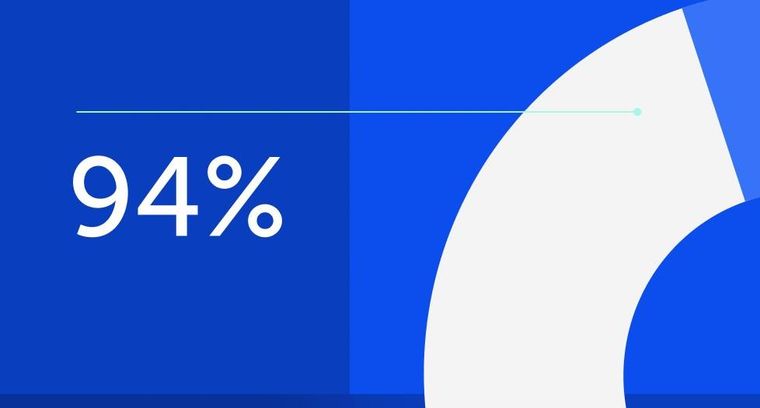
94% of researchers rate our articles as excellent or good
Learn more about the work of our research integrity team to safeguard the quality of each article we publish.
Find out more
ORIGINAL RESEARCH article
Front. Microbiol., 09 August 2022
Sec. Microbial Immunology
Volume 13 - 2022 | https://doi.org/10.3389/fmicb.2022.969490
Streptococcus pyogenes is one of the main pathogenic bacteria that causes disease in humans. It is reported that over 18 million cases of S. pyogenes disease occurred in the world, and more than 500,000 deaths occur annually worldwide. An effective vaccine is widely regarded as the most reliable way to control and prevent streptococcal infections. However, there is currently no approved vaccine for S. pyogenes. In this study, we evaluated the potential of lipoprotein FtsB as a new vaccine candidate to prevent S. pyogenes infection. Mice vaccinated with purified FtsB protein elicited high titers of IgG, IgG1 and IgG2a antibodies in mouse serum. Vaccinated with FtsB can reduce bacterial systemic dissemination in the blood, heart, and spleen and reduce organ damage in the mouse bacteremia model. In addition, active immunization with FtsB protected against streptococcal abscess formation. Furthermore, immunization with FtsB was efficient in inducing a mixed cellular immune response and promoting the maturation of dendritic cells in mice. The lipoprotein HtsA was served as a positive control because it has been reported to protect mice from S. pyogenes infection in both active and passive immunization. These findings demonstrated that lipoprotein FtsB may serve as a candidate vaccine for the prevention of S. pyogenes infection.
Streptococcus pyogenes is a gram-positive bacterium, which can produce a variety of toxins (streptolysin, scarlet fever toxin), lipoteichoic acid, streptokinase and other pathogenic factors, thereby causing a series of skin and mucous membrane infections and other diseases, such as pharyngitis, scarlet fever, pustular skin disease, invasion sexual deep tissue infection, and toxic heat shock syndrome, which are a major threat to human health (Dietrich and Steele, 2018). It is reported that over 18 million cases of S. pyogenes disease occurred in the world, resulting in more than 500,000 deaths annually, and most of these diseases occur mainly in low- and middle-income countries or regions (Carapetis et al., 2005; Commons et al., 2014; Vekemans et al., 2019). Due to the emergence of S. pyogenes resistance to erythromycin, clindamycin, and lincosamide and reduced sensitivity to beta-lactam antibiotics, it is becoming increasingly difficult to cure S. pyogenes disease (Seppala et al., 1992; Hasenbein et al., 2004; Hanage and Shelburne, 2020; Musser et al., 2020; Vannice et al., 2020; Johnson and LaRock, 2021). Thus, vaccination is regarded as the most reliable method to prevent S. pyogenes infections and reduce the global S. pyogenes disease burden, yet there is currently no approved S. pyogenes vaccine (Dale et al., 2016; Vekemans et al., 2019).
For several decades, current S. pyogenes vaccine strategies have mainly focused on M protein-based vaccines and non-M protein-based vaccines, such as GAS carbohydrate, streptococcal protective antigen, streptococcal pyrogenic exotoxin, and lipoproteins (Dale et al., 2016; Vekemans et al., 2019). Lipoproteins are a class of cell membrane-anchored proteins that are widely present in gram-negative and gram-positive bacteria and have a variety of biological functions, acting not only as bacterial virulence factors but also recognizing and activating the host’s immune system; thus, lipoproteins have become one of the most popular drug targets and vaccine candidates (Kovacs-Simon et al., 2011; Yang et al., 2020).
Iron is an essential element for bacterial growth and infection, and several iron-related lipoproteins have been investigated as vaccines, such as the iron-regulated surface determinants IsdA and IsdB (Kuklin et al., 2006; Kim et al., 2010), the streptococcal haem-binding lipoproteins Shp (Zhang et al., 2017) and HtsA (Song et al., 2020). In S. pyogenes, there are three iron uptake systems involved in iron uptake, including HtsABC, FtsABCD, and MtsABC (Janulczyk et al., 2003; Lei et al., 2003; Hanks et al., 2005). The lipoprotein FtsB is a substrate-binding protein of the ferrichrome transporter FtsABCD, which is highly conserved in S. pyogenes (Hanks et al., 2005). Moreover, the homologous proteins of FtsB protein also found in other bacterial species, including Streptococcus dysgalactiae subsp. equisimilis, Streptococcus parauberis, Streptococcus sanguinis, Streptococcus suis (Supplementary Table 1). The lipoprotein HtsA of the haem transporter HtsABC was reported as a candidate antigen for vaccine development (Song et al., 2020). In addition, the immunogenicity of the lipoprotein MtsA (SPy0453) from MtsABC system is weak (Mortensen et al., 2016). Thus, in this study, FtsB was purified and formulated with an aluminum adjuvant, and its immunogenicity and protective efficacy were determined in mouse bacteremia infection and skin infection models following challenge with the S. pyogenes MGAS5005 strain.
Six-week-old female BALB/c mice were purchased from Beijing Huafukang Biotechnology Company. All animal experiments were approved by the Committee on the Use of Live Animals in Teaching and Research of Zunyi Medical University and performed in accordance with the institutional and governmental guidelines and regulations.
S. pyogenes MGAS5005 was grown in 0.5% THYE medium (Todd-Hewitt broth (Oxoid, United Kingdom) containing 0.5% yeast extract (Oxoid, United Kingdom) at 37°C and 5% CO2. Escherichia coli strains BL21 (DE3) (for the pGEX-4T-ftsB recombinant expression plasmid) and Top10 (for the pBAD/HisA-htsA recombinant expression plasmid) were grown in Luria-Bertani (LB) broth with 100 μg/mL ampicillin at 37°C with shaking at 200 rpm.
For the expression and purification of the recombinant protein FtsB, the E. coli BL21 strain (DE) containing the recombinant expression plasmid pGEX-4T-ftsB was cultured in LB medium and induced with 0.5 mM isopropyl-b-D1-thiogalactopyranoside (IPTG) for 6 h at 37°C. The GST-FtsB fusion protein was purified by GST affinity chromatography, and the GST tag was removed with thrombin exonuclease to obtain the antigen protein FtsB.
For expression of the recombinant protein HtsA, the E. coli Top10 strain containing the recombinant expression plasmid pBAD/HisA-htsA was cultured in LB medium and induced with 0.04% L-(+)-arabinose for 6 h at 37°C. HtsA was purified by Ni-NTA affinity chromatography. The purity of the FtsB and HtsA proteins was analyzed by SDS-PAGE.
BALB/c mice (n = 10 per group) were immunized subcutaneously in the back with 20 μg of FtsB in 90 μL PBS that were formulated with 10 μL aluminum hydroxide gel adjuvant (InvivoGen, France) on days 0, 14, and 28. Mice immunized with 20 μg of HtsA protein plus aluminum adjuvant served as a positive control, and mice immunized with 100 μL PBS plus aluminum adjuvant served as a negative control. Blood samples were collected from the tail vein on days 0, 14, 28, and 35 for the enzyme-linked immunosorbent assay (ELISA) of antigen-specific antibodies. Wells of 96-well ELISA plates were coated with 5 μg/mL FtsB or HtsA protein in 50 mM carbonate coating buffer (pH 9.5) overnight at 4°C and then blocked with 200 μL of 5% milk at 37°C for 2 h. Five-fold serially diluted mouse serum samples were added to each well and incubated at 37°C for 1 h. The horseradish peroxidase (HRP)-conjugated goat anti-mouse IgG (Thermo, United States, 1:5,000), IgG1 (Thermo, United States, 1:1,000) and IgG2a (Thermo, United States, 1:1,000) were used as secondary antibodies and incubated at 37°C for 1 h. TMB solution was added to induce the color reaction, and the reaction was stopped with 100 μL of ELISA stop solution. Finally, the absorbance was read using a microplate reader at 450 nm.
Seven days after the third immunization (day 35), immunized mice in each group were injected intravenously in the tail vein with 5 × 106S. pyogenes MGAS5005 strain. For bacterial burdens and histopathology analysis, blood was collected at 6 h post- infection, and the heart, liver, spleen, lung and kidney were collected at 48 h after the challenge, then fixed with 4% paraformaldehyde at room temperature for 24 h and used for Gram staining and HE staining.
The immunized mice were anesthetized with pentobarbital sodium followed by subcutaneous injection of 20 μL sterile PBS-diluted bacterial solution containing 2 × 107 S. pyogenes on day 35. The body weight and abscess size of the infected mice were recorded every day for 9 days. The skin tissues of infected mice were collected 48 h after the challenge, then fixed and stained with HE, Masson’s trichrome and immunohistochemistry (IHC).
To test the cytokines, mice were sacrificed on day 35 and splenocytes were collected from each group and stimulated with 10 μg/mL FtsB or HtsA. Interleukin 2 (IL-2)-, IL- 4-, IL- 6-, IL- 10-, IL-17A- and gamma interferon (IFN-γ)-producing splenocytes from FtsB-vaccinated or positive or negative control mice were analyzed using corresponding mouse cytokine-specific ELISA kits (Biolegend, United States) according to the manufacturer’s instructions. The results were measured at OD450, and concentrations in pg/mL were calculated according to the standard curve.
To detect the dendritic cell (DC) cell subsets, splenocytes and homogenates were collected from mice immunized with FtsB, HtsA or PBS on day 35 and used for flow cytometry analysis. Briefly, splenocytes from mice were collected and adjusted to a concentration of 1 × 106 cells/mL. Then, 100 μL cell suspension (1 × 105 cells/mL) from each mouse was harvested and incubated with the fluorescently labeled antibodies FITC-anti-CD11c (Biolegend, United States, 1:200), PE/Cy5 anti-mouse MHC II (Biolegend, United States, 1:200), and PE anti-mouse CD80 (Biolegend, United States, 1:200) at 4°C for 30 min and then analyzed using flow cytometry. The proportion of CD11c + DC cells expressing MHC II and CD80 molecules was calculated.
All data in the paper are expressed as the means ± SEM. Differences between multiple groups were analyzed using one-way ANOVA followed by least significant difference (LSD) in GraphPad Prism 5 software (San Diego, CA, United States). Significance is indicated by p-values of < 0.05 (*), < 0.01 (**) and < 0.001 (***).
Recombinant FtsB and HtsA proteins were expressed in E. coli BL21 and Top10 and purified using GST affinity chromatography and Ni-NTA affinity chromatography, respectively. As shown in Figure 1, the purity of the FtsB and HtsA proteins was more than 95%, which can be used in further experiments.
Figure 1. Purified recombinant FtsB and HtsA proteins. The purity of the FtsB and HtsA proteins was analyzed by SDS–PAGE. M represents the protein marker, line 1 represents the HtsA protein, and line 2 represents the FtsB protein.
To investigate the immunogenicity of the FtsB protein, the titers of IgG, IgG1, and IgG2a antibodies in the sera of immunized mice were detected using ELISA on post-immunization days 14, 28 and 35. Immunization with the FtsB protein elicited a high level of IgG antibody on days 14, 28, and 35, and antibody production after two boost immunizations was considerably higher than that with one immunization, suggesting that FtsB is strongly antigenic (Figure 2A). Moreover, the titers of IgG antibodies against FtsB and HtsA were similar (Figure 2A).
Figure 2. Antigen-specific antibody responses elicited by FtsB immunization. (A) The level of FtsB-specific IgG antibodies was determined from serum samples on days 14, 28 and 35 by ELISA. (B) The level of FtsB-specific IgG1 was determined from serum samples on day 35 by ELISA. (C) The level of FtsB-specific IgG2a was determined from serum samples on day 35 by ELISA. (D) The ratio of IgG1/IgG2a. n = 10 mice per group, *p < 0.05, **p < 0.01, ***p < 0.001 vs. PBS control group, #represents p < 0.05 vs. HtsA group. The HtsA protein plus an aluminum adjuvant was used as a positive control, and PBS plus an aluminum adjuvant was used as a negative control.
IgG1 and IgG2a are Th2- and Th1-associated antigen-specific IgG isotypes, which reflect the possibility of a Th2- or Th1-biased immune response, respectively. Compared to the PBS control group, both IgG1 and IgG2a antibodies were significantly induced in the FtsB-immunized mice on day 35 (Figures 2B,C). The IgG1/Ig2a ratio was greater than 2 when immunized with FtsB, indicating a Th2-biased immune response (Figure 2D).
We first evaluated the protective efficacy of FtsB in a murine bacteremia model. Seven days following the third immunization (day 35), mice were challenged with 5 × 106 S. pyogenes MGAS5005. As shown in Figures 3A,B, the FtsB- and HtsA-immunized groups showed significantly lower bacterial loads in mouse blood than the PBS control group 6 h post-infection. Moreover, compared with the PBS control group, immunization with the FtsB protein decreased the bacterial burden in the heart and spleen 48 h post-infection, and similar results were also observed in HtsA-immunized mice (Figure 3C).
Figure 3. Immunization with the FtsB protein reduced the bacterial burden in a murine bacteremia model. (A,B) Bacterial loads in the blood of mice immunized with different antigens determined 6 h after infection with 5 × 106S. pyogenes MGAS5005 (n = 5). (C) The bacterial loads in the heart and spleen of mice. Gram staining of the heart and spleen of mice after 48 h of challenge with S. pyogenes (400×). n = 5 mice per group, **p < 0.01, ***p < 0.001 vs. PBS control group.
To further observe the effect of immunization with the FtsB protein on bacteremia, histological analysis was performed. The heart, liver, spleen, lung, and kidney were collected from mice challenged with S. pyogenes MGAS5005 48 h after infection. The smaller cardiac-mechanical gap in the heart tissue, more neat hepatocytes arrangement in the liver, the normal and visible germinal centers in the spleen, the less alveolar damage and inflammatory cell infiltration in the lung, and the less bleeding in the kidney were observed in mice immunization with the FtsB or HtsA protein when compared with the PBS control group (Figure 4). Collectively, these data suggested that vaccinated with the FtsB protein can reduce bacterial burden and spreading in the blood, heart and spleen and reduce organ damage.
Figure 4. Immunization with the FtsB protein decreased the pathology of the heart, liver, spleen, lung, and kidney in a murine bacteremia model. HE images of the heart, liver, spleen, lung, and kidney in mice 48 h after challenge with 5 × 106S. pyogenes MGAS5005 (400×).
We also used a murine skin infection model to assess the protective efficacy of FtsB against S. pyogenes. Seven days following the third immunization, mice were inoculated by subcutaneous injection in the shaved right flank with S. pyogenes. The body weight and skin abscess areas of all mice were monitored every day during a 9-day observation period. As shown in Figure 5, the body weights of mice in the vaccinated and control groups were almost unchanged; however, the S. pyogenes abscess sizes in mice immunized with FtsB were smaller than those in the PBS control group. Compared with the PBS control group, the skin wounds in mice immunized with FtsB or HtsA were almost healed by day 9 after infection (Figures 5B,C). In addition, there was no difference in abscess size between mice immunized with FtsB and HtsA (Figures 5B,C).
Figure 5. Immunization with the FtsB protein decreases the size of abscesses caused by S. pyogenes in a murine skin infection model. (A) The body weight of the mice. (B) The size of abscesses in mice. (C) Representative mouse skin lesions. n = 5 mice per group, **p < 0.01 represent FtsB vaccinated group vs. PBS control group.
We further investigated the possible mechanism for FtsB-mediated protection in the skin infection model. The HE results showed that the inflammation of infected skin wounds in mice immunized with FtsB was less than that in the PBS control group (Figure 6). F4/80 and CD31 are markers of macrophages and vascular endothelium, respectively. As shown in Figure 6, compared to the PBS control group, the expression of F4/80 in skin lesions was reduced significantly in mice vaccinated with FtsB or HtsA, while the expression of CD31 was induced significantly in mice vaccinated with FtsB or HtsA. In addition, the Masson staining results indicated that collagen fibers in the infected skin wounds of the mice vaccinated with FtsB or HtsA were significantly more abundant than those in the PBS control group (Figure 6). The above results demonstrated that immunization with FtsB alleviated the severity of S. pyogenes skin infections and accelerated skin wound healing.
Figure 6. In the skin infection model, immunization with the FtsB protein reduced inflammation at the mouse skin lesions, promoted vascular endothelial regeneration and accelerated wound healing. The skin pathological conditions of the mice were monitored 48 h after challenge with S. pyogenes. F4/80 is a marker of macrophages, and CD31 is a marker of vascular endothelium (400×). The brown in the figures represent F4/80- or CD31-positive cells.
To further investigate the possible mechanism of protective immunity initiated by FtsB, we measured the cytokine profiles of spleen cells from mice in each immunized group 7 days after the third immunization. As shown in Figure 7, elevated levels of the cytokines IL-2, IFN-γ (Th1), IL-4, IL-6, IL-10 (Th2), and the IL-17A (Th17) were observed in the FtsB- and HtsA-immunized groups compared to the PBS control group. These findings were consistent with the results from the antibody subtype assay, which suggested that immunization with FtsB or HtsA induced a strong mixed cellular immune response. Moreover, compared with the HtsA-immunized group, immunization with FtsB protein induced higher levels of IL-4 and IL-10.
Figure 7. Immunization with FtsB stimulated the secretion of the cytokines IL-2, IFN-γ, IL-4, IL-6, IL-10 and IL-17A. (A) The secretion of the cytokine IL-2. (B) The secretion of the cytokine IFN-γ. (C) The secretion of the cytokine IL-4. (D) The secretion of the cytokine IL-6. (E) The secretion of cytokine IL-10. (F) The secretion of the cytokine IL-17A. n = 5 mice per group, *p < 0.05, **p < 0.01, ***p < 0.001 vs. PBS control group, #represents p < 0.05 vs. HtsA group. The HtsA protein plus an aluminum adjuvant was used as a positive control, and PBS plus an aluminum adjuvant was used as a negative control.
To investigate the effect of immunization with FtsB protein on the maturation of DCs, we measured the expression of the MHC II and CD80 molecules on CD11c+ DCs from murine spleen cells in each immunized group by using flow cytometry. Compared with the PBS control group, the percentage of MHC II and CD80 molecule expression by CD11c+ DCs in mice vaccinated with FtsB or HtsA was significantly increased (Figure 8). These results indicated that immunization with FtsB can improve the maturation of DCs in mice, which is beneficial for inducing more effective antigen presentation and immune responses.
Figure 8. Immunization with FtsB stimulated the maturation of DCs. (A) Representative flow cytometry graph. (B) The percentage of the costimulatory molecule MHCII expressed on CD11c + DC cells. (C) The percentage of the costimulatory molecule CD80 expressed on CD11c + DCs. n = 5 mice per group, *p < 0.05, **p < 0.01 vs. PBS control group.
S. pyogenes is one of the major pathogens responsible for human disease, and iron plays an important role in its growth and infection. Iron uptake systems are the major virulence determinants for many pathogens, and their lipoproteins are exposed on the extracellular wall and thus have been proven to be promising vaccine candidates in many pathogens (Cassat and Skaar, 2013; Nguyen and Gotz, 2016; Kramer et al., 2020). In S. pyogenes, there are three iron uptake systems involved in iron uptake, HtsABC, FtsABCD and MtsABC, with the lipoproteins HtsA, FtsB and MtsA, respectively (Janulczyk et al., 2003; Lei et al., 2003; Hanks et al., 2005). It has been reported that the HtsA protein can be used as a vaccine candidate to protect against S. pyogenes infection (Song et al., 2020). Immunization with the HtsA protein can reduce the invasion of S. pyogenes, improve the survival rate, and reduce the size of skin lesions in mice (Song et al., 2020). However, whether the FtsB protein can serve as an S. pyogenes vaccine candidate has not yet been reported.
In the present study, we obtained the FtsB protein with high purity and thoroughly evaluated the immunogenicity it induced in BALB/c mice, as well as its protection against S. pyogenes infection. Immunization with the FtsB protein induced the production of high titers of antigen-specific IgG, IgG1 and IgG2a in mice. Moreover, the FtsB protein was able to induce a protective immune response against S. pyogenes infection in a murine bacteremia model and a skin infection model. In the murine bacteremia model, a low S. pyogenes bacterial load was recovered from the blood, heart, and spleen, and less organ damage, including less damage to the heart, liver, spleen, lung, and kidney, was observed in FtsB-vaccinated mice than in the PBS control group. In the murine skin infection model, there were smaller skin abscess areas and fewer inflammatory skin infection wounds in mice vaccinated with FtsB than in the PBS control group. These results indicate the protective efficacy of the FtsB vaccine.
In addition, we dissected the possible mechanisms of protection induced by FtsB from two perspectives: cytokine production and DC activation. Spleen cells from mice immunized with antigens can be stimulated in vitro to secrete specific cytokines that play important roles in antigen-specific immune responses. Cytokines play a key role in protecting the host from bacterial infection by modulating the innate immune response (Soderholm et al., 2018). DCs play a key role in activating innate immune cells and initiating adaptive immune responses, and they are also intermediate regulators responsible for sensing pathogens and activating effectors to eliminate them (Durai and Murphy, 2016). In this study, the protective effects of FtsB were accompanied by the secretion of IL-2, IFN-γ, IL-4, IL-6, IL-10, and IL-17A by stimulated splenocytes, as well as the maturation of DCs.
It has been reported that the HtsA protein can be used as a vaccine candidate against S. pyogenes infection (Song et al., 2020). Here, the IgG, IgG1 and IgG2a titers, the protective effects against S. pyogenes infection in two murine models, the levels of IL-2, IFN-γ, IL-6, and IL-17A production, and the percentage CD11c+ DCs expressing the MHC II and CD80 molecules were similar in mice immunized with FtsB protein compared to the HtsA positive control. However, FtsB induced an increased IgG1/Ig2a ratio, and the levels of the Th2-type cytokines IL-4 and IL-10 were higher than those of HtsA, which indicated that FtsB induced a Th2-biased immune response and can serve as an S. pyogenes vaccine candidate. On the other hand, compared to the M protein-based vaccine candidates, e.g., J8-DT (Pandey et al., 2015), the protective effect of the FtsB vaccination is weak, suggesting that the improvement and optimization of FtsB vaccine needs to be done in the future.
In conclusion, immunization with the FtsB protein could elicit immune responses, including the induction of high antigen-specific IgG, IgG1, and IgG2a titers, the secretion of the cytokines IL-2, IFN-γ, IL-4, IL-6, IL-10, and IL-17A, and the maturation of DCs, leading to protective immunity in a murine model. These results provide evidence for the FtsB protein as a vaccine candidate against S. pyogenes infection.
The raw data supporting the conclusions of this article will be made available by the authors, without undue reservation.
This animal study was reviewed and approved by the Committee on the Use of Live Animals in Teaching and Research of Zunyi Medical University.
L-YH and Y-BY designed the research, performed the experimental work, and wrote the manuscript. YL and Y-JL analyzed the data. X-YY and SL provided the initial idea, designed the research, and revised the manuscript. All authors contributed to manuscript revision and read and approved the submitted version.
This work was financially supported by the National Natural Science Foundation of China (32160183 to X-YY), the Guizhou Provincial Natural Science Foundation (QKH-J [2020]1Y352 to X-YY; QKH-ZK [2021]YB420 to SL; and QKH-[2018]5772-018 to SL), and the Excellent Young Talents Fund Program of Zunyi Medical University (18zy-005 to X-YY).
The authors declare that the research was conducted in the absence of any commercial or financial relationships that could be construed as a potential conflict of interest.
All claims expressed in this article are solely those of the authors and do not necessarily represent those of their affiliated organizations, or those of the publisher, the editors and the reviewers. Any product that may be evaluated in this article, or claim that may be made by its manufacturer, is not guaranteed or endorsed by the publisher.
The Supplementary Material for this article can be found online at: https://www.frontiersin.org/articles/10.3389/fmicb.2022.969490/full#supplementary-material
Carapetis, J. R., Steer, A. C., Mulholland, E. K., and Weber, M. (2005). The global burden of group A streptococcal diseases. Lancet Infect. Dis. 5, 685–694. doi: 10.1016/S1473-3099(05)70267-X
Cassat, J. E., and Skaar, E. P. (2013). Iron in infection and immunity. Cell Host Microbe 13, 509–519. doi: 10.1016/j.chom.2013.04.010
Commons, R. J., Smeesters, P. R., Proft, T., Fraser, J. D., Robins-Browne, R., and Curtis, N. (2014). Streptococcal superantigens: Categorization and clinical associations. Trends Mol. Med. 20, 48–62. doi: 10.1016/j.molmed.2013.10.004
Dale, J. B., Batzloff, M. R., Cleary, P. P., Courtney, H. S., Good, M. F., Grandi, G., et al. (2016). “Current Approaches to Group A Streptococcal Vaccine Development,” in Streptococcus Pyogenes: Basic Biology to Clinical Manifestations, eds J. J. Ferretti, D. L. Stevens, and V. A. Fischetti (Oklahoma City OK: University of Oklahoma Health Sciences Center).
Dietrich, M. L., and Steele, R. W. (2018). Group A Streptococcus. Pediatr. Rev. 39, 379–391. doi: 10.1542/pir.2017-0207
Durai, V., and Murphy, K. M. (2016). Functions of Murine Dendritic Cells. Immunity 45, 719–736. doi: 10.1016/j.immuni.2016.10.010
Hanage, W. P., and Shelburne, S. A. (2020). Streptococcus pyogenes With Reduced Susceptibility to beta-Lactams: How Big an Alarm Bell? Clin. Infect. Dis. 71, 205–206. doi: 10.1093/cid/ciz1006
Hanks, T. S., Liu, M., McClure, M. J., and Lei, B. (2005). ABC transporter FtsABCD of Streptococcus pyogenes mediates uptake of ferric ferrichrome. BMC Microbiol. 5:62. doi: 10.1186/1471-2180-5-62
Hasenbein, M. E., Warner, J. E., Lambert, K. G., Cole, S. E., Onderdonk, A. B., and McAdam, A. J. (2004). Detection of multiple macrolide- and lincosamide-resistant strains of Streptococcus pyogenes from patients in the Boston area. J. Clin. Microbiol. 42, 1559–1563. doi: 10.1128/JCM.42.4.1559-1563.2004
Janulczyk, R., Ricci, S., and Bjorck, L. (2003). MtsABC is important for manganese and iron transport, oxidative stress resistance, and virulence of Streptococcus pyogenes. Infect. Immun. 71, 2656–2664. doi: 10.1128/IAI.71.5.2656-2664.2003
Johnson, A. F., and LaRock, C. N. (2021). Antibiotic Treatment, Mechanisms for Failure, and Adjunctive Therapies for Infections by Group A Streptococcus. Front. Microbiol. 12:760255. doi: 10.3389/fmicb.2021.760255
Kim, H. K., DeDent, A., Cheng, A. G., McAdow, M., Bagnoli, F., Missiakas, D. M., et al. (2010). IsdA and IsdB antibodies protect mice against Staphylococcus aureus abscess formation and lethal challenge. Vaccine 28, 6382–6392. doi: 10.1016/j.vaccine.2010.02.097
Kovacs-Simon, A., Titball, R. W., and Michell, S. L. (2011). Lipoproteins of bacterial pathogens. Infect. Immun. 79, 548–561. doi: 10.1128/IAI.00682-10
Kramer, J., Ozkaya, O., and Kummerli, R. (2020). Bacterial siderophores in community and host interactions. Nat. Rev. Microbiol. 18, 152–163. doi: 10.1038/s41579-019-0284-4
Kuklin, N. A., Clark, D. J., Secore, S., Cook, J., Cope, L. D., Mcneely, T., et al. (2006). A novel Staphylococcus aureus vaccine: Iron surface determinant B induces rapid antibody responses in rhesus macaques and specific increased survival in a murine S. aureus sepsis model. Infect. Immun. 74, 2215–2223. doi: 10.1128/IAI.74.4.2215-2223.2006
Lei, B., Liu, M., Voyich, J. M., Prater, C. I., Kala, S. V., DeLeo, F. R., et al. (2003). Identification and characterization of HtsA, a second heme-binding protein made by Streptococcus pyogenes. Infect. Immun. 71, 5962–5969. doi: 10.1128/IAI.71.10.5962-5969.2003
Mortensen, R., Nissen, T. N., Fredslund, S., Rosenkrands, I., Christensen, J. P., Andersen, P., et al. (2016). Identifying protective Streptococcus pyogenes vaccine antigens recognized by both B and T cells in human adults and children. Sci. Rep. 6:22030. doi: 10.1038/srep22030
Musser, J. M., Beres, S. B., Zhu, L., Olsen, R. J., Vuopio, J., Hyyrylainen, H. L., et al. (2020). Reduced In Vitro Susceptibility of Streptococcus pyogenes to beta-Lactam Antibiotics Associated with Mutations in the pbp2x Gene Is Geographically Widespread. J. Clin. Microbiol. 58:e01993–19. doi: 10.1128/JCM.01993-19
Nguyen, M. T., and Gotz, F. (2016). Lipoproteins of Gram-Positive Bacteria: Key Players in the Immune Response and Virulence. Microbiol. Mol. Biol. Rev. 80, 891–903. doi: 10.1128/MMBR.00028-16
Pandey, M., Langshaw, E., Hartas, J., Lam, A., Batzloff, M. R., and Good, M. F. (2015). A synthetic M protein peptide synergizes with a CXC chemokine protease to induce vaccine-mediated protection against virulent streptococcal pyoderma and bacteremia. J. Immunol. 194, 5915–5925. doi: 10.4049/jimmunol.1500157
Seppala, H., Nissinen, A., Jarvinen, H., Huovinen, S., Henriksson, T., Herva, E., et al. (1992). Resistance to erythromycin in group A streptococci. N. Engl. J. Med. 326, 292–297. doi: 10.1056/NEJM199201303260503
Soderholm, A. T., Barnett, T. C., Sweet, M. J., and Walker, M. J. (2018). Group A streptococcal pharyngitis: Immune responses involved in bacterial clearance and GAS-associated immunopathologies. J. Leukoc. Biol. 103, 193–213.
Song, Y., Zhang, X., Cai, M., Lv, C., Zhao, Y., Wei, D., et al. (2020). Active and passive immunizations with HtsA, a streptococcal heme transporter protein, protect mice from subcutaneous group A Streptococcus infection. J. Microbiol. Immunol. Infect. 53, 87–93. doi: 10.1016/j.jmii.2018.03.002
Vannice, K. S., Ricaldi, J., Nanduri, S., Fang, F. C., Lynch, J. B., Bryson-Cahn, C., et al. (2020). Streptococcus pyogenes pbp2x Mutation Confers Reduced Susceptibility to beta-Lactam Antibiotics. Clin. Infect. Dis. 71, 201–204. doi: 10.1093/cid/ciz1000
Vekemans, J., Gouvea-Reis, F., Kim, J. H., Excler, J. L., Smeesters, P. R., O’Brien, K. L., et al. (2019). The Path to Group A Streptococcus Vaccines: World Health Organization Research and Development Technology Roadmap and Preferred Product Characteristics. Clin. Infect. Dis. 69, 877–883. doi: 10.1093/cid/ciy1143
Yang, X. Y., Zhu, K. X., Guo, Z., Deng, Y. D., Li, S., and Le, Y. J. (2020). Research Progress of Lipoprotein Functions and Their Application as Vaccine Candidates or Drug Targets in Streptococci. Prog. Biochem. Biophys. 47, 956–967.
Keywords: Streptococcus pyogenes, lipoprotein FtsB, vaccine, immunogenicity, protective immunity
Citation: He L-Y, Yu Y-B, Liu Y, Le Y-J, Li S and Yang X-Y (2022) Immunization with the lipoprotein FtsB stimulates protective immunity against Streptococcus pyogenes infection in mice. Front. Microbiol. 13:969490. doi: 10.3389/fmicb.2022.969490
Received: 15 June 2022; Accepted: 22 July 2022;
Published: 09 August 2022.
Edited by:
Tao Jin, Sahlgrenska University Hospital, SwedenReviewed by:
Jakub Kwiecinski, Jagiellonian University, PolandCopyright © 2022 He, Yu, Liu, Le, Li and Yang. This is an open-access article distributed under the terms of the Creative Commons Attribution License (CC BY). The use, distribution or reproduction in other forums is permitted, provided the original author(s) and the copyright owner(s) are credited and that the original publication in this journal is cited, in accordance with accepted academic practice. No use, distribution or reproduction is permitted which does not comply with these terms.
*Correspondence: Sha Li, bGlzaGFfam51QDE2My5jb20=; Xiao-Yan Yang, b3V5YW5neGlhbmd5YW5AMTI2LmNvbQ==
†These authors have contributed equally to this work
Disclaimer: All claims expressed in this article are solely those of the authors and do not necessarily represent those of their affiliated organizations, or those of the publisher, the editors and the reviewers. Any product that may be evaluated in this article or claim that may be made by its manufacturer is not guaranteed or endorsed by the publisher.
Research integrity at Frontiers
Learn more about the work of our research integrity team to safeguard the quality of each article we publish.