- 1Department of Microbiology and Biotechnology, University School of Sciences, Gujarat University, Ahmedabad, Gujarat, India
- 2Department of Biochemistry and Forensic Science, University School of Sciences, Gujarat University, Ahmedabad, Gujarat, India
- 3Finnish Museum of Natural History, University of Helsinki, Helsinki, Finland
- 4Department of Pharmacology, College of Pharmacy, Umm Al-Qura University, Makkah, Saudi Arabia
Microbial surfactants are amphiphilic surface-active substances aid to reduce surface and interfacial tensions by accumulating between two fluid phases. They can be generically classified as low or high molecular weight biosurfactants based on their molecular weight, whilst overall chemical makeup determines whether they are neutral or anionic molecules. They demonstrate a variety of fundamental characteristics, including the lowering of surface tension, emulsification, adsorption, micelle formation, etc. Microbial genera like Bacillus spp., Pseudomonas spp., Candida spp., and Pseudozyma spp. are studied extensively for their production. The type of biosurfactant produced is reliant on the substrate utilized and the pathway pursued by the generating microorganisms. Some advantages of biosurfactants over synthetic surfactants comprise biodegradability, low toxicity, bioavailability, specificity of action, structural diversity, and effectiveness in harsh environments. Biosurfactants are physiologically crucial molecules for producing microorganisms which help the cells to grasp substrates in adverse conditions and also have antimicrobial, anti-adhesive, and antioxidant properties. Biosurfactants are in high demand as a potential product in industries like petroleum, cosmetics, detergents, agriculture, medicine, and food due to their beneficial properties. Biosurfactants are the significant natural biodegradable substances employed to replace the chemical surfactants on a global scale in order to make a cleaner and more sustainable environment.
Introduction
Now-a-days, microbial surfactants are taking place in humans’ lifestyles abundantly, a lavish component of their routine products like cosmetics, food additives, and detergents. They are also widely used in the petroleum, medical, pharmaceutical, agricultural, and environmental sectors. Using biodegradable microbial surfactants instead of synthetic surfactants will help improve the economy and reduce environmental issues (Bhardwaj et al., 2013). The hazardous effluents produced during the manufacturing of synthetic surfactants have a negative impact on the environment. Hence, their market attractiveness has fallen despite their cost-effectiveness. Microbial surfactants are natural, biodegradable, and non-toxic, and as a result, their market demand is steadily increasing. The global market size of chemical surfactants is projected to reach a CAGR (compound annual growth rate) of 5.3% from 2020 to 2027 (Dixit et al., 2020), while for biosurfactants it is expected to grow over 5.5% CAGR between 2020 and 2026, especially for rhamnolipids it will possibly reach over USD 145 million (Ahuja and Singh, 2020).
The most extensively used microorganisms for biosurfactant production involve Pseudomonas spp. and Bacillus spp. from oil-contaminated sites, effluent, wastewater, etc. Besides these, some fungi like Candida spp., Torulopsis spp., Pichia spp., Aspergillus spp. (Bhardwaj et al., 2013) and marine microbes like Alcanivorax borkumensis, Alcaligenes spp., Arthrobacter spp., Myroides spp., Yarrowia lipolytica, Pseudomonas nautical (Maneerat, 2005) are also reported with a substantial amount of biosurfactants. The biosynthetic pathway for biosurfactant production in microorganisms depends on the substrates and the cultural conditions, making them assorted in chemical composition. Biosurfactants range from low molecular weight to high weight and comprise glycolipids, lipopeptides, neutral lipids, phospholipids, and polymeric biosurfactants (Shah et al., 2016).
The carbon source may come from hydrocarbons, carbohydrates, and lipids, which may be used separately or in combination. Various chromatographic and spectroscopic methods confirm these surface-active compounds’ chemical structure and functional groups. Biosurfactants can also be produced from cheap raw materials from large quantities of agricultural byproducts/waste (Bhardwaj et al., 2013). The process of economics and environmental credentials makes biosurfactants more attractive when produced using relatively simple and inexpensive waste products as substrates. The present review deals with fundamental aspects of microbial surfactants, including their classes, properties, producing microbes, biosynthesis, production, recovery, and characterization, along with the recent market potential, patents, and novel applications.
Classification
The nature of biosurfactants depends on the microbial origin and the nutrient availability, according to which they are classified into two categories based on their molecular weight and chemical composition (Figure 1). Based on the size, they are divided into two types, low molecular weight and high molecular weight biosurfactants. The low molecular weight biosurfactants can reduce the surface and interfacial tensions at the air and water interfaces. In contrast, high molecular weight biosurfactants are found effective in stabilizing the oil in water emulsions and are known as “bioemulsans.” They can work at low concentrations and have many substrate specificities, making them highly efficient emulsifiers. A few well-known biosurfactants’ chemical structures are given in Figure 2.
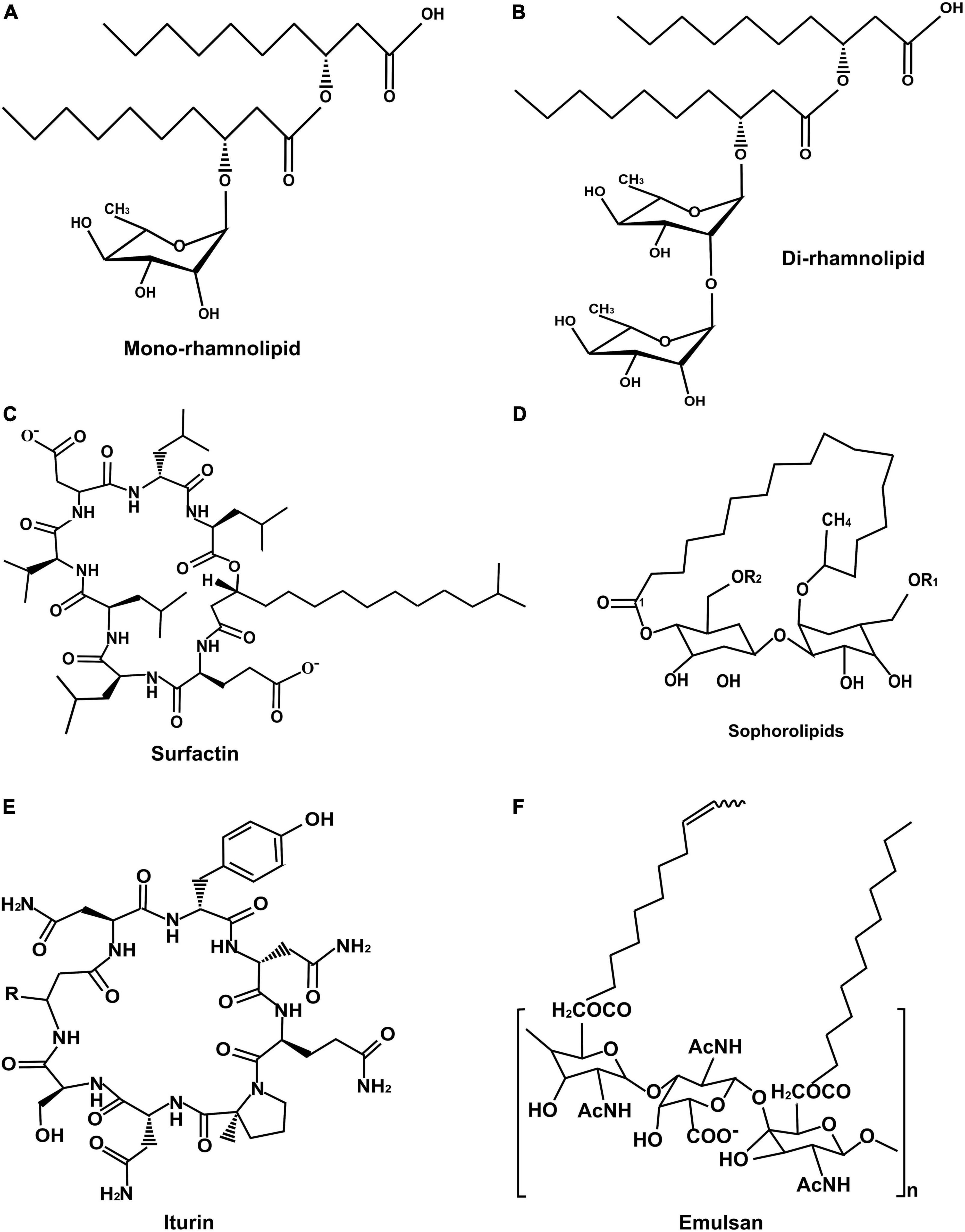
Figure 2. Structure of important biosurfactants: (A) Mono-rhamnolipid, (B) Di-rhamnolipid, (C) Surfactin, (D) Sophorolipid, (E) Iturin, and (F) Emulsan.
Furthermore, biosurfactants are classified based on their polarity as anionic or neutral compounds containing hydrophilic and hydrophobic domains. Carbohydrates, amino acids, phosphate groups, or other compounds are in the hydrophilic domain. In contrast, the hydrophobic domain is generally a long-chain fatty acid or derivative of fatty acids (Maneerat, 2005). Saranraj et al. (2022a) introduced some new biosurfactants like mannosylerythritol lipids (MELs), lichenysin, ituri, fengycin, viscosin, arthrofactin, amphisin, putisolvin, serrawettin, etc.
Properties
Synthetic surfactants are expensive and cause environmental problems because of toxicity and resistance to degradation. Microbial surfactants are the best alternative to synthetic surfactants as they show significant advantages over synthetic ones (Figure 3). The substantial properties of biosurfactants that makes them eligible to replace the synthetic surfactants are discussed here, which help evaluate their performance and selection of a potential microorganism.
Surface and interfacial activity
Surface tension is created when the water droplet molecules are whispered together by a strong intermolecular and attractive, cohesive force on the surface (Figure 4A). Biosurfactants can reduce different solutions’ surface and interfacial tensions (Figure 4B) at very low concentrations because of their lower critical micelle concentrations (CMC).
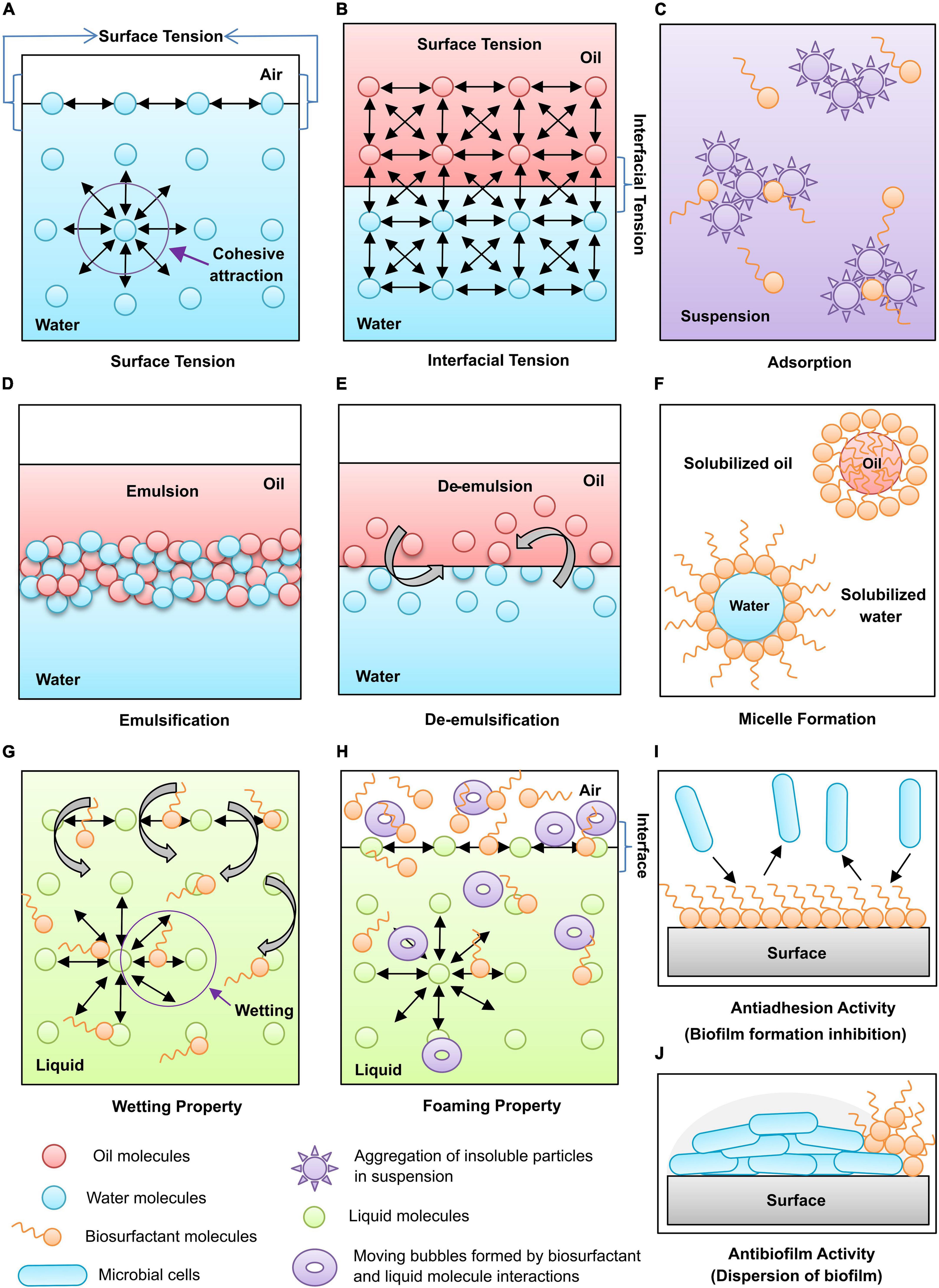
Figure 4. Functional properties of biosurfactants: (A) Surface tension, (B) Interfacial tension, (C) Adsorption, (D) Emulsification, (E) De-emulsification, (F) Micelle formation, (G) Wetting property, (H) Foaming property, (I) Antiadhesion activity, and (J) Antibiofilm activity.
Emulsification
Biosurfactants can play a dual role, an emulsifier or a de-emulsifier. Emulsions are of two types: oil-in-water and water-in-oil emulsions. Generally, the emulsions prepared with two different phase solutions are not stable. The addition of biosurfactants allows dispersion of one liquid into another and helps two immiscible liquids to be mixed, which signifies micellular solubilization with large particles (Figure 4D).
De-emulsification
The de-emulsification process breaks the emulsions by disrupting the stable surface between the internal and bulk phases (Figure 4E). This process helps to deal with the problems created by the natural emulsifying agents in oil recovery and production processes like corrosion of equipment used in the petroleum industries.
Solubilization
A high concentration of biosurfactants will form micellar structures (Figure 4F), which encapsulate and transport the insoluble molecules at higher levels in the solution. They increase the solubility of water-insoluble substances in aqueous solutions or organic solvents. Biosurfactants are proved more efficient than synthetic surfactants in solubilizing the complex mixture of molecules into an aqueous solution.
Wetting
A spreading and penetrating power of biosurfactants that reduces the surface tension of liquids by decreasing the attractive forces between similar particles and increasing affinity toward dissimilar surfaces is known as wetting ability. Biosurfactants can act as wetting agents by entering the pores rather than associating them with the surface tension (Figure 4G). Wetting agents is imperative when reconstructing dry compounds like powders, beads, or reagents in solid-phase devices.
Foaming
Biosurfactants are concentrated on the gas-liquid interface to form fizzes through the liquid, forming foam formation (Figure 4H). The bubbling techniques study surface-active molecules’ foaming properties, e.g., surfactin, sodium dodecyl sulfate (SDS), and bovine serum albumin (BSA).
Adsorption
Adsorption enables strong interactions between biosurfactants and hydrophobic substrates, which helps to enhance the recovery of biosurfactants from oil from rock or production media (Figure 4C). The biosurfactants’ adsorption property is the ability to act as an anti-adhesive agent (Figure 4I). Biosurfactants arbitrate the synthesis and stabilization of nanoparticles by adsorption which prevents aggregation and stabilization of nanoparticle formulations (Sadiq et al., 2022).
Dispersion
Some biosurfactants are used as a dispersant to prevent the aggregation of insoluble particles with one another in the suspension. The reduction in cohesive attraction among similar particles leads to dispersion (Figure 4J). It desorbs the hydrophobic molecules from rock surfaces to enhance their mobility and recovery, which is helpful in oilfield applications. The dispersion also helps to inhibit or remove the biofilm formation of harmful microbes, hence biosurfactant are useful in making wound healing formulations.
Flocculation
Flocculation is a process in which emulsion droplets stick together to form cluster-like structures called flocs. These flocs are not permanent and can be broken by mechanical action, thus restoring emulsions to their original form. Biosurfactants with flocculating ability have applications in environmental cleaning processes.
Biodegradability
Being a microbial product, biosurfactants can easily be degraded in nature or in treatment plants without producing harmful end products. This most significant feature makes them a superior environment-friendly compound (Saranraj et al., 2022b).
Low toxicity, biocompatibility, and digestibility
Biosurfactants are natural compounds with very low toxicity and can also be digested by humans, therefore widely used in the food and pharmaceutical industries. They also have righteous compatibility with many compounds used in cosmetics.
Tolerance to extreme conditions
The biosurfactants produced by some extremophiles are popular because of their ability to resist extreme environmental factors like temperature, pH, and ionic strength. Ibrahim (2017) reported the rhamnolipids produced by Ochrobactrum anthropic HM-1 and Citrobacter freundii HM-2 with excellent stability at 50–100°C for 30 min, 2.0–12.0 pH, and 2–10% NaCl.
Biosynthesis
Many researchers have studied biosynthetic pathways for the construction of biosurfactants. Being a biomolecule, each biosurfactant follows a different biosynthetic pathway as the nutritional and environmental conditions provided affect the microbial growth and its production, making them structurally diverse.
Rhamnolipid biosynthesis
The synthesis of fatty acid moieties for rhamnolipid differs from the general fatty acid biosynthesis at the ketoacyl reduction level (Kubicki et al., 2019). The de novo fatty acid biosynthesis supplies significant fatty acids to produce rhamnolipids by Pseudomonas aeruginosa as a model bacterium (Figure 5) for producing glycolipids. Rhamnose molecules are present in P. aeruginosa as a cell wall constituent in lipopolysaccharide (LPS). The rhamnose derives carbon from glycerol instead of acetate by condensing two carbon units formed by glycerol without splitting or rearranging their C–C bonds. Glycerol carbon provides all the carbons needed for rhamnolipid synthesis, whereas acetate can supply carbon for only β-hydroxydecanoic acid, an intermediate of β-oxidation.
Two glycosyltransferase units, i.e., rhamnosyltransferase I and rhamnosyltransferase II, primarily catalyze both mono- and di-rhamnolipids. The products of genes rhlA and rhlB organized by the bicistronic operon showed the sovereign activity of RhlA and RhlB proteins (Wittgens et al., 2017). The gene encodes for rhamnosyltransferase II, i.e., rhlC is localized at alternative chromosomal sites separately from rhlA and rhlB in P. aeruginosa. rhlA and rhlC genes are bound to the inner membrane, while rhlB is a membrane-bound gene. RhlA was studied to synthesize 3-(3-hydroxyalkanoyloxy) alkanoic acid (HAA) from the activated hydroxy fatty acid. In contrast, the glycosyltransferase RhlB catalyzes the condensation between dTDP-L-rhamnose (deoxy thymidine diphosphate L-rhamnose) and HAA to form mono-rhamnolipids. The RhlC involves di-rhamnolipid [L-rhamnose-L-rhamnose-3-(3-hydroxyalkanoyloxy) alkanoic acid] synthesis using mono-rhamnolipid as a substrate combined with dTDP-L-rhamnose. It shows sequence homology with rhamnosyltransferases linked in LPS synthesis (Pardhi et al., 2021b).
3-(3-Hydroxyalkanoyloxy) alkanoic acid already has surface-active properties and can be released in the cell’s environment as biosurfactants necessary for rhamnolipid production, but its function is unknown. RhlG enzyme is involved with rhamnolipid synthesis by draining the fatty acid precursors, and it also affects the polyhydroxyalkanoates (PHA) synthesis. HAA is a common compound involved in the origin of rhamnolipid and PHA synthesis, but PHA synthesis is not essential for rhamnolipids production. The RhlG provides the acyl carrier protein (ACP), a fatty acid precursor to synthesize the 4-hydroxy-2-alkylquinolines (HAQs) having QS-related Pseudomonas quinolone signal (PQS). The rhlA, rhlB, and rhlC genes are not only found in P. aeruginosa but are reported from other genera like Burkholderia paseudomallei, Bacillus thailandensis, and Escherichia coli as an essential protein for rhamnolipid synthesis (Varjani and Upasani, 2017).
Recent studies showed that the biosynthetic pathways involved with marine biosurfactants originated from non-marine bacteria (Kubicki et al., 2019). AlgC plays a central role in the biosynthetic pathway of dTDP-D-glucose, D-rhamnose, and dTDP-L-rhamnose. AlgC transforms D-glucose-6-phosphate to D-glucose-1-phosphate (precursor of dTDP-D-glucose and dTDP-L-rhamnose), which is used to produce LPS and exopolysaccharide alginate. RmlA, RmlB, RmlC, and RmlD are enzymes of the rmlABCD operon, catalyzing the dTDP-L-rhamnose pathway in P. aeruginosa.
Surfactin biosynthesis
The general biosynthetic pathway of surfactin produced by Bacillus subtilis is shown in Figure 6. A special character called non-ribosomal peptide synthetases (NRPS) catalyzed by multi-enzymatic thiotemplates are assembled modularly to synthesize surfactin, a lipopeptide biosurfactant. This multi-modular enzymatic assembly carries acyl chain initiation, elongation, and termination, catalyzed through protein molecules. The NRPS catalyzes reactions like incorporating lipids, lactonization, or epimerization. Each module contains different domains and helps incorporate and change one specific amino acid in the peptide chain. A prototypic module contains three domains, i.e., condensation, adenylation, and thiolation domain/peptidyl carrier protein (PCP) domain. The condensation domain catalyzes direct condensation of the thioesterified intermediates in the growing chain. An adenylation domain selects the amino acid for the respective module and releases the pyrophosphate by catalyzing the aminoacyl adenosine formation from adenosine triphosphate (ATP) and cognate amino acid. The thiolation domain supports the covalent bonding of activated amino acids, and the 4′-phosphopantetheine prosthetic group exists on the PCP through a thioester linkage (Shaligram and Singhal, 2010).
The epimerization domains usually help transform L- to D-amino acids. It shows that the composition of non-ribosomal peptides contains amino acids except proteinogenic ones. The operon srfA (25 kb) determines that NRPS comprises three multi-functional proteins encoded by srfA-A srfA-B, and srfA-C. The proteins SrfA-A (402 kDa), SrfA-B (401 kDa), SrfA-C (144 kDa), and a small subunit SrfA-D (40 kDa) are important for the initiation reactions of surfactin (Shaligram and Singhal, 2010). SrfA-A and SrfA-B are three-modular proteins; SrfA-C is a mono-modular with a thioesterase domain, and SrfA-D is a subunit (Figure 6; Kubicki et al., 2019).
A starter molecule, 3-hydroxy fatty acid, classically known as 3-hydroxy-13-methyl-myristic acid, was recognized by the first module’s condensation domain, containing seven amino acids (L-glutamate, L-leucine, D-leucine, L-valine, L-aspartate, D-leucine, and L-leucine) are successively added through seven modules. The thioesterase (TE) domain of termination module SrfA-C catalyzes the product and lactonization of the depsipeptide after the entire acyl chain is synthesized. These TE domains are chain-terminating protein moieties (25–30 kDa) generally found in the fatty acid biosynthesis. Some TE domains are reported as hydrolases and some for carrying regio- and stereo-specific reactions, while TE domains of SrfA-C are noted with a prominent intramolecular cyclization feature. An acyl-O-TE intermediate is engaged for intramolecular detention by a nucleophilic group of the acyl chain instead of undergoing hydrolysis (Kohli et al., 2001; Tanovic et al., 2008). Ali et al. (2022a) has discussed the influence of quorum sensing and CRISPRi technology on surfactin.
Microorganisms
Some microorganisms can use various substrates considered potentially harmful to other non-biosurfactant-producing microbes and produce structurally diverse biosurfactants. The composition and yield of the biosurfactant produced exclusively depend upon the sites from where the microorganisms are isolated, their genetic makeup, physiological conditions, and the various nutrients utilized by the organisms. Oil-contaminated sites like crude oil contaminated localities, petrochemical industrial waste, tannery effluents, used edible oils, and oil reservoirs are the major spots for the collection of samples for isolation of potential biosurfactant producers. Moreover, extremophiles are also reported from marine environments to produce extensively stable biosurfactants.
The genera Pseudomonas and Bacillus are very well explored for biosurfactant production contributing approximately 50–60% of the reported bacteria (Table 1). However, several fungi like Candida spp. and Pseudozyma spp. are also recognized as the principal biosurfactants producers. The bacterial producers are discovering each type of biosurfactant, while fungi are reported with a maximum production of glycolipids such as sophorolipids and mannosylerythritol lipid (Table 2).
Brevibacterium casei MSA19, Streptomyces spp. MAB36, Bacillus circulans, Aspergillus ustus MSF3, and Nocardiopsis alba MSA10 are a few marine microbes producing biosurfactants used in the medical field as they exhibit antimicrobial, anti-adhesive, and anti-biofilm activities against human pathogens (Gudiña et al., 2016). Besides natural strains, some mutant or recombinant strains like Pseudomonas aeruginosa 59C7, Bacillus licheniformis KGL11, Acinetobacter calcoaceticus RAG-1, Gordonia amarae gave 2–4 times more yield than the native strains (Mukherjee et al., 2006).
Production
Microorganisms utilize a wide range of complex organic substrates to get carbon and energy by converting them into simpler forms through fermentation. They produce significant products like ethanol, amino acids, vitamins, polysaccharides, etc. Biosurfactants are one of the secondary metabolites produced during such fermentation processes. Submerged and solid-state fermentations are used for biosurfactant production based on the microorganism’s nature.
Substrates
The choice of a suitable substrate is critical for commercially and economically effective biosurfactant manufacturing. Researchers have explored inexpensive resources to replace the costlier substrates, such as agro-industrial wastes, vegetable oil mill effluents (coconut, canola, olive, grape seed, palm, rapeseed, sunflower, soybean oil), dairy and sugar industry byproducts (buttermilk, whey, molasses), starch industry extract and wastes (corn, potatoes, tapioca, wheat) (Saranraj et al., 2022c). Using these substrates will reduce production costs while also helping conserve the environment. The low-cost carbon sources are utilized to increase the biosurfactant yield (Tables 1, 2).
Submerged fermentation
Submerged production processes are ideal for biosurfactant-producing bacteria and yeasts as they require water for optimum growth. Biosurfactants are extracellular compounds released by bacteria in the fermentation broth, making them simple to purify. However, some valuable compounds may have been known to leach out of the liquid portion during recovery, which is a disadvantage of submerged fermentation (SmF). Many researchers have designed the mineral salt medium and studied the submerged biosurfactant production using the shake flask method (Pardhi et al., 2020). De Rienzo et al. (2016) carried out a rhamnolipid production in a 10 L laboratory-scale bioreactor using Burkholderia thailandensis E264 and Pseudomonas aeruginosa ATCC 9027. Candida bombicola and Pseudomonas aeruginosa were reported with 34 and 20 g/L sophorolipids in 50 L bioreactor, respectively (Shah et al., 2007; Zhu et al., 2007).
Solid state fermentation
Solid state fermentation (SSF) generally uses solid materials such as molasses, wheat bran, cassava dregs, rice husk, cassava bagasse, coffee husk, banana peel, tapioca peel, etc., as a substrate are usually low-cost, carbon and protein-rich renewable wastes. Successful solid-state fermentations are reported for biosurfactant production by Aspergillus fumigatus, Phialemonium spp., and Pleurotus ostreatus using rice husk with defatted rice bran, soy oil or diesel oil, and sunflower seed oil, respectively (Martins et al., 2006; Velioğlu and Öztürk Ürek, 2015). In addition, some bacterial strains like Serratia rubidaea SNAU02, Brevibacterium aureum MSA13, and Bacillus pumilus UFPEDA 448 showed more rhamnolipids and lipopeptides production using SSF than SmF (Kiran G. et al., 2010; Slivinski et al., 2012; Nalini and Parthasarathi, 2014).
Recovery and purification
The economic recovery and downstream processes account for almost 60% of total production costs, will ensure the commercial viability of a bioprocess. Biosurfactants’ physicochemical features, such as surface or micelle forming activity, make them easier to recover than other secondary metabolites. The most often reported methods for biosurfactant recovery are listed in Table 3.
Biosurfactants are extracted mainly by organic solvents but most of them are toxic; hence researchers have replaced them with low toxic and cheap solvents that reduce the recovery expenses. A single downstream process is not sufficient to recover and purify the biosurfactant. Hence, multi-step recovery strategies with a series of purification and concentration steps are used, allowing for better quality recovered products at different stages. Crude biosurfactants can be obtained for environmental cleanup at a low cost with only a few early recovery processes.
Characterization
Various chromatographic and spectrophotometric methods are widely used for biosurfactant characterization individually or in combination, depending on the type of biosurfactant. The structural characterization of the biosurfactants will help to figure out their applications in different fields.
The phospholipids, rhamnolipids, and lipopeptides were separated by thin layer chromatography (TLC) using chloroform:methanol:water solvent system (Pekin et al., 2005; Daverey and Pakshirajan, 2009; Nwaguma et al., 2016). High-performance liquid chromatography (HPLC) is generally used to separate and identify the lipopeptide-type biosurfactants. For glycolipids, the HPLC device must be coupled with an evaporative light scattering detector (ELSD) or mass spectrometry (MS). It was observed that HPLC coupled with other devices like ultra-HPLC-MS are faster than the qualitative HPLC. Recently Bartal et al. (2018) identified surfactin isomers from Bacillus subtilis SZMC 6179J using HPLC-ESI-MS (electrospray ion-mass spectrometry).
Fourier Transform-Infrared Spectroscopy (FT-IR) analysis through classical KBr disk was used for lipopeptides produced by Bacillus spp. and Virgibacillus salaries (Elazzazy et al., 2015; López-prieto et al., 2019). In recent years, a new FT-IR approach has been introduced, i.e., attenuated total reflectance (ATR) crystal accessory which give rapid and more effective results. Reports of surfactin analysis through ATR confirmed it as a successful improved technique of FT-IR (Bezza and Chirwa, 2015; Pardhi et al., 2021a). Daverey and Pakshirajan (2009) identified the chemical configurations of sophorolipid and trehalose lipid through NMR. Mass spectrophotometry (MS) is generally coupled with other techniques for better performance like gas chromatography-MS (GC-MS), electrospray ion-MS (ESI-MS), secondary ion-MS (SIMS), liquid chromatography-ESI-MS (LC-ESI-MS), ultra-high-performance liquid-high-resolution-MS (UHPLC-HRMS), and matrix-assisted laser desorption/ionization-time of flight-MS (MALDI TOF-MS). The newly discovered biosurfactants, lichenysin-A, and aneurinifactin are purified and characterized by MALDI TOF-MS (Joshi et al., 2016; Balan et al., 2017).
Patents and worldwide production of biosurfactants
The demand for biosurfactants is progressively growing as the most desirable green surface-active product to replace the synthetic one. But the high cost of production prevents them from becoming the most considerable product in their field; therefore, researchers are emphasizing an ideal biosurfactant producing strains, alternative low-cost substrates, and minimal bioreactor process. To achieve these approaches, researchers have studied many biosurfactants and published the patents with their exclusive properties (Table 4).
Recently, Allied Market Research stated that the global chemical surfactants market size was valued at 41.3 billion USD in 2019 and is projected to reach 58.5 billion USD by 2027, registering a compound annual growth rate (CAGR) of 5.3% from 2020 to 2027 (Dixit et al., 2020). While according to the survey by Global Market Insight, the biosurfactants market size exceeded 1.5 billion USD in 2019 and is expected to grow at over 5.5% CAGR between 2020 and 2026 (Ahuja and Singh, 2020). Increasing emphasis on replacing petrochemical-based surfactants owing to high toxicity, low sustainability, and shelf-life should drive the product demand. The financial requirements of large-scale biosurfactant production are high, yet some companies manufacture biosurfactants globally (Table 5) to fulfill the public demand. Among all the biosurfactants, the rhamnolipids has the highest market share and is expected to grow over 5% CAGR in the future, especially in the Asia-Pacific region, owing to high consumption from countries like India, Japan, and China (Ahuja and Singh, 2020). After rhamnolipids, sophorolipids are the most selling products in the cosmetic sector (Table 5).
Applications of biosurfactants
Biosurfactants are significant compounds having the potential to replace synthetic surfactants. They have many applications in industrial sectors like petroleum, organic chemicals, pharmaceuticals, cosmetics, foods and beverages, bioremediation, petrochemicals, biological control, etc. (Figure 7). The potential biosurfactants and their applications are reported in Table 6.
Petroleum industry
Biosurfactants augment the removal and biodegradation of oil through mobilization, de-emulsification, solubilization, or emulsification. Rhamnolipids and surfactins showed better petroleum removal capacity than the synthetic surfactants from soil. The glycolipids from Ochrobactrum anthropic HM-1, Citrobacter freundii HM-2, and Pseudoxanthomonas spp. G3 efficiently recovered 70%, 67%, and 20% of residual oil from the sand-packed column (Ibrahim, 2017). In addition, Jain et al. (2012) recovered >90% lubricant oil from sandy soil using 1% (w/v) biosurfactant. Alike bacteria, Fusarium spp. BS-8 (JQ860113) was also reported with 46% enhanced oil recovery (Qazi et al., 2013). Rhamnolipid (0.4 mg/mL) was reported to remove 90% Mb, 30% Ni, and 70% Vd. In comparison, lipopeptide (17.34 mg/mL) removed 44.5% carbon from the harmful spent hydrodesulfurization (HDS) catalyst produced by petroleum refineries (Alsaqer et al., 2018). The cleaning and maintenance of oil storage containers are often problematic, as hazardous compounds used for cleaning generate a massive volume of harmful wastes. An oil sludge fraction deposited on the walls or bottom of the storage tanks is incredibly viscous semisolid particles and difficult to remove using conventional pumping. Oil-contaminated vessels were cleaned within 15 min using a biosurfactant of P. aeruginosa SH 29 (Diab and Din, 2013).
Environment
Biosurfactants are used in environmental protection for oil spill control and detoxifying oil-contaminated industrial effluents and soils. Their ability to stabilize oil/water emulsions and increase the hydrocarbon solubility enhances biodegradation and removal of hydrocarbon from the soil (Shah et al., 2022). An environment-friendly surfactin was reported with 100% biodegradation of activated sludge within 4 days (Fei et al., 2019). Rhamnolipids had efficiently removed Ni and Cd from soils (80–100%) and field samples (20–80%) (Mulligan and Wang, 2004). The crude oil (89%) was desorbed through lipopeptide (Al-dhabi and Esmail, 2020) and efficiently gas-oil was removed (86.7%) from soil by rhamnolipid (Gonzini et al., 2010). Obayori et al. (2009) reported 95.29% and 92.34% degradation of diesel and crude oil using biosurfactant. An emulsion of rhamnolipid-silica nanoparticles efficiently worked as a dispersant to remediate the crude oil-seawater system (Pi et al., 2015). For a sustainable environment, the most prominent field for the application of biosurfactants is bioremediation.
Agriculture
Biosurfactants are used for various purposes in agriculture, such as improving soil quality, removal of common water-soluble pollutants, helping to eliminate plant pathogens, supporting valuable plant-microbe interactions, pesticide preparations, etc. The rhamnolipid removed pentachlorophenol (PCP) from sand-soil (60%) and sandy-silt soils (61%) (Mulligan and Eftekhari, 2003). A biosurfactant reported with 72% degradation of anthracite related to Fe-stimulation within 48 days (Santos et al., 2008). Bee et al. (2019) observed efficient antifungal activity of rhamnolipid and surfactin against Fusarium oxysporum f. spp. ricini. A lipopeptide allegedly inhibited the anthracnose-causing pathogen Colletotrichum gloeosporioides in papaya leaves (Kim et al., 2010). A surfactin was used to treat the Rhizoctonia solani infected maize crop which led the production of defense enzymes (Ali et al., 2022b). Such properties make biosurfactants useful in phytopathogenic control. The biosurfactant from Serratia marcescens UCP 1549 was reported with 125% stimulation of cabbage seed germination (Araújo et al., 2019). A glycolipid significantly stimulated the growth promoting factors of Capsicum annuum L. (Ravinder et al., 2022).
Detergent industry
Now-a-days, public awareness is rising for the environmental risks linked with synthetic surfactants. Hence, a demand for eco-friendly biosurfactants which can substitute the laundry detergent is stimulated for soaps, shampoos, and washing liquids preparations. The biosurfactant forms micelles to remove the oily stains from the desired material by attracting their hydrophilic moieties. The detergent mixture of surfactin and subtilisin A efficiently removed immobilized rubisco stain from hydrophilic (75%) and hydrophobic (80%) surfaces (Onaizi et al., 2009). A rhamnolipid (0.01%) competently removed the marker stains from the whiteboard (Turbekar et al., 2014). The biosurfactant produced by Klebsiella spp. RJ-03 was reported to remove up to 80% lubricant oil from cotton cloth (Jain et al., 2012). Similarly, rhamnolipid, lipopeptide, and glycolipid removed 61.43% sunflower oil, 75% motor oil, 81% tea stains, and 86% burned engine oil from cotton fabric (Bafghi and Fazaelipoor, 2012; Bouassida et al., 2018).
Medical industry
The toxicity of biosurfactants is exerted on the permeability of cell membranes in a manner similar to that of detergents. Biosurfactants have biological properties such as antibacterial, anti-adhesive, anticancer, anti-mycoplasma, and hemolytic, making them a viable compound in the medical and cosmetic sectors. The rhamnolipids have shown antimicrobial activity against Aspergillus niger, Gliocladium virens, Chaetomium globosum, Penicillium chrysogenum, Aureobasidium pullulans, Botrytis cinerea, Rhizoctonia solani, Penicillium chrysogenum, Candida albicums, Bacillus pumilus, Micrococcus luteus, and Sarcina lutea (Abalos et al., 2001; El-Sheshtawy and Doheim, 2014). Lunasan, a new biosurfactant, has demonstrated antimicrobial activity against Streptococcus oralis (68%), Staphylococcus epidermidis (57.6%), Candida albicans (57%) and also exhibited anti-adhesive effect against Streptococcus agalactiae (100%), Streptococcus sanguis (100%), Pseudomonas aeruginosa (92%) (Luna et al., 2011). Thanomsub et al. (2007) reported rhamnolipid A and B having anti-proliferative activity against human breast cancer cell line and insect cell line C6/36 with a minimum inhibitory concentration of 6.25 μg/mL and 50 μg/mL, respectively. A water soluble polysaccharide kefiran produced by Lactobacillus kefiranofaciens ATCC 43761 showed anticancer activity with 193.89 μg/mL of IC50 against breast cancer (MCF-7) cells (Dailin et al., 2020). These properties make biosurfactants a suitable applicant for biomedical preparations.
Cosmetic industry
Cosmetic applications are one of the extraordinary parts of multifunctional biosurfactants. The applications depend on their excellent surface properties, including emulsification, detergency, solubilization, dispersion, wetting, and foaming effects. They also showed antioxidant activity, anti-irritating effects, and compatibility with skin with better moisturizing properties (Patel et al., 2022). Rhamnolipids, sophorolipids, and mannosylerythritol lipids (MELs) exhibit skin compatibility, low skin-irritation, and moisturizing properties, replacing the petrochemical-based surfactants applied in top cosmetic preparations like anti-wrinkle and anti-aging products (Table 4). MELs are introduced in the cosmetic field for exclusive liquid-crystal-forming and moisturizing assets and are mainly used in preparations preventing skin roughness. A sodium dodecyl sulfate (SDS)-damaged human skin cells showed 77.1% viability and self-assembling property after penetration of di-acylated MEL-B, which formed lyotropic liquid crystals to moisturize the skin (Morita et al., 2011). Concaix (2003) reported sophorolipids as stimulators of skin fibroblast metabolism, which helps in restoring, protecting, and repairing skin. They also reduce the subcutaneous fat overload by stimulating leptin synthesis in adipocytes, allowing cellulite treatment (Pellecier and André, 2004). MEL-A (0.5%) and MEA-B (0.5%) are studied for increasing the tensile strength of damaged hairs up to 122 gf/p and 119.4 gf/p; hence can be used for damaged hair treatment (Morita et al., 2010).
Food industry
Biosurfactants generally play a role in food formulating ingredients as fat stabilizers, food emulsifiers, and anti-adhesive agents. It is also used to control the agglomeration of fat globules, stabilize aerated systems, improve the texture and shelf-life of starch-containing products, modify the rheological properties of wheat dough, and improve the consistency and texture of fat-based products. Biosurfactants can decrease the adhesion of pathogenic organisms to solid surfaces or infection sites, hence used to protect the food products (Zaman et al., 2022). A biosurfactant extracted from Lactobacillus paracasei spp. paracasei A20 showed anti-adhesive activity against L. reuteri (77.6–78.8%), L. casei (56.5–63.8%), Streptomyces sanguis 12 (72.9%), S. mutans HG985 (31.4%), Staphylococcus aureus (76.8%), S. epidermidis (72.9%), S. agalactiae (66.6%), Pseudomonas aeruginosa (21.2%), E. coli (11.8%) (Gudiña et al., 2010). Long-term consumption of heavy metal contaminated vegetables may cause numerous human health hazards. A glycolipid was reported with 59% biofilm inhibition, 73% Cd removal from garlic, and antimicrobial activity against E. coli (Anjum et al., 2016). The biosurfactants increased the emulsion stability of fruit salad dressing from 51.4 to 62.8% (Sridhar et al., 2015). The muffins treated with lipopeptide were observed to reduce hardness and stickiness and showed improved softness (Kiran G. S. et al., 2010). A new glycolipid, diacyl mannosyl erythritol, showed an ice-packing factor of 35% for 8 h, thus helpful in improving ice slurry’s storage ability (Kitamoto et al., 2001).
Miscellaneous applications
Besides these, biosurfactants are commercially used in pulp, paper, paint, plastic, leather, and textile industries, along with ceramics and uranium ore processing. This is because the biosurfactants have de-resinification and pulp washing, defoaming, color smoothing, antistatic agent, pigment dispersion, coating, latex stabilization, retard sedimentation, emulsification, and wetting capability. The polymeric biosurfactant has shown potential as a wood adhesive material (Pervaiz and Sain, 2010). A biosurfactant producing Cobetia marina is patented as an additive of paint formulation for submersible surfaces (Dinamarca-Tapia et al., 2012). Rhamnolipid (Raza et al., 2014) and saponin (Leighs et al., 2018) are reported for scouring cotton fibers and wools, respectively. The biosurfactant-producing Meyerozyma guilliermondii and Acidithiobacillus spp. co-inoculated to solubilize the toxic metals like Zn (76.5%), Ni (59.8%), Cu (22%), Cr (9.8%), Cd (9.8%), and Pb (7.1%) from sewage sludge in 10 days, hence suitable for bioleaching (Camargo et al., 2018).
Conclusion
Biosurfactants possess the fundamental physico-chemical properties like surface tension reduction, micelle formation, emulsification and adsorption as like chemical surfactants but low toxicity and biodegradability give them edge over the synthetic one. Apart from known producers like Bacillus and Pseudomonas, many other genera like Burkholderia, Serratia, Klebsiella, Pseudozyma, and Fusarium were reported for biosurfactants. Rhamnolipids are the most widely used biosurfactants followed by sophorolipids in industries. A number of new biosurfactants with diverse applications are also introduced, namely aneurinifactin, ponctifactin, lichenysin-A, and friulimicin-B. Biosurfactants are in high demand as a prospective product in industries like petroleum, healthcare, cosmetics, detergents, agriculture, medicine, the environment, and food due to their beneficial characteristics. The potential of biosurfactants to replace synthetic surfactants and dominate the global market is hindered by their high manufacturing costs, despite the fact that they are a green surface-active product with steadily rising demand. Abundant opportunities exist to explore novel microbial strains that produce novel biosurfactants using inexpensive alternative substrates with minimal bioreactor process. The biodegradable microbial surfactants will be highlighted as one of nature’s most promising products for the environmental preservation and healthy future generations.
Author contributions
KR contributed to the conceptualization and supervision. DP contributed to the methodology and writing – original draft. RP, VR, and RJ contributed to the formal analysis. KR, PP, and WA contributed to the writing – review and editing. WA contributed to the fund acquisition. All authors contributed to the article and approved the submitted version.
Funding
This work was funded by Deanship of Scientific Research at Umm Al-Qura University for the supporting this work by Grant Code (Project Code: 22UQU4310387DSR12). Open access funding by the University of Helsinki, Helsinki, Finland.
Acknowledgments
We would like to thank the Deanship of Scientific Research at Umm Al-Qura University, Makkah, Saudi Arabia for supporting this work by Grant Code (Project Code: 22UQU4310387DSR12) and the University of Helsinki, Helsinki, Finland for providing open access support.
Conflict of interest
The authors declare that the research was conducted in the absence of any commercial or financial relationships that could be construed as a potential conflict of interest.
Publisher’s note
All claims expressed in this article are solely those of the authors and do not necessarily represent those of their affiliated organizations, or those of the publisher, the editors and the reviewers. Any product that may be evaluated in this article, or claim that may be made by its manufacturer, is not guaranteed or endorsed by the publisher.
Supplementary material
The Supplementary Material for this article can be found online at: https://www.frontiersin.org/articles/10.3389/fmicb.2022.982603/full#supplementary-material
References
Abalos, A., Pinazo, A., Infante, M., Casals, M., Garcia, F., and Manresa, A. (2001). Physicochemical and antimicrobial properties of new rhamnolipids produced by Pseudomonas aeruginosa AT10 from soybean oil refinery wastes. Langmuir 17, 1367–1371. doi: 10.1021/la0011735
Abd-al-hussan, G. F., Abd, F., Hussein, A. L., and Jassim, M. (2016). Extraction, purification and characterization of biosurfactant from Bacillus subtilis. J. Kerbala Univ. 14, 186–192.
Abouseoud, M., Maachi, R., and Amrane, A. (2007). Biosurfactant production from olive oil by Pseudomonas fluorescens. Commun. Curr. Res. Educ. Top Appl. Microbiol. 1, 340–347.
Adamu, A., Ijah, U. J. J., Riskuwa, M. L., Ismail, H. Y., and Ibrahim, U. B. (2015). Study on biosurfactant production by two Bacillus species. Int. J. Sci. Res. Knowl. 3, 13–20. doi: 10.12983/ijsrk-2015-p0013-0020
Ahimou, F., Jacques, P., and Deleu, M. (2001). Surfactin and iturin an effects on Bacillus subtilis surface hydrophobicity. Enzym. Microb. Technol. 27, 749–754. doi: 10.1016/S0141-0229(00)00295-7
Ahuja, K., and Singh, S. (2020). Biosurfactants Market size by Products. Glob Mark Insight. Availbale Online at: https://www.gminsights.com/industry-analysis/biosurfactants-market-report (accessed Apr 5, 2021).
Al-dhabi, N. A., and Esmail, G. A. (2020). Enhanced production of biosurfactant from Bacillus subtilis strain Al-Dhabi-130 under solid-state fermentation using date molasses from Saudi Arabia for bioremediation of crude-oil-contaminated soils. Int. J. Environ. Res. Public Health 17:8446. doi: 10.3390/ijerph17228446
Ali, S. A. M., Sayyed, R. Z., Reddy, M. S., Enshasy, H. E., and Hameeda, B. (2022a). “Delving through quorum sensing and CRISPRi strategies for enhanced surfactin production,” in the Biosurfatnats: Production and Applications in Bioremediation/Reclaimation, ed. R. Z. Sayyed (USA: CRC Press- Taylor & Francis group), 59–79. doi: 10.1201/9781003260165-4
Ali, S. A. M., Sayyed, R. Z., Mir, M. I., Hameeda, B., Khan, Y., Alkhanani, M. F., et al. (2022b). Induction of systemic resistance in maize and antibiofilm activity of surfactin from Bacillus velezensis MS20. Front. Microbiol. 13:879739. doi: 10.3389/fmicb.2022.879739
Alsaqer, S., Marafi, M., Banat, I. M., and Ismail, W. (2018). Biosurfactant-facilitated leaching of metals from spent hydrodesulphurization catalyst. J. Appl. Microbiol. 125, 1358–1369. doi: 10.1111/jam.14036
Anjum, F., Gautam, G., Edgard, G., and Negi, S. (2016). Bioresource Technology Biosurfactant production through Bacillus spp. MTCC 5877 and its multifarious applications in food industry. Bioresour. Technol. 213, 262–269. doi: 10.1016/j.biortech.2016.02.091
Araújo, H. W. C., Andrade, R. F. S., Rodríguez, D. M., Ribeaux, D. R., Alves, C. A., and Takaki, G. M. C. (2019). Sustainable biosurfactant produced by Serratia marcescens UCP 1549 and its suitability for agricultural and marine bioremediation applications. Microb. Cell Fact. 18:2. doi: 10.1186/s12934-018-1046-0
Astuti, I. D., Purwasena, I. A., Putri, R. K., Amaniyah, M., and Sugai, Y. (2019). Screening and characterization of biosurfactant produced by Pseudoxanthomonas spp. G3 and its applicability for enhanced oil recovery. J. Pet. Explor. Prod. Technol. 9, 2279–2289. doi: 10.1007/s13202-019-0619-8
Bafghi, M. K., and Fazaelipoor, M. H. (2012). Application of rhamnolipid in the formulation of a detergent. J. Surfactants Deterg. 15, 679–684. doi: 10.1007/s11743-012-1386-4
Balan, S. S., Kumar, C. G., and Jayalakshmi, S. (2016). Pontifactin, a new lipopeptide biosurfactant produced by a marine Pontibacter korlensis strain SBK-47: purification, characterization and its biological evaluation. Process Biochem. 51, 2198–2207. doi: 10.1016/j.procbio.2016.09.009
Balan, S. S., Kumar, C. G., and Jayalakshmi, S. (2017). Aneurinifactin, a new lipopeptide biosurfactant produced by a marine Aneurinibacillus aneurinilyticus SBP-11 isolated from Gulf of Mannar: purification, characterization and its biological evaluation. Microbiol. Res. 194, 1–9. doi: 10.1016/j.micres.2016.10.005
Bartal, A., Vigneshwari, A., Boka, B., Voros, M., Takacs, I., Kredics, L., et al. (2018). Effect of different cultivation parameters on the production of surfactin variants by a Bacillus subtilis strain. Molecules 23:2675. doi: 10.3390/molecules23102675
Bayoumi, R., Haroun, B., Ghazal, E., and Maher, Y. (2010). Structural analysis and characteristics of biosurfactants produced by some crude oil utilizing bacterial strains. J. Basic Appl. Sci. 4, 3484–3498.
Bednarski, W., Adamczak, M., Tomasik, J., and Plaszczyk, M. (2004). Application of oil refinery waste in the biosynthesis of glycolipids by yeast. Bioresour. Technol. 95, 15–18. doi: 10.1016/j.biortech.2004.01.009
Bee, H., Khan, M. Y., and Sayyed, R. Z. (2019). “Microbial surfactants and their significance in agriculture,” in in the PGPR: Prospects for Sustainable Agriculture, ed. S. R. Antonious (Singapore: Springer-Nature), 205–216. doi: 10.1007/978-981-13-6790-8_18
Bezza, F. A., and Chirwa, E. M. N. (2015). Production and applications of lipopeptide biosurfactant for bioremediation and oil recovery by Bacillus subtilis CN2. Biochem. Eng. J. 101, 168–178. doi: 10.1016/j.bej.2015.05.007
Bhardwaj, G., Cameotra, S. S., and Chopra, H. K. (2013). Biosurfactants from fungi: a review. Pet. Environ. Biotechnol. 4:160. doi: 10.4172/2157-7463.1000160
Bouassida, M., Fourati, N., Ghazala, I., Ellouze-Chaabouni, S., and Ghribi, D. (2018). Potential application of Bacillus subtilis SPB1 biosurfactants in laundry detergent formulations: compatibility study with detergent ingredients and washing performance. Eng. Life Sci. 18, 70–77. doi: 10.1002/elsc.201700152
Camargo, F., do Prado, P. F., Tonello, P. S., and Santos, A. C. A. (2018). Bioleaching of toxic metals from sewage sludge by co-inoculation of Acidithiobacillus and the biosurfactant producing yeast Meyerozyma guilliermondii. J. Environ. Manag. 211, 28–35. doi: 10.1016/j.jenvman.2018.01.045
Camargo-de-Morais, M., Ramos, S. A. F., Pimentel, M., de Morais, M., and Lima Filho, J. (2003). Production of an extra-cellular polysaccharide with emulsifier properties by Penicillium citrinum. World J. Microbiol. Biotechnol. 19, 191–194. doi: 10.1023/A:1023299111663
Cao, X., Wang, A., Jiao, R., Wang, C., Mao, D., Yang, L., et al. (2009). Evaluation of a lipopeptide biosurfactant from Bacillus natto TK-1 as a potential source of anti-adhesive, antimicrobial and antitumor activities. Brazil J. Microbiol. 4, 373–379. doi: 10.1590/S1517-83822009000200030
Chandran, P., and Das, N. (2010). Role of sophorolipid biosurfactant in degradation of diesel oil by Candida tropicalis. Int. J. Eng. Sci. Technol. 2, 6942–6953.
Christova, N., Tuleva, B., Kril, A., Georgieva, M., Konstantinov, S., and Terziyski, I. (2013). Chemical structure and in vitro antitumor activity of rhamnolipids from Pseudomonas aeruginosa BN10. Appl. Biochem. Biotechnol. 170, 676–689. doi: 10.1007/s12010-013-0225-z
Concaix, F. B. (2003). Use of Sophorolipids Comprising Diacetyl Lactones as Agent for Stimulating Skin Fibroblast Metabolism. US Patent 6.596.265. Washington, DC: U.S. Patent and Trademark Office.
Dailin, D. J., Elsayed, E. A., Malek, R. A., Hanapi, S. Z., Selvamani, S., Ramli, S., et al. (2020). Efficient kefiran production by Lactobacillus kefiranofaciens ATCC 43761 in submerged cultivation: influence of osmotic stress and nonionic surfactants, and potential bioactivities. Arab. J. Chem. 13, 8513–8523. doi: 10.1016/j.arabjc.2020.09.030
Daverey, A., and Pakshirajan, K. (2009). Production, characterization and properties of sophorolipids from the yeast Candida bombicola using a low-cost fermentative medium. Appl. Biochem. Biotechnol. 158, 663–674. doi: 10.1007/s12010-008-8449-z
De Rienzo, M. A. D., Kamalanathan, I. D., and Martin, P. J. (2016). Comparative study of the production of rhamnolipid biosurfactants by B. thailandensis E264 and P. aeruginosa ATCC 9027 using foam fractionation. Process Biochem. 51, 820–827. doi: 10.1016/j.procbio.2016.04.007
Desanto, K. (2012). Rhamnolipid-Based Formulations. US 8183198 B2. Washington, DC: U.S. Patent and Trademark Office.
Desanto, K. (2019). Preventing and Destroying Citrus Greening and Citrus Canker Using Rhamnolipid. US 2019/0029250.
DeSanto, K., and Keer, D. (2012). Use of Rhamnolipids in the Water Treatment Industry. PCT/US2012/032385.
Develter, D., Renkin, M., and Jacobs, I. (2003). Detergent Compositions- Glycolipids. US20040152613A1.
Diab, A., and Din, G. E. (2013). Applications of the biosurfactants produced by Bacillus spp. (SH 20 and SH 26) and Pseudomonas aeruginosa SH 29 isolated from the rhizosphere of an Egyptian salt march plant for the cleaning of oil-contaminated vessels and enhancing the biodegradation of. Afr. J. Enviro. Sci. Technol. 7, 671–679.
Dinamarca-Tapia, M. A., Ojeda-Herrera, J. R., and Cli, Q. (2012). Strain of Cobetia Marina and Biosurfactant Extract Obtained from Same. World Patent WO 2012164508 A1. Washington, DC: U.S. Patent and Trademark Office.
Dixit, S., Danekar, R., and Prasad, E. (2020). Surfactants market outlook – 2027. Allied Mark. Res. Available online at: https://www.alliedmarketresearch.com/surfactantmarket#:~:text=Surfactants%20Market%20Outlook%20%2D%202027,5.3%25%20from%202020%20to%202027
Dubeau, D., Deziel, E., Woods, D., and Lepine, F. (2009). Burkholderia thailandensis harbors two identical rhl gene clusters responsible for the biosynthesis of rhamnolipids. BMC Microbiol. 9:263. doi: 10.1186/1471-2180-9-263
Dubey, K. V., Juwarkar, A. A., and Singh, S. K. (2005). Adsorption-desorption process using woodbased activated carbon for recovery of biosurfactant from fermented distillery wastewater. Biotechnol. Process 21, 860–867. doi: 10.1021/bp040012e
Elazzazy, A. M., Abdelmoneim, T. S., and Almaghrabi, O. A. (2015). Isolation and characterization of biosurfactant production under extreme environmental conditions by alkali-halo-thermophilic bacteria from Saudi Arabia. Saudi J. Biol. Sci. 22, 466–475. doi: 10.1016/j.sjbs.2014.11.018
El-Sheshtawy, H. S., and Doheim, M. M. (2014). Selection of Pseudomonas aeruginosa for biosurfactant production and studies of its antimicrobial activity. Egypt. J. Pet. 23, 1–6. doi: 10.1016/j.ejpe.2014.02.001
Fei, D., Yu, G. Z. Z., and Liu, H. G. J. (2019). Low-toxic and nonirritant biosurfactant surfactin and its performances in detergent formulations. J. Surfactants Deterg. 23, 109–118. doi: 10.1002/jsde.12356
Franzetti, A., Caredda, P., Ruggeri, C., La-Colla, P., Tamburini, E., Papacchini, M., et al. (2009). Potential applications of surface active compounds by Gordonia spp. strain BS29 in soil remediation technologies. J. Chemosphere 75, 801–807. doi: 10.1016/j.chemosphere.2008.12.052
Fusconi, R., Assuncao, R. M. N., Guimaraes, R., Filho, G. R., and Machado, A. E. (2010). Exhopolysaccharide produced by Gordonia polyisoprenivorans CCT 7137 in GYM commercial medium and sugarcane molasses alternative medium: FT-IR study and emulsifying activity. Carbohydr. Polym. 79, 403–408. doi: 10.1016/j.carbpol.2009.08.023
Gandhi, N. R., and Skebba, V. L. P. (2011). Rhamnolipid Compositions and Related Methods of Use. US 7968499B2. Washington, DC: U.S. Patent and Trademark Office.
Gargouri, B., Contreras, M., Contreras, M. D., Ammar, S., Segura-Carretero, A., and Bouaziz, M. (2017). Biosurfactant production by the crude oil degrading Stenotrophomonas sp. B-2: chemical characterization, biological activities and environmental applications. Environ. Sci. Pollut. Res. 24, 3769–3779. doi: 10.1007/s11356-016-8064-4
Gautam, G., Mishra, V., Verma, P., Pandey, A. K., and Negi, S. (2014). A Cost effective strategy for production of bio-surfactant from locally isolated Penicillium chrysogenum SNP5 and its applications. J. Bioprocess. Biotech. 4:177. doi: 10.4172/2155-9821.1000177
Giessler-Blank, S., Schilling, M., Thum, O., and Sieverding, E. (2009). Use of Sophorolipids and Derivatives Thereof in Combination with Pesticides as Adjuvant/additive for Plant Protection and the Industrial Non-Crop Field. US9351485B2. Washington, DC: U.S. Patent and Trademark Office.
Gong, Z., Shen, H., Zhou, W., Wang, Y., Yang, X., and Zhao, Z. K. (2015). Efficient conversion of acetate into lipids by the oleaginous yeast Cryptococcus curvatus. Biotechnol. Biofuels 8:189. doi: 10.1186/s13068-015-0371-3
Gonzini, O., Plaza, A., Di Palma, L., and Lobo, M. C. (2010). Electrokinetic remediation of gasoil contaminated soil enhanced by rhamnolipid. J. Appl. Electrochem. 40, 1239–1248. doi: 10.1007/s10800-010-0095-9
Gross, R. A., and Shah, V. (2004). Antifungal Properties of Various Forms of Sophorolipids. WO2006069175A3.
Gross, R. A., Shah, V., and Doncel, G. (2004). Virucidal Properties and Various Forms of Sophorolipids. US 8648055B2. Washington, DC: U.S. Patent and Trademark Office.
Gudiña, E. J., Teixeira, J. A., and Rodrigues, L. R. (2016). Biosurfactants produced by marine microorganisms with therapeutic applications. Mar. Drugs 14:38. doi: 10.3390/md14020038
Gudiña, J., Rocha, V., Teixeira, A., and Rodrigues, R. (2010). Antimicrobial and antiadhesive properties of a biosurfactant isolated from Lactobacillus paracasei sspp. paracasei A20. Lett. Appl. Microbiol. 50, 419–424. doi: 10.1111/j.1472-765X.2010.02818.x
Hormann, B., Muller, M., Syldatk, C., and Hausmann, R. (2010). Rhamnolipid production by Burkholderia plantarii DSM 9509(T). Eur. J. Lipid Sci. Technol. 112, 674–680. doi: 10.1002/ejlt.201000030
Hultberg, M., Burgstrand, K.-J., Khalil, S., and Alsanius, B. (2008). Characterization of biosurfactant-producing strains of fluorescent pseudomonads in a soilless cultivation system. Antonie Van Leeuwenhoek 94, 329–334. doi: 10.1007/s10482-008-9250-2
Ibrahim, H. M. M. (2017). Characterization of biosurfactants produced by novel strains of Ochrobactrum anthropi HM-1 and Citrobacter freundii HM-2 from used engine oil-contaminated soil. Egypt. J. Pet. 27, 21–29. doi: 10.1016/j.ejpe.2016.12.005
Ishaq, U., Iqbal, Z., Rafiq, M., and Akrem, A. (2015). Production and characterization of novel self-assembling biosurfactants from Aspergillus flavus. J. Appl. Microbiol. 119, 1035–1045. doi: 10.1111/jam.12929
Jain, R. M., Mody, K., Mishra, A., and Jha, B. (2012). Physicochemical characterization of biosurfactant and its potential to remove oil from soil and cotton cloth. Carbohydr. Polym. 89, 1110–1116. doi: 10.1016/j.carbpol.2012.03.077
Jing, C., Xin, S., Hui, Z., and Yinbo, Q. (2007). Production, structure elucidation and anticancer properties of sophorolipid from Wickerhamiella domercqiae. Enzym. Microbiol. Technol. 39, 501–506. doi: 10.1016/j.enzmictec.2005.12.022
Joshi, P. A., and Shekhawat, D. B. (2014). Screening and isolation of biosurfactant producing bacteria from petroleum contaminated soil. Eur. J. Exp. Biol. 4, 164–169.
Joshi, S. J., Al-Wahaibi, Y., Al-Bahry, S. N., Elshafie, A. E., Al-Bemani, A. S., Al-Bahri, A., et al. (2016). Production, characterization and application of Bacillus licheniformis W16 biosurfactant in enhancing oil recovery. Front. Microbiol. 7:1853. doi: 10.3389/fmicb.2016.01853
Joshi-Navare, K., Singh, P., and Prabhune, A. (2014). New yeast isolate Pichia caribbica synthesizes xylolipid biosurfactant with enhanced functionality. Eur. J. Lipid Sci. Technol. 116, 1070–1079. doi: 10.1002/ejlt.201300363
Jourdier, E., and Chhabban, F. B. (2012). Process for the Production of Sophorose Starting from Sophorolipids. US20150336999A1.
Kakugawa, K., Tamai, M., Imamura, K., Miyamoto, K., Miyoshi, S., Morinaga, Y., et al. (2002). Isolation of yeast Kurtazmanomyces spp. I-11, novel producer of mannoasylerythritol lipid. Biosci. Biotechnol. Biochem. 66, 188–191. doi: 10.1271/bbb.66.188
Kamal, I., Blaghen, M., Lahlou, F. Z., and Hammoumi, A. (2015). Evaluation of biosurfactant production by Aeromonas salmonicida sp. degrading gasoline. Int. J. Appl. Microbiol. Biotechnol. Res. 3, 89–95. doi: 10.3126/ijasbt.v3i1.12200
Kanna, R., Gummadi, S. N., and Suresh Kumar, G. (2014). Production and characterization of biosurfactant by Pseudomonas putida MTCC 2467. J. Biol. Sci. 14, 436–445. doi: 10.3923/jbs.2014.436.445
Kim, P. Y., Ryu, J., Kim, Y. H., and Chi, Y. T. (2010). Production of biosurfactant lipopeptides iturin A, fengycin and surfactin A from Bacillus subtilis CMB32 for control of Colletotrichum gloeosporioides. J. Microbiol. Biotechnol. 20, 138–145. doi: 10.4014/jmb.0905.05007
Kiran, G. S., Thomas, T. A., and Selvin, J. (2010). Production of a new glycolipid biosurfactant from marine Nocardiopsis lucentensis MSA04 in solid-state cultivation. Coll. Surf. B Biointer. 78, 8–16. doi: 10.1016/j.colsurfb.2010.01.028
Kiran, G., Anto Thomas, T., Selvin, J., Sabarathnam, B., and Lipton, A. P. (2010). Optimization and characterization of a new lipopeptide biosurfactant produced by marine Brevibacterium aureum MSA13 in solid state culture. Bioresour. Technol. 101, 2389–2396. doi: 10.1016/j.biortech.2009.11.023
Kitamoto, D., Yanagishita, H., Endo, A., Nakaiwa, M., Nakane, T., and Akiya, T. (2001). Remarkable anti-agglomeration effect of yeast biosurfactant, diacylmannosylerythritol, on ice-water slurry for cold thermal storage. Biotechnol. Prog. 17, 362–365. doi: 10.1021/bp000159f
Kohli, R. M., Trauger, J. W., Schwarzer, D., Marahiel, M. A., and Walsh, C. T. (2001). Generality of peptide cyclization catalyzed by isolated thioesterase domains of non-ribosomal peptide synthetases. Biochemistry 40, 7099–7108. doi: 10.1021/bi010036j
Kubicki, S., Bollinger, A., Katzke, N., Jaeger, K., Loeschcke, A., and Thies, S. (2019). Marine biosurfactants: biosynthesis, structural diversity and biotechnological applications. Mar. Drugs 17:408. doi: 10.3390/md17070408
Kuyukina, M. S., Ivshina, I. B., Philip, J. C., Christofi, N., Dunbar, S. A., and Ritchkova, M. I. (2001). Recovery of Rhodococcus biosurfactants using methyl tertiary-butyl ether extraction. J. Microb. Methods 46, 149–156. doi: 10.1016/S0167-7012(01)00259-7
Leighs, S. J., McNeil, S. J., and Ranford, S. L. (2018). The application of biosurfactants for scouring wool. Color Technol. 1–5. doi: 10.1111/cote.12370
Leighton, A. (2013). Method for Treating Rhinitis and Sinusitis by Rhamnolipids. US 8592381, B2. Washington, DC: U.S. Patent and Trademark Office.
Liu, B., Liu, J., Ju, M., Li, X., and Yu, Q. (2016). Purification and characterization of biosurfactant produced by Bacillus licheniformis Y-1 and its application in remediation of petroleum contaminated soil. Mar. Pollut. Bull. 107, 46–51. doi: 10.1016/j.marpolbul.2016.04.025
López-prieto, A., Martínez-padrón, H., Rodríguez-lópez, L., Moldes, A. B., Cruz, J. M., Martínez-padrón, H., et al. (2019). Isolation and characterization of a microorganism that produces biosurfactants in corn steep water. CYTA J. Food 17, 509–516. doi: 10.1080/19476337.2019.1607909
Lu, J.-K., and Wang Hsin-Mei Xu, X.-R. (2016). Applications of Surfactin in Cosmetic Products. US2016/0030322A1.
Lukondeh, T., Ashbolt, N. J., and Rogers, P. L. (2003). Evolution of Klueveromyces maxianus FII 510700 grown on a lactose-based medium as a source of a natural bioemulsifier. J Ind. Microb. Biotechnol. 30, 715–720. doi: 10.1007/s10295-003-0105-6
Luna, J. M., Rufino, R. D., Sarubbo, L. A., Rodrigues, L. R. M., Teixeira, J. A. C., and Campos-Takaki, G. M. (2011). Evaluation antimicrobial and antiadhesive properties of the biosurfactant lunasan produced by Candida sphaerica UCP 0995. Curr. Microbiol. 62, 1527–34. doi: 10.1007/s00284-011-9889-1
Maneerat, S. (2005). Biosurfactants from marine microorganisms. Songklanakarin J. Sci. Technol. 27, 1264–1272. doi: 10.5772/intechopen.80493
Martins, V. G., Kalil, S. J., Bertolin, T. E., and Costa, J. A. V. (2006). Solid state biosurfactant production in a fixed-bed column bioreactor. Verlag Der Zeitschrift Für Naturforsch. 61, 721–726. doi: 10.1515/znc-2006-9-1019
Morita, T., Ishibashi, Y., Fukuoka, T., Imura, T., Sakai, H., Abe, M., et al. (2009). Production of glycolipid biosurfactants, mannosylerythritol lipids, by a smut fungus, Ustilago scitaminea NBRC 32730. Biosci. Biotechnol. Biochem. 73, 788–792. doi: 10.1271/bbb.80901
Morita, T., Ishibashi, Y., Hirose, N., Fukuoka, T., Imura, T., Sakai, H., et al. (2011). Production and characterization of a glycolipid biosurfactant, mannosylerythritol lipid B, from sugarcane juice by Ustilago scitaminea NBRC 32730. Biosci. Biotechnol. Biochem. 75, 1371–1376. doi: 10.1271/bbb.110221
Morita, T., Kitagawa, M., Yamamoto, S., Sogabe, A., Imaru, T., Fukuoka, T., et al. (2010). Glycolipids biosurfactants, mannoasylerythritol lipids, repair the damage hair. J. Oleo Sci. 59, 267–272.
Morita, T., Konishi, M., Fukuoka, T., Imura, T., and Kitamoto, D. (2008). Production of glycolipid biosurfactants, mannosylerythritol lipids, by Pseudozyma siamensis CBS 9960 and their interfacial properties. J. Biosci. Bioeng. 105, 493–502. doi: 10.1263/jbb.105.493
Morita, T., Konishi, M., Fukuoka, T., Imura, T., Kitamoto, H. K., and Kitamoto, D. (2006). Characterization of the genus Pseudozyma by the formation of glycolipid biosurfactants, mannosylerythritol lipids. FEMS Yeast Res. 7, 286–292. doi: 10.1111/j.1567-1364.2006.00154.x
Mukherjee, A. K. (2007). Potential application of cyclic lipopeptide biosurfactants produced by Bacillus subtilis strains in laundry detergent formulations. Lett. Appl. Microbiol. 45, 330–335. doi: 10.1111/j.1472-765X.2007.02197.x
Mukherjee, S., Das, P., and Sen, R. (2006). Towards commertial production of microbial surfactants. Trends Biotechnol. 24, 509–515. doi: 10.1016/j.tibtech.2006.09.005
Mulligan, C. N., and Eftekhari, F. (2003). Remediation with surfactant foam of PCP-contaminated soil. Eng. Geol. 70, 269–279. doi: 10.1016/S0013-7952(03)00095-4
Mulligan, C., and Wang, S. (2004). Rhamnolipid foam enhanced remediation of cadmium and nickel contaminated soil. J. Water Air Soil Pollut. 157, 315–330. doi: 10.1023/B:WATE.0000038904.91977.f0
Nalini, S., and Parthasarathi, R. (2014). Production and characterization of rhamnolipids produced by Serratia rubidaea SNAU02 under solid-state fermentation and its application as biocontrol agent. Bioresour. Technol. 173, 231–238. doi: 10.1016/j.biortech.2014.09.051
Nayak, A., Vijaykumar, M., and Karegoudar, T. (2009). Characterization of biosurfactant produced by Pseudoxanthomonas spp. PNK-04 and its application in bioremediation. Int. Biodeterior. Biodegrad. 63, 73–79. doi: 10.1016/j.ibiod.2008.07.003
Nitschke, M., Nitschke, M., and Pastore, G. M. (2006). Production and properties of a surfactant obtained from Bacillus subtilis grown on cassava wastewater. Bioresour. Technol. 97, 336–341. doi: 10.1016/j.biortech.2005.02.044
Noah, K. S., Fox, S. L., Bruhn, D. F., and Thompson, D. N. (2002). Development of continuous surfactin production from potato process effluent by Bacillus subtilis in an airlift reactor. Appl. Biochem. Biotechnol. 98–100, 803–813. doi: 10.1007/978-1-4612-0119-9_65
Nogueira, I. B., Rodrigues, D. M., Andradade, R. F., Lins, A. B., Bione, A. P., Silva, I. G. S., et al. (2020). Bioconversion of agroindustrial waste in the production of bioemulsifier by Stenotrophomonas maltophilia UCP 1601 and application in bioremediation process. Int. J. Chem. Eng. 2020, 1–9. doi: 10.1155/2020/9434059
Nwaguma, I. V., Chikere, C. B., and Okpokwasili, G. C. (2016). Isolation, characterization, and application of biosurfactant by Klebsiella pneumoniae strain IVN51 isolated from hydrocarbon- polluted soil in Ogoniland. Nigeria. Bioresour. Bioprocess 3:40. doi: 10.1186/s40643-016-0118-4
Obayori, O. S., Ilori, M. O., Adebusoye, S. A., Oyetibo, G. O., Omotayo, A. E., and Amund, O. O. (2009). Degradation of hydrocarbons and biosurfactant production by Pseudomonas spp. strain LP1. World J. Microbiol. Biotechnol. 25, 1615–1623. doi: 10.1007/s11274-009-0053-z
Onaizi, S. A., He, L., and Midellberg, A. P. J. (2009). Rapid screening of surfactant and biosurfactant Surface cleaning performance. Coll. Surf. B 72, 68–74. doi: 10.1016/j.colsurfb.2009.03.015
Ozdal, M., Gurkok, S., and Ozdal, O. G. (2017). Optimization of rhamnolipid production by Pseudomonas aeruginosa OG1 using waste frying oil and chicken feather peptone. 3 Biotech 7:117. doi: 10.1007/s13205-017-0774-x
Pardhi, D. S., Bhatt, R., Panchal, R. R., Raval, V. H., and Rajput, K. N. (2021b). “Rhamnolipid biosurfactants: Structure, biosynthesis, production and applications,” in Microb. Surfact: Production and Applications, eds R. Z. Sayyed, H. A. El-Enshasy, and B. Hameeda (Boca Raton: CRC Press). 1.
Pardhi, D. S., Panchal, R. R., Raval, V. H., and Rajput, K. N. (2021a). Statistical optimization of medium components for biosurfactant production by Pseudomonas guguanensis D30. Prep. Biochem. Biotechnol. 52, 171–180. doi: 10.1080/10826068.2021.1922919
Pardhi, D., Panchal, R., and Rajput, K. (2020). Screening of biosurfactant producing bactera and optimization of production conditions for Pseudomonas guguanensis D30. Biosci. Biotechnol. Res. Commun. 13, 170–179. doi: 10.21786/bbrc/13.1special
Parekh, V., and Pandit, A. (2012). Mango Kernel Fat: a novel lipid source for the fermentative production of sophorolipid biosurfactant using Starmerella bombicola NRRL-Y 17069. Ann. Biol. Res. 3, 1798–1803.
Patel, P., Bhatt, S., Patel, H., Marcelino, L. A., and Sayyed, R. Z. (2022). “Biosurfactant- A biomolecules and its potential applications,” in In the Biosurfatnats: Production and Applications in Food and Agriculture, eds R. Z. Sayyed and H. E. Enshasy (Milton Park: Taylor & Francis group), 133–149.
Pekin, G., Fazilet, V.-S., and Kosaric, N. (2005). Production of sophorolipids from Candida bombicola ATCC 22214 using Turkish corn oil and honey. Eng. Life Sci. 5, 357–362. doi: 10.1002/elsc.200520086
Pellecier, F., and André, P. (2004). Cosmetic use of Sophorolipids as Subcutaneous Adipose Cushion Regulation Agents and Slimming Application. World Patent 2004/108063.
Peng, F., Liu, Z., Wang, L., and Shao, Z. (2007). An oil-degrading bacterium: rhodococcus erythropolis strain 3C-9 and its biosurfactants. J. Appl. Microbiol. 102, 1603–1611. doi: 10.1111/j.1365-2672.2006.03267.x
Pereira, J. F. B., Gudiña, E. J., Costa, R., Vitorino, R., Teixeira, J. A., Coutinho, J. A. P., et al. (2013). Optimization and characterization of biosurfactant production by Bacillus subtilis isolates towards microbial enhanced oil recovery applications. Fuel 111, 259–268. doi: 10.1016/j.fuel.2013.04.040
Pervaiz, M., and Sain, M. (2010). Extraction and characterization of extra cellular polymeric substances (EPS) from waste sludge of pulp and papermill. Int. Rev. Chem. Eng. 2, 550–554.
Pi, G., Mao, L., Bao, M., Li, Y., Gong, H., and Zhang, J. (2015). Preparation of oil-in-seawater emulsions based on environmentally benign nanoparticles and biosurfactant for oil spill remediation. ACS Sustain. Chem. Eng. 3:150923133459004. doi: 10.1021/acssuschemeng.5b00516
Piljac, G. (2012). The use of Rhamnolipids as a Drug of Choice in the Case of Nuclear Disasters in the Treatment of the Combination Radiation Injuries and Illness in Humans and Animals. WO 2011/109200 A9.
Płaza, G. A., Łukasik, K., Wypych, J., Nałêcz-Jawecki, G., Berry, C., and Brigmon, R. L. (2008). Biodegradation of crude oil and distillation products by biosurfactant. Polish J. Environ. Stud. 17, 87–94.
Pradel, E., Zhang, Y., Pujol, N., Matsuyama, T., Bargmann, C., and Ewbank, J. (2007). Detection and avoidance of a natural product from the pathogenic bacterium Serratia marcescens by Caenorhabditis elegans. Natl. Acad. Sci. U.S.A. 104, 2295–2300. doi: 10.1073/pnas.0610281104
Qazi, M. A., Subhan, M., Fatima, N., Ali, M. I., and Ahmed, S. (2013). Role of biosurfactant produced by Fusarium sp. BS-8 in enhanced oil recovery (EOR) through sand pack column. Int. J. Biosci. Biochem. Bioinforma. 3, 598–604. doi: 10.7763/IJBBB.2013.V3.284
Rau, U., Nguyen, A. L., and Roeper, H. (2005). Downstream processing of mannosylerythrol lipids produced by Pseudozyma aphidis. Eur. J. Lipid Sci. Technol. 107, 373–380. doi: 10.1002/ejlt.200401122
Ravinder, R., Manasa, M., Roopa, D., Bukhari, N. A., Hatamleh, A. A., Khan, M. Y., et al. (2022). Biosurfactant producing multifarious Streptomyces puniceus RHPR9 of Coscinium fenestratum rhizosphere promotes plant growth in chilli. PLoS One 17:e0264975. journal.pone.0264975 doi: 10.1371/journal.pone.0264975
Raza, Z. A., Rehman, A., Hussain, M. T., Masood, R., Ul Haq, A., Saddique, M. T., et al. (2014). Production of rhamnolipid surfactant and its application in bioscouring of cotton fabric. Carbohydr. Res. 391, 97–105. doi: 10.1016/j.carres.2014.03.009
Rufino, R. D., Sarubbo, L. A., and Campos-Takaki, G. M. (2007). Enhancement of stability of biosurfactant produced by Candida lipolytica using industrial residue as substrate. World J. Microbiol. Biotechnol. 23, 729–734. doi: 10.1007/s11274-006-9278-2
Sadasivan, L. (2015). Aqueous Coatings and Paints Incorporating one or more Antimicrobial Biosurfactants and Methods for Using Same. US 8957129, B2. Washington, DC: U.S. Patent and Trademark Office.
Sadiq, M. B., Khan, M. R., and Sayyed, R. Z. (2022). “Biosurfactant mediated synthesis and stabilization of nanoparticles,” in In the Biosurfatnats: Production and Applications in Bioremediation/Reclaimation, ed. R. Z. Sayyed (Milton Park: Taylor & Francis group), 158–168. doi: 10.1201/9781003260165-8
Santhappan, R., and Pandian, M. R. (2017). Characterization of novel biosurfactants produced by the strain Fusarium oxysporum. J. Bioremed. Biodegrad. 8, 6–11. doi: 10.4172/2155-6199.1000416
Santos, E. C., Jacques, R. J., Bento, F. M., Peralba Mdo, C., Selbach, P. A., Sá, E. L., et al. (2008). Anthracene biodegradation and surface activity by an iron-stimulated Pseudomonas spp. Bioresour. Technol. 99, 2644–2649. doi: 10.1016/j.biortech.2007.04.050
Saranraj, P., Sivasakthivelan, P., Hamzah, K. J., Hasan, M. S., and Tawaha, A. R. M. A. (2022a). “Microbial fermentation technology for biosurfactants production,” in In the Biosurfatnats: Production and Applications in Food and Agriculture, eds R. Z. Sayyed and H. E. Enshasy (Milton Park: Taylor & Francis group), 63–81.
Saranraj, P., Sayyed, R. Z., Sivasakthivelan, P., Hasan, M. S., Al-Tawaha, A. R. M. A., and Amala, K. (2022b). “Microbial biosurfactants: methods of investigation, characterization, current market value and applications,” in In the Biosurfatnats: Production and Applications in Bioremediation/Reclaimation, ed. R. Z. Sayyed (Milton Park: Taylor & Francis group), 19–34. doi: 10.1201/9781003260165-2
Saranraj, P., Sayyed, R. Z., Hamzah, K. J., Asokan, N., Sivasakthivelan, P., and Al-Tawaha, A. R. M. (2022c). “Efficient substrates for microbial synthesis of biosurfactants,” in Biosurfatnats: Production and Applications in Bioremediation/Reclaimation, ed. R. Z. Sayyed (Milton Park: Taylor & Francis Group), 1–18. doi: 10.1201/9781003260165-1
Schloesser, T., Jakupovic, S., Katzer, W., Kluge, G., and Siems, K. (2011). Beverages Containing Glycolipid Preservatives. WO2013037818A3.
Schneider, T., Gries, K., Josten, M., Wiedemann, I., Pelzer, S., Labischinski, H., et al. (2009). The lipopeptide antibiotic Friulimicin B inhibits cell wall biosynthesis through complex formation with bactoprenol phosphate. Antimicrob. Agents Chemother. 53, 1610–1618. doi: 10.1128/AAC.01040-08
Sen, R., and Swaminathan, T. (2005). Characterization of concentration and purification parameters and operating conditions for the small-scale recovery of surfactin. Process Biochem. 40, 2953–2958. doi: 10.1016/j.procbio.2005.01.014
Shah, I., Hamid, B., Zaman, M., Fatima, S., Farooq, S., Datta, R., et al. (2022). “Microbial biosurfactants: an eco-friendly approach for bioremediation of contaminated environments,” in In the Biosurfatnats: Production and applications in Bioremediation/Reclaimation, ed. R. Z. Sayyed (Milton Park: Taylor & Francis group), 197–207. doi: 10.1201/9781003260165-11
Shah, N., Nikam, R., Gaikwad, S., Sapre, V., and Kaur, J. (2016). Biosurfactant: types, detection methods, importance and applications. Indian J. Microbiol. Res. 3:5. doi: 10.5958/2394-5478.2016.00002.9
Shah, V., Turjevic, M., and Badia, D. (2007). Utilization of restaurant waste oil as a precursor for sophorolipid production. Biotechnol. Prog. 23, 512–515. doi: 10.1021/bp0602909
Shaligram, N. S., and Singhal, R. S. (2010). Surfactin- A review on biosynthesis, fermentation, purification and applications. Food Technol. Biotechnol. 48, 119–134.
Shibahara, M., Zhao, X., and Wakamatsu, Y. (2000). Mannosylerythritol lipid increases levels of galactoceramide in and neurite out growth from PC12 pheochromocytoma cells. J. Cytotechnol. 33, 247–251.
Silva, N. R. A., Luna, M. A., Santiago, A. L., Franco, L. O., Silva, G. K., De Souza, P. M., et al. (2014). Biosurfactant-and bioemulsifier produced by a promising Cunninghamella echinulata isolated from caatinga soil in the northeast of Brazil. Int. J. Mol. Sci. 15, 15377–15395. doi: 10.3390/ijms150915377
Slivinski, C. T., Mallmann, E., Araujo, J. M., Mitchell, D. A., and Krieger, N. (2012). Production of surfactin by Bacillus pumilus UFPEDA 448 in solid-state fermentation using a medium based on okara with sugarcane bagasse as a bulking agent. Process Biochem. 47, 1848–1855. doi: 10.1016/j.procbio.2012.06.014
Souza, K. S. T., Gudina, E. J., Azevedo, Z., de Freitas, V., Schwan, R. F., Rodrigues, L. R., et al. (2017). New glycolipid biosurfactants produced by the yeast strain Wickerhamomyces anomalus CCMA 0358. Coll. Surf. B Biointer. 154, 373–382. doi: 10.1016/j.colsurfb.2017.03.041
Sridhar, B., Karthik, R., Pushpam, A. C., Vanitha, M. C., Nadu, T., Nadu, T., et al. (2015). Production and purification of biosurfactants from Saccharomyces cerevisiae and Pseudomonas aeruginosa and its application on fruit salads. Int. J. Adv. Res. Eng. Technol. 6, 97–104.
Stanburry, P., Whitaker, A., and Hall, S. (2016). Principles of Fermentation Technology, 2nd Edn. Amsterdam: Elsevier Science.
Tanovic, A., Samel, S. A., Essen, L.-O., and Marahiel, M. A. (2008). Crystal structure of the termination module of a nonribosomal peptide synthetase. Science 80, 659–663. doi: 10.1126/science.1159850
Thaniyavarn, J., Chianguthai, T., Sangvanich, P., Roongsawang, N., Washio, K., Morikawa, M., et al. (2008). Production of sophorolipid biosurfactant by Pichia anomala. Biosci. Biotechnol. Biochem. 72, 2061–2068. doi: 10.1271/bbb.80166
Thanomsub, B., Pumeechockchai, W., Limtrakul, A., Arunrattiyakorn, P., Petchleelaha, W., Nitoda, T., et al. (2007). Chemical structures and biological activities of rhamnolipids produced by Pseudomonas aeruginosa B189 isolated from milk factory waste. Bioresour. Technol. 98, 1149–1153. doi: 10.1016/j.biortech.2005.10.045
Toren, A., Segal, G., Ron, E., and Rosenberg, E. (2002). Structure-function studies of the recombinant protein bioemulsifier AlnA. Environ. Microbiol. 4, 257–261. doi: 10.1046/j.1462-2920.2002.00298.x
Tuleva, B., Christova, N., Cohen, R., Antonova, D., Todorov, T., and Stoineva, I. (2009). Isolation and characterization of trehalose tetraester biosurfactants from a soil strain Micrococcus luteus BN56. Process Biochem. 44, 135–141. doi: 10.1016/j.procbio.2008.09.016
Turbekar, R., Malik, N., Dey, D., and Thakare, D. (2014). Development of rhamnolipid based white board cleaner. Int. J. Appl. Sci. Biotechnol. 2, 570–573. doi: 10.3126/ijasbt.v2i4.11589
Vance-Harrop, M. H., Norma, B. G., and Campos-Takaki, G. M. (2003). New bioemulsifier produced by Candida lipolytica using D-glucose and Babassu oil as carbon sources. Braz. J. Microbiol. 34, 120–123. doi: 10.1590/S1517-83822003000200006
Varjani, S. J., and Upasani, V. N. (2017). Critical review on biosurfactant analysis, purification and characterization using rhamnolipid as a model biosurfactant. Bioresour. Technol. 232, 389–397. doi: 10.1016/j.biortech.2017.02.047
Velioğlu, Z., and Öztürk Ürek, R. (2015). Biosurfactant production by Pleurotus ostreatus in submerged and solid-state fermentation systems. Turk. J. Biol. 39, 160–166. doi: 10.3906/biy-1406-44
Vyas, T. K., and Dave, B. P. (2011). Production of biosurfactant by Nocardia otitidiscaviarum and its role in biodegradation of crude oil. Int. J. Environ. Sci. Tech. 8, 425–432. doi: 10.1007/BF03326229
Wadekar, S. D., Kale, S. B., Lali, A. M., Bhoumick, D. N., and Pratap, A. P. (2012). Microbial synthesis of rhamnolipids by Pseudomonas aeruginosa (ATCC 10145) on waste frying oil as low cost carbon source. Prep. Biochem. Biotechnol. 42, 249–266. doi: 10.1080/10826068.2011.603000
Wang, Q., Chen, S., Zhang, J., Sun, M., Liu, Z., and Yu, Z. (2008). Co-producing lipopeptides and poly-gamma-glutamic acid by solid state fermentation of Bacillus subtilis using soybean and sweet potato residues and its biocontrol and fertilizer synergistic effects. Bioresour. Technol. 99, 3318–3323. doi: 10.1016/j.biortech.2007.05.052
Whang, L., Liu, P., Ma, C., and Cheng, S. (2008). Application of biosurfactants, rhamnolipid and surfactin, for enhanced biodegradation of diesel-contaminated water and soil. J. Hazard. Mater. 151, 155–163. doi: 10.1016/j.jhazmat.2007.05.063
Wittgens, A., Kovacic, F., Müller, M. M., Gerlitzki, M., Santiago-Schübel, B., Hofmann, D., et al. (2017). Novel insights into biosynthesis and uptake of rhamnolipids and their precursors. Appl. Microbiol. Biotechnol. 101, 2865–2878. doi: 10.1007/s00253-016-8041-3
Yin, X., Maiqian, N., and Qirong, S. (2011). Rhamnolipid Biosurfactant from Pseudomonas Aeruginosa Strain NY3 and Methods of use. US 20110306569A1.
Zaman, M., Hassan, S., Fatima, S., Hamid, B., Farooq, S., Qayoom, I., et al. (2022). “Biosurfactants production and applications in food,” in In the Biosurfatnats: Production and Applications in Food and Agriculture, eds R. Z. Sayyed and H. E. Enshasy (Milton Park: Taylor & Francis group), 225–241.
Zaragoza, A., Aranda, F., Espuny, M., Teruel, J., Marques, A., and Manresa, A. (2010). Hemolytic activity of a bacterial trehalose lipid biosurfactant produced by Rhodococcus spp: evidence for a colloid-osmotic mechanism. Langmuir 26, 8567–8572. doi: 10.1021/la904637k
Zaragoza, A., Teruel, J., Aranda, F., and Ortiz, A. (2013). Interaction of a trehalose lipid biosurfactant produced by Rhodococcus erythropolis 51T7 with a secretory phospholipase A2. J. Coll. Inter. Sci. 15, 132–137. doi: 10.1016/j.jcis.2013.06.073
Keywords: biodegradable, emulsification, Pseudomonas spp., rhamnolipid, surface tension, surfactin
Citation: Pardhi DS, Panchal RR, Raval VH, Joshi RG, Poczai P, Almalki WH and Rajput KN (2022) Microbial surfactants: A journey from fundamentals to recent advances. Front. Microbiol. 13:982603. doi: 10.3389/fmicb.2022.982603
Received: 30 June 2022; Accepted: 11 July 2022;
Published: 04 August 2022.
Edited by:
Hameeda Bee, Osmania University, IndiaReviewed by:
Muhammad Bilal Sadiq, Forman Christian College, PakistanDibyajit Lahiri, University of Engineering and Management, India
Copyright © 2022 Pardhi, Panchal, Raval, Joshi, Poczai, Almalki and Rajput. This is an open-access article distributed under the terms of the Creative Commons Attribution License (CC BY). The use, distribution or reproduction in other forums is permitted, provided the original author(s) and the copyright owner(s) are credited and that the original publication in this journal is cited, in accordance with accepted academic practice. No use, distribution or reproduction is permitted which does not comply with these terms.
*Correspondence: Peter Poczai, peter.poczai@helsinki.fi; Kiransinh N. Rajput, rajputkn@gujaratuniversity.ac.in