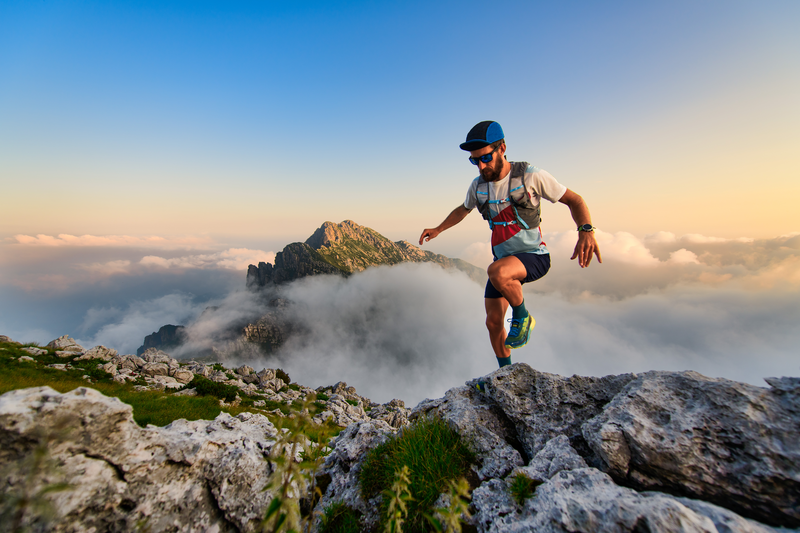
94% of researchers rate our articles as excellent or good
Learn more about the work of our research integrity team to safeguard the quality of each article we publish.
Find out more
ORIGINAL RESEARCH article
Front. Microbiol. , 10 February 2023
Sec. Antimicrobials, Resistance and Chemotherapy
Volume 14 - 2023 | https://doi.org/10.3389/fmicb.2023.1043838
This article is part of the Research Topic Pathoblockers and Antivirulence Agents of Plant-Origin for the Management of Multidrug Resistant Pathogens View all 5 articles
Aeromonas hydrophila is a significant pathogen to freshwater farmed animals, and antibiotics are usually used to control the bacterial septicemia caused by A. hydrophila. Due to the severe situation of development and spread of antibiotic resistance, there are stricter restrictions on antibiotics used in aquaculture. To evaluate the feasibility of glycyrrhetinic acid β (GA) as an alternative therapy against bacterial infection, in this study, an A. hydrophila isolated from diseased fish is used to test the antibacterial, anti-virulence activity and therapeutic effect of GA in vitro and in vivo, respectively. Results showed that GA did not affect the growth of A. hydrophila in vitro, while it could down-regulate (p < 0.05) the mRNA expression of the hemolysis-related genes hly and aerA, and significantly inhibited (p < 0.05) hemolytic activity of A. hydrophila. In addition, in vivo test showed that oral administration of GA was ineffective in controlling acute infections caused by A. hydrophila. In conclusion, these findings suggested that GA was a potential anti-virulence candidate against A. hydrophila, but the application of GA for the prevention and treatment of A. hydrophila-related diseases was still a long way.
Aeromonas hydrophila is a widespread opportunistic pathogen in freshwater environment. It is regarded as a significant risk factor in aquaculture because of its high morbidity and mortality (Harikrishnan and Balasundaram, 2007). Antibiotic therapy is still the main measure against A. hydrophila infection. However, due to the severe situation in the development and spread of antibiotic resistance, as well as drug residues and environmental pollution (Lulijwa et al., 2019), the alternatives to antibiotics are urgently needed. Anti-virulence strategies are considered as a promising alternative solution (Defoirdt, 2014), which exert less selective pressure on bacteria than antibiotic treatment, and therefore have apparent advantages in reducing the development of resistance.
Glycyrrhetinic acid β (GA), an oleanane-type triterpene, is the main active component of plant Glycyrrhiza glabra extract (El-Saber Batiha et al., 2020) and frequently employed in the therapy for human diseases. There are numerous publications on the antimicrobial effects of GA and its derivatives, including antibacterial activity in Staphylococcus aureus (Oyama et al., 2016), Pseudomonas aeruginosa (Darvishi et al., 2015) and Helicobacter pylori (Celik and Duran, 2019). GA is also effective at regulating pathogen virulence, as it can reduce the virulence of S. aureus (Li et al., 2012; Long et al., 2013; Huang et al., 2016) and Streptococcus mutans (Yamashita et al., 2019), as well as limit the production and release of α-hemolysin by S. aureus (Li et al., 2012). Furthermore, GA can effectively reduce pathological damage caused by the pathogen infection (Kim et al., 2013; Long et al., 2013).
Until now, there is limited data about GA in aquaculture, and even the available focus on its impact on farmed animals’ growth, nutrition, and metabolism, as well as non-specific immunity and antioxidation. For instance, Ietalurus punetaus (Jiang et al., 2016), Megalobrama amblycephala (Cai et al., 2014), Hypophthalmichthys molitrix (Harikrishnan et al., 2021), and Litopenaeus Vannamei (Chen et al., 2010). Only Schrader (2010) has reported that GA had antibacterial activity against Flavobacterium columnaria. To evaluate its potential as a therapeutic agent for prevention and treatment of bacterial diseases, in this study, the antibacterial and anti-virulence activity against pathogenic bacterial A. hydrophila in vitro and therapeutic effect in vitro of GA were tested.
Aeromonas hydrophila strain employed in this study was isolated from diseased Carassius auratus. Gibel carp (C. auratus gibelio, 5.3 ± 1.1 g), provided by the experimental station of Freshwater Fisheries Research Center, Chinese Academy of Fishery Sciences, were acclimatized in indoor water recirculation culture system at 28 ± 1°C for 2 weeks. A 2% commercial feed was given twice per day according to body weight. This study was carried out in strict accordance with the recommendations in the Guide for the Care and Use of Laboratory Animals. The protocol was approved by the Committee on the Ethics of Animal Experiments of the Freshwater Fisheries Research Center (Authorization Number: 20220214001). All surgery was performed under MS-222, and all efforts were made to minimize suffering.
Minimal inhibitory concentration (MIC) was determined as previous study (Zhu et al., 2019). GA (97%, Aladdin, China) was dissolved with 100% DMSO to obtain a stock solution of 25.6 mg/mL, then diluted with Nutrient Broth (NB) medium and the final concentrations of GA in culture medium were 256, 128, 64, 32, 16, 8 μg/mL, respectively. A. hyrophila at logarithmic growth period was adjusted to 1 × 107 CFU/ml, 50 μL cell suspension was inoculated into 5 mL NB medium with different concentration of GA, negative, solvent and positive controls containing only NB, NB with DMSO and Enrofloxacin (0.1 μg/mL) including bacteria, and then incubated at 28°C for 24 h with shaking (180 rpm). The test was repeated twice, and each group was detected with three parallels. The optical density (OD) of culture at 600 nm was measured using a Multiskan GO spectrophotometer. The MIC was defined as the lowest concentration of the drug that inhibited growth of target bacteria by >90%.
Aeromonas hydrophila was inocubated into NB medium with the different final concentration of GA (128 μg/ml, 64 μg/ml, 32 μg/ml, 16 μg/ml, 8 μg/ml, and 4 μg/ml), Enrofloxacin (0.1 μg/ml) and incubated at 28°C. The growth curve was detected with the OD600 at 0, 2, 4, 6, 8, 10, 12, 22, 24, and 26 h. The experiment was replicated in triplicate.
Bacterial cells (1 × 107 CFU/ml, 50 μl) were inoculated into NB medium (5 ml), and cultured with and without GA at 28°C for 24 h with shaking (180 rpm). OD600 was determined with a spectrophotometer. The supernatant was collected by centrifugation (10,000 g, 10 min, 4°C), and filtration with a nylon membrane (0.22 μm). The filtrate was stored at −80°C for protease and hemolytic activity assay. The experiment was repeated twice with six parallels in each group.
Azocasein assay was used to detect protease activity as previously reported (Li et al., 2019). Briefly, the filtrate (150 μl) and 0.3% azocasein (1 ml, dissolved in Tris–HCl, pH 7.5) were incubated at 37°C for 30 min, and the reaction was terminated with 500 μl 10% trichloroacetic acid. The supernatant was collected after centrifugation (10,000 g, 10 min, 4°C) and neutralized with NaOH (1 mol/l). Finally, OD400 of the supernatant was measured, and the relative activity of protease was calculated as OD400/OD600.
The hemolytic activity was determined as described previously (Li et al., 2019). Briefly, 100 μl filtrate, 100 μl 4% sheep blood cells and 800 μl sterile saline was incubated at 37°C for 30 min. The supernatant was collected by centrifugation (2000 g, 10 min, 4°C) and the OD405 was detected. Finally, the hemolysis rate was determined using the formula below: [(sample OD405 -blank control OD405) / sample OD 600] / (positive control OD405 -blank control OD405). By the same way, NB medium with Magnolol (98%, Aladdin, China) at 8 μg/ml was prepared as the positive drug in supplementary tests in hemolytic activity assay (Dong et al., 2017).
The lipase activity was measured using a modified agar-diffusion assay (Zhu et al., 2019). Bacterial cells in logarithmic growth period were adjusted to 1 × 105 CFU/ml and added 50 μl suspension to each well (diameter = 7 mm) in medium (NB base, 1.5% agar, 1% Tween 80) with and without GA and incubated at 28°C. The diameter of the precipitation region was measured at 24 h, 48 h, 72 h, and 96 h, respectively, to indicate the lipase activity. The experiment was done in triplicate, with three parallels in each group.
Motility assay was carried out as in the previous report (Zhu et al., 2019). Bacterial cells in logarithmic growth period were adjusted to 1 × 105 CFU/ml, planted onto the semi-soft medium (NB base, 0.5% agar) with and without GA, and then incubated at 28°C. To determine motility, the colony diameter was measured at 24, 48, 72, and 96 h. The experiment was repeated twice with six parallels in each group.
For virulence related gene expression analysis, A. hydrophila was inoculated in NB medium with and without GA (32 μg/ml), and cultured for 18 h at 28°C with shaking (180 rpm). Each group was conducted with three parallels. Bacterial cells were collected by centrifugation (10,000 g, 10 min, 4°C), washed and diluted with sterilized saline (0.85%), and the equal cell pellets were immediately immersed in RNAiso Plus (TaKaRa) and stored at −80°C.
Total RNA was extracted using a RNAiso Plus kit (Takara). RNA quantities and concentrations were measured using Nanodrop 2000 spectrophotometer (Thermo Scientific), then RNA concentrations were adjusted to 400 ng/μL. cDNA was synthesized using a HiScript® III RT SuperMiX for qPCR kit (Vazyme Biotech, China) following the instructions.
Quantitative real-time PCR was performed on a Bio-Rad CFX real-time PCR detection system (USA) using ChamQ Universal SYBR qPCR Master Mix kit (Vazyme, China). All primers of virulence related genes (Table 1) were synthesized by Sangon Biotech (China), and recA was chosen as the reference housekeeping gene (Pang, 2015; Gu et al., 2022). The experiments were conducted in triplicate, and the result was calculated using 2-△△Ct method.
Gibel carps (180) were randomly distributed into 1 control group and 3 experimental groups (infected only group, infected and treated with GA group, infected and treated with enrofloxacin group). Three parallels were set for each group.
Each fish in experimental groups were injected intraperitoneally with 1% (v/w) A. hydrophila suspension (1 × 107 CFU/ml) after anesthetizing with MS-222, while the control group received an injection of sterilized saline. When all fish in tank returned, basic or medicated feed were given to different groups. The control and infected only groups. Were fed with basic feed, while the infected and treated with GA group and the infected and treated with antibiotic group were fed with basic feed supplemented with GA (0.2%, w/w) and enrofloxacin (0.2%, w/w), respectively. Theoretical medication intake was 40 mg/kg of fish (Yang, 2005). The mortality was monitored for 7 days.
Statistical analyzes of data were performed in SPSS 23 software. T-test or T’-test was used for data in a normal distribution, while nonparametric test was employed for data not in a normal distribution. The survival curve was analyzed using the Kaplan–Meier method, and the survival rate was analyzed using one-way ANOVA. All data was present as mean ± standard deviation (x ± s), and the significance level was set at 0.05.
Solvent DMSO (1%) showed no noticeable bacteriostatic effect on A. hydrophila, (Figure 1A), GA treated groups with different concentrations all did not show significant inhibition to A. hydrophila (p > 0.05). Due to the saturated concentration of GA in NB is near 256 μg/ml, therefore the exact MIC of GA to A. hydrophila could not be detected here, and, the MIC should be above 128 μg/ml.
Figure 1. The effect of GA on the growth of Aeromonas hydrophila. (A) The bioassay results of GA’s MIC against A. hydrophila (n = 6). (B) Aeromonas hydrophila growth curves cultured with different doses of GA (n = 3). n.s., not significant.
The grow curves of A. hydrophila in each GA-treated groups were essentially coincident with that in the control group (Figure 1B). The result demonstrated that GA did not affect the growth of A. hydrophila.
The azocasein assay revealed that the DMSO significantly decreased the extracellular protease activity compared to the blank control group (p < 0.05), however, there was no significant difference between GA treated groups and DMSO group, except one group with GA concentration 64 μg/ml (p < 0.05) (Figure 2A). In hemolytic activity, lipase activity and motility tests, DMSO group showed no significant difference compared to the control group (Figures 2B–D) (p > 0.05). Furthermore, GA concentration over 4 μg/ml, dramatically reduced hemolytic activity of A. hydrophila (Figure 2B). It is generally consistent with the results in the supplementary experiment (Supplementary Figure S1). In aspects of motility, only the highest GA-treated group (128 μg/ml) decreased significantly at 24, 48, 72, and 96 h. According to the result in Figure 2D, there was no significantly difference between all the tested groups at all time points, and GA did not influence lipase activity (p > 0.05).
Figure 2. Effect of GA on virulence phenotype of A. hydrophila. (A) Protease activities of the A. hydrophila supernatants co-cultured with different concentrations of GA (n = 6). (B) Hemolytic activities of the A. hydrophila supernatants co-cultured with different concentrations of GA (n = 12). (C) Motility of the A. hydrophila co-cultured with different concentrations of GA (n = 12). (D) Lipase activities of the A. hydrophila co-cultured with different concentrations of GA(n = 9). n.s., not significant. #p < 0.05.
To further confirm the impact of GA on hemolytic activity of A. hydrophila, mRNA expressions of hemolysis-related genes (hly and aerA) were detected. The results showed that the DMSO did not affect the mRNA expressions of hly and aer A genes, whereas 32 μg/ml GAvsignificantly inhibited the mRNA expression of hly and aerA genes (Figure 3) and reduced the hly and aerA mRNA expressions to 51.8 and 52.8% of that in DMSO group, respectively.
Figure 3. Effects of GA on the mRNA expression of hemolysis-related genes in A. hydrophila. (A) The relative expression levels of hly of A. hydrophila GA (n = 3). (B) The relative expression levels of areA of A. hydrophila (n = 3). n.s., not significant. #p < 0.05.
GA significantly reduced the expression of hemolysis-related genes and suppressed the hemolytic activity of A. hydrophila in vitro. Therefore, we further tested therapeutic effect of GA in goldfish against A. hydrophila infection. The results showed that A. hydrophila infection induced acute histopathological damage in goldfish. Moribund goldfish showed redness and swelling around the injection site and cloaca, as well as blooding at the base of fin and at eye (Figure 4A). In addition, autopsy revealed ascites in intestine and body cavity, and enteritis. The survival of goldfish infected with A. hydrophila showed no significant difference between the enrofloxacin treated group and that of in control group (p > 0.05) (Figure 4B). However, GA treated groups did not markedly increase the survival of goldfish infected with A. hydrophila (p > 0.05).
Figure 4. Therapeutic effect of GA in goldfish against acute infection of A. hydrophila. (A) gross pathological changes (A1, diseased goldfish. A2, symptoms on the body surface. A3, symptoms of internal organs. A4, symptoms of intestinal). (B) survival curve (n = 15). CG, control group. IG, infected only group. GG, infected and treated with GA group. EG, infected and treated with enrofloxacin group. Different lower-case letters indicated significant differences among groups (p < 0.05).
In this study, the MIC and growth curve tests revealed that GA had no antibacterial effect on A. hydrophila. In Escherichia coli (Kim et al., 2002) and S. aureus (Oyama et al., 2016), the similar results also have been reported. The effect of GA on the growth of Bacillus subtilis was not consistent in different strains (Kim et al., 2002; Wang et al., 2012; Huang et al., 2016). Even it reported that some bacterial strain can use GA as carbon source (Yoshida et al., 2001). GA did not inhibit the growth of A. hydrophila isolated from diseased fish, so there was less selection pressure. Therefore, GA employed in aquaculture to control A. hydrophila infection will has an advantage in avoiding the development of drug resistance.
Like many other bacteria, the pathogenicity of A. hydrophila is mainly determined by its virulence. Pathogenic A. hydrophila usually possess a variety of virulence factors that can mediate the adhesion and invasion of the host tissues, evade host defense mechanisms and contribute to A. hydrophila survival and developing an infection. Furthermore, all these virulence factors are frequently associated with their phenotypes, such as hemolytic activity, extracellular enzyme activity and motility. Among these phenotypes, hemolytic activity of A. hydrophila is mostly mediated by the expression of hly and areA. They are the chief exotoxins of A. hydrophila, can interfere with host cell physiological activity and injure host tissues and cells directly or indirectly (Sarkar et al., 2021). Extracellular enzymes can degrade host mucus barrier and cause tissues damage, facilitate bacterial invasion into the host tissues (Chen et al., 2019). And the motility of A. hydrophila is depended on the structural components, flagella and fimbriae. They play an important role in the first contact between host and pathogen and involve in bacterial colonization and invasion (Qin et al., 2016). The current researches indicate that, inhibiting the expression of protease (Cascón et al., 2000), aerolysin (Li et al., 2011) hemolysis-related genes (Dong et al., 2017), interfering with flagella synthesis (Jiang et al., 2015), or blocking the effect of extracellular enzymes (Wu et al., 2012) and hemolytic exotoxins (Zhang et al., 2015), can effectively reduce the mortality from A. hydrophila.
For protection against the development and spread of antibiotic resistance, antibiotics use is becoming more restricted. Therefore, development of virulent-targeted medicines attracts much attention in the prevention and treatment of infection. In this investigation, results showed that GA had little effect on A. hydrophila lipase, protease, or motility, but did drastically inhibit A. hydrophila hemolysis ability, which was similar to these results reported in S. aureus (Li et al., 2012; Long et al., 2013; Huang et al., 2016). Furthermore, our results also indicated that GA inhibited aerA, and hly genes expression, which was consistent with these results in S. aureus (Li et al., 2012; Long et al., 2013; Huang et al., 2016). These results suggest that there is a similar mechanism, hemolytic activity is in inhibited via down-regulating the expressions of hemolysis-related genes, in these bacteria.
Now, anti-virulence strategies have become an important approach in novel drugs against antibiotic resistant bacterial infections. Then hemolysin and aerolysin as the chief exotoxins are important to the pathogenicity of A. hydrophila. Previous researches shown they are promising target for minimizing the virulence of the bacterium. There were some investigated focused on these targets, and have found some medications that had a substantial inhibitory impact on aerolysin and could effectively reduce the mortality of experimental fish from A. hydrophila, such as resveratrol (Dong et al., 2021), magnolol (Jiang et al., 2015). We also tested the effect of GA in vivo, however, the results were unsatisfactory. Further analysis demonstrated that is probably associated with the pharmacokinetics of different drugs. When compared to Dong (Jiang et al., 2015; Dong et al., 2021) and other researches, GA have little effect on the growth of A. hydrophila, the oral administration absorption process may further cause the optimal treatment window missed. Although this experiment was unable to prove GA’s therapeutic effect, we did learn from this failed animal experiment that GA’s application risk in the treatment of acute A. hydrophila infection. In the future, we will tweak the program even further and try to assess GA’s therapeutic properties.
The original contributions presented in the study are included in the article/Supplementary material, further inquiries can be directed to the corresponding authors.
The animal study was reviewed and approved by the Committee on the Ethics of Animal Experiments of the Freshwater Fisheries Research Center.
BX, JX, and XB contributed to conception and design of the study. TQ and LP participated in sample preparation and processing. KC performed the statistical analysis. KC wrote the first draft of the manuscript. BX, JX, TQ, and LP wrote sections of the manuscript. All authors contributed to manuscript revision, read, and approved the submitted version.
China Agriculture Research System of MOF and MARA (CARS-45) National Key R&D Program of China (2020YFD0900300).
The authors declare that the research was conducted in the absence of any commercial or financial relationships that could be construed as a potential conflict of interest.
All claims expressed in this article are solely those of the authors and do not necessarily represent those of their affiliated organizations, or those of the publisher, the editors and the reviewers. Any product that may be evaluated in this article, or claim that may be made by its manufacturer, is not guaranteed or endorsed by the publisher.
The Supplementary material for this article can be found online at: https://www.frontiersin.org/articles/10.3389/fmicb.2023.1043838/full#supplementary-material
SUPPLEMENTARY FIGURE S1 | Hemolytic activities of the A. hydrophila supernatants with different treatments (n = 6). n.s., not significant. #, p < 0.05.
Cai, D., Jiang, G., Wang, L., Lu, K., Qian, Y., Liu, W., et al. (2014). Effects of dietery glycyrrhetinc acid levels on growth performance, lipid deposition and antioxidant capacity of blunt snout bream (Megalobrama amblycephala). J. Fish. China 38, 1514–1521. doi: 10.3724/SP.J.1231.2014.49244
Cascón, A., Yugueros, J., Temprano, A., Sánchez, M., Hernanz, C., Luengo, J. M., et al. (2000). A major secreted elastase is essential for pathogenicity of Aeromonas hydrophila. American Society for Microbiology. Infect. Immun. 68, 3233–3241. doi: 10.1128/IAI.68.6.3233-3241.2000
Celik, M. M., and Duran, N. (2019). An experimental in-vitro study to evaluate the anti-helicobacter activity of Glycyrrhetinic acid. Rev. Rom. Med. Lab. 27, 63–71. doi: 10.2478/rrlm-2019-0003
Chen, D. D., Li, J. H., Yao, Y. Y., and Zhang, Y. A. (2019). Aeromonas hydrophila suppresses complement pathways via degradation of complement C3 in bony fish by metalloprotease. Fish Shellfish Immunol. 94, 739–745. doi: 10.1016/j.fsi.2019.09.057
Chen, X., Mai, K., Zhang, W., Wang, X., Ai, Q., Xu, W., et al. (2010). Effects of dietary glycyrrhizin on growth and nonspecific immunity of white shrimp, Litopenaeus vannamei. J. World Aquacult. Soc. 41, 665–674. doi: 10.1111/j.1749-7345.2010.00409.x
Darvishi, B., Manoochehri, S., Kamalinia, G., Samadi, N., Amini, M., Mostafavi, S. H., et al. (2015). Preparation and antibacterial activity evaluation of 18-β-glycyrrhetinic acid loaded PLGA nanoparticles. Iran. J. Pharm. Res. 14, 373–383. PMC4403053
Defoirdt, T. (2014). Virulence mechanisms of bacterial aquaculture pathogens and antivirulence therapy for aquaculture. Rev. Aquac. 6, 100–114. doi: 10.1111/raq.12030
Dong, J., Ding, H., Liu, Y., Yang, Q., Xu, N., Yang, Y., et al. (2017). Magnolol protects channel catfish from Aeromonas hydrophila infection via inhibiting the expression of aerolysin. Vet. Microbiol. 211, 119–123. doi: 10.1016/j.vetmic.2017.10.005
Dong, J., Zhang, L., Liu, Y., Zhou, S., Yang, Y., Xu, N., et al. (2021). Resveratrol influences the pathogenesis of Aeromonas hydrophila by inhibiting production of aerolysin and biofilm. Food Control 126:108083. doi: 10.1016/j.foodcont.2021.108083
El-Saber Batiha, G., Magdy Beshbishy, A., El-Mleeh, A., Abdel-Daim, M., and Prasad Devkota, H. (2020). Traditional uses, bioactive chemical constituents, and pharmacological and toxicological activities of Glycyrrhiza glabra L. (Fabaceae). Biomolecules 10:352. doi: 10.3390/biom10030352
Gao, J., Xi, B., Chen, K., Song, R., Qin, T., Xie, J., et al. (2019). The stress hormone norepinephrine increases the growth and virulence of Aeromonas hydrophila. Microbiology 8:e00664. doi: 10.1002/mbo3.664
Gu, Y., Chen, K., Xi, B., Xie, J., and Bing, X. (2022). Paeonol increases the antioxidant and anti-inflammatory capacity of gibel carp (Carassius auratus gibelio) challenged with Aeromonas hydrophila. Fish Shellfish Immunol. 123, 479–488. doi: 10.1016/j.fsi.2022.03.005
Harikrishnan, R., and Balasundaram, C. (2007). Modern trends in Aeromonas hydrophila disease management with fish. Rev. Fish. Sci. 13, 281–320. doi: 10.1080/10641260500320845
Harikrishnan, R., Devi, G., van Doan, H., Jawahar, S., Balasundaram, C., Saravanan, K., et al. (2021). Study on antioxidant potential, immunological response, and inflammatory cytokines induction of glycyrrhizic acid (GA) in silver carp against vibriosis. Fish Shellfish Immunol. 119, 193–208. doi: 10.1016/j.fsi.2021.09.040
Huang, L. R., Hao, X. J., Li, Q. J., Wang, D. P., Zhang, J. X., Luo, H., et al. (2016). 18beta-Glycyrrhetinic acid derivatives possessing a trihydroxylated a ring are potent gram-positive antibacterial agents. J. Nat. Prod. 79, 721–731. doi: 10.1021/acs.jnatprod.5b00641
Jiang, X., Qin, Y. X., Lin, G. F., Huang, L., Huang, B., Huang, W. S., et al. (2015). FlgN plays important roles in the adhesion of Aeromonas hydrophila to host mucus. Genet. Mol. Res. 14, 6376–6386. doi: 10.4238/2015.June.11.13
Jiang, G., Zhou, M., Li, X., Zhang, D., and Liu, W. (2016). Effect of glycyrrhetinic acid (GA) on the physiological responses and immunoreactions of channel catfish (Ictalurus punctatus) injection lipopolysaccharide (LPS). J. Fish. China 40, 1368–1375. doi: 10.11964/jfc.20160410347
Kim, S. R., Jeon, H. J., Park, H. J., Kim, M. K., Choi, W. S., Jang, H. O., et al. (2013). Glycyrrhetinic acid inhibits Porphyromonas gingivalis lipopolysaccharide-induced vascular permeability via the suppression of interleukin-8. Inflamm. Res. 62, 145–154. doi: 10.1007/s00011-012-0560-5
Kim, H. K., Park, Y., Kim, H. N., Choi, B. H., Jeon, H. G., Lee, D. G., et al. (2002). Antimicrobial mechanism of β-glycyrrhetinic acid isolated from licorice, Glycyrrhiza glabra. Biotechnol. Letters. 24, 1899–1902. doi: 10.1023/A:1020900124997
Li, J., Ni, X. D., Liu, Y. J., and Lu, C. P. (2011). Detection of three virulence genes alt, ahp and aerA in Aeromonas hydrophila and their relationship with actual virulence to zebrafish. J. Appl. Microbiol. 110, 823–830. doi: 10.1111/j.1365-2672.2011.04944.x
Li, H. E., Qiu, J. Z., Yang, Z. Q., Dong, J., Wang, J. F., Luo, M. J., et al. (2012). Glycyrrhetinic acid protects mice from Staphylococcus aureus pneumonia. Fitoterapia 83, 241–248. doi: 10.1016/j.fitote.2011.10.018
Li, M.-Y., Xi, B.-W., Qin, T., Chen, K., Ren, M.-C., and Xie, J. (2019). Indigenous AHL-degrading bacterium Bacillus firmus sw40 affects virulence of pathogenic Aeromonas hydrophila and disease resistance of gibel carp. Aquac. Res. 50, 3755–3762. doi: 10.1111/are.14338
Long, D. R., Mead, J., Hendricks, J. M., Hardy, M. E., and Voyich, J. M. (2013). 18beta-Glycyrrhetinic acid inhibits methicillin-resistant Staphylococcus aureus survival and attenuates virulence gene expression. Antimicrob. Agents Chemother. 57, 241–247. doi: 10.1128/AAC.01023-12
Lulijwa, R., Rupia, E. J., and Alfaro, A. C. (2019). Antibiotic use in aquaculture, policies and regulation, health and environmental risks: a review of the top 15 major producers. Rev. Aquac. 12, 640–663. doi: 10.1111/raq.12344
Oyama, K., Kawada-Matsuo, M., Oogai, Y., Hayashi, T., Nakamura, N., and Komatsuzawa, H. (2016). Antibacterial effects of glycyrrhetinic acid and its derivatives on Staphylococcus aureus. PLoS One 11:e0165831. doi: 10.1371/journal.pone.0165831
Pang, M. (2015). Genomic Characteristics and Virulence-associated Genes Analysis of Aeromonas hysrophila. Nanjing: Nanjing Agricultural University.
Qin, Y., Lin, G., Chen, W., Xu, X., and Yan, Q. (2016). Flagellar motility is necessary for Aeromonas hydrophila adhesion. Microb. Pathog. 98, 160–166. doi: 10.1016/j.micpath.2016.07.006
Sarkar, P., Issac, P. K., Raju, S. V., Elumalai, P., Arshad, A., and Arockiaraj, J. (2021). Pathogenic bacterial toxins and virulence influences in cultivable fish. Aquac. Res. 52, 2361–2376. doi: 10.1111/are.15089
Schrader, K. K. (2010). Plant natural compounds with antibacterial activity towards common pathogens of pond-cultured channel catfish (Ictalurus punctatus). Toxins 2, 1676–1689. doi: 10.3390/toxins2071676
Wang, J., Hu, X., Wen, W., Yang, L., and Zhu, Y. (2012). Synthesis and activity of 3-amino acid derivatives of glycyrrhetinic acid. Chin. J. Appl. Chem. 29, 873–877. doi: 10.3724/SP.J.1095.2012.00371
Wu, L., Jiang, Y. N., Tang, Q., Lin, H. X., Lu, C. P., and Yao, H. C. (2012). Development of an Aeromonas hydrophila recombinant extracellular protease vaccine. Microb. Pathog. 53, 183–188. doi: 10.1016/j.micpath.2012.07.007
Yamashita, T., Kawada-Matsuo, M., Katsumata, T., Watanabe, A., Oogai, Y., Nishitani, Y., et al. (2019). Antibacterial activity of disodium succinoyl glycyrrhetinate, a derivative of glycyrrhetinic acid against Streptococcus mutans. Microbiol. Immunol. 63, 251–260. doi: 10.1111/1348-0421.12717
Yoshida, K., Furihata, K., Yamane, H., and Omori, T. (2001). Metabolism of 18β-glycyrrhetinic acid in Sphingomonas paucimobilis strain G5. Biotechnol. Lett. 23, 253–258. doi: 10.1023/A:1005637809636
Zhang, D., Xu, D. H., and Shoemaker, C. A. (2015). Immunization with recombinant aerolysin and haemolysin protected channel catfish against virulent Aeromonas hydrophila. Aquac. Res. 48, 875–882. doi: 10.1111/are.12931
Keywords: glycyrrhetinic acid β, Aeromonas hydrophila, growth curve, virulence phenotypes, Carassius auratus
Citation: Chen K, Qin T, Pan L, Bing X, Xi B and Xie J (2023) Effects of glycyrrhetinic acid β on growth and virulence of Aeromonas hydrophila. Front. Microbiol. 14:1043838. doi: 10.3389/fmicb.2023.1043838
Received: 14 September 2022; Accepted: 24 January 2023;
Published: 10 February 2023.
Edited by:
Francesca Bonvicini, University of Bologna, ItalyReviewed by:
Yu Ying Fu, Fujian Chuanzheng Communications College, ChinaCopyright © 2023 Chen, Qin, Pan, Bing, Xi and Xie. This is an open-access article distributed under the terms of the Creative Commons Attribution License (CC BY). The use, distribution or reproduction in other forums is permitted, provided the original author(s) and the copyright owner(s) are credited and that the original publication in this journal is cited, in accordance with accepted academic practice. No use, distribution or reproduction is permitted which does not comply with these terms.
*Correspondence: Bingwen Xi, ✉ eGlid0BmZnJjLmNu; Jun Xie, ✉ eGllakBmZnJjLmNu
Disclaimer: All claims expressed in this article are solely those of the authors and do not necessarily represent those of their affiliated organizations, or those of the publisher, the editors and the reviewers. Any product that may be evaluated in this article or claim that may be made by its manufacturer is not guaranteed or endorsed by the publisher.
Research integrity at Frontiers
Learn more about the work of our research integrity team to safeguard the quality of each article we publish.