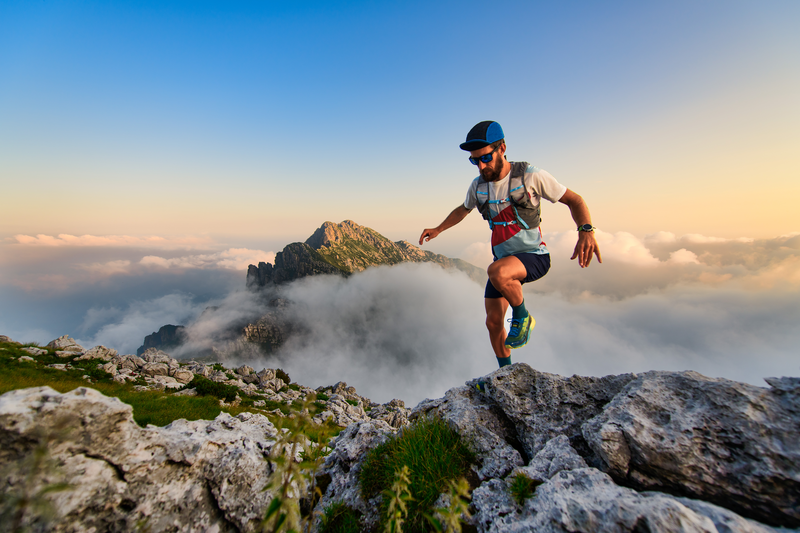
94% of researchers rate our articles as excellent or good
Learn more about the work of our research integrity team to safeguard the quality of each article we publish.
Find out more
REVIEW article
Front. Microbiol. , 09 February 2023
Sec. Microbial Symbioses
Volume 14 - 2023 | https://doi.org/10.3389/fmicb.2023.1061032
This article is part of the Research Topic Crosstalk Between the Microbiota and Host Mucosa: the Impact on Mucosa Development, Dysfunction and Diseases View all 9 articles
With the rapid development of metagenomic high-throughput sequencing technology, more and more oral mucosal diseases have been proven to be associated with oral microbiota shifts or dysbiosis. The commensal oral microbiota can greatly influence the colonization and resistance of pathogenic microorganisms and induce primary immunity. Once dysbiosis occurs, it can lead to damage to oral mucosal epithelial defense, thus accelerating the pathological process. As common oral mucosal diseases, oral mucositis and ulcers seriously affect patients’ prognosis and quality of life. However, from the microbiota perspective, the etiologies, specific alterations of oral flora, pathogenic changes, and therapy for microbiota are still lacking in a comprehensive overview. This review makes a retrospective summary of the above problems, dialectically based on oral microecology, to provide a new perspective on oral mucosal lesions management and aims at improving patients’ quality of life.
- We have produced an illustration representing the microbiota and its association with the oral cavity.
- The lotus leaf with lesions represents the subtle lesions and potential harm caused by dysbiosis, with the lotus flower expressing the health of oral mucosa.
The human body is similar to the planet, composed of many eco-systems made up of micro-eco-systems. Therefore, it is not hard to understand that the oral cavity (an integral part of the human body) hosts a great variety of microorganisms. Each microbiota has its own microenvironments, and the resident microorganisms living in this microenvironment have a harmonious balance (homeostasis) of nutrients, byproducts, pH, and temperature distributed either synergetically or symbiotically (Bugshan et al., 2017). Alteration by local or systematic factors will cause imbalance or what we call “dysbiosis,” leading to the proliferation of potentially pathogenic microorganisms, e.g., prolonged inflammation (Bugshan et al., 2017).
The oral cavity is the main point of entry into the human body. It is constantly exposed to extrinsic and intrinsic stimuli, thus resulting in it acting as both physical and immunological barriers (Wang et al., 2019). The physical nature of the mucosal layer is physically preventing the intrusion of external stimuli and the immunological barrier of it, are the ability it detecting microbial antigens and triggering immune responses (Presland and Jurevic, 2002; Novak et al., 2008). The mucosal layer is multilayered epithelial cells with cell-cell junctions, and the oral cavity is a unique site where both soft and hard tissue exist. Its polymorphous surface harbors several highly heterogeneous microbial niches. Shifts in microflora will result in a domino effect in those microbial niches. Therefore, it could be speculated that mucosal signs and conditions can be transient, persistent, and intermittent under biological, physical, and chemical exposures. Failure to respond to or eliminate the stimuli will result in prolonged inflammation and more complicated situations. Take candidiasis as an example of ulceration. Candida albicans infection was associated with microbiota dysbiosis which will result in epithelial junction protein E-cadherin degradation and C. albicans invasion (Bertolini et al., 2019). Several studies (Bugshan et al., 2017; France and Villa, 2020; Gasmi Benahmed et al., 2021) have proposed various hypotheses on microorganisms’ involvement in the processes of oral lesions, hoping to provide clues for the target for the relief of oral mucosal diseases. This current review focuses on how oral microbiota dysbiosis is associated with oral ulcers and what are the molecular biologic mechanisms of this association. The goal of this review is to try to provide possible ideas for future oral ulcer research and treatment intervention.
It has been proven that the occurrence of oral ulcers is closely related to the changes in the oral microbiota, such as shifts in the abundance of specific microorganisms and the emergence of new pathogenic microorganisms. Concurrently, the microbiota compositions in different oral habitats, such as mucosa, saliva, teeth, and tongue, are also different, which suggests that the microbiota changes in other oral habitats can influence the occurrence of ulcers, because saliva and chewing allow the microbiota of these habitats to interact with each other (Gao et al., 2018).
With the development of next-generation sequencing (NGS) and bioinformatics (Adami et al., 2021), more than 700 kinds of bacteria have been identified with various fungi, viruses, and protozoa in the oral cavity (Palmer, 2014). The Checkerboard DNA-DNA hybridization technique of the healthy host oral mucosal samples (comprised vestibule lip, attached gingiva, floor of mouth, buccal, tongue ventral, and hard palate) elucidated the abundance of native bacteria colonizing on these soft tissue surfaces, including S. mitis, C. gingivalis, G. morbillorum, V. parvula, E. corrodens, and etc. (Figure 1) (Mager et al., 2003). According to the Gram staining and bacterial morphology, the species of normal oral bacteria can be classified as follows:
Figure 1. Differences between dysbiosis and homeostasis of mucosal microbiota: (A) the dysbiosis of microbiota could be symbolized by the impaired epithelium, thinner biofilm attached to the epithelium, and dominant microflora being pathogens. (B) Microbiota homeostasis could be characterized by intact epithelium, thicker biofilm, and commensal microflora instead. (This figure was partially cited from Lin et al. (2021) with modifications).
Bac: Gram-positive:
1. Cocci–S. mitis, S. oralis, S. constellatus, P. micros, S. sanguis, S. anginosus, S. intermedius.
2. Rods–G. morbillorum, A. odontolyticus, E. saburreum, A. naeslundii 1, P. acnes, A. israelii, E. nodatum, A. naeslundii 2, A. gerencseriae, S. gordonii,
Bac: Gram-negative:
1. Cocci–V. parvula, N. mucosa.
2. Rods–C. gingivalis, E. corrodens, P. melaninogenica, F. peridonticum, L. buccalis, F. nucleatum ss polymorphum, P. nigrescens, F. nucleatum ss nucleatum, P. intermedia, C. gracilis, C. showae, C. rectus, A. actinomycetemcomitans, T. denticola, P. gingivalis, B. forsythus, S. C. sputigena, C. ochracea.
Fungi: Aureobasidium_sp, Filobasidium_wieringae, Podoscypha_involuta, Candida_albicans, Malassezia_sp, Cladosporium_sp, Malas-sezia_globosa, Debaryomyces_hansenii, Nigrospora_sp, Tremello-mycetes_sp, Alternaria_armoraciae, Aspergillus_sp, Daedaleopsis_confragosa (Stehlikova et al., 2019).
Protozoa: Entamoeba gingivalis, Trichomonas tenax (Zhou and Li, 2015; Deo and Deshmukh, 2019).
The microbiota imbalance and malfunction in the oral cavity is known as microbial dysbiosis. Studies have also suggested that specific microorganisms in the context of dysbiosis generate pathways that may potentially encourage the development of oral mucositis and prolonged the rehabilitation of existing ulcerations (Vasconcelos et al., 2016).
Clinical studies have used chemotherapy-induced mucositis patients as models for isolating bacterial strands, founding that the predominant strands were G. haemolysans and Streptococcus mitis, furthermore, the site where most bacterial changes were on the buccal mucosal layers (Napeñas et al., 2010). In lesions such as RAS (commonly found on the buccal, labial mucosa, and tongue areas), another study indicated that Rothia dentocariosa and Acinetobacter johnsonii are most likely to be linked with RAS and that the site of the most pronounced changes was the lower labial mucosa. Class Clostridia and genera Lachnoanaerobaculum, Cardiobacterium along with opportunistic pathogens such as Leptotrichia, and Fusobacterium increased in ulcerative samples, but a decrease in Streptococcus salivarius, Neisseria, and Veillonella species (Stehlikova et al., 2019). In active ulcers, the fungi Malassezia has its dominance followed by C. albicans, and the association between Malassezia and S. salivarius and Haemophilus is negative, whereas, the association is believed to be positive in Porphyromonas. The association between A. johnsonii and active ulceration was positive in the study, besides, an increase in A. johnsonii in the ulcerative site was also observed with a greater amount of Haemophilus parahaemolyticus or H. parainfluenza (Stehlikova et al., 2019).
In their experiments, Wang et al. (2019) concluded that the α diversity in normal and RAS patients was similar, but the fundamental microbial compositions of the two showed significant differences, embodying as that Firmicutes on the phylum level, Bacilli on the class level, Lactobacillales on the order level, Streptococcaceae on the family level, and Streptococcus on the genus level were prominent in the normal, whose result echoed the above findings that there was more taxonomic abundance of Streptococcus in normal patients than in RAS. In contrast, RAS patients exert more abundant Enterobacteriales on the order level, Enterobacteriaceae on the family level, Escherichia and Alloprevotella on the genus level, and E. coli on the species level. In summary, increased E. coli and Alloprevotella and decreased Streptococcus in microbial communities will most likely be associated with RAS.
The presence of oral mucosal commensal microbiota is important to the establishment of protective/homeostatic immune responses as it provides low dosage of pathogenic stimulation. This stimulation could sustain the activity of normal functional immune system.
The lipopolysaccharide (LPS) of Gram-negative bacteria can effectively activate toll like receptor 4 (TLR4) through the pathway of vesicular acidification. Meanwhile, the activation is weakened during the second induction to maintain the microbial immune homeostasis of the oral mucosa (Wu et al., 2015; Kantrong et al., 2019). Similarly, TLRs also mediate immune regulation toward fungi and viruses (Groeger and Meyle, 2019). Interleukin-17 (IL-17)-expressing cells (type 17 cells) also play an essential role in pathogen-associated molecular patterns (PAMPs) and inflammation recognition and response in oral mucosa through the binding of expressed C-C chemokine receptor 6 (CCR6) to mucosal C-C chemokine ligand 20 (CCL20) and the binding of IL-17 to its heterotrophic receptor (IL-17RA and IL-17RC) found mainly in epithelial cells (Acosta-Rodriguez et al., 2007; Li et al., 2019).
From the perspective of metabolites, nitric oxide (NO) produced as the metabolic products of oral microbiota exerts a positive influence on the prevention and control of oral diseases as it could activate macrophages, and then proceed with macrophage inhibition and phagocytosis of bacteria (Brennan et al., 2003; Hezel and Weitzberg, 2015; Bandara et al., 2019). Meanwhile, bacteriocin and hydrogen peroxide produced by some bacteria can antagonize other bacteria (Heilbronner et al., 2021). Rosier et al. (Maekawa et al., 2014) have confirmed that Porphyromonas gingivalis can affect the clearance of bacteria and inhibit the immune response. There are also inverse relationships in fungi, for instance, Pichia can inhibit the growth of potential pathogens, such as Candida, Aspergillus, and Fusarium (Baumgardner, 2019).
At the population level, the oral microbiota with higher α diversity can retain a higher capacity of resistance to invasive microorganisms and antibiotics. In contrast, extremely low β diversity of oral microbiota indicates that the microbiota of different hosts is highly homogenous and conserved (D’Agostino et al., 2022; Xu et al., 2022). The α diversity and salivary compositions assure that despite other influential factors, it will have certain degrees of buffer and resistance to assemble residency microbiota’s core functions in hosts without much variation in its microbial compositions (Rosier et al., 2018).
It has been reported that the number of smokers around the world is 1.14 billion, and the number of males is about five times that of females. Besides, about 30% of global smokers were from China in 2019 (Zhou et al., 2019; GBD 2019 Tobacco Collaborators, 2021). Smoking can inhibit the phagocytosis of macrophages and neutrophils by inhibiting bacterial stimulated expression of superoxide and surface receptors (e.g., TLR2), thus weakening the clearance of newly colonized microorganisms. Meanwhile, smoking may also induce the receptor activator of nuclear factor- κβ Ligand (RANKL) to upregulate the levels of IL-1 and IL-6, affecting the oral immune response to the microbiota (Matthews et al., 2012; Ahmed et al., 2021), as shown in Figure 2. In addition, smoking also changes the microbiota in the oral mucosa by affecting biofilm formation and adhesions of specific microorganisms (e.g., Staphylococcus aureus and Streptococcus pneumoniae) (Kulkarni et al., 2012; Mutepe et al., 2013). Studies have found that compared with non-smokers, smokers have higher oral microbiota α diversity with Firmicutes, Bacteroidetes and Actinobacteria significantly increasing, but the relative abundance of phyla Bacteroidetes, Fusobacteria, Proteobacteria, Neisseria, Branhamella, Porphyromonas, and Gemella was decreased (Morris et al., 2013; Vallès et al., 2018; Huang and Shi, 2019). It has been reported that compared with non-smokers, smokers are more prone to actinomycosis and have more significant levels of fugal CFU/ml (Abduljabbar et al., 2017; Angst et al., 2019). However, smoking is not the fundamental cause of RAS. Sawair (2010) found that smoking might increase the thickness of the squamous epithelial cell layer to reduce ulcer occurrence. In short, smoking does not show a causal relationship with the occurrence of ulcers, but the impact of smoking on oral mucosal microbiota and immunity does have a repercussion on the progress of ulcers and mucositis from other aspects.
Figure 2. Factors leading to the microbiota shifts: (A) smoking exerts a significant influence on dysbiosis by microbiota carried by immune reaction inhibition and upregulation of IL-1/6 induced by receptor activator of nuclear factor- κβ Ligand (RANKL). (B) Radiotherapy could initiate oral mucositis induced by damage-associated molecular patterns (DAMPs) along with lipopolysaccharide (LPS). (C) Hematopoietic stem cell transplantation impacts the flora alterations in a way undiscovered.
Radiotherapy and chemotherapy are the most commonly used methods for treating head and neck tumors in addition to surgery. However, more than 80% of patients will develop oral mucositis after radiotherapy, namely, radiation-induced oral mucositis (RIOM) (Al-Dasooqi et al., 2013; Bockel et al., 2018). Therefore, it is of clinical value to explore whether radiotherapy accelerates the occurrence of oral ulcerations and mucositis from the perspective of microbiota dysbiosis to improve patients’ prognosis and quality of life. The research of Hou et al. (2018) shows that radiotherapy has no significant effect on the overall microbiota abundance, but it has a dose-dependent effect on the specific components of the microbiota. At the level of phylum, with the increase of radiation dose, the relative abundance of Proteobacteria and Spirochaetes increases significantly, while Fusobacteria decreases. At the genus level, the relative abundance of Prevotella, Fusobacterium, Leptotrichia, Campylobacter, Peptostreptococcus, and Atopobium decreases, while Pseudomonas, Treponema, Granulicatella, and Capnocytophaga shows an upward trend (Hou et al., 2018). Another study on patients of oral cancer after radiotherapy showed that the increase of S. aureus and P. aeruginosa in oral mucosa after radiotherapy was statistically significant, while Escherichia coli dropped to a lower level after 6 weeks of radiotherapy (Subramaniam and Muthukrishnan, 2019). Stringer et al. further found that damage-associated molecular patterns (DAMPs) induced by radiotherapy, such as HMGB1, can bind to and activate TLR4. This leads to the initiation of the inflammatory cascade through interaction with bacterial LPS, which is shown in Figure 2. TLR4 signaling pathway can stimulate host cells to produce proinflammatory cytokines, such as tumor necrosis factor-α (TNF-α), thereby forming a full-thickness ulcer in the oral mucosa epithelium cell layers (Stringer and Logan, 2015). However, in combination with the role that TLR4 could induce basic protective immunoreaction, TLR4 plays dual roles in protection and injury; thus, it might be a drug-targeting site to prevent dysbiosis-induced ulcer deterioration.
Hematopoietic stem cell transplantation (HSCT) is a common treatment for hematological diseases. Almost 60–80% of the patients who receive treatment will develop oral mucositis (Villa and Sonis, 2016). It is worth noting that more and more studies have shown that HSCT can lead to oral microbiota dysbiosis and exaggerate inflammatory status (Hou et al., 2018; Laheij et al., 2019; Miranda-Silva et al., 2020), therefore, it is hypothesized that relieving microbiota dysbiosis would contribute to reduce the HSCT treatment complications. Previous studies have shown that bacteria and fungi, such as P. gingivalis, Enterococcus sp., and Candida are all associated with the occurrence of RAS after HSCT treatment (de Mendonça et al., 2012; Osakabe et al., 2017). Besides, Laheij et al. (2019) found that 3 months after the termination of HSCT treatment, the oral microbiota of the patients with RAS almost recovered to the same level as before. But within one or 2 weeks after the termination of HSCT treatment, there were significant component changes. Overall, Streptococcus australis/parasanguinis/OT057/OT066, Veillonella atypica/dispar, genus Actinomyces, Actinomyces sp. OT172, Actinomyces graevenitzii, and Gemella haemolysans/morbillorum/sanguinis are predominant before treatments, while S. aureus/caprae/epidermidis/warneri, Scardovia wiggsiae, Enterococcus faecalis, and Lactobacillus fermentum superseded the main species of the changed flora under HSCT treatment, although there is no significant changes in fungi (Laheij et al., 2019). Furthermore, the study by Muro et al. (2018) elucidated that after HSCT treatment, Staphylococcus spp., Enterococcus spp., and Lautropia mirabilis of, which are rarely detected in normal mucosal microbiota, were identified. The research of Ohbayashi et al. (2021) also showed that the normal flora of oral mucosa decreased to 25.5% after HSCT treatments, and the flora is mainly composed of coagulase-negative Staphylococci (39.4%), Enterococcus sp. (11.7%), Candida sp. (9.6%), and Stenotrophomonas maltophilia (2.1%), which was different from that before. However, the limitation of the above research is that chemotherapy or antibiotics will inevitably be used in HSCT treatments, and it is still challenging to determine whether HSCT is a direct factor in the alteration of the oral flora or not. Therefore, only when mouse models received only HSCT was created, the single factor affected microbiota variables can be obtained in turn.
Ultimately, it is unclear whether changes in oral microbiota caused by smoking, radiation, and HSCT are the causes or influencing factors of oral ulcers, with the timeline and correlation between the change of colony and the occurrence of ulcers to be evaluated. Nevertheless, the impact of the change of colony on the development and healing process of ulcers is undeniable; thus, the above research results are still of great significance in guiding ulcer treatments.
Research conducted by Hong et al. (2019) explained that patients under chemotherapy would have dysbiosis-mediated mucositis due to increasing inflammatory factors released into the oral cavity. Moreover, tissue damage will cause the release of reactive oxygen species (ROS) and their DNA materials. Then DNA breakage will lead to the activation of apoptotic pathways, which are regulated by p53 activation, caspase-3 elevation, and endogenous DAMPs (Triarico et al., 2022). The process mentioned above is the so-called phase I of mucositis development, synchronously marking the initiation of phase II mucositis. During phase II, inured mucosal cells promoted transcriptional factors such as nuclear NF-κB, which is an essential transcriptional mediator that governs over 200 genes associated with various proinflammatory cytokines involved in the early degradation of connective tissue, endothelium, and adhesion molecules apoptosis (e.g., TNF-α, IL-6, and IL-1β) (Triarico et al., 2022).
Meanwhile, other signaling pathways work coordinately by activating a positive feedback loop system, which is marked as phase III. TNF-α initiates mitogen-activated protein kinase (MAPK) activation on targeted cells while sustaining NF-κB processes. MAPK signaling pathways also mediate caspase-3 activation. Usually, at this stage, ulceration will be protracted as oral dysbiosis and leads to additional proinflammatory cytokines released by infiltrating mononuclear cells (phase IV). It is in phase IV that former studies have shown that cytotoxic T lymphocytes (CTLs) contributed to oral mucosal epithelial cells apoptosis, and enriched pathogens with their antigens at the ulcerative mucosa, such as LPS and hemolysin, can stimulate the host to produce a proliferative response (PR) of peripheral blood mononuclear cells (PBMCs) and T cells, then CTLs infiltrate oral mucosal epitheliums and lamina propria to deteriorate the mucosal damage (Sun et al., 2002; Suárez et al., 2020). In consequence, it can be seen that both innate immunity and adaptive immunity play important roles in dysbiosis-mediated oral ulcers. The lesions are believed to start repair during the phase V healing stage, which is characterized by epithelial cell proliferation and cellular differentiation aiming at restoring epithelial cells’ integrity (Singh and Singh, 2020). Subsequently, this section will elaborate on the role of oral dysbiosis in oral ulcers from the perspective of innate immunity and adaptive immunity shown in Figure 3.
Figure 3. Dysbiosis-induced immunoreaction: (a) innate immunity: lipopolysaccharide (LPS) and Peptidoglycan (PTG) contribute to the activation of immune and inflammatory responses in Th1/2. (b) Adaptive immunity: Porphyromonas gingivalis enhanced Th2 cytokine-mediated inflammatory immune response by prompting the secretion of IL-33 of epithelium, and antigen presentation by antigen–presenting cells (APCs) could activate Th1/2 by signal 1 and signal 2. (c) Candida albicans producing cytokines such as IL-1/IL-36 mediates the expression of IL-17 and the proliferation of Th17 cells, and short-chain fatty acids produced by microbiota facilitate the viability of Th17 with regulating the Tregs.
Peptidoglycan (PTG) and LPS are the components of bacteria that can act as ligands for TLR2 and TLR4, respectively, to activate PAMPs and then the innate immune system. Meanwhile, macrophages also respond to antigen stimulation by upregulating the expression of membrane TLR2/4 (Liang et al., 2013; Dabbagh et al., 2014). In Behçet’s disease (BD) patients with oral ulcers, the oral microbiota seems to lead to the excessive persistence of inflammation by inducing the abnormal polarization of M1/M2, which is related to the reduction of CCR1 of M2 in BD patients, with CCR1 attenuating the chemotaxis effect of M2 on MIP-1α and decreasing the expression of IL-10. Eventually, the macrophages at the ulcerative site gradually change into the M1 dominant paradigm, that is, inflammatory injury type (Hirahara et al., 2022). Rosier et al. ulteriorly have confirmed that P. gingivalis can cause crosstalk between the C5a receptor (C5aR) and TLR2, thereby increasing the inflammatory response, affecting the clearance of other bacteria and interfering with the healing. In the meantime, P. gingivalis can also inhibit the immune response by secreting a kind of LPS and serine phosphatase to inhibit the expression of TLR4 and IL-8 (Maekawa et al., 2014). Moreover, a large number of S. sanguinis-like microorganisms in the ulcers of RAS patients can promote Langerhans cells to present the bacteria-induced homologous peptides within epithelial heat shock proteins and then act as a bridge to activate humoral and cellular immunity simultaneously (Stehlikova et al., 2019).
In terms of humoral immunity, Stehlikova et al. (2019) found that anti-M. timidum IgG and anti-C. albicans IgA was significantly elevated in patients with RAS, indicating that specific microorganisms at the ulcer activated humoral immunity, and IgG and IgA secreted by B2 cells further induced antibody-dependent cell-mediated cytotoxicity (ADCC) to clear pathogenic bacteria. In cellular immunity, Groeger and Meyle (2019) illustrated that P. gingivalis could mediate epithelial cells to enhance the secretion of IL-33, thereby enhancing Th2 cytokine-mediated inflammatory immune response. Otherwise, intercellular adhesion molecules (ICAM), such as carcinoembryonic Ag-related cell adhesion molecule 1 (CEACAM1), are highly expressed in the junctional epithelium. They serve as receptors for various bacterial antigens, for instance, CEACAM1 will further interact with infiltrating polymorphonuclear (PMN) and T cells, ultimately enhancing the cytotoxicity (e.g., Perforin) of T cells and NK cells (Suárez et al., 2021).
In a healthy state, mechanical injury such as chewing can make oral mucosa produce IL-6 and promote the basic proliferation of Th17 (Kimura and Kishimoto, 2010). However, the activation of Th17 in oral diseases is more dependent on the change in microbiota. For instance, excessive hemolysin produced by C. albicans induces cell damage and c-Fos-driven intrinsic inflammatory reaction, during which damaged cells produced cytokines such as IL-1/IL-36, sequentially mediating the expression of IL-17 and the proliferation of Th17 cells (Conti and Gaffen, 2010). Besides, oral microbiota regulates Tregs and Th17 cells by producing short-chain fatty acids, whereby Tregs enhance the activity of Th17 cells by consuming IL-2 and reverse the inhibitory effect of IL-2 on Th17 cell differentiation, thereby, enhancing the Th17-mediated clearance of C. albicans in the oral cavity (Gaffen and Moutsopoulos, 2020).
The current hypothesis suggests factors: genetic predisposition, viral, bacterial, microelement deficiencies, hormonal, stress, mechanical stimuli, food allergies and immuno-deficiencies to be strongly associated with oral mucosal diseases (OMD) (Alrashdan et al., 2016; Abduljabbar et al., 2017; Vila et al., 2020). These factors, however, are highly correlated with microbiota dysbiosis in the oral cavity. Genetic expression analysis for patients under chemotherapy has found that during chemotherapy-induced dysbiosis, the abundance of F. nucleatum is increased at the mucositis lesion sites. This increase of F. nucleatum will provoke significant upregulation of TNF, CCL20, IL-17C, CXCL2, PMAIP1, DEFB4A, and DEFB103A, among which TNF could activate NF-κB and trigger apoptosis or necroptosis of epithelium via its extrinsic pathways (Hong et al., 2019). Another category of genes that may have a role in the induction of mucosal cell apoptosis in this process was the up-regulation of PMAIP. According to Hong et al. (2019) it was the gene encoding protein NOXA [it is a protein of proapoptotic Bcl2 homology 3 (BH3)], then it triggers a series of cascade-leading cell deaths. As mentioned previously, candidiasis is a common lesion for patients with compromised immune systems. When there is a disruption in the microbial equilibrium, i.e., immunosuppression activities, certain bacteria (such as Enterococcus species) will overgrow and establish a mutualistic network with C. albicans, and this change or dysbiosis will prolong the inflammatory responses and amplifies mucosal damage (Bertolini et al., 2019).
Compared with oral microbiota, the research on gastrointestinal microbiota is more in-depth. A recent review based on regulating microbiota to treat diseases mentioned that the intake of fecal microbiota transplantation (FMT), symbolic microbiological consortia, prebiotics, microbiota-derived proteins, and metabolites is expected to be effective in reconstructing a healthy microbiota (Sorbara and Pamer, 2022). This section focuses on the emerging methods for treating oral ulcers through microbiota regulation from three aspects: oral microbiota transplantation (OMT), probiotics, and photodynamic therapy (Figure 4).
Figure 4. Microbiota in prevention and therapy of mucositis and ulcerations: (A) oral microbiota transplantation (OMT) was carried out in dogs and found that the relative abundance of Chlorobi g-1 sp., Neisseria sp., and Bergeyella sp. increased rather than α-diversity; while downregulating the Lactobacillaceae but increasing the α-diversity in mice. (B) Photobiomodulation therapy is demonstrated to inhibit the Haemophilus, Neisseria, and Prevotella, instead benefiting the commensal Streptococcus, meanwhile which is lethal to HSV-1, too. (C) Probiotics including Lactobacillus, Bifidobacterium, Escherichia coli, and Escherichia faecalis, could neutralize the lipopolysaccharide (LPS) of Porphyromonas gingivalis by secreting the reuterin and inhibiting Pasteurella and anaerobic bacteria by downregulating the expression of gene encoding nitrate reduction (napA).
Compared with FMT, which has been widely used in clinics, the research on OMT is still constrained to animal experiments. Beikler et al. (2021) performed OMT in dogs with periodontitis, there is almost no difference in the abundance of the microbiota between the treated and control groups before receiving OMT. While 2 weeks after receiving OMT, the α-diversity of the oral microbiota of the treated group was significantly lower than that of the control and was most similar to the oral microbiota of the donor, with the relative abundance of ASV40 (Chlorobi g-1 sp.), ASV150 (Neisseria sp.) and ASV44 (Bergeyella sp.) increased. Another study (Xiao et al., 2021) in mice with RIOM showed that the level of IL-1/6 and TNF-α in tongue tissue and plasma of mice decreased after OMT treatments. From the perspective of microbiota, OMT reversed the rise of Lactobacillaceae in RIOM and gave the oral microbiota higher α-diversity. Later research further showed that S100A9 decreased in RIOM, but significantly increased after OMT, which might indicate that the level of S100A9 plays an important indicative role in both inflammation level and cancer prognosis (Xiao et al., 2021). The clinical application of OMT depends on a variety of factors, including the immune function of the recipient, the composition of the oral microbiota of the recipient, the screening of the healthy microbiota of the donor, and the pathogen screening of the donor microbiota (Beikler et al., 2021; Xiao et al., 2021). Accidentally, transplantation of pathogenic microorganisms could cause serious consequences such as periodontitis, caries, or even inflammatory bowel disease (IBD), arthritis, colorectal and pancreatic cancers, and Alzheimer’s disease (Tuganbaev et al., 2022), so OMT is not a long-term solution (Nascimento, 2017). Therefore, symbolic microbial consortia and engineered symbolic bacteria may become more targeted, universal, and ideal development directions.
Photobiomodulation therapy (PBMT) has gradually become a potential therapeutic method for many oral diseases, such as oral lichen planus (OLP), RAS, hyperpolarization, trigeminal neuralgia (TN), etc. PBMT can achieve anti-inflammatory and analgesic functions, promote wound healing and regeneration of damaged peripheral nerve tissues through the photoreceptors of mitochondria and Ca2+ channels of cell membranes (Kalhori et al., 2019), and there are no apparent side effects (Özberk et al., 2018). However, the specific effects of PBMT on oral microbiota need further elucidation. Some studies have shown that PBMT can form cytotoxic and highly lethal single oxygen to kill bacteria by laser excitation in the presence of endogenous oxygen (Kwiatkowski et al., 2018). A study on the application of PBMT on acute necrotizing ulcerative gingivitis treatment showed that the overall diversity of oral microbiota decreased after treatment, and the number of T. microdentium, F. nucleatum, and P. intermedia all decreased (Siddiqui et al., 2020). In addition, Zanotta et al. carried out a more in-depth test in rats, which showed that topical PBMT after indocyanine green (ICG) washing could produce a strong killing effect against Gram-positive bacteria and drug-resistant bacteria such as methicillin-resistant S. aureus, thereby inhibiting inflammation and promoting ulcer healing and fibrosis (Motamedifar et al., 2021). After PBMT for patients with RIOM, it was found that the number of pathogenic bacteria in the oral mucosa of patients decreased, including Haemophilus (from 15 to 11%), Neisseria (from 11 to 8%), and Prevotella (from 32 to 24%). However, it was accompanied by the increase of commensal bacteria Streptococcus (from 13 to 22%), which seems to indicate that the bactericidal effect of PBMT is selective and needs to be further explored (Zupin et al., 2018). In addition to bacteria, PBMT can also facilitate to repair oral ulcers caused by HSV-1 (Zupin et al., 2018). Owing to different light wavelengths having different antiviral mechanisms, the red laser may activate the antiviral immune response and inhibit virus replication, while the blue laser still needs to be further explored (Zanotta et al., 2020). In summary, compared with antibiotics, photosensitizers combined with PBMT may be very promising in microbial dysbiosis control, but the photosensitizer dose and light wavelength with unified standards still need to be clarified further. In addition, the oral mucosa of the recipient is required to have as low microbial abundance as possible before OMT, so PBMT combined with OMT may become a powerful method to treat oral ulcers in the future.
Probiotics can regulate the oral microbiota by colonizing the oral mucosa, producing specific metabolites to maintain the ecological balance of the host microbiota, and achieving a healthy state of moderate immunity (Sarao and Arora, 2017). This is different from the OMT mentioned above or symbolic microbiological consortia. For example, the introduction of probiotics is usually limited to a few species, without the disadvantage of introducing pathogenic bacteria. Simultaneously, taking probiotics is not to rebuild the flora, but to regulate the whole flora through the action of a few bacteria, therefore, the original microbiota of the subject is greatly preserved. Probiotics mainly include Lactobacillus, Bifidobacterium, E. coli, E. faecalis, and etc. It has been proven that probiotics can inhibit the growth, adhesion, proliferation, and biofilm formation of C. albicans (Janczarek et al., 2016; Hoare et al., 2017). Furthermore, Han et al. found that compared with pathogenic bacteria alone or the control group, the palate lesion treated with the mixture of L. reuteri (probiotic) and P. gingivalis (pathogen) has a faster healing rate. This phenomenon can be explained as reuterin produced by L. reuteri could degrade LPS of P. gingivalis, which ulteriorly inhibits LPS induced NLRP3 inflammasome (Han et al., 2020). Last but not least, Wang et al. (2021) tested in the mice of RIOM and found that S. salivarius K12 partially reconstituted the oral mucosa microbiota of RIOM mice, resulting in a significant reduction of Pasteurella and anaerobic bacteria by downregulating the expression of the gene encoding nitrate reduction (napA).
Oral microbiota dysbiosis is a multi-factor and multi-dimensional shift of the native microorganisms in the oral cavity. This microbiota shift will replace mutualistic/symbiotic microorganisms with potentially pathogenic microorganisms. The exact bacterial strands change in the microflora during the different onset and development stages of oral lesions is unknown, leaving room for future quantitative populational studies to be carried out. From the perspective of etiologies, medical treatments such as chemotherapy, antibiotics, and prosthodontic repairments can potentially also induce dysbiosis; thus, knowing the necessity of different risk factors will aid patient prognostic management (Brantes et al., 2019; Hong et al., 2019; Aguglia et al., 2020; Cardona et al., 2020; Neelakantan and Solomon, 2022). Based on current studies, once microbiota shifts participate in the progress of oral lesions, this dysbiosis will provide the foundation for the subsequent viral and fungal infections, such as Histoplasma capsulatum, cytomegalovirus, herpes simplex virus, Trichomonas spp, Leptospira, Treponema pallidum, etc. (Figueira et al., 2017; Folk and Nelson, 2017; Ito et al., 2019; Chen and Wang, 2020; Dmytrenko et al., 2020; Keah et al., 2022), which is principally attributed to their independent virulent factors, thereby they are not ulteriorly clarified in this review.
Microbiota dysbiosis may also lead to epigenetic changes by modification of genes and histones, regulation of non-coding RNA, etc., with gene serotonin transporter gene (SLC6A4), mannose-binding lectin 2 (MBL2), and so forth being susceptible to alterations of oral flora, whose molecular mechanism needs to be further ascertained (Bankvall et al., 2020; Slezakova et al., 2020; Baioumy et al., 2021; Bartakova et al., 2022). In the aspects of remedy of oral flora, some experiments proposed new approaches and hypotheses for utilizing herbal medicines or nutrients like curcumin, garlic, aloe vera, and oregano oil, which might be a promising domain of research in the future (Mahattanadul et al., 2018; Hoglund et al., 2020; Shi et al., 2020; Hosny et al., 2021). Our review focused mainly on oral mucositis RAS; however, mechanical trauma and psychological factors induced oral lesions are not mentioned; whether these proposed factors will influence microbiota shifts also remains to be explored.
ZM and LY contributed to the conception and design of the work, drafting the manuscript, made final approval of the version to be published, and agreed to be accountable for all aspects of the work in ensuring that questions related to the accuracy or integrity of any part of the work are appropriately investigated and resolved. YH contributed to the interpretation of data for the work, made the figures, drafting the manuscript, made final approval of the version to be published, and agreed to be accountable for all aspects of the work in ensuring that questions related to the accuracy or integrity of any part of the work are appropriately investigated and resolved. RH contributed to the conception and design of the work, revised the manuscript, made final approval of the version to be published, and agreed to be accountable for all aspects of the work in ensuring that questions related to the accuracy or integrity of any part of the work are appropriately investigated and resolved. All authors contributed to the article and approved the submitted version.
This work was partially supported by the National Natural Science Foundation of China (NSFC 31800114).
The authors declare that the research was conducted in the absence of any commercial or financial relationships that could be construed as a potential conflict of interest.
All claims expressed in this article are solely those of the authors and do not necessarily represent those of their affiliated organizations, or those of the publisher, the editors and the reviewers. Any product that may be evaluated in this article, or claim that may be made by its manufacturer, is not guaranteed or endorsed by the publisher.
Abduljabbar, T., Al-Askar, M., Baig, M. K., AlSowygh, Z. H., Kellesarian, S. V., and Vohra, F. (2017). Efficacy of photodynamic therapy in the inactivation of oral fungal colonization among cigarette smokers and non-smokers with denture stomatitis. Photodiagnosis Photodyn. Ther. 18, 50–53. doi: 10.1016/j.pdpdt.2017.01.182
Acosta-Rodriguez, E. V., Rivino, L., Geginat, J., Jarrossay, D., Gattorno, M., Lanzavecchia, A., et al. (2007). Surface phenotype and antigenic specificity of human interleukin 17-producing T helper memory cells. Nat. Immunol. 8, 639–646. doi: 10.1038/ni1467
Adami, G. R., Ang, M., and Kim, E. (2021). The oral microbiome: Methods and protocols. New York, NY: Springer. doi: 10.1007/978-1-0716-1518-8
Aguglia, A., Amerio, A., Proietti, L., Costanza, A., Serafini, G., and Amore, M. (2020). Oral mucositis induced by risperidone: Rare side effect of a frequently prescribed medication. Gen. Psychiatry 33:e100228. doi: 10.1136/gpsych-2020-100228
Ahmed, N., Arshad, S., Basheer, S. N., Karobari, M. I., Marya, A., Marya, C. M., et al. (2021). Smoking a dangerous addiction: A systematic review on an underrated risk factor for oral diseases. Int. J. Environ. Res. Public health 18:11003. doi: 10.3390/ijerph182111003
Al-Dasooqi, N., Sonis, S. T., Bowen, J. M., Bateman, E., Blijlevens, N., Gibson, R. J., et al. (2013). Emerging evidence on the pathobiology of mucositis. Support. Care 21, 3233–3241. doi: 10.1007/s00520-013-1900-x
Alrashdan, M. S., Cirillo, N., and McCullough, M. (2016). Oral lichen planus: A literature review and update. Arch. Dermatol. Res. 308, 539–551. doi: 10.1007/s00403-016-1667-2
Angst, P. D. M., Finger Stadler, A., Mendez, M., Oppermann, R. V., van der Velden, U., and Gomes, S. C. (2019). Supportive periodontal therapy in moderate-to-severe periodontitis patients: A two-year randomized clinical trial. J. Clin. Periodontol. 46, 1083–1093. doi: 10.1111/jcpe.13178
Baioumy, S. A., Fouad, S. H., Abdalgeleel, S. A., Baiomy, A. A., Sallam, D. E., and Taha, S. I. (2021). Mannose-binding lectin serum levels and (Gly54asp) gene polymorphism in recurrent aphthous stomatitis: A case-control study. Int. J. Immunopathol. Pharmacol. 35, 20587384211064454. doi: 10.1177/20587384211064454
Bandara, H., Panduwawala, C. P., and Samaranayake, L. P. (2019). Biodiversity of the human oral mycobiome in health and disease. Oral Dis. 25, 363–371. doi: 10.1111/odi.12899
Bankvall, M., Östman, S., Jontell, M., and Torinsson Naluai, Å (2020). A family-based genome-wide association study of recurrent aphthous stomatitis. Oral dis. 26, 1696–1705. doi: 10.1111/odi.13490
Bartakova, J., Deissova, T., Slezakova, S., Bartova, J., Petanova, J., Kuklinek, P., et al. (2022). Association of the angiotensin I converting enzyme (ACE) gene polymorphisms with recurrent aphthous stomatitis in the Czech population: Case-control study. BMC Oral Health 22:80. doi: 10.1186/s12903-022-02115-3
Baumgardner, D. J. (2019). Oral fungal microbiota: To thrush and beyond. J. Patient Cent. Res. Rev. 6, 252–261. doi: 10.17294/2330-0698.1705
Beikler, T., Bunte, K., Chan, Y., Weiher, B., Selbach, S., Peters, U., et al. (2021). Oral microbiota transplant in dogs with naturally occurring periodontitis. J. Dent. Res. 100, 764–770. doi: 10.1177/0022034521995423
Bertolini, M., Ranjan, A., Thompson, A., Diaz, P. I., Sobue, T., Maas, K., et al. (2019). Candida albicans induces mucosal bacterial dysbiosis that promotes invasive infection. PLoS Pathog 15:e1007717. doi: 10.1371/journal.ppat.1007717
Bockel, S., Vallard, A., Lévy, A., François, S., Bourdis, M., Le Gallic, C., et al. (2018). Pharmacological modulation of radiation-induced oral mucosal complications. Cancer Radiother. 22, 429–437. doi: 10.1016/j.canrad.2017.11.006
Brantes, M. F., Azevedo, R. S., Rozza-de-Menezes, R. E., Póvoa, H. C., Tucci, R., Gouvêa, A. F., et al. (2019). Analysis of risk factors for maxillary denture-related oral mucosal lesions: A cross-sectional study. Med Oral Patol. Oral Cir. Bucal 24, e305–e313. doi: 10.4317/medoral.22826
Brennan, P. A., Thomas, G. J., and Langdon, J. D. (2003). The role of nitric oxide in oral diseases. Arch. Oral Biol. 48, 93–100. doi: 10.1016/S0003-9969(02)00183-8
Bugshan, A., Farag, A. M., and Desai, B. (2017). Oral complications of systemic bacterial and fungal infections. Atlas Oral Maxillofac. Surg. Clin. North Am. 25, 209–220. doi: 10.1016/j.cxom.2017.04.012
Cardona, A. F., Ruiz-Patiño, A., Ricaurte, L., Zatarain-Barrón, Z. L., Barrón, F., and Arrieta, O. (2020). Chronic and severe non-lichenoid oral ulcers induced by nivolumab–diagnostic and therapeutic challenge: A case report. Case Rep. Oncol. 13, 314–320. doi: 10.1159/000505968
Chen, T. L., and Wang, L. S. (2020). Leptospiral presentation in Behçet’s disease: A case report. J. Formos. Med. Assoc. 119, 743–746. doi: 10.1016/j.jfma.2019.07.005
Conti, H. R., and Gaffen, S. L. (2010). Host responses to Candida albicans: Th17 cells and mucosal candidiasis. Microbes Infect 12, 518–527. doi: 10.1016/j.micinf.2010.03.013
Dabbagh, F., Haghighi, A. B., and Ghasemi, Y. (2014). Behcet’s disease: From heat shock proteins to infections. Asian Biomed. 8, 139–155. doi: 10.5372/1905-7415.0802.274
D’Agostino, S., Ferrara, E., Valentini, G., Stoica, S. A., and Dolci, M. (2022). Exploring oral microbiome in healthy infants and children: A systematic review. Int. J. Environ. Res. Public Health 19:11403. doi: 10.3390/ijerph191811403
de Mendonça, R. M., de Araújo, M., Levy, C. E., Morari, J., Silva, R. A., Yunes, J. A., et al. (2012). Prospective evaluation of HSV, Candida spp., and oral bacteria on the severity of oral mucositis in pediatric acute lymphoblastic leukemia. Support. Care Cancer 20, 1101–1107. doi: 10.1007/s00520-011-1190-0
Deo, P. N., and Deshmukh, R. (2019). Oral microbiome: Unveiling the fundamentals. J. Oral Maxillofac. Pathol. 23, 122–128. doi: 10.4103/jomfp.JOMFP_152_19
Dmytrenko, G., Tana, L., Cachau, M. V., Bravo, M., Gonzalez, S., Correa, F., et al. (2020). Presence of Trichomonas spp. in oral ulcerations of a patient with kidney transplant. A case report. J. Clin. Exp. Dent. 12, e1201–e1205. doi: 10.4317/jced.56922
Figueira, J. A., Camilo Júnior, D., Biasoli, ÉR., Miyahara, G. I., and Bernabé, D. G. (2017). Oral ulcers associated with bone destruction as the primary manifestation of histoplasmosis in an immunocompetent patient. J. Eur. Acad. Dermatol. Venereol. 31, e429–e430. doi: 10.1111/jdv.14257
Folk, G. A., and Nelson, B. L. (2017). Oral histoplasmosis. Head Neck Pathol. 11, 513–516. doi: 10.1007/s12105-017-0797-y
France, K., and Villa, A. (2020). Acute oral lesions. Dermatol. Clin. 38, 441–450. doi: 10.1016/j.det.2020.05.005
Gaffen, S. L., and Moutsopoulos, N. M. (2020). Regulation of host-microbe interactions at oral mucosal barriers by type 17 immunity. Sci. Immunol. 5, eaau4594. doi: 10.1126/sciimmunol.aau4594
Gao, L., Xu, T., Huang, G., Jiang, S., Gu, Y., and Chen, F. (2018). Oral microbiomes: More and more importance in oral cavity and whole body. Protein Cell 9, 488–500. doi: 10.1007/s13238-018-0548-1
Gasmi Benahmed, A., Noor, S., Menzel, A., and Gasmi, A. (2021). Oral aphthous: Pathophysiology, clinical aspects and medical treatment. Arch. Razi Inst. 76, 1155–1163.
GBD 2019 Tobacco Collaborators (2021). Spatial, temporal, and demographic patterns in prevalence of smoking tobacco use and attributable disease burden in 204 countries and territories, 1990-2019: A systematic analysis from the Global Burden of Disease Study 2019. Lancet 397, 2337–2360.
Groeger, S., and Meyle, J. (2019). Oral mucosal epithelial cells. Front. Immunol. 10:208. doi: 10.3389/fimmu.2019.00208
Han, N., Jia, L., Guo, L., Su, Y., Luo, Z., Du, J., et al. (2020). Balanced oral pathogenic bacteria and probiotics promoted wound healing via maintaining mesenchymal stem cell homeostasis. Stem Cell Res. Ther. 11:61. doi: 10.1186/s13287-020-1569-2
Heilbronner, S., Krismer, B., Brötz-Oesterhelt, H., and Peschel, A. (2021). The microbiome-shaping roles of bacteriocins. Nat. Rev. Microbiol. 19, 726–739. doi: 10.1038/s41579-021-00569-w
Hezel, M. P., and Weitzberg, E. (2015). The oral microbiome and nitric oxide homoeostasis. Oral Dis. 21, 7–16. doi: 10.1111/odi.12157
Hirahara, L., Takase-Minegishi, K., Kirino, Y., Iizuka-Iribe, Y., Soejima, Y., Yoshimi, R., et al. (2022). The roles of monocytes and macrophages in Behçet’s disease with focus on M1 and M2 polarization. Front. Immunol. 13:852297. doi: 10.3389/fimmu.2022.852297
Hoare, A., Marsh, P. D., and Diaz, P. I. (2017). Ecological therapeutic opportunities for oral diseases. Microbiol. Spectr. 5, 1–24. doi: 10.1128/microbiolspec.BAD-0006-2016
Hoglund, K. B., Barnett, B. K., Watson, S. A., Melgarejo, M. B., and Kang, Y. (2020). Activity of bioactive garlic compounds on the oral microbiome: A literature review. Gen. Dent. 68, 27–33.
Hong, B. Y., Sobue, T., Choquette, L., Dupuy, A. K., Thompson, A., Burleson, J. A., et al. (2019). Chemotherapy-induced oral mucositis is associated with detrimental bacterial dysbiosis. Microbiome 7:66. doi: 10.1186/s40168-019-0679-5
Hosny, K., Asfour, H., Rizg, W., Alhakamy, N. A., Sindi, A., Alkhalidi, H., et al. (2021). Formulation, optimization, and evaluation of oregano oil nanoemulsions for the treatment of infections due to oral microbiota. Int. J. Nanomed. 16, 5465–5478. doi: 10.2147/IJN.S325625
Hou, J., Zheng, H., Li, P., Liu, H., Zhou, H., and Yang, X. (2018). Distinct shifts in the oral microbiota are associated with the progression and aggravation of mucositis during radiotherapy. Radiother. Oncol. 129, 44–51. doi: 10.1016/j.radonc.2018.04.023
Huang, C., and Shi, G. (2019). Smoking and microbiome in oral, airway, gut and some systemic diseases. J. Transl. Med. 17:225. doi: 10.1186/s12967-019-1971-7
Ito, K., Okuno, T., Sawada, A., Sakai, K., Kato, Y., Muro, K., et al. (2019). Recurrent aphthous stomatitis caused by cytomegalovirus, herpes simplex virus, and Candida species in a kidney transplant recipient: A case report. Transplant. Proc. 51, 993–997. doi: 10.1016/j.transproceed.2019.01.058
Janczarek, M., Bachanek, T., Mazur, E., and Chałas, R. (2016). The role of probiotics in prevention of oral diseases. Postepy Hig. Med. Dosw. (Online) 70, 850–857. doi: 10.5604/17322693.1214381
Kalhori, K. A. M., Vahdatinia, F., Jamalpour, M. R., Vescovi, P., Fornaini, C., Merigo, E., et al. (2019). Photobiomodulation in oral medicine. Photobiomodul. Photomed. Laser Surg. 37, 837–861. doi: 10.1089/photob.2019.4706
Kantrong, N., To, T. T., and Darveau, R. P. (2019). Gingival epithelial cell recognition of lipopolysaccharide. Adv. Exp. Med. Biol. 1197, 55–67. doi: 10.1007/978-3-030-28524-1_5
Keah, N. M., Smetak, M. R., Lewis, J. S., and Topf, M. C. Jr. (2022). Secondary syphilis presenting as recurrent oral mucocutaneous lesions. Ear Nose Throat J. [Online ahead of print]. doi: 10.1177/01455613221078179
Kimura, A., and Kishimoto, T. (2010). IL-6: Regulator of Treg/Th17 balance. Eur. J. Immunol. 40, 1830–1835. doi: 10.1002/eji.201040391
Kulkarni, R., Antala, S., Wang, A., Amaral, F. E., Rampersaud, R., Larussa, S. J., et al. (2012). Cigarette smoke increases Staphylococcus aureus biofilm formation via oxidative stress. Infect. Immun. 80, 3804–3811. doi: 10.1128/IAI.00689-12
Kwiatkowski, S., Knap, B., Przystupski, D., Saczko, J., Kêdzierska, E., Knap-Czop, K., et al. (2018). Photodynamic therapy–mechanisms, photosensitizers and combinations. Biomed. Pharmacother. 106, 1098–1107. doi: 10.1016/j.biopha.2018.07.049
Laheij, A., Raber-Durlacher, J. E., Koppelmans, R. G. A., Huysmans, M., Potting, C., van Leeuwen, S. J. M., et al. (2019). Microbial changes in relation to oral mucositis in autologous hematopoietic stem cell transplantation recipients. Sci. Rep. 9:16929. doi: 10.1038/s41598-019-53073-w
Li, X., Bechara, R., Zhao, J., McGeachy, M. J., and Gaffen, S. L. (2019). IL-17 receptor-based signaling and implications for disease. Nat. Immunol. 20, 1594–1602. doi: 10.1038/s41590-019-0514-y
Liang, L., Tan, X., Zhou, Q., Zhu, Y., Tian, Y., Yu, H., et al. (2013). IL-1β triggered by peptidoglycan and lipopolysaccharide through TLR2/4 and ROS-NLRP3 inflammasome-dependent pathways is involved in ocular Behçet’s disease. Invest. Ophthalmol. Vis. Sci. 54, 402–414. doi: 10.1167/iovs.12-11047
Lin, D., Yang, L., Wen, L., Lu, H., Chen, Q., and Wang, Z. (2021). Crosstalk between the oral microbiota, mucosal immunity, and the epithelial barrier regulates oral mucosal disease pathogenesis. Mucosal Immunol. 14, 1247–1258. doi: 10.1038/s41385-021-00413-7
Maekawa, T., Krauss, J. L., Abe, T., Jotwani, R., Triantafilou, M., Triantafilou, K., et al. (2014). Porphyromonas gingivalis manipulates complement and TLR signaling to uncouple bacterial clearance from inflammation and promote dysbiosis. Cell Host Microbe 15, 768–778. doi: 10.1016/j.chom.2014.05.012
Mager, D. L., Ximenez-Fyvie, L. A., Haffajee, A. D., and Socransky, S. S. (2003). Distribution of selected bacterial species on intraoral surfaces. J. Clin. Periodontol. 30, 644–654. doi: 10.1034/j.1600-051X.2003.00376.x
Mahattanadul, S., Mustafa, M. W., Kuadkaew, S., Pattharachayakul, S., Ungphaiboon, S., and Sawanyawisuth, K. (2018). Oral ulcer healing and anti-Candida efficacy of an alcohol-free chitosan-curcumin mouthwash. Eur. Rev. Med. Pharmacol. Sci. 22, 7020–7023.
Matthews, J. B., Chen, F. M., Milward, M. R., Ling, M. R. I, and Chapple, L. (2012). Neutrophil superoxide production in the presence of cigarette smoke extract, nicotine and cotinine. J. Clin. Periodontol. 39, 626–634. doi: 10.1111/j.1600-051X.2012.01894.x
Miranda-Silva, W., Knebel, F. H., Tozetto-Mendozo, T. R., Palmieri, M., da Fonseca, F. P., Camargo, A. A., et al. (2020). Herpesviruses in the oral cavity of patients subjected to allogeneic hematopoietic stem cell transplantation and its relationship with oral mucositis. Clin. Oral Invest. 24, 3597–3608. doi: 10.1007/s00784-020-03234-3
Morris, A., Beck, J. M., Schloss, P. D., Campbell, T. B., Crothers, K., Curtis, J. L., et al. (2013). Comparison of the respiratory microbiome in healthy nonsmokers and smokers. Am. J. Respir. Crit. Care Med. 187, 1067–1075. doi: 10.1164/rccm.201210-1913OC
Motamedifar, M., Tanideh, N., Mardani, M., Daneshvar, B., and Hadadi, M. (2021). Photodynamic antimicrobial chemotherapy using indocyanine green in experimentally induced intraoral ulcers in rats. Photodermatol. Photoimmunol. Photomed. 37, 115–122. doi: 10.1111/phpp.12618
Muro, M., Soga, Y., Higuchi, T., Kataoka, K., Ekuni, D., Maeda, Y., et al. (2018). Unusual oral mucosal microbiota after hematopoietic cell transplantation with glycopeptide antibiotics: Potential association with pathophysiology of oral mucositis. Folia Microbiol. 63, 587–597. doi: 10.1007/s12223-018-0596-1
Mutepe, N. D., Cockeran, R., Steel, H. C., Theron, A. J., Mitchell, T. J., Feldman, C., et al. (2013). Effects of cigarette smoke condensate on pneumococcal biofilm formation and pneumolysin. Eur. Respir. J. 41, 392–395. doi: 10.1183/09031936.00213211
Napeñas, J. J., Brennan, M. T., Coleman, S., Kent, M. L., Noll, J., Frenette, G., et al. (2010). Molecular methodology to assess the impact of cancer chemotherapy on the oral bacterial flora: A pilot study. Oral Surg. Oral Med. Oral Pathol. Oral Radiol. Endod. 109, 554–560. doi: 10.1016/j.tripleo.2009.11.015
Nascimento, M. M. (2017). Oral microbiota transplant: A potential new therapy for oral diseases. J. Calif. Dent. Assoc. 45, 565–568.
Neelakantan, P., and Solomon, A. P. (2022). Dental implants and oral microbiome dysbiosis: An interdisciplinary perspective. Cham: Springer Nature. doi: 10.1007/978-3-030-99014-5
Novak, N., Haberstok, J., Bieber, T., and Allam, J.-P. (2008). The immune privilege of the oral mucosa. Trends Mol. Med. 14, 191–198. doi: 10.1016/j.molmed.2008.03.001
Ohbayashi, Y., Imataki, O., Uemura, M., Takeuchi, A., Aoki, S., Tanaka, M., et al. (2021). Oral microorganisms and bloodstream infection in allogeneic hematopoietic stem cell transplantation. Clin. Oral Invest. 25, 4359–4367. doi: 10.1007/s00784-020-03749-9
Osakabe, L., Utsumi, A., Saito, B., Okamatsu, Y., Kinouchi, H., Nakamaki, T., et al. (2017). Influence of oral anaerobic bacteria on hematopoietic stem cell transplantation patients: Oral mucositis and general condition. Transplant. Proc. 49, 2176–2182. doi: 10.1016/j.transproceed.2017.09.012
Özberk, S. S., Gündoðar, H., şenyurt, S. Z., and Erciyas, K. (2018). Adjunct use of low-level laser therapy on the treatment of necrotizing ulcerative gingivitis: A case report. J. Lasers Med. Sci. 9, 73–75. doi: 10.15171/jlms.2018.15
Palmer, R. J. Jr. (2014). Composition and development of oral bacterial communities. Periodontology 2000 64, 20–39. doi: 10.1111/j.1600-0757.2012.00453.x
Presland, R. B., and Jurevic, R. J. (2002). Making sense of the epithelial barrier: What molecular biology and genetics tell us about the functions of oral mucosal and epidermal tissues. J. Dent. Educ. 66, 564–574. doi: 10.1002/j.0022-0337.2002.66.4.tb03536.x
Rosier, B. T., Marsh, P. D., and Mira, A. (2018). Resilience of the oral microbiota in health: Mechanisms that prevent dysbiosis. J. Dent. Res. 97, 371–380. doi: 10.1177/0022034517742139
Sarao, L. K., and Arora, M. (2017). Probiotics, prebiotics, and microencapsulation: A review. Crit. Rev. Food Sci. Nutr. 57, 344–371. doi: 10.1080/10408398.2014.887055
Sawair, F. A. (2010). Does smoking really protect from recurrent aphthous stomatitis? Ther. Clin. Risk Manag. 6, 573–577. doi: 10.2147/TCRM.S15145
Shi, Y., Wei, K., Lu, J., Wei, J., Hu, X., and Chen, T. (2020). A clinic trial evaluating the effects of aloe vera fermentation gel on recurrent aphthous stomatitis. Can. J. Infect. Dis. Med. Microbiol. 2020:8867548. doi: 10.1155/2020/8867548
Siddiqui, A. Z., Vellappally, S., Fouad, H., and Muckarrum, S. M. (2020). Bactericidal and clinical efficacy of photochemotherapy in acute necrotizing ulcerative gingivitis. Photodiagn. Photodyn. Ther. 29:101668. doi: 10.1016/j.pdpdt.2020.101668
Singh, V., and Singh, A. K. (2020). Oral mucositis. Natl. J. Maxillofac. Surg. 11, 159–168. doi: 10.4103/njms.NJMS_10_20
Slezakova, S., Borilova Linhartova, P., Bartova, J., Petanova, J., Kuklinek, P., Fassmann, A., et al. (2020). Gene variability in matrix metalloproteinases in patients with recurrent aphthous stomatitis. J. Oral Pathol. Med. 49, 271–277. doi: 10.1111/jop.12993
Sorbara, M. T., and Pamer, E. G. (2022). Microbiome-based therapeutics. Nat. Rev. Microbiol. 20, 365–380. doi: 10.1038/s41579-021-00667-9
Stehlikova, Z., Tlaskal, V., Galanova, N., Roubalova, R., Kreisinger, J., Dvorak, J., et al. (2019). Oral microbiota composition and antimicrobial antibody response in patients with recurrent aphthous stomatitis. Microorganisms 7:636. doi: 10.3390/microorganisms7120636
Stringer, A. M., and Logan, R. M. (2015). The role of oral flora in the development of chemotherapy-induced oral mucositis. J. Oral pathol. Med. 44, 81–87. doi: 10.1111/jop.12152
Suárez, L. J., Arboleda, S., Angelov, N., and Arce, R. M. (2021). Oral versus gastrointestinal mucosal immune niches in homeostasis and allostasis. Front. Immunol. 12:705206. doi: 10.3389/fimmu.2021.705206
Suárez, L. J., Garzón, H., Arboleda, S., and Rodríguez, A. (2020). Oral dysbiosis and autoimmunity: From local periodontal responses to an imbalanced systemic immunity. A review. Front. Immunol. 11:591255. doi: 10.3389/fimmu.2020.591255
Subramaniam, N., and Muthukrishnan, A. (2019). Oral mucositis and microbial colonization in oral cancer patients undergoing radiotherapy and chemotherapy: A prospective analysis in a tertiary care dental hospital. J. Invest. Clin. Dent. 10:e12454. doi: 10.1111/jicd.12454
Sun, A., Chia, J. S., and Chiang, C. P. (2002). Increased proliferative response of peripheral blood mononuclear cells and T cells to Streptococcus mutans and glucosyltransferase D antigens in the exacerbation stage of recurrent aphthous ulcerations. J. Formos. Med. Assoc. 101, 560–566.
Triarico, S., Agresti, P., Rinninella, E., Mele, M. C., Romano, A., Attinà, G., et al. (2022). Oral microbiota during childhood and its role in chemotherapy-induced oral mucositis in children with cancer. Pathogens 11:448. doi: 10.3390/pathogens11040448
Tuganbaev, T., Yoshida, K., and Honda, K. (2022). The effects of oral microbiota on health. Science 376, 934–936. doi: 10.1126/science.abn1890
Vallès, Y., Inman, C. K., Peters, B. A., Ali, R., Wareth, L. A., Abdulle, A., et al. (2018). Types of tobacco consumption and the oral microbiome in the United Arab Emirates Healthy Future (UAEHFS) Pilot Study. Sci. Rep. 8:11327. doi: 10.1038/s41598-018-29730-x
Vasconcelos, R. M., Sanfilippo, N., Paster, B. J., Kerr, A. R., Li, Y., Ramalho, L., et al. (2016). Host-microbiome cross-talk in oral mucositis. J. Dent. Res. 95, 725–733. doi: 10.1177/0022034516641890
Vila, T., Sultan, A. S., Montelongo-Jauregui, D., and Jabra-Rizk, M. A. (2020). Oral candidiasis: A disease of opportunity. J. Fungi 6:15. doi: 10.3390/jof6010015
Villa, A., and Sonis, S. T. (2016). Pharmacotherapy for the management of cancer regimen-related oral mucositis. Expert. Opin. Pharmacother. 17, 1801–1807. doi: 10.1080/14656566.2016.1217993
Wang, S.-S., Tang, Y.-L., Pang, X., Zheng, M., Tang, Y.-J., and Liang, X.-H. (2019). The maintenance of an oral epithelial barrier. Life Sci. 227, 129–136. doi: 10.1016/j.lfs.2019.04.029
Wang, Y., Li, J., Zhang, H., Zheng, X., Wang, J., Jia, X., et al. (2021). Probiotic Streptococcus salivarius K12 alleviates radiation-induced oral mucositis in mice. Front. immunol. 12:684824. doi: 10.3389/fimmu.2021.684824
Wu, X., Zhang, G., Wang, X., Zhang, P., and Tan, Y. (2015). Endotoxin tolerance induction in human periodontal ligament fibroblasts stimulated with different bacterial lipopolysaccharides. Arch. Oral Biol. 60, 463–470. doi: 10.1016/j.archoralbio.2014.10.005
Xiao, H., Fan, Y., Li, Y., Dong, J., Zhang, S., Wang, B., et al. (2021). Oral microbiota transplantation fights against head and neck radiotherapy-induced oral mucositis in mice. Comput. Struct. Biotechnol. J. 19, 5898–5910. doi: 10.1016/j.csbj.2021.10.028
Xu, H., Tian, B., Shi, W., Tian, J., Wang, W., and Qin, M. (2022). Maturation of the oral microbiota during primary teeth eruption: A longitudinal, preliminary study. J. Oral. Microbiol. 14:2051352. doi: 10.1080/20002297.2022.2051352
Zanotta, N., Ottaviani, G., Campisciano, G., Poropat, A., Bovenzi, M., Rupel, K., et al. (2020). Photobiomodulation modulates inflammation and oral microbiome: A pilot study. Biomarkers 25, 677–684. doi: 10.1080/1354750X.2020.1825812
Zhou, M., Wang, H., Zeng, X., Yin, P., Zhu, J., Chen, W., et al. (2019). Mortality, morbidity, and risk factors in China and its provinces, 1990-2017: A systematic analysis for the Global Burden of Disease Study 2017. Lancet 394, 1145–1158. doi: 10.1016/S0140-6736(19)30427-1
Zhou, X., and Li, Y. (2015). Atlas of oral microbiology: From healthy microflora to disease. Singapore: Springer.
Keywords: oral microbiota, dysbiosis, oral mucositis, recurrent aphthous stomatitis (RAS), probiotics
Citation: Min Z, Yang L, Hu Y and Huang R (2023) Oral microbiota dysbiosis accelerates the development and onset of mucositis and oral ulcers. Front. Microbiol. 14:1061032. doi: 10.3389/fmicb.2023.1061032
Received: 04 October 2022; Accepted: 23 January 2023;
Published: 09 February 2023.
Edited by:
Heng Liu, The Wistar Institute, United StatesCopyright © 2023 Min, Yang, Hu and Huang. This is an open-access article distributed under the terms of the Creative Commons Attribution License (CC BY). The use, distribution or reproduction in other forums is permitted, provided the original author(s) and the copyright owner(s) are credited and that the original publication in this journal is cited, in accordance with accepted academic practice. No use, distribution or reproduction is permitted which does not comply with these terms.
*Correspondence: Ruijie Huang, cnVpam1odWFuZ0BnbWFpbC5jb20=
†These authors have contributed equally to this work
‡ORCID: Ziyang Min, orcid.org/0000-0002-1414-4799; Lei Yang, orcid.org/0000-0002-6641-0016; Ruijie Huang, orcid.org/0000-0003-3211-518X
Disclaimer: All claims expressed in this article are solely those of the authors and do not necessarily represent those of their affiliated organizations, or those of the publisher, the editors and the reviewers. Any product that may be evaluated in this article or claim that may be made by its manufacturer is not guaranteed or endorsed by the publisher.
Research integrity at Frontiers
Learn more about the work of our research integrity team to safeguard the quality of each article we publish.