- 1Department of Botany, Institute of Sciences, Banaras Hindu University, Varanasi, India
- 2Department of Botany, Thakur Prasad Singh (T.P.S.) College, Patna, Bihar, India
- 3Department of Botany, Mahila Mahavidyalaya (M.M.V.), Banaras Hindu University, Varanasi, India
This study was undertaken to bridge the knowledge gap pertaining to cyanobacteria’s response to pretreatment. The result elucidates the synergistic effect of pretreatment toxicity in cyanobacterium Anabaena PCC7120 on morphological and biochemical attributes. Chemical (salt) and physical (heat) stress-pretreated cells exhibited significant and reproducible changes in terms of growth pattern, morphology, pigments, lipid peroxidation, and antioxidant activity. Salinity pretreatment showed more than a five-fold decrease in the phycocyanin content but a six-fold and five-fold increase in carotenoid, lipid peroxidation (MDA content), and antioxidant activity (SOD and CAT) at 1 h and on 3rd day of treatment, respectively, giving the impression of stress-induced free radicals that are scavenged by antioxidants when compared to heat shock pretreatment. Furthermore, quantitative analysis of transcript (qRT-PCR) for FeSOD and MnSOD displayed a 3.6- and 1.8-fold increase in salt-pretreated (S-H) samples. The upregulation of transcript corresponding to salt pretreatment suggests a toxic role of salinity in synergizing heat shock. However, heat pretreatment suggests a protective role in mitigating salt toxicity. It could be inferred that pretreatment enhances the deleterious effect. However, it further showed that salinity (chemical stress) augments the damaging effect of heat shock (physical stress) more profoundly than physical stress on chemical stress possibly by modulating redox balance via activation of antioxidant responses. Our study reveals that upon pretreatment of heat, the negative effect of salt can be mitigated in filamentous cyanobacteria, thus providing a foundation for improved cyanobacterial tolerance to salt stress.
1. Introduction
Anthropogenic activities in agricultural fields such as overuse of chemical fertilizers and improper irrigation practices increase the salt and metal contents in the soil. Furthermore, an increase in greenhouse gas emissions leads to the accumulation of heat-trapping gases in the atmosphere and thus warms the climate, which eventually disturbs the soil ecosystem as well as soil microflora, particularly cyanobacteria. Thus, salinity and heat are considered agriculturally relevant abiotic stresses and are of particular interest. Cyanobacteria are photosynthetic prokaryotes, present in extreme environments, and serve as a dominant producer in paddy fields. They tolerate unfavorable environmental conditions and thus have evolved various responses to acclimatize to stresses (Wannigama et al., 2012). Anabaena, a filamentous nitrogen-fixing cyanobacterium, is known to fix a significant amount of nitrogen in Indian paddy fields. However, in paddy fields, they are exposed to various abiotic stresses, including heat and salinity. Salt stress is an abiotic element that has a huge impact on cyanobacterial survival and growth. In cells of photosynthetic organisms, salt stress leads to a decrease in cell volume and an increase in osmotic stress (Yang et al., 2020). It also inhibits processes like photosynthesis, protein synthesis, energetics, and lipid metabolism. Changes in ion and water homeostasis due to salt cause damage at the molecular level, arrest growth, and can even cause death (Demiral and Türkan, 2006). The low concentration of Na+ aids in the regulation of pH and nitrogen and carbon dioxide fixation in plants and cyanobacteria (Karandashova and Elanskaya, 2005; Han et al., 2012; Fan et al., 2019). Ion homeostasis is disrupted by excessive Na+ and Cl– fluxes into the cell, resulting in the build-up of ROS (Scarpeci et al., 2008; Feng et al., 2015; Song et al., 2020). ROS leads to the breakdown of the photosynthetic machinery and membrane lipid peroxidation, which has a negative impact on photosynthesis (Yang et al., 2020). Furthermore, high NaCl concentrations prevent the de novo synthesis of proteins, including several photosystem-related proteins like the D1 protein (Allakhverdiev et al., 2002).
However, heat damages the cellular membrane and leads to oxidative damage, thereby producing ROS such as superoxide anions, hydroxyl radicals, and peroxides (Liu and Huang, 2000). Furthermore, it is also known to inhibit growth, photosynthesis, metabolism, and RNA synthesis and induce protein denaturation and aggregation causing post-translational modifications leading to the loss of cell viability (Wen et al., 2005). Photosystem II (PSII) is regarded to be one of the most sensitive locations in photosynthetic machinery, and its activity is considerably reduced by high-temperature stress (Zhao et al., 2008).
However, most of the studies concerning stress are of single stress type (Rai et al., 2013). The pretreatment effect is distinctive and cannot be extrapolated from the responses to each of them when applied alone. It is reasonable to presume that the molecular signaling pathway and the complex network of interrelated genes may synergize and/or antagonize each other’s effects (Rai et al., 2013). Even though the pretreatment phenomenon has not yet been reported to exist in the environment but it can be applied to various biotechnological approaches, as mentioned in the study of Ellison et al. (2019) in where they studied the effect of pretreatment for enhanced lipid extraction. Pretreatment studies of salt, temperature, and copper followed by UV stress in Anabaena doliolum (Srivastava et al., 2006), pretreatment of copper followed by UV stress in Anabaena doliolum (Bhargava et al., 2008), and heat pretreatment followed by UV stress in Anabaena doliolum (Mishra et al., 2009) are one of the very few reports on cyanobacteria pertaining to pretreatment.
A thorough review of the literature has revealed the availability of facts of cyanobacteria subjected to single stresses as well as in combination (Narayan et al., 2011; Pandey et al., 2012; Rai et al., 2013, 2014; Agrawal et al., 2014; Panda et al., 2014; Shrivastava et al., 2015; Singh et al., 2015; Teikari et al., 2015). However, studies pertaining to pretreatments are very limiting. Furthermore, heat treatment has been found to offer cross-tolerance to different abiotic stresses such as salt (Mishra et al., 2009) but studies pertaining to the effects of salt (chemical stress) on heat shock (physical stresses) is limiting. Therefore, this study was conducted to bridge the above-mentioned gap in the knowledge regarding the response of cyanobacteria subjected to pretreatment. In light of this and the physicochemical characteristics of the relevant stresses, we postulated that salinity pretreatment should enhance the heat effect while heat pretreatment should counteract the salinity effect even if applied after 7 days. To test the aforementioned hypotheses, experiments were conducted to investigate the effect of pretreatment with salt and temperature on the morphology, pigment content, lipid peroxidation (MDA content), enzymatic (superoxide dismutase and catalase) modifications, and expression pattern of antioxidative genes in Anabaena PCC7120.
2. Materials and methods
2.1. Organism and growth condition
Anabaena PCC7120 was grown axenically in BG-11 medium (Rippka et al., 1979) buffered with 4-(2-hydroxyethyl)-1-piperazine-ethanesulfonic acid (HEPES) buffer (1.2 g L–1) at pH 7.5 in Erlenmeyer flask of capacity 250 ml containing 100 ml of culture at 24 ± 2oC under a day light fluorescent tube emitting 72 μmol m–2 s–1 photosynthetically active radiation (PAR) light intensity and a photoperiod of 14:10 h. The flasks were plugged with non-absorbent cotton and were shaken 2–3 times daily for proper aeration. All experiments were performed under an exponentially growing culture.
2.2. Experimental design and treatments
Anabaena PCC7120 culture (OD750 = 0.5) never exposed to stress was taken as control strain. The single, combined, and pretreatment of heat and salt treatment were given to the strain at LC50 (lethal concentration). LC50 was determined through the colony count method (Rai and Raizada, 1985).
2.2.1. For LC50NaCl determination
Exponentially growing Anabaena containing 104 cells mL–1 was aseptically spread on agar plates supplemented with various concentrations of NaCl (50–250 mM).
2.2.2. For LC50 heat shock determination
Exponentially growing Anabaena containing 104 cells mL–1 was aseptically spread on agar plates. The plates were shifted to the temperature-controlled incubator for heat treatment under continuous light of 72 μmol photon m–2 s–1 PAR provided by fluorescent lamps throughout the heat treatment. The plates were exposed to different temperatures (40–60oC) for 1 h.
Cells were counted after 3 weeks. LC50 was obtained at 150 mM and 45oC for NaCl and heat, respectively.
2.3. Experimental design
The experiments were performed in six sets: (1) cells never exposed to stress (C), (2) cells exposed to heat at 45oC for 1 h (H), (3) cells exposed to salt at 150 mM (S), (4) cells pretreated with heat (45oC for 1 h) followed by recovery for 7 days and application of salt at 150 mM (H-S), (5) cells pretreated with salt at 150 mM followed by recovery for 7 days and application of heat at 45oC (S-H), and (6) cells exposed to combined heat and salt stress at respective LC50 (H + S) (Figure 1). All the parameters used in the present study were analyzed from the aforementioned cultures. Experiments were performed in triplicates and were repeated two times to confirm the reproducibility.
2.4. Growth measurements
Growth was estimated by observing the optical density of the culture at OD750 nm in a UV/visible spectrometer (Shimadzu, 2017) on alternate days up to the 15th day using the basal medium as blank (Küpper et al., 2000). It was also measured by cell count through a hemocytometer under a microscope (Olympus, 2019) at 40X on alternate days up to the 15th day (Lawton et al., 1999).
2.5. Morphological alterations
The morphological alteration was obtained by taking images of the control culture and culture exposed to stress on 0, 3, 7, and 15 days using a light microscope (Olympus, 2019 model no. CX21iLEDFS1) at 40X, and the image was processed through a computer with Magcam DC-10 camera model and MagVision software (Image calibration: 40X; X:50.000000/40 Micron/Pixel; Y: 50.000000/40 Micron/Pixel).
2.6. Pigments estimation
Pigment estimation was done by the protocol of Myers and Kratz (1955). A known volume of cyanobacterial culture was centrifuged and the pellet was incubated overnight at 4oC in 80% acetone. The suspension was centrifuged and the supernatant was used to measure chlorophyll a and carotenoid at 663 and 480 nm, respectively. The pellet thus obtained was used for the estimation of phycocyanin. The pellet was resuspended in 30 mM Tris–HCl and sonicated. The suspension was centrifuged and the supernatant was used to measure the phycocyanin content at 610 nm.
2.7. Phycobiliproteins spectrum
The supernatant obtained in the pigment estimation for phycocyanin (Myers and Kratz, 1955) was used to study phycobiliproteins spectra by measuring the absorbance from the range of 400–700 nm (Adir and Lerner, 2003).
2.8. Malondialdehyde (MDA) estimation
The total content of 2-thiobarbituric acid (TBA) reactive substances expressed as MDA (malondialdehyde) was used to measure lipid peroxidation. These reactive substances were extracted by the protocol of Cakmak and Horst (1991). A known volume of culture was centrifuged and the pellet was suspended in 0.1% of TCA at 4oC. The suspension was sonicated and centrifuged. A total of 0.5% TBA in 20% TCA was added to the supernatant and the solution was incubated at 90oC, and then the reaction was terminated in ice-cold water. The suspension was again centrifuged. The absorbance of the supernatant was measured at 532 nm and corrected for non-specific turbidity by subtracting the absorbance at 600 nm. The concentration of MDA was calculated at its extinction coefficient (155 mM–1 cm1).
2.9. Antioxidant assay
Cell pellets of Anabaena PCC7120 suspended in lysis buffer (pH 7.0) were sonicated in ice-cold condition. Cell lysis buffer for enzyme assay contained 1 mM EDTA and 1% PVP. The sonicated sample was centrifuged at 4oC and the resulting supernatant containing crude extract or antioxidant enzymes was used for further assay. Total SOD activity was assayed by monitoring the inhibition of reduction of nitroblue tetrazolium (NBT) according to the method of Giannopolitis and Ries (1977). A 3 ml reaction mixture contained 50 mM potassium phosphate buffer (pH 7.8), 0.1 mM EDTA, 13 mM methionine, 75 μM NBT, enzyme extract, and 2 μM riboflavin. The reaction mixture was illuminated for 20 min at a light intensity of 5,000 μmol photon m–2 s–1. One unit of SOD activity was defined as the amount of enzyme required to cause 50% inhibition of NBT reduction monitored at 560 nm. Catalase activity was estimated by measuring the consumption of H2O2 (extinction coefficient 39.4 mM–1 cm–1) at 240 nm for 3 min (Aebi, 1984). A 3 ml reaction mixture contained 50 mM potassium phosphate buffer (pH 7), 10 mM H2O2, and enzyme extract. One unit of CAT activity was defined as the amount of enzyme utilized to decompose 1.0 μM of H2O2.
2.10. Transcript analysis
Total RNA extraction using the RNeasy Micro kit (Qiagen) was performed from 50 ml culture (OD750nm = 0.6) of Anabaena PCC7120 grown in BG11 before and after stress treatment. Total RNA (1 μg) was reverse transcribed in a 20 μl reaction mixture using the iScript cDNA synthesis kit (BioRad). Transcript analysis was performed using gene-specific primer for antioxidative genes, FeSOD, MnSOD, and catalase (all0070, alr3090, and alr2938) as well as reference gene rnpB (Table 1). For qRT-PCR using a CFX-96 (BioRad), 15 ng of cDNA extracted from each sample was used in 20 μl including 10 pmol of each forward and reverse primers, and 1x Sso fast evagreen qPCR supermix (BioRad). Transcript levels were normalized to rnpB transcript and calculated using the 2–ΔΔCt method to evaluate the relative quantities of each amplified product (Livak and Schmittgen, 2001). The threshold cycle (Ct) was automatically determined for each reaction by the system (default parameters). The specificity of the PCR was determined by melting curve analysis of the amplified products.
2.11. Statistical analysis
Values presented in the text indicate the mean ± S.E. of the three replicates. The experiments were done in triplicates and repeated two times to confirm the reproducibility. Results were statistically analyzed using two-way ANOVA, followed by Dunnett’s multiple comparisons test to compare changes within groups. A p-value of ≤ 0.05 was considered statistically significant. All the statistical analysis was performed using SPSS ver. 22.0 software.
3. Results
3.1. Growth measurements
The sharp decline in growth can be seen in cells subjected to S-H as shown in Figure 2A, and S-H density declined by 71.57, 56.90, and 50.06% on the 4, 6, and 10th days, respectively. The H-treated sample showed an appreciable decrease from the 6th day to the 10th day of treatment, thereafter there was an increase in its growth. S showed a decrease on the 10th day by 50.47%. However, H+S and pretreated sample, i.e., H-S did not show any stark difference in their growth and were always on par with the control sample. H, H+S, and H-S, did not show any significant change in their cell density. Control bears a sigmoid curve during its growth measurement.
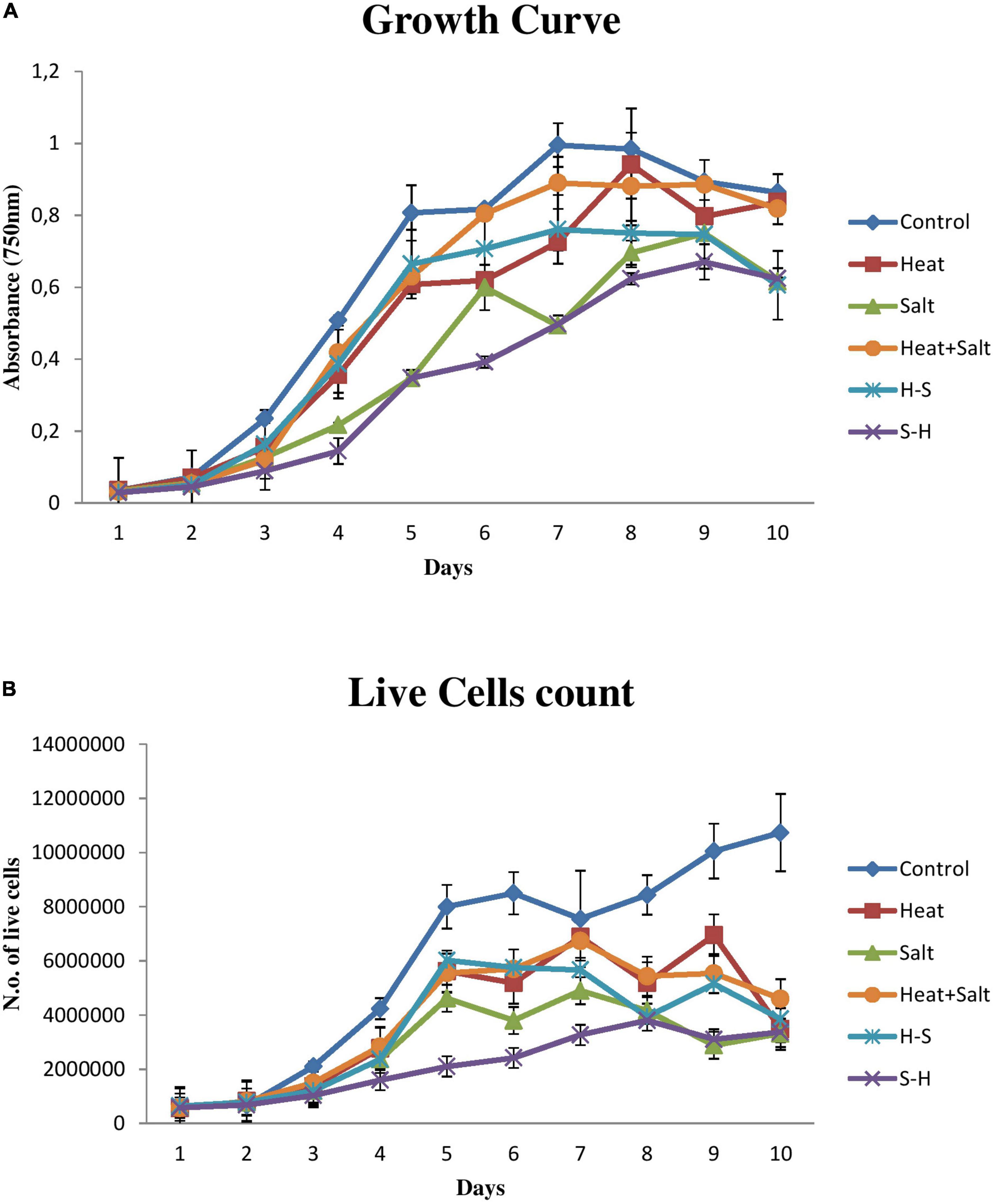
Figure 2. Growth behavior of Anabaena PCC 7120 exposed to stress. (A) Growth measurement via optical density. (B) Growth measurement via cell count.
Results pertaining to cell count (Figure 2B) portrayed that the sample treated with S-H was severely affected and showed a sharp decline in its number up to 15 days. H and H+S showed significant cell death by 67.54 and 57.14%, respectively, on the 15th day. S showed significant cell death from the 14th day onward. There was a decline of 71.31 and 69.09% on the 14th and 15 days, respectively. H-S showed a significant decrease on the 12th day by 53.35%, on the 14th day by 48.75%, and on the 15th day by 64.13%.
However, if a correlation has to be drawn between the optical density and cell count, it can be concluded that an increase in cell density does not correspond to an increase in live cell number. An increase in cell density and a decrease in live cell count in a sample can be attributed to the fact that optical density has included the density of live and dead cells together as can be observed in the case of samples treated with H, S, H-S, and H+S.
3.2. Morphological alterations
The morphological alterations of Anabaena PCC7120 were investigated through light microscopy under various stresses. There were significant differences in the morphological changes shown by the organism under study. Light microscopic studies on the interactions of the stress with Anabaena PCC7120 revealed extensive breakage of cyanobacterial filaments.
Significant variation was not observed in samples after 1 h of stress treatment sample and control. Slight yellowing of filaments could be observed in cells subjected to salt stress. Comparative shortening of filaments was observed in all samples on the 3rd day of stress treatment. The akinete formation could be observed in cultures exposed to H + S in order to survive stress conditions. Fragmentation of filaments was observed in the pretreated samples (H-S and S-H).
Abnormality in cell shape and size within the filaments was observed in treated cultures on the 7th day. The terminal akinete formation was observed in filaments exposed to stresses. Moreover, prominent intercalary cell death was observed in the culture treated with H and in S-H. Abnormal bulging of cells in the filament of cyanobacterium was observed in H + S. Intercalary cell death appeared in H on the 15th day, whereas cell enlargement and the terminal akinete cell were observed under salt treatment. H + S treatment showed distinctive anomalous cell enlargement in filaments owing to osmotic and heat stress. Fragmentation of filaments was observed in H-S; 4–5 cells/filaments were observed. A peculiar phenomenon of contraction of the protoplast of a plant cell, which may be due to loss of water from the cells, was observed in S-H which resulted in plasmolysis of cells in the filaments due to salinity pretreatment (Figures 3A–D).
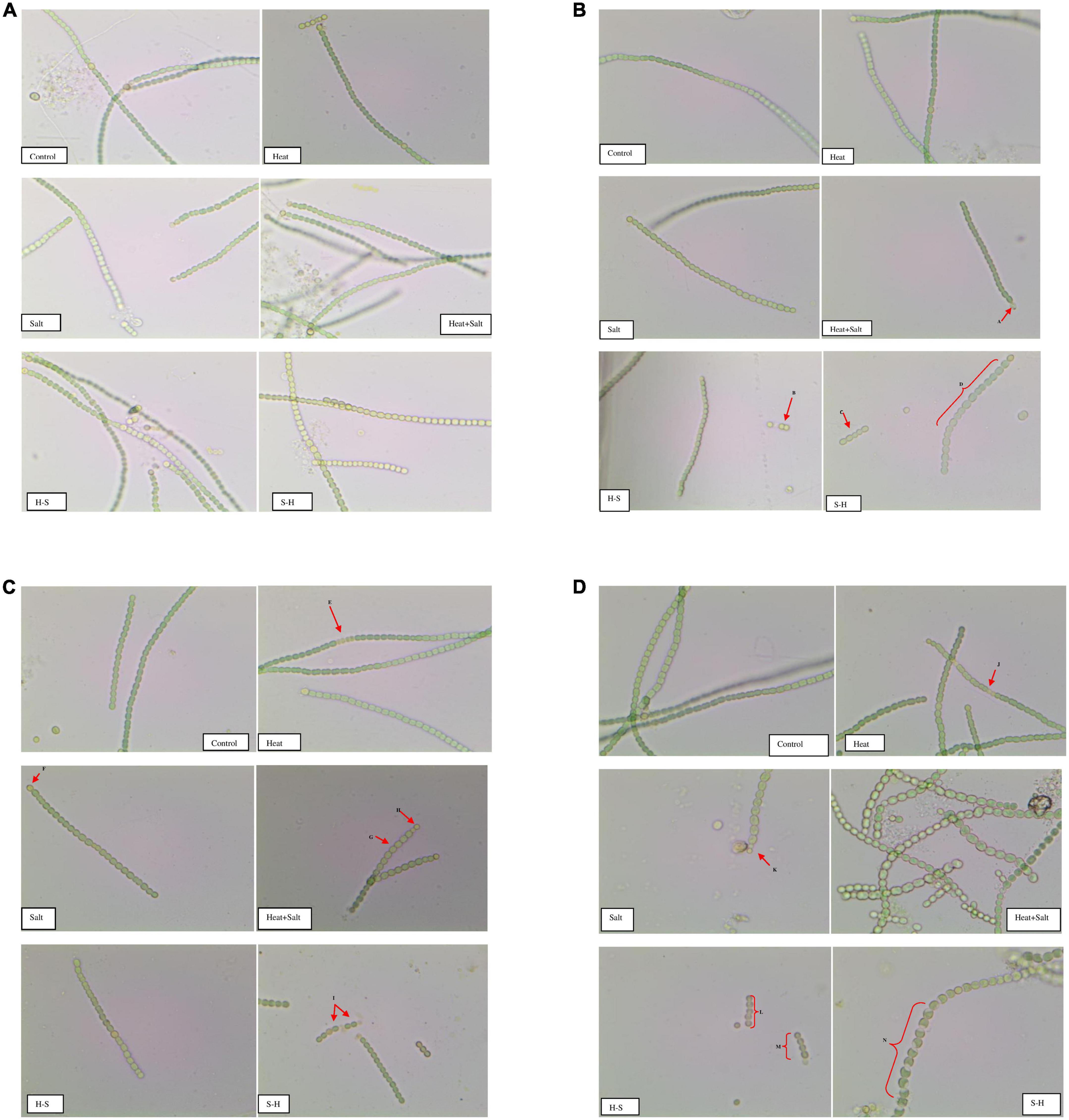
Figure 3. (A) Images of Anabaena PCC7120 subjected to selected stresses after 1 h (Image calibration: 40X; X:50.000000/40 Micron/Pixel; Y: 50.000000/40 Micron/Pixel). (B) Images of Anabaena PCC 7120 subjected to selected stresses on 3rd day. (Image calibration: 40X; X:50.000000/40 Micron/Pixel; Y: 50.000000/40 Micron/Pixel. Red arrows or braces indicate a morphological alteration in the filaments. A. Akinete formation; B. and C. Fragmentation of filaments; D. Bulging of cells in filaments). (C) Images of Anabaena PCC 7,120 subjected to selected stresses on the 7th day (Image calibration: 40X; X:50.000000/40 Micron/Pixel; Y: 50.000000/40 Micron/Pixel. E. & I. Intercalary cell death; F. and H. Akinetes formation; G. Cell bulging). (D) Images of Anabaena PCC7120 subjected to selected stresses on the 15th day (Image calibration: 40X; X:50.000000/40 Micron/Pixel; Y: 50.000000/40 Micron/Pixel. J. Intercalary cell death; K. Akinete formation; L. and M. Fragmentation of filaments; N. Plasmolysis of cells in filament).
3.3. Pigment analysis
3.3.1. Chlorophyll a
No significant change was observed after 1 h of stress exposure. The chlorophyll content of the culture subjected to treatment declined throughout the observation. On the 3rd day, S showed a significant decline in its chlorophyll concentration by 2.60-fold. Moreover, on the 15th day, cultures subjected to S and H + S treatments showed a significant decrease of 2.73- and 3.02-fold, respectively, in the chlorophyll a content. Nonetheless, pretreatment samples showed a decline in the content (approximately 1–1.5-fold change) unlike the other stressed exposed culture but it was on par with the control. It may be that pretreatment had an antagonistic effect on the latter-induced stress (Figure 4).
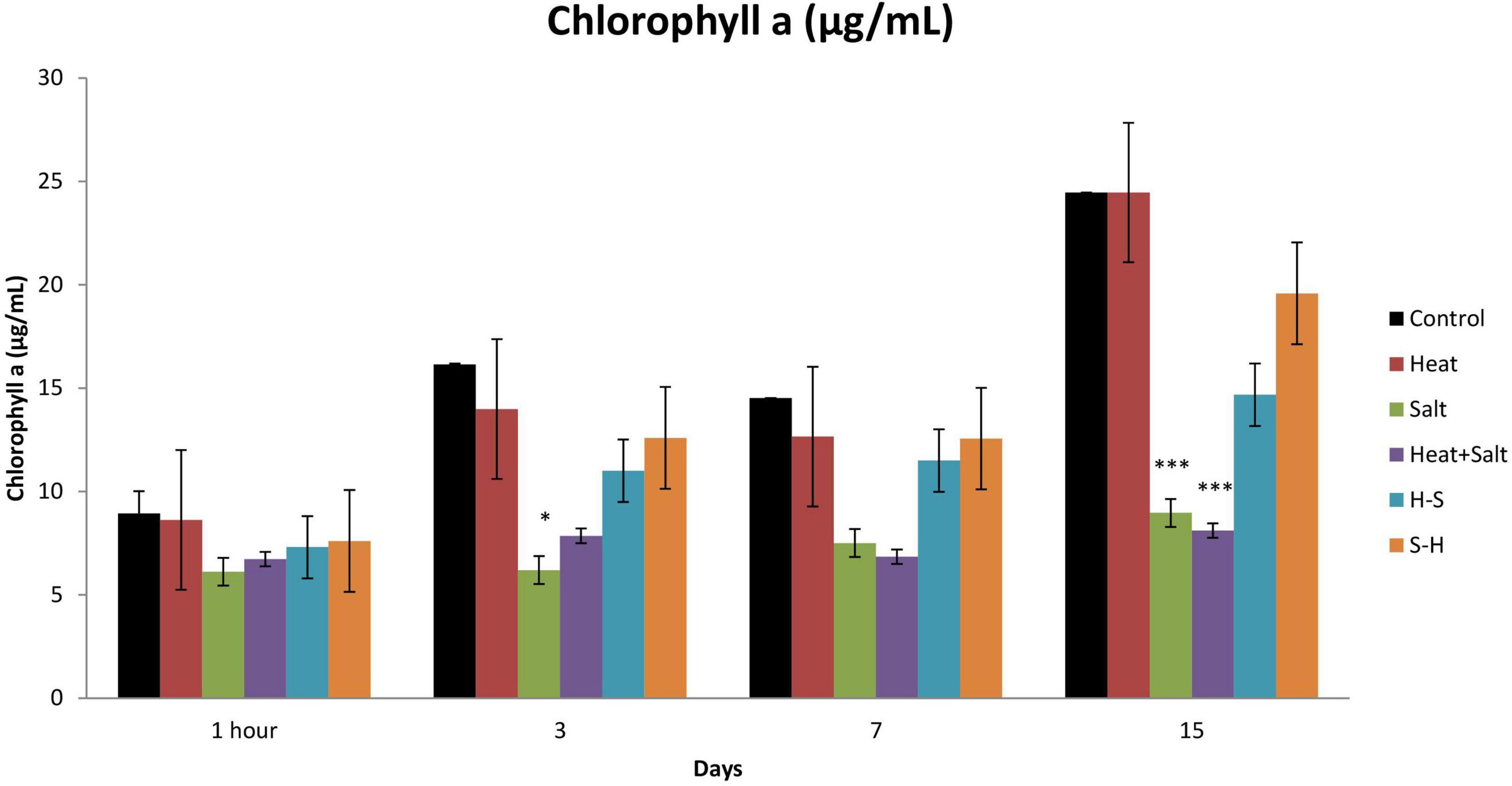
Figure 4. Effect of selected stresses on chlorophyll a content of Anabaena PCC 7120 up to the 15th day. Asterisk (*) indicates p < 0.05 and triple asterisk (***) indicates p < 0.001. (H-S indicates heat pretreatment; S-H indicates salt pretreatment).
3.3.2. Carotenoid
An increase in carotenoid content was observed throughout all cultures subjected to stress. However, H-S showed a significantly high amount of carotenoid from 1 h up to the 15th day. It showed an increase of 32.94, 33.98, 33.35, and 34.23% on 1 h and 3rd, 7th, and 15th day, respectively, in comparison to the control. Similarly, S-H also showed a significant increase in the amount of carotenoid on the 7 and 15th day (33.35 and 29.19%, respectively) after treatment (Figure 5). The increase of carotenoids in pretreated samples can be attributed as a positive strategy as it protects light-harvesting pigments against photochemical damage.
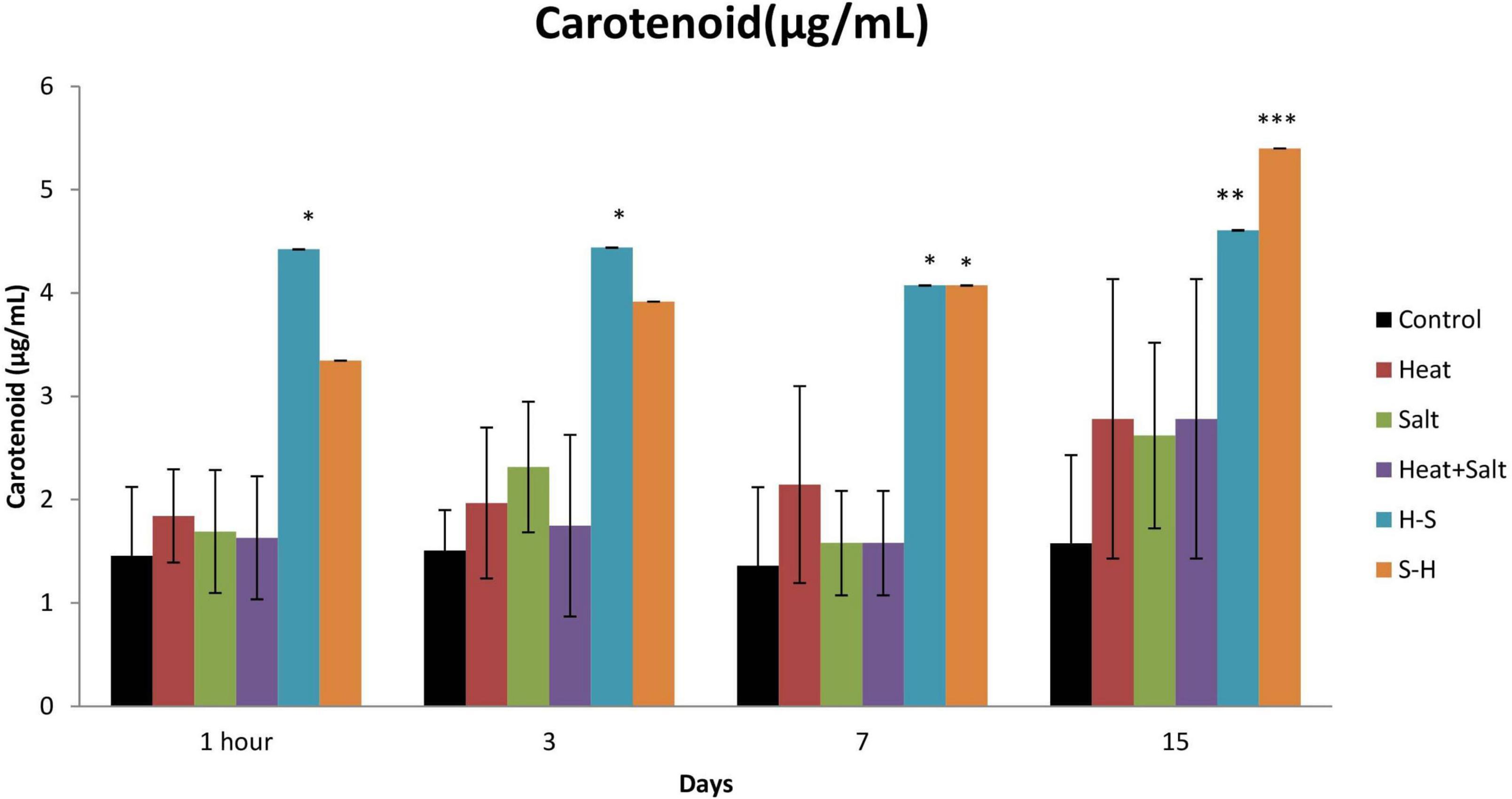
Figure 5. Effect of selected stresses on carotenoid content of Anabaena PCC 7120 up to 15th day. Asterisk (*) indicates p < 0.05, a double asterisk (**) indicates p < 0.01, and the triple asterisk (***) indicates p < 0.001. (H-S indicates heat pretreatment; S-H indicates salt pretreatment).
3.3.3. Phycocyanin
Phycocyanin content was always low for the treated sample up to the 15th day of observation in comparison to the control. There was no significant decline in the phycocyanin content in the H-treated sample. Significant changes were observed in all cultures subjected to stress on 1 h and 15th day except in H, whereas the pretreated sample also showed a significant decrease in the content on the 3rd and 7th day. There was a decrease of 2.45-, 2.22-, 2.29-, and 5.21-fold in S, H + S, H-S, and S-H, respectively, on 1 h after treatment, and 1.13-, 3.15-, 5.52-, and 3.74-fold in S, H + S, H-S, and S-H, respectively, on day 15. S-H showed a decrease in content by 2.49- and 4.426-fold on the 3rd and 7th day, respectively, and H-S showed a decrease of 3.15-fold on day 7 (Figure 6). Pretreatment enhanced the degradation of phycocyanin.
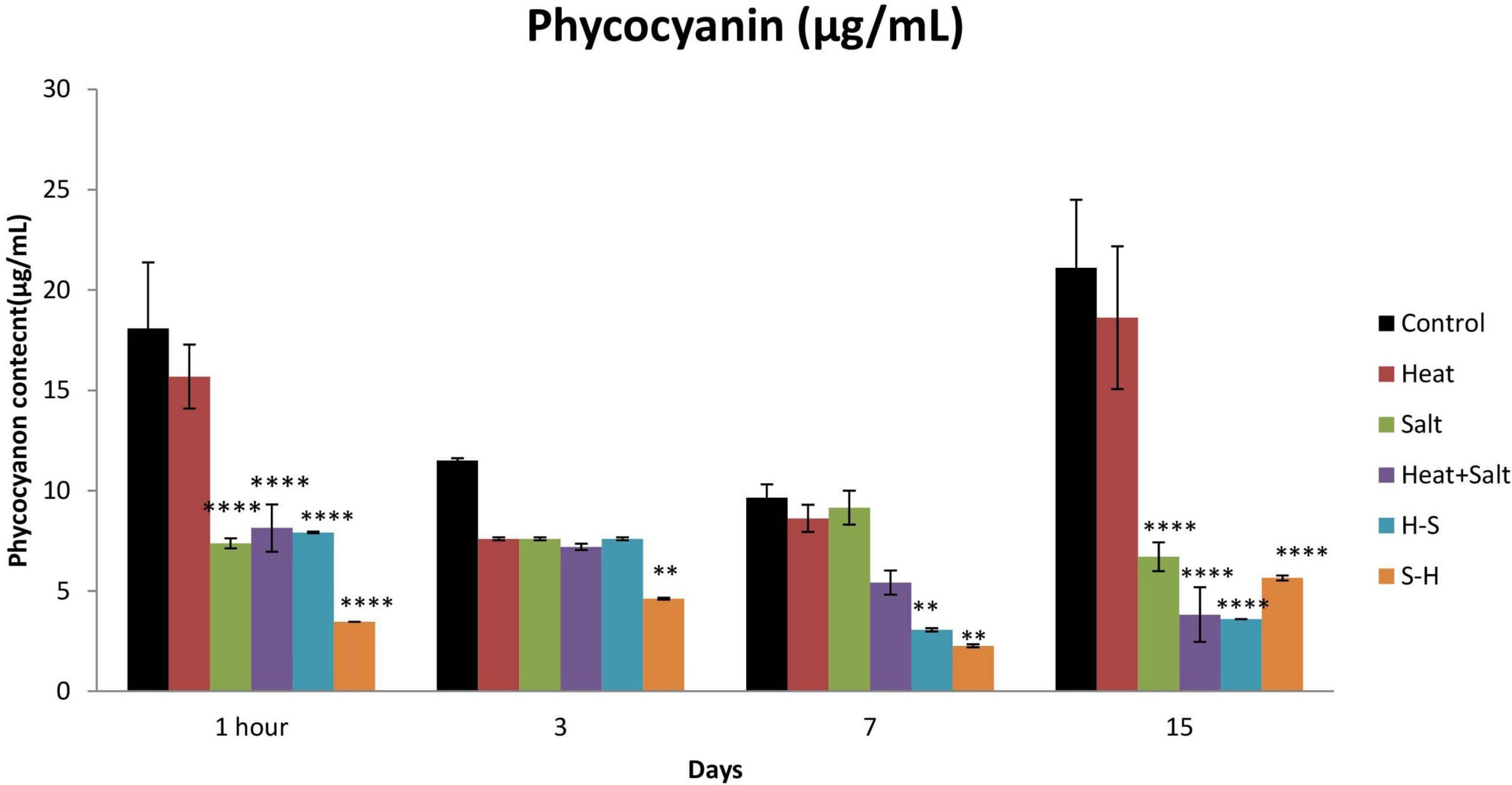
Figure 6. Effect of selected stresses on phycocyanin content of Anabaena PCC 7120 up to 15th day. A double asterisk (**) indicates p < 0.01, the triple asterisk (***) indicates p < 0.001, and the four asterisk (****) indicates p < 0.0001. (H-S indicates heat pretreatment; S-H indicates salt pretreatment).
3.4. Phycobiliprotein spectrum
A study was conducted to assess the effect of stress on phycobiliproteins of Anabaena PCC7120. It resulted in a large peak of allophycocyanin (620–660 nm) and phycocyanin (600–640 nm) in control. Phycoeyrthrocyanin peak was also higher in control than in stresses throughout the observation period. The peak area was least affected in H, whereas S-H was severely affected in terms of peak area except on the 15th day. Rest were showing intermediate results. H and H + S showed similar absorption for phycoerythrin and S and H-S also bear close resemblance in peak. A similar result was obtained on the 3rd day with the exception that H + S showed a further decrease in peak. On the 7th day, H was on par with control for the phycoerythrin peak but there was a significant decrease in peak in terms of allophycocyanin and phycocyanin. S and H-S samples were on par with each other in terms of the peak for phycocyanin, whereas the absorption of others remains almost the same as that of the 3rd day. H + S and H-S showed a decrease in their phycocyanin, allophycocyanin, and phycoerythrin peak but S-H showed a large peak on the 15th day (Figure 7). The change in absorption spectra of phycocyanin was similar to the change in the concentration of phycocyanin.
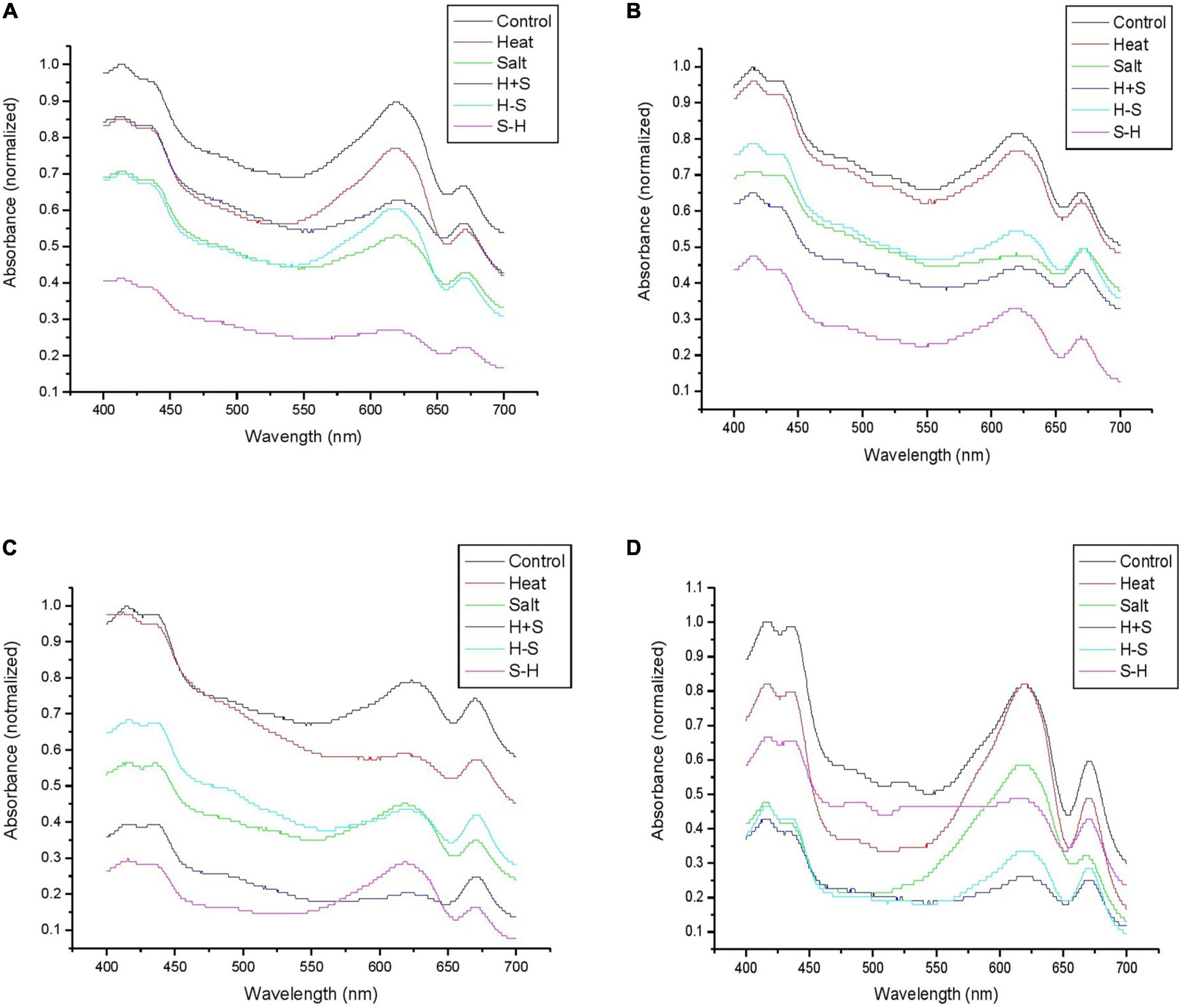
Figure 7. Graph representing the phycobiliprotein spectrum (460–670 nm) of Anabaena PCC 7120 exposed to stress on 1 h (A), 3rd (B), 7th (C), and 15th (D) day. (H-S indicates heat pretreatment; S-H indicates salt pretreatment).
3.5. Malondialdehyde (MDA)
The untreated sample showed a low level of MDA formation throughout the observation, whereas the treated one showed a comparatively high MDA level. MDA formation was significantly increased after 1 h of stress (4.25-fold increase) for H. The MDA content for S gradually increased up to the 7th day (5.25-, 2.66-, and 2.18-fold increase on 1 h and 3rd and 7th day, respectively). H + S showed significant production after 1 h of treatment (5.88-fold increases). H-S and S-H showed a significantly high content of MDA throughout the observation period. In pretreatment, the salt-pretreated sample showed the highest induction of MDA (Figure 8). The high amount of MDA marks high oxidative lipid injury under stress.
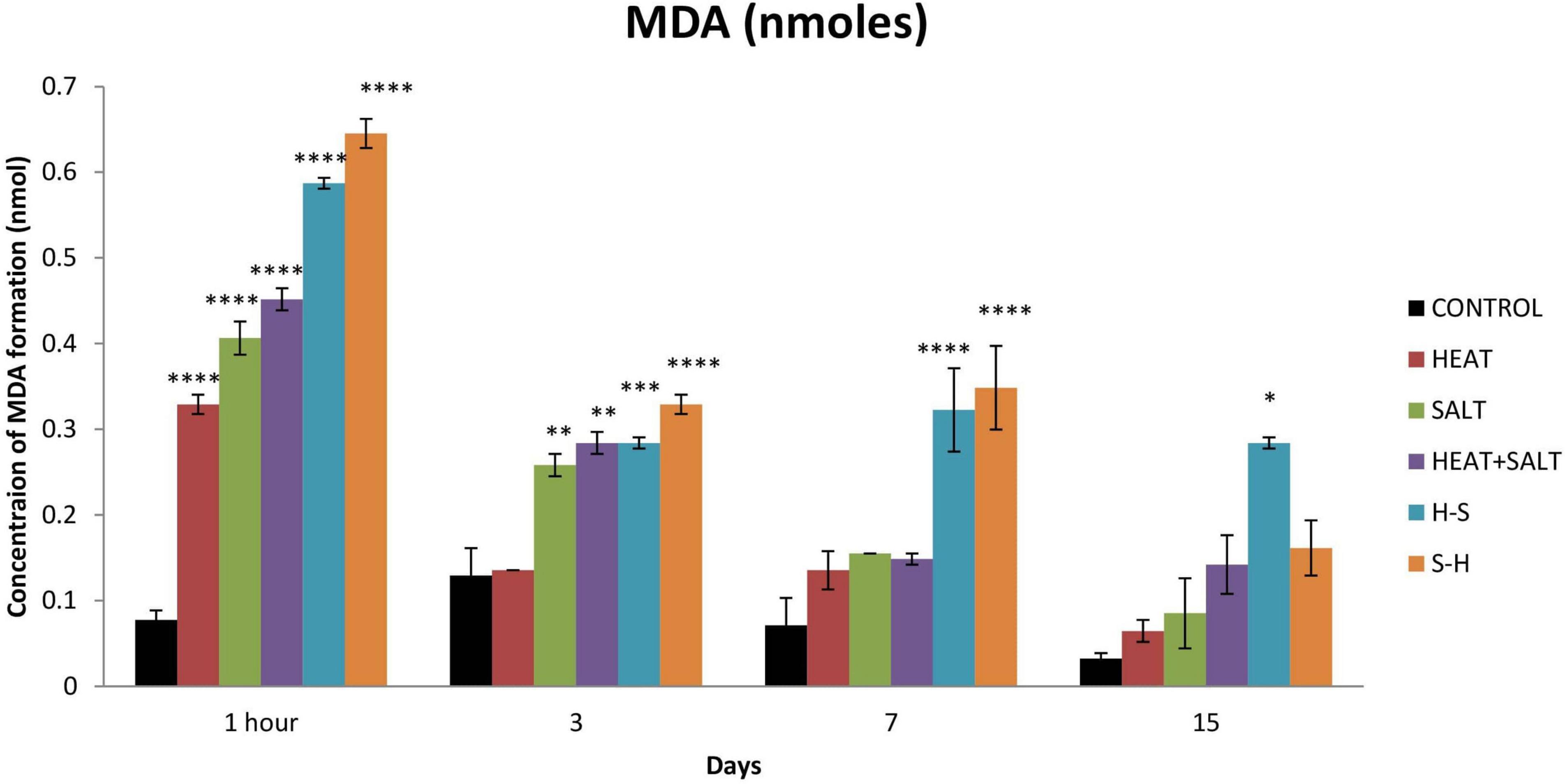
Figure 8. Effect of selected stresses on MDA production in Anabaena PCC 7120 up to the 15th day. Asterisk (*) indicates p < 0.05, a double asterisk (**) indicates p < 0.01, the triple asterisk (***) indicates p < 0.001, and the four asterisk (****) indicate p < 0.0001. (H-S indicates heat pretreatment; S-H indicates salt pretreatment).
3.6. Antioxidant assay
3.6.1. Superoxide dismutase
S-H showed the highest increase in SOD activity throughout the observation with 6.04-, 5.49-, 7.53-, and 2.18-fold increase on 1 h, and 3rd, 7th, and 15th day, respectively, in comparison with control which was followed by H-S. SOD activity was the highest on the 0th day (1-fold), followed by the 3rd day (3.58-fold), the 7th day (3.28-fold), and the 15th day (1.05-fold) for H-S. The least SOD activity among all the treatments shown by H. S showed an increase in activity up to the 7th day (4.45-, 1.67-, and 3.12-fold, respectively). H + S displayed an increase in its SOD activity on 1 h and 3rd day by 2.52- and 2.43-fold, respectively (Figure 9).
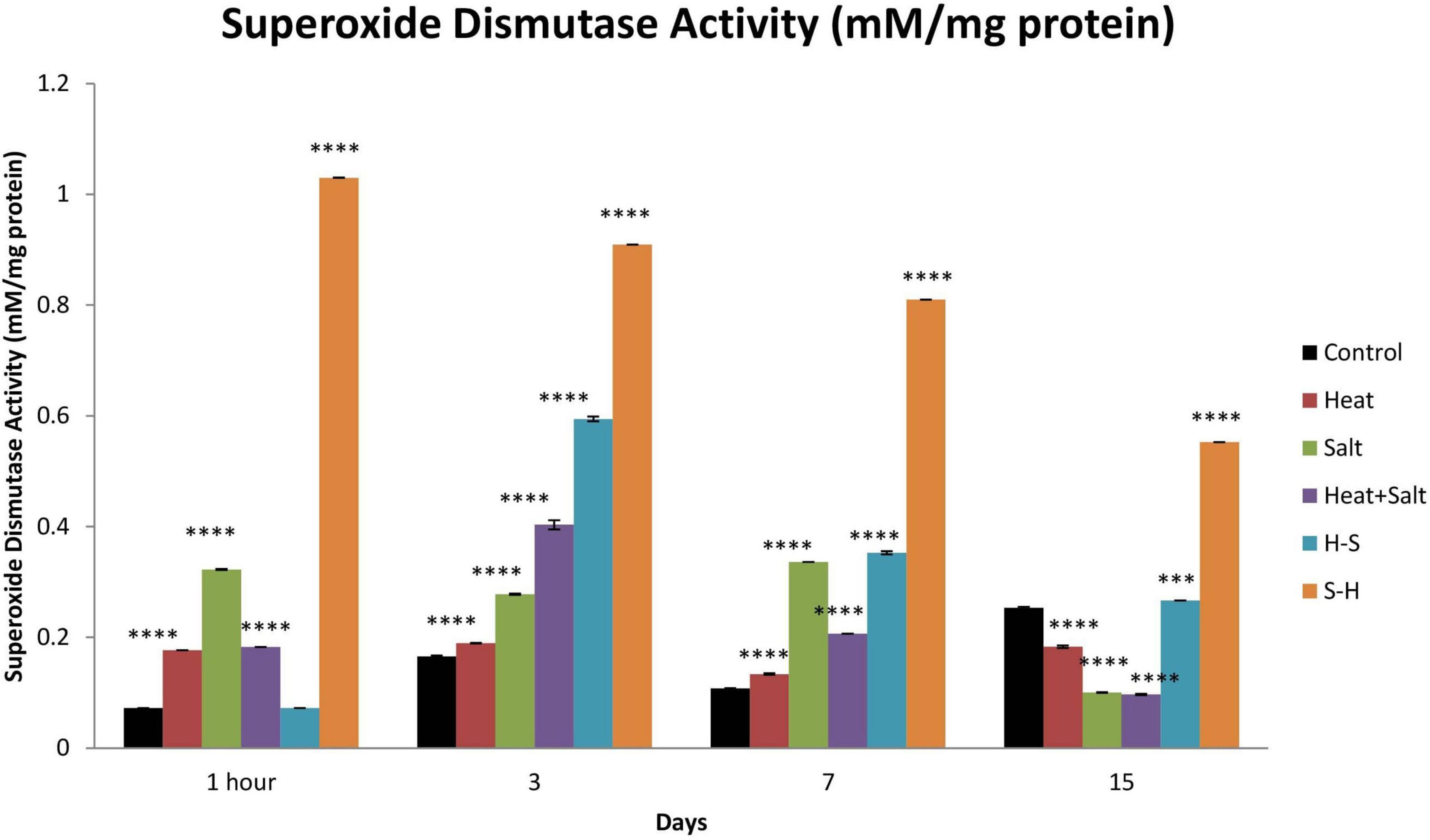
Figure 9. Effect of selected stresses on superoxide dismutase activity in Anabaena PCC 7120 up to the 15th day. Four asterisks (****) indicate p < 0.0001. (H-S indicates heat pretreatment; S-H indicates salt pretreatment).
3.6.2. Catalase
No significant changes were observed in catalase activity after 1 h of treatment. Catalase activity showed maximum induction throughout the observation in S-H. There were 5.96-, 1.79-, and 1.61-fold increase on 3rd, 7th, and 15th day, respectively. H-S showed an increase in its activity up to the 7th day with a significant increase on the 3rd day (3.97-fold). S and H + S showed similar activity to that of heat pretreatment. However, no significant increase in catalase activity was shown by H (Figure 10).
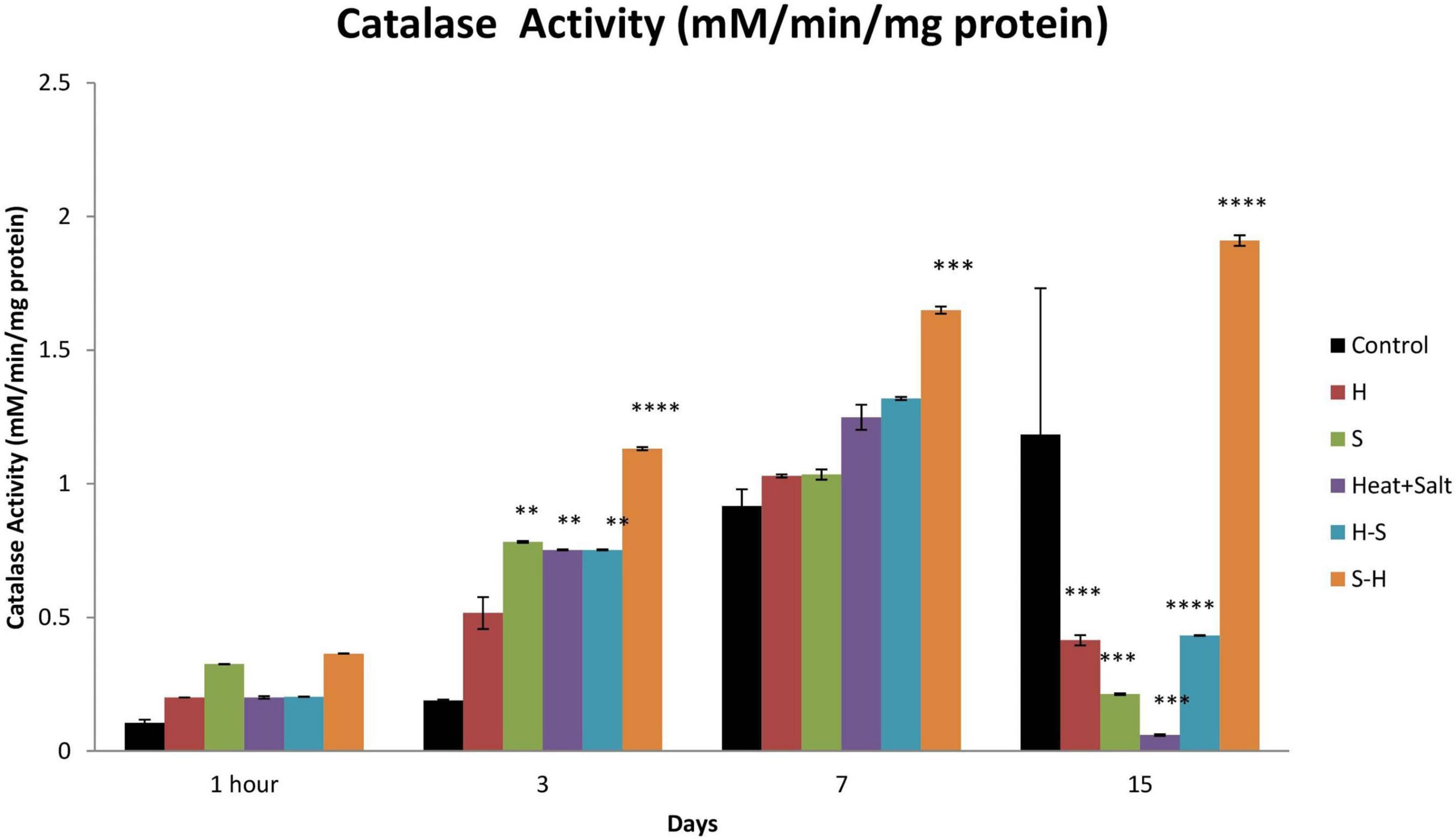
Figure 10. Effect of selected stresses on catalase activity in Anabaena PCC 7,120 up to the 15th day. A double asterisk (**) indicates p < 0.01, the triple asterisk (***) indicates p < 0.001, and the four asterisk (****) indicates p < 0.0001. (H-S indicates heat pretreatment; S-H indicates salt pretreatment).
3.7. Transcript analysis
Expression of three genes of the antioxidative defense system (FeSOD, MnSOD, and catalase) was observed in response to selected stresses, which displayed differential accumulation as compared to control. The expression of the genes alr2938 and all0070, which, respectively, code for FeSOD and MnSOD, increased by 3.6- and 1.8-fold in salt-pretreated (S-H) samples, indicating activation of stress defense pathways in response to ROS generation, supporting our biochemical findings. Likewise, cells exposed to H and S also displayed accumulation of alr2938 and all0070. Transcript levels of all0070 were downregulated under H-S and H + S samples. Similarly, catalase (alr3090) expression was also downregulated in H and H + S samples, whereas increased in S, S-H, and H-S samples (Figure 11).
4. Discussion
The present study deals with the effect of heat shock, salinity, their combination, and pretreatment stresses on growth, morphological alteration, pigments, lipid peroxidation, and antioxidant enzymes of Anabaena PCC 7120.
Morphological alterations through light microscopy demonstrated extensive shrinkage of cyanobacterial filament, cell degeneration, and terminal akinetes formation. It further revealed that the heat initially had no impact on the cellular structure, however, significant cell degeneration was observed in later phases. A similar result was observed under salinity stress, but anomalous swelling of cells was a unique feature observed on the 15th day, which could be due to osmotic stress leading to the accumulation of osmoprotectant (Singh and Montgomery, 2013). Heat pretreatment showed fragmentation of the filaments. H + S showed abnormal bulging of the cells in the filaments, which could be attributed to the fact that in addition to the cellular toxicity brought on by high ion concentrations, high salt concentrations in the growth media are known to reduce the water potential (Singh and Montgomery, 2013). Osmotic stress is thus caused by salt stress, and under these circumstances, many organisms, including cyanobacteria, store or synthesis suitable solutes or osmoprotectants to maintain reduced water potential inside the cell (Singh and Montgomery, 2013). In S-H, plasmolysis of cells in the filaments due to salinity pretreatment followed by heat was observed, which may be due to the hypertonic environment of the cyanobacterium. Similar to past observations on the response of cyanobacteria to abiotic stress conditions, particularly nitrogen constraint, akinete formations were observed. These findings require in-depth analysis because they are intriguing (Mahawar et al., 2018).
The decline in the chlorophyll a content in stressed samples could be attributed to the production of ROS that caused bleaching due to active oxygen-mediated peroxidation (Rai et al., 2021). Moreover, the comparative increase in the content of pretreated samples reflects the occurrence of cross-tolerance of heat-pretreated and salt-pretreated Anabaena to salt and heat stress, respectively. This result was concordant with the study of Mishra et al. (2009), which reported heat-mediated alleviation of UV-B toxicity in Anabaena doliolum.
The result of the pretreatment effect on carotenoid content can be further supported by the study of Mishra et al. (2009), where they reported an increase in carotenoid content on heat shock pretreatment followed by UV-B treatment. An increase in carotenoids in Anabaena PCC7120 under stress can be attributed to a positive strategy (Nonnengiesser et al., 1996). It is due to its role of protecting light harvesting pigments in the antenna complex against photochemical damage by allowing triplet energy transfer from chlorophyll a to carotenoid (Kim et al., 2005). They exert photoprotective and antioxidative functions by dissipating excess energy as heat by non-photochemical quenching (NPQ) or by scavenging excess ROS (El Bissati et al., 2000). Moreover, carotenoids are potent non-enzymatic antioxidants, which scavenge a variety of reactive oxygen species when cyanobacteria are exposed to stress. They play a role of a modulator of membrane homeostasis and photosynthetic apparatus against abiotic stress (Kirilovsky and Kerfeld, 2016).
Phycocyanin content significantly decreased under all treatments except heat up to the 15th day of observation compared to the control. The decrease in phycocyanin under S, H + S, H-S, and S-H may be due to the rapid entry of sodium ions leading to the dismantling of phycobilisomes from the thylakoid membranes causing photosynthesis inhibition and reduced energy transfers from phycobiliproteins to the reaction center (Lu and Vonshak, 2002; Rafiqul et al., 2003). Similar results have been earlier reported by Hemlata and Fatima (2009) on Anabaena NCCU-9, Singh et al. (2012) on Anabaena sp. PCC 7120, and Verma and Mohanty (2000) on Spirulina platensis, thus supporting present findings. Samples exposed to salt pretreatment were the most affected followed by heat pretreatment. Moreover, an increase in the intracellular concentration of NaCl inactivates ATP synthase and decreases the intracellular level of ATP (Murata et al., 2007). Moreover, ROS-induced protein peroxidation may also cause a damaging effect on phycocyanin.
Phycobiliproteins are water-soluble proteins that are covalently bonded with phycobilins (Hu, 2019). Due to phycobilins, phycobiliprotein have distinct absorption spectra from 460 to 670 nm (Adir and Lerner, 2003). Phycobiliproteins are divided into three different classes based on phycobilin energy or absorption spectra. Phycoerythrin (PEs) or phycoerythrocyanin (PECs) are of higher energy with main absorption at 480–580 nm, phycocyanin (PCs) of intermediate level with absorption at 600–640 nm, and allophycocyanins (APCs) with absorption at 620–660 nm. The change in the spectrum can be attributed to the fact that stresses led to photosynthetic inhibition. Inside the photosynthetic apparatus, the phycobilisome, which is involved in light capture, may be a target of photodamage and oxidative toxicity. These results find support from the studies of Wen et al. (2005) and Zhang et al. (2010) on Spirulina platensis under salt and heat stress, respectively.
Lipids play an important role in the tolerance to several physiological stressors in a variety of organisms including cyanobacteria (Parida and Das, 2005). An increase in the level of lipid peroxidation under various stresses can be attributed to the increased production of reactive oxygen species indicating oxidative stress in cyanobacteria, which can lead to cellular damage (Rai et al., 2021). In cyanobacteria, the membrane contains a high amount of polyunsaturated fatty acid (PUFA) (Halliwell, 1999), and increased MDA content is due to oxidative degradation of PUFA (Girotti, 1990). Increased lipid peroxidation in salt pretreatment (S-H) could be due to salinity-induced membrane damage, which was followed by heat treatment and enhanced lipid peroxidation. This finds support from the work of Srivastava et al. (2006), where a similar increase was reported in salt-pretreated samples followed by UV-B in Anabaena doliolum.
In order to scavenge stress-induced ROS, cyanobacteria activate their antioxidative defense system. SOD activity followed a general trend of S-H > H-S > S > H > C except on the 15th day of treatment suggesting recovery and suppression of the antioxidative defense system on the 15th day. Cyanobacteria induce a complex antioxidant defense system to combat the increasing level of ROS. SODs are the first line of defense against ROS. Superoxide dismutase is induced by a variety of cyanobacteria under stress and dismutates the superoxide radical (Zutshi et al., 2008). Superoxide dismutase is a major oxygen-free radical scavenger and it functions by disproportionating free radicals into hydrogen peroxide which is further scavenged by catalase (Macháèková, 1995).
A similar result was obtained for catalase, where pretreatment enhanced the activity. Peroxide radical produced by SOD during the scavenging of free radicals is further scavenged by catalase (Halliwell and Gutteridge, 2015). Catalases belong to the category of well-characterized antioxidant enzymes for hydroperoxide detoxification that cleaves the peroxide bond in hydrogen peroxide (H–O–O–H) to form molecular oxygen and water (Srivastava, 2010). Hence, it appears that peroxide can be detoxified by CAT.
Biochemical findings were further strengthened after analyzing the transcript levels of respective genes. Our findings imply that salinity pretreatment has an impact on the transcriptional control of genes involved in antioxidant production. Surprisingly, at heat pretreatment, the transcript levels of FeSOD and MnSOD were significantly downregulated. This may imply that the production of FeSOD and MnSOD is not alarm-driven by ROS or other cellular signals of temperature stress. Nevertheless, catalase gene expression was elevated. However, enhanced FeSOD and MnSOD under salt pretreatment (S-H), as compared with salinity, indicate an adaptive strategy of Anabaena for scavenging excessive ROS produced by the cyanobacteria.
5. Conclusion
The study was conducted to analyze the effect of stresses on cyanobacteria with the main aim to examine the effect of pretreatments on growth, development, pigment, lipid peroxidation, and antioxidant activity. Cyanobacteria were chosen due to their cosmopolitan nature, and particularly, Anabaena PCC7120 was chosen as a model organism as it bears a close resemblance to higher plant and easy maintenance of the culture in the laboratory. The cyanobacterium was able to effectively detoxify the surplus reactive oxygen species formed inside the cells of stressed Anabaena PCC7120 due to the enhanced expression of SOD in salt pretreatment. It can be concluded that the salinity pretreatments enhanced the deleterious effect. Salt was found to synergize the effect of heat on cyanobacteria as it caused osmotic stress in the cells that affect the cellular equilibrium whereas heat has been found to offer some cross tolerance to abiotic stresses due to the production of heat shock proteins. Heat shock proteins may be responsible for the protective effect of heat against salt. Pretreatment at sublethal temperatures increases the thermotolerance of cyanobacterial species, both unicellular and filamentous, and suggests that heat shock genes and proteins are involved in thermotolerance. Due to the constant synthesis of the two Hsp60 proteins throughout the heat stress and their great stability even after returning to normal growth circumstances, Anabaena demonstrates exceptional recovery from heat stress. On the other hand, salinity increased heat toxicity. The cellular equilibrium of the cell is disturbed by salt. Pretreatment with salinity impairs membrane permeability. In addition, it interferes with the cell’s ideal environment, which hinders enzyme activity, and is further hampered by heat shock (Figure 12). When exposed to heat, such pretreated cells become more fragile. Hence, it can be inferred that when physical and chemical stresses are superimposed 7 days apart, pretreatment chemical stress synergizes the deleterious effect of physical stress but pretreatment physical stress offers some tolerance to chemical stress. Therefore, this study is the first to provide how cyanobacteria respond in the presence of physical and chemical stresses and that chemical stress enhances the damaging effect of physical stress.
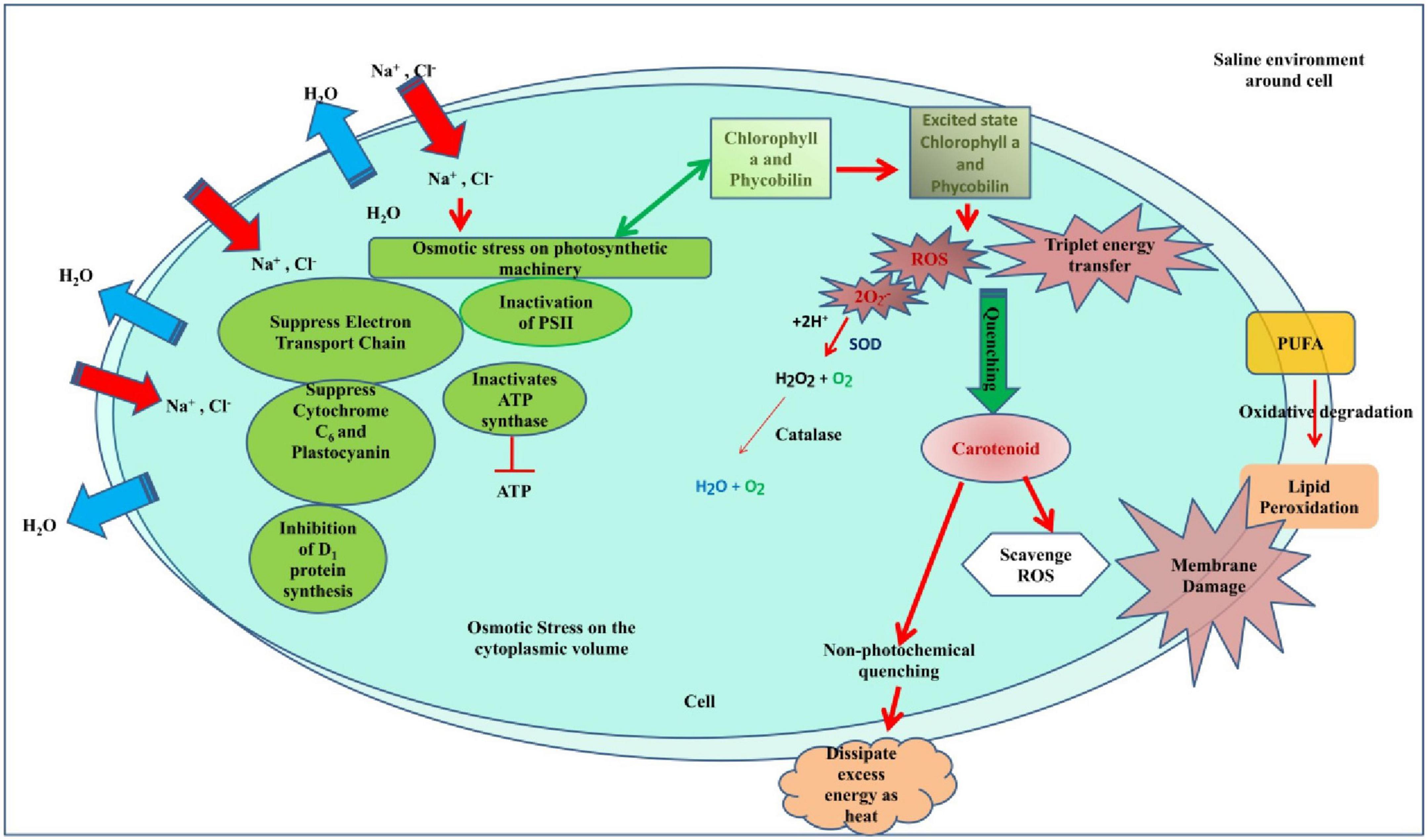
Figure 12. Hypothetical model showing how salinity pretreatment impairs the cell equilibrium and metabolism which when exposed to heat shock makes the cell more fragile.
Data availability statement
The original contributions presented in this study are included in the article/supplementary material, further inquiries can be directed to the corresponding author.
Author contributions
RS and NA conceived the idea and designed the experiments. RS conducted the experiments. VK performed the transcript analysis. RS, TK, SaY, NS, ShY, RP, VK, and NA analyzed the data. RS and ShY wrote the manuscript with input from other co-authors. All authors contributed to the article and approved the submitted version.
Acknowledgments
RS would like to thank the University Grant Commission (UGC), New Delhi for financial support in form of a research fellowship.
Conflict of interest
The authors declare that the research was conducted in the absence of any commercial or financial relationships that could be construed as a potential conflict of interest.
Publisher’s note
All claims expressed in this article are solely those of the authors and do not necessarily represent those of their affiliated organizations, or those of the publisher, the editors and the reviewers. Any product that may be evaluated in this article, or claim that may be made by its manufacturer, is not guaranteed or endorsed by the publisher.
Abbreviations
C, control; H, heat shock treatment; S, salinity; H + S, heat shock and salinity combined; H-S, heat shock pretreatment followed by salinity; S-H, salinity pretreatment followed by heat shock; SOD, superoxide dismutase; CAT, catalase.
References
Adir, N., and Lerner, N. (2003). The crystal structure of a novel unmethylated form of C-phycocyanin, a possible connector between cores and rods in phycobilisomes. J. Biol. Chem. 278, 25926–25932. doi: 10.1074/jbc.M302838200
Aebi, H. (1984). Catalase in vitro. Methods Enzymol. 105, 121–126. doi: 10.1016/S0076-6879(84)05016-3
Agrawal, C., Sen, S., Singh, S., Rai, S., Singh, P. K., Singh, V. K., et al. (2014). Comparative proteomics reveals association of early accumulated proteins in conferring butachlor tolerance in three N(2)-fixing Anabaena spp. J. Proteome 96, 271–290. doi: 10.1016/j.jprot.2013.11.015
Allakhverdiev, S. I., Nishiyama, Y., Miyairi, S., Yamamoto, H., Inagaki, N., Kanesaki, Y., et al. (2002). Salt stress inhibits the repair of photodamaged photosystem II by suppressing the transcription and translation of psbA genes in Synechocystis. Plant Physiol. 130, 1443–1453. doi: 10.1104/pp.011114
Bhargava, P., Kumar, A., Mishra, Y., and Rai, L. C. (2008). Copper pretreatment augments ultraviolet B toxicity in the cyanobacterium Anabaena doliolum: A proteomic analysis of cell death. Funct. Plant Biol. 35, 360–372. doi: 10.1071/FP07267
Cakmak, I., and Horst, W. J. (1991). Effect of aluminium on lipid peroxidation, superoxide dismutase, catalase, and peroxidase activities in root tips of soybean (Glycine max). Physiol. Plant. 83, 463–468.
Demiral, T., and Türkan, I. (2006). Exogenous glycinebetaine affects growth and proline accumulation and retards senescence in two rice cultivars under NaCl stress. Environ. Exp. Bot. 56, 72–79.
El Bissati, K., Delphin, E., Murata, N., Etienne, A.-L., and Kirilovsky, D. (2000). Photosystem II fluorescence quenching in the cyanobacterium Synechocystis PCC 6803: Involvement of two different mechanisms. Biochim. Biophys. Acta (BBA) Bioenergetics 1457, 229–242. doi: 10.1016/s0005-2728(00)00104-3
Ellison, C. R., Overa, S., and Boldor, D. (2019). Central composite design parameterization of microalgae/cyanobacteria co-culture pretreatment for enhanced lipid extraction using an external clamp-on ultrasonic transducer. Ultrason. Sonochem. 51, 496–503. doi: 10.1016/j.ultsonch.2018.05.006
Fan, Y., Yin, X., Xie, Q., Xia, Y., Wang, Z., Song, J., et al. (2019). Co-expression of SpSOS1 and SpAHA1 in transgenic Arabidopsis plants improves salinity tolerance. BMC Plant Biol. 19:74. doi: 10.1186/s12870-019-1680-7
Feng, Z. T., Deng, Y. Q., Zhang, S. C., Liang, X., Yuan, F., Hao, J. L., et al. (2015). K+ accumulation in the cytoplasm and nucleus of the salt gland cells of Limonium bicolor accompanies increased rates of salt secretion under NaCl treatment using NanoSIMS. Plant Sci. 238, 286–296. doi: 10.1016/j.plantsci.2015.06.021
Giannopolitis, C. N., and Ries, S. K. (1977). Superoxide dismutases: I. Occurrence in higher plants. Plant Physiol. 59, 309–314.
Girotti, A. W. (1990). Photodynamic lipid peroxidation in biological systems. Photochem. Photobiol. 51, 497–509.
Halliwell, B. (1999). Chemistry of free radical and related’reactive species’; Transition metal, Hydroxyl radical. Free Radic. Biol. Med. 53–55.
Halliwell, B., and Gutteridge, J. M. (2015). Free radicals in biology and medicine. Oxford: Oxford University Press.
Han, N., Lan, W., He, X., Shao, Q., Wang, B., and Zhao, X. (2012). Expression of a Suaeda salsa vacuolar H+/Ca2+ transporter gene in Arabidopsis contributes to physiological changes in salinity. Plant Molecular Biology Reporter 30, 470–477.
Hemlata Fatima, T. (2009). Screening of cyanobacteria for phycobiliproteins and effect of different environmental stress on its yield. Bull. Env. Contam. Toxicol. 83, 509–515.
Hu, I. (2019). Production of potential coproducts from microalgae, Biofuels from Algae. Amsterdam: Elsevier.
Karandashova, I., and Elanskaya, I. (2005). Genetic control and mechanisms of salt and hyperosmotic stress resistance in cyanobacteria. Russian J. Genet. 41, 1311–1321.
Kim, H., Dashdorj, N., Zhang, H., Yan, J., Cramer, W. A., and Savikhin, S. (2005). An anomalous distance dependence of intraprotein chlorophyll-carotenoid triplet energy transfer. Biophys. J. 89, L28–L30. doi: 10.1529/biophysj.105.069609
Kirilovsky, D., and Kerfeld, C. A. (2016). Cyanobacterial photoprotection by the orange carotenoid protein. Nat. Plants 2, 1–7.
Küpper, H., Spiller, M., and Kupper, F. C. (2000). Photometric method for the quantification of chlorophylls and their derivatives in complex mixtures: Fitting with Gauss-peak spectra. Anal. Biochem. 286, 247–256. doi: 10.1006/abio.2000.4794
Lawton, L., Marsalek, B., Padisak, J., and Chorus, I. (1999). “Determination in the laboratory,” in Toxic cyanobacteria in water: A guide to public health consequences, ed. F. Spon (New York, NY: Monitoring and Management), 347–367.
Liu, X., and Huang, B. (2000). Heat stress injury in relation to membrane lipid peroxidation in creeping bentgrass. Crop Sci. 40, 503–510.
Livak, K. J., and Schmittgen, T. D. (2001). Analysis of relative gene expression data using real-time quantitative PCR and the 2−ΔΔCT method. Methods 25, 402–408.
Lu, C., and Vonshak, A. (2002). Effects of salinity stress on photosystem II function in cyanobacterial Spirulina platensis cells. Physiol. Plant. 114, 405–413.
Macháèková, I. (1995). McKersie, BD, Leshem, YY: Stress and stress coping in cultivated plants. Biol. Plant. 37, 380–380.
Mahawar, H., Prasanna, R., Singh, S. B., and Nain, L. (2018). Influence of silver, zinc oxide and copper oxide nanoparticles on the cyanobacterium Calothrixelenkinii. BioNanoScience 8, 802–810.
Mishra, Y., Chaurasia, N., and Rai, L. C. (2009). Heat pretreatment alleviates UV-B Toxicity in the Cyanobacterium Anabaena doliolum: A proteomic analysis of cross tolerance. Photochem. Photobiol. 85, 824–833. doi: 10.1111/j.1751-1097.2008.00469.x
Murata, N., Takahashi, S., Nishiyama, Y., and Allakhverdiev, S. I. (2007). Photoinhibition of photosystem II under environmental stress. Biochim. Biophys. Acta (BBA) Bioenergetics 1767, 414–421.
Myers, J., and Kratz, W. (1955). Relations between pigment content and photosynthetic characteristics in a blue-green alga. J. General Physiol. 39, 11–22. doi: 10.1085/jgp.39.1.11
Narayan, O. P., Kumari, N., and Rai, L. (2011). Iron starvation-induced proteomic changes in Anabaena (Nostoc) sp. PCC 7120: Exploring Survival Strategy. J. Microbiol. Biotechnol. 21, 136–146. doi: 10.4014/jmb.1009.09021
Nonnengiesser, K., Schuster, A., and Koenig, F. (1996). Carotenoids and reaction center II-D1 Protein in light regulation of the photosynthetic Apparatus in Aphanocapsa. Botanica Acta 109, 115–124.
Panda, B., Basu, B., Rajaram, H., and Apte, S. K. (2014). Methyl viologen responsive proteomedynamics of Anabaena sp. strain PCC7120. Proteomics 14, 1895–1904.
Pandey, S., Rai, R., and Rai, L. (2012). Proteomics combines morphological, physiological and biochemical attributes to unravel the survival strategy of Anabaena sp. PCC7120 under arsenic stress. J. Proteome 75, 921–937. doi: 10.1016/j.jprot.2011.10.011
Parida, A. K., and Das, A. B. (2005). Salt tolerance and salinity effects on plants: A review. Ecotoxicol. Environ. Safety 60, 324–349.
Rafiqul, I. M., Hassan, A., Sulebele, G., Orosco, C. A., Roustaian, P., and Jalal, K. C. A. (2003). Salt stress culture of blue-green algae Spirulina fusiformis. Pak. J. Biol. Sci. (Pakistan) 6, 648–650.
Rai, L., and Raizada, M. (1985). Effect of nickel and silver ions on survival, growth, carbon fixation and nitrogenase activity in Nostoc muscorum: Regulation of toxicity by EDTA and calcium. J. General Appl. Microbiol. 31, 329–337.
Rai, R., Singh, S., Rai, K. K., Raj, A., Sriwastaw, S., and Rai, L. (2021). Regulation of antioxidant defense and glyoxalase systems in cyanobacteria. Plant Physiol. Biochem. 168, 353–372. doi: 10.1016/j.plaphy.2021.09.037
Rai, S., Agrawal, C., Shrivastava, A. K., Singh, P. K., and Rai, L. (2014). Comparative proteomics unveils cross species variations in Anabaena under salt stress. J. Proteome 98, 254–270. doi: 10.1016/j.jprot.2013.12.020
Rai, S., Sibgh, S., Shrivastava, A. K., and Rai, L. (2013). Salt and UV-B induced changes in Anabaena PCC 7120: Physiological, proteomic and bioinformatic perspectives. Photosynth. Res. 118, 105–114. doi: 10.1007/s11120-013-9931-1
Rippka, R., Deruelles, J., Waterbury, J. B., Herdman, M., and Stanier, R. Y. (1979). Generic assignments, strain histories and properties of pure cultures of cyanobacteria. Microbiology 111, 1–61.
Scarpeci, T. E., Zanor, M. I., Carrillo, N., Mueller-Roeber, B., and Valle, E. M. (2008). Generation of superoxide anion in chloroplasts of Arabidopsis thaliana during active photosynthesis: A focus on rapidly induced genes. Plant Mol. Biol. 66, 361–378. doi: 10.1007/s11103-007-9274-4
Shrivastava, A. K., Chatterjee, A., Yadav, S., Singh, P. K., Singh, S., and Rai, L. (2015). UV-B stress induced metabolic rearrangements explored with comparative proteomics in three Anabaena species. J. Proteome 127, 122–133. doi: 10.1016/j.jprot.2015.05.014
Singh, P. K., Shrivastava, A. K., Chatterjee, A., Pandey, S., Rai, S., Singh, S., et al. (2015). Cadmium toxicity in diazotrophic Anabaena spp. adjudged by hasty up-accumulation of transporter and signaling and severe down-accumulation of nitrogen metabolism proteins. J. Proteome 127, 134–146. doi: 10.1016/j.jprot.2015.05.019
Singh, S. P., and Montgomery, B. L. (2013). Salinity impacts photosynthetic pigmentation and cellular morphology changes by distinct mechanisms in Fremyelladiplosiphon. Biochem. Biophys. Res. Commun. 433, 84–89. doi: 10.1016/j.bbrc.2013.02.060
Singh, V. P., Srivastava, P. K., and Prasad, S. M. (2012). Differential effect of UV-B radiation on growth, oxidative stress and ascorbate–glutathione cycle in two cyanobacteria under copper toxicity. Plant Physiol. Biochem. 61, 61–70. doi: 10.1016/j.plaphy.2012.09.005
Song, Y., Li, J., Sui, Y., Han, G., Zhang, Y., Guo, S., et al. (2020). The sweet sorghum SbWRKY50 is negatively involved in salt response by regulating ion homeostasis. Plant Mol. Biol. 102, 603–614. doi: 10.1007/s11103-020-00966-4
Srivastava, A. K. (2010). Assessment of salinity-induced antioxidative defense system of diazotrophic cyanobacterium Nostoc muscorum. J. Microbiol. Biotechnol. 20, 1506–1512. doi: 10.4014/jmb.1005.05037
Srivastava, A. K., Bhargava, P., Mishra, Y., Shukla, B., and And Rai, L. C. (2006). Effect of pretreatment of salt, copper and temperature on ultraviolet-B-induced antioxidants in diazotrophic cyanobacterium Anabaena doliolium. J. Basic Microbiol. 46, 135–144. doi: 10.1002/jobm.200510059
Teikari, J., Österholm, J., Kopf, M., Battchikova, N., Wahlsten, M., Aro, E. M., et al. (2015). Transcriptomic and proteomic profiling of Anabaena sp. strain 90 under inorganic phosphorus stress. Appl. Environ. Microbiol. 81, 5212–5222. doi: 10.1128/AEM.01062-15
Verma, K., and Mohanty, P. (2000). Alterations in the structure of phycobilisomes of the cyanobacterium, Spirulina platensis in response to enhanced Na+ level. World J. Microbiol. Biotechnol. 16, 795–798.
Wannigama, D. L., Agrawal, C., and Rai, L. (2012). A comparative study on proteomic and biochemical alterations in the cyanobacterium Anabaena sp. PCC 7120 under short term exposure of abiotic stresses: Pesticide, salinity, heavy metal and UV-B 3rd world congress on biotechnology. Hyderabad, TG: International Convention Centre.
Wen, X., Gong, H., and Lu, C. (2005). Heat stress induces an inhibition of excitation energy transfer from phycobilisomes to photosystem II but not to photosystem I in a cyanobacterium Spirulina platensis. Plant Physiol. Biochem. 43, 389–395. doi: 10.1016/j.plaphy.2005.03.001
Yang, Z., Li, J. L., Liu, L. N., Xie, Q., and Sui, N. (2020). Photosynthetic regulation under salt stress and salt-tolerance mechanism of sweet sorghum. Front. Plant Sci. 10:1722. doi: 10.3389/fpls.2019.01722
Zhang, T., Gong, H., Wen, X., and Lu, C. (2010). Salt stress induces a decrease in excitation energy transfer from phycobilisomes to photosystem II but an increase to photosystem I in the cyanobacterium Spirulina platensis. J. Plant Physiol. 167, 951–958. doi: 10.1016/j.jplph.2009.12.020
Zhao, B., Wang, J., Gong, H., Wen, X., Ren, H., and Lu, C. (2008). Effects of heat stress on PSII photochemistry in a cyanobacterium Spirulina platensis. Plant Sci. 175, 556–564.
Keywords: cyanobacteria, Anabaena PCC7120, salinity, heat shock, pretreatments, stress
Citation: Srivastava R, Kanda T, Yadav S, Singh N, Yadav S, Prajapati R, Kesari V and Atri N (2023) Salinity pretreatment synergies heat shock toxicity in cyanobacterium Anabaena PCC7120. Front. Microbiol. 14:1061927. doi: 10.3389/fmicb.2023.1061927
Received: 05 October 2022; Accepted: 16 January 2023;
Published: 16 February 2023.
Edited by:
Prashant Kumar Singh, Mizoram University, IndiaReviewed by:
Riti Thapar Kapoor, Amity University Uttar Pradesh, IndiaAvinash Singh, New York University, United States
Vijay Pratap Singh, University of Allahabad, India
Copyright © 2023 Srivastava, Kanda, Yadav, Singh, Yadav, Prajapati, Kesari and Atri. This is an open-access article distributed under the terms of the Creative Commons Attribution License (CC BY). The use, distribution or reproduction in other forums is permitted, provided the original author(s) and the copyright owner(s) are credited and that the original publication in this journal is cited, in accordance with accepted academic practice. No use, distribution or reproduction is permitted which does not comply with these terms.
*Correspondence: Neelam Atri, bmVlbGFtMTQwOUBiaHUuYWMuaW4=