- 1Guangdong Provincial Key Laboratory of New Drug Screening, School of Pharmaceutical Sciences, Southern Medical University, Guangzhou, China
- 2Department of Critical Care Medicine, Zhujiang Hospital, Southern Medical University, Guangzhou, China
- 3Department of Pulmonary and Critical Care Medicine, Zhujiang Hospital, Southern Medical University, Guangzhou, China
- 4Department of Dermatology, Nanfang Hospital, Southern Medical University, Guangzhou, China
Antimicrobial peptide is one important component of the first protective barrier of organisms. They not only have potent antimicrobial activity which can protect the body from the invading pathogens, but also participate in the immune regulation of the body. In this study, a Brevinin-1 peptide named by Brevinin-1GHd was identified from Hoplobatrachus rugulosus, and the similarity of mature peptide sequence among Brevinin-1GHd, Brevinin-1HL and Brevinin-1GHa supported the close species relationship between H. rugulosus, Hylarana latouchii and Hylarana guertheri. Moreover, the secondary structure of Brevinin-1GHd was found to possess α-helical characteristics and high thermal stability. In addition, Brevinin-1GHd could bind to LPS with a Kd value of 6.49 ± 5.40 mM and suppress the release of TNF-α, NO, IL-6 and IL-1β by inactivation of MAPK signaling pathway in RAW 264.7 cells induced by LPS. Furtherly, Brevinin-1GHd had a significant inhibitory effect on acute edema development in the right paw of mice injected by carrageenan. Thus, the significant LPS-neutralizing and anti-inflammatory activities of Brevinin-1GHd were demonstrated in this study, which made it become the first Brevinin-1 family peptide with anti-inflammatory activity reported so far, and the biological activity of Brevinin-1GHd made it promising to be a novel therapeutic drug for infectious inflammation.
1. Introduction
Antimicrobial peptides (AMPs) make amphibians survive under the harsh and complex living environment with direct threat of abundant microorganisms and the infection hazard. More and more researchers focus on the functions and structure identification of amphibian AMPs (Xu and Lai, 2015; Annunziato and Costantino, 2020), which are reported to have antimicrobial, LPS-neutralization, immune regulation, anti-virus, anti-tumor, anti-oxidation, wound healing, promoting insulin release and other functions at present (Zhang et al., 2021). AMPs from the skin secretions of amphibians are extremely diverse and more than 100 families have been identified according to their sequence similarity (Xu and Lai, 2015). Brevinin family is one of the richest members, which is composed of two sub-families: Brevinin-1 and Brevinin-2 (Conlon, 2008; Nicolas and El Amri, 2009). The first Brevinin peptide was discovered in 1992 and about 350 Brevinin peptides have been found so far (Savelyeva et al., 2014). Most Brevinins shows broad-spectrum antibacterial and high hemolytic activity. In addition, these peptides are usually linear, amphiphilic and cationic and have a C-terminal Rana box (Cys-(Xaa) 5-Cys), which is bridged by a disulfide (Novkovic et al., 2012). Furtherly, although both Brevinin-1 and-2 generally have four unchanged residues, namely Ala9, Cys18, Lys23, Cys24 and Lys7, Cys27, Lys28, and Cys33, the amino acid sequences of Brevinin peptides are not conservative among different species, which makes Brevinin family rich in biological functions including antimicrobial activity, insulin releasing, and anti-cancer activities (Conlon et al., 2004, 2005). Noteworthy, immunoregulatory activity has been reported from the members from Brevinin-2 rather than Brevinin-1 (Popovic et al., 2012).
Hoplobatrachus rugulosus is the only species of Hoplobatrachus recorded in China (Frost, 2016). According to Chinese medical literature, “Northeast Animal Medicine,” H. rugulosus can be used as a medicine with the functions reducing swelling, detoxification and relieving cough (The Fourth Clinical College of Jilin Medical University, 1972). Although Tigerinin-1R and HR-CATH with antimicrobial activity are identified from H. rugulosus skin (Ojo et al., 2011; Chen et al., 2021), its other components remain stills indistinct. At present, a Brevinin-1 peptide, Brevinin-1GHd (Bre-1GHd in abbreviation) with LPS-neutralizing and anti-inflammatory activities in vitro and in vivo, was identified from the skin of H. rugulosus. It is so far the first report about Brevinin-1 peptide with anti-inflammatory activity, and this finding may be the beginning of studying the anti-inflammatory function in Brevinin-1 family peptides and provide a novel therapeutic drug for infectious inflammation.
2. Materials and methods
2.1. Animals and ethical statement
Adult H. rugulosus (n = 3) were purchased from various farms from Guangzhou, Guangdong province, China. The frogs were humanely euthanized with CO2 and cleaned with distilled water, and their dorsal skins were cut off and stored in liquid nitrogen. Mice from the Experimental Animal Center of Southern Medical University (Guangdong, China) were raised at 25°C with 12 h light/dark cycle and normal rodent rearing protocols. All experimental procedure related to animals were authorized by the Animal Care and Use Ethics Committee of Southern Medical University and strictly carried out in light of the guidelines of the Animal Care and Use Ethics Committee.
2.2. Brevinin-1GHd cDNA sequencing analysis
The cDNA sequence encoding Brevinin-1GHd from H. rugulosus was identified from the skin transcriptome (unpublished data). Molecular mass and theoretical isoelectric point were analyzed using ProtParam.1 Signal peptide was predicted with SignalP-6.0.2 The simulated secondary structure model of Brevinin-1GHd was predicted by trRosettaX-Single3 and visualized by PyMOL. The trRosetta algorithm built a three-dimensional structural model based on direct energy minimization (Du et al., 2021; Supplementary Figure S1). The Brevinin-1 family peptide sequences with high homology to Brevinin-1GHd were obtained by Basic Local Alignment Search Tool (BLAST) and were aligned using the ClustalW method. Phylogenetic Tree was constructed with MEGA X package4 by using the neighbor-joining method (Kumar et al., 2018).
2.3. Peptide synthesis
Brevinin-1GHd (FLGALFKVASKLVPAAICSISKKC) was synthesized and purified by GL Biochem Ltd. (Shanghai, China). The peptide was eluted with 46% acetonitrile solution at the flow rate of 1 ml/min with an inert silica gel ODS-SP column (Shimazu, Sumi, Japan) via HPLC. When the peptide purity was over 95% according to the ratio of different front areas, the peak was gathered and lyophilized, and further determined by MALDI-TOF mass spectrometry (Supplementary Figures S2, S3).
2.4. Circular dichroism analysis
Circular dichroism (CD) spectroscopy of Brevinin-1GHd was subjected using Chirascan plus ACD spectropolarimeter (Applied Photophysics Ltd., Leatherhead, United Kingdom) as previously described by us to estimate its secondary structure and interaction with LPS (L2880, Escherichia coli O55: B5, Sigma-Aldrich, St. Louis, Missouri, MO) (Wu et al., 2021). Shortly, Brevinin-1GHd at the final concentration of 50 μM was dissolved in SDS solution (0, 30, 60, 90, and 120 mM) or in 60 mM SDS solution before incubation at different temperatures (25, 37, 50, 70, and 90°C) for 1 h, and the CD spectrum was subsequently determined. To explore the binding of Brevinin-1GHd to LPS, 0.2 mg/ml of LPS was dissolved in H2O or 30 mM SDS solution, and then was mixed with 50 μM Brevinin-1GHd for 1 h at 25°C. Soon afterwards, the CD spectrum was determined. The binding of Brevinin-1GHd with LPS was identified by comparing the changes of spectrogram of each sample. CD data are presented as the mean residue ellipticity of three consecutive scans per sample in θ, deg.·cm2·dmol−1. The mean residue ellipticity (θ, deg.·cm2·dmol−1) is calculated by following equation: , where θobs is the observed ellipticity (mdeg), c is the concentration (mol/L) of Brevinin-1GHd solution, l is the path length (cm), and n is the number of Brevinin-1GHd residues.
2.5. SPRi assay
The real-time binding reaction of Brevinin-1GHd with LPS was further measured with PlexArrayTM HTA100 system (Plexera LLC, Bothell, Washington, DC) and bare gold SPRi chip (Nanocapture gold chip, with a gold layer of 47.5 nm thickness) as previously described by us (Chai et al., 2021). Briefly, 2 mM Brevinin-1GHd was immobilized onto the surface of gold chips and LPS (1.25, 2.5, and 5 μM) as the analyte was flowed through the chip. The PLEXEA data analysis module and Prism 6.0 were used to analyze the data finally displayed as a curve.
2.6. Isothermal titration calorimetry assay
Interactions between Brevinin-1GHd and LPS were further determined with MicroCal PEAQ-ITC (Malvern; United Kingdom). Both Brevinin-1GHd and LPS powders were added to PBS solution to prepare stock solutions at concentrations of 0.5 mM and 50 μM, respectively. 280 μl of 50 μM LPS was applied into the sample cell with constant stirring speed of 250 rpm/s at 25°C before a 1.5 μl aliquot of Brevinin-1GHd at 0.5 mM was titrated into the sample cell. Instrument operation and data analysis were finished as previously described by us (Chai et al., 2021).
2.7. Membrane binding assay
Membrane binding assays were performed with Brevinin-1GHd as previously described with slight modification (Tian et al., 2021). Shortly, FITC-labeled Brevinin-1GHd was generated with FITC conjugation kit (Sangon Biotech, Shanghai, China) according to the manufacture’s manual. RAW 264.7 cells at a density of 1× 105 cells/ml were incubated with different concentrations of FITC-labeled Brevinin-1GHd (0, 1.25, 2.5, 5 and 10 μM) at 37°C for 30 min. The unbound peptide was washed out with PBS for three times. The fluorescence intensity was measured with a FACscan flow cytometer (Becton Dickinson, United States).
2.8. Cytotoxicity assay
The cytotoxicity of Brevinin-1GHd toward RAW 264.7 cells was determined by cell counting kit-8 (CCK-8; Biosharp, China). RAW 264.7 macrophages at 2× 104 cells/well were grown in 96-well plates containing DMEM medium with 10% FBS, 100 U/ml ampicillin plus 100 μg/ml streptomycin at 37°C. After adhesion, the cells were treated with different concentrations of Brevinin-1GHd (0, 1.25, 2.5, 5, 10 and 20 μM) for 24 h. The absorbance values of all wells at 450 nm was measured with microplate reader (Tecan Trading AG, Männedorf, Switzerland). All experiments were performed with at least three repeats.
2.9. Quantitative real-time PCR (qRT-PCR)
The cells were pre-treated with Brevinin-1GHd (1, 2, and 4 μM) for 30 min and were cultured with MDEM medium containing 100 ng/ml LPS for 8 h at 37°C. The cells lacking LPS and Brevinin-1GHd treatment were regarded as the negative control. Cells were lysed with trizol and isolated with chloroform, isopropanol to obtain total RNA. After the first-strand cDNA was synthesized by reverse transcription kit (Takara Company, Dalian, China) in light of the manufacturer’s manual, qRT-PCR was performed using an Applied Biosystems 7,500 instrument (Applied Biosystems, America) for estimation of mRNA expression of IL-1β, IL-6, TNF-α, and iNOS. qRT-PCR procedure and data analysis were performed as previously reported (Zeng et al., 2018). All experiments were performed with at least three repeats. The primer sequences used for the different tested genes are shown in the Supplementary Table S1.
2.10. No and inflammatory cytokine determination
RAW 264.7 cells at 2× 104/well were grown in 96-well plates overnight and then were treated with Brevinin-1GHd (1, 2, and 4 μM) or PBS for 1 h before incubation with 100 ng/ml LPS at 37°C for another 24 h. The cells lacking LPS and Brevinin-1GHd treatment were regarded as the negative control. The contents of NO, IL-1β, IL-6 and TNF-α in the culture supernatants were, respectively, measured by Griess reagent (Beyotime Biotechnology, China) and ELISA kits (Thermo Fisher Scientific, United States) in light of the manufacturers’ manuals. Nitrite contents reflecting NO production in supernatants were obtained from the standard curve produced with NaNO2 (Sigma-Aldrich, St. Louis, Missouri, United States). All experiments were performed with at least three repeats.
2.11. Western blot analysis
RAW 264.7 cells were plated in 6-well plates at the number of 1× 106 cells per well overnight for cell adhesion. Then, the macrophages were treated with different concentrations of Brevinin-1GHd (1, 2, and 4 μM) for 30 min, and then co-incubated with 100 ng/ml LPS for another 30 min before collected for WB analysis of phospho-JNK/JNK, phospho-ERK/ERK, phospho-p38/p38 and GAPDH contents (Cell Signaling Technology, Beverly, Massachusetts, USA) as previously described (Zeng et al., 2018). The cells lacking LPS and Brevinin-1GHd treatment were regarded as the negative control. All experiments were undertaken in triplicates.
2.12. Paw edema assay
The in vivo anti-inflammatory effects of Brevinin-1GHd were evaluated with the carrageenan-induced mice as described previously (Tian et al., 2021). Shortly, at the beginning of the experiment, the paw volume up to the ankle was determined in all mice using a volume meter (Taimeng PV-2007500, China). Then mice were pre-injected with Indometacin (10 mg/kg) or Brevinin-1GHd (5 mg/kg) through intraperitoneal injection. One hour after the administration, the modeling treatment was performed. Except that the right paw of the mice in the control group was treated with 50 ul of normal saline through subcutaneous injection, the other mice were treated with 50 ul of normal saline containing 1% carrageenan in the right paw through subcutaneous injection. Δpaw thickness was calculated from incremental volumes (a–b), where “a” and “b” were the volumes of the mouse’s right hind paw at different time points before and after carrageenan administration. In order to obtain mouse peak right paw tissue, another batch of mice were reprocessed as described above. Four hours after injection of carrageenan, the mouse right hind paws were surgically removed for myeloperoxidase (MPO) activity and histological analysis.
2.13. Myeloperoxidase activity assay
Myeloperoxidase (MPO) abundantly express in neutrophil granulocytes, and its presence can directly reflect the content of neutrophil granulocytes. Therefore, MPO kinetic-colorimetric assay was carried out to evaluate the neutrophil migrated to the hind paw. The frozen paws from paw edema assay were homogenated in 600 μl PBS (pH = 7.4), centrifuged at 10,000 rpm/min at 4°C for 15 min to take the supernatant and set it to a uniform concentration. Subsequently, the MPO Detection kit (Nanjing Jiancheng Bioengineering Institute, China) was used to detect the MPO content of each supernatant according to the manual of the kit. The supernatant was added to a 96-well plate pre-filled with PBS solution containing 1% cetyltrimethylammonium, 0.17 mg/ml of 3, 3′-dimethoxybenzidine and 0.0005% H2O2, and reacted in dark at 37°C for 30 min, then the absorbance values of 460 nm was detected with microplate reader (Tecan Trading AG, Ma¨nnedorf, Switzerland). MPO activity in the tissues was expressed as units of enzyme/g of tissue. One unit of MPO is the amount of enzyme that oxidizes MPO substrate to produce 1 μM luciferin per minute at 37°C.
2.14. Statistical analysis
Statistical analysis was carried out using Igor and GraphPad Prism 6.0 software (GraphPad Software Inc., La Jolla, CA, United States). The multiple groups were analyzed using one-way analysis of variance and Tukey’s post-hoc test while the significance between two experimental groups was implemented with unpaired Student’s t-test. All experiments were repeated at least three times and a p-value < 0.05 was statistically regarded as significant difference. All the values are shown as mean ± SEM.
3. Results
3.1. cDNA identification and cladistic analysis
The cDNA sequence obtained by the skin transcriptome analysis was 305 bp and encoded a precursor of classic AMP which contained the signal peptide, acidic domain and KR site. BLAST results showed that it was highly identity to Brevinin-1HL and Brevinin-1GHd reported from the skin of H. latouchii and H. guentheri with the exception of a substitution (Phe21 to Leu21) in the signal peptide (Jiang et al., 2020; Lin et al., 2021; Figures 1A,B). Thus, the mature peptide sequence was predicted to be FLGALFKVASKLVPAAICSISKKC. The secondary structure model of Brevinin-1GHd predicted by trRosetta was composed of two α-helices, which were composed of nine amino acids near N terminus (Ala4-Leu12) and five amino acids near C terminus (Ala15-Ser19), respectively. The predicted helices and coils account for 58.3 and 41.6%, respectively (Supplementary Figure S1). Considering that some biological activities of Brevinin-1GHd have been reported, Brevinin-1GHd was continued to use as the name of the peptide from H. rugulosus skin to unify this study with the previous report (Jiang et al., 2020). Like other members of Brevinin-1 family, the deduced peptide contained a “Rana box” structure which was composed by a disulfide bond between two Cys residues at the C terminus (Figures 1A,B). The phylogenetic tree analysis showed that the bootstrapping values among the three was 97, which mean a high affinity existed among these species (Figure 1C).
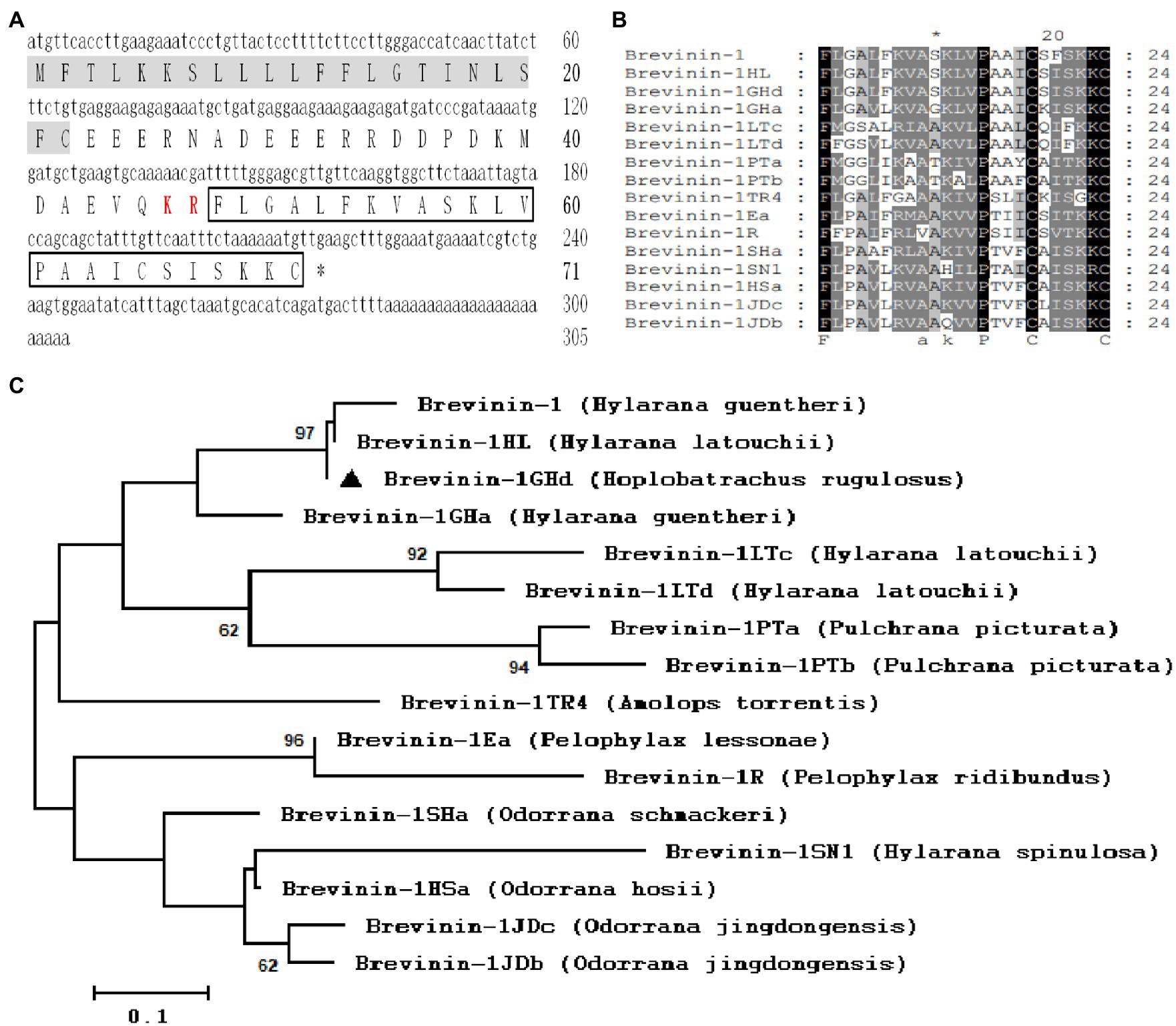
Figure 1. Sequence Analysis of Brevinin-1GHd. (A) The cDNA and predicted amino acid sequences of Brevinin-1GHd. The signal peptide and KR protease cleavage site are labeled with a gray background and red style, respectively. The amino acid sequence of mature Brevinin-1GHd is boxed, and the stop codon is indicated by an asterisk (*). (B) Amino acid sequence alignment of mature Brevinin-1GHd with other Brevinins-1 peptides from different frogs. The identical residues are marked with a black background. (C) Phylogenetic analysis of Brevinins-1 from different frogs. Numbers at the forks symbol the percentage of trees in which this group occurs after bootstrapping (1,000 replicates; shown only when >60%). Scale bar shows the number of substitutions per base. Replicates are applied to prove the reliability of each branch.
3.2. Circular dichroism determination
The secondary structure of Brevinin-1GHd was measured by CD determination in different solutions or temperature environments. As showed in Figures 2A,B, the CD curves of Brevinin-1GHd in different concentrations of SDS solutions and under different ambient temperatures were almost the same. Even when the environmental temperature rose to 90°C, the peak of the spectrum decreased slightly while its shape was also basically unchanged, which indicated that Brevinin-1GHd had high stability. In addition, the two negative shoulder band peaks at 222 nm and 208 nm and the positive peak near 192 nm were appear as a characteristic of the α-helical structure of Brevinin-1GHd. By comparing the CD spectra of Brevinin-1GHd after incubation with or without LPS in 30 mM SDS solution, the presence of LPS could rise the peak at 195 nm and the trough at 208 nm on the CD curve of Brevinin-1GHd (Figure 2C), which indicating that LPS bound to the polypeptide and changed the secondary structure of the peptide.
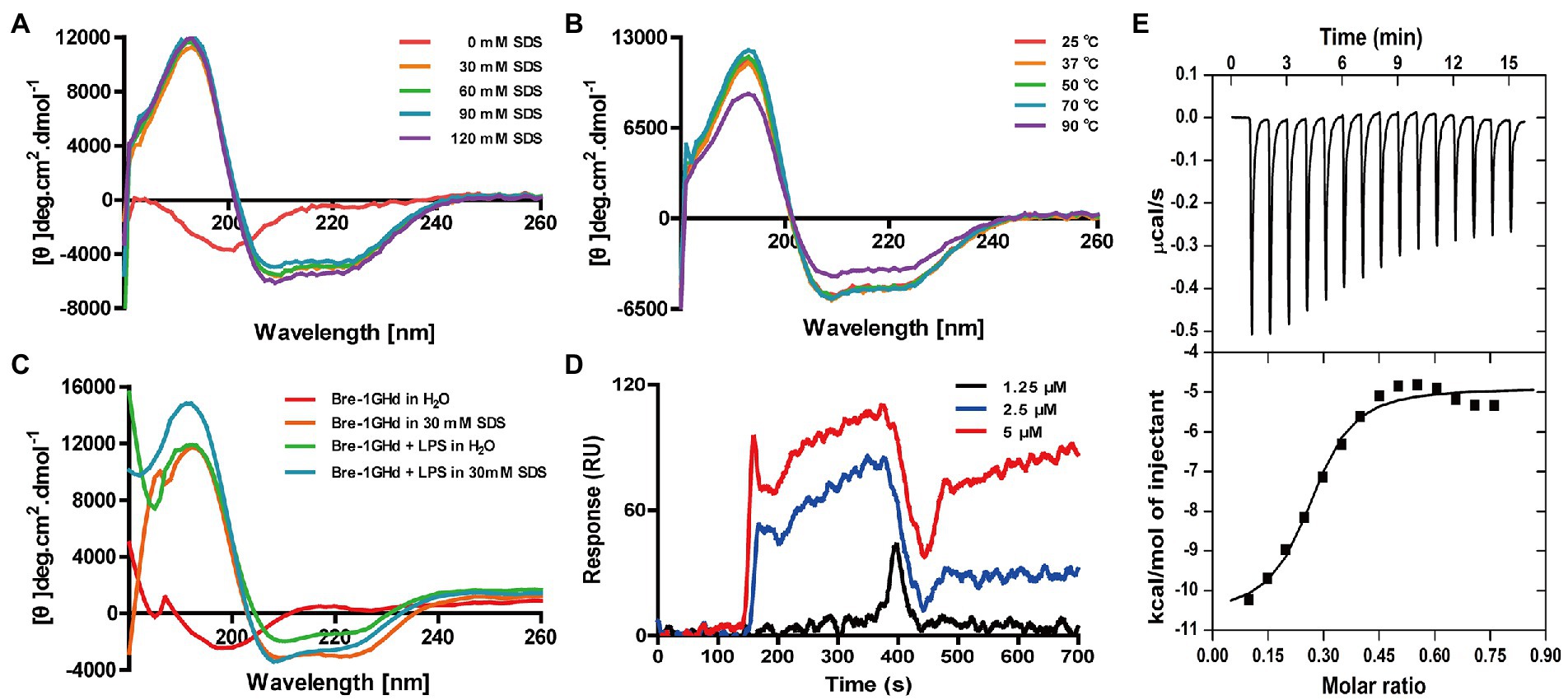
Figure 2. CD analysis of Brevinin-1GHd and binding reaction of Brevinin-1GHd with LPS. (A–C) The CD spectra of Brevinin-1GHd in different conditions. (D) Representative SPRi sensorgrams of LPS binding to Brevinin-1GHd immobilized on a gold chip. The black, blue, and red lines indicate the SPRi signal generated by 1.25, 2.5, and 5 μM LPS flowing through Brevini-1GHd immobilized on the chip, respectively. (E) ITC measurement of Brevinin-1GHd binding to LPS. The top and low panels display thermo changes of each administration and enthalpy changes as a function of ligand/target molar ratio, respectively.
3.3. Direct binding reaction with LPS
The binding activity of Brevinin-1GHd to LPS was explored with ITC and SPRi experiments. As showed in Figure 2D, when Brevinin-1GHd was immobilized on the SPRi chip and different concentrations of LPS flowed through the immobilized peptide, strong SPRi signal was appeared and resonance unit increase were concentration-dependent, indicating that LPS could directly bind to Brevinin-1GHd. Furtherly, during the reaction between Brevinin-1GHd and LPS in the ITC reflection pool, the enthalpy decreased and heat was released, indicating that they had combined. In addition, the Kd value of their binding affinity was 6.49 ± 5.40 mM (Figure 2E).
3.4. Inhibition of NO and inflammatory cytokines production induced by LPS
It is well known that LPS can induce the inflammatory action of macrophages (Luo and Song, 2021). The anti-inflammatory activity of Brevinin-1GHd was thus tested in LPS-induced mouse macrophages. Firstly, the proliferation inhibition activity of Brevinin-1GHd was tested on RAW 264.7 cells by the CCK-8 method to ensure that the concentrations used in subsequent experiments would not affect cell viability. As shown by Figure 3A, the IC50 value of Brevinin-1GHd on RAW 264.7 cells was about 12.16 μM (Figure 3A). Subsequently, the mRNA expression levels of four inflammatory factors were measured in RAW 264.7 cells by real-time PCR. After LPS stimulation, the mRNA levels of iNOS, TNF-α, IL-6 and IL-1β in RAW 264.7 cells were significantly increased while Brevinin-1GHd could concentration-dependently inhibit the stimulation effect of LPS. In the group pretreated with 4 μM Brevinin-1GHd for 30 min, the expression levels of iNOS, TNF-α, IL-6 and IL-1β mRNA were reduced by about 50.69, 80.58, 91.61 and 95.19% compared to the LPS-stimulated group, respectively (Figures 3B–E). Similar to the results of real-time PCR, RAW 264.7 cells stimulated by LPS released a large amount of NO, TNF-α, IL-6 and IL-1β, and these effects were significantly suppressed by Brevinin-1GHd in a concentration-dependent manner. As showed in Figures 3F–I, after treatment with 4 μM Brevinin-1GHd, the release of NO, TNF-α, IL-6 and IL-1β were reduced by about 87.31, 44.09, 72.10 and 67.20%, respectively. Thus, Brevinin-1GHd could significantly suppress the transcription and expression of inflammatory factors in RAW 264.7 cells stimulated by LPS at a concentration that was non-toxic to cells.
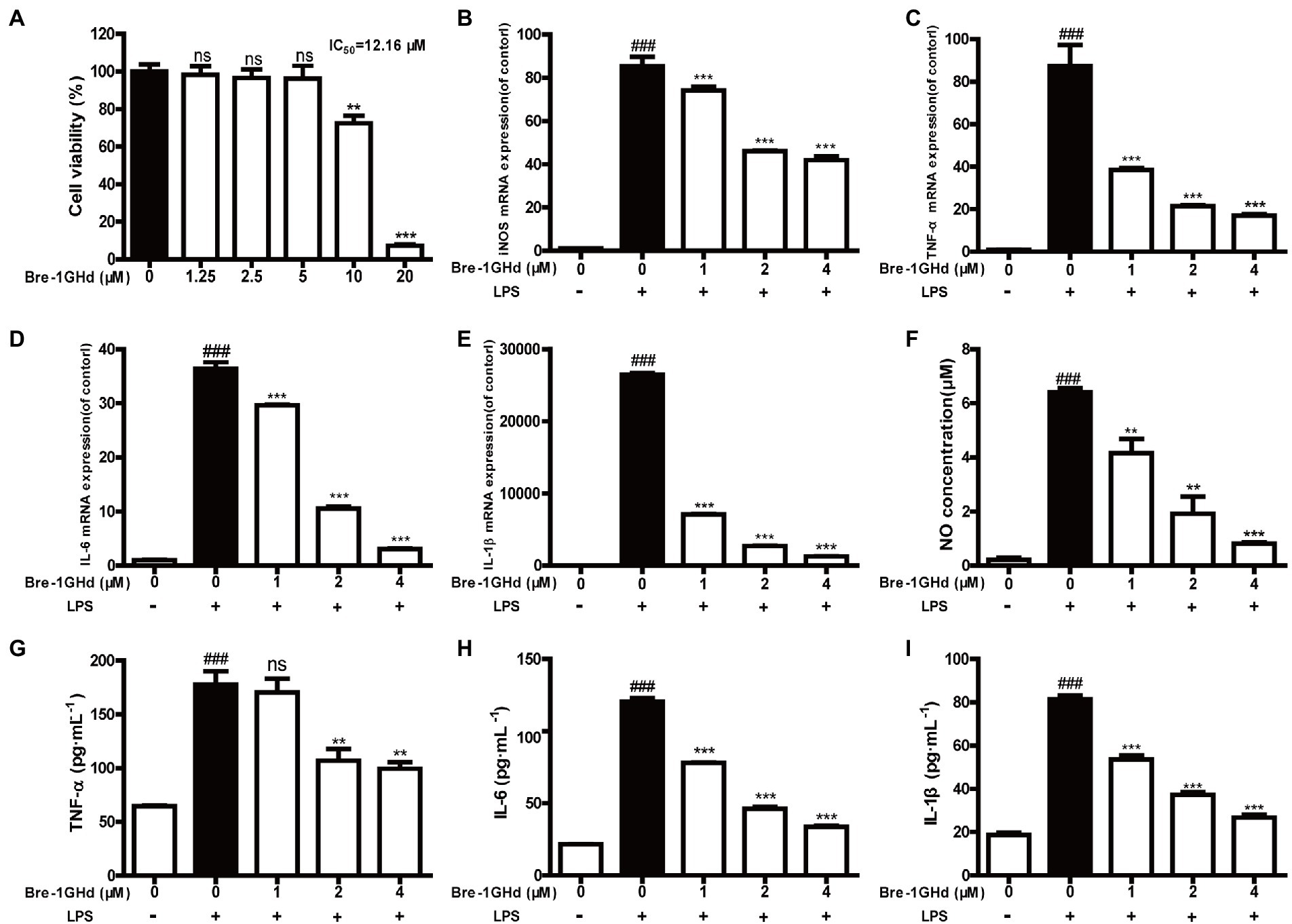
Figure 3. Effects of Brevinin-1GHd on the generation of nitrite and cytokines induced by LPS. (A) Proliferation inhibition activity of Brevinin-1GHd on RAW 264.7 cells. The cells were incubated with Brevinin-1GHd (1.25–20 μM) for 24 h, and then the IC50 value of Brevinin-1GHd on RAW264.7 cells was determined by CCK-8. (B–I) The effect of Brevinin-1GHd on production of NO, TNF-α, IL-6 and IL-1β in RAW 264.7 cells recruited with LPS. Data are mean ± SEM (n = 3). ∗∗p < 0.01 and ∗∗∗ p < 0.001 as well as ### p < 0.001 indicate significant difference in comparison with the control incubated with 100 ng/ml LPS and the control without LPS plus Brevinin-1GHd, respectively.
3.5. Suppression of LPS-induced inflammatory response pathways
It is well known that LPS can induce the phosphorylation of JNK, ERK and p38 protein which are main components of MAPK pathway (Negishi et al., 2006; Cao et al., 2019). The effect of Brevinin-1GHd was thus investigated on the inflammation-related signaling pathways of RAW 264.7 cells activated by LPS with WB assays. As presented in Figure 4A, compared with the control without LPS stimulation, the expressions of phosphorylated JNK, ERK and p38 in the LPS stimulation group were increased significantly, which indicated that LPS successfully activated the MAPK pathway of RAW 264.7 cells. Meanwhile, this effect of LPS was significantly reduced by Brevinin-1GHd in a concentration-dependent manner. The ratios of p-JNK/JNK, p-ERK/ERK and p-p38/p38 in the 4 μM Brevinin-1GHd group were reduced by about 27.68, 36.80 and 51.29%, respectively, compared with the LPS-stimulated group (Figure 4B). This result indicated that Brevinin-1GHd mainly acted on the MAPK signaling pathway of RAW 264.7 cells, thereby inhibiting the inflammatory response induced by LPS.
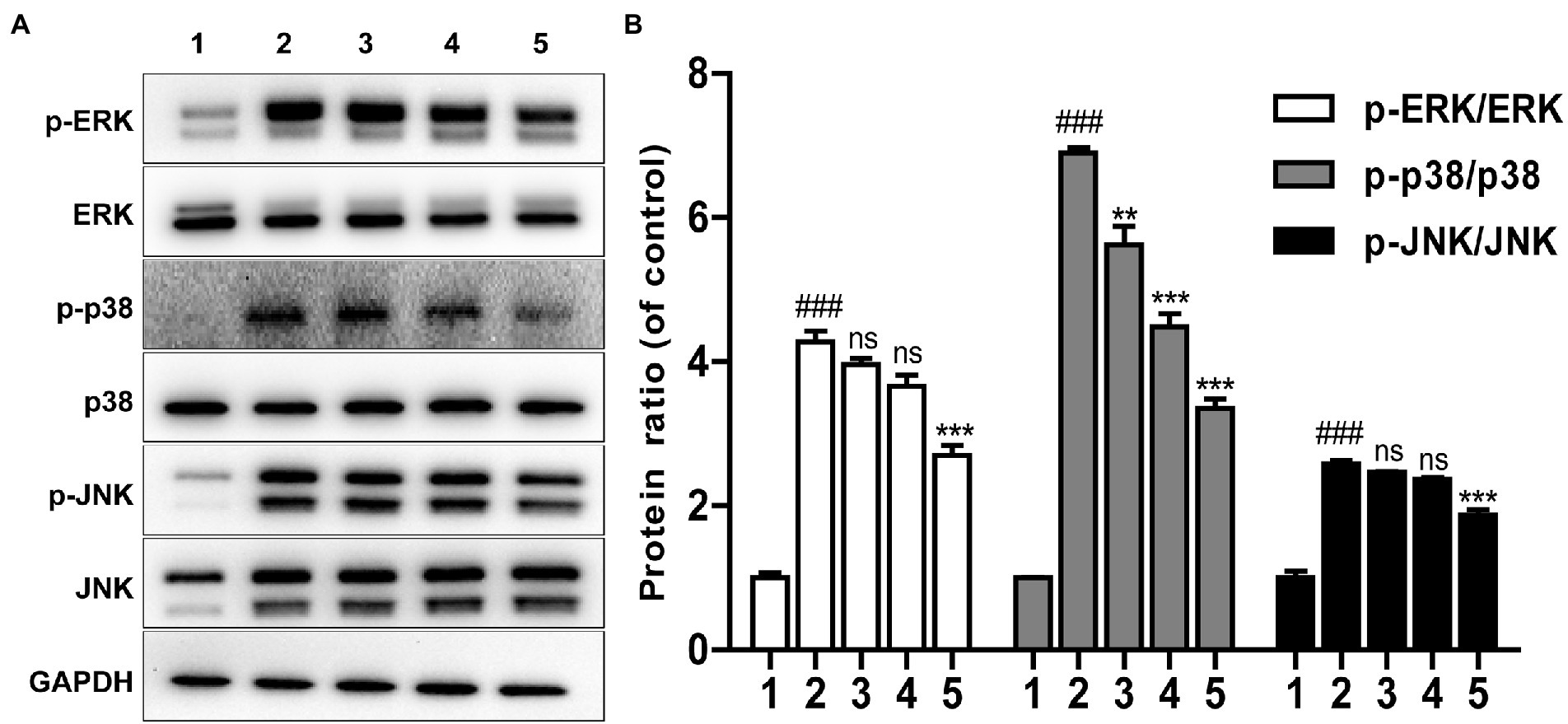
Figure 4. Effects of Brevinin-1GHd on inflammatory pathway activation inspired by LPS. (A) Typical WB bands of ERK, p38, JNK in RAW 264.7 cells. The cells were pre-treated with or without 1, 2, and 4 μM Brevinin-1GHd for 30 min and an additional 30 min after LPS addition, then were collected for WB analysis. ICONS 1–5 are the control group, LPS treatment group, and 100 ng/ml LPS plus 1, 2, and 4 μM Brevinin-1GHd treatment groups, respectively. (B) Quantification analysis of band densities in A figure. Data are expressed as mean ± SEM (n = 3). ∗∗p < 0.01 and ∗∗∗p < 0.001 as well as ### p < 0.001 suggest significant difference in comparison with the control incubated with 100 ng/ml LPS and with the control without both LPS and Brevinin-1GHd, respectively.
3.6. Paw edema assay
Carrageenan-induced mice paw edema assay was carried out to study the anti-inflammatory effects of Brevinin-1GHd in vivo (Park and Im, 2021). As shown in Figure 5A, carrageenan administration obviously promoted the development of the inflammatory vascular phase of the right paw of mice, resulting in increased skin edema on the hind paw, however, intraperitoneal injection of 10 mg/kg Indomethacin or 5 mg/kg Brevinin-1GHd into mice before carrageenan injection could significantly alleviate the swelling of the right paw of mice. The measurement results showed that the swelling volume of the right paw treated by Brevinin-1GHd and Indomethacin at 4 h was reduced by about 57.62 and 40.67%, respectively, compared with the mice in the model group and the swelling volume of the right paw in 24 h was also reduced by about 40.00 and 16.66%, respectively (Figure 5B). In addition, MPO levels in the right paw tissue grinding fluid of mice injected with carrageenan increased dramatically. Compared with the mice in the model group injected with carrageenan alone, the MPO concentration in the right paw tissue grinding fluid of mice injected with Indomethacin or Brevinin-1GHd before carrageenan injection decreased by about 20.03 and 27.98%, respectively (Figure 5C). Furthermore, histopathological evaluation showed that the paw tissue of mice in the model group only exposed to carrageenan had epithelial hyperplasia, inflammatory cell infiltration and sub-epidermal edema, while Indomethacin and Brevinin-1GHd significantly prevented these signs of inflammation (Figure 5D). The above data suggested that Brevinin-1GHd had a significant inhibitory effect on the development of acute edema in the right paw of mice.
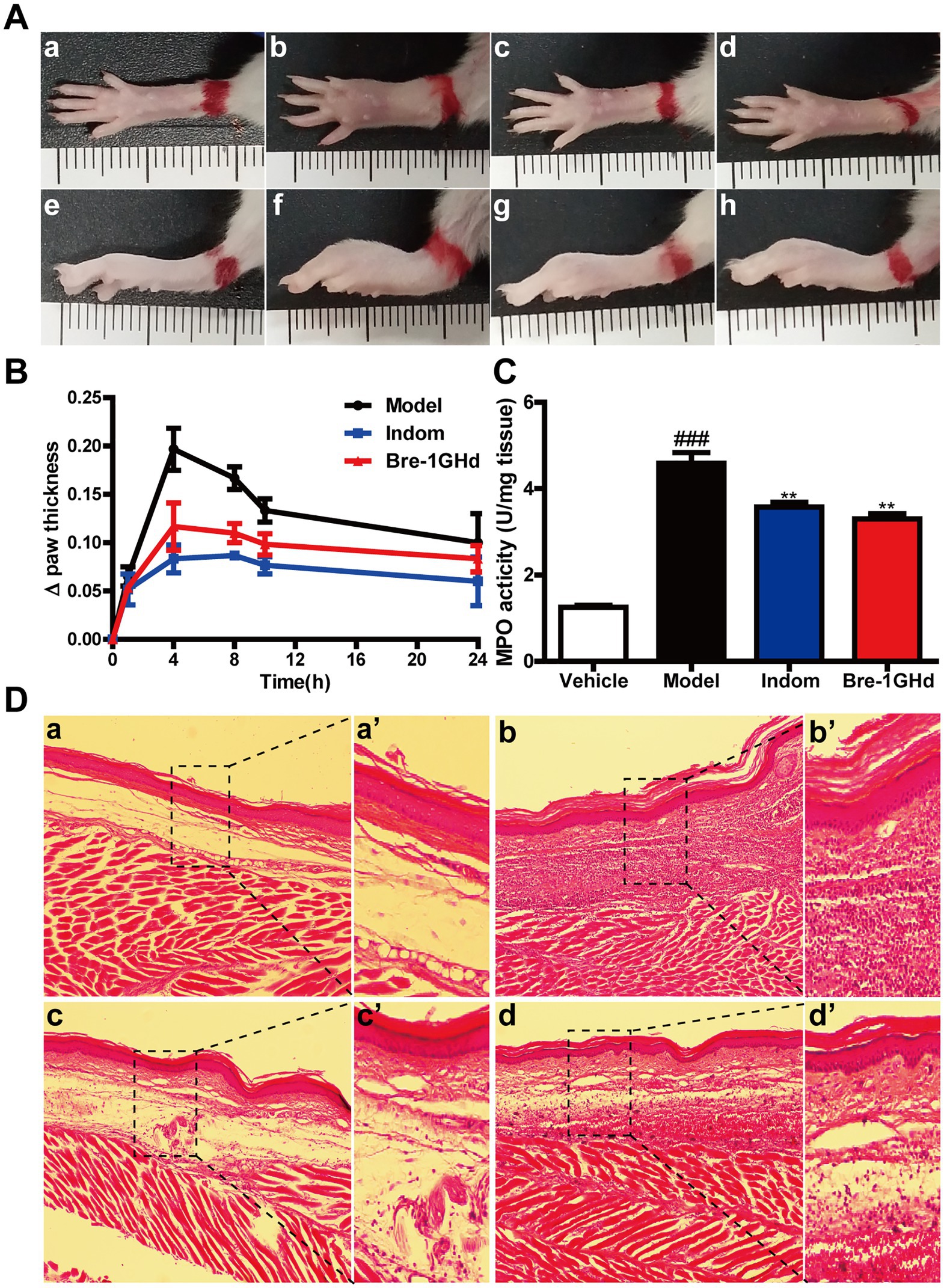
Figure 5. Treatment of carrageenan-induced acute inflammation in mice paw by Brevinin-1GHd. (A) Mice paws at 4 h after carrageenan administration. Panels a–d and e–h indicate the corresponding bottom and lateral views of the paws. Images from left to right are the control group (a,e), the model group (b,f), Indomethacin group (c,g) and Brevinin-1GHd group (d,h). (B) Thickness of right paw of mice at different time points within 24 h. (C) MPO activity in the right paw of mice at 4 h after administration. (D) HE staining of the right paw of mice at 4 h after administration. Labels (a,a’)–(d,d’) refer to the control group, model group, Indomethacin group and Brevinin-1GHd group, respectively. The scale bars of a-d and a’-d’ were 50 and 200 μm, respectively.
4. Discussion and conclusions
The increasing number of AMPs have been demonstrated to show both antimicrobial and immunomodulatory activities due to their unique physicochemical properties and mechanism of action, which make them have great potential in the treatment of infectious inflammation (Mahlapuu et al., 2016; Luo and Song, 2021). The immunoregulatory activity of Brevinin-1 family, however, has not been reported so far. In this study, Brevinin-1GHd was proved to possess LPS neutralizing as well as anti-inflammatory activity in vitro and in vivo.
The evolution of AMPs in amphibians is characterized by frequent gene duplication and diversification driven by positive selection, which can cause the generation of resistance genes with new functional properties. Furthermore, functionally divergent AMPs can cope with new emerging pathogens in the environment or old pathogens that have developed resistance (Lazzaro et al., 2020). As a result, it makes the molecular variation within a particular AMP family is extremely large, meaning a peptide from one species little show an identical amino acid sequence in another, even when two species are quite close in the evolutionary relationship (Tennessen and Blouin, 2007). For the members belonging to Brevinin-1 family widely identified in most Eurasian and New World species, the discovery of Brevinin-1 peptides with the same amino acid sequence in different species occurs occasionally (Savelyeva et al., 2014). In this study, a peptide identified from the skin of H. rugulosus frog is with complete identity to known AMP in H. guentheri and H. latouchi. The repeated expression of Brevinin-1GHd in the three species may be an important basis for their common evolutionary origin. After all, it has been reported that Hoplobatrachus and Hylarana species are most likely originated from southern Asia (Sultana et al., 2017; Reilly et al., 2022). In addition, H. rugulosus is mainly distributed in southern China and broadly inhabits rice fields, pools or stone crevices, which are similar to H. guentheri and H. latouchi. The resembling geographical environment is probably one of the key factors to maintain conservative expression of Brevinin-1GHd in three species during the evolutionary process (AmphibiaChina, 2022). Furthermore, it is possible that other AMPs like Brevinin-1GHd are co-expressed in different species, and the reason why these AMPs remain in the process of different species evolution are worth further being explored.
LPS is a momentous component in the cell wall of Gram-negative bacteria (Zhang et al., 2013) and often released into the blood circulation during infection, inducing monocytes and phagocytes to produce a large amount of cytokines and local inflammation (Maldonado et al., 2016). Therefore, the neutralization of LPS produced by bacteria is the key to the treatment of infectious inflammation. Coincidentally, most AMPs are positive charges and have a high content of hydrophobic residues. Positive charges can promote strong electrostatic interaction between AMPs and negatively charged LPS, and hydrophobicity makes them easy to be embedded in LPS micelles. Thus, some AMPs have the ability to bind LPS (Sun and Shang, 2015; Schromm et al., 2021). Considering that Brevinin-1GHd contains not only four positively charged lysine residues, but also hydrophobic amino acids such as alanine, leucine, valine, proline, isoleucine and phenylalanine, it was speculated to have LPS-neutralizing activity. In agreement, the overwhelming evidences from ITC, SPRi, CD and WB experiments demonstrated this speculation. Interestingly, although the secondary structures of Brevinin-1GHd and Cath-MH are very similar and both dominated by α-helices with short random coils at both ends, the LPS-neutralization ability of Brevinin-1GHd is significantly weaker than that of Cath-MH (Chai et al., 2021). By comparing their sequences and physicochemical properties, Cath-MH is found to contain more positively charged residues than Brevinin-1GHd. Furtherly, Brevinin-1GHd showed more significant anti-inflammatory activity than Brevinin-2MP in the treatment of carrageenan-induced acute inflammation in animal models when comparing present results with data reported by Tian (Tian et al., 2021). Although Brevinin-2MP has one more cation (Arg) than Brevinin-1GHd, it also has two more negatively charged residuals (Asp + Glu). This may prove that cationic property is one of the keys to affect the anti-inflammatory activity of AMPs (Sun and Shang, 2015). Beyond all doubt, such inferences need be verified in future experiments.
It is worth noting that Brevinin-1GHd also shows good anti-inflammatory activity in carrageenan-induced mouse paw swelling which lacks LPS (Figure 5). Some AMPs have been described to exert their anti-inflammatory activities by directly acting on cell surface receptors. For example, PS-K18 can inhibit TLR4-mediated NF-κB pathway and activate innate defense (Jang et al., 2019). In addition, Brevinin-1GHd could bind cell surface in a concentration dependent manner which indicate that it can also directly bind to some receptors on RAW 264.7 cells (Supplementary Figure S4). Therefore, the anti-inflammatory mechanism of Brevinin-1GHd maybe be also related to its receptors on the cell surface and further study is necessary to clarify its anti-inflammatory mechanism completely.
In conclusion, including broad-spectrum antibacterial activity, Brevinin-1GHd can not only neutralize LPS rapidly, but also effectively inhibit the inflammation of RAW 264.7 cells induced by LPS. At the same time, Brevinin-1GHd also shows significant therapeutic effect on carrageenan-induced acute inflammation of mice paws. These biological activities make Brevinin-1GHd very likely to be a new anti-infective agent to assist or replace antibiotics.
Data availability statement
The original contributions presented in the study are included in the article/Supplementary material, further inquiries can be directed to the corresponding authors.
Author contributions
MT, KW, YL, JC, JW, HZ, and XH performed experiments and analyzed data. XC and XX designed experiments, supervised the study, evaluated the data, and wrote and revised the manuscript for publication. All authors contributed to the article and approved the submitted version.
Funding
This study was supported in part by the National Natural Science Foundation of China (Nos, 31772476, 31911530077, 31861143050, and 82000040).
Conflict of interest
The authors declare that the research was conducted in the absence of any commercial or financial relationships that could be construed as a potential conflict of interest.
Publisher’s note
All claims expressed in this article are solely those of the authors and do not necessarily represent those of their affiliated organizations, or those of the publisher, the editors and the reviewers. Any product that may be evaluated in this article, or claim that may be made by its manufacturer, is not guaranteed or endorsed by the publisher.
Supplementary material
The Supplementary material for this article can be found online at: https://www.frontiersin.org/articles/10.3389/fmicb.2023.1102576/full#supplementary-material
Footnotes
1. ^https://web.expasy.org/protparam/
2. ^https://www.cbs.dtu.dk/services/SignalP/
References
AmphibiaChina. (2022). The Database of Chinese Amphibians. Kunming, Yunnan, China: Kunming Institute of Zoology (CAS).
Annunziato, G., and Costantino, G. (2020). Antimicrobial peptides (AMPs): a patent review (2015-2020). Expert Opin. Ther. Pat. 30, 931–947. doi: 10.1080/13543776.2020.1851679
Cao, Y., Chen, J., Ren, G., Zhang, Y., Tan, X., and Yang, L. (2019). Punicalagin prevents inflammation in LPS-induced RAW264.7 macrophages by inhibiting FoxO3a/autophagy signaling pathway. Nutrients 11:2794. doi: 10.3390/nu11112794
Chai, J., Chen, X., Ye, T., Zeng, B., Zeng, Q., Wu, J., et al. (2021). Characterization and functional analysis of cathelicidin-MH, a novel frog-derived peptide with anti-septicemic properties. Elife 10:e64411. doi: 10.7554/eLife.64411
Chen, J., Lin, Y. F., Chen, J. H., Chen, X., and Lin, Z. H. (2021). Molecular characterization of cathelicidin in tiger frog (Hoplobatrachus rugulosus): antimicrobial activity and immunomodulatory activity. Comp. Biochem. Physiol. C Toxicol. Pharmacol. 247:109072. doi: 10.1016/j.cbpc.2021.109072
Conlon, J. M. (2008). Reflections on a systematic nomenclature for antimicrobial peptides from the skins of frogs of the family Ranidae. Peptides 29, 1815–1819. doi: 10.1016/j.peptides.2008.05.029
Conlon, J. M., Abraham, B., Sonnevend, A., Jouenne, T., Cosette, P., Leprince, J., et al. (2005). Purification and characterization of antimicrobial peptides from the skin secretions of the carpenter frog Rana virgatipes (Ranidae, Aquarana). Regul. Pept. 131, 38–45. doi: 10.1016/j.regpep.2005.06.003
Conlon, J. M., Kolodziejek, J., and Nowotny, N. (2004). Antimicrobial peptides from ranid frogs: taxonomic and phylogenetic markers and a potential source of new therapeutic agents. Biochim. Biophys. Acta 1696, 1–14. doi: 10.1016/j.bbapap.2003.09.004
Du, Z., Su, H., Wang, W., Ye, L., Wei, H., Peng, Z., et al. (2021). The trRosetta server for fast and accurate protein structure prediction. Nat. Protoc. 16, 5634–5651. doi: 10.1038/s41596-021-00628-9
Frost, D. R.. (2016). Amphibian Species of the World: An Online Reference. Version 6. 0 (Date of Access). New York, USA: American Museum of Natural History.
Jang, M., Kim, J., Choi, Y., Bang, J., and Kim, Y. (2019). Antiseptic effect of Ps-K18: mechanism of its antibacterial and anti-inflammatory activities. Int. J. Mol. Sci. 20:4895. doi: 10.3390/ijms20194895
Jiang, Y., Wu, Y., Wang, T., Chen, X., Zhou, M., Ma, C., et al. (2020). Brevinin-1GHd: a novel Hylarana guentheri skin secretion-derived Brevinin-1 type peptide with antimicrobial and anticancer therapeutic potential. Biosci. Rep. 40:BSR20200019. doi: 10.1042/BSR20200019
Kumar, S., Stecher, G., Li, M., Knyaz, C., and Tamura, K. (2018). MEGA X: molecular evolutionary genetics analysis across computing platforms. Mol. Biol. Evol. 35, 1547–1549. doi: 10.1093/molbev/msy096
Lazzaro, B. P., Zasloff, M., and Rolff, J. (2020). Antimicrobial peptides: application informed by evolution. Science 368:eaau5480. doi: 10.1126/science.aau5480
Lin, Y., Lin, T., Cheng, N., Wu, S., Huang, J., Chen, X., et al. (2021). Evaluation of antimicrobial and anticancer activities of three peptides identified from the skin secretion of Hylarana latouchii. Acta Biochim. Biophys. Sin. Shanghai 53, 1469–1483. doi: 10.1093/abbs/gmab126
Luo, Y., and Song, Y. (2021). Mechanism of antimicrobial peptides: antimicrobial, anti-inflammatory and antibiofilm activities. Int. J. Mol. Sci. 22:11401. doi: 10.3390/ijms222111401
Mahlapuu, M., Hakansson, J., Ringstad, L., and Bjorn, C. (2016). Antimicrobial peptides: an emerging category of therapeutic agents. Front. Cell. Infect. Microbiol. 6:194. doi: 10.3389/fcimb.2016.00194
Maldonado, R. F., Sa-Correia, I., and Valvano, M. A. (2016). Lipopolysaccharide modification in gram-negative bacteria during chronic infection. FEMS Microbiol. Rev. 40, 480–493. doi: 10.1093/femsre/fuw007
Negishi, H., Fujita, Y., Yanai, H., Sakaguchi, S., Ouyang, X., Shinohara, M., et al. (2006). Evidence for licensing of IFN-gamma-induced IFN regulatory factor 1 transcription factor by MyD88 in toll-like receptor-dependent gene induction program. Proc. Natl. Acad. Sci. U. S. A. 103, 15136–15141. doi: 10.1073/pnas.0607181103
Nicolas, P., and El Amri, C. (2009). The dermaseptin superfamily: a gene-based combinatorial library of antimicrobial peptides. Biochim. Biophys. Acta 1788, 1537–1550. doi: 10.1016/j.bbamem.2008.09.006
Novkovic, M., Simunic, J., Bojovic, V., Tossi, A., and Juretic, D. (2012). DADP: the database of anuran defense peptides. Bioinformatics 28, 1406–1407. doi: 10.1093/bioinformatics/bts141
Ojo, O. O., Abdel-Wahab, Y. H., Flatt, P. R., Mechkarska, M., and Conlon, J. M. (2011). Tigerinin-1R: a potent, non-toxic insulin-releasing peptide isolated from the skin of the Asian frog, Hoplobatrachus rugulosus. Diabetes Obes. Metab. 13, 1114–1122. doi: 10.1111/j.1463-1326.2011.01470.x
Park, S. J., and Im, D. S. (2021). 2-Arachidonyl-lysophosphatidylethanolamine induces anti-inflammatory effects on macrophages and in carrageenan-induced paw edema. Int. J. Mol. Sci. 22:4865. doi: 10.3390/ijms22094865
Popovic, S., Urban, E., Lukic, M., and Conlon, J. M. (2012). Peptides with antimicrobial and anti-inflammatory activities that have therapeutic potential for treatment of acne vulgaris. Peptides 34, 275–282. doi: 10.1016/j.peptides.2012.02.010
Reilly, S. B., Arifin, U., Stubbs, A. L., Karin, B. R., Kaiser, H., Frederick, J. H., et al. (2022). Phylogenetic relationships of southern Wallacean ranid frogs (Anura: Ranidae: Hylarana). Zootaxa 5150, 591–599. doi: 10.11646/zootaxa.5150.4.7
Savelyeva, A., Ghavami, S., Davoodpour, P., Asoodeh, A., and Los, M. J. (2014). An overview of Brevinin superfamily: structure, function and clinical perspectives. Adv. Exp. Med. Biol. 818, 197–212. doi: 10.1007/978-1-4471-6458-6_10
Schromm, A. B., Paulowski, L., Kaconis, Y., Kopp, F., Koistinen, M., Donoghue, A., et al. (2021). Cathelicidin and PMB neutralize endotoxins by multifactorial mechanisms including LPS interaction and targeting of host cell membranes. Proc. Natl. Acad. Sci. U. S. A. 118:21118. doi: 10.1073/pnas.2101721118
Sultana, N., Igawa, T., Islam, M. M., Hasan, M., Alam, M. S., Komaki, S., et al. (2017). Inter-and intra-specific genetic divergence of Asian tiger frogs (genus Hoplobatrachus), with special reference to the population structure of H. tigerinus in Bangladesh. Genes Genet. Syst. 91, 217–227. doi: 10.1266/ggs.16-00006
Sun, Y., and Shang, D. (2015). Inhibitory effects of antimicrobial peptides on lipopolysaccharide-induced inflammation. Mediat. Inflamm. 2015:167572. doi: 10.1155/2015/167572
Tennessen, J. A., and Blouin, M. S. (2007). Selection for antimicrobial peptide diversity in frogs leads to gene duplication and low allelic variation. J. Mol. Evol. 65, 605–615. doi: 10.1007/s00239-007-9045-5
The Fourth Clinical College of Jilin Medical University. (1972). Animal Medicine of Northeast Province. Changchun, China: Jilin People’s Press.
Tian, M., Liu, J., Chai, J., Wu, J., and Xu, X. (2021). Antimicrobial and anti-inflammatory effects of a novel peptide from the skin of frog Microhyla pulchra. Front. Pharmacol. 12:783108. doi: 10.3389/fphar.2021.783108
Wu, J., Zhang, H., Chen, X., Chai, J., Hu, Y., Xiong, W., et al. (2021). FM-CATH, a novel cathelicidin from Fejervarya multistriata, shows therapeutic potential for treatment of CLP-induced sepsis. Front. Pharmacol. 12:731056. doi: 10.3389/fphar.2021.731056
Xu, X., and Lai, R. (2015). The chemistry and biological activities of peptides from amphibian skin secretions. Chem. Rev. 115, 1760–1846. doi: 10.1021/cr4006704
Zeng, B., Chai, J., Deng, Z., Ye, T., Chen, W., Li, D., et al. (2018). Functional characterization of a novel lipopolysaccharide-binding antimicrobial and anti-inflammatory peptide in vitro and in vivo. J. Med. Chem. 61, 10709–10723. doi: 10.1021/acs.jmedchem.8b01358
Zhang, G., Meredith, T. C., and Kahne, D. (2013). On the essentiality of lipopolysaccharide to gram-negative bacteria. Curr. Opin. Microbiol. 16, 779–785. doi: 10.1016/j.mib.2013.09.007
Keywords: antimicrobial peptide, amphibian, Brevinin-1, lipopolysaccharide, anti-inflammation
Citation: Tian M, Wang K, Liang Y, Chai J, Wu J, Zhang H, Huang X, Chen X and Xu X (2023) The first Brevinin-1 antimicrobial peptide with LPS-neutralizing and anti-inflammatory activities in vitro and in vivo. Front. Microbiol. 14:1102576. doi: 10.3389/fmicb.2023.1102576
Edited by:
Einar Ringo, UiT the Arctic University of Norway, NorwayReviewed by:
Heng Zheng, China Pharmaceutical University, ChinaEugene A. Rogozhin, Institute of Bioorganic Chemistry (RAS), Russia
Copyright © 2023 Tian, Wang, Liang, Chai, Wu, Zhang, Huang, Chen and Xu. This is an open-access article distributed under the terms of the Creative Commons Attribution License (CC BY). The use, distribution or reproduction in other forums is permitted, provided the original author(s) and the copyright owner(s) are credited and that the original publication in this journal is cited, in accordance with accepted academic practice. No use, distribution or reproduction is permitted which does not comply with these terms.
*Correspondence: Xueqing Xu, xu2003@smu.edu.cn; Xin Chen, hxkd006@smu.edu.cn
†These authors have contributed equally to this work and share first authorship