- 1Chengdu Institute of Organic Chemistry, Chinese Academy of Sciences, Chengdu, China
- 2Beijing Key Laboratory of Ionic Liquids Clean Process, CAS Key Laboratory of Green Process and Engineering, State Key Laboratory of Multiphase Complex Systems, Institute of Process Engineering, Chinese Academy of Sciences, Beijing, China
- 3University of Chinese Academy of Sciences, Beijing, China
- 4Innovation Academy for Green Manufacture, Chinese Academy of Sciences, Beijing, China
- 5Beijing Key Laboratory of Lignocellulosic Chemistry, Beijing Forestry University, Beijing, China
- 6Senior Department of Orthopedics, Chinese PLA Medical School, Beijing, China
Ionic liquids (ILs) have garnered increasing attention in the biomedical field due to their unique properties. Although significant research has been conducted in recent years, there is still a lack of understanding of the potential applications of ILs in the biomedical field and the underlying principles. To identify the antibacterial activity and mechanism of ILs on bacteria, we evaluated the antimicrobial potency of imidazole chloride ILs (CnMIMCl) on Staphylococcus aureus (S. aureus). The toxicity of ILs was positively correlated to the length of the imidazolidinyl side chain. We selected C12MIMCl to study the mechanism of S. aureus. Through the simultaneous change in the internal and external parts of S. aureus, C12MIMCl caused the death of the bacteria. The production of large amounts of reactive oxygen species (ROS) within the internal parts stimulated oxidative stress, inhibited bacterial metabolism, and led to bacterial death. The external cell membrane could be destroyed, causing the cytoplasm to flow out and the whole cell to be fragmented. The antibacterial effect of C12MIMCl on skin abscesses was further verified in vivo in mice.
1. Introduction
In recent years, diseases caused by bacteria have plagued human beings. Although antibiotics have alleviated this problem, excessive use also causes problems such as bacterial resistance. Considering the overuse of antibiotics and the increasing resistance to antibacterial agents, there is an urgent need to develop tunable antibacterial compounds and drug delivery systems to treat high-mortality diseases. Moreover, the COVID-19 pandemic has garnered more attention than ever regarding the need for more effective medical methods to diagnose and cure the disease. New, high-performing antimicrobial and antiviral materials have to be developed. As a combination of salt and organics, ionic liquids (ILs) have been endowed with abundant and diverse properties (Zhang et al., 2014), including low volatility, a wide electrochemical window, a highly tunable structure, and thermal stability, which have been widely used as solvents (Liang et al., 2019; Shamshina and Berton, 2020), extractants (Gao et al., 2020; Wang et al., 2022), catalysts (Li et al., 2021; Sadjadi, 2021; Yuan et al., 2023), electrolytes (Fu et al., 2022), and so on. ILs could be self-assembled into low-dimensional nanoparticles due to their strong interactions between the cations and anions, H-bond direction, and large molecular volume (Dupont, 2011; Chen et al., 2014). Their unique properties have attracted increasing attention in the biomedical field, such as the extraction and conservation of proteins (Schröder, 2017; Veríssimo et al., 2021; Xue et al., 2022) and nuclear acids (Dinis et al., 2020), drug transport enhancers (Md Moshikur et al., 2020; Zhang et al., 2020), drug carriers (Lu et al., 2020; Moshikur et al., 2021), drug additives (Tang et al., 2016), and disease diagnosis (Zhu et al., 2020) and therapy (Albadawi et al., 2021; Gao et al., 2021). The nanoparticle structure of ILs provides a variety of possibilities for biomedical applications. Staphylococcus aureus is a common foodborne pathogenic gram-positive bacterium which can cause a variety of serious infections (e.g., pneumonia, enteritis, pericarditis, and sepsis) in humans and animals through the skin and food (Shangguan et al., 2015; Nithya and Sundrarajan, 2020). In the treatment process, S. aureus has strong resistance and low susceptibility to drugs; thus, it is urgent to develop new ingredients to counteract the problem of drug resistance (Hess et al., 2005). In this study, S. aureus was selected to study the toxicity and mechanism of ILs on the bacterium.
Although ILs have been extensively studied in various directions for biomedical applications (Egorova et al., 2017), they currently remain in the preliminary stage due to a lack of systematic research and theoretical basis. In addition, there is little relevant research on ILs as an agent in vivo. An exciting avenue for research is to determine whether ILs can be used in developing antibacterial drugs due to their wide variety, adjustable structure, excellent properties, and the mechanism by which they interact with bacteria. Florio et al. (2019) compared and evaluated the antimicrobial efficacy of 15 ILs, including 1-methyl-3-dodecylimidazolium bromide, 1-dodecyl-1-methylpyrrolidinium bromide, and 1-dodecyl-1-methylpiperidinium bromide, which had strong inhibitory effects on the biofilm formation of S. aureus or Pseudomonas aeruginosa (P. aeruginosa) (Florio et al., 2019). Brunel et al. (2016) found that triphenylamine phosphonium ILs could self-assemble into nanoparticles, which have a good antibacterial effect on S. aureus. The IL nanoparticles were likely to strongly affect bacterial metabolism (Brunel et al., 2016). Although some researchers have speculated on the mechanism by which ILs interact with S. aureus, it remains unknown how ILs affect bacterial membranes and influence bacterial metabolism. Further studies are needed to better understand the mechanisms involved in antibacterial activity.
Because imidazole chloride ILs are widely available and studied in a variety of fields, a series of CnMIMCl were chosen to investigate toxicity mechanisms on bacteria and gain additional insight into the effects of IL structure on antibacterial properties. This study explored the toxicity and mechanism of ILs in gram-positive bacteria (S. aureus) and designed and developed ILs for infection prevention and control. A skin abscess model was established to demonstrate the antimicrobial efficacy of ILs in vivo.
2. Materials and methods
2.1. Strains, culture conditions, and ILs
The S. aureus BNCC 186335 and USA300-sfgfp strains were purchased from Forhigh Biotech. The S. aureus strain was cultivated in LB medium (18 g/L Nutrient Broth (NB), Solarbio) for 24 h at 37°C and 180 r/min in a shaker incubator. A microplate reader (Tecan, Infinite M2000) was used to measure OD600. ILs (C2MIMCl, C3MIMCl, C4MIMCl, C6MIMCl, C8MIMCl, C12MIMCl, C16MIMCl, ≥99%) were prepared by Shanghai Cheng Jie Chemical Co., Ltd. Then, the ILs were diluted in sterile, distilled water. Cy5 NHS Ester (Cy5, SE) was purchased from Beijing Fanbo Biochemicals Co., Ltd. Then, 4% of the paraformaldehyde fix solution was purchased from Solarbio.
2.2. Antibacterial assay
Iconic liquids were diluted in sterile, distilled water following the corresponding concentration gradient. Then, 50 μL of bacteria solutions and different ILs were added to 5 ml of LB medium. Afterward, mixed solutions were hatched at 37°C and 180 r/min in a shaker incubator for 24 h. Before measuring OD600, the solution was fully shaken to distribute S. aureus and ensure the accuracy of the measurement. Finally, 100 μL of solutions were removed from each sample and placed into 96-well plates to measure OD600. To avoid human or machine error, every OD600 value was repeated at least three times. EC50s were determined from OD600 by GraphPad Prism8. The activity of S. aureus cultured with ILs was observed by a confocal microscope, and the fluorescence was quantified.
Then, 200 μL of IL was added to 10 mL of solid LB medium (18 g/L NB, 1L water, and 15 g agarose), which was melted to liquid in advance and put in 10-cm plates. Then, 100 μL of bacterial suspension (OD600 = 0.15) with further dilution was spread on solid LB plates and cultured at 37°C for 48 h. The CFUs were counted after being cultured, and the cultures were photographed with a camera.
2.3. Oxidative stress in S. aureus
C3MIMCl or C12MIMCl was added to S. aureus (OD600 = 0.15) and mixed with the ROS Assay Kit (DCFH-DA), and the mixed solution was placed in a 96-well plate for 30 min at 37oC. Then, the ROS value was detected with the microplate reader at the excitation wavelength of 488 nm and the emission wavelength of 525 nm. Following the above experimental procedure, 200 μL of the mixture was added to a confocal dish, and fluorescence was photographed using confocal laser scanning microscopy (Nikon Corporation, A1, CLSM) at the excitation wavelength of 488 nm with green as the pseudo color.
2.4. Surface morphology characterization and status observation of S. aureus
Staphylococcus aureus (OD600 = 0.15) was cultured in a liquid LB medium with ILs within 3 h at 37°C. Then, the bacteria were washed with PBS three times and fixed in 4% of the paraformaldehyde fix solution at 4°C overnight. The next day, bacteria were washed with PBS three times and treated with ethanol gradient dehydration at different concentrations (30, 40, 50, 60, 70, 80, 90, 95, and 100%). Bacteria were successfully mixed with different ethanol solution concentrations, were left to stand, were dehydrated for 15 min, and were collected by centrifugation. Finally, the bacteria dehydrated with 100% ethanol onto the tin foil was dropped and dried at 37°C for 2 h. The morphological changes of S. aureus treated with ILs were characterized by scanning electron microscopy (SEM, HITACHI, SU8020).
S. aureus was cultured with ILs, centrifuged (8000 r/min, 8 min), washed with water (3 times), and fixed with glutaraldehyde solution (PH = 7.4) overnight. They were taken in cross-sections and stained for further transmission electron microscopy (TEM, HITACHI, H-7650B) observation.
2.5. Measurement of S. aureus surface zeta potential
S. aureus (OD600 = 0.25) was treated with C3MIMCl and C12MIMCl (the final concentrations: 0.01 mM, 0.04 mM, and 0.16 mM) in liquid LB medium at 37°C for 3 h with gentle shaking. Then, S. aureus was washed with PBS three times and sterilized with water one time a day. The obtained bacteria were dispersed in 1 mL of water. The uniform suspension was measured for zeta potential using a Zetasizer Nano ZS (Malvern Instruments Ltd., Zetasizer Nano ZS90). As a control group, the bacteria were treated with an LB medium containing corresponding volumes of water at the same conditions. At the same time, we used Cy5, SE labeled C12MIMCl to interact with S. aureus and CLSM to observe the interaction between IL and bacteria.
2.6. Mice
BALB/c mice (6–8 weeks old, female) were obtained from Vital River Laboratories (Beijing, China). The Institutional Animal Care and Use Committees approved the animal protocol of the Institute of Process Engineering, Chinese Academy of Sciences (approval ID: IPEAECA 2022103). This study was performed in strict accordance with the Regulations for the Care and Use of Laboratory Animals and the Guideline for the Ethical Review of Animals.
2.7. Skin abscess model and antibacterial activity of ionic liquid in vivo
For the skin abscess model, mice were divided into three groups (controls, infected group, and ILs). Mice were anesthetized with pentobarbital sodium (1%) and inoculated with 100 μl of PBS containing 1013 cfu/L S. aureus or sterile PBS in the right flank by subcutaneous injection. After abscess formation, 50 μl of C12MIMCl (2 mM) was injected daily at the abscess site for 3–4 days. We observed the abscess healing continuously. Skin biopsy samples collected on day 11 were fixed and prepared for histopathological evaluation. The tissues were stained with hematoxylin and eosin (H&E) and 4′,6-diamidino-2-phenylindole (DAPI) and visualized by the scanner (3DHISTECH, P250 FLASH).
3. Results and discussion
3.1. Antibacterial activities of CnMIMCl against S. aureus
The antibacterial activities of a series of imidazole chloride ILs against S. aureus were determined. To evaluate different ILs' antimicrobial activity against S. aureus, different concentrations of six ILs were used to measure EC50 (Figure 1A). The EC50 values in the LB medium of C2MIMCl, C3MIMCl, C4MIMCl, C6MIMCl, C8MIMCl, C12MIMCl, C16MIMCl were 76.7, 28.7, 18.2, 3.5, 0.3, 1.9 x 10−3, and 0.5 x 10−3 mM for S. aureus, respectively. Imidazolium chloride ILs with substituents of 12 and 16 carbon lengths had strong antibacterial effects on S. aureus. The toxicity of S. aureus increased with the length of the cation side chain. It may be due to the increased hydrophobicity. With an increase in CnMIMCl concentration, the inhibition rate of S. aureus increased. For example, when the concentration of C12MIMCl was 0.0015, 0.0020, 0.0025, and 0.0030 mM, the inhibition rates of S. aureus were 23.2, 60.5, 83.1, and 87.7%, respectively (Figure 1B). It showed that the toxicity of CnMIMCl varied for each concentration. S. aureus transfected with a green fluorescent protein (GFP) was incubated with C3MIMCl or C12MIMCl and observed by CLSM. There was no difference in GFP fluorescence intensity between the C3MIMCl group and the control group, while S. aureus GFP fluorescence intensity in the C12MIMCl group was significantly reduced (Figures 1C, D).
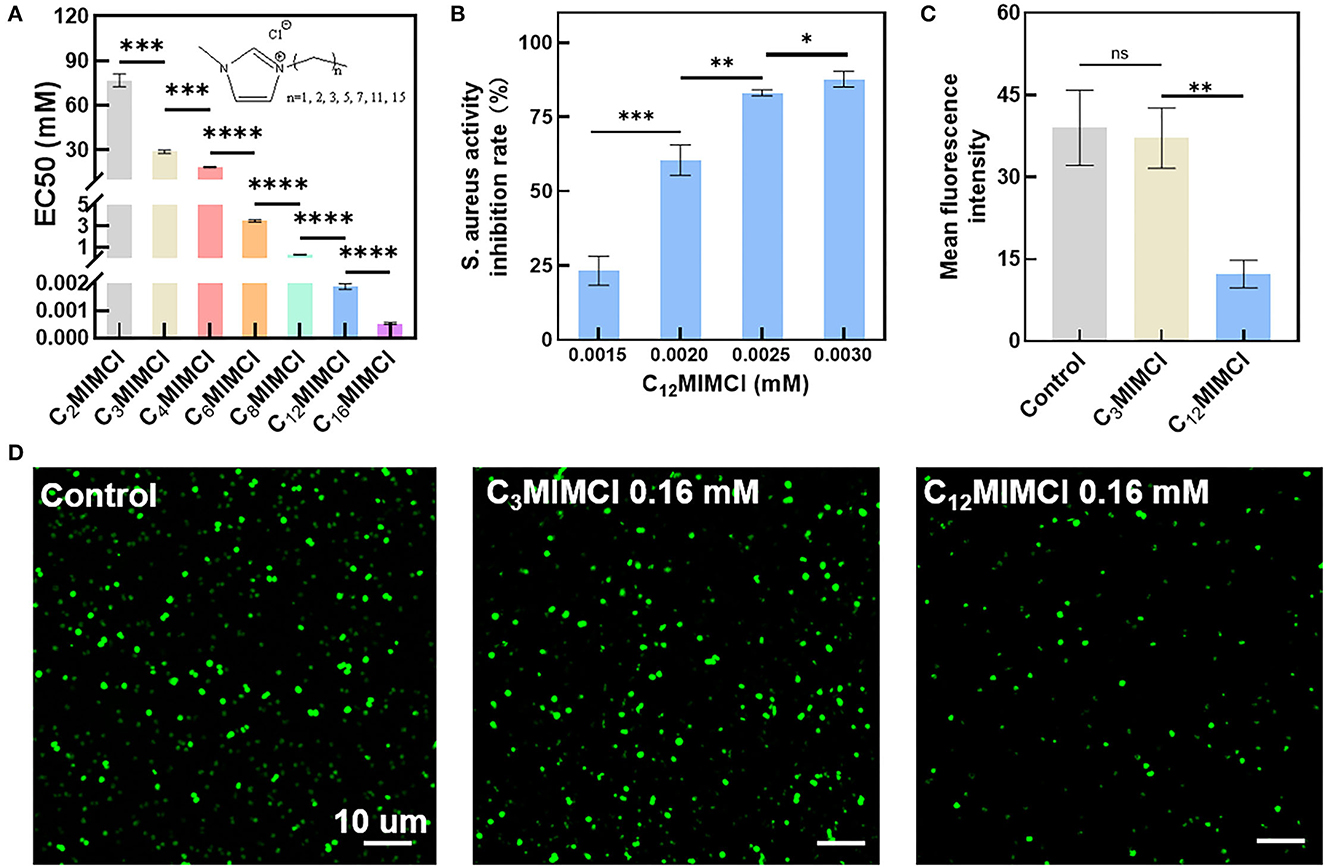
Figure 1. Toxicity test of CnMIMCl against Staphylococcus aureus. (A) EC50 values of Staphylococcus aureus incubated with CnMIMCl for 24 h. (B) Effects of C12MIMCl on Staphylococcus aureus at different concentrations. (C) Quantitative analysis of bacterial activity. (D) CLSM images of bacterial activity. Data in (A–C) represent the mean ± s.d. Statistical significance was calculated via two-tailed unpaired Student's t-test (A–C). Ns means no significant difference, *P<0.05, **P < 0.01, ***P < 0.001, ****P < 0.0001.
To further verify the toxicity of CnMIMCl, the less toxic C3MIMCl and the more toxic C12MIMCl were selected for plate coating experiments (Figure 2). Compared with the control group, there was no bacterial inhibition when the concentration of C3MIMCl was 0.0040 mM. The inhibition rates were 65.8 ± 0.8%, 89.1 ± 5.1%, and 94.6 ± 4.7% when the C3MIMCl concentration was 26 mM, 32 mM, and 40 mM, respectively. C12MIMCl showed a significant inhibitory effect on S. aureus compared with C3MIMCl. When the concentrations of C12MIMCl were 0.0026 mM, 0.0032 mM, and 0.0040 mM, the inhibition rates were 9.7 ± 3.9%, 23.0 ± 5.1%, and 98.4 ± 0.8%, respectively. With ~95% inhibition of S. aureus, the concentration used for C3MIMCl is about 104 times higher than that used for C12MIMCl.
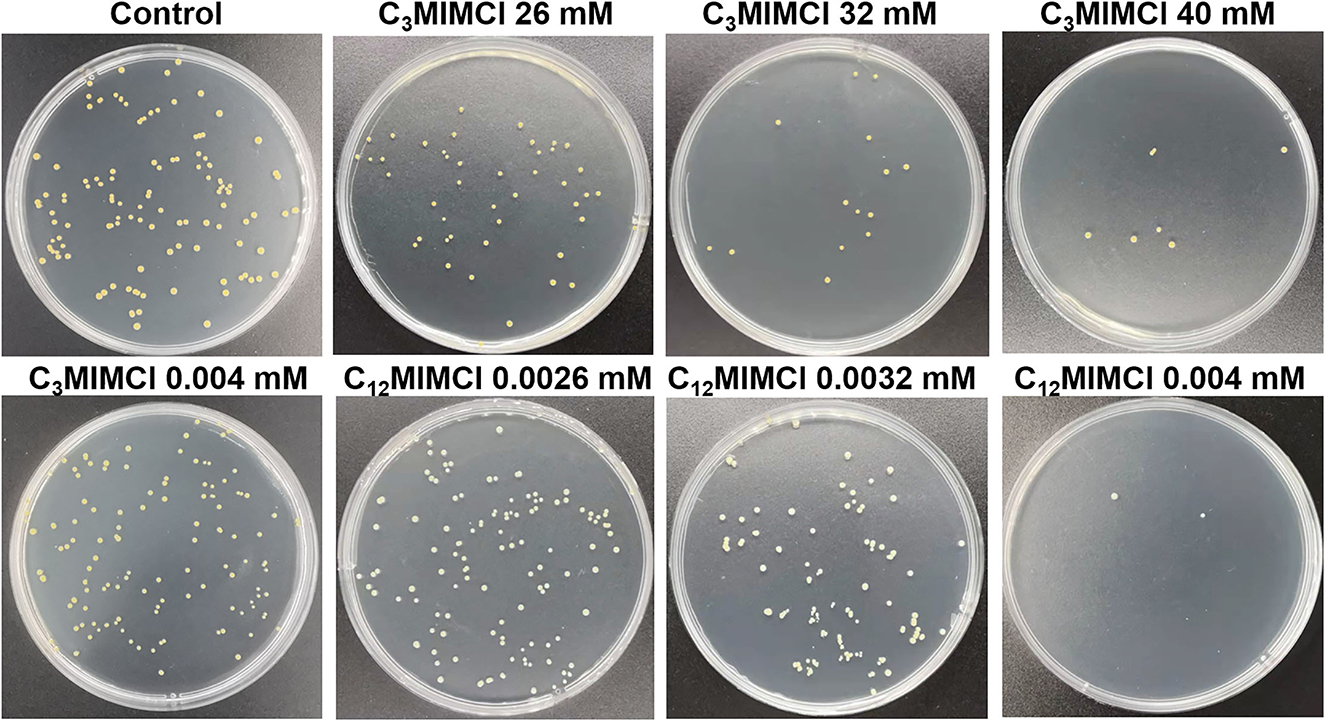
Figure 2. Bacterial colony photos of Staphylococcus aureus incubated with various concentrations of C3MIMCl and C12MIMCl for 48 h.
The toxicity of CnMIMCl to S. aureus was proportional to the length of the imidazolidinyl side chain. At the same time, the longer the alkyl side chain length of the imidazole, the greater the hydrophobicity of CnMIMCl. Therefore, the toxicity of CnMIMCl to S. aureus increased with the enhancement of hydrophobicity. It is well known that phospholipid is one of the main components of the cell membrane, and the outer surface of the cell membrane is electronegative. The ILs were easily adsorbed to the bacterial surface due to the positive charge of the cationic part, and then, the ILs interacted with the phospholipid bilayer due to hydrophobicity. Therefore, C3MIMCl with a short carbon chain and C12MIMCl with a long carbon chain were selected for the subsequent research.
3.2. Oxidative stress of S. aureus by C12MIMCl
To further investigate the mechanisms leading to the inhibitory and destructive effects of C12MIMCl on S. aureus, as well as the significant reduction of the viability of the exposed bacteria induced by ILs, the release of reactive oxygen species (ROS) within S. aureus was explored. ROS is a general term for a class of molecules with oxidative activity produced by cells during energy metabolism under aerobic conditions. In bacteria, excess ROS can damage nucleic acids, proteins, and lipids (Brynildsen et al., 2013). Moreover, it can also lead to bacterial oxidative stress and inhibit bacterial metabolism (Ning et al., 2019; Zhao et al., 2022). Bacterial death occurs when intracellular ROS exceeds the cell's ability to detoxify and repair. Therefore, it is necessary to determine the amount of ROS in bacteria to reveal the mechanism of IL acting on bacteria. Figure 3 shows CLSM images of ROS (green). No significant changes in ROS fluorescence values were observed with 0.16 mM C3MIMCl compared with the control group. It was found that C12MIMCl could significantly increase ROS in bacteria compared with C3MIMCl at the same concentration, and the ROS gradually increased with an increase in C12MIMCl concentration. Therefore, C12MIMCl could induce ROS release intracellularly.
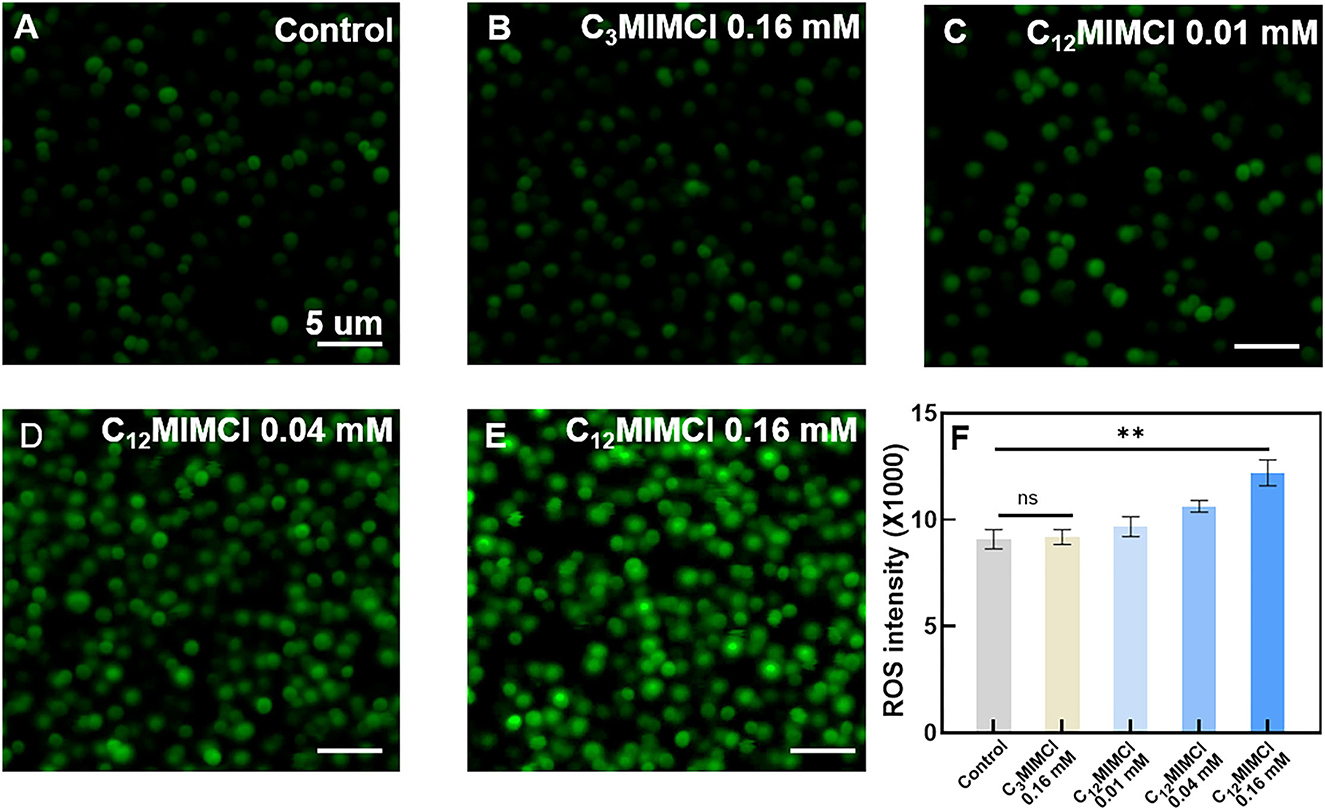
Figure 3. Oxidative stress of Staphylococcus aureus. (A) CLSM images of ROS release from bacteria in control. (B–E) CLSM images of ROS release from bacteria in the presence of 0.16 mM C3MIMCl (B), 0.01 mM C12MIMCl (C), 0.04 mM C12MIMCl (D), 0.16 mM C12MIMCl (E). (F) Quantitative analysis of ROS release by a microplate reader. Data in (F) represent the mean ± s.d. Statistical significance of (F) was calculated via two-tailed unpaired Student's t-test. Ns means no significant difference, **P < 0.01.
3.3. Damage to the S. aureus membrane by C12MIMCl
3.3.1. Surface morphology observation of S. aureus
SEM images were utilized to observe the morphological changes of S. aureus after incubation with ILs for 3 h. It could be seen that the normal form of S. aureus strains displayed regular and clear edges, smooth surfaces with rounded projections, and complete cell walls. To further investigate the antibacterial activities of short and long cation side chains, the damage of C3MIMCl and C12MIMCl on the bacterial membrane of S. aureus was evaluated (Figure 4). Furthermore, similar to their control counterparts, the morphologies of the bacteria treated with 0.16 mM C3MIMCl for 3 h remained intact and smooth. However, the morphology of S. aureus treated with C12MIMCl was collapsed by depression or wrinkling on the surface, indicating that partial or complete membrane lysis might occur. As the concentration of C12MIMCl increased, a greater effect on the surface morphology of S. aureus could be observed. This result indicated that C12MIMCl could damage the membranes of S. aureus.
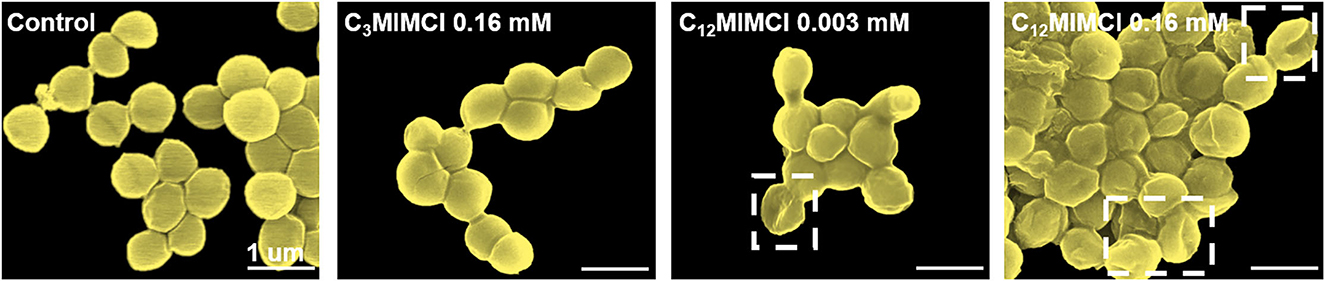
Figure 4. SEM characterization of Staphylococcus aureus morphologies and membrane integrities after 3h co-culture with water (control group), 0.16 mM C3MIMCl or different concentrations of C12MIMCl (0.003 mM and 0.16 mM).
3.3.2. Changes on the S. aureus surface
Bacterial membrane potential is the proton motive force of bacterial metabolism and respiration. Antibacterial activity strongly correlates with the dissipation of the membrane potential in bacteria (Schilling et al., 2019). When studying the antibacterial mechanisms, the integrity of bacterial membranes is a non-negligible factor. In our study, when the concentration of C12MIMCl was 0.16 mM, C12MIMCl increased the bacterial membrane potential to 16.3%, and the change in membrane potential increased with an increase in ILs concentration. Compared with C3MIMCl, S. aureus showed an increase in zeta potentials after co-culturing with C12MIMCl (0.16 mM) (Figure 5A), indicating that the positively charged C12MIMCl underwent electrostatic interactions with the negatively charged components of the S. aureus membrane. With an increase in C12MIMCl concentration, the change in S. aureus membrane potential increased. The potential of S. aureus is in full correlation with their EC50 values and is concentration dependent. It could be seen that the bacterial color changed after incubation with Cy5, SE-C12MIMCl, indicating that C12MIMCl was adsorbed on the bacterial surface (Figure 5B). Because the S. aureus surface was negatively charged, it could attract the IL cation with a positive charge to gather on the bacterial surface by CLSM (Figures 5C–E). It was once again demonstrated that C12MIMCl could interact with bacterial cell membranes.
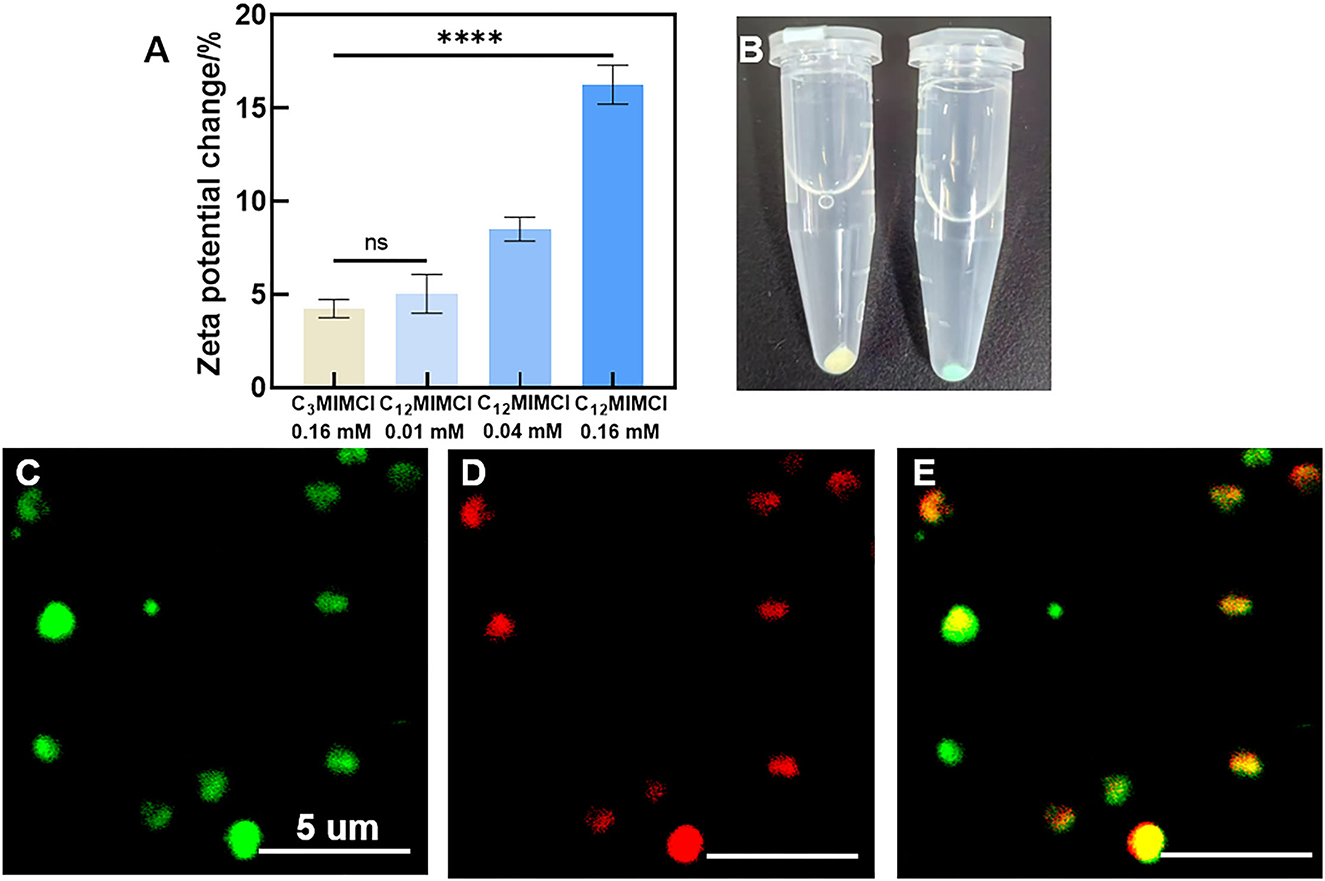
Figure 5. The changes of Staphylococcus aureus surface. (A) Zeta potentials of Staphylococcus aureus co-cultured with C3MIMCl or C12MIMCl in PBS for 3h. (B) Picture of Staphylococcus aureus incubated with Cy5, SE-C12MIMCl. (C–E) CLSM images of C12MIMCl interacting with Staphylococcus aureus. Green: Staphylococcus aureus transfected with GFP (C), Red: Cy5, SE-C12MIMCl (D), merged (E). Data in (A) represent the mean ± s.d. Statistical significance of (A) was calculated via two-tailed unpaired Student's t-test. Ns means no significant difference, ****P < 0.0001.
3.3.3. Observation of the destruction process of S. aureus by ILs
TEM images further confirmed that S. aureus was treated with C12MIMCl, which showed membrane detachment, content leakage, and cell disruption. Figure 6 shows the whole process of S. aureus being destroyed. The control group and C3MIMCl group showed intact and smooth cell membranes. Initially, part of the cell membrane was shed from the bacterial surface in the presence of C12MIMCl (Figure 6A). Subsequently, the cytoplasm flowed out from the broken cell membrane (Figure 6B). Then, the cell membrane of S. aureus was completely disrupted (Figure 6C). Finally, S. aureus was dead (Figure 6D).

Figure 6. TEM images of Staphylococcus aureus within 3 h treatment with 0.16 mM of C12MIMCl. (A-D): The destruction process of Staphylococcus aureus by C12MIMCl.
3.4. Antibacterial mechanism of ionic liquids against S. aureus
The cell membrane mainly consists of phospholipids with a negative charge on the surface and hydrophobicity. ILs are amphiphilic, and long cationic side chains can be inserted into the phospholipid bilayer, causing cell membrane disorder (Kaur et al., 2020; Liu et al., 2021). According to the above experimental contents, C12MIMCl could be adsorbed on the surface of S. aureus by electrostatic action. Afterward, C12MIMCl was inserted into cell membranes driven by hydrophobicity. This led to an oxidative stress reaction in bacteria, that is, the rapid production of a large amount of ROS. At the same time, it changed the permeability of the bacterial cell membrane, which could change the bacterial osmotic pressure, destroying the cell membrane and allowing cytoplasm to flow out of the broken cell membrane. Figure 7 depicts a plausible antibacterial mechanism for interacting C12MIMCl with S. aureus.
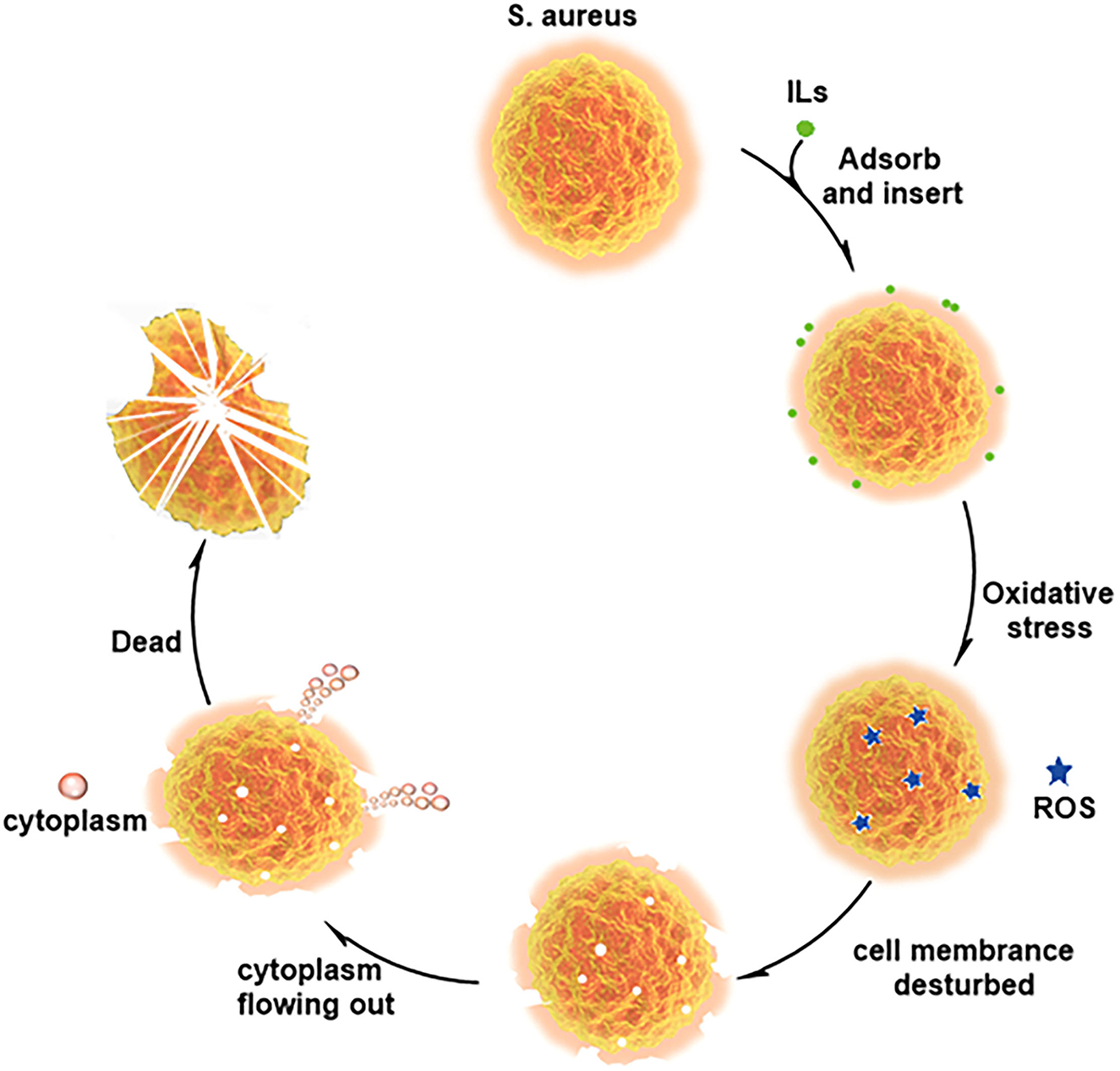
Figure 7. Plausible antibacterial mechanism for interaction between C12MIMCl and Staphylococcus aureus.
3.5. The therapeutic effect of C12MIMCl on skin abscess in vivo
3.5.1. Observation of a skin abscess
The skin of S. aureus-infected mice developed visible abscesses, redness, edema, and ulcers within 1–2 days of infection. The abscess was injected with 2 mM C12MIMCl (50 μl) for 3–4 days. On the second day of C12MIMCl administration, the abscess showed a distinct black scab, and the abscess under the skin gradually decreased, while the abscess in the control group gradually grew larger (Figure 8). In the following days, the scabbed area gradually expanded. The subcutaneous abscess did not disappear completely until 3–4 days after the C12MIMCl injection. After 8 days, the scab gradually fell off. The skin in the treatment group could be healed for 10–12 days. However, the subcutaneous abscess remained in the control group.
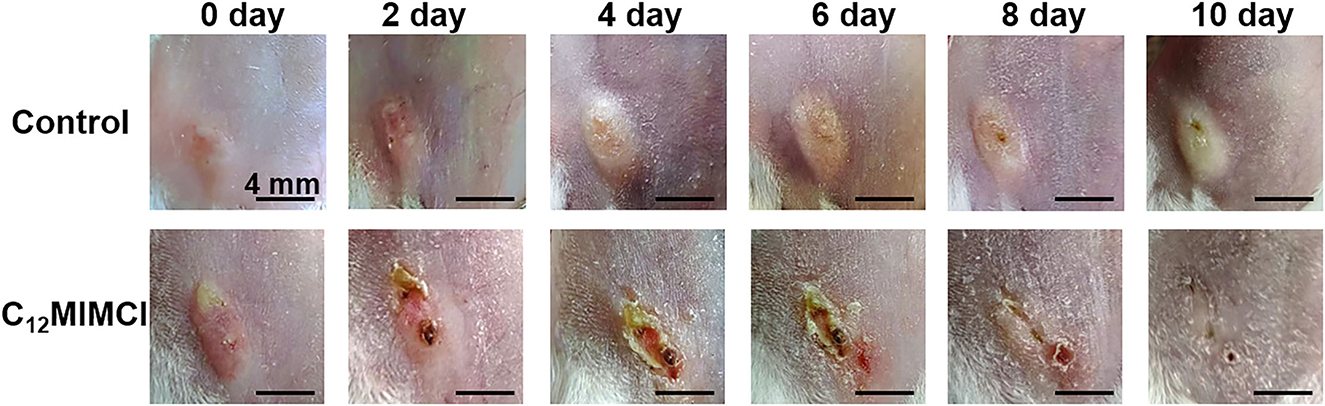
Figure 8. Changes of skin abscess in mice after Staphylococcus aureus infection and C12MIMCl treatment.
3.5.2. Analysis of skin tissue
Skin biopsy samples collected on day 11 were fixed and prepared for histopathological evaluation (Figures 9A–C). Compared with the control group, the wavy curves of the epidermis and dermis at the subcutaneous abscess sites of S. aureus infection disappeared, indicating that the epidermis and dermis were severely damaged. In addition, there was a large infiltration of monocytes in the dermis. After the C12MIMCl injection, the morphology of the epidermis and dermis gradually recovered, and the number of inflammatory cells decreased significantly. H&E staining of skin sections from the mice revealed that C12MIMCl had a good therapeutic effect on the subcutaneous abscess. After DAPI staining and GFP spontaneous fluorescence scanning, it could be seen that the number of S. aureus in the skin was nearly zero after the C12MIMCl injection. The number of S. aureus cells in the skin remained largely unchanged in the untreated group (Figures 9D–F). In conclusion, C12MIMCl could improve the skin abscess infected with S. aureus in mice.
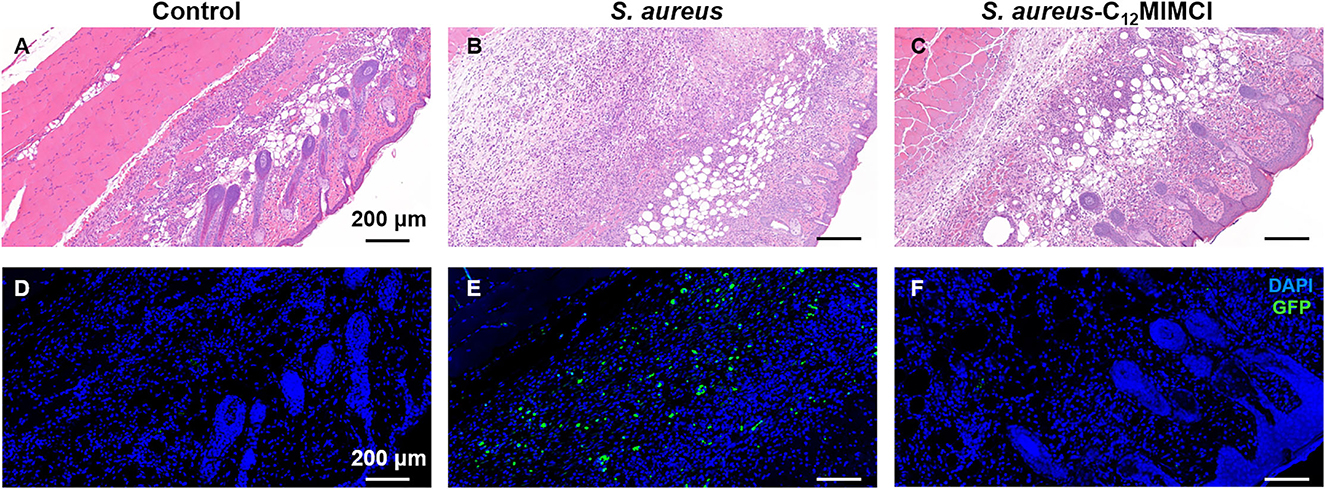
Figure 9. Histopathology of skin abscess and fluorescent scan in mice after Staphylococcus aureus infection and C12MIMCl treatment. (A) Histopathology of the control group (PBS). (B) Histopathology of skin abscess after Staphylococcus aureus injection. (C) Histopathology of C12MIMCl treatment in skin abscess. (D) Fluorescent scan of the control group (PBS). (E) Fluorescent scan of skin abscess after Staphylococcus aureus injection. (F) Fluorescent scan of C12MIMCl treatment in skin abscess. DAPI (blue), GFP-Staphylococcus aureus (green).
4. Conclusion
A variety of imidazole chloride ILs with varying length substituents was investigated for their antibacterial mechanisms against S. aureus. Imidazole chloride ILs containing twelve and sixteen carbon lengths had strong antibacterial and anti-biofilm activity against S. aureus. The results showed that the antibacterial efficiency of ILs could be improved by changing the alkyl chain length due to the tunable structure of ILs. The above research demonstrated that long cationic side-chain ILs effectively inhibited S. aureus. This study provided new ideas for creating new antibacterial drugs. ROS release tests confirmed that long cationic side-chain ILs have a strong impact on the metabolism of S. aureus. SEM and membrane potential test experiments revealed that ILs could collapse and damage the surface of S. aureus. TEM images clearly showed how C12MIMCl disrupts the cell membrane of S. aureus, causing the contents to leak. Ultimately, the antibacterial mechanism of C12MIMCl against S. aureus was proven. In vivo tests, C12MIMCl had significant antibacterial effects and accelerated the healing of skin abscesses. After the new coronavirus pandemic in 2019, it is urgent to develop new antibacterial and antiviral drugs. ILs have not been found to be appropriate drugs for clinical use. At present, we know that the toxicity of ILs to S. aureus depends on the length of cationic alkyl chain substituents. In the future, we will expand the types of organism models (e.g., Gram-negative bacteria, viruses, cells, and animals) and design specific properties for different diseases by regulating different types of ILs substituents.
Data availability statement
The original contributions presented in the study are included in the article/supplementary material, further inquiries can be directed to the corresponding authors.
Ethics statement
The animal study was reviewed and approved by the Institutional Animal Care and Use Committees at the Institute of Process Engineering, Chinese Academy of Sciences.
Author contributions
YH, GW, and YD contributed to the conception and design of the study. YH, PY, and HY performed the experiments. YH, PY, and XM contributed significantly to data analyses. YH and YX organized the pictures of the experiment. YH wrote the manuscript. HY, YX, and YS revised the manuscript. GW and YD oversaw the completion of this study. All authors contributed to the article and approved the submitted version.
Funding
This study was supported by the National Natural Science Foundation of China (21978292 and 21676280), the Innovation Academy for Green Manufacture, the Chinese Academy of Sciences (IAGM2020C20), the Major Program of the National Natural Science Foundation of China (21890762), and the International Partnership Program of the Chinese Academy of Sciences (grant no. 122111KYSB20190060).
Acknowledgments
The authors sincerely appreciate Prof. Suojiang Zhang (IPE, CAS) for his careful academic guidance and great support.
Conflict of interest
The authors declare that the research was conducted in the absence of any commercial or financial relationships that could be construed as a potential conflict of interest.
Publisher's note
All claims expressed in this article are solely those of the authors and do not necessarily represent those of their affiliated organizations, or those of the publisher, the editors and the reviewers. Any product that may be evaluated in this article, or claim that may be made by its manufacturer, is not guaranteed or endorsed by the publisher.
References
Albadawi, H., Zhang, Z., Altun, I., Hu, J., Jamal, L., Ibsen, K. N., et al. (2021). Percutaneous liquid ablation agent for tumor treatment and drug delivery. Sci. Transl. Med. 13, eabe3889. doi: 10.1126/scitranslmed.abe3889
Brunel, F., Lautard, C., Garzino, F., Giorgio, S., Raimundo, J. M., Bolla, J. M., et al. (2016). Antibacterial activities of fluorescent nano assembled triphenylamine phosphonium ionic liquids. Bioorg. Med. Chem. Lett. 26, 3770–3773. doi: 10.1016/j.bmcl.2016.05.055
Brynildsen, M. P., Winkler, J. A., Spina, C. S., MacDonald, I. C., and Collins, J. J. (2013). Potentiating antibacterial activity by predictably enhancing endogenous microbial ROS production. Nat. Biotechnol. 31, 160–165. doi: 10.1038/nbt.2458
Chen, S. M., Zhang, S. J., Liu, X. M., Wang, J. Q., Wang, J. J., Dong, K., et al. (2014). Ionic liquid clusters: structure, formation mechanism, and effect on the behavior of ionic liquids. Phys. Chem. Chem. Phys. 16, 5893–5906. doi: 10.1039/C3CP53116C
Dinis, T. B. V., Sousa, F., and Freire, M. G. (2020). Insights on the DNA stability in aqueous solutions of ionic liquids. Front. Bioeng. Biotechnol. 8, 547857. doi: 10.3389/fbioe.2020.547857
Dupont, J. (2011). From molten salts to ionic liquids a “nano” journey. Accounts Chem. Res. 44, 1223–1231. doi: 10.1021/ar2000937
Egorova, K. S., Gordeev, E. G., and Ananikov, V. P. (2017). Biological activity of ionic liquids and their application in pharmaceutics and medicine. Chem. Rev. 117, 7132–7189. doi: 10.1021/acs.chemrev.6b00562
Florio, W., Becherini, S., D'Andrea, F., Lupetti, A., Chiappe, C., and Guazzelli, L. (2019). Comparative evaluation of antimicrobial activity of different types of ionic liquids. Mater. Sci. Eng. C 104, 109907. doi: 10.1016/j.msec.2019.109907
Fu, D., Sun, Y., Zhang, F., Sun, Z., Li, W., Wang, L., et al. (2022). Enabling polymeric ionic liquid electrolytes with high ambient ionic conductivity by polymer chain regulation. Chem. Eng. J. 431, 133278. doi: 10.1016/j.cej.2021.133278
Gao, S. R., Fang, S. F., Song, R. Z., Chen, X. C., and Yu, G. R. (2020). Extractive denitrogenation of shale oil using imidazolium ionic liquids. Green Energ. Environ. 5, 173–182. doi: 10.1016/j.gee.2020.04.002
Gao, Y. R., Cao, J. F., Shu, Y., and Wang, J. H. (2021). Research progress of ionic liquids-based gels in energy storage, sensors and antibacterial. Green Chem. Eng. 2, 368–383. doi: 10.1016/j.gce.2021.07.012
Hess, D. J., Garni, R. M., Henry-Stanley, M. J., and Wells, C. L. (2005). Escherichia colimodulates extraintestinal spread of Staphylococcus aureus. Shock 24, 376–381. doi: 10.1097/01.shk.0000180615.75822.fe
Kaur, N., Fischer, M., Kumar, S., Gahlay, G. K., Scheidt, H. A., and Mithu, V. S. (2020). Role of cationic head-group in cytotoxicity of ionic liquids: probing changes in bilayer architecture using solid-state NMR spectroscopy. J. Colloid. Interface Sci. 581, 954–963. doi: 10.1016/j.jcis.2020.08.115
Li, M., Chen, J., Li, L., Ye, C., Lin, X., and Qiu, T. (2021). Novel multi–SO3H functionalized ionic liquids as highly efficient catalyst for synthesis of biodiesel. Green Energ. Environ. 6, 271–282. doi: 10.1016/j.gee.2020.05.004
Liang, L., Yan, J. P., He, Q., Luong, T., Pray, T. R., Simmons, B. A., et al. (2019). Scale-up of biomass conversion using 1-ethyl-3-methylimidazolium acetate as the solvent. Green Energ. Environ. 4, 432–438. doi: 10.1016/j.gee.2018.07.002
Liu, J., Wang, Y., Wang, C., Gao, J., Cui, W., Zhao, B., et al. (2021). Thermodynamical origin of nonmonotonic inserting behavior of imidazole ionic liquids into the lipid bilayer. J. Phys. Chem. Lett. 12, 9926–9932. doi: 10.1021/acs.jpclett.1c02566
Lu, B. B., Zhou, G. X., Xiao, F., He, Q. J., and Zhang, J. H. (2020). Stimuli-responsive poly(ionic liquid) nanoparticles for controlled drug delivery. J. Mater. Chem. B 8, 7994–8001. doi: 10.1039/D0TB01352H
Md Moshikur, R., Chowdhury, M. R., Fujisawa, H., Wakabayashi, R., Moniruzzaman, M., and Goto, M. (2020). Design and characterization of fatty acid-based amino acid ester as a new “green” hydrophobic ionic liquid for drug delivery. ACS Sustain. Chem. Eng. 8, 13660–13671. doi: 10.1021/acssuschemeng.0c03419
Moshikur, R. M., Ali, M. K., Moniruzzaman, M., and Goto, M. (2021). Recent advances in surface-active ionic liquid-assisted self-assembly systems for drug delivery. Curr. Opin. Colloid In. 56, 101515. doi: 10.1016/j.cocis.2021.101515
Ning, H.-Q., Li, Y.-Q., Tian, Q.-W., Wang, Z.-S., and Mo, H.-Z. (2019). The apoptosis of Staphylococcus aureus induced by glycinin basic peptide through ROS oxidative stress response. LWT-Food Sci. Technol. 99, 62–68. doi: 10.1016/j.lwt.2018.09.028
Nithya, P., and Sundrarajan, M. (2020). Ionic liquid functionalized biogenic synthesis of Ag-Au bimetal doped CeO2 nanoparticles from Justicia Adhatoda for pharmaceutical applications: antibacterial and anti-cancer activities. J. Photochem. Photobiol. B 202, 111706. doi: 10.1016/j.jphotobiol.2019.111706
Sadjadi, S. (2021). Magnetic (poly) ionic liquids: a promising platform for green chemistry. J. Mol. Liq. 323, 114994. doi: 10.1016/j.molliq.2020.114994
Schilling, N. A., Berscheid, A., Schumacher, J., Saur, J. S., Konnerth, M. C., Wirtz, S. N., et al. (2019). Synthetic lugdunin analogues reveal essential structural motifs for antimicrobial action and proton translocation capability. Angew. Chem. Int. Ed. Engl. 58, 9234–9238. doi: 10.1002/anie.201901589
Schröder, C. (2017). Proteins in ionic liquids: current status of experiments and simulations. Top. Curr. Chem. 375, 25. doi: 10.1007/s41061-017-0110-2
Shamshina, J. L., and Berton, P. (2020). Use of ionic liquids in chitin biorefinery: a systematic review. Front. Bioeng. Biotechnol. 8, 11. doi: 10.3389/fbioe.2020.00011
Shangguan, J., Li, Y., He, D., He, X., Wang, K., Zou, Z., et al. (2015). A combination of positive dielectrophoresis driven on-line enrichment and aptamer-fluorescent silica nanoparticle label for rapid and sensitive detection of Staphylococcus aureus. Analyst 140, 4489–4497. doi: 10.1039/C5AN00535C
Tang, W. T., Liu, B., Wang, S. P., Liu, T. L., Fu, C. H., Ren, X. L., et al. (2016). Doxorubicin-loaded ionic liquid-polydopamine nanoparticles for combined chemotherapy and microwave thermal therapy of cancer. RSC Adv. 6, 32434–32440. doi: 10.1039/C6RA02434C
Veríssimo, N. V., Saponi, C. F., Ryan, T. M., Greaves, T. L., and Pereira, J. F. B. (2021). Imidazolium-based ionic liquids as additives to preserve the enhanced green fluorescent protein fluorescent activity. Green Chem. Eng. 2, 412–422. doi: 10.1016/j.gce.2021.08.001
Wang, Y., Wang, S., and Liu, L. (2022). Recovery of natural active molecules using aqueous two-phase systems comprising of ionic liquids/deep eutectic solvents. Green Chem. Eng. 3, 5–14. doi: 10.1016/j.gce.2021.07.007
Xue, Y., Zhang, X. G., Lu, Z. P., Xu, C., Xu, H. J., and Hu, Y. (2022). Enhancing the catalytic performance of candida antarctica lipase b by chemical modification with alkylated betaine ionic liquids. Front. Bioeng. Biotechnol. 10, 850890. doi: 10.3389/fbioe.2022.850890
Yuan, X., Liu, J., Wang, Y., Jie, X., Qin, J., and He, H. (2023). Ionic liquids enable highly efficient knoevenagel reaction by dual-responsive emulsion microreactor. Chem. Eng. J. 451, 138941. doi: 10.1016/j.cej.2022.138941
Zhang, S. J., Sun, J., Zhang, X. C., Xin, J. Y., Miao, Q. Q., and Wang, J. J. (2014). Ionic liquid-based green processes for energy production. Chem. Soc. Rev. 43, 7838–7869. doi: 10.1039/C3CS60409H
Zhang, Y., Cao, Y., Meng, X., Li, C., Wang, H., and Zhang, S. (2020). Enhancement of transdermal delivery of artemisinin using microemulsion vehicle based on ionic liquid and lidocaine ibuprofen. Colloid. Surfaces B 189, 110886–110886. doi: 10.1016/j.colsurfb.2020.110886
Zhao, Y., Wei, J., Li, C., Ahmed, A. F., Liu, Z., and Ma, C. (2022). A comprehensive review on mechanism of natural products against Staphylococcus aureus. J. Future Foods 2, 25–33. doi: 10.1016/j.jfutfo.2022.03.014
Keywords: ionic liquids, Staphylococcus aureus, antibacterial activity, mechanism, skin abscess
Citation: Hu Y, Xing Y, Ye P, Yu H, Meng X, Song Y, Wang G and Diao Y (2023) The antibacterial activity and mechanism of imidazole chloride ionic liquids on Staphylococcus aureus. Front. Microbiol. 14:1109972. doi: 10.3389/fmicb.2023.1109972
Received: 06 December 2022; Accepted: 09 January 2023;
Published: 06 February 2023.
Edited by:
Zhen Liu, Beijing University of Chemical Technology, ChinaCopyright © 2023 Hu, Xing, Ye, Yu, Meng, Song, Wang and Diao. This is an open-access article distributed under the terms of the Creative Commons Attribution License (CC BY). The use, distribution or reproduction in other forums is permitted, provided the original author(s) and the copyright owner(s) are credited and that the original publication in this journal is cited, in accordance with accepted academic practice. No use, distribution or reproduction is permitted which does not comply with these terms.
*Correspondence: Gongying Wang, wanggongying1102@126.com; Yanyan Diao,
yydiao@ipe.ac.cn