- 1College of Life Science and Agricultural Engineering, Nanyang Normal University, Nanyang, China
- 2Key Laboratory of Agricultural Environmental Microbiology, Ministry of Agriculture, College of Life Sciences, Nanjing Agricultural University, Nanjing, China
- 3Jiangsu Academy of Agricultural Sciences, Institute of Germplasm Resources and Biotechnology, Nanjing, China
- 4The Environmental Monitoring Center of Gansu Province, Lanzhou, China
- 5College of Rural Revitalization, Jiangsu Open University, Nanjing, China
Dicamba, an important hormone-type systemic herbicide, is widely used to control more than 200 kinds of broadleaf weeds in agriculture. Due to its broad-spectrum, high efficiency and effectively killing glyphosate-resistant weeds, dicamba is considered as an excellent target herbicide for the engineering of herbicide-resistant crops. In this study, an efficient dicamba-degrading microbial consortium was enriched from soil collected from the outfall of a pesticide factory. The enriched consortium could almost completely degrade 500 mg/L of dicamba within 12 h of incubation. A novel tetrahydrofolate (THF)-dependent dicamba demethylase gene, named dmt06, was cloned from the total DNA of the enriched consortium. Dmt06 shared the highest identity (72.3%) with dicamba demethylase Dmt50 from Rhizorhabdus dicambivorans Ndbn-20. Dmt06 was expressed in Escherichia coli BL21 and purified to homogeneity using Co2+-charged nitrilotriacetic acid affinity chromatography. The purified Dmt06 catalyzed the transfer of methyl from dicamba to THF, generating the herbicidally inactive metabolite 3,6-dichlorosalicylate (3,6-DCSA) and 5-methyl-THF. The optimum pH and temperature for Dmt06 were detected to be 7.4 and 35°C, respectively. Under the optimal condition, the specific activity of Dmt06 reached 165 nmol/min/mg toward dicamba, which was much higher than that of Dmt and Dmt50. In conclusion, this study cloned a novel gene, dmt06, encoding an efficient THF-dependent dicamba demethylase, which was a good candidate for dicamba-resistant transgenic engineering.
Introduction
Dicamba (3,6-dichloro-2-methoxybenzoate) is an important hormone-type systemic herbicide. It has been widely used to control more than 200 kinds of broadleaf weeds in the farmland of gramineous crops such as wheat, corn and rice (Stevens and Sumner, 1991; Huang et al., 2017). At present, the global annual usage of dicamba has reached 30,000 tons. Furthermore, the biotechnology giant Monsanto company has successfully developed transgenic soybean and cotton that were highly resistant to dicamba using the dicamba demethylase gene DMO (Behrens et al., 2007). These dicamba-resistant crops were approved by the US department of agriculture in 2015 and have been commercially planted since 2017 (Li et al., 2018). By 2019, the planting area of dicamba-resistant crops reached 50 million acres, accounting for 50% of the total planting area of soybeans in the United States.1 Therefore, it has important theoretical and application value to explore dicamba-degrading and detoxifying strain and gene resources (Barrows et al., 2014).
Microbial metabolism was the main factor in the dissipation of dicamba in the soil (Krueger et al., 1989; Taraban et al., 1993; Werwath et al., 1998). So far, many dicamba-degrading strains, such as Stenotrophomonas maltophilia DI-6, Pseudomonas sp. DI-8 (Krueger et al., 1989; Wang et al., 1997), Sphingobium sp. Ndbn-10, Rhizorhabdus dicambivorans (formerly Sphingomonas sp.) Ndbn-20, have been isolated (Yao et al., 2015). The initial degradation step of dicamba was demethylation in all of these isolates, generating the herbicidally inactive metabolite 3,6-dichlorosalicylate (3,6-DCSA; Krueger et al., 1989; Behrens et al., 2007; Yao et al., 2015). Two main types of dicamba demethylases have been identified: (1) monooxygenase type demethylase: Herman et al. (2005) identified a dicamba monooxygenase DMO from S. maltophilia DI-6, DMO was a three-component monooxygenase using NADH as the electron donor. (2) tetrahydrofolate (THF)-dependent methyltransferase: Yao et al. (2016) and Chen et al. (2019) identified two dicamba demethylases Dmt and Dmt50 from R. dicambivorans Ndbn-20. Both Dmt and Dmt50 were THF-dependent methyltransferases. They catalyzed the methyl transfer from dicamba to THF, generating 3,6-DCSA and 5-methyl-THF. The activities of Dmt and Dmt50 were severely inhibited by the product 5-methyl-THF, resulting in significantly lower demethylation effect than DMO, which limited their application values.
In this study, a highly efficient dicamba-degrading consortium was enriched from the soil collected from the outlet of a pesticide plant. The enriched consortium was able to almost completely degrade 500 mg/L of dicamba within 12 h of incubation. A THF-dependent dicamba demethylase gene, named dmt06, was cloned from the total DNA of the consortium. Dmt06 was heterogeneously expressed in Escherichia coli and purified by affinity chromatography. Furthermore, the enzymatic characteristics of Dmt06 was also investigated. Our results indicated that Dmt06 showed higher demethylation activity than previously reported THF-dependent demethylases Dmt and Dmt50 (Yao et al., 2016; Chen et al., 2019), indicating that the Dmt06 has good potential application in the construction of dicamba-resistant crops.
Materials and methods
Chemicals and media
Dicamba, 3,6-DCSA, NADH and THF were analytically pure. Methanol, acetonitrile, and acetic acid were chromatographically pure. All the chemicals were purchased from Sigma-Aldrich Company (Shanghai). Luria-Bertani (LB) broth and LB agar were obtained from Difco Laboratories (Detroit, MI). The minimal salt medium (MSM) consisted of the following components: 1.3 g K2HPO4, 0.86 g KH2PO4, 0.66 g (NH4)2SO4, 0.097 g MgSO4, 0.025 g MnSO4·H2O, 0.005 g FeSO4·7H2O, 0.0013 g CaSO4·6H2O per liter water, pH 7.0. For solid media, 15 g per liter of agar powder was added. All media were sterilized by autoclaving at 121°C for 20 min.
Bacterial strains, plasmids, and primers
The E. coli strains and plasmids used in this study are listed in Table 1, and the primers used in this study are listed in Table 2. All E. coli strains were aerobically cultured in LB broth or agar at 37°C. Antibiotics were added at the following concentrations: kanamycin, 50 mg/L; ampicillin, 100 mg/L.
Enrichment of dicamba-degrading consortium
Soil sample used in this study was collected from the outfall of a pesticide factory. 10 g of the soil sample was transferred into a 250 ml Erlenmeyer flask containing 90 ml MSM supplemented with 500 mg/L dicamba. The Erlenmeyer flask was incubated at 30°C and 180 rpm on a shaker. At certain intervals, the remained dicamba concentration in the microbial consortium was determined using high-performance liquid chromatography (HPLC) as described below. When ~70% of the added dicamba was degraded, 10 ml of the enrichment culture was transferred into 90 ml fresh medium. The transfer was repeated for 5–6 times until the enriched consortium acquired high dicamba-degrading ability.
Determination of the dicamba demethylase activity in the cell extract of the enriched consortium
To investigate which type of dicamba demethylase was in the enriched consortium. The bacterial cells of the final transferred consortium were collected by centrifugation at 6000 rpm for 10 min. After wishing twice with MSM, the cells were resuspended with ice-cold phosphate-buffered saline (PBS) buffer (50 mM, pH 7.4), and then disrupted by pulse sonication on ice with 15 s burst and 10 s pause for 15 min (Auto Science, UH-650B ultrasonic processor, 40% intensity), and the cell lysate was centrifuged at 12,000 rpm for 10 min at 4°C. The supernatant was collected as the cell extract. The protein concentration of the cell extract was quantified by the bicinchoninic acid (BCA) method using bovine serum albumin as the standard (BCA Protein Assay Kit, Sangon Biotech Shanghai Co., Ltd.). The demethylase activity of the cell extract toward dicamba was determined in a 300 μl mixture containing 100 mM PBS buffer, 2.0 mM NADH or 2.0 mM THF, 0.5 mM substrate, and 50 μl crude enzyme. The mixture was incubated for 5 min at 30°C, then the reaction was terminated by boiling at 100°C for 1.0 min. The conversion of substrate was analyzed by HPLC. One unit of dicamba demethylase activity was defined as the amount of enzyme that catalyzed the conversion of 1.0 nmol of dicamba per min.
Cloning of the dicamba demethylase gene from the enriched consortium
The total DNA of the enriched consortium was extracted by Sodium dodecyl sulfate (SDS) high-salt method (Sambrook and Russell, 2001). To clone the dicamba demethylase gene from the total DNA of the enriched consortium, four degenerate primers including two forward primers and two reverse primers (Table 2) were designed according the conserved region of the reported THF-dependent demethylase gene sequences. These primers were paired for PCR amplification using the total DNA of the enrichment as a template. Amplification conditions: 94°C 3 min; 94°C 30 s, 55°C 30 s, 72°C 2 min, 33 cycles; 72°C 10 min. The acquired fragment was ligated into T-vector and transformed into E. coli DH5α. Sequencing of the fragment in the T-vector was performed using the ABI 3730xl DNA sequencer (Applied Biosystems). Then, the upstream sequence and downstream sequence of the acquired sequence were amplified by SEFA-PCR method, a DNA walking technology developed in our Lab (Wang et al., 2007), using the primers listed in Table 2.
Expression of the dicamba demethylase gene and purification of the product
The dicamba demethylase gene was amplified by PCR using 2 × Phanta Master Mix (Vazyme Biotech Co., Ltd) using the primers listed in Table 2 and the total DNA extracted from the enrichment as the template. The PCR products were inserted into the NdeI-HindIII site of pET29(+) to generate the recombinant plasmid pET-dmt06 using a one-step cloning kit (Vazyme Biotech). Then, pET-dmt06 was transformed into E. coli BL21 (DE3) for expression. Escherichia coli BL21 (DE3) cells harboring pET-dmt06 was grown in 100 ml of LB broth supplemented with 50 mg/L kanamycin at 37°C. When the absorbance at 600 nm reached 1.0, the cultures were induced with 0.4 mM isopropyl-β-D-thiogalactopyranoside (IPTG) for 10 h at 16°C. Then, cells were harvested by centrifugation at 12,000 rpm for 5 min. After washed twice with 100 mM PBS buffer (pH 7.4), the cells were resuspended in ice-cold PBS buffer, and then disrupted by sonication as described above, and the undisrupted cells and cell debris was removed by centrifugation at 12,000 rpm for 10 min at 4°C. The supernatant was charged onto 1-ml His-bind resin columns (HiTrap Talon crude; GE Healthcare Life Sciences), which had been activated by Co2+ and equilibrated with binding buffer. Following washing with binding/washing buffer containing different concentration of imidazole, the target protein was eluted with 5 ml of elution buffer [50 mM NaH2PO4, 300 mM NaCl, 100 mM imidazole (pH 8.0)]. The resultant fractions were dialyzed overnight at 4°C to remove imidazole in PBS buffer (100 mM, pH 7.4). The purities and molecular weights of the expressed protein was determined by 12% SDS-polyacrylamide gel electrophoresis (PAGE). The protein concentration of the cell extract was quantified by the BCA method. The dicamba demethylase activity was determined as described above. The metabolite was identified by mass spectrometry (MS) as described below.
Study on the enzymatic characteristics
The temperature range was investigated at 50 mM PBS buffer (pH 7.4) under different temperatures (4°C–70°C), and the relative activity was calculated by assuming that the activity at 30°C was 100%. The pH range was investigated at pH values from 3.6 to 10.6 in three different buffering systems: 20 mM HAc-NaAc buffer (pH 3.6–5.8), 50 mM PBS buffer (pH 5.5–8.5), and 20 mM glycine-NaOH buffer (pH 8.6–10.6). The activity observed at pH 7.4 in PBS buffer was set as 100%, each value was the average from three independent experiments. For pH stability investigation, the enzyme was preincubated in buffers with different pH (pH 3.6–10.6) at 30°C for 4 h. Then, the remaining activity was assayed under the optimal condition. For thermostability investigation, the enzyme was preincubated in a water bath at different temperatures (30°C–70°C) for 120 min, and then the residual activity was assayed. To investigate the effects of potential inhibitors on demethylase activity, the enzyme mixture was preincubated for 30 min at 35°C, then the chemical agents (EDTA and SDS, final concentration of 5.0 mM) and metal ions (Li+, Na+, Mg2+, K+, Hg2+, Mn2+, Ni2+, Co2+, Zn2+, Cu2+, Ba2+, Al3+, Cd2+, Ag+, Fe2+, Fe3+, final concentration of 1.0 mM) were individually added, and the reactions were performed at 35°C for 30 min. Dicamba demethylase activity was assayed as described above and expressed as a percentage of the activity obtained without addition of the above compound.
Chemical analysis
The collected samples were freeze-dried and dissolved in 1.0 ml of methanol. The solution was filtered through a 0.22 μm-pore-size Millipore membrane to remove particles. The concentrations of dicamba and metabolite were analyzed on an UltiMate 3,000 titanium system (Thermo Fisher Scientific) equipped with a C18 reversed-phase column (internal diameter, 4.6 mm; length, 250 mm; Agilent Technologies). The mobile phase was a mixture of ultrapure water (58.4%), acetonitrile (31.7%), methanol (7.5%), and 2.4% acetic acid (Yao et al., 2015). The flow rate was 1.0 ml min−1. A VWD-3100 single-wavelength detector was used to detect the UV absorption, the wavelengths for dicamba and 3,6-DCSA were 275 nm and 319 nm, respectively. The MS analysis was performed according to the method described by Liu et al. (2020).
Accession Number. The gene dmt06 sequence is deposited in the GenBank database under accession number ON828423.
Results
Enrichment of an efficient dicamba-degrading microbial consortium
In this study, we used dicamba as a sole source of carbon to enrich dicamba-degrading microbial consortium. It took 11 days to degrade ~70% of the added 500 mg/L of dicamba for the first round of consortium. During the acclimation, the degradation ability of the consortium became stronger and stronger. After six rounds of transfer, the enriched consortium could almost completely degrade the added 500 mg/L of dicamba within 12 h incubation (Figure 1). HPLC analysis indicated that an intermediate metabolite was accumulated during the degradation, the retention time of the metabolite was equal to that of the 3,6-DCSA standard, and this metabolite disappeared with prolonged incubation (Figure 2). The results indicated that the enriched microbial consortium could efficiently degrade dicamba, and the first degradation step was demethylation to generate 3,6-DCSA, which could be further degraded by the enriched consortium.
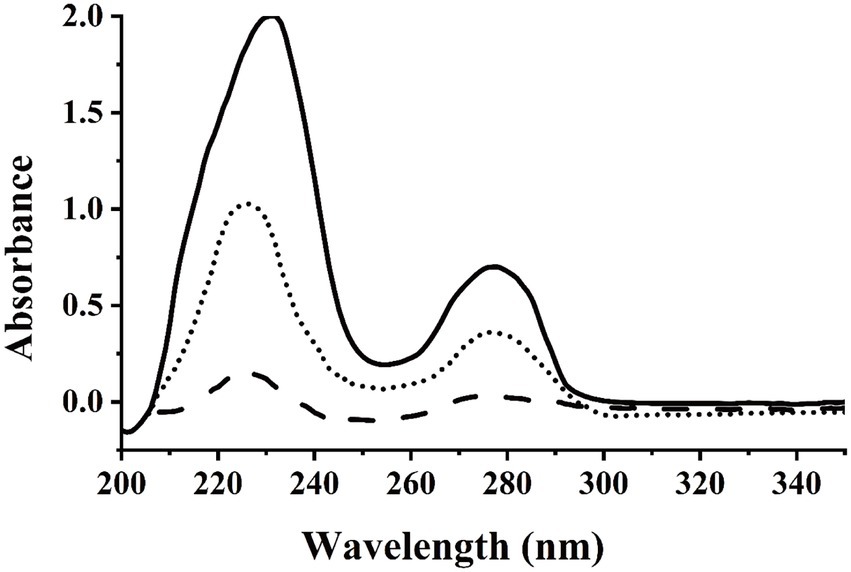
Figure 1. UV scanning detection of dicamba degradation by the enriched consortium. The sixth generation of the enriched consortium was inoculated into MSM supplemented with 500 mg/L of dicamba, which then was incubated in at 30°C and 180 rpm on a shaker. Solid line indicated the sample collected at 0 h, dotted line indicated the sample collected at 6 h, dashed line indicated the sample collected at 12 h.
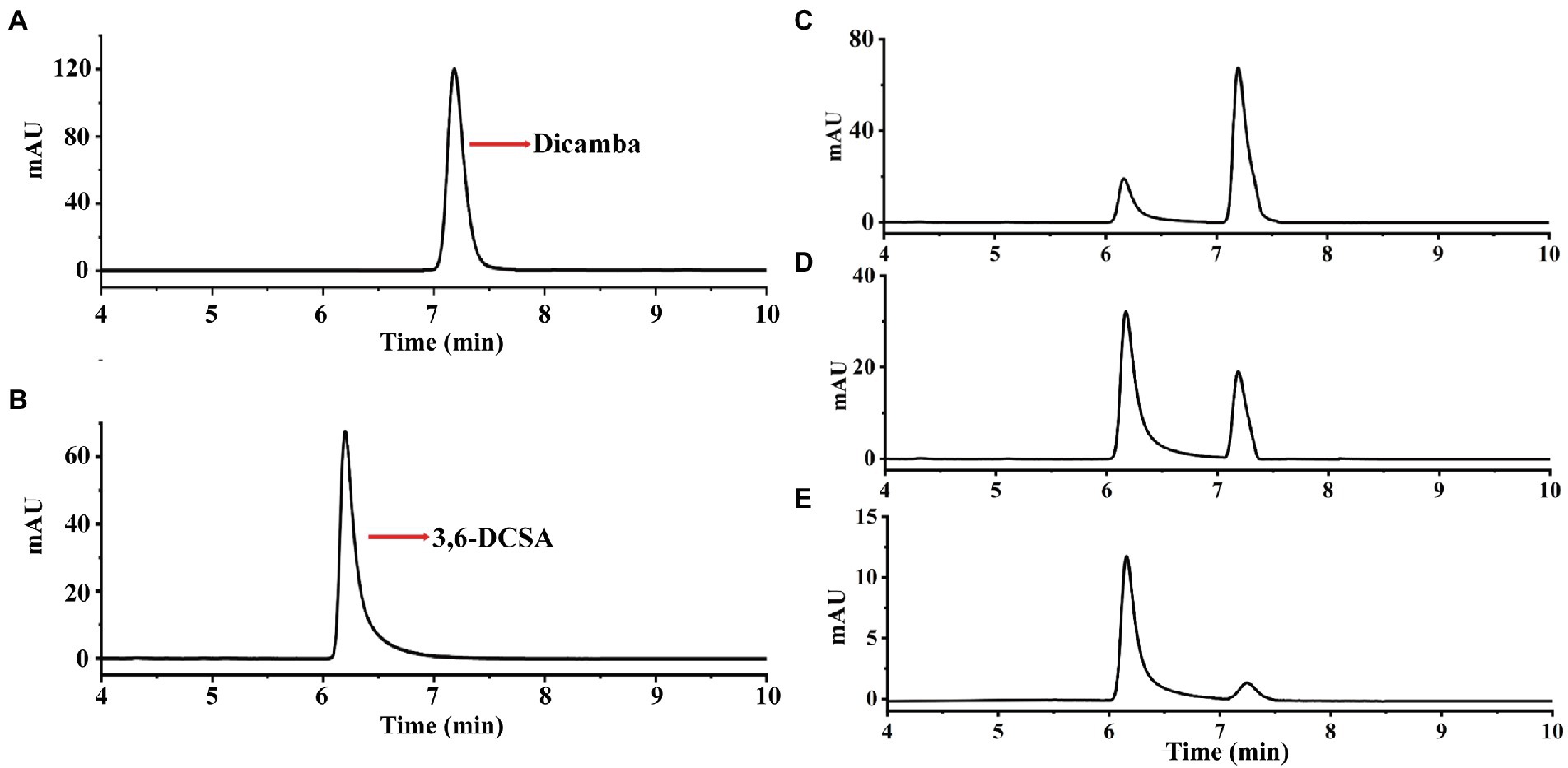
Figure 2. HPLC analysis of dicamba degradation by the enriched consortium. (A) The dicamba standard. (B) The 3,6-DCSA standard. (C) Sample collected at 4 h. (D) Sample collected at 8 h. (E) Sample collected at 12 h.
Degradation of dicamba by the crude enzyme of the enriched consortium
To date, two types of demethylases catalyzing the demethylation of methyl group-containing aromatics have been reported, one was the NADH-dependent monooxygenase, and another was the THF-dependent methyltransferase. In order to determine which type of dicamba demethylase was in the enrichment culture, the cell extract of the enriched consortium was obtained by ultrasonic disruption, and the dicamba demethylase activity in the cell extract was assayed in the presence of NADH and THF, respectively. The results showed that the cell extract added with THF acquired the dicamba demethylase activity, while the cell extract added with NADH could not convert dicamba (Table 3). The results suggested that the dicamba-degrading bacteria in the enriched consortium employed a THF-dependent demethylase to convert dicamba to 3,6-DCSA.
Cloning of the THF-dependent demethylase gene from the enriched consortium
To date, four THF-dependent demethylase genes have been reported: syringate demethylase DesA (Masai et al., 2004) and vanillate demethylase LigM (Abe et al., 2005) from Sphingomonas paucimobilis SYK-6, and dicamba demethylase Dmt (Yao et al., 2016) and Dmt50 (Chen et al., 2019) from R. dicambivorans Ndbn-20. To clone the dicamba demethylase gene, four degenerate primers (Table 2) including two forward primers (dF1 and dF2) and two reverse primers (dR1 and dR2) were designed according the conserved regions of the four THF-dependent demethylase genes, and then the primers were paired to amplify the dicamba demethylase gene from the total DNA of the enriched consortium. The results indicated that one pair of primers (dF1 and dR1) successfully amplified a fragment with clear band on gel electrophoresis (Figure 3A), the size of the acquired fragment was ~600 bp, which was consistent with the theoretical value. Sequencing results showed that the sequence of this fragment was homologous with 191–818 bp of dmt50 with a similarity of 74.3%. Then, to clone the whole gene, primers of SEFA-PCR, were designed in the upstream and downstream of the acquired fragment. After two steps of SEFA-PCR amplification, clear bands with length of ~1–1.5 kb were obtained at both upstream and downstream of the acquired fragment (Figure 3B).
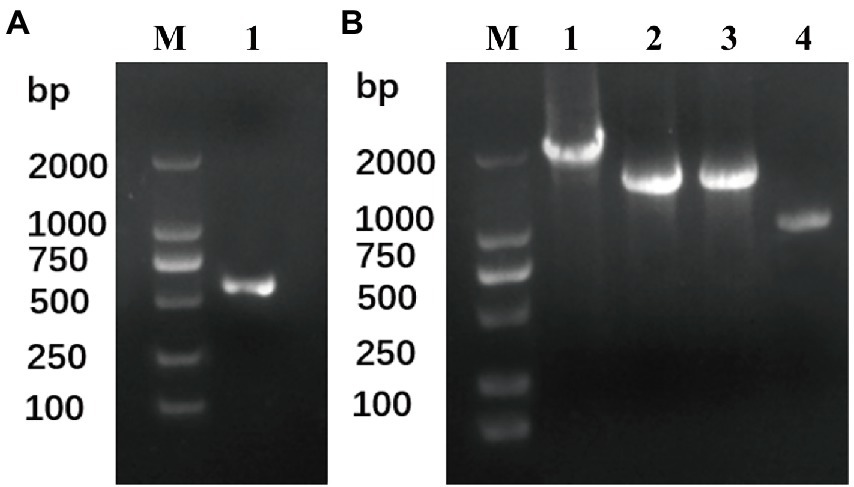
Figure 3. Amplification of the THF-dependent demethylase gene from the total DNA of the enriched consortium. (A) Fragment amplification from the total DNA of the enriched consortium using degenerate primers, Line M: DNA marker, line 1 amplified product using primer pair dF1 and dR1. (B) Amplified products from the upstream and downstream of the acquired fragment by SEFA-PCR, Line M: DNA marker, line 1: the first round SEFA-PCR from the fragment upstream, line 2: the first round SEFA-PCR from the fragment downstream, line 3: the second round SEFA-PCR from the fragment upstream, line 4: the second round SEFA-PCR from the fragment downstream.
The acquired SEFA-PCR products were sequenced and finally assembled a complete gene, which named dmt06 in this study. dmt06 was 1,422 bp in size, encoding a 473 amino acid protein. Blast in GenBank of the NCBI indicated that Dmt06 was most related to some THF-dependent demethylases or aminomethyltransferase family protein. Interestingly, Dmt06 shared 100% identity with a putative aminomethyltransferase family protein from Actinomadura parvosata subsp. kistnae, and of the proteins with known function, Dmt06 showed the highest identity (72.3%) with dicamba demethylase Dmt50 from R. dicambivorans Ndbn-20, and shared 46.2% identity with Dmt.
Expression and purification of Dmt06
To investigate the function of Dmt06, the dmt06 gene was ligated into plasmid pET29a(+) and expressed in E. coli BL21(DE3) under the induction of IPTG. Then, the recombinant Dmt06 was purified to homogeneity using Co2+-charged nitrilotriacetic acid affinity chromatography (Figure 4). SDS-PAGE analysis indicated that the molecular mass of the denatured protein was ~55 kDa, which was consistent with the theoretical mass of the tagged protein (52.3 kDa). Dmt06 was stored at −80°C in 100 mM PBS buffer (pH 7.4) with 10% glycerol and 0.3 mM EDTA, 90% of its activity was retained when stored for 2 months, indicating that Dmt06 was very stable.
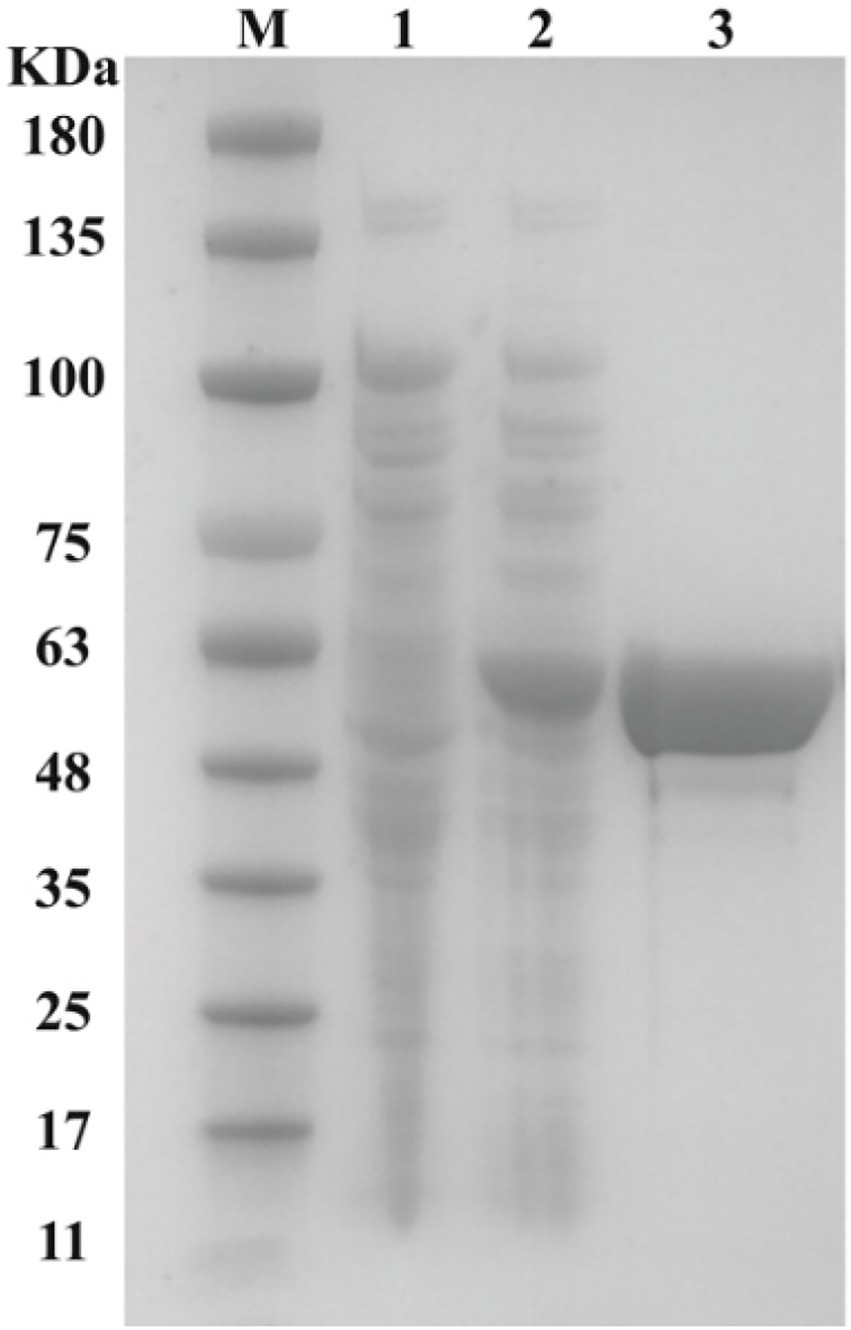
Figure 4. SDS-PAGE analysis of the purified Dmt06. Lane M: protein molecular marker; lane 1: crude extract of E. coli BL21 harboring pET29a; lane 2: crude extract of E. coli BL21 harboring pET-dmt06; lane 3: purified Dmt06.
Enzymatic assays showed that Dmt06 could transfer dicamba to a product with a retention time at 6.25 min, which was equal to that of the 3,6-DCSA standard (Figure 5). MS analysis showed that the product had a prominent deprotonated molecular ion peak at m/z 204.95 (M-H)− with a fragment peak at m/z 106.95 (Figure 6), this ion peak characteristic was also consistent with that of 3,6-DCSA. Thus, the product was identified as 3,6-DCSA. The above results demonstrated that Dmt06 was a demethylase catalyzing the conversion of dicamba to 3,6-DCSA.
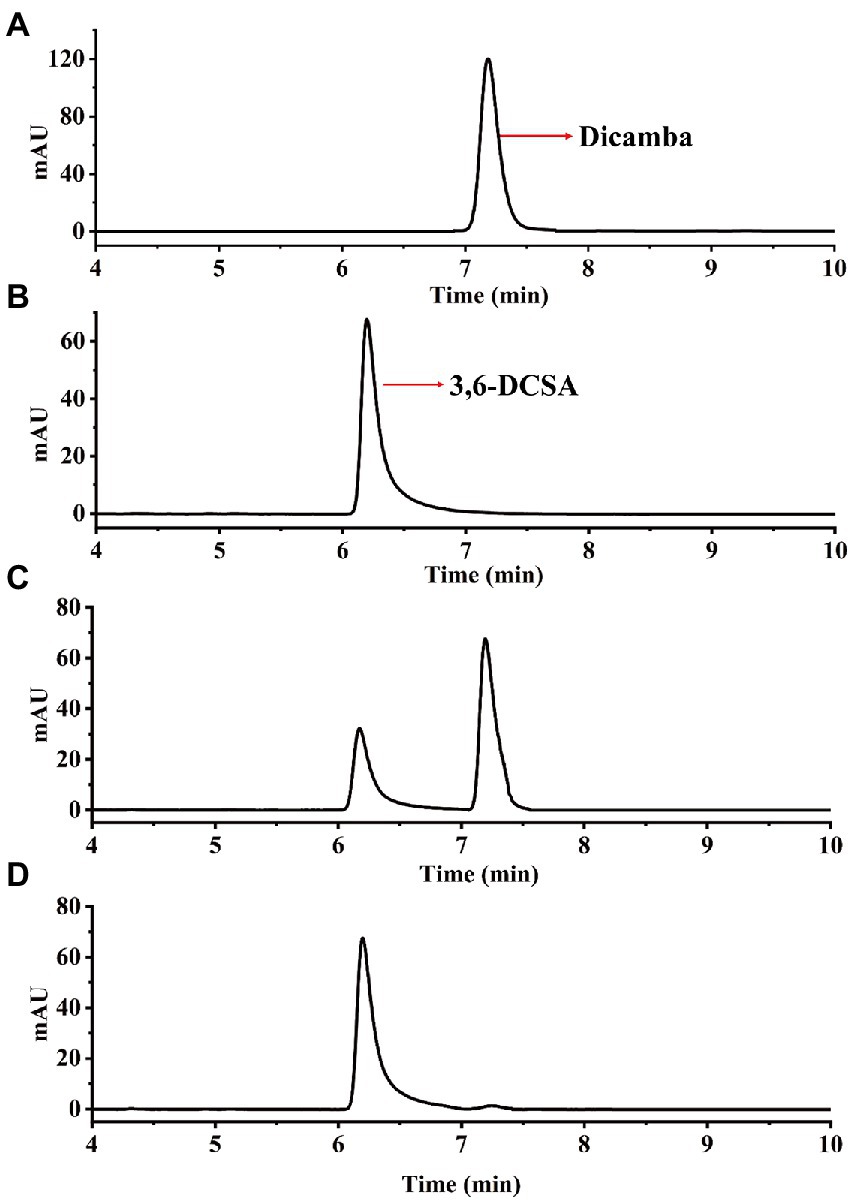
Figure 5. HPLC analysis of the product produced during dicamba conversion by Dmt06. (A) The dicamba standard. (B) The 3,6-DCSA standard. (C) Sample collected at 5 min. (D) Sample collected at 10 min.
Enzymatic characteristics of the purified Dmt06
The purified Dmt06 displayed dicamba demethylase activity only in the presence of THF, indicating that Dmt06 was a THF-dependent demethylase. The activity of Dmt06 was detected from 10°C to 60°C and at pH ranging from 5.5 to 10.0. The optimum pH and temperature for Dmt06 was 7.4 and 35°C, respectively (Supplementary Figures S1, S2). In the stability studies, more than 70% of the activity of Dmt06 was retained after incubation at 50°C for 120 min, and <20% of its activity was retained after incubation at 60°C for 120 min. The results indicated that Dmt06 was stable at 50°C, but become unstable when temperature raised to 60°C. The effects of various metal ions and potential inhibitors on the activity of Dmt06 are shown in Supplementary Figure S3. The activity of Dmt06 was severely inhibited by 1.0 mM of Hg2+, Co2+, Zn2+, Cd2+, Ag2+, and 5.0 mM of SDS, and was moderately inhibited by 1.0 mM of Mn2+, Ni2+, Cu2+, Ba2+, and Al3+. On the other hand, 1.0 mM of Li+, Na+, K+, Mg2+, Ca2+, Fe2+, Fe3+, and 5.0 mM EDTA had no obviously effect on the activity of Dmt06. At the optimal condition, the incubation of 0.1 mg of Dmt06 for 5 min resulted in a specific activity of 165 nmol/min/mg toward dicamba. Dmt06 could not catalyze the methyl transfer of vanillate, syringate, isoproturon, and alachlor, indicating that Dmt06 possessed a very narrow substrate spectrum.
Discussion
The planting of glyphosate-resistant transgenic crops combined with the application of glyphosate can effectively kill weeds without damaging the crops, thus proving an efficient and low-cost weed control strategy (Barrows et al., 2014). Therefore, the planting area of glyphosate-resistant GM crops have been rapidly expanded since their commercialization in the early 1990s, reaching ~150 million hectares in 2018 (See footnote 1). However, long-term high-intensive use of glyphosate has resulted in increasing resistance of weeds (Cerdeira and Duke, 2006). It was reported that at least 41 kinds of weeds in 29 countries have developed strong resistance to glyphosate, which makes the weeding strategy of glyphosate-resistant transgenic crop ineffective. Therefore, it is necessary to screen new target herbicides and construct corresponding transgenic crops. Dicamba is a broad-spectrum herbicide that can effectively kill glyphosate-resistant weeds. In particular, dicamba has been commercially used for more than 60 years since it was developed in the 1960s (Tomlin, 2006), but so far, few weeds have developed resistance to dicamba. Therefore, dicamba is an ideal target herbicide for the next generation of herbicide-resistant engineering, in which the dicamba detoxification genes have great application potential.
Previous studies have indicated that dicamba could be degraded by soil bacteria, and the initial step of microbial degradation was demethylation to generate 3,6-DCSA, which was herbicidally inactive (Li et al., 2018). Thus, dicamba demethylase gene is an ideal herbicide-resistant gene for herbicide-resistant engineering. So far, a NADH-dependent dicamba monooxygenase DMO and two THF-dependent dicamba demethylases Dmt and Dmt50 have been identified. Biological giant Monsanto has successfully used the oxygenase gene of DMO to construct dicamba-resistant soybean and corn, which have been commercially planted on a large scale. Compared with DMO, the advantage of the THF-dependent demethylases Dmt and Dmt50 is that they do not need reducing force NADH, so it is more energy-saving. In addition, the 5-methyl-THF generated during dicamba demethylation can be used for the synthesis of purine, pyrimidine, glycine and methionine, as well as the methylation of DNA, fatty acids and enzymes (Sullivan et al., 2021; Menezo et al., 2022). However, the disadvantage of Dmt and Dmt50 is that their activities were lower than that of DMO, which greatly limits their abilities to detoxify dicamba.
In this study, we enriched a highly efficient dicamba-degrading microbial consortium, the sixth generation of the enrichment could almost completely degrade 500 mg/L dicamba within 12 h incubation. In previous reports, Sphingobium sp. Ndbn-10 and R. dicambivorans Ndbn-20 degraded 2.25 mM (~ 497 mg/L) of dicamba within 36 h and 72 h, respectively, and Pseudomonas maltophilia DI-6 degraded 97% of 1,000 mg/L dicamba within 30 h (Krueger et al., 1989; Yao et al., 2016). Thus, the enriched consortium had a relatively high dicamba-degrading efficiency when compared with previous studies. To isolate pure strains that capable of degrading dicamba, the enriched consortium was serially diluted, the diluent was spread on LB plate and incubated at 30°C for 5 days. Colonies with different morphology were selected to test their dicamba-degrading abilities. We selected ~100 colonies, unexpectedly, none of them could degrade dicamba. The possible reason might be that the bacteria responsible for dicamba degradation in the enriched consortium were unculturable. Activities study of the crude extract of the consortium indicated that the degradation of dicamba was initiated by demethylation, which catalyzed by an unknown THF-dependent demethylase (named Dmt06 in this study). To clone the gene, a fragment of dmt06 was firstly successfully amplified from the total DNA of the enriched consortium by PCR using two pairs of degenerate primers, which designed according to the conserved region of reported THF-dependent demethylases, and then the full length of dmt06 was obtained by DNA walking using SEFA-PCR method. Interestingly, results of blastp in the Non-redundant protein sequences (nr) database of NCBI showed that Dmt06 shared 100% identities with a putative aminomethyltransferase family protein from Actinomadura parvosata subsp. kistnae, and ~70%–98% identities with a lot of putative aminomethyltransferase family proteins from indigenous soil bacteria Rhizobiales, Nonomuraea, Rhizorhabdus, Proteobacteria, Rhizobium, Mesorhizobium, Sinorhizobium, Tianweitania, and Microbacterium. The wide distribution of this gene in soil bacteria suggested that it may have important functions, e.g., it is possible that the gene participates in the demethylation process of some natural occurred methyl-containing aromatic compounds. Results of blastp in the UniProtKB/Swiss-Prot (swissprot) database of NCBI indicated that Dmt06 was most related to the two reported THF-dependent demethylases Dmt and Dmt50. However, the identities between them were <75%, these analyses suggested that dmt06 might encode a novel THF-dependent methyltransferase gene that differs from dmt and dmt50. Furthermore, Dmt06 was also obviously different from Dmt and Dmt50 in size and enzymatic characteristics. E.g., Dmt06 has 473 amino acids, while Dmt and Dmt50 have 466 and 475 amino acids, respectively. Dmt06 had a relatively narrow pH range (5.5–10.0) than that of Dmt (5.0–10.0) and Dmt50 (3.6–10.0), Dmt06 could tolerate 50°C, which was higher than that of Dmt (45°C) but much lower than that of Dmt50 (75°C). The optimal pH and temperature for Dmt06 (7.4 and 35°C, respectively) were also different from that of Dmt (8.0 and 30°C, respectively) and Dmt50 (8.0 and 45°C, respectively). Most important, the specific activity of Dmt06 reached 165 nmol/min/mg toward dicamba, which was significantly higher than that of Dmt (114 nmol/min/mg; Yao et al., 2016) and Dmt50 (146 nmol/min/mg; Chen et al., 2019), indicating that Dmt06 has stronger dicamba detoxification ability than Dmt and Dmt50. Thus, dmt06 is a potential candidate for the engineering of dicamba-resistant transgenic crops and bioremediation of dicamba residue pollution in environment. At the same time, the sequence comparison and structure analysis of Dmt06, Dmt and Dmt50 can provide a basis to elucidate the catalytic mechanism of the THF-dependent demethylase. In the future, we will study the structure and the key active sites of this new dicamba demethylase, and improve the activity of Dmt06 through directed evolution technology and rational protein design.
Conclusion
In conclusion, this study cloned a novel THF-dependent dicamba demethylase gene dmt06 from an efficient dicamba-degrading microbial consortium. Dmt06 were synthesized in E. coli BL21 and purified as His-tagged enzymes. Enzymatic assay showed that the dicamba demethylation activity of Dmt06 was much higher than that of Dmt and Dmt50, indicating that it has good application value in the dicamba-resistant transgenic engineering.
Data availability statement
The data presented in the study are deposited in the NCBI GenBank repository, accession number ON828423.
Author contributions
NL and LC conceived the presented idea. NL and JH contributed to the writing and prepared the figures and tables. EC, CY and HZ participated in revising the manuscript. All authors contributed to the article and approved the submitted version.
Funding
This work was financially supported by the National Natural Science Foundation of China (no. 31900082); the China Postdoctoral Science Foundation (no. 2021M701735); and the High-qualified Talents Scientific Research Startup Foundation of Nanyang Normal University (2019ZX013).
Conflict of interest
The authors declare that the research was conducted in the absence of any commercial or financial relationships that could be construed as a potential conflict of interest.
Publisher’s note
All claims expressed in this article are solely those of the authors and do not necessarily represent those of their affiliated organizations, or those of the publisher, the editors and the reviewers. Any product that may be evaluated in this article, or claim that may be made by its manufacturer, is not guaranteed or endorsed by the publisher.
Supplementary material
The Supplementary material for this article can be found online at: https://www.frontiersin.org/articles/10.3389/fmicb.2022.978577/full#supplementary-material
Footnotes
References
Abe, T., Masai, E., Miyauchi, K., Katayama, Y., and Fukuda, M. (2005). A tetrahydrofolate-dependent O-demethylase, LigM, is crucial for catabolism of vanillate and syringate in Sphingomonas paucimobilis SYK-6. J. Bacteriol. 187, 2030–2037. doi: 10.1128/JB.187.6.2030-2037.2005
Behrens, M. R., Mutlu, N., Chakraborty, S., Dumitru, R., Jiang, W. Z., LaVallee, B. J., et al. (2007). Dicamba resistance: enlarging and preserving biotechnology-based weed management strategies. Science 316, 1185–1188. doi: 10.1126/science.1141596
Barrows, G., Sexton, S., and Zilberman, D. (2014). Agricultural biotechnology: the promise and prospects of genetically modified crops. J. Econ. Perspect. 28, 99–120. doi: 10.1257/jep.28.1.99
Chen, L., Yao, S. G., Chen, T., Tao, Q., Xie, X. T., Xiao, X., et al. (2019). Coexpression of methyltransferase gene dmt50 and methylene tetrahydrofolate reductase gene increases Arabidopsis thaliana dicamba resistance. J. Agric. Food Chem. 67, 1443–1452. doi: 10.1021/acs.jafc.8b04944
Cerdeira, A. L., and Duke, S. O. (2006). The current status and environmental impacts of glyphosate-resistant crops. J. Environ. Qual. 35, 1633–1658. doi: 10.2134/jeq2005.0378
Huang, X., He, J., Yan, X., Hong, Q., Chen, K., He, Q., et al. (2017). Microbial catabolism of chemical herbicides: microbial resources, metabolic pathways and catabolic genes. Pestic. Biochem. Physiol. 143, 272–297. doi: 10.1016/j.pestbp.2016.11.010
Herman, P. L., Behrens, M., Chakraborty, S., Chrastil, B. M., Barycki, J., and Weeks, D. P. (2005). A three-component dicamba O-demethylase from Pseudomonas maltophilia strain DI-6. J. Biol. Chem. 280, 24759–24767. doi: 10.1074/jbc.M500597200
Krueger, J. P., Butz, R. G., Atallah, Y. H., and Cork, D. J. (1989). Isolation and identification of microorganisms for the degradation of dicamba. J. Agric. Food Chem. 37, 534–538. doi: 10.1021/jf00086a057
Li, N., Yao, L., He, Q., Qiu, J. G., Cheng, D., Ding, D. R., et al. (2018). The catabolism of 3,6-dichlorosalicylate is initiated by the cytochrome P450 monooxygenase system DsmABC in Rhizorhabdus dicambivorans Ndbn-20. Appl. Environ. Microbiol. 84, e02133–e02117. doi: 10.1128/AEM.02133-17
Liu, J. W., Zhang, X., Xu, J. Y., Qiu, J. G., Zhu, J. C., Cao, H., et al. (2020). Anaerobic biodegradation of acetochlor by acclimated sludge and its anaerobic catabolic pathway. Sci. Total Environ. 748:141122. doi: 10.1016/j.scitotenv.2020.141122
Masai, E., Sasaki, M., Minakawa, Y., Abe, T., Sonoki, T., Katayama, Y., et al. (2004). A novel tetrahydrofolate-dependent O-demethylase gene is essential for growth of Sphingomonas paucimobilis SYK-6 with syringate. J. Bacteriol. 186, 2757–2765. doi: 10.1128/JB.186.9.2757-2765.2004
Menezo, Y., Elder, K., Clement, A., and Clement, P. (2022). Folic acid, folinic acid, 5-methyl tetrahydrofolate supplementation for mutations that affect epigenesis through the folate and one-carbon cycles. Biomol. Ther. 12, 197. doi: 10.3390/biom12020197
Stevens, J. T., and Sumner, D. D. (1991). Handbook of Pesticide Toxicology. Academic Press, New York, NY, 1317–1408.
Sambrook, J., and Russell, D. (2001). Molecular Cloning: A Laboratory Manual, 3rd ed. Cold Spring Harbor Laboratory, Cold Spring Harbor, NY.
Sullivan, M. R., Darnell, A. M., Reilly, M. F., Lewis, C. A., and Heiden, M. (2021). Methionine synthase is essential for cancer cell proliferation in physiological folate environments. Nat. Metab. 3, 1500–1511. doi: 10.1038/s42255-021-00486-5
Taraban, R. H., Berry, D. F., Berry, D. A., and Walker, H. L. Jr. (1993). Degradation of dicamba by an anaerobic consortium enriched from wetland soil. Appl. Environ. Microbiol. 59, 2332–2334. doi: 10.1128/aem.59.7.2332-2334.1993
Tomlin, C. D. S. (2006). The Pesticide Manual: A World Compendium, 14th ed. British Crop Protection Council, Farnham.
Wang, S. M., He, J., Cui, Z. L., and Li, S. P. (2007). Self-formed adaptor PCR: a simple and efficient method for chromosome walking. Appl. Environ. Microbiol. 73, 5048–5051. doi: 10.1128/AEM.02973-06
Werwath, J., Arfmann, H. A., Pieper, D. H., Timmis, K. N., and Wittich, R. M. (1998). Biochemical and genetic characterization of a gentisate 1,2-dioxygenase from Sphingomonas sp. strain RW5. J. Bacteriol. 180, 4171–4176. doi: 10.1128/JB.180.16.4171-4176.1998
Wang, X. Z., Li, B., Herman, P. L., and Weeks, D. P. (1997). A three-component enzyme system catalyzes the O-demethylation of the herbicide dicamba in Pseudomonas maltophilia DI-6. Appl. Environ. Microbiol. 63, 1623–1626. doi: 10.1128/aem.63.4.1623-1626.1997
Yao, L., Jia, X. J., Zhao, J. D., Cao, Q., Xie, X. T., Yu, L. L., et al. (2015). Degradation of the herbicide dicamba by two sphingomonads via different O-demethylation mechanisms. Int. Biodeterior. Biodegr. 104, 324–332. doi: 10.1016/j.ibiod.2015.06.016
Keywords: dicamba, microbial consortium, gene clone, tetrahydrofolate-dependent demethylase, Dmt06
Citation: Li N, Chen L, Chen E, Yuan C, Zhang H and He J (2022) Cloning of a novel tetrahydrofolate-dependent dicamba demethylase gene from dicamba-degrading consortium and characterization of the gene product. Front. Microbiol. 13:978577. doi: 10.3389/fmicb.2022.978577
Edited by:
Shaohua Chen, South China Agricultural University, ChinaReviewed by:
Zhiyong Ruan, Institute of Agricultural Resources and Regional Planning (CAAS), ChinaChunyan Li, Northeast Agricultural University, China
Copyright © 2022 Li, Chen, Chen, Yuan, Zhang and He. This is an open-access article distributed under the terms of the Creative Commons Attribution License (CC BY). The use, distribution or reproduction in other forums is permitted, provided the original author(s) and the copyright owner(s) are credited and that the original publication in this journal is cited, in accordance with accepted academic practice. No use, distribution or reproduction is permitted which does not comply with these terms.
*Correspondence: Jian He, 530241892@qq.com; hejian@njau.edu.cn; Cansheng Yuan, yuancs@jsou.edu.cn