- 1Hainan University, Haikou, Hainan, China
- 2Tropical Crops Genetic Resources Institute, Chinese Academy of Tropical Agricultural Sciences, Haikou, Hainan, China
In order to better understand the effect of wilting treatment on silage, we study analyzed the fermentation quality of unwilted (CK) and wilted (WT) king grass silage, and the dynamic changes of microorganisms in silage and aerobic exposure. After 30 days of silage, WT silage significantly reduced the pH of the silage (p < 0.05) and increased the contents of lactic acid and acetic acid (p < 0.05), but did not reduce the content of Ammonia-N (p > 0.05). Wilting treatment increased bacterial and fungal diversity during silage but decreased fungal diversity during aerobic exposure. The relative abundance of Lactococcus and Enterococcus in wilting silage increased. In the aerobic exposure stage, the relative abundance of Klebsiella decreased, but the relative abundance of Enterobacter increased in wilting treatment silage. In addition, the relative abundance of Acinetobacter and Ignatzschineria increased after 5 days of aerobic exposure. In contrast with unwilted silage, wilting treatment silage after aerobic exposure had no Candida, but the relative abundance of Wickerhamomyces increased. The results showed that wilting treatment could raise the silage quality of king grass. However, WT silage did not inhibit the reproduction of harmful microorganisms during aerobic exposure and did not significantly improve the aerobic stability of silage.
Introduction
King grass (Pennisetum purpureum Schumacher × Penicillium glaucum (Linnaeus) R. Brown) is a kind of high-quality grass, which is suitable for tropical and subtropical climates and likes warm and humid climates (Zhao et al., 2019). King grass is a nutritionally rich and high-yielding forage grass, which can be harvested 5–8 times per year for 75–180 t, which makes it an important animal feed resource (Botero-Londono et al., 2021; Long et al., 2022). In addition, king grass can absorb heavy metal ions from soil to purify soil (Li et al., 2021), which is rich in lignocellulosic biomass and can also be used to produce ethanol (Cardona et al., 2016). The growth of king grass is seasonal. It grows exuberant in the summertime or rainy season, but it grows slowly and has a low yield in winter or dry season, which is easy to leads to the imbalance between supply and demand (Li et al., 2014). According to the growth characteristics of king grass, using appropriate methods to store it during the bloom season can better provide a sustainable supply for ruminants. Ensiling is a common method for long-term storage of forage to improve feed palatability (Zhang et al., 2019a). The fermentation quality of silage quality is closely related to the nature of raw materials and the succession of microbial communities (Zi et al., 2021). After aerobic exposure, some harmful aerobic microorganisms such as yeasts and molds in the silage will multiply rapidly with the increase in temperature and pH, leading to the deterioration of the silage (Da Silva et al., 2015). Zhang et al. (2019a) reported the dynamic changes of microorganisms during sugarcane silage and after aerobic exposure. It is important to understand fully these changes for regulating the quality of silage fermentation. At present, the research on king grass silage mainly focuses on the mixed fermentation with other forages and the effects of adding starter on its silage quality and bacterial community (Li et al., 2014, 2019b; Zhang et al., 2018). However, there are no reports on the dynamic changes of microorganisms during the silage of king grass, and the microbial succession in the silage process is still unclear. Therefore, it is important to study the microbial dynamic changes during king grass silage and aerobic exposure to control the quality of king grass silage.
The fermentation quality of silage is influenced by many factors, among which raw material moisture and soluble carbohydrate content are important factors (Borreani et al., 2018). When the moisture content of the raw materials is too high, the growth of harmful microorganisms during silage is promoted, thereby reducing silage quality (Zhang et al., 2019b). Wilting is a conventional method to reduce the water content of raw materials. It has been shown that wilting treatment can inhibit the propagation of undesirable microorganisms and improves the quality of silage (Liu et al., 2011; Nishino et al., 2012; Wang et al., 2018). Guo et al. (2021) study showed that the relative abundance of Lactobacillus increased, while the relative abundances of Enterobacter and Pseudomonas decreased in paper mulberry silage after wilting treatment, and the results indicated that wilting treatment could improve paper mulberry silage quality. However, the quality of direct silage of king grass is not ideal because the dry matter content and soluble carbohydrates were low (Li et al., 2014; Zi et al., 2021). At present, there have been no reports on the microbial changes of wilted king grass silage. In addition, the effect of wilting on the quality, bacterial community, and fungal community of king grass silage is not clear.
The purpose of this study was to study the dynamic changes of bacterial and fungal communities of unwilted and wilted king grass during silage and aerobic exposure. It provides a theoretical basis for the structure of the king grass microbial strain bank and better improves the quality of king grass silage.
Materials and methods
Silage preparation
King grass (Reyan No. 4) was planted in the experimental base of the Chinese Academy of Tropical Agricultural Sciences (109°58′E, 19°52′N). Harvest the half-a-year vegetative king grass with strong growth and no diseases and pests as the experimental raw material. Select the period time without precipitation for 7 consecutive days for the sample, and the sampling time is the afternoon of November 2, 2020. All samples were taken from the same test field. After sampling, immediately put it into a sterile bag and take it back to the laboratory. The king grass is cut into small pieces of about 2 cm by a grass chopper (Donghong No. 1, Donghong Mechanical Equipment Co., Ltd., China). King grass was evenly divided into two parts, a part of king grass is silage directly without wilting treatment (CK). Another part of king grass is wilted until the water content is 63% (Wither at 30°C for 12 h), and then silage (WT). Before silage, a portion of each of the two groups was used for microbial diversity analysis. The unwilted and wilted king grass are packed in vacuum packaging bags (30 cm × 20 cm; Guo Zhong Packaging Co., Ltd., Haikou, China) according to 200 g per bag. The vacuum packaging machine (Xiangshan brand intelligent vacuum packaging machine; Xiangshan Tea Machinery Factory, Anxi County, Fujian Province, China) vacuums and stores it at a normal temperature of 30°C. A total of 60 bags (two treatment groups × six silage times × four aerobic exposure times × three repetitions). Samples were taken on days 1, 3, 5, 7, 14, and 30 of the fermentation process, and 1, 3, 5, and 7 after aerobic exposure. A part of the sample was used for microbial diversity analysis and a part of the sample was used for organic acid, pH, and Ammonia-N determination.
Silage fermentation analysis
Place 25 g of the sample into a 250 ml volumetric flask containing 225 ml of sterile water and shake at 30°C for 2 h. After preliminary treatment, 40 ml of the samples were placed in 50 ml centrifuge tubes and centrifuged at 4°C, 10,000 × g for 15 min (Dong et al., 2020; Zi et al., 2021). After centrifugation, the supernatant was retained and the pH was measured using a pH meter (Starter 2,100; Ohaus Instruments Co. Ltd., Shanghai, China), organic acids (lactic acid, acetic acid, propionic acid, and butyric acid), and ammonia nitrogen determination, and the precipitate was retained at −80°C for microbial diversity analysis. The retained supernatant was filtered through a 0.22 μM filtration one part was assayed for pH with a pH glass electrode pH meter and one part was analyzed for lactic, acetic, propionic, butyric acid using an Agilent 1,200 HPLC (column: Athena C18-WP; eluent: 3 mM HClO4; flow rate:1 ml/min; temperature: 45°C; UVD:210 nm). Determination of ammonia-N content using a phenol-hypo-chlorite colorimetric method (Broderick and Kang, 1980).
King grass silage microbial analysis
DNA extraction
Unwilted and wilted raw materials and silage from each ensiling period and aerobic exposure period were used for microbial community analysis. Briefly, 40 ml to 50 ml centrifuge tubes were taken from each bag of the treated filtrate, centrifuged at 10,000 r/m for 10 min at 4°C to remove the supernatant, and the precipitate was collected. Microbial extraction is carried out with reference to Zi et al. (2021) method.
PCR amplification and sequencing
The V3–V4 hypervariable region of bacterial 16S rRNA was amplified by primers 338F (5′-ACTCCTACGGGAGGCAGCAG-3′) and 806R (5′-GGACTACHVGGGTWTCTAAT-3′). Bacterial 16S amplification was performed as described by Tian et al. (2017). Fungal ITS amplicon sequencing used BITS (5′-NNNNNNNNCTACCTGCGGARGGA-TCA-3′) and B58S3 (5′-GAGATCCRTTGYTRAAAGTT-3′) universal primers. Fungal ITS-1 amplification was conducted according to the description of Romero et al. (2017). Illumina Miseq 2500 platform (Illumina, Inc., San Diego, CA, United States) was used for sequencing. UCHIME algorithm is used to obtain valid data. Calculate Shannon, Chao1, ACE, and Good coverage by QIIME (version 2.15.3). The sequencing data of this study has been submitted to the NCBI database, and the Bioproject registration number is PRJNA76755.
Statistical analysis
The SPSS2016 software was used to conduct a one-way analysis of variance (ANOVA) of each index of king grass silage, and the general linear model (GLM) was used to conduct a two-way analysis of variance on treatment method, silage duration, and their interaction. Comparisons at the 5% significance level were made using multiple comparison tests of Duncan. The online platform (Illumina hiseq sequencing platform) was used to analyze sequencing data for the bacterial and fungal communities.
Results
Fermentation profiles of king grass silage during ensiling and aerobic exposure
The fermentation quality of CK silage and WT silage are shown in Table 1. The pH value of silage decreased gradually with the prolonged silage time. The contents of lactic acid and acetic acid increased and decreased during silage, but showed an overall increasing trend. Propionic acid and butyric acid were not detected in CK silage and WT silage. After 30 days of silage, WT silage exhibited significantly lower pH than CK silage (p<0.01). After 30 days of silage, the contents of lactic and acetic acids in WT silage were significantly higher than in CK silage (p < 0.01). In addition, the present study found that ammonia nitrogen (Ammonia-N) content was low in king grass silage. After 30 days of silage, there was no notable difference in ammonia-N content between CK silage and WT silage (p > 0.05).
As shown in Table 2, although the pH of king grass silage increased with the extension of aerobic exposure time, the pH of the WT silage was significantly lower than that of the CK silage (P<0.01). In addition, the pH values of CK silage and WT silage increased slowly 3 days before aerobic exposure. However, after being exposed to the air for 5 days, the pH increased rapidly. After being exposed to the air for 7 days, the content of lactic acid in WT silage was prominent higher than in CK silage (p < 0.01), while the content of acetic acid was significantly lower than in CK silage (p < 0.01). After being exposed to the air for 7 days, the Ammonia-N content in CK silage was prominent higher than that in WT silage (p < 0.01).
Bacterial diversity of king grass silage during silage and aerobic exposure
Table 3 is Alpha diversity of bacteria in king grass ensiling process and after exposure to air. As presented in Table 3, Good’s coverage value was about 1, which illustrated that the sample assay covered most of the bacteria, reflecting the real situation of the bacteria in the sample. It can be seen from the indexes of Outs and Chao 1 that with the extension of silage time, the bacterial colony richness in CK silage and WT silage is relatively stable and has no obvious change. Compared with CK silage, the bacterial community richness and diversity of WT silage were lower than CK silage.
Under aerobic exposure, although the bacterial colony richness in CK silage and WT silage decreased with time, the bacterial richness of CK silage was lower than that of WT silage after being exposed to the air for 7 days. Aerobic exposure of silage on days 3 and 5, the Shannon index was higher in WT silage than in CK silage. However, on day 7 of exposure to air, the Shannon index of WT silage was noteworthy lower than CK silage.
Bacterial community dynamics during king grass silage and aerobic exposure
The bacterial dynamic changes during the king grass ensiling period and aerobic exposure are presented in Figure 1. Where Figures 1A,B are the bacterial community dynamics at the phylum level. Before silage, the dominant bacteria in king grass were mainly Proteobacteria and Firmicutes. Compared with unwilted, after wilted treatment, the relative abundance of Proteobacteria (70.56 ~ 77.19%) increased, while that of Firmicutes (28.22 ~ 17.96%) decreased. The relative abundance of Cyanobacteria was higher in CK silage (6.99 and 3.21%, respectively) on days 7 and 14 of ensiling in contrast with WT silage (0.63 and 0.17%, respectively). In addition, Bacteroidetes (10.21%) and Actinobacteria (2.11%) were more abundant in CK silage on the 7 day of ensiling. On day 30 of ensiling, the relative abundance of Proteobacteria in WT silage (78.36%) was lower than that of CK silage (89.73%), but the relative abundance of Firmicutes was higher in WT silage (18.87%) than in CK silage (7.61%). Proteobacteria remain the dominant phylum in CK silage and WT silage aerobic exposure. The relative abundance of Proteobacteria increased, while that of Firmicutes decreased in CK silage and WT silage 5 days before aerobic exposure. However, on day 7 of exposure to air, the relative abundance of Proteobacteria in CK silage and WT silage decreased, while the relative abundance of Firmicutes increased. Totality, Proteobacteria and Firmicutes were the two phyla with the highest relative abundance in king grass silage at any time of ensiling and aerobic exposure.
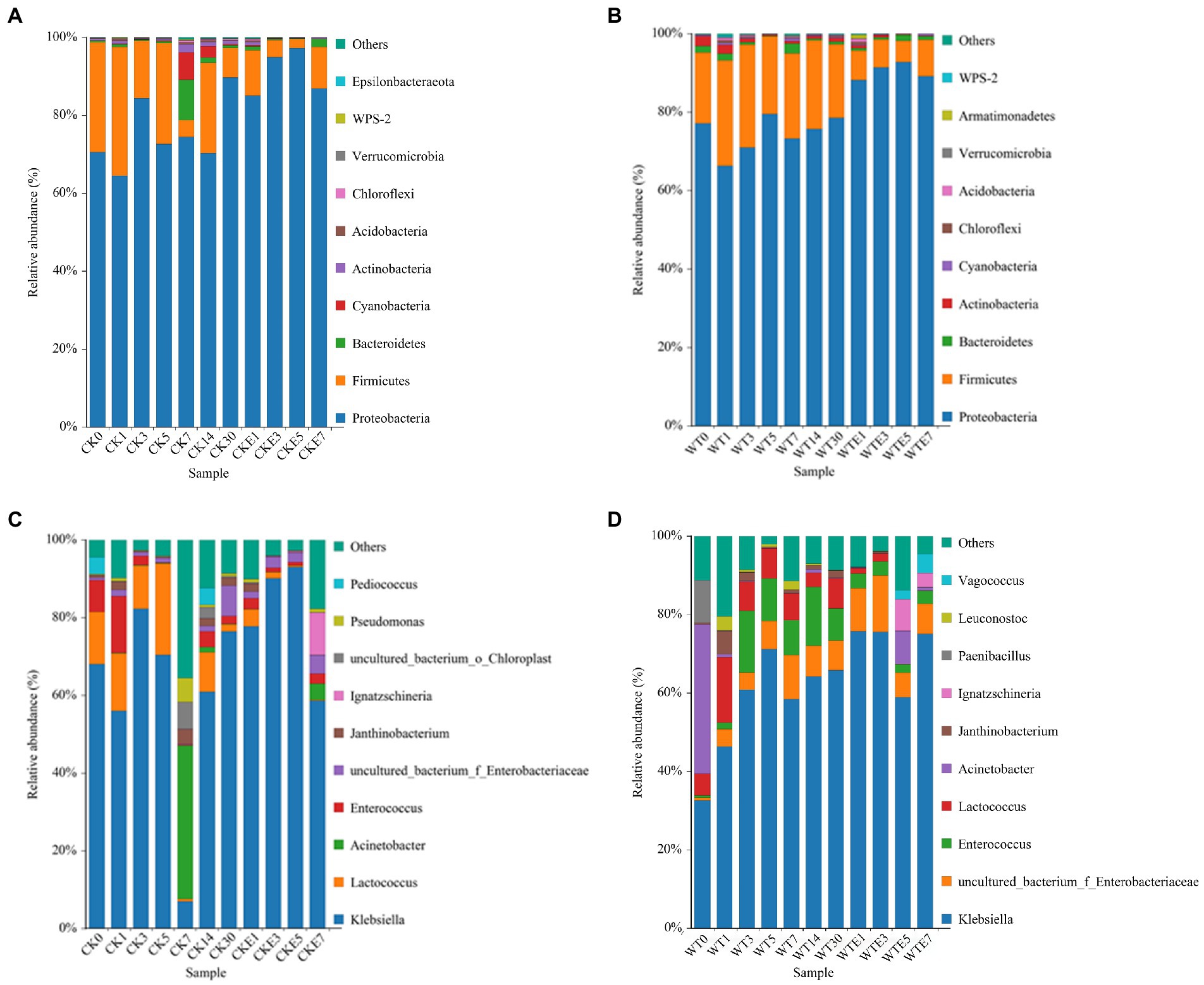
Figure 1. Relative abundance of bacterial community during ensiling and aerobic exposure of King grass silage. (A,C) show the relative abundance at phylum and genus level for CK, respectively, while (B,D) show the relative abundance at phylum and genus level for WT, respectively. CK, unwilted silage; CKE, unwilted silage aerobic exposure; WT, wilting silage; WTE, wilting silage aerobic exposure; Arabic numerals indicate the time of silage.
Figures 1C,D. show the dynamic changes of bacterial genus level. In this study, lactic acid bacteria present in both unwilted and wilted king grass before silage were mainly Lactococcus and Enterococcus. In addition, Pediococcus was present in unwilted king grass, whereas Leuconostoc was present in wilting treatment king grass. During the ensiling, regardless of CK silage (68.02% ~ 76.43%) or WT silage (32.55% ~ 65.78%), Klebsiella was the dominant bacterial genus during the silage of king grass. In contrast with CK silage, on the 3 and 5 days of ensiling, the relative abundance of Lactococcus was lower and that of Enterococcus was higher in the WT silage. On days 5 to 7 of silage, the relative abundance of Klebsiella decreased from 70.38 to 6.99%, the relative abundance of Lactococcus decreased from 23.54 to 0.63%, and the relative abundance of Acinetobacter increased from 0.17 to 39.51% in CK silage. WT silage (7.67 and 8.19%, respectively) showed higher abundances of Lactococcus and Enterococcus than CK silage (1.83 and 2.03%, respectively) at day 30 of ensiling. In addition, after 30 days of ensiled, Lactococcus and Enterobacter were the most different species between direct silage and withered silage (Figure 2). The relative abundance of Enterobacteriaceae in CK silage (7.87%) and WT silage (7.57%) did not change much after 30 days of ensiled. After aerobic exposure, Klebsiella remained the dominant genus in both CK silage and WT silage. On day 1 of aerobic exposure, the relative abundance of Lactococcus increased from 2.03 to 2.78% and that of Enterococcus increased from 1.83 to 4.39% in CK silage. In contrast, the relative abundance of Lactococcus decreased from 7.67 to 1.36% and the relative abundance of Enterococcus decreased from 8.19 to 3.69% in WT silages on day 1 of aerobic exposure. Lactococcus abundance decreased rapidly in both CK silage and WT silage from days 3 to 7 of aerobic exposure. The relative abundance of Lactococcus decreased from 4.39 to 0.19% in the CK silage and from 2 to 0.09% in the WT silage. The abundance of Enterobacteriaceae in WT silage increased from 7.58 to 14.37% on days 1 to 3 of aerobic exposure. The relative abundance of Acinetobacter increased in silage after being exposed to the air for 5 days. On days 5 and 7 of aerobic exposure, the relative abundance of Acinetobacter in CK silage and WT silage was significantly different (Figure 2).
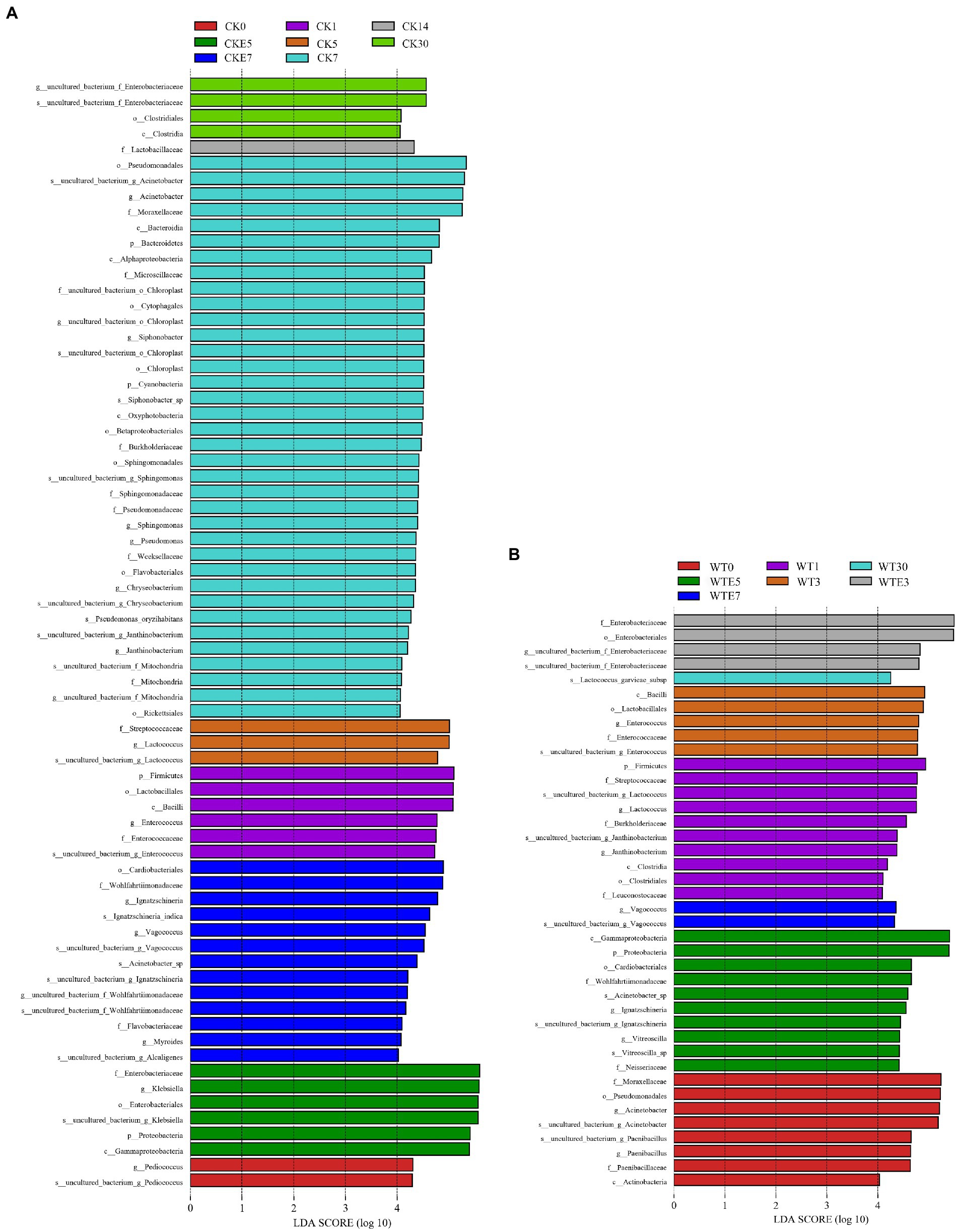
Figure 2. Comparison of bacterial changes during ensiling and aerobic exposure using LEfSe analysis. (A, CK group; B, WT group; CK, unwilted silage; CKE, unwilted silage aerobic exposure; WT, Wilting silage; WTE, Wilting silage aerobic exposure; Arabic numerals indicate the time of silage).
Fungal diversity of king grass silage during silage and aerobic exposure
Table 4 is Alpha diversity of fungal in king grass ensiling process and after exposure to air. It can be seen from Table 4 that the CK silage Chao1 and ACE indicators showed a decreasing trend during days 1–30 of ensiling, while WT silage Chao1 and ACE indicators presented an increasing trend. After 30 days of silage, the Chao1, ACE, and Shannon indices were higher in WT silage than in CK silage, indicating that wilt treatment increased the king grass fungal richness and diversity.
After aerobic exposure, the Chao1 and ACE indexes in the CK silage showed an increasing trend, while those in the WT silage showed a decreasing trend, indicating that the relative abundance of fungi decreased in the WT silage with aerobic exposure. After being exposed to the air for 7 days, fungal diversity was lower in WT dried silage than in CK silage.
Fungal community dynamics during king grass silage and aerobic exposure
The fungal dynamic changes during the king grass ensiling period and aerobic exposure are presented in Figure 3. Where Figures 3A,B are the fungal community dynamics at the phylum level. Before silage, the main fungal phyla in unwilted king grass and wilted king grass are Ascomycota, Basidiomycota and Mortierellomycota. In addition, Figure 4 shows that there is a significant difference in the relative abundance of Basidiomycota between CK silage and WT silage. Ascomycota relative abundance was decreased while Basidiomycota relative abundance was increased in wilting (50.84 and 41.01%, respectively) king grass compared to unwilted (65.04 and 20.22%, respectively) king grass. Ascomycota and Basidiomycota were both the dominant phyla in CK silage and WT silage during ensiling. Ascomycota and Basidiomycota remained the dominant phyla in CK silage and WT silage after aerobic exposure. After being exposed to the air, the relative abundance of Ascomycota began to increase. After 7 days of aerobic exposure, the relative abundance of Ascomycota in CK silage increased from 67.61 to 85.58%, and that in WT silage increased from 62.5 to 82.22%.
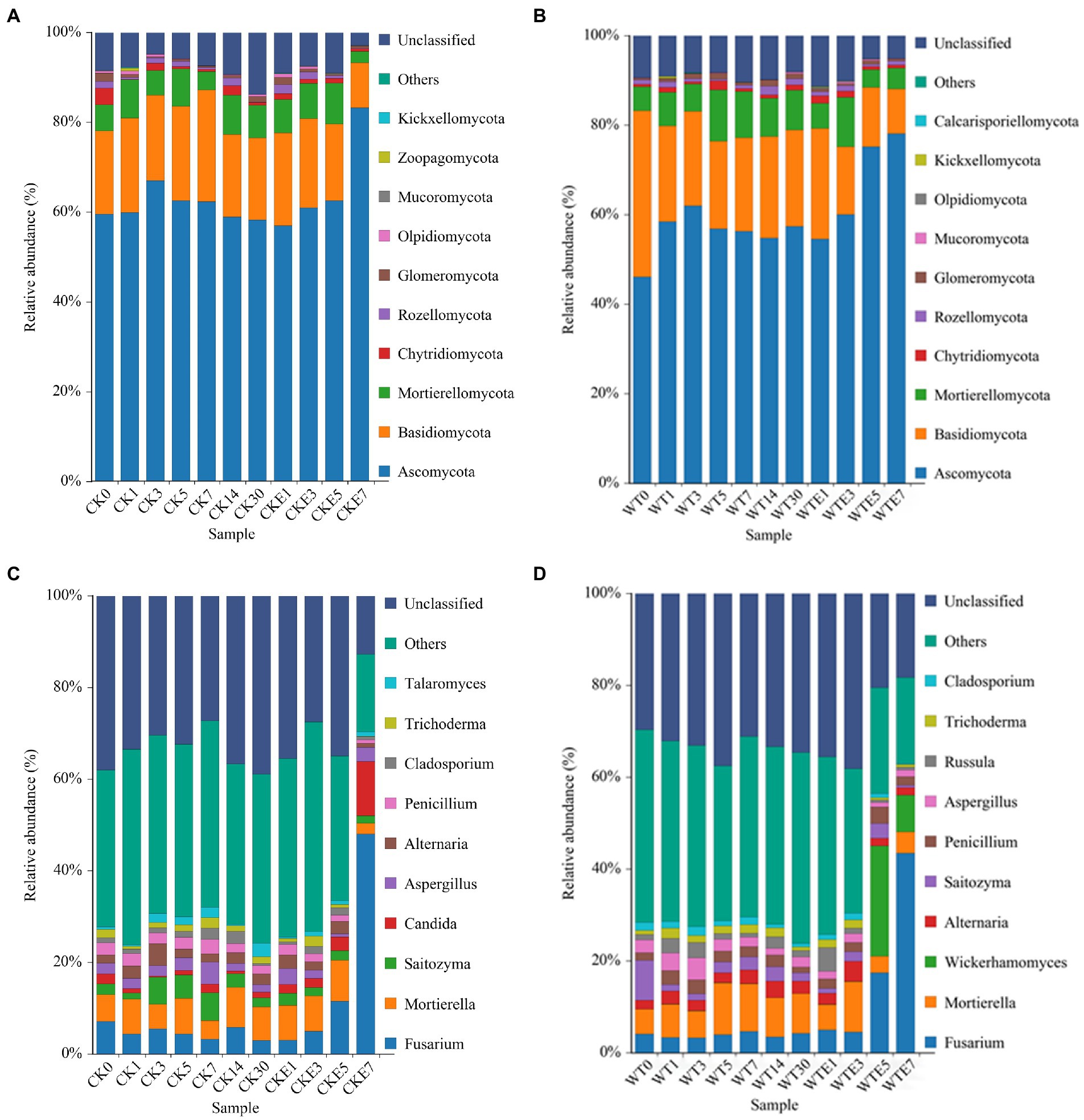
Figure 3. Relative abundance of fungal community during ensiling and aerobic exposure of King grass silage. (A,C) show the relative abundance at phylum and genus level for CK, respectively, while (B,D) show the relative abundance at phylum and genus level for WT, respectively. CK, unwilted silage; CKE, unwilted silage aerobic exposure; WT, wilting silage; WTE, wilting silage aerobic exposure; Arabic numerals indicate the time of silage.
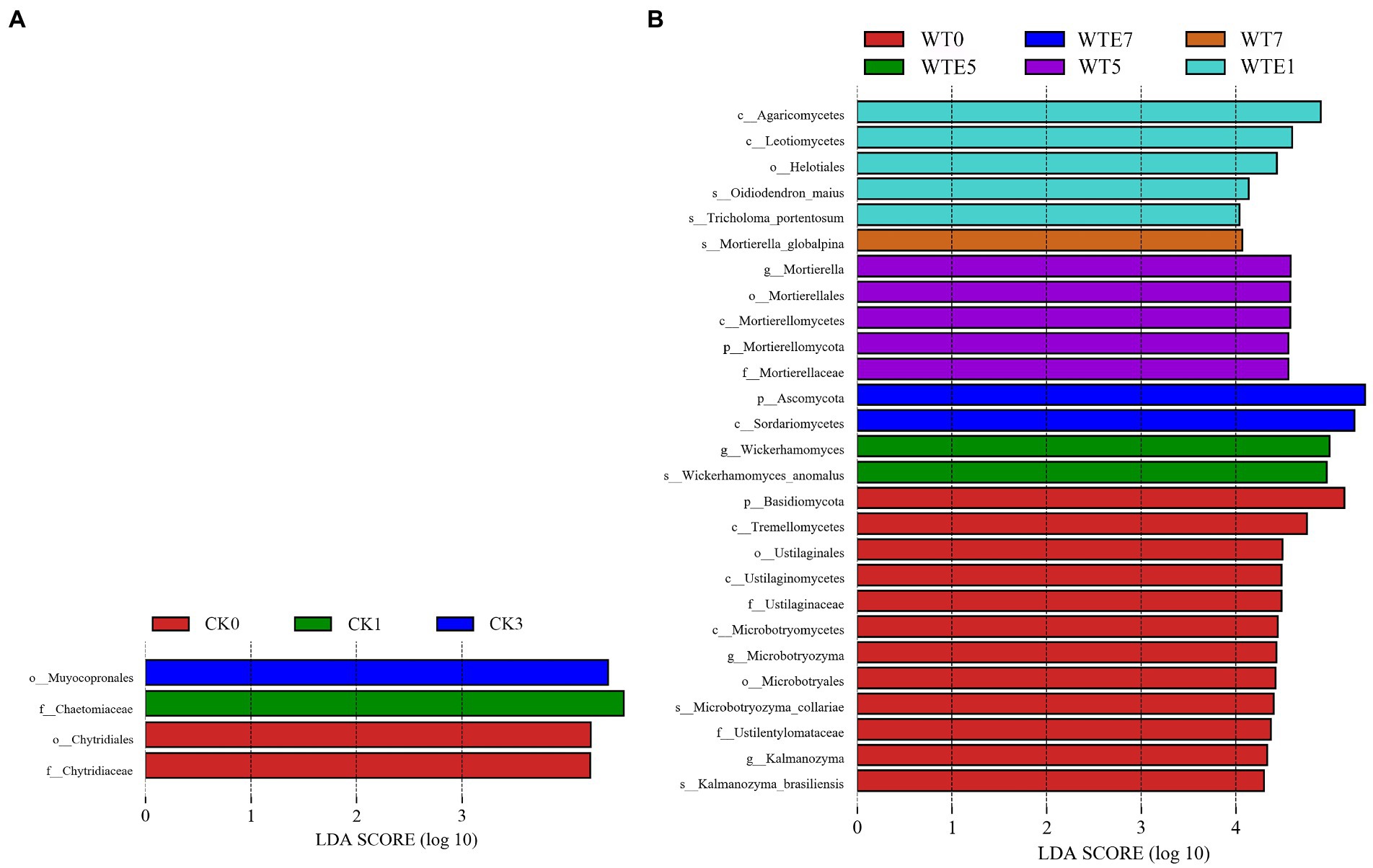
Figure 4. Comparison of fungal changes during ensiling and aerobic exposure using LEfSe analysis. (A, CK group; B, WT group; CK, unwilted silage; CKE, unwilted silage aerobic exposure; WT, wilting silage; WTE, wilting silage aerobic exposure; Arabic numerals indicate the time of silage.)
Figures 3C,D show the dynamic changes of the fungal genus level. Fusarium, Mortierella, Aspergillus, Penicillium, Saitozyma, and Alternaria were found in CK silage and WT silage during ensiling. In addition, Candida was also identified in CK silage (2.1%), however, there was no Candida in the WT silage. The relative abundance of Wickerhamomyces in WT silage increased to 24.09% on day 5 of aerobic exposure, but decreased to 7.98% on day 7 of aerobic exposure. Wickerhamomyces were not detected in CK silage. After exposure to air, the richness of the Fusarium in CK silage and WT silage was raised. On the 7th day of aerobic exposure, the relative abundance of Fusarium in CK silage increased from 2.94 to 48.03%, and that in WT silage increased from 4.2 to 43.48%. After exposure to air for 7 days, the relative abundance of Candida in CK silage increased from 1.27 to 11.92%. After 7 days of aerobic exposure, the relative abundance of Penicillium in CK silage decreased from 1.85 to 0.77%, while the relative abundance of Penicillium in WT silage increased from 1.3 to 1.84%.
Discussion
In this study, wilting treatment reduced silage pH, raised the contents of lactic acid and acetic acid, and improved silage fermentation quality. pH and organic acid are important indexes to evaluate silage quality. In contrast with CK silage, after 30 days of silage, the pH of WT silage is lower, and the contents of lactic acid and acetic acid are higher, which indicates that the quality of WT silage is better. Besides, the content of acetic acid in king grass silage was higher than that of lactic acid, indicating that king grass silage was a combination of lactic acid fermentation and acetic acid fermentation, but acetic acid fermentation was dominant, which may also be the reason for the high pH of silage. Studies have shown that acetic acid is the main organic acid in tropical forages (Nishino et al., 2012; Li et al., 2019a). In addition, high concentrations of lactic acid and acetic acid contribute to silage fermentation, which may be because high concentrations of lactic acid and acetic acid inhibit the multiplication of unwelcome microorganisms. Ammonia-N is the product of protein hydrolysis and an important index for assessing the quality of silage (Li et al., 2018). The protein in silage will be hydrolyzed into Ammonia-N and other substances, reducing the absorption and utilization of protein by ruminants (Kung et al., 2018). It was found that there was no notable difference in Ammonia-N content between CK silage and WT silage, indicating that wilting treatment did not affect the hydrolytic activity of the protease. After 5 days of aerobic exposure, the pH in the feed increased rapidly, indicating that the aerobic spoilage of silage was aggravated. Combined with the increase in the relative abundance of yeasts and molds after 5 days of aerobic exposure in Figure 3, it shows that wilting does not improve the aerobic stability of king grass silage.
During silage and aerobic exposure, Proteobacteria and Firmicum are the two phyla with high relative abundance, which is the same as other people’s research (Hu et al., 2018; Zhang et al., 2019a). On day 7 of silage, the richness of Bacteroidetes and Actinobacteria increased suddenly. The reason for this phenomenon is difficult to explain, so we need to conduct more in-depth research. Zi et al. (2021) showed that after 30 days of silage, the relative abundance of Firmicutes in king grass silage was higher than that of Proteobacteria, which was just the opposite in this study. It has been reported that with the increase in silage time, the dominant phylum will change from Proteobacteria to Firmicutes (Xu et al., 2017; Yuan et al., 2019). However, this transformation needs to be carried out in a low pH and anaerobic environment (Dong et al., 2019). In this study, the higher pH (> 4.3) of king grass silage, resulted in no shift from Proteobacteria to Firmicutes. This is consistent with studies by others (Long et al., 2022). After exposure to air, the relative abundance of Proteobacteria raised and that of Firmicutes decreased, which is consistent with the research of others (McGarvey et al., 2013; Liu et al., 2019). Lactic acid bacteria related to silage mainly include Lactobacillus, Lactococcus, Pediococcus, Leuconostoc, Enterococcus, etc. (Tohno et al., 2012). Lactococcus plays a key role in silage and has been widely used at present (Ni et al., 2018). Lactococcus begin to produce lactic acid early in silage, decreasing the pH of silage and providing an acidic environment for the growth of other lactic acid bacteria. Most studies showed that during the late stage of silage, Lactococcus would be replaced by Lactobacilli with better acid tolerance properties (Keshri et al., 2018; Yuan et al., 2019). Yang et al. (2016) research that Lactobacillus was the dominant species in fruit residue, could survive at a lower acid base, and produced more lactic acid. But in this study, after 30 days of silage, there was no Lactobacillus in silage and Lactococcus was still the dominant genus. The possible reason was that the pH of silage was higher, Lactococcus could still survive and play a significant role. Besides, Enterococcus, Pediococcus, and Leuconostoc also play a significant role in the early silage. Parvin et al. (2010) discover that Enterococcus existed in the wilted guinea grass silage compared to direct silage. Moreover, Ni et al. (2017) also reported that Enterococcus in withered alfalfa silage is the dominant genus. In this research, the richness of Enterococcus raised in silage after wilting treatment, is consistent with previous studies. Klebsiella is a facultative anaerobic bacterium and a harmful microorganism in silage (Lin et al., 2021). Klebsiella has been found in many silages, such as corn, tea, mulberry leaves, etc. (Hu et al., 2018; Wang et al., 2020; Lin et al., 2021). Studies have shown that pH < 4 can inhibit the growth of Klebsiella (Junges et al., 2017). In this study, Klebsiella has always been the dominant bacteria, which may be related to the pH > 4.3 of king grass silage, and too high pH not inhibit its growth. Enterobacteriaceae bacteria are generally undesirable bacteria in silage, which ferment lactic acid into acetic acid and other products (Santos et al., 2016). It has been shown that the occurrence of Enterobacteriaceae is associated with an acetic acid content, and the relative abundance of Enterobacteriaceae tends to be higher in silages with high acetic acid content (Nishino et al., 2012; Li et al., 2019a). This research further confirms this view. Acinetobacter is an aerobic bacterium that can be detected in diverse surroundings (Kampfer and Glaeser, 2012). Studies have shown that Acinetobacter does not exist in silage (Li and Nishino, 2011). However, Ogunade et al. (2017) found the presence of Acinetobacter in silage after 120 days of ECLB silage and speculated that the raised relative abundance of Acinetobacter may be related to the raised acetic acid concentration. It has been shown that some Acinetobacter can survive when acetate is sufficient in the surrounding environment (Fuhs and Chen, 1975). In this study, given the increased relative abundance of Acinetobacter and higher-level WT of acetic acid in silage on day 7 of CK silage and WT silage, we speculate that a proportion of Acinetobacter species utilize acetic acid and thus survive the anaerobic phase of silage.
Ascomycota and Basidiomycota are the most abundant fungal phyla during silage and aerobic exposure, which is the same as other people’s research (Romero et al., 2017; Zhang et al., 2019a). Fusarium, Aspergillus, and Penicillium are common undesirable molds (Vu et al., 2019). Fusarium can produce a variety of mycotoxins, which affect the feeding safety of silages (Niderkorn et al., 2007). Myllykoski et al. (2009) reported that beef cattle cause their jejuna hemorrhagic syndrome after consuming a diet containing various mycotoxin-producing species including Fusarium, Aspergillus, and Penicillium. Yeast propagates rapidly after aerobic exposure, resulting in silage corruption. The most common yeast genera involved in silage aerobic spoilage are Pichia, Candida, and Issatchenkia (Liu et al., 2019; Zhang et al., 2019a). Yeasts consume sugars and organic acids, resulting in increased silage pH and temperature, extensive growth of other aerobic microorganisms, and finally complete deterioration of the silage by molds (Borreani and Tabacco, 2010). In the present study, the presence of Candida was detected only after being exposed to the air for 5 days from CK silage. Candida is a lactic acid assimilating yeast that raised aerobic spoilage of silage (Bai et al., 2020). Besides, the presence of Wickerhamomyces was detected after being exposed to the air for 5 days in WT silage, but fewer studies are currently available on Wickerhamomyces in silage. We speculate that Wickerhamomyces promotes the aerobic deterioration of king grass silage. In addition, Fusarium grew abundantly in both CK and WT silages after 5 days of aerobic exposure. The above results indicated that wilting could not inhibit the growth of harmful microorganisms after aerobic exposure, indicating that wilting did not improve the aerobic stability of king grass silage.
Conclusion
The findings show that the wilting treatment significantly raised the content of lactic acid and acetic acid, and lowered the pH. The wilting treatment increased the bacterial and fungal diversity of silage. Besides, the wilting treatment increased the relative abundance of Lactococcus and Enterococcus in king grass silage. But the wilting treatment did not raise the aerobic stability of silage. After exposure to air, the relative abundance of Lactococcus decreased and that of Acinetobacter and Ignatzschineria increased. After being exposed to the air for 7 days, CK silage and WT ensiling fungal colony were dominated by Fusarium. Candida and Wickerhamomyces belong to the Saccharomyces and are important microorganisms in silage deterioration upon aerobic exposure.
Data availability statement
The datasets presented in this study can be found in online repositories. The names of the repository/repositories and accession number(s) can be found at: https://submit.ncbi.nlm.nih.gov/PRJNA767755.
Author contributions
RC, ML, and XZ carried out the experimental design. RC, ML, LC, and XZ performed the experiments. RC, ML, LC, JY, XZ, JT, and HZ analyzed the experimental data. RC wrote the first draft. ML and XZ revised the manuscript. All authors contributed to the article and approved the submitted version.
Funding
This study was funded by the National Natural Science Foundation of China (Nos. 31860680 and 31960678), the Key Research and Development Program of Hainan Province (ZDYF2022XDNY153), and the Central Public-Interest Scientific Institution Basal Research Fund for Chinese Academy of Tropical Agricultural Sciences (1630032022011).
Conflict of interest
The authors declare that the research was conducted in the absence of any commercial or financial relationships that could be construed as a potential conflict of interest.
Publisher’s note
All claims expressed in this article are solely those of the authors and do not necessarily represent those of their affiliated organizations, or those of the publisher, the editors and the reviewers. Any product that may be evaluated in this article, or claim that may be made by its manufacturer, is not guaranteed or endorsed by the publisher.
References
Bai, J., Xu, D., Xie, D., Wang, M., Li, Z., and Guo, X. (2020). Effects of antibacterial peptide-producing Bacillus subtilis and Lactobacillus buchneri on fermentation, aerobic stability, and microbial community of alfalfa silage. Bioresour. Technol. 315:123881. doi: 10.1016/j.biortech.2020.123881
Borreani, G., and Tabacco, E. (2010). The relationship of silage temperature with the microbiological status of the face of corn silage bunkers. J. Dairy Sci. 93, 2620–2629. doi: 10.3168/jds.2009-2919
Borreani, G., Tabacco, E., Schmidt, R. J., Holmes, B. J., and Muck, R. E. (2018). Silage review: factors affecting dry matter and quality losses in silages. J. Dairy Sci. 101, 3952–3979. doi: 10.3168/jds.2017-13837
Botero-Londono, J. M., Celis-Celis, E. M., and Botero-Londono, M. A. (2021). Nutritional quality, nutrient uptake and biomass production of Pennisetum purpureum cv King Grass. Sci. Rep. 11:13799. doi: 10.1038/s41598-021-93301-w
Broderick, G., and Kang, J. (1980). Automated simultaneous determination of ammonia and total amino acids in ruminal fluid and in vitro media. J. Dairy Sci. 63, 64–75. doi: 10.3168/jds.S0022-0302(80)82888-8
Cardona, E., Rios, J., Peña, J., Peñuela, M., and Rios, L. (2016). King grass: A very promising material for the production of second generation ethanol in tropical countries. Biomass Bioenergy 95, 206–213. doi: 10.1016/j.biombioe.2016.10.008
Da Silva, T. C., Smith, M. L., Barnard, A. M., and Kung, L. (2015). The effect of a chemical additive on the fermentation and aerobic stability of high-moisture corn. J. Dairy Sci. 98, 8904–8912. doi: 10.3168/jds.2015-9640
Dong, Z., Li, J., Chen, L., Wang, S., and Shao, T. (2019). Effects of freeze-thaw event on microbial community dynamics during red clover ensiling. Front. Microbiol. 10:1559. doi: 10.3389/fmicb.2019.01559
Dong, L., Zhang, H., Gao, Y., and Diao, Q. (2020). Dynamic profiles of fermentation characteristics and bacterial community composition of Broussonetia papyrifera ensiled with perennial ryegrass. Bioresour. Technol. 310:123396. doi: 10.1016/j.biortech.2020.123396
Fuhs, G. W., and Chen, M. (1975). Microbiological basis of phosphate removal in the activated sludge process for the treatment of wastewater. Microb. Ecol. 2, 119–138. doi: 10.1007/BF02010434
Guo, L. N., Wang, X. K., Lin, Y. L., Yang, X. P., Ni, K. K., and Yang, F. Y. (2021). Microorganisms that are critical for the fermentation quality of paper mulberry silage. Food Energy Secur. 10:e304. doi: 10.1002/fes3.304
Hu, Z., Chang, J., Yu, J., Li, S., and Niu, H. (2018). Diversity of bacterial community during ensiling and subsequent exposure to air in whole-plant maize silage. Asian-Australas J. Anim. Sci. 31, 1464–1473. doi: 10.5713/ajas.17.0860
Junges, D., Morais, G., Spoto, M. H. F., Santos, P. S., Adesogan, A. T., Nussio, L. G., et al. (2017). Short communication: influence of various proteolytic sources during fermentation of reconstituted corn grain silages. J. Dairy Sci. 100, 9048–9051. doi: 10.3168/jds.2017-12943
Kampfer, P., and Glaeser, S. P. (2012). Prokaryotic taxonomy in the sequencing era--the polyphasic approach revisited. Environ. Microbiol. 14, 291–317. doi: 10.1111/j.1462-2920.2011.02615.x
Keshri, J., Chen, Y., Pinto, R., Kroupitski, Y., Weinberg, Z. G., and Sela Saldinger, S. (2018). Microbiome dynamics during ensiling of corn with and without Lactobacillus plantarum inoculant. Appl. Microbiol. Biotechnol. 102, 4025–4037. doi: 10.1007/s00253-018-8903-y
Kung, L. M., Shaver, R. D., Grant, R. J., and Schmidt, R. J. (2018). Silage review: interpretation of chemical, microbial, and organoleptic components of silages. J. Dairy Sci. 101, 4020–4033. doi: 10.3168/jds.2017-13909
Li, D., Ni, K., Zhang, Y., Lin, Y., and Yang, F. (2019a). Fermentation characteristics, chemical composition and microbial community of tropical forage silage under different temperatures. Asian-Australas J. Anim. Sci. 32, 665–674. doi: 10.5713/ajas.18.0085
Li, Y., and Nishino, N. (2011). Effects of inoculation of lactobacillus rhamnosus and lactobacillus buchneri on fermentation, aerobic stability and microbial communities in whole crop corn silage. Grassl. Sci. 57, 184–191. doi: 10.1111/j.1744-697X.2011.00226.x
Li, X., Tian, J., Zhang, Q., Jiang, Y., Wu, Z., and Yu, Z. (2018). Effects of mixing red clover with alfalfa at different ratios on dynamics of proteolysis and protease activities during ensiling. J. Dairy Sci. 101, 8954–8964. doi: 10.3168/jds.2018-14763
Li, D., Zheng, X., Lin, L., An, Q., Jiao, Y., Li, Q., et al. (2021). Remediation of soils co-contaminated with cadmium and dichlorodiphenyltrichloroethanes by king grass associated with Piriformospora indica: insights into the regulation of root excretion and reshaping of rhizosphere microbial community structure. J. Hazard. Mater. 422:126936. doi: 10.1016/j.jhazmat.2021.126936
Li, M., Zi, X. J., Tang, J., Zhou, H. L., and Cai, Y. M. (2019b). Silage fermentation, chemical composition and ruminal degradation of king grass, cassava foliage and their mixture. Grassl. Sci. 65, 210–215. doi: 10.1111/grs.12235
Li, M., Zi, X. J., Zhou, H. L., Hou, G. Y., and Cai, Y. M. (2014). Effects of sucrose, glucose, molasses and cellulase on fermentation quality and in vitro gas production of king grass silage. Anim. Feed Sci. Tech. 197, 206–212. doi: 10.1016/j.anifeedsci.2014.06.016
Lin, H., Lin, S., Awasthi, M. K., Wang, Y., and Xu, P. (2021). Exploring the bacterial community and fermentation characteristics during silage fermentation of abandoned fresh tea leaves. Chemosphere 283:131234. doi: 10.1016/j.chemosphere.2021.131234
Liu, B., Huan, H., Gu, H., Xu, N., Shen, Q., and Ding, C. (2019). Dynamics of a microbial community during ensiling and upon aerobic exposure in lactic acid bacteria inoculation-treated and untreated barley silages. Bioresour. Technol. 273, 212–219. doi: 10.1016/j.biortech.2018.10.041
Liu, Q., Zhang, J., Shi, S., and Sun, Q. (2011). The effects of wilting and storage temperatures on the fermentation quality and aerobic stability of stylo silage. Anim. Sci. J. 82, 549–553. doi: 10.1111/j.1740-0929.2011.00873.x
Long, S., Li, X., Yuan, X., Su, R., Pan, J., Chang, Y., et al. (2022). The effect of early and delayed harvest on dynamics of fermentation profile, chemical composition, and bacterial Community of King Grass Silage. Front. Microbiol. 13:864649. doi: 10.3389/fmicb.2022.864649
McGarvey, J. A., Franco, R. B., Palumbo, J. D., Hnasko, R., Stanker, L., and Mitloehner, F. M. (2013). Bacterial population dynamics during the ensiling of Medicago sativa (alfalfa) and subsequent exposure to air. J. Appl. Microbiol. 114, 1661–1670. doi: 10.1111/jam.12179
Myllykoski, J., Lindstrom, M., Keto-Timonen, R., Soderholm, H., Jakala, J., Kallio, H., et al. (2009). Type C bovine botulism outbreak due to carcass contaminated non-acidified silage. Epidemiol. Infect. 137, 284–293. doi: 10.1017/S0950268808000939
Ni, K., Minh, T. T., Tu, T. T., Tsuruta, T., Pang, H., and Nishino, N. (2017). Comparative microbiota assessment of wilted Italian ryegrass, whole crop corn, and wilted alfalfa silage using denaturing gradient gel electrophoresis and next-generation sequencing. Appl. Microbiol. Biotechnol. 101, 1385–1394. doi: 10.1007/s00253-016-7900-2
Ni, K., Zhao, J., Zhu, B., Su, R., Pan, Y., Ma, J., et al. (2018). Assessing the fermentation quality and microbial community of the mixed silage of forage soybean with crop corn or sorghum. Bioresour. Technol. 265, 563–567. doi: 10.1016/j.biortech.2018.05.097
Niderkorn, V., Morgavi, D. P., Pujos, E., Tissandier, A., and Boudra, H. (2007). Screening of fermentative bacteria for their ability to bind and biotransform deoxynivalenol, zearalenone and fumonisins in an in vitro simulated corn silage model. Food Addit. Contam. 24, 406–415. doi: 10.1080/02652030601101110
Nishino, N., Li, Y., Wang, C., and Parvin, S. (2012). Effects of wilting and molasses addition on fermentation and bacterial community in Guinea grass silage. Lett. Appl. Microbiol. 54, 175–181. doi: 10.1111/j.1472-765X.2011.03191.x
Ogunade, I. M., Jiang, Y., Kim, D. H., Cervantes, A. A. P., Arriola, K. G., Vyas, D., et al. (2017). Fate of Escherichia coli O157:H7 and bacterial diversity in corn silage contaminated with the pathogen and treated with chemical or microbial additives. J. Dairy Sci. 100, 1780–1794. doi: 10.3168/jds.2016-11745
Parvin, S., Wang, C., Li, Y., and Nishino, N. (2010). Effects of inoculation with lactic acid bacteria on the bacterial communities of Italian ryegrass, whole crop maize, Guinea grass and Rhodes grass silages. Anim. Feed Sci. Tech. 160, 160–166. doi: 10.1016/j.anifeedsci.2010.07.010
Romero, J. J., Zhao, Y., Balseca-Paredes, M. A., Tiezzi, F., Gutierrez-Rodriguez, E., and Castillo, M. S. (2017). Laboratory silo type and inoculation effects on nutritional composition, fermentation, and bacterial and fungal communities of oat silage. J. Dairy Sci. 100, 1812–1828. doi: 10.3168/jds.2016-11642
Santos, A. O., Avila, C. L., Pinto, J. C., Carvalho, B. F., Dias, D. R., and Schwan, R. F. (2016). Fermentative profile and bacterial diversity of corn silages inoculated with new tropical lactic acid bacteria. J. Appl. Microbiol. 120, 266–279. doi: 10.1111/jam.12980
Tian, J., He, N., Hale, L., Niu, S., Yu, G., Liu, Y., et al. (2017). Soil organic matter availability and climate drive latitudinal patterns in bacterial diversity from tropical to cold temperate forests. Funct. Ecol. 32, 61–70. doi: 10.1111/1365-2435.12952
Tohno, M., Kobayashi, H., Nomura, M., Uegaki, R., and Cai, Y. (2012). Identification and characterization of lactic acid bacteria isolated from mixed pasture of timothy and orchardgrass, and its badly preserved silage. Anim. Sci. J. 83, 318–330. doi: 10.1111/j.1740-0929.2011.00955.x
Vu, V. H., Li, X., Wang, M., Liu, R., Zhang, G., Liu, W., et al. (2019). Dynamics of fungal community during silage fermentation of elephant grass (Pennisetum purpureum) produced in northern Vietnam. Asian-Australas J. Anim. Sci. 32, 996–1006. doi: 10.5713/ajas.18.0708
Wang, C., Pian, R., Chen, X., Lv, H., Zhou, W., and Zhang, Q. (2020). Beneficial effects of tannic acid on the quality of bacterial communities present in high-moisture mulberry leaf and Stylo silage. Front. Microbiol. 11:586412. doi: 10.3389/fmicb.2020.586412
Wang, Y., Wang, C., Zhou, W., Yang, F. Y., Chen, X. Y., and Zhang, Q. (2018). Effects of wilting and Lactobacillus plantarum addition on the fermentation quality and microbial community of Moringa oleifera leaf silage. Front. Microbiol. 9:1817. doi: 10.3389/fmicb.2018.01817
Xu, Z., He, H., Zhang, S., and Kong, J. (2017). Effects of inoculants Lactobacillus brevis and Lactobacillus parafarraginis on the fermentation characteristics and microbial communities of corn stover silage. Sci. Rep. 7:13614. doi: 10.1038/s41598-017-14052-1
Yang, J., Tan, H., and Cai, Y. (2016). Characteristics of lactic acid bacteria isolates and their effect on silage fermentation of fruit residues. J. Dairy Sci. 99, 5325–5334. doi: 10.3168/jds.2016-10952
Yuan, X., Dong, Z., Li, J., and Shao, T. (2019). Microbial community dynamics and their contributions to organic acid production during the early stage of the ensiling of Napier grass (Pennisetum purpureum). Grass Forage Sci. 75, 37–44. doi: 10.1111/gfs.12455
Zhang, Y. C., Li, D. X., Wang, X. K., Lin, Y. L., Zhang, Q., Chen, X. Y., et al. (2019b). Fermentation dynamics and diversity of bacterial community in four typical woody forages. Ann. Microbiol. 69, 233–240. doi: 10.1007/s13213-018-1398-z
Zhang, Y. G., Li, M., Zhou, H. L., Hu, L., Li, W., and Xu, T. S. (2018). Associative effects of stylo and king grass silage different ratios on in vitro rumen fermentation. Legume Res. 41, 584–588. doi: 10.18805/Lr-335
Zhang, L., Zhou, X., Gu, Q., Liang, M., Mu, S., Zhou, B., et al. (2019a). Analysis of the correlation between bacteria and fungi in sugarcane tops silage prior to and after aerobic exposure. Bioresour. Technol. 291:121835. doi: 10.1016/j.biortech.2019.121835
Zhao, J., Xia, B., Meng, Y., Yang, Z., Pan, L., Zhou, M., et al. (2019). Transcriptome analysis to shed light on the molecular mechanisms of early responses to cadmium in roots and leaves of king grass (Pennisetum americanum × P. purpureum). Int. J. Mol. Sci. 20:2532. doi: 10.3390/ijms20102532
Keywords: king grass, silage, bacterial community, fungal community, aerobic exposure
Citation: Chen R, Li M, Yang J, Chen L, Zi X, Zhou H and Tang J (2022) Exploring the effect of wilting on fermentation profiles and microbial community structure during ensiling and air exposure of king grass silage. Front. Microbiol. 13:971426. doi: 10.3389/fmicb.2022.971426
Edited by:
Qing Zhang, South China Agricultural University, ChinaReviewed by:
Huaxin Niu, Inner Mongolia Minzu University, ChinaLin Sun, Inner Mongolia Academy of Agricultural and Animal Husbandry Sciences, China
Jie Zhao, Nanjing Agricultural University, China
Copyright © 2022 Chen, Li, Yang, Chen, Zi, Zhou and Tang. This is an open-access article distributed under the terms of the Creative Commons Attribution License (CC BY). The use, distribution or reproduction in other forums is permitted, provided the original author(s) and the copyright owner(s) are credited and that the original publication in this journal is cited, in accordance with accepted academic practice. No use, distribution or reproduction is permitted which does not comply with these terms.
*Correspondence: Jinsong Yang, food868@163.com; Xuejuan Zi, zixuejuan@163.com
†These authors have contributed equally to this work