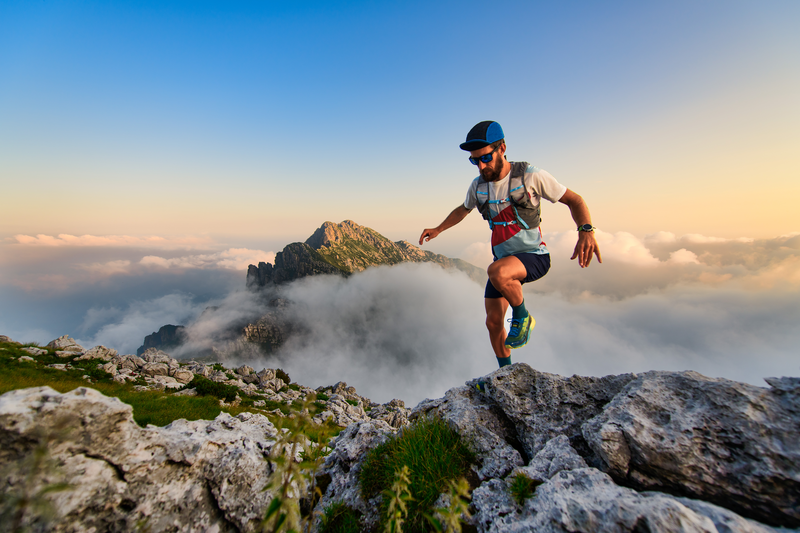
94% of researchers rate our articles as excellent or good
Learn more about the work of our research integrity team to safeguard the quality of each article we publish.
Find out more
REVIEW article
Front. Microbiol. , 16 February 2023
Sec. Microbiotechnology
Volume 14 - 2023 | https://doi.org/10.3389/fmicb.2023.1040901
This article is part of the Research Topic Bioinoculants with Nano-compounds to Improve Soil Health: A Step Toward Sustainable Agriculture View all 12 articles
A correction has been applied to this article in:
Corrigendum: Biopesticides as an alternative to synthetic pesticides: a case for nanopesticides, phytopesticides and microbial pesticides
Over the years, synthetic pesticides like herbicides, algicides, miticides, bactericides, fumigants, termiticides, repellents, insecticides, molluscicides, nematicides, and pheromones have been used to improve crop yield. When pesticides are used, the over-application and excess discharge into water bodies during rainfall often lead to death of fish and other aquatic life. Even when the fishes still live, their consumption by humans may lead to the biomagnification of chemicals in the body system and can cause deadly diseases, such as cancer, kidney diseases, diabetes, liver dysfunction, eczema, neurological destruction, cardiovascular diseases, and so on. Equally, synthetic pesticides harm the soil texture, soil microbes, animals, and plants. The dangers associated with the use of synthetic pesticides have necessitated the need for alternative use of organic pesticides (biopesticides), which are cheaper, environment friendly, and sustainable. Biopesticides can be sourced from microbes (e.g., metabolites), plants (e.g., from their exudates, essential oil, and extracts from bark, root, and leaves), and nanoparticles of biological origin (e.g., silver and gold nanoparticles). Unlike synthetic pesticides, microbial pesticides are specific in action, can be easily sourced without the need for expensive chemicals, and are environmentally sustainable without residual effects. Phytopesticides have myriad of phytochemical compounds that make them exhibit various mechanisms of action, likewise, they are not associated with the release of greenhouse gases and are of lesser risks to human health compared to the available synthetic pesticides. Nanobiopesticides have higher pesticidal activity, targeted or controlled release with top-notch biocompatibility and biodegradability. In this review, we examined the different types of pesticides, the merits, and demerits of synthetic pesticides and biopesticides, but more importantly, we x-rayed appropriate and sustainable approaches to improve the acceptability and commercial usage of microbial pesticides, phytopesticides, and nanobiopesticides for plant nutrition, crop protection/yield, animal/human health promotion, and their possible incorporation into the integrated pest management system.
From antiquity, the use of synthetic (chemical) pesticides to control crop pests for improved crop production is known (Anani et al., 2020). Synthetic pesticides are made from chemicals and carriers, such as polymers (Rakhimol et al., 2020), which are specific for different pests. They range from those employed in the control of weeds (herbicides), algae (algicides), fungi (fungicide), mites or ticks (miticides/acaricides), bacteria (bactericides), rodents (rodenticide), termites (termiticides), insects (insecticides), molluscs (molluscicides), and nematodes (nematicides), which form the basis of their classification (Anakwue, 2019). Another mode of pesticide classification can be based on their active ingredients, which include organochlorines, dichlorvos, diazinon, diamide, chlorpyrifos, etc. Although synthetic pesticides have positive effects on crop yield and productivity, they also have some negative impacts on soil biodiversity, animals, aquatic life, and humans (Farooq et al., 2019). Synthetic pesticides usually render the soil brittle, reduce soil respiration, and lessen the activities of some macroorganisms in the soil, such as earthworms (Pertile et al., 2020; Pelosi et al., 2021). They also reduce the characteristics of animal offspring, animal immunity to diseases, vitality, and the success of mating in animals (Syromyatnikov et al., 2020). They negatively affect soil microorganisms by limiting their biological services in the production of certain plant growth-promoting traits, such as siderophores, nitrogen, indole-3-acetic, etc. (Kumar and Kumar, 2019). When synthetic pesticides get into the environment through different means, such as vapormovements, indiscriminate disposal, droplet drift, erosion, and leaching, some non-targeted plants are encountered, thus resulting in a decline in the plant’s photosynthetic ability and seed production (Hashimi et al., 2020). The intrusion of pesticides into the water bodies during runoff can lead to the death of aquatic life and water pollution. Also, the accumulation of pesticides in the water bodies can be transitional from the aquatic lives to the animals and humans, and their biomagnification can result in deadly diseases, such as cancer, kidney diseases, skin rashes, diabetes, etc. (Jayaraj et al., 2017; Sabarwal et al., 2018; Manfo et al., 2020). However, biopesticides have emerged and have been very useful in the control of pests with lot of merits.
Biopesticides are cheap, environment-friendly, specific in their mode of action, sustainable, do not leave residues, and are not associated with the release of greenhouse gases (Borges et al., 2021). These biopesticides can be in the form of phytopesticides (plant origin; Idris et al., 2022), microbial pesticides (microbial origin; Harish et al., 2021), and nanobiopesticides (nanoparticles produced from biological agents; Abdollahdokht et al., 2022; Pan et al., 2023). Unlike synthetic pesticides, microbial pesticides are specific in action, can be easily sourced without the need for expensive chemicals, and are environmentally sustainable without residual effects (Harish et al., 2021; Hummadi et al., 2021). Phytopesticides have myriad of phytochemical compounds that make them exhibit various mechanisms of action, likewise, they are not associated with the release of greenhouse gases and are of lesser risks to human health compared to the available synthetic pesticides (Malahlela et al., 2021; Idris et al., 2022). Nanobiopesticides have higher pesticidal activity, targeted or controlled release with top-notch biocompatibility, and biodegradability compared to the synthetic pesticides (Abdollahdokht et al., 2022; Pan et al., 2023). Biopesticides act through different mechanisms, which include the inhibition and destruction of the plasma membrane and protein translation of pathogens/pests. Although, a few drawbacks have reduced their acceptability and commercial utilization, yet, biopesticides are highly specific in their target, have a short shelf life, are less persistent in the soil environment, and originate from sustainable raw materials, unlike synthetic pesticides (Kumar et al., 2021). Some of the merits of biopesticides mentioned above could also serve as their demerits. For example, the specificity in their target toward pest could be a demerit if the desire is to control many pests simultaneously. Also, their short shelf life means they are easily degradable and persist less in the environment, but this turns to a demerit if the goal is to completely eliminate the existing pests and prevent the growth of the pests that will come after the application of the biopesticides. The critical assessment of these merits and demerits, and the possible measures to improve on these seeming drawbacks has become very important. Therefore, this review examined the types, effects, advantages, and disadvantages of both synthetic and biopesticides. Also, different measures to improve on biopesticides (that is, microbial pesticides, phytopesticides, and nanobiopesticides) for possible incorporation into the integrated pest management system to reduce yield and quality loss were adequately discussed.
Pesticides can be classified based on their active ingredients, functions, and sources. According to their active ingredients, pesticides are classified into organochlorines, propanil, and so on (Table 1). In terms of their functions, they can be classified into herbicides, fungicides, algicides, rodenticides, and so on (Figure 1). However, according to their sources, pesticides are classified into synthetic pesticides and biopesticides. Many pesticides, which include carbofuran, carbendazim, dichlorvos, anthraquinone, dinocap, paraquat, methomyl, aldicarb, and diuron have been banned for use in a lot of countries due to their toxicity to humans (Boucaud-Maitre et al., 2019). These banned pesticides are usually preferred by farmers because they are more affordable and more available compared to unbanned pesticides (Kehinde and Tijani, 2021). It is therefore important for World Health Organization (WHO), Food and Agriculture Organization (FAO), and other regulatory bodies to impose a ban on such products worldwide and also sanction companies that produce them.
Pesticides can be classified according to the functions they perform. For instance, algicides (acaricides) destroy algae on different surfaces (Zheng et al., 2018), and antifeedants prevent the destruction of plants or harvested and preserved crops by other pests which could feed on them (Peprah-Yamoah et al., 2022). Herbicides prevent the growth of weeds and eliminate them (Loddo et al., 2019), while miticides are used to kill termites or ticks that destroy crops (Murcia-Morales et al., 2021). Similarly, bactericides and fungicides get rid of harmful bacteria and fungi, respectively, or inhibit their growth without tampering with the beneficial ones (Ullah and Dijkstra, 2019; Akanmu et al., 2021). Fumigants exhibit broad-spectrum activity against fungi, insects, and bacteria (Fang et al., 2020). Termiticides suppress the activities of termites on the soil (Singh et al., 2020). Repellents are used to repel insect pests and birds. Acaricides are used to control arachnids (Benelli, 2022) and insecticides help to destroy insects that affect plants, animals, and humans (Matsuda et al., 2020). Equally, the effective use of nematicides in the control of nematodes, rodenticides in the control of mice and other rodents, and molluscicides in the control of molluscs have been documented (Horgan and Kudavidanage, 2020; Figure 1). Attractants lure and attract pests to a trap or bait (Souto et al., 2021). Insect growth regulators disrupt the molting, maturity from pupal stage to adult, or other life processes of insects (Jindra and Bittova, 2020).
Pesticides are classified into chemical and biological pesticides according to their source. Chemical pesticides are very effective and rapid in the control of pests. They are made from inorganic or synthetic salts, such as sulfur, copper sulfate, lime, and ferrous sulfate. Their chemical compositions are simple and highly soluble in water, which makes them easily absorbable by pests, thereby enhancing their activity and durability in the environment (Kim et al., 2017; Abubakar et al., 2020).
Biological pesticides (biopesticides) are substances produced from biological agents that manage pests in agriculture to enhance crop production (Samada and Tambunan, 2020). They can be sourced from microorganisms, plants, or nanoparticles (Kidd et al., 2017; Samada and Tambunan, 2020; Adeleke et al., 2022d). Microbes release certain metabolites, which protect plants from pests and are useful as microbial pesticides (Samada and Tambunan, 2020). Active compounds from plants used as phytopesticides include phenols, alkaloids, and terpenes (Abubakar et al., 2020). Generally, nanoparticles can be produced from chemical or biological agents (mainly plants or microbes; Omole et al., 2018). Nanoparticles of biological origins that are used as pesticides are termed nanobiopesticides and are also very important as plant protectants (Pan et al., 2023). Nanobiopesticides have found application as pesticidal agents in agriculture because of their excellent physicochemical characteristics like size, reactivity, surface area, and so on. Besides, nanobiopesticides have unambiguous biological interactions with plants, as well as clear transport and fate in the environment (Bratovcic et al., 2021; Kumar et al., 2022; Pan et al., 2023).
Synthetic pesticides are faced with drawbacks, which include the cost of purchase and production, persistence in the soil, pest resistance, impacts on health and the environment, economic harm to organic producers due to pesticide drift, disposal of contaminated crops, removal of stockpiles of unused pesticides as well as regular containers, and the disposal of expired/unused products, which can affect organic farms or innocent populace (Hicks et al., 2018; Essiedu et al., 2020).
A large portion of pesticides when applied on the soil for agricultural purposes remains non-degradable. Hence, they are more persistent in the environment and leach to underground and surface water, thus leading to loss of biodiversity and pollution. Of all the pesticides applied on the soil, about 98% affect organisms that are not targeted. For instance, in Europe, pesticides decrease soil respiration by 35%, reduce insect biomass by 70%, and decrease the number of farm birds by 50%; and in America and Europe, it reduces the honeybee population by 30% (Ali et al., 2021). Research by Tongo et al. (2022) revealed Aldrin pesticide as a major pesticide detected in the Ikpoba river in the Southern part of Nigeria. Although, other chemicals, such as diazinon, endrin, glyphosate, aldrin, endosulfan I, heptachlor, heptachlor epoxide, and carbofuran present with the tendencies of being biomagnified need proper monitoring. Furthermore, pesticides (e.g., carbamate and organophosphate) have been reported to negatively affect soil’s nutrients, as they chelate some important metal ions, thus making them unavailable for plant uptake (Kaur et al., 2017). Likewise, plant photosynthesis, reproduction, and seed production can be adversely affected by pesticides (Hashimi et al., 2020).
Residues of pesticides in food crops can be consumed directly by humans or used in the production of animal feeds (Choudhary et al., 2018). This can come to play when pesticides are applied toward harvesting (Jallow et al., 2017). Biomagnification of pesticides occurs in animals when they feed on accidentally or deliberately contaminated harvested food crops or forage. Topical pesticides are applied on food crops to control parasites, and through other means, such as disposal, spraying, and formulations of pesticides (Choudhary et al., 2018). Pesticide accumulation in the granular tissues of animals can lead to the death of cells, necrosis (causing a reduced hormone production), ovarian follicles (resulting in a reduced progesterone level), reduced oestrogen production, reduced libido, and a reduced sperm concentration and quality in male animals (Li et al., 2022).
Accumulation of pesticides in birds (e.g., bald eagles, ospreys, grebes, cormorants, seagulls, pelicans, and peregrine falcons) living in pesticide-polluted areas can lead to reproduction problems (Garces et al., 2020). Pesticides lead to crossed bill deformity in birds. For example, a high concentration of DDT pesticides led to crossed-bill deformity in a wild bald eagle (Garces et al., 2020). In reptiles inhabiting areas close to rivers where water from agricultural farms is washed, deformities could be observed. For example, snapping turtles living in Erie and Lake Ontario in Canada were found to have deformities, such as deformed jaws, limbs, cranium, carapaces, nostrils, and tails, enlarged yolk sacs, dwarfism, missing eyes, unhatched eggs, and these were traced to chemical pesticides contamination (Garces et al., 2020). In soils, the reduction in the function and population of fungi, actinomycetes, and bacteria has been linked to the usage of three pesticides, namely glyphosate, malathion, and alphacypermethrin (Kumar et al., 2019). All the negative effects of synthetic pesticides lead to the loss of biodiversity and genetic conservation in animals. Furthermore, it also alters soil biodiversity and health, by affecting the microbial functions in the soil, which directly or indirectly enhances soil nutrients and plant health.
Consumption of vegetables, food crops, fruits, milk, and meat from animals that contain high pesticide residue can lead to different diseases in humans (Omoyajowo et al., 2018; Li et al., 2022). Onwujiogu et al. (2022) found pesticides in Bambara groundnut quantity, which is beyond the Maximum Residual Limit (MRL) recommended by the WHO and could pose a threat to the health of humans, especially children who feed on them. Omoyajowo et al. (2018) also experimented to unravel the level of pesticides in three fruits and realized that the pesticide level of watermelon was above the MRL level specified by the WHO/FAO, which equally poses a health threat to the consumers. Similarly, pesticides are used to protect harvested food crops, vegetables, and fruits and those used for other purposes aside that which they are manufactured. For instance, the use of calcium carbide to ripen fruits poses health threats to humans. Calcium carbide contains calcium arsenide and calcium phosphide, and when reacts with water, forms arsine and phosphide, thus leading to headache, vomiting, dizziness, nausea, unconsciousness, and fatigue in humans (Andrew et al., 2018). Equally, ethepon, a pesticide used to hasten the ripening of fruits, vegetables, and cereals exhibited hepatocyte properties when tested on albino rats (Bhadoria et al., 2018).
Furthermore, in humans, biomagnification of pesticides through food (such as fish), drinking water, skin pores (while spraying), post-harvest crop preservation, and inhalation, give rise to diseases, such as cancer, Parkinson’s diseases, eye irritation, diabetes, kidney diseases, hypertension, skin rashes, liver dysfunction, eczema, birth defects, Alzheimer’s diseases, neurological destruction, cardiovascular diseases, and endocrine disorder (Damalas and Koutroubas, 2016; Jayaraj et al., 2017; Sabarwal et al., 2018; Manfo et al., 2020). Likewise, high pesticides level can lead to about 25–30% increase in mental ailments, and a 50% increase in severe brain cancer, leukaemia, lymphoma, and cancer.
Due to the drawbacks of synthetic pesticides, an alternative means of pest control is being encouraged, which is the use of biopesticides (Ojuederie et al., 2021; Ayilara et al., 2022b; Figure 2). Biopesticides are effective and safer means of controlling pests, they have a mild effect on the environment compared to their synthetic counterpart, and they are specific in their target, hence preventing bioaccumulation (Saberi et al., 2020; Kumar et al., 2021). Biopesticides are made from natural substances, such as plants, microbes, and nanoparticles of biological origin, thus, making them a sustainable means of pest control (Kumar et al., 2021).
Some successes have been recorded in the use of biopesticides in the control of some pests to which chemical pesticides are being applied (Table 2).
The effectiveness of biopesticides in pest management comes from various modes of action, which include actions that regulate gut disruption, pest growth, and pest metabolism. Biopesticides work by denaturing protein, causing metabolic disorder and paralysis, activating target-poisoning mechanisms, exhibiting multisite inhibitory actions, and releasing neuromuscular toxins and bioactive compounds (Figure 3; Sparks and Nauen, 2015; Dar et al., 2021; Fenibo et al., 2021). These multiple actions offer biopesticides the capacity to alter the course of pest resistance as compared to chemical pesticides. Studies have indicated that biopesticides are eco-friendly, possess low toxicity properties, are biodegradable, and specific in action with little or no negative impact on non-target organisms (Deravel et al., 2014; Kalpana and Anil, 2021), Unlike biopesticides, conventional pesticides are a major source of environmental pollution, which promotes pest resistance with high post-harvest contamination and bioaccumulation in food crops (Fenibo et al., 2021).
However, there are various limitations to the full adoption, development, and use of biopesticides in agriculture. Biopesticides are often ranked as having low efficacy and a slower rate in the control of pests and diseases (Damalas and Koutroubas, 2016; Delgado‐Carrillo et al., 2018). Commercial biopesticide products are highly expensive and not readily available in the global market. In addition to the problem of commercialization, biopesticides also face quality control problems and concise shelf-life (Arthurs and Dara, 2019).
Many farmers also worry about dosage recommendations and fear the evaluation of new pest species that may be resistant to the existing biopesticides (Stevenson et al., 2017). The advantages and disadvantages of biopesticides are summarized in Table 3. Biopesticides have also been classified into three groups based on their extraction source and the constituting molecule/compound. The three groups include; (i) Microbial Biopesticides; (ii) Biochemical Pesticides; and (iii) GMO-Based Biopesticides. The source characteristics and the consisting molecules of biopesticide influence the mechanisms by which biopesticides protect the crops from the attack of pathogens. For example, fungicides and bactericides derived from microorganisms act by inhibiting or disrupting the process of protein translation, or cause a major disruption in plasma membrane permeability, thus leading to cell death, while some may prevent glucose formation in target pathogens (Parker and Sperandio, 2009; Svidritskiy, et al., 2013; Gwinn, 2018).
Microbial pesticides consist of substances derived from microorganisms like bacteria, fungi, viruses, protozoa, and algae, which are used in the control of pests (Adeleke et al., 2022c). Microbes use the toxic metabolites produced to destroy and prevent the growth of pests. Microbial pesticides are applied to the environment through different techniques, such as emulsion, electrospraying system, fluidized bed, spray drying, extrusion, lyophilization, spray cooling, and coacervation (De Oliveira et al., 2021). The major categories of microbes used as biopesticides include bacteria genera, Chromobacterium, Pseudomonas, and Yersinia, fungal genera Beauveria, Paecilomyces, Verticillium, Hirsutella, Metarhizium, and Lecanicillium and nematodes belonging to the genera Steinernema and Heterorhabditis (Chang et al., 2003; Kumar et al., 2021; Adeleke et al., 2022b).
A fungi species, Trichoderma sp. has been reported to prevent the activity of numerous fungi inhabiting the soil that cause root rot, black gram, and green gram in chickpeas, and groundnut (Samada and Tambunan, 2020). Likewise, Beauveria bassiana and M. brunneum have been reported in the control of thrips, beetles, weevil, aphids, whiteflies, and mites infestation in ornamental crops, fruits, and vegetables (Dara, 2017; Arthurs and Dara, 2019). Other examples of microbial pesticides are listed in Table 2.
Of all the bacterial pesticides, Bacillus thuringiensis (Bt) is well-known and have been made into products available for commercial purpose (Ujvary, 2010; Ruiu, 2018). Bacillus thuringiensis is a Gram-positive bacteria that acts as an insecticide by producing exudates, such as poisonous parasporal crystals and endospores which when consumed by insects get dissolved in their midgut by the alkaline environment and release delta-endotoxin, a protein that has a lethal effect on insects (Xiao and Wu, 2019). Bacillus thuringiensis is used to reduce pest infestation in plants, such as cabbage and potato, and is capable of controlling lepidopterans in different plants (Berini et al., 2018; Samada and Tambunan, 2020). As good as the positive effects of commercially available Bt sounds, they come with some drawbacks which include quick deactivation when exposed to light, short activity time, slow lethal rate, and low awareness and sensitivity to the environment (Xiao and Wu, 2019). The short life and environmental sensitivity of microbial pesticides, which reduce their awareness and usage are the major challenges associated with their use (Pathak et al., 2017). For instance, baculoviruses only survive in their host and cannot reproduce outside their host; hence, they cannot be used outside their host (Borges et al., 2021). Their host may have an adverse environmental impact on the environment, and their use might be dangerous.
Fungi are also used to control plant pests. An example is the mycoinsecticide, which is a microbial insecticide whose active ingredient is a living fungus that exhibits an antagonistic effect on insects or other arthropod pests, with some strains releasing metabolites while inside the pest that may also injure or kill it (Zaki et al., 2020). Only a few rare fungal strains have been developed as commercial mycoinsecticides, hence, the technology is still in its early stages. Attachments, germination, penetration, invasion, replication, and host death are the six general phases of action for mycoinsecticides (Zaki et al., 2020).
Spores can land on and attach to the target host’s cuticle when the formulated product is diluted and applied according to label instructions. Adhesion is primarily achieved through hydrophobic interactions between the cuticle and the spore. The number of spores attached to the host’s body determines their efficacy. The spore germinates in response to chemical cues on the cuticle and then develops an aspersorium, which is the penetration structure. The fungus penetrates the layers of the cuticle through a combination of mechanical pressure and enzyme degradation (Zaki et al., 2020).
Generally, microbial pesticides exert no adverse effects on the environment, producers, and consumers of agricultural products because their ingredients are generally considered safe and are target-specific (Guven et al., 2021). In addition, their usage lower greenhouse gas emissions compared to chemical pesticides (Llamas et al., 2021). Furthermore, there is a wide variety of organisms from which microbial biopesticides can be derived to solve the problem of resistance and ensures sustainability. Since different microbes used as biopesticides might require different storage condition, it might be cumbersome for sellers, producers, marketers, and end users to cope with their storage and transportation. Hence, more research is needed to ensure a sustainable and extended shelf-ability of microbial pesticides.
Essential oil and extract from different parts of plants have been successfully used to control plant diseases (Ayilara et al., 2022a). They attract, repel, prevent respiration, detect host plants from specific pests, destroy the eggs and larvae of pests, and destroy pests from feeding on plants (Tripathi et al., 2009; Halder et al., 2013; Ali et al., 2017). Essential oil from Coleus aromaticus Benth., Hyptis suaveolens (L.), Azadirachta indica, Ageratum conyzoides L., and Achillea sp., have been reported to control the infestation of Tribolium castaneum (Herbst), a red flour beetle that destroys many crop species (Singh et al., 2014; Jaleel et al., 2015; Upadhyay et al., 2018). Other plant parts, such as bark, flowers, roots, leaves, peels, seeds, and buds can be used to control different plant pathogens (Tongnuanchan and Benjakul, 2014).
Plant families that have been reported to contain bioactive compounds with activity against important crop pests include Myrtaceae, Lauraceae, Rutaceae, Lamiaceae, Asteraceae, Apiaceae, Cupressaceae, Poaceae, Zingiberaceae, Piperaceae, Liliaceae, Apocynaceae, Solanaceae, Caesalpinaceae, and Sapotaceae (Gakuubi et al., 2016). They are easily available which makes them inexpensive and can be easily incorporated into agricultural production systems. Secondary metabolites, such as steroids, alkaloids, tannins, terpenes, phenols, flavonoids, and resins are commonly found in botanical pesticides and have shown antifungal, antibacterial, antioxidant, or insecticidal properties (Ahmad et al., 2017). The specific compounds found in certain plant species make them effective against a specific category of pests and also determine their mode of action on the target pests (Lengai et al., 2020). Botanical pesticides contain bioactive compounds that act in a variety of ways against pests, such as insects, fungi, bacteria, nematodes, and plant host cells infected with viral pathogens (Lengai et al., 2020). Depending on the botanical compound and pest, the modes of action may include repellence, inhibition, protein denaturation, and other effects. Pesticides derived from pyrethrum target insect nerve cells, thus causing paralysis and death. Also, neem-based pesticides with antifeedant and repellent properties, induce moulting abnormalities, hinder oviposition, and disrupt the endocrine system (Lengai et al., 2020).
Pesticides from plants have been well-reported to interfere with the normal metabolism of insect pests, which include the octopamine and acetylcholinesterase pathways (Polsinelli et al., 2010; Pang et al., 2012; Dassanayake et al., 2021). Acetylcholinesterase is an enzyme used by insects in their neuronal communication and neuromuscular functions and can be toxic to insects by destroying the membrane of the postsynaptic junction and the current of the nerve. Octapamine on the other hand is a hormone involved in neuromodulation and neurotransmission in insects and can impair the muscle juncture and homeostasis of the body fluids of insects (Dassanayake et al., 2021). Equally, plant pesticides can prevent cell wall biosynthesis, cell membrane structure, ATPases function, quorum sensing, efflux pumps, and biofilm formation (Lang and Buchbauer, 2012; Hu et al., 2017). Extracts from four weed plants, namely Lippia javanica, Tithonia diversifolia, Vernonia amygdalina, and ephrosia vogelii, in Tanzania were used to control insects in common bean (Mkenda et al., 2015). Similarly, Lovatto et al. (2004) carried out an experiment where nine different aqueous plant extracts from the leaves, fruits, and flowers of nine plants were used to repel and kill Brevicoryne brassicae. Solanum pseudocapsicum L., and Solanum guaraniticum A were reported to be the most effective.
Nanobiopesticides can be defined as biological protection products that are developed using nanotechnology to enhance efficacy and reduce an environmental load of pesticides (Chaudhary et al., 2021b,d). Nanobiopesticides are formulated from nanomaterials and applied specially fixed on a hybrid substrate, encapsulated in a matrix or functionalized nanocarriers for external stimuli or enzyme-mediated triggers (Agostini et al., 2012; Khati et al., 2018; Kumari et al., 2020; Agri et al., 2021, 2022; Chaudhary et al., 2022; Pan et al., 2023). They are nanostructures with two or three dimensions used for carrying agrochemical ingredients and can help increase water solubility and bioavailability, and protect agrochemicals against environmental degradation. It also helps revolutionize the control of pathogens, weeds, and insects in crops (Yadav et al., 2020). They are available in different forms, such as nano-gel, nano-encapsulation, nano-fibres, nano-sphere, etc. (Rajna and Paschapur, 2019; Pan et al., 2023).
Nanoparticles in recent years are being reported to be very helpful in agriculture (Omole et al., 2018). They have been used as active ingredients and carriers to stabilize many agrochemicals and their products from them include nanofertilizers, nanopesticides, etc. (Chaudhary and Sharma, 2019; Chaudhary et al., 2021a). For instance, pesticides from nanomaterials, such as magnesium oxide, magnesium hydroxide, copper oxide, and zinc oxide derived from aqueous extracts of Chamaemelum nobile flowers, Punica granatum peels, green peach aphid (GPA) and Olea europaea leaves have been reported in the control of insects (Grillo et al., 2021; Konappa et al., 2021). Also, silver nanoparticles derived from the leaf extract of Euphorbia hirta have been explored in the control of the causative agent of cotton bollworm, Helicoverpa armigera (Devi et al., 2014). The ability of copper oxide nanoparticles and zinc oxide nanoparticles to control Alternaria citri, a causative agent of citrus black rot disease in the plant has as well been reported (Lasso-Robledo et al., 2022). In addition, Sardar et al. (2022) used combined and individual zinc oxide and copper oxide to control citrus black rot disease in a potato dextrose medium. The fungal and insecticidal effects of copper nanoparticles have been demonstrated against Tribolium castaneum, a pest that affects grain (El-Saadony et al., 2020). The major interactions which occur between plants and nanoparticles have been studied using different techniques, which include fluorescence spectroscopy, microscopy, and magnetic resonance imaging (Chhipa, 2019). The effectiveness of nanobiopesticides can be determined by the composition, surface charge, concentration, size, and chemical and physical changes (Chhipa, 2019).
The critical role of nanoformulations in reducing active ingredient degradation, improving water solubility equilibrium, and increasing the biological availability of active ingredients is well understood, and this has helped in avoiding endemic pest infestation, plant injury, and economic loss by lowering the quality and quantity of agricultural products and foods (Syafrudin et al., 2021; Chaudhary et al., 2021a,c).
Because of their small size and larger surface area, nanopesticides’ chemical properties differ significantly from conventional pesticides, and these properties can be used to develop an efficient assemble of a structure with several advantages, such as the possibility of better interaction and mode of action at a target site of the desired pest. Nano-sized products exhibit greater selectivity without impairing compound bioactivity against the target pathogen. Their increased toxicity can also increase pest penetration (Priya et al., 2018). Nanoparticle application reduces drifting and leaching issues and allows for the use of a smaller amount of active compound per area, as long as the formulation can provide optimal concentration delivery for the target insecticide for longer periods. There are several methods for creating pesticide nanoproducts, such as nanoemulsions, nanocapsules, and inorganic engineered nanoparticles (such as metal oxides, metals, and clays), and can be further developed to improve the efficacy of existing pesticides, reduce their environmental toxicity, or both.
On a general note, biopesticides have been reported to be capable of controlling pests but their sole use for sustainable agriculture may not be realistic, majorly because they are not readily available in many locations and their mode of action can be very slow. Hence, they should be incorporated with the existing synthetic pesticides and be applied majorly close to the harvest period of crops since residual chemicals observed in plants are those majorly applied close to harvest periods. Furthermore, this will help to maintain suitable agriculture, pending the improvements of biopesticides.
It is very important to understand the molecular mechanisms underlying the action of biopesticides at each stage of action to ensure better control strategies over pests. Understanding the biopesticides mechanisms of action against insect pests at the molecular level will allow for synergistic approaches among biopesticides, which have different mechanisms of action without an overlapping mechanism. This will also give allowance for the exploration of different toxic molecules present in biopesticides that can enlarge the pesticidal arsenal of these biopesticides. The widely used biopesticides and their mechanisms of action at the biochemical level have been described. However, the entomopathogenic fungus, Beauveria bassiana has gained wide acceptance and can be used as a model to describe the molecular mechanism of biopesticides’ application.
Beauveria bassiana is an example of an entomopathogenic fungus that has been widely used as biopesticide because it is highly efficacious against a lot of arthropod hosts (Boomsma et al., 2014). However, to understand their effectiveness and sustainability against pests, there is a need to fully evaluate their molecular mechanism of pathogenicity beyond the conventional approach. The mechanism of pathogenicity of B. bassiana begins with adhesion to the host pest, penetration of cuticle, and colonization of the pest heaemocoel (Wojda et al., 2009).
The hydrophobins-coated aerial conidia of B. bassiana allow its hydrophobic interaction with the cuticles of insects (Holder and Keyhani, 2005). This hydrophobicity of the B. bassiana aerial conidia can be influenced by the role that several genes expressed by B. bassiana play in lipid homeostasis. It has been revealed by transcriptomics analyses that there is an upregulation of gene expressions for hydrophobins and Metarhizium adhesion-like protein 1, 2 (MAD 1, MAD2) by B. bassiana which are crucial for its hydrophobic attachment to the cuticle of insect (Wang and St Leger, 2007). The transportation and storage of lipids in the conidia, and maintenance of the lipid homeostasis of B. bassiana is possible when mammalian-like perilipin 1 (MPL1) genes are over-expressed (Chen et al., 2018). The role that the MPL1 gene plays is crucial because its deletion causes a reduction in the turgor pressure of the appressoria impairing the adhesiveness of B. bassiana (Wang and Leger, 2007). Also, the surface sensing and signaling for the germination of conidia and formation of appressoria is made possible by CFEM-domain-containing genes in B. bassiana (Sabnam and Barman, 2017). Proteomics has also revealed that B. bassiana secretes sphingomyelin phosphodiesterase, which allows it to disrupt the membrane of the host insect upon contact with the cuticles of the insect (Santi et al., 2019).
Once B. bassiana completed adhesion to the host insect, its conidia germinate and develop appressoria to allow penetration into the cuticle of the host. The penetration efficiency of B. bassiana usually increased when the structural outlook of the appressorium allows the synergistic functioning of enzymatic digestion and mechanical pressure (Singh et al., 2017). The hyphae of B. bassiana germinate in the exoskeleton of the insect as the penetration proceeds and B. bassiana produces secondary hyphae inside the cuticle. The hyphae switch to blastospores (motile, more hydrophilic, and better evade the insect’s host immunity) when exposed to hyperosmotic environment in the haemocoel (Ortiz-Urquiza and Keyhani, 2016). Through transcriptomics, it has been reported that chitin synthase is responsible for chitin production, and β-1,3-glucanases soften the cell wall to allow germination, while several cell wall protein-conferring genes give the cell wall of B. bassiana its building blocks (Tartar et al., 2005; Mouyna et al., 2013; Chen et al., 2018). Genes necessary for the cell body differentiation in B. bassiana include osmosensor Mos1, signaling-related genes, and mitogen-activated-protein kinases (MAPKs) like protein kinase A (PKA; Chen et al., 2018; Zhou et al., 2019). For penetration into the cuticle of the host insect, notable proteases, lipases, chitinases, and carboxypeptidases have been reported and these include subtilisin-like protease (Pr) isoform 1A (Pr1A) and 1B (Pr1B), cytochrome P450s (CYPs) and GH18 family chitinases (Lai et al., 2017).
In response to the penetration into the cuticle of the insect, the insect activates melanization and produces antimicrobial peptides (AMPs), reactive oxygen species (ROS), and protease inhibitors (Ortiz-Urquiza and Keyhani, 2016). Stress management and immune-evasion-related genes are upregulated to overcome the host insect defense mechanisms. Glutathione S-transferases (GSTs), catalases, peroxidases, superoxide dismutase (SODs), thioredoxins, and oxidoreductases are anti-oxidative enzyme-producing genes over-expressed in B. bassiana (Lai et al., 2017). Heat shock proteins (HSPs) are expressed to maintain internal cellular integrity against diverse types of stress (Santi et al., 2019). Another mechanism used by B. bassiana is the production of secondary metabolites that are toxic to the insect cell. These metabolites include oosporeins, beauvericin, isarolides, beauverolides, tenellins, and bassianolide (Chandler, 2017). The biosynthesis of oosporein happens in the haemocoel and it is mediated by the over-expression of polyketide synthase (PKS) gene (Lai et al., 2017). It is interesting to note that a greater amount of beauverolides secreted by B. bassiana usually occur when live insect tissues are present than in the presence of dead insect tissues (de Bekker et al., 2013). With these fantastic mechanisms of action, B. bassiana stands out among the entomopathogenic fungi, thus making it an attractive and widely used biopesticide against a lot of arthropod hosts.
Lastly, it is good to note that the complex mechanism of pathogenesis exhibited by B. bassiana cannot be fully understood by a singular omics approach, there is a need to examine the total expressions of different proteins, secondary metabolites, and their genes at every infection stage. Hence, researchers in different fields of omics need to collaborate to work with the same parameters to have a holistic view of the mechanism of action of different biopesticides.
Integrated Pest Management (IPM) system refers to the mechanism of controlling pests using different techniques, such as habitat manipulation, biological and chemical control measures, use of pest-resistant varieties, and the modification of cultural practices. These techniques can be merged to ensure the long-term protection of plants (Deguine et al., 2021). For instance, IPM has been used in the control of Tuta absoluta, a deadly pest that affects tomatoes globally, and has developed resistance to insecticides (Desneux et al., 2021). Here, the synthetic pesticides and biological pesticides include the release and conservation of sex pheromones and arthropod natural enemies (Desneux et al., 2021). The use of IPM has been reported to be cost-effective and reduces the loss of crop yield (Hagstrum and Flinn, 2018). Currently, the adoption of IPM is limited owing to several factors, which include awareness, user preference, production industry, technology, policy, and culture (Deguine et al., 2021). It is, therefore, necessary to increase awareness of the inclusion of biological pesticides from microorganisms, plants, and nanobiopesticides in IPM. The awareness of many people about IPM will be an advantage to encourage producers to produce more of it, enhance its adoption and encourage researchers to carry out more research on it.
A lot of crops are lost yearly to pest, but the emergence of synthetic pesticides have helped to reduce the loss. Nevertheless, the adverse effects of synthetic pesticides limit their use; thus, promoting the use of biological pesticides. Since biopesticides have proven as good alternative to chemical pesticides, it will be very important to explore them for maximum use in agriculture. The demand and availability of biopesticides are very poor, hence discouraging the producers and the users, respectively. Therefore, making grants or capital available for researchers, entrepreneurs, producers, and marketers will help to enhance the production and availability of biopesticides.
The shelf-life of biopesticides is short, as they require special temperatures and conditions for survival during transportation and storage. Hence, more research to unravel the mechanisms to make biopesticides more stable and improve their shelf-life will go a long way in increasing their efficiency.
The fact that biopesticides have no residual effects on the environment could serve as an advantage and a disadvantage. An advantage because it will not remain long enough to be dangerous to the plants, humans, and animals (which is one of the major demerits of synthetic pesticides), and it is a disadvantage because it will only protect crops as long as it has contacts with the pests, and pests that infest after their application would not be affected and might need another application, thus leading to a higher cost implication and labor for farmers. Consequently, more research should be carried out to incorporate bio-carriers and other sustainable methods, which can be used to enhance the persistence of biopesticides in the environment. Since biopesticides are highly specific in their mode of action, chemical reactions may occur if more than a biopesticide is applied to a crop that is affected by different pests. Hence, it is important to carry out more research on the compatibility of different biopesticides, which are likely to be used together on the same crop. Furthermore, most research carried out on biopesticides was focused on yield and not the nutritional quality of the crops, an insight into the nutritional quality of biopesticides will enhance their use.
The Maximum Residual Limit for pesticides in local markets (not only for food crops that would be exported) should be enforced and awareness should be created on the effectiveness of biopesticides so that farmers can explore them. In addition, a mobile meter, device, or strip could be developed, made affordable and easily available to enhance the easy and rapid detection of pesticide levels in food crops. This will help farmers to take caution and also help the populace to avoid feeding on crops with a Maximum Residual Limit greater than the WHO specified value. Awareness of the effects of the indiscriminate use and health effects of biopesticides in humans will also help to promote a good environment and health. Due to the numerous challenges still encountered with the use of biopesticides, the sole use of biopesticides might not be feasible. Therefore, their incorporation with the existing synthetic pesticides will be a better means of preventing crops from pests and ensuring sustainable agriculture.
MA, BA, and SA conceived the idea and were involved in the writing of the manuscript. CF, UA, LG, RO, RJ, and QU contributed to the writing of the manuscript. MA, BA, and RO revised the manuscript. OB reviewed and edited the final draft of the manuscript. All authors contributed to the article and approved the submitted version.
This study was funded through research grants from the National Research Foundation, South Africa (UID: 123634 and 132595).
OB appreciates the research grants from the National Research Foundation, South Africa.
The authors declare that the research was conducted in the absence of any commercial or financial relationships that could be construed as a potential conflict of interest.
All claims expressed in this article are solely those of the authors and do not necessarily represent those of their affiliated organizations, or those of the publisher, the editors and the reviewers. Any product that may be evaluated in this article, or claim that may be made by its manufacturer, is not guaranteed or endorsed by the publisher.
Aba, S., Eze, S., Ishieze, P., Omeje, T., and Ugwuoke, K. (2018). Controlling phytophthora blight of cucumber (Cucumis sativus) in a derived savannah, using different spray intervals of a registered fungicide. Plant Pathol. Quar. 8, 122–130. doi: 10.5943/ppq/8/2/3
Abbas, M. S. T. (2020). Interactions between entomopathogenic fungi and entomophagous insects. Adv. Entomol. 08, 130–146. doi: 10.4236/ae.2020.83010
Abbas, M. S. T. (2022). Pathogenicity of entomopathogenic nematodes to dipteran leaf miners, house flies and mushroom flies. Egypt. J. Biol. Pest Control 32, 1–9. doi: 10.1186/s41938-022-00566-y
Abdollahdokht, D., Gao, Y., Faramarz, S., Poustforoosh, A., Abbasi, M., Asadikaram, G., et al. (2022). Conventional agrochemicals towards nano-biopesticides: an overview on recent advances. Chem. Biol. Technol. Agric. 9:13. doi: 10.1186/s40538-021-00281-0
Abdullahi, G., Obeng-Ofori, D., Afreh-Nuamah, K., and Billah, M. (2020). Acute and residual concentration-dependent toxicities of some selected insecticides to adult Bactrocera invadens drew, Tsuruta and white (Diptera: Tephritidae). J. Basic Appl. Zool. 81, 1–10. doi: 10.1186/s41936-020-00144-4
Abraham, D. A., and Vasantha, V. S. (2020). Hollow polypyrrole composite synthesis for detection of trace-level toxic herbicide. ACS Omega 5, 21458–21467. doi: 10.1021/acsomega.0c01870
%Abubakar, Y., Tijjani, H., Egbuna, C., Adetunji, C. O., Kala, S., Kryeziu, T. L., et al. (2020). “Pesticides, history, and classification” in Natural Remedies for Pest, Disease and Weed Control. eds. E. Chukwuebuka and S. Barbara (Netherlands: Elsevier), 29–42.
Adeleke, B. S., Akinola, S. A., Adedayo, A. A., Glick, B. R., and Babalola, O. O. (2022a). Synergistic relationship of endophyte-nanomaterials to alleviate abiotic stress in plants. Front. Environ. Sci. 10:1015897. doi: 10.3389/fenvs.2022.1015897
Adeleke, B. S., Ayangbenro, A. S., and Babalola, O. O. (2022b). In vitro screening of sunflower associated endophytic bacteria with plant growth-promoting traits. Front. Sustain. Food Syst. 6:903114. doi: 10.3389/fsufs.2022.903114
Adeleke, B. S., Ayilara, M. S., Akinola, S. A., and Babalola, O. O. (2022c). Biocontrol mechanisms of endophytic fungi. Egypt. J. Biol. Pest Control 32, 1–17. doi: 10.1186/s41938-022-00547-1
Adeleke, B. S., Fadiji, A. E., Ayilara, M. S., Igiehon, O. N., Nwachukwu, B. C., and Babalola, O. O. (2022d). Strategies to enhance the use of endophytes as bioinoculants in agriculture. Horticulturae 8:498. doi: 10.3390/horticulturae8060498
Agostini, A., Mondragón, L., Coll, C., Aznar, E., Marcos, M. D., Martínez-Máñez, R., et al. (2012). Dual enzyme-triggered controlled release on capped nanometric silica mesoporous supports. Chemistry Open 1, 17–20. doi: 10.1002/open.201200003
Agri, U., Chaudhary, P., and Sharma, A. (2021). In vitro compatibility evaluation of agriusable nanochitosan on beneficial plant growth-promoting rhizobacteria and maize plant. Nat Acad. Sci. Lett. 44, 555–559. doi: 10.1007/s40009-021-01047-w
Agri, U., Chaudhary, P., Sharma, A., and Kukreti, B. (2022). Physiological response of maize plants and its rhizospheric microbiome under the influence of potential bioinoculants and nanochitosan. Plant Soil 474, 451–468. doi: 10.1007/s11104-022-05351-2
Ahmad, W., Singh, S., and Kumar, S. (2017). Phytochemical screening and antimicrobial study of Euphorbia hirta extracts. J. Med. Plant Study 5, 183–186.
Aioub, A. A., El-Ashry, R. M., Hashem, A. S., Elesawy, A. E., and Elsobki, A. E. (2021). Compatibility of entomopathogenic nematodes with insecticides against the cabbage white butterfly, Pieris rapae L.(Lepidoptera: Pieridae). Egypt. J. Biol. Pest Control 31, 1–12. doi: 10.1186/s41938-021-00498-z
Akanmu, A. O., Babalola, O. O., Venturi, V., Ayilara, M. S., Adeleke, B. S., Amoo, A. E., et al. (2021). Plant disease management: leveraging on the plant-microbe-soil interface in the biorational use of organic amendments. Front. Plant Sci. 12:700507. doi: 10.3389/fpls.2021.700507
Ali, A. M., Mohamed, D. S., Shaurub, E., and Elsayed, A. M. (2017). Antifeedant activity and some biochemical effects of garlic and lemon essential oils on Spodoptera littoralis (Boisduval)(Lepidoptera: Noctuidae). J. Entomol. Zool. Stud. 5, 1476–1482.
%Ali, S., Ullah, M. I., Sajjad, A., Shakeel, Q., and Hussain, A. (2021). “Environmental and health effects of pesticide residues” in Sustainable Agriculture Reviews. eds. Inamuddin, M. I. Ahamed and E. Lichtfouse (New York, NY: Springer), 311–336.
Allen, K. C., Luttrell, R. G., Sappington, T. W., Hesler, L. S., and Papiernik, S. K. (2018). Frequency and abundance of selected early-season insect pests of cotton. J. Integr. Pest Manag. 9, 1–20. doi: 10.1093/jipm/pmy010
Anakwue, R. (2019). Cardiotoxicity of pesticides: are Africans at risk? Cardiovasc. Toxicol. 19, 95–104. doi: 10.1007/s12012-018-9486-7
%Anani, O. A., Mishra, R. R., Mishra, P., Enuneku, A. A., Anani, G. A., and Adetunji, C. O. (2020). “Effects of toxicant from pesticides on food security: current developments” in Innovations in Food Technology. eds. P. Mishra, R. R. Mishra and C. O. Adetunji (New York, NY: Springer), 313–321.
Andrew, G. S., Simon, U. T., John, A. U., Godwin, O. O., Alexander, N. I., and Ikagu, Y. M. (2018). Studies on changes in some haematological and plasma biochemical parameters in wistar rats fed on diets containing calcium carbide ripened mango fruits. Int. J. Food Sci. Nutr. Eng. 8, 27–36. doi: 10.5923/j.food.20180802.02
Anikwe, J. C., Okelana, F. A., Mokwunye, I. U., and Oyedokun, A. Y. (2021). History and recent development of pesticide usage on cocoa in Nigeria. Afric. J. Gen. Agric. 5, 211–219.
Arthurs, S., and Dara, S. K. (2019). Microbial biopesticides for invertebrate pests and their markets in the United States. J. Invertebr. Pathol. 165, 13–21. doi: 10.1016/j.jip.2018.01.008
Ayilara, M. S., Abberton, M., Oyatomi, O. A., Babalola, O. O., and Odeyemi, O. (2022a). Potentials of underutilized legumes in food security. Front. Soil Sci. 2:1020193. doi: 10.3389/fsoil.2022.1020193
Ayilara, M. S., Adeleke, B. S., and Babalola, O. O. (2022b). Bioprospecting and challenges of plant microbiome research for sustainable agriculture, a review on soybean endophytic bacteria. Microb. Ecol. 2022, 1–23. doi: 10.1007/s00248-022-02136-z
Basiev, S., Bekmurzov, A., Bekuzarova, S., Dulaev, T., Sokolova, L., Bolieva, Z., et al. (2019). Phytoinsecticides to fight against Colorado beetle. KnE Life Sci. 2022, 562–569. doi: 10.18502/kls.v4i14.5643
Benelli, G. (2022). Insecticide, acaricide, repellent and antimicrobial development. Molecules 27:386. doi: 10.3390/molecules27020386
Berini, F., Katz, C., Gruzdev, N., Casartelli, M., Tettamanti, G., and Marinelli, F. (2018). Microbial and viral chitinases: attractive biopesticides for integrated pest management. Biotechnol. Adv. 36, 818–838. doi: 10.1016/j.biotechadv.2018.01.002
Bhadoria, P., Nagar, M., Bharihoke, V., and Bhadoria, A. S. (2018). Ethephon, an organophosphorous, a fruit and vegetable ripener: has potential hepatotoxic effects? J. Fam. Med. Primary Care 7:179. doi: 10.4103/jfmpc.jfmpc_422_16
Boomsma, J. J., Jensen, A. B., Meyling, N. V., and Eilenberg, J. (2014). Evolutionary interaction networks of insect pathogenic fungi. Annu. Rev. Entomol. 59, 467–485. doi: 10.1146/annurev-ento-011613-162054
Borges, S., Alkassab, A. T., Collison, E., Hinarejos, S., Jones, B., McVey, E., et al. (2021). Overview of the testing and assessment of effects of microbial pesticides on bees: strengths, challenges and perspectives. Apidologie 52, 1256–1277. doi: 10.1007/s13592-021-00900-7
Boucaud-Maitre, D., Rambourg, M.-O., Sinno-Tellier, S., Puskarczyk, E., Pineau, X., Kammerer, M., et al. (2019). Human exposure to banned pesticides reported to the French poison control centers: 2012–2016. Environ. Toxicol. Pharmacol. 69, 51–56. doi: 10.1016/j.etap.2019.03.017
Bratovcic, A., Hikal, W. M., Said-Al Ahl, H. A. H., Tkachenko, K. G., Baeshen, R. S., Sabra, A. S., et al. (2021). Nanopesticides and Nanofertilizers and agricultural development: scopes, advances and applications. OJE 11, 301–316. doi: 10.4236/oje.2021.114022
Campos, J. N. D., Rabelo, M. M., Bann, P. L., Unruh, J. B., and de Paula-Moraes, S. V. (2022). Insecticide efficacy against tropical sod webworm in concentration-mortality bioassay, 2021. Arthropod. Manag. Tests 47:tsac009. doi: 10.1093/amt/tsac009
%Chandler, D. (2017). “Basic and applied research on entomopathogenic fungi” in Microbial Control of Insect and Mite Pests. ed. L. A. Lacey (Amsterdam: Elsevier), 69–89.
Chang, J. H., Choi, J. Y., Jin, B. R., Roh, J. Y., Olszewski, J. A., Seo, S. J., et al. (2003). An improved baculovirus insecticide producing occlusion bodies that contain bacillus thuringiensis insect toxin. J. Invertebr. Pathol. 84, 30–37. doi: 10.1016/S0022-2011(03)00121-6
Chaudhary, P., Chaudhary, A., Bhatt, P., Kumar, G., Khatoon, H., Rani, A., et al. (2022). Assessment of soil health indicators under the influence of nanocompounds and bacillus spp. in field condition. Frontiers in environmental. Science 9:648. doi: 10.3389/fenvs.2021.769871
Chaudhary, P., Chaudhary, A., Parveen, H., Rani, A., Kumar, G., Kumar, R., et al. (2021a). Impact of nanophos in agriculture to improve functional bacterial community and crop productivity. BMC Plant Biol. 21, 1–12. doi: 10.1186/s12870-021-03298-7
Chaudhary, P., Khati, P., Chaudhary, A., Gangola, S., Kumar, R., and Sharma, A. (2021b). Bioinoculation using indigenous bacillus spp. improves growth and yield of Zea mays under the influence of nanozeolite. Biotechnology 11, 1–12. doi: 10.1007/s13205-020-02561-2
Chaudhary, P., Khati, P., Chaudhary, A., Maithani, D., Kumar, G., and Sharma, A. (2021c). Cultivable and metagenomic approach to study the combined impact of nanogypsum and Pseudomonas taiwanensis on maize plant health and its rhizospheric microbiome. PLoS One 16:e0250574. doi: 10.1371/journal.pone.0250574
Chaudhary, P., and Sharma, A. (2019). Response of nanogypsum on the performance of plant growth promotory bacteria recovered from nanocompound infested agriculture field. Environ. Ecol. 37, 363–372.
Chaudhary, P., Sharma, A., Chaudhary, A., Khati, P., Gangola, S., and Maithani, D. (2021d). Illumina based high throughput analysis of microbial diversity of maize rhizosphere treated with nanocompounds and bacillus sp. Appl. Soil Ecol. 159:103836. doi: 10.1016/j.apsoil.2020.103836
Chen, A., Wang, Y., Shao, Y., Zhou, Q., Chen, S., Wu, Y., et al. (2018). Genes involved in Beauveria bassiana infection to galleria mellonella. Arch. Microbiol. 200, 541–552. doi: 10.1007/s00203-017-1456-0
%Chhipa, H. (2019). “Applications of nanotechnology in agriculture” in Methods in Microbiology. eds. V. Gurtler, A. S. Ball and S. Soni (Amsterdam: Elsevier), 115–142.
Choudhary, S., Yamini, N. R., Yadav, S. K., Kamboj, M., and Sharma, A. (2018). A review: pesticide residue: cause of many animal health problems. J. Entomol. Zool. Stud. 6, 330–333.
Damalas, C. A., and Koutroubas, S. D. (2016). Farmers’ exposure to pesticides: toxicity types and ways of prevention. Toxics 4, 1–10. doi: 10.3390/toxics4010001
Damalas, C. A., and Koutroubas, S. D. (2020). “Botanical pesticides for eco-friendly pest management: drawbacks and limitations” in Pesticides in Crop Production: Physiological Biochemical Action, vol. 10, 181–193.
%Dar, S. A., Wani, S. H., Mir, M., Showkat, A., Dolkar, T., and Dawa, T. (2021). Biopesticides: mode of action, efficacy and scope in pest management. J. Adv. Res. Biochem. Pharmacol. 4, 1–8.
%Dara, S. (2017). “Microbial control of arthropod pests in small fruits and vegetables in temperate climate” in Microbial Control of Insect and Mite Pests. ed. L. A. Lacey (Amsterdam: Elsevier), 209–221.
Dassanayake, M. K., Chong, C. H., Khoo, T.-J., Figiel, A., Szumny, A., and Choo, C. M. (2021). Synergistic field crop pest management properties of plant-derived essential oils in combination with synthetic pesticides and bioactive molecules: a review. Foods 10:2016. doi: 10.3390/foods10092016
de Bekker, C., Smith, P. B., Patterson, A. D., and Hughes, D. P. (2013). Metabolomics reveals the heterogeneous secretome of two entomopathogenic fungi to ex vivo cultured insect tissues. PLoS One 8:e70609. doi: 10.1371/journal.pone.0070609
De Oliveira, J. L., Fraceto, L. F., Bravo, A., and Polanczyk, R. A. (2021). Encapsulation strategies for bacillus thuringiensis: from now to the future. J. Agric. Food Chem. 69, 4564–4577. doi: 10.1021/acs.jafc.0c07118
Deguine, J.-P., Aubertot, J.-N., Flor, R. J., Lescourret, F., Wyckhuys, K. A., and Ratnadass, A. (2021). Integrated pest management: good intentions, hard realities. Agron. Sustain. Dev. 41, 1–35. doi: 10.1007/s13593-021-00689-w
Delgado‐Carrillo, O., Martén‐Rodríguez, S., Ashworth, L., Aguilar, R., Lopezaraiza‐Mikel, M., and Quesada, M. (2018). Temporal variation in pollination services to Cucurbita moschata is determined by bee gender and diversity. Ecosphere 9:e02506. doi: 10.1002/ecs2.2506
Deravel, J., Krier, F., and Jacques, P. (2014). Biopesticides, a complementary and alternative approach to the use of agrochemicals. A review. Biotechnologie, Agronomie, Société et Environnement 18, 220–232.
Desneux, N., Han, P., Mansour, R., Arnó, J., Brévault, T., Campos, M. R., et al. (2021). Integrated pest management of Tuta absoluta: practical implementations across different world regions. J. Pest. Sci. 1, 1–23. doi: 10.1007/S10340-021-01442-8
Devi, G. D., Murugan, K., and Selvam, C. P. (2014). Green synthesis of silver nanoparticles using Euphorbia hirta (Euphorbiaceae) leaf extract against crop pest of cotton bollworm, Helicoverpa armigera (Lepidoptera: Noctuidae). J. Biopest. 7:54.
Dogara, A. M., Hamad, S. W., Halliru, B. S., Wada, N., and Dalorima, T. (2022). Influences of different concentrations of herbicides on the growth and yield of maize as well as their effects on the associated weeds in North-Western Nigeria. Passer J. Basic Appl. Sci. 4, 31–36. doi: 10.24271/psr.2021.309284.1097
Dugje, I., Menkir, A., Kamai, N., Aminu, D., Kamara, A., Odo, P., et al. (2020). Participatory evaluation of provitamin a-rich maize (Zea mays L.) hybrids in savanna zones of Northeast Nigeria. Abuja J. Agric. Environ. 1, 133–154.
Ebrahimi, L., Sheikhigarjan, A., and Ghazavi, M. (2022). Entomopathogenic nematodes for control of potato tuber moth Phthorimaea operculella [Zeller], (Lepidoptera: Gelechiidae) in infested tubers. Int. J. Pest Manag. 2022, 1–8. doi: 10.1080/09670874.2022.2058709
Ebuehi, O. A., Oluwatosin, R., and Olufemi, A.-O. (2017). Sniper-organophosphate pesticide exposure induces neurotoxicity and biochemical agitation in male Sprague-Dawley rats. Int. J. Adv. Biotechnol. Res. 1, 20–25. doi: 10.33545/26174693.2017.v1.i2a.99
Ejilibe, C. O., Nwamba, H. O., Atama, I. C., Chiamaka, L. A., Aguzie, I. O., Madu, J. C., et al. (2019). Biochemical responses of Bufo regularis (Reuss, 1833) tadpole exposed to Butaforce® and Termex® pesticides. Pak. J. Zool. 51, 1–6. doi: 10.17582/journal.pjz/2019.51.6.2175.2180
El-Saadony, M. T., Abd El-Hack, M. E., Taha, A. E., Fouda, M. M., Ajarem, J. S., Maodaa, S. N., et al. (2020). Ecofriendly synthesis and insecticidal application of copper nanoparticles against the storage pest Tribolium castaneum. Nano 10:587. doi: 10.3390/nano10030587
Elsakhawy, T., Alkahtani, M. D., Sharshar, A. A., Attia, K. A., Hafez, Y. M., and Abdelaal, K. A. (2020). Efficacy of mushroom metabolites (Pleurotus ostreatus) as a natural product for the suppression of broomrape growth (Orobanche crenata Forsk) in faba bean plants. Plan. Theory 9, 1–18. doi: 10.3390/plants9101265
Essiedu, J. A., Adepoju, F. O., and Ivantsova, M. N. (2020). Benefits and limitations in using biopesticides: a review. AIP Conf. Proc. 2313:080002. doi: 10.1063/5.0032223
Falqueto, S. A., Pitaluga, B. F., de Sousa, J. R., Targanski, S. K., Campos, M. G., de Oliveira Mendes, T. A., et al. (2021). Bacillus spp. metabolites are effective in eradicating Aedes aegypti (Diptera: Culicidae) larvae with low toxicity to non-target species. J. Invertebr. Pathol. 179:107525. doi: 10.1016/j.jip.2020.107525
Fang, W., Wang, X., Huang, B., Zhang, D., Liu, J., Zhu, J., et al. (2020). Comparative analysis of the effects of five soil fumigants on the abundance of denitrifying microbes and changes in bacterial community composition. Ecotoxicol. Environ. Saf. 187:109850. doi: 10.1016/j.ecoenv.2019.109850
Farooq, M. A., Arif, M. J., Gogi, M. D., Nawaz, A., and Atta, B. (2019). Comparative efficacy of different pesticide residue mitigation modules in mango. Am. J. Biomed. Sci. Res. 4, 214–219. doi: 10.34297/AJBSR.2019.04.000801
Fenibo, E. O., Ijoma, G. N., and Matambo, T. (2021). Biopesticides in sustainable agriculture: A critical sustainable development driver governed by green chemistry principles. Front. Sus. Food Syst. 5:619058. doi: 10.3389/fsufs.2021.619058
Gakuubi, M. M., Wanzala, W., Wagacha, J. M., and Dossaji, S. F. (2016). Bioactive properties of Tagetes minuta L. (Asteraceae) essential oils: a review. Am. J. Essent. Oil Nat. Products 2, 27–36.
Garces, A., Pires, I., and Rodrigues, P. (2020). Teratological effects of pesticides in vertebrates: a review. J. Environ. Sci. Health A 55, 75–89. doi: 10.1080/03601234.2019.1660562
Gautam, U.K. (2020). Effect of the entomopathogenic fungus Isaria fumosorosea on physiological processes in insects. Faculty of Science, University of South Bohemia in České Budějovice
Ghelichpour, M., Mirghaed, A. T., Hoseini, S. M., and Jimenez, A. P. (2020). Plasma antioxidant and hepatic enzymes activity, thyroid hormones alterations and health status of liver tissue in common carp (Cyprinus carpio) exposed to lufenuron. Aquaculture 516:734634. doi: 10.1016/j.aquaculture.2019.734634
Godjo, A., Zadji, L., Decraemer, W., Willems, A., and Afouda, L. (2018). Pathogenicity of indigenous entomopathogenic nematodes from Benin against mango fruit fly (Bactrocera dorsalis) under laboratory conditions. Biol. Control 117, 68–77. doi: 10.1016/j.biocontrol.2017.10.009
Gozel, U., and Gozel, C. (2021). Entomopathogenic nematodes in Pest management. Integrated Pest management. Environ. Sound Pest Manag. 55:63894. doi: 10.5772/63894
Grillo, R., Fraceto, L. F., Amorim, M. J., Scott-Fordsmand, J. J., Schoonjans, R., and Chaudhry, Q. (2021). Ecotoxicological and regulatory aspects of environmental sustainability of nanopesticides. J. Hazard. Mater. 404:124148. doi: 10.1016/j.jhazmat.2020.124148
Guven, O., Aydin, T., Karaca, I., and Butt, T. (2021). Biopesticides offer an environmentally friendly solution for control of pine processionary moth (Thaumetopoea wilkinsoni tams) larvae and pupae in urban areas. Biocontrol Sci. Tech. 31, 35–52. doi: 10.1080/09583157.2020.1826905
Gwinn, K. D. (2018). Bioactive natural products in plant disease control. Studies in Natural Products Chemistry 56, 229–246. doi: 10.1016/B978-0-444-64058-1.00007-8
Hagag, E. S. (2021). Evaluation of metabolites of Myrothecium verrucaria as biological nematicide against root-knot nematode, Meloidogyne incognita in vitro and in vivo on sugar beet plants. J. Plant Protect. Pathol. 12, 47–53. doi: 10.21608/jppp.2021.52745.1007
%Hagstrum, D. W., and Flinn, P. W. (2018). “Integrated pest management” in Integrated Management of Insects in Stored Products. eds. B. Subramanyam and D. W. Hagstrum (Florida: CRC Press), 399–407.
Halder, J., Rai, A., and Kodandaram, M. (2013). Compatibility of neem oil and different entomopathogens for the management of major vegetable sucking pests. Nat Acad. Sci. Lett. 36, 19–25. doi: 10.1007/s40009-012-0091-1
%Harish, S., Murugan, M., Kannan, M., Parthasarathy, S., Prabhukarthikeyan, S., and Elango, K. (2021). “Entomopathogenic viruses” in Microbial Approaches for Insect Pest Management. ed. Omkar (New York, NY: Springer), 1–57.
Hashimi, M. H., Hashimi, R., and Ryan, Q. (2020). Toxic effects of pesticides on humans, plants, animals, pollinators and beneficial organisms. Asian Plant Res. J. 5, 37–47. doi: 10.9734/aprj/2020/v5i430114
Hicks, H. L., Comont, D., Coutts, S. R., Crook, L., Hull, R., Norris, K., et al. (2018). The factors driving evolved herbicide resistance at a national scale. Nature Ecol. Evol. 2, 529–536. doi: 10.1038/s41559-018-0470-1
Holder, D. J., and Keyhani, N. O. (2005). Adhesion of the entomopathogenic fungus Beauveria (Cordyceps) bassiana to substrata. Appl. Environ. Microbiol. 71, 5260–5266. doi: 10.1128/AEM.71.9.5260-5266.2005
Horgan, F. G., and Kudavidanage, E. P. (2020). Use and avoidance of pesticides as responses by farmers to change impacts in rice ecosystems of southern Sri Lanka. Environ. Manag. 65, 787–803. doi: 10.1007/s00267-020-01272-x
Hu, Y., Zhang, J., Kong, W., Zhao, G., and Yang, M. (2017). Mechanisms of antifungal and anti-aflatoxigenic properties of essential oil derived from turmeric (Curcuma longa L.) on aspergillus flavus. Food Chem. 220, 1–8. doi: 10.1016/j.foodchem.2016.09.179
Hummadi, E. H., Dearden, A., Generalovic, T., Clunie, B., Harrott, A., Cetin, Y., et al. (2021). Volatile organic compounds of Metarhizium brunneum influence the efficacy of entomopathogenic nematodes in insect control. Biol. Control 155:104527. doi: 10.1016/j.biocontrol.2020.104527
Husak, V. (2015). Copper and copper-containing pesticides: metabolism, toxicity and oxidative stress. J. Vasyl Stefanyk Precarp. Nat. Univ. Ser. Soc. Hum. Sci. 2, 39–51. doi: 10.15330/jpnu.2.1.38-50
Idris, H., Suryani, E., Gustia, H., and Ramadhan, A. I. J. R. I. E. (2022). The effect of various essential oil and solvent additives on the botanical pesticide of Piper aduncum essential oil on formulation antifungal activity. Results Eng. 16:100644. doi: 10.1016/j.rineng.2022.100644
Imoloame, E. O. (2021). Agronomic and economic performance of maize (Zea mays L.) as influenced by seed bed configuration and weed control treatments. Open Agriculture 6, 445–455. doi: 10.1515/opag-2021-0030
Indratin, Poniman, Sukarjo, and Helmi, M. (2021). Distribution of endosulfan insecticide residues on intensive shallot agriculture farming in Brebes regency, Indonesia. IOP Conf. Ser. Earth Environ. Sci. 648:012080. doi: 10.1088/1755-1315/648/1/012080
Jaleel, W., Saeed, Q., Saeed, S., Ansari, T., Naqqash, M. N., Iqbal, N., et al. (2015). Efficacy and time mortality of Tribolium castaneum (Herbst)(Coleoptera: Tenebrionidae) by some essential oils through contact and fumigant methods. Appl. Sci. Bus. Econom. 2, 1–7.
Jallow, M. F., Awadh, D. G., Albaho, M. S., Devi, V. Y., and Ahmad, N. (2017). Monitoring of pesticide residues in commonly used fruits and vegetables in Kuwait. Int. J. Environ. Res. Public Health 14:833. doi: 10.3390/ijerph14080833
Jayaraj, R., Megha, P., and Sreedev, P. (2017). Organochlorine pesticides their toxic effects on living organisms and their fate in the environment. Interdiscip. Toxicol. 9, 90–100. doi: 10.1515/intox-2016-0012
Jindra, M., and Bittova, L. (2020). The juvenile hormone receptor as a target of juvenoid “insect growth regulators”. Arch. Insect Biochem. Physiol. 103:e21615. doi: 10.1002/arch.21615
Jones, J. G., Korir, R. C., Walter, T. L., and Everts, K. L. (2020). Reducing chlorothalonil use in fungicide spray programs for powdery mildew, anthracnose, and gummy stem blight in melons. Plant Dis. 104, 3213–3220. doi: 10.1094/PDIS-04-20-0712-RE
Kaczmarek, A., and Boguś, M. I. (2021). Fungi of entomopathogenic potential in Chytridiomycota and Blastocladiomycota, and in fungal allies of the Oomycota and microsporidia. IMA fungus 12, 1–13. doi: 10.1186/s43008-021-00074-y
Kalpana, T., and Anil, T. (2021). A review of biopesticides and their plant phytochemicals information. Ann. Romanian Soc. Cell Biol. 25, 3576–3588.
Kanissery, R., Gairhe, B., Kadyampakeni, D., Batuman, O., and Alferez, F. (2019). Glyphosate: its environmental persistence and impact on crop health and nutrition. Plan. Theory 8:499. doi: 10.3390/plants8110499
Kaur, S., Kumar, V., Chawla, M., Cavallo, L., Poater, A., and Upadhyay, N. (2017). Pesticides curbing soil fertility: effect of complexation of free metal ions. Front. Chem. 5:43. doi: 10.3389/fchem.2017.00043
Kehinde, A. D., and Tijani, A. A. (2021). Effects of access to livelihood capitals on adoption of European Union (EU) approved pesticides among cocoa producing households in Osun state. Agri. Trop. Subtrop. 54, 57–70. doi: 10.2478/ats-2021-0007
Khati, P., Bhatt, P., Kumar, R., and Sharma, A. (2018). Effect of nanozeolite and plant growth promoting rhizobacteria on maize. Biotech 8, 1–12. doi: 10.1007/s13205-018-1142-1
Kidd, P. S., Alvarez-Lopez, V., Becerra-Castro, C., Cabello-Conejo, M., and Prieto-Fernandez, A. (2017). Potential role of plant-associated bacteria in plant metal uptake and implications in phytotechnologies. Adv. Bot. Res. 83, 87–126. doi: 10.1016/bs.abr.2016.12.004
Kim, K.-H., Kabir, E., and Jahan, S. A. (2017). Exposure to pesticides and the associated human health effects. Sci. Total Environ. 575, 525–535. doi: 10.1016/j.scitotenv.2016.09.009
Konappa, N., Krishnamurthy, S., Arakere, U. C., Chowdappa, S., Akbarbasha, R., and Ramachandrappa, N. S. (2021). “Nanofertilizers and nanopesticides: recent trends, future prospects in agriculture” in Advances in Nano-Fertilizers Nano-Pesticides in Agriculture. ed. P. Opender Koul (Amsterdam: Woodhead Publishing Series in Food Science), 281–330.
Kovendan, K., Chandramohan, B., Govindarajan, M., Jebanesan, A., Kamalakannan, S., Vincent, S., et al. (2018). Orchids as sources of novel nanoinsecticides? Efficacy of Bacillus sphaericus and Zeuxine gracilis-fabricated silver nanoparticles against dengue, malaria and filariasis mosquito vectors. J. Clust. Sci. 29, 345–357. doi: 10.1007/s10876-018-1331-4
Kumar, V., and Chandel, S. (2018). Effect of different fungicides against Podosphaera pannosa causing rose powdery mildew under greenhouse conditions. J. Crop Weed 14, 168–173.
Kumar, V., and Kumar, P. (2019). “Pesticides in agriculture and environment: impacts on human health” in Contaminants in Agriculture Environment: Health Risks Remediation, Vol. 1. eds. V. Kumar, R. Kumar, J. Singh and P. Kumar (India: ture and Environmental Science Academy), 76–95.
Kumar, R., Kumar, N., Rajput, V. D., Mandzhieva, S., Minkina, T., Saharan, B. S., et al. (2022). Advances in biopolymeric Nanopesticides: a new eco-friendly/eco-protective perspective in precision agriculture. Nano 12:3964. doi: 10.3390/nano12223964
Kumar, J., Ramlal, A., Mallick, D., and Mishra, V. (2021). An overview of some biopesticides and their importance in plant protection for commercial acceptance. Plan. Theory 10:1185. doi: 10.3390/plants10061185
Kumar, V., Singh, S., and Upadhyay, N. (2019). Effects of organophosphate pesticides on siderophore producing soils microorganisms. Biocatal. Agric. Biotechnol. 21:101359. doi: 10.1016/j.bcab.2019.101359
Kumari, S., Sharma, A., Chaudhary, P., and Khati, P. (2020). Management of plant vigor and soil health using two agriusable nanocompounds and plant growth promotory rhizobacteria in fenugreek. Biotech 10:461. doi: 10.1007/s13205-020-02448-2
Lai, Y., Chen, H., Wei, G., Wang, G., Li, F., and Wang, S. (2017). In vivo gene expression profiling of the entomopathogenic fungus Beauveria bassiana elucidates its infection stratagems in Anopheles mosquito. Sci. China Life Sci. 60, 839–851. doi: 10.1007/s11427-017-9101-3
Lang, G., and Buchbauer, G. (2012). A review on recent research results (2008–2010) on essential oils as antimicrobials and antifungals. A review. Flavour Fragr. J. 27, 13–39. doi: 10.1002/ffj.2082
Lasso-Robledo, J. L., Torres, B., and Peralta-Videa, J. R. (2022). Do all cu nanoparticles have similar applications in nano-enabled agriculture? Plant Nano Biol. 1:100006. doi: 10.1016/j.plana.2022.100006
Lee, Y. K., Jin, N. Y., Kim, H. J., Yu, Y. M., Yasunaga-Aoki, C., and Youn, Y. N. (2021). Investigation and characteristics of novel biological control agents for controlling dipteran pests. J. Faculty Agric. 66, 183–190.
Lengai, G. M., Muthomi, J. W., and Mbega, E. R. (2020). Phytochemical activity and role of botanical pesticides in pest management for sustainable agricultural crop production. Sci. Afric. 7:e00239. doi: 10.1016/j.sciaf.2019.e00239
Li, Z., Xiong, J., and Fantke, P. (2022). Screening of pesticide distributions in foods of animal origin: a matrix-based approach for biotransfer factor modeling of grazing mammals. Environ Sci Process Impacts 24, 609–624. doi: 10.1039/d1em00454a
Llamas, B., Suarez-Rodriguez, M. C., Gonzalez-Lopez, C. V., Mora, P., and Acien, F. G. (2021). Techno-economic analysis of microalgae related processes for CO2 bio-fixation. Algal Res. 57:102339. doi: 10.1016/j.algal.2021.102339
Loddo, D., Scarabel, L., Sattin, M., Pederzoli, A., Morsiani, C., Canestrale, R., et al. (2019). Combination of herbicide band application and inter-row cultivation provides sustainable weed control in maize. Agronomy 10:20. doi: 10.3390/agronomy10010020
Lourenço, A. M., Haddi, K., Ribeiro, B. M., Corrêia, R. F., Tomé, H. V., Santos-Amaya, O., et al. (2018). Essential oil of Siparuna guianensis as an alternative tool for improved lepidopteran control and resistance management practices. Sci. Rep. 8, 1–13. doi: 10.1038/s41598-018-25721-0
Lovatto, P. B., Goetze, M., and Thomé, G. C. H. (2004). Extracts effect of wild plants of the Solanaceae family on Brevicoryne brassicae control in cabbage (Brassica oleracea var. acephala). Cienc. Rural 34, 971–978. doi: 10.1590/S0103-84782004000400001
Malahlela, M., Thibane, V. S., and Mudau, F. N. (2021). Nematocidal activity of fermented extracts from Lantana camara plant parts against Meloidogyne javanica on tomato. Int. J. Veg. Sci. 27, 20–28. doi: 10.1080/19315260.2019.1697981
Manfo, F. P. T., Mboe, S. A., Nantia, E. A., Ngoula, F., Telefo, P. B., Moundipa, P. F., et al. (2020). Evaluation of the effects of agro pesticides use on liver and kidney function in farmers from Buea. J. Toxicol. 2020:2305764. doi: 10.1155/2020/2305764
Matsuda, K., Ihara, M., and Sattelle, D. B. (2020). Neonicotinoid insecticides: molecular targets, resistance, and toxicity. Annu. Rev. Pharmacol. Toxicol. 60, 241–255. doi: 10.1146/annurev-pharmtox-010818-021747
Maurer, M., Kerns, D., and Tellez, T. (2018). Citrus Peel miner Marmara salictella monitoring techniques and control measures 1996-971. Citrus Decid. Fruit Nut Res. Rep. 1, 1–8.
McGuire, A. V., and Northfield, T. D. (2020). Tropical occurrence and agricultural importance of Beauveria bassiana and Metarhizium anisopliae. Front. Sustain. Food Syst. 4:6. doi: 10.3389/fsufs.2020.00006
Mkenda, P., Mwanauta, R., Stevenson, P. C., Ndakidemi, P., Mtei, K., and Belmain, S. R. (2015). Extracts from field margin weeds provide economically viable and environmentally benign pest control compared to synthetic pesticides. PLoS One 10:e0143530. doi: 10.1371/journal.pone.0143530
Moreno-Gavíra, A., Huertas, V., Diánez, F., Sánchez-Montesinos, B., and Santos, M. (2020). Paecilomyces and its importance in the biological control of agricultural pests and diseases. Plan. Theory 9:1746. doi: 10.3390/plants9121746
Mouyna, I., Hartl, L., and Latgé, J.-P. (2013). β-1, 3-glucan modifying enzymes in aspergillus fumigatus. Front. Microbiol. 4:81. doi: 10.3389/fmicb.2013.00081
Murcia-Morales, M., Díaz-Galiano, F. J., Guitérrez-Tirado, I., Flores, J. M., Van der Steen, J. J., and Fernández-Alba, A. R. (2021). Dissipation and cross-contamination of miticides in apiculture. Evaluation by APIStrip-based sampling. Chemosphere 280:130783. doi: 10.1016/j.chemosphere.2021.130783
%Narware, J., Yadav, R., Keswani, C., Singh, S., and Singh, H. (2019). “Silver nanoparticle-based biopesticides for phytopathogens: scope and potential in agriculture” in Nano-Biopesticides Today and Future Perspectives. ed. K. Opender (Amsterdam: Elsevier), 303–314.
Ojuederie, O. B., Chukwuneme, C. F., Samuel, O., Olanrewaju, M. A., Adegboyega, T. T., and Babalola, O. O. (2021). Contribution of microbial inoculants in sustainable maintenance of human health, including test methods and evaluation of safety of microbial pesticide microorganisms. Biopestic. Bot. Microorgan. Improv. Agric. Hum. Health 1, 207–240.
Okoroiwu, H. U., and Iwara, I. A. (2018). Dichlorvos toxicity: a public health perspective. Interdiscip. Toxicol. 11:129. doi: 10.2478/intox-2018-0009
Omole, R. K., Torimiro, N., Alayande, S. O., and Ajenifuja, E. (2018). Silver nanoparticles synthesized from Bacillus subtilis for detection of deterioration in the post-harvest spoilage of fruit. Sustain. Chem. Pharm. 10, 33–40. doi: 10.1016/j.scp.2018.08.005
Omoyajowo, K., Njok, K., Amiolemen, S., Ogidan, J., Adenekan, O., Olaniyan, K., et al. (2018). Assessment of pesticide residue levels in common fruits consumed in Lagos state, Nigeria. LASU J. Res. Rev. Sci. 2018, 56–62.
Onwujiogu, V. C., Abugu, H. O., and Okoye, C. O. (2022). Health risk assessment of pesticide residue in freshly harvested Vigna subtserranea (Bambara nut) in Nsukka south eastern Nigeria. Environ. Monit. Assess. 194, 1–15. doi: 10.1007/s10661-022-10201-w
Opeyemi, B. S., Temidayo, B. R., Babalola, Y. O., Emmanuel, I. B., Ojubolamo, M. T., and Folake, A. B. (2018). Biological control of anthracnose disease of tomato using ethanolic extracts of Azadirachta indica and Nicotiana tabacum. Int. Ann. Sci. 4, 20–26. doi: 10.21467/ias.4.1.20-26
Ortiz-Urquiza, A., and Keyhani, N. (2016). Molecular genetics of Beauveria bassiana infection of insects. Adv. Genet. 94, 165–249. doi: 10.1016/bs.adgen.2015.11.003
Oso, A., and Awe, G. (2019). Effects of different water regimes and spacing on insect pest infestation and efficacy of control measures in cucumber (Cucumis sativus). J. Exp. Agric. Int. 41, 1–8. doi: 10.9734/jeai/2019/v41i530415
Pan, X., Guo, X., Zhai, T., Zhang, D., Rao, W., Cao, F., et al. (2023). Nanobiopesticides in sustainable agriculture: developments, challenges, and perspectives. Environ. Sci. Nano. 10, 41–61. doi: 10.1039/D2EN00605G
Pang, Y.-P., Brimijoin, S., Ragsdale, D. W., Yan Zhu, K., and Suranyi, R. (2012). Novel and viable acetylcholinesterase target site for developing effective and environmentally safe insecticides. Curr. Drug Targets 13, 471–482. doi: 10.2174/138945012799499703
Parker, C. T., and Sperandio, V. (2009). Cell‐to‐cell signalling during pathogenesis. Cellular Microbiology 11, 363–369. doi: 10.1111/j.1462-5822.2008.01272.x
%Pathak, D., Yadav, R., and Kumar, M. (2017). “Microbial pesticides: development, prospects and popularization in India” in Plant-microbe interactions in agro-ecological perspectives. eds. D. Singh, H. Singh and R. Prabha (US: Springer), 455–471.
Pathania, A., Dutta, J., and Mhatre, P. H. (2022). In-vitro efficacy of Verticillium lecanii (Zimm.) Viegas against Estonian cyst nematode, Cactodera estonica. Indian Phytopathol. 75, 1167–1171. doi: 10.1007/s42360-022-00526-4
Pelosi, C., Bertrand, C., Daniele, G., Coeurdassier, M., Benoit, P., Nélieu, S., et al. (2021). Residues of currently used pesticides in soils and earthworms: a silent threat? Agric. Ecosyst. Environ. 305:107167. doi: 10.1016/j.agee.2020.107167
Peprah-Yamoah, E., Afrifa-Yamoah, E., Ofori, H., and Adua, E. (2022). Antifeedant potency of Mentha spicata aqueous extracts against fall armyworm (Spodoptera frugiperda). Res. Square 1, 1–15. doi: 10.21203/rs.3.rs-1314042/v1
Pereira, S. P., Santos, S. M., Fernandes, M. A., Deus, C. M., Martins, J. D., de Lima, M. C. P., et al. (2021). Improving pollutants environmental risk assessment using a multi model toxicity determination with in vitro, bacterial, animal and plant model systems: the case of the herbicide alachlor. Environ. Pollut. 286, 117239–117211. doi: 10.1016/j.envpol.2021.117239
Pertile, M., Antunes, J. E. L., Araujo, F. F., Mendes, L. W., Van den Brink, P. J., and Araujo, A. S. F. (2020). Responses of soil microbial biomass and enzyme activity to herbicides imazethapyr and flumioxazin. Sci. Rep. 10, 1–9. doi: 10.1038/s41598-020-64648-3
Polsinelli, G. A., Singh, S. K., Mishra, R. K., Suranyi, R., Ragsdale, D. W., Pang, Y.-P., et al. (2010). Insect-specific irreversible inhibitors of acetylcholinesterase in pests including the bed bug, the eastern yellowjacket, German and American cockroaches, and the confused flour beetle. Chem. Biol. Interact. 187, 142–147. doi: 10.1016/j.cbi.2010.01.036
Priya, S., Sarvendra, K., Vinod, K.S., and Kapil, A.C. (2018). Nanopesticides: Manage food security and environmental pollution. Available at: https://www.biotecharticles.com/Agriculture-Article/Nanopesticides-Manage-Food-Security-and-Environmental-Pollution-4391.html (Accessed April 10, 2022).
Rabelo, M. M., Paula-Moraes, S. V., Pereira, E. J. G., and Siegfried, B. D. (2020). Contrasting susceptibility of lepidopteran pests to diamide and pyrethroid insecticides in a region of overwintering and migratory intersection. Pest Manag. Sci. 76, 4240–4247. doi: 10.1002/ps.5984
Rajna, S., and Paschapur, A. (2019). Nanopesticides: its scope and utility in pest management. Indian Farmer 6, 17–21.
%Rakhimol, K., Thomas, S., Volova, T., and Jayachandran, K. (2020). Controlled Release of Pesticides for Sustainable Agriculture. New York, NY: Springer.
Rodrigues, D., Costa-Pinto, A. R., Sousa, S., Vasconcelos, M. W., Pintado, M. M., Pereira, L., et al. (2019). Sargassum muticum and Osmundea pinnatifida enzymatic extracts: chemical, structural, and cytotoxic characterization. Mar. Drugs 17:209. doi: 10.3390/md17040209
Roseline, T. A., Murugan, M., Sudhakar, M., and Arunkumar, K. (2019). Nanopesticidal potential of silver nanocomposites synthesized from the aqueous extracts of red seaweeds. Environ. Technol. Innov. 13, 82–93. doi: 10.1016/j.eti.2018.10.005
Ruiu, L. (2018). Microbial biopesticides in agroecosystems. Agronomy 8:235. doi: 10.3390/agronomy8110235
Sabarwal, A., Kumar, K., and Singh, R. P. (2018). Hazardous effects of chemical pesticides on human health–cancer and other associated disorders. Environ. Toxicol. Pharmacol. 63, 103–114. doi: 10.1016/j.etap.2018.08.018
Saberi, F., Marzban, R., Ardjmand, M., Shariati, F. P., and Tavakoli, O. (2020). Optimization of culture media to enhance the ability of local bacillus thuringiensis var. tenebrionis. Journal of the Saudi society of. Agric. Sci. 19, 468–475. doi: 10.1016/j.jssas.2020.08.004
Sabnam, N., and Barman, S. R. (2017). WISH, a novel CFEM GPCR is indispensable for surface sensing, asexual and pathogenic differentiation in rice blast fungus. Fungal Genet. Biol. 105, 37–51. doi: 10.1016/j.fgb.2017.05.006
Sabry, A.-K. H. (2020). Role of nanopesticides in agricultural development. Intellect. Prop. Issues Nanotechnol. 2020, 107–120. doi: 10.1201/9781003052104-7
Saleh, T. A. (2020). Trends in the sample preparation and analysis of nanomaterials as environmental contaminants. Trends Environ. Analy. Chem. 28:e00101. doi: 10.1016/j.teac.2020.e00101
Samada, L. H., and Tambunan, U. S. F. (2020). Biopesticides as promising alternatives to chemical pesticides: a review of their current and future status. Online J. Biol. Sci. 20, 66–76. doi: 10.3844/ojbsci.2020.66.76
Santi, L., Coutinho-Rodrigues, C. J., Berger, M., Klein, L. A., De Souza, E. M., Rosa, R. L., et al. (2019). Secretomic analysis of Beauveria bassiana related to cattle tick, Rhipicephalus microplus, infection. Folia Microbiol. 64, 361–372. doi: 10.1007/s12223-018-0659-3
Saranya, S., Ramaraju, K., and Jeyarani, S. (2021). Ovicidal action of different fungal pathogens against two spotted spider mite, Tetranychus urticae (Koch) under laboratory conditions. J. Biol. Control. 35, 37–40. doi: 10.18311/jbc/2021/27410
Sardar, M., Ahmed, W., Al Ayoubi, S., Nisa, S., Bibi, Y., Sabir, M., et al. (2022). Fungicidal synergistic effect of biogenically synthesized zinc oxide and copper oxide nanoparticles against Alternaria citri causing citrus black rot disease. Saudi J. Biol. Sci. 29, 88–95. doi: 10.1016/j.sjbs.2021.08.067
Sari, A., and Lubis, L. (2021). The effectiveness of contact fungicides mancozeb in controlling potato leaf blight disease (Phytophthora infestans (Mont) de Barry) in Karo District in the wet month and in the laboratory. IOP Conf. Ser. IOP Publ. Earth Environ. Sci. 782:042022. doi: 10.1088/1755-1315/782/4/042022
Scariot, F. J., Delamare, A. P. L., and Echeverrigaray, S. (2022). The effect of chlorothalonil on Saccharomyces cerevisiae under alcoholic fermentation. Pestic. Biochem. Physiol. 182, 1–8. doi: 10.1016/j.pestbp.2021.105032
%Singh, A., Bhardwaj, R., and Singh, I. K. (2019). “Biocontrol agents: potential of biopesticides for integrated pest management” in Biofertilizers for Sustainable Agriculture and Environment. eds. B. Giri, R. Prasad, Q. S. Wu and A. Varma (New York, NY: Springer), 413–433.
Singh, S., Bhuva, B. V., Solanki, V. H., Patel, K. N., and Patel, K. (2020). Persistence and downward movement of four termiticides in three representative soils of Gujarat under laboratory conditions. J. Entomol. Zool. Studi. 8, 1803–1811.
Singh, P., Prakash, B., and Dubey, N. (2014). Insecticidal activity of Ageratum conyzoides L., Coleus aromaticus Benth. And Hyptis suaveolens (L.) Poit essential oils as fumigant against storage grain insect Tribolium castaneum Herbst. J. Food Sci. Technol. 51, 2210–2215. doi: 10.1007/s13197-012-0698-8
Singh, D., Raina, T. K., and Singh, J. (2017). Entomopathogenic fungi: an effective biocontrol agent for management of insect populations naturally. J. Pharm. Sci. 9:833.
Souto, A. L., Sylvestre, M., Tölke, E. D., Tavares, J. F., Barbosa-Filho, J. M., and Cebrián-Torrejón, G. (2021). Plant-derived pesticides as an alternative to pest management and sustainable agricultural production: prospects, applications and challenges. Molecules 26:4835. doi: 10.3390/molecules26164835
Sparks, T. C., and Nauen, R. (2015). IRAC: Mode of action classification and insecticide resistance management. Pest. Biochem. Physiol. 121, 122–128. doi: 10.1016/j.pestbp.2014.11.014
Stevenson, P. C., Isman, M. B., and Belmain, S. R. (2017). Pesticidal plants in Africa: A global vision of new biological control products from local uses. Industrial Crops and Products 110, 2–9. doi: 10.1016/j.indcrop.2017.08.034
Sumekar, Y., Widayat, D., Umiyati, U., and Fitri, A. (2022). Persistence and effectiveness of atrazine herbicide in sweet corn (Zea mays saccharata Sturt) cultivation with different organic ingredients in doses. Research square 1, 1–13. doi: 10.21203/rs.3.rs-1677256/v1
Surma, M., Sadowska-Rociek, A., and Cieślik, E. (2021). Application of quechers method for the determination of phenylurea herbicides in beetroot by HPLC with uv-Vis detection. J. Microbiol. Biotechnol. Food Sci. 2021, 145–147. doi: 10.15414/jmbfs.2015.4.special3.145-147
Svidritskiy, E., Ling, C., Ermolenko, D. N., and Korostelev, A. A. (2013). Blasticidin S inhibits translation by trapping deformed tRNA on the ribosome. Proceedings of the National Academy of Sciences 110, 12283–12288. doi: 10.1073/pnas.1304922110
Syafrudin, M., Kristanti, R. A., Yuniarto, A., Hadibarata, T., Rhee, J., Al-Onazi, W. A., et al. (2021). Pesticides in drinking water—a review. Int. J. Environ. Res. Public Health 18:468. doi: 10.3390/ijerph18020468
Syromyatnikov, M. Y., Isuwa, M. M., Savinkova, O. V., Derevshchikova, M. I., and Popov, V. N. (2020). The effect of pesticides on the microbiome of animals. Agriculture 10, 1–14. doi: 10.3390/agriculture10030079
Tartar, A., Shapiro, A. M., Scharf, D. W., and Boucias, D. G. (2005). Differential expression of chitin synthase (CHS) and glucan synthase (FKS) genes correlates with the formation of a modified, thinner cell wall in in vivo-produced Beauveria bassiana cells. Mycopathologia 160, 303–314. doi: 10.1007/s11046-005-0134-y
Tongnuanchan, P., and Benjakul, S. (2014). Essential oils: extraction, bioactivities, and their uses for food preservation. J. Food Sci. 79, R1231–R1249. doi: 10.1111/1750-3841.12492
Tongo, I., Onokpasa, A., Emerure, F., Balogun, P., Enuneku, A., Erhunmwunse, N., et al. (2022). Levels, bioaccumulation and biomagnification of pesticide residues in a tropical freshwater food web. Int. J. Environ. Sci. Technol. 19, 1467–1482. doi: 10.1007/s13762-021-03212-6
Tripathi, A., Upadhyay, S., Bhuiyan, M., and Bhattacharya, P. (2009). A review on prospects of essential oils as biopesticide in insect-pest management. Pharmacog. Phytotherap. 1, 052–063. doi: 10.5897/JPP.9000003
Tsufac, A. R., Awazi, N. P., and Yerima, B. P. K. (2020). Determinants and policy ramifications of cocoa farmers’ use of agrochemicals in cocoa-based (Theobroma cacao) agroforestry systems in Cameroon. J. Exp. Agric. Int. 42, 26–37. doi: 10.9734/jeai/2020/v42i1030611
%Ujvary, I. (2010). “Pest control agents from natural products” in Hayes' Handbook of Pesticide Toxicology. ed. B. T. Ikem (Massachusetts: Elsevier), 119–229.
Ullah, M. R., and Dijkstra, F. A. (2019). Fungicide and bactericide effects on carbon and nitrogen cycling in soils: a meta-analysis. Soil Systems 3:23. doi: 10.3390/soilsystems3020023
Upadhyay, N., Dwivedy, A. K., Kumar, M., Prakash, B., and Dubey, N. K. (2018). Essential oils as eco-friendly alternatives to synthetic pesticides for the control of Tribolium castaneum (Herbst)(Coleoptera: Tenebrionidae). J. Essent. Oil Bear. Plants 21, 282–297. doi: 10.1080/0972060X.2018.1459875
Wang, C., and Leger, R. J. S. (2007). The Metarhizium anisopliae perilipin homolog MPL1 regulates lipid metabolism, appressorial turgor pressure, and virulence. J. Biol. Chem. 282, 21110–21115. doi: 10.1074/jbc.M609592200
Wang, C., and St Leger, R. (2007). The MAD1 adhesin of Metarhizium anisopliae links adhesion with blastospore production and virulence to insects, and the MAD2 adhesin enables attachment to plants. Eukaryot. Cell 6, 808–816. doi: 10.1128/EC.00409-06
Wojda, I., Kowalski, P., and Jakubowicz, T. (2009). Humoral immune response of galleria mellonella larvae after infection by Beauveria bassiana under optimal and heat-shock conditions. J. Insect Physiol. 55, 525–531. doi: 10.1016/j.jinsphys.2009.01.014
Xiao, Y., and Wu, K. (2019). Recent progress on the interaction between insects and bacillus thuringiensis crops. Philos. Trans. R. Soc. B 374:20180316. doi: 10.1098/rstb.2018.0316
Yadav, R. K., Singh, N., Singh, A., Yadav, V., Bano, C., and Khare, S. (2020). Expanding the horizons of nanotechnology in agriculture: recent advances, challenges and future perspectives. Vegetos 33, 203–221. doi: 10.1007/s42535-019-00090-9
Zaki, O., Weekers, F., Thonart, P., Tesch, E., Kuenemann, P., and Jacques, P. (2020). Limiting factors of mycopesticide development. Biol. Control 144:104220. doi: 10.1016/j.biocontrol.2020.104220
Zheng, N., Ding, N., Gao, P., Han, M., Liu, X., Wang, J., et al. (2018). Diverse algicidal bacteria associated with harmful bloom-forming Karenia mikimotoi in estuarine soil and seawater. Sci. Total Environ. 631, 1415–1420. doi: 10.1016/j.scitotenv.2018.03.035
Keywords: nanoparticles, biopesticides, synthetic pesticides, soil health, pesticides
Citation: Ayilara MS, Adeleke BS, Akinola SA, Fayose CA, Adeyemi UT, Gbadegesin LA, Omole RK, Johnson RM, Uthman QO and Babalola OO (2023) Biopesticides as a promising alternative to synthetic pesticides: A case for microbial pesticides, phytopesticides, and nanobiopesticides. Front. Microbiol. 14:1040901. doi: 10.3389/fmicb.2023.1040901
Received: 09 September 2022; Accepted: 17 January 2023;
Published: 16 February 2023.
Edited by:
Parul Chaudhary, National Dairy Research Institute (ICAR), IndiaReviewed by:
Remember Roger Adjei, University of Energy and Natural Resources, GhanaCopyright © 2023 Ayilara, Adeleke, Akinola, Fayose, Adeyemi, Gbadegesin, Omole, Johnson, Uthman and Babalola. This is an open-access article distributed under the terms of the Creative Commons Attribution License (CC BY). The use, distribution or reproduction in other forums is permitted, provided the original author(s) and the copyright owner(s) are credited and that the original publication in this journal is cited, in accordance with accepted academic practice. No use, distribution or reproduction is permitted which does not comply with these terms.
*Correspondence: Olubukola O. Babalola, ✉ b2x1YnVrb2xhLmJhYmFsb2xhQG53dS5hYy56YQ==
Disclaimer: All claims expressed in this article are solely those of the authors and do not necessarily represent those of their affiliated organizations, or those of the publisher, the editors and the reviewers. Any product that may be evaluated in this article or claim that may be made by its manufacturer is not guaranteed or endorsed by the publisher.
Research integrity at Frontiers
Learn more about the work of our research integrity team to safeguard the quality of each article we publish.