- 1Department of Food Science, Czech University of Life Sciences Prague, Prague, Czechia
- 2Department of Botany, Faculty of Science, Charles University, Prague, Czechia
Cannabis preparations are gaining popularity among patients with various skin diseases. Due to the lack of scientific evidence, dermatologists remain cautious about their prescriptions. So far, only a few studies have been published about the effects of high-potency cannabis extracts on microorganisms (especially dermatophytes) causing skin problems that affect more than 25% of the worldwide population. Even though, the high-potency cannabis extracts prepared by cold extraction are mostly composed of non-psychoactive tetrahydrocannabinolic acid (THCA) and only low amount of THC, their use in topical treatment can be stigmatized. The in vitro antimicrobial and antifungal activity of two high potent cannabis strains extracted by three solvents traditionally or currently used by cannabis users (ethanol; EtOH, butane; BUT, dimethyl ether; DME) was investigated by broth dilution method. The chemical profile of cannabis was determined by high-performance liquid chromatography with ultraviolet detection and gas chromatography with mass spectrometer and flame ionization detector. The extraction methods significantly influenced chemical profile of extracts. The yield of EtOH extracts contained less cannabinoids and terpenes compared to BUT and DME ones. Most of the extracts was predominantly (>60%) composed of various cannabinoids, especially THCA. All of them demonstrated activity against 18 of the 19 microorganisms tested. The minimal inhibitory concentrations (MICs) of the extracts ranged from 4 to 256 μg/mL. In general, the bacteria were more susceptible to the extracts than dermatophytes. Due to the lower content of biologically active substances, the EtOH extracts were less effective against microorganisms. Cannabis extracts may be of value to treat dermatophytosis and other skin diseases caused by various microorganisms. Therefore, they could serve as an alternative or supportive treatment to commonly used antibiotics.
Introduction
Human is a unique organ inhabited by a diverse collection of bacteria, fungi, and viruses where the microorganisms usually live in homeostasis (Schommer and Gallo, 2013). Dysbiosis of the microbiome can lead to the development of various skin diseases, which are understood as the 4th most common contributors to the global burden of non-fatal diseases worldwide (Hay et al., 2014). Among them, skin infections of fungal and bacterial origin are the most common ones. Bacterial infections are commonly caused by, e.g., Staphylococcus epidermidis, S. aureus, and Streptococcus pyogenes. These opportunistic pathogens can cause serious infections, such as endocarditis, sepsis, or streptococcal toxic shock syndrome. However, more frequently they cause painful skin infections such as folliculitis, impetigo, etc. (Johansson et al., 2010; Becker et al., 2014).
The superficial fungal diseases (dermatomycosis) are predominantly caused by Epidermophyton, Microsporum or Trichophyton spp. It is estimated that 20–25% of the global population suffer with them (Havlickova et al., 2008; Panda and Verma, 2017) and due to globalization, the number of the cases of dermatophytosis is increasing (Begum et al., 2020). For example, the prevalence of dermatophytosis in India is greater than 50% (Ramaraj et al., 2016). Although the symptoms of fungal infection are generally mild and painless, their psychological and social burden on patients can be significant (Rajagopalan et al., 2018).
Nowadays, bacterial and fungal skin diseases are treated with topical or systemic antibiotics. Unfortunately, increasing antibiotic and antifungal resistance is becoming a significant worldwide problem. For example, 17–32% of clinical Trichophyton isolates in India were identified to be resistant to the widely used antibiotic terbinafine (TB) (Singh et al., 2018). The situation is even more alarming in the case of bacteria, as the increasing number of multidrug-resistant strains such as Methicillin-resistant S. aureus (MRSA) (Alves et al., 2018) is identified every year. The international strategies to tackle the antimicrobial resistance are known and slowly adopted by countries worldwide (WHO, 2019). However, there is no doubt that the discovery of new agents with antibiotic activity remains of great importance.
Cannabis sativa, after its legalization for medicinal purposes, is in the scope of many researches. It has been used in traditional medicine for various purposes including treatment of skin infections (Zuardi, 2006). Currently, cannabis is recommended for the treatment of various dermatologic conditions, i.e., psoriasis, lupus or acne, however, the scientific evidence on its activity is limited (Dhadwal and Kirchhof, 2018; Lim and Kirchhof, 2019).
Its positive effects on various diseases are attributed to cannabinoids and terpenes. Among other biological activities, antimicrobial action has been described for all of them, especially for cannabigerol (CBG), detla-9-tetrahydrocannabinol (THC), cannabigerolic acid (CBGA), and detla-9-tetrahydrocannabiolic acid (THCA) (Farha et al., 2020; Pasquali et al., 2020; Blaskovich et al., 2021) and also for most of the abundant terpenes – myrcene, limonene, β-pinene and linalool (Pepeljnjak et al., 2005; Sanguinetti et al., 2007; Singh et al., 2010). Moreover, it has been demonstrated that the synergistic effect between cannabinoids and terpenes may contribute to more effective treatment (Russo, 2011).
Unfortunately, high-potency cannabis is mainly associated with its psychoactive potential caused by THC, which is formed from THCA during the thermal processing of the naïve extract. At the same time, THCA is non-psychoactive cannabinoid with anti-inflammatory, anticancer and neuroprotective effect. For the topical application cannabis is currently prescribed in the form of dried flowers, creams, tinctures, or extracts (Zajicek et al., 2012; Peschel, 2016; Palmieri et al., 2019). Even though the extracts added to the preparations are usually not thermally processed, its topical application can be stigmatized and not acceptable for many people. Moreover, increasing trend in the use of cannabis extracts that are not heated can be observed among people (Anderson et al., 2019). The efficiency of each preparation is influenced not only by the cannabis variety (chemotype) but also by the type of extraction method, solvent used, and final formulation. Organic solvents with different polarities such as methanol, ethanol, acetone, or hexane are the most commonly used for the preparation of cannabis extracts (Wang et al., 2018). The solvent extraction is very simple; on the other hand, the solvent evaporation can be demanding.
For this reason, cannabis concentrates prepared by gases are gaining popularity among recreational users and medicinal marijuana patients (Daniulaityte et al., 2017). Supercritical CO2 extraction of cannabis become widely accepted technique in industry (Gallo-Molina et al., 2019). While recreational users prefer to use butane (Chan et al., 2017) and quite recently dimethyl ether (DME) for the extraction. Both gases are highly inflammable, butane is also highly toxic and can contain various impurities (Al-Zouabi et al., 2018). On the other hand, DME is understood to be of low toxicity and is also used in food industry for extraction of animal protein (EFSA, 2009). Therefore. could become one of the alternative techniques for cannabis extraction. So far, only a limited number of studies have investigated the influence of extraction techniques on the activity of cannabis extract. Moreover, although cannabis contains two groups of biologically active substances with high antimicrobial potential, its role in the treatment of bacterial or superficial fungal infections has also not been intensively investigated so far. Therefore, the aim of our research was to evaluate the antimicrobial and antifungal activity of two medicinal cannabis strains extracted by various methods against selected bacteria and dermatophytes.
Materials and methods
Chemicals
All solvents used for the HPLC and GC analysis were of analytical grade. Acetonitrile (ACN) and formic acid (FA) together with terpene standards [(+)-3-carene, camphene, α-pinene, ß-myrcene, (+)-limonene, terpinolene, linalool, fenchol, α-terpineol, caryophyllene, α-bergamotene, humulene, farnesene mix, caryophyllene oxide] and n-alkane standard were purchased from Sigma-Aldrich (Prague, Czechia). Methanol, n-hexane, dimethyl sulfoxide (DMSO), and ethanol (EtOH) were purchased from VWR Chemicals (Prague, Czechia). Standards of cannabinoids, namely, cannabidivarin (CBDV), cannabidivarinic acid (CBDVA), cannabigerol (CBG), cannabigerolic acid (CBGA), cannabinol (CBN), cannabinolic acid (CBNA), cannabidiol (CBD), cannabidiolic acid (CBDA), cannabichromene (CBC), tetrahydrocannabivarin (THCV), Δ9-tetrahydrocannabinol (THC), and tetrahydrocannabinolic acid A (THCA-A) were obtained from Cayman Chemicals (Ann Arbor, United States). Extraction gas butane (BUT), and dimethylether (DME) were provided by RSonic (Berlin, DE) and Dexso GmbH (Pratteln, CH), respectively. Microbiological growth media Mueller-Hinton Broth (MHB), Sabourad dextrose agar (SDA) were bought from OXOID (Prague, Czechia), horse defibrinated blood was purchased from Thermo Fisher Scientific (Waltham, United States), antibiotics clotrimazole (CLT), chloramphenicol (CLP), ampicilin (AMP), terbinafine (TB), and RPMI 1640 medium from Sigma-Aldrich.
Plant material and extract preparation
The dried inflorescences from two cannabis genotypes – Forbidden Fruit (FF) (Czech Seed Bank, Czechia) and Chocolope (CHP) (DNA Genetics, NL) were used for the preparation of extracts. The plants were cultivated under controlled indoor conditions at the Department of Food Science, Faculty of Agrobiology Food and Natural Resources, Czech University of Life Sciences in Prague in 2020. Each genotype was cultivated on the table (2 m2) equipped with drip irrigation system. Plants were placed in pots containing Euro Pebbles (expanded clay) as growth medium. Air ventilation unit maintained the room temperature and humidity between 22 and 30°C, and 40 and 70%, respectively. The microclimatic conditions were adjusted according to the plant growth phase. Double-ended high-pressure sodium lamps were used to provide a suitable spectrum of light at a power of 1,000 W. The detailed information about plant nutrition were described previously by Janatová et al. (2018). Each cannabis genotype was extracted by three types of solvents – ethanol (EtOH), butane (BUT), and dimethyl ether (DME). To prepare first type of extract, 60 g of dried homogenized cannabis inflorescences were macerated for 48 h in 96% ethanol in the ratio 6:1 (solvent: flower; v/w). Subsequently, the extract was filtered, and the solvent evaporated using a Rotavapor® R-100 vacuum evaporator (Buchi, CHE) at 40°C. The Dexso extractor was used for the preparation of butane and dimethyl ether extracts. The extractor was filled with 30 g of plant material to which 500 mL bottle containing compressed BUT or DME was connected. The gas flow was allowed to enter, and the extract was collected at the bottom to the teflon paper. To remove the residues of the gases, the extracts were placed for 2 h under the vacuum at Glass Vac (BVV, United States).
Determination of the cannabinoid content in extracts by high-performance liquid chromatography with ultraviolet detection
Ten milligrams of each extract were dissolved in 1 mL of MeOH, filtered through 0.45 μm nylon filters (Agilent, United States), transferred to a HPLC vial, and submitted for analysis.
The apparatus consisted of UltiMate 3000 HPLC system (Thermo Fisher Scientific, United States) and was equipped with UV detector. Cannabinoids analysis was performed on an Excel SuperC18 column (250 × 4.6 mm, 3 μm, 90 A; ACE, Scotland). Compounds were separated using a gradient elution employing water + 0.075% FA (A) and ACN + 0.5% FA (B) as mobile phase under following gradient (A:B): 0′, 30:70; 1′, 30:70; 10′, 0:100; 12′, 0:100; 14′, 30:70. Temperature of the column compartment was set at 40°C. Injection was 10 μL and flow rate was 1 mL/min. Cannabinoids were detected at wavelengths between 190 and 400 nm. Quantification was done under 210 nm. The evaluation of the acquired data was performed using the Chromeleon 7.2 software (Thermo Fisher Scientific, United States). Standard calibration curves were prepared in a concentration range of 2–100 μg/mL with six concentration levels (100, 50, 20, 10, 5, and 2 μg/mL). UV peak areas of the standards (at each concentration) were plotted against the corresponding standard concentrations (in μg/mL) using weighted linear regression to generate a standard curve.
Determination of terpene profile of the extracts by gas chromatography coupled with flame ionization or mass detector
The extracts were dissolved in methanol at a concentration of 2 mg/mL, transferred to the vial, and submitted to the GC-FID or GC-MS analysis.
To identify the terpenic profile, the cannabis extracts were analyzed by GC 7200 coupled with 7890 B qTOF mass detector (Agilent). Separation of individual volatile components was performed on a HP-5MS column (30 ms, 0.25 mm i.d., 0.25 μm; Agilent). Helium was used as a carrier gas; the flow was set at 1 mL.min–1. The oven temperature program began at 60°C with a 3.5 min hold; the temperature was increased at a rate of 3.5°C/min to 155°C and then again at a 30°C min–1 rate to 300°C and held for 10 min (total run time: 45 min). The temperature of the quadrupole was maintained at 230°C and of the ion source at 230°C. The compounds were measured in a scan mode in a range of 55–700 Da. The retention indices of each analyte were calculated from the retention times of n-alkanes by linear interpolation as previously described by Kováts. Identification of the analyzed volatiles was carried out by comparing the spectra with the spectra of available standards and/or by comparing their spectra and retention indices with the NIST Mass Spectral Search Program, version 2.2. The relative quantification of terpenes was performed by 7890A GC coupled with FID detector (Agilent) under the same chromatographic conditions. The detector conditions were set up as follows: t = 300°C; flow of gases - air: 400 mL/min; H2: 30 mL/min; and N2 make up flow: 5 mL/min. All samples were analyzed in triplicate.
Determination of antimicrobial and antifungal activity of extracts
Tested microorganisms
The antibacterial activity of the extracts was tested against seven bacterial strains, namely, Staphylococcus aureus ATCC 25923 and 29213, S. epidermidis CCM 50, S. saprophyticus CCM 2727, S. lugdunensis CCM 4069, S. epidermidis CCM 4418, Streptococcus pyogenes CCM 4425. Their antifungal activity was determined against 12 dermatophytes, namely 3 strains of Nannizzia fulva (CCF 6025; 5338; 5782) two strains of Trichophyton rubrum (CCF 4934; 4879, Arthroderma insingulare (CCF 5417; 5943), Trichophyton tonsurans CCF 4930, Nannizzia gypsea CCF 5215, Epidermophyton floccosum CCM 8339, Microsporum canis CCM 8353, and Trichophyton interdigitale CCM 8377. The strains were obtained from the American Type Culture Collection (ATCC), Czech Collection of Microorganisms (CCM) or Culture Collection of Fungi, Department of Botany, Charles University, Prague (CCF).
Broth microdilution method
To determine antimicrobial and antifungal activity expressed as minimal inhibitory concentration (MIC), slightly modified broth microdilution methods CLSI (CLSI, 2008, 2009) were used for bacteria and fungi, respectively. Both experiments were carried out in 3 technical and 3 independent replicates.
Determination of minimal inhibitory concentration for bacteria
The crude extracts were dissolved in DMSO to prepare the stock solution (c = 51.2 mg/mL). Subsequently, the two-fold serial dilution of the extracts was prepared at concentrations ranging from 4 to 512 μg/mL was prepared to 96 microtiter plates containing MHB as growth medium. In case of S. pyogenes, the MHB was supplemented with 7% horse defibrinated blood. The microplates were inoculated with bacteria at a final density of 0.5 McF (i.e., 1.5 × 108 CFU/mL). The inocula were prepared from 1 day old bacterial cultures cultivated in MHB at 37°C. The degree of inhibition of bacterial growth was evaluated after 24 h cultivation at 37°C using the BioTek Synergy H1 microplate reader (Agilent) at 512 nm. The lowest concentration that inhibited bacterial growth by 80% compared to the growth control was considered the MIC. Chloramphenicol and ampicilin were used as positive controls.
Determination of minimal inhibitory concentration for dermatophytes
The stock solution of the extracts (c = 51.2 mg/mL) was by two-fold serial dilution dosed to 96 microtiter plates, resulting in concentrations ranging from 8 to 1,024 μg/mL. RPMI 1640 medium with pH 7 was used as growth medium. The microplates were inoculated with dermatophytes at density 3–7 × 105 CFU/mL. Dermatophyte inocula were prepared from 14-day old fungal cultures grown on SDA at 27°C. The degree of fungal growth inhibition was evaluated after 5 days cultivation at 27°C by microplate reader BioTek Synergy H1 at 495 nm. The lowest concentration that inhibited the growth of the dermatophyte by 80% compared to the control was considered MIC. Terbinafine and clotrimazole were used as positive controls.
Statistical evaluation of the data
The data were processed in Excel and STATISTICA 12 software (StatSoft, Tulsa, United States). As the data did not show normal distribution, the significant differences were evaluated by non-parametric Kruskal-Wallis test.
Results and discussion
Most of the extracts prepared from two cannabis strains by three different solvents were predominantly (>60%) composed of various cannabinoids, especially THCA. All of them demonstrated activity against 18 of the 19 microorganisms tested. In general, the bacteria were more susceptible to the extracts in comparison to dermatophytes. The MICs of the extracts ranged from 4 to 256 μg/mL. The ethanolic ones were, in general, less potent than the DME and BUT ones. This fact can be attributed to lower content of the active compounds in EtOH extracts.
Yield and chemical profile of cannabis extracts
The extraction yield by both gases was on average 4% higher compared to traditional maceration in ethanol (Table 1). The color of BUT and DME extracts was golden, while EtOH extracts were dark green. This indicates a higher content of chlorophyll content in EtOH extracts. High variability in cannabinoid content was observed if different solvents were used for the extraction of a single strain (Table 1). The most significant differences in the cannabinoid composition were determined in extracts prepared with ethanol. They contained less than half of the cannabinoids compared to the BUT and DME extracts (that is, CBGA ranged from 5.7 to 9.5 mg/g and 19 to 21 mg/g in EtOH and BUT/DME extracts, respectively (Table 1). The THC content in all extracts was similar. In general, the predominant cannabinoid in all extracts was THCA (190–617 mg/g), while the least present was THCV which was found only in Chocolope EtOH extract. The lower content of cannabinoids in EtOH extracts can probably be attributed to the higher polarity of the solvent and thus lower extraction ability of these compounds into the resulting extract (Politi et al., 2008; Romano and Hazekamp, 2013).
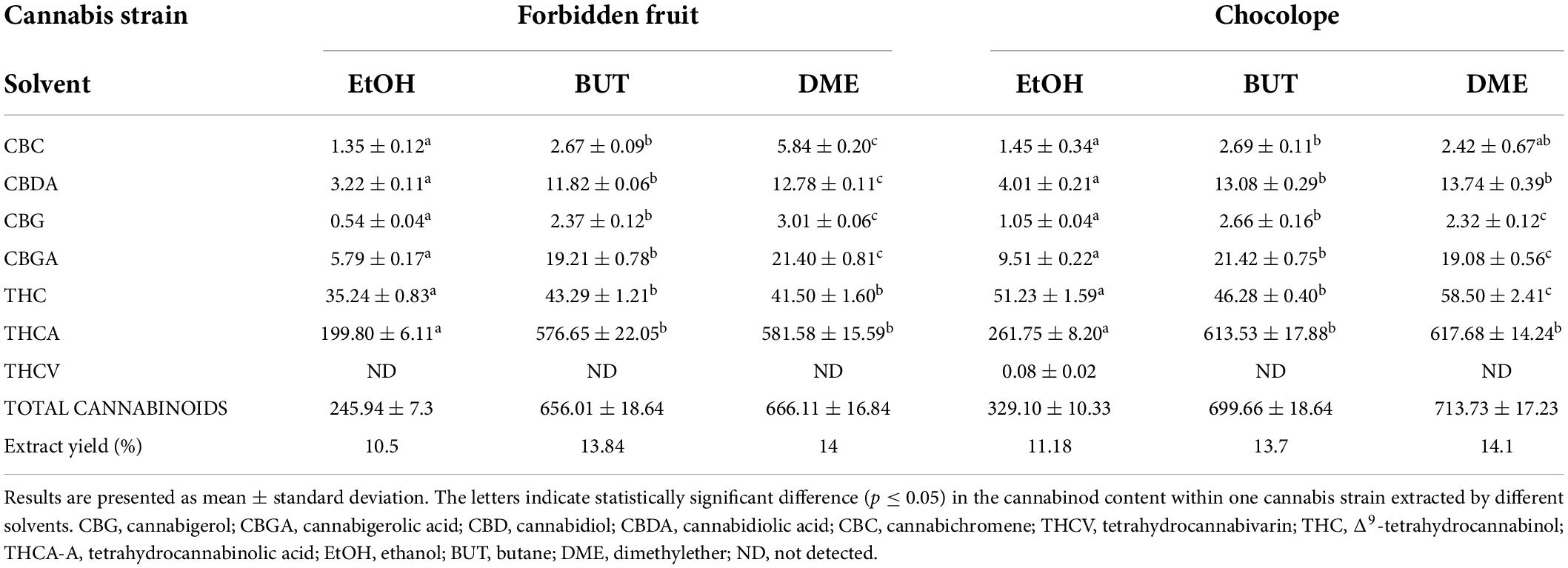
Table 1. Extract yield (%) and average cannabinoid content (mg/g) in extracts prepared by different solvents.
The higher variability in the terpene profile was observed between strains and between extracts of one strain prepared with different solvents (Table 2). The main terpenes (>7% each) in Forbidden Fruit extracts were ß-caryophyllene, ß-myrcene, selina-4(15),7(11)-diene, selina-3,7(11)-diene followed by humulene and ß-farnesene. The Chocolope strain extracts contained mostly ß-caryophyllene, ß-myrcene, (+)-limonene, linalool, and selina-3,7(11)-diene. The results indicate that both strains belong to caryophyllene-dominated cultivars (Lewis et al., 2018), which are also characterized by relatively high levels of humulene (Fischedick, 2017) and low levels of the often predominant α-pinene (Lewis et al., 2018).
The solvents had a statistically significant effect on the presence and relative ratio of individual terpenes in the extracts (Table 2). Both EtOH extracts compared to BUT and DME ones lacked most of the identified monoterpenes (i.e., thujene, α-pinene, ß-myrcene, and (+)-limonene). These terpenes were probably lost during the evaporation of the solvent under vacuum and increased temperature (Romano and Hazekamp, 2013). In conclusion, the gas extraction was more effective, BUT and DME extracts contained significantly higher amounts of active compounds. On top of that, the extraction was less time demanding (did not require the solvent evaporation step). Moreover, DME is a non-toxic gas commercially used for collagen extraction (EFSA, 2015). Therefore, it could be considered as a suitable alternative to ethanolic extraction.
Antifungal and antibacterial activity of extracts
All extracts showed very good to moderate activity against 18 of the 19 microorganisms tested. The MIC of the extracts ranged from 4 to 128 μg/mL and 32 to 256 μg/mL (Table 3) for bacteria and dermatophytes, respectively.
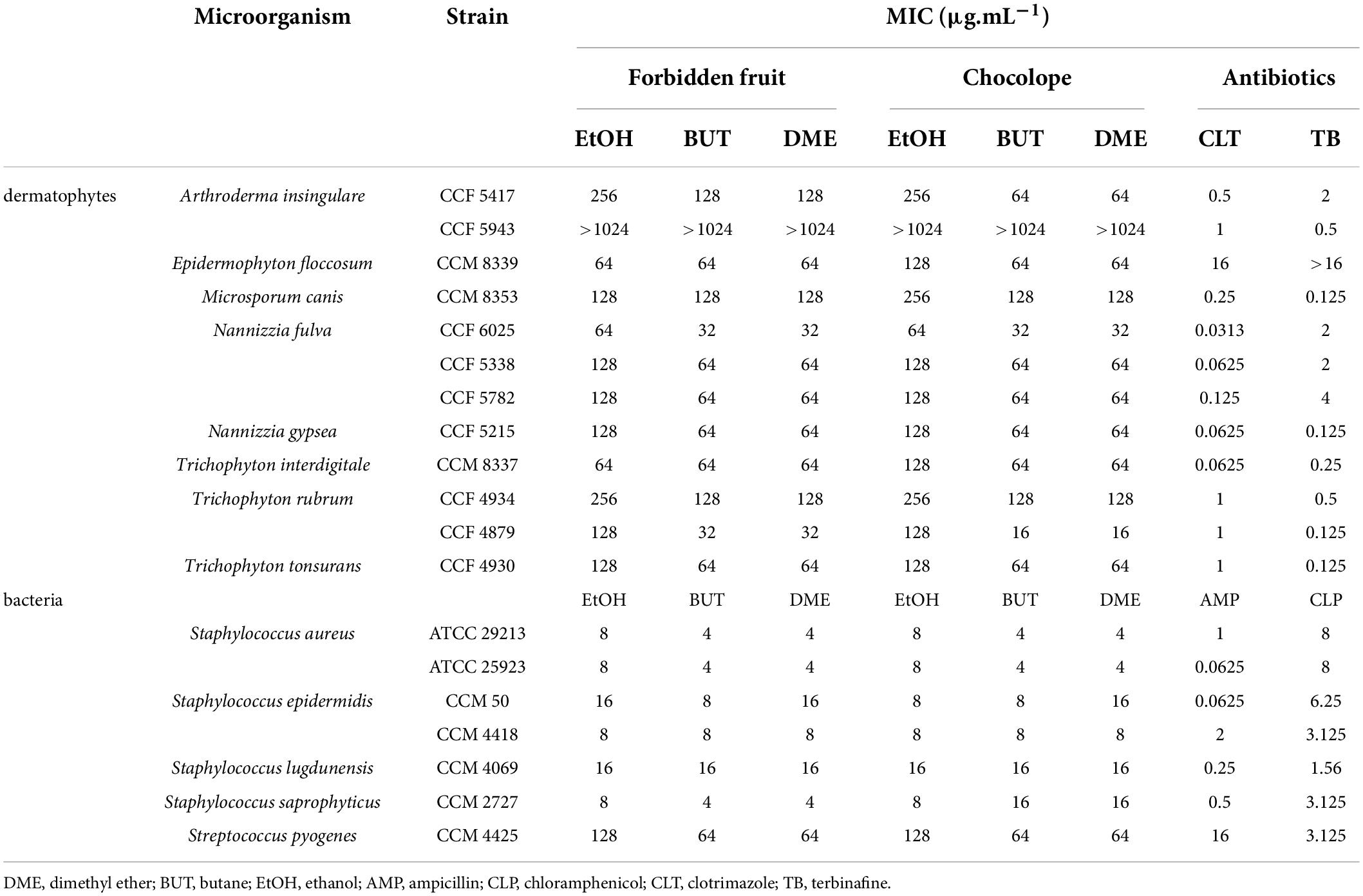
Table 3. Minimal inhibitory concentrations (MIC) (μg/mL) of cannabis extracts against selected bacteria and dermatophytes.
The most susceptible bacteria were S. aureus strains (MIC = 4–8 μg/mL). However, almost 10 times higher MIC values (MIC = 64–128 μg/mL) were determined for S. pyogenes. Such a significant decrease in the activity of cannabis extracts could be attributed to the presence of blood in the cultivation medium. As previously reported, in this type of media, cannabinoids become less effective due to their strong affinity to blood proteins (Garrett and Hunt, 1974; van Klingeren and Ten Ham, 1976). All extracts were also slightly less effective against S. aureus strains compared to isolated THC, CBD, CBG, and CBC (MIC = 0.5–2 μg/mL) (Appendino et al., 2008; Blaskovich et al., 2021). Generally, all the extracts except the EtOH ones were from more than 60% composed of cannabinoids (Table 1), therefore, they can be considered antimicrobial principles of cannabis. However, the role of the remaining biologically active compounds, especially terpenes, should not be omitted. The antimicrobial activity of the terpenes identified in extracts (Table 2) has been confirmed by many studies (Schofs et al., 2021). For example, ß-caryophyllene and α-pinene are very effective against S. aureus, S. epidermidis, S. saprophyticus and S. lugdunensis (Dahham et al., 2015; Ranarivelo et al., 2020). While myrcene has proven effects against S. epidermidis (Inoue et al., 2004) and limonene also has strong bactericidal effects against a diverse range of bacteria (Han et al., 2020, 2021).
The most sensitive dermatophytes were T. rubrum CCF 4879 (the most common species causing dermatophytosis in developed countries) and M. canis CCF 6025, while A. insingulare was resistant even to the highest concentrations of the extracts tested. The mode values of the MICs demonstrated that the EtOH extracts were slightly less effective (MIC = 128 μg/mL) compared to the DME and BUT extracts (MIC = 64 μg/mL). Even though some of the cannabis skin preparations are among other disorders also indicated for the treatment of bacterial and fungal skin diseases (Hashim et al., 2017; Anastassov et al., 2019), the scientific evidence about their activity against dermatophytes is very limited. So far, only Turner and Elsohly (1981) reported significant antifungal effects of CBC and its’ analogs against Trichophyton mentagrophytes (MIC = 6.25 – 50 μg/mL–1). Also hemp water extracts proved to have moderate activity against T. rubrum and interdigitale (MIC = 500 μg/mL) but not against N. gypsea (Orlando et al., 2020). There is no doubt that cannabinoids have a beneficial effect on various skin problems such as skin inflammation, fibrosis, itch, pain, and improved wound healing (Hashim et al., 2017; Cintosun et al., 2020). However, their contribution to the antifungal activity remains questionable and a possible mechanism of action is yet to be elucidated. On the other hand, all extracts contained terpenes with established activity against dermatophytes. For example, essential oils from cannabis with high concentrations of ß-caryophyllene or ß-caryophyllene alone were very effective against various dermatophytes (Iordache et al., 2016; Orlando et al., 2021). Tavares et al. (2010) reported a strong MIC (1–5 μg/mL) of myrcene against a variety of microorganisms, including dermatophytes. Moreover, limonene, α-pinene, γ-eudesmol, germacrene (separated or as a apart of essential oil) also proved significant to moderate antidermatophytic activity (MIC = 0.8–250 μg/mL) which was sometimes even better than some used antifungals, e.g., griseofulvin (Sanguinetti et al., 2007; Singh et al., 2010; Pinto et al., 2013; Alves et al., 2018; Danielli et al., 2018). It is very probable that both groups of biologically active substances, i.e., cannabinoids and terpenes, have an important role in the antifungal activity of cannabis extract.
The MIC values of cannabis extracts presented in this study are higher in comparison to the currently used antifungals. Furthermore, as mentioned above, some of the isolated compounds can be more active than the extracts. Therefore, the presented results may raise the question about the real therapeutic potential of cannabis extracts in the treatment of various skin infections. Current antimicrobial and antifungal agents were design solely to kill the target microorganism (Gnat et al., 2020). On the other hand, the effect of cannabis extract can be more complex. Not only it can inhibit or kill the microorganism growth, but both cannabinoids and terpenes due to their anti-inflammatory, antipruritics and antinociceptive properties can have positive effect on other symptoms accompanying the skin infections. Cannabinoids can also interact with the skin endocannabinoid system that plays an important role in its homeostasis (Martins et al., 2022). Terpenes are understood as skin penetration enhancers (Aqil et al., 2007), thus can facilitate the penetration of highly lipophilic cannabinoids to deeper skin layers or by other ways enhance their activity. This so called “entourage effect” was quite recently confirmed for some of the terpenes present in cannabis (LaVigne et al., 2021). From the empirical evidence provided by many users of cannabis topicals, it is known that the preparations are usually well accepted and reported to be effective (Martins et al., 2022). Although topicals are often homemade or from unofficial sources and contain unknown concentrations of various cannabis chemotypes (Mahmood et al., 2022). Therefore, their low toxicity during long-term use can be expected. These facts confirm that the therapeutic potential of cannabis in the treatment of skin infections should not be overlooked. Especially for the treatment of dermatomycosis that is usually long and can be associated with adverse effects. The side effects are unacceptable for elderly, pregnant women and children (Gnat et al., 2020).
To confirm the safety and efficacy of cannabis extracts that will lead to their use in medical practice, a lot of work has to be done. Among others identification of most active cannabis chemotypes, standardization of the extracts, determination of effective dose, pharmacokinetics, toxicological risk assessment and clinical studies are needed.
Conclusion
All tested extracts demonstrated significant activity against 18 of 19 tested bacterial and fungal skin pathogens. To the best of our knowledge, this is the first report on anti-dermatophyte activity of high potency cannabis strains extracted by different solvents. The extracts prepared traditionally by maceration in ethanol had lower amounts of active compounds, which led to their lower activity against the tested microorganisms. While the extraction by gas (DME, BUT) was more effective and less laborious. Since DME is successfully used in food industry, its pharmaceutical and commercial potential for plant extraction should be further considered and investigated.
It is already known that cannabinoids have a relatively wide spectrum of biological activities for which they are useful in the treatment of skin diseases. However, not much attention has been paid to their effectiveness against bacteria and fungi, which are often accompanied by skin problems. Our research brought new evidence that cannabis extracts may be of value to treat dermatophytosis and other skin diseases caused by various microorganisms and showed that cannabis could serve as an alternative or supportive treatment to commonly used antibiotics.
Data availability statement
The raw data supporting the conclusions of this article will be made available by the authors, without undue reservation.
Author contributions
TS and ZK: writing – original draft and investigation. JT: investigation, validation, and writing – review and editing. AJ: resources. PK: writing – review and editing. VH: resources and writing – review and editing. AF: conceptualization, data curation, methodology, writing – original draft, review and editing, and supervision. All authors contributed to the article and approved the submitted version.
Funding
This work was supported by SGS Project No. SV20-11-21310, METROFOOD-CZ Research Infrastructure Project (MEYS Grant No. LM2018100) and NutRisk Research Program (Project No. CZ.02.1.01/0.0/0.0/16_019/0000845).
Conflict of interest
The authors declare that the research was conducted in the absence of any commercial or financial relationships that could be construed as a potential conflict of interest.
Publisher’s note
All claims expressed in this article are solely those of the authors and do not necessarily represent those of their affiliated organizations, or those of the publisher, the editors and the reviewers. Any product that may be evaluated in this article, or claim that may be made by its manufacturer, is not guaranteed or endorsed by the publisher.
References
Appendino, G., Gibbons, S., Giana, A., Pagani, A., Grassi, G., Stavri, M., et al. (2008). Antibacterial cannabinoids from Cannabis sativa: A structure-activity study. J. Nat. Prod. 71, 1427–1430. doi: 10.1021/np8002673
Alves, F. A. R., Morais, S. M. de, Nogueira Sobrinho, A. C., Silva, I. N. G. da, Martins, C. G., Silva, A. A., et al. (2018). Chemical composition, antioxidant and antifungal activities of essential oils and extracts from Plectranthus s. against dermatophytes fungi. Revista Brasileira de Saúde e Produção Anim. 19, 105–115. doi: 10.1590/s1519-99402018000100010
Al-Zouabi, I., Stogner, J. M., Miller, B. L., and Lane, E. S. (2018). Butane hash oil and dabbing: Insights into use, amateur production techniques, and potential harm mitigation. Subst. Abuse Rehabil. 9, 91–101. doi: 10.2147/sar.s135252
Anastassov, G. E., Changoer, L., and Van Damme, P. A. (2019). Anti-microbial composition comprising cannabinoids. (U.S. Patent No. 10441552). Alexandria, VA: U.S. Patent and Trademark Office.
Anderson, L. L., Low, I. K., Banister, S. D., McGregor, I. S., and Arnold, J. C. (2019). Pharmacokinetics of Phytocannabinoid Acids and Anticonvulsant Effect of Cannabidiolic Acid in a Mouse Model of Dravet Syndrome. J. Nat. Prod. 82, 3047–3055. doi: 10.1021/acs.jnatprod.9b00600
Aqil, M., Ahad, A., Sultana, Y., and Ali, A. (2007). Status of terpenes as skin penetration enhancers. Drug Discov. Today 12, 1061–1067. doi: 10.1016/J.DRUDIS.2007.09.001
Becker, K., Heilmann, C., and Peters, G. (2014). Coagulase-negative staphylococci. Clin. Microbiol. Rev. 27, 870–926. doi: 10.1128/CMR.00109-13
Begum, J., Mir, N. A., Lingaraju, M. C., Buyamayum, B., and Dev, K. (2020). Recent advances in the diagnosis of dermatophytosis. J. Basic Microbiol. 60, 293–303. doi: 10.1002/jobm.201900675
Blaskovich, M. A. T., Kavanagh, A. M., Elliott, A. G., Zhang, B., Ramu, S., Amado, M., et al. (2021). The antimicrobial potential of cannabidiol. Commun. Biol. 4:7. doi: 10.1038/s42003-020-01530-y
Chan, G. C. K., Hall, W., Freeman, T. P., Ferris, J., Kelly, A. B., Winstock, A., et al. (2017). User characteristics and effect profile of Butane Hash Oil: An extremely high-potency cannabis concentrate. Drug Alcohol. Depend. 178, 32–38. doi: 10.1016/j.drugalcdep.2017.04.014
Cintosun, A., Lara-Corrales, I., and Pope, E. (2020). Mechanisms of Cannabinoids and Potential Alicability to Skin Diseases. Clin. Drug Investig. 40, 293–304. doi: 10.1007/s40261-020-00894-7
CLSI (2008). Reference Method for Broth Dilution Antifungal Susceptibility Testing of Filamentous Fungi; Aroved Standard—Second Edition. CLSI document M38-A2. Wayne: Clinical and Laboratory Standards Institute.
CLSI (2009). Methods for Dilution Antimicrobial Susceptibility Tests for Bacteria that Grow Aerobically; Aroved Standard-Eight Ed. CLSI Document M07-A8. Wayne: Clinical and Laboratory Standards Institute.
Dahham, S. S., Tabana, Y. M., Iqbal, M. A., Ahamed, M. B., Ezzat, M. O., Majid, A. S., et al. (2015). The anticancer, antioxidant and antimicrobial properties of the sesquiterpene β-caryophyllene from the essential oil of Aquilaria crassna. Molecules 20, 11808–11829. doi: 10.3390/molecules200711808
Danielli, L. J., Pippi, B., Duarte, J. A., Maciel, A. J., Lopes, W., Machado, M. M., et al. (2018). Antifungal mechanism of action of Schinus lentiscifolius Marchand essential oil and its synergistic effect in vitro with terbinafine and ciclopirox against dermatophytes. J. Pharm. Pharmacol. 70, 1216–1227. doi: 10.1111/jphp.12949
Daniulaityte, R., Lamy, F. R., Barratt, M., Nahhas, R. W., Martins, S. S., Boyer, E. W., et al. (2017). Characterizing marijuana concentrate users: A web-based survey. Drug Alcohol. Depend. 178, 399–407. doi: 10.1016/j.drugalcdep.2017.05.034
Dhadwal, G., and Kirchhof, M. G. (2018). The risks and benefits of cannabis in the dermatology. J. Cutan. Med. Surg. 22, 194–199. doi: 10.1177/1203475417738971
EFSA (2009). Scientific Opinion of the Panel on Food Contact Materials, Enzymes, Flavourings and Processing aids (CEF) on dimethyl ether as an extraction solvent. EFSA J. 984, 1–13.
EFSA (2015). Scientific Opinion on the safety of use of dimethyl ether as an extraction solvent under the intended conditions of use and the proposed maximum residual limits. EFSA J. 13:4174
Farha, M. A., MacNair, C. R., Carfrae, L. A., Zahed, S. S. El, Ellis, M. J., Tran, H. R., et al. (2020). Uncovering the Hidden Antibiotic Potential of Cannabis. ACS Infect. Dis. 6, 338–346. doi: 10.1021/acsinfecdis.9b00419
Fischedick, J. T. (2017). Identification of Terpenoid Chemotypes Among High (À)-trans-D 9-Tetrahydrocannabinol-Producing Cannabis sativa L Cultivars. Cannabis Cannabinoid Res. 2, 34–47. doi: 10.1089/can.2016.0040
Gallo-Molina, A. C., Castro-vargas, H. I., Garzón-méndez, W. F., Ramírez, J. A., Rivera Monroy, Z. J., King, J. W., et al. (2019). Extraction, isolation and purification of tetrahydrocannabinol from the Cannabis sativa L. plant using supercritical fluid extraction and solid phase extraction. J. Supercrit. Fluids 146, 208–216. doi: 10.1016/j.supflu.2019.01.020
Garrett, E. R., and Hunt, C. A. (1974). Physicochemical Properties, Solubility, and Protein Binding of Δ9 -Tetrahydrocannabinol. J. Pharm. Sci. 63, 1056–1064. doi: 10.1002/jps.2600630705
Gnat, S., Łagowski, D., and Nowakiewicz, A. (2020). Major challenges and perspectives in the diagnostics and treatment of dermatophyte infections. J. Alied Microbiol. 129, 212–232. doi: 10.1111/JAM.14611
Han, Y., Chen, W., and Sun, Z. (2021). Antimicrobial activity and mechanism of limonene against Staphylococcus aureus. J. Food Saf. 41:e12918. doi: 10.1111/jfs.12918
Han, Y., Sun, Z., and Chen, W. (2020). Antimicrobial susceptibility and antibacterial mechanism of limonene against listeria monocytogenes. Molecules 25:33. doi: 10.3390/molecules25010033
Hashim, P. W., Cohen, J. L., Pompei, D. T., and Goldenberg, G. (2017). Topical cannabinoids in dermatology. Cutis 100, 50–52.
Havlickova, B., Czaika, V. A., and Friedrich, M. (2008). Epidemiological trends in skin mycoses worldwide. Mycoses 51, 2–15. doi: 10.1111/j.1439-0507.2008.01606.x
Hay, R. J., Johns, N. E., Williams, H. C., Bolliger, I. W., Dellavalle, R. P., Margolis, D. J., et al. (2014). The global burden of skin disease in 2010: An analysis of the prevalence and impact of skin conditions. J. Invest. Dermatol. 134, 1527–1534. doi: 10.1038/jid.2013.446
Inoue, Y., Shiraishi, A., Hada, T., and Hamashima, H. (2004). The Antibacterial Effects of Myrcene on Staphylococcus aureus and Its Role in the Essential Oil of the Tea Tree (Melaleuca alternifolia). Nat. Med. 58, 10–14.
Iordache, O., Cozea, A., Vǎrzaru, E., Stoica, E., Platon, C., Rodino, S., et al. (2016). Antimicrobial activity of textiles treated with rosemary and orange essential oils against a selection of pathogenic fungi. Sci. Bull. 20, 362–369.
Janatová, A., Fraňková, A., Tlustoš, P., Božik, M., and Klouček, P. (2018). Yield and cannabinoids contents in different cannabis (Cannabis sativa L.) genotypes for medical use. Ind. Crops Prod. 112, 363–367. doi: 10.1016/j.indcrop.2017.12.006
Johansson, L., Thulin, P., Low, D. E., and Norrby-Teglund, A. (2010). Getting under the skin: The immunopathogenesis of Streptococcus pyogenes deep tissue infections. Clin. Infect. Dis. 51, 58–65. doi: 10.1086/653116
LaVigne, J. E., Hecksel, R., Keresztes, A., and Streicher, A. (2021). Cannabis sativa terpenes are cannabimimetic and selectively enhance cannabinoid activity. Sci. Rep. 11:8232. doi: 10.1038/s41598-021-87740-8
Lewis, M. A., Russo, E. B., and Smith, K. M. (2018). Pharmacological Foundations of Cannabis Chemovars. Planta Med. 84, 225–233. doi: 10.1055/s-0043-122240
Lim, M., and Kirchhof, M. G. (2019). Dermatology-Related Uses of Medical Cannabis Promoted by Dispensaries in Canada, Europe, and the United States. J. Cutan. Med. Surg. 23, 178–184. doi: 10.1177/1203475418808761
Mahmood, F., Lim, M. M., and Kirchhof, M. G. (2022). A Survey of Topical Cannabis Use in Canada. J. Cutan. Med. Surg. 26, 156–161. doi: 10.1177/12034754211059025
Martins, A. M., Gomes, A. L., Boas, I. V., Marto, J., and Ribeiro, H. M. (2022). Cannabis-based products for the treatment of skin inflammatory diseases: A timely review. Pharmaceuticals 15:210. doi: 10.3390/ph15020210
Orlando, G., Adorisio, S., Delfino, D., Chiavaroli, A., Brunetti, L., Recinella, L., et al. (2021). Comparative investigation of composition, antifungal, and anti-inflammatory effects of the essential oil from three industrial hemp varieties from Italian cultivation. Antibiotics 10:334. doi: 10.3390/antibiotics10030334
Orlando, G., Recinella, L., Chiavaroli, A., Brunetti, L., Leone, S., Carradori, S., et al. (2020). Water Extract from Inflorescences of Industrial Hemp Futura 75 Variety as a Source of Anti-Inflammatory, Anti-Proliferative and Antimycotic Agents: Results from In Silico, In Vitro and Ex Vivo Studies. Antioxidants 9:437. doi: 10.3390/antiox9050437
Palmieri, B., Laurino, C., and Vadala, M. (2019). A therapeutic effect of cbd-enriched ointment in inflammatory skin diseases and cutaneous scars. Clin. Ter. 170, e93–e99. doi: 10.7417/CT.2019.2116
Panda, S., and Verma, S. (2017). The menace of dermatophytosis in India: The evidence that we need. Indian J. Dermatol. Venereol. Leprol. 83, 281–284. doi: 10.4103/ijdvl.IJDVL_224_17
Pasquali, F., Schinzari, M., Lucchi, A., Mandrioli, M., Toschi, T. G., De Cesare, A., et al. (2020). ‘Preliminary data on the antimicrobial effect of Cannabis sativa L. variety Futura 75 against food-borne pathogens in vitro as well as against naturally occurring microbial populations on minced meat during storage. Ital. J. Food Saf. 9, 80–87. doi: 10.4081/ijfs.2020.8581
Pepeljnjak, S., Kosalec, I., Kalodera, Z., and Blazević, N. (2005). ‘Antimicrobial activity of juniper berry essential oil (Juniperus communis L Cupressaceae). Acta Pharm. 55, 417–422.
Peschel, W. (2016). Quality control of traditional Cannabis tinctures: Pattern, markers, and stability. Sci. Pharm. 84, 567–584. doi: 10.3390/scipharm84030567
Pinto, E., Hrimpeng, K., Lopes, G., Vaz, S., Gonçalves, M. J., Cavaleiro, C., et al. (2013). Antifungal activity of Ferulago capillaris essential oil against Candida, Cryptococcus, Aspergillus and dermatophyte species. Eur. J. Clin. Microbiol. Infect. Dis. 32, 1311–1320. doi: 10.1007/s10096-013-1881-1
Politi, M., Peschel, W., Wilson, N., Prieto, J. M., and Heinrich, M. (2008). Direct NMR analysis of cannabis water extracts and tinctures and semi-quantitative data on Δ9-THC and Δ9-THC-acid. Phytochemistry 69, 562–570. doi: 10.1016/j.phytochem.2007.07.018
Rajagopalan, M., Inamadar, A., Mittal, A., Miskeen, A. K., Srinivas, C. R., Sardana, K., et al. (2018). Expert consensus on the management of dermatophytosis in India (ECTODERM India). BMC Dermatol. 18:6. doi: 10.1186/s12895-018-0073-1
Ramaraj, V., Vijayaraman, R. S., Rangarajan, S., and Kindo, A. J. (2016). Incidence and prevalence of dermatophytosis in and around Chennai, Tamilnadu, India. Int. J. Res. Med. Sci. 4, 695–700. doi: 10.18203/2320-6012.ijrms20160483
Ranarivelo, L. R., Randriamialinoro, F., Rakotonandrasana, S., Ratsimbason, M., Vérité, P., Lecso-Bornet, M., et al. (2020). Chemical Composition and Antimicrobial Activity of Leaf Essential Oil of Tetradenia nervosa Codd from Madagascar, Collected at Different Stages of Vegetative Growth and Age’, in ACS Symposium Series. J. Am. Chem. Soc. 1361, 285–296. doi: 10.1021/bk-2020-1361.ch015
Romano, L. L., and Hazekamp, A. (2013). Cannabis oil: Chemical evaluation of an upcoming cannabis- based medicine. Cannabinoids 1, 1–11.
Russo, E. B. (2011). Taming THC: Potential cannabis synergy and phytocannabinoid-terpenoid entourage effects. Br. J. Pharmacol. 163, 1344–1364. doi: 10.1111/j.1476-5381.2011.01238.x
Sanguinetti, M., Posteraro, B., Romano, L., Battaglia, F., Lopizzo, T., De Carolis, E., et al. (2007). In vitro activity of Citrus bergamia (bergamot) oil against clinical isolates of dermatophytes. J. Antimicrob. Chemother. 59, 305–308. doi: 10.1093/jac/dkl473
Schofs, L., Sparo, M. D., and Sánchez Bruni, S. F. (2021). The antimicrobial effect behind Cannabis sativa. Pharmacol. Res. Perspect. 9:e00761. doi: 10.1002/prp2.761
Schommer, N. N., and Gallo, R. L. (2013). Structure and function of the human skin microbiome. Trends Microbiol. 21, 660–668. doi: 10.1016/j.tim.2013.10.001
Singh, A., Masih, A., Khurana, A., Singh, P. K., Gupta, M., Hagen, F., et al. (2018). High terbinafine resistance in Trichophyton interdigitale isolates in Delhi, India harbouring mutations in the squalene epoxidase gene. Mycoses 61, 477–484. doi: 10.1111/myc.12772
Singh, P., Shukla, R., Prakash, B., Kumar, A., Singh, S., Mishra, P. K., et al. (2010). ‘Chemical profile, antifungal, antiaflatoxigenic and antioxidant activity of Citrus maxima Burm. and Citrus sinensis (L.) Osbeck essential oils and their cyclic monoterpene DL-limonene. Food Chem. Toxicol. 48, 1734–1740. doi: 10.1016/j.fct.2010.04.001
Tavares, A. C., Gonçalves, M. J., Cruz, M. T., Cavaleiro, C., Lopes, M. C., Canhoto, J., et al. (2010). Essential oils from Distichoselinum tenuifolium: Chemical composition, cytotoxicity, antifungal and anti-inflammatory properties. J. Ethnopharmacol. 130, 593–598. doi: 10.1016/j.jep.2010.05.054
Turner, C. E., and Elsohly, M. A. (1981). Biological activity of cannabichromene, its homologs and isomers. J. Clin. Pharmacol. 21, 283S–291S. doi: 10.1002/j.1552-4604.1981.tb02606.x
van Klingeren, B., and Ten Ham, M. (1976). Antibacterial activity of Δ9-tetrahydrocannabinol and cannabidiol. Antonie van Leeuwenhoek 42, 9–12. doi: 10.1007/BF00399444
Wang, Y. H., Avula, B., ElSohly, M. A., Radwan, M. M., Wang, M., Wanas, A. S., et al. (2018). Quantitative Determination of Δ 9 -THC, CBG, CBD, Their Acid Precursors and Five Other Neutral Cannabinoids by UHPLC-UV-MS. Planta Med. 84, 260–266. doi: 10.1055/s-0043-124873
WHO (2019). Monitoring and Evaluation of the Global Action Plan on Antimicrobial Resistance Framework and Recommended Indicators. Geneva: World Health Organization
Zajicek, J. P., Hobart, J. C., Slade, A., Barnes, D., and Mattison, P. G., Musec Research Group, et al. (2012). MUltiple sclerosis and extract of cannabis: Results of the MUSEC trial. J. Neurol. Neurosurg. Psychiatry 83, 1125–1132. doi: 10.1136/jnnp-2012-302468
Keywords: extraction method, Cannabis sativa, antimicrobial activity, dermatophytes, dimethyl ether, skin infection
Citation: Skala T, Kahánková Z, Tauchen J, Janatová A, Klouˇcek P, Hubka V and Fraˇnková A (2022) Medical cannabis dimethyl ether, ethanol and butane extracts inhibit the in vitro growth of bacteria and dermatophytes causing common skin diseases. Front. Microbiol. 13:953092. doi: 10.3389/fmicb.2022.953092
Received: 25 May 2022; Accepted: 11 July 2022;
Published: 20 September 2022.
Edited by:
Floriana D’Angeli, Università Telematica San Raffaele, ItalyReviewed by:
Atte Von Wright, University of Eastern Finland, FinlandSueli Fumie Yamada-Ogatta, State University of Londrina, Brazil
Copyright © 2022 Skala, Kahánková, Tauchen, Janatová, Klouˇ cek, Hubka and Fra ˇ nková. This is an open-access article distributed under the terms of the Creative Commons Attribution License (CC BY). The use, distribution or reproduction in other forums is permitted, provided the original author(s) and the copyright owner(s) are credited and that the original publication in this journal is cited, in accordance with accepted academic practice. No use, distribution or reproduction is permitted which does not comply with these terms.
*Correspondence: Adéla Fraˇnková, frankovaa@af.czu.cz