- Key Laboratory of Animal Feed and Nutrition of Inner Mongolia Autonomous Region, College of Animal Science, Inner Mongolia Agricultural University, Hohhot, China
The objective of this study was to evaluate the effect of Allium mongolicum Regel ethanol extract (AME) on the concentration of three branched-chain fatty acids (BCFAs) related to flavor, fermentation parameters and the bacteria and their correlations in the rumen of lambs. A total of thirty 3-month-old male, Small-tailed Han sheep (33.60 ± 1.23 kg) were randomly distributed into 2 groups as follows: control group (CON) was fed a basal diet and AME group was fed a basal diet supplemented with 2.8 g⋅lamb–1⋅d–1 A. mongolicum Regel ethanol extract. AME supplementation decreased (P = 0.022) 4-methyloctanoic acid (MOA) content and tended to lower (P = 0.055) 4-methylnonanoic acid (MNA) content in the rumen. Compared to CON group, the ruminal concentrations of valerate and isovalerate were higher (P = 0.046 and P = 0.024, respectively), and propionate was lower (P = 0.020) in the AME group. At the phylum level, the AME group had a lower abundance of Bacteroidetes (P = 0.014) and a higher abundance of Firmicutes (P = 0.020) than the CON group. At the genus level, the relative abundances of Prevotella (P = 0.001), Christensenellaceae_R-7_group (P = 0.003), Succiniclasticum (P = 0.004), and Selenomonas (P = 0.001) were significantly lower in the AME group than in the CON group, while the relative abundances of Ruminococcus (P < 0.001), Quinella (P = 0.013), and Lachnospiraceae_XPB1014_group (P = 0.001) were significantly higher. The relative abundances of Prevotella (P = 0.029, R = 0.685; P = 0.009, R = 0.770), Christensenellaceae_R-7_group (P = 0.019, R = 0.721; P = 0.029, R = 0.685), and Succiniclasticum (P = 0.002, R = 0.842; P = 0.001, R = 0.879) was positively correlated with MOA and MNA levels, and the relative abundance of Lachnospiraceae_XPB1014_group (P = 0.033, R = −0.673) was negatively correlated with MOA. The relative abundance of Christensenellaceae_R-7_group (P = 0.014, R = −0.744) and Prevotellaceae_UCG-003 (P = 0.023, R = −0.706) correlated negatively with the EOA content. In conclusion, these findings suggest that the AME affected the concentration of BCFAs, fermentation parameters and the rumen bacteria in the rumen of lambs.
Introduction
Sheep meat and milk have a characteristic “mutton flavor,” which is mainly associated with branched-chain fatty acids (BCFAs), especially 4-methyloctanoic acid (MOA), 4-ethyloctanoic acid (EOA) and 4-methylnonanoic acid (MNA) (Salles et al., 2002). MOA and MNA are de novo synthesized by the use of methylmalonyl-CoA in chain lengthening with acetyl-CoA using propionate as a precursor in the liver (Massart et al., 1983; Vlaeminck et al., 2006). Propionate from rumen fermentation through the rumen epithelium into the portal vein arrives at the liver, and then the liver synthesize BCFAs when propionate concentrations exceed the capacity of gluconeogenesis (Wong et al., 1975; Aschenbach et al., 2010). The biosynthesis pathway of EOA is less well known and may follow the same biosynthesis pathway of MOA and MNA, which uses butyrate and ethylmalonyl-CoA instead of propionate and methylmalonyl-CoA (Kaffarnik et al., 2015). Methylmalonyl-CoA and ethylmalonyl-CoA originate from the carboxylation of propionyl-CoA and butyryl-CoA, respectively, by acetyl-CoA carboxylase (Dewulf et al., 2019). In addition, ruminal microbiota also participate in the formation of BCFAs, which by using the carbon skeleton of iso-butyrate, iso-valerate and branched-chain amino acids (Shivani et al., 2016).
The composition of a diet is one of the most important factors affecting the concentration of BCFAs. High-concentrate diets lead to a higher proportion of VFAs, especially propionate, in the rumen than low-concentrate diets (Plaizier et al., 2008), which will provide sufficient precursors for the synthesis of BCFAs. Young et al. (2003) reported that ram lambs fed with an alfalfa pellet or a corn-based diet had greater concentrations of MOA and MNA in the subcutaneous fat than ram lambs grazing ryegrass/clover pasture. Teng et al. (2020) found that milk from lambs offered perennial ryegrasses/white clover has lower levels of MOA and MNA than milk from lambs offered Lucerne silage and soy meal. In recent years, researchers have made substantial efforts to reduce mutton flavor by adding natural plant extracts, such as tannins, flavonoids, and essential oils, to lamb diets. Vasta et al. (2013) stated that essential oils from Rosmarinus officinalis or Artemisia herba alba can change the volatile compound composition in lamb meat. Del Bianco et al. (2021) reported that supplementation with 4% tannins from Acacia mearnsii, Castanea sativa, or Caesalpinia spinosa in the diet decreased the concentrations of MOA and 8-methylnonanoic acid, which also conferred a “mutton” flavor (Brennand et al., 1989) to the perirenal fat of Sarda × Comisana lambs.
Allium Mongolicum Regel is a traditional Mongolian medicinal herb belonging to the genus Allium of the Liliaceae family and grows extensively in northwest China (Wang et al., 2019). A. Mongolicum Regel contains polysaccharides, flavonoids, polyphenols, and other bioactive compounds (Li et al., 2019). Our previous studies indicated that A. Mongolicum Regel ethanol extract (AME) significantly decreased the concentration of BCFAs in the longissimus dorsi muscle and dorsal subcutaneous, omental and perirenal adipose tissues of Small-tailed Han sheep (Liu et al., 2019; Liu and Ao, 2021). However, it is not clear whether AME affects the synthesis of three BCFAs related to flavor in the rumen of lambs. Thus, the objective of this study was to investigate the effects of AME on the concentrations of three rumen BCFAs related to flavor, rumen fermentation parameters, the diversity of rumen bacteria and their correlations in lambs.
Materials and methods
All experimental procedures involving animals were evaluated and approved according to the guidelines of the Animal Care and Use Committee of Inner Mongolia Agriculture University (Hohhot, China). This study was conducted at a commercial farm in Bayannaoer, Inner Mongolia Autonomous Region, China (latitude 40°13′–42°28′; longitude 105°12′–109°53′) between April and June 2021.
Animals and experimental design
A total of thirty 3-month-old male, Small-tailed Han sheep (33.60 ± 1.23 kg) were randomly distributed into 2 groups as follows: control group (CON) was fed a basal diet and AME group was fed a basal diet supplemented with 2.8 g⋅lamb–1⋅d–1 A. Mongolicum Regel ethanol extract. One group was composed of three pens, with five lambs in each pen (3.95 m × 5.12 m). A. Mongolicum Regel powder was purchased from Hao Hai Biological Company (Alxa League, Inner Mongolia, China), and the ethanol extraction process was conducted as previously described by Ding et al. (2021). The obtained AME primarily contained 26.43% flavonoids, 18.57% organic acids and their derivatives, 14.43% nucleotides and their derivatives, 11.14% amino acids and others. The dose of the ethanol extract (2.8 g⋅lamb–1⋅d–1) in the diet has been proven to be most beneficial for the lamb based on our previous studies (Zhao et al., 2021). We mixed 2.8 g AME with 50 g concentrate for each lamb. Thus, the total dose of five lambs (a pen) is 14 g AME mixed with 250 g concentrate, divided into two equal amounts, and provided for each pen twice daily to ensure that the lambs completely consumed the AME. Each pen of CON group was fed 250 g of concentrate without AME in the same manner. Then, total mixed ration was provided, and the lambs were allowed to feed ad libitum. The basal diet met the requirements for sheep, as described by the National Research Council (NRC, 2007), and its composition and nutritional level are shown in Table 1.
The experimental period lasted for 75 days, with 15 days of adaptation to the experimental environment and 60 days for the experimental feeding period. The lambs were given free access to water and TMR was provided twice daily at 7:00 and 18:00. During the experimental period, feed was provided to the lambs and refusals were recorded to calculate the dry matter intake (DMI). The body weight of each lamb was also recorded before morning feeding at 0, 15, 30, 45, and 60 days, to calculate average daily gain (ADG), meanwhile, initial body weight (IBW), and final body weight (FBW) were recorded.
Sampling
At 60 days of the experimental period, rumen fluid samples were collected before the morning feeding using an oral stomach tube connected to a 50 mL syringe from six randomly selected lambs from each group. The initial part of the rumen fluid was discarded to avoid contamination with saliva, and then rumen fluid (approximately 50 mL) was collected and filtered through four layers of gauze. The pH was measured using a portable pH meter (PHB-4; INESA Scientific Instrument Co., Ltd, Shanghai, China). 10 mL of rumen fluid was mixed with 2 mL of 25% HPO3 and stored at −20°C until volatile fatty acid (VFA) analysis. 10 mL of rumen fluid was stored at −20°C until ammonium nitrogen (NH3-N) analysis. The remaining rumen fluid was immediately frozen in liquid nitrogen and stored at −80°C until BCFAs analysis and microbial community analysis.
Chemical analyses of feeds
Samples of feed were dried at 55°C for 48 h and then ground to pass through a 2 mm sieve before chemical analysis. Crude protein (N × 6.25, method 981.10) and minerals content were measured using an analytical method provided by the Association of Official Agricultural Chemists (AOAC, 2000). The neutral detergent fiber, acid detergent fiber were determined as described by Van Soest et al. (1991).
Branched-chain fatty acid analyses
Three BCFAs, namely, MOA, MNA, and EOA, in the rumen were analyzed by gas chromatography–mass spectrometry (GC–MS). Prior to GC–MS analysis, fatty acids in the rumen were converted to their corresponding methyl esters, which was carried out according to the method of Kaffarnik et al. (2014). Undecanoic acid (Sigma–Aldrich, USA) was used as an internal standard.
A GC–MS system (Thermo TRANCE GC 1300) equipped with an autosampler was used in EI mode (70 eV). Solutions (1 μL) were injected in split mode (split ratio 10:1) onto a TG-WAX column (30 m, 0.25 mm i.d., 0.25 μm film thickness). The GC oven was held at 60°C for 2 min, and then, the temperature was raised at 20°C min–1 to 250°C and held for 5 min. The flow rate of the helium carrier gas was 1.2 mL/min. The mass spectrometer transfer line was operated at 250°C. Mass spectra were acquired using an ion source temperature of 220°C. GC–MS analyses were performed in full-scan mode (m/z 50–350).
Ruminal fermentation analyses
Ruminal NH3-N concentrations were analyzed using a microplate reader (Epoch, BioTek Instruments, Inc., USA) according to the method described by Chaney and Marbach (1962). The concentration of VFA was determined using a gas chromatograph (GC-2014; Shimadzu, Japan) with a fused silica column (60 m × 0.25 mm × 0.50 μm; DB-FFAP, Agilent Technologies, USA) according to Erwin et al. (1961). 2-Ethyl butyric acid was used as an internal standard. The column temperature was increased from 80 to 180°C at 20°C/min and held for 3 min. The injector and detector temperatures were set at 220 and 250°C, respectively.
16S rDNA extraction and sequencing
Microbial community genomic DNA was extracted from rumen fluid samples using the E.Z.N.A.® soil DNA Kit (Omega Bio-Tek, Norcross, GA, U.S.) according to the manufacturer’s instructions. The DNA quality and quantity were determined by a NanoDrop 2000 UV–vis spectrophotometer (Thermo Fisher Scientific, Wilmington, USA). The V3–V4 region of the bacterial 16S rRNA gene was amplified with the primer pair 338F (5′-ACTCCTACGGGAGGCAGCAG-3′) and 806R (5′-GGACTACHVGGGTWTCTAAT-3′) by an ABI GeneAmp® 9700 PCR thermocycler (ABI, CA, USA). The PCR amplification of the 16S rRNA gene was performed as follows: initial denaturation at 95°C for 3 min, followed by 27 cycles of denaturing at 95°C for 30 s, annealing at 55°C for 30 s and extension at 72°C for 45 s, and a single extension at 72°C for 10 min, ending at 4°C. The PCR mixtures contained 4 μL of 5 × TransStart FastPfu buffer, 2 μL of 2.5 mM deoxynucleoside triphosphates, 0.8 μL of each primer (5 μM), 0.4 μL of TransStart FastPfu DNA polymerase, 10 ng of template DNA, and ddH2O to reach a total volume of 20 μL. The PCR products were purified using the AxyPrep DNA Gel Extraction Kit (Axygen Biosciences, Union City, CA, USA) and quantified using a Quantus™ Fluorometer (Promega, USA). Purified amplicons were sequenced on an Illumina MiSeq PE300 platform (Illumina, San Diego, USA).
Sequencing data analyses
Fastp v0.19.6 software1 and FLASH v1.2.11 software2 were used for quality control and splicing, respectively. UPARSE v7.0.1090 software was used to cluster operational taxonomic units (OTUs) with 97% similarity (Edgar, 2013). The taxonomy of each OTU representative sequence was analyzed by ribosomal database project (RDP) classifier v2.113 compared with Silva v1384 using a comparison threshold of 70% (Han et al., 2015). Alpha diversity analysis was performed by Mothur v1.30.25.
Statistical analysis
Sequencing data followed normality (Shapiro–Wilk’s tests, P > 0.2) after log transformations, and their differences were homogenous (Levene’s test, P > 0.05). Non-transformed values are shown in this study. Data for growth performance, the concentration of BCFAs, rumen fermentation parameters and bacterial communities between CON and AME group lambs were analyzed using one-way ANOVA by SPSS 26.0 (IBM, New York, USA). The statistical model was Yi = μ + Ai + e, where Yi is the dependent variable; μ is the overall mean; Ai is the fixed effect of treatment (i = 1.2, CON or AME); and e is the random effect. The degrees of freedom of ANOVA are as follows: total: dfT = n - 1 (n = total sample size); group: dfG = k - 1 (k = total group size); error: dfE = dfT - dfG. Statistical significance was defined at P < 0.05, and trending toward significance was defined at 0.05 ≤ P ≤ 0.1. Spearman’s rank correlation coefficient analysis between BCFA levels, VFA levels and rumen bacterial taxa abundances was carried out using tools on the Majorbio platform (Majorbio Bio-Pharm Technology Co., Ltd.; Shanghai, China)6. Significant correlations were defined at P < 0.05.
Results
Growth performance
The results of the effect of AME on the growth performance of lambs are presented in Table 2. There were no differences in FBM and DMI between the CON and AME groups. Compared with the CON group, AME supplementation tended to increase (P = 0.098) the ADG of lambs.
Three branched-chain fatty acids concentrations and fermentation parameters in the rumen
There was no difference in the concentration of EOA in rumens between the CON and AME groups (Figure 1). AME supplementation decreased (P = 0.022) the concentration of MOA and tended to lower (P = 0.055) the concentration of MNA in the rumen.
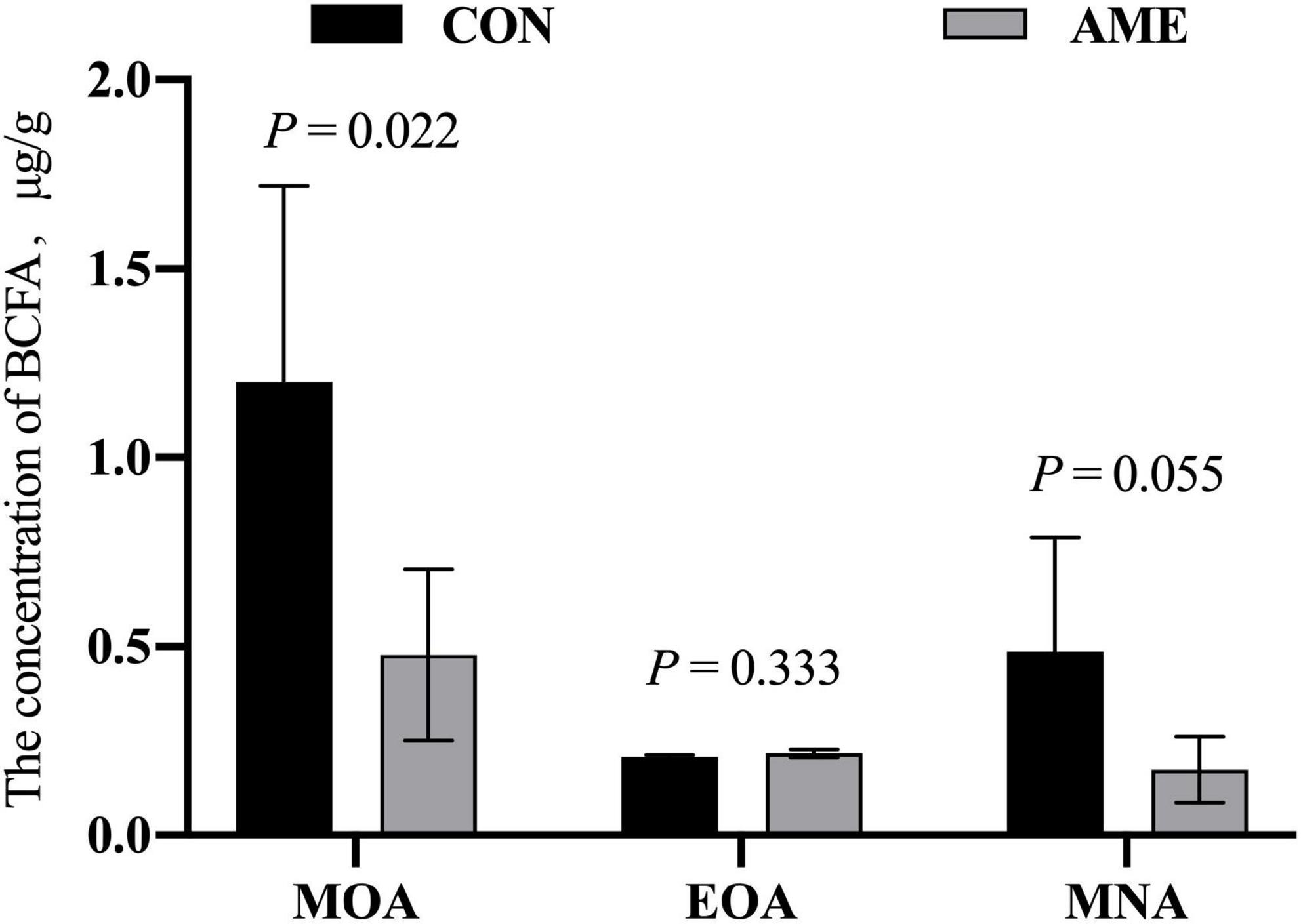
Figure 1. Effect of AME on the concentration of BCFAs in the rumen of lambs. CON, basal diet; AME, basal diet + 2.8 g⋅lamb–1⋅d–1 A. mongolicum Regel ethanol extract (AME).
The results of the rumen fermentation parameters are presented in Table 3. There were no differences in ruminal pH, TVFA level, acetate level, butyrate level, iso-butyrate level, or acetate/propionate ration between the CON and AME groups. The concentration of rumen NH3-N (P = 0.078) tended to be lower in the AME group than in the CON group. Lambs consuming the AME had higher ruminal concentrations of valerate and iso-valerate (P = 0.046 and P = 0.024, respectively) than lambs consuming the CON diet and had a lower ruminal concentration of propionate (P = 0.020).
Abundance and diversity of rumen bacteria
In total, 664,062 raw sequence reads were obtained by sequencing from the lamb ruminal fluid in the CON and AME groups. After quality control and filtering, a total of 556,763 clean tags were generated, and an average of 46,397 clean tags were analyzed for each sample. According to 97% similarity, 1,243 OTUs were identified in the two groups (Table 4). No significant differences were found in the OTU number, ACE, Chao 1 index, or Shannon and Simpson diversity indices between the two treatment groups. The coverage (P = 0.07) tended to higher in AME group than CON group.
At the phylum level, the relative abundances of the top 10 rumen bacterial phyla are presented in Table 5. Bacteroidetes and Firmicutes were the predominant phyla for the two groups and accounted for approximately 96% of the total bacterial abundance. The relative composition of the bacterial phyla was changed when the diet was supplemented with AME. Compared to that in the CON group, the richness of Bacteroidetes (P = 0.014) was significantly decreased in the AME group, while the richness of Firmicutes (P = 0.020) was significantly increased. The relative abundances of Proteobacteria (P = 0.001) and Cyanobacteria (P = 0.001) were higher in the AME group than in the CON group, and that of Desulfobacterota (P = 0.052) tended to be higher.
At the genus level, 22 rumen bacterial genera with a relative abundance > 1% in at least one group are presented in Table 6. The dominant bacterial genera were Prevotella, norank_f_bacteroidales_RF16_group, Rikenellaceae_RC9_gut_group and Ruminococcus in both groups. The relative abundances of Prevotella (P = 0.001), norank_f_bacteroidales_RF16_group (P = 0.001), Christensenellaceae_R-7_group (P = 0.003), norank_f_p-251-o5 (P = 0.006), Succiniclasticum (P = 0.004), and Selenomonas (P = 0.001) were significantly lower in the AME group than in the CON group. In addition, the relative abundances of Ruminococcus (P < 0.001), Quinella (P = 0.013), norank_f_Muribaculaceae (P < 0.001), unclassified_f_Ruminococcus (P < 0.001), and Lachnospiraceae_XPB1014_group (P = 0.001) were significantly higher in the AME group than in the CON group.
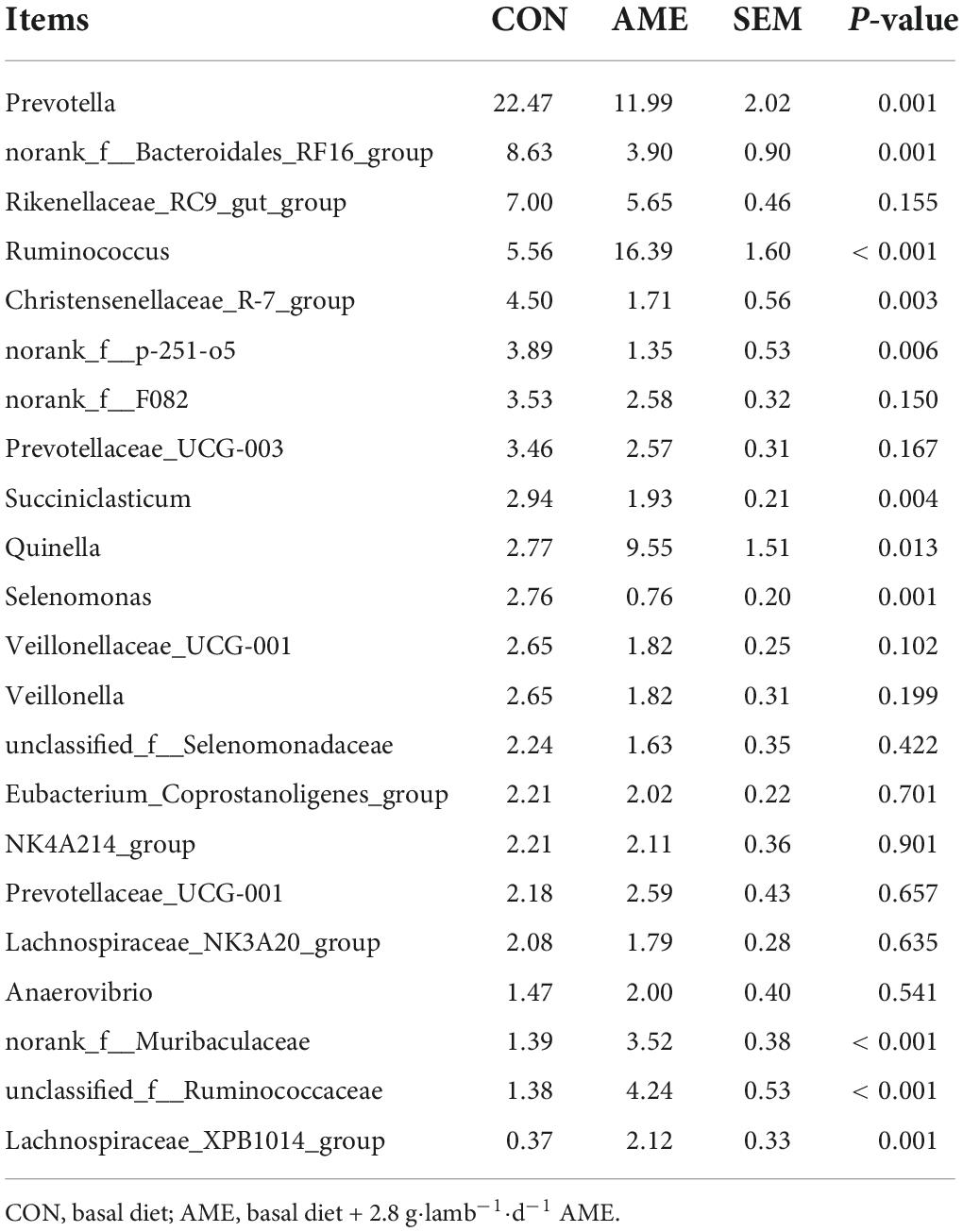
Table 6. Effect of AME on bacterial abundance at the genus level in the rumen for lambs (>1% at least in one group).
Relationships between volatile fatty acid levels, rumen bacteria and branched-chain fatty acid levels
The relationships between BCFA levels, rumen fermentation parameters and the bacterial community are presented in Figure 2. Iso-butyrate (P = 0.015, R = −0.736; P = 0.003, R = −0.827) and iso-valerate (P = 0.011, R = −0.758; P = 0.001, R = −0.879) levels correlated negatively with the MOA and MNA levels. At the phylum level, the relative abundances of Desulfobacterota (P = 0.016, R = −0.733) and Proteobacteria (P = 0.025, R = −0.697) correlated negatively with the MOA content. The relative abundances of Desulfobacterota (P = 0.009, R = −0.770) and Cyanobacteria (P = 0.038, R = −0.661) negatively correlated with the MNA content.
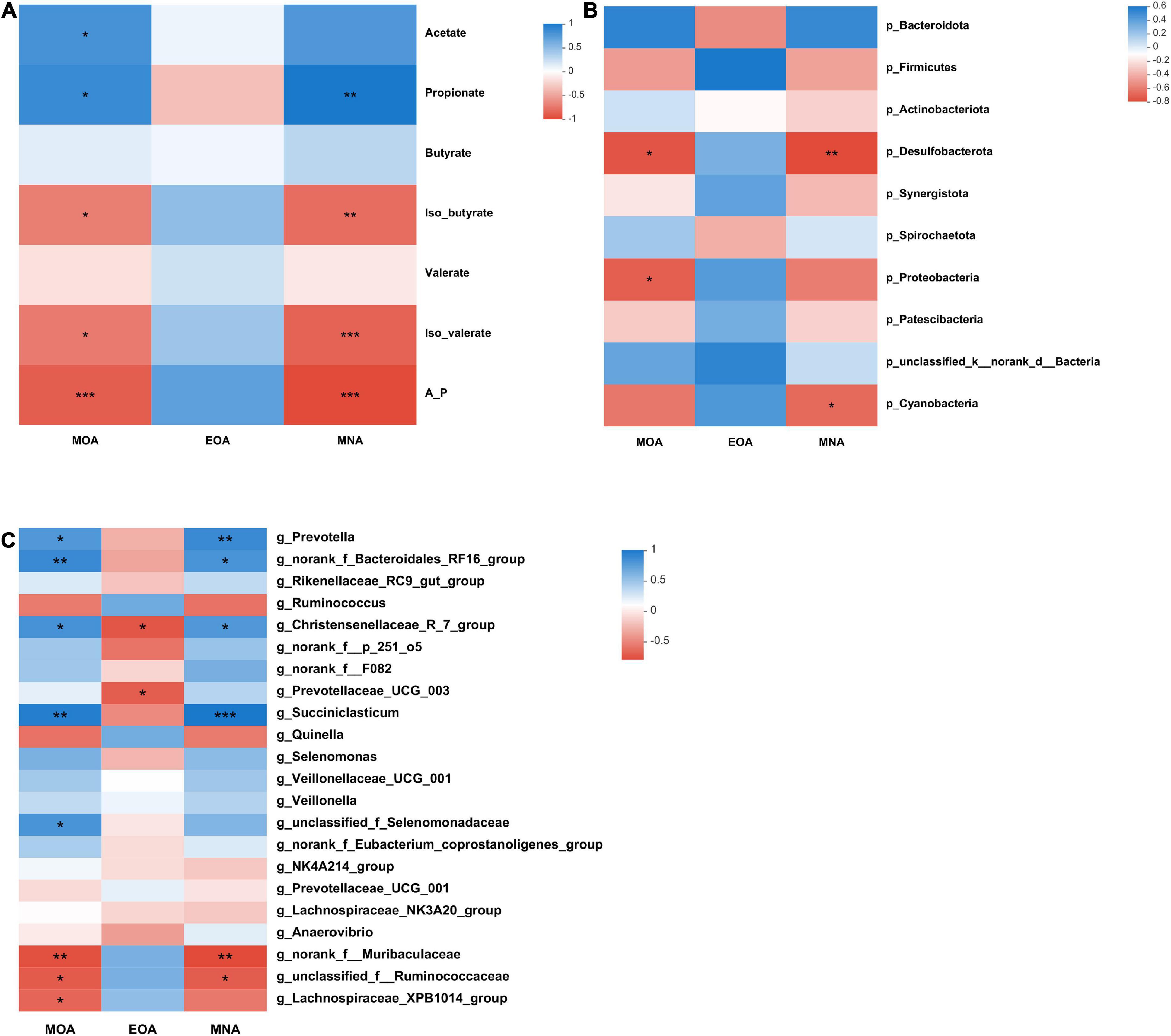
Figure 2. Correlations between VFA contents, microbial taxa and BCFA contents (A–C). (A) Correlation between the concentration of BCFAs and VFAs. (B) Correlation between the concentration of BCFAs and the top 10 microbial taxa at the phylum level. (C) Correlation between the concentration of BCFAs and genera with relative abundances > 1% (at least in one group). Significant correlations are shown by *P < 0.05, **P < 0.01, and ***P < 0.001. Blue represents positive correlation coefficients. Red represents negative correlation coefficients.
At the genus level, there were positive correlations among the following: MOA content with the Prevotella (P = 0.029, R = 0.685), norank_f_Bacteroidales_RF16_group (P = 0.009, R = 0.770), Christensenellaceae_R-7_group (P = 0.019, R = 0.721), Succiniclasticum (P = 0.002, R = 0.842), and unclassified_f_Selenomonadaceae (P = 0.022, R = 0.709) abundances and MNA content with the Prevotella (P = 0.009, R = 0.770), norank_f_Bacteroidales_RF16_group (P = 0.022, R = 0.709), Christensenellaceae_R-7_group (P = 0.029, R = 0.685), and Succiniclasticum (P = 0.001, R = 0.879) abundances. Moreover, there were negative correlations between the MOA content with the norank_f__Muribaculaceae (P = 0.009, R = −0.770), unclassified_f__Ruminococcaceae (P = 0.019, R = −0.721), and Lachnospiraceae_XPB1014_group (P = 0.033, R = −0.673) abundances; EOA content with the Christensenellaceae_R-7_group (P = 0.014, R = −0.744) and Prevotellaceae_UCG-003 (P = 0.023, R = −0.706) abundances; and MNA content with the norank_f__Muribaculaceae (P = 0.006, R = −0.794) and unclassified_f__Ruminococcaceae (P = 0.022, R = −0.709) abundances.
Discussion
Effect of Allium mongolicum Regel ethanol extract on the growth performance of Lambs
In this study, there were no differences in the FBW and DMI between the CON and AME groups. In accordance with this study, Ding et al. (2021) reported that AME did not affect the FBW and DMI, which suggested that AME had no effect on the palatability of the diet. AME supplementation tended to increase the ADG of lambs, which may be attributed to the high content of flavonoids in the AME. Muqier et al. (2017) reported that flavonoids from Allium Mongolicum Regel promote the secretion of growth hormone, insulin-like growth factor and adrenocorticotropic hormone, leading to a significant increase in the ADG. This result proved that AME could improve the growth performance of lambs.
Effect of Allium mongolicum Regel ethanol extract on three branched-chain fatty acids concentrations and fermentation parameters in the rumen of lambs
The rumen is a complex ecosystem that has a strong relationship with the formation of BCFAs. Acetate, propionate and butyrate are produced by rumen fermentation, which through the rumen epithelium into the portal vein arrives at the liver to increase the synthesis of BCFAs in the liver of lambs (Chilliard et al., 2003). Berthelot et al. (2001) further verified this view and suggested that a diet supplemented with propionate significantly increased the content of BCFAs in subcutaneous and visceral fat of lambs. This could also explain why concentrate-based diets promote animal growth, while meat has a strong mutton flavor. In the present study, we observed that AME supplementation decreased the concentrations of MOA and MNA in the rumen of lambs. The lower concentrations of MOA and MNA in the AME group might be due to the active ingredients, such as flavonoids and organic acids, of the AME inhibiting the isomerization of unsaturated fatty acids to BCFAs after triglyceride degradation.
In the present study, there was no significant difference in pH between the CON and AME groups. However, the pH was in the optimal range between 6.2 and 7.2, which reflected the stability of the rumen microbial ecosystem (Calsamiglia et al., 2002). NH3-N is an essential indicator of rumen function and is the primary substrate for microbial protein synthesis (Wallace et al., 1994). The AME group had a lower concentration of NH3-N, which may be attributed to the high content of flavonoids in the AME. A similar result was found by Jelali and Ben Salem (2014) in a study with buffalo calves offered Moringa oleifera leaf that is rich in flavonoids. Liu et al. (2020) also reported that flavonoids decrease ruminal NH3-N concentrations in in vitro experiments. This would indicate that the AME may reduce ruminal ammonia concentrations by increasing the utilization of amino acids or promoting the synthesis of microbial proteins.
VFAs produced by ruminal microorganisms degrade nutrients, which meet 60–80% of the energy requirements of ruminants (Lane and Jesse, 1997). In the current study, there were no differences in the TVFA, acetate, butyrate, or iso-butyrate levels or acetate/propionate ratio among the treatments. In accordance with this study, Seradj et al. (2018) suggested that citrus flavonoids did not affect the concentrations of TVFAs, butyrate and isobutyrate in vitro. The AME did not affect the concentration of TVFAs, probably due to a significant lack of effect on ruminal pH (Gabel et al., 2002). Furthermore, we also found that supplementation with AME in the diet decreased the concentration of propionate and increased the concentration of valerate and iso-valerate. Consistent with our results, Oskoueian et al. (2013) reported that the addition of 4.5% flavonoid substrate caused a lower propionate concentration in vitro. Moreover, there was no difference in acetate/propionate ratio between the CON and AME groups, although the concentration of propionate was lower in the AME group than in the CON group. Ruminal BCFAs (iso-butyrate and iso-valerate) originate from the microbial deamination of branched amino acids (Apajalahti et al., 2019). A relatively high valerate content is conducive to improving the growth performance of ruminants (Bergman, 1990).
Effects of Allium mongolicum Regel ethanol extract on the abundance and diversity of rumen bacteria in lambs
Previous studies have suggested that extracts from natural plants could affect the composition of rumen microorganisms in ruminants (Jiao et al., 2021; Wang et al., 2021). Bacteroidetes and Firmicutes are the predominant phyla in the ruminal microbial community, which is consistent with our results (Scharen et al., 2017). Proteobacteria are positively correlated with fiber intake (Faniyi et al., 2019). In the current study, we observed that the abundances of Bacteroidetes were lower in the AME group than in the CON group, and the abundances of Firmicutes, Proteobacteria, and Cyanobacteria were higher. Zhan et al. (2017) reported that supplementation with flavonoid extracts from alfalfa increased the ruminal abundance of Firmicutes in dairy cows. Jiang et al. (2021) observed that the relative abundances of Firmicutes and Proteobacteria were relatively high in yak calves offered root extracts from Codonopsis pilosula that contained high concentrations of flavonoids. Consequently, the flavonoids of the AME could explain the decreased abundance of Bacteroidetes and increased abundances of Firmicutes and Proteobacteria in the rumen of lambs.
At the genus level, Prevotella, Succiniclasticum, and Selenomonas are associated with propionate production (Henderson et al., 2015; Yuste et al., 2020). In the present study, adding AME to the diet significantly decreased the relative abundances of Prevotella, Succiniclasticum, and Selenomonas. The reduction in the abundances of Prevotella, Succiniclasticum, and Selenomonas resulted in a lower concentration of propionate in the rumen. The decrease in the relative abundance of Prevotella (within Bacteroidetes) could be due to the AME decreasing the relative abundance of Bacteroidetes at the phylum level. In addition, the abundance of Prevotella was negatively associated with the ruminal pH (Chiquette et al., 2012). In this study, AME increased the ruminal pH but not significantly, which may also have causes a decrease in the relative abundance of Prevotella. Charistensenellaceae_R-7_group is involved in biofilm formation (Mao et al., 2015). Studies have suggested that flavonoids can increase the relative abundance of the Charistensenellaceae_R-7_group (Harlow et al., 2018; Jiang et al., 2021). However, in the current study, the relative abundance of Charistensenellaceae_R-7_group was lower in the AME group than in the CON group, which could be due to its growth being inhibited by other active compounds of the AME.
The relationships of volatile fatty acid levels, rumen bacteria and branched-chain fatty acid levels
The phenotypic characteristics of ruminants are determined to some extent by rumen microbiota (Scharen et al., 2018). The feed was fermented by microorganisms in the rumen to produce precursors (e.g., propionate and butyrate) of BCFA biosynthesis. Some of the precursors are formed into BCFAs in the rumen, while the others are absorbed into the blood through the rumen wall and then transported to the liver to synthesize BCFAs (Victoria and Robert, 1993). Therefore, rumen VFAs and microorganisms are key factors that directly or indirectly affect BCFA biosynthesis. In the present study, Spearman’s rank correlation coefficient analysis was conducted to analyze the relationip between VFA levels, rumen bacteria and BCFA levels related to mutton flavor. We found that MOA and MNA levels negatively correlated with iso-butyrate and iso-valerate levels. Moreover, the relative abundances of Prevotella, Christensenellaceae_R-7_group, and Succiniclasticum correlated positively with MOA and MNA contents, and the Lachnospiraceae_XPB1014_group abundance correlated negatively with MOA and MNA levels. The relative abundance of Lachnospiraceae_XPB1014_group correlated negatively with the EOA content. These findings reveal the relationship between the rumen bacteria and three flavor-related BCFAs in the rumen of lambs.
Conclusion
In the present study, a diet supplemented with AME significantly decreased the concentrations of MOA and MNA in the rumen of lambs. AME supplementation also changes the rumen fermentation pattern and the composition of rumen bacteria. Moreover, this study also found that MOA and MNA were positively correlated with Prevotella, Christensenellaceae_R-7_group, and Succiniclasticum but negatively correlated with iso-butyrate, iso-valerate, and Lachnospiraceae_XPB1014_group.
Data availability statement
The datasets presented in this study can be found in online repositories. The names of the repository/repositories and accession number(s) can be found in the article/supplementary material.
Ethics statement
This animal study was reviewed and approved by the Animal Care and Use Committee of Inner Mongolia Agriculture University.
Author contributions
YZ involved in investigation, formal analysis, and writing—original draft. YmZ involved in data curation. EK involved in writing—review and editing. CB involved in supervision. CA involved in project administration and funding acquisition. All authors contributed to the article and approved the submitted version.
Funding
This work was supported by the Key Grant for Special for College of Animal Science in Inner Mongolia Agricultural University (BZCG202102) and the Scientific Research Project for Advanced Talents, Inner Mongolia Agricultural University (NDYB2018-26).
Acknowledgments
We gratefully acknowledge the support by the Fuchuan Inner Mongolia Farming Polytron Technologies, Inc., for the care of animals.
Conflict of interest
The authors declare that the research was conducted in the absence of any commercial or financial relationships that could be construed as a potential conflict of interest.
Publisher’s note
All claims expressed in this article are solely those of the authors and do not necessarily represent those of their affiliated organizations, or those of the publisher, the editors and the reviewers. Any product that may be evaluated in this article, or claim that may be made by its manufacturer, is not guaranteed or endorsed by the publisher.
Footnotes
- ^ https://github.com/OpenGene/fastp
- ^ https://ccb.jhu.edu/software/FLASH/index.shtml
- ^ https://sourceforge.net/projects/rdp-classifier/
- ^ https://www.arb-silva.de/
- ^ https://www.mothur.org/
- ^ www.majorbio.com
References
AOAC (2000). AOAC Official Methods of Analysis, 17th Edn. Washington, D.C: Association of Official Agricultural Chemists.
Apajalahti, J., Vienola, K., Raatikainen, K., Holder, V., and Moran, C. A. (2019). Conversion of branched-chain amino acids to corresponding isoacids - an in vitro tool for estimating ruminal protein degradability. Front. Vet. Sci. 6:311. doi: 10.3389/fvets.2019.00311
Aschenbach, J. R., Kristensen, N. B., Donkin, S. S., Hammon, H. M., and Penner, G. B. (2010). Gluconeogenesis in dairy cows: the secret of making sweet milk from sour dough. Iubmb Life. 62, 869–877. doi: 10.1002/iub.400
Berthelot, V., Bas, P., Schmidely, P., and Duvaux-Ponter, C. (2001). Effect of dietary propionate on intake patterns and fatty acid composition of adipose tissues in lambs. Small Rumin Res. 40, 29–39. doi: 10.1016/s0921-4488(00)00217-0
Bergman, E. N. (1990). Energy contributions of volatile fatty acids from the gastrointestinal tract in various species. Physiol. Rev. 70, 567–590. doi: 10.1007/BF00370249
Brennand, C. P., Ha, J. K., and Lindsay, R. C. (1989). Aroma properties and thresholds of some branched-chain and other minor volatile fatty acids occurring in milkfat and meat lipids. J. Sens Stud. 4, 105–120.
Calsamiglia, S., Ferret, A., and Devant, M. (2002). Effects of pH and pH fluctuations on microbial fermentation and nutrient flow from a dual-flow continuous culture system. J. Dairy Sci. 85, 574–579. doi: 10.3168/jds.S0022-0302(02)74111-8
Chaney, A. L., and Marbach, E. P. (1962). Modified reagents for determination of urea and ammonia. Clin. Chem. 8, 130–132. doi: 10.1093/clinchem/8.2.130
Chilliard, Y., Ferlay, A., Rouel, J., and Lamberet, G. (2003). A review of nutritional and physiological factors affecting goat milk lipid synthesis and lipolysis. J. Dairy Sci. 86, 1751–1770. doi: 10.3168/jds.S0022-0302(03)73761-8
Chiquette, J., Allison, M. J., and Rasmussen, M. (2012). Use of Prevotella bryantii 25A and a commercial probiotic during subacute acidosis challenge in midlactation dairy cows. J. Dairy Sci. 95, 5985–5995. doi: 10.3168/jds.2012-5511
Del Bianco, S. A., Natalello, G., Luciano, B., Valenti, L., Campidonico, V., Gkarane, F., et al. (2021). Influence of dietary inclusion of tannin extracts from mimosa, chestnut and tara on volatile compounds and flavour in lamb meat. Meat Sci. 172:108336. doi: 10.1016/j.meatsci.2020.108336
Dewulf, J. P., Gerin, I., Rider, M. H., Veiga-da-Cunha, M., Van Schaftingen, E., and Bommer, G. T. (2019). The synthesis of branched-chain fatty acids is limited by enzymatic decarboxylation of ethyl- and methylmalonyl-CoA. Biochem. J. 476, 2427–2447. doi: 10.1042/BCJ20190500
Ding, H., Liu, W., Erdene, K., Du, H., and Ao, C. (2021). Effects of dietary supplementation with Allium mongolicum Regel extracts on growth performance, serum metabolites, immune responses, antioxidant status, and meat quality of lambs. Anim. Nutr. 7, 530–538. doi: 10.1016/j.aninu.2021.04.001
Edgar, R. C. (2013). UPARSE: highly accurate OTU sequences from microbial amplicon reads. Nat. Methods. 10, 996–998. doi: 10.1038/nmeth.2604
Erwin, E. S., Marco, G. J., and Emery, E. M. (1961). Volatile fatty acid analyses of blood and rumen fluid by gas chromatography. J. Dairy Sci. 44, 1768–1771. doi: 10.3168/jds.S0022-0302(61)89956-6
Faniyi, T. O., Adegbeye, M. J., Elghandour, M., Pilego, A. B., Salem, A. Z. M., Olaniyi, T. A., et al. (2019). Role of diverse fermentative factors towards microbial community shift in ruminants. J. Appl. Microbiol. 127, 2–11. doi: 10.1111/jam.14212
Gabel, G., Aschenbach, J. R., and Muller, F. (2002). Transfer of energy substrates across the ruminal epithelium: implications and limitations. Anim. Health Res. Rev. 3, 15–30. doi: 10.1079/ahrr200237
Han, X., Yang, Y., Yan, H., Wang, X., Qu, L., and Chen, Y. (2015). Rumen bacterial diversity of 80 to 110-day-old goats using 16S rRNA sequencing. PLoS One. 10:e0117811. doi: 10.1371/journal.pone.0117811
Harlow, B. E., Flythe, M. D., and Aiken, G. E. (2018). Biochanin a improves fibre fermentation by cellulolytic bacteria. J. Appl. Microbiol. 124, 58–66. doi: 10.1111/jam.13632
Henderson, G., Cox, F., Ganesh, S., Jonker, A., Young, W., Collaborators, G. R. C., et al. (2015). Rumen microbial community composition varies with diet and host, but a core microbiome is found across a wide geographical range. Sci. Rep. 5:14567. doi: 10.1038/srep14567
Jelali, R., and Ben Salem, H. (2014). Daily and alternate day supplementation of Moringa oleifera leaf meal or soyabean meal to lambs receiving oat hay. Livest. Sci. 168, 84–88. doi: 10.1016/j.livsci.2014.07.005
Jiang, C., Ding, L., Dong, Q., Wang, X., Wei, H., Hu, C., et al. (2021). Effects of root extracts of three traditional Chinese herbs as dietary supplements on dry matter intake, average daily gain, rumen fermentation and ruminal microbiota in early weaned yak calves. Anim. Feed Sci. Technol. 278:115002. doi: 10.1016/j.anifeedsci.2021.115002
Jiao, P., Hu, G., Liang, G., Chen, M., An, N., Wang, Z., et al. (2021). Dietary supplementation with Macleaya cordata extract inclusion affects growth performance, rumen fermentation, bacterial communities, and immune responses of weaned lambs. Anim. Feed Sci. Technol. 282:115127. doi: 10.1016/j.anifeedsci.2021.115127
Kaffarnik, S., Heid, C., Kayademir, Y., Eibler, D., and Vetter, W. (2015). High enantiomeric excess of the flavor relevant 4-alkyl-branched Fatty acids in milk fat and subcutaneous adipose tissue of sheep and goat. J. Agric. Food Chem. 63, 469–475. doi: 10.1021/jf505452u
Kaffarnik, S., Kayademir, Y., Heid, C., and Vetter, W. (2014). Concentrations of volatile 4-alkyl-branched fatty acids in sheep and goat milk and dairy products. J. Food Sci. 79, C2209–C2214. doi: 10.1111/1750-3841.12673
Lane, M. A., and Jesse, B. W. (1997). Effect of volatile fatty acid infusion on development of the rumen epithelium in neonatal sheep. J. Dairy Sci. 80, 740–746. doi: 10.3168/jds.S0022-0302(97)75993-9
Li, M. Y., Guo, W. Q., Guo, G. L., Zhu, X. M., Niu, X. T., Shan, X. F., et al. (2019). Effect of sub-chronic exposure to selenium and Allium mongolicum Regel flavonoids on Channa argus: Bioaccumulation, oxidative stress, immune responses and immune-related signaling molecules. Fish Shellfish Immunol. 91, 122–129. doi: 10.1016/j.fsi.2019.05.002
Liu, S., Zhang, Z., Hailemariam, S., Zheng, N., Wang, M., Zhao, S., et al. (2020). Biochanin A inhibits ruminal nitrogen-metabolizing bacteria and alleviates the decomposition of amino acids and urea in vitro. Animals 10:368. doi: 10.3390/ani10030368
Liu, W., Ding, H., Erdene, K., Chen, R., Mu, Q., Ao, C., et al. (2019). Effects of flavonoids from Allium mongolicum Regel as a dietary additive on meat quality and composition of fatty acids related to flavor in lambs. Can. J. Anim Sci. 99, 15–23. doi: 10.1139/cjas-2018-0008
Liu, W. J., and Ao, C. J. (2021). Effect of dietary supplementation with Allium mongolicum Regel extracts on growth performance, carcass characteristics, and the fat color and flavor-related branched-chain fatty acids concentration in ram lambs. Anim Biosci. 34, 1134–1145. doi: 10.5713/ajas.20.0246
Mao, S., Zhang, M., Liu, J., and Zhu, W. (2015). Characterising the bacterial microbiota across the gastrointestinal tracts of dairy cattle: membership and potential function. Sci. Rep. 5:16116. doi: 10.1038/srep16116
Massart, A. M., Roets, E., Peeters, G., and Verbeke, R. (1983). Propionate for fatty acid synthesisby the mammary glandof the lactating goat. J. Dairy Sci. 66, 1145–1154. doi: 10.3168/jds.S0022-0302(83)81958-4
Muqier, S., Qi, T., Wang, R. W., Chen, C. F., Wang, C., and Ao, C. (2017). Effects of flavonoids from Allium Mongolicum Regel on growth performance and growth-related hormones in meat sheep. Anim Nutr. 3, 33–38. doi: 10.1016/j.aninu.2017.01.003
NRC (2007). Nutrient requirements of small ruminants: sheep, goats, cervids, and new world camelids, 6th Edn. Washington, DC: National Academies Press.
Oskoueian, E., Abdullah, N., and Oskoueian, A. (2013). Effects of flavonoids on rumen fermentation activity, methane production, and microbial population. Biomed Res. Int. 2013:349129. doi: 10.1155/2013/349129
Plaizier, J. C., Krause, D. O., Gozho, G. N., and McBride, B. W. (2008). Subacute ruminal acidosis in dairy cows: the physiological causes, incidence and consequences. Vet. J. 176, 21–31. doi: 10.1016/j.tvjl.2007.12.016
Shivani, S., Srivastava, A., Shandilya, U. K., Kale, V., and Tyagi, A. K. (2016). Dietary supplementation of Butyrivibrio fibrisolvens alters fatty acids of milk and rumen fluid in lactating goats. J. Sci. Food Agr. 96, 1716–1722. doi: 10.1002/jsfa.7277
Salles, C., Sommerer, N., Septier, C., Issanchou, S., Chabanet, C., Garem, A., et al. (2002). Goat cheese flavor sensor evaluation of branched-chain fatty acids and small peptides. J. Food Sci. 67, 835–841. doi: 10.1111/j.1365-2621.2002.tb10686.x
Scharen, M., Drong, C., Kiri, K., Riede, S., Gardener, M., Meyer, U., et al. (2017). Differential effects of monensin and a blend of essential oils on rumen microbiota composition of transition dairy cows. J. Dairy Sci. 100, 2765–2783. doi: 10.3168/jds.2016-11994
Scharen, M., Frahm, J., Kersten, S., Meyer, U., Hummel, J., Breves, G., et al. (2018). Interrelations between the rumen microbiota and production, behavioral, rumen fermentation, metabolic, and immunological attributes of dairy cows. J. Dairy Sci. 101, 4615–4637. doi: 10.3168/jds.2017-13736
Seradj, A. R., Gimeno, A., Fondevila, M., Crespo, J., Armengol, R., and Balcells, J. (2018). Effects of the citrus flavonoid extract Bioflavex or its pure components on rumen fermentation of intensively reared beef steers. Anim Prod. Sci. 58, 553–560. doi: 10.1071/an15146
Teng, F., Reis, M. G., Broadhurst, M., Lagutin, K., Samuelsson, L., Ma, Y., et al. (2020). Factors affecting levels of volatile 4-alkyl branched-chain fatty acids in sheep milk from 2 contrasting farming systems in New Zealand. J. Dairy Sci. 103, 2419–2433. doi: 10.3168/jds.2019-17192
Van Soest, P. J., Robertson, J. B., and Lewis, B. A. (1991). Methods for dietary fiber, neutral detergent fiber, and nonstarch polysaccharides in relation to animal nutrition. J. Dairy Sci. 74, 3583–3597. doi: 10.3168/jds.S0022-0302(91)78551-2
Vasta, V., Aouadi, D., Brogna, D. M., Scerra, M., Luciano, G., Priolo, A., et al. (2013). Effect of the dietary supplementation of essential oils from rosemary and artemisia on muscle fatty acids and volatile compound profiles in Barbarine lambs. Meat Sci. 95, 235–241. doi: 10.1016/j.meatsci.2012.12.021
Victoria, L., and Robert, C. L. (1993). Metabolic conjugates as precursors for characterizing flavor compounds in ruminant milks. J. Agric. Food Chem. 41, 446–454. doi: 10.1021/jf00027a01
Vlaeminck, B., Fievez, V., Cabrita, A. R. J., Fonseca, A. J. M., and Dewhurst, R. J. (2006). Factors affecting odd- and branched-chain fatty acids in milk: A review. Anim. Feed Sci. Technol. 131, 389–417. doi: 10.1016/j.anifeedsci.2006.06.017
Wallace, R. J., Arthaud, L., and Newbold, C. J. (1994). Influence of Yucca shidigera extract on ruminal ammonia concentrations and ruminal microorganisms. Appl. Environ. Microbiol. 60, 1762–1767. doi: 10.1128/aem.60.6.1762-1767.1994
Wang, W., Li, J., Zhang, H., Wang, X., Fan, J., and Zhang, X. (2019). Phenolic compounds and bioactivity evaluation of aqueous and methanol extracts of Allium mongolicum Regel. Food Sci. Nutr. 7, 779–787. doi: 10.1002/fsn3.926
Wang, Y., Nan, X., Zhao, Y., Jiang, L., Wang, H., Hua, D., et al. (2021). Dietary supplementation with inulin improves lactation performance and serum lipids by regulating the rumen microbiome and metabolome in dairy cows. Anim. Nutr. 7, 1189–1204. doi: 10.1016/j.aninu.2021.09.007
Wong, E., Nixon, L. N., and Johnson, C. B. (1975). Volatile medium chain fatty acids and mutton flavor. J. Agric. Food Chem. 23, 495–498. doi: 10.1021/jf60199a044
Zhao, Y. X., Erdene, K., Changjin, A., Zhibi, B., Hongxi, D., Zejun, F., et al. (2021). Effects of Allium mongolicum Regel and its extracts supplementation on the growth performance, carcass parameters and meat quality of sheep. Ital J. Anim Sci. 20, 1899–1908. doi: 10.1080/1828051x.2021.1971572
Young, O. A., Lane, G. A., Priolo, A., and Fraser, K. (2003). Pastoral and species flavour in lambs raised on pasture, lucerne or maize. J. Sci. Food Agr. 83, 93–104. doi: 10.1002/jsfa.1282
Yuste, S., Amanzougarene, Z., de la Fuente, G., Fondevila, M., and de Vega, A. (2020). Effects of partial substitution of barley with maize and sugar beet pulp on growth performance, rumen fermentation and microbial diversity shift of beef calves during transition from a milk and pasture regimen to a high-concentrate diet. Livest. Sci. 238:104071. doi: 10.1016/j.livsci.2020.104071
Keywords: Allium mongolicum Regel ethanol extract, branched-chain fatty acids, mutton flavor, rumen bacteria, lamb
Citation: Zhao Y, Zhang Y, Khas E, Ao C and Bai C (2022) Effects of Allium mongolicum Regel ethanol extract on three flavor-related rumen branched-chain fatty acids, rumen fermentation and rumen bacteria in lambs. Front. Microbiol. 13:978057. doi: 10.3389/fmicb.2022.978057
Received: 25 June 2022; Accepted: 31 August 2022;
Published: 15 September 2022.
Edited by:
Zhixiong He, Key Laboratory of Agro-Ecological Processes in Subtropical Region, Institute of Subtropical Agriculture (CAS), ChinaReviewed by:
Grzegorz Bełńecki, Kielanowski Institute of Animal Physiology and Nutrition (PAN), PolandChong Li, Gansu Agricultural University, China
Copyright © 2022 Zhao, Zhang, Khas, Ao and Bai. This is an open-access article distributed under the terms of the Creative Commons Attribution License (CC BY). The use, distribution or reproduction in other forums is permitted, provided the original author(s) and the copyright owner(s) are credited and that the original publication in this journal is cited, in accordance with accepted academic practice. No use, distribution or reproduction is permitted which does not comply with these terms.
*Correspondence: Changjin Ao, Y2hhbmdqaW5hb0BhbGl5dW4uY29t; Chen Bai, b3N0cmljaDIwMTJAMTYzLmNvbQ==