- 1School of Biotechnology and Bioengineering, West Yunnan University, Lincang, China
- 2Chongqing Key Laboratory of Economic Plant Biotechnology, College of Landscape Architecture and Life Science/Institute of Special Plants, Chongqing University of Arts and Sciences, Yongchuan, China
- 3Lincang Academy of Forestry, Lincang, China
- 4Department of Biological Sciences, Virginia Polytechnic Institute and State University, Blacksburg, VA, United States
Macadamia (Macadamia integrifolia) trees are an important source of revenue in rainforest ecosystems. Their nuts are rich in vitamins, minerals, fiber, antioxidants, and monounsaturated oils. The fungus Lasiodiplodia theobromae, however, is a major disease problem, causing kernel rot and other disease symptoms. In the present study, a dual confrontation assay was used to evaluate the inhibitory effect of an endophytic strain of Trichoderma hamatum C9 from macadamia root against L. theobromae. Volatiles and cell-free culture filtrate of T. hamatum were also used to assess their antifungal activity against L. theobromae. Results suggested that T. hamatum exhibited a significant inhibitory effect against L. theobromae in vitro. Further results of a biocontrol assay indicated that a spray treatment of T. hamatum conidial suspension significantly decreased the size of lesions caused by artificially inoculated L. theobromae on macadamia leaves, as well as the disease index in young trees inoculated with L. theobromae, relative to sterile water controls. Collectively, our findings indicate that T. hamatum C9 represents a potential biocontrol agent that can be used to manage L. theobromae on macadamia.
Introduction
Macadamia (Macadamia integrifolia) is an evergreen tree native to rainforest regions of southeastern Australia (Trueman, 2013). Over the past century, macadamia nuts have become an important internationally traded product (Carr, 2013). They have a high content of oil (69–78 g per 100 g fresh weight) and a relatively low percentage of saturated fatty acids. The consumption of oils with low levels of saturated fatty acids helps to improve blood lipid profiles and decreases inflammation and oxidative stress, thus, contributing to lowering body mass and generally reducing risk factors associated with cardiovascular disease (Aquino-Bolaños et al., 2017). Moreover, macadamia kernels are a rich source of tocotrienols and squalene, which are also considered to be nutraceuticals, and macadamia oil is a common product obtained from the processing of macadamia nuts (Wall, 2010; Navarro and Rodrigues, 2016).
The introduction and trial planting of macadamia in China began in the 1970s, and now macadamia is cultivated in the provinces of Guangdong, Yunnan, Guangxi, and Guizhou. The planted area of macadamia in China exceeded 301,206 hm2 by the end of 2018, and now China has the largest and fastest growing macadamia industry, accounting for over 1/3 of global production acreage (Shuai et al., 2022). Macadamia plants have been reported to be susceptible to a variety of fungal pathogens that can variously infect flower, leaf, fruit, stem, and root tissues (Akinsanmi and Drenth, 2006, 2017; Akinsanmi et al., 2016a,b; Prasannath et al., 2021a,b; Li et al., 2022). In this regard, species in the genus Lasiodiplodia represent a serious concern for the crop. Lasiodiplodia sp. (Akinsanmi and Drenth, 2017) and Lasiodiplodia pseudotheobromae (Chang et al., 2019) are responsible for causing husk rot resulting in diffuse soft and spongy black lesions on the fruit pericarp. Lasiodiplodia theobromae can also cause trunk cankers and shoot necrosis (Fischer et al., 2017). It has been reported that L. theobromae has a wide host range and geographical distribution, particularly in tropical and subtropical regions (Salvatore et al., 2020). Its pycnidia are stromatic, globose, and ostiolate. Conidia are initially hyaline, 1-celled and subovoid. When mature, typical conidia are 1-septate, brown and measured 26–31 × 12–16 μm (Fischer et al., 2017). Although macadamia cultivation still relies on the use of synthetic chemicals like carbendazim and pyraclostrobin (Akinsanmi et al., 2008; Khun et al., 2021) to minimize disease problems, there is a broad trend to explore and develop biocontrol agents, including the use of beneficial fungal endophytes, to manage tree diseases (Sosso et al., 2021).
Different species and strains of Trichoderma have been extensively studied and employed as biocontrol agents, due to their ubiquitous presence in soils, high efficacy, and established regulatory approval (Alghuthaymi et al., 2022). Trichoderma has been reported to be a dominant component of various soil mycobiomes, as well as a common fungal endophyte with biocontrol potential and plant growth promotion activity (Castro-Restrepo et al., 2022; Siebatcheu et al., 2022; Tyśkiewicz et al., 2022). Thus, Trichoderma has been widely used as a component of environmentally friendly agricultural management practices (Zin and Badaluddin, 2020). In this regard, Trichoderma hamatum has been recognized for its ability to induce systemic resistance in host plants and secrete antifungal compounds (Shaw et al., 2016; Abdelkhalek et al., 2022). It is known Trichoderma serves as a producer of volatile organic compounds; in particular, 6-n-pentyl-2H-pyran-2-one (6-PAP) (Jeleń et al., 2014) is very considered recently as a determinant of effects in plant protection. Studies utilizing Trichoderma as a biocontrol agent to manage macadamia tree diseases, however, are limited. The main objective of the present study was to evaluate the ability of T. hamatum to inhibit L. theobromae in vitro, as well to limit disease on macadamia leaves and whole plants. The antifungal activity of volatiles and cell-free culture filtrate of T. hamatum against L. theobromae was also assessed.
Materials and methods
Biocontrol and pathogenic fungi
The endophytic fungus, T. hamatum strain C9, was originally isolated in our laboratory from a root of a healthy macadamia tree (M. integrifolia × M. tetraphylla hybrid cv. A4) growing in a major production area in Lincang City, Yunnan Province, China (24°1′-24°11′N, 99°33′-99°43′E). The fungal pathogen, L. theobromae strain L1, was originally isolated from a root of an infected macadamia tree growing in the same region. Both fungal isolates were identified based on their morphology and the nucleotide sequence of ITS rDNA (Malachová et al., 2020; Baazeem et al., 2021). Specifically, the partial nucleotide sequences of ITS rDNA of the strain C9 (Figure 1A) and the strain L1 (Figure 1B) obtained in our study were 100% identical to those of T. hamatum isolate F4 (NCBI Accession: MT341773.1) and L. theobromae isolate FH14K03 (NCBI Accession: MK886711.1), respectively. Both fungi were cultured on PDA (potato dextrose agar) and grown at 25°C prior to use.
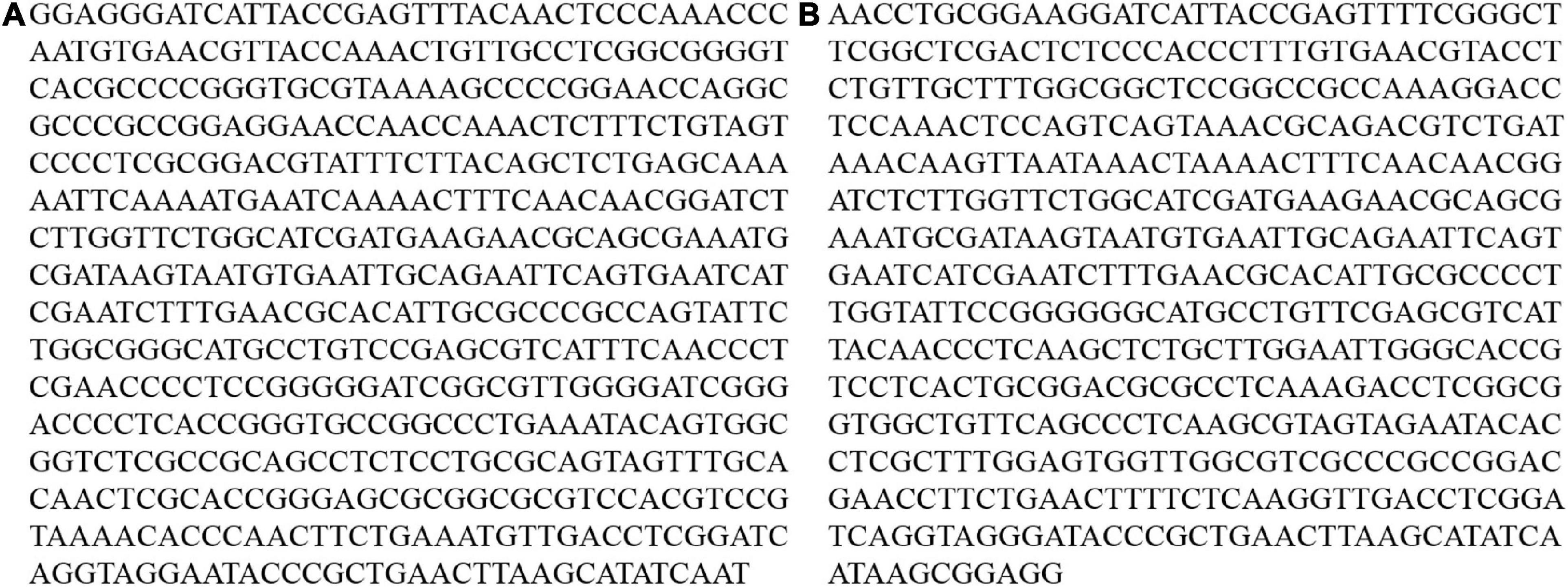
Figure 1. The partial nucleotide sequences of ITS rDNA of the strain C9 (A) and the strain L1 (B) obtained in our study.
Plant material
Macadamia (M. integrifolia × M. tetraphylla hybrid cv. A4) plants were purchased in September 2021, from suppliers in the macadamia production area where the fungi were collected. Three-year-old plants with a height of 1 – 1.3 m and fully expanded leaves from the top third of the plants were used in this study.
Dual confrontation assay
A dual confrontation assay was used to quantify the interaction between T. hamatum C9 and L. theobromae L1 in vitro (Stracquadanio et al., 2020). Mycelial disks (7 mm in diameter) obtained from the margins of 5-day-old PDA cultures of T. hamatum and L. theobromae were placed on opposite sides (50-mm distance) of a 90-mm PDA plate. The PDA plates were then incubated at 25°C for 72 h. The radial growth of L. theobromae (the smallest colony diameter) was then measured in the presence (treatment) or absence (control) of T. hamatum. Three biological replicates were used in each assay and the assay was repeated three times.
Assessment of Trichoderma hamatum C9 cell-free culture filtrate and volatiles on the growth of Lasiodiplodia theobromae L1
A conidial suspension of T. hamatum was obtained from 5-day-old PDA cultures and adjusted to a concentration of 1 × 106 spores/ml that was quantified with a hemocytometer. Then, 100 μl of the conidial suspension was added to 100 ml of PDB and the inoculated broth was incubated for 3 day at 25°C on a rotary shaker set at 180 rpm. The 3-day-old cultures were subsequently used to obtain cell-free culture filtrate as previously described (Wonglom et al., 2019). Briefly, the PDB cultures were first filtered through Whatman filter paper Grade 44 using a vacuum filtration system and then filtered again through a 0.20-μm cellulose acetate syringe filter to obtain T. hamatum cell-free culture filtrate. Mycelial disks (7 mm in diameter) from 5-day-old PDA cultures of L. theobromae were placed in the center of Petri dishes (90 mm in diameter) containing 20 ml of PDA amended with different concentrations of the cell-free culture filtrate (0 [control], 0.5, 1, 5, and 10% v/v) and incubated at 25°C. The radial growth of L. theobromae was determined by measuring colony diameter after 48 h of incubation on the PDA plates.
A confrontation culture assay was conducted in a Petri dish with two-sections to determine the presence of antifungal activity of the volatiles produced by T. hamatum. Mycelial disks (7 mm in diameter) from a 5-day-old PDA culture of T. hamatum and L. theobromae were separately placed at the center of each section of the PDA petri plate and incubated at 25°C (Mao et al., 2019). Mycelial plugs from 5-day-old PDA plates without T. hamatum were used as a control. Antifungal activity of T. hamatum volatiles against L. theobromae was assessed after 48 h of coincubation using the following formula: Percent inhibition by T. hamatum volatiles = [(the largest diameter of L. theobromae colony in the control plates – the largest diameter of L. theobromae colony in the treatment plates)/the largest diameter of L. theobromae colony in control plates] × 100. Three biological replicates were utilized in each assay and the assay was repeated three times.
Biocontrol efficacy of Trichoderma hamatum against Lasiodiplodia theobromae L1 on macadamia leaves in vitro
A conidial suspension of T. hamatum (1 × 106 spores/ml) was sprayed evenly on the upper surface of fully expanded macadamia leaves that had been removed from macadamia plants. The leaves were air-dried and then two wounds were made on each leaf using sterilized needles. Each of the wounds on each wounded leaf was subsequently inoculated by placing a mycelial disk (5 mm in diameter) of L. theobromae over the wound site. Leaves sprayed with T. hamatum and inoculated with blank PDA disk without L. theobromae served as a positive (healthy) control, while leaves that were sprayed with sterilized water, wounded, and then inoculated with L. theobromae served as a negative (disease) control. All of the treated leaves were incubated on sterilized wet filter papers in Petri dishes for 4 days, after which average lesion area on each wound was determined. Three biological replicates (10 leaves for each replicate) were used in each assay and the assay was repeated three times.
Biocontrol efficacy of Trichoderma hamatum against Lasiodiplodia theobromae on macadamia plants
Three-year-old potted macadamia plants ranging between 1 and 1.3 m in height were used in the biocontrol assay. The plant trunk (3 cm above the ground soil) of each plant was wounded with a 5-mm punch and injected with 5-ml of a conidial suspension (1 × 106 spores/ml) of T. hamatum, and after air drying, subsequently inoculated with mycelial disks (5 mm in diameter) of L. theobromae. Each wound was covered with a wet, sterilized piece of cloth and sealed with plastic wrap. Wounded plants inoculated with 5-ml conidial suspension of T. hamatum (1 × 106 spores/ml) and sterile PDA disks served as a positive (healthy) control, while wounded plants inoculated with 5-ml sterilized water and mycelial disks of L. theobromae served as a negative (disease) control. Three biological replicates (five plants for each replicate) were used in each assay and the assay was repeated three times. Disease severity was assessed at 20 days after inoculation using the following disease lesion scale: 0 = no spots, 1 = spot area 0–20%, 2 = 20–40%, 3 = 40–60%, 4 = 60–80% with 50% of the spots coalesced 50%, 5 = 80–100% with 75% of the spots coalesced. The disease lesion scale scores were converted to a disease severity index (DSI) using the following formula (Promwee et al., 2017):
Statistical analysis
All statistical analyses were performed using SPSS version 20.0 (SPSS Inc., United States) software. Data with a single variable (treatment) were analyzed by a one-way ANOVA. Mean separations in Figure 2 were performed using a Student’s t-test, while mean separations in Figures 3–5 were performed using a Duncan’s multiple range test. Differences at P < 0.05 were considered significant. Data presented were pooled across three independent repeated experiments. As the experiment was not a significant variable, the statistical analyses were conducted on the pooled data (n = 9).
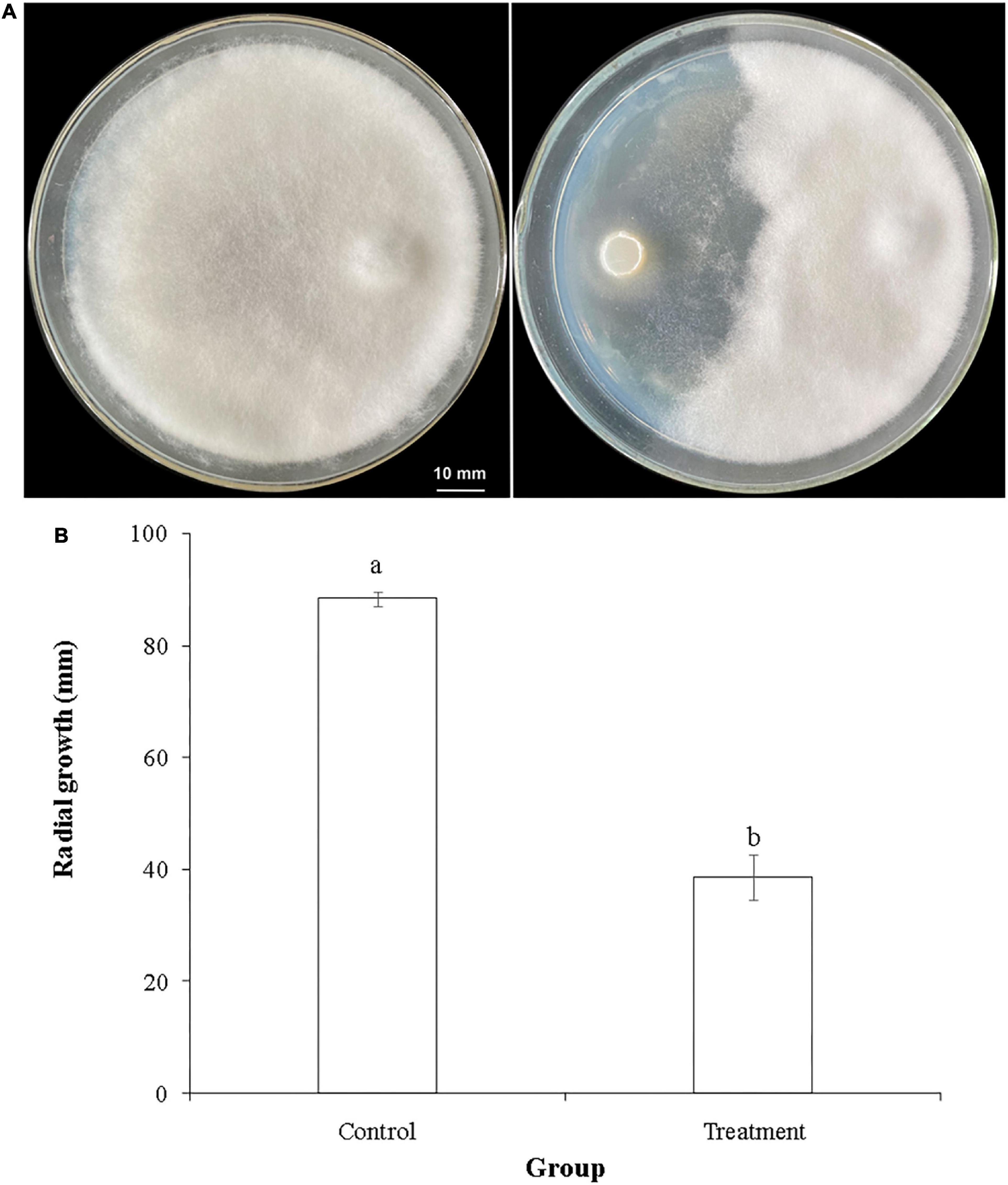
Figure 2. Inhibitory activity of T. hamatum against L. theobromae in a dual confrontation assay. (A) Representative photo of the radial growth of L. theobromae in the absence of (left panel) or presence of (right panel) T. hamatum. (B) Quantitative assessment of radial growth (PIRG) of L. theobromae in the absence (control) or presence (treatment) of T. hamatum. Different letters above each column indicate a significant difference (P < 0.05) between control and treatment groups according to Student’s t-test. Data represent the mean ± SD (n = 9).
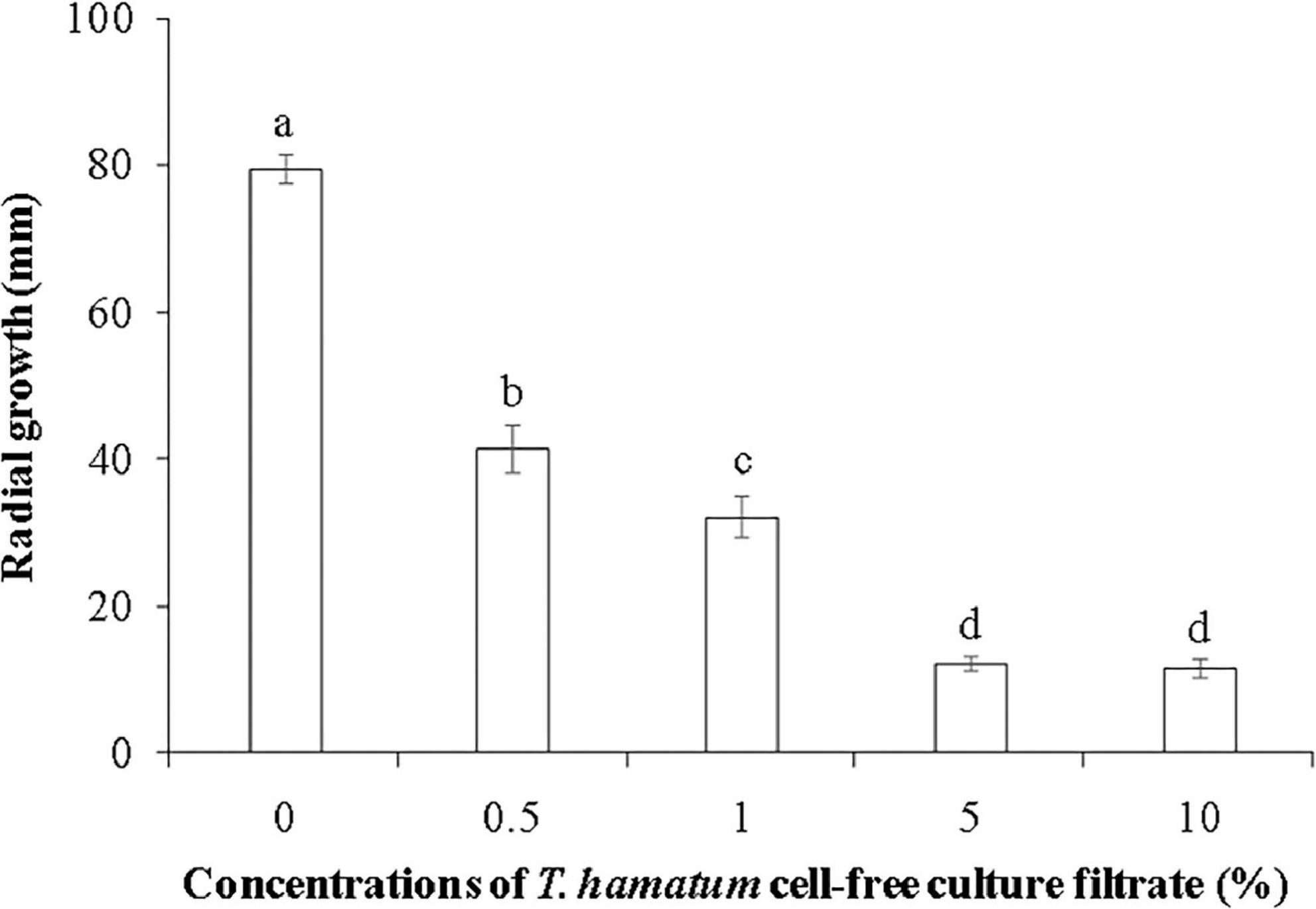
Figure 3. The effect of different concentrations of T. hamatum cell-free culture filtrate on the radial growth of L. theobromae. L. theobromae was grown on PDA medium amended with various concentrations of T. hamatum cell-free culture filtrate. Radial growth measurements (mm) were taken after 48 h of culture. Different letters above each column indicate a significant difference (P < 0.05) according to Duncan’s multiple range test. Data represent the mean ± SD (n = 9).
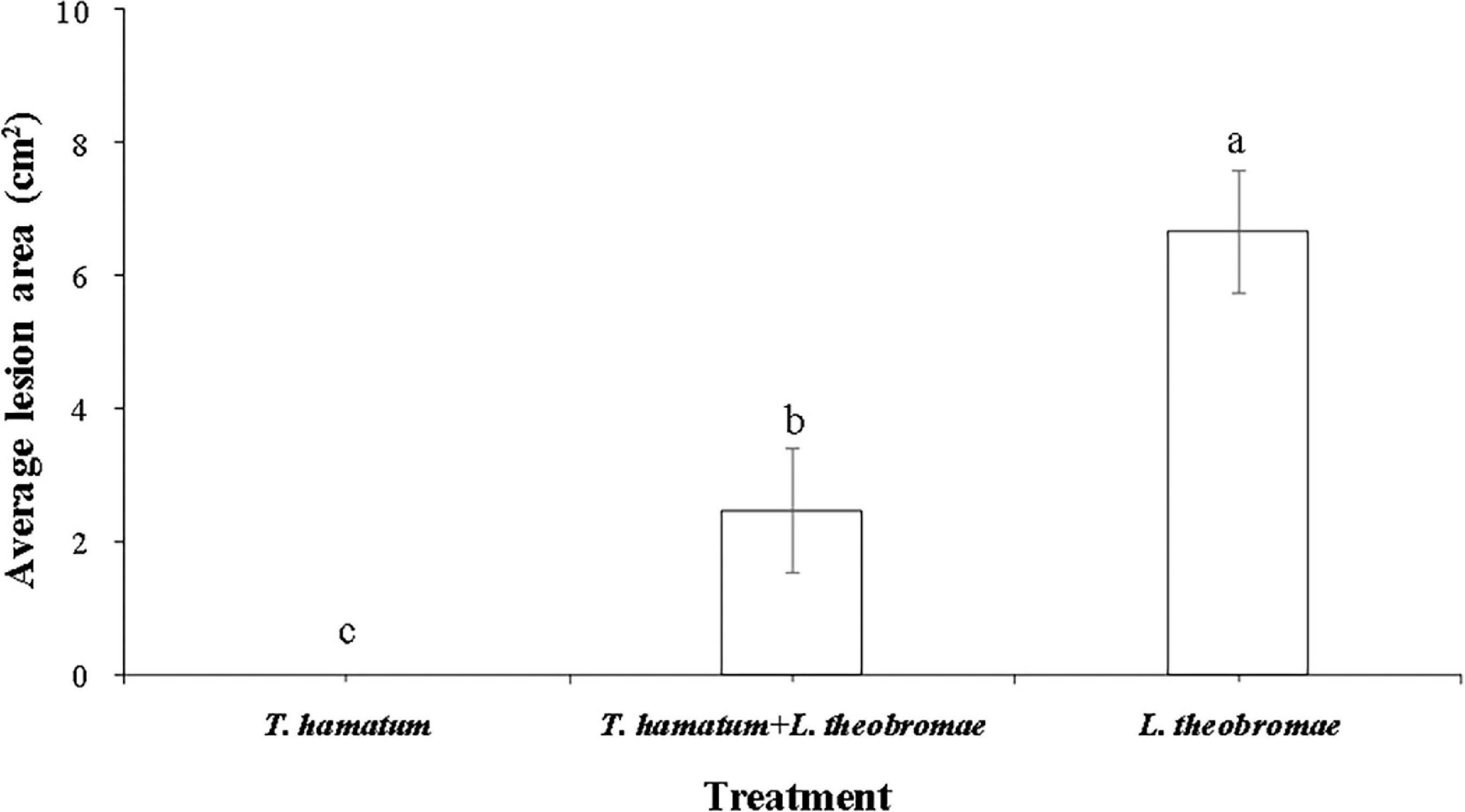
Figure 4. The quantitative data of lesion areas caused by L. theobromae on macadamia leaves 4 days after inoculation for the three treatment groups. (I) Leaves treated only with T. hamatum (healthy control); (II) Leaves treated with T. hamatum + L. theobromae; (III) Leaves treated only with L. theobromae (disease control). Different letters above each column indicate a significant difference (P < 0.05) according to Duncan’s multiple range test. Data represent the mean ± SD (n = 9).
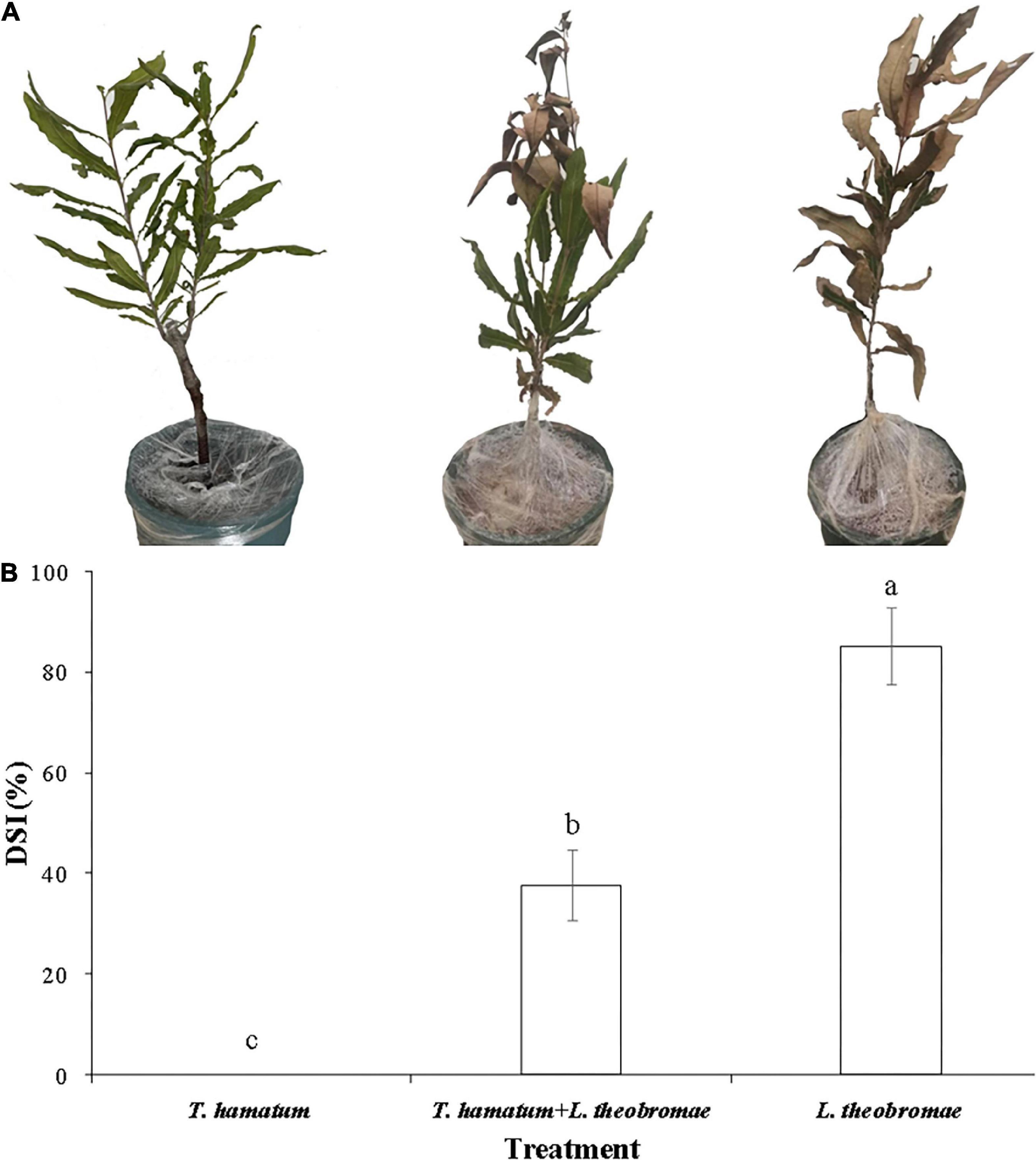
Figure 5. Disease symptoms and disease severity index (DSI) of potted macadamia plants infected with L. theobromae 20 days after inoculation. (A) Representative photos of potted, three-year-old macadamia trees untreated or treated with a conidial suspension of T. hamatum and then inoculated or non-inoculated with L. theobromae. Photos from left to right represent plants treated only with T. hamatum (healthy control), plants treated with T. hamatum + L. theobromae, and plants treated only with L. theobromae (disease control). (B) The corresponding disease severity index (DSI) of the three treatment groups. Different letters above each column indicate a significant difference (P < 0.05) according to a Duncan’s multiple range test. Data represent the mean ± SD (n = 9).
Results and discussion
Trichoderma hamatum has been reported to have biocontrol activity against several fungal plant pathogens, including Sclerotinia spp. (Rabeendran et al., 2006), Fusarium oxysporum (Mao et al., 2020), Rhizoctonia solani, and Pythium ultimum (Lewis et al., 1996). The genome of T. hamatum GD12 has been sequenced and has provided fundamental information for studying its beneficial traits (Studholme et al., 2013). Results of the dual confrontation assay conducted in the present study indicated that T. hamatum had a significant inhibitory effect against L. theobromae, one of the major fungal pathogens of macadamia (Figure 2A). The radial growth of L. theobromae was significantly inhibited by T. hamatum (Figure 2B), exhibiting a percent inhibition of 56.3%. We hypothesized that non-volatile metabolites and/or volatiles produced by T. hamatum might contribute to its inhibitory activity against L. theobromae. Therefore, we assessed the inhibitory activity of cell-free culture filtrate and volatiles against L. theobromae.
Cell-free culture filtrates of Trichoderma spp., including T. hamatum, have been reported to have antifungal properties (Reino et al., 2008; Baiyee et al., 2019; Baazeem et al., 2021). Padder and Sharma (2011) reported that culture filtrate of T. hamatum had a significant inhibitory effect on spore germination of Colletotrichum lindemuthianum, and the inhibitory activity of culture filtrates of Trichoderma sp. have also been reported against other fungal pathogens, including Fusarium solani (Dugassa et al., 2021) and F. oxysporum (Shanmugam et al., 2008). In the current study, the cell-free culture filtrate of T. hamatum at a concentration ranging from 0.5 to 10% markedly inhibited the mycelial growth of L. theobromae, with inhibitory activity increasing with concentration. No difference in inhibitory activity was observed, however, between the 5 and 10% concentrations of cell-free culture filtrate (Figure 3).
Volatiles produced by Trichoderma spp. may also contribute to the inhibitory activity displayed against fungal pathogens (Li et al., 2018; Guo et al., 2019). Results of our culture assays support this premise. The culture assay utilizing Petri dishes with two separate sections clearly indicated that the volatiles produced by T. hamatum had a significant inhibitory effect on the growth of L. theobromae, with the percentage inhibition reaching 32.4%. The volatiles produced by Trichoderma species have been reported to include sesquiterpenes, diterpenes, and tetraterpenes (Lee et al., 2016), with Trichoderma species differing in the profile of the volatiles they produce based on specific fungal interactions (Guo et al., 2019). Therefore, the specific volatiles produced by T. hamatum in the presence of L. theobromae and the contribution of specific volatiles to the inhibitory activity need to be further investigated.
Lasiodiplodia theobromae can cause cankers on the trunks of macadamia tree and shoot necrosis (Fischer et al., 2017). In the present study, we found that L. theobromae can also cause lesions on macadamia leaves. When leaves were sprayed with a conidial suspension of T. hamatum (1 × 106 spores/ml), however, the lesion area resulting from L. theobromae infection significantly decreased, relative to leaves treated only with L. theobromae (Figure 4). At present, there is limited information on the biocontrol efficacy of Trichoderma species against macadamia leaf diseases. We speculate that the non-volatile and/or volatile antifungal metabolites (Figures 2, 3) produced by T. hamatum may contribute to its biocontrol aptitude. The efficacy of biocontrol agents in vitro, however, does not guarantee their efficacy in planta (Collinge et al., 2022). Therefore, we also assessed the biocontrol efficacy of T. hamatum against L. theobromae on potted, three-year-old, macadamia plants. Macadamia trees are susceptible to a variety of fungal pathogens (Akinsanmi et al., 2016b; Wrona et al., 2020) and insects (Khun et al., 2021), which can result in significant economic losses. Studies on the biocontrol of insect have been significantly more numerous than studies on the biocontrol of plant diseases (Gutierrez-Coarite et al., 2018; Polaszek et al., 2020). In our present study, T. hamatum exhibited a high level of biocontrol efficacy against L. theobromae on three-year-old, potted macadamia trees (Figure 5A). The application of a conidial suspension (1 × 106 spores/ml) of T. hamatum to wounds on the main stem of macadamia trees significantly decreased the DSI of L. theobromae from 85.1 to 37.7% (Figure 5B), indicating the good potential for use of T. hamatum as a biocontrol agent for the management of fungal diseases on macadamia.
Conclusion
The present study demonstrated that T. hamatum can inhibit the growth of L. theobromae in vitro, and decrease lesion size on detached leaves, and disease severity on potted, three-year-old macadamia plants. Our study also indicates that non-volatile and volatile metabolites of T. hamatum may contribute to its inhibitory properties. Further, detailed studies on the mechanisms responsible for biocontrol activity, however, are needed. In particular, other potential modes of action of T. hamatum against L. theobromae, such as the induction of disease resistance and mycoparasitism, may also contribute and need to be investigated.
Data availability statement
The original contributions presented in this study are included in the article/supplementary material, further inquiries can be directed to the corresponding authors.
Author contributions
XiL, JLe, LY, JLi, and YS: conceptualization. XiL and YS: project administration. HB: resources. JLe and XuL: data curation. XiL, LY, JLi, and YS: writing – original draft. MW, LY, JLi, and YS: writing – review and editing. All authors contributed to the article and approved the submitted version.
Funding
This study was supported by the Yunnan Fundamental Research Projects (grant no. 2019FD123) and Chongqing Talents – Innovation Leader Project to YS.
Conflict of interest
The authors declare that the research was conducted in the absence of any commercial or financial relationships that could be construed as a potential conflict of interest.
Publisher’s note
All claims expressed in this article are solely those of the authors and do not necessarily represent those of their affiliated organizations, or those of the publisher, the editors and the reviewers. Any product that may be evaluated in this article, or claim that may be made by its manufacturer, is not guaranteed or endorsed by the publisher.
References
Abdelkhalek, A., Al-Askar, A. A., Arishi, A. A., and Behiry, S. I. (2022). Trichoderma hamatum strain Th23 promotes tomato growth and induces systemic resistance against tobacco mosaic virus. J. Fungi 8:228. doi: 10.3390/jof8030228
Akinsanmi, O. A., and Drenth, A. (2006). First report of Tubercularia lateritia as the causal agent of canker on macadamia. Australas. Plant Dis. Notes 1, 49–51. doi: 10.1071/DN06019
Akinsanmi, O. A., and Drenth, A. (2017). Characterisation of husk rot in macadamia. Ann. Appl. Biol. 170, 104–115. doi: 10.1111/aab.12320
Akinsanmi, O. A., Miles, A. K., and Drenth, A. (2008). Alternative fungicides for controlling husk spot caused by Pseudocercospora macadamiae in macadamia. Australas. Plant Pathol. 37, 141–147. doi: 10.1071/AP08001
Akinsanmi, O. A., Nisa, S., Jeff-Ego, O. S., Shivas, R. G., and Drenth, A. (2016a). Dry flower disease of macadamia in Australia caused by Neopestalotiopsis macadamiae sp. nov. and Pestalotiopsis macadamiae sp. nov. Plant Dis. 101, 45–53. doi: 10.1094/PDIS-05-16-0630-RE
Akinsanmi, O. A., Wang, G., Neal, J., Russell, D., Drenth, A., and Topp, B. (2016b). Variation in susceptibility among macadamia genotypes and species to Phytophthora root decay caused by Phytophthora cinnamomi. Crop Prot. 87, 37–43. doi: 10.1016/j.cropro.2016.04.021
Alghuthaymi, M. A., Abd-Elsalam, K. A., AboDalam, H. M., Ahmed, F. K., Ravichandran, M., Kalia, A., et al. (2022). Trichoderma: An eco-friendly source of nanomaterials for sustainable agroecosystems. J. Fungi 8:367. doi: 10.3390/jof8040367
Aquino-Bolaños, E. N., Mapel-Velazco, L., Martín-del-Campo, S. T., Chávez-Servia, J. L., Martínez, A. J., and Verdalet-Guzmán, I. (2017). Fatty acids profile of oil from nine varieties of Macadamia nut. Int. J. Food Prop. 20, 1262–1269. doi: 10.1080/10942912.2016.1206125
Baazeem, A., Almanea, A., Manikandan, P., Alorabi, M., Vijayaraghavan, P., and Abdel-Hadi, A. (2021). In vitro antibacterial, antifungal, nematocidal and growth promoting activities of Trichoderma hamatum FB10 and its secondary metabolites. J. Fungi 7:331. doi: 10.3390/jof7050331
Baiyee, B., Pornsuriya, C., Ito, S. I., and Sunpapao, A. (2019). Trichoderma spirale T76-1 displays biocontrol activity against leaf spot on lettuce (Lactuca sativa L.) caused by Corynespora cassiicola or Curvularia aeria. Biol. Control 129, 195–200. doi: 10.1016/j.biocontrol.2018.10.018
Carr, M. K. V. (2013). The water relations and irrigation requirements of macadamia (Macadamia Spp.): A review. Exp. Agric. 49, 74–90. doi: 10.1017/S0014479712000804
Castro-Restrepo, D., Dominguez, M. I., Gaviria-Gutiérrez, B., Osorio, E., and Sierra, K. (2022). Biotization of endophytes Trichoderma asperellum and Bacillus subtilis in Mentha spicata microplants to promote growth, pathogen tolerance and specialized plant metabolites. Plants 11:1474. doi: 10.3390/plants11111474
Chang, J. M., Zhan, R. L., Liu, F., and Wu, J. B. (2019). First report of Lasiodiplodia pseudotheobromae causing husk rot in macadamia. Plant Dis. 103:153. doi: 10.1094/PDIS-06-18-1048-PDN
Collinge, D. B., Jensen, D. F., Rabiey, M., Sarrocco, S., Shaw, M. W., and Shaw, R. H. (2022). Biological control of plant diseases – What has been achieved and what is the direction? Plant Pathol. 71, 1024–1047. doi: 10.1111/ppa.13555
Dugassa, A., Alemu, T., and Woldehawariat, Y. (2021). In-vitro compatibility assay of indigenous Trichoderma and Pseudomonas species and their antagonistic activities against black root rot disease (Fusarium solani) of faba bean (Vicia faba L.). BMC Microbiol. 21:115. doi: 10.1186/s12866-021-02181-7
Fischer, I. H., Perdona, M. J., Cruz, J. C. S., and Firmino, A. C. (2017). First report of Lasiodiplodia theobromae on Macadamia integrifolia in Brazil. Summa Phytopathol. 43:70. doi: 10.1590/0100-5405/169154
Guo, Y., Ghirardo, A., Weber, B., Schnitzler, J. P., Benz, J. P., and Rosenkranz, M. (2019). Trichoderma species differ in their volatile profiles and in antagonism toward ectomycorrhiza Laccaria bicolor. Front. Microbiol. 10:891. doi: 10.3389/fmicb.2019.00891
Gutierrez-Coarite, R., Mollinedo, J., Cho, A., and Wright, M. G. (2018). Canopy management of macadamia trees and understory plant diversification to reduce macadamia felted coccid (Eriococcus ironsidei) populations. Crop Prot. 113, 75–83. doi: 10.1016/j.cropro.2018.07.014
Jeleń, H., Błaszczyk, L., Chełkowski, J., Rogowicz, K., and Strakowska, J. (2014). Formation of 6-n-pentyl-2H-pyran-2-one (6-PAP) and other volatiles by different Trichoderma species. Mycol. Prog. 13, 589–600. doi: 10.1007/s11557-013-0942-2
Khun, K. K., Ash, G. J., Stevens, M. M., Huwer, R. K., and Wilson, B. A. (2021). Compatibility of Metarhizium anisopliae and Beauveria bassiana with insecticides and fungicides used in macadamia production in Australia. Pest Manag. Sci. 77, 709–718. doi: 10.1002/ps.6065
Lee, S., Yap, M., Behringer, G., Hung, R., and Bennett, J. W. (2016). Volatile organic compounds emitted by Trichoderma species mediate plant growth. Fungal Biol. Biotechnol. 3:7. doi: 10.1186/s40694-016-0025-7
Lewis, J. A., Lumsden, R. D., and Locke, J. C. (1996). Biocontrol of damping-off diseases caused by Rhizoctonia solani and Pythium ultimum with alginate prills of Gliocladium virens, Trichoderma hamatum and various food bases. Biocontrol Sci. Technol. 6, 163–174. doi: 10.1080/09583159650039368
Li, N., Alfiky, A., Wang, W., Islam, M., Nourollahi, K., Liu, X., et al. (2018). Volatile compound-mediated recognition and inhibition between Trichoderma biocontrol agents and Fusarium oxysporum. Front. Microbiol. 9:2614. doi: 10.3389/fmicb.2018.02614
Li, Y. P., Finnegan, P. M., Liu, M., Zhao, J. X., Nie, Y. L., and Tang, L. (2022). First report of Neofusicoccum parvum causing leaf spot disease on Macadamia integrifolia in China. Plant Dis. doi: 10.1094/PDIS-02-22-0299-PDN [Epub ahead of print].
Malachová, K., Novotný, Č, Adamus, G., Lotti, N., Rybková, Z., Soccio, M., et al. (2020). Ability of Trichoderma hamatum isolated from plastics-polluted environments to attack petroleum-based, synthetic polymer films. Processes 8:467. doi: 10.3390/pr8040467
Mao, L. J., Chen, J. J., Xia, C. Y., Feng, X. X., Kong, D. D., Qi, Z. Y., et al. (2019). Identification and characterization of new Muscodor endophytes from gramineous plants in Xishuangbanna, China. MicrobiologyOpen 8:e00666. doi: 10.1002/mbo3.666
Mao, T., Chen, X., Ding, H., Cheng, X., and Jiang, X. (2020). Pepper growth promotion and Fusarium wilt biocontrol by Trichoderma hamatum MHT1134. Biocontrol Sci. Technol. 30, 1228–1243. doi: 10.1080/09583157.2020.1803212
Navarro, S. L. B., and Rodrigues, C. E. C. (2016). Macadamia oil extraction methods and uses for the defatted meal byproduct. Trends Food Sci. Technol. 54, 148–154. doi: 10.1016/j.tifs.2016.04.001
Padder, B. A., and Sharma, P. N. (2011). In vitro and in vivo antagonism of biocontrol agents against Colletotrichum lindemuthianum causing bean anthracnose. Arch. Phytopathol. Plant Protect. 44, 961–969. doi: 10.1080/03235400903460619
Polaszek, A., Noyes, J. S., Russell, S., and Ramadan, M. M. (2020). Metaphycus macadamiae (Hymenoptera: Encyrtidae) – a biological control agent of macadamia felted coccid Acanthococcus ironsidei (Hemiptera: Eriococcidae) in Hawaii. PLoS One 15:e0230944. doi: 10.1371/journal.pone.0230944
Prasannath, K., Shivas, R. G., Galea, V. J., and Akinsanmi, O. A. (2021a). Neopestalotiopsis species associated with flower diseases of Macadamia integrifolia in Australia. J. Fungi 7:771. doi: 10.3390/jof7090771
Prasannath, K., Shivas, R. G., Galea, V. J., and Akinsanmi, O. A. (2021b). Novel Botrytis and Cladosporium species associated with flower diseases of macadamia in Australia. J. Fungi 7, 898. doi: 10.3390/jof7110898
Promwee, A., Yenjit, P., Issarakraisila, M., Intana, W., and Chamswarng, C. (2017). Efficacy of indigenous Trichoderma harzianum in controlling Phytophthora leaf fall (Phytophthora palmivora) in Thai rubber trees. J. Plant Dis. Prot. 124, 41–50. doi: 10.1007/s41348-016-0051-y
Rabeendran, N., Jones, E. E., Moot, D. J., and Stewart, A. (2006). Biocontrol of Sclerotinia lettuce drop by Coniothyrium minitans and Trichoderma hamatum. Biol. Control 39, 352–362. doi: 10.1016/j.biocontrol.2006.06.004
Reino, J. L., Guerrero, R. F., Hernández-Galán, R., and Collado, I. G. (2008). Secondary metabolites from species of the biocontrol agent Trichoderma. Phytochem. Rev. 7, 89–123. doi: 10.1007/s11101-006-9032-2
Salvatore, M. M., Andolfi, A., and Nicoletti, R. (2020). The thin line between pathogenicity and endophytism: The case of Lasiodiplodia theobromae. Agriculture 10:488. doi: 10.3390/agriculture10100488
Shanmugam, V., Sharma, V., and Ananthapadmanaban. (2008). Genetic relatedness of Trichoderma isolates antagonistic against Fusarium oxysporum f.sp. Dianthi inflicting carnation wilt. Folia Microbiol. 53, 130–138. doi: 10.1007/s12223-008-0019-9
Shaw, S., Le Cocq, K., Paszkiewicz, K., Moore, K., Winsbury, R., de Torres Zabala, M., et al. (2016). Transcriptional reprogramming underpins enhanced plant growth promotion by the biocontrol fungus Trichoderma hamatum GD12 during antagonistic interactions with Sclerotinia sclerotiorum in soil. Mol. Plant Pathol. 17, 1425–1441. doi: 10.1111/mpp.12429
Shuai, X., Dai, T., Chen, M., Liang, R., Du, L., Chen, J., et al. (2022). Comparative study on the extraction of macadamia (Macadamia integrifolia) oil using different processing methods. LWT Food Sci. Technol. 154:112614. doi: 10.1016/j.lwt.2021.112614
Siebatcheu, E. C., Wetadieu, D., Youassi, Y. O., Boat, M. A. B., Bedane, K. G., Tchameni, N. S., et al. (2022). Secondary metabolites from an endophytic fungus Trichoderma erinaceum with antimicrobial activity towards Pythium ultimum. Nat. Prod. Res. doi: 10.1080/14786419.2022.2075360 [Epub ahead of print].
Sosso, J., Zakeel, M. C. M., and Akinsanmi, O. A. (2021). Culturable fungal endophytes in Australian macadamia nursery plants. Australas. Plant Pathol. 50, 739–746. doi: 10.1007/s13313-021-00824-x
Stracquadanio, C., Quiles, J. M., Meca, G., and Cacciola, S. O. (2020). Antifungal activity of bioactive metabolites produced by Trichoderma asperellum and Trichoderma atroviride in liquid medium. J. Fungi 6:263. doi: 10.3390/jof6040263
Studholme, D. J., Harris, B., Le Cocq, K., Winsbury, R., Perera, V., Ryder, L., et al. (2013). Investigating the beneficial traits of Trichoderma hamatum GD12 for sustainable agriculture-insights from genomics. Front. Plant Sci. 4:258. doi: 10.3389/fpls.2013.0025
Trueman, S. J. (2013). The reproductive biology of macadamia. Sci. Hortic. 150, 354–359. doi: 10.1016/j.scienta.2012.11.032
Tyśkiewicz, R., Nowak, A., Ozimek, E., and Jaroszuk-Ściseł, J. (2022). Trichoderma: The current status of its application in agriculture for the biocontrol of fungal phytopathogens and stimulation of plant growth. Int. J. Mol. Sci. 23:2329. doi: 10.3390/ijms23042329
Wall, M. M. (2010). Functional lipid characteristics, oxidative stability, and antioxidant activity of macadamia nut (Macadamia integrifolia) cultivars. Food Chem. 121, 1103–1108. doi: 10.1016/j.foodchem.2010.01.057
Wonglom, P., Daengsuwan, W., Ito, S. I., and Sunpapao, A. (2019). Biological control of Sclerotium fruit rot of snake fruit and stem rot of lettuce by Trichoderma sp. T76-12/2 and the mechanisms involved. Physiol. Mol. Plant Pathol. 107, 1–7. doi: 10.1016/j.pmpp.2019.04.007
Wrona, C. J., Mohankumar, V., Schoeman, M. H., Pan, Y. P., Shivas, R. G., Jeff-Ego, O. S., et al. (2020). Phomopsis husk rot of macadamia in Australia and South Africa caused by novel Diaporthe species. Plant Pathol. 69, 911–921. doi: 10.1111/ppa.13170
Keywords: biological control, Lasiodiplodia theobromae, macadamia, Trichoderma hamatum, fungal disease
Citation: Li X, Leng J, Yu L, Bai H, Li X, Wisniewski M, Liu J and Sui Y (2022) Efficacy of the biocontrol agent Trichoderma hamatum against Lasiodiplodia theobromae on macadamia. Front. Microbiol. 13:994422. doi: 10.3389/fmicb.2022.994422
Received: 14 July 2022; Accepted: 15 August 2022;
Published: 31 August 2022.
Edited by:
Rosario Nicoletti, Council for Agricultural Research and Economics (CREA), ItalyReviewed by:
Julian Rafael Dib, CONICET Planta Piloto de Procesos Industriales Microbiológicos (PROIMI), ArgentinaNengguo Tao, Xiangtan University, China
Copyright © 2022 Li, Leng, Yu, Bai, Li, Wisniewski, Liu and Sui. This is an open-access article distributed under the terms of the Creative Commons Attribution License (CC BY). The use, distribution or reproduction in other forums is permitted, provided the original author(s) and the copyright owner(s) are credited and that the original publication in this journal is cited, in accordance with accepted academic practice. No use, distribution or reproduction is permitted which does not comply with these terms.
*Correspondence: Longfeng Yu, 364130476@qq.com; Jia Liu, jialiu1983@163.com; Yuan Sui, suiyuan-mine@163.com
†These authors have contributed equally to this work