128/jb.178.7.1990-1995.1996
Reichmann, D., Voth, W., and Jakob, U. (2018). Maintaining a healthy proteome during oxidative stress. Mol. Cell 69, 203–213. doi: 10.1016/j.molcel.2017.12.021
Reniere, M. L., Whiteley, A. T., Hamilton, K. L., John, S. M., Lauer, P., Brennan, R. G., et al. (2015). Glutathione activates virulence gene expression of an intracellular pathogen. Nature 517, 170–173. doi: 10.1038/nature14029
Scortti, M., Monzo, H. J., Lacharme-Lora, L., Lewis, D. A., and Vazquez-Boland, J. A. (2007). The PrfA virulence regulon. Microbes Infect. 9, 1196–1207. doi: 10.1016/j.micinf.2007.05.007
Sun, J., Hang, Y., Han, Y., Zhang, X., Gan, L., Cai, C., et al. (2019). Deletion of glutaredoxin promotes oxidative tolerance and intracellular infection in listeria monocytogenes. Virulence 10, 910–924. doi: 10.1080/21505594.2019.1685640
Ursini, F., Maiorino, M., Brigelius-Flohe, R., Aumann, K. D., Roveri, A., Schomburg, D., et al. (1995). Diversity of glutathione peroxidases. Methods Enzymol. 252, 38–53. doi: 10.1016/0076-6879(95)52007-4
van der Oost, R., Beyer, J., and Vermeulen, N. P. (2003). Fish bioaccumulation and biomarkers in environmental risk assessment: a review. Environ. Toxicol. Pharmacol. 13, 57–149. doi: 10.1016/s1382-6689(02)00126-6
Wang, Y., Feng, H., Zhu, Y., and Gao, P. (2017). Structural insights into glutathione-mediated activation of the master regulator PrfA in listeria monocytogenes. Protein Cell 8, 308–312. doi: 10.1007/s13238-017-0390-x
Keywords: Listeria monocytogenes , glutathione peroxidase, glutathione, oxidative stress, virulence factor, pathogenicity
Citation: Zhang Y, Guo Q, Fang X, Yuan M, Hu W, Liang X, Liu J, Yang Y and Fang C (2023) Destroying glutathione peroxidase improves the oxidative stress resistance and pathogenicity of Listeria monocytogenes. Front. Microbiol. 14:1122623. doi: 10.3389/fmicb.2023.1122623
Edited by:
Peng Fei, Nanyang Institute of Technology, ChinaReviewed by:
Brandan Pedre Perez, KU Leuven, BelgiumChaoxin Man, Northeast Agricultural University, China
Copyright © 2023 Zhang, Guo, Fang, Yuan, Hu, Liang, Liu, Yang and Fang. This is an open-access article distributed under the terms of the Creative Commons Attribution License (CC BY). The use, distribution or reproduction in other forums is permitted, provided the original author(s) and the copyright owner(s) are credited and that the original publication in this journal is cited, in accordance with accepted academic practice. No use, distribution or reproduction is permitted which does not comply with these terms.
*Correspondence: Chun Fang, fangchun@yangtzeu.edu.cn
†These authors have contributed equally to this work