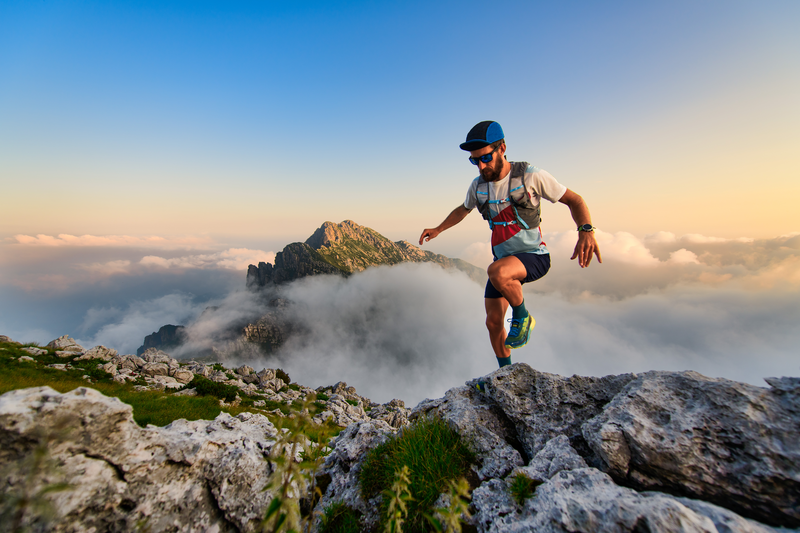
94% of researchers rate our articles as excellent or good
Learn more about the work of our research integrity team to safeguard the quality of each article we publish.
Find out more
MINI REVIEW article
Front. Microbiol. , 20 February 2023
Sec. Aquatic Microbiology
Volume 14 - 2023 | https://doi.org/10.3389/fmicb.2023.1097712
This article is part of the Research Topic The Biosynthesis, Emission, and Ecophysiological Functions of Algal Volatile Organic Compounds (VOCs) View all 3 articles
Cyanobacteria are photosynthetic prokaryotes and one of dominant species in eutrophicated waters, which easily burst blooms in summer with high irradiance and temperature conditions. In response to high irradiance, high temperature, and nutrient conditions, cyanobacteria release abundant of volatile organic compounds (VOCs) by up-regulating related gene expression and oxidatively degrading β-carotene. These VOCs not only increase offensive odor in waters, but also transfer allelopathic signals to algae and aquatic plants, resulting in cyanobacteria dominating eutrophicated waters. Among these VOCs, β-cyclocitral, α-ionone, β-ionone, limonene, longifolene, and eucalyptol have been identified as the main allelopathic agents, which even directly kill algae by inducing programmed cell death (PCD). The VOCs released from cyanobacteria, especially the ruptured cells, exhibit repelling effects on the herbivores, which is beneficial to survival of the population. Cyanobacterial VOCs might transfer aggregating information among homogeneous species, so the acceptors initiate aggregation to resist the coming stresses. It can be speculated that the adverse conditions can promote VOC emission from cyanobacteria, which play important roles in cyanobacteria dominating eutrophicated waters and even bursting blooms.
Human activities lead to the continuous inputs of nutrients, especially nitrogen (N) and phosphorus (P), into inland waters, which aggravates the eutrophication (Peñuelas et al., 2013; Yang et al., 2020). Cyanobacteria are photosynthetic prokaryotes, which are quite different from eukaryotic algae. In eutrophicated waters, cyanobacteria massively multiply and grow, become the dominant species, and even burst blooms (Hudnell and Dortch, 2008). However, other aquatic organisms gradually reduce and even disappear at the end, including zooplankton, algae, aquatic plants, and fishes (Körner, 2001; Abrantes et al., 2006; Wu et al., 2019; Landsberg et al., 2020).
Cyanobacteria release a wide spectrum of volatile organic compounds (VOCs) through secondary metabolic pathways, mainly including terpenoids, sulfocompounds, benzenes, alkenes, furans, alkanes, alcohols, ketones, aldehydes, and esters (Walsh et al., 1998; Xu et al., 2017; Ye et al., 2018; Zuo et al., 2018a,b; Zuo, 2019). Their emission rate during cyanobacterial blooms can reach to approximately 81.2 μg·m−2·h−1 in Chaohu Lake in China (Liu M. et al., 2021), and the emission is sensitive to environmental conditions, such as nutrients (Xu et al., 2017; Ye et al., 2018; Zuo et al., 2018a,b), high irradiance (Walsh et al., 1998; Wang and Li, 2015; Zheng et al., 2020), and high temperature (Wang and Li, 2015; Zheng et al., 2020).
Cyanobacterial VOCs result in offensive odor in waters, of which geosmin, 2-methylisoborneol, β-cyclocitral, and β-ionone provide main contribution (Watson et al., 2016; Liu et al., 2017). For geosmin and 2-methylisoborneol, they are responsible for musty and earthy odors, and easily sensed by human, due to their extremely low odor thresholds (< 10 ng·L−1) (Watson et al., 2016). The toxicological effects of these odor compounds on human are still unknown, but they profoundly lower the water aesthetics and quality, and even cause drinking water crisis to more than two million residents for 5 days in Wuxi in China (Ma et al., 2013). Moreover, these VOCs serve important functions in cyanobacteria dominating eutrophicated waters, such as inhibiting and even killing algae and aquatic plants (Xu et al., 2017; Zuo et al., 2018b; Sun et al., 2020; Du et al., 2022), repelling herbivores (Zuo, 2019; Havaux, 2020; Saha and Fink, 2022), and possibly transferring aggregating information to induce the formation of blooms. In this review, the emission of VOCs from cyanobacteria and their functions in blooms are summarized to provide helps for uncovering cyanobacteria blooms from VOC perspective. To the best of my knowledge, this is the first specific review about the research advances of cyanobacterial VOCs.
In eutrophicated waters, cyanobacteria massively grow in spring with suitable conditions, and they reach to the biomass peak and easily burst blooms in summer (June to August or September in the northern hemisphere) with high irradiance and temperature conditions (Beal et al., 2021). Under high irradiance, a remarkable improvement is detected in the emission amount of VOCs from cyanobacteria (Walsh et al., 1998; Wang and Li, 2015; Zheng et al., 2020). 2-Methylisoborneol and geosmin are two typical cyanobacterial VOCs, which can be released by over 70 cyanobacterial species, such as Anabaena, Lyngbya, Nostoc, Phormidium, Oscillatoria, Aphanizomenon, and Planktothrix (Izaguirre and Taylor, 2004; Watson et al., 2016). The emission of the two compounds varied with season and exhibited an increase trend from spring to late summer (Westerhoff et al., 2005), and their content can reach to 51.4 and 120.9 ng·L−1, respectively, in Eagle Creek Reservoir, Indiana (Clercin et al., 2022). 2-Methylisoborneol and geosmin belong to terpenoids, and are synthesized with geranyl diphosphate (GPP) from methylerythritol-4-phosphate pathway (MEP) and farnesyl diphosphate (FPP) from mevalonate pathway (MVA) as the precursor, respectively. In the formation of 2-methylisoborneol, GPP is transferred into a methyl from S-adenosylmethionine to form 2-methyl GPP, and then converted into 2-methylisoborneol by catalyzing with 2-methylisoborneol synthase (Figure 1A) (Giglio et al., 2011; Watson et al., 2016). In the generation of geosmin, FPP is transformed to germacradienol in the catalysis of geosmin synthase N-terminus, and then converted into geosmin through an intermediate compound 8,10-dimethyl-1-octalin by catalyzing with the enzyme C-terminus (Figure 1B) (Jiang et al., 2007; Watson et al., 2016). Compared with low irradiance, high irradiance obviously promoted the emission of 2-methylisoborneol from Pseudanabaena sp. and geosmin from Anabaena ucrainica (Wang and Li, 2015), which might be caused by up-regulating expression of the genes associated with the two compound biosynthesis (Devi et al., 2021).
β-Cyclocitral and β-ionone are another two typical compounds in cyanobacterial VOCs. Their emission amount can reach to a high level during cyanobacterial blooms in summer, e.g., β-cyclocitral and β-ionone were separately detected about 284.3 and 185.0 ng·L−1 in Lake Taihu, and about 538.12 and 50.44 ng·L−1 in Western Lake Chaohu, China (Ma et al., 2013; Jiang et al., 2016). The two compounds are derived from β-carotene degradation by oxidizing with reactive oxygen species (ROS), especially 1O2, and catalyzing with carotenoid cleavage dioxygenases (CCDs), respectively (Figure 1C) (Havaux, 2020; Zheng et al., 2020). There were three CCD homologs (NSCs) were identified in Nostoc sp. PCC 7120, of which two NSCs can cleave β-carotene at the C9=C10 double bonds to form β-ionone (Scherzinger et al., 2006). In cyanobacteria, no a CCD was found to be responsible for β-cyclocitral formation by cleaving β-carotene (Havaux, 2020; Zheng et al., 2020). Under high irradiance, an increase was found in the emission of β-cyclocitral and β-ionone from Microcystis aeruginosa (Walsh et al., 1998; Zheng et al., 2020). High irradiance elevated the 1O2 level in M. aeruginosa, which raised the emission of β-cyclocitral by oxidizing β-carotene at C7=C8 double bonds (Zheng et al., 2020).
High temperature, another typical environmental condition in summer, can also promote VOC emission from cyanobacteria (Wang and Li, 2015; Zheng et al., 2020). In contrast with 25°C, high temperature at 35°C promoted the emission of 2-methylisoborneol from Pseudanabaena sp. and geosmin from A. ucrainica (Wang and Li, 2015). This can be explained by up-regulation of the related genes in the two compound formation (Kakimoto et al., 2014; Watson et al., 2016). Lobaria pulmonaria is a symbiont of cyanobacterium Nostoc, green alga Dyctiochloropsis and fungus. Heat shock promoted the symbiont releasing carotenoid degradants as well as C6 green leaf volatiles (GLVs) that were derived from the oxidative degradation of fatty acids (García-Plazaola et al., 2017). Under the temperature at 25, 30, and 35°C, the emission amount of β-cyclocitral from M. aeruginosa gradually raised with elevating the temperature, due to the gradually increased 1O2 enhancing β-carotene oxidative degradation (Zheng et al., 2020).
There are multiple forms of N and P in eutrophicated waters, which have different effects on the VOC emission from cyanobacteria. Microcystis aeruginosa and Microcystis flos-aquae mainly released terpenoids, sulfocompounds, benzenes, furans, hydrocarbons, aldehydes, and esters. These VOC emission amount and compound types were quite different among nine N sources, with the maximum emission amount by using NH4Cl and maximum compound types by using urea (Xu et al., 2017; Zuo et al., 2018a). When the two Microcystis were supplied with sodium pyrophosphate, K2HPO4, and sodium hexametaphosphate as the sole P source, K2HPO4 and sodium hexametaphosphate separately promoted the VOC emission from M. aeruginosa and M. flos-aquae (Ye et al., 2018; Zuo et al., 2018b).
In water bodies, P is regarded as the limiting factor for cyanobacterial growth, of which concentration affects the VOC emission from cyanobacteria. The geosmin level in reservoirs was negatively related with the P concentration (Dzialowski et al., 2009). With declining the P content, the VOC emission amount from M. aeruginosa and M. flos-aquae gradually increased, and the highest emission amount was found under non-P condition (Ye et al., 2018; Zuo et al., 2018b). During 35-day cultivation, the emission amount of 2-methyl-1-butanol, 3-methyl-1-butanol, and 2-phenylethanol from M. aeruginosa gradually increased with exhausting the N nutrient, which might be caused by the raised activity of 2-keto-acid decarboxylase (Hasegawa et al., 2012). Similarly, M. aeruginosa and M. flos-aquae increased VOC emission with declining the N concentration, and the highest emission amount was detected under non-N condition, due to up-regulation of the genes in precursor formation of the VOCs, especially terpenoids and benzenes (Xu et al., 2017; Zuo et al., 2018a). These results indicate that the relative lack of nutrients resulted from cyanobacterial massive growth may promote the VOC emission. In addition, extremely high N level also improved VOC emission from Microcystis, due to the stress effects (Gan et al., 2015).
When M. aeruginosa was co-cultured with a diatom (Cyclotella meneghiniana) and two green algae (Scenedesmus quadricauda and Chlorella pyrenoidosa), it showed allelopathic effects on the growth of algae (Wang et al., 2017). In field works, a positive relationship between cyanobacterial VOCs and the seasonal succession of algal species has been found, and Microcystis finally became the dominant species (Arii et al., 2021). When Chlorella vulgaris and Chlamydomonas reinhardtii were separately treated with the VOCs from M. flos-aquae and M. aeruginosa under non-N condition, the cell growth and photosynthetic properties declined remarkably (Xu et al., 2017; Zuo et al., 2018a). Similarly, the VOCs from M. flos-aquae and M. aeruginosa under non-P condition also exhibited inhibitory effects on the cell growth and photosynthetic properties in C. vulgaris and C. reinhardtii (Ye et al., 2018; Zuo et al., 2018b). Although there are plentiful nutrients in eutrophicated waters, the massive growth of cyanobacteria may result in the relative lack of nutrients. In that case, cyanobacteria release an abundance of VOCs to inhibit other competitors and promote themselves to dominant the waters (Zuo, 2019). In addition, high irradiance and temperature also exhibit promoting effects on the VOC emission, which should contribute to the allelopathic effects of cyanobacteria for offsetting the adverse effects of environmental conditions on cyanobacterial growth.
Among cyanobacterial VOCs, some components have been identified as the allelopathic agents, e.g., geosmin, β-cyclocitral, α-ionone, β-ionone, and geranylacetone exhibited inhibitory effects on the growth of C. pyrenoidosa (Ikawa et al., 2001). Limonene and eucalyptol were two main components in Microcystis VOCs, and can inhibit the growth of C. vulgaris and C. reinhardtii by degrading photosynthetic pigments and lowering photosynthetic properties (Zhao et al., 2016; Zhou et al., 2016). In addition, 0.4 mM β-cyclocitral, 0.2 mM α-ionone, 0.2 mM β-ionone, 0.2 mM limonene, 0.4 mM longifolene, and 1.2 mM eucalyptol even directly killed C. reinhardtii by inducing programmed cell death (PCD), with appearance of some typical characteristics, such as caspase-like activation, nuclei concentration firstly following by a broken process, and DNA ladders (Sun et al., 2020; Liu J. et al., 2021; Yin et al., 2021). In exposure to 0.1–0.5 mg·ml−1 β-cyclocitral, a rupture was found in Nitzschia palea cells (Chang et al., 2011). In addition, 21.2 mg·ml−1 (110.4 mM) β-ionone and 5 mg·ml−1 (32.9 mM) β-cyclocitral even poisoned their emitter M. aeruginosa (Chang et al., 2011; Shao et al., 2011). However, this extreme high concentration is hard to reach in natural waters, and algae have been killed in that case.
Besides algae, aquatic plants are also primary producers in aquatic ecosystems, and they massively decrease and even disappear during cyanobacterial blooms, leading to a macrophytic lake changing into an algal lake (Wu et al., 2019). In Lake Müggelsee in Germany, cyanobacterial blooms resulted in the reduction of aquatic plants for approximately 90% (Körner, 2001). When submerged plants were exposed to cyanobacterial exudates and extracts, their growth was impacted by increasing ROS levels and declining photosynthetic properties (Zheng et al., 2013; Zhang et al., 2021). Recently, the allelopathic effects of β-cyclocitral and β-ionone on duckweed (Lemna turionifera) were detected. The two compounds exhibited inhibitory effects on duckweed growth and photosynthetic properties by down-regulating expression of the genes related with photosynthetic pigment synthesis, photosynthetic electron transport chain, CO2 fixation, and growth-promoting hormone synthesis (Du et al., 2022). This indicates that cyanobacterial VOCs act as signal molecules to play allelopathic roles.
Daphnia (water fleas) are dominant herbivores in waters and primarily feed eukaryotic algae rather than cyanobacteria, as cyanobacteria contain more biochemical substances (Lürling, 2003; von Elert et al., 2003). When Daphnia magna was exposed to β-cyclocitral and 2,4,7-decatrienal, it exhibited an avoidance behavior by obviously increasing the swimming rate (Watson et al., 2007). Jüttner et al. (2010) reported that Microcystis cell rupture raised β-cyclocitral emission by activating a rapid β-carotene oxidation, and then the compound served repelling function to D. magna. This model is similar with damaged higher plants releasing VOCs to repel herbivores, but the repelling signal emission is beneficial to cyanobacterial population with sacrificing single celled individual. In addition, geosmin has also exhibited a repellent activity against herbivores (Saha and Fink, 2022).
In terrestrial ecosystems, it has been well-studied in the VOC message transfer between plants (Baldwin et al., 2006; Loreto and D'Auria, 2022). This phenomenon has also been detected in green alga C. reinhardtii, indicating that it might be a retention mechanism during biological evolution (Zuo, 2019). The VOCs from C. reinhardtii undergoing PCD can induce health C. reinhardtii improving antioxidant enzyme activities for defense (Zuo et al., 2012a). The VOCs from C. reinhardtii stressed by NaCl and Na2CO3 also showed the similar inducing effects on health C. reinhardtii (Zuo et al., 2012b). During C. reinhardtii PCD triggered by wasp venom, the released NO and ethylene improved the tolerance of health C. reinhardtii cells to wasp venom (Yordanova et al., 2010). Cyanobacterial cells aggregate together and float onto the water surface to form blooms. This aggregation is important for enhancing cyanobacteria tolerance to the adverse environments, such as high irradiance, high temperature, nutrient deficiency, and herbivores (Ma et al., 2014; Zhu et al., 2014; Li et al., 2015). We have found that β-cyclocitral, limonene, longifolene, and eucalyptol can induce M. aeruginosa cell aggregation (unpublished data), indicating that the VOCs released under adverse conditions might transfer information to other cyanobacterial cells and induce them aggregating together (bursting blooms) to resist the coming stresses.
Cyanobacteria easily burst blooms in summer with high irradiance and high temperature, and their massive reproduction and growth can result in relative lack of nutrients. These adverse environmental conditions are not beneficial to cyanobacterial growth (Wang and Li, 2015; Zuo, 2019; Zheng et al., 2020), but promote cyanobacteria releasing a wide spectrum of VOCs by up-regulating related gene expression and oxidatively degrading β-carotene. These VOCs can transfer allelopathic signals to inhibit the growth of algae and aquatic plants, and even directly kill these competitors, which may offset the disadvantageous effects of the environmental conditions and promote cyanobacteria to dominate eutrophicated waters. The VOCs from cyanobacteria and the ruptured cells have repelling function on herbivores, which improves cyanobacterial population survival by reducing the risk of encountering predators. In addition, cyanobacterial VOCs might transfer aggregating information between homogeneous species, then these cells aggregate together (bursting blooms) to resist the adverse conditions (Figure 2). Therefore, cyanobacteria increase the emission of VOCs in response to the adverse environmental conditions, and these VOCs serve important functions in cyanobacterial massive reproduction and bursting blooms.
These findings in cyanobacterial VOCs provide a new thought for recognizing the formation of water blooms, but are still in a primary stage. During water blooms, how do cyanobacteria release VOCs to respond the complex environmental conditions not only a single condition? What is the exact allelopathic mechanism of cyanobacterial VOCs on the competitors? What is the exact repelling mechanism of cyanobacterial VOCs on the herbivores? How are cyanobacterial acceptor cells to identify and sense VOC aggregating information and perform an aggregation response? Among plentiful cyanobacterial VOCs, what is the exact information of each component for different acceptors? These issues should be investigated in future by using protocols in molecular biology, whose answers will promote us to deeply recognize cyanobacteria blooms from VOC perspective for preventing and controlling the blooms.
The author confirms being the sole contributor of this work and has approved it for publication.
This research was supported by the National Natural Science Foundation of China (No. 31870585).
I am grateful to Sun Xu in Zhejiang A&F University for drawing the Figure 2 for this paper.
The author declares that the research was conducted in the absence of any commercial or financial relationships that could be construed as a potential conflict of interest.
All claims expressed in this article are solely those of the authors and do not necessarily represent those of their affiliated organizations, or those of the publisher, the editors and the reviewers. Any product that may be evaluated in this article, or claim that may be made by its manufacturer, is not guaranteed or endorsed by the publisher.
Abrantes, N., Antunes, S. C., Pereira, M. J., and Gonçalves, F. (2006). Seasonal succession of cladocerans and phytoplankton and their interactions in a shallow eutrophic lake (Lake Vela, Portugal). Acta Oecol. 29, 54–64. doi: 10.1016/j.actao.2005.07.006
Arii, S., Yamashita, R., Tsuji, K., Tomita, K., Hasegawa, M., Bober, B., et al. (2021). Differences in susceptibility of cyanobacteria species to lytic volatile organic compounds and influence on seasonal succession. Chemosphere 284, 131378. doi: 10.1016/j.chemosphere.2021.131378
Baldwin, I. T., Halitschke, R., Paschold, A., Von Dahl, C. C., and Preston, C. A. (2006). Volatile signaling in plant-plant interactions: “talking trees” in the genomics era. Science 311, 812–815. doi: 10.1126/science.1118446
Beal, M. R. W., O'Reilly, B., Hietpas, K. R., and Block, P. (2021). Development of a sub-seasonal cyanobacteria prediction model by leveraging local and global scale predictors. Harmful Algae 108, 102100. doi: 10.1016/j.hal.2021.102100
Chang, D. W., Hsieh, M. L., Chen, Y. M., Lin, T. F., and Chang, J. S. (2011). Kinetics of cell lysis for Microcystis aeruginosa and Nitzschia palea in the exposure to β-cyclocitral. J. Hazard. Mater. 185, 1214–1220. doi: 10.1016/j.jhazmat.2010.10.033
Clercin, N. A., Koltsidou, I., Picard, C. J., and Druschel, G. K. (2022). Prevalence of actinobacteria in the production of 2-methylisoborneol and geosmin, over cyanobacteria in a temperate eutrophic reservoir. Chem. Eng. J. Adv. 9, 100226. doi: 10.1016/j.ceja.2021.100226
Devi, A., Chiu, Y.-T., Hsueh, H.-T., and Lin, T.-F. (2021). Quantitative PCR based detection system for cyanobacterial geosmin/2-methylisoborneol (2-MIB) events in drinking water sources: current status and challenges. Water Res. 188, 116478. doi: 10.1016/j.watres.2020.116478
Du, S., Xu, H., Yang, M., Pan, N., Zheng, T., Xu, C., et al. (2022). Toxic mechanism of two cyanobacterial volatiles β-cyclocitral and β-ionone on the photosynthesis in duckweed by altering gene expression. Environ. Pollut. 308, 119711. doi: 10.1016/j.envpol.2022.119711
Dzialowski, A. R., Smith, V. H., Huggins, D. G., deNoyelles, F., Lim, N.-C., Baker, D. S., et al. (2009). Development of predictive models for geosmin-related taste and odor in Kansas, USA, drinking water reservoirs. Water Res. 43, 2829–2840.
Gan, L., Xu, Q., Yang, W., Zhao, J., Zhou, L., and Zuo, Z. (2015). The effects of NaNO3 stress on the release of VOCs from two Microcystis. Acta Hydrobiol. Sin. 39, 782–788. doi: 10.7541/2015.102
García-Plazaola, J. I., Portillo-Estrada, M., Fernández-Marín, B., Kännaste, A., and Niinemets, Ü. (2017). Emissions of carotenoid cleavage products upon heat shock and mechanical wounding from a foliose lichen. Environ. Exp. Bot. 133, 87–97. doi: 10.1016/j.envexpbot.2016.10.004
Giglio, S., Chou, W. K., Ikeda, H., Cane, D. E., and Monis, P. T. (2011). Biosynthesis of 2-methylisoborneol in cyanobacteria. Environ. Sci. Technol. 45, 992–998. doi: 10.1021/es102992p
Hasegawa, M., Nishizawa, A., Tsuji, K., Kimura, S., and Harada, K. (2012). Volatile organic compounds derived from 2-keto-acid decarboxylase in Microcystis aeruginosa. Microbes Environ. 27, 525–528. doi: 10.1264/jsme2.ME12099
Havaux, M. (2020). β-Cyclocitral and derivatives: emerging molecular signals serving multiple biological functions. Plant Physiol. Biochem. 155, 35–41. doi: 10.1016/j.plaphy.2020.07.032
Hudnell, K. H., and Dortch, Q. (2008). “A synopsis of research needs identified at the interagency, international symposium on cyanobacterial harmful algal blooms (ISOCHAB),” in Cyanobacterial Harmful Algal Blooms: State of the Science and Research Needs, ed K. H. Hudnell (New York, NY: Springer), 17–44. doi: 10.1007/978-0-387-75865-7_2
Ikawa, M., Sasner, J. J., and Haney, J. F. (2001). Activity of cyanobacterial and algal odor compounds found in lake waters on green alga Chlorella pyrenoidosa growth. Hydrobiologia 443, 19–22. doi: 10.1023/A:1017535801766
Izaguirre, G., and Taylor, W. D. (2004). A guide to geosmin- and MIB-producing cyanobacteria in the United States. Water Sci. Technol. 49, 19–24. doi: 10.2166/wst.2004.0524
Jiang, J., He, X., and Cane, D. E. (2007). Biosynthesis of the earthy odorant geosmin by a bifunctional Streptomyces coelicolor enzyme. Nat. Chem. Biol. 3, 711–715. doi: 10.1038/nchembio.2007.29
Jiang, Y., Cheng, B., Liu, M., and Nie, Y. (2016). Spatial and temporal variations of taste and odor compounds in surface water, overlying water and sediment of the Western Lake Chaohu, China. Bull. Environ. Contam. Toxicol. 96, 186–191. doi: 10.1007/s00128-015-1698-y
Jüttner, F., Watson, S. B., von Elert, E., and Köster, O. (2010). β-Cyclocitral, a grazer defence signal unique to the cyanobacterium Microcystis. J. Chem. Ecol. 36, 1387–1397. doi: 10.1007/s10886-010-9877-0
Kakimoto, T., Yamaguchi, M., Uchimiya, H., and Kawai-Yamada, M. (2014). Culture temperature affects gene expression and metabolic pathways in the 2-methylisoborneol-producing cyanobacterium Pseudanabaena galeata. J. Plant Physiol. 171, 292–300. doi: 10.1016/j.jplph.2013.09.005
Körner, S. (2001). Development of submerged macrophytes in the shallow Lake Müggelsee (Berlin, Germany) before and after its switch to the phytoplankton dominated state. Arch. Hydrobiol. 152, 395–409. doi: 10.1127/archiv-hydrobiol/152/2001/395
Landsberg, J. H., Hendrickson, J., Tabuchi, M., Kiryu, Y., Williams, B. J., and Tomlinson, M. C. (2020). A large-scale sustained fish kill in the St. Johns River, Florida: a complex consequence of cyanobacteria blooms. Harmful Algae 92, 101771. doi: 10.1016/j.hal.2020.101771
Li, M., Nkrumah, P. N., and Peng, Q. (2015). Different tolerances to chemical contaminants between unicellular and colonial morph of Microcystis aeruginosa: excluding the differences among different strains. J. Hazard. Mater. 285, 245–249. doi: 10.1016/j.jhazmat.2014.10.064
Liu, J., Yu, Q., Ye, B., Zhu, K., Yin, J., Zheng, T., et al. (2021). Programmed cell death of Chlamydomonas reinhardtii induced by three cyanobacterial volatiles β-ionone, limonene and longifolene. Sci. Total Environ. 762, 144539. doi: 10.1016/j.scitotenv.2020.144539
Liu, M., Wu, T., Zhao, X., Zan, F., Yang, G., and Miao, Y. (2021). Cyanobacteria blooms potentially enhance volatile organic compound (VOC) emissions from a eutrophic lake: field and experimental evidence. Environ. Res. 202, 111664. doi: 10.1016/j.envres.2021.111664
Liu, X., Shi, C., Xu, X., Li, X., Xu, Y., Huang, H., et al. (2017). Spatial distributions of β-cyclocitral and β-ionone in the sediment and overlying water of the west shore of Taihu Lake. Sci. Total Environ. 579, 430–438. doi: 10.1016/j.scitotenv.2016.11.079
Loreto, F., and D'Auria, S. (2022). How do plants sense volatiles sent by other plants? Trends Plant Sci. 27, 29–38. doi: 10.1016/j.tplants.2021.08.009
Lürling, M. (2003). Daphnia growth on microcystin-producing and microcystin-free Microcystis aeruginosa in different mixtures with the green alga Scenedesmus obliquus. Limnol. Oceanogr. 48, 2214–2220. doi: 10.4319/lo.2003.48.6.2214
Ma, J., Brookes, J. D., Qin, B., Paerl, H. W., Gao, G., Wu, P., et al. (2014). Environmental factors controlling colony formation in blooms of the cyanobacteria Microcystis spp. in Lake Taihu, China. Harmful Algae 31, 136–142. doi: 10.1016/j.hal.2013.10.016
Ma, Z., Niu, Y., Xie, P., Chen, J., Tao, M., and Deng, X. (2013). Off-flavor compounds from decaying cyanobacterial blooms of Lake Taihu. J. Environ. Sci. 25, 495–501. doi: 10.1016/S1001-0742(12)60101-6
Peñuelas, J., Poulter, B., Sardans, J., Ciais, P., van der Velde, M., Bopp, L., et al. (2013). Human-induced nitrogen-phosphorus imbalances alter natural and managed ecosystems across the globe. Nat. Commun. 4, 2934. doi: 10.1038/ncomms3934
Saha, M., and Fink, P. (2022). Algal volatiles – the overlooked chemical language of aquatic primary producers. Biol. Rev. Camb. Philos. Soc. (2022) 97, 2162–2173. doi: 10.1111/brv.12887
Scherzinger, D., Ruch, S., Kloer, D. P., Wilde, A., and Al-Babili, S. (2006). Retinal is formed from apo-carotenoids in Nostoc sp. PCC7120: in vitro characterization of an apo-carotenoid oxygenase. Biochem. J. 398, 361–369. doi: 10.1042/BJ20060592
Shao, J., Xu, Y., Wang, Z., Jiang, Y., Yu, G., Peng, X., et al. (2011). Elucidating the toxicity targets of β-ionone on photosynthetic system of Microcystis aeruginosa NIES-843 (Cyanobacteria). Aquat. Toxicol. 104, 48–55. doi: 10.1016/j.aquatox.2011.03.014
Sun, Q., Zhou, M., and Zuo, Z. (2020). Toxic mechanism of eucalyptol and β-cyclocitral on Chlamydomonas reinhardtii by inducing programmed cell death. J. Hazard Mater. 389, 121910. doi: 10.1016/j.jhazmat.2019.121910
von Elert, E., Martin-Creuzburg, D., and Le Coz, J. R. (2003). Absence of sterols constrains carbon transfer between cyanobacteria and a freshwater herbivore (Daphnia galeata). Proc. Roy. Soc. Lond. Biol. Sci. 270, 1209–1214. doi: 10.1098/rspb.2003.2357
Walsh, K., Jones, G. J., and Dunstan, R. H. (1998). Effect of high irradiance and iron on volatile odour compounds in the cyanobacterium Microcystis aeruginosa. Phytochemistry 49, 1227–1239. doi: 10.1016/S0031-9422(97)00943-6
Wang, L., Zi, J., Xu, R., Hilt, S., Hou, X., and Chang, X. (2017). Allelopathic effects of Microcystis aeruginosa on green algae and a diatom: evidence from exudates addition and co-culturing. Harmful Algae 61, 56–62. doi: 10.1016/j.hal.2016.11.010
Wang, Z., and Li, R. (2015). Effects of light and temperature on the odor production of 2-methylisoborneol-producing Pseudanabaena sp. and geosmin-producing Anabaena ucrainica (cyanobacteria). Biochem. Syst. Ecol. 58, 219–226. doi: 10.1016/j.bse.2014.12.013
Watson, S. B., Jüttner, F., and Köster, O. (2007). Daphnia behavioural responses to taste and odour compounds: ecological significance and application as an inline treatment plant monitoring tool. Water Sci. Technol. 55, 23–31. doi: 10.2166/wst.2007.158
Watson, S. B., Monis, P., Baker, P., and Giglio, S. (2016). Biochemistry and genetics of taste- and odor-producing cyanobacteria. Harmful Algae 54, 112–127. doi: 10.1016/j.hal.2015.11.008
Westerhoff, P., Rodriguez-Hernandez, M., Baker, L., and Sommerfeld, M. (2005). Seasonal occurrence and degradation of 2-methylisoborneol in water supply reservoirs. Water Res. 39, 4899–4912. doi: 10.1016/j.watres.2005.06.038
Wu, Y., Huang, L. C., Wang, Y. L., Li, L., Li, G. B., Xiao, B. D., et al. (2019). Reducing the phytoplankton biomass to promote the growth of submerged macrophytes by introducing artificial aquatic plants in shallow eutrophic waters. Water 11, 1370. doi: 10.3390/w11071370
Xu, Q., Yang, L., Yang, W., Bai, Y., Hou, P., Zhao, J., et al. (2017). Volatile organic compounds released from Microcystis flos-aquae under nitrogen sources and their toxic effects on Chlorella vulgaris. Ecotoxicol. Environ. Saf. 135, 191–200. doi: 10.1016/j.ecoenv.2016.09.027
Yang, M. D., Li, X. D., Huang, J., and Yi, Y. B. (2020). Damming effects on river sulfur cycle in karst area: a case study of the Wujiang cascade reservoirs. Agric. Ecosyst. Environ. 294, 106857. doi: 10.1016/j.agee.2020.106857
Ye, C., Yang, Y., Xu, Q., Ying, B., Zhang, M., Gao, B., et al. (2018). Volatile organic compound emissions from Microcystis aeruginosa under different phosphorus sources and concentrations. Phycol. Res. 66, 15–22. doi: 10.1111/pre.12201
Yin, J., Zhu, K., Ye, B., Liu, J., Yu, Q., Xu, S., et al. (2021). Programmed cell death in Chlamydomonas reinhardtii induced by cyanobacterial volatile α-ionone. Acta Microbiol. Sin. 61, 2883–2890. doi: 10.13343/j.cnki.wsxb.20200725
Yordanova, Z. P., Iakimova, E. T., Cristescu, S. M., Harren, F. J. M., Kapchina-Toteva, V. M., and Woltering, E. J. (2010). Involvement of ethylene and nitric oxide in cell death in mastoparan-treated unicellular alga Chlamydomonas reinhardtii. Cell Biol. Int. 34, 301–308. doi: 10.1042/CBI20090138
Zhang, W., Gu, P., Zheng, X., Wang, N., Wu, H., He, J., et al. (2021). Ecological damage of submerged macrophytes by fresh cyanobacteria (FC) and cyanobacterial decomposition solution (CDS). J. Hazard Mater. 401, 123372. doi: 10.1016/j.jhazmat.2020.123372
Zhao, J., Yang, L., Zhou, L., Bai, Y., Wang, B., Hou, P., et al. (2016). Inhibitory effects of eucalyptol and limonene on the photosynthetic abilities in Chlorella vulgaris (Chlorophyceae). Phycologia 55, 696–702. doi: 10.2216/16-38.1
Zheng, G., Xu, R., Chang, X., Hilt, S., and Wu, C. (2013). Cyanobacteria can allelopathically inhibit submerged macrophytes: effects of Microcystis aeruginosa extracts and exudates on Potamogeton malaianus. Aquat. Bot. 109, 1–7. doi: 10.1016/j.aquabot.2013.02.004
Zheng, T., Zhou, M., Yang, L., Wang, Y., Wang, Y., Meng, Y., et al. (2020). Effects of high light and temperature on Microcystis aeruginosa cell growth and β-cyclocitral emission. Ecotoxicol. Environ. Saf. 192, 110313. doi: 10.1016/j.ecoenv.2020.110313
Zhou, L., Zhao, J., Xu, Q., Yang, W., and Zuo, Z. (2016). Effects of eucalyptol and limonene on the photosynthetic abilities in Chlamydomonas reinhardtii. Acta Hydrobiol. Sin. 40, 294–300.
Zhu, X., Nan, H., Zhang, L., Zhu, C., Liu, J., and Yang, Z. (2014). Does microcystin disrupt the induced effect of Daphnia kairomone on colony formation in Scenedesmus? Biochem. Syst. Ecol. 57, 169–174. doi: 10.1016/j.bse.2014.08.001
Zuo, Z. (2019). Why algae release volatile organic compounds — the emission and roles. Front. Microbiol. 10, 491. doi: 10.3389/fmicb.2019.00491
Zuo, Z., Yang, L., Chen, S., Ye, C., Han, Y., Wang, S., et al. (2018a). Effects of nitrogen nutrients on the volatile organic compound emissions from Microcystis aeruginosa. Ecotoxicol. Environ. Saf. 161, 214–220. doi: 10.1016/j.ecoenv.2018.05.095
Zuo, Z., Yang, Y., Xu, Q., Yang, W., Zhao, J., and Zhou, L. (2018b). Effects of phosphorus sources on volatile organic compound emissions from Microcystis flos-aquae and their toxic effects on Chlamydomonas reinhardtii. Environ. Geochem. Health 40, 1283–1298. doi: 10.1007/s10653-017-0055-y
Zuo, Z., Zhu, Y., Bai, Y., and Wang, Y. (2012a). Acetic acid-induced programmed cell death and release of volatile organic compounds in Chlamydomonas reinhardtii. Plant Physiol. Biochem. 51, 175–184. doi: 10.1016/j.plaphy.2011.11.003
Keywords: allelopathic effect, cyanobacterial blooms, repelling herbivore, transferring information, volatile organic compounds
Citation: Zuo Z (2023) Emission of cyanobacterial volatile organic compounds and their roles in blooms. Front. Microbiol. 14:1097712. doi: 10.3389/fmicb.2023.1097712
Received: 14 November 2022; Accepted: 06 February 2023;
Published: 20 February 2023.
Edited by:
Francesco Pomati, Swiss Federal Institute of Aquatic Science and Technology, SwitzerlandReviewed by:
Ernani Pinto, University of São Paulo, BrazilCopyright © 2023 Zuo. This is an open-access article distributed under the terms of the Creative Commons Attribution License (CC BY). The use, distribution or reproduction in other forums is permitted, provided the original author(s) and the copyright owner(s) are credited and that the original publication in this journal is cited, in accordance with accepted academic practice. No use, distribution or reproduction is permitted which does not comply with these terms.
*Correspondence: Zhaojiang Zuo, enVvemhhb2ppYW5nQDEyNi5jb20=
Disclaimer: All claims expressed in this article are solely those of the authors and do not necessarily represent those of their affiliated organizations, or those of the publisher, the editors and the reviewers. Any product that may be evaluated in this article or claim that may be made by its manufacturer is not guaranteed or endorsed by the publisher.
Research integrity at Frontiers
Learn more about the work of our research integrity team to safeguard the quality of each article we publish.