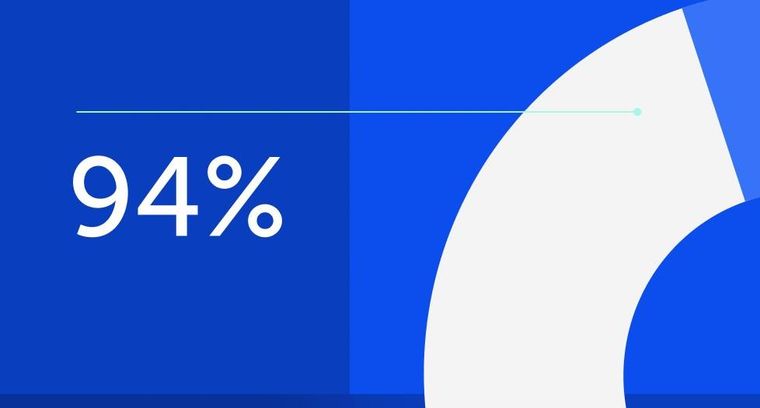
94% of researchers rate our articles as excellent or good
Learn more about the work of our research integrity team to safeguard the quality of each article we publish.
Find out more
ORIGINAL RESEARCH article
Front. Microbiol., 16 February 2023
Sec. Microorganisms in Vertebrate Digestive Systems
Volume 14 - 2023 | https://doi.org/10.3389/fmicb.2023.1113730
This article is part of the Research TopicThe Potential Role of Gut Microbiome in Animal Gut-Linked DiseasesView all 16 articles
Weining cattle is a precious species with high tolerance to cold, disease, and stress, and accounts for a large proportion of agricultural economic output in Guizhou, China. However, there are gaps in information about the intestinal flora of Weining cattle. In this study, high-throughput sequencing were employed to analyze the intestinal flora of Weining cattle (WN), Angus cattle (An), and diarrheal Angus cattle (DA), and explore the potential bacteria associated with diarrhea. We collected 18 fecal samples from Weining, Guizhou, including Weining cattle, Healthy Angus, and Diarrheal Angus. The results of intestinal microbiota analysis showed there were no significant differences in intestinal flora diversity and richness among groups (p > 0.05). The abundance of beneficial bacteria (Lachnospiraceae, Rikenellaceae, Coprostanoligenes, and Cyanobacteria) in Weining cattle were significantly higher than in Angus cattle (p < 0.05). The potential pathogens including Anaerosporobacter and Campylobacteria were enriched in the DA group. Furthermore, the abundance of Lachnospiraceae was very high in the WN group (p < 0.05), which might explain why Weining cattle are less prone to diarrhea. This is the first report on the intestinal flora of Weining cattle, furthering understanding of the relationship between intestinal flora and health.
Weining is located in southwest China at an average altitude of 2,200 meters. It is a large livestock county where cattle raising is the main economic income of farmers. In 2021, the stock of cattle in Weining was 153,600, accounting for 6.5% of the local agricultural output, with the most farmed species being Weining cattle and Angus cattle. The Weining cattle are an ancient ruminant with hypoxia tolerance, anti-oxidant action, and disease resistance, and have become the symbol of Weining Guizhou. They resemble ordinary cows with short horns and yellow hair, but also have many characteristics of their own. Due to the nutritious quality of their meat, their strength, and their docility, Weining play a significant role in local farming and prosperity. In addition, the incidence of intestinal diseases is fairly low compared to other types of cattle, one of the most important reasons for local people keeping Weining cattle.
The normal intestine harbors over 100 trillion microorganisms including bacteria (98%), fungi (0.1%), viruses, protists, archaea, and these microbial communities (Liu J. et al., 2019; Li et al., 2021). The gut microbiota colonizing the intestinal tract forms a symbiotic relationship with the host and plays a vital role in maintaining nutrient intake, immune regulation, and intestinal barrier integrity. In addition, the gut microbiome is believed to be a biochemical transformation that exerts beneficial substances such as antimicrobial peptides, vitamins, and enzymes. Increasing evidence suggests that the diversity and composition of the gut microbiome have been linked to species and health, with species being the primary cause of the gut microbiota, followed by health status. However, to date, knowledge of the gut microbiota characteristics of Weining and Angus cattle is limited.
As is well known, diarrhea occurs in all animals, especially in newborns, and causes death in about half of the ruminants (Li et al., 2018; Bu et al., 2020). Calf mortality due to diarrhea remains very high in most countries, e.g., 17% in Germany and 5% in the United States (Urie et al., 2018; Eibl et al., 2021). Several studies have also indicated that intestinal microbial dysbiosis drives the development of diarrhea (Han et al., 2017; Shao et al., 2020). Healthy and balanced intestinal flora reduce the risk of diarrhea (Huang et al., 2019; Zuo et al., 2021). Previous studies have shown that intestinal bacteria in some ruminants alternate between dominant and weak populations with diarrheal symptoms (Yang et al., 2017). Thus, there may be some unavoidable links between the alteration of intestinal microbial communities and diarrhea. In the last few years, we have found that other types of cattle like Angus were more susceptible to diarrhea in Weining China. The mortality rate of other types of cattle was 9%, whereas in Weining cattle it was 3%. However, so far, there is little information about the relationship between diarrhea and gut microbiota in Angus cattle.
Regarding this phenomenon, we speculated about whether the low incidence of diarrhea in Weining cattle was related to intestinal flora. Possible reasons are that the Weining cattle’s ancestors lived in relative isolation after entering the mountains and developed individual gut flora. However, the characteristics of gut microbiota in Weining cattle remain unclear. Here we seek to investigate and compare the composition and variability of gut bacteria in Weining cattle (WN), healthy Angus cattle (An), and diarrheal Angus cattle (DA). In addition, although there have been recent studies on gut microbiota and diarrhea, very few studies have been conducted on Angus cattle, and our study aims to better understand how gut microbes affect organismal health, exploring potential pathogenic microbes. Meanwhile, we aimed to explore the potential bacteria associated with disease resistance. These findings will aid in the future development of dietary interventions that may resolve or prevent enteric and diarrheal diseases in ruminants.
Samples were taken between June and August 2022 in Weining China, the peak period for diarrhea incidence in cattle. In total, 18 individual fresh fecal samples were taken from 6 Weining cattle, 6 healthy Angus cattle, and 6 diarrheal cattle. All the cattle were a half-year-old and had lived in the same conditions. Prior to sampling, all specimens are tested by a professional veterinarian to assess their health, and the samples collected are immediately placed in liquid nitrogen fixation and transported back to the laboratory in dry ice as soon as possible.
DNA was extracted from 200 mg of feces using the QIAamp DNA Mini Kit (QIAGEN, Hilden, Germany). A fragment from the V3-V4 hypervariable region of the bacterial 16S rRNA gene was amplified using the linker primer 338F (ACTCCTACGGGAGGCAGCA) and the reverse primer 806R (GGACTACHVGGGTWTCTAAT). PCR reactions contained: 1 μL forward index primer (10 mM), 1 μL reverse index primer (10 mm), 1 μL 10 ng/μL DNA template, and 17 μL mixPfx AccuPrime master (Invitrogen, United States). The reaction conditions are as follows: initial denaturation at 95°C for 5 min, followed by 30 cycles of denaturation at 95°C for 30 s, annealing at 55°C for 30 s, and extension at 72°C for 1 min, and final elongation for 5 min at 72°C. The PCR amplification was performed in duplicate under the same conditions to ensure the accuracy of the results. In addition, we constructed the quality libraries with a single peak and concentration of more than 2 nM using a bioanalyzer (Agilent Technologies, United States) and quantitative PCR (qPCR). At last, the qualified library was sequenced on the Hiseq6000 platform (Illumina, United States), targeting the sequences with paired-end reads (Wang et al., 2022).
Quality screening of raw data generated by high-throughput sequencing using QIIME software (Qiime1.9.1). Questionable sequences such as short sequences (<200 bp), mismatched primers, and chimeras were removed. The resulting eligible sequences were segmented and clustered by OTU based on 97% similarity. The αlpha diversity indices of gut diversity were calculated based on the relative abundance distribution of OTUs in each sample. Meanwhile, βeta diversity indices were used to dissect the differences and similarities of the major components of the gut flora. Additionally, sparsity curves were generated for each sample to assess the sequencing depth. The data were statistically analyzed using GraphPad Prism (version 9.0c). Data are expressed as Mean ± SD, and p < 0.05 was considered statistically significant.
In the 16S rDNA high-throughput sequencing, 18 stool samples yielded a total of 1,441,028 raw sequences, of which the AN (healthy Angus), DA (Diarrheal Angus), and WN (Weining cattle) groups contained 480,043, 480,101, and 480,884 sequences, respectively (Table 1). The quality of the raw data was assessed and a total of 1,434,904 qualified sequences were obtained (An: 479260, DA: 479311, WN: 480104). Both the sparsity curve and the rank abundance curve showed a saturation trend, indicating that the depth and uniformity of sequencing could meet the requirements of the subsequent analysis (Figures 1A–C). The qualified sequences were clustered into 2,736 OTUs based on 97% nucleic acid sequence similarity, with the number of OTUs per sample ranging from 495 to 806 (Figures 1D,E). There were 841 shared OTUs in AN, DA, and WN groups and unique OTUs in each group were 232, 241, and 503, respectively.
Figure 1. Feasibility analysis and OTUs distribution of amplicon sequencing. (A,B) Rarefaction curves. (C) rank abundance curves. (D) Venn diagram. (E) The numbers OTUs in each sample.
Alpha analysis was applied to discover the difference in intestinal microbial community richness and diversity. The averages of the Shannon index were 8.64, 8.79, and 8.96 in the WN, An, and DA groups (p < 0.05). Moreover, there were no significant differences in the Chao1 (An = 714.67, DA = 772.67, WN = 657.25), Simpson (An = 0.99, DA = 0.99, WN = 0.99), and ACE (An = 714.40, DA = 772.78, WN = 657.29) index, indicating that the diversity of intestinal flora in groups WN, An and DA were not significantly different (Figures 2A–D). PCoA was applied to dissect the gut microbial variability and similarity among intergroup and intragroup individuals. The results of PCoA and NMDS showed that the samples from An and DA were clustered together and separated from the WN group, indicating that the intestinal flora composition of Weining cattle was different from Angus cattle, and the intestinal microbiota community diversity index was slightly affected by diarrhea in the An and DA groups (Figures 2E,F).
Figure 2. Changes of gut microbial diversity associated with species and diarrhea. (A–D) Chao1, ACE, Shannon, and Simpson indices. (E,F) PCoA plots based on the weighted and unweighted UniFrac distance.
The relative proportions of different levels of sample-dominated flora were detected by microbial taxon assignment. At the phyla level, a total of 12 phyla were detected from all samples, ranging from 8 to 11 per sample. Firmicutes (63.89, 64.87, and 67.80%), Bacteroidota (32.42, 31.42, and 26.86%), Desulfobacterota (0.93, 0.93, and 1.52%) were the most abundant in the An, DA, and WN groups under phyla level, occupying more than 90% of all bacteria composition (Figure 3A). Proteobacteria (0.63, 0.50, and 1.22%), Cyanobacteria (0.38, 0.61, and 1.01%), Fibrobacterota (0.04, 0.13, and 0.23%), Spirochaetota (0.02, 0.12, and 0.09%), Patescibacteria (0.90, 0.49, and 0.57%), Verrucomicrobiota (0.55, 0.54, and 0.56%), and Campylobacterota (0.19, 0.32, and 0.01%) were observed with a lower abundance in the An, DA, and WN groups.
Figure 3. Changes of gut microbial composition associated with species and diarrhea. (A,B) Composition and the relative ratio of preponderant bacteria at the phylum and genus levels. (C) Heatmap of the 50 most abundant bacterial genera.
Among the genus identified, the Lachnospiraceae (12.23%) was notably enriched in the WT group, closely followed by UCG_005 (10.38), unclassified_UCG_010 (0.73%), Rikenellaceae_RC9_gut_group (0.55%), unclassified_[Eubacterium]_coprostanoligenes_group (6.8%), Alistipes (3.0%) and Monoglobus (4.3%). The results showed a very high proportion of beneficial bacteria in the Weining cattle.
In addition, the Rikenellaceae_RC9 (6.2 and 6.3%), UCG_005 (11.79 and 10.55%), Lachnospiraceae (6.29 and 4.82%), unclassified_[Eubacterium]_coprostanoligenes_group (5.57 and 5.56%), Prevotellaceae_UCG_003 (4.74 and 4.86%), Monoglobus (3.12 and 3.09%), and unclassified_Oscillospiraceae (3.23 and 2.98%) were the main bacterial genus in An and DA groups (Figure 3B). The data indicated that most of the bacteria that are beneficial for health are significantly higher in the An group than in the DA group. The heatmap showed higher intra-group similarity and greater inter-group variability, revealing differences in gut microbiota composition between the An, DA, and WN groups (Figure 3C).
Metastatic analysis was performed to explore differences in gut microbiota between the WN, An, and DA groups. A comparison of the An and DA groups showed a significant decline in the abundances of 7 genus (unclassified_rumen_bacterium_YS2, unclassified_[Clostridium]_methylpentosum_group, unclassified_Butyricicoccaceae, uncultured_Ruminococcaceae_bacterium, unclassified_Peptostreptococcaceae, Anaerosporobacter, and unclassified_Oscillospirales) as well as a significant increase in the abundances of 3 genus (Defluviitaleaceae_UCG_011, Dorea, and UCG_009; Figure 4). At the phyla level, the An group showed dramatically higher abundances of Campylobacterota and Bacteroidota, whereas the WN group enriched for Cyanobacteria and Elusimicrobiota. Compared with the An group, the gut microbiota in the WN group showed a distinct decrease in the relative abundances of Prevotellaceae_UCG_003, unclassified_Clostridia_vadinBB60_group, Campylobacter, Faecalibacterium, Erysipelotrichaceae_UCG_009, UCG_004, unclassified_Paludibacteraceae, Candidatus_Soleaferrea, unclassified_gir_aah93h0, unclassified_UCG_010, Saccharofermentans, unclassified_Erysipelatoclostridiaceae, Parabacteroides, NK4A214_group, unclassified_Rikenellaceae, Dorea, unclassified_Oscillospiraceae, EMP_G18, dgA_11_gut_group, unclassified_Barnesiellaceae, Parasutterella, Anaerofustis, Romboutsia, Papillibacter, uncultured_compost_bacterium, UCG_005, unclassified_Bacteroidales_RF16_group and Blautia, whereas Anaerosporobacter, unclassified_Lachnospiraceae, Lachnospiraceae_UCG_001, Ruminobacter, unclassified_Gastranaerophilales, unclassified_Hydrogenoanaerobacterium, unclassified_[Eubacterium]_coprostanoligenes_group, uncultured_rumen_bacterium, uncultured_Clostridium_sp., Peptococcus, Frisingicoccus, unclassified_Oscillospirales, Anaerovorax, Caproiciproducens, uncultured_Alphaproteobacteria_bacterium, [Eubacterium]_ruminantium_group, Ruminococcus, Paludicola, unclassified_Clostridia_UCG_014, unclassified_Muribaculaceae, and Monoglobus increased significantly (Figure 5). Moreover, the cladogram was generated by applying LefSe to further investigate variability in bacterial taxa composition. In addition to the significantly different bacteria mentioned above, we observed that several bacteria such as Campylobacteria and Anaerosporobacter were reached in the DA group, whereas beneficial bacteria such as Lachnospiraceae were significantly overrepresented in the WN group (Figure 6).
Figure 4. Statistical analysis of differential bacteria between An and DA groups at the phylum and genus levels.
Figure 5. Statistical analysis of differential bacteria between An and WN groups at the phylum and genus levels.
Figure 6. LEfSe integrated with LDA scores recognized differentially abundant taxon related to species and diarrhea. (A,B) Cladogram shows the phylogenetic distribution of differential taxon. (C) LDA scores >2 are considered significant.
Prevotellaceae_UCG_003 was positively associated with unclassified_UCG_010. Ruminobacter was negatively related to dgA_11_gut_group, uncultured_Ruminococcaceae_bacterium, unclassified_UCG_010, UCG_009, NK4A214_group, unclassified_Clostridia_vadinBB60_group, Prevotellaceae_UCG_003 but positively associated with unclassified_Lachnospiraceae, UCG_002 and Monoglobus (Figure 7).
Figure 7. Correlation network analysis of gut microbiota. Positive and negative correlations are indicated by red and green lines, respectively.
Diarrhea is the most common disease in calves and severely affects the animal’s growth and development. According to previous reports, the economic damage caused by diarrhea is enormous and difficult to control (Fischer et al., 2016; Lorenz et al., 2021). Studies indicated that the etiology of bovine diarrhea is multifactorial, with pathogens and management factors (housing, feeding, and sanitary conditions) playing an important role (Bendali et al., 1999). A study in diarrheal calves in Belgium estimated the prevalence of E. coliRotavirus, Coronavirus, and C. parvum at 4, 20, 8, and 31%, respectively. In a recent Swiss study on diarrheal calves, the prevalence of these Enteropathogens remained at high levels. Moreover, diarrhea inevitably accompanies intestinal damage, suggesting that the intestinal microbiota may be altered (Xia et al., 2018; Zhai et al., 2019). As the habitat of intestinal flora, the gastrointestinal tract is more susceptible to various diseases such as inflammatory bowel disease and diarrhea due to the influence of intestinal flora (Liu C. S. et al., 2019; Yue et al., 2019; Zhang et al., 2022). In addition, although the gut microbiota inhabits the gut, significant changes in the gut microbiota may also lead to the development of other diseases such as liver disease, diabetes, and obesity, etc. (Guo et al., 2022; Ye et al., 2022). More importantly, significant changes in gut microbiota also affect gut permeability, which may lead to a leaky gut and increased rates of pathogenic bacterial infection (Yue et al., 2020; Xu et al., 2021). Therefore, the characterization of gut microbiota is crucial for the prevention, control, and diagnosis of diarrhea (Bjorkman et al., 2003; Singh et al., 2015).
Gut microbial diversity and richness constantly decrease under the influence of diarrhea (Zhang L. et al., 2020; Cui et al., 2021; Ren et al., 2022). In our study, we found no significant difference in gut microbiota diversity and richness between the An group and DA group, the lack of difference is most likely due to an increase in pathogenic bacteria due to diarrhea and a decrease in beneficial bacteria in the DA group. In Han’s report, there was no significant difference in gut microbial diversity between healthy and diarrheal yaks, which was consistent with our findings (Han et al., 2017). Similarly, He′s results are also similar to ours: diarrhea does not significantly alter the diversity and richness of gut microbiota in pigs (He et al., 2020). Although the diversity and richness of intestinal microbiota did not change significantly, the composition of bacteria did. Anaerosporbacter were rich in the DA group but were not detected in the An group. Anaerosporbacter is likely to be associated with the occurrence of colorectal cancer. In Yu’s study, the results manifested that the Anaerosporbacter were abundant in the colorectal cancer group compared to the healthy group (Yu et al., 2017). Campylobacter is recognized as the most common cause of bacterial enteritis (Liu et al., 2018). Among bacterial infections reported in recent years, Campylobacter spp. predominated. In Singh’s report, Campylobacter usually causes asymptomatic infections, diarrhea, and hemorrhagic colitis (Singh et al., 2015; Shin et al., 2021). Interestingly, the aforementioned potentially pathogenic bacteria exhibited a significant relative abundance in the DA group compared to the An group. Candidatus Soleaferrea secretes homeostatic protective properties and has anti-inflammatory effects (Zhang et al., 2015), there was no significant difference in the proportion of Candidatus Soleaferrea in the An and DA groups. Conversely, the beneficial bacteria including Dorea, Muribaculaceae, UCG-009, and Monoglobus were significantly lower in the DA group compared with healthy Angus cattle. Monoglobus is a beneficial bacteria that modulates the metabolism (Kim et al., 2019). Unique among known human gut flora, Monoglobus plays an active role in pectin degradation and sugar utilization (Kim et al., 2019). Muribaculaceae can produce propionate, which is closely related to gut health. Related studies have reported that Muribaculaceae are closely related to acarbose consumption (Smith et al., 2021). In addition, Dorea and UCG-009 are capable of regulating health and absorbing nutrients. In the current study, the gut microbiota of Angus cattle with diarrhea was significantly altered, implying an imbalance in gut homeostasis. Our study showed that gut microbiota dysbiosis is an important factor driving the development of diarrhea. At the same time, the findings shed light on potential pathogens including Anaerosporbacter and Campylobacter, which cause diarrhea in Angus cattle.
It is well known that gut microbiota are an important indicator for evaluating gut function and homeostasis (Xia et al., 2018; Duan et al., 2020; Reese et al., 2021). However, the diversity of gut microbiota is easily affected by various factors such as species, age, and various diseases (Ding et al., 2019; Wu et al., 2020, 2022). Species are the most important factor affecting gut microbiota (Yang et al., 2018; Huang et al., 2020; Zhong et al., 2021). Animals of various species need to evolve different intestinal flora structures to adapt to their habitats, and diet characteristics, etc. (Liu et al., 2021, 2022). For example, herbivores have a higher intestinal flora structure to digest cellulose and realize energy conversion (Dong et al., 2020; Yang et al., 2022). In addition, different breeds of sheep and chickens also have different intestinal flora structures (Rettedal et al., 2019; Qin et al., 2020). Captive horses, for example, have lower gut microbiota composition and lower numbers of pathogenic bacteria than wild donkeys (Zhou et al., 2022). In addition, compared with cattle living in plain areas, yaks need to evolve a more diverse intestinal flora structure to adapt to the high-altitude hypoxic environment of the Qinghai-Tibet Plateau (Fu et al., 2021; Wang et al., 2021). Intestinal flora are executors of intestinal function and the supervisor of intestinal health, so changes in intestinal flora also affect the health of the host (Yin et al., 2019; Zhang P. et al., 2020; Chen et al., 2022).
Weining cattle are a native breed in Guizhou, while Angus cattle are an exotic breed introduced to Weining in the last 10 years. Weining cattle and Angus cattle are currently the main breeds in Weining Guizhou, occupying more than 90% of the local cattle industry. In the past 3 years, the incidence of diarrhea was less than 3% in Weining cattle. However, the rate of diarrhea in other breeds of cattle was significantly higher than 5%. Previous studies have demonstrated that the gut microbiome is correlated with species and health. In some studies, greater quantities of Firmicutes were found in the WN group compared to the An groups. Previous research has reported that Firmicutes are closely related to the health of gut microbiota (Eckburg et al., 2005), which contribute to maintaining gut microbiota balance, regulating the gut environment, and inhibiting pathogens (Sun et al., 2016). In addition, Lachnospiraceae, Rikenellaceae, and Coprostanoligenes were the most dominant genus in the WN group. Lachnospiraceae is closely linked to host health by producing short-chain fatty acids, converting primary to secondary bile acids, and inhibiting intestinal pathogens (Sorbara et al., 2020). The Rikenellaceae and Coprostanoligenes were regarded as a beneficial bacterium in modulating health and serum dyslipidemia (Tavella et al., 2021). Rikenellaceae plays an essential role in maintaining intestinal mucosal immunity, Previous studies have demonstrated that HIV infection is distinctly involved with the loss of Rikenellaceae (Dubourg et al., 2017). Similarly, in Teresa Tavella’s research, Rikenellaceae could significantly reduce visceral adipose tissue and help maintain a healthier metabolic profile, which proved that adequate Rikenellaceae could improve the body’s health and metabolism (Backhed et al., 2015; Dubin et al., 2016). Meanwhile, Wei et al. (2021) reported that Coprostanoligenes have the ability to modulate serum dyslipidemia. Overall, the highest abundance of beneficial bacteria was present in the WN group compared to the An and DA groups. In particular, the Lachnospiraceae are over-represented in Weining cattle, showing significantly higher abundances compared to Angus cattle. Interestingly, the Muribaculaceae, which plays an important role in anti-inflammatory action was also enriched in the WN group. Conversely, the potential pathogens including Alistipes and Campylobacteria were lower present in the WN group compared to the An group. Overall, the greater abundance and diversity of beneficial bacteria indicated the potential of Weining cattle for diarrhea prevention and health modulation. The data revealed that a good gut microbiome structure improves the body’s disease resistance and health status. In addition, Weining cattle have great potential as an isolated source of probiotics.
This study characterized the gut microbiota diversity and composition in Weining cattle and Angus cattle. The WN group had a greater abundance of beneficial bacteria and a lower abundance of potential pathogens. While there was no significant difference between healthy Angus and diarrheal Angus, there was a significant change in the type and proportion of bacteria. The potential pathogens including Anaerosporbacter and Campylobacter were higher in diarrheal cattle, conversely, the beneficial bacteria including Dorea, Muribaculaceae, UCG-009, and Monoglobus were significantly lower compared to healthy cattle. This is the first report of gut microbiota in Weining cattle and broadens the knowledge of gut microbiota. Our results convey the message that diarrhea not only directly modifies the diversity and abundance of gut microbiota but also indirectly affects some functional bacteria. In addition, this study revealed potentially pathogenic bacteria and provided basic data for the subsequent treatment of diarrhea in Angus cattle.
The datasets presented in this study can be found in online repositories. The names of the repository/repositories and accession number(s) can be found at: https://www.ncbi.nlm.nih.gov/, PRJNA931445.
The study was conducted under the guidance and approval of the Animal Welfare and Ethics Committee of Huazhong Agricultural University.
LW, JM, and MW provided the research idea. DW, JM, and MW contributed reagents, materials, and analysis tools. LW wrote the manuscript. KL and YZ revised the manuscript. All authors participated in writing and reviewing the manuscript, contributed to the article and approved the submitted version.
The study was supported by Research and Demonstration of Key Technology for Nutritional Control and Efficient Utilization of Roughage for Weining Cattle [Guizhou Science (2021) General project No. 153], the Weining Cattle Breeding Base of Weining County, Guizhou Province, and the Sixth Batch of Talent Base Project of Guizhou Province [Guizhou people Hair Collar (2018) No. 3].
The authors declare that the research was conducted in the absence of any commercial or financial relationships that could be construed as a potential conflict of interest.
All claims expressed in this article are solely those of the authors and do not necessarily represent those of their affiliated organizations, or those of the publisher, the editors and the reviewers. Any product that may be evaluated in this article, or claim that may be made by its manufacturer, is not guaranteed or endorsed by the publisher.
The Supplementary material for this article can be found online at: https://www.frontiersin.org/articles/10.3389/fmicb.2023.1113730/full#supplementary-material
Backhed, F., Roswall, J., Peng, Y., Feng, Q., Jia, H., Kovatcheva-Datchary, P., et al. (2015). Dynamics and stabilization of the human gut microbiome during the first year of life. Cell Host Microbe 17, 690–703. doi: 10.1016/j.chom.2015.04.004
Bendali, F., Bichet, H., Schelcher, F., and Sanaa, M. (1999). Pattern of diarrhoea in newborn beef calves in south-West France. Vet. Res. 30, 61–74.
Bjorkman, C., Svensson, C., Christensson, B., and de Verdier, K. (2003). Cryptosporidium parvum and giardia intestinalis in calf diarrhoea in Sweden. Acta Vet. Scand. 44, 145–152. doi: 10.1186/1751-0147-44-145
Bu, D., Zhang, X., Ma, L., Park, T., Wang, L., Wang, M., et al. (2020). Repeated inoculation of young calves with rumen microbiota does not significantly modulate the rumen prokaryotic microbiota consistently but decreases diarrhea. Front. Microbiol. 11:1403. doi: 10.3389/fmicb.2020.01403
Chen, A. S., Liu, D. H., Hou, H. N., Yao, J. N., Xiao, S. C., Ma, X. R., et al. (2022). Dietary pattern interfered with the impacts of pesticide exposure by regulating the bioavailability and gut microbiota. Sci. Total Environ. 858:159936. doi: 10.1016/j.scitotenv.2022.159936
Cui, M., Wang, Y., Elango, J., Wu, J., Liu, K., and Jin, Y. (2021). Cereus sinensis polysaccharide alleviates antibiotic-associated diarrhea based on modulating the gut microbiota in c57bl/6 mice. Front. Nutr. 8:751992. doi: 10.3389/fnut.2021.751992
Ding, J., An, X. L., Lassen, S. B., Wang, H. T., Zhu, D., and Ke, X. (2019). Heavy metal-induced co-selection of antibiotic resistance genes in the gut microbiota of collembolans. Sci. Total Environ. 683, 210–215. doi: 10.1016/j.scitotenv.2019.05.302
Dong, H., Liu, B., Li, A., Iqbal, M., Mehmood, K., Jamil, T., et al. (2020). Microbiome analysis reveals the attenuation effect of lactobacillus from yaks on diarrhea via modulation of gut microbiota. Front. Cell. Infect. Microbiol. 10:610781. doi: 10.3389/fcimb.2020.610781
Duan, H., Yu, L. L., Tian, F. W., Zhai, Q. X., Fan, L. P., and Chen, W. (2020). Gut microbiota: a target for heavy metal toxicity and a probiotic protective strategy. Sci. Total Environ. 742:140429. doi: 10.1016/j.scitotenv.2020.140429
Dubin, K., Callahan, M. K., Ren, B., Khanin, R., Viale, A., Ling, L., et al. (2016). Intestinal microbiome analyses identify melanoma patients at risk for checkpoint-blockade-induced colitis. Nat. Commun. 7:10391. doi: 10.1038/ncomms10391
Dubourg, G., Surenaud, M., Levy, Y., Hue, S., and Raoult, D. (2017). Microbiome of hiv-infected people. Microb. Pathog. 106, 85–93. doi: 10.1016/j.micpath.2016.05.015
Eckburg, P. B., Bik, E. M., Bernstein, C. N., Purdom, E., Dethlefsen, L., Sargent, M., et al. (2005). Diversity of the human intestinal microbial flora. Science 308, 1635–1638. doi: 10.1126/science.1110591
Eibl, C., Bexiga, R., Viora, L., Guyot, H., Felix, J., Wilms, J., et al. (2021). The antibiotic treatment of calf diarrhea in four european countries: a survey. Antibiotics (Basel) 10:910. doi: 10.3390/antibiotics10080910
Fischer, S., Bauerfeind, R., Czerny, C. P., and Neumann, S. (2016). Serum interleukin-6 as a prognostic marker in neonatal calf diarrhea. J. Dairy Sci. 99, 6563–6571. doi: 10.3168/jds.2015-10740
Fu, H., Zhang, L., Fan, C., Liu, C., Li, W., Cheng, Q., et al. (2021). Environment and host species identity shape gut microbiota diversity in sympatric herbivorous mammals. Microb. Biotechnol. 14, 1300–1315. doi: 10.1111/1751-7915.13687
Guo, Z., Pan, J., Zhu, H., and Chen, Z. Y. (2022). Metabolites of gut microbiota and possible implication in development of diabetes mellitus. J. Agric. Food Chem. 70, 5945–5960. doi: 10.1021/acs.jafc.1c07851
Han, Z., Li, K., Shahzad, M., Zhang, H., Luo, H., Qiu, G., et al. (2017). Analysis of the intestinal microbial community in healthy and diarrheal perinatal yaks by high-throughput sequencing. Microb. Pathog. 111, 60–70. doi: 10.1016/j.micpath.2017.08.025
He, K., Yan, W., Sun, C., Liu, J., Bai, R., Wang, T., et al. (2020). Alterations in the diversity and composition of gut microbiota in weaned piglets infected with balantioides coli. Vet. Parasitol. 288:109298. doi: 10.1016/j.vetpar.2020.109298
Huang, A., Cai, R., Wang, Q., Shi, L., Li, C., and Yan, H. (2019). Dynamic change of gut microbiota during porcine epidemic diarrhea virus infection in suckling piglets. Front. Microbiol. 10:322. doi: 10.3389/fmicb.2019.00322
Huang, S. M., Wu, Z. H., Li, T. T., Liu, C., Han, D. D., Tao, S. Y., et al. (2020). Perturbation of the lipid metabolism and intestinal inflammation in growing pigs with low birth weight is associated with the alterations of gut microbiota. Sci. Total Environ. 719:137382. doi: 10.1016/j.scitotenv.2020.137382
Kim, C. C., Healey, G. R., Kelly, W. J., Patchett, M. L., Jordens, Z., Tannock, G. W., et al. (2019). Genomic insights from monoglobus pectinilyticus: a pectin-degrading specialist bacterium in the human colon. ISME J. 13, 1437–1456. doi: 10.1038/s41396-019-0363-6
Li, A., Liu, B., Li, F., He, Y., Wang, L., Fakhar-e-Alam Kulyar, M., et al. (2021). Integrated bacterial and fungal diversity analysis reveals the gut microbial alterations in diarrheic giraffes. Front. Microbiol. 12:712092. doi: 10.3389/fmicb.2021.712092
Li, K., Mehmood, K., Zhang, H., Jiang, X., Shahzad, M., Dong, X., et al. (2018). Characterization of fungus microbial diversity in healthy and diarrheal yaks in gannan region of Tibet autonomous prefecture. Acta Trop. 182, 14–26. doi: 10.1016/j.actatropica.2018.02.017
Liu, C. S., Liang, X., Wei, X. H., Jin, Z., Chen, F. L., Tang, Q. F., et al. (2019). Gegen qinlian decoction treats diarrhea in piglets by modulating gut microbiota and short-chain fatty acids. Front. Microbiol. 10:825. doi: 10.3389/fmicb.2019.00825
Liu, F., Ma, R., Wang, Y., and Zhang, L. (2018). The clinical importance of campylobacter concisus and other human hosted campylobacter species. Front. Cell. Infect. Microbiol. 8:243. doi: 10.3389/fcimb.2018.00243
Liu, J., Wang, H. W., Lin, L., Miao, C. Y., Zhang, Y., and Zhou, B. H. (2019). Intestinal barrier damage involved in intestinal microflora changes in fluoride-induced mice. Chemosphere 234, 409–418. doi: 10.1016/j.chemosphere.2019.06.080
Liu, W., Wang, Q., Song, J., Xin, J., Zhang, S., Lei, Y., et al. (2021). Comparison of gut microbiota of yaks from different geographical regions. Front. Microbiol. 12:666940. doi: 10.3389/fmicb.2021.666940
Liu, J., Wang, X., Zhang, W., Kulyar, M. F., Ullah, K., Han, Z., et al. (2022). Comparative analysis of gut microbiota in healthy and diarrheic yaks. Microb. Cell Factories 21:111. doi: 10.1186/s12934-022-01836-y
Lorenz, I., Huber, R., and Trefz, F. M. (2021). A high plane of nutrition is associated with a lower risk for neonatal calf diarrhea on bavarian dairy farms. Animals (Basel) 11:3251. doi: 10.3390/ani11113251
Qin, W., Song, P., Lin, G., Huang, Y., Wang, L., Zhou, X., et al. (2020). Gut microbiota plasticity influences the adaptability of wild and domestic animals in co-inhabited areas. Front. Microbiol. 11:125. doi: 10.3389/fmicb.2020.00125
Reese, A. T., Chadaideh, K. S., Diggins, C. E., Schell, L. D., Beckel, M., Callahan, P., et al. (2021). Effects of domestication on the gut microbiota parallel those of human industrialization. elife 10:60197. doi: 10.7554/eLife.60197
Ren, S., Wang, C., Chen, A., Lv, W., and Gao, R. (2022). The probiotic Lactobacillus paracasei ameliorates diarrhea cause by escherichia coli o (8) via gut microbiota modulation (1). Front. Nutr. 9:878808. doi: 10.3389/fnut.2022.878808
Rettedal, E. A., Altermann, E., Roy, N. C., and Dalziel, J. E. (2019). The effects of unfermented and fermented cow and sheep milk on the gut microbiota. Front. Microbiol. 10:458. doi: 10.3389/fmicb.2019.00458
Shao, H., Zhang, C., Xiao, N., and Tan, Z. (2020). Gut microbiota characteristics in mice with antibiotic-associated diarrhea. BMC Microbiol. 20:313. doi: 10.1186/s12866-020-01999-x
Shin, J., Noh, J. R., Choe, D., Lee, N., Song, Y., Cho, S., et al. (2021). Ageing and rejuvenation models reveal changes in key microbial communities associated with healthy ageing. Microbiome 9:240. doi: 10.1186/s40168-021-01189-5
Singh, P., Teal, T. K., Marsh, T. L., Tiedje, J. M., Mosci, R., Jernigan, K., et al. (2015). Intestinal microbial communities associated with acute enteric infections and disease recovery. Microbiome 3:45. doi: 10.1186/s40168-015-0109-2
Smith, B. J., Miller, R. A., and Schmidt, T. M. (2021). Muribaculaceae genomes assembled from metagenomes suggest genetic drivers of differential response to acarbose treatment in mice. Msphere 6:e85121. doi: 10.1128/msphere.00851-21
Sorbara, M. T., Littmann, E. R., Fontana, E., Moody, T. U., Kohout, C. E., Gjonbalaj, M., et al. (2020). Functional and genomic variation between human-derived isolates of lachnospiraceae reveals inter-and intra-species diversity. Cell Host Microbe 28, 134–146.e4. doi: 10.1016/j.chom.2020.05.005
Sun, B., Wang, X., Bernstein, S., Huffman, M. A., Xia, D. P., Gu, Z., et al. (2016). Marked variation between winter and spring gut microbiota in free-ranging tibetan macaques (macaca thibetana). Sci. Rep. 6:26035. doi: 10.1038/srep26035
Tavella, T., Rampelli, S., Guidarelli, G., Bazzocchi, A., Gasperini, C., Pujos-Guillot, E., et al. (2021). Elevated gut microbiome abundance of christensenellaceae, porphyromonadaceae and rikenellaceae is associated with reduced visceral adipose tissue and healthier metabolic profile in italian elderly. Gut Microbes 13, 1–19. doi: 10.1080/19490976.2021.1880221
Urie, N. J., Lombard, J. E., Shivley, C. B., Kopral, C. A., Adams, A. E., Earleywine, T. J., et al. (2018). Preweaned heifer management on us dairy operations: part i. descriptive characteristics of preweaned heifer raising practices. J. Dairy Sci. 101, 9168–9184. doi: 10.3168/jds.2017-14010
Wang, Y. P., An, M., Zhang, Z., Zhang, W. Q., Kulyar, M., Iqbal, M., et al. (2022). Effects of milk replacer-based lactobacillus on growth and gut development of yaks’ calves: a gut microbiome and metabolic study. Microbiol. Spectrum. 10:e0115522. doi: 10.1128/spectrum.01155-22
Wang, Y., Fu, Y., He, Y., Kulyar, M. F., Iqbal, M., Li, K., et al. (2021). Longitudinal characterization of the gut bacterial and fungal communities in yaks. J Fungi (Basel) 7:559. doi: 10.3390/jof7070559
Wei, W., Jiang, W., Tian, Z., Wu, H., Ning, H., Yan, G., et al. (2021). Fecal g. streptococcus and g. Eubacterium_coprostanoligenes_group combined with sphingosine to modulate the serum dyslipidemia in high-fat diet mice. Clin. Nutr. 40, 4234–4245. doi: 10.1016/j.clnu.2021.01.031
Wu, N., Wang, X. B., Xu, X. Y., Cai, R. J., and Xie, S. Y. (2020). Effects of heavy metals on the bioaccumulation, excretion and gut microbiome of black soldier fly larvae (hermetia illucens). Ecotoxicol. Environ. Saf. 192:110323. doi: 10.1016/j.ecoenv.2020.110323
Wu, H. F., Zheng, L., Tan, M. T., Li, Y. N., Xu, J. S., Yan, S., et al. (2022). Cd exposure-triggered susceptibility to Bacillus thuringiensis in lymantria dispar involves in gut microbiota dysbiosis and hemolymph metabolic disorder. Ecotoxicol. Environ. Saf. 241:113763. doi: 10.1016/j.ecoenv.2022.113763
Xia, J., Jin, C., Pan, Z., Sun, L., Fu, Z., and Jin, Y. (2018). Chronic exposure to low concentrations of lead induces metabolic disorder and dysbiosis of the gut microbiota in mice. Sci. Total Environ. 631-632, 439–448. doi: 10.1016/j.scitotenv.2018.03.053
Xu, B., Qin, W., Xu, Y., Yang, W., Chen, Y., Huang, J., et al. (2021). Dietary quercetin supplementation attenuates diarrhea and intestinal damage by regulating gut microbiota in weanling piglets. Oxidative Med. Cell. Longev. 2021:6221012. doi: 10.1155/2021/6221012
Yang, X., Fan, X., Jiang, H., Zhang, Q., Basangwangdui,, Zhang, Q., et al. (2022). Simulated seasonal diets alter yak rumen microbiota structure and metabolic function. Front. Microbiol. 13:1006285. doi: 10.3389/fmicb.2022.1006285
Yang, Q., Huang, X., Zhao, S., Sun, W., Yan, Z., Wang, P., et al. (2017). Structure and function of the fecal microbiota in diarrheic neonatal piglets. Front. Microbiol. 8:502. doi: 10.3389/fmicb.2017.00502
Yang, H., Xiang, Y., Robinson, K., Wang, J. J., Zhang, G. L., Zhao, J., et al. (2018). Gut microbiota is a major contributor to adiposity in pigs. Front. Microbiol. 9:3045. doi: 10.3389/fmicb.2018.03045
Ye, J., Wu, Z., Zhao, Y., Zhang, S., Liu, W., and Su, Y. (2022). Role of gut microbiota in the pathogenesis and treatment of diabetes mullites: advanced research-based review. Front. Microbiol. 13:1029890. doi: 10.3389/fmicb.2022.1029890
Yin, N., Gao, R., Knowles, B., Wang, J., Wang, P., Sun, G., et al. (2019). Formation of silver nanoparticles by human gut microbiota. Sci. Total Environ. 651, 1489–1494. doi: 10.1016/j.scitotenv.2018.09.312
Yu, T., Guo, F., Yu, Y., Sun, T., Ma, D., Han, J., et al. (2017). Fusobacterium nucleatum promotes chemoresistance to colorectal cancer by modulating autophagy. Cells 170, 548–563.e16. doi: 10.1016/j.cell.2017.07.008
Yue, Y., He, Z., Zhou, Y., Ross, R. P., Stanton, C., Zhao, J., et al. (2020). Lactobacillus plantarum relieves diarrhea caused by enterotoxin-producing escherichia coli through inflammation modulation and gut microbiota regulation. Food Funct. 11, 10362–10374. doi: 10.1039/d0fo02670k
Yue, S. J., Liu, J., Wang, W. X., Wang, A. T., Yang, X. Y., Guan, H. S., et al. (2019). Berberine treatment-emergent mild diarrhea associated with gut microbiota dysbiosis. Biomed. Pharmacother. 116:109002. doi: 10.1016/j.biopha.2019.109002
Zhai, Q., Wang, J., Cen, S., Zhao, J., Zhang, H., Tian, F., et al. (2019). Modulation of the gut microbiota by a galactooligosaccharide protects against heavy metal lead accumulation in mice. Food Funct. 10, 3768–3781. doi: 10.1039/c9fo00587k
Zhang, L., Gu, X., Wang, J., Liao, S., Duan, Y., Li, H., et al. (2020). Effects of dietary isomaltooligosaccharide levels on the gut microbiota, immune function of sows, and the diarrhea rate of their offspring. Front. Microbiol. 11:588986. doi: 10.3389/fmicb.2020.588986
Zhang, Y. J., Li, S., Gan, R. Y., Zhou, T., Xu, D. P., and Li, H. B. (2015). Impacts of gut bacteria on human health and diseases. Int. J. Mol. Sci. 16, 7493–7519. doi: 10.3390/ijms16047493
Zhang, Y., Liu, H., Yue, Z., Tan, P., Sun, M., Ji, L., et al. (2022). Wickerhamomyces anomalus relieves weaning diarrhea via improving gut microbiota and redox homeostasis using a piglet model. Food Funct. 13, 11223–11235. doi: 10.1039/d2fo01861f
Zhang, P., Lu, G., Liu, J., Yan, Z., and Wang, Y. (2020). Toxicological responses of carassius auratus induced by benzophenone-3 exposure and the association with alteration of gut microbiota. Sci. Total Environ. 747:141255. doi: 10.1016/j.scitotenv.2020.141255
Zhong, Y., Cao, J., Deng, Z., Ma, Y., Liu, J., and Wang, H. (2021). Effect of fiber and fecal microbiota transplantation donor on recipient mice gut microbiota. Front. Microbiol. 12:757372. doi: 10.3389/fmicb.2021.757372
Zhou, Z., Tang, L., Yan, L., Jia, H., Xiong, Y., Shang, J., et al. (2022). Wild and captive environments drive the convergence of gut microbiota and impact health in threatened equids. Front. Microbiol. 13:832410. doi: 10.3389/fmicb.2022.832410
Keywords: Weining cattle, Angus cattle, diarrhea, gut microbiota, 16S
Citation: Wang L, Wu D, Zhang Y, Li K, Wang M and Ma J (2023) Dynamic distribution of gut microbiota in cattle at different breeds and health states. Front. Microbiol. 14:1113730. doi: 10.3389/fmicb.2023.1113730
Received: 01 December 2022; Accepted: 23 January 2023;
Published: 16 February 2023.
Edited by:
Tang Zhaoxin, South China Agricultural University, ChinaReviewed by:
Zeeshan Ahmad Bhutta, Chungbuk National University, Republic of KoreaCopyright © 2023 Wang, Wu, Zhang, Li, Wang and Ma. This is an open-access article distributed under the terms of the Creative Commons Attribution License (CC BY). The use, distribution or reproduction in other forums is permitted, provided the original author(s) and the copyright owner(s) are credited and that the original publication in this journal is cited, in accordance with accepted academic practice. No use, distribution or reproduction is permitted which does not comply with these terms.
*Correspondence: Mingjin Wang, ✉ d2FuZ21qMTk2N0AxNjMuY29t; Jinping Ma, ✉ d3VkYW95aTEyMzMyMUAxNjMuY29t
Disclaimer: All claims expressed in this article are solely those of the authors and do not necessarily represent those of their affiliated organizations, or those of the publisher, the editors and the reviewers. Any product that may be evaluated in this article or claim that may be made by its manufacturer is not guaranteed or endorsed by the publisher.
Research integrity at Frontiers
Learn more about the work of our research integrity team to safeguard the quality of each article we publish.