- Wuzhoufeng Agricultural Science and Technology Co., Ltd., Yantai, China
Alginate oligosaccharides (AOS) can be obtained by acidolysis and enzymatic hydrolysis. The products obtained by different methods have different structures and physiological functions. AOS have received increasing interest because of their many health-promoting properties. AOS have been reported to exert protective roles for intestinal homeostasis by modulating gut microbiota, which is closely associated with intestinal inflammation, gut barrier strength, bacterial infection, tissue injury, and biological activities. However, the roles of AOS in intestinal inflammation network remain not well understood. A review of published reports may help us to establish the linkage that AOS may improve intestinal inflammation network by affecting T helper type 1 (Th1) Th2, Th9, Th17, Th22 and regulatory T (Treg) cells, and their secreted cytokines [the hub genes of protein–protein interaction networks include interleukin-1 beta (IL-1β), IL-2, IL-4, IL-6, IL-10 and tumor necrosis factor alpha (TNF-α)] via the regulation of probiotics. The potential functional roles of molecular mechanisms are explored in this study. However, the exact mechanism for the direct interaction between AOS and probiotics or pathogenic bacteria is not yet fully understood. AOS receptors may be located on the plasma membrane of gut microbiota and will be a key solution to address such an important issue. The present paper provides a better understanding of the protecting functions of AOS on intestinal inflammation and immunity.
Introduction
The human gut contains over 100 trillion microorganisms, including gram-negative and gram-positive bacteria, archaea, bacteriophages, fungi, and protozoa (Subramanian et al., 2018; Nishida et al., 2022). Lipopolysaccharides (LPS), which are also known as lipoglycan or endotoxin, are the main components of the outer membrane of Gram-negative bacteria and induce human gut inflammation and obesity development(Du et al., 2022). Endotoxin affects the composition of the intestinal flora, destroy intestinal mucosal barrier, leads to a large increase in the reproduction and translocation of harmful bacteria, increases the serum endotoxin level, and eventually causes endotoxemia (Fuke et al., 2019). The intestinal alterations, including gut barrier dysfunction, dysbiosis, and endotoxemia, will affect intestinal homeostasis (Kühn et al., 2020). Intestinal homeostasis is critical for health, which is dependent on multifaceted interactions between the gut microbiota, the intestinal epithelium and mucosal immune system(Ahlawat et al., 2021). Maintaining the balance of gut microbiota is important to promote intestinal homeostasis(Huang P. et al., 2021).
Intestinal inflammation has been regarded as a serious, worldwide public health issue, and especially inflammatory bowel disease (IBD) is a persistent and worsening inflammatory gut disease (Khan et al., 2019). There are 10 million people globally living with IBD according to the European Federation of Crohn’s and Ulcerative Colitis Associations (EFCCA)(Zhao et al., 2021). Gut microbiota interacts with the host via metabolites, such as bile acids, short-chain fatty acids (SCFA) and tryptophan metabolites, which affect host immune development, immune homeostasis, and energy metabolism. Alterations in gut microbiota and their metabolites have been described in much work on IBD (Lavelle and Sokol, 2020). Therefore, gut microbiota imbalance is an important factor in abnormal intestinal inflammation (Lobionda et al., 2019). Fecal microbiota transplantation and probiotic intervention are promising approaches in the prevention of IBD (Dang et al., 2020). Orally administered probiotics can be beneficial to restore dysbiotic microbiota and to prevent obesity or IBD (Lavelle and Sokol, 2020). Nature products present a promising potential to treat IBD by improving the growth of probiotics in gut microbiota (Zhang N. et al., 2021).
Alginate is an active substance derived from the ocean, which is widely present in the cell walls of marine algae and is a polymer compound composed of D-mannuronic acid (M-block) and L-guluronic acid (G-block). Alginate oligosaccharides (AOS) can be obtained from alginate by acidolysis and enzymatic hydrolysis. The products obtained by different methods have different structures and different physiological functions. Alginate can be degraded into AOS with an alginate to water ratio of 1:25 (w/v) and 1.0% formic acid, and the hydrolysate water showed high antioxidant properties (Meillisa et al., 2015). Sulfuric acid hydrolysis is a typical method to prepare AOS, which can promote the growth of Nannochloropsis oculata (Park et al., 2011). Alginate is treated with trifluoroacetic acid (TFA) and used in innovative biomedical devices (Hajiali et al., 2015). Alginate lyases play a critical role to produce AOS via alginate degradation (Cheng et al., 2020). Enzymatic hydrolysis is the key method to prepare AOS with the specific polymerization degree (DPs) with certain purity and activities (Cao et al., 2021; Ming et al., 2021).
AOS have received increasing attention not only because of its low molecular weight and viscosity but also its good solubility in water, which makes them useful in medicine (Liu J. et al., 2019). AOS possess various applications in food and biomedical industries, and exert multiple health-promoting properties such as anti-inflammatory, anti-microbial, anti-oxidant, and immunomodulation (Wang et al., 2021; Zhang et al., 2023). Meanwhile, many functional oligosaccharides including AOS have been reported as prebiotics to ameliorate ulcerative colitis (UC) via the SCFAs produced from the oligosaccharide metabolized by gut microbiota (Liu et al., 2022). AOS have antibacterial infection activities and have been prepared as wound dressings to maintain a physiologically moist environment, and minimize bacterial infections (Aderibigbe and Buyana, 2018). AOS have been reported to exert protective functions for intestinal damage by regulating gut microbiota (Zhang P. et al., 2021), improving immunity (Hu et al., 2021), reducing endotoxemia (Gotteland et al., 2020), and gut inflammation (Zhang P. et al., 2020).
However, intestinal inflammatory disease, such as IBD, is often associated with inflammation networks (Friedrich et al., 2019; Zhang P. et al., 2020). The effects of AOS on the inflammation network remain widely unclear. In this review, AOS are used as the intervention substances, and the effects of AOS on gut homeostasis and inflammation are analyzed by analyzing animal gut microbiota and relevant inflammatory factors. The possible effects of AOS on gut microbiota and inflammation network are explored.
AOS modulate intestinal homeostasis via the regulation of gut microbiota
Gut microbiota consists of pathogenic bacteria and beneficial bacteria, and the balance between them will be critical to maintain gut-healthy status. Here, we tried to explore the effects of AOS on gut probiotics and pathogenic bacteria.
AOS strengthen gut health via the metabolites of probiotics.
Probiotics produce large amounts of postbiotic metabolites, which play important roles in regulating human health (Pelton, 2020). Vitamin K has been regarded as an underappreciated mediator of gut microbiota community dynamics (Ellis et al., 2021). B vitamins are responsible of crucial microbial bioactivities, metabolism and signaling. Vitamins C, E and B2 are widely reported antioxidants, which affect luminal redox balance (Pham et al., 2021). On the other hand, most gut probiotic, are capable of synthesizing vitamin K and most of B vitamins, including biotin, cobalamin, folates, nicotinic acid, pantothenic acid, pyridoxine, riboflavin, and thiamine (Figure 1A; Gu and Li, 2016). GSH is a major antioxidant and capable of eliminating ROS-caused damage to the most cells, and can be synthesized by Lactobacillus salivarius (Yuan et al., 2022). Antimicrobial peptides (AMPs) are a class of small peptides, which play a key role in the innate immune system of gut health (Zong et al., 2020). Some probiotic lactic acid bacteria produce bacteriocins (Figure 1A), a kind of small cationic peptides that kill the pathogen cells via pore formation (Tiwari, 2022). Defensins belong to cationic antimicrobial peptides, which prevent bacterial infection (Pero et al., 2019) and are critical elements of innate immunity in gut health (Chung and Raffatellu, 2019; Shulman et al., 2021). Phenyllactic acid, a product of phenylalanine catabolism, is the main bioactive metabolite produced by Saccharomyces boulardii (Fu et al., 2022). Phenyl lactic acid improves Samonella Typhimurium-induced colitis by modulating regulating the components of gut microbiota, SCFA production and inflammatory activities (Zhou et al., 2021). Volatile organic compounds (VOCs) are well-known biomarkers of gastrointestinal diseases and nutritional situation (Rondanelli et al., 2019). Bacillus amyloliquefaciens and some yeasts synthesize high level of VOCs (Ngo et al., 2020; Shruthi et al., 2022). AOS has been report to affect the most related lactic acid bacteria (Zhuge et al., 2020; Le et al., 2021; Yudiati et al., 2021), yeasts (Chávez-Falcón et al., 2022), and other probiotics (Guleria et al., 2016) under simulated gastrointestinal conditions.
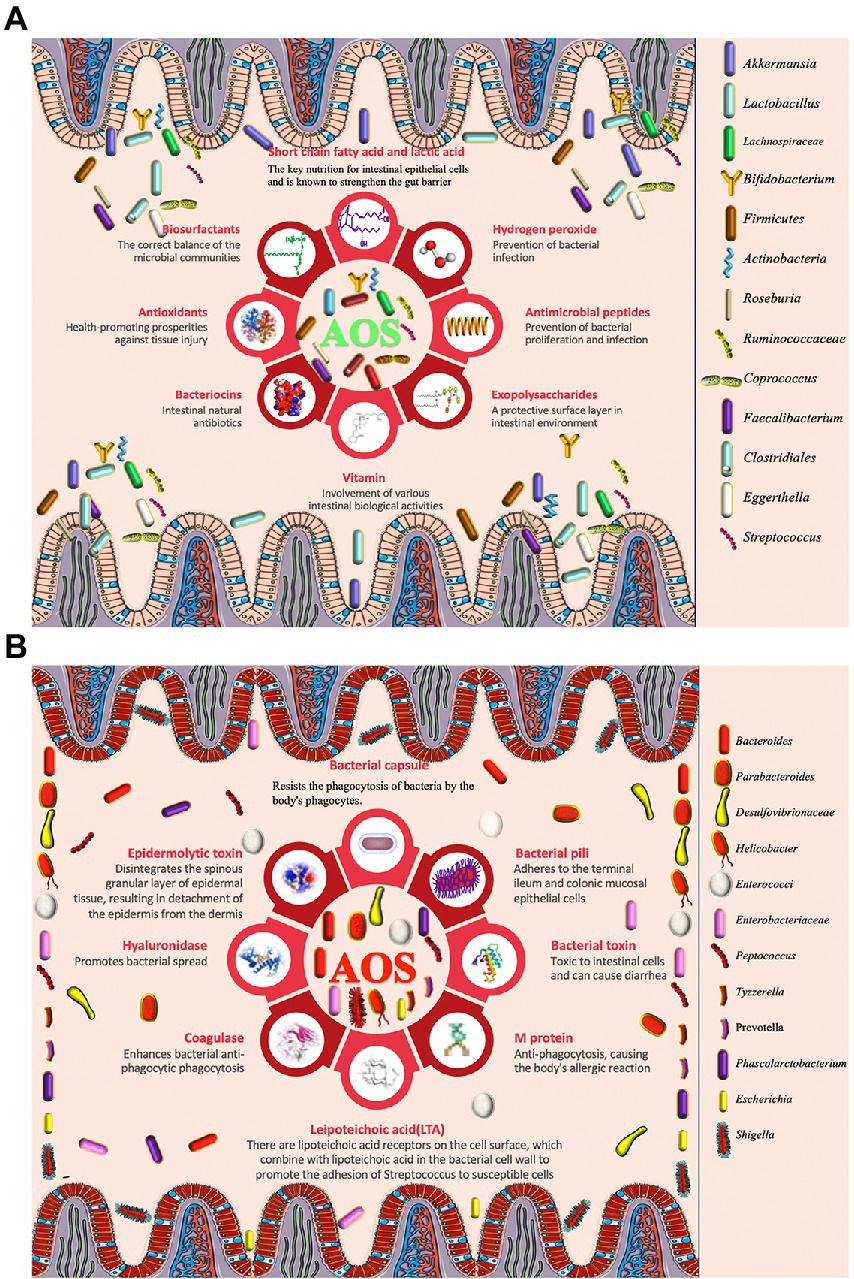
Figure 1. The effects of alginate oligosaccharides (AOS) on intestinal homeostasis via modulation of gut microbiota. (A) AOS exert protective roles for intestinal homeostasis by increasing the proportion of probiotics. (B) AOS exert protective roles for intestinal homeostasis by reducing the proportion of harmful pathogens.
Akkermansia muciniphila is considered to be favorable intestinal probiotics by improving gut metabolic activities and immune capacity (Zhang et al., 2019; Xu et al., 2020). Both probiotics Lactobacillus gasseri and Lactobacillus reuteri strains have clinically proven to have health-promoting effects by improving lipid metabolism and inflammation (De Gregorio et al., 2020; Wang et al., 2020). AOS intervention promotes the growth of A. muciniphila (Table 1), L. reuteri (Table 2), and L. gasseri (Table 2), which play important roles in the improvement of lipid metabolism (Wang et al., 2020). Meanwhile, AOS diet increases concentrations of the metabolites, such as SCFA via gut microbiota (Tables 1, 2; Mizuno et al., 2020), including acetic acid, propionic acid, and butyric acid (Wang et al., 2020). SCFAs are the main metabolites of gut microbiota, and closely associated with intestinal barrier integrity. SCFAs exert protective functions for intestinal homeostasis by strengthening gut barrier (Figure 1A; De Gregorio et al., 2020; Liu P. et al., 2021). The receptors and transporters of SCFA regulate the antibiotic long-term effects on the colonic mucosa and main the insusceptibility to experimental colitis (Holota et al., 2019). SCFAs exert protective effects on intestinal barrier function by inhibiting NOD-, LRR- and pyrin domain-containing protein 3 (NLRP3, expressed predominantly in macrophages) inflammasome and autophagy (Feng et al., 2018).
SCFA-producing bacteria include Lactobacillus (Kusumo et al., 2019), Bifidobacterium (Fang et al., 2021), Clostridiales (Gargari et al., 2018), and Lachnospiraceae species (Vacca et al., 2020). Bacteroides have been regarded as the predominant genus in the gastrointestinal tract and are associated with a higher concentration of beneficial SCFA (Fernandez-Julia et al., 2021). AOS may stimulate the growth of Bacteroides and Lachnospiraceae species (Cherry, 2020). Alginate microcapsules are reported to improve the bioactivities of Bifidobacterium species (Zhang Z. et al., 2021). Other work also shows AOS treatment increases the probiotic species Lactobacillus (Table 2) and Akkermansia species (Table 1), and reduces pathogenic species Bacteroides and Parabacteroides (Figure 1A; Table 1; Li et al., 2020). Moreover, the correlation analysis shows AOS improve gut homeostasis by increasing SCFAs production via the probiotics Roseburia, Bifidobacterium (Table 2), and Akkermansia (Table 1) in the model with fumonisin B1-induced intestinal damage (Figure 1A; Li et al., 2022). Other work indicates that AOS treatment maintains mucosal barrier function and inhibits immune injury by increasing Firmicutes and Actinobacteria and reducing Bacteroidetes species (He et al., 2021). AOS increase the proportions of SCFA probiotic producers by increasing the abundance of Ruminococcaceae, Coprococcus, Roseburia, and Faecalibacterium (Figure 1A; Table 2; Han et al., 2021). AOS has been found to reduce Salmonella colonization and promote the improvement of intestinal barrier in broiler chickens (Table 1; Yan et al., 2011). AOS ameliorate high-salt-induced intestinal injury by increasing barrier and absorption functions by increasing the abundance of Lactobacillus, Bifidobacterium, Faecalibaculum (Table 2) and Mucispirillum (Table 1; Zhang Z. et al., 2022). AOS Administration significantly upregulate the levels of IL-10 and TLR-3, and gut barrier biomarkers claudin-1 and mucin 2 (MUC2; Zhuge et al., 2020). MUC2 is a key secretory protein observed in the human intestinal system (Liu et al., 2020).
AOS improve antibacterial infection of the intestine.
Figure 1A shows AOS may improve gut microbiota by inducing probiotics producing biosurfactants, SCFA, hydrogen peroxide, antimicrobial peptides, expolysaccharides, vitamin and antioxidants so on. Biosurfactants are active compounds that are produced from cell surface and the most biosurfactants obtained from a large number of lactic acid bacteria. Probiotic biosurfactants exert beneficial biological activity on the gut microbiome and against pathogen infection via an immense antimicrobial, anti-adhesive, and antibiofilm potential (Figure 1A; Patel et al., 2021; Satria, 2022). Exopolysaccharides are the long-chain polymers of carbohydrates and can produce a protective surface layer in intestinal environment for gut microbiota (Figure 1A). Exopolysaccharide from L. rhamnosus controls dextran sulfate sodium (DSS) -induced colitis in mice by improving gut microbiota (Wan et al., 2022). Probiotics also produce hydrogen peroxide (Figure 1A), which plays a critical role in the treatment of H pylori infection (Alipour and Mofarrah, 2022; Nabavi-Rad et al., 2022).
Staphylococcus aureus can cause a wide variety of infections from skin to life-threating infections (Scolari et al., 2020). It has been widely reported that S. aureus infections are associated with intestinal symptoms, and its influence may be related to lipid raft-associated trafficking of sucrase–isomaltase and thereby may trigger secondary functional gastrointestinal diseases (Mergani et al., 2021). The film which is made of AOS shows the antimicrobial activity against two common pathogenic bacteria S. aureus and E. coli and two pathogenic fungi Aspergillus niger and Penicillium digitatum (Aloui et al., 2021). AOS films are also found to prevent S. aureus and methicillin-resistant S. epidermidis infections by inducing very high antibacterial activity against these life-threatening pathogens (Martí et al., 2019). AOS exert protective functions against enterotoxigenic E. coli-induced animal intestinal barrier injury and its infection (Wan et al., 2021).
Diarrhea is the main symptom of intestinal bacterial infection. AOS have been found to prevent diarrhea by regulating the abundance of Alloprevotella, Bacteroides, Parabacteroides and Rikenellaceae (Figure 1A; Yao et al., 2021). The probiotic mixtures with L. casei, L. bulgaricus, and Streptococcus thermophiles can prevent diarrhea in the elderly (Figure 1A; Mallina et al., 2018). There is a synergic effect between AOS and probiotics (L. bulgaricus and S. thermophilus; Yudiati et al., 2021).
Figure 1B shows that AOS also reduce the abundance of some pathogenic bacteria, which include Escherichia, Shigella, and Peptoniphilus species so on (Figure 1B; Han et al., 2021). These pathogens threaten gut health by producing bacterial toxin (Asadpoor et al., 2021a), hyaluronidase (Tomlin and Piccinini, 2018), lipoteichoic acid (Szentirmai et al., 2021), M protein(Kolesinski et al., 2022), and other structures (bacterial capsule and pili) to help them stay in gut tracts (Gupta et al., 2019). Some pathogens are closely associated with intestinal infection and diarrhea (Figure 1B; Peh et al., 2022). Bacterial toxin produced by the pathogenic species may be the main reason for causing diarrhea (Figure 1B; Dubreuil, 2019).
AOS improve antioxidant properties of the intestine.
AOS increase the abundance of Clostridium orbiscindens, Ruminococcus gnavus, Eggerthella lenta, Clostridium spp. and Clostridiales species in intestine by improving their fermentation levels (Figure 1A; An et al., 2013). Clostridium butyricum increases intestinal antioxidant properties and resistance to adverse stress (Duan et al., 2019). Gut Clostridia species display antioxidant activities by producing the antioxidants glutathione, ascorbic acid and uric acid (Million et al., 2020). The probiotics L. casei, L. bulgaricus, and Streptococcus thermophiles also show antioxidant functions in the elderly (Figure 1A; Mallina et al., 2018; Shori et al., 2022). AOS significantly repair FB1-induced intestinal damage, inflammation, and oxidative stress (including T-SOD and MDA) by increasing the probiotic abundance, such as Roseburia, Bifidobacterium, and Akkermansia, and SCFAs production (Li et al., 2022). SCFAs, such as acetic, propionic, and butyric acids, can exert health-promoting properties by increasing antioxidant activities. Acetic acid promotes the enzymatic antioxidant ability and stimulates antioxidant responses (Gurdo et al., 2018). Propionic exerts anti-inflammatory and antioxidant properties in addition to its antimycobacterial activity. It has therapeutic potential for the treatment of the patient populations driven by excessive inflammation and tissue damage (Negatu et al., 2020). Sodium butyrate is found to increase the oxidative status by activating of Nrf2-dependent signaling (Ma et al., 2018). AOS improve oxidative stress in kidney-damaged model by increasing the levels of SOD and CAT, and reducing the levels of MDA by increasing the abundance of L. johnsonii and L. reuteri.
AOS increase anti-inflammatory properties of the intestine by improving gut microbiota.
AOS also reduce the abundance of pathogenic bacteria including Escherichia, Shigella, and Peptoniphilus species (Han et al., 2021), which are closely associated with intestinal inflammation (Peh et al., 2022). AOS protect against intestinal injury by decreasing the abundance of Enterobacteriaceae, Enterococci (Wang et al., 2006), Bacteroidetes (He et al., 2021), Desulfovibrionaceae, Helicobacter, Peptococcus, and Tyzzerella in the intestine (Figure 1B; Huang J. et al., 2021). The abundance of Bacteroides is negatively associated with the amounts of inflammatory monocytes and positively linked with the levels of the metabolites in intestine (Ejima et al., 2021). Most of these species have capsules to resist bacterial phagocytosis (Figure 1B) and can induce intestinal inflammation and affect intestine permeability (Zhang Y. et al., 2020). AMP-activated protein kinase (AMPK) and NF-κB p65 are critical integrators of cytokine signals and have been observed to be reduced after AOS intervention (Tran et al., 2019; Wan et al., 2020, 2021; Huang J. et al., 2021). AOS can improve obesity-related metabolic abnormalities and inflammation. AOS intervention reverses the gut dysbiosis by increasing the relative abundance of Lactobacillus (Table 2) and Akkermansia species (Table 1) and decreasing the abundance of Bacteroides and Parabacteroides species (Table 1; Li et al., 2020b). AOS supplementary diet reduces the levels of inflammatory cytokines IL-1β and CD11c. AOS supplement ameliorates the inflammatory responses in a DSS-induced colitis model by reducing the levels of TNF-α, COX-2, IL-1β, IL-6, and increasing IL-10 level via the regulation of the abundance of Firmicutes, Actinobacteria, and Bacteroidetes (He et al., 2021). AOS reduce gut inflammation by decreasing D-Lactic acid and LPS levels, and TLR-4 and MAPK expression. Furthermore, AOS also considerably improves the abundance of Lactobacillus, Roseburia, and Lachnospiraceae) and reduce the abundance of Helicobacter, Peptococcus, and Tyzzerella (Huang J. et al., 2021).
AOS regulate intestinal inflammation network via changes of metabolites of gut microbiota
Although anti-inflammatory properties of AOS have been widely reported, the roles of AOS in intestinal inflammation networks remain widely unclear. Figure 2A shows AOS may improve intestinal inflammation cells [Th1, Th2, Th9, Th17, Th22 and regulatory T (Treg) cells] and their secreted inflammatory cytokines via SCFA, butyrate and essential metabolites produced from probiotics. These factors are closely associated with various gut inflammatory diseases or gut health. T Helper (Th) Cells (T helper type 1 (Th1; IFN-γ, IL-2, and TNF-β; Wang J. et al., 2018; Pradhan et al., 2019), Th2 (IL-4, IL-5, IL-9, IL-10, IL-13, IL-25, IL-31, and IL-33; Pradhan et al., 2019), Th9 (IL-9, IL-10, IL-21, IL-33, and IL-36; Hoeppli et al., 2019), Th17 (IL-6, IL-8, IL-17A, IL-17F, IL-21, IL-22, and IL-26; Kulkarni et al., 2018; Wang J. et al., 2018; Alrafas et al., 2019), Th22 (IL-13, IL-22, IL-26 and TNF-α; Sanaii et al., 2019; Shohan et al., 2020) and regulatory T (Treg) cells (IL-10, TGF-β and IL-35; Kulkarni et al., 2018; Alrafas et al., 2019) are involved with gut inflammation (Figure 2A). The effects of AOS on these cells or cytokines are explored. Th cell responses may be affected by the metabolites of probiotics during the prevention of gut inflammation (Figure 2A; Liu X.-J. et al., 2019; Di Gangi et al., 2020). Figure 2B shows the protein–protein interaction (PPI) networks of main inflammatory cytokines, which are also closely associated with gut inflammation and health and may be affected by AOS treatment. Among their secreted cytokines, Cytoscape analysis shows the hub genes of protein–protein interaction networks include IL-10, IL-6, IL-4, IL-2, IL-1β, and TNF-α (Figure 2B), which are closely associated with gut inflammation and health. AOS treatment enhances IL-10 secretion by affecting gut microbiota when compared with LPS treated animal models (Zhang et al., 2022a). AOS intervention decreases the levels of IL-1β, IL-6, and TNF-α, and increases the levels of IL-10 (Zhang et al., 2022a). AOS treatment affects the levels of Th1 cytokines (IL-2 and IFN-γ), and Th2 cytokines (IL-4 and IL-6; Wang W. et al., 2018). IL-10-deficiency will induce colitis in an animal model (Kang et al., 2018) while deletion of IL-6 can exacerbate colitis by inducing systemic inflammation (Ye et al., 2020). IL-1β plays an important role in the pathogenesis of IBD (Mao et al., 2018). IL-2 induces colitis by activating STAT5, which is required for optimal IL-22 production (Bauché et al., 2020). Circulating pro-inflammatory cytokine Il-4 is found to be increased in an IBD model (Zhou et al., 2019). TNF-α-producing CD4+ effector memory T cells stimulate intestinal development and regulate inflammatory responses (Schreurs et al., 2019). Anti-TNF-α therapy inhibits proinflammatory activities of mucosal neutrophils in IBD (Zhang et al., 2018).
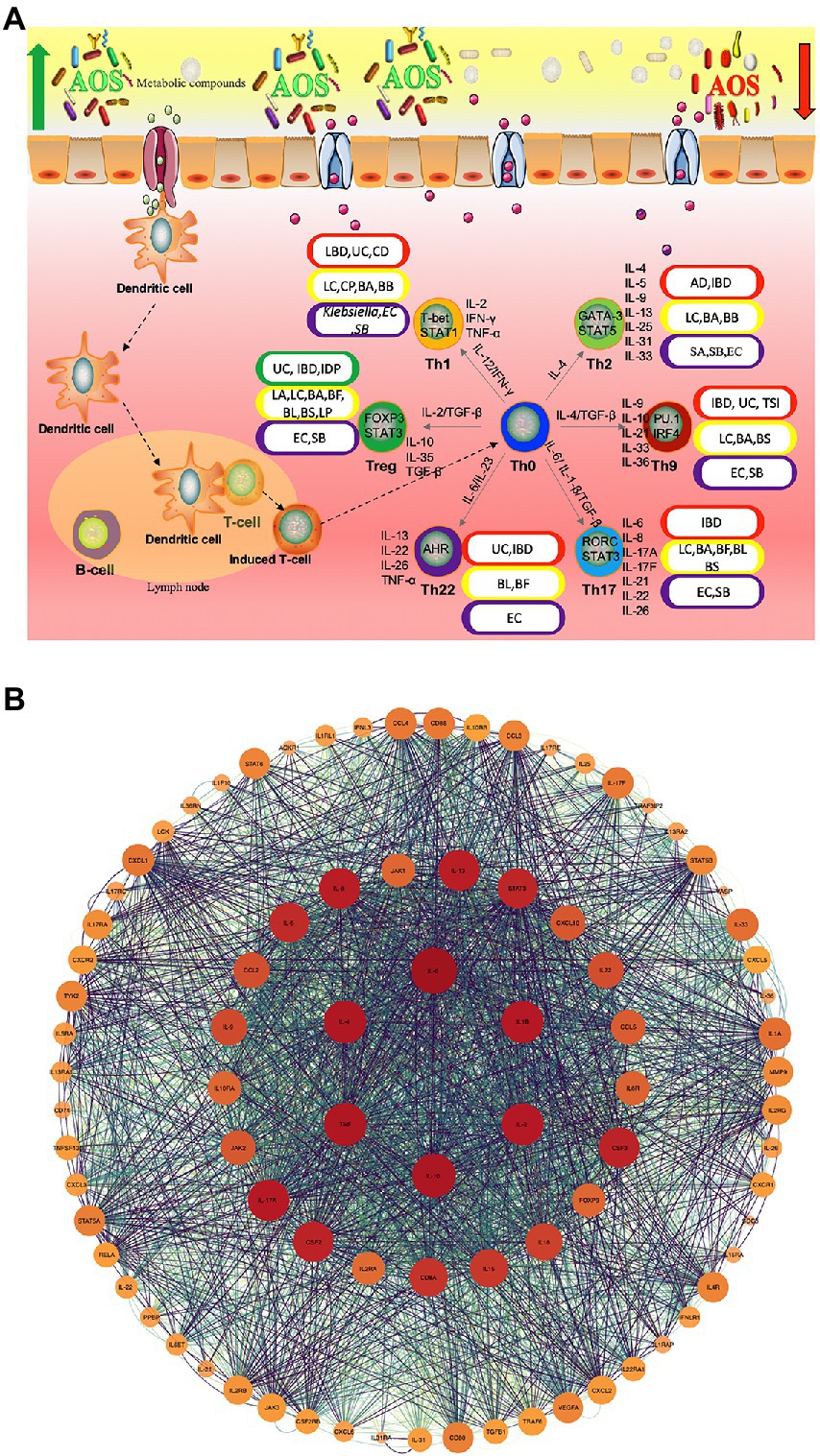
Figure 2. The roles of AOS in the inflammatory immunology process of intestine possible by increasing the beneficial metabolites of probiotics. (A) AOS may improve intestinal inflammation network by affecting T helper cells and regulatory T (Treg) cells and their secreted cytokines. Red, yellow, purple and green capsules stand for the occurrence of intestinal diseases, probiotics, pathogens and reduction in intestinal diseases because of different Th-type cell responses. (B) protein–protein interaction (PPI) networks are constructed via webservice String (Szklarczyk et al., 2021) and visualized via Cytoscape software (Doncheva et al., 2018). IBD, Inflammatory bowel disease; UC, ulcerative colitis; CD, Crohn ‘s disease; AD, allergic diarrhea; TSI, Trichinella spiralis infection; IDP, intestinal dysplastic progression; BA, Bifidobacterium adolescentis; BB, (B). breve; BS, B. spp; BL, B. longum DJO10A; BF, Bacteroides fragilis; LC, Lactobacillus casei; LC, (L). casei CRL431; LP, L. paracasei CNCMI-1518; LA, L. acidophilus; SA, Staphylococcal aureus; EC, E. coli; SB, Saccharomyces boulardii.
AOS can affect many different types of T helper cells and regulate immune-induced diseases. AOS and their derivatives have been found to reduce allergic responses by altering Th1/Th2 balance forward to Th1 cells, inhibiting IgE production, and maintaining the amounts of mast cells (Vo et al., 2015). Oral administration of β-d-mannuronic acid (the main component of AOS) down-regulates the levels of Th17 cell, IL-17 and IL-6 in patients with ankylosing spondylitis (Figure 2; Fattahi et al., 2018). AOS can significantly increase the immunosuppressive activity of Treg cells (CHEN and YAO, 2019).
AOS can increase the abundance of most Lactobacillus species in intestine. L. casei CRL431 and L. paracasei CNCMI-1518 have been found to show protective function against Salmonella typhimurium, which can cause Th1-type cell-mediated immunity by increasing IFN-γ/IL-4 ratio (Figure 2; Lemme-Dumit et al., 2021). The over-expression of IL-12p40 contributes to Th2-type inflammatory responses in the large intestine of mice with allergic diarrhea (Hino et al., 2004). IBD, including ulcerative colitis (UC) and Crohn’s disease (CD), are related to the imbalances of gut microbiota. B. adolescentis regularly treatment may improve the therapeutic effects for IBD by stimulating protective Treg/Th2 response and gut microbiota remodeling (Fan et al., 2021). Th9 cells also promote IBD risk by increasing IL-9 levels and Bifidobacterium species may be related to the prevention of IBD (Vyas and Goswami, 2018; Jakubczyk et al., 2020). The pro-inflammatory responses induced by Th1, Th2, and Th17 is also associated with IBD pathogenesis. IL-25, an IL-17 regulate Th2- and Th9-type immune responses, and IL-25 is important cytokine against T. spiralis infection (Angkasekwinai et al., 2017). AOS may also affect Th9- and Th17-type inflammatory responses by increasing the abundance of Bifidobacterium species (Figure 2; Li et al., 2022).
Th22 cells are closely associated with the severity of IBD, and may be involved in the inflammatory process of IBD (Xia and Li, 2019). Th2/Th17/Th22 responses are common to E. coli-derived vesicles but specific differences are found in Th1 and Treg cell responses (Diaz-Garrido et al., 2019). The balance of Th17/Treg cells plays an important role in the prevention of IBD progression and development (Yan et al., 2020). Lactobacillus acidophilus has been found to inhibit IBD development by regulating the balance between Th17 and Treg cells (Park et al., 2018). Bifidobacterium longum and L. plantarum alleviate allergic rhinitis in mice by revering Th2/Treg balance (Kim et al., 2019). AOS extend the viability of Lactobacillus species (Tiani et al., 2018), increase the abundance of Bifidobacterium species (Li et al., 2022), reduce the abundance of E. coli (Han et al., 2021), and may also affect Th2/Th17/Th22/Treg cell response (Figure 2). All the results suggest that there may exist a linkage between AOS and Th cells or their cytokines via the regulation of gut microbiota.
AOS show protective roles for intestinal homeostasis by increasing the abundance of probiotics (Table 1), which are linked with reducing intestinal inflammation (Zhang T. et al., 2021), improved gut barrier (Ottman et al., 2017), reduced bacterial infection (Peng et al., 2020), against tissue injury (Si et al., 2022), the balance of gut microbiota (Aabed et al., 2019), and protective surface layers (Meng et al., 2021; Figure 1A). However, the most other functions are not deeply explored in the present study. For instance, AOS show anti-adhesive properties by hampering pathogenic bacteria E. coli (Asadpoor et al., 2021b). Bacterial adhesion pili is also associated with pathogenic adhesion in intestine (Figure 1B; Shori et al., 2022) but the possible association with AOS intervention has been seldom reported. There are many great challenges to elucidate the mechanism for the effects of AOS on intestinal homeostasis and inflammation networks. The exact mechanism for the direct interaction between AOS and gut probiotics or pathogenic bacteria has not been established yet. There may be some different receptors between probiotic and pathogenic bacteria, which show different susceptibilities with AOS. Further investigation in IPEC-J2 cells found that AOS acts its function through mannose receptor signaling pathway (Zhao et al., 2020). The polysaccharide utilization loci have been found in Bacteroides species, which may include secreted glycosidases, a complement of cell surface glycan-binding proteins, oligosaccharide receptor or transporters, and a series of metabolic enzymes (Zafar and Saier, 2021). Such a receptor is expected to be discovered in some gut probiotics or gut pathogens to address the important issue.
Conclusion
AOS have caught increasing attention recently because of their antioxidant and anti-inflammatory properties. AOS also exert their numerous functions for gut homeostasis by reducing intestinal inflammation, bacterial infection, and tissue injury, increasing biological responses and improving gut barrier. AOS may affect intestinal inflammation network by regulating the levels of Th1, Th2, Th9, Th17, Th22, and regulatory T (Treg) cells, and their secreted cytokines via the increase in the proportion of probiotics. However, the mechanism for the direct interaction between AOS and probiotics or pathogenic bacteria remains unclear. Possible existence of some AOS receptors on the outer membrane of gut microbiota may provide a key clue to explore the mechanism. Much work needs to be done to address such an important issue in the future.
Author contributions
ZZ and XW were involved in the initial conceptualization of this manuscript. ZZ and FL led the literature review, writing of the first draft, and involved in the visualization of concepts. XW provided revisions and additional conceptual input into the manuscript. All authors contributed to the article and approved the submitted version.
Funding
This study received funding from Wuzhoufeng Agricultural Science and Technology Co., Ltd. The funder was not involved in the study design, collection, analysis, interpretation of data, the writing of this article, or the decision to submit it for publication.
Conflicts of interest
Authors ZZ, XW, and FL were employed by Wuzhoufeng Agricultural Science & Technology Co., Ltd.
Publisher’s note
All claims expressed in this article are solely those of the authors and do not necessarily represent those of their affiliated organizations, or those of the publisher, the editors and the reviewers. Any product that may be evaluated in this article, or claim that may be made by its manufacturer, is not guaranteed or endorsed by the publisher.
References
Aabed, K., Shafi Bhat, R., Moubayed, N., Al-Mutiri, M., Al-Marshoud, M., Al-Qahtani, A., et al. (2019). Ameliorative effect of probiotics (Lactobacillus paracaseii and Protexin(R)) and prebiotics (propolis and bee pollen) on clindamycin and propionic acid-induced oxidative stress and altered gut microbiota in a rodent model of autism. Cell. Mol. Biol. (Noisy-le-Grand) 65, 1–7. doi: 10.14715/cmb/2019.65.1.1
Aderibigbe, B. A., and Buyana, B. (2018). Alginate in wound dressings. Pharmaceutics 10:42. doi: 10.3390/pharmaceutics10020042
Ahlawat, S., Kumar, P., Mohan, H., Goyal, S., and Sharma, K. K. (2021). Inflammatory bowel disease: tri-directional relationship between microbiota, immune system and intestinal epithelium. Crit. Rev. Microbiol. 47, 254–273. doi: 10.1080/1040841X.2021.1876631
Alipour, M., and Mofarrah, R. (2022). Probiotics for treatment of helicobacter pylori infections and gastric cancer prevention. J. Genet. Resour. 8, 244–254. doi: 10.22080/JGR.2022.23949.1324
Aloui, H., Deshmukh, A. R., Khomlaem, C., and Kim, B. S. (2021). Novel composite films based on sodium alginate and gallnut extract with enhanced antioxidant, antimicrobial, barrier and mechanical properties. Food Hydrocoll. 113:106508. doi: 10.1016/j.foodhyd.2020.106508
Alrafas, H. R., Busbee, P. B., Nagarkatti, M., and Nagarkatti, P. S. (2019). Resveratrol modulates the gut microbiota to prevent murine colitis development through induction of Tregs and suppression of Th17 cells. J. Leukoc. Biol. 106, 467–480. doi: 10.1002/JLB.3A1218-476RR
An, C., Kuda, T., Yazaki, T., Takahashi, H., and Kimura, B. (2013). FLX pyrosequencing analysis of the effects of the brown-algal fermentable polysaccharides alginate and laminaran on rat cecal microbiotas. Appl. Environ. Microbiol. 79, 860–866. doi: 10.1128/AEM.02354-12
Angkasekwinai, P., Sodthawon, W., Jeerawattanawart, S., Hansakon, A., Pattanapanyasat, K., and Wang, Y.-H. (2017). ILC2s activated by IL-25 promote antigen-specific Th2 and Th9 functions that contribute to the control of Trichinella spiralis infection. PLoS One 12:e0184684. doi: 10.1371/journal.pone.0184684
Asadpoor, M., Ithakisiou, G.-N., Henricks, P. A., Pieters, R., Folkerts, G., and Braber, S. (2021a). Non-digestible oligosaccharides and short chain fatty acids as therapeutic targets against enterotoxin-producing bacteria and their toxins. Toxins 13:175. doi: 10.3390/toxins13030175
Asadpoor, M., Varasteh, S., Pieters, R. J., Folkerts, G., and Braber, S. (2021b). Differential effects of oligosaccharides on the effectiveness of ampicillin against Escherichia coli in vitro. PharmaNutrition 16:100264. doi: 10.1016/j.phanu.2021.100264
Bauché, D., Joyce-Shaikh, B., Fong, J., Villarino, A. V., Ku, K. S., Jain, R., et al. (2020). IL-23 and IL-2 activation of STAT5 is required for optimal IL-22 production in ILC3s during colitis. Sci. Immunol. 5:eaav1080. doi: 10.1126/sciimmunol.aav1080
Cao, S., Li, Q., Xu, Y., Tang, T., Ning, L., and Zhu, B. (2021). Evolving strategies for marine enzyme engineering: recent advances on the molecular modification of alginate lyase. Mar. Life Sci. Technol. 4, 106–116. doi: 10.1007/s42995-021-00122-x
Chávez-Falcón, M. S., Buitrago-Arias, C., Avila-Reyes, S. V., Solorza-Feria, J., Arenas-Ocampo, M. L., Camacho-Díaz, B. H., et al. (2022). Kinetics and mechanisms of saccharomyces boulardii release from optimized whey protein–agavin–alginate beads under simulated gastrointestinal conditions. Bioengineering 9:460. doi: 10.3390/bioengineering9090460
Chen, K.-W., and Yao, Y.-M. (2019). Effects of alginate/glycol chitosan double-network hydrogel on immune response of regulatory T cells of mice. Med. J. Chin. People’s Lib. Army 44, 91–97. doi: 10.11855/j.issn.0577-7402.2019.02.01
Cheng, D., Jiang, C., Xu, J., Liu, Z., and Mao, X. (2020). Characteristics and applications of alginate lyases: a review. Int. J. Biol. Macromol. 164, 1304–1320. doi: 10.1016/j.ijbiomac.2020.07.199
Cherry, P. (2020). Seaweeds as a Source of Non-Digestible Complex Polysaccharide Components for the Development of Novel Prebiotic Ingredients for the Functional Food Industry. Ulster: Ulster University.
Chung, L. K., and Raffatellu, M. (2019). “GI pros: antimicrobial defense in the gastrointestinal tract”. in Seminars in Cell & Developmental Biology (London, United Kingdom: Elsevier Ltd.), 129–137.
Dang, X., Xu, M., Liu, D., Zhou, D., and Yang, W. (2020). Assessing the efficacy and safety of fecal microbiota transplantation and probiotic VSL# 3 for active ulcerative colitis: a systematic review and meta-analysis. PLoS One 15:e0228846. doi: 10.1371/journal.pone.0228846
De Gregorio, P. R., Maldonado, N. C., Pingitore, E. V., Terraf, M. L., Tomás, M. J., De Ruiz, C. S., et al. (2020). Intravaginal administration of gelatine capsules containing freeze-dried autochthonous lactobacilli: a double-blind, randomised clinical trial of safety. Benefic. Microbes 11, 5–17. doi: 10.3920/BM2019.0081
Di Gangi, A., Di Cicco, M. E., Comberiati, P., and Peroni, D. G. (2020). Go with your gut: the shaping of T-cell response by gut microbiota in allergic asthma. Front. Immunol. 11:1485. doi: 10.3389/fimmu.2020.01485
Diaz-Garrido, N., Fábrega, M.-J., Vera, R., Giménez, R., Badia, J., and Baldomà, L. (2019). Membrane vesicles from the probiotic Nissle 1917 and gut resident Escherichia coli strains distinctly modulate human dendritic cells and subsequent T cell responses. J. Funct. Foods 61:103495. doi: 10.1016/j.jff.2019.103495
Doncheva, N. T., Morris, J. H., Gorodkin, J., and Jensen, L. J. (2018). Cytoscape StringApp: network analysis and visualization of proteomics data. J. Proteome Res. 18, 623–632. doi: 10.1021/acs.jproteome.8b00702
Du, L., Lei, X., Wang, J., Wang, L., Zhong, Q., Fang, X., et al. (2022). Lipopolysaccharides derived from gram-negative bacterial pool of human gut microbiota promote inflammation and obesity development. Int. Rev. Immunol. 41, 45–56. doi: 10.1080/08830185.2021.1996573
Duan, Y., Zhang, J., Huang, J., and Jiang, S. (2019). Effects of dietary Clostridium butyricum on the growth, digestive enzyme activity, antioxidant capacity, and resistance to nitrite stress of Penaeus monodon. Probiotics Antimicrob. Proteins 11, 938–945. doi: 10.1007/s12602-018-9421-z
Dubreuil, J. D. (2019). EAST1 toxin: An enigmatic molecule associated with sporadic episodes of diarrhea in humans and animals. J. Microbiol. 57, 541–549. doi: 10.1007/s12275-019-8651-4
Ejima, R., Akiyama, M., Sato, H., Tomioka, S., Yakabe, K., Kimizuka, T., et al. (2021). Seaweed dietary fiber sodium alginate suppresses the migration of colonic inflammatory monocytes and diet-induced metabolic syndrome via the gut microbiota. Nutrients 13:2812. doi: 10.3390/nu13082812
Ellis, J. L., Karl, J. P., Oliverio, A. M., Fu, X., Soares, J. W., Wolfe, B. E., et al. (2021). Dietary vitamin K is remodeled by gut microbiota and influences community composition. Gut Microbes 13, 1–16. doi: 10.1080/19490976.2021.1887721
Fan, L., Qi, Y., Qu, S., Chen, X., Li, A., Hendi, M., et al. (2021). B. adolescentis ameliorates chronic colitis by regulating Treg/Th2 response and gut microbiota remodeling. Gut Microbes 13, 1–17. doi: 10.1080/19490976.2020.1826746
Fang, Z., Li, L., Lu, W., Zhao, J., Zhang, H., Lee, Y.-K., et al. (2021). Bifidobacterium affected the correlation between gut microbial composition, SCFA metabolism, and immunity in mice with DNFB-induced atopic dermatitis. Food Biosci. 41:100648. doi: 10.1016/j.fbio.2020.100648
Fattahi, M. J., Ahmadi, H., Jafarnezhad-Ansariha, F., Mortazavi-Jahromi, S. S., Rehm, B. H., Cuzzocrea, S., et al. (2018). Oral administration effects of β-d-mannuronic acid (M2000) on Th17 and regulatory T cells in patients with ankylosing spondylitis. Biomed. Pharmacother. 100, 495–500. doi: 10.1016/j.biopha.2018.02.059
Feng, Y., Wang, Y., Wang, P., Huang, Y., and Wang, F. (2018). Short-chain fatty acids manifest stimulative and protective effects on intestinal barrier function through the inhibition of NLRP3 inflammasome and autophagy. Cell. Physiol. Biochem. 49, 190–205. doi: 10.1159/000492853
Fernandez-Julia, P. J., Munoz-Munoz, J., and Van Sinderen, D. (2021). A comprehensive review on the impact of β-glucan metabolism by Bacteroides and Bifidobacterium species as members of the gut microbiota. Int. J. Biol. Macromol. 181, 877–889. doi: 10.1016/j.ijbiomac.2021.04.069
Friedrich, M., Pohin, M., and Powrie, F. (2019). Cytokine networks in the pathophysiology of inflammatory bowel disease. Immunity 50, 992–1006. doi: 10.1016/j.immuni.2019.03.017
Fu, J., Liu, J., Wen, X., Zhang, G., Cai, J., Qiao, Z., et al. (2022). Unique probiotic properties and bioactive metabolites of saccharomyces boulardii. Probiotics Antimicrob. Proteins 1–16 [Epub ahead of print].
Fu, X., Zhan, Y., Li, N., Yu, D., Gao, W., Gu, Z., et al. (2021). Enzymatic preparation of low-molecular-weight Laminaria japonica polysaccharides and evaluation of its effect on modulating intestinal microbiota in high-fat diet-fed mice. Front. Bioeng. Biotechnol. :1438. doi: 10.3389/fbioe.2021.820892
Fuke, N., Nagata, N., Suganuma, H., and Ota, T. (2019). Regulation of gut microbiota and metabolic endotoxemia with dietary factors. Nutrients 11:2277. doi: 10.3390/nu11102277
Gargari, G., Taverniti, V., Gardana, C., Cremon, C., Canducci, F., Pagano, I., et al. (2018). Fecal Clostridiales distribution and short-chain fatty acids reflect bowel habits in irritable bowel syndrome. Environ. Microbiol. 20, 3201–3213. doi: 10.1111/1462-2920.14271
Gotteland, M., Riveros, K., Gasaly, N., Carcamo, C., Magne, F., Liabeuf, G., et al. (2020). The pros and cons of using algal polysaccharides as prebiotics. Front. Nutr. 7:163. doi: 10.3389/fnut.2020.00163
Gu, Q., and Li, P. (2016). Probiotics and Prebiotics in Human Nutrition and Health Chapter 7. Toronto: University of Toronto.
Guleria, S., Walia, A., Chauhan, A., and Shirkot, C. K. (2016). Immobilization of bacillus amyloliquefaciens SP1 and its alkaline protease in various matrices for effective hydrolysis of casein. 3 Biotech 6:208. doi: 10.1007/s13205-016-0519-2
Gupta, N., Maurya, S., Verma, H., and Verma, V. K. (2019). Unraveling the factors and mechanism involved in persistence: host-pathogen interactions in Helicobacter pylori. J. Cell. Biochem. 120, 18572–18587. doi: 10.1002/jcb.29201
Gurdo, N., Novelli Poisson, G. F., Juárez, Á., Rios De Molina, M., and Galvagno, M. A. (2018). Improved robustness of an ethanologenic yeast strain through adaptive evolution in acetic acid is associated with its enzymatic antioxidant ability. J. Appl. Microbiol. 125, 766–776. doi: 10.1111/jam.13917
Hajiali, H., Heredia-Guerrero, J. A., Liakos, I., Athanassiou, A., and Mele, E. (2015). Alginate nanofibrous mats with adjustable degradation rate for regenerative medicine. Biomacromolecules 16, 936–943. doi: 10.1021/bm501834m
Han, Z.-L., Chen, M., Fu, X.-D., Yang, M., Hrmova, M., Zhao, Y.-H., et al. (2021). Potassium alginate oligosaccharides Alter gut microbiota, and have potential to prevent the development of hypertension and heart failure in spontaneously hypertensive rats. Int. J. Mol. Sci. 22:9823. doi: 10.3390/ijms22189823
Han, Z. L., Yang, M., Fu, X. D., Chen, M., Su, Q., Zhao, Y. H., et al. (2019). Evaluation of prebiotic potential of three marine algae oligosaccharides from enzymatic hydrolysis. Mar. Drugs 17:173. doi: 10.3390/md17030173
Hao, Y., Feng, Y., Yan, X., Chen, L., Ma, X., Tang, X., et al. (2022a). Gut microbiota-testis Axis: FMT mitigates high-fat diet-diminished male fertility via improving systemic and testicular metabolome. Microbiol. Spectr. 10:e0002822. doi: 10.1128/spectrum.00028-22
Hao, Y., Feng, Y., Yan, X., Chen, L., Zhong, R., Tang, X., et al. (2022b). Gut microbiota-testis axis: FMT improves systemic and testicular micro-environment to increase semen quality in type 1 diabetes. Mol. Med. 28:45. doi: 10.1186/s10020-022-00473-w
He, N., Yang, Y., Wang, H., Liu, N., Yang, Z., and Li, S. (2021). Unsaturated alginate oligosaccharides (UAOS) protects against dextran sulfate sodium-induced colitis associated with regulation of gut microbiota. J. Funct. Foods 83:104536. doi: 10.1016/j.jff.2021.104536
Hino, A., Kweon, M.-N., Fujihashi, K., Mcghee, J. R., and Kiyono, H. (2004). Pathological role of large intestinal IL-12p40 for the induction of Th2-type allergic diarrhea. Am. J. Pathol. 164, 1327–1335. doi: 10.1016/S0002-9440(10)63219-1
Hoeppli, R., Macdonald, K., Leclair, P., Fung, V., Mojibian, M., Gillies, J., et al. (2019). Tailoring the homing capacity of human Tregs for directed migration to sites of Th1-inflammation or intestinal regions. Am. J. Transplant. 19, 62–76. doi: 10.1111/ajt.1493
Holota, Y., Dovbynchuk, T., Kaji, I., Vareniuk, I., Dzyubenko, N., Chervinska, T., et al. (2019). The long-term consequences of antibiotic therapy: role of colonic short-chain fatty acids (SCFA) system and intestinal barrier integrity. PLoS One 14:e0220642. doi: 10.1371/journal.pone.0220642.eCollection2019
Hu, J., Zhang, J., and Wu, S. (2021). The growth performance and non-specific immunity of juvenile grass carp (Ctenopharyngodon idella) affected by dietary alginate oligosaccharide. 3 Biotech 11, 1–8. doi: 10.1007/s13205-020-02589-4
Huang, J., Huang, J., Li, Y., Wang, Y., Wang, F., Qiu, X., et al. (2021). Sodium alginate modulates immunity, intestinal mucosal barrier function, and gut microbiota in cyclophosphamide-induced immunosuppressed BALB/c mice. J. Agric. Food Chem. 69, 7064–7073. doi: 10.1021/acs.jafc.1c02294
Huang, P., Jiang, A., Wang, X., Zhou, Y., Tang, W., Ren, C., et al. (2021). NMN maintains intestinal homeostasis by regulating the gut microbiota. Front. Nutr. 8:714604. doi: 10.3389/fnut.2021.714604
Jakubczyk, D., Leszczyńska, K., and Górska, S. (2020). The effectiveness of probiotics in the treatment of inflammatory bowel disease (IBD)—a critical review. Nutrients 12:1973. doi: 10.3390/nu12071973
Kang, Y., Yang, G., Zhang, S., Ross, C. F., and Zhu, M. J. (2018). Goji berry modulates gut microbiota and alleviates colitis in IL-10-deficient mice. Mol. Nutr. Food Res. 62:1800535. doi: 10.1002/mnfr.201800535
Khan, I., Ullah, N., Zha, L., Bai, Y., Khan, A., Zhao, T., et al. (2019). Alteration of gut microbiota in inflammatory bowel disease (IBD): cause or consequence? IBD treatment targeting the gut microbiome. Pathogens 8:126. doi: 10.3390/pathogens8030126
Kim, W.-G., Kang, G.-D., Kim, H., Han, M., and Kim, D.-H. (2019). Bifidobacterium longum IM55 and lactobacillus plantarum IM76 alleviate allergic rhinitis in mice by restoring Th2/Treg imbalance and gut microbiota disturbance. Benefic. Microbes 10, 55–67. doi: 10.3920/BM2017.0146
Kolesinski, P., Wang, K.-C., Hirose, Y., Nizet, V., and Ghosh, P. (2022). An M protein coiled coil unfurls and exposes its hydrophobic core to capture LL-37. bioRxiv [Preprint].
Kühn, F., Adiliaghdam, F., Cavallaro, P. M., Hamarneh, S. R., Tsurumi, A., Hoda, R. S., et al. (2020). Intestinal alkaline phosphatase targets the gut barrier to prevent aging. JCI Insight 5:e134049. doi: 10.1172/jci.insight.134049
Kulkarni, N., Sonar, S. A., and Lal, G. (2018). Plasticity of Th17 and Tregs and its clinical importance as therapeutic target in inflammatory bowel disease. Indian J. Inflamm. Res. 1, 1–17. doi: 10.15305/ijir/v1i1/258
Kusumo, P. D., Maulahela, H., Utari, A. P., Surono, I. S., Soebandrio, A., and Abdullah, M. (2019). Probiotic lactobacillus plantarum IS 10506 supplementation increase SCFA of women with functional constipation. Iranian J. Microbiol. 11:389.
Lavelle, A., and Sokol, H. (2020). Gut microbiota-derived metabolites as key actors in inflammatory bowel disease. Nat. Rev. Gastroenterol. Hepatol. 17, 223–237. doi: 10.1038/s41575-019-0258-z
Le, N. T. M., Ae-Young, M., Choi, Y. N., Sang-Hoon, H., and Seung-Moon, P. (2021). Comparison of gut microbiota from Jeju black-swine fed different feed additives containing Rhodobacter sphaeroides. Lactobacillus Alginate Oligosaccharide. 한국생물공학회 학술대회 :352.
Lemme-Dumit, J. M., Cazorla, S. I., Perdigón, G. D. V., and Maldonado-Galdeano, C. (2021). Probiotic bacteria and their cell walls induce Th1-type immunity against salmonella typhimurium challenge. Front. Immunol. 12:1770. doi: 10.3389/fimmu.2021.660854
Li, T., Huang, S., Wang, J., Yin, P., Liu, H., and Sun, C. (2022). Alginate oligosaccharides protect against fumonisin B1-induced intestinal damage via promoting gut microbiota homeostasis. Food Res. Int. 152:110927. doi: 10.1016/j.foodres.2021.110927
Li, S., Wang, L., Liu, B., and He, N. (2020). Unsaturated alginate oligosaccharides attenuated obesity-related metabolic abnormalities by modulating gut microbiota in high-fat-diet mice. Food Funct. 11, 4773–4784. doi: 10.1039/C9FO02857A
Liu, H., Cai, Z., Wang, F., Hong, L., Deng, L., Zhong, J., et al. (2021). Colon-targeted adhesive hydrogel microsphere for regulation of gut immunity and Flora. Adv Sci (Weinh) 8:e2101619. doi: 10.1002/advs.202101619
Liu, P., Wang, Y., Yang, G., Zhang, Q., Meng, L., Xin, Y., et al. (2021). The role of short-chain fatty acids in intestinal barrier function, inflammation, oxidative stress, and colonic carcinogenesis. Pharmacol. Res. 165:105420. doi: 10.1016/j.phrs.2021.105420
Liu, N., Wang, H., Yang, Z., Zhao, K., Li, S., and He, N. (2022). The role of functional oligosaccharides as prebiotics on ulcerative colitis. Food Funct. doi: 10.1039/d2fo00546h
Liu, J., Wu, S., Cheng, Y., Liu, Q., Su, L., Yang, Y., et al. (2021). Sargassum fusiforme alginate relieves hyperglycemia and modulates intestinal microbiota and metabolites in type 2 diabetic mice. Nutrients 13:2887. doi: 10.3390/nu13082887
Liu, J., Yang, S., Li, X., Yan, Q., Reaney, M. J., and Jiang, Z. (2019). Alginate oligosaccharides: production, biological activities, and potential applications. Compr. Rev. Food Sci. Food Saf. 18, 1859–1881. doi: 10.1111/1541-4337.12494
Liu, Y., Yu, X., Zhao, J., Zhang, H., Zhai, Q., and Chen, W. (2020). The role of MUC2 mucin in intestinal homeostasis and the impact of dietary components on MUC2 expression. Int. J. Biol. Macromol. 164, 884–891. doi: 10.1016/j.ijbiomac.2020.07.191
Liu, X.-J., Yu, R., and Zou, K.-F. (2019). Probiotic mixture VSL# 3 alleviates dextran sulfate sodium-induced colitis in mice by downregulating T follicular helper cells. Curr. Med. Sci. 39, 371–378. doi: 10.1007/s11596-019-2045-z
Lobionda, S., Sittipo, P., Kwon, H. Y., and Lee, Y. K. (2019). The role of gut microbiota in intestinal inflammation with respect to diet and extrinsic stressors. Microorganisms 7:271. doi: 10.3390/microorganisms7080271
Ma, N., Abaker, J. A., Bilal, M. S., Dai, H., and Shen, X. (2018). Sodium butyrate improves antioxidant stability in sub-acute ruminal acidosis in dairy goats. BMC Vet. Res. 14, 1–13. doi: 10.1186/s12917-018-1591-0
Mallina, R., Craik, J., Briffa, N., Ahluwalia, V., Clarke, J., and Cobb, A. (2018). Probiotic containing lactobacillus casei, Lactobacillus bulgaricus, and streptococcus thermophiles (ACTIMEL) for the prevention of Clostridium difficile associated diarrhoea in the elderly with proximal femur fractures. J. Infect. Public Health 11, 85–88. doi: 10.1016/j.jiph.2017.04.001
Mao, L., Kitani, A., Strober, W., and Fuss, I. J. (2018). The role of NLRP3 and IL-1β in the pathogenesis of inflammatory bowel disease. Front. Immunol. 9:2566. doi: 10.3389/fimmu.2018.02566
Martí, M., Frigols, B., Salesa, B., and Serrano-Aroca, Á. (2019). Calcium alginate/graphene oxide films: reinforced composites able to prevent Staphylococcus aureus and methicillin-resistant Staphylococcus epidermidis infections with no cytotoxicity for human keratinocyte HaCaT cells. Eur. Polym. J. 110, 14–21. doi: 10.1016/j.eurpolymj.2018.11.012
Meillisa, A., Woo, H.-C., and Chun, B.-S. (2015). Production of monosaccharides and bio-active compounds derived from marine polysaccharides using subcritical water hydrolysis. Food Chem. 171, 70–77. doi: 10.1016/j.foodchem.2014.08.097
Meng, J., Wang, Y. Y., and Hao, Y. P. (2021). Protective function of surface layer protein from lactobacillus casei fb05 against intestinal pathogens in vitro. Biochem. Biophys. Res. Commun. 546, 15–20. doi: 10.1016/j.bbrc.2021.01.101
Mergani, A., Wanes, D., Schecker, N., Branitzki-Heinemann, K., Naim, H. Y., and Von Köckritz-Blickwede, M. (2021). Staphylococcus aureus infection influences the function of intestinal cells by altering the lipid raft-dependent sorting of Sucrase-Isomaltase. Front. Cell Dev. Biol. 9:699970. doi: 10.3389/fcell.2021.699970
Million, M., Armstrong, N., Khelaifia, S., Guilhot, E., Richez, M., Lagier, J.-C., et al. (2020). The antioxidants glutathione, ascorbic acid and uric acid maintain butyrate production by human gut clostridia in the presence of oxygen in vitro. Sci. Rep. 10, 1–11. doi: 10.1038/s41598-020-64834-3
Ming, L., Lei, L., Zhang, H.-F., Bao, Y., and Everaert, N. (2021). Alginate oligosaccharides preparation, biological activities and their application in livestock and poultry. J. Integr. Agric. 20, 24–34. doi: 10.1016/S2095-3119(20)63195-1
Mizuno, H., Bamba, S., Abe, N., and Sasaki, M. (2020). Effects of an alginate-containing variable-viscosity enteral nutrition formula on defecation, intestinal microbiota, and short-chain fatty acid production. J. Funct. Foods 67:103852. doi: 10.1016/j.jff.2020.103852
Nabavi-Rad, A., Sadeghi, A., Asadzadeh Aghdaei, H., Yadegar, A., Smith, S. M., and Zali, M. R. (2022). The double-edged sword of probiotic supplementation on gut microbiota structure in helicobacter pylori management. Gut Microbes 14:2108655. doi: 10.1080/19490976.2022.2108655
Negatu, D. A., Gengenbacher, M., Dartois, V., and Dick, T. (2020). Indole propionic acid, an unusual antibiotic produced by the gut microbiota, with anti-inflammatory and antioxidant properties. Front. Microbiol. 11:575586. doi: 10.3389/fmicb.2020.575586
Ngo, T. T., Bang, N. N., Dart, P., Callaghan, M., Klieve, A., Hayes, B., et al. (2020). Feed preference response of weaner bull calves to bacillus amyloliquefaciens H57 probiotic and associated volatile organic compounds in high concentrate feed pellets. Animals 11:51. doi: 10.3390/ani11010051
Nishida, A., Nishino, K., Ohno, M., Sakai, K., Owaki, Y., Noda, Y., et al. (2022). Update on gut microbiota in gastrointestinal diseases. World J. Clin. Cases 10, 7653–7664. doi: 10.12998/wjcc.v10.i22.7653
Ottman, N., Reunanen, J., Meijerink, M., Pietila, T. E., Kainulainen, V., Klievink, J., et al. (2017). Pili-like proteins of Akkermansia muciniphila modulate host immune responses and gut barrier function. PLoS One 12:e0173004. doi: 10.1371/journal.pone.0173004
Park, J.-S., Choi, J. W., Jhun, J., Kwon, J. Y., Lee, B.-I., Yang, C. W., et al. (2018). Lactobacillus acidophilus improves intestinal inflammation in an acute colitis mouse model by regulation of Th17 and Treg cell balance and fibrosis development. J. Med. Food 21, 215–224. doi: 10.1089/jmf.2017.3990
Park, H.-J., Kim, Y.-H., and Lee, J.-H. (2011). Effect of alginate on the growth of Nannochloropsis oculata NIES-2145. KSBB J. 26, 206–210. doi: 10.7841/ksbbj.2011.26.3.206
Patel, M., Siddiqui, A. J., Hamadou, W. S., Surti, M., Awadelkareem, A. M., Ashraf, S. A., et al. (2021). Inhibition of bacterial adhesion and antibiofilm activities of a glycolipid biosurfactant from lactobacillus rhamnosus with its physicochemical and functional properties. Antibiotics 10:1546. doi: 10.3390/antibiotics10121546
Peh, A., O’donnell, J. A., Broughton, B. R., and Marques, F. Z. (2022). Gut microbiota and their metabolites in stroke: a double-edged sword. Stroke 53:036800, 1788–1801. doi: 10.1161/STROKEAHA.121.036800
Pelton, R. (2020). Postbiotic metabolites: how probiotics regulate health. Integr. Med. Clin. J. 19:25.
Peng, M., Tabashsum, Z., Patel, P., Bernhardt, C., Biswas, C., Meng, J., et al. (2020). Prevention of enteric bacterial infections and modulation of gut microbiota with conjugated linoleic acids producing lactobacillus in mice. Gut Microbes 11, 433–452. doi: 10.1080/19490976.2019.1638724
Pero, R., Brancaccio, M., Laneri, S., De Biasi, M.-G., Lombardo, B., and Scudiero, O. (2019). A novel view of human helicobacter pylori infections: interplay between microbiota and beta-defensins. Biomol. Ther. 9:237. doi: 10.3390/biom9060237
Pham, V. T., Dold, S., Rehman, A., Bird, J. K., and Steinert, R. E. (2021). Vitamins, the gut microbiome and gastrointestinal health in humans. Nutr. Res. 95, 35–53. doi: 10.1016/j.nutres.2021.09.001
Pradhan, B., Guha, D., Naik, A. K., Banerjee, A., Tambat, S., Chawla, S., et al. (2019). Probiotics L. acidophilus and B. clausii modulate gut microbiota in Th1-and Th2-biased mice to ameliorate salmonella typhimurium-induced diarrhea. Probiotics Antimicrob. Proteins 11, 887–904. doi: 10.1007/s12602-018-9436-5
Rondanelli, M., Perdoni, F., Infantino, V., Faliva, M. A., Peroni, G., Iannello, G., et al. (2019). Volatile organic compounds as biomarkers of gastrointestinal diseases and nutritional status. J. Anal. Methods Chem. 2019, 1–14. doi: 10.1155/2019/7247802
Sanaii, A., Shirzad, H., Haghighian, M., Rahimian, G., Soltani, A., Shafigh, M., et al. (2019). Role of Th22 cells in helicobacter pylori-related gastritis and peptic ulcer diseases. Mol. Biol. Rep. 46, 5703–5712. doi: 10.1007/s11033-022-07470-6
Satria, A. H. B. M. (2022). Isolation and characterization of biosurfactant-producing lactic acid bacteria from sauerkraut. Enhanced Knowl. Sci. Technol. 2, 060–069.
Schreurs, R. R., Baumdick, M. E., Sagebiel, A. F., Kaufmann, M., Mokry, M., Klarenbeek, P. L., et al. (2019). Human fetal TNF-α-cytokine-producing CD4+ effector memory T cells promote intestinal development and mediate inflammation early in life. Immunity 50:e468. doi: 10.1016/j.immuni.2018.12.010
Scolari, I. R., Páez, P. L., Musri, M. M., Petiti, J. P., Torres, A., and Granero, G. E. (2020). Rifampicin loaded in alginate/chitosan nanoparticles as a promising pulmonary carrier against Staphylococcus aureus. Drug Deliv. Transl. Res. 10, 1403–1417. doi: 10.1007/s13346-019-00705-3
Shohan, M., Dehghani, R., Khodadadi, A., Dehnavi, S., Ahmadi, R., Joudaki, N., et al. (2020). Interleukin-22 and intestinal homeostasis: protective or destructive? IUBMB Life 72, 1585–1602. doi: 10.1002/iub.229
Shori, A. B., Albalawi, A., Al Zahrani, A. J., Al-Sulbi, O. S., and Baba, A. S. (2022). Microbial analysis, antioxidant activity, and sensory properties of yoghurt with different starter cultures during storage. Int. Dairy J. 126:105267. doi: 10.1016/j.idairyj.2021.105267
Shruthi, B., Deepa, N., Somashekaraiah, R., Adithi, G., Divyashree, S., and Sreenivasa, M. (2022). Exploring biotechnological and functional characteristics of probiotic yeasts: a review. Biotechnol. Rep. 34:e00716. doi: 10.1016/j.btre.2022.e00716
Shulman, R. J., Devaraj, S., and Heitkemper, M. (2021). Activation of the innate immune system in children with irritable bowel syndrome evidenced by increased fecal human β-defensin-2. Clin. Gastroenterol. Hepatol. 19, 2121–2127. doi: 10.1016/j.cgh.2020.09.034
Si, X., Jia, H., Liu, N., Li, J., Pan, L., Wang, J., et al. (2022). Alpha-ketoglutarate attenuates colitis in mice by increasing lactobacillus abundance and regulating stem cell proliferation via Wnt-hippo signaling. Mol. Nutr. Food Res. 66:e2100955. doi: 10.1002/mnfr.202100955
Subramanian, B., Balakrishnan, S., Seshadri, K. G., and Valeriote, F. A. (2018). Insights into the human gut microbiome—a review. J. Basic Clin. Appl. Health Sci 1, 103–110. doi: 10.5005/jp-journals-10082-01133
Szentirmai, É., Massie, A. R., and Kapás, L. (2021). Lipoteichoic acid, a cell wall component of gram-positive bacteria, induces sleep and fever and suppresses feeding. Brain Behav. Immun. 92, 184–192. doi: 10.1016/j.bbi.2020.12.008
Szklarczyk, D., Gable, A. L., Nastou, K. C., Lyon, D., Kirsch, R., Pyysalo, S., et al. (2021). The STRING database in 2021: customizable protein–protein networks, and functional characterization of user-uploaded gene/measurement sets. Nucleic Acids Res. 49, D605–D612. doi: 10.1093/nar/gkaa1074
Takei, M. N., Kuda, T., Taniguchi, M., Nakamura, S., Hajime, T., and Kimura, B. (2020). Detection and isolation of low molecular weight alginate- and laminaran-susceptible gut indigenous bacteria from ICR mice. Carbohydr. Polym. 238:116205. doi: 10.1016/j.carbpol.2020.116205
Tiani, K. A., Yeung, T. W., Mcclements, D. J., and Sela, D. A. (2018). Extending viability of lactobacillus plantarum and Lactobacillus johnsonii by microencapsulation in alginate microgels. Int. J. Food Sci. Nutr. 69, 155–164. doi: 10.1080/09637486.2017.1343285
Tiwari, S. K. (2022). Bacteriocin-producing probiotic lactic acid bacteria in controlling Dysbiosis of the gut microbiota. Front. Cell. Infect. Microbiol. 12:851140. doi: 10.3389/fcimb.2022.851140
Tomlin, H., and Piccinini, A. M. (2018). A complex interplay between the extracellular matrix and the innate immune response to microbial pathogens. Immunology 155, 186–201. doi: 10.1111/imm.12972
Tran, V. C., Cho, S.-Y., Kwon, J., and Kim, D. (2019). Alginate oligosaccharide (AOS) improves immuno-metabolic systems by inhibiting STOML2 overexpression in high-fat-diet-induced obese zebrafish. Food Funct. 10, 4636–4648. doi: 10.1039/c9fo00982e
Vacca, M., Celano, G., Calabrese, F. M., Portincasa, P., Gobbetti, M., and De Angelis, M. (2020). The controversial role of human gut lachnospiraceae. Microorganisms 8:573. doi: 10.3390/microorganisms8040573
Vo, T. S., Ngo, D. H., Kang, K. H., Jung, W. K., and Kim, S. K. (2015). The beneficial properties of marine polysaccharides in alleviation of allergic responses. Mol. Nutr. Food Res. 59, 129–138. doi: 10.1002/mnfr.201400412
Vyas, S. P., and Goswami, R. (2018). A decade of Th9 cells: role of Th9 cells in inflammatory bowel disease. Front. Immunol. 9:1139. doi: 10.1002/mnfr.201400412
Wan, C., Qian, W. W., Liu, W., Pi, X., Tang, M. T., Wang, X. L., et al. (2022). Exopolysaccharide from lactobacillus rhamnosus ZFM231 alleviates DSS-induced colitis in mice by regulating gut microbiota. J. Sci. Food Agric. 102, 7087–7097. doi: 10.1002/jsfa.12070
Wan, J., Zhang, J., Chen, D., Yu, B., Huang, Z., Mao, X., et al. (2020). Alterations in intestinal microbiota by alginate oligosaccharide improve intestinal barrier integrity in weaned pigs. J. Funct. Foods 71:104040. doi: 10.1016/j.jff.2020.104040
Wan, J., Zhang, J., Xu, Q., Yin, H., Chen, D., Yu, B., et al. (2021). Alginate oligosaccharide protects against enterotoxigenic Escherichia coli-induced porcine intestinal barrier injury. Carbohydr. Polym. 270:118316 doi: 10.1016/j.carbpol.2021.118316
Wang, M., Chen, L., and Zhang, Z. (2021). Potential applications of alginate oligosaccharides for biomedicine–a mini review. Carbohydr. Polym. 271:118408. doi: 10.1016/j.carbpol.2021.118408
Wang, J., Goepfert, C., Mueller, N., Piersigilli, A., Lin, R., Wen, H., et al. (2018). Larval Echinococcus multilocularis infection reduces dextran sulphate sodium-induced colitis in mice by attenuating T helper type 1/type 17-mediated immune reactions. Immunology 154, 76–88. doi: 10.1111/imm.12860
Wang, Y., Han, F., Hu, B., Li, J., and Yu, W. (2006). In vivo prebiotic properties of alginate oligosaccharides prepared through enzymatic hydrolysis of alginate. Nutr. Res. 26, 597–603. doi: 10.1016/j.nutres.2006.09.015
Wang, W., Jing, W., and Liu, Q. (2018). Astragalus oral solution ameliorates allergic asthma in children by regulating relative contents of CD4+ CD25highCD127low Treg cells. Front. Pediatr. 6:255. doi: 10.3389/fped.2018.00255
Wang, Y., Li, L., Ye, C., Yuan, J., and Qin, S. (2020). Alginate oligosaccharide improves lipid metabolism and inflammation by modulating gut microbiota in high-fat diet fed mice. Appl. Microbiol. Biotechnol. 104, 3541–3554. doi: 10.1007/s00253-020-10449-7
Xia, L., and Li, L. (2019). Changes and clinical significance of levels of Th22 cell subsets and IL-22 in patients with inflammatory bowel disease. Zhongguo Weishengtaxixue Zazhi/Chinese Journal of Microecology 31, 1203–1206.
Xu, Y., Wang, N., Tan, H.-Y., Li, S., Zhang, C., and Feng, Y. (2020). Function of Akkermansia muciniphila in obesity: interactions with lipid metabolism, immune response and gut systems. Front. Microbiol. 11:219. doi: 10.3389/fmicb.2020.00219
Yan, G., Guo, Y., Yuan, J., Liu, D., and Zhang, B. (2011). Sodium alginate oligosaccharides from brown algae inhibit salmonella Enteritidis colonization in broiler chickens. Poult. Sci. 90, 1441–1448. doi: 10.3382/ps.2011-01364
Yan, J.-B., Luo, M.-M., Chen, Z.-Y., and He, B.-H. (2020). The function and role of the Th17/Treg cell balance in inflammatory bowel disease. J. Immunol. Res. 2020, 1–8. doi: 10.1155/2020/8813558
Yao, L., Yang, P., Lin, Y., Bi, D., Yu, B., Lin, Z., et al. (2021). The regulatory effect of alginate on ovalbumin-induced gut microbiota disorders. J. Funct. Foods 86:104727. doi: 10.1016/j.jff.2021.104727
Ye, M., Joosse, M. E., Liu, L., Sun, Y., Dong, Y., Cai, C., et al. (2020). Deletion of IL-6 exacerbates colitis and induces systemic inflammation in IL-10-deficient mice. J. Crohn’s Colitis 14, 831–840. doi: 10.1093/ecco-jcc/jjz176
Yu, X., Zou, L. F., Xiong, J. H., Pan, J. Z., Li, P. J., and Chen, C. G. (2022). Effects of different ionic polysaccharides in cooked lean pork batters on intestinal health in mice. Foods 11:1372. doi: 10.3390/foods11101372
Yuan, Y., Yang, J., Zhuge, A., Li, L., and Ni, S. (2022). Gut microbiota modulates osteoclast glutathione synthesis and mitochondrial biogenesis in mice subjected to ovariectomy. Cell Prolif. 55:e13194.
Yudiati, E., Wijayanti, D., Azhar, N., Chairunnisa, A., Sedjati, S., and Arifin, Z. (2021). “Alginate oligosaccharide/polysaccharide and lactic acid bacteria (Lactobacillus bulgaricus FNCC–0041 & Streptococcus thermophilus FNCC–0040) as immunostimulants against pathogenic Vibrio spp. using Artemia bio model”, in IOP Conference Series: Earth and Environmental Science: IOP Publishing), 012060.
Zafar, H., and Saier, M. H. Jr. (2021). Gut Bacteroides species in health and disease. Gut Microbes 13, 1–20. doi: 10.1080/19490976.2020.1848158
Zhang, Y., Chen, L., Hu, M., Kim, J. J., Lin, R., Xu, J., et al. (2020). Dietary type 2 resistant starch improves systemic inflammation and intestinal permeability by modulating microbiota and metabolites in aged mice on high-fat diet. Aging (Albany NY) 12:9173. doi: 10.18632/aging.103187
Zhang, P., Feng, Y., Li, L., Ge, W., Yu, S., Hao, Y., et al. (2021). Improvement in sperm quality and spermatogenesis following faecal microbiota transplantation from alginate oligosaccharide dosed mice. Gut 70, 222–225. doi: 10.1136/gutjnl-2020-320992
Zhang, Z., Gu, M., You, X., Sela, D. A., Xiao, H., and Mcclements, D. J. (2021). Encapsulation of bifidobacterium in alginate microgels improves viability and targeted gut release. Food Hydrocoll. 116:106634. doi: 10.1016/j.foodhyd.2021.106634
Zhang, Y., Guo, C., Li, Y., Han, X., Luo, X., Chen, L., et al. (2022a). Alginate oligosaccharides ameliorate DSS-induced colitis through modulation of AMPK/NF-κB pathway and intestinal microbiota. Nutrients 14:2864. doi: 10.3390/nu14142864
Zhang, T., Ji, X., Lu, G., and Zhang, F. (2021). The potential of Akkermansia muciniphila in inflammatory bowel disease. Appl. Microbiol. Biotechnol. 105, 5785–5794.
Zhang, P., Jia, J., Jiang, P., Zheng, W., Li, X., Song, S., et al. (2022). Polysaccharides from edible brown seaweed Undaria pinnatifida are effective against high-fat diet-induced obesity in mice through the modulation of intestinal microecology. Food Funct. 13, 2581–2593. doi: 10.1039/d1fo04012j
Zhang, N., Jin, M., Wang, K., Zhang, Z., Shah, N. P., and Wei, H. (2021). Functional oligosaccharide fermentation in the gut: improving intestinal health and its determinant factors: A review. Carbohydr. Polym. 119043.
Zhang, T., Li, Q., Cheng, L., Buch, H., and Zhang, F. (2019). Akkermansia muciniphila is a promising probiotic. Microb. Biotechnol. 12, 1109–1125. doi: 10.1111/1751-7915.13410
Zhang, C., Li, M., Rauf, A., Khalil, A. A., Shan, Z., Chen, C., et al. (2023). Process and applications of alginate oligosaccharides with emphasis on health beneficial perspectives. Crit. Rev. Food Sci. Nutr. 63, 303–329. doi: 10.1080/10408398.2021.1946008
Zhang, Z., Liu, J., Li, M., Yang, B., Liu, W., Chu, Z., et al. (2022). Lactobacillus rhamnosus encapsulated in alginate/chitosan microgels manipulates the gut microbiome to ameliorate salt-induced Hepatorenal injury. Front. Nutr. 9:872808. doi: 10.3389/fnut.2022.872808
Zhang, P., Liu, J., Xiong, B., Zhang, C., Kang, B., Gao, Y., et al. (2020). Microbiota from alginate oligosaccharide-dosed mice successfully mitigated small intestinal mucositis. Microbiome 8, 1–15. doi: 10.1186/s40168-020-00886-x
Zhang, Y., Qin, S., Song, Y., Yuan, J., Hu, S., Chen, M., et al. (2022b). Alginate oligosaccharide alleviated cisplatin-induced kidney oxidative stress via lactobacillus genus-FAHFAs-Nrf2 Axis in mice. Front. Immunol. 13:857242. doi: 10.3389/fimmu.2022.857242
Zhang, C., Shu, W., Zhou, G., Lin, J., Chu, F., Wu, H., et al. (2018). Anti-TNF-α therapy suppresses proinflammatory activities of mucosal neutrophils in inflammatory bowel disease. Mediat. Inflamm. 2018, 1–12. doi: 10.1155/2018/3021863
Zhao, Y., Feng, Y., Liu, M., Chen, L., Meng, Q., Tang, X., et al. (2020). Single-cell RNA sequencing analysis reveals alginate oligosaccharides preventing chemotherapy-induced mucositis. Mucosal Immunol. 13, 437–448. doi: 10.1038/s41385-019-0248-z
Zhao, J., Han, H., Zhong, B., Xie, W., Chen, Y., and Zhi, M. (2021). Health information on social media helps mitigate Crohn’s disease symptoms and improves patients’ clinical course. Comput. Hum. Behav. 115:106588
Zheng, W., Duan, M., Jia, J., Song, S., and Ai, C. (2021). Low-molecular alginate improved diet-induced obesity and metabolic syndrome through modulating the gut microbiota in BALB/c mice. Int. J. Biol. Macromol. 187, 811–820. doi: 10.1016/j.ijbiomac.2021.08.003
Zhou, Q., Gu, R., Xue, B., Li, P., and Gu, Q. (2021). Phenyl lactic acid alleviates Samonella typhimurium-induced colitis via regulating microbiota composition, SCFA production and inflammatory responses. Food Funct. 12, 5591–5606. doi: 10.1039/D1FO00166C
Zhou, X., Li, W., Wang, S., Zhang, P., Wang, Q., Xiao, J., et al. (2019). YAP aggravates inflammatory bowel disease by regulating M1/M2 macrophage polarization and gut microbial homeostasis. Cell Rep. 27:e1175, 1176–1189.e5. doi: 10.1016/j.celrep.2019.03.028
Zhuge, A., Li, B., Yuan, Y., Lv, L., Li, Y., Wu, J., et al. (2020). Lactobacillus salivarius LI01 encapsulated in alginate-pectin microgels ameliorates d-galactosamine-induced acute liver injury in rats. Appl. Microbiol. Biotechnol. 104, 7437–7455. doi: 10.1007/s00253-020-10749-y
Keywords: alginate oligosaccharides, gut microbiota, inflammation network, T helper cells, cytokines, probiotics
Citation: Zhang Z, Wang X and Li F (2023) An exploration of alginate oligosaccharides modulating intestinal inflammatory networks via gut microbiota. Front. Microbiol. 14:1072151. doi: 10.3389/fmicb.2023.1072151
Edited by:
Fengqin Feng, Zhejiang University, ChinaReviewed by:
Mehdi Fatahi-Bafghi, Shahid Sadoughi University of Medical Sciences and Health Services, IranMuhammad Hussain, Northeast Agricultural University, China
Mengyu Yang, Zhejiang University of Technology, China
Copyright © 2023 Zhang, Wang and Li. This is an open-access article distributed under the terms of the Creative Commons Attribution License (CC BY). The use, distribution or reproduction in other forums is permitted, provided the original author(s) and the copyright owner(s) are credited and that the original publication in this journal is cited, in accordance with accepted academic practice. No use, distribution or reproduction is permitted which does not comply with these terms.
*Correspondence: Zhikai Zhang, ✉ zzk1140515379@163.com