- 1Faculty of Medicine and Health Sciences, UCSI University, Bandar Springhill, Negeri Sembilan, Malaysia
- 2AGTC Genomics Sdn Bhd, Pusat Perdagangan Bandar, Persiaran Jalil 1, Bukit Jalil, Wilayah Persekutuan Kuala Lumpur, Malaysia
Nasopharyngeal carcinoma (NPC) is a metastasis-prone malignancy closely associated with the Epstein–Barr virus (EBV). Despite ubiquitous infection of EBV worldwide, NPC incidences displayed predominance in certain ethnic groups and endemic regions. The majority of NPC patients are diagnosed with advanced-stage disease, as a result of anatomical isolation and non-specific clinical manifestation. Over the decades, researchers have gained insights into the molecular mechanisms underlying NPC pathogenesis as a result of the interplay of EBV infection with several environmental and genetic factors. EBV-associated biomarkers were also used for mass population screening for the early detection of NPC. EBV and its encoded products also serve as potential targets for the development of therapeutic strategies and tumour-specific drug delivery. This review will discuss the pathogenic role of EBV in NPC and efforts in exploiting the potential of EBV-associated molecules as biomarkers and therapeutic targets. The current knowledge on the role of EBV and its associated products in NPC tumorigenesis, development and progression will offer a new outlook and potential intervention strategy against this EBV-associated malignancy.
1. Introduction
Epstein–Barr virus (EBV), formally known as human herpesvirus 4, is classified under the Lymphocrytovirus genus of the Gammaherpesvirinae subfamily within the Herpesviridae family. It was first discovered in Burkitt lymphoma cells during the 1960s (Young et al., 2016; Wong et al., 2022). Classified as a group I carcinogen, the virus is linked to 1.5% of all human malignancies and 1.8% of all cancer-related deaths, including Burkitt lymphoma, Hodgkin’s lymphoma, B-cell, T-cell, and NK-cell lymphoma, gastric carcinoma and nasopharyngeal carcinoma. Nasopharyngeal carcinoma (NPC) and gastric carcinoma accounted for 82% of EBV-attributed malignancies and 89% of deaths attributed to EBV-associated neoplasms. EBV is known to cause lifelong persistent infection asymptomatically in over 90% of the global population. Despite that, only a small proportion of the global community developed NPC, the incidences particularly displayed remarkable predilection towards certain populations and geographical areas. In endemic regions of NPC, EBV contributes to 95% of NPC incidences and 100% of NPC-related mortalities. In contrast, 20% of NPC incidences and 80% of NPC mortalities are attributed to EBV in low incidences areas (Khan and Hashim, 2014; Khan et al., 2020). Furthermore, NPC displays predominance in males, whereby the NPC incidences are two- to three-fold higher in men than women. The unique distribution of NPC cases implied the role of multiple factors, including host genetics and environmental factors that interplay with viral factors in NPC tumorigenesis (Chua et al., 2016; Chen et al., 2019).
NPC is histologically classified into keratinizing squamous cell carcinoma (type 1), nonkeratinizing squamous cell carcinoma (type 2), and undifferentiated carcinoma (type 3). The prevalence of EBV is 100% in type 2 and type 3 NPC, which is predominant in endemic areas (Chen et al., 2019). Currently, the Union for International Cancer Control or American Joint Committee on Cancer (UICC/AJCC) staging system is used for treatment guidance and prognosis prediction in NPC patients. The adoption of intensity-modulated radiotherapy alone or with chemotherapy for the treatment of NPC has achieved a favourable clinical outcome, with a decline in the age-standardised mortality rate over the decade. The 5-year overall survival (OS) rate is as high as 94% for patients diagnosed at an early stage, whereas a drastic decline in the 5-year OS rate (73.7%) is observed in patients with late-stage (stage III and IV) NPC (Jen et al., 2020). Nevertheless, owing to nonspecific symptoms of NPC, including headache, epistaxis, and facial pain, early detection of NPC remains a major challenge. Furthermore, 5 to 30% of NPC patients will develop post-treatment locoregional or distant recurrence (Li et al., 2014a; Lee et al., 2015; Tang et al., 2016; Jen et al., 2020; Yu et al., 2021b). Thus, diagnostic, predictive and prognostic biomarkers with high accuracy, precision, sensitivity and specificity are needed for the early diagnosis of NPC and prediction of treatment response of NPC patients. Furthermore, effective therapeutic strategies targeting the specific molecular targets in NPC could improve the clinical outcome of NPC patients. The current review discusses the oncogenic role of EBV in NPC and its translation into clinical application.
2. The pathogenic role of EBV in NPC
The non-keratinizing carcinoma, accounting for the majority of NPC cases in the endemic regions, is strongly associated with EBV infection. The disease is characterised by an enigmatic geographical and ethnic distribution, wherein East and Southeast Asia accounted for over 70% of NPC global incidences, followed by South-Central Asia (6.3%), North Africa (2.6%) and South Africa (2.4%; Ferlay et al., 2020; Sung et al., 2021; Figure 1). The disease displays predilection towards some ethnic groups, including the Cantonese population residing the Southern China, the Bidayuh in Borneo, and the Inuits living in the Arctic (Tsao et al., 2014; Chua et al., 2016). The distinctive epidemiological pattern implied that NPC pathogenesis is a multistep process involving the interplay between EBV, host genetic predisposition, and environmental factors including tobacco smoking, dietary components, and occupational exposure. Over the decades, the implementation of advanced techniques contributed to deeper insights into the genomic and epigenomic landscape, the tumour microenvironment, and the pathogenic role of EBV in NPC. Figure 2 illustrates the pathogenic model of NPC, while Table 1 briefly summarises the pathogenic role of EBV-encoded products in NPC.
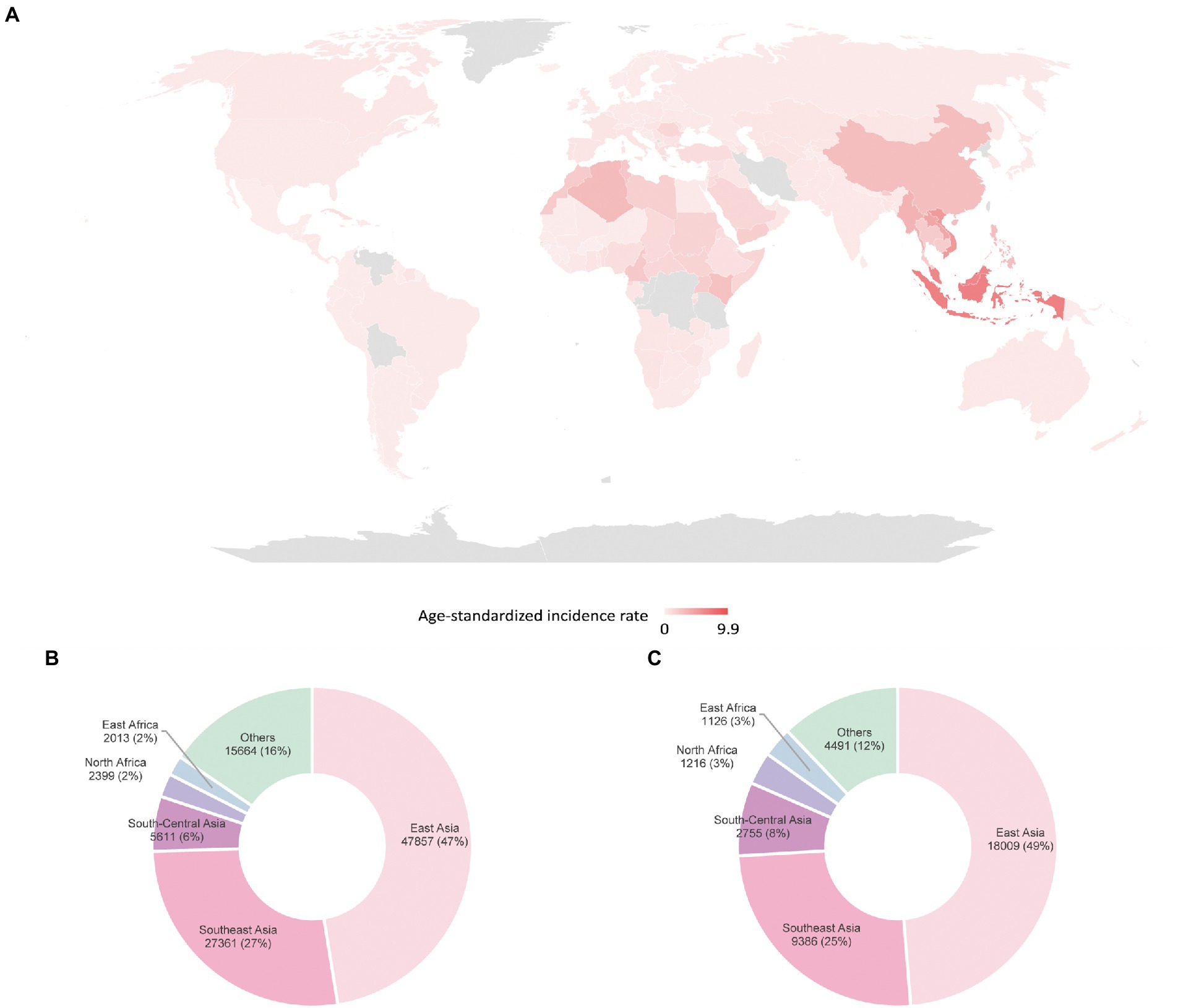
Figure 1. Global distribution of NPC estimated by GLOBOCAN in 2020 (A) age-standardised incidence rates (B) incidences in males (C) incidences in females (Sung et al., 2021).
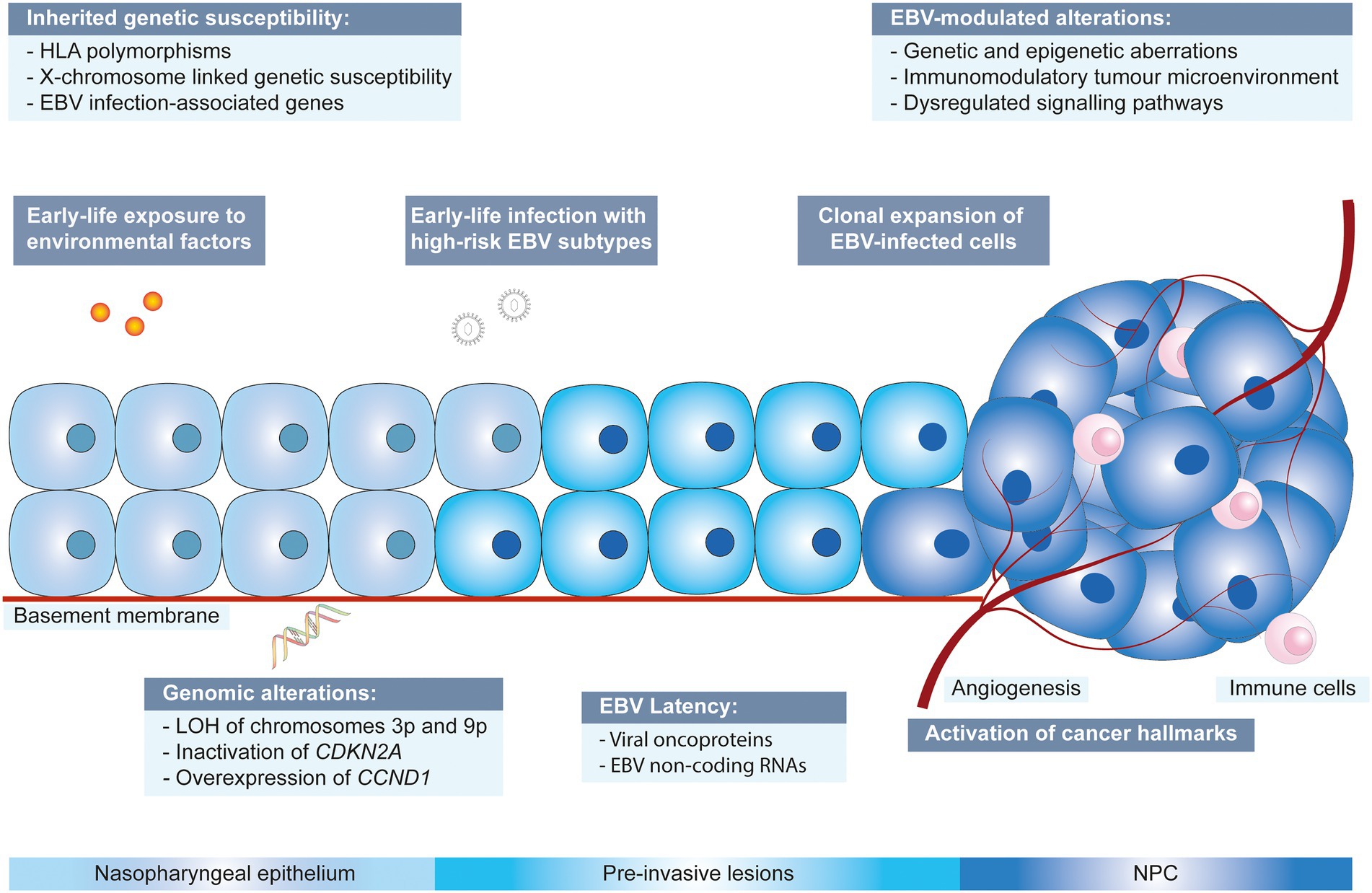
Figure 2. Multistep developmental model of NPC driven by collaboration between EBV, environmental factors and genetic susceptibility. CCND1: cyclin D1; CDKN2A: cyclin dependent kinase inhibitor 2A; EBV: Epstein–Barr virus; HLA: human leukocyte antigen; LOH: loss of heterozygosity.
Several candidate genes that predispose to NPC were reported, including genes involved in immune responses. The genetic polymorphisms of the human leukocyte antigen (HLA) complex located at the major histocompatibility complex (MHC) region on chromosome 6p21 might confer susceptibility towards NPC due to impaired EBV antigen presentation to cytotoxic cells, hence contributing to ethnicity predisposition of EBV-associated NPC (Wee et al., 2012). Furthermore, given the male predominance in NPC incidences, it is hypothesised that the involvement of an X-chromosome-linked polymorphism, which affects the innate immune response, could enhance the susceptibility towards EBV infection. A possible candidate is a gene encoding the Toll-like receptor 8 (TLR8) which displays specific polymorphisms in East Asians (Wee et al., 2012). A next-generation sequencing technology-guided single nucleotide polymorphism (SNP) analysis revealed an association between SNPs of IL-10 and SPLUNC1 (short-palate lung and nasal epithelial clone 1; also known as BPI fold containing family A member 1) with increased susceptibility to NPC (Wu et al., 2017). In addition, whole-exome sequencing on multiplex families reported gene variants potentially involved in EBV viral infection, for instance, gene encoding integrin αvβ6 ITGB6 that facilitates EBV fusion to epithelial cells. BCL2L12 (B-cell lymphoma 2-like 12) and NEDD4L (Neural precursor cell expressed developmentally downregulated gene 4-like), which encode for LMP-interacting proteins, are also associated with familial NPC (Yu et al., 2019).
Exposure to carcinogens such as acetaldehyde from alcohol and polycyclic aromatic hydrocarbons from tobacco smoking induces the formation of DNA adducts, causing impairment of DNA repair and generation of reactive oxygen species that subsequently induce genetic alterations in the nasopharyngeal epithelium and results in the formation of low-grade dysplastic lesions that favours EBV infection and persistence (Basria et al., 2019). Particularly, reports on frequent loss of heterozygosity on chromosomes 3p and 9p in the normal nasopharyngeal epithelium of individuals with a high risk of developing NPC and in low-grade dysplastic lesions indicate that the genetic events take place during early pathogenesis of NPC and may precede EBV infection (Chan et al., 2000, 2002). The deletion of cyclin-dependent kinase inhibitor 2A (CDKN2A) on chromosome 9p leads to augmented expression of cyclin D1 (CCND1) and contributes to persistent latent EBV infection through suppressing EBV-induced growth arrest and senescence (Tsang et al., 2012). On the other hand, environmental factors, particularly cigarette smoking, are linked to EBV reactivation, which generates infectious products and modulates signalling cascades involved in carcinogenesis, contributing to NPC. Cigarette smoke extract promotes EBV replication and induces the expression of lytic genes in vitro. Epidemiological studies revealed the association of smoking with persistent reactivation of EBV, as reflected by elevated plasma levels of its antibodies, which increase the risk of carcinogenesis (Xu et al., 2012; Chen et al., 2022).
The clonal origin of EBV infection in NPC implies its involvement in the early initiation of NPC (Ajadurai et al., 1995; Lo et al., 2012). It is postulated that viral-induced epithelial changes occur at the transformational zone present in the fossa of Rosenmüller during early life when regulation of the host immune response relies on TLR8. Thus, EBV infection during early childhood potentially predisposes an individual to EBV-associated NPC (Poh et al., 2016). The exact mechanism for the entry of EBV into nasopharyngeal epithelial cells remains vague. Early studies suggested that EBV-specific polymeric IgA may induce a shift of EBV tissue tropism, facilitating its infection in epithelial cells. EBV bound to IgA may enter epithelial cells via endocytosis, a process mediated by the secretory component, a transmembrane protein expressed on the basolateral surface of epithelial cells (Sixbey and Yao, 1992; Lin et al., 1997). On the other hand, as demonstrated in both in vitro and in vivo models, it is surmised that EBV infection is established in nasopharyngeal epithelial cells primarily through cell-mediated infection (Yua et al., 2018). Studies also reported the involvement of integrins αvβ5, αvβ6 and αvβ8 in triggering epithelial cell fusion mediated by EBV glycoprotein gHgL (Chesnokova et al., 2009; Chesnokova and Hutt-Fletcher, 2011). Moreover, recent studies revealed two receptors that promote EBV internalisation and fusion with nasopharyngeal epithelial cells, including Neuropilin 1 (NRP1) and ephrin receptor tyrosine kinase A2 (EphA2; Wang et al., 2015; Zhang et al., 2018). The EBV infection in NPC is typically represented by a type II latency pattern, in which Epstein–Barr nuclear antigen 1 (EBNA1), latent membrane protein (LMP)1 and 2, and non-coding RNAs are expressed and induce further genetic and epigenetic changes, contributing to the acquisition of several cancer hallmarks. These hallmark capabilities, as enumerated by Hanahan and Weinberg, include [1] infinite proliferative potential; [2] evasion of growth suppressors; [3] resistance towards apoptosis; [4] immortal replication; [5] angiogenesis; [6] invasion and metastasis; [7] metabolic reprogramming and [8] elusion from immune destruction (Hanahan and Weinberg, 2011). The dysregulation of multiple signalling pathways induced by somatic mutations or EBV latent gene expression results in the modulation of the tumour microenvironment and immune response. The latent proteins further promote tumour progression by driving a rapid clonal expansion of EBV-infected cells, leading to the accumulation of genetic and epigenetic events, resulting in NPC progression (Lo et al., 2012; Tsang et al., 2012; Wong et al., 2021).
Several studies unveiled pieces of evidence of the involvement of the EBV lytic phase, characterised by the expression of EBV lytic genes in three stages, immediate early (IE), early (E), and late (L). in oncogenesis. The IE genes, BZLF1 and BRLF1, are vital initiators of the lytic cascade that activate the E lytic genes that encode enzymes required for viral replication such as BALF3. Subsequently, L lytic genes are expressed and cell lysis takes place to release viral particles (Liang et al., 2022; Yap et al., 2022). Serological studies revealed the association between IE and E lytic genes with tumour progression and immune cell infiltration, suggesting the presence of intermittent abortive reactivation of EBV in NPC patients, the frequency whereof increases following disease progression (Guo et al., 2019). The lytic phase contributes to tumorigenesis by producing infectious virions that spread EBV infection to other cells and regulating cellular oncogenic pathways, including immune response, angiogenesis, invasion, apoptosis, and others (Rosemarie and Sugden, 2020).
2.1. EBV induces genetic instability in NPC
EBV induces genetic instability in infected premalignant cells through dysregulation of genes involved in [1] DNA repair; [2] cell cycle checkpoint; [3] anti-oncogenic activities. Through the PI3K/Akt/FOXO3a pathway, EBV-encoded LMP1 represses DNA damage-binding protein 1 (DDB1)-mediated nucleotide excision repair (Chen et al., 2008). Moreover, LMP1 induces G2 checkpoint defect by impairing Chk1 activation, allowing unrepaired chromatid breaks to undergo mitosis, which further propagates and accumulates, leading to chromosomal instability (Deng et al., 2012). On the other hand, EBV-encoded miRNAs, including miR-BART5-5p, miR-BART7-3p, miR-BART9-3p, miR-BART14-3p, modulate the activity of ataxia telangiectasia mutated (ATM) gene, which is involved in the repair of DNA double-strand break (Lung et al., 2018). EBNA1 is shown to promote the survival of cells harbouring DNA damage, contributing to NPC development. EBNA1 disrupts the promyelocytic leukaemia nuclear bodies (PML NBs) through interaction with cellular ubiquitin-specific protease (USP)7 or HAUSp, interfering with p53 acetylation, DNA repair and apoptosis (Sivachandran et al., 2008). EBV-encoded lytic gene product BALF3 could induce chromosome aberrations and DNA strand breaks in the host cells (Chiu et al., 2014).
EBV contributed to non-mutational genetic instability through the regulation of gene expression by inducing aberrant epigenetic alterations including DNA methylation and histone modification in NPC. A high frequency of hypermethylation was detected within or near the CpG islands (CGI) of malignant tissue, with significant enrichment of histone-bivalent marks at the methylated regions (Dai et al., 2015). EBV latent proteins, including EBNA1 and LMP1, could interact with DNA methyltransferases (DNMTs) or demethylases to modulate epigenetic modifications (Tsai et al., 2006; Shi et al., 2019). Via NF-κB signalling, LMP1 induces the hypermethylation and inactivation of the tumour suppressor phosphatase and tensin homologue (PTEN) by activating DNMT3b (Peng et al., 2016). DNA methylation and histone modification liaise to induce epigenetic deregulation in NPC. EBV infection dysregulates MLH1, a DNA repair pathway gene, via aberrant histone bivalent switches. The MLH1 downregulation is associated with the reduced trimethylation of histone H3 lysine 4 (H3K4me3) and enhanced H3 lysine 27 trimethylation (H3K27me3; Leong et al., 2019).
2.2. EBV contributes to the construction of tumour microenvironment and immune evasion
The tumour microenvironment of NPC is featured by its abundance of immune cells, albeit with limited antitumour activities. EBV contributes to the accumulation of immune cells in the NPC microenvironment and immunosuppression in NPC, thus promoting tumour initiation, growth and development. Through CTAR1 and 2, LMP1 induces the upregulation of chemokines, including T-lymphocytes recruiting macrophage inflammatory protein (MIP)-1α and -1β, via NF-κB and JNK signalling cascades (Lai et al., 2010). EBV-encoded RNAs (EBERs) trigger inflammatory responses characterised by a rise in TNF-α levels in NPC via the TLR3 pathway. EBERs also collaborate with LMP1 through an NF-κB-mediated positive feedback loop to amplify the inflammatory response in NPC (Li et al., 2015).
EBV-encoded latent proteins and miRNAs contribute to viral persistent infection and tumorigenesis by compromising the host immune responses through the interference of cytokine signalling networks and antigen presentation, promoting the infiltration of immune-regulating cells in the NPC immune microenvironment, and inducing immune cells anergy. EBV modulates the innate immune response by the disruption of chemokine and cytokine signalling pathways. LMP2A and 2B modulate interferon (IFN) activity by accelerating the internalisation of IFN receptors (IFNRs; Shah et al., 2009). LMP1 and EBV lytic transactivator BZLF1/Zta induce the upregulation of immunosuppressive cytokines such as interleukin (IL)-10 that potentiates regulatory function in CD4+ T helper cells and reduces cytotoxic T-cell infiltration. IL-10 is positively associated with the expression of Fas ligand (FasL) expression, which might trigger Fas-mediated apoptosis in antitumour immune cells (Yao et al., 1997; Ogino et al., 2007; Pai et al., 2007; Lee et al., 2011). EBV-miR-BART6p targets the pattern recognition receptor retinoic acid-inducible gene (RIG)-I mRNA to inhibit EBV-triggered IFN-β response (Lu et al., 2017). EBV recruits regulatory immune cells into the NPC immune microenvironment, promoting immune evasion. EBNA1 promotes the accumulation of immunosuppressive Foxp3+ regulatory T-lymphocytes (Tregs) by driving C-C motif ligand (CCL)20- and transforming growth factor (TGF)-β-mediated infiltration of Tregs into the tumour site and stimulates the conversion of naïve T-lymphocytes into Tregs via upregulation of TGF-β and polarisation of M2 macrophages (Huo et al., 2020; Wang et al., 2020). Alteration of the metabolic pathway leads to the expansion of myeloid-derived suppressor cells (MDSCs) in the NPC microenvironment (Cai et al., 2017). EBV induces exhaustion in immune cells by upregulating co-stimulatory molecules. Through STAT3, AP-1, and NF-κB pathways, LMP1 modulates the immune checkpoint programmed cell death protein 1 (PD-1)/ programmed death ligand 1 (PD-L1), facilitating immune evasion (Fang et al., 2014). EBV-miR-BART11 and EBV-miR-BART17-3p enhance PD-L1 transcription by inhibiting FOXP1 and PBRM1, respectively (Wang et al., 2022).
EBV drives angiogenesis in NPC, which is necessary for the transport of oxygen and nutrients to the malignant tissue. EBV infection induces store-operated Ca2+ entry (SOCE)-mediated angiogenesis through upregulation of its regulator, stromal interaction molecule 1 (STIM1; Ye et al., 2021). EBV-infected cells induce chemokine CCL5-mediated tumour angiogenesis via PI3K/AKT and HIF-1α pathways (Ma et al., 2018). Enhanced expression of transcription factor activator protein (AP)-1 by EBNA1 elevates the expression of angiogenic cytokine IL-8 and vascular endothelial growth factor (VEGF), leading to angiogenesis in NPC (Oneil et al., 2008). Exosome-packaged EBERs upregulate the expression of vascular cell adhesion molecule 1 (VCAM-1) expression to promote angiogenesis, the process involving TLR3 and RIG-I (Cheng et al., 2019). EBV-miR-BART1-5p induces angiogenesis by regulating the AMPK/mTOR/HIF-1 pathway (Wang et al., 2018). Besides classic angiogenesis, EBV induces vasculogenic mimicry, a process whereby malignant cells form vessel-like structures independent of endothelial cells. Activation of the PI3K/AKT/mTOR/HIF-1α signalling cascades by LMP2A promotes vasculogenic mimicry in NPC (Xiang et al., 2018). On the other hand, LMP1 induces the formation of vasculogenic mimicry by VEGF/VEGFR1 signalling (Xu et al., 2018).
2.3. EBV promotes metastasis and invasion
Metastasis is a complex process involving a series of events, including [1] invasion of tumour cells into adjacent tissues, [2] intravasation into the circulatory system, [3] survival in the peripheral blood circulation, [4] extravasation into distant tissues, and [5] colonisation (Lambert et al., 2017). Epithelial cells undergo epithelial-mesenchymal transition (EMT) to enhance their motility and invasiveness, the outcome marked by a decrease in epithelial markers such as E-cadherin and an increase in mesenchymal markers including vimentin, and morphological change into spindle-like shape. Studies revealed the prominence of cells with spindle-like morphology and expression of molecular markers associated with cancer stem cells and EMT at the invasive front of NPC tumours, these characteristics highly correlated with EBER and LMP1 (Luo and Yao, 2013). LMP2A was also reported to be associated with side population stem-like cancer cells, but not morphologic change (Kong et al., 2010). LMP1 promotes EMT through the regulation of transcription factors, including Snail and Twist (Horikawa et al., 2007, 2011). LMP1 increases the expression of calreticulin, promoting EMT via the TGF-β/Smad-3/NRP1 axis (Ye et al., 2020). Through the mTOR pathway, LMP2A induces the overexpression of metastatic tumour antigen 1 (MTA1) to promote EMT in NPC (Lin et al., 2014). By mediating the TGF-β1/ zinc finger E-box binding homeobox (ZEB)/ miR-200 feedback loop, EBNA1 induces EMT in NPC (Li et al., 2014b). EBV-miR-BART7-3p, BART8-3p, BART10-3p, BART13-3p, and BART22 induce the EMT process by targeting PTEN, RNF38, BTRC, ABI2 and MOSPD2 genes, respectively, in NPC cells (Cai et al., 2015; Yan et al., 2015; Lin et al., 2018a; Huang et al., 2020; Zhang et al., 2022).
EBV targets cell adhesion molecules including cadherin and integrin to induce a mesenchymal-like phenotype of the host malignant cells. LMP1 downregulates the expression of E-cadherin by inducing hypermethylation of its gene promoter CDH1 (Tsai et al., 2002, 2006). EBV-encoded miRNA-BART9 elicits inhibitory action on E-cadherin, promoting the invasiveness property of NPC cells (Hsu et al., 2014). LMP2A promotes the motility of NPC cells by regulating the localization of the integrin β4 (ITGβ4) subunit at the cellular protrusions. LMP2A competitively bind to the spleen tyrosine kinase (Syk), which is responsible for the internalisation of the ITGβ4 subunit and inhibition of cell migration. Moreover, LMP2A accelerates the cleavage of ITGβ4 through the EGFR/ Ca2+/ calpain/ ITGβ4 pathway (Zhou et al., 2014; Liang et al., 2017).
Studies unveiled other metastatic mechanisms utilised by EBV, including the expression of matrix metalloproteinases (MMPs), which degrade the basement membrane and allow the invasion of NPC cells. LMP1 interacts with the tumour necrosis factor receptor family-associated factors (TRAFs) through CTAR-1 and CTAR-2, contributing to the activation of the MMP-9 promoter. It further induces the MMP-9 enzymatic activity through the activation of the NF-κB and AP-1 transcription factors (Takeshita et al., 1999). LMP2A triggers the expression of MMP9 through the ERK1/2-Fra-1 axis through its PY motifs (Lan et al., 2012). BRLF1/Rta promotes invasiveness in NPC through paracrine secretion of MMP-9 (Lan et al., 2018). Other than that, LMP1 upregulates neurotrophic tyrosine kinase receptor type 2 (NTRK2 or TrkB) expression to enhance anoikis resistance, which is necessary for survival after detachment from the tumour, of NPC cells through NF-κB signalling (Li et al., 2020).
2.4. EBV promotes metabolic reprogramming In NPC
The Warburg effect, or aerobic glycolysis, is commonly observed in malignant cells. EBV modulates the metabolic switch in NPC cells through the alteration of mitochondrial function, contributing to elevated glycolysis, enhanced proliferation, and apoptotic resistance (Xiao et al., 2014; Sung et al., 2017). LMP1 downregulates PTEN/AKT signalling through by upregulating the expression and activity of DNMT1 to activate the glycolytic flux. Furthermore, LMP1 promotes the mitochondrial translocation of DNMT1, thus inducing the hypermethylation of the mitochondrial DNA D-loop region and downregulation of oxidative phosphorylation (OXPHOS) complexes causing metabolic reprogramming in NPC (Luo et al., 2018). EBV is involved in the regulation of glucose metabolism through multiple signalling pathways. Through AKT/ERK/IKK signalling, LMP1 induces mTORC1 activation, which subsequently induces NF-κB signalling and enhances the transcription of glucose transporter (GLUT)1, thereby increasing glucose uptake in NPC cells (Zhang et al., 2017). Furthermore, LMP1 induces the expression of fibroblast growth factor receptor (FGFR)1 and activates its signalling, thus facilitating aerobic glycolysis through the phosphorylation of glycolytic pathway-associated proteins such as lactate dehydrogenase (Lo et al., 2015). BART1-5p activates the AMPK/mTOR/HIF1 pathway, facilitating the conversion to aerobic glycolysis (Wang et al., 2018). EBV modulates multiple transcription factors such as HIF-1α, c-Myc, and HoxC8 to alter glucose metabolism in NPC. LMP1 extends the half-life of c-Myc, a master regulator of energy metabolism, by attenuating the PI3K/Akt-GSK3β-FBW7 signalling axis, which mediates the ubiquitination-dependent degradation of c-Myc. C-Myc subsequently participates in LMP1-mediated upregulation of hexokinase (HK)2, a key player in glycolysis (Xiao et al., 2014). Through stalling RNA polymerase II, LMP1 represses Homeobox (Hox) genes, including a glycolytic regulator HoxC8 (Jiang et al., 2015). The shift in glucose metabolism facilitates tumorigenesis through its contribution to EBV-mediated immortalisation of infected cells, sustained EBV latency, apoptotic resistance and modulation of host immune response (Yang et al., 2022).
Besides glycolytic flux, metabolic shifts in lipids and amino acids were reported in NPC. LMP2A downregulates adipose triglyceride lipase, inhibiting lipid catabolism. This results in the accumulation of lipid droplets in NPC cells, thereby enhancing their migratory capabilities (Zheng et al., 2020). Plasma lipidomic revealed the association of plasma lipid profile with the titre of EBV antibodies reflecting the EBV lytic-latent cycle and with disease progression. Therefore, it is suggested that EBV potentially affect the prognosis of NPC by regulating lipid metabolism (Huang et al., 2022). Furthermore, NPC cells expressing LMP1 display enhanced consumption of glutamine (Lo et al., 2015).
The unique geographical and ethnic predominance of NPC highlights the importance of interplay among EBV, genetic susceptibility and environmental factors in the initiation and development of NPC. The advancement in multi ‘omic’ technologies has shed light on the understanding of the complex interactions in NPC carcinogenesis. The chemical carcinogen-induced genetic aberrations and inherited genetic susceptibility potentially enhanced individual predisposition towards EBV infection, while facilitating the establishment of latency and contributing to malignant alterations in host cells. Further elucidation of the precise pathogenic role of EBV in NPC would assist in the discovery of useful biomarkers and therapeutic targets. The development of more efficient in vitro systems for the investigation of the EBV life cycle in epithelial cells would allow more comprehensive studies of viral-host interaction in the oncogenesis of NPC. Future studies are required to define the biological role of the identified genes underlying genetic susceptibility towards NPC and their interactions with viral factors. The increase in sample size is suggested to obtain results with improved reliability and reproducibility, thus allowing the stratification of individual risk towards NPC and the development of cost-effective NPC screening strategies in high-risk populations. Furthermore, as environmental factors are associated with the development of NPC, the establishment of lifestyle modification programmes or guidelines targeting high-risk communities is recommended to minimise their risk of developing NPC. The strong association between EBV and NPC urges the development of prophylactic vaccines against EBV and validation of their long-term efficacy in preventing NPC, particularly in endemic regions.
3. EBV subtypes
Despite the widespread EBV infection which affects 90% of the global adult population, EBV-associated NPC displayed a skewed geographical distribution, suggesting the role of EBV sequence variation in NPC pathogenesis. This spurred studies into the geographical and ethnic correlation of various EBV subtypes and their role in the development of specific EBV-associated neoplasms. The major variation of the alleles at the EBNA2 and 3 s gene sequences clustered EBV into type 1 and type 2 strains. Type 1 EBV, including B95.8, Akata, Mutu, C666-1, M81, GD1, and GD2, displayed global prevalence and is commonly associated with NPC. On the other hand, type 2 EBV such as AG876, Jijoye, and Wewak is prevalent in Papua New Guinea, Alaska, and sub-Saharan Africa (Palser et al., 2015; Weiss et al., 2018).
EBV viral genomic analysis is imperative for the study of the association between viral sequence variation and EBV-associated diseases. Various EBV strains were isolated from NPC and fully sequenced, including two strains isolated from Cantonese NPC patients, Guangdong (GD)-1 (Zeng et al., 2005) and -2 (Liu et al., 2011), strains isolated from Hong Kong patients with NPC, HKNPC1-9 (Kwok et al., 2012, 2014) and M81 (Tsai et al., 2013), Yu103 (Yu et al., 2021a) from a Singaporean NPC patient, and an NPC cell line-derived C666-1 strain (Tso et al., 2013; Table 2). The EBV isolates exhibit distinct phenotypic properties, for instance, the M81 strain displays enhanced tropism towards epithelial cells and reduced tropism towards B-lymphocytes, which may be due to the abundance of the glycoprotein gp110 (Tsai et al., 2013). On the other hand, Yu103 simultaneously infects and transforms both B-lymphocytes and epithelial cells, thus possessing the capacity to drive both haematological and epithelial malignancies (Yu et al., 2021a).
The latency-associated genes possess the highest frequency of SNP (Palser et al., 2015). Notably, the LMP1 gene sequence displays a high degree of polymorphism when compared with other EBV genes. These include a 30 base-pair (bp) deletion at the cytoplasmic carboxyl (C)-terminal tail, which is associated with a more aggressive phenotype of EBV-associated tumour. It is reported that a 30 bp deletion results in codon variation of the T-cell epitope, leading to potential escape from host cell immune recognition. Furthermore, a point mutation involving a single nucleotide substitution at position 169,425 results in the loss of the XhoI restriction site of the cytoplasmic amino (N)-terminal tail. These mutations are suggested to be associated with NPC susceptibility (Lin et al., 2003; Tang et al., 2008; da Costa et al., 2015; Banko et al., 2021). The LMP1 gene heterogeneity defines several variants, which are Alaskan, China 1, China 2, China 3, Mediterranean (Med)+, Med-, and North Carolina (NC; Edwards et al., 1999).
The polymorphisms at the codon 487 of EBNA-1 at the C-terminal give rise to 5 subtypes, including two prototypes P-Ala and P-Thr and three variants V-Val, V-Leu, and V-Pro. The V-Val subtype, which is commonly detected in NPC biopsies from Asian patients, might contribute to NPC tumorigenesis by enhancing the expression of viral or cellular genes (Zhang et al., 2004; Van Do et al., 2008; Mai et al., 2010; Lha et al., 2019). NPC-derived EBNA1 genes typically possess polymorphisms in their DNA-binding domain, leading to defective DNA replication and episome maintenance that potentially increases NPC risk due to unstable latent infection and aberrant expression of lytic cycle genes (Dheekollu et al., 2017).
Variations in the EBV lytic gene contribute to increased NPC risk. The BALF2 H-H-H endemic strain carrying three non-synonymous single nucleotide variants (BALF2 162215A > C [V700L], 162,476 T > C [I613V], and 163364C > T [V317M]) in the EBV genome is associated with NPC in southern China. The SNPs 162,476_C and 163364_T confer an increase in risk exceeding 6-fold for NPC and cumulatively contribute to 83% of overall NPC risk in southern China (Xu et al., 2019; Miller et al., 2021; Kondo et al., 2022). Furthermore, the amino acid substitution in V1222I of BNRF1 is found to be associated with NPC risk in China. The variations in BALF2 and BNRF1 may result from viral interaction with the host immune system (Xue et al., 2021).
Recent studies identified several polymorphisms associated with EBV risk within the EBV genome. These include a single nucleotide polymorphism within the EBV-encoded RPMS1 gene (G155391A) which was reported to be associated with a high risk of NPC (Feng et al., 2015). Further investigation of the RPMS1 gene polymorphisms revealed four genotypes, RPMS1-A to D, whereby the variation is correlated with geographical region and type of malignancy (Wu et al., 2018). Other than that, a four-base deletion within the EBER locus was detected in approximately 97% of NPC cases (Fung Hui et al., 2018).
Viral genetic variation potentially elicits an influential effect on NPC carcinogenesis, though the relationship between EBV genetic variation and the risk of NPC is yet to be established. Furthermore, the majority of studies on EBV genotyping were limited to certain regions. Moreover, it was reported that EBV sequence variation is associated with host sequence variants, hence, joint analyses of host and viral genome variation would help decipher the pathogenesis of NPC and identify novel diagnostic and therapeutic targets (Rüeger et al., 2021). Studies recruiting larger samples involving individuals with different conditions from different ethnic groups and geographical regions are needed to determine the exact association of EBV strains with the geographical distribution and development of the disease. Further characterisation of mutations in the high-risk EBV variants would facilitate the delineation of molecular mechanisms that take place in the infected host cells. The implementation of new techniques, such as clustered regularly interspaced short palindromic repeats (CRISPR)/Cas9 system would allow rapid cloning and sequence determination of EBV variants (Yajima et al., 2018).
4. EBV-based biomarkers in NPC
The insignificant clinical manifestations of NPC, including otologic conditions, neck mass, nasal issues, and headache, and its anatomic isolation led to delayed diagnosis and treatment, whereby patients only start seeking medical attention 6 weeks after the onset of symptoms. A misdiagnosis rate of as high as 43.4% was reported in NPC patients (Wu et al., 2016; Wang et al., 2017). Based on the AJCC/IUCC’s 8th edition TNM staging system for NPC, the five-year OS for NPC patients diagnosed at early stages (stages I and II) are more than 90%, whereas in patients diagnosed with advanced stages NPC, the five-year OS decline drastically, whereby a 5-year OS of 73.7% is predicted in patients with stage IVA NPC (Jen et al., 2020). Thus, early diagnosis of NPC is essential to improve the survival and prognosis of patients. Nasoendoscopy examination combined with pathologic analysis of biopsy remains the current gold standard for clinical NPC detection. The approach is however invasive and not suitable for asymptomatic individuals (Zhang et al., 2021b). This warrants the development of non-invasive tests for the diagnosis of NPC which offers high specificity, sensitivity, accuracy and reliability. Given the close association between EBV and NPC, EBV represents a promising target for the development of screening, diagnostic and prognostic biomarkers for NPC.
A two-stage screening model is used for population screening in endemic regions. The model consists of a primary screening method using serological or molecular markers, followed by secondary screening using imaging or endoscopy methods. Conventionally combined detection of VCA/ IgA and EA/IgA using immunoenzymatic assay was performed in endemic areas of NPC, allowing the early diagnosis of NPC with a rate ranging from 55 to 87% (Zeng et al., 1980, 1982; Ji et al., 2002; Lo et al., 2022). The increased titres of EBV-specific IgA and IgG antibodies in the serum of NPC patients, as first reported in the 1970s, provide a rationale for the intervention (Henle and Henle, 1976; Ho et al., 1976). Studies revealed the presence of a serologic window wherein serological EBV-VCA IgA antibody levels are elevated and sustained which precedes the clinical onset of NPC, suggesting EBV-VCA IgA as an early marker of NPC (Ji et al., 2007). The development of the ELISA technique for the detection of multiple EBV serological markers, including immunoglobulins to VCA, EA, EBNA1, Zta, and replication and transcription activator (Rta), aims to improve the accuracy, sensitivity and specificity of the screening assay. Plasma EBNA1-IgA demonstrates a remarkable distinguishing ability between early-stage NPC and normal subjects with specificity and sensitivity as high as 98.7 and 91.5%, respectively. Combined detection of VCA-IgA with EBNA1-IgA by ELISA enables the diagnosis of NPC patients with improved specificity and sensitivity (Fachiroh et al., 2006; Liu et al., 2012, 2020).
By using conventional PCR targeting EBNA, Mutirangura et al. detected the presence of EBV DNA, which might be originated from NPC tumour, in 31% of blood samples obtained from NPC patients and suggested its potential as a marker for the diagnosis of NPC (Mutirangura et al., 1998). Case–control studies employing the real-time PCR (qPCR) strategy to target the BamHI-W and EBNA1 regions of the viral genome and detected cell-free EBV DNA in over 95% of plasma from NPC patients (Lo et al., 1999; Lin et al., 2004). Large-scale screening of high-risk populations combining qPCR-based detection of EBV DNA, nasal endoscopic examination and magnetic resonance imaging (MRI) allowed the identification of early-stage NPC, with specificity and sensitivity of 98.6 and 97.1%, respectively, (Lam et al., 2017). The sensitivity of EBV DNA for the detection of early-stage NPC (90%) was limited when compared to advanced-stage NPC (98%), implying the need for parallel screening to enhance diagnostic sensitivity. For instance, the combination of EBV DNA and IgA-VCA as marker panels could enhance the diagnostic sensitivity to 99% (Leung et al., 2004). Moreover, high levels of EBV DNA load can be detected in the nasopharyngeal swab of NPC patients with a lower rate of false-positive and -negative when compared to plasma EBV DNA, suggesting nasopharyngeal EBV DNA as an alternative with less invasiveness and higher accuracy than plasma EBV DNA (Zheng et al., 2015a). Other than that, sequencing-based detection of plasma EBV DNA displayed superior positive predictive value over qPCR and was able to distinguish the molecular characteristic of circulating EBV DNA between NPC and non-NPC individuals, thus reducing false positives for the diagnosis of NPC (Lam et al., 2018).
The plasma EBV DNA concentration is correlated with the disease stage, clinical outcome and overall survival of NPC patients, indicating its potential as a prognostic indicator for staging and monitoring of therapeutic effect (Lo et al., 1999; Lin et al., 2004). Patients with undetectable post-treatment plasma EBV DNA had better survival, while the presence of EBV DNA in plasma following treatment predicts distant metastasis. It is suggested that the integration of EBV DNA with other clinicopathologic parameters before, during, and after treatment could facilitate outcome prediction, treatment strategy tailoring, response monitoring, and post-treatment surveillance. Prognostic nomograms incorporating EBV DNA load and the UICC/AJCC staging system were established for prognosis prediction and treatment stratification in patients with locally advanced NPC (Xu et al., 2017). A recent large-scale study established a recursive partitioning analysis model that classified elderly patients with NPC into risk groups based on EBV DNA load and T stage and the chemotherapy benefits in each group were assessed. In the poor prognosis group, defined by EBV DNA load of fewer than 4,000 copies/mL with stage T3–4 or EBV DNA of more than 4,000 copies/mL with any T stage, patients receiving chemoradiotherapy had significantly improved OS compared with patients receiving only radiotherapy (Wu et al., 2022). Pre- and post-treatment plasma EBV DNA titres were predictive of the risk of disease progression, metastasis, recurrence, and death, and thus are promising biomarkers for prognosis stratification and post-treatment tumour surveillance (Li et al., 2021). Nevertheless, the heterogeneity of the assay methodologies and lack of a standardised reference system leads to interlaboratory discrepancies. Thus, standardisation of methods and cut-off values for EBV DNA assays is required to ensure reproducibility and improve the efficacy of the marker (Lee et al., 2021).
Besides that, EBV-encoded miRNAs emerge as potential diagnostic and prognostic biomarkers of NPC. miRNA profiling using qPCR detected the elevation of a series of EBV-encoded BART miRNAs, including BART2-5p, BART6-3p, BART7-3p, BART7-5p, BART9-5p, BART11-3p, BART17-5p, and BART19-5p in the plasma samples of patients with primary NPC, wherein BART19-5p displayed the best performance for the detection of NPC in patients with undetectable sera EBV DNA (Gao et al., 2019). Sera miRNA BART2-5p allows the identification of preclinical NPC from high-risk populations with 90.9% sensitivity and 54.5% specificity (Jiang et al., 2018). Moreover, enhanced plasma levels of BART2-3p, BART2-5p, BART5-3p, BART5-5p, BART6-3p, BART8-3p, BART9-5p, BART17-5p, BART19-3p, and BART20-3p were associated with recurrent NPC, among which BART8-3p and BART10-3p demonstrated the highest ability to distinguish EBV DNA undetectable recurrent NPC (Gao et al., 2019). Abundant levels of miRNAs BART7 and BART13 are detected in the plasma samples from NPC patients, the levels increase with disease progression. miRNAs BART7 and BART13 might serve as prognostic biomarkers that provide useful clinical information on disease stage and treatment efficacy (Zhang et al., 2015; Lu et al., 2020). Large-scale studies should be conducted to further validate the efficacy of EBV-encoded miRNAs as biomarkers in NPC.
To facilitate the integration of these biomarkers into clinical use, the method and cut-off value should be standardised to produce accurate and reproducible results. Moreover, large-scale clinical studies with consideration of geographical, genetic, and environmental factors should be conducted. Research on big data analytics would shed light on the disease pattern and its associated factors. The integration of patient information from the aspect of genomics, proteomics, imaging, serological profile, demographics and others allows for the tailoring of a personalised treatment strategy for each individual. Artificial intelligence is developed to provide insights into intervention strategies for precision medicine. Researchers have developed machine learning and deep learning models for the prediction of survival, disease progression, and treatment response for NPC patients by integrating several clinical parameters, including images, demographic characteristics, plasma EBV-DNA, serum biochemical profile, treatment regimen and others (Jing et al., 2019; Zhong et al., 2021; Chen et al., 2021a; Zhang et al., 2021a; Ng et al., 2022).
5. Ongoing clinical and preclinical studies on therapeutic targeting EBV in NPC
Over the last few decades, advances in the management of NPC have brought about a significant improvement in the prognosis of patients. The current treatment strategies for NPC patients, as outlined in the National Cancer Comprehensive Network (NCCN) guideline (v 1.2018) and ESMO-EURACAN guideline, are mainly based on AJCC/IUCC staging. IMRT is the current benchmark for the management of early-stage NPC (stage I-II), with locoregional control exceeding 90%. Unfortunately, nearly 70% of patients are presented with locoregionally advanced disease upon diagnosis, leading to poor outcomes. The addition of concurrent cisplatin chemotherapy to radiation in patients with advanced disease (stage III-IVb) significantly improves the prognostic outcomes (Au et al., 2018; Colevas et al., 2018; Bossi et al., 2021; Yang et al., 2021). Despite remarkable improvement in the clinical benefit of NPC patients, 5 to 15% of patients receiving IMRT will develop locoregional failures, while 15 to 30% of patients will experience distant failure. Furthermore, patients also suffer from late toxicities, such as neurologic damage and hearing loss (Lee et al., 2015). The development of effective therapeutic strategies which address specific molecular targets of NPC would improve the efficacy of cancer therapy while reducing its toxicity and cost. The presence of EBV in undifferentiated NPC cases and premalignant lesions imply the significant role of EBV in the development of NPC. Given the oncogenic role of EBV in NPC, studies have exploited various approaches targeting EBV, including immunotherapy, lytic reactivation, latent proteins-targeting peptides, and genetic therapy (Table 3).
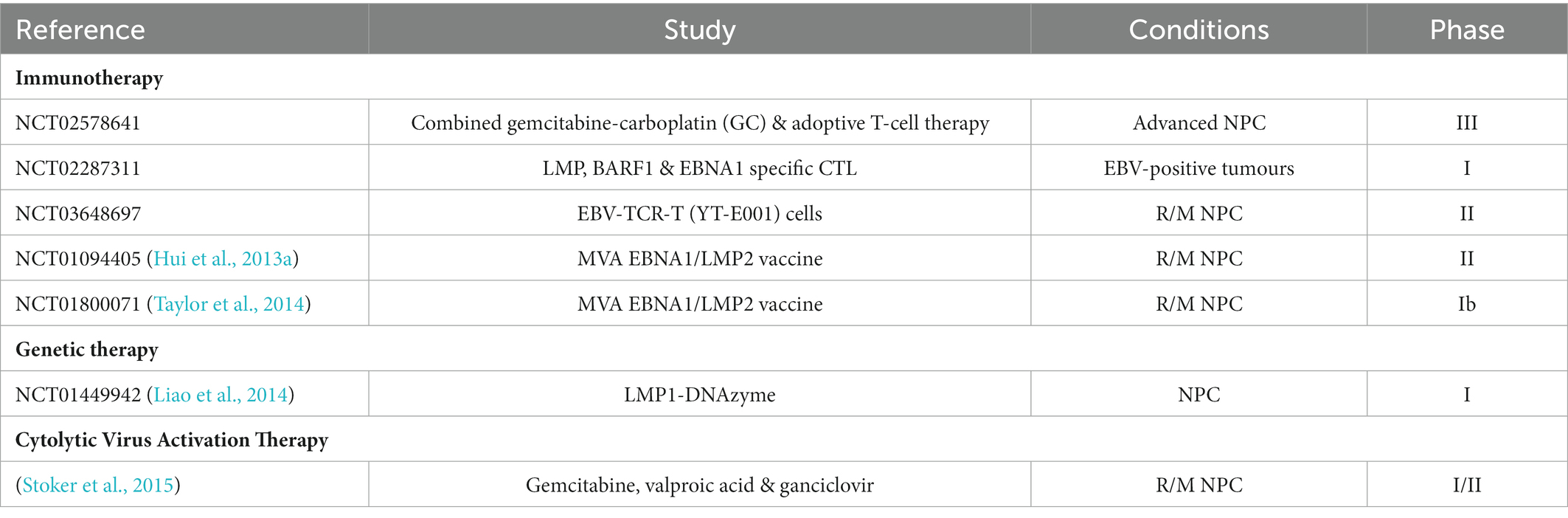
Table 3. Clinical studies on EBV-targeting therapeutics for NPC. R/M NPC: recurrent/ metastatic NPC.
5.1. Immunotherapy
Cellular-based immunotherapy targeting EBV in NPC can be further classified into two approaches, passive immunotherapy or adoptive immunotherapy, and active immunotherapy or cancer vaccines. Adoptive immunotherapy involved the infusion of EBV-targeting cytotoxic T-lymphocytes (CTLs) into NPC patients. Autologous EBV-specific effector T-cells include polyclonal T-cell lines reactivated ex vivo using autologous EBV-transformed lymphoblastoid cell line (LCL) and T-cells selectively reactivated using recombinant viral vectors or peptides. Phase I/II clinical studies reported the safety and well-tolerance of immunotherapy involving the adoptive transfer of autologous EBV-CTLs generated ex vivo by stimulation with EBV-transformed LCLs, albeit with limited efficacy (Straathof et al., 2005; Louis et al., 2010; Chia et al., 2014). Following infusion, the patients displayed a reduction in EBV viral load and improved response to chemotherapy. Patients with advanced NPC administered with chemotherapeutic agents, gemcitabine and carboplatin, combined with adoptive transfer of EBV-CTL demonstrated improved clinical outcomes when compared with patients receiving only chemotherapy, with an improved median overall survival from 17.7 months to 29.9 months, and an increase in 2-years OS rates from 29.5 to 62.9% (Chia et al., 2014). A phase III clinical study (NCT02578641) is currently being conducted across Asia and the United States to further evaluate the safety and efficacy of combined EBV-CTL adoptive immunotherapy with gemcitabine-carboplatin chemotherapy. Although potent antitumour activity was observed in patients with locoregional disease, patients with metastatic disease displayed only limited antitumour activity (Louis et al., 2010). Furthermore, the approach preferentially expanded EBNA3-specific T-cells, which are not expressed in NPC (Straathof et al., 2005). The generation of LCL-expanded EBV-specific CTLs is time-consuming, taking about 8 to 22 weeks, and labour-intensive (Chia et al., 2014).
Alternatively, EBV-specific CTLs are generated by stimulation with adenovirus-based vector encoding truncated EBNA1 fused to CTL epitopes from LMP1 and 2 (AdE1-LMPpoly). The method is rapid and generates T-cells with improved functional and cytolytic potential compared to LCL-expanded EBV-specific CTLs. In both prophylactic and therapeutic settings, adoptive immunotherapy with AdE1-LMPpoly-expanded T cells was well-tolerated in NPC patients. Patients receiving CTLs have a prolonged median OS of 523 days when compared to patients who did not receive CTLs (220 days). The immune response was however transient, and only a minimal decline in EBV DNA load was observed (Smith et al., 2012, 2017). Another approach involves genetic modification of T-cells to recognise tumour-specific antigens through the expression of chimeric antigen receptors (CAR). LMP1-targeting CAR-T cells displayed LMP1-specific cytolytic action and significant growth inhibitory effect on tumour overexpressing LMP1 in in vivo xenograft model (Tang et al., 2014). On the other hand, T-cell receptor (TCR) gene transfer has been exploited as an alternative approach with improved efficiency, reliability and reduced time consumed to generate a large number of T-cells targeting EBV-encoded oncoproteins with high avidity. A significant reduction of tumour growth in mice was observed (Zheng et al., 2015b). An ongoing phase II clinical study (NCT03648697) in China is currently being conducted to evaluate the efficacy of EBV-TCR-T (YT-E001) cells in NPC patients.
Adoptive immunotherapy targeting EBV is a promising therapeutic strategy for NPC with minimal toxicities and long-term clinical benefits. Several strategies are proposed to improve the quality and efficacy of the therapeutic approach. This include the discovery of predictive biomarkers such as IP-10 and MIP-3a and prognostic biomarkers such as EBV-DNA would facilitate in identifying patients who can benefit from the therapeutic plan (Chia et al., 2014). The limited expansion of EBV-specific CTLs in vivo might restrict the antitumour activities. CD45 monoclonal antibody (mAb)-mediated lymphodepletion could stimulate the expansion of infused EBV-specific CTLs through elevating plasma levels of cytokines IL-7 and 15, depletion of regulatory T-cells and increasing antigenic stimulation (Louis et al., 2009). The expression of co-inhibitory receptors such as PD-1 and CTLA-4 on EBV-specific CTLs might be associated with their limited antitumour cytotoxicity, hence, immune checkpoint blockade might help potentiate the therapeutic efficacy (Smith et al., 2017). The lack of appropriate animal models of NPC for the evaluation of the therapeutic potential of EBV-targeted immunotherapy is a major hurdle for its preclinical validation, leading to disparity in preclinical and clinical results. This highlights the need for preclinical models which recapitulate the human immune system and tumour microenvironment.
Apart from that, tumour vaccines delivering EBV-specific antigens, including LMP1, LMP2, and EBNA1, through viral vectors or antigen-presenting cells are designed to enhance tumour-specific immunity. MVA-EL, a recombinant Modified Vaccinia Ankara expressing a chimeric antigen construct composed of HLA class I-restricted epitopes in LMP2 and HLA class II-restricted epitopes in EBNA1, efficiently elicits CD8+ and CD4+ T-cells responses in vitro. In phase I clinical trials involving NPC patients in remission or with persisting diseases in Hong Kong and the United Kingdom, the vaccine was reported to demonstrate satisfactory safety and tolerability, and immunogenicity across diverse EBV strains and HLA variations (Taylor et al., 2004, 2014; Hui et al., 2013a). In vitro study suggested that LMP2-specific CTLs induced by EBV-LMP2 recombinant adenovirus vaccine elicit cytotoxicity effect on tumour cells via the perforin/ granzyme and Fas/FasL pathways (Ge et al., 2020).
The other strategy involves autologous antigen-presenting cells expressing EBV-specific antigens generated ex vivo. A dendritic cell (DC) vaccine generated by transduction with an adenovirus encoding a truncated LMP1 and full-length LMP2 (Ad-ΔLMP1-LMP2) displayed only limited efficacy in phase II clinical trials on metastatic patients (Chia et al., 2012). Another LMP2-DC vaccine, generated by infecting autologous DCs with an adenovirus vector expressing LMP2 (rAd-LMP2), can elicit CTL responses specific to LMP2 that contribute to preventing the recurrence and metastasis, particularly in the early stage of NPC (Zeng et al., 2020).
Tumour vaccines targeting EBV-encoded products are well tolerated across diverse ethnicity and have minimal adverse events. Limited efficacy was however reported in patients with advanced NPC, which may be due to the prevalence of Tregs and the presence of co-inhibitory molecules in the tumour microenvironment (Chia et al., 2012; Hui et al., 2013a). Future multicentre clinical trials recruiting larger cohorts are required to further assess the efficacy of these vaccines for the treatment of NPC, identify groups that could benefit from the intervention, and evaluate the long-term therapeutic effects of EBV-targeting vaccines. Studies should also investigate the potential synergism between therapeutic vaccination and other modalities, such as chemotherapy and adoptive immunotherapy to improve their efficacy. Virotherapy using oncolytic viruses represents a novel form of cancer therapeutics that act as cancer vaccines which stimulate the host’s anti-tumour immunity besides directly inducing cancer cell death. These include herpes simplex virus G47Δ and Pteropine Orthoreovirus 7S which demonstrated antitumour activity against NPC in vitro. Oncolytic viruses have been genetically engineered to express cytokines, costimulatory molecules, immune checkpoint inhibitors, or bispecific T-cell engager (BiTE) molecules to boost anti-tumour immune response and suppress tumour tolerance. For instance, an oncolytic adenovirus MG1 encoding HPV oncogenes E6 and E7 demonstrated tumour-specific responses in preclinical studies and is currently undergoing a phase I clinical trial involving patients with HPV-associated malignancies (Wang et al., 2011; Loh et al., 2022; Tan et al., 2022). Thus, oncolytic viruses represent attractive targets for the development of therapeutic tools against EBV-associated NPC.
5.2. Cytolytic virus activation therapy
Cytolytic virus activation therapy aims to reactivate the lytic cycle in latent EBV, thus inducing the expression of immunogenic proteins and viral kinases that sensitises cells to antiviral immune responses or therapies. The chemical lytic inducers involved include conventional chemotherapeutic agents, histone deacetylase inhibitors, proteasome inhibitors, protein kinase C (PKC) modulators and others (Wildeman et al., 2012). Chemotherapeutic drugs, including cis-platinum and 5-fluorouracil, induce a latent-lytic switch in EBV infecting malignant cells through p38 stress MAPK, P13 kinase, and PKC-δ pathways, thereby triggering de novo expression of viral kinases such as protein kinase (PK) that converts analogue anti-viral prodrug ganciclovir into active cytotoxic form to kill EBV-positive NPC cells (Feng et al., 2002; Meng et al., 2010). Inhibitors of histone deacetylase such as suberoylanilide hydroxamic acid (SAHA), or Vorinostat, and romidepsin, induce EBV lytic cycle with the expression of lytic proteins, leading to apoptosis and suppression of tumour growth in NPC (Hui et al., 2012, 2016). Sodium phenylbutyrate (NaPB), a PKC modulator, can upregulate EBV thymidine kinase (TK) activity in EBV-positive tumour cells, sensitising them to the antiviral drug ganciclovir (Chung et al., 2000). Iron chelators, including C7, and di-2-pyridyl ketone 4,4-dimethyl-3-thiosemicarbazone (Dp44mT) reactivate EBV lytic cycle through the extracellular signal-regulated kinase (ERK)-mediated autophagy and hypoxic signalling through HIF-1α induction (Choi et al., 2015; Yiu et al., 2018). Studies reported synergistic effects in the combination of chemical lytic inducers. For instance, the chemotherapeutic agent gemcitabine with histone deacetylase inhibitor valproic acid leads to stronger induction of the lytic cycle of EBV (Novalić et al., 2017). The combination of proteasome inhibitor bortezomib and SAHA synergistically activates EBV lytic cycle and induces ROS-driven caspase-dependent apoptosis, thus suppressing tumour growth in vivo (Hui et al., 2013b).
Pilot and phase I-II clinical studies reported that cytolytic virus activation therapy combining gemcitabine, valproic acid, and ganciclovir was well tolerated and contributed to disease stabilisation in patients with advanced NPC. Immune responses were restricted, probably due to the immunomodulatory effect of the tumour microenvironment (Wildeman et al., 2012). Future studies should look into the exact molecular mechanism underlying tumour and immune responses following lytic induction to enhance the efficacy of the therapeutic strategy. The effect of intracellular iron chelation suggested the incorporation of clinically available iron chelators for NPC patients. Further clinical studies would aid in the evaluation of the optimised dose and drug combination regimen in NPC patients.
5.3. Others
Peptides targeting EBV-encoded latent proteins were designed for cancer monitoring and precision cancer therapy. A probe combining the fluorophore L2 and EBNA1-specific binding peptide P4 was synthesised as a theragnostic agent that permits the visualisation of tumours when localised at the nucleus of EBV-positive NPC cells and inhibition of tumour growth by interfering with the homodimerization of EBNA1 (Jiang et al., 2017). The incorporation of a zinc chelator (ZRL5) into P4 could interrupt the oligomerization of EBNA1 more effectively to inhibit growth and reactivate EBV lytic cycle, leading to shrinkage of EBV-positive NPC tumours (Jiang et al., 2019). In another study, the conjugation of P4 with the lanthanide upconversion nanoparticles to form UCNP-P4 further enhances its stability and biocompatibility and provides stronger signalling for imaging and more specific targeting of tumours (Zha et al., 2018). The construction of a dual-EBV-oncoproteins-targeting pH-responsive peptide, UCNP-P5 further enhanced the specificity and efficacy of the drug while reducing the undesired side effects in preclinical in vivo and in vitro models (Zha et al., 2021).
Genetic manipulation of LMP1 expression via RNA-cleaving deoxyribozyme (DNAzyme) has been actively investigated as a potential treatment approach for NPC. DNAzymes showed an inhibitory effect on the expression of LMP1 and its downstream pathways, including the AP1, NF-κB and JAK/STAT pathways, which results in DNA damage and cell cycle arrest, promotion of apoptosis, and enhancement of radiosensitivity in both in vitro and in vivo NPC models. LMP1-targeted DNAzymes contribute to the inhibition of LMP1-induced radio-resistance in NPC by repressing the LMP1/JNKs/HIF-1/VEGF-mediated angiogenesis and inhibiting the LMP1/Akt-induced telomerase activity (Ma et al., 2013; Yang et al., 2014, 2015). Early phase clinical trial of intratumoral administration of EBV-LMP1 targeted DNAzyme in conjunction with radiotherapy reported a significant reduction in short-term tumour regression with minimal adverse events associated with the combined treatment (Cao et al., 2014; Liao et al., 2014).
Several strategies targeting EBV have been proposed and experimented with over the last few decades, including adoptive immunotherapy and cancer vaccines, cytolytic virus activation therapy, EBV-encoded oncoproteins-targeting peptides, and genetic therapy. Besides the widely explored EBNA1 and LMPs, the inevitable oncogenic roles of EBV-derived RNAs, including EBERs, miRNAs urged further investigation as promising therapeutic targets. NPC patients respond to EBV-targeting treatment variably, thus, the identification of predictive molecular markers is vital for the stratification of personalised treatment strategies for individual patients. This is however hindered by the limited availability of reliable NPC models which possess stable genetic aberrations. Therefore, patient-derived NPC models which are more genomically characterised are demanded to unravel the specific molecular players that determine patients’ responses to EBV-targeting therapy (Lee et al., 2015). Besides that, EBV is also an attractive target for tumour-specific drug delivery, which could enhance the potency of drugs while minimising undesired adverse effects in patients. Besides the aforementioned probed nanoparticles, exosomes are also promising vehicles for tumour-specific drug delivery (Wan et al., 2020). Genomic editing tools, such as CRISPR-Cas potentially mediate precise targeting of the viral genome for antitumour therapy (Huo and Hu, 2019). Lastly, studies combining different therapeutic strategies are also recommended to study their synergistic effect and design an optimal therapeutic regimen for NPC patients.
6. Concluding remarks and future opportunities
EBV is an oncogenic virus closely associated with NPC. The role of EBV infection in the initiation and development of NPC may be due to the aberrant induction of latency in premalignant epithelial cells, the exact molecular mechanisms remained unexplored. The preclinical research on the EBV association with NPC is impeded by the limited availability of representative models. Rapid loss of EBV episomes following cell propagation and genetic contamination was reported in cell cultures of NPC (Dittmer et al., 2008). The addition of ROCK inhibitor Y-27632 to epithelial cells suppressed their differentiation and promotes the establishment of EBV-positive cell line NPC43 (Lin et al., 2018b). The establishment of more efficient in vitro models for the investigation of EBV infection and cell transformation would offer insight into the interaction between host cells, viruses and the tumour microenvironment. Furthermore, patient-derived xenografts could recapitulate the mutational profile of NPC patients and facilitate the translational research and development of precision medicine.
NPC displayed skewed geographical and ethnic distribution despite the widespread EBV infection, suggesting the role of EBV gene heterogeneity. The implementation of more sensitive and effective technology for viral genome sequencing and cloning of EBV strains, together with phenotypic investigation of EBV sequence heterogeneity would shed light on identifying the high-risk EBV variant associated with NPC and the role of sequence variation in tumorigenesis, cancer progression, survival outcome, and therapeutic response. This knowledge would also facilitate the identification of high-risk groups with a predisposition to NPC and the development of prevention strategies or EBV-based therapeutics for NPC.
EBV serology detecting levels of EBV DNA and antibodies towards EBV oncoproteins aids in the detection of early-stage NPC, prediction of prognosis, and monitoring of patient’s response. The development of diagnostic and predictive biomarkers with high sensitivity and specificity is essential to detect NPC at an early stage and provide prognostic information with higher accuracy and precision. Large-scale, international studies with standardised methods and cut-off values are required to improve the efficacy of the markers. Studies integrating EBV-based signature with other NPC biomarkers, for instance, NPC-associated genomic polymorphisms, are required to further enhance their efficiency in early diagnosis and predict the treatment response and recurrence risk. The use of artificial intelligence integrating the genotypic and phenotypic profiles of patients could facilitate risk stratification and decision-making in a cost- and time-effective manner.
Author contributions
ZS, PS and S-CC: conceptualization. ZS: writing—original draft preparation. PS, C-OL and S-CC: writing—review and editing. S-CC: funding acquisition and supervision. All authors contributed to the article and approved the submitted version.
Funding
This study is supported by the Ministry of Higher Education Malaysia (MOHE) through Fundamental Research Grant Scheme FRGS (FGRS/1/2020/SKK0/UCSI/02/2).
Acknowledgments
This is a short text to acknowledge the contributions of specific colleagues, institutions, or agencies that aided the efforts of the authors.
Conflict of interest
C-OL was employed by AGTC Genomics Sdn Bhd.
The remaining authors declare that the research was conducted in the absence of any commercial or financial relationships that could be construed as a potential conflict of interest.
Publisher’s note
All claims expressed in this article are solely those of the authors and do not necessarily represent those of their affiliated organizations, or those of the publisher, the editors and the reviewers. Any product that may be evaluated in this article, or claim that may be made by its manufacturer, is not guaranteed or endorsed by the publisher.
References
Ajadurai, R., Athmanathan, P., Mapati, U., Rasad, P., Obert, R., Adler, S., et al. (1995). Clonal proliferations of cells infected with Epstein–Barr virus in Preinvasive lesions related to nasopharyngeal carcinoma. N. Engl. J. Med. 333, 693–698. doi: 10.1056/NEJM199509143331103
Au, K. H., Ngan, R. K. C., Ng, A. W. Y., Poon, D. M. C., Ng, W. T., Yuen, K. T., et al. (2018). Treatment outcomes of nasopharyngeal carcinoma in modern era after intensity modulated radiotherapy (IMRT) in Hong Kong: a report of 3328 patients (HKNPCSG 1301 study). Oral Oncol. 77, 16–21. doi: 10.1016/j.oraloncology.2017.12.004
Banko, A., Miljanovic, D., Lazarevic, I., and Cirkovic, A. (2021). A systematic review of Epstein–Barr virus latent membrane protein 1 (Lmp1) gene variants in nasopharyngeal carcinoma. Pathogens 10, 1–31. doi: 10.3390/pathogens10081057
Basria, R., Mydin, S. M. N., and Okekpa, S. I. (2019). Molecular pathways for nasopharyngeal carcinoma focused on acetaldehyde, nitrosamines and nicotine exposures. Malaysian J. Med. Health Sci. 15, 64–70.
Bossi, P., Chan, A. T., Licitra, L., Trama, A., Orlandi, E., Hui, E. P., et al. (2021). Nasopharyngeal carcinoma: ESMO-EURACAN clinical practice guidelines for diagnosis, treatment and follow-up. Ann. Oncol. 32, 452–465. doi: 10.1016/j.annonc.2020.12.007
Cai, L. M., Lyu, X. M., Luo, W. R., Cui, X. F., Ye, Y. F., Yuan, C. C., et al. (2015). EBV-miR-BART7-3p promotes the EMT and metastasis of nasopharyngeal carcinoma cells by suppressing the tumor suppressor PTEN. Oncogene 34, 2156–2166. doi: 10.1038/onc.2014.341
Cai, T.-T., Ye, S.-B., Liu, Y.-N., He, J., Chen, Q.-Y., Mai, H.-Q., et al. (2017). LMP1-mediated glycolysis induces myeloid-derived suppressor cell expansion in nasopharyngeal carcinoma. PLoS Pathog. 13:e1006503. doi: 10.1371/journal.ppat.1006503
Cao, Y., Yang, L., Jiang, W., Wang, X., Liao, W., Tan, G., et al. (2014). Therapeutic evaluation of Epstein-Barr virus-encoded latent membrane protein-1 targeted DNAzyme for treating of nasopharyngeal carcinomas. Mol. Ther. 22, 371–377. doi: 10.1038/mt.2013.257
Chan, A. S. C., Fai To, K., and Wai Lo, K. (2000). High frequency of chromosome 3p deletion in histologically normal nasopharyngeal epithelia from southern Chinese. Cancer Res. 60, 5365–5370.
Chan, A. S. C., To, K. F., Lo, K. W., Ding, M., Li, X., Johnson, P., et al. (2002). Frequent chromosome 9p losses in histologically normal nasopharyngeal epithelia from southern Chinese. Int. J. Cancer 102, 300–303. doi: 10.1002/ijc.10689
Chen, Y. P., Chan, A. T. C., Le, Q. T., Blanchard, P., Sun, Y., and Ma, J. (2019). Nasopharyngeal carcinoma. Lancet 394, 64–80. doi: 10.1016/S0140-6736(19)30956-0
Chen, Y., Chang, E. T., Liu, Q., Cai, Y., Zhang, Z., Chen, G., et al. (2022). Environmental factors for Epstein-Barr virus reactivation in a high-risk area of nasopharyngeal carcinoma: a population-based study. Open Forum Infect. Dis. 9:128. doi: 10.1093/ofid/ofac128
Chen, X., Li, Y., Li, X., Cao, X., Xiang, Y., Xia, W., et al. (2021a). An interpretable machine learning prognostic system for locoregionally advanced nasopharyngeal carcinoma based on tumor burden features. Oral Oncol. 118:105335. doi: 10.1016/j.oraloncology.2021.105335
Chen, Y.-R., Liu, M.-T., Chang, Y.-T., Wu, C.-C., Hu, C.-Y., and Chen, J.-Y. (2008). Epstein-Barr virus latent membrane protein 1 represses DNA repair through the PI3K/Akt/FOXO3a pathway in human epithelial cells. J. Virol. 82:8124. doi: 10.1128/JVI.00430-08
Chen, Y., Zhou, C., Li, H., Li, H., and Li, Y. (2021b). Identifying key genes for nasopharyngeal carcinoma by prioritized consensus differentially expressed genes caused by aberrant methylation. J. Cancer 12, 874–884. doi: 10.7150/jca.49392
Cheng, S., Li, Z., He, J., Fu, S., Duan, Y., Zhou, Q., et al. (2019). Epstein–Barr virus noncoding RNAs from the extracellular vesicles of nasopharyngeal carcinoma (NPC) cells promote angiogenesis via TLR3/RIG-I-mediated VCAM-1 expression. Biochim. Biophys. Acta (BBA) - Mol. Basis Dis. 1865, 1201–1213. doi: 10.1016/j.bbadis.2019.01.015
Chesnokova, L. S., and Hutt-Fletcher, L. M. (2011). Fusion of Epstein-Barr virus with epithelial cells can be triggered by αvβ5 in addition to αvβ6 and αvβ8, and integrin binding triggers a conformational change in glycoproteins gHgL. J. Virol. 85:13223. doi: 10.1128/JVI.05580-11
Chesnokova, L. S., Nishimura, S. L., and Hutt-Fletcher, L. M. (2009). Fusion of epithelial cells by Epstein-Barr virus proteins is triggered by binding of viral glycoproteins gHgL to integrins αvβ6 or αvβ6. Proceed. National Academy of Sci. 106, 20464–20469. doi: 10.1073/pnas.0907508106
Chia, W. K., Teo, M., Wang, W. W., Lee, B., Ang, S. F., Tai, W. M., et al. (2014). Adoptive T-cell transfer and chemotherapy in the first-line treatment of metastatic and/or locally recurrent nasopharyngeal carcinoma. Mol. Ther. 22, 132–139. doi: 10.1038/mt.2013.242
Chia, W. K., Wang, W. W., Teo, M., Tai, W. M., Lim, W. T., Tan, E. H., et al. (2012). A phase II study evaluating the safety and efficacy of an adenovirus-ΔLMP1-LMP2 transduced dendritic cell vaccine in patients with advanced metastatic nasopharyngeal carcinoma. Ann. Oncol. 23, 997–1005. doi: 10.1093/annonc/mdr341
Chiu, S. H., Wu, C. C., Fang, C. Y., Yu, S. L., Hsu, H. Y., Chow, Y. H., et al. (2014). Epstein-Barr virus BALF3 mediates genomic instability and progressive malignancy in nasopharyngeal carcinoma. Oncotarget 5:8583. doi: 10.18632/oncotarget.2323
Choi, C. K., Ho, D. N., Hui, K. F., Kao, R. Y., and Chiang, A. K. S. (2015). Identification of novel small organic compounds with diverse structures for the induction of Epstein-Barr virus (EBV) lytic cycle in EBV-positive epithelial malignancies. PLoS One 10:5994. doi: 10.1371/journal.pone.0145994
Chua, M. L. K., Wee, J. T. S., Hui, E. P., and Chan, A. T. C. (2016). Nasopharyngeal carcinoma. Lancet 387, 1012–1024. doi: 10.1016/S0140-6736(15)00055-0
Chung, Y. L., Lee, Y. H. W., Yen, S. H., and Chi, K. H. (2000). A novel approach for nasopharyngeal carcinoma treatment uses phenylbutyrate as a protein kinase C modulator: implications for radiosensitization and EBV-targeted therapy. Clin. Cancer Res. 6, 1452–1458.
Colevas, A. D., Yom, S. S., Pfister, D. G., Spencer, S., Adelstein, D., Adkins, D., et al. (2018). NCCN guidelines® insights: head and neck cancers, version 1.2018 featured updates to the NCCN guidelines. J. Natl. Compr. Cancer Netw. 16, 479–490. doi: 10.6004/jnccn.2018.0026
da Costa, V. G., Marques-Silva, A. C., and Moreli, M. L. (2015). The Epstein-Barr virus latent membrane protein-1 (LMP1) 30-bp deletion and XhoI-polymorphism in nasopharyngeal carcinoma: a meta-analysis of observational studies. Syst. Rev. 4:46. doi: 10.1186/s13643-015-0037-z
Dai, W., Cheung, A. K. L., Ko, J. M. Y., Cheng, Y., Zheng, H., Ngan, R. K. C., et al. (2015). Comparative methylome analysis in solid tumors reveals aberrant methylation at chromosome 6p in nasopharyngeal carcinoma. Cancer Med. 4, 1079–1090. doi: 10.1002/cam4.451
Deng, W., Pang, P. S., Tsang, C. M., Hau, P. M., Yip, Y. L., Cheung, A. L. M., et al. (2012). Epstein-barr virus-encoded latent membrane protein 1 impairs G2 checkpoint in human nasopharyngeal epithelial cells through defective Chk1 activation. PLoS One 7, 1–9. doi: 10.1371/journal.pone.0039095
Dheekollu, J., Malecka, K., Wiedmer, A., Delecluse, H.-J., Chiang, A. K. S., Altieri, D. C., et al. (2017). Carcinoma-risk variant of EBNA1 deregulates Epstein-Barr virus episomal latency. Oncotarget 8, 7248–7264. doi: 10.18632/oncotarget.14540
Dittmer, D. P., Hilscher, C. J., Gulley, M. L., Yang, E., Chen, M., and Glaser, R. (2008). Multiple pathways for Epstein-Barr virus episome loss from nasopharyngeal carcinoma. Int. J. cancer. Journal international du cancer 123, 2105–2112. doi: 10.1002/ijc.23685
Edwards, R. H., Seillier-Moiseiwitsch, F., and Raab-Traub, N. (1999). Signature amino acid changes in latent membrane protein 1 distinguish Epstein-Barr virus strains. Virology 261, 79–95. doi: 10.1006/viro.1999.9855
Fachiroh, J., Paramita, D. K., Hariwiyanto, B., Harijadi, A., Dahlia, H. L., Indrasari, S. R., et al. (2006). Single-assay combination of Epstein-Barr virus (EBV) EBNA1- and viral capsid antigen-p18-derived synthetic peptides for measuring anti-EBV immunoglobulin G (IgG) and IgA antibody levels in sera from nasopharyngeal carcinoma patients: options for field screening. J. Clin. Microbiol. 44, 1459–1467. doi: 10.1128/JCM.44.4.1459-1467.2006
Fang, W., Zhang, J., Hong, S., Zhan, J., Chen, N., Qin, T., et al. (2014). EBV-driven LMP1 and IFN-γ up-regulate PD-L1 in nasopharyngeal carcinoma: implications for oncotargeted therapy. Oncotarget 5, 12189–12202. doi: 10.18632/oncotarget.2608
Feng, F.-T., Cui, Q., Liu, W.-S., Guo, Y.-M., Feng, Q.-S., Chen, L.-Z., et al. (2015). A single nucleotide polymorphism in the Epstein-Barr virus genome is strongly associated with a high risk of nasopharyngeal carcinoma. Chin. J. Cancer 34:61. doi: 10.1186/s40880-015-0073-z
Feng, W. H., Israel, B., Raab-Traub, N., Busson, P., and Kenney, S. C. (2002). Chemotherapy induces lytic EBV replication and confers ganciclovir susceptibility to EBV-positive epithelial cell tumors. Cancer Res. 62, 1920–1926.
Ferlay, J., Ervik, M., Lam, F., Colombet, M., Mery, L., Piñeros, M., et al. (2020). Global cancer observatory: cancer today. Available at: https://gco.iarc.fr/today/home [Accessed September 10, 2022].
Fung Hui, K., Fung Chan, T., Yang, W., Jane Shen, J., Pui Lam, K., Kwok, H., et al. (2018). High risk Epstein-Barr virus variants characterized by distinct polymorphisms in the EBER locus are strongly associated with nasopharyngeal carcinoma 144, 3031–3042. doi: 10.1002/ijc.32049
Gao, W., Wong, T. S., Lv, K. X., Zhang, M. J., Tsang, R. K. Y., and Chan, J. Y. W. (2019). Detection of Epstein–Barr virus (EBV)-encoded microRNAs in plasma of patients with nasopharyngeal carcinoma. Head Neck 41, 780–792. doi: 10.1002/hed.25544
Ge, Y., Zhou, Z., Wang, X., Zhou, Y., Liu, W., Teng, Z., et al. (2020). In vitro evaluation of the therapeutic effectiveness of EBV-LMP2 recombinant adenovirus vaccine in nasopharyngeal carcinoma. Biomed. Pharmacother. 121:109626. doi: 10.1016/j.biopha.2019.109626
Guo, X., Li, T., Li, F., Xu, Y., Wang, H., Cheng, W., et al. (2019). Intermittent abortive reactivation of Epstein-Barr virus during the progression of nasopharyngeal cancer as indicated by elevated antibody levels. Oral Oncol. 93, 85–90. doi: 10.1016/j.oraloncology.2019.04.024
Hanahan, D., and Weinberg, R. A. (2011). Hallmarks of cancer: the next generation. Cells 144, 646–674. doi: 10.1016/j.cell.2011.02.013
Henle, G., and Henle, W. (1976). Epstein-barr virus-specific IgA serum antibodies as an outstanding feature of nasopharyngeal carcinoma. Int. J. Cancer 17, 1–7. doi: 10.1002/ijc.2910170102
Ho, H. C., Ng, A. H., Kwan, H. C., and Chau, J. C. W. (1976). Epstein-Barr-virus-specific IgA and IgG serum antibodies in nasopharyngeal carcinoma. Br. J. Cancer 34:655. doi: 10.1038/bjc.1976.228
Horikawa, T., Yang, J., Kondo, S., Yoshizaki, T., Joab, I., Furukawa, M., et al. (2007). Twist and epithelial-mesenchymal transition are induced by the EBV Oncoprotein latent membrane protein 1 and are associated with metastatic nasopharyngeal carcinoma. Cancer Res. 67, 1970–1978. doi: 10.1158/0008-5472.CAN-06-3933
Horikawa, T., Yoshizaki, T., Kondo, S., Furukawa, M., Kaizaki, Y., and Pagano, J. S. (2011). Epstein-Barr virus latent membrane protein 1 induces snail and epithelial-mesenchymal transition in metastatic nasopharyngeal carcinoma. Br. J. Cancer 104, 1160–1167. doi: 10.1038/bjc.2011.38
Hsu, C. Y., Yi, Y. H., Chang, K. P., Chang, Y. S., Chen, S. J., and Chen, H. C. (2014). The Epstein-Barr virus-encoded MicroRNA MiR-BART9 promotes tumor metastasis by targeting E-cadherin in nasopharyngeal carcinoma. PLoS Pathog. 10:e1003974. doi: 10.1371/journal.ppat.1003974
Huang, Y., Liang, J., Hu, W., Liang, Y., Xiao, X., Zhao, W., et al. (2022). Integration profiling between plasma Lipidomics, Epstein–Barr virus and clinical phenomes in nasopharyngeal carcinoma patients. Front. Microbiol. 0:2462. doi: 10.3389/FMICB.2022.919496
Huang, J., Qin, Y., Yang, C., Wan, C., Dai, X., Sun, Y., et al. (2020). Downregulation of ABI2 expression by EBV-miR-BART13-3p induces epithelial-mesenchymal transition of nasopharyngeal carcinoma cells through upregulation of c-JUN/SLUG signaling. Aging 12:340. doi: 10.18632/aging.102618
Hui, K. F., Cheung, A. K. L., Choi, C. K., Yeung, P. L., Middeldorp, J. M., Lung, M. L., et al. (2016). Inhibition of class I histone deacetylases by romidepsin potently induces Epstein-Barr virus lytic cycle and mediates enhanced cell death with ganciclovir. Int. J. Cancer 138, 125–136. doi: 10.1002/ijc.29698
Hui, K. F., Ho, D. N., Tsang, C. M., Middeldorp, J. M., Tsao, G. S. W., and Chiang, A. K. S. (2012). Activation of lytic cycle of Epstein-Barr virus by suberoylanilide hydroxamic acid leads to apoptosis and tumor growth suppression of nasopharyngeal carcinoma. Int. J. Cancer 131, 1930–1940. doi: 10.1002/ijc.27439
Hui, K. F., Lam, B. H. W., Ho, D. N., Tsao, S. W., and Chiang, A. K. S. (2013b). Bortezomib and SAHA synergistically induce ROS-driven caspase-dependent apoptosis of nasopharyngeal carcinoma and block replication of epstein-barr virus. Mol. Cancer Ther. 12, 747–758. doi: 10.1158/1535-7163.MCT-12-0811/85176/AM/BORTEZOMIB-AND-SAHA-SYNERGISTICALLY-INDUCE-ROS
Hui, E. P., Taylor, G. S., Jia, H., Ma, B. B. Y., Chan, S. L., Ho, R., et al. (2013a). Phase 1 trial of recombinant modified vaccinia Ankara (MVA) encoding Epstein-Barr viral tumor antigens in nasopharyngeal carcinoma patients. Cancer Res. 73:1676. doi: 10.1158/0008-5472.CAN-12-2448
Huo, H., and Hu, G. (2019). CRISPR/Cas9-mediated LMP1 knockout inhibits Epstein-Barr virus infection and nasopharyngeal carcinoma cell growth. Infect Agent Cancer 14, 1–10. doi: 10.1186/s13027-019-0246-5
Huo, S., Luo, Y., Deng, R., Liu, X., Wang, J., Wang, L., et al. (2020). EBV-EBNA1 constructs an immunosuppressive microenvironment for nasopharyngeal carcinoma by promoting the chemoattraction of Treg cells. J. Immunother. Cancer 8:e001588. doi: 10.1136/jitc-2020-001588
Jen, C.-W., Tsai, Y.-C., Wu, J.-S., Chen, P.-L., Yen, J.-H., Chuang, W.-K., et al. (2020). Prognostic classification for patients with nasopharyngeal carcinoma based on American joint committee on cancer staging system T and N categories. Ther Radiol Oncol 4, 2–13. doi: 10.21037/tro.2020.02.01
Ji, M., Ou, X., Zong, Y., Zheng, S., Guo, Y., Liang, J., et al. (2002). A follow-up survey of 42 048 subjects tested with epstein-barr viral serology in a high-incidence area of nasopharyngeal carcinoma. Chinese-German J. Clinical Oncology 1, 222–225. doi: 10.1007/BF02838437
Ji, M. F., Wang, D. K., Yu, Y. L., Guo, Y. Q., Liang, J. S., Cheng, W. M., et al. (2007). Sustained elevation of Epstein-Barr virus antibody levels preceding clinical onset of nasopharyngeal carcinoma. Br. J. Cancer 96, 623–630. doi: 10.1038/sj.bjc.6603609
Jiang, C., Chen, J., Xie, S., Zhang, L., Xiang, Y., Lung, M., et al. (2018). Evaluation of circulating EBV microRNA BART2-5p in facilitating early detection and screening of nasopharyngeal carcinoma. Int. J. Cancer 143, 3209–3217. doi: 10.1002/ijc.31642
Jiang, L., Lan, R., Huang, T., Chan, C. F., Li, H., Lear, S., et al. (2017). EBNA1-targeted probe for the imaging and growth inhibition of tumours associated with the Epstein-Barr virus. Nat Biomed Eng 1, 1–10. doi: 10.1038/s41551-017-0042
Jiang, L., Lung, H. L., Huang, T., Lan, R., Zha, S., Chan, L. S., et al. (2019). Reactivation of Epstein-Barr virus by a dual-responsive fluorescent EBNA1-targeting agent with Zn2+-chelating function. Proc. Natl. Acad. Sci. U. S. A. 116, 26614–26624. doi: 10.1073/pnas.1915372116
Jiang, Y., Yan, B., Lai, W., Shi, Y., Xiao, D., Jia, J., et al. (2015). Repression of Hox genes by LMP1 in nasopharyngeal carcinoma and modulation of glycolytic pathway genes by HoxC8. Oncogene 34, 6079–6091. doi: 10.1038/onc.2015.53
Jing, B., Zhang, T., Wang, Z., Jin, Y., Liu, K., Qiu, W., et al. (2019). A deep survival analysis method based on ranking. Artif. Intell. Med. 98, 1–9. doi: 10.1016/j.artmed.2019.06.001
Khan, G., Fitzmaurice, C., Naghavi, M., and Ahmed, L. A. (2020). Global and regional incidence, mortality and disability-adjusted life-years for Epstein-Barr virus-attributable malignancies, 1990-2017. BMJ Open 10:e037505. doi: 10.1136/bmjopen-2020-037505
Khan, G., and Hashim, M. J. (2014). Global burden of deaths from Epstein-Barr virus attributable malignancies 1990-2010. Infect Agent Cancer 9, 1–11. doi: 10.1186/1750-9378-9-38
Kondo, S., Okuno, Y., Murata, T., Dochi, H., Wakisaka, N., Mizokami, H., et al. (2022). EBV genome variations enhance clinicopathological features of nasopharyngeal carcinoma in a non-endemic region. Cancer Sci. 113, 2446–2456. doi: 10.1111/cas.15381
Kong, Q. L., Hu, L. J., Cao, J. Y., Huang, Y. J., Xu, L. H., Liang, Y., et al. (2010). Epstein-Barr virus-encoded LMP2A induces an epithelial–mesenchymal transition and increases the number of side population stem-like cancer cells in nasopharyngeal carcinoma. PLoS Pathog. 6:e1000940. doi: 10.1371/journal.ppat.1000940
Kwok, H., Tong, A. H. Y., Lin, C. H., Lok, S., Farrell, P. J., Kwong, D. L. W., et al. (2012). Genomic sequencing and comparative analysis of Epstein-Barr virus genome isolated from primary nasopharyngeal carcinoma biopsy. PLoS One 7:e36939. doi: 10.1371/journal.pone.0036939
Kwok, H., Wu, C. W., Palser, A. L., Kellam, P., Sham, P. C., Kwong, D. L. W., et al. (2014). Genomic diversity of Epstein-Barr virus genomes isolated from primary nasopharyngeal carcinoma biopsy samples. J. Virol. 88, 10662–10672. doi: 10.1128/JVI.01665-14
Lai, H. C., Hsiao, J. R., Chen, C. W., Wu, S. Y., Lee, C. H., Su, I. J., et al. (2010). Endogenous latent membrane protein 1 in Epstein-Barr virus-infected nasopharyngeal carcinoma cells attracts T lymphocytes through upregulation of multiple chemokines. Virology 405, 464–473. doi: 10.1016/j.virol.2010.06.037
Lam, W. K. J., Chan, S. L., Chu, S. W. I., Sc, B., Mak, C., Tse, I. O. L., et al. (2017). Analysis of plasma Epstein–Barr virus DNA to screen for nasopharyngeal cancer. N. Engl. J. Med. 377, 513–522. doi: 10.1056/NEJMoa1701717
Lam, W. K. J., Jiang, P., Chan, K. C. A., Cheng, S. H., Zhang, H., Peng, W., et al. (2018). Sequencing-based counting and size profiling of plasma Epstein–Barr virus DNA enhance population screening of nasopharyngeal carcinoma. Proc. Natl. Acad. Sci. U. S. A. 115, E5115–E5124. doi: 10.1073/pnas.1804184115
Lambert, A. W., Pattabiraman, D. R., and Weinberg, R. A. (2017). Emerging biological principles of metastasis. Physiol. Behav. 176, 139–148. doi: 10.1016/j.cell.2016.11.037.EMERGING
Lan, Y. Y., Chang, F. H., Tsai, J. H., and Chang, Y. (2018). Epstein-Barr virus Rta promotes invasion of bystander tumor cells through paracrine of matrix metalloproteinase 9. Biochem. Biophys. Res. Commun. 503, 2160–2166. doi: 10.1016/j.bbrc.2018.08.006
Lan, Y.-Y., Hsiao, J.-R., Chang, K.-C., Chang, J. S.-M., Chen, C.-W., Lai, H.-C., et al. (2012). Epstein-Barr virus latent membrane protein 2A promotes invasion of nasopharyngeal carcinoma cells through ERK/Fra-1-mediated induction of matrix metalloproteinase 9. J. Virol. 86:6667. doi: 10.1128/JVI.00174-12
Lee, A. W. M., Lee, V. H. F., Ng, W. T., Strojan, P., Saba, N. F., Rinaldo, A., et al. (2021). A systematic review and recommendations on the use of plasma EBV DNA for nasopharyngeal carcinoma. Eur. J. Cancer 153, 109–122. doi: 10.1016/j.ejca.2021.05.022
Lee, A. W. M., Ma, B. B. Y., Ng, W. T., and Chan, A. T. C. (2015). Management of nasopharyngeal carcinoma: current practice and future perspective. J. Clin. Oncol. 33, 3356–3364. doi: 10.1200/JCO.2015.60.9347
Lee, C.-H., Yeh, T.-H., Lai, H.-C., Wu, S.-Y., Su, I.-J., Takada, K., et al. (2011). Epstein-Barr virus Zta-induced Immunomodulators from nasopharyngeal carcinoma cells upregulate Interleukin-10 production from monocytes. J. Virol. 85, 7333–7342. doi: 10.1128/JVI.00182-11
Leong, M. M. L., Cheung, A. K. L., Dai, W., Tsao, S. W., Tsang, C. M., Dawson, C. W., et al. (2019). EBV infection is associated with histone bivalent switch modifications in squamous epithelial cells. Proc. Natl. Acad. Sci. U. S. A. 116, 14144–14153. doi: 10.1073/pnas.1821752116
Leung, S. F., Tam, J. S., Chan, A. T. C., Zee, B., Chan, L. Y. S., Huang, D. P., et al. (2004). Improved accuracy of detection of nasopharyngeal carcinoma by combined application of circulating Epstein-Barr virus DNA and anti-Epstein-Barr viral capsid antigen IgA antibody. Clin. Chem. 50, 339–345. doi: 10.1373/clinchem.2003.022426
Lha, T., Author, C., Le Huyen Ai, T., Professor, A., Minh City, C., Chi Minh City, H., et al. (2019). Novel patterns of the Epstein-Barr nuclear antigen (EBNA-1) V-val subtype in EBV-associated nasopharyngeal carcinoma from Vietnam. Balkan J. Medical Genetics 22, 61–68. doi: 10.2478/bjmg-2019-0011
Li, Z., Duan, Y., Cheng, S., Chen, Y., Hu, Y., Zhang, L., et al. (2015). EBV-encoded RNA via TLR3 induces inflammation in nasopharyngeal carcinoma. Oncotarget 6, 24291–24303. doi: 10.18632/oncotarget.4552
Li, K., Lin, G.-Z., Shen, J.-C., and Zhou, Q. (2014a). Time trends of nasopharyngeal carcinoma in urban Guangzhou over a 12-year period (2000-2011): declines in both incidence and mortality. Asian Pac. J. Cancer Prev. 15, 9899–9903. doi: 10.7314/APJCP.2014.15.22.9899
Li, X. P., Wang, L., Tian, W. D., Xu, X., Nie, B., Lu, J., et al. (2014b). Epstein-Barr virus nuclear antigen 1 (EBNA1) protein induction of epithelial-mesenchymal transition in nasopharyngeal carcinoma cells. Cancer 120, 363–372. doi: 10.1002/CNCR.28418
Li, W., Yang, C., Lv, Z., Li, J., Li, Z., Yuan, X., et al. (2021). Integrating pre-and post-treatment plasma Epstein-Barr virus DNA levels for better prognostic prediction of nasopharyngeal carcinoma. J. Cancer 12, 2715–2722. doi: 10.7150/jca.56397
Li, Z., Zhou, Z., Wu, X., Zhou, Q., Liao, C., Liu, Y., et al. (2020). LMP1 promotes nasopharyngeal carcinoma metastasis through NTRK2-mediated anoikis resistance. Am. J. Cancer Res. 10:2083.
Liang, Y., Zhang, Y., and Luo, B. (2022). The lytic phase of Epstein–Barr virus plays an important role in tumorigenesis. Virus Genes 1, 1–12. doi: 10.1007/S11262-022-01940-6/FIGURES/5
Liang, J., Zheng, S., Xiao, X., Wei, J., Zhang, Z., Ernberg, I., et al. (2017). Epstein-Barr virus-encoded LMP2A stimulates migration of nasopharyngeal carcinoma cells via the EGFR/Ca2+/calpain/ITGβ4 axis. Biol Open 6, 914–922. doi: 10.1242/bio.024646
Liao, W.-H., Yang, L.-F., Liu, X.-Y., Zhou, G.-F., Jiang, W.-Z., Hou, B.-L., et al. (2014). DCE-MRI assessment of the effect of Epstein-Barr virus-encoded latent membrane protein-1 targeted DNAzyme on tumor vasculature in patients with nasopharyngeal carcinomas. BMC Cancer 14:835. doi: 10.1186/1471-2407-14-835
Lin, C. T., Lin, C. R., Tan, G. K., Chen, W., Dee, A. N., and Chan, W. Y. (1997). The mechanism of Epstein-Barr virus infection in nasopharyngeal carcinoma cells. Am. J. Pathol. 150:1745.
Lin, Z., Wan, X., Jiang, R., Deng, L., Gao, Y., Tang, J., et al. (2014). Epstein-Barr virus-encoded latent membrane protein 2A promotes the epithelial-mesenchymal transition in nasopharyngeal carcinoma via metastatic tumor antigen 1 and mechanistic target of rapamycin signaling induction. J. Virol. 88, 11872–11885. doi: 10.1128/JVI.01867-14
Lin, J.-C., Wang, W.-Y., Chen, K. Y., Wei, Y.-H., Liang, W.-M., Jan, J.-S., et al. (2004). Quantification of plasma Epstein-Barr virus DNA in patients with advanced nasopharyngeal carcinoma. N. Engl. J. Med. 350, 2461–2470. doi: 10.1056/NEJMoa032260
Lin, W., Yip, Y. L., Jia, L., Deng, W., Zheng, H., Dai, W., et al. (2018b). Establishment and characterization of new tumor xenografts and cancer cell lines from EBV-positive nasopharyngeal carcinoma. Nat. Commun. 9, 1–17. doi: 10.1038/s41467-018-06889-5
Lin, C., Zong, J., Lin, W., Wang, M., Xu, Y., Zhou, R., et al. (2018a). EBV-miR-BART8-3p induces epithelial-mesenchymal transition and promotes metastasis of nasopharyngeal carcinoma cells through activating NF-κB and Erk1/2 pathways. J. Exp. Clin. Cancer Res. 37:283. doi: 10.1186/s13046-018-0953-6
Lin, S.-X., Zong, Y.-S., Wu, Q.-L., Han, A.-J., and Liang, Y.-J. (2003). Loss of an Xho I-site within N-terminal region of Epstein-Barr virus LMP1 gene in nasopharyngeal carcinoma. Chin. J. Cancer 22, 1147–1151.
Liu, P., Fang, X., Feng, Z., Guo, Y.-M., Peng, R.-J., Liu, T., et al. (2011). Direct sequencing and characterization of a clinical isolate of Epstein-Barr virus from nasopharyngeal carcinoma tissue by using next-generation sequencing technology. J. Virol. 85, 11291–11299. doi: 10.1128/JVI.00823-11
Liu, Y., Huang, Q., Liu, W., Liu, Q., Jia, W., Chang, E., et al. (2012). Establishment of VCA and EBNA1 IgA-based combination by enzyme-linked immunosorbent assay as preferred screening method for nasopharyngeal carcinoma: a two-stage design with a preliminary performance study and a mass screening in southern China. Int. J. Cancer 131, 406–416. doi: 10.1002/ijc.26380
Liu, W., Li, H., Sheng, H., Liu, X., Chi, P., Wang, X., et al. (2020). A randomized controlled trial on evaluation of plasma Epstein-Barr virus biomarker for early diagnosis in patients with nasopharyngeal carcinoma. Adv. Ther. 37, 4280–4290. doi: 10.1007/s12325-020-01461-4
Lo, Y. M. D., Chan, L. Y. S., Lo, K. W., Leung, S. F., Zhang, J., Chan, A. T. C., et al. (1999). Quantitative analysis of cell-free Epstein-Barr virus DNA in plasma of patients with nasopharyngeal carcinoma. Cancer Res. 59, 1188–1191.
Lo, Y. M. D., Chan, L. Y. S., Lo, K. W., Leung, S. F., Zhang, J., Chan, A. T. C., et al. (2022). Epstein-Barr virus-based nasopharyngeal carcinoma population screening. Annals of Nasopharynx Cancer 6:3. doi: 10.21037/anpc-21-6
Lo, K. W., Chung, G. T. Y., and To, K. F. (2012). Deciphering the molecular genetic basis of NPC through molecular, cytogenetic, and epigenetic approaches. Semin. Cancer Biol. 22, 79–86. doi: 10.1016/j.semcancer.2011.12.011
Lo, A. K.-F., Dawson, C. W., Young, L. S., Ko, C.-W., Hau, P.-M., and Lo, K.-W. (2015). Activation of the FGFR1 signalling pathway by the Epstein-Barr virus-encoded LMP1 promotes aerobic glycolysis and transformation of human nasopharyngeal epithelial cells. J. Pathol. 237, 238–248. doi: 10.1002/path.4575
Loh, A., Shaibie, N. A., Chan, P. Q., Leong, P. P., Segeran, S., Tan, B. K., et al. (2022). Pteropine Orthoreovirus, PRV7S (Sikamat virus) demonstrates Oncolysis in nasopharyngeal carcinoma cell lines. Front Biosci (Landmark Ed) 27:138. doi: 10.31083/j.fbl2704138
Louis, C. U., Straath, K., Bollard, C. M., Gerken, C., Huls, M. H., Gresik, M. V., et al. (2009). Enhancing the in vivo expansion of adoptively transferred EBV- specific CTL with lymphodepleting CD45 monoclonal antibodies in NPC patients. Blood 113, 2442–2450. doi: 10.1182/blood-2008-05-157222
Louis, C. U., Straathof, K., Bollard, C. M., Ennamuri, S., Gerken, C., Lopez, T. T., et al. (2010). Adoptive transfer of EBV-specific T cells results in sustained clinical responses in patients with locoregional nasopharyngeal carcinoma. J. Immunother. 33, 983–990. doi: 10.1097/CJI.0b013e3181f3cbf4
Lu, T., Guo, Q., Lin, K., Chen, H., Chen, Y., Xu, Y., et al. (2020). Circulating Epstein-Barr virus microRNAs BART7-3p and BART13-3p as novel biomarkers in nasopharyngeal carcinoma. Cancer Sci. 111, 1711–1723. doi: 10.1111/cas.14381
Lu, Y., Qin, Z., Wang, J., Zheng, X., Lu, J., Zhang, X., et al. (2017). Epstein-Barr virus miR-BART6-3p inhibits the RIG-I pathway. J. Innate Immun. 9, 574–586. doi: 10.1159/000479749
Lung, R. W. M., Hau, P. M., Yu, K. H. O., Yip, K. Y., Tong, J. H. M., Chak, W. P., et al. (2018). EBV-encoded miRNAs target ATM-mediated response in nasopharyngeal carcinoma. J. Pathol. 244, 394–407. doi: 10.1002/path.5018
Luo, X., Hong, L., Cheng, C., Li, N., Zhao, X., Shi, F., et al. (2018). DNMT1 mediates metabolic reprogramming induced by Epstein-Barr virus latent membrane protein 1 and reversed by grifolin in nasopharyngeal carcinoma. Cell Death Dis. 9:619. doi: 10.1038/S41419-018-0662-2
Luo, W., and Yao, K. (2013). Molecular characterization and clinical implications of spindle cells in nasopharyngeal carcinoma: a novel molecule-morphology model of tumor progression proposed. PLoS One 8:e83135. doi: 10.1371/journal.pone.0083135
Ma, W., Feng, L., Zhang, S., Zhang, H., Zhang, X., Qi, X., et al. (2018). Induction of chemokine (C-C motif) ligand 5 by Epstein-Barr virus infection enhances tumor angiogenesis in nasopharyngeal carcinoma. Cancer Sci. 109, 1710–1722. doi: 10.1111/cas.13584
Ma, X., Xu, Z., Yang, L., Xiao, L., Tang, M., Lu, J., et al. (2013). EBV-LMP1-targeted DNAzyme induces DNA damage and causes cell cycle arrest in LMP1-positive nasopharyngeal carcinoma cells. Int. J. Oncol. 43, 1541–1548. doi: 10.3892/ijo.2013.2098
Mai, S.-J., Xie, D., Huang, Y.-F., Wang, F.-W., Liao, Y.-J., Deng, H.-X., et al. (2010). The enhanced transcriptional activity of the V-val subtype of Epstein-Barr virus nuclear antigen 1 in epithelial cell lines. Oncol. Rep. 23:1417. doi: 10.3892/or_00000779
Meng, Q., Hagemeier, S. R., Fingeroth, J. D., Gershburg, E., Pagano, J. S., and Kenney, S. C. (2010). The Epstein-Barr virus (EBV)-encoded protein kinase, EBV-PK, but not the thymidine kinase (EBV-TK), is required for ganciclovir and acyclovir inhibition of lytic viral production. J. Virol. 84, 4534–4542. doi: 10.1128/JVI.02487-09
Miller, J. A., Sahoo, M. K., Yamamoto, F., Huang, C., Wang, H., Zehnder, J. L., et al. (2021). Multiplex Epstein-Barr virus BALF2 genotyping detects high-risk variants in plasma for population screening of nasopharyngeal carcinoma. Mol. Cancer 21:154. doi: 10.1186/s12943-022-01625-6
Mutirangura, A., Pornthanakasem, W., Theamboonlers, A., Sriuranpong, V., Lertsanguansinchi, P., Yenrudi, S., et al. (1998). Epstein-Barr viral DNA in serum of patients with nasopharyngeal carcinoma. Clin. Cancer Res. 4, 665–669.
Ng, W. T., But, B., Choi, H. C. W., de Bree, R., Lee, A. W. M., Lee, V. H. F., et al. (2022). Application of artificial intelligence for nasopharyngeal carcinoma management – a systematic review. Cancer Manag. Res. 14:339. doi: 10.2147/CMAR.S341583
Novalić, Z., Verkuijlen, S. A. W. M., Verlaan, M., Eersels, J. L. H., de Greeuw, I., Molthoff, C. F. M., et al. (2017). Cytolytic virus activation therapy and treatment monitoring for Epstein-Barr virus associated nasopharyngeal carcinoma in a mouse tumor model. J. Med. Virol. 89, 2207–2216. doi: 10.1002/jmv.24870
Ogino, T., Moriai, S., Ishida, Y., Ishii, H., Katayama, A., Miyokawa, N., et al. (2007). Association of immunoescape mechanisms with Epstein-Barr virus infection in nasopharyngeal carcinoma. Int. J. Cancer 120, 2401–2410. doi: 10.1002/ijc.22334
Oneil, J. D., Owen, T. J., Wood, V. H. J., Date, K. L., Valentine, R., and Chukwuma, M. B.,, et al. (2008). Epstein-Barr virus-encoded EBNA1 modulates the AP-1 transcription factor pathway in nasopharyngeal carcinoma cells and enhances angiogenesis in vitro. J. Gen. Virol. 89, 2833–2842. doi: 10.1099/vir.0.2008/003392-0
Pai, S., O’Sullivan, B., Abdul-Jabbar, I., Peng, J., Connoly, G., Khanna, R., et al. (2007). Nasopharyngeal carcinoma-associated Epstein-Barr virus-encoded oncogene latent membrane protein 1 potentiates regulatory T-cell function. Immunol. Cell Biol. 85, 370–377. doi: 10.1038/sj.icb.7100046
Palser, A. L., Grayson, N. E., White, R. E., Corton, C., Correia, S., Ba Abdullah, M. M., et al. (2015). Genome diversity of Epstein-Barr virus from multiple tumor types and Normal infection. J. Virol. 89:5222. doi: 10.1128/JVI.03614-14
Peng, H., Chen, Y., Gong, P., Cai, L., Lyu, X., Jiang, Q., et al. (2016). Higher methylation intensity induced by EBV LMP1 via NF-κB/ DNMT3b signaling contributes to silencing of PTEN gene. Oncotarget 7, 40025–40037. doi: 10.18632/oncotarget.9474
Poh, S. S., Chua, M. L. K., and Wee, J. T. S. (2016). Carcinogenesis of nasopharyngeal carcinoma: an alternate hypothetical mechanism. Chin. J. Cancer 35:9. doi: 10.1186/S40880-015-0068-9
Rosemarie, Q., and Sugden, B. (2020). Epstein-Barr virus: how its lytic phase contributes to oncogenesis. Microorganisms 8:1824. doi: 10.3390/microorganisms8111824
Rüeger, S., Hammer, C., Loetscher, A., Mclaren, P. J., Lawless, D., Naret, O., et al. (2021). The influence of human genetic variation on Epstein-Barr virus sequence diversity. Sci. Rep. 11:4586. doi: 10.1038/s41598-021-84070-7
Shah, K. M., Stewart, S. E., Wei, W., Woodman, C. B. J., O’Neil, J. D., Dawson, C. W., et al. (2009). The EBV-encoded latent membrane proteins, LMP2A and LMP2B, limit the actions of interferon by targeting interferon receptors for degradation. Oncogene 28:3903. doi: 10.1038/onc.2009.249
Shi, F., Zhou, M., Shang, L., Du, Q., Li, Y., Xie, L., et al. (2019). EBV(LMP1)-induced metabolic reprogramming inhibits necroptosis through the hypermethylation of the RIP3 promoter. Theranostics 9, 2424–2438. doi: 10.7150/thno.30941
Sivachandran, N., Sarkari, F., and Frappier, L. (2008). Epstein-Barr nuclear antigen 1 contributes to nasopharyngeal carcinoma through disruption of PML nuclear bodies. PLoS Pathog. 4:170. doi: 10.1371/journal.ppat.1000170
Sixbey, J. W., and Yao, Q. Y. (1992). Immunoglobulin A-induced shift of Epstein-Barr virus tissue tropism. Science 1979, 1578–1580. doi: 10.1126/SCIENCE.1312750
Smith, C., Lee, V., Schuessler, A., Beagley, L., Rehan, S., Tsang, J., et al. (2017). Pre-emptive and therapeutic adoptive immunotherapy for nasopharyngeal carcinoma: phenotype and effector function of T cells impact on clinical response. Onco. Targets. Ther. 6:3311. doi: 10.1080/2162402X.2016.1273311
Smith, C., Tsang, J., Beagley, L., Chua, D., Lee, V., Li, V., et al. (2012). Effective treatment of metastatic forms of Epstein-Barr virus-associated nasopharyngeal carcinoma with a novel adenovirus-based adoptive immunotherapy. Cancer Res. 72, 1116–1125. doi: 10.1158/0008-5472.CAN-11-3399
Stoker, S. D., Novalic, Z., Wildeman, M. A., Huitema, A. D. R., Verkuijlen, S. A. W. M., Juwana, H., et al. (2015). Epstein-Barr virus-targeted therapy in nasopharyngeal carcinoma. J. Cancer Res. Clin. Oncol. 141, 1845–1857. doi: 10.1007/s00432-015-1969-3
Straathof, K. C. M., Bollard, C. M., Popat, U., Huls, M. H., Lopez, T., Morriss, M. C., et al. (2005). Treatment of nasopharyngeal carcinoma with Epstein-Barr virus–specific T lymphocytes. Blood 105, 1898–1904. doi: 10.1182/blood-2004-07-2975
Sung, W. W., Chen, P. R., Liao, M. H., and Lee, J. W. (2017). Enhanced aerobic glycolysis of nasopharyngeal carcinoma cells by Epstein-Barr virus latent membrane protein 1. Exp. Cell Res. 359, 94–100. doi: 10.1016/j.yexcr.2017.08.005
Sung, H., Ferlay, J., Soerjomataram, I., Siegel, R. L., Bray, F., and Jemal, A. (2021). Global cancer statistics 2020: GLOBOCAN estimates of incidence and mortality worldwide for 36 cancers in 185 countries. CA Cancer J. Clin. 71, 7–33. doi: 10.3322/caac.21660
Takeshita, H., Yoshizaki, T., Miller, W. E., Sato, H., Furukawa, M., Pagano, J. S., et al. (1999). Matrix metalloproteinase 9 expression is induced by Epstein-Barr virus latent membrane protein 1 C-terminal activation regions 1 and 2. J. Virol. 73:5548. doi: 10.1128/JVI.73.7.5548-5555.1999
Tan, E. W., Abd-Aziz, N., and Onn Tan, K. (2022). Engineered oncolytic adenoviruses: an Emerging approach for cancer therapy. Pathogens 11:1146. doi: 10.3390/pathogens11101146
Tang, L. L., Chen, W. Q., Xue, W. Q., He, Y. Q., Zheng, R. S., Zeng, Y. X., et al. (2016). Global trends in incidence and mortality of nasopharyngeal carcinoma. Cancer Lett. 374, 22–30. doi: 10.1016/j.canlet.2016.01.040
Tang, Y. L., Lu, J. H., Cao, L., Wu, M. H., Peng, S. P., Zhou, H. D., et al. (2008). Genetic variations of EBV-LMP1 from nasopharyngeal carcinoma biopsies: potential loss of T cell epitopes. Braz. J. Med. Biol. Res. 41, 110–116. doi: 10.1590/S0100-879X2008000200006
Tang, X., Zhou, Y., Li, W., Tang, Q., Chen, R., Zhu, J., et al. (2014). T cells expressing a LMP1-specific chimeric antigen receptor mediate antitumor effects against LMP1-positive nasopharyngeal carcinoma cells in vitro and in vivo. J. Biomedical Res. 28, 468–475. doi: 10.7555/JBR.28.20140066
Taylor, G. S., Haigh, T. A., Gudgeon, N. H., Phelps, R. J., Lee, S. P., Steven, N. M., et al. (2004). Dual stimulation of Epstein-Barr virus (EBV)-specific CD4+- and CD8+-T-cell responses by a chimeric antigen construct: potential therapeutic vaccine for EBV-positive nasopharyngeal carcinoma. J. Virol. 78:768. doi: 10.1128/JVI.78.2.768-778.2004
Taylor, G. S., Jia, H., Harrington, K., Lee, L. W., Turner, J., Ladell, K., et al. (2014). A recombinant modified vaccinia Ankara vaccine encoding Epstein-Barr virus (EBV) target antigens: a phase I trial in UK patients with EBV-positive cancer. Clin. Cancer Res. 20, 5009–5022. doi: 10.1158/1078-0432.CCR-14-1122-T
Tsai, C. L., Li, H. P., Lu, Y. J., Hsueh, C., Liang, Y., Chen, C. L., et al. (2006). Activation of DNA methyltransferase 1 by EBV LMP1 involves c-Jun NH 2-terminal kinase signaling. Cancer Res. 66, 11668–11676. doi: 10.1158/0008-5472.CAN-06-2194
Tsai, M. H., Raykova, A., Klinke, O., Bernhardt, K., Gärtner, K., Leung, C. S., et al. (2013). Spontaneous lytic replication and Epitheliotropism define an Epstein-Barr virus strain found in carcinomas. Cell Rep. 5, 458–470. doi: 10.1016/j.celrep.2013.09.012
Tsai, C.-N., Tsai, C.-L., Tse, K.-P., Chang, H.-Y., and Chang, Y.-S. (2002). The Epstein-Barr virus oncogene product, latent membrane protein 1, induces the down-regulation of E-cadherin gene expression via activation of DNA methyltransferases. Proc. Natl. Acad. Sci. 99, 10084–10089. doi: 10.1073/pnas.152059399
Tsang, C. M., Yip, Y. L., Lo, K. W., Deng, W., To, K. F., Hau, P. M., et al. (2012). Cyclin D1 overexpression supports stable EBV infection in nasopharyngeal epithelial cells. Proc. Natl. Acad. Sci. U. S. A. 109, E3473–E3482. doi: 10.1073/pnas.1202637109
Tsao, S. W., Yip, Y. L., Tsang, C. M., Pang, P. S., Lau, V. M. Y., Zhang, G., et al. (2014). Etiological factors of nasopharyngeal carcinoma. Oral Oncol. 50, 330–338. doi: 10.1016/j.oraloncology.2014.02.006
Tso, K. K. Y., Yip, K. Y. L., Mak, C. K. Y., Chung, G. T. Y., Lee, S. D., Cheung, S. T., et al. (2013). Complete genomic sequence of Epstein-Barr virus in nasopharyngeal carcinoma cell line C666-1. Infect Agent Cancer 8, 1–4. doi: 10.1186/1750-9378-8-29
Do, N.Van, Ingemar, E., Phi, P. T. P., Jenny, A., Chinh, T. T., Zeng, Y. X., et al. (2008). A major EBNA1 variant from Asian EBV isolates shows enhanced transcriptional activity compared to prototype B95.8. Virus Res. 132, 15–24. doi: 10.1016/j.virusres.2007.10.020
Wan, F.-Z., Chen, K.-H., Sun, Y.-C., Chen, X.-C., Liang, R.-B., Chen, L., et al. (2020). Exosomes overexpressing miR-34c inhibit malignant behavior and reverse the radioresistance of nasopharyngeal carcinoma. J. Transl. Med. 18:12. doi: 10.1186/s12967-019-02203-z
Wang, K. H., Austin, S. A., Chen, S. H., Sonne, D. C., and Gurushanthaiah, D. (2017). Nasopharyngeal carcinoma diagnostic challenge in a nonendemic setting: our experience with 101 patients. Perm. J. 21, 16–180. doi: 10.7812/TPP/16-180
Wang, J., Ge, J., Wang, Y., Xiong, F., Guo, J., Jiang, X., et al. (2022). EBV miRNAs BART11 and BART17-3p promote immune escape through the enhancer-mediated transcription of PD-L1. Nat. Commun. 13:866. doi: 10.1038/s41467-022-28479-2
Wang, J., Guo, X., Wu, G. I., Jiao, Y., Damola Faleti, O., Liu, P., et al. (2018). EBV-miR-BART1-5P activates AMPK/mTOR/ HIF1 pathway via a PTEN independent manner to promote glycolysis and angiogenesis in nasopharyngeal carcinoma. PLoS Pathog. 14:e1007484. doi: 10.1371/journal.ppat.1007484
Wang, J. N., Hu, P., Zeng, M. S., and Liu, R. bin (2011). Anti-tumor effect of oncolytic herpes simplex virus G47delta on human nasopharyngeal carcinoma. Chin. J. Cancer 30:41. doi: 10.5732/CJC.011.10301
Wang, J., Luo, Y., Bi, P., Lu, J., Wang, F., Liu, X., et al. (2020). Mechanisms of Epstein-Barr virus nuclear antigen 1 favor Tregs accumulation in nasopharyngeal carcinoma. Cancer Med. 9, 5598–5608. doi: 10.1002/cam4.3213
Wang, H. B., Zhang, H., Zhang, J. P., Li, Y., Zhao, B., Feng, G. K., et al. (2015). Neuropilin 1 is an entry factor that promotes EBV infection of nasopharyngeal epithelial cells. Nat. Commun. 6:7240. doi: 10.1038/ncomms7240
Wee, J., Nei, W. L., Yeoh, K. W., Yeo, R. M., Loong, S. L., and Qian, C. N. (2012). Why are east Asians more susceptible to several infection-associated cancers (carcinomas of the nasopharynx, stomach, liver, adenocarcinoma of the lung, nasal NK/T-cell lymphomas)? Med. Hypotheses 79, 833–842. doi: 10.1016/j.mehy.2012.09.003
Weiss, E. R., Lamers, S. L., Henderson, J. L., Melnikov, A., Somasundaran, M., Garber, M., et al. (2018). Early Epstein-Barr virus genomic diversity and convergence toward the B95.8 genome in primary infection. J. Virol. 92, 1466–1483. doi: 10.1128/JVI.01466-17
Wildeman, M. A., Novalić, Z., Verkuijlen, S. A. W. M., Juwana, H., Huitema, A. D. R., Tan, I. B., et al. (2012). Cytolytic virus activation therapy for Epstein-Barr virus-driven tumors. Clin. Cancer Res. 18, 5061–5070. doi: 10.1158/1078-0432.CCR-12-0574
Wong, K. C. W., Hui, E. P., Lo, K. W., Lam, W. K. J., Johnson, D., Li, L., et al. (2021). Nasopharyngeal carcinoma: an evolving paradigm. Nat. Rev. Clin. Oncol. 18, 679–695. doi: 10.1038/s41571-021-00524-x
Wong, Y., Meehan, M. T., Burrows, S. R., Doolan, D. L., and Miles, J. J. (2022). Estimating the global burden of Epstein–Barr virus-related cancers. J. Cancer Res. Clin. Oncol. 148, 31–46. doi: 10.1007/s00432-021-03824-y
Wu, M.-Y., Huang, S.-J., Yang, F., Qin, X.-T., Liu, D., Ding, Y., et al. (2017). Detection of nasopharyngeal carcinoma susceptibility with single nucleotide polymorphism analysis using next-generation sequencing technology. Oncotarget 8, 52708–52723. doi: 10.18632/oncotarget.17085
Wu, S., Liu, W., Li, H., Zhao, Z., Yang, Y., Xiao, H., et al. (2018). Conservation and polymorphism of EBV RPMS1 gene in EBV-associated tumors and healthy individuals from endemic and non-endemic nasopharyngeal carcinoma areas in China. Virus Res. 250, 75–80. doi: 10.1016/j.virusres.2018.04.009
Wu, Z.-X., Xiang, L., Rong, J.-F., He, H.-L., and Li, D. (2016). Nasopharyngeal carcinoma with headaches as the main symptom: a potential diagnostic pitfall. J. Cancer Res. Ther. 12, 209–214. doi: 10.4103/0973-1482.157334
Wu, Y.-L., Yang, K.-B., Huang, Y., Shi, J.-R., He, Q.-S., Chen, L., et al. (2022). Selection and validation of chemotherapy beneficiaries among elderly nasopharyngeal carcinoma (NPC) patients treated with intensity-modulated radiation therapy (IMRT): a large real-world study. Radiat. Oncol. 17, 1–14. doi: 10.1186/s13014-022-02095-2
Xiang, T., Lin, Y.-X., Ma, W., Zhang, H.-J., Chen, K.-M., He, G.-P., et al. (2018). Vasculogenic mimicry formation in EBV-associated epithelial malignancies. Nat. Commun. 9:5009. doi: 10.1038/s41467-018-07308-5
Xiao, L., Hu, Z.-Y., Dong, X., Tan, Z., Li, W., Tang, M., et al. (2014). Targeting Epstein-Barr virus oncoprotein LMP1-mediated glycolysis sensitizes nasopharyngeal carcinoma to radiation therapy. Oncogene 33, 4568–4578. doi: 10.1038/onc.2014.32
Xu, S., Bai, J., Zhuan, Z., Li, B., Zhang, Z., Wu, X., et al. (2018). EBV-LMP1 is involved in vasculogenic mimicry formation via VEGFA/VEGFR1 signaling in nasopharyngeal carcinoma. Oncol. Rep. 40, 377–384. doi: 10.3892/or.2018.6414
Xu, C., Chen, Y. P., Liu, X., Li, W. F., Chen, L., Mao, Y. P., et al. (2017). Establishing and applying nomograms based on the 8th edition of the UICC/AJCC staging system to select patients with nasopharyngeal carcinoma who benefit from induction chemotherapy plus concurrent chemoradiotherapy. Oral Oncol. 69, 99–107. doi: 10.1016/j.oraloncology.2017.04.015
Xu, F. H., Xiong, D., Xu, Y. F., Cao, S. M., Xue, W. Q., Qin, H.De, et al. (2012). An Epidemiological and molecular study of the relationship between smoking, risk of nasopharyngeal carcinoma, and Epstein–Barr virus activation. JNCI: J. National Cancer Institute 104, 1396–1410. doi: 10.1093/jnci/djs320
Xu, M., Yao, Y., Chen, H., Zhang, S., Cao, S.-M., Zhang, Z., et al. (2019). Genome sequencing analysis identifies Epstein-Barr virus subtypes associated with high risk of nasopharyngeal carcinoma. Nat. Genet. 51, 1131–1136. doi: 10.1038/s41588-019-0436-5
Xue, W.-Q., Wang, T.-M., Huang, J.-W., Zhang, J.-B., He, Y.-Q., Wu, Z.-Y., et al. (2021). A comprehensive analysis of genetic diversity of EBV reveals potential high-risk subtypes associated with nasopharyngeal carcinoma in China. Virus Evol 7:10. doi: 10.1093/ve/veab010
Yajima, M., Ikuta, K., and Kanda, T. (2018). Rapid CRISPR/Cas9-mediated cloning of full-length Epstein-Barr virus genomes from latently infected cells. Viruses 10:171. doi: 10.3390/v10040171
Yan, Q., Zeng, Z., Gong, Z., Zhang, W., Li, X., He, B., et al. (2015). EBV-miR-BART10-3p facilitates epithelial-mesenchymal transition and promotes metastasis of nasopharyngeal carcinoma by targeting BTRC. Oncotarget 6, 41766–41782. doi: 10.18632/oncotarget.6155
Yang, S.-S., Guo, J.-G., Liu, J.-N., Liu, Z.-Q., Chen, E.-N., Chen, C.-Y., et al. (2021). Effect of induction chemotherapy in nasopharyngeal carcinoma: an updated meta-analysis. Analysis. Front. Oncol 10:591205. doi: 10.3389/fonc.2020.591205
Yang, L., Liu, L., Xu, Z., Liao, W., Feng, D., Dong, X., et al. (2015). EBV-LMP1 targeted DNAzyme enhances radiosensitivity by inhibiting tumor angiogenesis via the JNKs/HIF-1 pathway in nasopharyngeal carcinoma. Oncotarget 6, 5804–5817. doi: 10.18632/oncotarget.3331
Yang, L., Xu, Z., Liu, L., Luo, X., Lu, J., Sun, L., et al. (2014). Targeting EBV-LMP1 DNAzyme enhances radiosensitivity of nasopharyngeal carcinoma cells by inhibiting telomerase activity. Cancer Biol. Ther. 15, 61–68. doi: 10.4161/CBT.26606
Yang, T., You, C., Meng, S., Lai, Z., Ai, W., and Zhang, J. (2022). EBV infection and its regulated metabolic reprogramming in nasopharyngeal tumorigenesis. Front. Cell. Infect. Microbiol. 12:5205. doi: 10.3389/fcimb.2022.935205
Yao, M., Ohshima, K., Suzumiya, J., Kume, T., Shiroshita, T., and Kikuchi, M. (1997). Interleukin-10 expression and cytotoxic-T-cell response in Epstein-Barr-virus-associated nasopharyngeal carcinoma. J. Cancer 72, 398–402. doi: 10.1002/(sici)1097-0215(19970729)72:3<398::aid-ijc4>3.0.co;2-k
Yap, L. F., Kang, A., Wong, C., Paterson, I. C., and Young, L. S. (2022). Functional implications of Epstein-Barr virus lytic genes in carcinogenesis. Cancers (Basel) 14:5780. doi: 10.3390/cancers14235780
Ye, J., Wei, J., Luo, Y., Deng, Y., Que, T., Zhang, X., et al. (2021). Epstein-barr virus promotes tumor angiogenesis by activating STIM1-dependent Ca2+ signaling in nasopharyngeal carcinoma. Pathogens 10:1275. doi: 10.3390/pathogens10101275
Ye, D., Zhu, J., Zhao, Q., Ma, W., Xiao, Y., Xu, G., et al. (2020). LMP1 up-regulates calreticulin to induce epithelial-mesenchymal transition via TGF-β/Smad3/NRP1 pathway in nasopharyngeal carcinoma cells. J. Cancer 11, 1257–1269. doi: 10.7150/jca.37415
Yiu, S. P. T., Hui, K. F., Choi, C. K., Kao, R. Y. T., Ma, C. W., Yang, D., et al. (2018). Intracellular iron chelation by a novel compound, C7, reactivates Epstein–Barr virus (EBV) lytic cycle via the ERK-autophagy Axis in EBV-positive epithelial cancers. Cancers (Basel) 10:505. doi: 10.3390/cancers10120505
Young, L. S., Yap, L. F., and Murray, P. G. (2016). Epstein-Barr virus: more than 50 years old and still providing surprises. Nat. Rev. Cancer 16, 789–802. doi: 10.1038/nrc.2016.92
Yu, G., Hsu, W. L., Coghill, A. E., Yu, K. J., Wang, C. P., Lou, P. J., et al. (2019). Whole-exome sequencing of nasopharyngeal carcinoma families reveals novel variants potentially involved in nasopharyngeal carcinoma. Sci. Rep. 9:9916. doi: 10.1038/S41598-019-46137-4
Yu, F., Syn, N. L., Lu, Y., Chong, Q. Y., Lai, J., Tan, W. J., et al. (2021a). Characterization and establishment of a novel EBV strain simultaneously associated with nasopharyngeal carcinoma and B-cell lymphoma. Front. Oncol. 11:626659. doi: 10.3389/fonc.2021.626659
Yu, H., Yin, X., Mao, Y., Chen, M., Tang, Q., and Yan, S. (2021b). The global burden of nasopharyngeal carcinoma from 2009 to 2019: an observational study based on the global burden of disease study 2019. Eur. Arch. Otorhinolaryngol. 1:3. doi: 10.1007/s00405-021-06922-2
Yua, F., Lu, Y., Tay, K. J., Yoshiyam, H., and Seng Loh, K. (2018). Establishment of EBV latency in nasopharyngeal tumor epithelial cells by in vivo cell-mediated transfer infection. Otorhinolaryngol Head Neck Surg 3:174. doi: 10.15761/OHNS.1000174
Zeng, M.-S., Li, D.-J., Liu, Q.-L., Song, L.-B., Li, M.-Z., Zhang, R.-H., et al. (2005). Genomic sequence analysis of Epstein-Barr virus strain GD1 from a nasopharyngeal carcinoma patient. J. Virol. 79, 15323–15330. doi: 10.1128/JVI.79.24.15323-15330.2005
Zeng, Y., Liu, Y., Liu, C., Chen, S., Wei, J., Zhu, J., et al. (1980). Application of an immunoenzymatic method and an immunoautoradiographic method for a mass survey of NPC. Intervirology 13, 162–168.
Zeng, Y., Si, Y. F., Lan, G. P., Wang, Z., Zhou, L., Tang, M. Z., et al. (2020). LMP2-DC vaccine elicits specific EBV-LMP2 response to effectively improve immunotherapy in patients with nasopharyngeal cancer. Biomed. Environ. Sci. 33, 849–856. doi: 10.3967/bes2020.115
Zeng, Y., Zhang, L., Jml, M., Zhang, Q., Wu, Y., WmG, Y., et al. (1982). Serological mass survey for early detection of nasopharyngeal carcinoma in Wuzhou City. Int. J. Cancer 29, 139–141. doi: 10.1002/ijc.2910290204
Zha, S., Chau, H. F., Chau, W. Y., Chan, L. S., Lin, J., Lo, K. W., et al. (2021). Dual-targeting peptide-guided approach for precision delivery and cancer monitoring by using a safe Upconversion Nanoplatform. Advanced Science 8:2002919. doi: 10.1002/advs.202002919
Zha, S., Fung, Y. H., Chau, H. F., Ma, P., Lin, J., Wang, J., et al. (2018). Responsive upconversion nanoprobe for monitoring and inhibition of EBV-associated cancers via targeting EBNA1. Nanoscale 10, 15632–15640. doi: 10.1039/C8NR05015E
Zhang, T., Chen, Z., Deng, J., Xu, K., Che, D., Lin, J., et al. (2022). Epstein–Barr virus-encoded microRNA BART22 serves as novel biomarkers and drives malignant transformation of nasopharyngeal carcinoma. Cell Death Dis. 13, 1–14. doi: 10.1038/s41419-022-05107-x
Zhang, J., Jia, L., Lin, W., Yip, Y. L., Lo, K. W., Lau, V. M. Y., et al. (2017). Epstein-Barr virus-encoded latent membrane protein 1 upregulates glucose transporter 1 transcription via the mTORC1/NF-κB signaling pathways. J. Virol. 91:e02168-16. doi: 10.1128/JVI.02168-16
Zhang, H., Li, Y., Wang, H. B., Zhang, A., Chen, M. L., Fang, Z. X., et al. (2018). Ephrin receptor A2 is an epithelial cell receptor for Epstein-Barr virus entry. Nat. Microbiol. 3, 164–171. doi: 10.1038/s41564-017-0080-8
Zhang, S. Q., Pan, S. M., Liang, S. X., Han, Y. S., Chen, H. B., and Li, J. C. (2021b). Research status and prospects of biomarkers for nasopharyngeal carcinoma in the era of high-throughput omics (review). Int. J. Oncol. 58:188. doi: 10.3892/IJO.2021.5188
Zhang, X. S., Wang, H. H., Hu, L. F., Li, A., Zhang, R. H., Mai, H. Q., et al. (2004). V-val subtype of Epstein-Barr virus nuclear antigen 1 preferentially exists in biopsies of nasopharyngeal carcinoma. Cancer Lett. 211, 11–18. doi: 10.1016/j.canlet.2004.01.035
Zhang, L., Wu, X., Liu, J., Zhang, B., Mo, X., Chen, Q., et al. (2021a). MRI-based deep-learning model for distant metastasis-free survival in Locoregionally advanced nasopharyngeal carcinoma. J. Magn. Reson. Imaging 53, 167–178. doi: 10.1002/jmri.27308
Zhang, G., Zong, J., Lin, S., Verhoeven, R. J. A., Tong, S., Chen, Y., et al. (2015). Circulating Epstein-Barr virus microRNAs miR-BART7 and miR-BART13 as biomarkers for nasopharyngeal carcinoma diagnosis and treatment. Authors Int. J. Cancer 136, E301–E312. doi: 10.1002/ijc.29206
Zheng, X. H., Lu, L. X., Li, X. Z., and Jia, W. H. (2015a). Quantification of Epstein-Barr virus DNA load in nasopharyngeal brushing samples in the diagnosis of nasopharyngeal carcinoma in southern China. Cancer Sci. 106, 1196–1201. doi: 10.1111/CAS.12718
Zheng, S., Matskova, L., Zhou, X., Xiao, X., Huang, G., Zhang, Z., et al. (2020). Downregulation of adipose triglyceride lipase by EB viral-encoded LMP2A links lipid accumulation to increased migration in nasopharyngeal carcinoma. Mol. Oncol. 14, 3234–3252. doi: 10.1002/1878-0261.12824
Zheng, Y., Parsonage, G., Zhuang, X., Machado, L. R., James, C. H., Salman, A., et al. (2015b). Human leukocyte antigen (HLA) a∗1101-restricted Epstein-Barr virus-specific T-cell receptor gene transfer to target nasopharyngeal carcinoma. Cancer Immunol. Res. 3, 1138–1147. doi: 10.1158/2326-6066.CIR-14-0203-T
Zhong, L., Dong, D., Fang, X., Zhang, F., Zhang, N., Zhang, L., et al. (2021). A deep learning-based radiomic nomogram for prognosis and treatment decision in advanced nasopharyngeal carcinoma: a multicentre study. EBioMedicine 70:103522. doi: 10.1016/j.ebiom.2021.103522
Keywords: nasopharyngeal carcinoma, Epstein–Barr virus, biomarker, therapeutic, diagnostic, screening
Citation: Su ZY, Siak PY, Leong C-O and Cheah S-C (2023) The role of Epstein–Barr virus in nasopharyngeal carcinoma. Front. Microbiol. 14:1116143. doi: 10.3389/fmicb.2023.1116143
Edited by:
Mei-Ru Chen, National Taiwan University, TaiwanReviewed by:
Yonggang Pei, Southern University of Science and Technology, ChinaTakayuki Murata, Fujita Health University, Japan
Copyright © 2023 Su, Siak, Leong and Cheah. This is an open-access article distributed under the terms of the Creative Commons Attribution License (CC BY). The use, distribution or reproduction in other forums is permitted, provided the original author(s) and the copyright owner(s) are credited and that the original publication in this journal is cited, in accordance with accepted academic practice. No use, distribution or reproduction is permitted which does not comply with these terms.
*Correspondence: Shiau-Chuen Cheah, ✉ cheahsc@ucsiuniversity.edu.my