- 1Key Laboratory of Livestock and Poultry Multi-omics, Ministry of Agriculture and Rural Affairs, College of Animal and Technology (Institute of Animal Genetics and Breeding), Sichuan Agricultural University, Chengdu, Sichuan, China
- 2Farm Animal Genetic Resources Exploration and Innovation, Key Laboratory of Sichuan Province, Sichuan Agricultural University, Chengdu, Sichuan, China
In order to investigate the effects of dietary probiotics supplementation on laying performance, egg quality, serum hormone levels, immunity, antioxidant, and gut microbiota of layers at different laying stages, a total of 168 Tianfu green shell laying hens (28-day-old) were randomly divided into 2 treatments: a non-supplemented control diet (NC), and diet supplemented with 10 g/kg of probiotics, respectively. Each treatment had 6 replicates with 14 hens per replicate. The feeding trial lasted for 54 weeks. The results showed that the supplementation of probiotics significantly increased the average egg weight, improved egg quality (p < 0.05) and ovarian development. Meanwhile, probiotics increased the serum hormone levels of E2 and FSH, and antioxidant indices T-AOC and T-SOD (p < 0.05) of laying hens at different laying stages (p < 0.05), decreased the expression of proinflammatory factors including IL-1, IL-6 and TNF-α (p < 0.05). Furthermore, using 16S rRNA sequencing, we observed that the addition of probiotics increased the distribution of Firmicutes, Bacteroidota and Synergistota at early laying period. Meanwhile, Bacteroidota, Actinobacteriota, Verrucomicrobiota and Deferribacterota showed an increasing trend at the peak of egg production. The relative abundance of Firmicutes, Desulfobacterota and Actinobacteriota were significantly increased at the late laying period. Moreover, PICRUSt2 and BugBase analysis revealed that at the late laying period, the probiotics supplementation not only enriched many significant gene clusters of the metabolism of terpenoids and polyketide, genetic information processing, enzyme families, translation, transcription, replication and repair, and nucleotide metabolism, but also decreased the proportion of potential pathogenic bacteria. To sum up, these data show that the addition of probiotics not only improves the performance, egg quality, ovarian development and immune function of laying hens at different laying period, but also improves the gut microbiota of layers, thus enhances production efficiency.
Introduction
Laying hens are known to be a kind of highly productive and powerful animals, and their reproductive performance determines the economic benefits of laying hens breeding. However, after the peak laying rate of high-intensity metabolism, laying hens gradually entered the late laying stage and occupied a long time in the whole production cycle. In the late stage of laying, laying hens were more susceptible to external factors due to the decline of ovarian function, and weakened resistance to stress and disease, which were often accompanied by low laying rate, low albumen height, poor eggshell quality and a variety of diseases (Liu et al., 2013, 2019), thus increasing the difficulty and cost of breeding. Therefore, it is necessary to adopt strategies to improve the performance and quality of layers in the late laying period, prolong the laying cycle and improve the reproductive efficiency of laying hens (Zhang et al., 2019; Guo et al., 2020). To reduce the use of drugs in farming and avoid antibiotic residues in eggs, producers rely on safe alternatives to improve the health and persistency of egg production in aged layers (Qu et al., 2018). So, dietary supplementation with prebiotics, probiotics and synbiotics to improve the performance and health of laying hens has attracted increasing attention (Liu et al., 2019; Lv et al., 2019).
Probiotics, also known as active bacterial preparation and growth enhancers, are generally defined as “live microorganisms which, when administered in adequate amounts, confer health benefits on the host” (Gerritsen et al., 2011). It has a wide range of species, which are typically classified as Lactobacillus, Bifidobacterium and Gram-positive cocci, and yeast (Saccharomyces) genera (Fijian, 2014), with health benefits, growth promotion, non-toxic side effects, and other characteristics. Probiotics supplementation in laying hen diets have been proven to improve hen’s performance parameters including egg production, feed conversion ratio and egg quality, disease resistance and animal welfare (Smith, 2014; Villageliu and Lyte, 2017; Khan et al., 2020; Sjofjan et al., 2021; Bindari and Gerber, 2022; Jiang et al., 2022). For instance, Macit et al. (2021) reported that supplementation of humate and probiotics into diets could improve the content of monounsaturated fatty acids in yolk, feed conversion ratio and yolk color. Yan et al. (2019) demonstrated that confirmed that dietary probiotics reduced shell-less egg production and improved bone mineralization in laying hens. Park et al. (2016) confirmed that probiotics (Enterobacter faecium) supplementation significantly increased laying rate, eggshell thickness and nutrient digestibility, and reduced ammonia emission of laying hens. The mechanism of probiotics improving hen performance and egg quality may be related to changes in intestinal microbial composition (Fuller, 1989; Mead, 2000). Probiotics have been shown in studies to promote the growth of non-pathogenic co-anaerobic bacteria and gram-positive bacteria while inhibiting pathogen proliferation and promoting nutrient digestion and utilization (Yeo and Kim, 1997; Mountzouris et al., 2010). Furthermore, probiotics may stimulate immune regulation in a non-inflammatory manner to preserve intestinal integrity (Murugesan et al., 2014; Waititu et al., 2014; Lee et al., 2015) and metabolic homeostasis (O’Mahony et al., 2015), and ultimately regulating host behavior (Bienenstock et al., 2015; Neijat et al., 2019). However, to date, numerous studies on probiotics in laying hen diets have focused on peak or late laying period (Mikulski et al., 2012; Abdelqader et al., 2013), and studies that directly link probiotics to changes in the gut microbiota of laying hens during early, peak and late laying period are scarce.
The gut microbiota of chicken is a diverse community of hundreds of different microbes, which was frequently influenced by factors such as nutrition, gender, age, breed, feeding style and breeding density (Pourabedin and Zhao, 2015; Ding et al., 2017; Wang et al., 2020). Previous studies have shown that intricate gut microbiota frequently affects the health and performance of chicken (Bai et al., 2021). For example, the Clostridium species in the chicken cecum, especially clusters IV and XIVa, are the dominant microbiota in the chicken cecum and has a significantly contributing to growth (Eeckhaut et al., 2011). Moreover, numerous studies have proposed multiple potential mechanisms by which prebiotic-mediated changes in gut microbiota may confer health benefits, such as producing antimicrobial factors (Muñoz et al., 2012), improving intestinal morphology (Pourabedin et al., 2014) and stimulating the adaptive immune system of the host (Yitbarek et al., 2012). In the comprehensive review by Gaggia et al. (2010), dietary prebiotic supplementation increased the abundance of Lactobacillus and bifidobacterium in the gut microbiota of chickens. However, the effects of probiotics supplementation on the gut microbiota of laying hens at different laying stage are poorly known. Hence, the aim of this study was to evaluate the effects of supplemental dietary probiotics on laying performance, egg quality, antioxidant capacity, serum hormone levels, expression level of reproductive and immune related genes and gut microbiota in laying hens at early laying, peak laying and late laying period.
Materials and methods
Animals, diets and experimental design
A total of 168 healthy 28-days-old Tianfu green shell laying hens with similar initial body weight (215.40 ± 0.15 g) were obtained from the poultry breeding farm of Sichuan Agricultural University (Ya’an, China) and then randomly divided into experimental group and control group. Each group had 6 replicates and each replicate included 14 chickens. From 28 days of age, the control group (NC) was only fed the base feed and water, while the experimental group (PC) was fed the basal diet plus the probiotics. The main components of the compound probiotics used in this study were Bacillus subtilis (≥1 × 109 CFU/g), Lactic Acid Bacteria (≥2 × 107 CFU/g) and Saccharomyces (≥1 × 107 CFU/g), and were provided by Shandong Sukehan Biotechnology Co., Ltd. (Shandong, China). The dosage of probiotics should follow the commercial recommendations of the company, which is to add 10 g/kg of dietary weight to the diet. Meanwhile, the basal diets were based on the recommended nutrient content by the National Research Council (1994). All laying hens were housed in a fully enclosed chicken house and kept in wired three-level battery cages, with the room temperature kept at 22–26°C, the relative humidity at 65% and provided free access to feed and water throughout the experimental period. The photoperiod was set at 10 h (5 lux) from 17 weeks. and then increased 1 h per week until 16 h at 22 weeks. with an intensity of 10–15 lux. Meanwhile, the chickens were fed probiotics from 5 to 58 weeks of age, and the entire experimental period lasted 54 weeks. Furthermore, feces were cleaned regularly throughout the rearing period and layers were immunized according to normal immunization procedures.
Sample collection
12 birds per treatment were randomly chosen for slaughter at the end of the three different experimental period: early laying period (23 weeks), peak laying period (27 weeks) and late laying period (58 weeks), respectively. Before slaughter, 4 mL blood samples were collected from the wing vein and were subsequently centrifuged for 10 min at 3000 r/min at 4°C to separate the serum. Serum was stored in a new 1.5 mL tube at −20°C until analyzed. Each hen was eviscerated immediately after slaughter, and the cecum segment was identified and lapped prior to removal. Cecal contents were collected and stored at −80°C until further analysis. The ovarian tissues were collected and weighted, and parts of ovarian tissue was frozen with liquid nitrogen and stored at −80°C for subsequent RNA extraction and qRT-PCR analysis.
Laying performance and egg quality
Eggs were collected twice a day (11:00 am and 16:00 pm). The data on egg production, egg weight and unqualified eggs were recorded, and the average weekly egg production rate, average daily egg weight and broken egg rate of the whole laying cycle were calculated.
At the age of weeks 23, 27 and 58, 12 eggs from each treatment (balanced with egg weight, a total of 36 eggs per group during the experiment) were collected and the egg quality indices including egg weight (EW), egg shape index (ESI), eggshell strength (ESS), eggshell weight (ESW), eggshell thickness (ET), yolk weight (YW), yolk color (YC), albumen height (AH), and Haugh Unit (HU) were determined. The EW of individual egg were measured by an electronic scale. ESI was calculated using the formula: ESI (%) = (egg width in mm/egg length in mm) × 100. The width and length of eggs were measured by 0.01 mm Vernier calipers (Hoffmann Quality Tools Trading (Shanghai) Co., Ltd., China). ESS was measured by eggshell strength gauge (Robotmation, Tokyo, Japan). Subsequently, broken the egg and transferred the contents to a glass plate, using a trip-pod micrometer to measure the AH. The average AH at different locations (one near the yolk and the other at the end of the dense protein) was combined with egg weight to obtain the Haugh unit score for each egg according to the Haugh (1937) formula. The formula as follows: HU = 100 × log (height of albumen in mm-1.7 × EW0.37 in g + 7.6). Next, the egg yolks and albumen were separated and weighted on an electronic scale. To determine ESW, cleaned off any adhering albumen with water, dried them in a fume hood and weighted them. ET was measured in 3 different parts (blunt end, sharp end and middle) by a Vernier caliper. YC was evaluated according to Roche Yolk color fan (1, light yellow; 15, orange). The eggshell ratio (ESR, %) and the yolk ratio (YR, %) were calculated by ESW/EW × 100 and YW/EW × 100, respectively.
Cholesterol analysis
After determination of egg quality, yolk samples from each replicate were separated from the broken eggs. One gram of each yolk sample was mechanically homogenized with 9 times the volume of absolution ice-cold ethanol (1:9 weight/volume) under ice bath condition and subsequently centrifuged at 2500 r/min at 4°C for 10 min. The supernatant was aspirated, and the cholesterol concentration was determined using a cholesterol assay kit according to manufacturer’s instruction (Baolai Biotechnology Co. Ltd., Yancheng, China).
Measurement of serum hormone, apolipoprotein and antioxidant indexes
On the last day of week 23, 27 and 58, serum samples (n = 12) of per treatment groups were used to evaluate the concentration of follicle-stimulating hormone (FSH), luteinizing hormone (LH), estradiol (E2), vitellogenin (VTG) and very low-density lipoprotein (VLPL) by using commercially enzyme-linked immunosorbent assay (ELISA) kits obtained from Baolai Biotechnology Co., Ltd. (Yancheng, China) according to the manufacturer’s instructions. Also, the levels of total antioxidant capacity (T-AOC), total superoxide dismutase (T-SOD), methane dicarboxylic aldehyde (MDA) and glutathione peroxidase (GSH-Px) in serum were assessed using commercial ELISA kits and following the guidelines provided by the manufacturer (Baolai Biotechnology Co. Ltd., Yancheng, China).
Follicle counts
The ovaries (n = 12) including large and small yellow follicles, obtained at weeks 23, 27 and 58 in the control group and the treatment group were weighted. Then, the follicles were dissected and placed on a filter paper moistened with physiological saline. The long and short axes of follicles between basement membranes were measured with Vernier calipers, and the pre-hierarchical follicles were separated into 4 groups according to their morphology and diameter, namely small yellow follicle (SYFs, 6–8 mm), large yellow follicle (LYFs, 8–10 mm), small white follicle (SWFs, 2–4 mm), and large white follicle (LWFs, 4–6 mm) (Johson, 2015). However, the diameter of other follicles >10 mm were hierarchical follicles (F1–F5). After classification, all types of ovarian follicles were counted and weighted.
mRNA relative expression levels of genes related to reproduction and immunity
The relative expression levels including follicle-stimulating hormone receptor (FSHR), luteinizing hormone receptor (LHR), estrogen receptors alpha (ERSα), steroidogenic acute regulatory protein (StAR), bone morphogenetic protein 15 (BMP-15), anti-mullerian hormone (AMH), interleukin 1 (IL-1), interleukin 6 (IL-6), toll-like receptor 4 (TLR4), tumor necrosis factor-α (TNF-α), and cyclooxygenase-2 (COX2) were conducted by the quantitative real-time polymerase chain reaction (PCR) analysis.
TRIzol reagent (TakaRa, Dalian, China) was used to extract total RNA from ovarian tissues (n = 12) at 3 different periods (23, 27, and 58 week) in control group and treatment group according to manufacturer’s instructions. The resulting total RNA was reversely transcribed into cDNA using the PrimeScrip RT reagent kit (TakaRa, Dalian, China). Then, the cDNA was amplified in a CFX96-Touch™ Real-time PCR System (Bio-Rad Laboratories, CA, USA) using the SsoFastTM EvaGreen® supermix (Takara, Dalian, China). The reaction mixture (10 μl) for qPCR contained 5 μl 2 × SsoFastTM EvaGreen® supermix, 1 μl cDNA sample, 0.5 μl each of forward and reverse primers and 3 μl RNase free water. The reaction procedure as follows: 1 min at 98°C, and 38 cycles of 98°C for 15 s, annealing temperature for 20 s. The primer sequences are listed in Table 1. Each sample was run in triplicate, and the glyceraldehyde 3-phosphate dehydrogenase (GAPDH) was used as the housekeeping gene to calculate relative mRNA levels by using the 2−△△Ct method.
Cecal microbial sequencing analysis
Total genomic DNA was extracted from cecal content samples at 3 different periods (21, 27, and 58 weeks) in control group and treatment group using the QIAamp Fast DNA stool Mini Kit (Qiagen, Germany) according to the procedure provided by manufacturer. The concentration and integrity of DNA was assessed by a NanoDrop 2000 spectrophotometer (Thermo Fisher Scientific, Waltham, MA, USA) and 1% agarose gel, respectively. The V3-V4 region of the 16S rRNA gene was amplified using the primer 341F (5’-CCTACGGGNGGCWGCAG-3′) and 805R (5′- GACTACHVGGGTATCTAATCC-3′) in the ABI GeneAmp 9700 PCR System. A 25 μL mixture of 12.5 μL Phusion® Hot Start Flex 2× Master Mixart version, 5.0 μL of forward and reverse primers (1 μM), and 50 ng of template DNA was prepared for PCR. Thermal cycling as follows: 98°C for 30 s, 98°C for 10 s for 35 cycles, 54°C for 30 s, 72°C for 45 s, and 72°C for 10 min. The final amplified products were purified by an AxyPrep DNA gel extraction kit (Axygen Bioscience, Union City, USA) and quantified by a Qubit dsDNA BR Assay Kit (Invitrogen, Shanghai, China). At last, the purified PCR products were sequenced on the Illumina MiSeq platform according to the standard protocols of the LC-Biotechnology Co., Ltd. (Hangzhou, Zhejiang Province, China).
Sequence processing and bioinformatical analysis
After the original pairing sequence were demultiplexed, FLASH software (version 1.2.7) was used to merge the resulting sequences, and fastp (version 0.19.6) was used to filter the quality (Magoc and Salzberg, 2011; Chen et al., 2018). High-quality reads were de-noised using the divisive amplicon denoising algorithm (DADA2) (Callahan et al., 2016) plugin in QIIME2 (version 2020.2). RDP classification algorithm was used to classify each 16S rRNA gene sequences from the SILVA database (v138).1 Alpha diversity indices including Chao 1, ACE, Observed-species, Good-coverage, Shannon and Simpson were calculated using the QIIME (version 1.7.0). The beta (Bray–Curtis dissimilarity metric) diversity was analyzed by the “phyloseq” in R package. Analysis of similarities (ANOSIM) was carried out in the R package vegan. Linear discriminant analysis effect size (LEfSe) analysis was conducted to analyze the statistical significance and biological relevance. PICRUSt2 was used to predict the functional differences of cecal microbiota in chickens at different laying stages (Parks et al., 2014), and KEGG metabolic pathway was used to observe the functional differences among different groups.
Statistical analysis
The results of laying performance, serum parameters, egg quality, and relative gene expression were all tested by one-way ANOVA using the SPSS 22.0 software (SPSS Inc., Chicago, IL, United States) followed by Duncan’s multiple comparison test. If p < 0.05, the difference was significant. All data in this study were presented as mean ± standard error of mean (SEM), and the graphs were generated by GraphPad Prism software 8.0 (GraphPad Inc., San Diego, USA).
Results
Probiotics supplementary improved hen productivity, ovarian weight and numbers of the different-sized follicles
The laying rate of Tianfu green shell laying hens in each group were shown in Figure 1A. Compared with the NC group, dietary supplementation with probiotics significantly increased the laying rate of hens during the whole laying period (including early laying, peak laying and late laying) (p < 0.05). However, it is worth noting that after 52 weeks, the egg production rate of the PC group was slightly lower than that of the NC group, but the difference not significant (p > 0.05). Moreover, probiotic supplementation increased the average weekly egg weight, especially the average weekly egg weight at weeks 37, 41, 42, 48, 52 and 53 was significantly higher than that of the control group (p < 0.05) (Figure 1B), indicating that dietary probiotics can improve the egg weight of laying hens in the late laying period. The broken egg rate data are summarized in Table 2. Before 45 weeks of age, compared with the NC group, the egg breaking rate of PC group was significantly decreased, but after 46 weeks of age, the egg breaking rate was slightly increased.
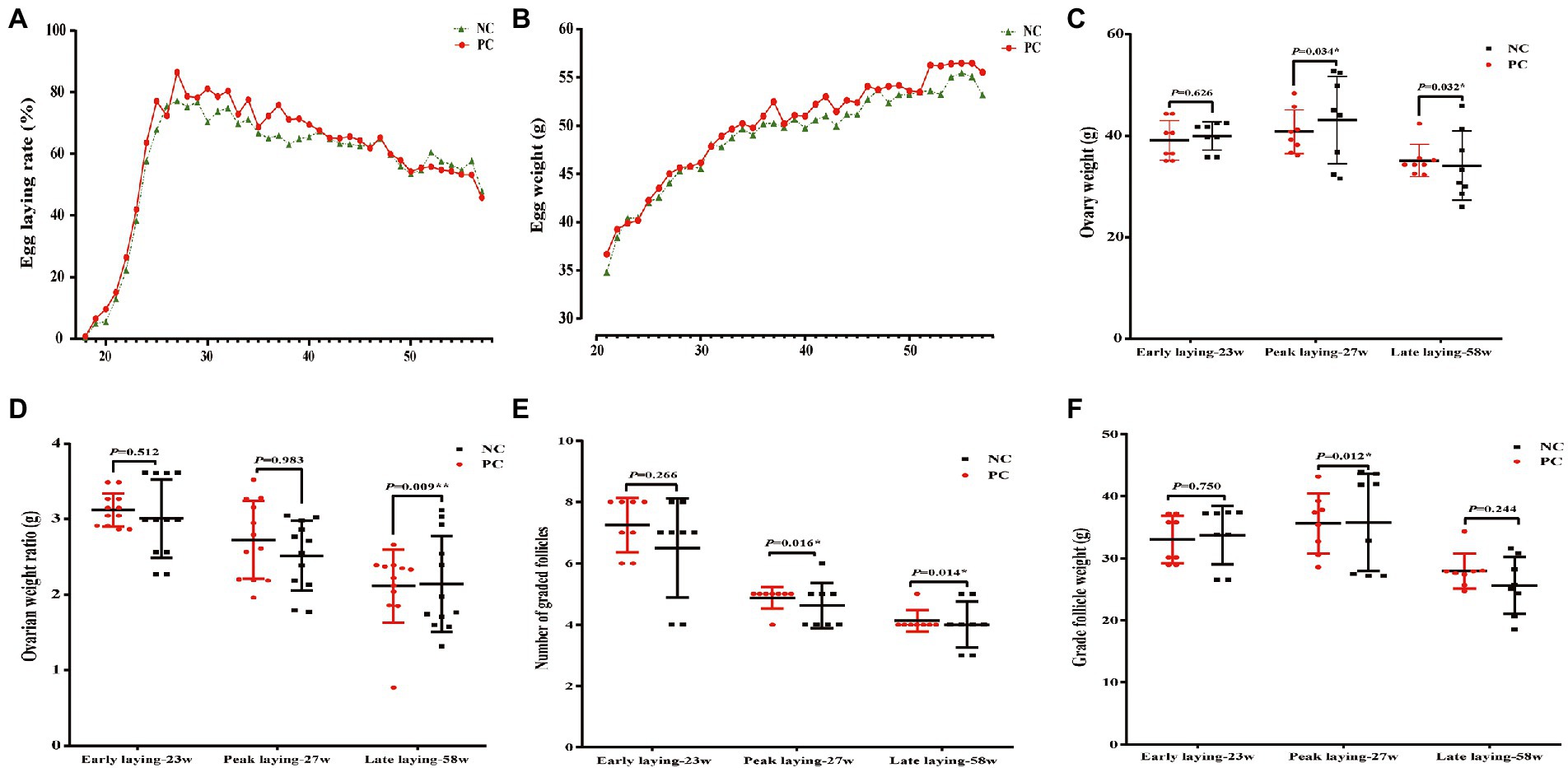
Figure 1. Effect of dietary supplementation of probiotics on performance, number and weight of follicles of laying hens at different laying period. (A) Lay rate; (B) Egg weight; (C) Ovary weight; (D) Ovarian weight ratio; (E) Number of grade follicles; (F) Grade follicles weight. Data present the mean ± SEM, *p < 0.05, **p < 0.01.
In addition, the ovarian weight and number of follicles of different sizes were determined. The results showed that probiotics had certain effects on the development of reproductive organs of laying hens. Compared with the NC group, the number of grade follicles in the PC group increased throughout the laying period, and the ovarian weight and graded follicles weight also increased in the late laying period (Figures 1C, E, F). However, the ovarian weight ratio decreased significantly in the late laying period (Figure 1D, p < 0.01). Meanwhile, as shown in Table 3, the weight and number of SYFs, SWFs and LWFs tended to increase with dietary probiotics supplementation during the whole laying cycle, but the difference was not significant (p > 0.05). However, the number of LYFs in the PC group were decreased at late laying period, but the difference was not significant (p > 0.05).
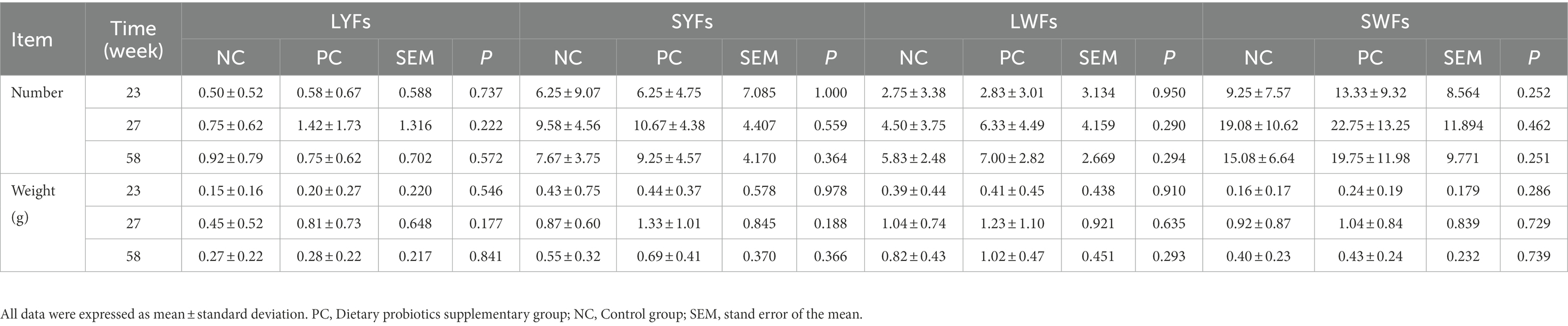
Table 3. The effect of probiotics on pregrade follicle weight and number in laying hens at different laying period.
Probiotics supplementary improved egg quality at different laying stages
Table 4 shows the effects of dietary probiotics supplementation on egg quality of laying hens at different laying stage. Compared with NC group, probiotics supplementation increased ESS, EW, AH, HU, yolk percentage and ESI during the whole laying period, especially in the late laying period (58w), the difference in ESS (p = 0.001) and ESI (p = 0.019) between the two groups was significant (p < 0.01, p < 0.05). The ET of the PC group was significantly higher than that of NC group at early laying (23w) and late laying (58w) (p < 0.01, p < 0.05), but lower than that of NC group at peak laying (27w), and the difference was not significant (p > 0.05). Moreover, compared with the NC group, YC in PC group was slightly increased and decreased at early laying and peak laying period, respectively, but not significant (p > 0.05).
Probiotics supplementary affected cholesterol and apolipoprotein levels in yolk
To investigate the effects of probiotic supplementation on cholesterol and apolipoprotein, the contents of cholesterol, VLDL and VTG in yolk were measured. Compared with NC group, the level of cholesterol and VTG in yolk of PC group were significantly increased and decreased in early laying and peak laying period (p < 0.01), respectively, but were opposite in late laying period (Figures 2A, C). Moreover, the VLDL level of yolk in the PC group were decreased compared with the NC group during the whole laying period, and reached a significant level in the late laying period (p < 0.05, Figure 2B).
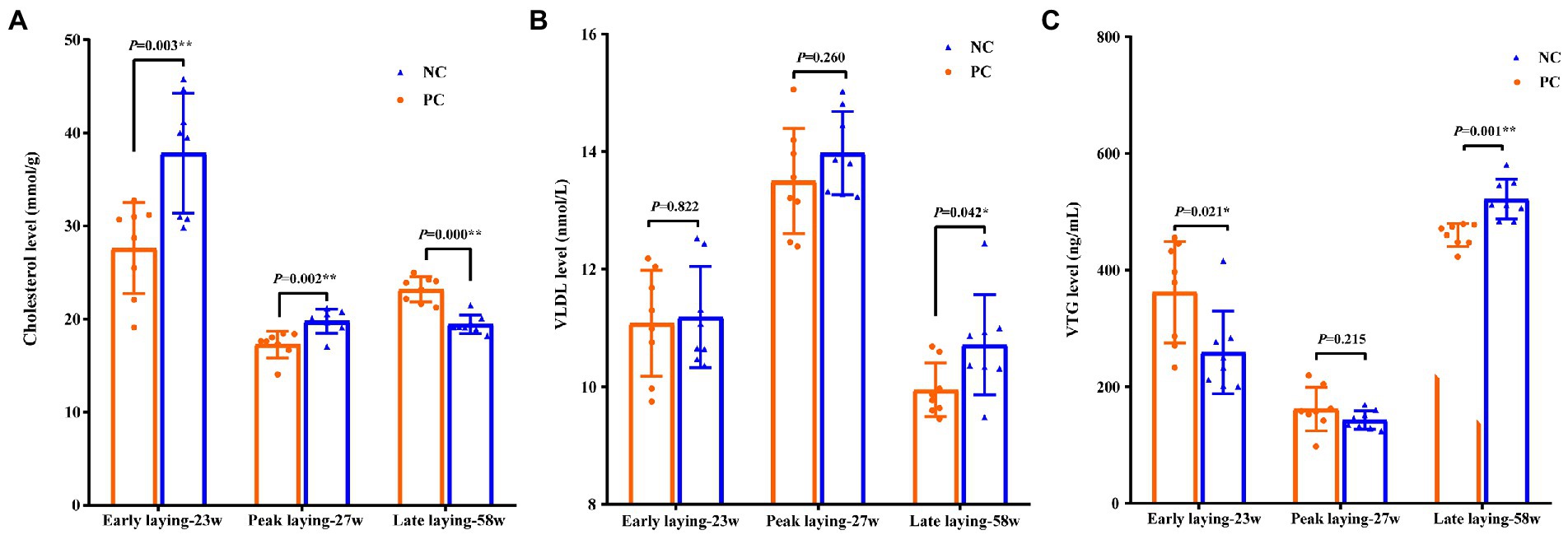
Figure 2. Effects of probiotic supplementation on lipid metabolism in serum and cholesterol content of laying hens in egg yolk at different laying period. (A) Cholesterol; (B) VLDL; (C) VTG. Data present the mean ± SEM, *p < 0.05, **p < 0.01.
Effects of probiotics on the serum hormone level
The content of serum hormone can reflect the development and maturity of ovarian follicles of laying hens. As shown in Figures 3A, C, compared with the NC group, FSH and E2 levels in the PC group were increased during the whole laying period, and there was no significant difference between the two groups (p > 0.05) at other laying periods except that the E2 content in PC group was significantly higher than that in the NC group at early laying period (p < 0.05). Moreover, an increase in the serum concentration of LH was observed in the PC group compared to the NC one during the peak laying (p < 0.05), but decreased during the early laying and late laying period (Figure 3B).
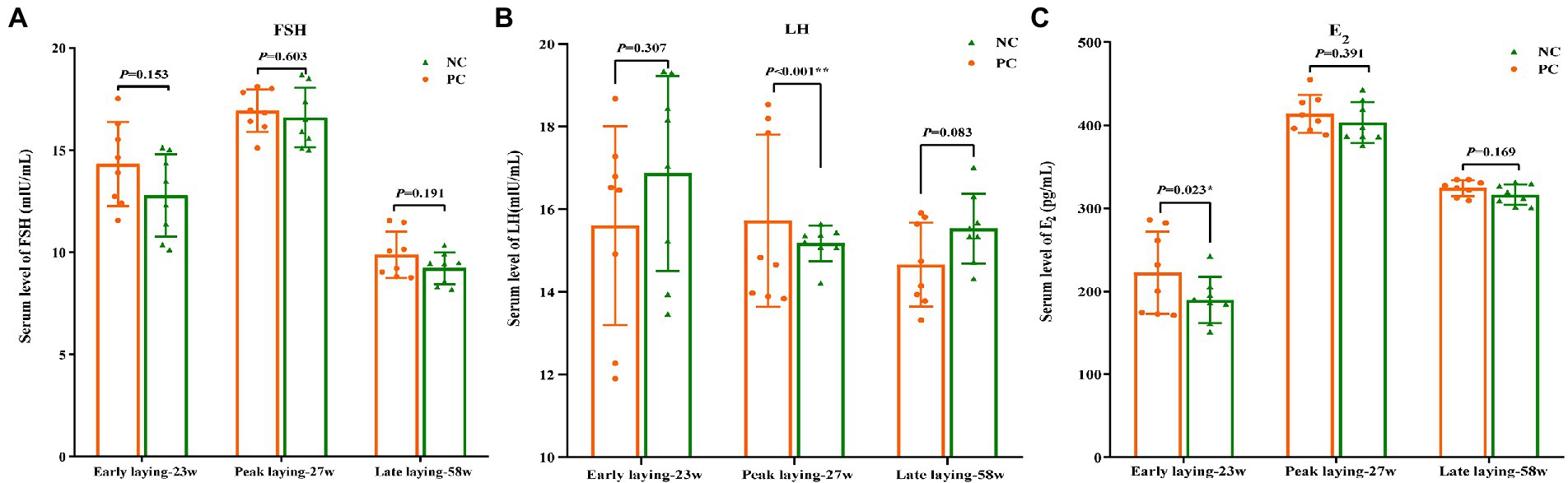
Figure 3. Effect of dietary probiotics on serum hormone levels of laying hens at different laying period. (A) Follicle-stimulating hormone (FSH); (B) Luteinizing hormone (LH); (C) Estradiol (E2). Data present the mean ± SEM, *p < 0.05, **p < 0.01.
Probiotics supplementation reduced oxidative stress
Using serum data from laying hens at different laying period, Figure 4 presents the antioxidant stress status. The data indicated that compared with the NC group, probiotics supplementation increased T-AOC and T-SOD levels during the whole laying period, as well as reduced the level of MDA, especially at the late laying period, the difference between the two groups was significant (p < 0.05 or p < 0.01, Figures 4A–C). However, the serum GSH-Px level in PC group was slightly higher than that of NC group at the early stage of laying, but significantly lower than that of NC group at the peak and late stage of laying (p < 0.05, p < 0.01, Figure 4D).
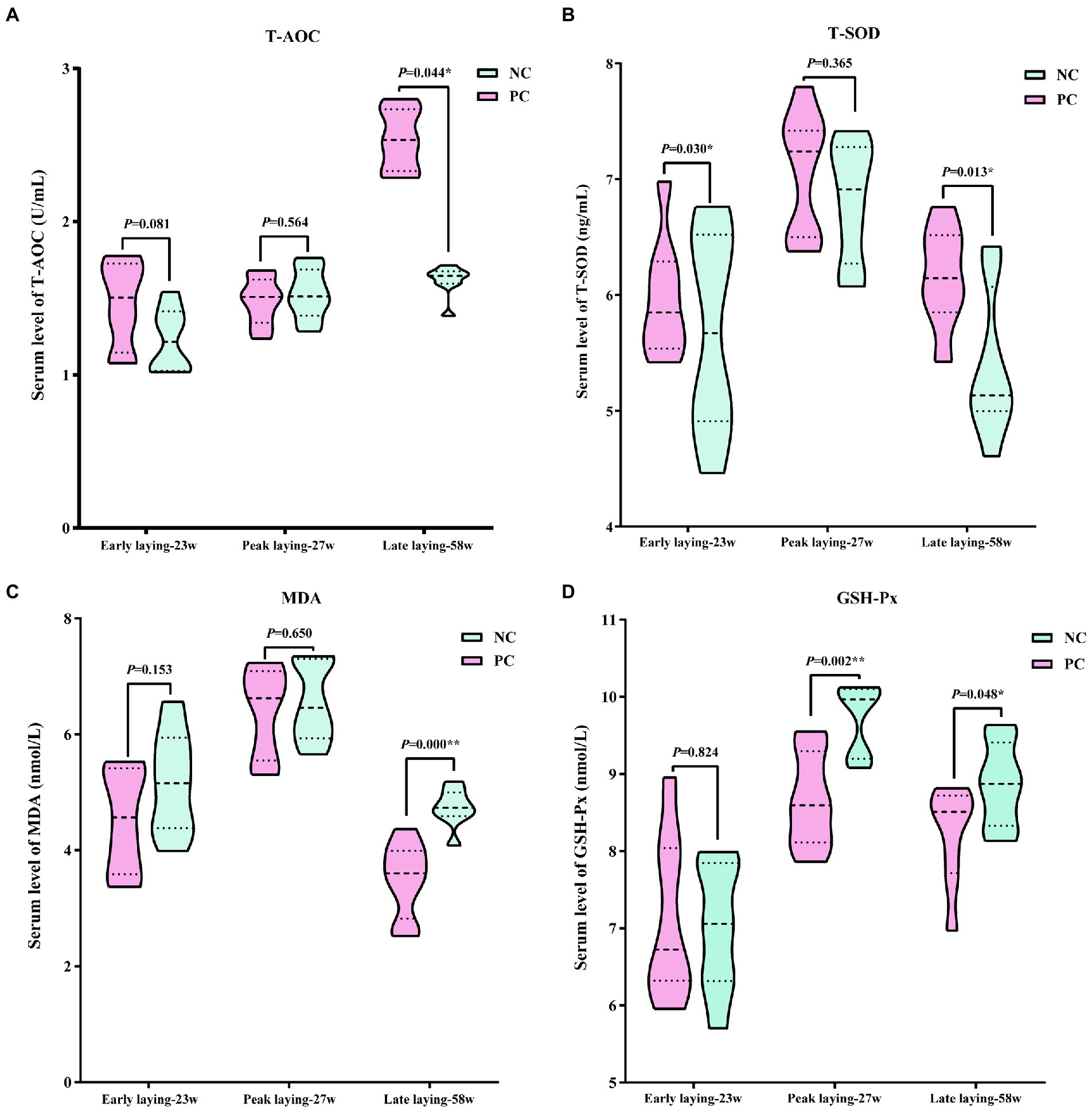
Figure 4. Effect of dietary probiotics on serum antioxidant index levels of laying hens at different laying period. (A) T-AOC; (B) T-SOD; (C) MDA; (D) GSH-Px. Data present the mean ± SEM, *p < 0.05, **p < 0.01.
Probiotics supplement improved ovarian function by increasing the gene level associated with ovarian development
To assess whether probiotics can improve ovarian development and function, we examined the expression of ovarian cytohormone receptor genes including FSHR, LHR and ESRα, ovarian StAR, BMP15 and AMH. As shown in Figures 5A–C, the ovarian expression level of FSHR, LHR and ESRα in the PC group during the whole laying period were increased compared with the NC group, especially in the late laying period, reaching a significant or extremely significant level (p < 0.05, p < 0.01). After probiotics supplement, the expression levels of StAR and BMP15 in ovary were significantly higher than those in the NC group at the late stage of laying (p < 0.05, Figures 5D, E), indicating an improvement in reproductive performance at the late stage. Interestingly, compared with the NC group, the expression level of AMH in the PC group increased significantly at the early laying and peak laying period (p < 0.05), but decreased at the late laying period (Figure 5F), as well as the AMH expression level in the ovarian tissues decreased with time in both the NC group and PC group.
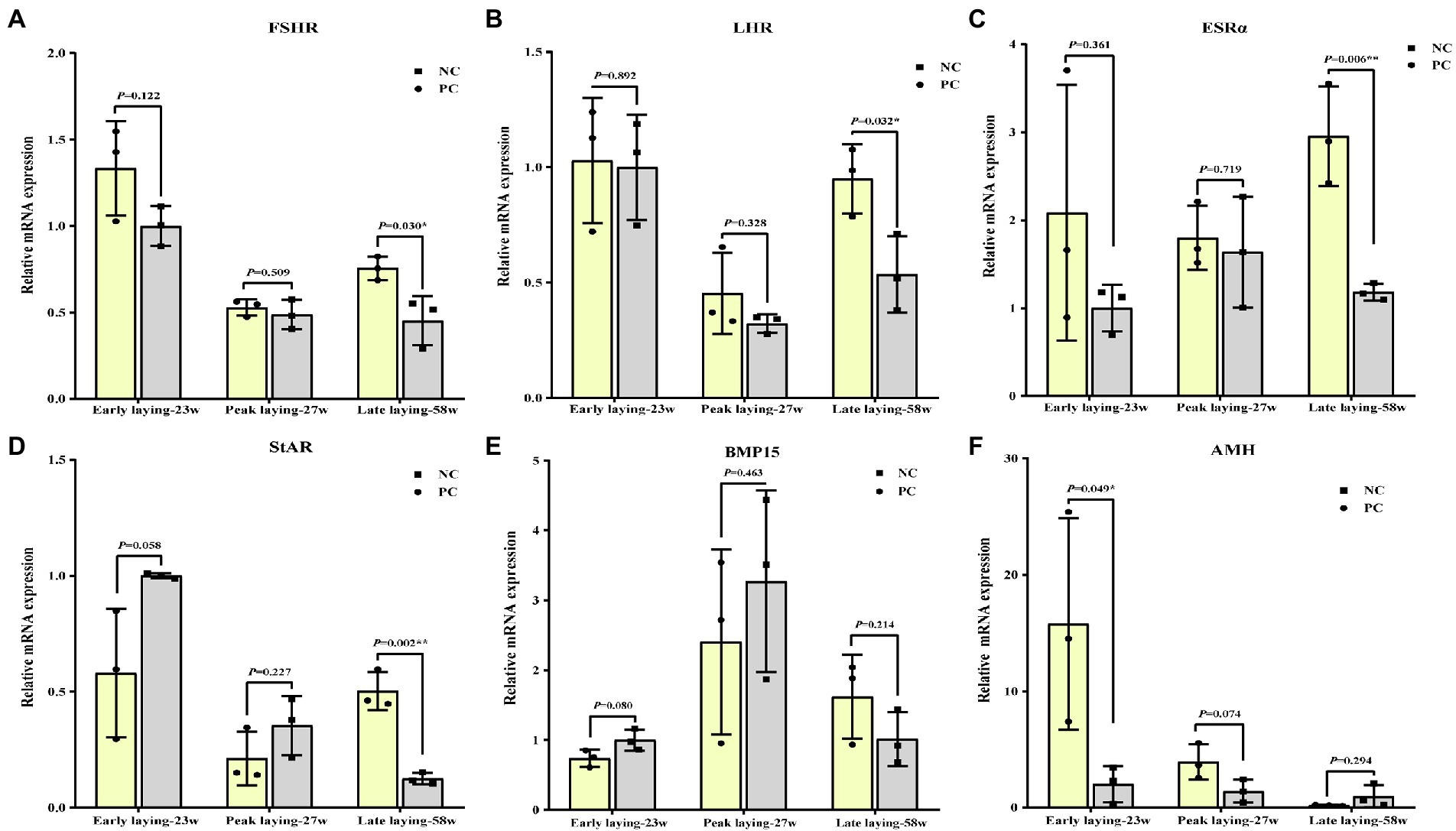
Figure 5. Effects of dietary probiotics on mRNA expression of genes related to ovarian development of laying hens at different laying period. (A) Follicle-stimulating hormone receptor (FSHR); (B) Luteinizing hormone receptor (LHR); (C) Estrogen receptors alpha (ERSα); (D) Steroidogenic acute regulatory protein (StAR); (E) Bone morphogenetic protein 15 (BMP15); (F) Anti-mullerian hormone (AMH). Data present the mean ± SEM, *p < 0.05, **p < 0.01.
Probiotics supplement improved ovarian immune function
Figure 6 summarized the relative mRNA expression of inflammation related cytokines in the ovarian. The expression level of proinflammatory cytokines such as IL-1, IL-6 and TNF-α in ovary were decreased at three different laying stages due to the effect of probiotics as compared to NC group (Figures 6A–C), while the level of anti-inflammatory cytokines TLR4 was increased (Figure 6E). There was no significant difference between the two groups at the late laying period, except that TLR 4 and IL-6 expression at the early laying period and TNF-α expression at the peak laying period were significantly different (Figures 6B, C, E, p < 0.05). Moreover, compared with the NC group, the expression level of Cox2 in PC group was increased in the early laying and peak laying, as well as decreased in the late laying period, but the difference was not significant (Figures 6D, p > 0.05).
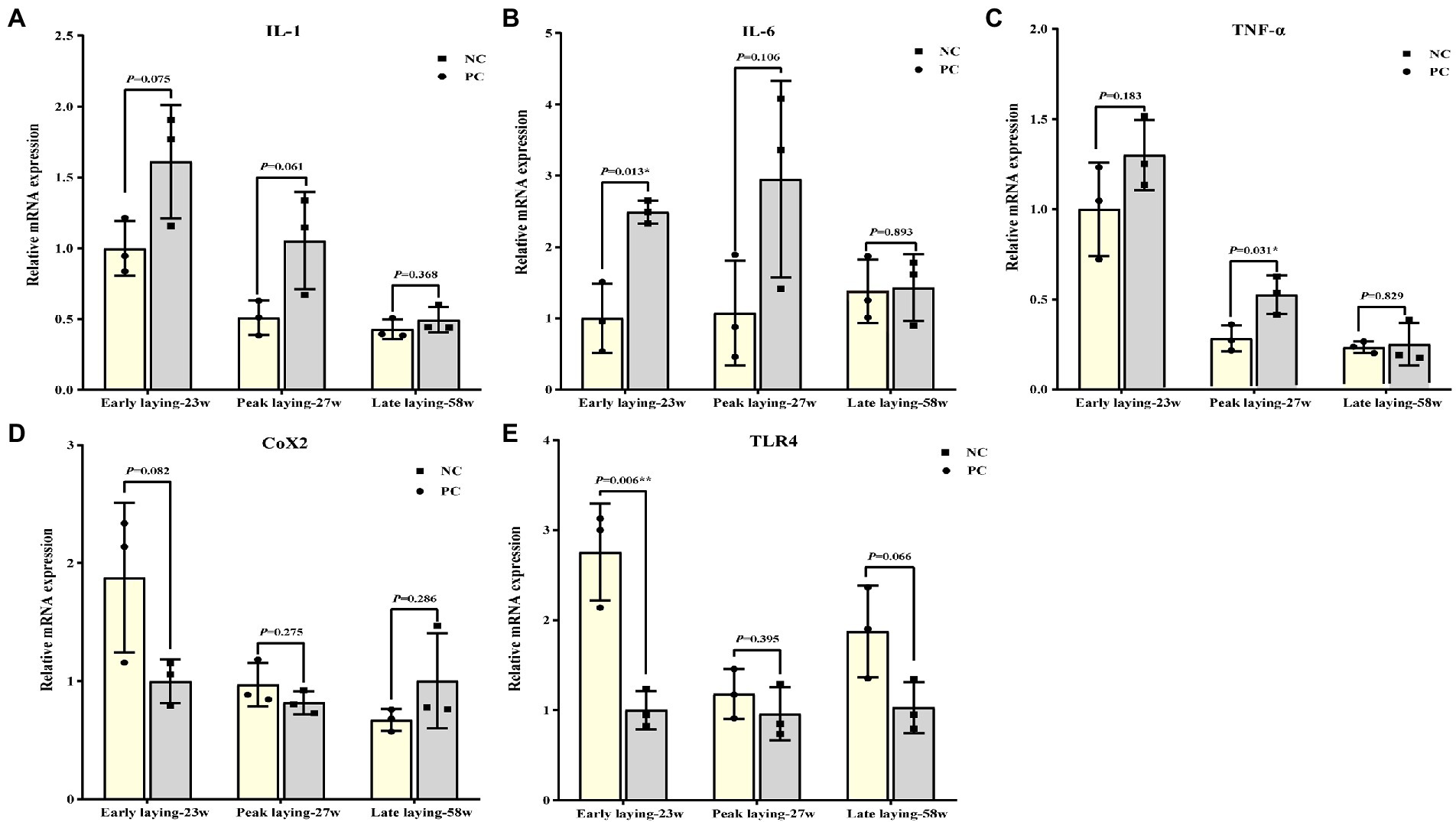
Figure 6. Effects of dietary probiotics supplementary on the mRNA expression of pro- and anti- inflammation related cytokines related genes in ovary at different laying period. (A–D) mRNA expression of proinflammatory cytokines including interleukin 1 (IL-1), interleukin 6 (IL-6), tumor necrosis factor-α (TNF-α), and cyclooxygenase-2 (COX2); (E) mRNA expression of anti-inflammatory cytokines related gene toll-like receptor 4 (TLR4). Data present the mean ± SEM, *p < 0.05, **p < 0.01.
Microbial composition in the cecal content
To further investigate whether probiotics could affect intestinal microbiota at different laying stages, we analyzed the composition of cecal flora composition by 16S rDNA gene sequencing. A total of 1,170,991 V3-V4 16S rDNA effective sequences were obtained from the 18 samples, with an average of 65,055 sequences per sample. A total of 21,940 distinct operational taxonomic units (OTUs) were identified at the 97% identity level with high threshold identity and with an average of 1,219 OTUs for each group sample. The curves for the observed OUTs and species rank approached a plateau, suggested that the samples had sufficient sequence coverage to allow nearly all bacterial species in cecal samples to be identified (Figures 7A, B). Alpha diversity analysis revealed that Chao1 index were decreased in the PC group during the whole laying period (Figure 7C), and Shannon index in the PC group decreased in the peak and late laying period except that it was higher at the early laying period as compared with NC group; however, it had no difference between two groups (Figure 7D). Simpson indices showed that species evenness was the highest in the late laying period, reaching the maximum value of 1 (Figure 7E; Supplementary Table S1). Moreover, the principal coordinate analysis (PCoA) based on weighted unifrac distances further showed that there were differences in microbiota among all groups (Figure 7F). A Venn diagram showed that there were 151 OTUs common at different laying period in the caecum of laying hens. Additionally, 1,657 and 1,454 OUTs were uniquely present in the NC group and PC group at early laying period (23w), respectively; 3,042 and 2,897 OTUs were uniquely present in NC group and PC group at peak laying period (27w), respectively; 3,536 and 2,834 OTUs were uniquely present in the NC group and PC group at late laying period (58w), respectively (Figure 7G).
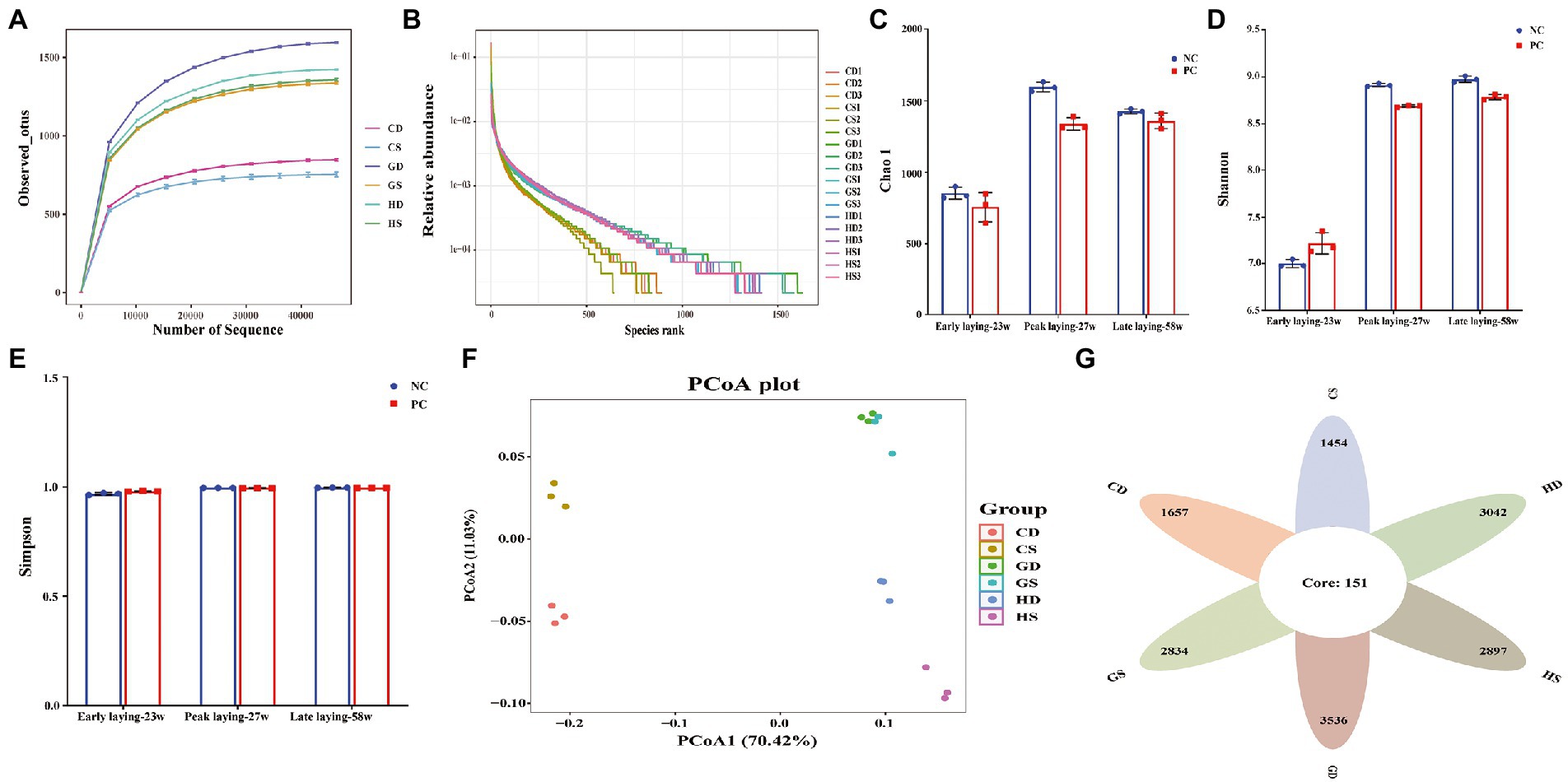
Figure 7. Effects of dietary probiotics supplementary on cecum microbiota diversity and composition in layers at different laying period. (A) Rarefaction curves. Sequences sampled represent sequencing reads that were grouped into operational taxonomic units (OTUs) based on their 97% similarity; (B) Abundance curve of species identified; (C) Chao 1 diversity index boxplot; (D) Shannon diversity index boxplot; (E) Simpson diversity index plot; (F) The principal coordinate analysis (PCoA) of the cecum microbiota based on unweighted UniFrac metric; (G) Venn diagram showing the number of control and probiotic-supplemented cecal microbial communities of layers at different laying stages sharing OTUs (97% similarity) and those that are unique.
Taxonomic composition of bacterial community
Intestinal microbiota taxonomic analysis showed that the probiotic supplementation changed the composition of intestinal microbiota at different stages of the whole laying period. At the phylum level of the microbiota in the cecal, Firmicutes Bacteroidota and Bacteroidetes were the predominant bacteria from each group in this study, with relative abundance of 40.86, 30.77 and 16.93%, respectively. Desulfobacterota (2.73%) and Actinobacteriota (1.43%) were relatively lower in abundance, but they performed well across the whole experimental group. Other minor phyla such as Proteobacteria (0.43%), Verrucomicrobiota (0.33%), Synergistota (0.31%), Deferribacterota (0.29%), Spirochaetota (0.23%) and Fusobacteriota (0.14%) were also found (Figure 8A). We found a significant increase in relative abundance of Firmicutes, Bacteroidota and Synergistota in the PC group compared with the NC group at early laying (23w) (Figure 8B). Beyond that, compared with the NC group, Bacteroidota, Desulfobacterota, Actinobacteriota, Verrucomicrobiota and Deferribacterota in the PC group have increased significantly at peak laying (27w) (Figure 8C), and the relative abundance of Firmicutes, Desulfobacterota and Actinobacteriota also significantly increased at late laying (58w) (Figure 8D), but other bacterial phyla were decreased.
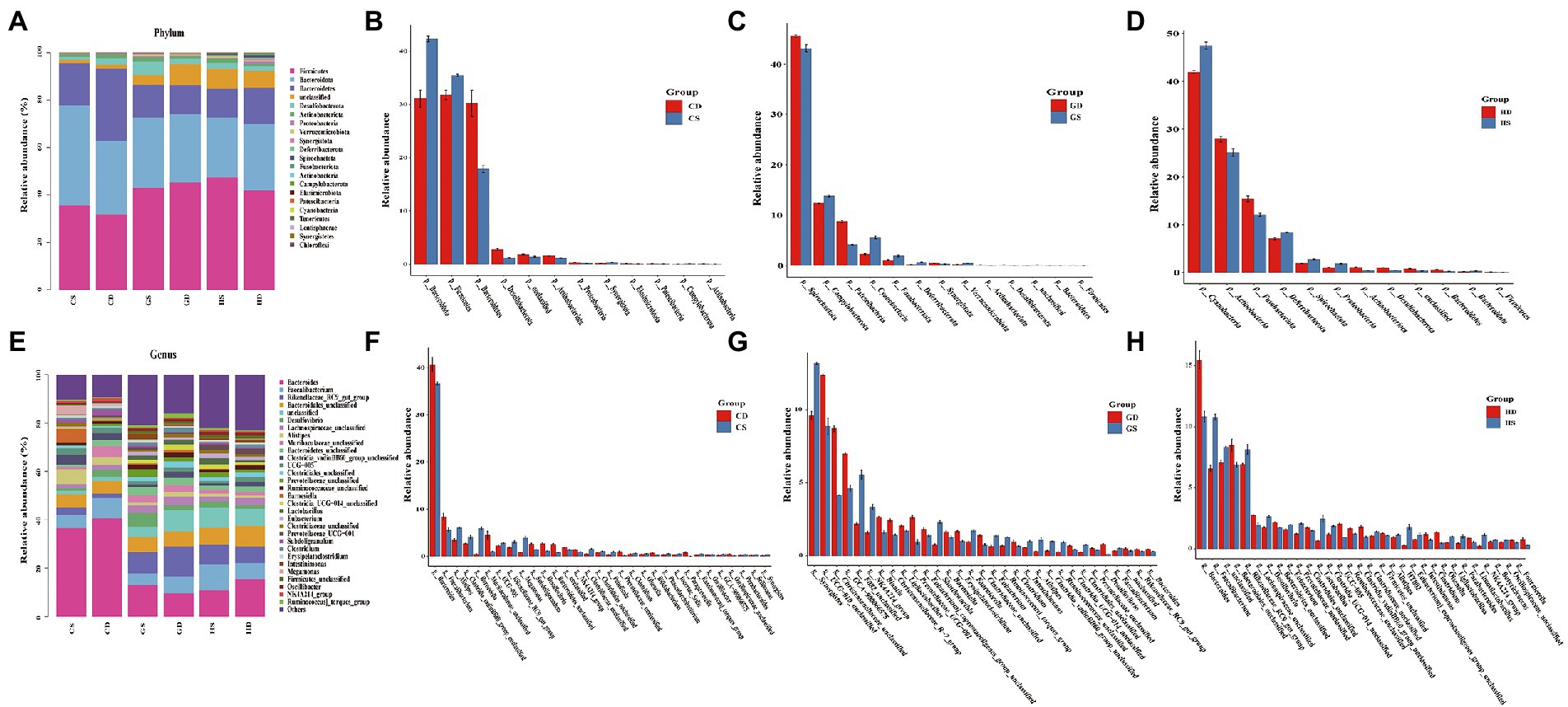
Figure. 8. Relative abundance and significant difference of top 30 OTUs at the phylum and genus level in the cecal microbiota of laying hens at different laying stages. (A) The relative abundance of cecal microbiota at phylum level in laying hens; (B) Barplot difference analysis of cecal microbiota in phylum level of laying hens at early laying period; (C) Barplot difference analysis of cecal microbiota in phylum level of laying hens at peak laying period; (D) Barplot difference analysis of cecal microbiota in phylum level of laying hens at late laying period; (E) The relative abundance of cecal microbiota at genus level in laying hens; (F) Barplot difference analysis of cecal microbiota in genus level of laying hens at early laying period; (G) Barplot difference analysis of cecal microbiota in genus level of laying hens at peak laying period; (H) Barplot difference analysis of cecal microbiota in genus level of laying hens at late laying period.
At the genus level, the dominant genera were Bacteroidetes, Faecalibacterium, Rikenellaceae_RC9_gut_group, and Bacteroidales_unclassified of all the groups (Figure 8E). Moreover, the relative abundance level of Alistipes, Clostridia_vadinBB60_group_unclassified, Barnesiella, UCG-005, Rikenellaceae_RC9_gut_group and Megamonas increased significantly after probiotics supplement at the early laying period (23w) (Figure 8F). Compared with the NC group, the relative abundance of Bacteroides, Desulfovibrio and Prevotellaceae_unclassified increased significantly, and the relative abundance of the Rikenellaceae_RC9_gut_group, Faecalibacterium, Clostridiales_unclassified, Clostridia_vadinBB60_group_unclassified and Alistipes decreased significantly at peak laying period (27w) (Figure 8G). It is noticeable that at late laying (58w), most cecal microorganisms in the PC group including Faecalibacterium, Rikenellaceae_RC9_gut_group, Desulfovibrio, Eubacterium and Lactobacillus increased dramatically (Figure 8H).
Changes in the cecum microbiome between NC group and probiotics supplement group (PC) at different laying period using linear discriminant analysis (LDA) effect size (LEfSe) was further compared based on the threshold of LDA score > 3. The results showed that there were 54, 80 and 75 dominant taxa of PC group in cecal content samples of Tianfu laying hens at the early, peak and late laying period, respectively, which could be used as potential biomarker bacteria (Supplementary Figure S1). It was observed that the PC group at the peak of laying period had more differential biomarkers, followed by the late laying period and the least at early laying period.
Correlation analysis between the cecal bacteria
To investigate the possible interactions among members of the intestinal bacterial community in laying hens, we used Spearman correlation coefficient method to analyze the correlation between the top 30 microorganisms in genus level abundance. In this study, Bacteroidetes_unclassified, Ruminococcaceae_unclassified, Clostridia_UCG-014_unclassified, Lactobacillus, Firmicutes_unclassified, Oscillibacter and Lachnospiraceae_unclassified had a positive correlation with most bacteria (Figure 9A), but Firmicutes_unclassified and Bacteroidetes_unclassified had a significant negative correlation with Muribaculaceae_unclassified (Figure 9B). Furthermore, Bacteroides, UCG-005, Alistipes, Subdoligranulum, NK4A214_group and Megamonas had a significant negatively correlation with most bacteria, while Megamonas had a positively correlated with NK4A214_group (Figures 9A,B).
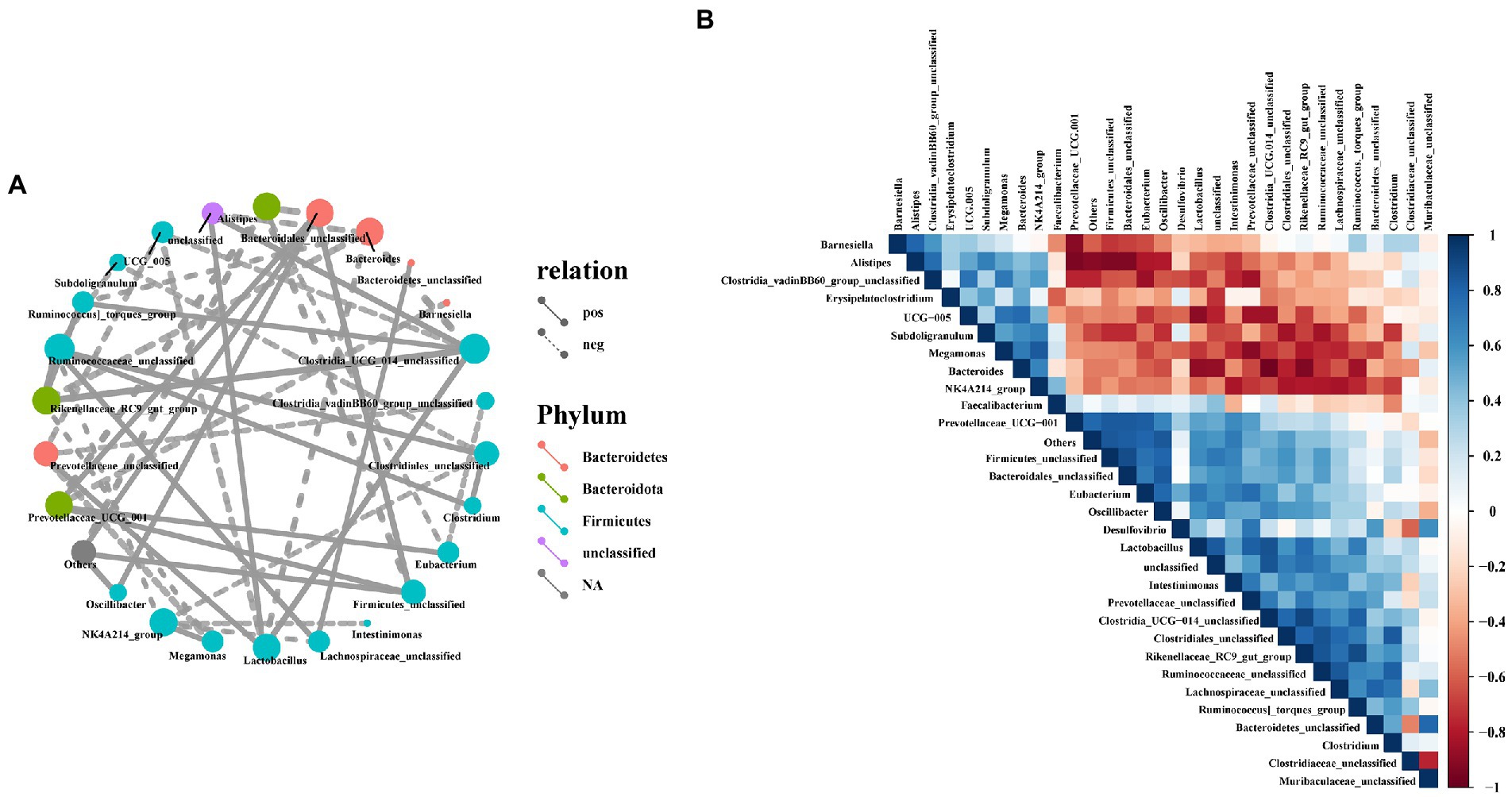
Figure 9. Correlation analysis of cecal microbiota in laying hens. (A) Network analysis at the genus level. Different nodes represent different dominant genera, the color of nodes indicates the phylum level species to which the species belongs, and the connection between nodes indicates the correlation between the two genera. A connecting line represents a significant correlation, whose width correlates with the strength of the correlation, while the line’s type indicated what type of interaction it is (solid line: positive correlation, dash line: negative correlation); (B) Heatmaps of Spearman correlation analyses among the abundance of top 30 gut microbiota in the cecum.
Predictive functional profiling of microbial communities
To further investigate the changes of cecal microbiota function in different laying stages after probiotics supplementation, we used PICRUSt2 technology to predict and analyze cecal microbiota function. Based on PICRUSt2 functional secondary classification results, the functional richness of cecal microbiota of hens at different laying stages was compared between the NC group and the PC group. The results shown that at the early laying period (23w), compared with NC group, PC group had extremely significantly increased gene clusters in amino acid metabolism, cardiovascular diseases, excretory system, immune system disease and metabolic diseases (Figure 10A; Supplementary Table S2; p < 0.01), and significantly increased gene clusters in cancer, genetic information processing, immune system and nervous system (Figure 10A; Supplementary Table S2; p < 0.05). Moreover, at the peak laying period (27w), 7 gene clusters of cell motility and carbohydrate metabolism in the PC group was extremely significantly higher than those in the NC group (Figure 10B; Supplementary Table S2; p < 0.01), while 3 gene clusters of signaling molecules and interaction, metabolism of cofactors and vitamins, and cardiovascular diseases in PC group were significantly higher than those in NC group (Figure 10B; Supplementary Table S2; p < 0.05). However, at the late laying period (58w), compared with the NC group, 9 gene clusters of the metabolism of terpenoids and polyketide, genetic information processing, enzyme families, immune system diseases, nucleotide metabolism, poorly characterized, replication and repair, transcription and translation were extremely significantly increased in PC group (Figure 10C; Supplementary Table S2; p < 0.01), as well as 1 gene clusters of cell growth and death enriched in the PC group (Supplementary Table S2; p < 0.05).
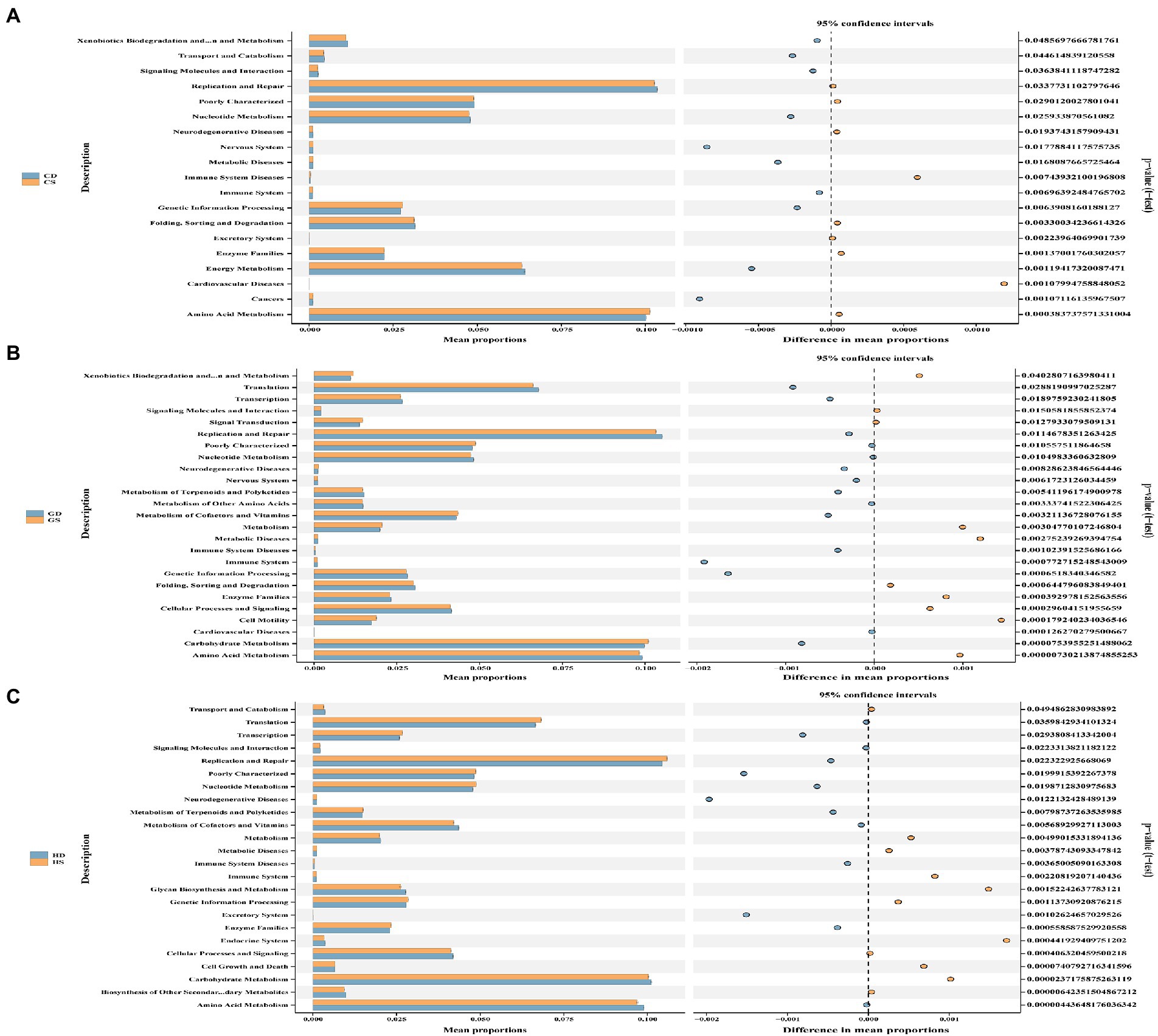
Figure 10. Functional analysis of microbial communities. PICRUSt2-predicted relative abundance of KEGG pathways (level 2) was compared between NC and PC groups of laying hens at different laying periods. (A) The early laying period (23w); (B) The peak laying period (27w); (C) The late laying period (58w). Welch’s correction was applied to the t test in order to analyze the differences between two groups at the same laying period. Statistical significance was determined by p < 0.01.
Meanwhile, we mapped 16S rRNA data to the KEGG pathway to predict the metabolic characteristics of gut microbiota at different laying period. At the early laying period (23w), compared with the NC group, probiotics supplementation significantly increased the terpenoid backbone biosynthesis, pyruvate metabolism, glycosyltransferases, glycine, serine and threonine metabolism, D-glutamine and D-glutamate metabolism, cell motility and secretion and benzoate degradation (Supplementary Figure S2A). Subsequently, the significant up of the tyrosine metabolism, two-component system, sulfur metabolism, pentose and glucuronate interconversions, inositol phosphate metabolism, naphthalene degradation, general function prediction only, fructose and mannose metabolism, bacterial motility proteins and bacterial chemotaxis in the PC group at the peak laying period (28w) (Supplementary Figure S2B). However, at the late laying period (58w), probiotics supplementation increased the pyrimidine metabolism, purine metabolism, peptidoglycan biosynthesis, peptidases, nucleotide excision repair, glycolysis/gluconeogenesis, glutathione metabolism, DNA repair and recombination proteins, bacterial secretion system and aminoacyl-tRNA biosynthesis compared to NC group (Supplementary Figure S2C).
The influence of probiotics supplementation on microbiome characteristics at different laying period
BugBase was used to predict the bacterial composition of each group at different laying period. Nine potential phenotypes, including aerobic, anaerobic, contains mobile elements, facultatively anaerobic, forms biofilms, gram negative, gram positive, potentially pathogenic and stress tolerant were significantly predicted in the NC and PC group (p < 0.05), as shown in Supplementary Figure S3 and Supplementary Table S3. Among all the phenotypes, the PC group tended to have more anaerobic, gram-positive, stress tolerant, facultatively anerobic and containing mobile elements bacteria at early laying period (23w) and have more aerobic and gram-negative at peak laying period (27w). Additionally, the proportion of aerobic, facultatively anaerobic, forms biofilms, gram-positive and stress tolerant bacteria were observed to be significantly enriched in the PC group at the late laying period (58w). What is noteworthy is that the sum of potentially pathogenic bacteria was particularly lower in the PC group than in NC group at different laying time during the whole laying cycle (p < 0.01).
Discussion
The reproduction performance of laying hens in the late laying period is directly related to the economic benefit of chicken farm. To our knowledge, this is the first study to add probiotics to the basic diet of Tianfu green shell laying hens in order to study its effects on egg quality, antioxidant capacity, immune function, and intestinal microflora at different laying stages. These results of this study can provide data reference and theoretical support for the application of probiotics in Tianfu green shell laying hens, and they have important implications for improving laying hen production performance at different laying stages, laying hen efficiency, and egg quality.
It is well known that maintaining egg shell quality and internal composition is a top priority for the laying hen’s industry. In the study, our findings showed that adding probiotics to the diet could increase the AH and HU at the peak and late laying period, and increased eggshell thickness and strength at late laying periods, which was similar to the results in laying hens in Mazanko et al. (2019). Also, Wang et al. (2021) reported that Clostridium butyricum can improve eggshell thickness and eggshell strength. Probiotics have been found to promote the growth of beneficial bacteria, and the proliferation of these microorganism ultimately leads to the accumulation of short-chain fatty acid (SCFAs) (Forte et al., 2016). Therefore, the improvement in eggshell quality may be related to the ability of probiotics to improve the serum calcium absorption and retention levels of laying hens, which promotes calcium deposition on shell glands (Attia et al., 2020). Conversely, some studies have found that probiotic supplementation has no effect on eggshell strength or thickness (Upadhaya et al., 2019; Wang et al., 2020). The reason may be related to the age and laying period of laying hens.
Ovary is the foundation of laying hens, and it is also one of the parts that commercial laying hen manufacturers pay special attention to, because changes in or destruction of their pathology affect the number and growth of follicles, which in turn affects egg production and quality, ultimately reducing economic benefits. In the present study, the number and weight of grade follicles, as well as the number of SYFs and LWFs in late laying period were improved by the addition of probiotics. However, little was known about the effects of probiotics on ovaria-related variables at different stages of laying hens for comparison with the current result. However, according to Lei et al. (2013), probiotics (Bacillus licheniformis) may increase serum FSH and E2 content in laying hens, which stimulated the growth and development of follicles. Meanwhile, Zhou et al. (2020) also found that the addition of probiotics (Bacillus amyloliquefaciens) would increase contents of FSH and E2, thus increasing the ovarian weight and promoting the growth and maturation of follicle. Thus, the addition of probiotics may regulate ovarian development by inducing FSH and E2 secretion, improving reproductive ability and follicles quality.
Oxidative stress occurs when there is a loss of balance between the antioxidant system and reactive oxygen species (ROS) as an output, which impairs reproductive performance and leads to tissue damage (Surai et al., 2019; Pisoschi et al., 2021). Therefore, antioxidant stress has become one of the important factors affecting the performance and egg quality of laying hens. There are pieces of evidence that probiotics could effectively mask the adverse effects of oxidative stress, and promote the activity of antioxidant enzymes (Deng et al., 2012). For instance, Zhan et al. (2019) investigated that effect of Clostridium butyricum on laying hens and found that the concentrations of GSH-Px, CAT and T-SOD were significantly increased in serum. Aluwong et al. (2013) showed that addition of yeast probiotics could significantly improve the GSH-Px activity of broilers. Also, Liao et al. (2015) revealed that dietary Clostridium butyricum supplementation could increase the activity of antioxidant enzymes and the concentration of major non-enzymatic antioxidant GSH in intestinal mucosa of broilers, while reducing the concentration of MDA. However, to our study, information is lacking on the effects of probiotics on Tianfu green shell laying hens’ antioxidation. Our present findings revealed that probiotics significantly increased serum T-AOC and SOD concentrations while decreasing the MDA content throughout the laying cycle, particularly in the late laying period. Overall, these findings suggest that complex probiotics could reduce oxidative stress in laying hens by stimulating enzyme components, thereby increasing laying rate and egg quality. It is suggested that these probiotics with antioxidant effect can be used as probiotic antioxidants in laying hen production, but the mechanism needs to be further investigated.
Furthermore, some studies have shown that probiotics or their cellular components can improve immune function in animals (Chen et al., 2022). For example, Zhao et al. (2022) revealed that dietary supplementation with the small peptides can reduce the proinflammatory factors including IL-6, IL-8 and IL-12 expression, thereby protecting the body from inflammatory damage. Also, Wang et al. (2021) showed that dietary addition of Clostridium butyricum and butyric acid glycerides decreased IL-6 content in jejunum of yellow-feathered breeder hens, while those of IL-4, IL-6, IL-1β and IgY were decreased by sodium butyrate. The present results showed that the dietary supplementation with probiotics upregulated the ovarian tissue of TLR-4 expression while decreasing the mRNA level of proinflammatory cytokines (IL-1, IL-6 and TNF-α) at three different time points during the laying period, implying that probiotics can regulate the ovarian immune function of laying hen. This is similar with the findings of Amevor et al. (2022) who reported that the dietary combination of quercetin and vitamin E decreased the mRNA levels of 1L-1β and IL-6 and increased the expression of anti-inflammatory cytokines (IL-10) in liver and testis, thus reduced the inflammatory response of the body improved the immune function. These results indicate that dietary probiotics can stimulate the local immune system in ovary of laying hens. The immunomodulatory effect of probiotics on laying hens was evidence in the reduced the expression level of proinflammatory cytokines. Therefore, we believe that the improvement of chicken immune status has a great promotion effect on chicken health, and is conducive to the improvement of egg production performance and egg shell and protein quality.
It is well known that the balance of gut microbiota is important for maintaining physiological and behavioral balance of chickens and is also essential for optimal growth, reproduction, health and welfare (Clavijo and Flórez, 2018). To better elucidate the effect of dietary probiotics on the intestinal microbiota of laying hens at different laying stages, 16S rRNA method is used to evaluate the effects. In this study, there was no significant difference in the diversity and richness of gut microbiota between the probiotic supplementation group and the control group at different laying stages. In line with previous studies, probiotics (Bacillus licheniformis DSM5749) had no significant effect on alpha diversity of cecal microorganisms of laying hens (Pan et al., 2022).
We also observed that at the phylum level, Firmicutes, Bacteroidota and Bacteroidetes accounted for the largest proportion of the total cecal microbial community of laying hens from each group, which was consistent with the results of previous studies (Zhang et al., 2021). During peak laying period, not only Bacteroidota increased, Actinobacteriota, Verrucomicrobiota and Deferribacterota also increased. By late laying period, the relative abundance of Firmicutes, Desulfobacterota and Actinobacteriota increased significantly, while other phyla have decreased. More detail indicated that at the genus level, the abundance of Bacteroides and Prevotellaceae_unclassified increased with the addition of probiotics at the peak laying period. Previous studies have shown that the Bacteroidetes, as a kind of probiotic bacteria, can improve the nutrient utilization and immunity of the host by increasing polysaccharide decomposition (Bai et al., 2013; Lee and Hase, 2014). Furthermore, Prevotella has an important role in the utilization of carbohydrates within the gut microbial ecosystem (Wang et al., 2017). This may explain why laying hens have greater feed intake and optimal performance at peaky laying period. Notably, the relative abundance of some beneficial bacteria such as Faecalibacterium, Eubacterium and Lactobacillus increased with the addition of probiotics at the late laying period. Faecalibacterium has important immune and metabolic functions, which was related to inflammation and possibly obesity (Balamurugan et al., 2010; Quevrain et al., 2016). It has been found that the abundance of Faecalibacterium in cecum and feces of chickens was associated with their health and growth (Singh et al., 2012; Wu et al., 2019). Meanwhile, Foditsch et al. (2015) also found that oral administration of Faecalibacterium to preweaning cows did improve weight gain and reduce diarrhea. Additionally, Eubacterium is also known to plays a critical role in regulating inflammation, immune responses, and maintaining the integrity of the intestinal barrier (Mukherjee et al., 2020). Therefore, we hypothesized that the improvement of ovarian immune function of laying hens by probiotics in the late laying period might be related to the changes of these microbiota levels. The exact mechanism needs to be further determined.
Notably, the bacterial composition predicted by BugBase demonstrated that probiotics supplementation significantly increased the proportion of aerobic, facultatively anaerobic, forms biofilms, gram-positive and stress tolerant bacteria, while decreased the proportion of potentially pathogenic bacteria at the late laying period. Previous studies have shown that biofilm formation is a protective growth pattern that against the threat of antibiotics and harsh environments (Davies, 2003; Hall and Mah, 2017), thus explaining why the probiotic supplementation can enhance the immune function of hens at late laying period. However, these results are based on BugBase predictions and need to be verified experimentally.
Conclusion
In conclusion, dietary supplementation with probiotics was effective in improving egg quality, promoting antioxidant capacity and immune function, and benefiting follicle development and cecal microflora of laying hens at different laying stages, especially at the late laying period (Supplementary Figure S4).
Data availability statement
The original contributions presented in the study are publicly available. This data can be found at: NCBI, PRJNA874899.
Ethics statement
The animal study was reviewed and approved by Institution Animal Care and Use Committee of Sichuan Agricultural University, Sichuan, China. Written informed consent was obtained from the owners for the participation of their animals in this study.
Author contributions
HX and YW conceived and designed this study. YXL, DL, CY, YJ, ZH, ZZ, and RD performed the experiments. HX and YW analyzed the data and wrote the manuscript. YXL, DL, and CY assisted in the 16S rRNA gene sequencing data analysis and the phenotypic data analysis. XZ, YZ, YT, QZ, and YPL collected all samples of this study. XZ, YPL, and YW edited the paper. YW obtained the funding. All authors reviewed and approved the final manuscript.
Funding
This study was supported by the 14th Five-year Plan for Breeding Program in Sichuan (2021YFYZ0007).
Acknowledgments
We appreciate all the workers from the poultry breeding farm of Sichuan Agricultural University for the management of chickens.
Conflict of interest
The authors declare that the research was conducted in the absence of any commercial or financial relationships that could be construed as a potential conflict of interest.
Publisher’s note
All claims expressed in this article are solely those of the authors and do not necessarily represent those of their affiliated organizations, or those of the publisher, the editors and the reviewers. Any product that may be evaluated in this article, or claim that may be made by its manufacturer, is not guaranteed or endorsed by the publisher.
Supplementary material
The Supplementary material for this article can be found online at: https://www.frontiersin.org/articles/10.3389/fmicb.2023.1041072/full#supplementary-material
Footnotes
References
Abdelqader, A., AI-Fataftah, A. R., and Das, G. (2013). Effects of dietary Bacillus subtilis and inulin supplementation on performance, eggshell quality, intestinal morphology and microflaora composition of laying hens in the late phase of production. Anim. Feed Sci. Technol. 179, 103–111. doi: 10.1016/j.anifeedsci.2012.11.003
Aluwong, T., Kawu, M., Raji, M., Dzenda, T., Govwang, F., Sinkalu, V., et al. (2013). Effect of yeast probiotic on growth, antioxidant enzyme activities and malondialdehyde concentration of broiler chickens. Antioxidants 2, 326–339. doi: 10.3390/antiox2040326
Amevor, F. K., Cui, Z., Ning, Z., Shu, G., Du, X., Jin, N., et al. (2022). Dietary quercetin and vitamin E supplementation modulates the reproductive performance and antioxidant capacity of aged male breeder chickens. Poult. Sci. 101:101851. doi: 10.1016/j.psj.2022.101851
Attia, Y. A., AI-Harthi, M. A., and Abo EI-Maaty, H. M. (2020). Calcium and cholecalciferol levels in late-phase laying hens: effects on productive traits, egg quality, blood biochemistry, and immune responses. Front. Vet. Sci. 7:389. doi: 10.3389/fvets.2020.00389
Bai, Y., Wang, R., Yang, Y., Li, R., and Wu, X. (2021). Folic acid absorption characteristics and effect on cecal microbiota of laying hens. Front. Vet. Sci. 8:720851. doi: 10.3389/fvets.2021.720851
Bai, S. P., Wu, A. M., Ding, X. M., Lei, Y., Bai, J., Zhang, K. Y., et al. (2013). Effects of probiotic-supplemented diets on growth performance and intestinal immune characteristics of broiler chickens. Poult. Sci. 92, 663–670. doi: 10.3382/ps.2012-02813
Balamurugan, R., George, G., Kabeerdoss, J., Hepsiba, J., Chandragunasekaran, A. M., and Ramakrishna, B. S. (2010). Quantitative differences in intestinal Faecalibacterium prausnitzii in obese Indian children. Br. J. Nutr. 103, 335–338. doi: 10.1017/S0007114509992182
Bienenstock, J., Kunze, W., and Forsythe, P. (2015). Microbiota and the gut-brain axis. Nutr. Rev. 73, 28–31. doi: 10.1093/nutrit/nuv019
Bindari, Y. R., and Gerber, P. F. (2022). Centennial review: factors affecting the chicken gastrointestinal microbial composition and their association with gut health and productive performance. Poult. Sci. 101:101612. doi: 10.1016/j.psj.2021.101612
Callahan, B. J., McMurdie, P. J., Rosen, M. J., Han, A. W., Johnson, A. J. A., and Holmes, S. P. (2016). DADA2: high-resolution sample inference from Illumina amplicon data. Nat. Methods 13, 581–583. doi: 10.1038/nmeth.3869
Chen, X., Chen, W., Ci, W., Zheng, Y., Han, X., Huang, J., et al. (2022). Effects of dietary supplementation with Lactobacillus acidophilus and Bacillus subtilis on mucosal immunity and intestinal barrier are associated with its modulation of gut metabolites and microbiota in late-phase laying hens. Probiotics Aantimicrob. Proteins 14, 1–13. doi: 10.1007/s12602-022-09923-7
Chen, S., Zhou, Y., Chen, Y., and Gu, J. (2018). Fastp: a ultra-fast all-in-one FASTQ preprocessor. Bioinformatics 34, i884–i890. doi: 10.1093/bioinformatics/bty560
Clavijo, V., and Flórez, M. J. V. (2018). The gastrointestinal microbiome and its association with the control of pathogens in broiler chicken production: a review. Poult. Sci. 97, 1006–1021. doi: 10.3382/ps/pex359
Davies, D. (2003). Understanding biofilm resistance to antibacterial agents. Nat. Rev. Drug Discov. 2, 114–122. doi: 10.1038/nrd1008
Deng, W., Dong, X. F., Tong, J. M., and Zhang, Q. (2012). The probiotic bacillus licheniformis ameliorates heat stress-induced impairment of egg production, gut morphology, and intestinal mucosal immunity in laying hens. Poult. Sci. 91, 575–582. doi: 10.3382/ps.2010-01293
Ding, J. M., Dai, R. H., Yang, L. Y., He, C., Xu, K., Liu, S. Y., et al. (2017). Inheritance and establishment of gut microbiota in chickens. Front. Microbiol. 8:11. doi: 10.3389/fmicb.2017.01967
Eeckhaut, V., Van Immerseel, F., Croubels, S., De Baere, S., Haesebrouck, F., Ducatelle, R., et al. (2011). Butyrate production in phylogenetically diverse Firmicutes isolated from the chicken caecum. Microbial. Biotechnol. 4, 503–512. doi: 10.1111/j.1751-7915.2010.00244.x
Fijian, S. (2014). Microorganisms with claimed probiotic properties: an overview of recent literature. Int. J. Environ. Res. Public Health 11, 4745–4767. doi: 10.3390/ijerph110504745
Foditsch, C., Pereira, R. V., Ganda, E. K., Gomez, M. S., Marques, E. C., Santin, T., et al. (2015). Oral administration of Faecalibacterium prausnitzii decreased the incidence of severe diarrhea and related mortality rate and increased weight gain in preweaned dairy heifers. PLoS One 10:e0145485. doi: 10.1371/journal.pone.0145485
Forte, C., Moscati, L., Acuti, G., Mugnai, C., Franciosini, M., Costarelli, S., et al. (2016). Effects of dietary Lactobacillus acidophilus and Bacillus subtilis on laying performance, egg quality, blood biochemistry and immune response of organic laying hens. J. Anim. Phys. Anim. Nutr. 100, 977–987. doi: 10.1111/jpn.12408
Gaggia, F., Mattarelli, P., and Biavati, B. (2010). Probiotics and prebiotics in animal feeding for safe food production. Int. J. Food Microbiol. 141, S15–S28. doi: 10.1016/j.ijfoodmicro.2010.02.031
Gerritsen, J., Smidt, H., Rijkers, G. T., and de Vos, W. M. (2011). Intestinal microbiota in human health and disease: the impact of probiotics. Genes Nutr. 6, 209–240. doi: 10.1007/s12263-011-0229-7
Guo, Y., Zhao, Z. H., Pan, Z. Y., An, L. L., Balasubramanian, B., and Liu, W. C. (2020). New insights into the role of dietary marine-derived polysaccharides on productive performance, egg quality, antioxidant capacity, and jejunal morphology in late-phase laying hens. Poult. Sci. 99, 2100–2107. doi: 10.1016/j.psj.2019.12.032
Hall, C. W., and Mah, T. F. (2017). Molecular mechanisms of biofilm-based antibiotic resistance and tolerance in pathogenic bacteria. FEMS Microbiol. Rev. 41, 276–301. doi: 10.1093/femsre/fux010
Jiang, S., Hu, J. Y., and Cheng, H. W. (2022). The impact of probiotic Bacillus subtilis on injurious behavior in laying hens. Animals 12:870. doi: 10.3390/ani12070870
Johson, A. L. (2015). Ovarian follicle selection and granulosa cell differentiation. Poult. Sci. 94, 781–785. doi: 10.3382/ps/peu008
Khan, S., Moore, R. J., Stanley, D., and Chouslkar, K. K. (2020). The gut microbiota of laying hens and its manipulation with prebiotics and probiotics to enhance gut health and food safety. Appl. Environ. Microbiol. 86, e00600–e00620. doi: 10.1128/AEM.00600-20
Lee, W. J., and Hase, K. (2014). Gut microiota-generated metabolites in animal health and disease. Nat. Chem. Biol. 10, 416–424. doi: 10.1038/nchembio.1535
Lee, K. W., Kim, D. K., Lillehoj, H. S., Jang, S. I., and Lee, S. H. (2015). Immune modulation by Bacillus subtilis-based direct-fed microbials in commercial broiler chickens. Anim. Feed Sci. Technol. 200, 76–85. doi: 10.1016/j.anifeedsci.2014.12.006
Lei, K., Li, Y. L., Yu, D. Y., Rajput, I. R., and Li, W. F. (2013). Influence of dietary inclusion of Bacillus licheniformis on laying performance, egg quality, antioxidant enzyme activities, and intestinal barrier function of laying hens. Poult. Sci. 92, 2389–2395. doi: 10.3382/ps.2012-02686
Liao, X., Wu, R., Ma, G., Zhao, L., Zheng, Z., and Zhang, R. (2015). Effects of Clostridium butyricum on antioxidant properties, meat quality and fatty acid composition of broiler birds. Lipids Health Dis. 14:36. doi: 10.1186/s12944-015-0035-0
Liu, Y., Li, Y., Liu, H. N., Suo, Y. L., Hu, L. L., Peng, X. A., et al. (2013). Effect of quercetin on performance and egg quality during the late laying period of hens. Br. Poult. Sci. 54, 510–514. doi: 10.1080/00071668.2013.799758
Liu, W., Yuan, Y., Sun, C., Balasubramanian, B., Zhao, Z., and An, L. (2019). Effects of dietary betaine on growth performance, digestive function, carcass traits, and meat quality in indigenous yellow-feathered broilers under long-term heat stress. Animals 9:506. doi: 10.3390/ani9080506
Lv, Z. P., Yan, S. J., Li, G., Liu, D., and Guo, Y. M. (2019). Genistein improves the reproductive performance and bone status of breeder hens during the late egg-laying period. Poult. Sci. 98, 7022–7029. doi: 10.3382/ps/pez367
Macit, M., Karaoglu, M., Celebi, S., Esenbuga, N., Yoruk, M. A., and Kaya, A. (2021). Effects of supplementation of dietary humate, probiotic, and their combination on performance, egg quality, and yolk fatty acid composition of laying hens. Trop. Anim. Health Prod. 53:63. doi: 10.1007/s11250-020-02546-6
Magoc, T., and Salzberg, S. L. (2011). FLASH: fast length adjustment of short reads to improve genome assemblies. Bioinformatics 27, 2957–2963. doi: 10.1093/bioinformatics/btr507
Mazanko, M. S., Makarenko, M. S., Chistyakov, V. A., Usatov, A. V., Prazdnova, E. V., Bren, A. B., et al. (2019). Probiotic intake increases the expression of vitellogenin genes in laying hens. Probiotics Antimicrob. Proteins 11, 1324–1329. doi: 10.1007/s12602-019-9519-y
Mead, G. C. (2000). Prospects for competitive exclusion treatment to control salmonellas and other foodborne pathogens in poultry. Vet. J. 159, 111–123. doi: 10.1053/tvjl.1999.0423
Mikulski, D., Jankowski, J., Naczmanski, J., Mikulska, M., and Demey, V. (2012). Effects of dietary probiotic (Pediococcus acidilactici) supplementation on performance, nutrient digestibility, egg traits, egg yolk cholesterol, and fatty acid profile in laying hens. Poult. Sci. 91, 2691–2700. doi: 10.3382/ps.2012-02370
Mountzouris, K. C., Tsitrsikos, P., Palamidi, I., Arvaniti, A., Mohnl, M., Schatzmayr, G., et al. (2010). Effects of probiotic inclusion levels in broiler nutrition on growth performance, nutrient digestibility, plasma immunoglobulins, and cecal microflora composition. Poult. Sci. 89, 58–67. doi: 10.3382/ps.2009-00308
Mukherjee, A., Lordan, C., Paul Ross, R., and Cotter, P. D. (2020). Gut microbes from the phylogenetically diverse genus Eubacterium and their various contributions to gut health. Gut Microbes 12:1802866. doi: 10.1080/19490976.2020.1802866
Muñoz, M., Mosquera, A., Alméciga-Díaz, C. J., Melendez, A. P., and Sánchez, O. F. (2012). Fructooligosaccharides metabolism and effect on bacteriocin production in Lactobacillus strains isolated from ensiled corn and molasses. Anaerobe 18, 321–330. doi: 10.1016/j.anaerobe.2012.01.007
Murugesan, G. R., Gabler, N. K., and Persia, M. E. (2014). Effects of direct-fed microbial supplementation on broiler performance, intestinal nutrient transport and integrity under experimental conditions with increased microbial challenge. Br. Poult. Sci. 55, 89–97. doi: 10.1080/00071668.2013.865834
National Research Council (1994). Nutrient Requirements of Poultry: Ninth Revised Edition. Washington DC: The National Academies Press.
Neijat, M., Shirley, R. B., Barton, J., Thiery, P., Welsher, A., and Kiarie, E. (2019). Effect of dietary supplementation of bacillus subilis DSM29784 on hen performance, egg quality indices, and apparent retention of dietary components in laying hens from 19 to 48 weeks of age. Poult. Sci. 98, 5622–5635. doi: 10.3382/ps/pez324
O’Mahony, S. M., Clarke, G., Borre, Y. E., Dinan, T. G., and Cryan, J. F. (2015). Serotonin, tryptophan metabolism and the brain-gut-microbiome axis. Behav. Brain Res. 277, 32–48. doi: 10.1016/j.bbr.2014.07.027
Pan, X., Cai, Y., Kong, L., Xiao, C., Zhu, Q., and Song, Z. (2022). Probiotic effects of Bacillus licheniformis DSM5749 on growth performance an intestinal microecological balance of laying hens. Front. Nutr. 9:868093. doi: 10.3389/fnut.2022.868093
Park, J. W., Jeong, J. S., Lee, S. I., and Kim, I. H. (2016). Effect of dietary supplementation with a probiotic (Enterococcus faecium) on production performance, excreta microflaora, ammonia emission, and nutrient utilization in ISA brown laying hens. Poult. Sci. 95, 2829–2835. doi: 10.3382/ps/pew241
Parks, D. H., Tyson, G. W., Hugenholtz, P., and Beiko, R. G. (2014). STAMP: statistical analysis of taxonomic and functional profiles. Bioinformatics 30, 3123–3124. doi: 10.1093/bioinformatics/btu494
Pisoschi, A. M., Pop, A., Iordache, F., Stanca, L., Predoi, G., and Serban, A. I. (2021). Oxidative stress mitigation by antioxidants-an overview on their chemistry and influences on health status. Eur. J. Med. Chem. 209:112891. doi: 10.1016/j.ejmech.2020.112891
Pourabedin, M., Xu, Z., Baurhoo, B., Chevaux, E., and Zhao, X. (2014). Effects of mannan oligosaccharide and virginiamycin on the cecal microbial community and intestinal morphology of chickens raised under suboptimal conditions. Can. J. Microbiol. 60, 255–266. doi: 10.1139/cjm-2013-0899
Pourabedin, M., and Zhao, X. (2015). Prebiotics and gut microbiota in chickens. FEMS Microbiol. Lett. 362:fnv122. doi: 10.1093/femsle/fnv122
Qu, X. Y., Chen, J. F., He, C. Q., Chi, F., and Johnston, S. L. (2018). Effects of modified montmorillonite adsorbent on performance, egg quality, serum biochemistry, oxidation status, and immune response of laying hens in late production. Livest. Sci. 210, 15–20. doi: 10.1016/j.livsci.2018.01.021
Quevrain, E., Maubert, M. A., Michon, C., Chain, F., Marquant, R., and Tailhades, J. (2016). Identification of an anti-inflammatory protein from Faecalibacerium prausnitzii, a commensal bacterium deficient in Crohn’s disease. Gut 65, 415–425. doi: 10.1136/gutjnl-2014-307649
Singh, K. M., Shah, T., Deshpande, S., Jakhesara, S. J., Koringa, P. G., Rank, D. N., et al. (2012). High through put 16S rRNA gene-based pyrosequencing analysis of the fecal microbiota of high FCR and low FCR broiler growers. Mol. Biol. Rep. 39, 10595–10602. doi: 10.1007/s11033-012-1947-7
Sjofjan, O., Adli, D. N., Harahap, R. P., Jayanegara, A., Utama, D. T., and Seruni, A. P. (2021). The effects of lactic acid bacteria and yeasts as probiotics on the growth performance, relative organ weight, blood parameters, and immune responses of broiler: a meta-analysis. F1000Res 10:183. doi: 10.12688/f1000research.51219.3
Smith, J. M. (2014). A review of avian probiotics. J. Avian Med. Surg. 28, 87–94. doi: 10.1647/2012-031
Surai, P. F., Kochish, I. I., Fisinin, V. I., and Kidd, M. T. (2019). Antioxidant defense systems and oxidative stress in poultry biology: an update. Antioxidants 8:235. doi: 10.3390/antiox8070235
Upadhaya, S. D., Rudeaux, F., and Kim, I. H. (2019). Efficacy of dietary Bacillus subtilis and bacillus licheniformis supplementation continuously in pullet and lay period on egg production, excreta microflora, and egg quality of Hyline-Brown birds. Poult. Sci. 98, 4722–4728. doi: 10.3382/ps/pez184
Villageliu, D. N., and Lyte, M. (2017). Microbial endocrinology: why the intersection of microbiology and neurobiology matters to poultry health. Poult. Sci. 96, 2501–2508. doi: 10.3382/ps/pex148
Waititu, S. M., Yitbarek, A., Matini, E., Echeverrry, H., Kiarie, E., Rodriguez-Lecompte, J. C., et al. (2014). Effect of supplementing direct-fed microbials on broiler performance, nutrient digestibilities, and immune responses. Poult. Sci. 93, 625–635. doi: 10.3382/ps.2013-03575
Wang, Y., Sun, J., Zhong, H., Li, N., Xu, H., Zhu, Q., et al. (2017). Effect of probiotics on meat flavor and gut microbiota of chicken. Sci. Rep. 7:6400. doi: 10.1038/s41598-017-06677-z
Wang, Y., Wang, Y., Lin, X., Gou, Z., Fan, Q., and Jiang, S. (2021). Effects of Clostridium butyricum, sodium butyrate, and butyric acid glycerides on the reproductive performance, egg quality, intestinal health, and offspring performance of yellow-feathered breeder hens. Front. Microbiol. 12:657542. doi: 10.3389/fmicb.2021.657542
Wang, W. W., Wang, J., Zhang, H. J., Wu, S. G., and Qi, G. H. (2020). Effects of Clostridium butyricum on production performance and intestinal absorption function of laying hens in the late phase of production. Anim. Feed Sci. Technol. 264:114476. doi: 10.1016/j.anifeedsci.2020.114476
Wang, Y., Xu, L., Sun, X., Wan, X., Sun, G., Jiang, R., et al. (2020). Characteristics of the fecal microbiota of high- and low-yield hens and effects of fecal microbiota transplantation on egg production performance. Res. Vet. Sci. 129, 164–173. doi: 10.1016/j.rvsc.2020.01.020
Wu, Y., Wang, B., Zeng, Z., Liu, R., Tang, L., Gong, L., et al. (2019). Effects of probiotics Lactobacillus plantarum 16 and Paenibacillus polymyxa 10 on intestinal barrier function, antioxidative capacity, apoptosis, immune response, and biochemical parameters in broilers. Poult. Sci. 98, 5028–5039. doi: 10.3382/ps/pez226
Yan, F. F., Murugesan, G. R., and Cheng, H. W. (2019). Effects of probiotic supplementation on performance traits, bone mineralization, cecal microbial composition, cytokines and corticosterone in laying hens. Animal 13, 33–41. doi: 10.1017/S175173111800109X
Yeo, J., and Kim, K. I. (1997). Effect of feeding diets containing an antibiotic, a probiotic, or yucca extract on growth and intestinal urease activity in broiler chicks. Poult. Sci. 76, 381–385. doi: 10.1093/ps/76.2.381
Yitbarek, A., Echeverry, H., Brady, J., Hernandez-Doria, J., Camelo-Jaimes, G., Sharif, S., et al. (2012). Innate immune response to yeast-derived carbohydrates in broiler chickens fed organic diets and challenged with Clostridium perfringens. Poult. Sci. 91, 1105–1112. doi: 10.3382/ps.2011-02109
Zhan, H. Q., Dong, X. Y., Li, L. L., Zheng, Y. X., Gong, Y. J., and Zou, X. T. (2019). Effects of dietary supplementation with Clostridium butyricum on laying performance, egg quality, serum parameters, and cecal microflora of laying hens in the late phase of production. Poult. Sci. 98, 896–903. doi: 10.3382/ps/pey436
Zhang, Y., Ma, W., Zhang, Z., Liu, F., Wang, J., Yin, Y., et al. (2019). Effects of enterococcus faecalis on egg production, egg quality and caecal microbiota of hens during the late laying period. Arch. Anim. Nutr. 73, 208–221. doi: 10.1080/1745039X.2019.1591128
Zhang, G., Wang, H., Zhang, J., Tang, X., Raheem, A., and Wang, M. (2021). Modulatory effects of Bacillus subtilis on the performance, morphology, cecal microbiota and gut barrier function of laying hens. Animals 11:1523. doi: 10.3390/ani11061523
Zhao, X., Zhang, Y., He, W., Wei, Y., Han, S., Xia, L., et al. (2022). Effects of small peptide supplementation on growth performance, intestinal barrier of laying hens during the brooding and growing periods. Front. Immunol. 13:925256. doi: 10.3389/fimmu.2022.925256
Keywords: probiotics, laying hen, egg quality, antioxidant capacity, ovarian, gut microbiota
Citation: Xu H, Lu Y, Li D, Yan C, Jiang Y, Hu Z, Zhang Z, Du R, Zhao X, Zhang Y, Tian Y, Zhu Q, Liu Y and Wang Y (2023) Probiotic mediated intestinal microbiota and improved performance, egg quality and ovarian immune function of laying hens at different laying stage. Front. Microbiol. 14:1041072. doi: 10.3389/fmicb.2023.1041072
Edited by:
Weiwei Wang, South China Agricultural University, ChinaReviewed by:
Wu Shugeng, Feed Research Institute (CAAS), ChinaDoaa Ibrahim, Zagazig University, Egypt
Copyright © 2023 Xu, Lu, Li, Yan, Jiang, Hu, Zhang, Du, Zhao, Zhang, Tian, Zhu, Liu and Wang. This is an open-access article distributed under the terms of the Creative Commons Attribution License (CC BY). The use, distribution or reproduction in other forums is permitted, provided the original author(s) and the copyright owner(s) are credited and that the original publication in this journal is cited, in accordance with accepted academic practice. No use, distribution or reproduction is permitted which does not comply with these terms.
*Correspondence: Yan Wang, as519723614@163.com
†These authors have contributed equally to this work