- 1School of Grassland Science, Beijing Forestry University, Beijing, China
- 2College of Resources and Environment/International Magnesium Institute, Fujian Agriculture and Forestry University, Fuzhou, China
The riparian zone is an important ecological corridor connecting the upstream and downstream rivers. Its highly complex biological and physical environments significantly affect the biogeographical pattern of species and various ecosystem functions. However, in alpine riparian ecosystems, the distribution patterns and drivers of arbuscular mycorrhizal (AM) fungi, a group of functionally important root-associated microorganisms, remain poorly understood. In this study, we investigated the AM fungal diversity and community composition in near-bank (wetland) and far-bank (alpine meadows) soils along the Niaqu River in the Nam Co watershed, and assessed the relative importance of abiotic and biotic filtering in shaping these distributions. Overall, 184 OTUs were identified in the riparian ecosystem, predominantly belonging to the genus Glomus, especially in the downstream soils, and Claroideoglomus in near-bank soils. AM fungal colonization, spore density, and α diversity showed an overall increasing trend along the river, while the extraradical hyphae declined dramatically from the middle of the river. AM fungal communities significantly varied between the wetland and alpine meadows in the riparian zone, mainly driven by the geographic distance, soil water content, soil pH, and plant communities. Specifically, soil pH was the principal predictor of AM fungal community in near-bank wetland soils, while soil water content had a most substantial direct effect in alpine meadows. These findings indicate that abiotic factors are the most important divers in shaping AM fungal communities at the watershed scale, which could be helpful in alpine riparian biodiversity conservation and management.
Introduction
Arbuscular mycorrhizal (AM) fungi are widespread root-associated microorganisms and functionally important (Smith and Read, 2008). Generally, AM fungi can provide the plant with soil phosphorus and nitrogen in exchange for carbohydrates (van der Heijden et al., 2015), and improves the host plant’s resistance to drought (Gruemberg et al., 2015), heavy metals (Almeida-Rodriguez et al., 2016) and pathogens (Sikes et al., 2009; Mirshad and Puthur, 2016; Miozzi et al., 2019). There is increasing evidence that mycorrhizas are essential for regulating belowground biogeochemical cycling, soil structure, plant diversity and productivity, and ecosystem function (Van and Klironomos, 1998; Bender et al., 2015; Powell and Rillig, 2018). These mutual benefits of AM fungi are generally considered to be largely dependent on their community composition, which is mainly structured by abiotic and biotic filtering (Hempel, 2018). Therefore, the in-depth exploration of AM fungal community dynamics and their drivers is crucial if we are to manipulate these beneficial organisms to enhance ecological restoration, especially in the alpine ecosystem (Coban et al., 2022).
In recent years, with the development and application of high-throughput sequencing techniques, the development of AM fungi biogeography research has been comprehensively promoted. A meta-analysis of sequences from the global AM fungal database showed that geographic distance, soil temperature, moisture, and plant communities significantly affect the AM fungal community structure (Kivlin et al., 2011). Lekberg and Waller (2016) found that, on a global scale, plant species identity may not be more important for local AM fungal community assembly than other factors, such as environmental conditions, fungal interactions, and even random dispersal. At the landscape scale, AM fungal community composition was mainly influenced by local abiotic factors (e.g., pH, rainfall, and soil type) (Hazard et al., 2013), or land use and geographic distance (Zhu et al., 2020; Yang et al., 2021). In four typical grassland ecosystems of Sanjiangyuan National Park, we found that soil water content and plant community composition are the key factors in determining AM fungal community (Li et al., 2021a). Together, these studies suggest that both abiotic and biotic factors had strong effects on AM fungal community assembly. However, most of the related studies targeted the typical terrestrial ecosystems, and the biogeographical patterns of AM fungal communities in riparian ecosystems remain poorly understood (Li et al., 2021b).
The riparian ecosystem is an ecological transition zone between the river and the terrestrial ecosystems and provides various ecosystem services (Gonzalez et al., 2017). It is potentially a susceptible zone for interacting with biomes and environmental factors (Larsen et al., 2016). Vegetation and soils are the important components of riparian ecosystems, carrying various ecosystem service functions. For example, riparian soils intercept water resources and nutrients in rivers by regulating plant resource acquisition, soil conservation, and microbial fixation or slowly release downstream through hydrological processes, thereby forming natural nutrient and water gradients (Mitsch and Gosselink, 2000). Nevertheless, most of the current research on riparian zones has focused on the above-ground plant community composition and their distribution patterns (Acker et al., 2003; Bosompemaa et al., 2021; Qin et al., 2021). This unique natural environment also provides suitable conditions for microbial biogeography research and helps answer fundamental scientific questions in microbial ecology, such as the spatial distribution pattern of microbial communities and their maintaining mechanism. Moreover, it is also helpful for fully understanding and utilizing riparian microbial resources and further comprehending the functioning of riparian ecosystems.
Qinghai-Tibet Plateau (QTP), known as the Asian Water Tower, is dotted with numerous glaciers and lakes and is the source of large rivers in Asia (Immerzeel et al., 2010). Currently, there are 12 large watersheds on the QTP, each composed of many small watersheds with independent hydrological processes. The small watersheds with an area of 40-500 km2 account for about 73% of the total watershed area and about 95% of the total watershed number (unpublished data). In the ubiquitous alpine small watershed, different ecosystems (grassland, meadow, wetland, etc.) from the ice edge to the estuary are connected by the water resource and material cycling driven by the hydrological process, forming a continuous riparian ecosystem. Our previous studies found that AM fungal distribution pattern and their symbiosis with host plants are related to annual precipitation (Zhang et al., 2016, 2019). But it should be noted that the precipitation gradient of the QTP is different from that of the riparian zone within a small watershed. Besides natural precipitation, the riparian zone also includes the water flowing from rivers fed by glacial meltwater and underground water. Whenever the river flow increases during the monsoon period (Yu et al., 2019), the AM fungal propagules on the riparian zone may be dispersed into the sediments and riparian soils on the river bank or downstream by flood shocks (Harner et al., 2011), thereby affecting the distribution of AM fungi. The hydrological processes in the alpine watershed are active, and the material turnover rate is fast and continuous, so the different locations and types of ecosystems in the riparian zone are not completely independent. Therefore, the rules associated with static soil environments may not apply to riparian continuum environments (Read et al., 2015). However, AM fungal population and community dynamics in riparian along the alpine watershed are not yet explicit.
In this study, riparian soils were collected along the Niaqu river, a representative area of the small alpine watershed in QTP (Yu et al., 2019), to reveal the AM fungal distribution and the role of the local environment and geographical distance in determining community composition. To account for the vertical and horizontal dimensions of these small watersheds, samples were taken from 6 sites along the 35-km riparian zones near and away from the river, respectively. We hypothesized that; H1, AM fungal propagules, i.e., spores and extraradical hyphae, and α diversity would increase along the river from upstream to downstream, especially near-bank due to the water connectivity. H2, environmental factors, especially the heterogeneity of soil moisture caused by distance from the river, are the more important factors in structuring the fungal communities at the small watershed scale.
Materials and methods
Study site and sampling design
Nam Co (30°30′∼30°55′N, 90°16′∼91°03′) is located at the northern foot of the Nyenchen Tanggula Mountains, with an area of 2,020 km2 and an average elevation of 4,718 m. It is the highest saltwater lake in the world (Anslan et al., 2020). The Nam Co basin is surrounded by high mountains and hills, forming a well-closed internal flow area. The lake water is supplied by the glacial meltwater and many small or large streams along the lake, forming many small watersheds (Zhu et al., 2010). Niaqu river is located on the east bank of the Nam Co. This area is characterized by high terrain, cold climate, and large temperature difference between day and night. A total of 12 sampling sites were set up, i.e., near-bank and far-bank along the river water flow direction (Figure 1). The vegetation types are divided into wetland (close to the river) and alpine meadows (about 250 m away from the river). The wetland is dominated by Kobresia tibetica, Kobresia pygmaea, and Polygonum viviparum, and the alpine meadows are dominated by Kobresia pygmaea, Carex moorcroftii, and Leontopodium pusillum.
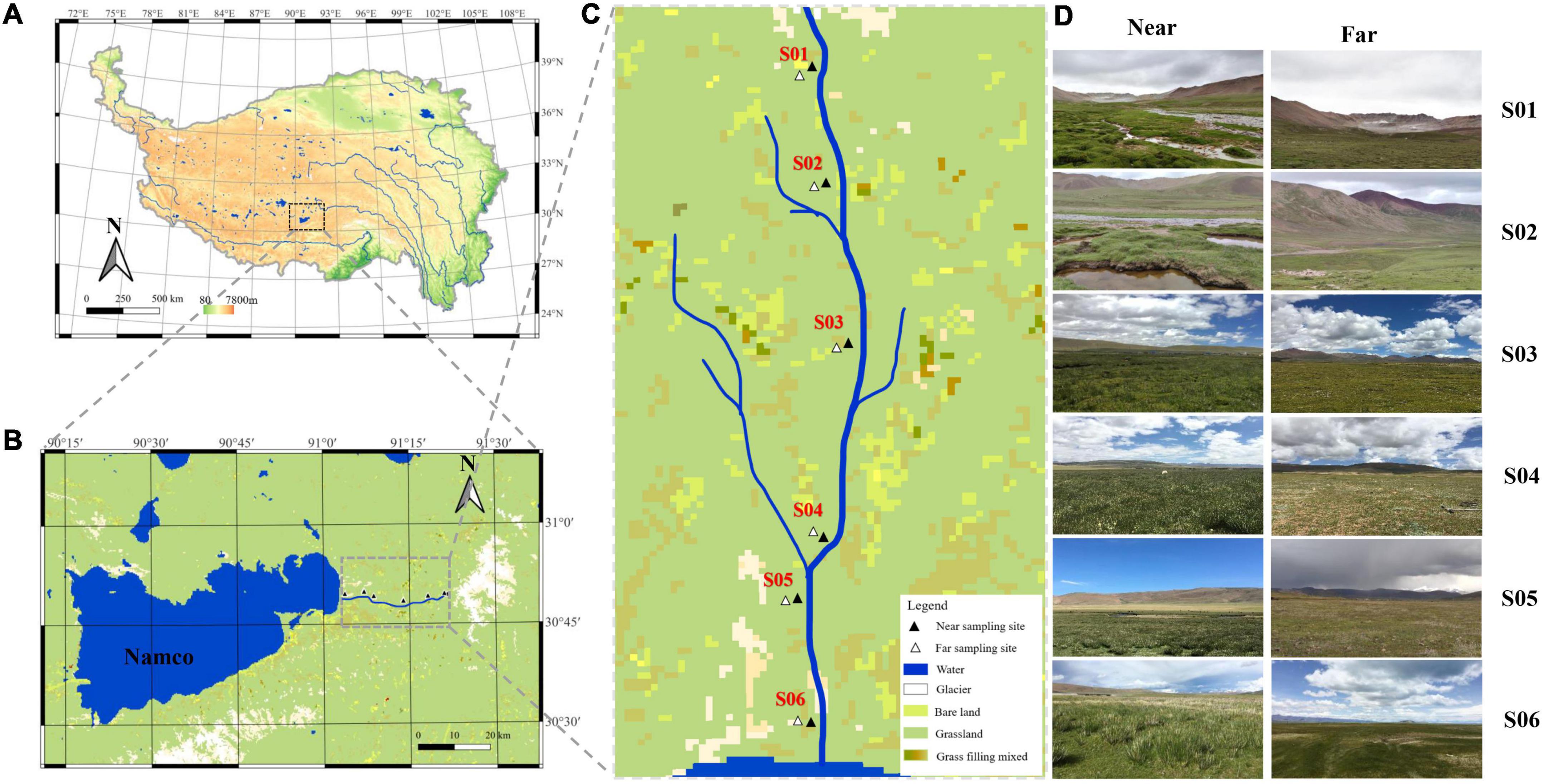
Figure 1. Maps showing locations of the sampling sites: (A) Location of Nam Co in the Qinghai-Tibet Plateau. (B) Map of a panoramic view of the Nam Co area marked with Niyaquriver. (C) Map of a magnified view of the Niyaqu basin with the six sampling sites labeled as S01–S06 along the river. (D) View of the sampling sites along the Niyaquriver in the Nam Co area.
By the end of August 2019, three 50 × 50 cm quadrats, with a separation distance of not less than 10 m, were randomly selected at each sample site. In each quadrat, the aboveground biomass was calculated by collecting all plant shoot and oven-dried at 80°C to a constant weight. Three soil cores (0-20 cm depth; 7 cm in diameter) were randomly collected and pooled into one sample. All the soil was passed through a 2 mm sieve in situ, and the fine living roots were first picked out. All samples were stored at 4°C and transported to the laboratory quickly for subsequent analysis. The living roots were thoroughly mixed and used for the determination of AM fungal colonization. The sieved soil was divided into two sub-samples (about 100 g each). The sub-sample 1 was stored at −20°C for the analysis of DNA extraction, available phosphorus, ammonium nitrogen, and nitrate nitrogen. The sub-sample 2 was air-dried and kept for later use analysis of soil physicochemical properties, such as mycelium density, spore density, pH, soil organic carbon, soil total nitrogen, and soil total phosphorus.
Determination of soil physicochemical properties
Soil water content (SWC) was determined by drying soil samples at 105°C for 24 h. Soil pH value was detected with a soil suspension, a soil-water ratio of 1:2.5 (w/v), and a composite glass electrode (Lu, 1999). Soil organic carbon (SOC) was measured by the potassium dichromate external heating method (Nelson and Sommers, 1996). The Kjeldahl method determined total nitrogen (TN) (Bremner and Mulvaney, 1982). Ammonium nitrogen (NH4+) and nitrate-nitrogen (NO3–) were both extracted with 2 mol ⋅ L–1 KCl solution and then measured by flow analyzer (TRAACS 2000) (Lu, 1999). Soil total phosphorus (TP) and available phosphorus (AP) were, respectively, extracted with KClO4-H2SO4 and NaHCO3, and determined by the molybdenum-antimony colorimetric method (Bray and Kurtz, 1945; Olsen, 1954).
Analyses of arbuscular mycorrhizal fungal colonization, spores, and extraradical hyphae
Roots were washed carefully with tap water and cut into segments of 1 cm in length. Approximately 100 root segments were randomly chosen and cleared in 10% KOH at 90 °C and stained with 0.05% Trypan blue. Roots segments were randomly selected and quantified for the percent root length of AM fungal colonization (% RLC) using the magnified intersection method at 200 × magnification (Mcgonigle et al., 1990; Brundrett et al., 1994). Spores of AM fungi were separated from 20 g of dry soil in each soil sample by wet sieving and sucrose centrifugation (Brundrett et al., 1994). The spore density was counted using a dissecting microscope. Extraradical hyphae of AM fungi in each dry soil sample were extracted by the membrane filter technique and stained with Trypan blue following the protocols of Brundrett et al. (1994) and Miller et al. (1995). Hyphal length density (HLD) (m ⋅ g–1 dry soil) was calculated using a line intersection method (Brundrett et al., 1994).
DNA extraction and PCR
DNA was extracted from 0.5 g of soil samples (fresh weight) using the PowerSoil® DNA Isolation Kit (MoBio Laboratories, Inc., Carlsbad, CA, USA) according to the instructions of the manufacturer. The quality and quantity of the extracted DNA were determined by electrophoresis on a 1.0% agarose gel and spectroscopic analysis (NanoDrop Technologies, Wilmington, DE, USA). DNA extracts were stored at −20°C and then 10-fold diluted DNA was used as templates of subsequent PCRs. Partial small subunit (SSU) ribosomal RNA gene fragments were amplified using nested PCR. DNA was first amplified using the primers NS31 (TTGGAGGGCAAGTCTGGTGCC) and AML2 (GAACCCAAACACTTTGGTTTCC) to amplify the 18S rRNA gene (Lumini et al., 2010). PCR was carried out in a final volume of 25 μl consisting of 2 μl extracted DNA dilution and 1 μl (10 μM) of each primer using the 2 × Taq PCR master mix system (Tiangen Biotech.) with the following cycling conditions: initial denaturation at 94°C for 3 min, followed by 30 cycles at 94°C for 30 s, 59°C for 1 min, 72°C for 2 min, and a final extension period at 72°C for 10 min. An aliquot of 2 μl of the first PCR product was diluted 1/100 with ddH2O and used as a template for the second PCR reaction using primers AMV4.5NF (AAGCTCGTAGTTGAATTTCG) and AMDGR (CCCAA CTATCCCTATTAATCAT) (Geel et al., 2014). The second PCR was carried out in a final volume of 50 μl with the following cycles: 94°C for 3 min, followed by 30 cycles at 94°C for 30 s, 58°C for 1 min, 72°C for 1 min, and a final extension period at 72°C for 10 min. Second-step PCR products were purified with an agarose gel DNA purification kit (AP-GX-250G; Axygen, United States) and quantified using a NanoDrop 8000. The purified products were pooled in equimolar amounts and then sequenced on the Illumina-MiSeq platform (Guangdong Magigene Inc., Guangdong, China).
Bioinformatics analysis
The raw data obtained after high-throughput sequencing were mainly used in the Quantitative Insights Into Microbial Ecology (QIIME) and the UPARSE pipeline to analyze (Caporaso et al., 2010; Edgar, 2013). Quality control was conducted with QIIME, and high-quality sequences were imported into a Usearch (vision 11). Then, the Usearch was used for dereplication and merging of paired-end reads. The singletons were removed, and the chimeras were detected and removed using the de novo approach. The operational taxonomic units (OTUs) were clustered at 97% similarity. The sequence with the most occurrences in each OTU was selected as the representative sequence, and the QIIME was used for OTU classification and OTUs with non-AMF. OTUs with less than 3 samples and sequences with less than 0.01% of the total sequences were removed to reduce errors. All samples are resampled according to the minimum sample sequence size to reduce errors caused by different sample sizes. Taxonomic assignment was performed by blasting the representative sequence of each OTU against the GenBank database1 and the Maarj AM database using BLAST software. The criteria were set to >97% similarity, >90% coverage, and >200 BLAST score values. The sequences obtained in this study have been submitted to the GenBank database (PRJNA843517).
Statistical analysis
All statistical analyses were undertaken using R2. Before further analysis, all data were tested for normality. Any data which did not meet normality distribution were converted using the appropriate method. The α diversity of AM fungi was characterized by calculating the OTU numbers (richness) and Shannon-Wiener index using the “diversity” function of the “Vegan” package in the R. The Kruskal-Wallis Test was used to analyze the differences in the α diversity of AM fungi, soil, and plant parameters at different locations in the riparian zone. Differences in plant community and AM fungi community composition (based on Bray-Curtis distance) were analyzed using non-metric multidimensional scaling analysis (NMDS) ordination (“Vegan” package, “metaMDS” function). Differences in community composition between groups were calculated and compared using non-parametric multivariate analysis of variance (PERMANOVA). Correlations between geographic distance, environmental and plant factors, and AM fungal communities were determined by the mantel test analysis based on the Pearson correlation. Canonical correspondence analysis (CCA) was carried out to explore the association between AM fungi community composition and soil factors using the “Vegan” package in R. Geographic distances are calculated using the “distm” function of the “geosphere” package in R. In this study, a structural equation model was constructed by Amos 25 to analyze how geographic distance, soil factors, and plant factors directly or indirectly affect AM fungal diversity and community composition. The plant and environmental variables were selected after collinearity testing based on driver analysis of AM fungal abundance, α diversity, and β diversity. Among them, geographic distance is represented by the actual longitudinal distance from the first sampling site to each of the other sites in near-bank and far-bank, respectively. Soil fertility index (SFI) was a synthetic index generated from the first principle component (PC1) scores of principal component analysis (PCA) of SOC (loading value = 0.50), TN (0.55), and TC (0.54), with 66.72% of the total explainable variance. Plant community and AM fungal community composition are characterized by the corresponding NMDS score values, respectively. The reference standard of the model fit is: chi-square/df < 2, P > 0.05; root mean square error of approximation (RMSEA) < 0.05; goodness of fit index (goodness of fit index, GFI) > 0.95 (Hooper et al., 2008). All graphs in this article were drawn using R and SigmaPlot 13.0.
Results
Soil physicochemical properties and plant characteristics
Soil physicochemical properties changed significantly along the riparian zone (Figure 2). SOC showed an overall decreasing trend along the river (Figure 2A). In contrast, soil TN (Figure 2B) and TC (Figure 2C) showed a first increasing trend and then decreasing trend in the near-bank but a continuous decreasing trend in the far-bank. Soil water content also showed decreasing trend along the river (Figure 2D). Vertical to the river, the SOC and soil water content in the near-bank were higher than those in the far-bank, except for the S01 point near the ice edge (Figures 2A,D). And except for the S01 and S02 points, the soil TN and TC in the near-bank were also higher than those in the far-bank (Figures 2B,C). The remaining soil factors, such as soil TP, AP, NH4+, and NO3–, showed no noticeable change (Supplementary Table 1).
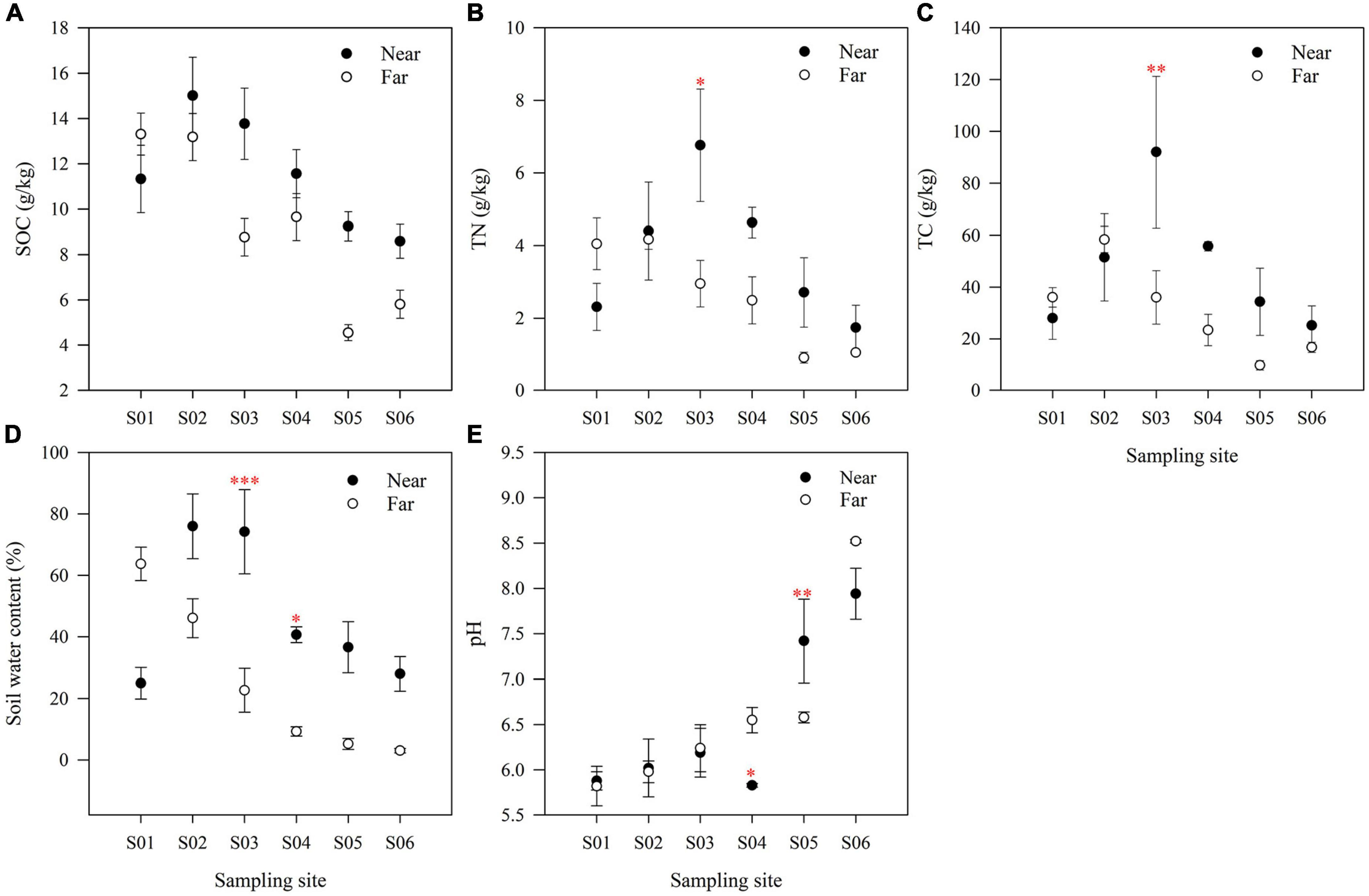
Figure 2. The soil organic C (A), soil total N (B), soil total C (C), soil water content (D) and soil pH (E) at each sampling site in the riparian zone. The data are means ± SE (n = 3). Significant differences between the near-bank and the far-bank samples at the same sampling site were determined based on the Kruskal-Wallis Test (*P < 0.05; **P < 0.01; ***P < 0.001).
Among the plant variables, there were no significant changes in plant aboveground biomass and richness from upstream to downstream. The aboveground biomass in the near-bank was significantly higher than that in the far-bank (P < 0.05), but the plant richness showed no significant difference (Supplementary Table 1). As shown on the NMDS ordination (Supplementary Figure 1), the plant species composition in the far-bank was relatively dispersed and well separated from that in the near-bank, which was verified by PERMANOVA (Supplementary Table 2).
Identification of arbuscular mycorrhizal fungal community
The results of the OTU rarefaction curve indicated that the sampling intensity was sufficient (Supplementary Figure 2), which also showed that much more AM fungal sequences were detected in the far-bank than the near-bank. In this study, a total of 184 AM fungal OTUs were identified, belonging to 9 families and 12 genera (Figure 3). Among them, the most dominant genus was identified as Glomus (23.84% of all AM fungal sequences), mainly concentrated in the downstream riparian zone (Figure 3), followed by Claroideoglomus (23.32%), which mostly gathered in the near-bank.
Arbuscular mycorrhizal fungal abundance, diversity, and community composition
AM fungal abundance was significantly different along the river and showed contrasting patterns (Figure 4). The RLC (Figure 4A) and spore density (Figure 4B) showed an overall upward trend along the river, while the HLD (Figure 4C) first increased and then decreased, reaching a peak at point S03. Vertical to the river, the colonization of AM fungi was not significantly different between the near- and far-bank soils. The spore density in the far-bank was much higher than that in the near-bank soil (P < 0.05), while the HLD showed a reverse trend (P < 0.05; Supplementary Table 3).
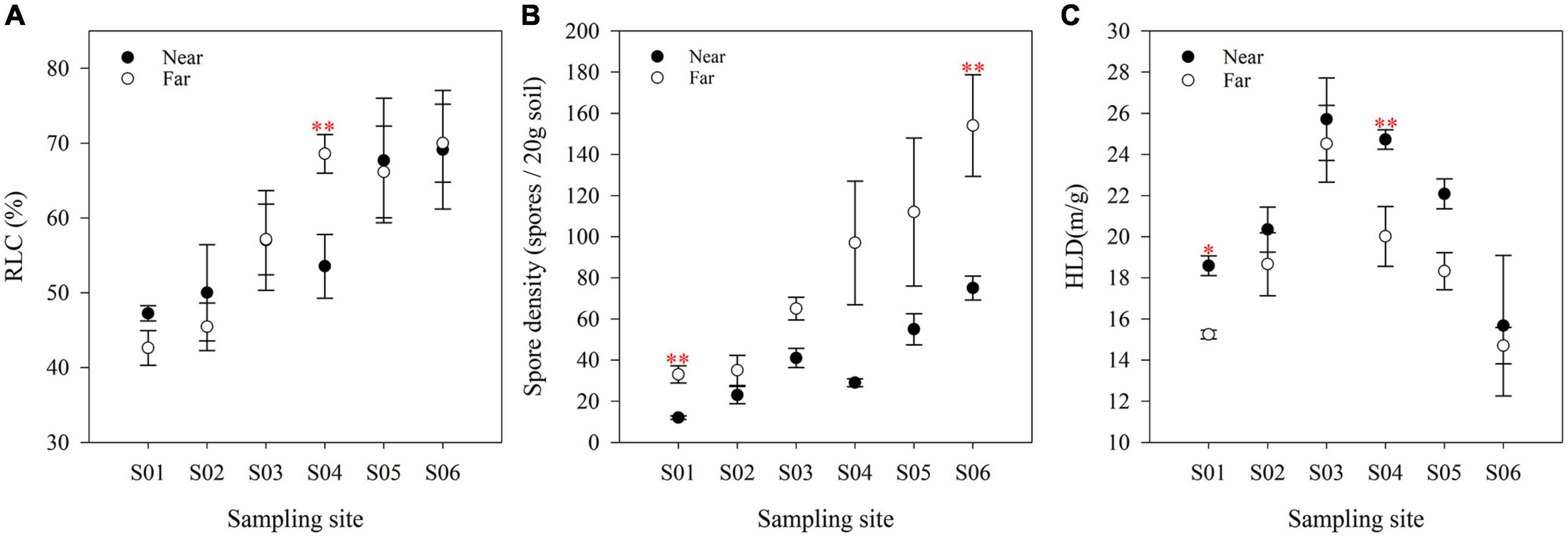
Figure 4. The RLC (A), spore density (B) and HLD (C) of soils at various sites in the riparian zone. The data are means ± SE (n = 3). Significant differences between the near-bank and the far-bank samples at the same sampling site were determined based on the Kruskal-Wallis Test (*P < 0.05; **P < 0.01; ***P < 0.001).
In the near-bank soils, the AM fungal α diversity (species richness and Shannon-wiener index) increased overall from upstream to downstream along the river (Figure 5), and there was a significant difference in the community composition (P = 0.001; Figure 6). While in the far-bank soils, AM fungal community also showed a distinct distribution pattern along the river (P < 0.05; Figure 5), but its α diversity increased and then decreased at the two sampling sites near the lake (Figure 5). On the whole, the α diversity in the near-bank was not significantly different from that in the far-bank, except for the specific S03, S04, and S06 (Supplementary Table 4).
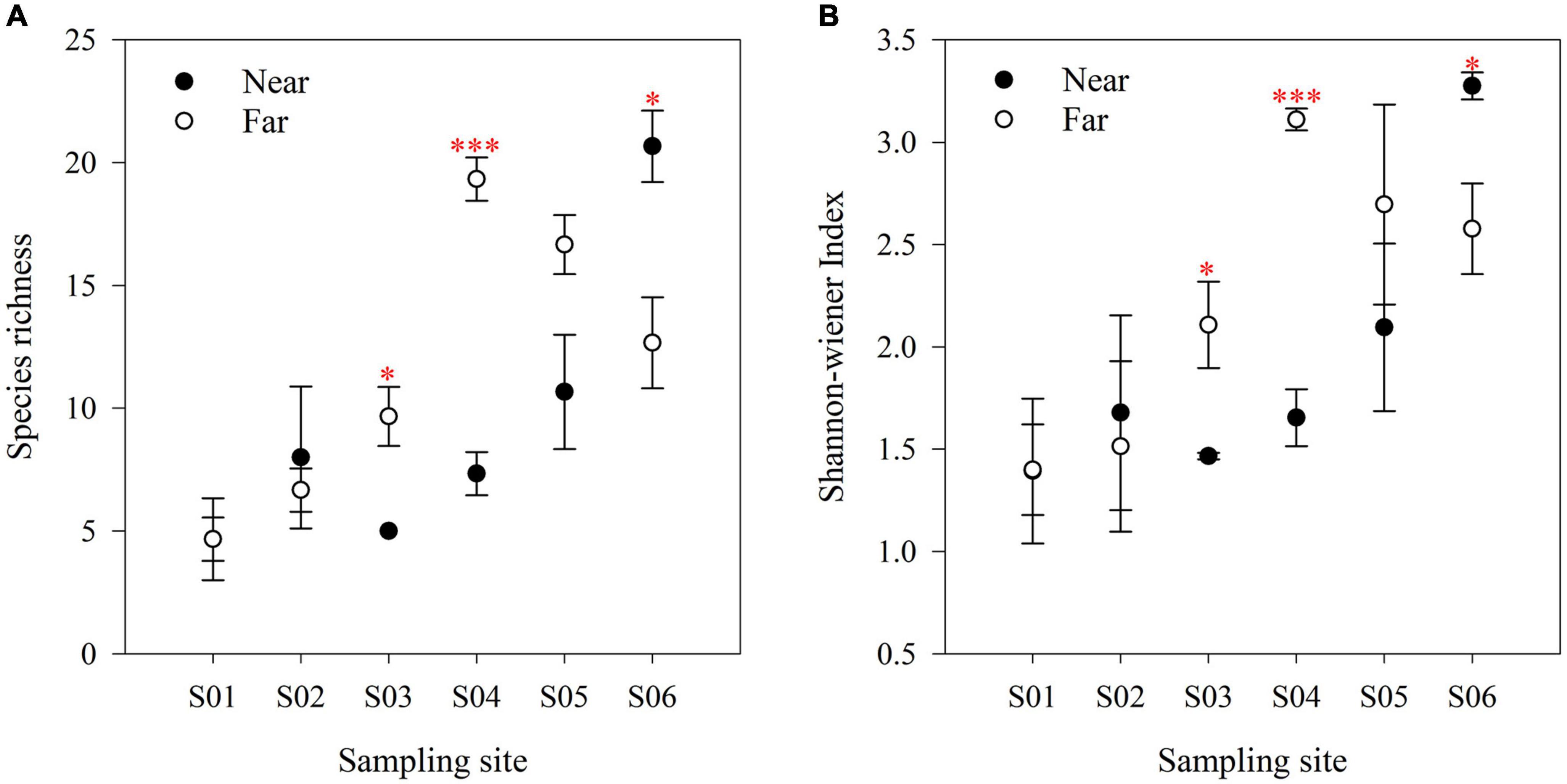
Figure 5. AM fungal species richness (A) and Shannon-wiener index (B) in riparian zone. The data are means ± SE (n = 3). Significant differences between the near-bank and the far-bank samples at the same sampling site were determined based on the Kruskal-Wallis Test (*P < 0.05; **P < 0.01; ***P < 0.001).
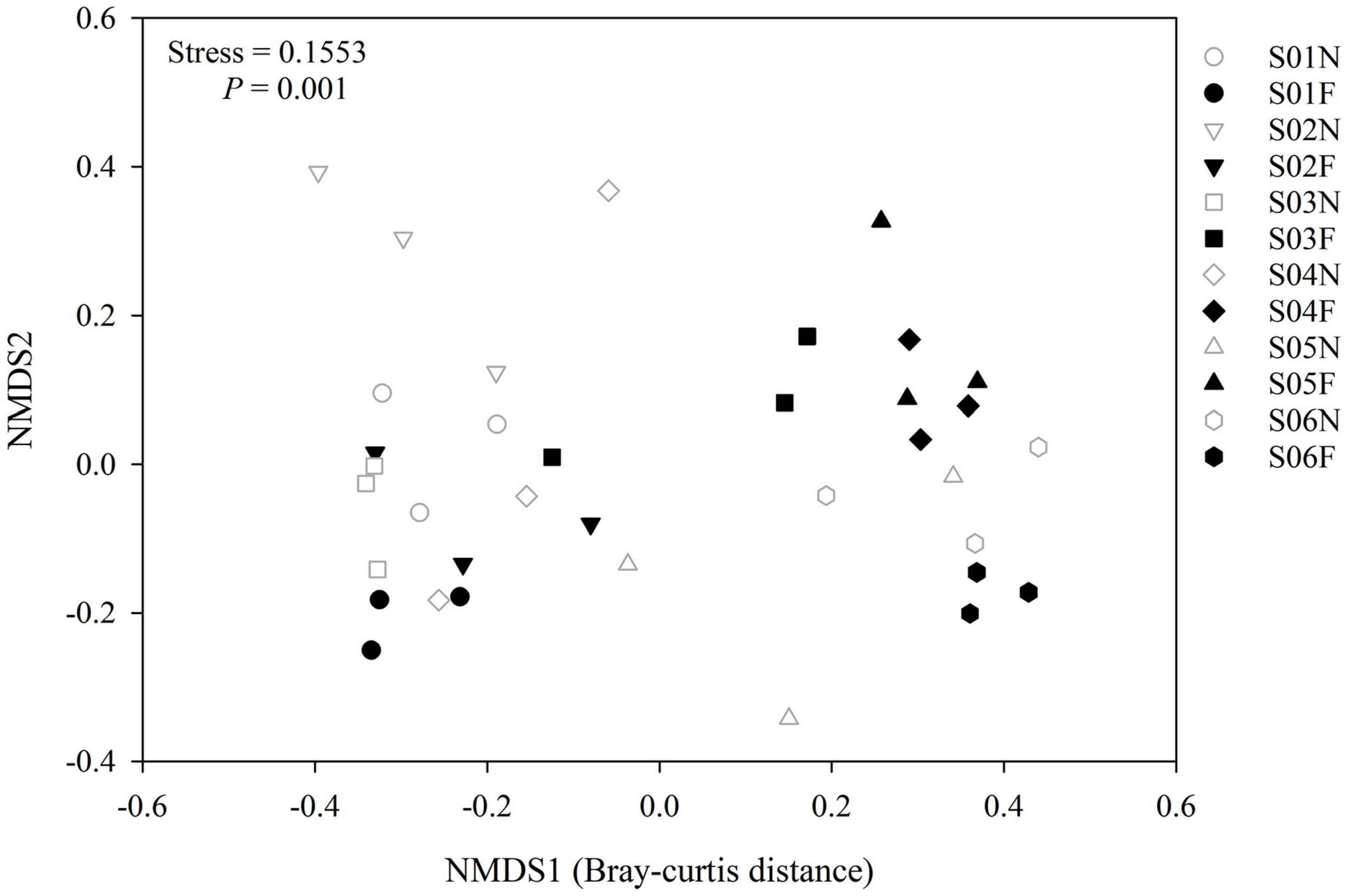
Figure 6. Non-metric multidimensional scaling (NMDS) ranking according to the AM fungal community differences (Bray-Curtis distance) identified at each sampling site. Permutational multivariate analysis of variance (PERMANOVA) was adopted to compare community composition among different soil samples.
Drivers of arbuscular mycorrhizal fungal diversity and community composition
The results of the Mantel test showed that a variety of environmental factors such as geographic distance, plant community, and soil physicochemical were significantly correlated with AM fungal abundance, α diversity, and community composition (P < 0.05, Figure 7). In the near-bank soils, the AM fungal spore density significantly correlated with geographic distance, elevation, soil pH, NH4+ content, and AP content, respectively (Figure 7A). The α diversity showed significant correlations with soil pH, elevation, plant richness, and geographic distance. In contrast, AM fungal community composition was only significantly correlated with soil pH. In the far-bank soils, AM fungi were influenced by more complex biotic, and abiotic factors (Figure 7B). Geographic distance, soil water content and elevation significantly correlated with AM fungal communities. Moreover, the output of the Canonical correspondence analysis (CCA) with environmental factors indicated that the AM fungal community composition was significantly impacted by soil pH (P < 0.001), geographic distance (P < 0.001), and soil water content (P < 0.05; Supplementary Figure 3). It is worth noting that a valid (P < 0.001) distance-decay relationship was observed for the AM fungal community over geographic distance with similar slopes (turnover rates) in the near (slope = -0.017, R2 = 0.150) and far (slope = −0.015, R2 = 0.369) river-bank soils (Supplementary Figure 4).
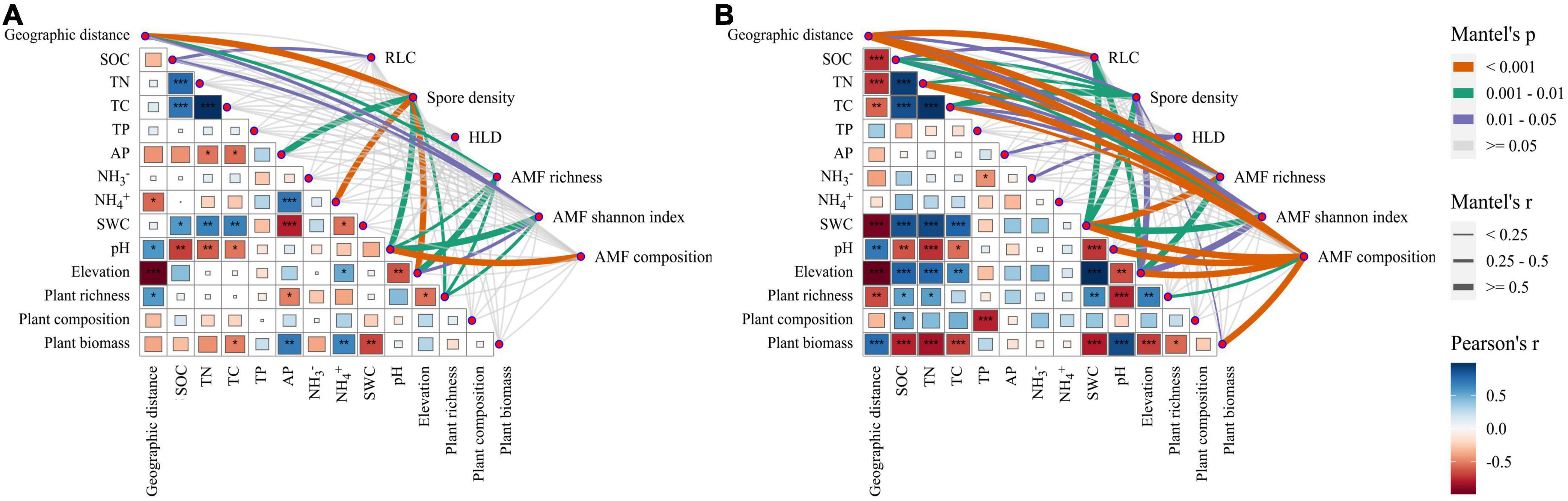
Figure 7. Relationships between AM fungal abundance, α diversity, and community composition with environmental factors in the near (A) and far (B) bank soils. The relationships among environmental variables were illustrated by Pearson correlation (*P < 0.05; **P < 0.01; ***P < 0.001).
The structural equation model (SEM) further better assessed the impact of environmental variables on AM fungal community composition through direct and indirect pathways. In total, AM fungal species richness and community composition in the riparian soils were mainly driven by geographic distance and soil water content, followed by soil pH and plant community composition (Supplementary Figure 5 and Supplementary Table 5). Among them, the SEM model (Chi_square/df = 0.039, P = 0.843, RMSEA < 0.001; GFI = 0.999) explained 71 and 90% of the AM fungal richness and community composition variance in the near-bank soils, respectively (Figure 8A and Table 1). In this model, soil pH had direct effects on AM fungal species richness (standard estimates = 0.73, P < 0.01) and community composition (standard estimates = 0.28, P < 0.05), while geographic distance and soil water content had indirect effects on AM fungi via changes in soil pH. The far-bank SEM model (Chi_square/df = 0.508, P = 0.730, RMSEA < 0.001; GFI = 0.971) explained 60 and 97% of the AMF richness and community composition variance, respectively (Figure 8B and Table 2). In the far-bank soil, other than the direct effect of soil water content, geographic distance indirectly affected AM fungal species richness and community composition through soil moisture (P < 0.05). Soil pH showed a significant direct effect on AM fungal community composition. Moreover, plant community composition also significantly affected AM fungal community composition in the total riparian zone.
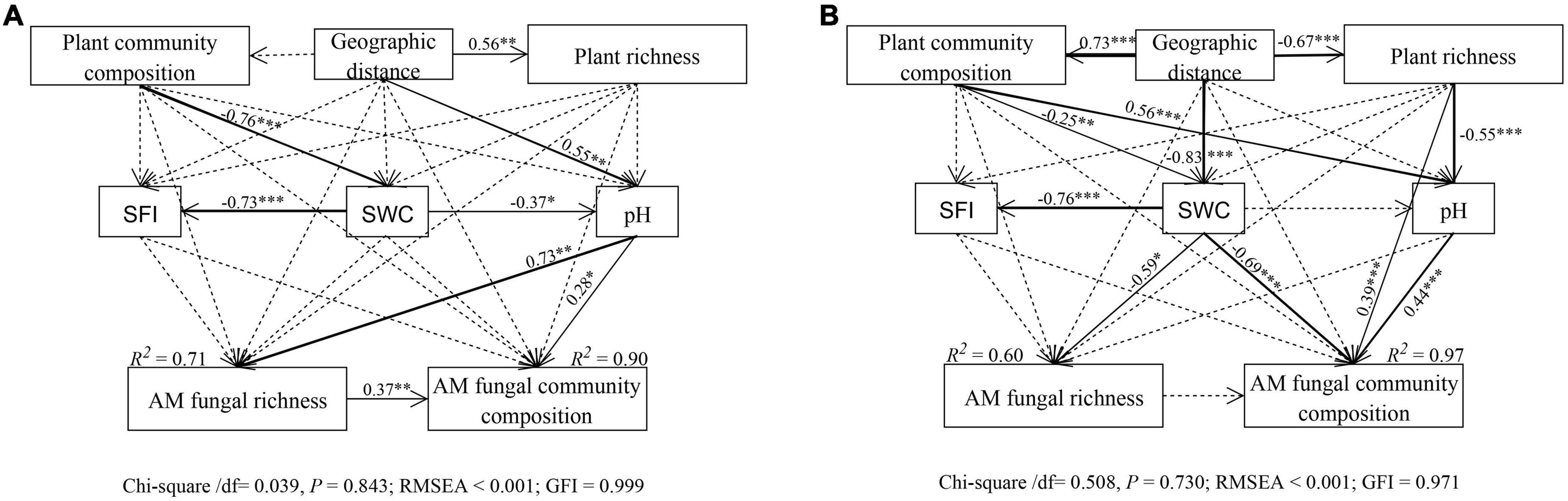
Figure 8. Structural equation model (SEM) showing causal relationships among geographic distances, plant variables, soil variables, AM fungal richness and community composition in the near (A) and far (B) bank soils. Solid and dashed lines indicate significant and non-significant pathways, respectively. The width of the solid line indicates the strength of the causal effect. The R2 value represents the proportion of variance explained for each variable.
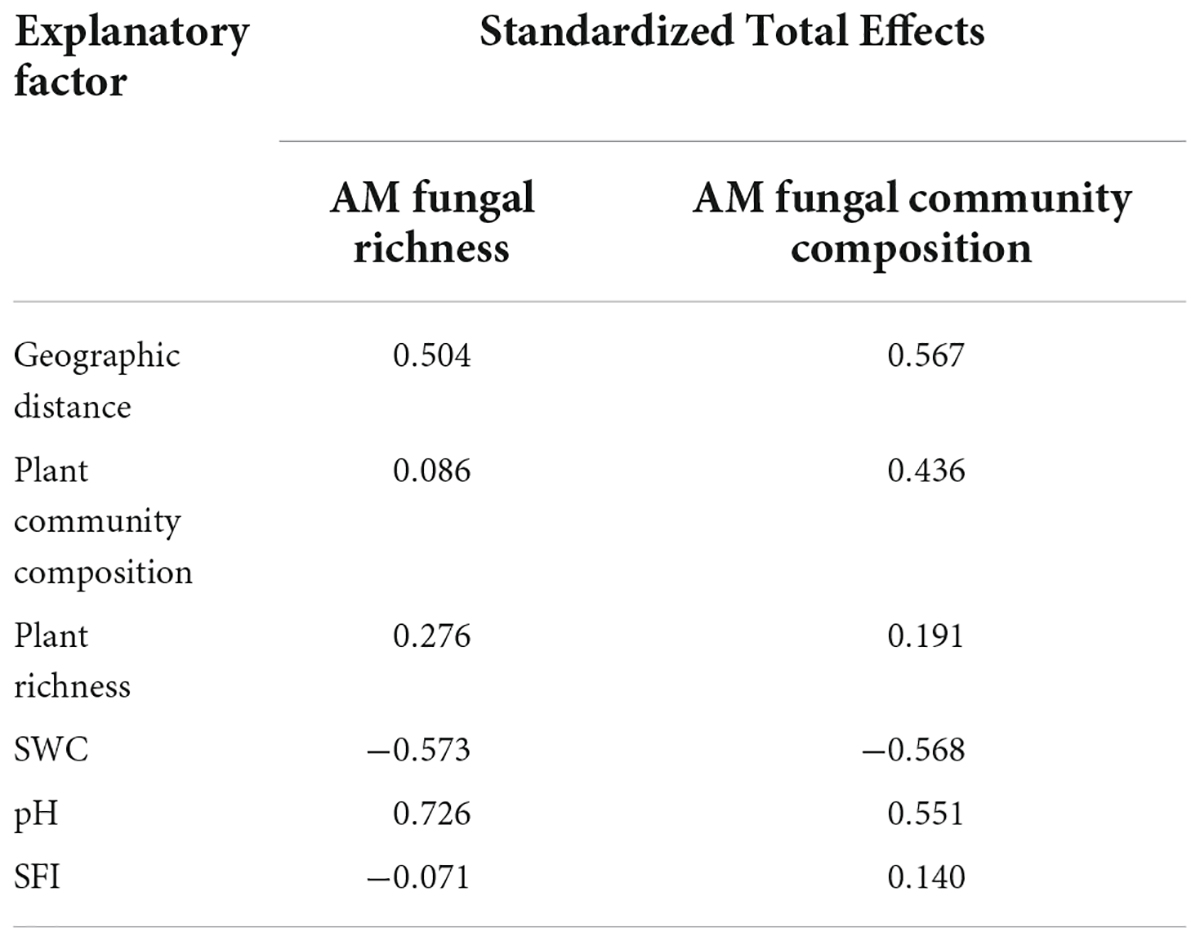
Table 1. Standardized total contribution rate of main factors driving AM fungal richness and community composition in the near-bank soils.
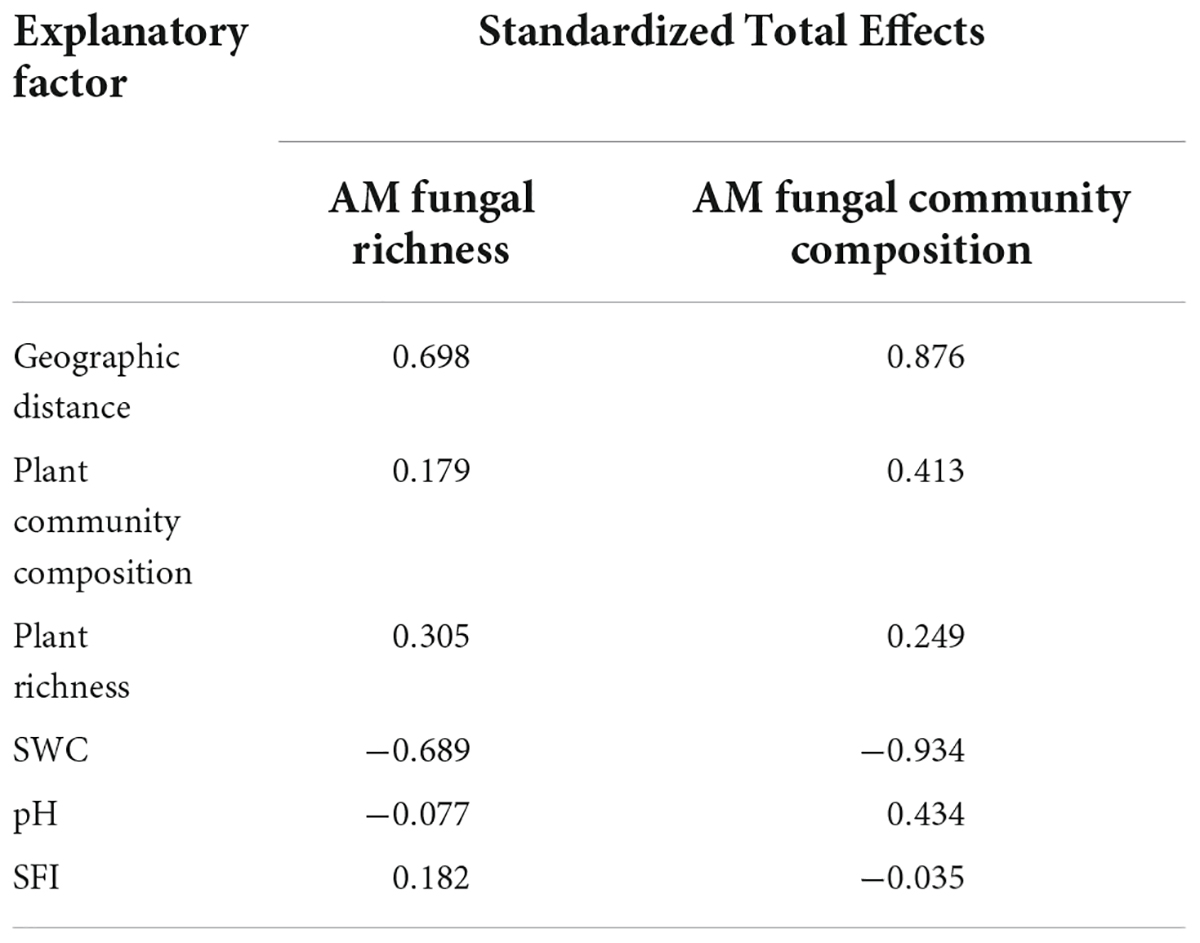
Table 2. Standardized total contribution rate of main factors driving AM fungal richness and community composition in the far-bank soils.
Discussion
Arbuscular mycorrhizal fungal abundance and diversity in the riparian zone of small alpine watershed
Previous studies have shown that arbuscular mycorrhizal symbionts commonly exist in extreme alpine stress habitats on the Qinghai-Tibet Plateau (Gai et al., 2009; Li et al., 2018) and play an essential role in typical alpine grassland plants growth and community succession (Jiang et al., 2018; Mao et al., 2019). Our study also proved that a relatively high AM fungal abundance was present in the riparian zone of the alpine watershed. The dominant genera Glomus and Claroideoglomus are common AM fungi groups in typical alpine grassland (Shi et al., 2017; Jiang et al., 2018; Bahaduiv et al., 2019). Moreover, we found that the relative abundance of Glomus significantly increased along the river while the Claroideoglomus was the dominant genus in the near-bank soils. Fungi in the Glomus are considered to be more disturbance-tolerant due to their “r” selected traits, which can produce a large number of spores and mycelium in the process of growth and reproduction, thus enhancing their adaptive ability (Ohsowski et al., 2014; van der Heyde et al., 2017b). Therefore, the potential reasons for the higher abundance of Glomus in the downstream riparian zone are as follows. First, the subsurface flow in the riparian zone will enhance the accumulation of the Glomus spores with a small diameter and large production. Second, the frequent wildlife and livestock activities downstream near the lake are also conducive to promoting the predominance of the Glomus, which are considered more disturbance tolerant (Ba et al., 2012; van der Heyde et al., 2017a,b). While the Claroideoglomus usually showed higher salt tolerance and predominated in high saline soils (Dai et al., 2013; Dashtebani et al., 2014; Analia Silvani et al., 2017; Yang et al., 2020). Influenced by the carbonate minerals of the river water (Gao et al., 2008; Keil et al., 2010), the soil salinity in the near-bank may be relatively high than that in the far-bank soils, which might be why the relative abundance of Claroideoglomus was much higher in near-bank soil. Thus, further research is needed to carry out to test the relative importance of soil salinity for AM fungal assembly in the riparian zone.
It is worth noting that the same high AM colonization levels observed in wetland plants (near-bank) and alpine meadow plants (far-bank) were inconsistent with other previous work, which showed that AM fungal colonization is lower in wetland-aquatic habitats than in terrestrial ecosystems (Fusconi and Mucciarelli, 2018). On the one hand, there is no significant difference in plant species diversity between the wetland and alpine meadow in this study, which might provide equally rich ecological niches for AM fungi. On the other hand, the near-river alpine wetlands, usually classified as wet meadows, are just inundated with river water temporarily during the growing season in this area. The effect of flooding intensities on AM colonization is also weaker than that of aquatic habitats, which is featured in typical oxygen limitations unsuitable for AM fungal survival (Bohrer et al., 2004). However, the higher soil moisture near-bank did affect the mycorrhizal growth and development, as confirmed by the lower spore density and α diversity. Conversely, the higher aboveground biomass meaning more allocation of carbon to AM fungi in the near-bank may result in increased external hyphae, which concurs with our findings that HLD in the near-bank soils was much higher than that in the far-bank.
This study demonstrated that AM fungal spores and α diversity along the Niaqu river showed an overall increasing trend from upstream to downstream, which supports our first hypothesis (H1) and corroborates with a recent study showing the increased microbial abundance and diversity along the Nu River (Zhang et al., 2022). The potential reasons for these results may be due to the great elevation drop from upstream to downstream (about 400 m), which might enhance the accumulation of the microbial propagules under the transportation of subsurface flow and increase the AM fungal community α diversity. Furthermore, a large amount of the Glomus group gathered downstream might provide many asexual spores (Chagnon et al., 2013). At last, the gradually favorable soil moisture and pH from upstream to downstream might support multiple AM fungal phylotypes colonization (Deepika and Kothamasi, 2015), which can be confirmed by the strong correlations between soil water content and soil pH and AM fungi.
Different major driving factors on arbuscular mycorrhizal fungal community between the wetland and alpine meadows in the riparian zone
Exploring the driving factors of AM fungal community composition in grassland ecosystems is one of the primary aims of mycorrhizal ecology (Ma et al., 2021). Previous studies have shown that the main factors influencing the grassland AM fungal community composition included host plants, geographical, climatic, and soil characteristics (Davison et al., 2015). Still, the relative importance of these driving factors is conflicting, especially at different scales (Hempel, 2018). Our results proved a strong effect of geographic distance on AM fungal diversity and community composition at the alpine small watershed scale. This is in line with previous studies reporting that potential spatial constraints may be a significant determinant of AM fungal community formation even at tiny scales (Hazard et al., 2013; Horn et al., 2014, 2017). However, the spatial isolation would be somewhat weakened by the water connectivity, especially in near-bank soils, thus showing a weaker distance-decay relationship. Notably, geographic distance only indirectly affected AM fungi through soil pH in near-bank and soil water content in far-bank, respectively. These findings suggest that local abiotic environmental variation caused by spatial differences is more critical in shaping AM fungal community in the Niaqu small watershed, supporting our second hypothesis (H2).
The effects of soil water content and pH on the microbial community have been widely reported, which can vitally shape the distribution pattern of soil microbes (Liu et al., 2018; Neupane et al., 2022). In this study, we also found the dominant role of these two factors in AM fungal distribution in the alpine riparian ecosystems. However, it is worth noting that in the near-bank soils, soil pH rather than soil water content exerted a substantial direct effect on AM fungal species richness and compositions, contrary to our second hypothesis. But in the far-bank soils, our results indicated soil moisture to be the strongest predictor of AM fungal community compositions. We assumed that the relative importance of these two drivers in determining the observed fungal distribution patterns mainly depends on the limiting factors of habitats in the riparian zone.
Unlike the near-bank wetland, where the soil is nearly saturated, soil moisture is likely to be the limiting factor of the typical alpine meadow ecosystem in the far-bank. Especially the sharp declined soil water content from upstream to downstream could influence AM fungal species directly through water limitation (Fu et al., 2022). Soil moisture can directly affect AM fungal colonization (Fitzsimons et al., 2008), the growth and distribution of extra-radical mycelium (Clark et al., 2009), and the specific species assemblages (Staddon et al., 2004; Deepika and Kothamasi, 2015). At the same time, soil aeration can influence fungal growth and diversity due to their high oxygen requirement (Miller and Sharitz, 2000). Although the soil water content also showed a decreasing trend from upstream to downstream in the near-bank wetland, the overall high soil moisture level might reduce the community sensitivity of AM fungi to soil water change (Garcia et al., 2008). Here, we found that soil pH, even within a limited range of about 2.0 pH units, was the main direct factor controlling the fungal community distribution in the near-bank soils. Moreover, soil moisture also indirectly affected the AM fungal community via changes in the soil pH. Soil pH is likely to affect the arbuscular mycorrhizal sporulation, extraradical mycelium growth, and the niche space, especially for specific genera (Van Aarle et al., 2002; Dumbrell et al., 2010). For instance, the relative abundance of the Glomus was shown to increase with increased soil pH along the river, either in the near or far-bank soils in this study.
Host plants are essential in regulating AM fungal structure and community (Neuenkamp et al., 2018). However, in this study, we did not find a strong effect of host plants on AM fungal community compared to abiotic factors, consistent with the results by Yang et al. (2021) which reported a minor contribution of crop types. This may be due to the specific plant types in this watershed ecosystem. The near-bank wetland and far-bank alpine meadows are dominated by the Cyperaceae plants, which do not strongly depend on AM fungi (Newman and Reddell, 1987; Fuchs and Haselwandter, 2004). Thus the biotic filtering is relatively unimportant in driving AM fungal community composition compared to abiotic parameters in this area. Similarly, very little AM fungal community variation was explained by soil fertility, mainly regulated by soil water content both in the near- and far-bank soil, confirming the driving effect of soil moisture in this watershed ecosystem.
Conclusion
This is the first study investigating the composition and distribution of AM fungal community in the riparian zone of the alpine river on the QTP. By studying the biotic and abiotic factors related to riparian soil, we undertook an extensive assessment of the factors driving AM fungal communities. Our study reveals that AM fungal communities significantly varied between the wetland and alpine meadows in the riparian zone, mainly driven by the geographic distance, soil water content, soil pH, and plant communities. Especially, soil pH was the principal predictor of AM fungal community in near-bank wetland soils, while soil water content had a most substantial direct effect in alpine meadows. In addition, we also found that geographic distance strongly influences AM fungal community at the alpine small watershed scale, but indirectly through soil water content or pH. In contrast, host plants have minimal influence on it. Our results indicate that the abiotic factors are the most important drivers in shaping AM fungal communities at the watershed scale. The results of this study lay a foundation for fully understanding and utilizing riparian microbial resources, further comprehending the service functions of riparian ecosystems, and providing theoretical support for the construction of microbial biogeographic models.
Data availability statement
The datasets presented in this study can be found in online repositories. The names of the repository/repositories and accession number(s) can be found below: https://www.ncbi.nlm.nih.gov/genbank/, PRJNA843517.
Author contributions
YZ, JZ, and BJ designed the experiments, which were then carried out by YZ, and CL. YZ, KC, HS, and YT collected the data and conducted the statistical analyses. YZ wrote the first draft of the manuscript. JZ, MM, KC, BJ, and YZ reviewed and edited the manuscript before submission. All authors made substantial contributions to the discussion of content.
Funding
This work was supported by The Second Tibetan Plateau Scientific Expedition and Research Program (2019QZKK0304 and 2019QZKK0302).
Acknowledgments
We thank Qiang Dong for his help in providing lab analysis and data analysis methods, Zhouyuan Li for helping to draw geographic map, and Liyuan Ma, Liwen Shan, Jiaqing Xu, Jiayin Pan, Yu Wu, Qiang Liu, Wencong Lv, and Youqing Yang for their help in field sampling.
Conflict of interest
The authors declare that the research was conducted in the absence of any commercial or financial relationships that could be construed as a potential conflict of interest.
Publisher’s note
All claims expressed in this article are solely those of the authors and do not necessarily represent those of their affiliated organizations, or those of the publisher, the editors and the reviewers. Any product that may be evaluated in this article, or claim that may be made by its manufacturer, is not guaranteed or endorsed by the publisher.
Supplementary material
The Supplementary Material for this article can be found online at: https://www.frontiersin.org/articles/10.3389/fmicb.2022.994918/full#supplementary-material
Footnotes
References
Acker, S. A., Gregory, S., Lienkaemper, G., McKee, W. A., Swanson, F. J., and Miller, S. D. (2003). Composition, complexity, and tree mortality in riparian forests in the central Western Cascades of Oregon. Forest Ecol. Manag. 173, 293–308. doi: 10.1016/S0378-1127(02)00005-1
Almeida-Rodriguez, A. M., Gomes, M. P., Loubert-Hudon, A., Joly, S., and Labrecque, M. (2016). Symbiotic association between Salix purpurea L. and Rhizophagus irregularis: modulation of plant responses under copper stress. Tree Physiol. 36, 407–420. doi: 10.1093/treephys/tpv119
Analia Silvani, V., Paula Colombo, R., Victoria Scorza, M., Fernandez Bidondo, L., Paola Rothen, C., Scotti, A., et al. (2017). Arbuscular mycorrhizal fungal diversity in high-altitude hypersaline Andean wetlands studied by 454-sequencing and morphological approaches. Symbiosis 72, 143–152. doi: 10.1007/s13199-016-0454-3
Anslan, S., Rad, M. A., Buckel, J., Galindo, P. E., Kai, J., Kang, W., et al. (2020). Reviews and syntheses: How do abiotic and biotic processes respond to climatic variations in the Nam Co catchment (Tibetan Plateau)? Biogeosciences 17, 1261–1279. doi: 10.5194/bg-17-1261-2020
Ba, L., Ning, J., Wang, D., Facelli, E., Facelli, J. M., Yang, Y., et al. (2012). The relationship between the diversity of arbuscular mycorrhizal fungi and grazing in a meadow steppe. Plant Soil 352, 143–156. doi: 10.1007/s11104-011-0985-6
Bahaduiv, A., Jin, Z., Jiang, S., Chai, Y., Zhang, Q., Pan, J., et al. (2019). Arbuscular mycorrhizal spores distribution across different ecosystems of the qinghai tibetan plateau. Pak. J. Bot. 51, 1481–1492. doi: 10.30848/PJB2019-4(10)
Bender, S. F., Conen, F., and Van der Heijden, M. G. A. (2015). Mycorrhizal effects on nutrient cycling, nutrient leaching and N2O production in experimental grassland. Soil Biol. Biochem. 80, 283–292. doi: 10.1016/j.soilbio.2014.10.016
Bohrer, K. E., Friese, C. F., and Amon, J. P. (2004). Seasonal dynamics of arbuscular mycorrhizal fungi in differing wetland habitats. Mycorrhiza 14, 329–337. doi: 10.1007/s00572-004-0292-7
Bosompemaa, P., Peterson, E. W., Perry, W., and Seyoum, W. M. (2021). Recycling of nitrate and organic matter by plants in the vadose zone of a saturated riparian buffer. Water Air Soil Poll. 232:245. doi: 10.1007/s11270-021-05202-3
Bray, R. H., and Kurtz, L. (1945). Determination of total, organic, and available forms of phosphorus in soils. Soil Sci. 59, 39–46. doi: 10.1097/00010694-194501000-00006
Bremner, J., and Mulvaney, C. (1982). “Nitrogen-Total,” in Methods of soil analysis, part 2: chemical and microbiological properties, 2nd Edn, eds A. L. Page, R. H. Miller, and D. R. Keeney (Madison WI: American Society of Agronomy).
Brundrett, M., Melville, L., and Peterson, L. (1994). Practical methods in mycorrhiza research: based on a workshop organized in conjunction with the Ninth North American Conference on Mycorrhizae. Ontario: University of Guelph.
Caporaso, J. G., Kuczynski, J., Stombaugh, J., Bittinger, K., Bushman, F. D., and Costello, E. K. (2010). QIIME allows analysis of high-throughput community sequencing data. Nat. Methods 7, 335–336. doi: 10.1038/nmeth.f.303
Chagnon, P., Bradley, R. L., Maherali, H., and Klironomos, J. N. (2013). A trait-based framework to understand life history of mycorrhizal fungi. Trends Plant Sci. 18, 484–491. doi: 10.1016/j.tplants.2013.05.001
Clark, N. M., Rillig, M. C., and Nowak, R. S. (2009). Arbuscular mycorrhizal fungal abundance in the mojave desert: seasonal dynamics and impacts of elevated CO2. J. Arid Environ. 73, 834–843. doi: 10.1016/j.jaridenv.2009.03.004
Coban, O., De Deyn, G. B., and van der Ploeg, M. (2022). Soil microbiota as game-changers in restoration of degraded lands. Science 375:990. doi: 10.1126/science.abe0725
Dai, M., Bainard, L. D., Hamel, C., Gan, Y., and Lynch, D. (2013). Impact of land use on arbuscular mycorrhizal fungal communities in rural Canada. Appl. Environ. Microb. 79, 6719–6729. doi: 10.1128/AEM.01333-13
Dashtebani, F., Hajiboland, R., and Aliasgharzad, N. (2014). Characterization of salt-tolerance mechanisms in mycorrhizal (Claroideoglomus etunicatum) halophytic grass, Puccinellia distans. Acta Physiol. Plant 36, 1713–1726. doi: 10.1007/s11738-014-1546-4
Davison, J., Moora, M., Oepik, M., Adholeya, A., Ainsaar, L., Ba, A., et al. (2015). Global assessment of arbuscular mycorrhizal fungus diversity reveals very low endemism. Science 349, 970–973. doi: 10.1126/science.aab1161
Deepika, S., and Kothamasi, D. (2015). Soil moisture-a regulator of arbuscular mycorrhizal fungal community assembly and symbiotic phosphorus uptake. Mycorrhiza 25, 67–75. doi: 10.1007/s00572-014-0596-1
Dumbrell, A. J., Nelson, M., Helgason, T., Dytham, C., and Fitter, A. H. (2010). Relative roles of niche and neutral processes in structuring a soil microbial community. ISME J. 4, 337–345. doi: 10.1038/ismej.2009.122
Edgar, R. C. (2013). Uparse: highly accurate OTU sequences from microbial amplicon reads. Nat. Methods 10, 996–998. doi: 10.1038/nmeth.2604
Fitzsimons, M. S., Miller, R. M., and Jastrow, J. D. (2008). Scale-dependent niche axes of arbuscular mycorrhizal fungi. Oecologia 158, 117–127. doi: 10.1007/s00442-008-1117-8
Fu, W., Chen, B., Rillig, M. C., Jansa, J., Ma, W., Xu, C., et al. (2022). Community response of arbuscular mycorrhizal fungi to extreme drought in a cold-temperate grassland. New Phytologist 234, 2003–2017. doi: 10.1111/nph.17692
Fuchs, B., and Haselwandter, K. (2004). Red list plants: colonization by arbuscular mycorrhizal fungi and dark septate endophytes. Mycorrhiza 14, 277–281. doi: 10.1007/s00572-004-0314-5
Fusconi, A., and Mucciarelli, M. (2018). How important is arbuscular mycorrhizal colonization in wetland and aquatic habitats? Environ. Exp. Bot. 155, 128–141. doi: 10.1016/j.envexpbot.2018.06.016
Gai, J. P., Christie, P., Cai, X. B., Fan, J. Q., Zhang, J. L., Feng, G., et al. (2009). Occurrence and distribution of arbuscular mycorrhizal fungal species in three types of grassland community of the Tibetan Plateau. Ecol. Res. 24, 1345–1350. doi: 10.1007/s11284-009-0618-1
Gao, T. G., Kang, S. C., Zhang, Q. G., Zhou, S. Q., and Xu, Y. W. (2008). Major ionic features and their sources in the nam co basin over the tibetan plateau. Environ. Sci. 11, 3009–3016. doi: 10.13227/j.hjkx.2008.11.045
Garcia, I., Mendoza, R., and Pomar, M. C. (2008). Deficit and excess of soil water impact on plant growth of Lotus tenuis by affecting nutrient uptake and arbuscular mycorrhizal symbiosis. Plant Soil 304, 117–131. doi: 10.1007/s11104-007-9526-8
Geel, M. V., Busschaert, P., Honnay, O., and Lievens, B. (2014). Evaluation of six primer pairs targeting the nuclear rRNA operon for characterization of arbuscular mycorrhizal fungal (AMF) communities using 454 pyrosequencing. J. Microbiol. Methods 106, 93–100. doi: 10.1016/j.mimet.2014.08.006
Gonzalez, E., Felipe-Lucia, M. R., Bourgeois, B., Boz, B., Nilsson, C., Palmer, G., et al. (2017). Integrative conservation of riparian zones. Biol. Conserv. 211, 20–29. doi: 10.1016/j.biocon.2016.10.035
Gruemberg, B. C., Urcelay, C., Shroeder, M. A., Vargas-Gil, S., and Luna, C. M. (2015). The role of inoculum identity in drought stress mitigation by arbuscular mycorrhizal fungi in soybean. Biol. Fert. Soils 51, 1–10. doi: 10.1007/s00374-014-0942-7
Harner, M. J., Opitz, N., Geluso, K., Tockner, K., and Rillig, M. C. (2011). Arbuscular mycorrhizal fungi on developing islands within a dynamic river floodplain: an investigation across successional gradients and soil depth. Aquat. Sci. 73, 35–42. doi: 10.1007/s00027-010-0157-4
Hazard, C., Gosling, P., van der Gast, C. J., Mitchell, D. T., Doohan, F. M., and Bending, G. D. (2013). The role of local environment and geographical distance in determining community composition of arbuscular mycorrhizal fungi at the landscape scale. ISME J. 7, 498–508. doi: 10.1038/ismej.2012.127
Hempel, S. (2018). Passengers and drivers of arbuscular mycorrhizal fungal communities at different scales. New Phytologist 220, 952–953. doi: 10.1111/nph.15139
Hooper, D., Mullen, J., Hooper, D., Coughlan, J., and Mullen, M. R. (2008). Structural equation modeling: Guidelines for determining model fit. Electron. J. Bus. Res. Methods 6, 53–60. doi: 10.0000/PMID35188134
Horn, S., Caruso, T., Verbruggen, E., Rillig, M. C., and Hempel, S. (2014). Arbuscular mycorrhizal fungal communities are phylogenetically clustered at small scales. ISME J. 8, 2231–2242. doi: 10.1038/ismej.2014.72
Horn, S., Hempel, S., Verbruggen, E., Rillig, M. C., and Caruso, T. (2017). Linking the community structure of arbuscular mycorrhizal fungi and plants: a story of interdependence? ISME J. 11, 1400–1411. doi: 10.1038/ismej.2017.5
Immerzeel, W. W., van Beek, L. P. H., and Bierkens, M. F. P. (2010). Climate change will affect the asian water towers. Science 328, 1382–1385. doi: 10.1126/science.1183188
Jiang, S., Liu, Y., Luo, J., Qin, M., Johnson, N. C., Opik, M., et al. (2018). Dynamics of arbuscular mycorrhizal fungal community structure and functioning along a nitrogen enrichment gradient in an alpine meadow ecosystem. New Phytologist 220, 1222–1235. doi: 10.1111/nph.15112
Keil, A., Berking, J., Muegler, I., Schuett, B., Schwalb, A., and Steeb, P. (2010). Hydrological and geomorphological basin and catchment characteristics of Lake Nam Co, South-Central Tibet. Quatern. Int. 218, 118–130. doi: 10.1016/j.quaint.2009.02.022
Kivlin, S. N., Hawkes, C. V., and Treseder, K. K. (2011). Global diversity and distribution of arbuscular mycorrhizal fungi. Soil Biol. Biochem. 43, 2294–2303. doi: 10.1016/j.soilbio.2011.07.012
Larsen, S., Muehlbauer, J. D., and Marti, E. (2016). Resource subsidies between stream and terrestrial ecosystems under global change. Global Change Biol. 22, 2489–2504. doi: 10.1111/gcb.13182
Lekberg, Y., and Waller, L. P. (2016). What drives differences in arbuscular mycorrhizal fungal communities among plant species? Fungal Ecol. 24, 135–138. doi: 10.1016/j.funeco.2016.05.012
Li, C. C., Zhou, Y. X., Gu, Q., Yang, M. X., Zhu, C. L., Peng, Z. Y., et al. (2021a). The species diversity and community assembly of arbuscular mycorrhizal fungi intypical alpine grassland in Sanjiangyuan region. Acta Pratacult. Sin. 30, 46–58.
Li, K., Hu, J., Li, T., Liu, F., Tao, J., Liu, J., et al. (2021b). Microbial abundance and diversity investigations along rivers: Current knowledge and future directions. Wiley Interdiscipl. Rev. Water 8:e1547. doi: 10.1002/wat2.1547
Li, X., Xu, M., Christie, P., Li, X., and Zhang, J. (2018). Large elevation and small host plant differences in the arbuscular mycorrhizal communities of montane and alpine grasslands on the Tibetan Plateau. Mycorrhiza 28, 605–619. doi: 10.1007/s00572-018-0850-z
Liu, J., Yu, Z., Yao, Q., Sui, Y., Shi, Y., Chu, H., et al. (2018). Ammonia-oxidizing archaea show more distinct biogeographic distribution patterns than ammonia-oxidizing bacteria across the black soil zone of Northeast China. Front. Microbiol. 9:171. doi: 10.3389/fmicb.2018.00171
Lu, K. (1999). Analytical Methods of Soil and Agricultural Chemistry. Beijing: Chinese Agriculture Science and Technology Press, doi: 10.1016/S0016-7061(01)00127-6
Lumini, E., Orgiazzi, A., Borriello, R., Bonfante, P., and Bianciotto, V. (2010). Disclosing arbuscular mycorrhizal fungal biodiversity in soil through a land-use gradient using a pyrosequencing approach. Environ. Microbiol. 12, 2165–2179. doi: 10.1111/j.1462-2920.2009.02099.x
Ma, X., Geng, Q., Zhang, H., Bian, C., Chen, H. Y. H., Jiang, D., et al. (2021). Global negative effects of nutrient enrichment on arbuscular mycorrhizal fungi, plant diversity and ecosystem multifunctionality. New Phytologist 229, 2957–2969. doi: 10.1111/nph.17077
Mao, L., Pan, J., Jiang, S., Shi, G., Qin, M., Zhao, Z., et al. (2019). Arbuscular mycorrhizal fungal community recovers faster than plant community in historically disturbed Tibetan grasslands. Soil Biol. Biochem. 134, 131–141. doi: 10.1016/j.soilbio.2019.03.026
Mcgonigle, T. P., Miller, M. H., Evans, D. G., and Swan, G. L. F. A. (1990). A New Method which Gives an Objective Measure of Colonization of Roots by Vesicular-Arbuscular Mycorrhizal Fungi. New Phytologist 115, 495–501. doi: 10.1111/j.1469-8137.1990.tb00476.x
Miller, R. M., Jastrow, J. D., and Reinhardt, D. R. (1995). External hyphal production of vesicular-arbuscular mycorrhizal fungi in pasture and tallgrass prairie communities. Oecologia 103, 17–23. doi: 10.1007/BF00328420
Miller, S. P., and Sharitz, R. R. (2000). Manipulation of flooding and arbuscular mycorrhiza formation influences growth and nutrition of two semiaquatic grass species. Funct. Ecol. 14, 738–748.
Miozzi, L., Vaira, A. M., Catoni, M., Fiorilli, V., Accotto, G. P., and Lanfranco, L. (2019). Arbuscular mycorrhizal symbiosis: plant friend or foe in the fight against viruses? Front. Microbiol. 10:1238. doi: 10.3389/fmicb.2019.01238
Mirshad, P. P., and Puthur, J. T. (2016). Arbuscular mycorrhizal association enhances drought tolerance potential of promising bioenergy grass (Saccharum arundinaceum retz.). Environ. Monit. Assess. 188:425. doi: 10.1007/s10661-016-5428-7
Mitsch, W. J., and Gosselink, J. G. (2000). The value of wetlands: importance of scale and landscape setting. Ecol. Econ. 35, 25–33. doi: 10.1016/s0921-8009(00)00165-8
Nelson, D. W., and Sommers, L. E. (1996). “Total carbon, organic carbon and organic matter, part 3: chemical methods,” in Soil Science Society of America Book Series, Number 5, eds D. L. Sparks, A. L. Page, P. A. Helmke, R. H. Loeppert, P. N. Soltanpour, M. A. Tabatabai, et al. (Madison, WI: Soil Science Society America).
Neuenkamp, L., Moora, M., Opik, M., Davison, J., Gerz, M., Mannisto, M., et al. (2018). The role of plant mycorrhizal type and status in modulating the relationship between plant and arbuscular mycorrhizal fungal communities. New Phytologist 220, 1236–1247. doi: 10.1111/nph.14995
Neupane, J., Guo, W., Cao, G., Zhang, F., Slaughter, L., and Deb, S. (2022). Spatial patterns of soil microbial communities and implications for precision soil management at the field scale. Precis. Agric. 23, 1008–1026. doi: 10.1007/s11119-021-09872-1
Newman, E. I., and Reddell, P. (1987). The distribution of mycorrhizas among families of vascular plants. New Phytol. 106, 745–751. doi: 10.1111/j.1469-8137.1987.tb00175.x
Ohsowski, B. M., Zaitsoff, P. D., Oepik, M., and Hart, M. M. (2014). Where the wild things are: looking for uncultured Glomeromycota. New Phytologist 204, 171–179. doi: 10.1111/nph.12894
Olsen, S. R. (1954). Estimation of available phosphorus in soils by extraction with sodium bicarbonate. Washington, DC: United States Department of Agriculture.
Powell, J. R., and Rillig, M. C. (2018). Biodiversity of arbuscular mycorrhizal fungi and ecosystem function. New Phytologist 220, 1059–1075. doi: 10.1111/nph.15119
Qin, J., Sun, Y., Qiu, X., Liu, H., Zhang, E., and Mao, X. (2021). Distribution pattern simulation of multiple emergent plants in river riparian zones. River Res. Appl. 37, 1180–1190. doi: 10.1002/rra.3721
Read, D. S., Gweon, H. S., Bowes, M. J., Newbold, L. K., Field, D., Bailey, M. J., et al. (2015). Catchment-scale biogeography of riverine bacterioplankton. ISME J. 9, 516–526. doi: 10.1038/ismej.2014.166
Shi, G., Yao, B., Liu, Y., Jiang, S., Wang, W., Pan, J., et al. (2017). The phylogenetic structure of AMF communities shifts in response to gradient warming with and without winter grazing on the Qinghai-Tibet Plateau. Appl. Soil Ecol. 121, 31–40. doi: 10.1016/j.apsoil.2017.09.010
Sikes, B. A., Cottenie, K., and Klironomos, J. N. (2009). Plant and fungal identity determines pathogen protection of plant roots by arbuscular mycorrhizas. J. Ecol. 97, 1274–1280. doi: 10.1111/j.1365-2745.2009.01557.x
Staddon, P. L., Gregersen, R., and Jakobsen, I. (2004). The response of two Glomus mycorrhizal fungi and a fine endophyte to elevated atmospheric CO2, soil warming and drought. Global Change Biol. 10, 1909–1921.
Van, D. H. M. G., and Klironomos, J. N. (1998). Mycorrhizal fungal diversity determines plant biodiversity, ecosystem variability and productivity. Nature 396, 69–72.
Van Aarle, I. M., Olsson, P. A., and Soderstrom, B. (2002). Arbuscular mycorrhizal fungi respond to the substrate pH of their extraradical mycelium by altered growth and root colonization. New Phytologist 155, 173–182. doi: 10.1046/j.1469-8137.2002.00439.x
van der Heijden, M. G. A., Martin, F. M., Selosse, M., and Sanders, I. R. (2015). Mycorrhizal ecology and evolution: the past, the present, and the future. New Phytologist 205, 1406–1423. doi: 10.1111/nph.13288
van der Heyde, M., Ohsowski, B., Abbott, L. K., and Hart, M. (2017b). Arbuscular mycorrhizal fungus responses to disturbance are context-dependent. Mycorrhiza 27, 431–440. doi: 10.1007/s00572-016-0759-3
van der Heyde, M., Bennett, J. A., Pither, J., and Hart, M. (2017a). Longterm effects of grazing on arbuscular mycorrhizal fungi. Agr. Ecosyst. Environ. 243, 27–33. doi: 10.1016/j.agee.2017.04.003
Yang, R., Qin, Z., Wang, J., Xu, S., Zhao, W., Zhang, X., et al. (2020). Salinity changes root occupancy by arbuscular mycorrhizal fungal species. Pedobiologia 81–82:150665. doi: 10.1016/j.pedobi.2020.150665
Yang, W., Li, S., Wang, X., Liu, F., Li, X., and Zhu, X. (2021). Soil properties and geography shape arbuscular mycorrhizal fungal communities in black land of China. Appl. Soil Ecol. 167:104109. doi: 10.1016/j.apsoil.2021.104109
Yu, Z., Wu, G., Keys, L., Li, F., Yan, N., Qu, D., et al. (2019). Seasonal variation of chemical weathering and its controlling factors in two alpine catchments, Nam Co basin, central Tibetan Plateau. J. Hydrol. 576, 381–395. doi: 10.1016/j.jhydrol.2019.06.042
Zhang, J., Wang, F., Che, R., Wang, P., Liu, H., Ji, B., et al. (2016). Precipitation shapes communities of arbuscular mycorrhizal fungi in Tibetan alpine steppe. Sci. Rep. 6:23488. doi: 10.1038/srep23488
Zhang, J., Wang, P., Xue, K., Hao, Y., Wang, Y., and Cui, X. (2019). Trait complementarity between fine roots of Stipa purpurea and their associated arbuscular mycorrhizal fungi along a precipitation gradient in Tibetan alpine steppe. J. Mt. Sci.-Engl. 16, 542–547. doi: 10.1007/s11629-018-5311-9
Zhang, S., Li, K., Hu, J., Wang, F., Chen, D., Zhang, Z., et al. (2022). Distinct assembly mechanisms of microbial sub-communities with different rarity along the Nu River. J. Soil. Sediment. 22, 1530–1545. doi: 10.1007/s11368-022-03149-4
Zhu, L., Xie, M., and Wu, Y. (2010). Quantitative analysis of lake area variations and the influence factors from 1971 to 2004 in the Nam Co basin of the Tibetan Plateau. Chin. Sci. Bull. 55, 1294–1303. doi: 10.1007/s11434-010-0015-8
Keywords: alpine riparian soils, arbuscular mycorrhizal fungi, community distribution, driving factor, Nam Co watershed
Citation: Zhou Y, Chen K, Muneer MA, Li C, Shi H, Tang Y, Zhang J and Ji B (2022) Soil moisture and pH differentially drive arbuscular mycorrhizal fungal composition in the riparian zone along an alpine river of Nam Co watershed. Front. Microbiol. 13:994918. doi: 10.3389/fmicb.2022.994918
Received: 15 July 2022; Accepted: 08 September 2022;
Published: 30 September 2022.
Edited by:
Decai Jin, Research Center for Eco-Environmental Sciences (CAS), ChinaReviewed by:
Xiangnan Li, Northeast Institute of Geography and Agroecology (CAS), ChinaXi-En Long, Nantong University, China
Copyright © 2022 Zhou, Chen, Muneer, Li, Shi, Tang, Zhang and Ji. This is an open-access article distributed under the terms of the Creative Commons Attribution License (CC BY). The use, distribution or reproduction in other forums is permitted, provided the original author(s) and the copyright owner(s) are credited and that the original publication in this journal is cited, in accordance with accepted academic practice. No use, distribution or reproduction is permitted which does not comply with these terms.
*Correspondence: Jing Zhang, zhangjing_2019@bjfu.edu.cn