- College of Agriculture and Biotechnology, Hunan University of Humanities, Science and Technology, Loudi, China
Background and aims: Land abandonment and selective farming are two common management methods to restore the soil conditions of low-pollution farmland in mining areas. The soil bacterial community plays an important role in farmland soil restoration; however, few studies have compared the composition and diversity of soil bacteria between the abandoned farmlands (AFS) and selective-farming farmlands (FFS). Here, the effects of AFS and FFS on soil properties and bacterial diversity were evaluated in an antimony (Sb) mining area in southern China. This study aimed to identify effective land management methods in terms of positive or negative changes in soil environment and bacterial diversity.
Methods: 16S rRNA high-throughput sequencing was used to compare the diversity and composition of soil bacteria between AFS and FFS in the Xikuangshan (the largest Sb mine in the world).
Results: Compared to AFS, FFS had higher Sb concentration and nutritional properties (e.g., available N, P, and K) and lower Zn concentration (p < 0.05). The bacterial alpha diversity including Chao1 index, Simpson index, Shannon index and Pielou−e index in FFS was higher than AFS (p < 0.05). At the phylum level, FFS had higher relative abundances of Chloroflexi, Acidobacteria, Gemmatimonadetes, and Rokubacteria, and lower relative abundances of Firmicutes, Actinobacteria, and Bacteroidetes. At the genus level, FFS had higher relative abundances of Acidothermus, Haliangium, and Rokubacteriales, and lower relative abundances of Bacillus, Rhodococcus, Sphingomonas, and 67-14. Redundancy analysis indicated that soil heavy metal content and soil fertility were closely correlated with the soil bacterial community. Altogether, selective farming of low-pollution farmland in the mining area can improve soil properties and soil bacterial diversity.
Highlights
–Selective farming of farmland can improve soil nutritional properties.
–Selective farming significantly improved the bacterial alpha diversity.
–Selective farming changed the composition and abundances of soil dominant bacteria.
Introduction
Due to many environmental problems related to heavy metal soil pollution, concern about the soil quality of farmlands near metal mining areas is increasing (Tang et al., 2020; Castellano-Hinojosa et al., 2021; Han et al., 2022; Khmelevtsova et al., 2022). Farmlands with heavy metal pollution can show low productivity and poor safety of crops, resulting in the agricultural production activities stopped (Deng et al., 2015). However, to meet the production and living needs of local residents, it is unrealistic to abandon large areas of polluted farmland (especially low-pollution farmland). To address this problem, different strategies including metal removal, selective farming and safe farming with agronomic measures have been explored in the past decades (Li, 2019; Jin et al., 2020; Chen et al., 2021; Deng et al., 2021; Han et al., 2022; Khmelevtsova et al., 2022). Among these, selective farming on low-pollution farmland is one of the common farming methods for some countries (including China) with large populations and less cultivated lands (Hou et al., 2018; Han et al., 2022; Khmelevtsova et al., 2022).
Farming of farmlands can affect soil physicochemical properties and biological processes (García-Orenes et al., 2010; Wang et al., 2019; Khmelevtsova et al., 2022). Previous studies have shown that farmland planting can destroy the size of soil aggregates and increase soil porosity, thus changing the stability of soil structure (Deng et al., 2015). Farmland planting can also affect the activity, biomass and diversity of soil microbe, and change the content, valence and stability of heavy metals in soil (Deng et al., 2015; Huang et al., 2020). These changes can reduce or increase the degree of pollution by heavy metals in soil, thereby affecting microbial and biochemical processes in farm ecosystems (Raiesi, 2006). In addition, the health of cultivated land is closely related to soil bacterial characteristics (Zhou and Ding, 2007; Wang et al., 2019; Khmelevtsova et al., 2022). Soil bacterial diversity can be used to evaluate the soil quality of contaminated soil, as it can reflect the effects of farming methods, nitrogen input and land restoration (Jusselme et al., 2013; Nivelle et al., 2016). Therefore, the composition and diversity of soil bacteria can be used as an essential indicator to evaluate cultivated land restoration.
There is little information on the impact of land abandonment and selective farming on soil properties and soil bacteria in mining areas, although these are typical management methods in China (Sun et al., 2018; Bai et al., 2021). It is assumed that selective farming and abandonment will lead to different soil characteristics and soil bacteria. In this study, we sampled the soil of local selective-farming farmlands and abandoned farmlands for high-throughput sequencing of bacteria and detected soil properties (heavy metal content and nutrient status). We compared the differences in soil bacterial composition and diversity between the two farming methods to provide a basis for follow-up research on the remediation of heavy metal polluted farmlands from the perspective of bacteria.
Materials and methods
Experimental site and sampling
The study sites were located in the Sb mining area (Xikuangshan, the largest Sb mine in the world) of Hunan Province, China (Supplementary Figure S1). Mining activities gradually stopped in the 1990s. According to previous research, there are different degrees of combined pollution of Sb, As, Cd, Pb, Hg, and Zn in the surrounding farmland soil (Zhou et al., 2019; Bai et al., 2021).
A preliminary investigation and visit to the farmlands around the mining area showed although the pollution conditions of these farmland were similar, due to the large number of manual relocation in the mining area, some farmlands close to the residence was selectively planted with sweet potato (Ipomoea batatas L.) and maize (Zea mays L.) for 10–15 years, while some farmlands far away were abandoned for 10–15 years. In the study area, selective-farming land was cultivated, fertilized in the form of complex fertilizer (NPK) and irrigated in spring/summer, and the annual application rates of N, P and K were about 310–360, 120–140, 230–270 kg/ha, respectively. In these abandoned farmlands, the dominant plants were Boehmeria nivea, Pteridium aquilinum var. Latiusculum, Miscanthus floridulus, and Erigeron annuus. Maize (Guo et al., 2011; Wang et al., 2016) and sweet potato (Huang et al., 2020) are cropped in low-pollution farmland as their low accumulation of heavy metals. Planting maize and sweet potato instead of rice in low-pollution areas of heavy metals are one of the measures to protect human health and the agricultural economy (Wang et al., 2016; Huang et al., 2020).
The collection of farmland soil and determination of soil properties
To study the impact of different farming methods on farmland restoration in mining areas, five abandoned farmlands (AFS, representing natural restoration) and five farming farmlands (FFS, representing the conventional soil management) were selected randomly to sample farm soils of about 10–15 cm deep. In total, 10 farmlands were sampled. In each farmland, five soil sub-samples were collected and then they were fully mixed to obtain a uniform sample of each site (Sun et al., 2020). Visible coarse roots, stones and soil animals were removed. The soil samples were sieved (2 mm). All collected composite samples were placed in a polyethylene sealed plastic bag, labeled, and carried back to the laboratory in an iced box. Each soil sample was artificially divided into two subgroups. The first subgroup was air-dried at ambient temperature to test the contents of soil nutrients (e.g., available N, P, and K) and heavy metals (e.g., Sb, As, Cd, Pb, Zn, and Hg). The second subset was stored at −80°C for soil bacterial DNA extraction.
The contents of heavy metals (Sb, As, Cd, Pb, Zn, and Hg) were measured by PinAAcle atomic absorption spectrometer (Perkineller Inc., United States). The determination of soil nutrient properties was carried out using the method of Zhang and Fang (2007) and Sun et al. (2020). Briefly, the nitrogen content was determined by micro-kjeldahl digestion. NH4+-N and NO3−-N in soil were extracted with 2 M KCl (w/v, 1:5), and their sum was the available N (AN) content. After digestion with H2SO4-HClO4 and extraction with 0.5 M NaHCO3, the available phosphorus (AP) content was determined by the molybdenum blue colorimetry method. After digestion with HF-HClO4 and extraction with 1 M NH4OAC, the available potassium (AK) content was measured by flame photometer.
Illumina MiSeq platform sequencing and sequence analysis
The total bacterial DNA was purified from 0.5 g of each homogenized soil samples using the E.Z.N.A.® Soil DNA Kit (Omega Bio-Tek, United States). The V3-V4 regions of bacterial 16S rRNA gene were amplified and sequenced with 338F (forward primers, 5′-ACTCCTACGGGAGGCAGCAG-3′) and 806R (reverse primers, 5′-GGACTACHVGGGTWTCTAAT-3′; Deng et al., 2020a; Duan et al., 2021). PCR conditions included an initial denaturation step at 98°C for 5 min, followed by 25 cycles including denaturation at 98°C for 15 s, annealing at 55°C for 30 s, extension at 72°C for 30 s, and a final extension at 72°C for 5 min. High-throughput sequencing of 16S rRNA amplicons was performed on the Illumina MiSeq platform at Shanghai Personal Biotechnology Co., Shanghai, China.
Statistical analysis
The ambiguous sequence readings in the raw 16S rRNA data were discarded by QIIME software (version 1.8.0), and then the high-quality sequences were clustered with 97% similarity. The sequence data visualization and statistical analyses for the soil bacterial community were carried out using the R software package (version 3.2.0) and QIIME software. A Venn diagram was created with R software to visualize the similarity and overlap of OTU numbers between FFS and AFS (Fouts et al., 2012). The Chao1 richness index, Simpson index, Shannon index and Pielou evenness index were used to evaluate the differences of alpha diversity indices. Principal coordinate analysis (PCoA), Non-metric multidimensional scaling analysis (NMDS) and hierarchical cluster analysis were used to quantify the differences and similarities of bacterial beta diversity between samples (Deng et al., 2020a). The correlation between soil parameters and bacterial abundance was studied by redundancy analysis (RDA; Guo et al., 2019). A Kruskal–Wallis test was used to test the statistical differences of bacterial communities between FFS and AFS.
Results
Nutrient and heavy metal concentration in soil
Compared to AFS, the contents of heavy metals and soil nutrients in FFS were significantly different (Table 1). Compared to AFS, the concentration of Sb in FFS was significantly higher (p < 0.05), while the concentration of Zn was significantly lower (p < 0.05), and there was no significant difference in the contents of other heavy metals (e.g., As, Cd, Pb, and Hg; p > 0.05; Table 1). Compared to AFS, due to the influence of long-term fertilization in selective-farming farmlands, the soil nutritional status in FFS was significantly improved (p < 0.05). The available N, available P, and available K in farmed farmlands improved by 79.96%, 369.68%, and 312.99%, respectively (Table 1).
Alpha diversity index and beta diversity analysis
The number of OTUs identified in FFS and AFS was 13,228 and 13,140, respectively. There were 2,964 overlapping OTUs in the two groups, and 10,264 and 10,176 unique OTUs in the soil from FFS and FFS, respectively (Figure 1A). The sparse curves reached a plateau, indicating that the sequencing depth was sufficient (Figure 1B).
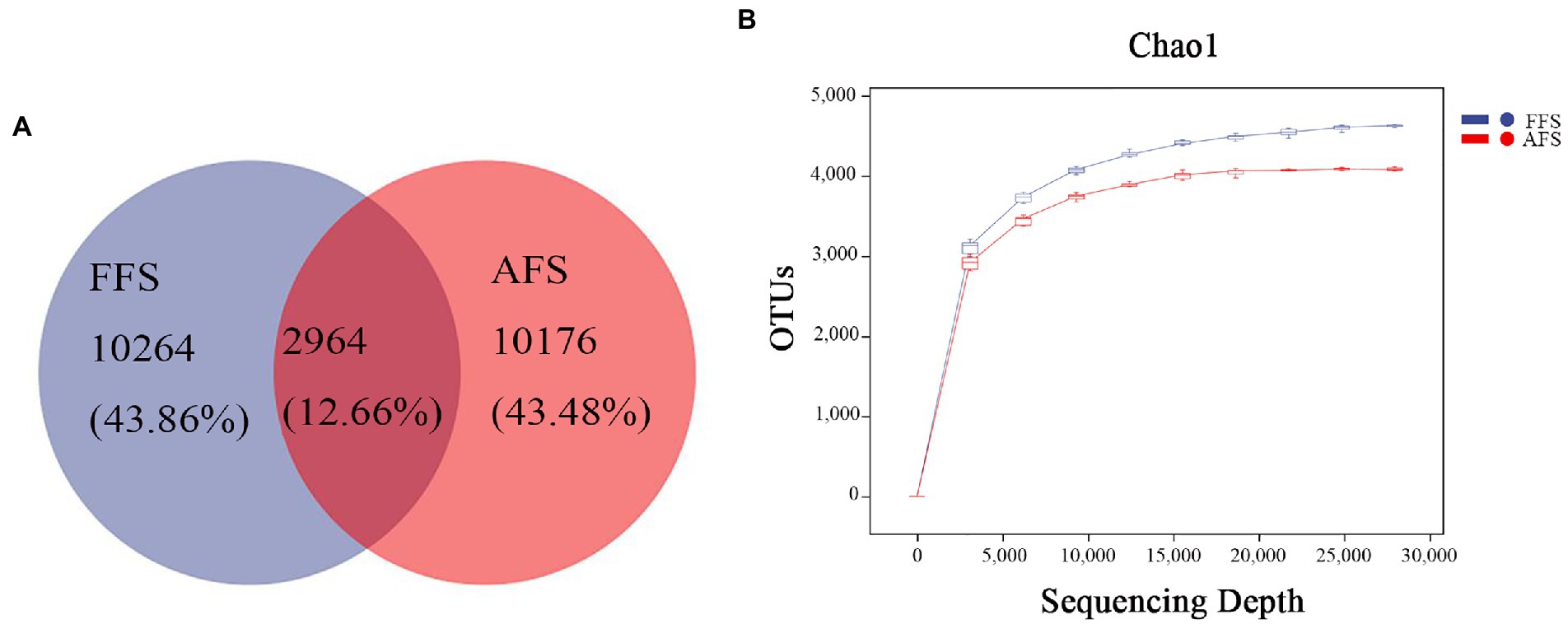
Figure 1. Venn diagrams (A) and the sparse curve (B) in bacterial communities in the selective-farming farmlands (FFS) and the abandoned farmlands (AFS). Different colored shapes represent different groups.
Alpha diversity between FFS and AFS was estimated by Chao1 (Figure 2A), Shannon (Figure 2B), Simpson (Figure 2C), and Pielou−e indexes (Figure 2D). These indexes in the FFS were significantly higher than in AFS (Kruskal–Wallis tests, p < 0.05; Figure 2).
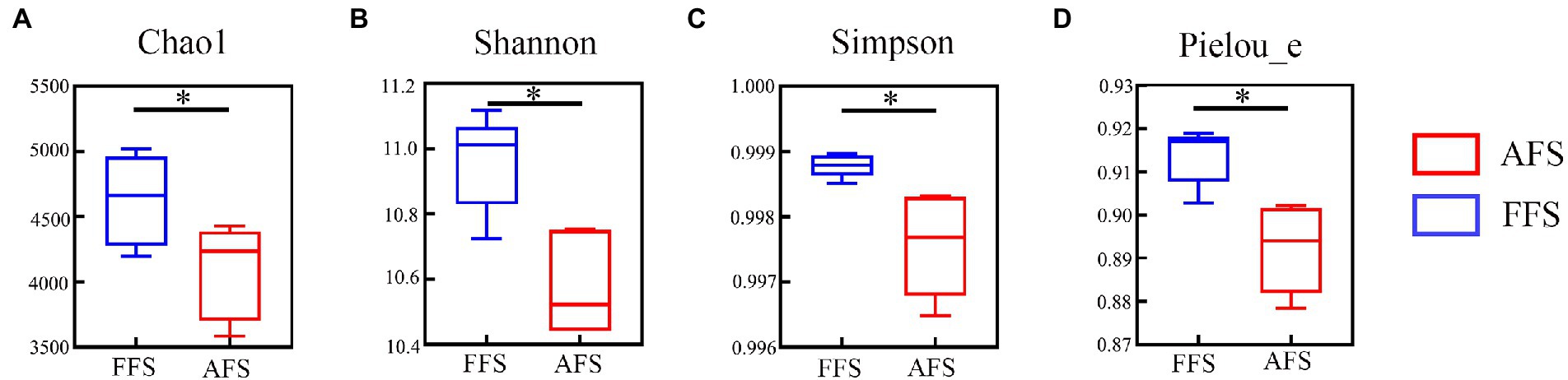
Figure 2. Alpha diversity of soil bacterial communities (A-D) in the FFS and the AFS. *p < 0.05, mean ± SD, Kruskal-Wallis test.
Beta diversity was assessed to quantify the impact of farming methods on the soil bacterial community structure (Figure 3). The PCoA plots indicated that the soil bacterial communities could be divided into two groups (Figure 3). PCoA plots could explain 64.9% (PC1) and 17.6% (PC2) of the variation in soil bacteria, respectively. NMDS plots showed that there were some inter-group differences (stress = 0.00237), in which FFS and AFS did not overlap, indicating that the soil bacterial community of FFS was significantly different from that of AFS (Figure 3).
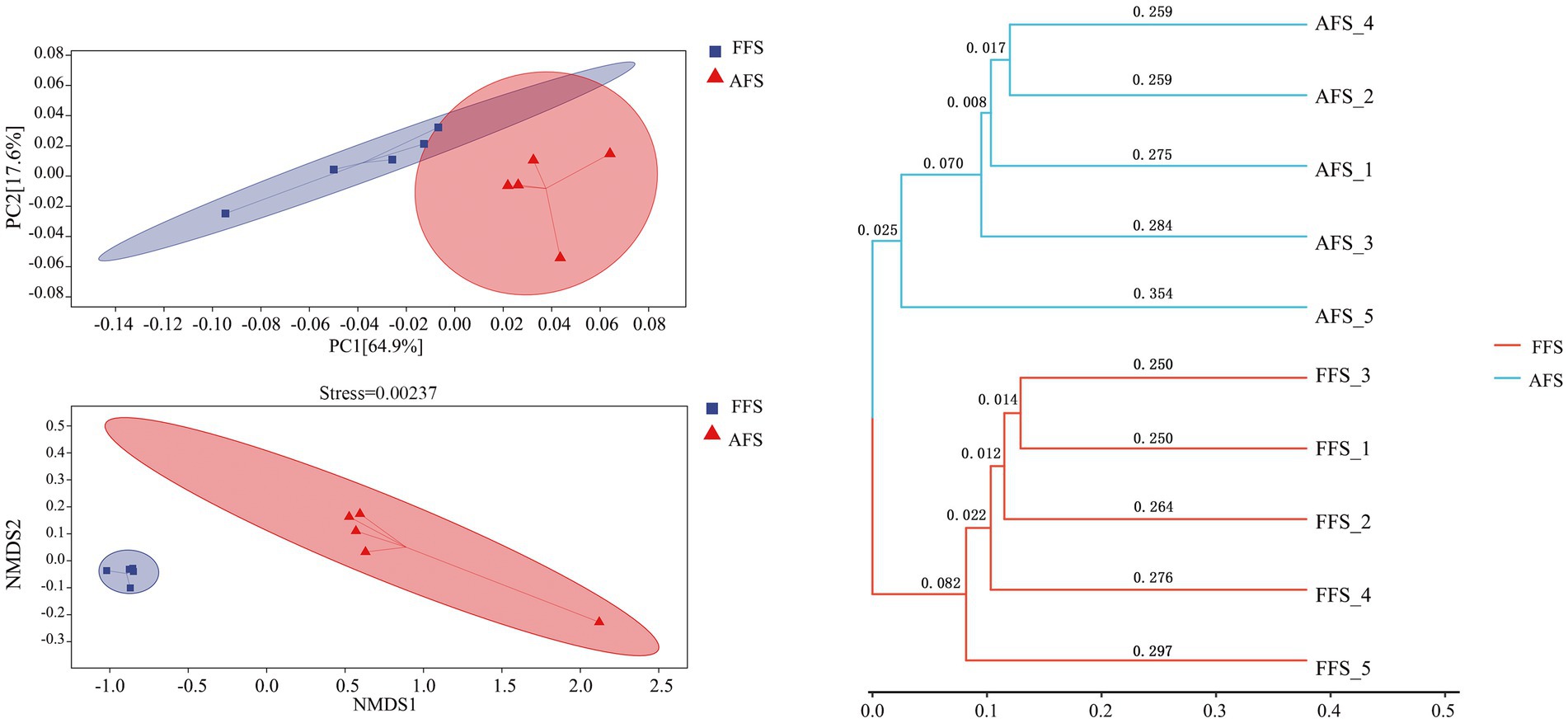
Figure 3. Principal coordinates analysis (PCoA) and non-metric multidimensional scaling analysis (NMDS) based on Bray–Curtis distance represent the differences in the soil bacteria between the FFS and the AFS. The hierarchical cluster analysis shows clusters of bacterial communities based on weighted UniFrac with 100% support at all nodes. Different colored shapes represent different groups.
Taxonomic composition analysis
To further study the change in soil bacteria between FFS and AFS, we analyzed the compositional changes of soil bacteria in different categories (Figures 4A,B). At the phylum level, 10 domain bacterial phyla (relative abundance >1% in at least one sample) were obtained, including Proteobacteria, Actinobacteria, Chloroflexi, Acidobacteria, Firmicutes, Gemmatimonadetes, Bacteroidetes, Patescibacteria, Cyanobacteria, and Rokubacteria (Figure 4A). The dominant phyla with relative abundances >10% were Proteobacteria (29.46%–36.75%) and Actinobacteria (23.14%–34.66%; Figure 4C). Proteobacteria, the most dominant bacteria, showed 33.90% and 32.14% abundances in AFS and FFS, respectively. Notably, the abundance of Actinobacteria, Chloroflexi, Acidobacteria, Firmicutes, Gemmatimonadetes, Bacteroidetes, and Rokubacteria showed significant differences between FFS and AFS (Kruskal–Wallis test, p < 0.05, Figure 4C). Compared to AFS, the relative abundances of Actinobacteria, Firmicutes, and Bacteroidetes significantly decreased in FFS, while that of Chloroflexi, Acidobacteria, Gemmatimonadetes, and Rokubacteria significantly increased in FFS (Kruskal–Wallis test, p < 0.05, Figure 4C). The seed network diagram showed that Actinobacteria was a node phylum in the networks under FFS and AFS (Supplementary Figure S2).
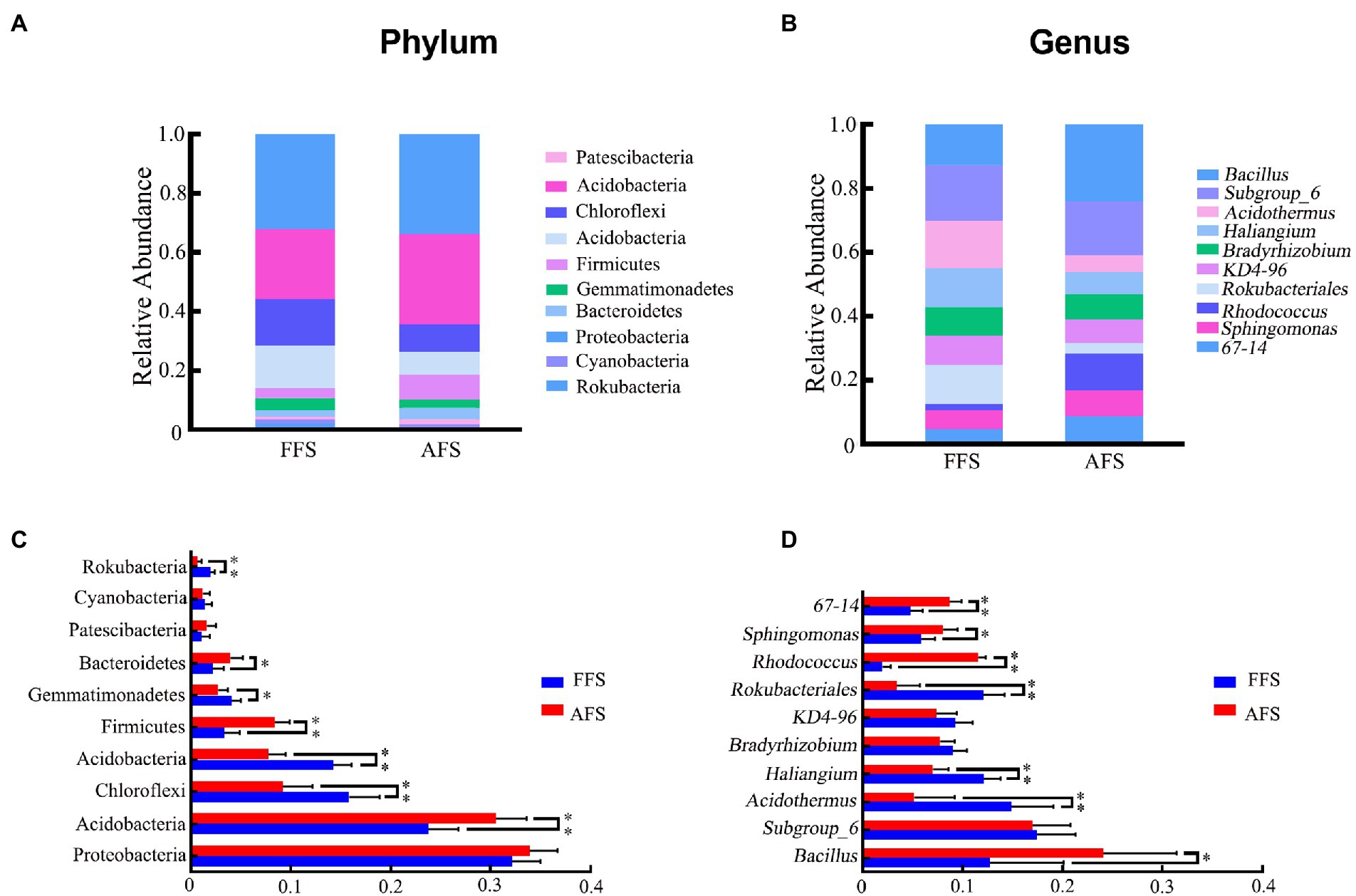
Figure 4. Relative abundance of 10 dominant bacterial phyla (A, C) and 10 dominant genera (B, D) (relative abundance >1% in at least one sample) in the soil bacteria for the FFS and the AFS (mean ± SD, Kruskal–Wallis test, *p < 0.05, **p < 0.01). Different colored shapes represent different phyla or genus.
At the genus level, the top 10 identified genera were Bacillus, Subgroup_6, Acidothermus, Haliangium, Bradyrhizobium, KD4-96, Rokubacteriales, Rhodococcus, Sphingomonas, and 67-14 (Figure 4B). Bacillus, Sphingomonas, 67-14, Acidothermus, Haliangium, Rokubacteriales, and Rhodococcus showed significant differences in abundance between FFS and AFS (Kruskal–Wallis test, p < 0.05, Figure 4D). In addition, the average abundances of Bacillus, Rhodococcus, Sphingomonas, and 67-14 were significantly lower in FFS than in AFS (Kruskal–Wallis test, p < 0.05, Figure 4D). On the contrary, the average abundances of Acidothermus, Haliangium, and Rokubacteriales were significantly higher in FFS compared to AFS (Kruskal–Wallis test, p < 0.05, Figure 4D).
To compare the differences in the domain species composition of soil bacteria at the genus level between FFS and AFS, the top 20 genera with average abundances were selected as the analysis objects, and their abundance data were used to draw the genus-level species composition heat map of species clusters (Figure 5). Through horizontal comparison, the top 20 genera in average abundance showed obvious differences between FFS and AFS.
The redundancy analysis (RDA) explained 78.69% (RDA1) and 12.61% (RDA2) of the variation, respectively (Figure 6). Figure 6 shows that the heavy metals (Sb, Hg, As, Cd, and Pb) and soil indicators (AN) were positively correlated with Cyanobacteria, Rokubacteria, Gemmatimonadetes, Acidobacteria, and Chloroflexi. Heavy metal Zn and soil indicators (AK and AP) were positively correlated with Patescibacteria, Bacteroidetes, Firmicutes, Actinobacteria, and Proteobacteria (Figure 6).
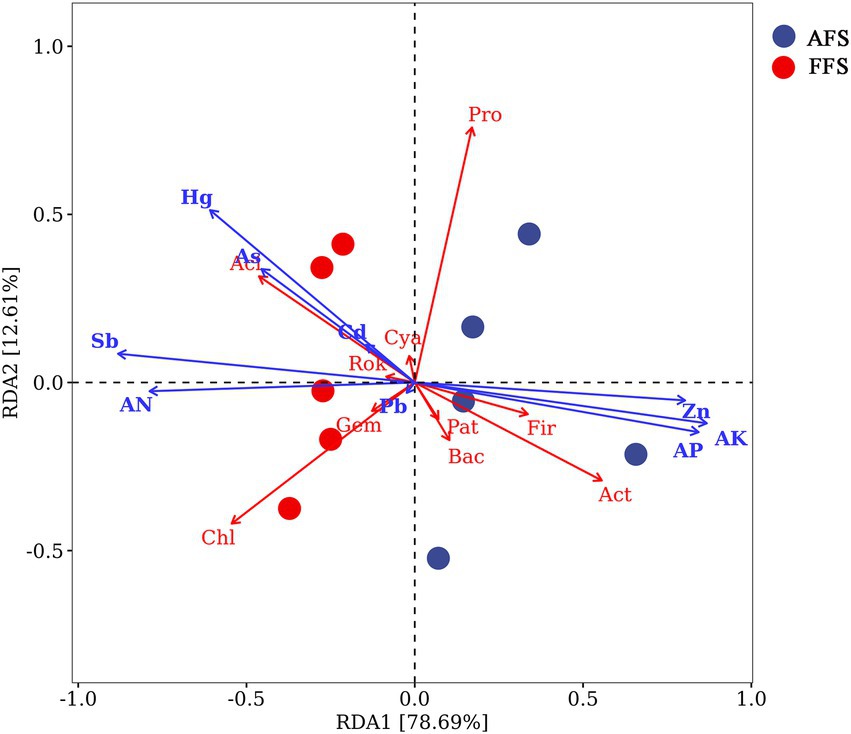
Figure 6. Redundancy analysis (RDA) of bacterial communities using soil properties as environmental parameters in the FFS and the AFS. The different colored points represent the two different types of management. The arrows represent soil properties, including available phosphorus (AP), available nitrogen (AN), and available potassium (AK). The two different circle colors represent FFS and AFS. On the RDA axis, the relationships between environmental variables and the axis were determined by arrow length and angle size. AK, available K; AN, available N; AP, available P; Aci, Acidobacteria; Act, Actinobacteria; Bac, Bacteroidetes; Chl, Chloroflexi; Cya, Cyanobacteria; Fir, Firmicutes; Gem, Gemmatimonadetes; Rok, Rokubacteria; Pat, Patescibacteria; and Pro, Proteobacteria.
Discussion
Soil quality is important for plant establishment and growth (Shrestha et al., 2019). Selective planting in the Sb mining area significantly decreased the Zn concentration and increased Sb concentration, while other heavy metals (e.g., As, Cd, Pb, and Hg) showed no significant difference when compared to land abandonment. These results indicate that the effects of AFS and FFS on soil heavy metals were diverse. The vegetation of abandoned farmland is formed with natural succession, which is a form of self-repair for farmland and can lead to farmland restoration to some extent. There are many changes in plant compositions in the process of succession, and these plants have different absorption capacities for soil heavy metals. Our previous investigation found that some plants (e.g., B. nivea, Pteridium aquilinum var. latiusculum) have a high ability to absorb and transport the heavy metal Sb (Bai et al., 2021). The emergence of these plants with high Sb enrichment capacity during natural restoration may be the main reason for the obvious decline of Sb content in abandoned farmland. In addition, the investigation of Tang et al. (2020) on the regional distribution characteristics of heavy metals in Xikuangshan also showed that the contents of Sb in different land use were significantly different, among which the contents of ecological restoration grassland were relatively low. Interestingly, farmed farmland significantly reduces the content of the heavy metal Zn, which means that selective farming of maize and sweet potato may have the ability to absorb and transport Zn. Previous study also showed that crops (including maize and sweet potato) can absorb zinc in soil, and the absorption capacity at the root-soil interface is affected by root dry weight and morphology (e.g.,Ogiyama et al., 2010; Zhang et al., 2017). The application of farming fertilizer and soil microorganisms can affect the dry weight and morphology of roots in maize and sweet potato, and then change the accumulation of zinc in these crops (Ogiyama et al., 2010; Zhang et al., 2017).
Soil properties (e.g., heavy metals and soil physicochemical properties) are the main drivers of soil bacterial communities (Sibanda et al., 2019; Deng et al., 2020b; Duan et al., 2021; Khmelevtsova et al., 2022). Changes in soil properties caused by different agricultural land activities, such as abandonment and selective farming, are an important driving force for bacterial community dynamics. Our results observed that FFS increased the bacterial alpha diversity including Chao1 index, Simpson index, Shannon index and Pielou−e index and changed the beta diversity. These bacterial change might be caused by the farmland fertilization. Some studies documented that the farmland fertilization could increase bacterial alpha diversity and change bacterial beta diversity (Huang et al., 2019; Mei et al., 2021).
At the phylum level, Proteobacteria and Acidobacteria are the abundant phyla in both AFS and FFS, indicating that they have high survival and reproduction ability in the metal mining areas. This result is similar to other studies, showing these are the dominant phyla in Sb mines (Deng et al., 2020b; Duan et al., 2021), Zn mines (Luo et al., 2018), Cu mines (Sun et al., 2018), and gold mines (Sibanda et al., 2019). In this study, selective planting led to a significant decrease in the relative abundance of Actinobacteria, Firmicutes, and Bacteroidetes, and an increase in the relative abundance of Chloroflexi, Acidobacteria, Gemmatimonadetes, and Rokubacteria. A 40-year farmland fertilization experiment in Jilin Province, China also showed a significant increase in bacterial relative abundance of Acidobacteria and Gemmatimonadetes and a significant decrease in the Actinobacteria, Bacteroidetes and Firmicutes (Mei et al., 2021). In addition, our results also observed that Actinobacteria existed as a node phylum in the dominant seed network. The significant differences of this phylum caused by different farmland farming will further lead to the differences in the composition and abundance of other bacteria in the soil.
At the genus level, FFS significantly decreased the average abundances of Bacillus, Rhodococcus, Sphingomonas, and 67-14, and increased the average abundances of Haliangium, Acidothermus, and Rokubacteriales. The abundance of different kinds of bacteria depends on the soil’s physical and chemical properties resulting from farming methods. For example, Bacillus, a bacterium with a strong tolerance for heavy metals, can effectively alleviate the toxicity of heavy metals for plants by biological accumulation and biotransformation (Aka and Babalola, 2016; Jan et al., 2019). Our previous research confirmed that Bacillus is more abundant at the beginning of succession with higher heavy metal pollution (Duan et al., 2021). Sphingomonas is favored in heavy metal contaminated soil and possess the ability to degrade pollutants including fungicides (Leys et al., 2004; Degrune et al., 2017). Studies have also confirmed that agricultural planting can significantly change the abundance of this genus (Babin et al., 2019; Chen et al., 2019). Haliangium has a higher abundance in the fertile soil of continuous cropping strawberries and mangoes (Li et al., 2019). Our previous research on rhizosphere bacteria in different restoration stages of the Sb mine confirmed that natural restoration would improve soil conditions and soil fertility, resulting in a significant increase in Haliangium content in the Sb mining area (Duan et al., 2021). So, we speculate that Haliangium may prefer soils with higher soil fertility. In addition, to our knowledge, there were no reports on the significant changes in the abundance of Rhodococcus, 67-14, Acidothermus, and Rokubacteriales caused by agricultural management, further tests were needed to clarify the potential link to agricultural management-driven soil bacterial properties.
Our experiments indicate that selective farming in the mining area not only changed the bacterial composition, but also significantly improved the soil bacterial richness, species evenness, and species diversity. The soil bacterial composition and diversity was affected by multiple environmental factors (e.g., Griffiths et al., 2011; Zeng et al., 2016). The main reasons for differences between planting methods may be the following:
1. Farming methods. The natural restoration of abandoned farmland polluted by heavy metals can lead to a change in bacterial community in the soil. The cultivation of agricultural soil polluted by heavy metals will also lead to a change in soil bacterial community composition. The research of Le Guillou et al. (2019) indicated that farming practices could effectively shape the richness and diversity of the soil bacterial community, and cultivation during rotation has an important impact on the formation of the bacterial community.
2. Heavy metals. Different concentrations of heavy metals can lead to various reactions of bacteria (Ippolito et al., 2010; Cavani et al., 2016). For example, Lejon et al. (2010) observed that Cu reduced the bacterial diversity in poor organic soil; on the contrary, Cu increased the bacterial diversity in soil rich in organic modifiers. The redundancy analysis from our experiment showed that Pb and Zn contents were closely related to the composition of the bacterial community in AFS, while the heavy metals Sb, As and Hg were closely related to the bacterial composition in FFS. In addition, studies have confirmed that heavy metals can form new complexes with organic matter, minerals, salts, and other components (Zhu et al., 2020). Forms of heavy metals also have various impacts on soil bacteria.
3. Soil fertility. The higher soil fertility of selective-farming farmland may improve bacterial diversity. Nitrogen availability is a limiting factor for bacterial activity in disturbed ecosystems (Raiesi, 2012). Large amounts of urea input into these N-poor soils may stimulate bacterial activity and respiration (Raiesi, 2004). Soil and crop management measures, including tillage and nitrogen fertilizer, can affect soil bacterial diversity by affecting the content and distribution of soil N, P, and K (García-Orenes et al., 2010; Castellano-Hinojosa et al., 2021; Khmelevtsova et al., 2022). Chao et al. (2016) and Santonja et al. (2018) confirmed that the composition of the bacterial community is mainly driven by soil nutrients at an abandoned mine site. Similarly, most of the communities were positively correlated with available N, P, and K in our study, which we held to be evidence that different farming methods indirectly regulated the composition and diversity of bacteria by changing soil factors.
Conclusion
This study provides evidence to support the hypothesis that selective farming has a positive impact on measured soil quality indicators, compared to abandoned farmland. The abundances of Haliangium, Acidothermus, and Rokubacteriales in selective-farming farmlands significantly increased, while that of Bacillus, Rhodococcus, Sphingomonas, and 67-14 showed a significant decrease. The improvement of nutritional status and the increase in bacterial diversity in FFS showed that appropriate agricultural practice is conducive to the gradual restoration of cultivated land. Further investigation integrating bacterial functions and bacterial community analysis may be helpful to evaluate the impact of different agricultural practices on soil bacterial quality and function.
Data availability statement
The datasets presented in this study can be found in online repositories. The names of the repository/repositories and accession number(s) can be found at: https://www.ncbi.nlm.nih.gov/, SAMN24619870, SAMN24619871, SAMN24619873, SAMN24619874, SAMN24619875, SAMN24619876, SAMN24619877, SAMN24619878, and SAMN24619879. The sequences from rhizosphere bacterial samples are available at NCBI SRA: BioProject PRJNA794295.
Author contributions
RD conceived the research idea, conducted the field work and experiments, contributed to visualization, writing, and editing. YD, YZ, and YL conceived the research idea, writing, and editing. ZC contributed to sampling and conducted the statistical analysis. WH contributed to data curation and investigation. LY and GX conducted the field work and experiments. All authors contributed to the article and approved the submitted version.
Funding
This work was supported by the Science and Technology Innovation Project of Hunan (2021NK2030, 2020NK2001, and 2021RC1016), the Graduate Innovation Project of Hunan Province (CX20211219), and the Agricultural Science and Technology Innovation Project of Hunan Province (2020cx84).
Conflict of interest
The authors declare that the research was conducted in the absence of any commercial or financial relationships that could be construed as a potential conflict of interest.
Publisher’s note
All claims expressed in this article are solely those of the authors and do not necessarily represent those of their affiliated organizations, or those of the publisher, the editors and the reviewers. Any product that may be evaluated in this article, or claim that may be made by its manufacturer, is not guaranteed or endorsed by the publisher.
Supplementary materials
The Supplementary materials for this article can be found online at: https://www.frontiersin.org/articles/10.3389/fmicb.2022.953624/full#supplementary-material
Supplementary Figure S1 | Site location of the study area.
Supplementary Figure S2 | The seed network of dominant bacterial phyla.
Abbreviations
AFS, Abandoned farmlands; FFS, Selective-farming farmlands; AN, Available N; AP, Available P; AK, Available K; PCoA, Principal coordinate analysis; RDA, Redundancy analysis.
References
Aka, R. J. N., and Babalola, O. O. (2016). Effect of bacterial inoculation of strains of Pseudomonas aeruginosa, Alcaligenes feacalis and Bacillus subtilis on germination, growth and heavy metal (cd, Cr, and Ni) uptake of Brassica juncea. Int. J. Phytoremediation 18, 200–209. doi: 10.1080/15226514.2015.1073671
Babin, D., Deubel, A., Jacquiod, S., Sørensen, S. J., Geistlinger, J., Grosch, R., et al. (2019). Impact of long-term agricultural management practices on soil prokaryotic communities. Soil Biol. Biochem. 129, 17–28. doi: 10.1016/j.soilbio.2018.11.002
Bai, J., Zhang, W., Zhang, S. S., Xiang, G. H., Xu, H. J., Zheng, Y., et al. (2021). Ecological and health risk assessment of heavy metals in soils and their distribution in dominant plants in the Xikuangshan mining area. J. Agr. Resour. Environ. 38, 411–421. doi: 10.13254/j.jare.2020.0783
Castellano-Hinojosa, A., Strauss, S. L., González-López, J., and Bedmar, E. J. (2021). Changes in the diversity and predicted functional composition of the bulk and rhizosphere soil bacterial microbiomes of tomato and common bean after inorganic N-fertilization. Rhizosphere 18:100362. doi: 10.1016/j.rhisph.2021.100362
Cavani, L., Manici, L. M., Caputo, F., Peruzzi, E., and Ciavatta, C. (2016). Ecological restoration of a copper polluted vineyard: long-term impact of farmland abandonment on soil bio-chemical properties and microbial communities. J. Environ. Manage. 182, 37–47. doi: 10.1016/j.jenvman.2016.07.050
Chao, Y., Liu, W., Chen, Y., Chen, W., Zhao, L., Ding, Q., et al. (2016). Structure, variation, and co-occurrence of soil microbial communities in abandoned sites of a rare earth elements mine. Environ. Sci. Technol. 50, 11481–11490. doi: 10.1021/acs.est.6b02284
Chen, Y., Liu, D., Ma, J., Jin, B., Peng, J., and He, X. (2021). Assessing the influence of immobilization remediation of heavy metal contaminated farmland on the physical properties of soil. Sci. Total Environ. 781:146773. doi: 10.1016/j.scitotenv.2021.146773
Chen, L., Redmile-Gordon, M., Li, J., Zhang, J., Xin, X., Zhang, C., et al. (2019). Linking cropland ecosystem services to microbiome taxonomic composition and functional composition in a sandy loam soil with 28-year organic and inorganic fertilizer regimes. Appl. Soil Ecol. 139, 1–9. doi: 10.1016/j.apsoil.2019.03.011
Degrune, F., Theodorakopoulos, N., Colinet, G., Hiel, M. P., Bodson, B., Taminiau, B., et al. (2017). Temporal dynamics of soil microbial communities below the seedbed under two contrasting tillage regimes. Front. Microbiol. 8:1127. doi: 10.3389/fmicb.2017.01127
Deng, J., Bai, X., Zhou, Y., Zhu, W., and Yin, Y. (2020a). Variations of soil microbial communities accompanied by different vegetation restoration in an open-cut iron mining area. Sci. Total Environ. 704:135243. doi: 10.1016/j.scitotenv.2019.135243
Deng, R., Chen, Y., Deng, X., Huang, Z., Zhou, S., Ren, B., et al. (2021). A critical review of resistance and oxidation mechanisms of Sb-oxidizing bacteria for the bioremediation of Sb (III) pollution. Front. Microbiol. 12:738596. doi: 10.3389/fmicb.2021.738596
Deng, R., Tang, Z., Hou, B., Ren, B., Wang, Z., Zhu, C., et al. (2020b). Microbial diversity in soils from antimony mining sites: geochemical control promotes species enrichment. Environ. Chem. Lett. 18, 911–922. doi: 10.1007/s10311-020-00975-1
Deng, L., Zeng, G., Fan, C., Lu, L., Chen, X., Chen, M., et al. (2015). Response of rhizosphere microbial community structure and diversity to heavy metal co-pollution in arable soil. Appl. Microbiol. Biotechnol. 99, 8259–8269. doi: 10.1007/s00253-015-6662-6
Duan, R., Lin, Y., Zhang, J., Huang, M., Du, Y., Yang, L., et al. (2021). Changes in diversity and composition of rhizosphere bacterial community during natural restoration stages in antimony mine. PeerJ 9:e12302. doi: 10.7717/peerj.12302
Fouts, D. E., Szpakowski, S., Purushe, J., Torralba, M., Waterman, R. C., MacNeil, M. D., et al. (2012). Next generation sequencing to define prokaryotic and fungal diversity in the bovine rumen. PLoS One 7:e48289. doi: 10.1371/journal.pone.0048289
García-Orenes, F., Guerrero, C., Roldán, A., Mataix-Solera, J., Cerdá, A., Campoy, M., et al. (2010). Soil microbial biomass and activity under different agricultural management systems in a semiarid Mediterranean agroecosystem. Soil Tillage Res. 109, 110–115. doi: 10.1016/j.still.2010.05.005
Griffiths, R. I., Thomson, B. C., James, P., Bell, T., Bailey, M., and Whiteley, A. S. (2011). The bacterial biogeography of British soils. Environ. Microbiol. 13, 1642–1654. doi: 10.1111/j.1462-2920.2011.02480.x
Guo, D., Fan, Z., Lu, S., Ma, Y., Nie, X., Tong, F., et al. (2019). Changes in rhizosphere bacterial communities during remediation of heavy metal-accumulating plants around the Xikuangshan mine in southern China. Sci. Rep. 9, 1947–1911. doi: 10.1038/s41598-018-38360-2
Guo, X. F., Wei, Z. B., Wu, Q. T., Qiu, J. R., and Zhou, J. L. (2011). Cadmium and zinc accumulation in maize grain as affected by cultivars and chemical fixation amendments. Pedosphere 21, 650–656. doi: 10.1016/S1002-0160(11)60167-7
Han, J., Liang, L., Zhu, Y., Xu, X., Wang, L., Shang, L., et al. (2022). Heavy metal (loid) s in farmland soils on the karst plateau, Southwest China: an integrated analysis of geochemical baselines, source apportionment, and associated health risk. Land Degrad. Dev. 33, 1689–1703. doi: 10.1002/ldr.4257
Hou, D., Ding, Z., Li, G., Wu, L., Hu, P., Guo, G., et al. (2018). A sustainability assessment framework for agricultural land remediation in China. Land Degrad. Dev. 29, 1005–1018. doi: 10.1002/ldr.2748
Huang, Q., Wang, J., Wang, C., and Wang, Q. (2019). The 19-years inorganic fertilization increased bacterial diversity and altered bacterial community composition and potential functions in a paddy soil. Appl. Soil Ecol. 144, 60–67. doi: 10.1016/j.apsoil.2019.07.009
Huang, F., Zhou, H., Gu, J., Liu, C., Yang, W., Liao, B., et al. (2020). Differences in absorption of cadmium and lead among fourteen sweet potato cultivars and health risk assessment. Ecotox. Environ. Safe. 203:111012. doi: 10.1016/j.ecoenv.2020.111012
Ippolito, J. A., Ducey, T., and Tarkalson, D. (2010). Copper impacts on corn, soil extractability, and the soil bacterial community. Soil Sci. 175, 586–592. doi: 10.1097/SS.0b013e3181fe2960
Jan, M., Shah, G., Masood, S., Shinwari, K. I., and Jamil, M. (2019). Bacillus cereus enhanced phytoremediation ability of rice seedlings under cadmium toxicity. Biomed. Res. Int. 2019, 1–12. doi: 10.1155/2019/8134651
Jin, C. S., Deng, R. J., Ren, B. Z., Hou, B. L., and Hursthouse, A. S. (2020). Enhanced biosorption of Sb (III) onto living rhodotorula mucilaginosa strain DJHN070401: optimization and mechanism. Curr. Microbiol. 77, 2071–2083. doi: 10.1007/s00284-020-02025-z
Jusselme, M. D., Miambi, E., Mora, P., Diouf, M., and Rouland-Lefèvre, C. (2013). Increased lead availability and enzyme activities in root-adhering soil of Lantana camara during phytoextraction in the presence of earthworms. Sci. Total Environ. 445–446, 101–109. doi: 10.1016/j.scitotenv.2012.12.054
Khmelevtsova, L. E., Sazykin, I. S., Azhogina, T. N., and Sazykina, M. A. (2022). Influence of agricultural practices on bacterial community of cultivated soils. Agriculture 12:371. doi: 10.3390/agriculture12030371
Le Guillou, C., Chemidlin Prévost-Bouré, N., Karimi, B., Akkal-Corfini, N., Dequiedt, S., Nowak, V., et al. (2019). Tillage intensity and pasture in rotation effectively shape soil microbial communities at a landscape scale. MicrobiologyOpen 8:e00676. doi: 10.1002/mbo3.676
Lejon, D. P. H., Pascault, N., and Ranjard, L. (2010). Differential copper impact on density, diversity and resistance of adapted culturable bacterial populations according to soil organic status. Eu. J. Soil Biol. 46, 168–174. doi: 10.1016/j.ejsobi.2009.12.002
Leys, N. M. E. J., Ryngaert, A., Bastiaens, L., Verstraete, W., Top, E. M., and Springael, D. (2004). Occurrence and phylogenetic diversity of Sphingomonas strains in soils contaminated with polycyclic aromatic hydrocarbons. Appl. Environ. Microbiol. 70, 1944–1955. doi: 10.1128/AEM.70.4.1944-1955.2004
Li, X. (2019). Technical solutions for the safe utilization of heavy metal-contaminated farmland in China: a critical review. Land Degrad. Dev. 30, 1773–1784. doi: 10.1002/ldr.3309
Li, M. J., Wang, Q. X., Liu, Z. B., Pan, X. X., and Zhang, Y. Y. (2019). Silicon application and related changes in soil bacterial community dynamics reduced ginseng black spot incidence in Panax ginseng in a short-term study. BMC Microbiol. 19:263. doi: 10.1186/s12866-019-1627-z
Luo, Y. F., Wu, Y. G., Qiu, J., Wang, H., and Yang, L. (2018). Bacterial community structure and diversity responses to the direct revegetation of an artisanal zinc smelting slag after 5 years. Environ. Sci. Pollut. Res. Int. 25, 14773–14788. doi: 10.1007/s11356-018-1573-6
Mei, N., Zhang, X., Wang, X., Peng, C., Gao, H., Zhu, P., et al. (2021). Effects of 40 years applications of inorganic and organic fertilization on soil bacterial community in a maize agroecosystem in Northeast China. Eur. J. Agron. 130:126332. doi: 10.1016/j.eja.2021.126332
Nivelle, E., Verzeaux, J., Habbib, H., Kuzyakov, Y., Decocq, G., Roger, D., et al. (2016). Functional response of soil microbial communities to tillage, cover crops and nitrogen fertilization. Appl. Soil Ecol. 108, 147–155. doi: 10.1016/j.apsoil.2016.08.004
Ogiyama, S., Suzuki, N., Sakamoto, K., and Inubushi, K. (2010). Absorption of zinc and copper by maize and sweet potato in an arable field after pig farm manure application—contribution of arbiscular mycorrhizal fungi and effects of wood charcoal application. HortResearch 64, 9–18.
Raiesi, F. (2004). Soil properties and N application effects on microbial activities in two winter wheat cropping systems. Biol. Fertil. Soils 40, 88–92. doi: 10.1007/s00374-004-0741-7
Raiesi, F. (2006). Carbon and N mineralization as affected by soil cultivation and crop residue in a calcareous wetland ecosystem in Central Iran. Agric. Ecosyst. Environ. 112, 13–20. doi: 10.1016/j.agee.2005.07.002
Raiesi, F. (2012). Soil properties and C dynamics in abandoned and cultivated farmlands in a semi-arid ecosystem. Plant Soil 351, 161–175. doi: 10.1007/s11104-011-0941-5
Santonja, M., Foucault, Q., Rancon, A., Gauquelin, T., Fernandez, C., Baldy, V., et al. (2018). Contrasting responses of bacterial and fungal communities to plant litter diversity in a Mediterranean oak forest. Soil Biol. Biochem. 125, 27–36. doi: 10.1016/j.soilbio.2018.06.020
Shrestha, P., Gautam, R., and Ashwath, N. (2019). Effects of agronomic treatments on functional diversity of soil microbial community and microbial activity in a revegetated coal mine spoil. Geoderma 338, 40–47. doi: 10.1016/j.geoderma.2018.11.038
Sibanda, T., Selvarajan, R., Msagati, T., Venkatachalam, S., and Meddows-Taylord, S. (2019). Defunct gold mine tailings are natural reservoir for unique bacterial communities revealed by high-throughput sequencing analysis. Sci. Total Environ. 650, 2199–2209. doi: 10.1016/j.scitotenv.2018.09.380
Sun, L., Li, J., Wang, Q., Zhang, Y., Xu, Z., Wang, R., et al. (2020). The effects of eight years of conservation tillage on the soil physicochemical properties and bacterial communities in a rain-fed agroecosystem of the loess plateau, China. Land Degrad. Dev. 31, 2475–2489. doi: 10.1002/ldr.3619
Sun, X. Y., Zhou, Y. L., Tan, Y. J., Wu, Z. X., Lu, P., Zhang, G. H., et al. (2018). Restoration with pioneer plants changes soil properties and remodels the diversity and structure of bacterial communities in rhizosphere and bulk soil of copper mine tailings in Jiangxi Province, China. Environ. Sci. Pollut. Res. Int. 25, 22106–22119. doi: 10.1007/s11356-018-2244-3
Tang, Z. E., Deng, R. J., Zhang, J., Ren, B. Z., and Hursthouse, A. (2020). Regional distribution characteristics and ecological risk assessment of heavy metal pollution of different land use in an antimony mining area–Xikuangshan, China. Hum. Ecol. Risk Assess. 26, 1779–1794. doi: 10.1080/10807039.2019.1608423
Wang, H., Han, J., Deng, N., and An, S. (2019). Effects of the natural restoration time of abandoned farmland in a semiarid region on the soil denitrification rates and abundance and community structure of denitrifying bacteria. Appl. Microbiol. Biotechnol. 103, 1939–1951. doi: 10.1007/s00253-018-09575-0
Wang, A. Y., Wang, M. Y., Liao, Q., and He, X. Q. (2016). Characterization of Cd translocation and accumulation in 19 maize cultivars grown on Cd-contaminated soil: implication of maize cultivar selection for minimal risk to human health and for phytoremediation. Environ. Sci. Pollut. Res. 23, 5410–5419. doi: 10.1007/s11356-015-5781-z
Zeng, Q., Dong, Y., and An, S. (2016). Bacterial community responses to soils along a latitudinal and vegetation gradient on the loess plateau, China. PLoS One 11:e0152894. doi: 10.1371/journal.pone.0152894
Zhang, W., Chen, X. X., Liu, Y. M., Liu, D. Y., Chen, X. P., and Zou, C. Q. (2017). Zinc uptake by roots and accumulation in maize plants as affected by phosphorus application and arbuscular mycorrhizal colonization. Plant Soil 413, 59–71. doi: 10.1007/s11104-017-3213-1
Zhang, M., and Fang, L. (2007). Effect of tillage, fertilizer and green manure cropping on soil quality at an abandoned brick making site. Soil Tillage Res. 93, 87–93. doi: 10.1016/j.sti11.2006.03.016
Zhou, L. X., and Ding, M. (2007). Soil microbial characteristics as bioindicators of soil health. Biodivers. Sci. 15, 162–171. doi: 10.1360/biodiv.060290
Zhou, S., Hursthouse, A., and Chen, T. (2019). Pollution characteristics of Sb, As, Hg, Pb, Cd, and Zn in soils from different zones of Xikuangshan antimony mine. J. Anal. Methods. Chem. 2019:2754385. doi: 10.1155/2019/2754385
Keywords: soil pollution, agricultural practices, bacteria, 16S rRNA, heavy metal
Citation: Duan R, Du Y, Chen Z, Zhang Y, Hu W, Yang L, Xiang G and Luo Y (2022) Diversity and composition of soil bacteria between abandoned and selective-farming farmlands in an antimony mining area. Front. Microbiol. 13:953624. doi: 10.3389/fmicb.2022.953624
Edited by:
Saowaluck Tibpromma, Qujing Normal University, ChinaReviewed by:
Suhail Asad, Yunnan Agricultural University, ChinaRenjian Deng, Hunan University of Science and Technology, China
Copyright © 2022 Duan, Du, Chen, Zhang, Hu, Yang, Xiang and Luo. This is an open-access article distributed under the terms of the Creative Commons Attribution License (CC BY). The use, distribution or reproduction in other forums is permitted, provided the original author(s) and the copyright owner(s) are credited and that the original publication in this journal is cited, in accordance with accepted academic practice. No use, distribution or reproduction is permitted which does not comply with these terms.
*Correspondence: Renyan Duan, duanrenyan78@163.com; Yucai Luo, 1016484783@qq.com