- 1National Demonstration Center for Experimental Plant Science Education, College of Agriculture, Guangxi University, Nanning, Guangxi, China
- 2State Key Laboratory for Conservation and Utilization of Subtropical Agro-Bioresource and College of Life Science and Technology, Nanning, China
- 3State Key Laboratory for Conservation and Utilization of Subtropical Agro-Bioresources, South China Agricultural University, Guangzhou, China
- 4Key Laboratory of Natural Pesticide and Chemical Biology, Ministry of Education, South China Agricultural University, Guangzhou, China
Biological control is an effective measure in the green control of rice diseases. To search for biocontrol agents with broad-spectrum and high efficiency against rice diseases, in this study, a strain of antagonistic bacterium BR-01 with strong inhibitory effect against various rice diseases was isolated from Bolbostemmatis Rhizoma by plate confrontation method. The strain was identified as Bacillus velezensis by morphological observation, physiological and biochemical identification, and molecular characterization by 16S rDNA and gyrB gene sequencing analysis. The confrontation test (dual culture) and Oxford cup assays demonstrated that B. velezensis BR-01 had strong antagonistic effects on Magnaporthe oryzae, Ustilaginoidea virens, Fusarium fujikuroi, Xanthomonas oryzae pv. Oryzicola, and Xanthomonas oryzae pv. oryzae, the major rice pathogens. The genes encoding antimicrobial peptides (ituA, ituD, bmyB, bmyC, srfAA, fenB, fenD, bacA, and bacD) were found in B. velezensis BR-01 by PCR amplification with specific primers. B. velezensis BR-01 could produce protease, cellulase, β-1,3-glucanase, chitinase, indoleacetic acid, siderophore, and 1-aminocyclopropane-1-carboxylate (ACC) deaminase, and might produce three lipopeptide antibiotics, surfactin, iturin, and fengycin based on Liquid chromatography–mass spectrometry (LC-MS) results. Furthermore, the plant assays showed that B. velezensis BR-01 had significant control effects on rice bacterial blight and bacterial leaf streak by pot experiments in greenhouse. In conclusion, B. velezensis BR-01 is a broad-spectrum antagonistic bacterium and has the potential as the ideal biocontrol agent in controlling multiple rice diseases with high efficiency.
Introduction
Rice (Oryza sativa L.) is one of the most important staple crops, which is widely cultivated around the world and mainly in Asia. It is also one of the earliest domesticated crop species. Due to the continuously human intervening, rice plants might be more fragile to stresses than many other crops, and more susceptible at different seasons and their growth stages (Ou et al., 2014; Zhao et al., 2014; Quibod et al., 2020). Rice diseases have caused great losses in rice production, both in yield and quality. Chemical control has played a major role in rice disease control with the characteristics of convenience, rapidity, and the remarkable effects. However, the non-negligible side effects of many chemical agents, such as chemical residues, environmental pollution, and pest resistances, are clearly inconsistent with the requirements in sustainable agriculture (Jepson et al., 2020). The selection and application of disease-resistant varieties is the preferential choice for green agriculture, but the long breeding cycles and the uncertain resistant persistency of the resistant cultivars are still major challenges in rice production (Deng et al., 2020). Biological control, a sustainable and practical approach for plant disease management, is a promising alternative strategy in rice disease prevention and control (Ab Rahman et al., 2018). So far, a variety of microorganisms, mainly including Bacillus, Pantoea, Streptomyces, Trichoderma, Clonostachys, Pseudomonas, Burkholderia, Lysobacter, and yeasts, have been shown to have the antagonistic ability against rice pathogens and some of which have been registered as the biopesticides used in controlling rice diseases in fields (Qian et al., 2009; Limtong et al., 2020; Rani et al., 2021; Doni et al., 2022; Tan, 2022; Zhang H. J. et al., 2022).
Among the most commonly used antagonistic microorganisms, Bacillus is a prominent genus used as the biocontrol agents in rice production, with strong stress resistance and good environmental adaptability (Doni et al., 2022). Bacillus is a large and heterogeneous collection of aerobic or facultatively anaerobic, rod-shaped, endospore-forming bacteria that are widely distributed throughout the environment (Rabbee and Baek, 2020). Currently, B. subtilis, B. cereus, B. pumilus, B. amyloliquefaciens, B. firmus, B. megaterium, B. safensis, and B. velezensis, etc., have been reported to have the antagonist effects on plant pathogens, including bacteria, fungi, and nematodes (Lopes et al., 2018; Chakraborty et al., 2021). Numerous studies have shown that different taxa of Bacillus, even different strains of the same species in Bacillus, might have the distinct biological functions on plant pathogens. Genomic analysis has revealed that Bacillus spp. possess species- or strain-specific clusters of genes related to the biosynthesis of secondary metabolites, which play different roles in both pathogen suppression and plant growth promotion (Rabbee and Baek, 2020; Doni et al., 2022). The biological control mechanisms of Bacillus are mainly described as the production of direct antifungal substances such as volatile organic compounds (VOCs), non-volatile metabolites, and extracellular lytic enzymes. B. subtilis is the well-studied antagonist organism group in controlling rice blast, sheath blight, and bacterial leaf blight, which can produce mainly bacteriocin and lipopeptide compounds, organic acids, and chitinases to suppress pathogen either directly or through enhancing the plant defense mechanisms (Amruta et al., 2016; Kaspar et al., 2019; Doni et al., 2022). In addition to B. subtilis, other Bacillus spp. were also demonstrated to exert effectively antagonistic on rice pathogens (Chakraborty et al., 2021). Yu et al. (2002) found that production of iturin A by B. amyloliquefaciens suppressing Rhizoctonia solani. Saechow et al. (2018) isolated a strain of B. amyloliquefaciens BAS23 from rice field soil. Based on dual culture method results, BAS23 showed strong inhibitory activity against a broad range of dirty panicle fungal pathogens of rice. It also has a variety of characters to promote plant growth and improve the growth of rice seedlings in the test tube. Jain et al. (2020) demonstrated that synergistic consortium of beneficial microbes B. amyloliquefaciens and Aspergillus spinulosporus in rice rhizosphere promoted host defense Xanthomonas oryzae pv. oryzae. B. cereus, B. firmus, B. megaterium, B. pumilus, and B. safensis, etc., have also positively evaluated having multiple functions in biocontrol of rice diseases (Kanjanamaneesathian et al., 2009; Rong et al., 2020; Sha et al., 2020; Chakraborty et al., 2021).
Bacillus velezensis is a newly reclassified Bacillus species, which consists of the original B. velezensis strains (Ruiz-García et al., 2005) and B. amyloliquefaciens subsp. plantarum, B. methylotrophicus, and B. oryzicola, also known as later heterotypic synonyms of B. velezensis, based on phylogenomics (Dunlap et al., 2016; Fan et al., 2017; Adeniji et al., 2019). Studies showed that many strains of this species have the ability to suppress the growth of rice pathogens and promote plant growth, largely relying on the gene clusters related to the biosynthesis of secondary metabolites, e.g., the cyclic lipopeptides (i.e., surfactin, bacillomycin-D, fengycin, and bacillibactin) and polyketides (i.e., macrolactin, bacillaene, and difficidin) (Rabbee and Baek, 2020). At present, hundreds of B. velezensis strains have been isolated from different environments, some of which were demonstrated to have antagonistic ability to rice pathogens in laboratories or/and in the fields. B. velezensis (synonyms B. methylotrophicus) strain BC79, isolated from primeval forest soil in Qinling Mountains, China, was able to suppress mycelial growth and conidial germination of M. oryzae in dual cultures on solid media, mainly by the phenaminomethylacetic acid (Shan et al., 2013). B. velezensis (formerly B. amyloliquefaciens) FZB42, the model strain for Gram-positive plant-growth-promoting and biocontrol rhizobacteria, was shown to possess biocontrol activity against X. oryzae pv. oryzae and X. oryzae pv. oryzicola by producing the antibiotic compounds difficidin and bacilysin (Wu et al., 2015; Fan et al., 2018). B. velezensis (synonyms B. oryzicola) strain YC7007, an endophytic bacterium isolated from the roots of rice, could inhibit the growth of important fungal and bacterial pathogens of rice, such as Fusarium fujikuroi and Burkholderia glumae via antibiotic production and systemic resistance inducing activities (Chung et al., 2015; Hossain et al., 2016). B. velezensis G341, isolated from Korean ginseng root with rot symptoms, could inhibit mycelial growth of M. oryzae and R. solani. It was found that the diffusible and volatile antifungal compounds emitted from strain G341 were found to have strong effects on R. solani than M. oryzae (Lim et al., 2017). B. velezensis NKG-2 could inhibit the growth of U. virens on potato glucose broth (PDB), which had the ability to produce chitinase, cellulase, β-1,3-glucanase, amylase, indole-3-acetic acid (IAA), and siderophore (Myo et al., 2019). B. velezensis E69, isolated from rice leaf, could strongly inhibit the conidial germination and appressorial formation of M. oryzae (Sha et al., 2019). B. velezensis XT1, a halotolerant bacterium isolated from a saline habitat in Spain, had antifungal activity in vitro by inhibiting the mycelial growth of M. oryzae and promoting the growth of many plants (Torres et al., 2019). A marine-derived strain B. velezensis 11-5 could produce cyclic lipopeptide iturin A which could inhinbit M. oryzae growth (Ma et al., 2020). C15surfactin A, produced by B. velezensis HN-2, displayed antibacterial activity against Xoo and effectively inhibited its infection of rice (Jin et al., 2020). B. velezensis CMRP 4490 had antagonistic activity against R. solani (Teixeira et al., 2021). B. velezensis JK23, a radiation-resistant strain isolated from radioactive contaminated soil, had strong inhibition against F. moniliforme (Zhang et al., 2021). B. velezensis ZW10, isolated from Sichuan basin neutral purplish soil, was reported to have strong antagonistic on M. oryzae growth on plate and the capacity to stimulate rice defenses (Chen et al., 2021). B. velezensis J17-4, isolated from healthy and sterilized stems of rice stem, could significantly inhibit the growth of Dickeya zeae and could significantly reduce the inhibitory effect of D. zeae strain EC1 against rice seed germination under laboratory inoculation conditions (Shi et al., 2022). These researches indicated that B. velezensis has strong antagonistic effects on a variety of rice diseases, from numerous individual cases and a few of B. velezensis strains had already been registered as biopesticides playing important roles in rice disease prevention and control (Doni et al., 2022; Zhang Y. H. et al., 2022). However, the studies also showed that B. velezensis strains seemingly differ in their abilities to control certain rice diseases, which might be insufficient to meet the requirements of high efficiency and low-carbon in green rice production. The broad-spectrum B. velezensis strains with versatility in antagonistic, host immunity inducing, and plant-growth-promoting, are urgently needed to protect paddies from the attacks of an armada of diseases simultaneously (Shasmita et al., 2022).
In recent years, endophytic microorganisms from medicinal plants and their active components have shown good development value in agriculture, fermentation industry and pharmaceutical industry (Zhao et al., 2011; Gond et al., 2012; Kuo et al., 2021; Zhang G. Y. et al., 2022). However, these studies mainly focus on endophytic fungi. In this study, a broad-spectrum biocontrol bacterium, B. velezensis BR-01, was identified from Bolbostemmatis Rhizoma, a Chinese herbal medicine, and its antagonistic effects on important rice pathogens were assessed and its antimicrobial active substances were preliminarily determined and analyzed.
Materials and methods
Bacterial strains isolation from Chinese herbal medicine
The antagonistic microbial strains were deliberately isolated from the traditional Chinese medicines, i.e., Bolbostemmatis Rhizoma, Radix isatidis, and Flos Lonicerae. Bolbostemmatis Rhizoma (Tubeimu) is the dried tuber of Bolbostemma paniculatum (Maxim.) Franquet, belonging to Cucurbitaceae family (Liu et al., 2004). Radix isatidis (Banlangen) is the dried root of Isatis indigotica, a biennial herbal plant belonging to Cruciferae. Flos Lonicerae (Jinyinhua) is the flower bud and early flower of Lonicera japonica Thund., which is a semi-evergreen and perennial woody climbing shrub belonging to the Caprifoliaceae family. In this study, the samples Bolbostemmatis Rhizoma, Radix isatidis, and Flos Lonicerae were collected from Huyi District, Xian City, Shanxi Province, China (33°46′N, 108°22′E), Anguo City, Hebei Province, China (38°42′N, 115°33′E), and Jingxi County, Guangxi Province, China (23°13′N, 106°42′E), respectively.
The collected Chinese herbal materials were cut into small segments of 1 cm in size, sterilized on the surface, smashed and ground in a sterile mortar containing 10 mL sterile water, and then put into a triangular flask containing 90 ml sterile water. The bacterial suspension was prepared by shaking culture at 200 rpm for 30 min. 1 mL bacterial suspension was diluted with sterile water to concentrations of 10–2, 10–3, and 10–4, respectively. Dip 100 μL diluted bacterial solution evenly spread on agar plates of NA medium (Supplementary Table 1), repeat 3 times. After 48 h culture at 28°C, single colonies with different shape were selected for subculture.
Rice and pathogens
Rice (Oryza sativa L.) cultivar Nipponbare was used as a host for the respective pathogens including Xoc, Xoo, M. oryzae, R. solani, F. fujikuroi, and U. virens in this study (Table 1).
Xoc and Xoo strains were grown in NA medium at 28°C. U. virens was grown on potato sucrose agar medium (PSA) (Supplementary Table 1) at 28°C. M. oryzae, R. solani, F. fujikuroi, and U. virens were cultivated on potato dextrose agar medium (PDA) (Supplementary Table 1) at 28°C.
Initial screening of antagonistic bacteria
Given that there have been considerable reports about B. velezensis biological control of M. oryzae, the rice fungal pathogen, in this study, X. oryzae pv. oryzicola, the rice bacterial pathogen, were deliberately chosen as the initial control target indicator.
The isolated antagonist bacteria and Xoc were inoculated in NB medium (Supplementary Table 1), and cultured in a shaker at 28°C, respectively. After overnight culture at 200 rpm, the bacterial concentration was adjusted to OD600 = 1.0.
Firstly, a layer of 5 ml water agar was placed at the bottom of the petri dish to ensure that the bottom of the petri dish was smooth. After cooling, an Oxford cup was placed in the middle of the petri dish. The completely melted NA agar medium was cooled to about 45°C, and 1 mL indicator bacteria liquid was added to each 100 mL medium. After shaking evenly, it was poured into the petri dish, about 15 ml per dish. After cooling, the Oxford cup was taken out, and 0.1 mL antagonistic bacteria liquid was added to each hole. The same amount of NB medium was added as the blank control, with three repeated treatments. Inverted culture at 28°C for 2 days, the diameter of inhibition zone was observed and measured (Li et al., 2019).
Evaluation of antagonistic spectrum of the antagonistic bacterium
For determination of the antagonistic spectrum of the antagonistic bacterium, the pathogens of the major rice diseases, i.e., Xoo, M. oryzae, R. solani, F. fujikuroi, and U. virens, were used as targeted indicators (Table 1), after the initial screening.
Almost same procedures in the antagonistic test on Xoc were used on Xoo. Determination of antagonistic spectrum against pathogenic fungi were carried out by using plate confrontation method (Pastrana et al., 2016). The indicator fungi block was inoculated in the center of PDA plate with a puncher of 5 mm in diameter, and 1 μL of antagonistic bacterial suspension (OD600 = 1.0) was dotted around the indicator fungi. The dotted point was 2 cm away from the fungi block. The plates inoculated with the same amount of NB medium were taken as the control group, and the treatment was repeated for three times. Inverted culture in a constant temperature incubator at 28°C, when the diameter of the pathogen in the control group grew to about 3/4 of the diameter of the petri dish, the diameter of the pathogen was measured by Cross-measurement method (Yang et al., 2017), and the antifungal rate of the strain was calculated according to the following formula.
Where I = antifungal rate; d0 = diameter of the control group; d1 = diameter of the treatment group; d = diameter of the punch.
Physiological, biochemical, and molecular characterization
The antagonistic bacteria were primarily identified by observing the morphology, size, color, wet or dry, smooth or rough of individual colonies according to the methods of Berger’s Manual of Bacterial Identification (Holt et al., 1994) and Common Bacterial Systems Identification Manual (Dong and Cai, 2001). A number of physiological and biochemical characterization tests such as Gram staining, contact enzyme, nitrate reduction, liquefaction of gelatin, hydrogen sulfide production, carbon source utilization, V-P (Voges–Proskauer) test and MR (methyl red) test were performed on antagonistic bacteria. The treatment was repeated three times.
Genomic DNA was extracted and purified using the whole genome DNA sequencing kit (Tiangen Biochemical Technology Co., Ltd., Beijing, China). 16S rDNA and gyrB genes of the antagonistic bacterium were amplified by primer pairs 27F (5′-AGAGTTTGATCCTGGCTCAG-3′), 1492R (5′-GGTTACCTTGTTACGACTT-3′) (Mahmood et al., 2006) and UP1 (5′-GAAGTCATCATGACCGTT CTGCAYGCNGGNGGNAARTTYGA-3′), UP2r (5′-AGCAG GATACGGATGTGCGAGCCRTCNACRTCNGCRTCNGTCAT-3′) (Yamamoto and Harayama, 1995), respectively.
The PCR amplification system was 50 μL of 5 × PCR buffer 10 μL, primer F 1.2 μL, primer R 1.2 μL, 2.5 mM dNTPs 5 μL, DNA template 1 μL, DNA polymerase 0.6 μL, and add ddH2O to 50 μL. PCR cycle conditions: denaturation at 95°C for 30 s, annealing at 60°C for 30 s, extension at 72°C for 30 s, a total of 30 cycles; then the products were kept at 72°C for 10 min and detected by 1% agarose gel electrophoresis. The amplified products were sequenced by Nanning Qingke Biotechnology Co., Ltd. The sequencing results were compared with the known sequences in NCBI database by nucleotide BLAST, and the phylogenetic tree was constructed by N-J method in MEGA 11.0.
Detection of antagonist related lytic enzymes and secondary metabolites
The activities of protease, cellulase, glucanase, and chitinase of BR-01 were detected on agar plates containing skim milk, sodium carboxymethyl cellulose, β-glucan, and colloidal chitin, respectively (Ghose, 1987; Roberts and Selitrennikoff, 1988; Shakeel et al., 2015; Li et al., 2018). The antagonistic bacterial suspension (1 μL, OD600 = 1) was inoculated on four testing media in plates (Supplementary Table 1) and cultured at 28°C for 2–4 days. The ability of antagonistic bacterium to produce various hydrolytic enzymes was judged by observing the growth of bacterial colonies and the size of hydrolytic circles around colonies. The hydrolysis circle of cellulase was first stained with Congo red and then observed.
Chrome-azurol S (CAS) medium (Supplementary Table 1) was used to detect the ability of antagonistic bacterium to produce siderophore. The antagonistic bacterial suspension (1 μL, OD600 = 1) was inoculated on CAS medium plates and cultured at 28°C for 2–4 days. The yellow-orange halo around the colonies was considered to be caused by the presence of siderophore (Schwyn and Neilands, 1987).
The ability of antagonistic bacterium to produce IAA was determined by observing whether the fermentation supernatant of YMB medium (Supplementary Table 1) mixed Salkowski reagent will appear obvious red (Gordon and Weber, 1951).
To check whether antagonistic bacterium can be continuously passaged three times in ADF medium (Supplementary Table 1) to determine whether antagonistic bacterium can produce ACC deaminase (Penrose and Glick, 2003).
Detection of synthesis-related genes for antimicrobial peptides
The antimicrobial lipopeptide synthesis-related genes were detected by using specific primers (Mora et al., 2011; Plaza et al., 2015; Yang et al., 2015; Sajitha and Dev, 2016) listed in Supplementary Table 2. The PCRs were carried out using approximately 0.5 μg of total bacterial DNA (1 μL), 10 μL of 5× PCR buffer, 5 μL of dNTPs (2 mM), 1.5 μL of each primer (10 μM), 0.6 μL of DNA polymerase, and adequate ddH2O so that the final volume of the mixture was 50 μL. The PCR mixtures were denatured at 95°C for 5 min, which was followed by 30 cycles of 94°C for 30 s, 51°C∼60°C for 30 s, and 72°C for 1 min and then a final extension at 72°C for 5 min. PCR products were checked for sizes by electrophoresis on a 1.5% agarose gel.
Preparation of culture extract and its antimicrobial activity test
In this study, the antimicrobial substance from cell-free supernatants of B. velezensis BR-01 was purified using phosphate-buffered saline (PBS) (Supplementary Table 1) and methanol extraction after ammonium sulfate precipitation at 60% saturation (Xu et al., 2018). The methanol extract was dried under reduced pressure in a rotating evaporator at 43°C to obtain the dry powder of antibacterial peptide crude extract (Jasim et al., 2014).
The inhibitory sample was prepared by dissolving 3 mg crude extract in 1 ml methanol and filtered through 0.22 μm filter (Yang Y. Y. et al., 2021). Xoc and Xoo were used as indicator bacteria and the antibacterial activity was detected by Oxford cup method. M. oryzae and U. virens were used as indicator fungi, and the inhibitory samples were mixed with PDA medium at a ratio of 1:40 by radial growth assay (Pastrana et al., 2016). With the addition of the same amount of methanol as the control, each treatment was repeated three times. For antagonistic to bacterial pathogens, the treatment and control groups were cultured at 28°C for 2 days. For antagonistic to fungal pathogens, the treatment and control groups were cultured at 28°C, when the diameter of the fungal colonies in the control group grew to about 3/4 of the diameter of the petri dish, the diameter of the pathogen was measured by Cross-measurement method (refer to 1.4) (Yang et al., 2017).
Compound identification from culture extract using liquid chromatograph-mass spectrometer
Liquid Chromatograph-Mass Spectrometer (LC-MS) analysis was performed to identify purified bioactive substances in crude extract from B. velezensis BR-01 (Xu et al., 2018). Using Thermo Xcalibur 4.0 software, the relative molecular mass of the target substance was measured according to the mass-to-charge ratio (m/z) of the sample, and the types of lipopeptides produced by the strain were preliminarily determined by comparison (Xia et al., 2019).
Chromatographic conditions: ACQUITY UPLCBEHC18 column (50 mm × 2.1 mm, 1.7 μm); in the positive ion (ESI+) mode, the mobile phase A was 0.1% formic acid water, and B was methanol. Sample gradient elution program: 0∼2 min, 95% A; 2∼13 min, 95% A∼5% A; 13∼16 min, 5% A; 16∼16.1 min, 5% A∼95% A; 16.1∼18 min, 95% A. The flow rate was 0.3 mL/min, and the injection volume was 2 μL.
Mass spectrum conditions: The ion source is spray electrothermal spray (HESI) with a temperature of 300°C. The spray voltage is 3.0 kV under positive ion mode. The temperature of transmission capillary is 320°C, the sheath gas is 30 psi, and the auxiliary gas flow velocity is 10 psi; the scanning mode is full MS/dd-MS2, the quality range is 200∼2,000 m/z, and the resolutions of primary scanning and secondary scanning are 70,000 and 17,500, respectively. Collision gas: high purity nitrogen.
Biocontrol efficacy assays under greenhouse conditions
Biocontrol efficacy assays were carried out in a greenhouse at constant temperature of 28°C and relative humidity of 90%. 45-day-old rice plants in pots were used in infection challenges. Xoc, Xoo, and B. velezensis BR-01 strains were inoculated into NB medium at 28°C and cultured at 200 rpm for 18 h until logarithmic growth period, collected and then diluted with NB medium to OD600 = 0.5. The infiltration method was used for inoculation of Xoc, by which the Xoc culture suspension was infiltrated into rice leaves using needleless syringe (Yang and Bogdanove, 2013). The leaf-cutting method was used for inoculation of Xoo, by which rice leaves were clipped using scissors dipped in Xoo culture suspension (Ke et al., 2017).
Four different treatment groups were set up (Supplementary Table 4; Li et al., 2019; Huang et al., 2021). CK group: inoculated with rice pathogenic bacteria, 12 h after inoculation spray NB medium as control treatment. NB medium group: replace the inoculated bacterial suspension with NB medium and spray NB medium 12 h after inoculation. Treatment group: inoculated with rice pathogenic bacteria, 12 h after inoculation spray B. velezensis BR-01 bacterial suspension. Preventive group: inoculated with rice pathogenic bacteria, 12 h before inoculation spray B. velezensis BR-01 bacterial suspension. Each treatment group of each rice pathogenic bacterium was inoculated with 10 rice plants and 5 leaves per plant. About 10 days after inoculation, the expansion of the lesions was observed and recorded, and the control effect was calculated according to the formula (Li et al., 2019).
Where C = control effect; L1 = length of spots in control group; L2 = length of spots in treatment group.
Data analysis
Each experiment was independently repeated at least three times. The software SPSS v20.0 (SPSS, Chicago, IL, USA) was used for statistical analysis. Statistical significance between multiple groups was determined using analysis of variance (ANOVA). All the results were expressed as mean ± SD (n = 3). P < 0.05 were considered statistically significant.
Results and analysis
Isolation of antagonistic bacteria and evaluation of their inhibition effects
To screen the microbial resources for controlling various diseases of rice, three kinds of Chinese herbal medicines were collected from three provinces in China. Eight bacterial strains were isolated, including three strains from Bolbostemmatis Rhizoma, three strains from Radix isatidis, and two strains from Flos Lonicerae, which had antagonistic effects on Xoc in the Oxford cup assays (Figure 1). Among them, the strongest antagonistic strain was isolated from Bolbostemmatis Rhizoma, with an average inhibition zone diameter of 46.33 mm, designated BR-01. The plate co-culture test showed that BR-01 had antagonistic activity against M. oryzae, U. virens, and F. fujikuroi, and the relative inhibition rates of mycelial growth were 83.71, 96.65, and 57.92%, respectively, but had no significant antagonistic effect against R. solani. The results of the Oxford cup method showed that BR-01 also had a strong antagonistic effect against Xoo, with an average inhibition zone diameter of 36.70 mm (Figure 2).
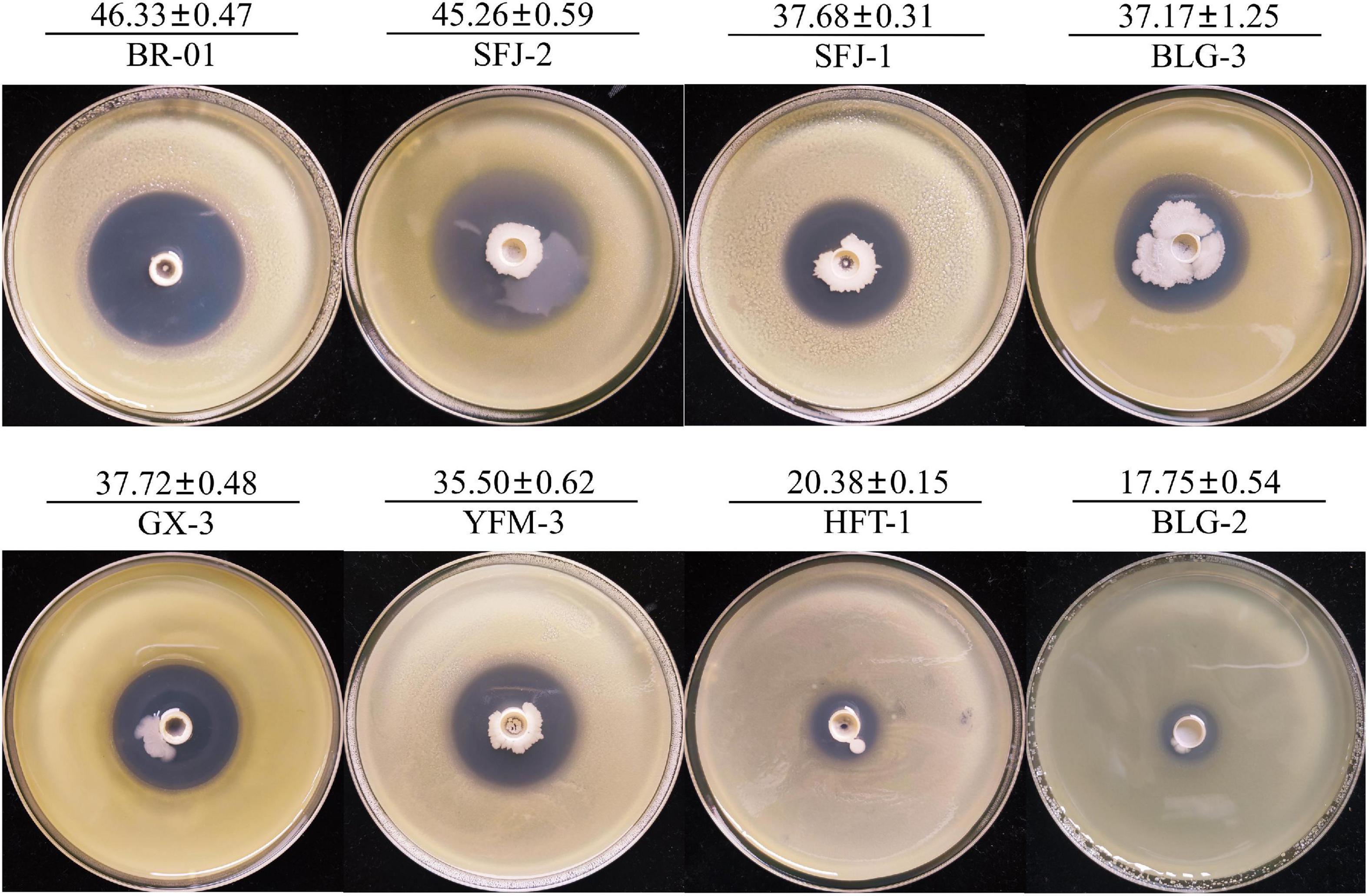
Figure 1. Antagonistic effect of antagonistic bacteria on Xanthomonas oryzae pv. oryzicola. Values given are the means ± standard deviations of triplicate measurements. The unit of measurement for inhibition zones is millimeter.
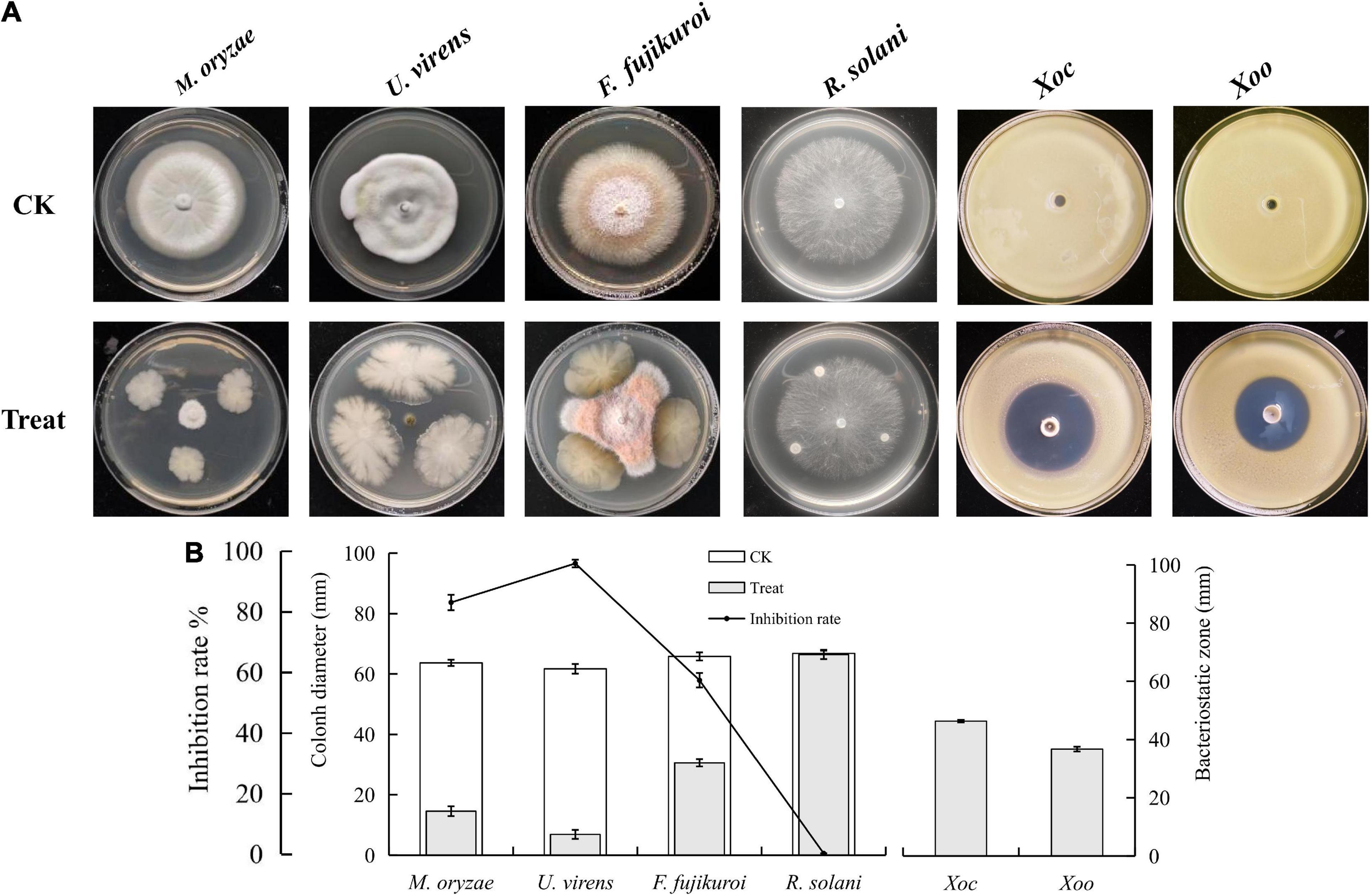
Figure 2. (A) Antagonistic effects of BR-01 against a variety of rice pathogens and (B) data statistics. Inhibition rate of BR-01 on pathogenic fungi was measured when pathogenic fungi in control group grew to about 3/4 of culture dish. Values given are the means ± standard deviations of triplicate measurements.
Characterization of antagonistic bacterium BR-01
The morphological observation showed that the single colonies of BR-01 were white, flat, opaque, rough, and wrinkled with irregular edges on the NA medium, and Gram staining was positive (Figure 3). Physiological and biochemical characterization showed that BR-01 could utilize sucrose, D-glucose, D-maltose, and D-mannitol. Contact enzyme reaction, gelatin hydrolysis, and starch hydrolysis were positive reactions. Hydrogen sulfide production, citrate utilization, and phenylalanine dehydrogenase test were negative reactions (Table 2). According to the physiological and biochemical characteristics of BR-01, combined with the morphological characteristics of colonies, and referring to Bergey’s universal methods (Holt et al., 1994), BR-01 was preliminarily identified as Bacillus sp.
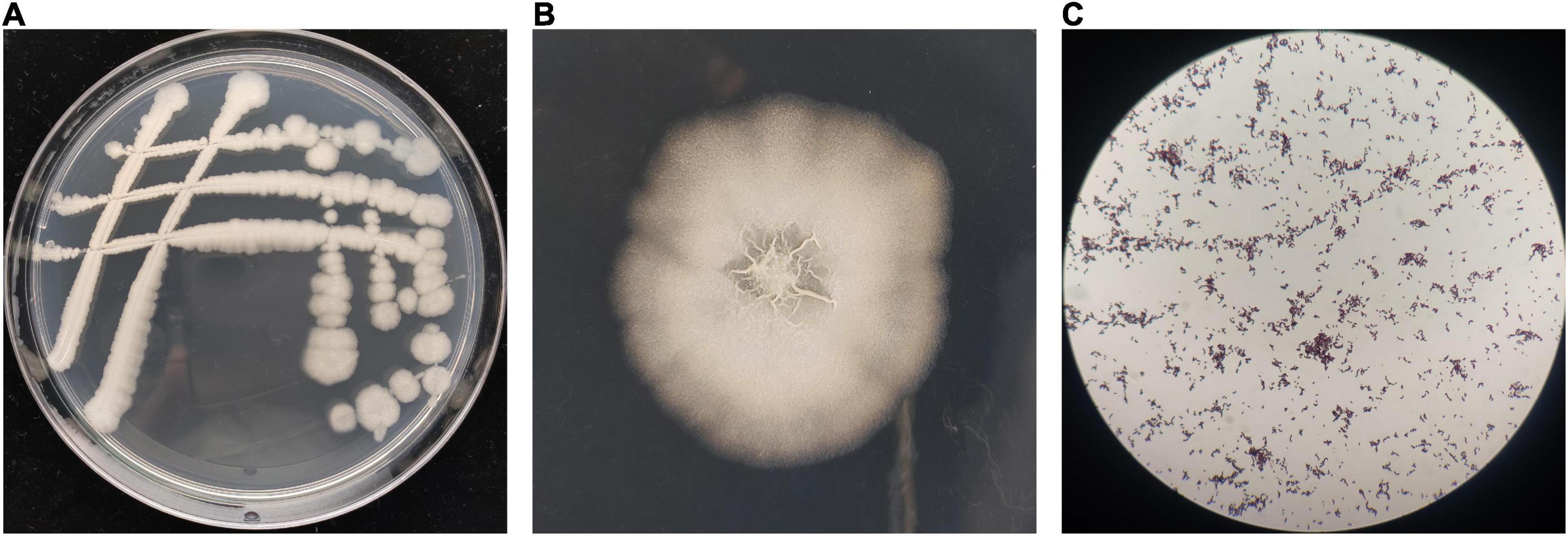
Figure 3. Colony morphological characteristics of BR-01. (A) Appearance of antagonistic bacterium BR-01 on NA medium. (B) Antagonistic bacterium BR-01 single colony morphology. (C) Gram staining of antagonistic bacterium BR-01.
To clarify the taxonomic status of BR-01, its 16S rDNA sequence was analyzed by BLAST in NCBI. It was found that it had the highest homology with B. velezensis CBMB205 (NR116240.1) and the identity was 99.58%. BLAST analysis of the gyrB gene sequence of BR-01 also showed that it had 100% identity with the gyrB gene of B. velezensis JS25R (NZ CP009679.1). Using MEGA 11.0 software, phylogenetic trees based on 16S rDNA and gyrB gene sequences were constructed, respectively. The results showed that the 16S rDNA and gyrB sequences of BR-01 were clustered into one branch with B. velezensis (Figure 4). Thus, the antagonistic strain BR-01 was identified as B. velezensis by combining the morphological characteristics, physiological and biochemical characteristics, and sequence analysis results.
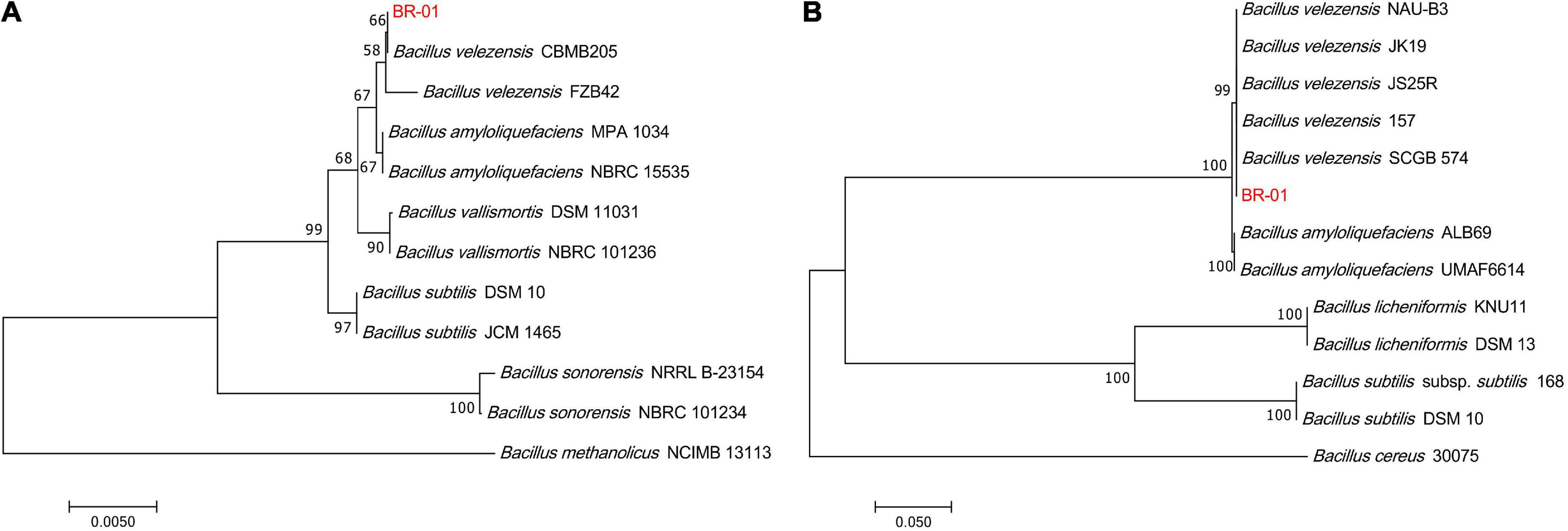
Figure 4. Neighbor-joining phylogenetic tree of the antagonistic bacterium BR-01 were constructed by MEGA 11.0. (A) Based on 16S rDNA gene. (B) Based on gyrB gene. The numbers at the branches indicate the confidence level calculated by bootstrap analysis (1000). The scale bar shows the evolutionary distance between species.
Detection of antagonist related lytic enzymes and secondary metabolites from BR-01
To study the antagonistic mechanism of B. velezensis BR-01, We examined the ability of B. velezensis BR-01 to produce antagonist related lytic enzymes and secondary metabolites. Translucent hydrolysis circles were formed around all colonies of B. velezensis BR-01 on the medium for protease, cellulase and β-1,3-glucanase assays, indicating that B. velezensis BR-01 could produce protease, cellulase and β-1,3-glucanase. In addition, B. velezensis BR-01 grew well on the medium with chitin as the sole carbon source, so it was considered to have the ability to produce chitinase, although there was no obvious hydrolysis circle around the colony of B. velezensis BR-01. The study on the production of fungal cell wall degrading enzymes by B. velezensis BR-01 can explain the swelling of fungal cells and the loss of cell wall integrity to a certain extent, leading to cell lysis and death (Muleta et al., 2007), providing a basis for the further development and application of B. velezensis BR-01 (Figure 5 and Table 3).
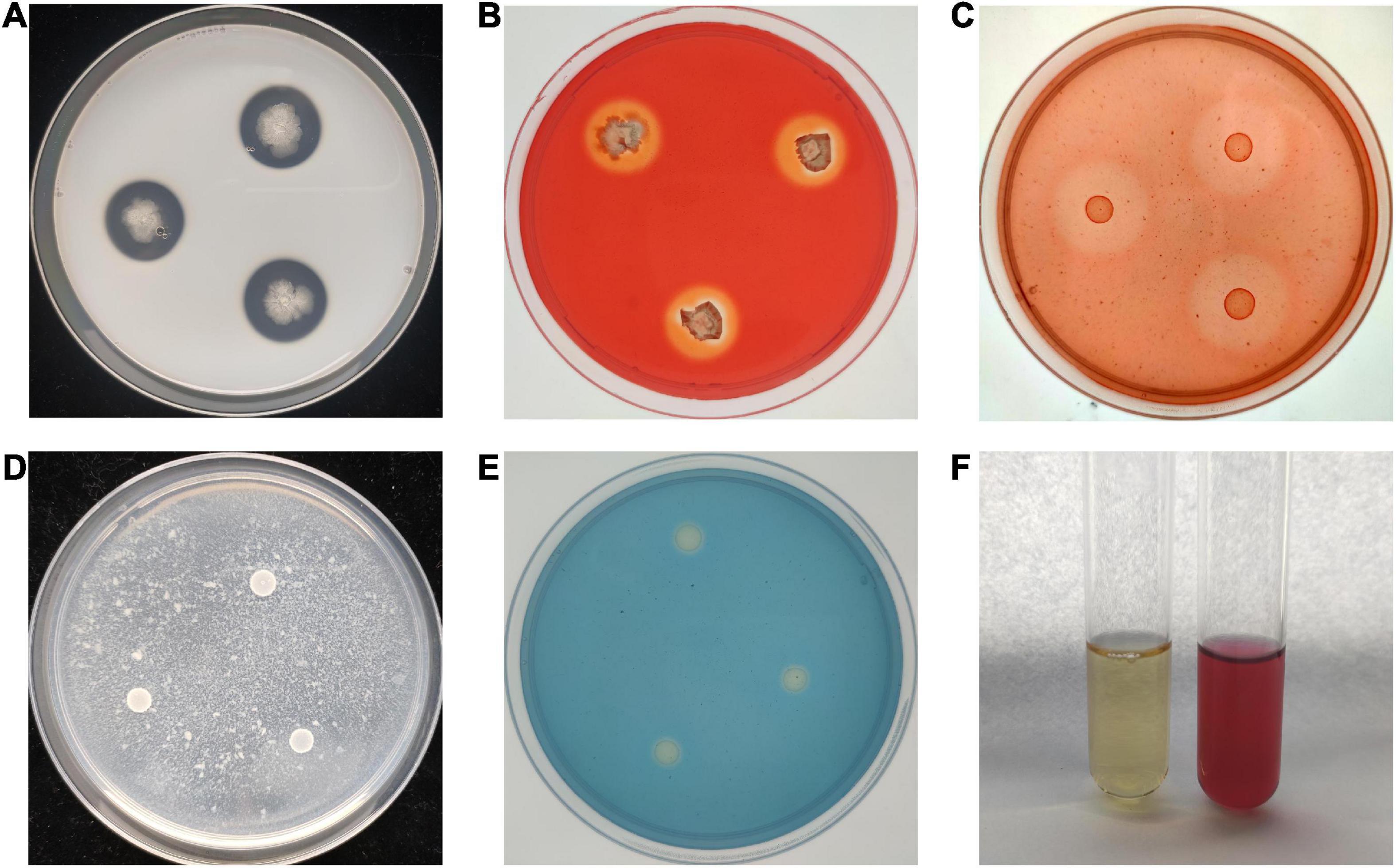
Figure 5. Detection of antagonistic enzymes and secondary metabolites in B. velezensis BR-01. (A) Protease detection; (B) cellulase detection; (C) β-1,3-glucanase detection; (D) chitinase detection; (E) siderophore detection; (F) indole-3-acetic acid (IAA) detection.
Compared with the control group, the color of the YMB liquid medium fermentation supernatant of B. velezensis BR-01 was significantly red after mixing with Salkowski reagent, indicating that B. velezensis BR-01 could synthesize IAA using tryptophan as the precursor. B. velezensis BR-01 could be continuously passaged three times on ADF medium with ACC as the sole nitrogen source, indicating that B. velezensis BR-01 could produce ACC deaminase. B. velezensis BR-01 could change CAS medium from blue to light orange, indicating that it could produce Siderophore. IAA is a plant auxin that can effectively promote plant growth and affect plant physiological metabolism. ACC deaminase can degrade ethylene precursor ACC, thereby reducing ethylene levels during plant growth and contributing to plant growth (Nascimento et al., 2014). Siderophore absorb ferric ion in the surrounding environment and provide more ferric ion to plants for their use, so that plant pathogenic microorganisms lack iron nutrition and cannot grow and reproduce (Wang et al., 2014). B. velezensis BR-01 could promote plant growth by releasing these substances and indirectly improve plant disease resistance.
Identification of the synthetic genes for antimicrobial peptides in BR-01
Bacillus velezensis can produce a variety of antimicrobial peptides. To identify the synthetic genes for antimicrobial peptides in BR-01, the target genes involving in the synthesis of iturin, bacillomycin, surfactin, fengycin, and bacilysin were subjected to be amplified with specific primers for certain genes using the genomic DNA of B. velezensis BR-01 as the template (Chen et al., 2009; Sumi et al., 2015; Yang and Du, 2022). The PCR results showed that BR-01 might contain ituA and ituD genes related to iturn synthesis, bmyB and bmyC genes for bacillomycinD synthesis, srfAA for surfactin synthesis, fenB and fenD fengycin synthesis, and bacA and bacD involving in bacilysin synthesis (Figure 6), indicating that B. velezensis BR-01 has the potential to produce the common antimicrobial peptides in B. velezensis.
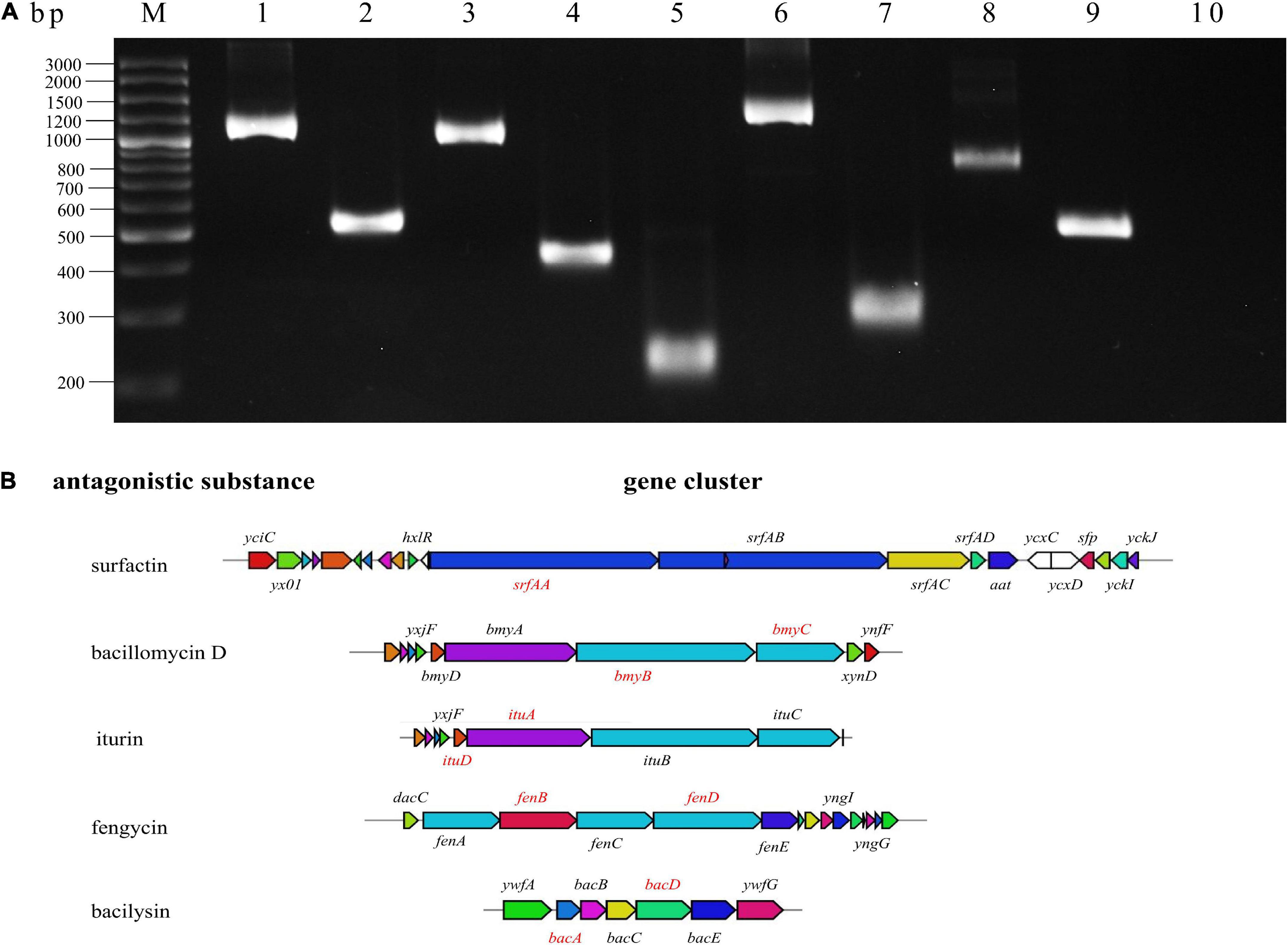
Figure 6. Amplification of antimicrobial peptide synthesis gene of B. velezensis BR-01. (A) Detection of antimicrobial peptide biosynthetic gene in B. velezensis BR-01 by PCR. M, DNA marker; 1, ituA; 2, ituD; 3, bmyC; 4, bmyB; 5, srfAA; 6, fenB; 7, fenD; 8, bacD; 9, bacA; 10, non-template control. (B) Five antimicrobial peptide biosynthetic gene clusters. The red gene denote the successful antibacterial peptide biosynthesis gene amplified by PCR. Pictures of antimicrobial peptide gene cluster were from antiSMASH (http://antismash.secondarymetabolites.org/).
Antagonistic activity test and liquid chromatograph-mass spectrometer preliminary identification of BR-01 culture extract
In this study, the antimicrobial peptide crude extract was successfully extracted from cell-free supernatants of B. velezensis BR-01. The results of the antagonistic activity assay of the antibacterial peptide crude extract showed that when an appropriate amount of crude extract methanol solution was added to the medium, the growth of the four indicator microbes was inhibited to varying degrees, indicating that crude extract of antimicrobial peptide from B. velezensis BR-01 has an antagonistic effect (Figure 7).
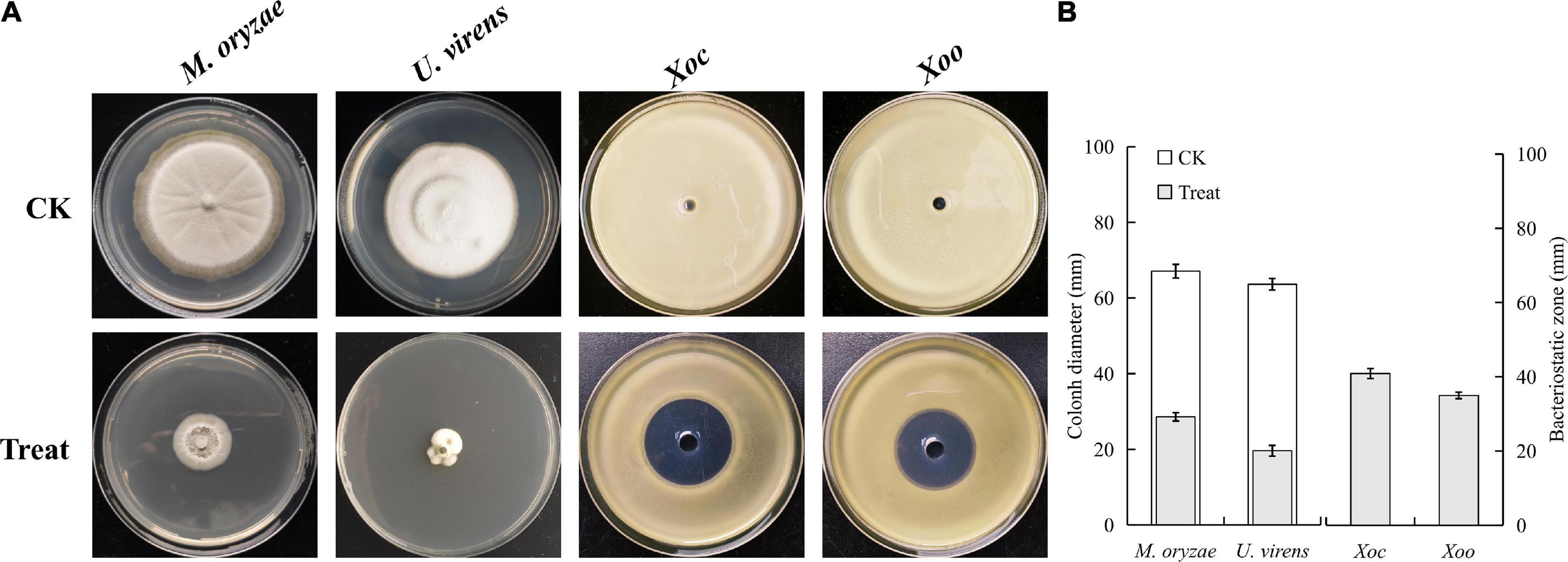
Figure 7. (A) Antibacterial activity test of B. velezensis BR-01 culture extract and (B) data statistics. Fungal plate photographs were taken when pathogenic fungi in the control group grew to about 3/4 of the culture dish. Bacterial plate photographs were taken after 2 days of culture. All plates were placed at 28°C.
The crude extract of antimicrobial peptide from B. velezensis BR-01 was analyzed by LC-MS detection and analysis technology (Xu et al., 2018). Studies have shown that antimicrobial peptides metabolized by Bacillus mainly include iturin, fengycin, and surfactin. Their specific composition and molecular weight are known. Combined with the peaks appearing in each time period of the chromatogram corresponding to the mass spectrum, a series of single peaks in the mass spectrum were analyzed according to the relative molecular mass and the structural characteristics of the “-CH2-” fat chain (Ma and Hu, 2019; Xia et al., 2019; Hasan et al., 2020). The mass spectra in the range of m/z 1000–2000 were mainly as follows: (1) m/z 1008, m/z 1022, m/z 1036, and m/z 1050 were speculated to be surfactin homologues; (2) m/z 1044, m/z 1058, m/z 1072, m/z 1086, and m/z 1100 were speculated as iturin homologues; (3) m/z 1463, m/z 1477, and m/z 1491 were speculated to be fengycin homologues. Based on the above results, it was speculated that the strain might produce three types of lipopeptide antibiotics: surfactin, iturin, and fengycin (Supplementary Figure 1 and Supplementary Table 3).
Biocontrol efficacy of BR-01 on rice bacterial streak and bacterial leaf blight
Under greenhouse conditions, the biological control effects of B. velezensis BR-01 on rice bacterial leaf streak and rice bacterial blight were determined (Li et al., 2019; Huang et al., 2021). After 10 days of inoculation, obvious lesions were found on the leaves of rice inoculated with Xoc and Xoo, respectively. The control effects of spraying B. velezensis BR-01 fermentation broth on rice bacterial leaf streak and rice bacterial blight in greenhouse were determined. The results showed that after spraying B. velezensis BR-01 fermentation broth, the incidence of the treatment group and the prevention group was significantly lower than that of the control group, and the control effects of the treatment group on rice bacterial leaf streak and rice bacterial blight were 64.19 and 66.16%, respectively. The control effects of B. velezensis BR-01 fermentation broth on rice bacterial leaf streak and rice bacterial blight in the prevention group were 56.54 and 55.41%, respectively, indicating that B. velezensis BR-01 fermentation broth had obvious control effects on rice bacterial leaf streak and rice bacterial blight (Figure 8).
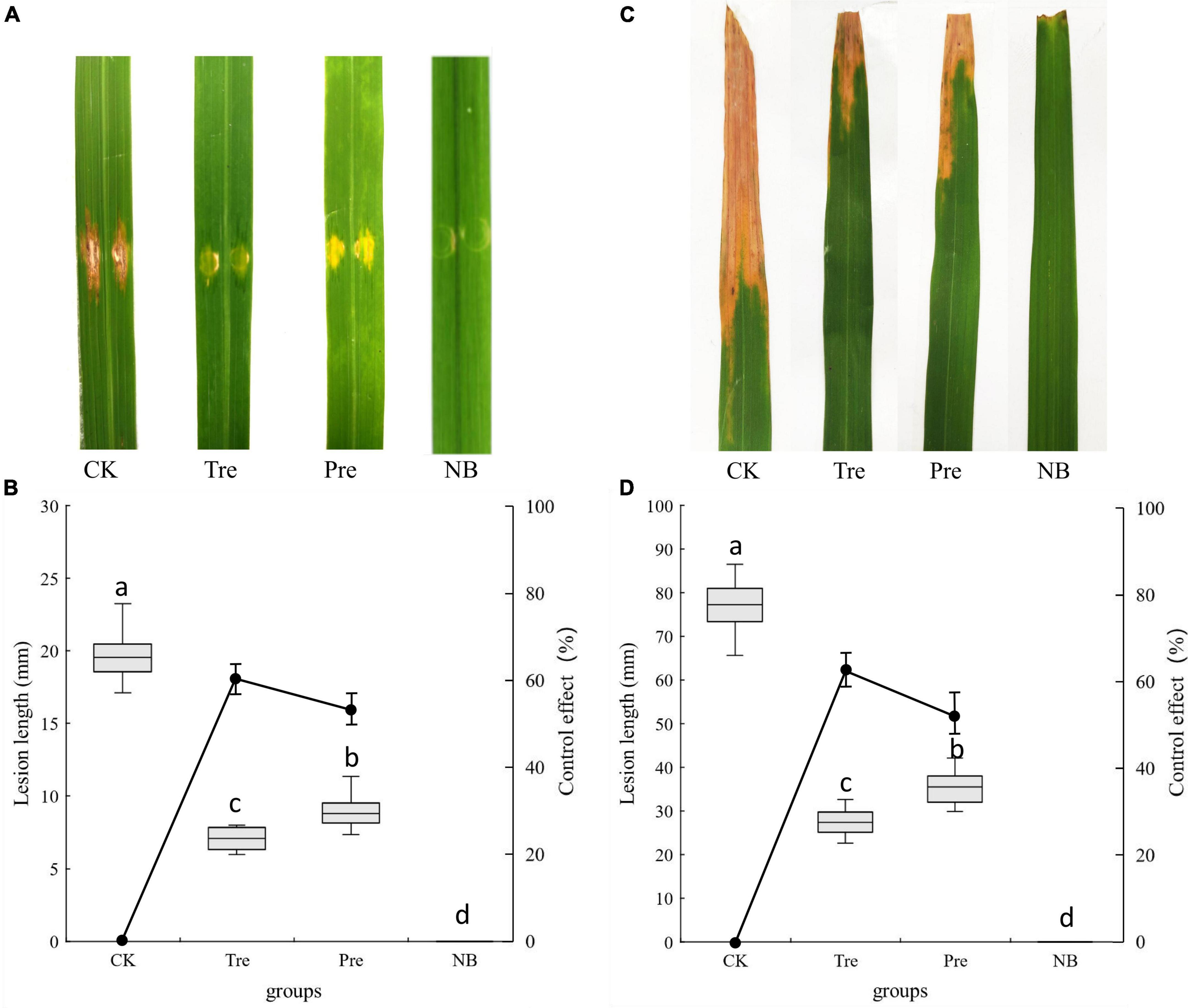
Figure 8. Effect of B. velezensis BR-01 on inhibition of rice bacterial leaf streak and rice bacterial blight under greenhouse conditions. (A) Representative lesions on rice leaves of rice bacterial leaf streak. (B) Lesions length of rice bacterial leaf streak and biocontrol effect of B. velezensis BR-01. (C) Representative lesions on rice leaves of rice bacterial blight. (D) Lesions length of rice bacterial blight and biocontrol effect of B. velezensis BR-01. Different lowercase letters represented significant difference (P < 0.05).
Discussion
With the improvement of low-carbon and sustainable agriculture, biological approaches are urgently needed to minimize crop yield losses resulting from pest activity and reduce impacts of pest management on human health and the environment (Baker et al., 2020). Rice, one of the most important staple crops, is especially concerned owing to its huge chemical consumption, including pesticides, plant growth regulators and chemical fertilizers. The biological control of rice diseases is particularly important both on economic and ecological aspects in the present world fraught with potential food crises.
Considering the vulnerability of rice to the infection of a variety of diseases, in this study, a B. velezensis BR-01 was isolated from Bolbostemmatis Rhizoma, a traditional Chinese medicine. The confrontation culture and Oxford cup assays demonstrated that B. velezensis BR-01 had strong inhibitory effect on M. oryzae, U. virens, F. fujikuroi, Xoc, and Xoo, five major rice pathogens but R. solani (Supplementary Table 4). B. velezensis BR-01 could produce protease, cellulase, β-1,3-glucanase, chitinase, indoleacetic acid, siderophore, and ACC deaminase, and might produce three lipopeptide antibiotics, surfactin, iturin, and fengycin based on LC-MS results. Furthermore, the plant assays showed that B. velezensis BR-01 had significant control effects on rice bacterial leaf streak and rice bacterial blight by pot experiments in greenhouse. To our knowledge, this is the only report about a B. velezensis strain which has a broad-spectrum against five major rice diseases. It was reasonably to expect reducing the frequency of farming operations using the multiple antagonistic agents.
Although the antagonistic efficacies between the different biocontrol agents were not compliant to be quantitively compared when the individual tests were not conducted on the same conditions, from the comparative literature analysis, we might still evaluate B. velezensis BR-01 to have the potential being a strong and broad-spectrum biocontrol agent in rice disease control. Currently, there have been numerous reports about the antagonistic effects and biocontrol efficacies of B. velezensis strains on M. oryzae, some of which were registered as the bacterial pesticides used in rice production (Chen et al., 2021). From our dual culture tests, B. velezensis BR-01 has an 83.7% relative inhibition rate on M. oryzae, which is comparable to most of other B. velezensis strains on M. oryzae (Sha et al., 2020; Chakraborty et al., 2021). B. velezensis BR-01 also showed antifungal activity against U. virens and F. fujikuroi, with relative inhibition rates 96.6 and 57.9%, respectively. U. virens is the causal agent of rice false smut disease, one of the most devastating rice fungal diseases in China (Miao et al., 2020). Rice false smut disease not only causes severe yield loss and grain quality reduction, but also threatens food safety due to its production of mycotoxins. U. virens specifically infects rice panicles before heading. The hyphae colonize in florets, and eventually form rice false smuts with powdery chlamydospores on rice grains (Fan et al., 2016; Baite et al., 2022). Once the disease occurs, it is difficult to control. F. fujikuroi is a prevalent plant pathogen, which causes the bakanae disease of the rice plant (Hossain et al., 2016). The infection sites of bakanae disease and false smut are totally different, however, their latent periods in the disease courses are long and intangible, which might result in inconvenience to precise prevention and control by using chemicals or fungicides. It is highly expected that biological agents might play important roles in preventing bakanae disease and false smut in niche competitions (Hossain et al., 2016; Baite et al., 2022).
As to rice bacterial diseases, B. velezensis BR-01 was demonstrated to have strong antagonistic effects on Xoc and Xoo on plate confrontation tests, and have the significant biocontrol efficacies on rice bacterial leaf streak and leaf blight in the pot experiments. Previously, some other B. velezensis strains have been reported having inhibitory effects on X. oryzae, for example, strain FZB42 isolated from the rhizosphere soil of lettuce (Wu et al., 2015), strain 504 from water spinach rhizosphere soil (Li et al., 2019), and HN-2 from soil (Jin et al., 2020), which were emphasized specifically antagonistic on Xoo and Xoc of rice pathogens. Recently, strain SF327 isolated from orchard soil has been demonstrated with functions of promoting plant growth and the inhibitory effects on three rice pathogens, i.e., M. oryzea, Xoo, and Xoc (Fang et al., 2022). The relative inhibition rate of B. velezensis BR-01 on Xoo PXO99A is similar to that of SF27 on Xoo PXO99A, which provided a strong support for biocontrol effect of B. velezensis BR-01 on rice bacterial diseases.
To data, there are several isolation approaches for B. velezensis strains used in rice disease control. First, soil microorganisms (including paddy field and non-paddy field). Soil is the “natural medium” of microorganisms and the most abundant strain resource pool (Wu et al., 2015; Jin et al., 2020; Fang et al., 2022). The second is plant rhizosphere bacteria. Because plant rhizosphere growth promoting bacteria (PGPR) have strong rhizosphere colonization ability and unique microbiological functions, they have become a hot spot in the research of biocontrol microorganisms (Liu et al., 2018). Plant endophytes are also an important source of biocontrol microorganisms. Their stable living space and strong colonization ability make them have excellent characteristics as biocontrol factors of plant diseases (Wen et al., 2004; Hossain et al., 2016). In addition, microorganisms in complex habitats (such as marine, lake, and river) show great potential as biocontrol factors of plant diseases and producers of bioactive substances because of their unique biodiversity. The B. velezensis isolates from different environments showed different antagonistic spectrum and growth promoting functions. It is reasonable to attribute the diversities of biological functions of certain bacteria to their different environmental adaptation, which prompts to isolate and screen more versatile antagonistic bacteria from different habitats.
In this study, strains were isolated from Chinese medicinal plants different from most crops for screening biocontrol microorganisms. Medicinal plants are a group of plants with special chemical composition and physiological functions, and are one of the main raw materials for screening natural medicinal ingredients (Lv et al., 2017). Because endophytes form a friendly kinship with host plants in the long-term coevolution process, some endophytes have the ability to produce the same or similar bioactive compounds as their host plants (Zhao et al., 2011; Lv et al., 2017). Therefore, in general, endophytic bacteria contained in plants with medicinal activity are more likely to produce antibiotic activity (Deng and Yang, 2010). Ding et al. (2010) analyzed the secondary metabolites of endophytic bacteria from the tissue of a Bruguiera Gymnorrhiza. For the first time, the anti-HIV activity of the indole semiterpenoid Xiamycin was obtained from bacteria. Chen et al. (2013) isolated a strain of B. amyloliquefaciens from Ophiopogon japonicus. The exopolysaccharide secreted by it has antitumor activity. Wang et al. (2021) isolated a pseudomonas fluorescens strain from the Bletilla striata, which had inhibitory effects on Staphylococcus aureus, Escherichia coli, Micrococcus luteus, and Pectobacterium carotovorum subsp. carotovorum. It was speculated that strain BSR2010 inhibited the growth of four pathogens by producing 2,4-diacetylphloroglucinol (2,4-DAPG). These studies indicate that endophytic bacteria of medicinal plants can produce a wide variety of secondary metabolites with novel structures, from which new antibacterial, antiviral, and antitumor active substances can be screened. The exploitation and utilization of endophytic bacteria in medicinal plants has potential theoretical and practical value.
Bolbostemmatis Rhizoma is the dry tuber of Bolbostemma paniculatum (Maxim.) Franquet, which contains triterpenoids, sterols and glycosides, anthraquinones, and alkaloids. Triterpenoids from Bolbostemmatis Rhizoma have anti-tumor, anti-inflammatory, and antiviral pharmacological effects (Yang Z. H. et al., 2021). So far, no reports have been reported on the isolation of endophytic bacteria from Bolbostemmatis Rhizoma with antagonistic activity against rice pathogens. In this study, endophytic bacteria were isolated from the tubers of Bolbostemma paniculatum (Maxim.) Franquet, and their antibacterial activities against a variety of rice pathogens were studied, to screen out excellent strains with broad-spectrum and high antibacterial activity, and then study the antibacterial components of their metabolites, so as to lay the foundation for the further development of Bolbostemmatis Rhizoma endophytic bacteria resources.
In conclusion, all parameters tested in vitro of strains isolated from Bolbostemmatis Rhizoma of B. velezensis RB-01 showed that they could antagonize a variety of major rice pathogens. This indicates that B. velezensis RB-01 is a new microbial resource for biological control of various rice diseases.
Data availability statement
The original contributions presented in this study are included in the article/Supplementary material, further inquiries can be directed to the corresponding authors.
Author contributions
Y-QH, JZ, and WJ conceived and designed the research and drafted the manuscript. JZ, YX, YL, and SL isolated and identified the bacterial strains. JZ, XL, and YML isolated the genome DNA and performed the PCR analysis. JZ, XM, and YX conducted the plant assays. JZ, SML, and YL carried out the LC-MS assays. YL, FL, and WJ conducted bioinformatical and statistical analysis. WJ and YX contributed to revising the manuscript. All authors contributed to the interpretation of data and approved the final manuscript.
Funding
This work was supported by the National Key R&D Program of China (2018YFD0200302), the Natural Science Foundation of Guangxi (2019GXNSFAA245052), and State Key Laboratory for Conservation and Utilization of Subtropical Agro-Bioresources (SKLCUSA-a201917).
Conflict of interest
The authors declare that the research was conducted in the absence of any commercial or financial relationships that could be construed as a potential conflict of interest.
Publisher’s note
All claims expressed in this article are solely those of the authors and do not necessarily represent those of their affiliated organizations, or those of the publisher, the editors and the reviewers. Any product that may be evaluated in this article, or claim that may be made by its manufacturer, is not guaranteed or endorsed by the publisher.
Supplementary material
The Supplementary Material for this article can be found online at: https://www.frontiersin.org/articles/10.3389/fmicb.2022.983781/full#supplementary-material
References
Ab Rahman, S. F. S., Singh, E., Pieterse, C. M. J., and Schenk, P. M. (2018). Emerging microbial biocontrol strategies for plant pathogens. Plant Sci. 267, 102–111. doi: 10.1016/j.plantsci.2017.11.012
Adeniji, A. A., Loots, D. T., and Babalola, O. O. (2019). Bacillus velezensis: phylogeny, useful applications, and avenues for exploitation. Appl. Microbiol. Biotechnol. 103, 3669–3682. doi: 10.1007/s00253-019-09710-5
Amruta, N., Prasanna Kumar, M. K., Narayanaswamy, S., Gowda, M., Channakeshava, B. C., Vishwanath, K., et al. (2016). Isolation and identification of rice blast disease-suppressing antagonistic bacterial strains from the rhizosphere of rice. J. Pure Appl. Microbiol. 10, 1043–1054.
Baite, M. S., Prabhukarthikeyan, S. R., and Raghu, S. (2022). Biological control of a fungus Ustilaginoidea virens causing false smut of rice. Biocontrol 67, 357–363. doi: 10.1007/s10526-022-10148-4
Baker, B. P., Green, T. A., and Loker, A. J. (2020). Biological control and integrated pest management in organic and conventional systems. Biol. Control. 140:104095. doi: 10.1016/j.biocontrol.2019.104095
Chakraborty, M., Mahmud, N. U., Ullah, C., Rahman, M., and Islam, T. (2021). Biological and biorational management of blast diseases in cereals caused by Magnaporthe oryzae. Crit. Rev. Biotechnol. 41, 994–1022. doi: 10.1080/07388551.2021.1898325
Chen, X. H., Koumoutsi, A., Scholz, R., Schneider, K., Vater, J., Sussmuth, R., et al. (2009). Genome analysis of Bacillus amyloliquefaciens FZB42 reveals its potential for biocontrol of plant pathogens. J. Biotechnol. 140, 27–37. doi: 10.1016/j.jbiotec.2008.10.011
Chen, Y. T., Yuan, Q., Shan, L. T., Lin, M. A., Cheng, D. Q., and Li, C. Y. (2013). Antitumor activity of bacterial exopolysaccharides from the endophyte Bacillus amyloliquefaciens sp. isolated from Ophiopogon japonicus. Oncol. Lett. 5, 1787–1792. doi: 10.3892/ol.2013.1284
Chen, Z., Zhao, L., Dong, Y., Chen, W., Li, C., Gao, X., et al. (2021). The antagonistic mechanism of Bacillus velezensis ZW10 against rice blast disease: evaluation of ZW10 as a potential biopesticide. PLoS One 16:e0256807. doi: 10.1371/journal.pone.0256807
Chung, E. J., Hossain, M. T., Khan, A., Kim, K. H., Jeon, C. O., and Chung, Y. R. (2015). Bacillus oryzicola sp. nov., an endophytic bacterium isolated from the roots of rice with antimicrobial, plant growth promoting, and systemic resistance inducing activities in rice. Plant Pathol. J. 31, 1–13. doi: 10.5423/PPJ.OA.12.2014.0136
Deng, X. P., and Yang, F. (2010). Study on endophytes in pharmaceutical plants and the related active substances. Anhui Agric. Sci. Bull. 16, 78–80. doi: 10.16377/j.cnki.issn1007-7731.2010.0102472
Deng, Y. W., Ning, Y. S., Yang, D. L., Zhai, K., Wang, G. L., and He, Z. H. (2020). Molecular basis of disease resistance and perspectives on breeding strategies for resistance improvement in crops. Mol. Plan. 13, 1402–1419. doi: 10.1016/j.molp.2020.09.018
Ding, L., Munich, J., Goerls, H., Maier, A., Fiebig, H. H., and Lin, W. H. (2010). Xiamycin, a pentacyclic indolosesquiterpene with selective anti-HIV activity from a bacterial mangrove endophyte. Bioorg. Med. Chem. Lett. 20, 6685–6687. doi: 10.1016/j.bmcl.2010.09.010
Dong, X. Z., and Cai, M. Y. (2001). Manual for Systematic Identification of Common Bacteria. Beijing: Science Press, 364–398.
Doni, F., Suhaimi, N. S. M., Mispan, M. S., Fathurrahman, F., Marzuki, B. M., Kusmoro, J., et al. (2022). Microbial contributions for rice production: from conventional crop management to the use of ‘omics’ technologies. Front. Microbiol. 23:737. doi: 10.3390/ijms23020737
Dunlap, C. A., Kim, S. J., Kwon, S. W., and Rooney, A. P. (2016). Bacillus velezensis is not a later heterotypic synonym of Bacillus amyloliquefaciens; Bacillus methylotrophicus, Bacillus amyloliquefaciens subsp. plantarum and ‘Bacillus oryzicola ’ are later heterotypic synonyms of Bacillus velezensis based on phylogenomics. Int. J. Syst. Evol. Microbiol. 66, 1212–1217. doi: 10.1099/ijsem.0.000858
Fan, B., Blom, J., Klenk, H. P., and Borriss, R. (2017). Bacillus amyloliquefaciens, Bacillus velezensis, and Bacillus siamensis form an “operational group B. amyloliquefaciens” within the B. subtilis species complex. Front. Microbiol. 8:22. doi: 10.3389/fmicb.2017.00022
Fan, B., Wang, C., and Song, X. F. (2018). Bacillus velezensis FZB42 in 2018: the gram-positive model strain for plant growth promotion and biocontrol. Front. Microbiol. 10:1279. doi: 10.3389/fmicb.2018.02491
Fan, J., Yang, J., Wang, Y. Q., Li, G. B., Li, Y., Huang, F., et al. (2016). Current understanding on Villosiclava virens, a unique flower-infecting fungus causing rice false smut disease. Mol. Plant Pathol. 17, 1321–1330. doi: 10.1111/mpp.12362
Fang, Y., Peng, Y., Liao, C., Chen, L., Zhou, Q., Huang, J., et al. (2022). Bacillus velezensis SF327, a potential biocontrol agent with the functions of preventing plant diseases and promoting plant growth. Acta Microbiol. Sin. doi: 10.13343/j.cnki.wsxb.20220128 Epub ahead of print].
Ghose, T. K. (1987). Measurement of cellulase activities. Pure Appl. Chem. 2, 257–268. doi: 10.1351/pac198759020257
Gond, S. K., Mishra, A., Sharma, V. K., Verma, S. K., Kumar, J., Kharwar, R. N., et al. (2012). Diversity and antimicrobial activity of endophytic fungi isolated from Nyctanthes arbor-tristis, a well-known medicinal plant of India. Mycoscience 53, 113–121. doi: 10.1007/s10267-011-0146-z
Gordon, S. A., and Weber, R. P. (1951). Colorimetric estimation of indoleacetic acid. Plant Physiol. 26, 192–195. doi: 10.1104/pp.26.1.192
Hasan, N., Farzand, A., Heng, Z., Khan, I. U., Moosa, A., Zubair, M., et al. (2020). Antagonistic potential of novel endophytic Bacillus strains and mediation of plant defense against Verticillium wilt in upland cotton. Plants Basel. 9:1438. doi: 10.3390/plants9111438
Holt, J. G., Krieg, N. R., Sneath, P. H. A., Staley, J. T., and Williams, S. T. (1994). Bergey’s Manual of Determinative Bacteriology. Baltimore, MD: Williams and Wilkins Press, 195–256.
Hossain, M. T., Khan, A., Chung, E. J., Rashid, H., and Chung, Y. R. (2016). Biological control of rice bakanae by an endophytic Bacillus oryzicola YC7007. Plant Pathol. J. 32, 228–241. doi: 10.5423/PPJ.OA.10.2015.0218
Huang, M. S., Yang, R. H., Yan, Y. C., Li, Y. L., Fang, Y., Zhou, Q., et al. (2021). Bacillus altitudinis 181-7, a potential biocontrol agent against bacterial leaf streak of rice. Acta Phytopathol. Sin. 56, 962–974. doi: 10.13926/j.cnki.apps.000555
Jain, A., Chatterjee, A., and Das, S. (2020). Synergistic consortium of beneficial microorganisms in rice rhizosphere promotes host defense to blight-causing Xanthomonas oryzae pv. oryzae. Planta 252:106. doi: 10.1007/s00425-020-03515-x
Jasim, B., Anisha, C., Rohini, S., Kurian, J. M., Jyothis, M., and Radhakrishnan, E. K. (2014). Phenazine carboxylic acid production and rhizome protective effect of endophytic Pseudomonas aeruginosa isolated from Zingiber officinale. World J. Microbiol. Biotechnol. 30, 1649–1654. doi: 10.1007/s11274-013-1582-z
Jepson, P. C., Murray, K., Bach, O., Bonilla, M. A., and Neumeister, L. (2020). Selection of pesticides to reduce human and environmental health risks: a global guideline and minimum pesticides list. Lancet Planet. Health. 4, E56–E63. doi: 10.1016/S2542-5196(19)30266-9
Jin, P., Wang, Y., Tan, Z., Liu, W., and Miao, W. (2020). Antibacterial activity and rice-induced resistance, mediated by C15surfactin A, in controlling rice disease caused by Xanthomonas oryzae pv. oryzae. Pest. Biochem. Physiol. 169:104669. doi: 10.1016/j.pestbp.2020.104669
Kanjanamaneesathian, M., Chumthong, A., Pengnoo, A., and Wiwattanapatapee, R. (2009). Bacillus megaterium suppresses major Thailand rice disease. Asian J. Food Agro. Ind. 2, S154–S159.
Kaspar, F., Neubauer, P., and Gimpel, M. (2019). Bioactive secondary metabolites from Bacillus subtilis: a comprehensive review. J. Nat. Prod. 82, 2038–2053. doi: 10.1021/acs.jnatprod.9b00110
Ke, Y. G., Hui, S. G., and Yuan, M. (2017). Xanthomonas oryzae pv. oryzae inoculation and growth rate on rice by leaf clipping method. Bio Protocol. 7:e2568. doi: 10.21769/BioProtoc.2568
Kuo, J., Chan, C. F., and Chi, W. C. (2021). Isolation of endophytic fungi with antimicrobial activity from medicinal plant Zanthoxylum simulans Hance. Folia Microbiol. 53, 113–121.
Li, X. Y., Zhang, T., Yang, H. M., Chu, M., Gao, Y., Zeng, J., et al. (2018). Detection of extracellular enzymes of antagonizing cotton Verticillium wilt bacteria and determination of enzyme activity. Xinjiang Agric. Sci. 55, 1663–1673. doi: 10.6048/j.issn.1001-4330.2018.09.012
Li, S. Z., Chen, Y., Yang, R. H., Zhang, C., Liu, Z., Li, Y., et al. (2019). Isolation and identification of a Bacillus velezensis strain against plant pathogenic Xanthomonas spp. Acta Microbiol. Sin. 59, 1969–1983. doi: 10.13343/j.cnki.wsxb.20180513
Lim, S. M., Yoon, M. Y., Choi, G. J., Choi, Y. H., Jang, K. S., Shin, T. S., et al. (2017). Diffusible and volatile antifungal compounds produced by an antagonistic Bacillus velezensis G341 against various phytopathogenic fungi. Plant Pathol J. 33, 488–498. doi: 10.5423/PPJ.OA.04.2017.0073
Limtong, S., Into, P., and Attarat, P. (2020). Biocontrol of rice seedling rot disease caused by Curvularia lunata and Helminthosporium oryzae by epiphytic yeasts from plant leaves. Microorganisms 8:647. doi: 10.3390/microorganisms8050647
Liu, K., Mclnroy, J. A., Hu, C. H., and Kloepper, J. W. (2018). Mixtures of plant-growth-promoting rhizobacteria enhance biological control of multiple plant diseases and plant-growth promotion in the presence of pathogens. Plant Dis. 102, 67–72. doi: 10.1094/PDIS-04-17-0478-RE
Liu, W. Y., Chen, W. G., Zhang, W. D., Chen, H. S., Gu, Z. B., and Li, Y. T. (2004). Studies on chemical constituents in bulbs of Bolbostemma paniculatum. China J. Chin. Mater. Med. 29, 953–956.
Lopes, R., Tsui, S., Goncalves, P., and de Queiroz, M. V. (2018). A look into a multifunctional toolbox: endophytic Bacillus species provide broad and underexploited benefits for plants. World J. Microbiol. Biotechnol. 34:94. doi: 10.1007/s11274-018-2479-7
Lv, Y. N., Sun, X. M., Zhao, B., Li, W. J., Liu, X. J., and Pu, G. Z. (2017). Diversity and biological effects of endophytic bacteria in medicinal plants. Chin. Wild Plant Resour. 36, 45–49. doi: 10.3969/j.issn.1006-9690.2017.06.010
Ma, Z. W., and Hu, J. C. (2019). Complete genome sequence of a marine-sediment-derived bacterial strain Bacillus velezensis SH-B74, a cyclic lipopeptides producer and a biopesticide. 3 Biotech 9:162. doi: 10.1007/s13205-019-1694-8
Ma, Z., Zhang, S., Sun, K., and Hu, J. (2020). Identification and characterization of a cyclic lipopeptide iturin A from a marine-derived Bacillus velezensis 11-5 as a fungicidal agent to Magnaporthe oryzae in rice. J. Plant. Dis. Prot. 127, 15–24. doi: 10.1007/s41348-019-00282-0
Mahmood, S., Freitag, T. E., and Prosser, J. I. (2006). Comparison of PCR primer-based strategies for characterization of ammonia oxidizer communities in environmental samples. FEMS Microbiol. Ecol. 56, 482–493. doi: 10.1111/j.1574-6941.2006.00080.x
Miao, J. K., Xu, H., Yang, H., Yan, H., Chu, J., Bai, Y. J., et al. (2020). Resistance evaluation of main rice cultivars to false smut rice in Liaoning province. Crops. 36, 38–46. doi: 10.16035/j.issn.1001-7283.2020.06.006
Mora, I., Cabrefiga, J., and Montesinos, E. (2011). Antimicrobial peptide genes in Bacillus strains from plant environments. Int. Microbiol. 14, 213–223. doi: 10.2436/20.1501.01.151
Muleta, D., Assefa, F., and Granhall, U. (2007). In vitro antagonism of rhizobacteria isolated from Coffea arabica L. against emerging fungal coffee pathogens. Eng. Life Sci. 7, 577–586. doi: 10.1002/elsc.200700004
Myo, E. M., Liu, B. H., Ma, J. J., Shi, L. M., Jiang, M. G., Zhang, K. C., et al. (2019). Evaluation of Bacillus velezensis NKG-2 for bio-control activities against fungal diseases and potential plant growth promotion. Biol. Control. 134, 23–31. doi: 10.1016/j.biocontrol.2019.03.017
Nascimento, F. X., Rossi, M. J., Soares, C. R. F. S., McConkey, B. J., and Glick, B. R. (2014). New insights into 1-aminocyclopropane-1-carboxylate (ACC) deaminase phylogeny, evolution and ecological significance. PLoS One 9:e99168. doi: 10.1371/journal.pone.0099168
Ou, Y. F., Men, X. Y., and Ge, F. (2014). Analysis of biological disasters in main food crops of China. Biol. Disaster Sci. 37:1. doi: 10.3969/j.issn.2095-3704.2014.01.001
Pastrana, A. M., Basallote-Ureba, M. J., Aguado, A., Akdi, K., and Capote, N. (2016). Biological control of strawberry soil-borne pathogens Macrophomina phaseolina and Fusarium solani, using Trichoderma asperellum and Bacillus spp. Phytopathol. Mediterr. 55, 109–120. doi: 10.14601/Phytopathol_Mediterr-16363
Penrose, D. M., and Glick, B. R. (2003). Methods for isolating and characterizing ACC deaminase-containing plant growth-promoting rhizobacteria. Physiol. Plant. 118, 10–15. doi: 10.1034/j.1399-3054.2003.00086.x
Plaza, G., Chojniak, J., Rudnicka, K., Paraszkiewicz, K., and Bernat, P. (2015). Detection of biosurfactants in Bacillus species: genes and products identification. J. Appl. Microbiol. 119, 1023–1034. doi: 10.1111/jam.12893
Qian, G. L., Hu, B. S., Jiang, Y. H., and Liu, F. Q. (2009). Identification and characterization of Lysobacter enzymogenes as a biological control agent against some fungal pathogens. Agric. Sci. China 8, 68–75. doi: 10.1016/S1671-2927(09)60010-9
Quibod, I. L., Atieza-Grande, G., Oreiro, E. G., Palmos, D., Nguyen, M. H., Coronejo, S. T., et al. (2020). The green revolution shaped the population structure of the rice pathogen Xanthomonas oryzae pv. oryzae. ISME J. 14, 492–505. doi: 10.1038/s41396-019-0545-2
Rabbee, M. F., and Baek, K. H. (2020). Antimicrobial activities of lipopeptides and polyketides of Bacillus velezensis for agricultural applications. Molecules 25:4973. doi: 10.3390/molecules25214973
Rani, A., Saini, K. C., Bast, F., Varjani, S., Mehariya, S., Bhatia, S. K., et al. (2021). A review on microbial products and their perspective application as antimicrobial agents. Biomolecules 11:1860. doi: 10.3390/biom11121860
Roberts, W. K., and Selitrennikoff, C. P. (1988). Plant and bacterial chitinases differ in antifungal activity. Microbiology 134, 169–176. doi: 10.1099/00221287-134-1-169
Rong, S. H., Xu, H., Li, L. H., Chen, R. J., Gao, X. L., and Xu, Z. J. (2020). Antifungal activity of endophytic Bacillus safensis B21 and its potential application as a biopesticide to control rice blast. Pest. Biochem. Physiol. 162, 69–77. doi: 10.1016/j.pestbp.2019.09.003
Ruiz-García, C., Béjar, V., Martínez-Checa, F., Llamas, I., and Quesada, E. (2005). Bacillus velezensis sp. nov., a surfactant-producing bacterium isolated from the river Vélez in Málaga, southern Spain. Int. J. Syst. Evol. Microbiol. 55, 191–195. doi: 10.1099/ijs.0.63310-0
Saechow, S., Thammasittirong, A., Kittakoop, P., Prachya, S., and Thammasittirone, S. N. R. (2018). Antagonistic activity against dirty panicle rice fungal pathogens and plant growth-promoting activity of Bacillus amyloliquefaciens BAS23. J. Microbiol. Biotechnol. 28, 1527–1535. doi: 10.4014/jmb.1804.04025
Sajitha, K. L., and Dev, S. A. (2016). Quantification of antifungal lipopeptide gene expression levels in Bacillus subtilis B1 during antagonism against sapstain fungus on rubberwood. Biol. Control. 96, 78–85. doi: 10.1016/j.biocontrol.2016.02.007
Schwyn, B., and Neilands, J. B. (1987). Universal chemical assay for the detection and determination of siderophores. Anal. Biochem. 160, 47–56. doi: 10.1016/0003-2697(87)90612-9
Sha, Y. X., Shuting, S., Qingchao, Z., and Shen, R. Q. (2019). Biocontrol potential of Bacillus velezensis strain E69 against rice blast and other fungal diseases. Chin. Agric. Sci. 52, 1908–1917. doi: 10.3864/j.issn.0578-1752.2019.11.006
Sha, Y., Zeng, Q., and Sui, S. (2020). Screening and application of Bacillus strains isolated from nonrhizospheric rice soil for the biocontrol of rice blast. Plant Pathol. J. 36, 231–243. doi: 10.5423/PPJ.OA.02.2020.0028
Shakeel, M., Rais, A., Hassan, M. N., and Hafeez, F. Y. (2015). Root associated Bacillus sp improves growth, yield and zinc translocation for basmati rice (Oryza sativa) varieties. Front. Microbiol. 6:1286. doi: 10.3389/fmicb.2015.01286
Shan, H., Zhao, M., Chen, D., Cheng, J., Li, J., Feng, J., et al. (2013). Biocontrol of rice blast by the phenaminomethylacetic acid producer of Bacillus methylotrophicus strain BC79. Crop Prot. 44, 29–37. doi: 10.1016/j.cropro.2012.10.012
Shasmita, Swain, B. B., Mohapatra, P. K., Naik, S. K., and Mukherjee, A. K. (2022). Biopriming for induction of disease resistance against pathogens in rice. Planta 255:113. doi: 10.1007/s00425-022-03900-8
Shi, Z. R., Hong, W. W., and Wang, Q. W. (2022). Complete genome resource of Bacillus velezensis J17-4, an endophyte isolated from stem tissues of rice. Plant Dis. 106, 727–729. doi: 10.1094/PDIS-05-21-0996-A
Sumi, C. D., Yang, B. W., Yeo, I. C., and Hahm, Y. T. (2015). Antimicrobial peptides of the genus Bacillus: a new era for antibiotics. Can. J. Microbiol. 61, 93–103. doi: 10.1139/cjm-2014-0613
Tan, H. J. (2022). Review and prospect of biological pesticides in China. World Pestic. 44, 16–27. doi: 10.16201/j.cnki.cn10-1660/tq.2022.04.03.2022
Teixeira, G. M., Mosela, M., Nicoletto, M. L. A., Ribeiro, R. A., Hungria, M., Youssef, K., et al. (2021). Genomic insights into the antifungal activity and plant growth-promoting ability in Bacillus velezensis CMRP 4490. Front. Microbiol. 11:618415. doi: 10.3389/fmicb.2020.618415
Torres, M., Llamas, I., Torres, B., Toral, L., Sampedro, I., and Bejar, V. (2019). Growth promotion on horticultural crops and antifungal activity of Bacillus velezensis XT1. Appl. Soil Ecol. 150:103453. doi: 10.1016/j.apsoil.2019.103453
Wang, H. Y., Li, C. Y., Song, F. J., Lin, A. H., and Meng, Y. Y. (2021). Isolation and identification of an endophytic bacterium from Bletilla striata and preliminary study of antibacterial mechanism. J. South Cent. Univ. Natl. Nat. Sci. Ed. 40, 246–251. doi: 10.12130/znmdzk.20210305
Wang, W. F., Qiu, Z. Q., Tan, H. M., and Wang, W. F. (2014). Siderophore production by actinobacteria. Biometals 27, 623–632. doi: 10.1007/s10534-014-9739-2
Wen, C. Y., Wu, Y. H., and Tian, X. L. (2004). Recent advances and issues on the endophyte. Chin. J. Plant Ecol. 23, 86–91. doi: 10.13292/j.1000-4890.2004.0054
Wu, L., Wu, H., Chen, L., Yu, X., Borriss, R., and Gao, X. (2015). Difficidin and bacilysin from Bacillus amyloliquefaciens FZB42 have antibacterial activity against Xanthomonas oryzae rice pathogens. Sci. Rep. 5:12975. doi: 10.1038/srep12975
Xia, J. J., Chen, J. W., Song, Y., Liu, Y. T., Dong, J., Wu, Y., et al. (2019). Identification of antibacterial substances from Bacillus amyloliquefaciens HE and analysis of antibacterial characteristics. South China Fish. Sci. 15, 41–49. doi: 10.12131/20190054
Xu, B. H., Ye, Z. W., Zheng, Q. W., Wei, T., Lin, J. F., and Guo, L. Q. (2018). Isolation and characterization of cyclic lipopeptides with broad-spectrum antimicrobial activity from Bacillus siamensis JFL15. 3 Biotech 8:444. doi: 10.1007/s13205-018-1443-4
Yamamoto, S., and Harayama, S. (1995). PCR amplification and direct sequencing of gyrB genes with universal primers and their application to the detection and taxonomic analysis of Pseudomonas putida Strains. Appl. Environ. Microbiol. 61:3768. doi: 10.1128/aem.61.3.1104-1109.1995
Yang, B., and Bogdanove, A. (2013). Inoculation and virulence assay for bacterial blight and bacterial leaf streak of rice. Methods Mol. Biol. 956, 249–255. doi: 10.1007/978-1-62703-194-3_18
Yang, B., and Du, C. M. (2022). Review on secondary antibiotic metabolites of Bacillus velezensis. Chin. J. Biol. Control. 38, 502–512. doi: 10.16409/j.cnki.2095-039x.2022.02.007
Yang, L. P., Quan, X., Xue, B. G., Goodwin, P. H., Lu, S. B., Wang, J. H., et al. (2015). Isolation and identification of Bacillus subtilis strain YB-05 and its antifungal substances showing antagonism against Gaeumannomyces graminis var. tritici. Biol. Control. 85, 52–58. doi: 10.1016/j.biocontrol.2014.12.010
Yang, S. Q., Zhang, F., and Ma, G. L. (2017). Purification and inhibitive mechanism of antagonist substances from Bacillus velezensis S6 Strain. Agrochemicals 56:660. doi: 10.16820/j.cnki.1006-0413.2017.09.007
Yang, Y. Y., Ji, Q. Q., Sun, J. D., Wang, J. W., Qi, H. Q., Liu, Y., et al. (2021). Screening of Bacillus methylotrophicus producing broad-spectrum antibacterial active substances and identification of lipopeptides. J. Dalian Polytech. Univ. 40, 401–406. doi: 10.19670/j.cnki.dlgydxxb.2021.0603
Yang, Z. H., Ruan, Z. J., Liao, H., and Huang, X. H. (2021). Research progress on the anti-tumor function of tubeimosides. J. Biol. 38, 86–90. doi: 10.3969/j.issn.2095-1736.2021.05.086
Yu, G. Y., Sinclair, J. B., Hartman, G. L., and Bertagnolli, B. L. (2002). Production of iturin A by Bacillus amyloliquefaciens suppressing Rhizoctonia solani. Soil Biol. Biochem. 34, 955–963. doi: 10.1016/S0038-0717(02)00027-5
Zhang, G. Y., Wang, Y. H., Kuo, M., Zhang, T. T., Wang, B., and Liu, H. M. (2022). Isolation and identification of a cellulase-producing endophytic fungus in paris polyphylla var. yunnanensis. Biotechnol. Bull. 38, 95–104. doi: 10.13560/j.cnki.biotech.bull.1985.2021-0266
Zhang, H. J., Tao, L. M., Liu, X., and Huang, X. Z. (2022). Review on registration and management of bio-pesticide in China. Chin. J. Bio. Control. 38, 9–17. doi: 10.16409/j.cnki.2095-039x.2022.01.012
Zhang, Y. H., Zhao, M. X., Chen, W., Yu, H. L., Jia, W. T., Pan, H. Y., et al. (2022). Multi-omics techniques for analysis antifungal mechanisms of lipopeptides produced by Bacillus velezensis GS-1 against Magnaporthe oryzae In vitro. Int. J. Mol. Sci. 23:3762. doi: 10.3390/ijms23073762
Zhang, L., Huang, W., Wang, N., Song, B., Zhu, J., Abdukeyim, R., et al. (2021). Identification of radiation-resistant Bacillus velezensis JK23 and its biocontrol activity. Chin. J. Bio. Control. 37, 885–862. doi: 10.16409/j.cnki.2095-039x.2021.06.011
Zhao, J., Shan, T., Mou, Y., and Zhou, L. (2011). Plant-derived bioactive compounds produced by endophytic fungi. Mini Rev. Med. Chem. 11, 159–168. doi: 10.2174/138955711794519492
Keywords: Bacillus velezensis, Bolbostemmatis Rhizoma, biological control, broad-spectrum antagonistic activities, rice pathogens
Citation: Zhou J, Xie Y, Liao Y, Li X, Li Y, Li S, Ma X, Lei S, Lin F, Jiang W and He Y-Q (2022) Characterization of a Bacillus velezensis strain isolated from Bolbostemmatis Rhizoma displaying strong antagonistic activities against a variety of rice pathogens. Front. Microbiol. 13:983781. doi: 10.3389/fmicb.2022.983781
Received: 01 July 2022; Accepted: 30 August 2022;
Published: 28 September 2022.
Edited by:
Tofazzal Islam, Bangabandhu Sheikh Mujibur Rahman Agricultural University, BangladeshReviewed by:
Aniruddha Sarker, Kyungpook National University, South KoreaMeixiang Zhang, Nanjing Agricultural University, China
Copyright © 2022 Zhou, Xie, Liao, Li, Li, Li, Ma, Lei, Lin, Jiang and He. This is an open-access article distributed under the terms of the Creative Commons Attribution License (CC BY). The use, distribution or reproduction in other forums is permitted, provided the original author(s) and the copyright owner(s) are credited and that the original publication in this journal is cited, in accordance with accepted academic practice. No use, distribution or reproduction is permitted which does not comply with these terms.
*Correspondence: Wei Jiang, d2VpamlhbmdAZ3h1LmVkdS5jbg==; Yong-Qiang He, eXFoZUBneHUuZWR1LmNu