- 1College of Landscape Architecture and Tourism, Hebei Agricultural University, Baoding, China
- 2Hebei Key Laboratory for Tree Genetic Resources and Forest Protection, Baoding, China
Fertilization is an effective agronomic strategy to improve the efficiency of phytoextraction by Salix integra Thunb. However, the specific effects of the simultaneous application of nitrogen (N) and sulfur (S) fertilizers in the rhizosphere remain unclear. We investigated the bioavailability of lead (Pb) and Cadmium (Cd) along with the microbial metabolic functions and community structure in the rhizosphere soil of S. integra after the application of N (0, 100, and 200 kg·ha−1·year−1) and S (0, 100, and 200 kg·ha−1·year−1) fertilizers for 180 days. The simultaneous application of N and S fertilizers significantly enhanced the absorption of Pb and Cd by S. integra, whereas this effect was not observed for the single application of N or S fertilizer. The contents of acid-soluble Pb and Cd in the rhizosphere soil significantly increased after either single or combined fertilize applications. The microbial metabolic activity was enhanced by the N and S fertilizers, whereas the microbial diversity markedly decreased. The metabolic patterns were mainly affected by the concentration of N fertilizer. The dominant fungi and bacteria were similar under each treatment, although the relative abundances of the dominant and special species differed. Compared to the N200S100 and N200S200 treatments, the N100S100 and N100S200 treatments resulted in fewer pathogenic fungi and more rhizosphere growth-promoting bacteria, which promoted phytoremediation by S. integra. Redundancy analysis indicated that the pH and nitrate content were the key factors affecting the structure of the microbial community. Collectively, the results suggest interactive effects between N and S fertilizers on the rhizosphere soil, providing a potential strategy for plant-microbial remediation by S. integra.
Introduction
Numerous environmental problems have emerged with the acceleration of industrialization and urbanization, the most serious of which is heavy metal pollution (Manta et al., 2002). Lead (Pb) and cadmium (Cd) are the most significant heavy metal pollutants in China (Hu et al., 2014). Many physical, chemical, and biological processes have been developed to reduce the threats posed by Pb and Cd pollution in ecosystems and humans; however, most of these approaches are costly and cause secondary pollution (Ma et al., 2016). Due to these problems, phytoremediation, a low-cost approach that does not generate secondary contamination, has emerged as a research hotspot in recent years. Plants can absorb, transfer, and stabilize various heavy metals, making them ideal candidates for the bioremediation of heavy metals in contaminated soils (Gong et al., 2018). However, the low remediation efficiency of plants has restricted their large-scale application, because of low biomass and bioavailability of heavy metals (Zhang et al., 2019; Wan et al., 2020).
The bioavailability of heavy metals is a key factor affecting phytoremediation. Compared with other fertilizers (phosphate and potash), nitrogen (N) and sulfur (S) have greater impacts on the availability of heavy metals (Hou et al., 2017). Both N and S are essential nutrients that promote plant growth (Wang et al., 2016), and these nutrients can also alter the bioavailability of heavy metals to affect the absorption of heavy metals by plants (Wang et al., 2008). The acid-soluble is the main form of heavy metals that is absorbed by plants (Moharami and Jalali, 2015). Previous studies have shown that N fertilizer can increase the accumulation of Cd in wheat (Triticum aestivum) and rice (Oryza sativa; Wang et al., 2019, 2020). Maqbool et al. (2020) reported that different forms of N fertilizers could improve the absorption efficiency of Cd by Solanum nigrum. The application of moderate N fertilizer improved the phytoremediation efficiency of Sedum alfredii in Zn/Cd-contaminated soils (Lin et al., 2020). The absorption of Cd by Brassica juncea and Nicotiana tabacum was significantly promoted by the application of S fertilizer (9.98 g·kg−1 and 0.51 g·kg−1, respectively; Dede and Ozdemir, 2016; Li et al., 2019). Thus, different types and concentrations of fertilizers have different effects on phytoremediation. However, till now, studies on the effects of N and S fertilizers on phytoremediation have focused primarily on crops and hyperaccumulators, and the interactive effects of N and S fertilizers on phytoremediation by fast-growing trees remain unclear. In addition, certain parameters such as pH and the type and content of metal available in the rhizosphere environment are considered as the most important factor in phytoextraction. In the long term, N changes the form of Cd in soil, therby affecting heavy metal absorption (Wang et al., 2020). Iqbal et al. (2012) reported that the application of S fertilizer improved the extraction efficiency of Cd by Salix, thereby promoting the S oxidation process of microorganisms and increasing the bioavailability of Cd in the rhizosphere soil. Rhizobacteria are directly beneficial to root patterns and provide nutrients to plants (Buée et al., 2009).
Some plant growth-promoting bacteria such as Bacillus, Ensifer secrete IAA and can stimulate plant growth and heavy metal accumulation (Mesa et al., 2017; Sajid et al., 2020). Geopora, an arbuscular mycorrhizal fungi, forms symbionts with plants to improve resistance to heavy metals (Sessitsch et al., 2013; Konkolewska et al., 2020). Soil microbiota are closely associated with soil quality and fertility (Khan et al., 2016; Mitra et al., 2018; Pramanik et al., 2018). The effects of fertilization on soil microbial communities have been widely reported, and fertilizers are known to influence the size, composition, function, and activity of the soil microbial community. The forms and concentration of N also have significant effects on the composition and diversity of the soil bacterial community (Liu et al., 2018a; Zheng et al., 2019). Fierer et al. (2012) reported that the N content has a significant effect on the composition of the bacterial community but little effect on the diversity. Fertilization with S reduced soil heterogeneity by changing the soil physical and chemical properties, thereby driving variation in the bacterial community structure (Philippot et al., 2013; Masuda et al., 2016). In general, the concentrations and forms of N and S have important effects on the composition and diversity of the microbial community (Liu et al., 2018a; Wang et al., 2018a; Zheng et al., 2019). However, few studies have addressed the simultaneous application of N and S fertilizers on soil microbial communities involved in phytoextraction. These microbes might be crucial for phytoextraction in the soil (Iqbal et al., 2012; Wang et al., 2020).
Salix integra Thunb. grows quickly and produces a large quantify of biomass and a deep root system. Previous studies have reported that S. integra had stronger absorption and tolerance to various heavy metals, for example Pb, Cu, Cd, and Zn (Yang et al., 2014, 2018; Shi et al., 2017; Cao et al., 2020). Thus, S. integra can be used to absorb metals. Most previous studies have focused on exposure to a single fertilizer; however, the phytoextraction of Pb and Cd by S. integra was enhanced by the simultaneous application of N and S fertilizers but not by the single application of N or S fertilizer. In addition, our previous research showed that the phytoremediation efficiency of S. integra could be affected by the rhizosphere microbes and inoculation brain (Niu et al., 2020, 2021). However, the mechanism by which simultaneous N and S fertilization affects the soil physicochemical parameters and soil microbial activity and diversity remains unclear. We hypothesized that (1) N and S fertilizers have interactive effects on soil properties, and (2) the composition and function of the rhizosphere soil microbial community are affected by N and S fertilization. A better understanding of the response of soil microbial communities to N and S in the contaminated soil is also expected to provide a theoretical basis for the phytoremediation of contaminated soil using fast-growing trees.
Materials and methods
Study site and plant material
Experiments were carried out at the Experimental Station of Hebei Agricultural University, Baoding City, Hebei Province (E 115°42′, N 38°81′), China. Annual rainfall at this site is approximately 532 mm, and the annual mean temperature is approximately 13.4°C. The soil is a typical meadow cinnamon soil. Contaminated soils with different Pb and Cd concentrations were added artificially in 2017. The soil properties and metal concentrations were as follows: pH = 8.42, soil organic matter = 18.13 g·kg−1, total nitrogen = 319.9 mg·kg−1, total phosphorus = 205.9 mg·kg−1, total potassium = 6.37 mg·kg−1, Pb concentration = 296.4 mg·kg−1, and Cd concentration = 23.7 mg·kg−1. 1-year-old S. integra cuttings with similar growth and vigor were used in this study.
Experimental setup
Experiments were carried out using pots (height = 30 cm, diameter = 30 cm) in April 2019. Every pot was filled with 20 kg of air-dried loam soil. The soil water content was maintained at 60% of its water holding capacity during the experiment. Three N and S concentrations were established according to the fertilization concentration of 1-year-old seedlings (Cheng, 2012). N was added as CO(NH2)2, and S was added as Na2SO4. The contents of applied N and S fertilizers (in terms of N and S contents) are shown in Supplementary Table S1. The experiments included nine treatments with 12 replicates per treatment.
Fertilizer was added every 15 days from May 15 to July 15. For the N100 and N200 fertilization levels, 0.3031 and 0.6062 g CO(NH2)2, corresponding to 0.1413 and 0.2826 g N, respectively, were applied each time. For the S100 and S200 fertilization levels, 0.6270 and 1.2540 g Na2SO4, corresponding to 0.1413 and 0.2826 g S, respectively, were applied. The fertilizers were dissolved in water and then sprayed on the soil surface of each pot. All pots were placed randomly and subjected to the same treatment conditions (watering, soil loosening, and weeding) during the growing season.
Plant and soil sampling
After growth for 180 days, the rhizosphere and bulk soils of the plants (three replicates each) were collected for each treatment in October 2019. The roots, stems, and leaves were harvested separately. The roots were soaked in 25 mmol·L−1 Na2-EDTA solution for 15 min to remove heavy metal ions adsorbed on the surface and then gently washed with distilled water. To sample the rhizosphere soil, the plants were removed from the pots and shaken to remove large soil aggregates. The rhizosphere soil was obtained using a brush that attached firmly to the root surface. Five randomly selected roots were pooled into a single sample, and approximately 3 g of soil for molecular analyses was stored at −80°C until DNA extraction. Residual soils for measuring the soil chemical properties and the contents of Pb and Cd were air-dried. All tools were sterilized between the sampling of different rhizosphere soils.
Soil chemical properties
The soil was air-dried and passed through a 60-mesh sieve. After digestion with concentrated sulfuric acid, the organic matter content (SOM) was determined by the potassium dichromate heating method. Available phosphorus (AP) was determined by the molybdenum antimony colorimetric method after extraction with sodium bicarbonate. Available potassium (AK) was determined with an atomic spectrophotometer after extraction with acetic acid. After dissolving the soil in deionized water, the pH (1:2.5 m/V) was measured using an electronic pH meter, nitrate N was determined using phenol disulfonic acid colorimetry, ammonium N was determined using KCl leaching-indophenol blue colorimetric method, available sulfur (AS) was determined using the phosphate extraction-barium sulfate turbidimetric method, and cation exchange capacity (CEC) was determined using the chlorine barium-sulfuric acid exchange method (Lu, 2000).
Determination of heavy metals in plant and soil samples
Dried and homogenized S. integra tissue powders were thoroughly digested with HNO3 and HClO4 (10:1, v/v). The samples were then analyzed using an atomic absorption spectrometer (Shimadzu AA-680, Kyoto, Japan).
The soil (0.5 g) was digested in 10 ml of a mixture of HNO3 and HClO4 (10:1, v/v) via microwave digestion (Sined MDS-6, Shanghai, China) at 180°C until the solution become transparent (approximately 3.5 h). The solution was then filtered through a 0.45-μm membrane and diluted to 50 ml. Soil samples were analyzed by atomic absorption spectrophotometry.
Soil Pb and Cd fractions were collected by BCR sequential extraction using a soil sample (0.5 g) containing the acid-soluble, reducible, oxidizable, and residual fractions (Rauret et al., 1999). The acid-soluble fraction was extracted with 0.11 mol·L−1 acetic acid for 16 h. The reducible fraction was extracted with 0.5 mol·L−1 hydroxylamine hydrochloride for 16 h (pH = 1.5). The oxidizable fraction was extracted using 8.8 mol·L−1 H2O2 at 85°C for 2 h. The residual fraction was obtained by extraction with 1.0 mol·L−1 ammonium acetate and digested by adding three acids (2 mlHNO3, 4 ml HCl, and 2 mL HF). The different fractions were evaluated by atomic absorption spectrophotometry.
Community-level physiological profiling
Community-level physiological profiling (CLPP) was used to assess the metabolic function and functional diversity of the microbial community (Ma et al., 2018; Zhao et al., 2018). The carbon source utilization pattern (CSUP) of the soil microbial community was assessed using a Biolog ECO microplate (Category No. 1506, United States). Detailed information about the experimental procedure can be found in Niu et al. (2019). For further analysis of the CLPP data, the absorbance reading at 240 h was identified as the time point for all samples. The data were analyzed to determine metabolic diversity indices according to Sofo et al. (2018).
Microbial community analysis
The structures of the rhizosphere bacterial and fungal communities were analyzed for five treatments: the control (N0S0), two treatments that enhanced the absorption of heavy metals by plants (N100S100 and N100S200), and two treatments that suppressed the absorption of heavy metals by plants (N200S100, and N200S200). Three replicates for each of the five treatments were analyzed, resulting in a total of 15 samples analyzed. Extracted total DNA was prepared for the analysis of microbial community structure via high-throughput sequencing. Microbial DNA was extracted from each fresh soil sample using a Fast Soil DNA kit (Omega Biotek, Inc.) following the manufacturer’s protocol. DNA samples were quantified using a nano spectrophotometer (Thermo Scientific, Wilmington, DE, United States) and 1% (w/v) agarose gel to verify the concentration and purity. The V3–V4 hypervariable region of the bacterial 16S rRNA gene was selected for PCR amplification with the primers 341F (5-CCTAYGGGRBGCASCAG-3′) and 806R (5′-GGAC TACNNG GGTAT-CTAAT-3′) for bacterial identification. The internal transcribed spacer (ITS) hypervariable regions of the fungal genes were amplified with primers ITS5-1737F (GGAAGTAAAAGTCGTAACAAGG) and ITS2-2043R (GCTGCGTTCTTCA TCGTGC) for fungal identification. Details of the PCR program are described in Xu et al. (2018). Each sample was amplified three times. The amplified products were mixed in equal amounts and then sequenced using the Novaseq 6,000 PE 250 platform (Illumina, United States; Allwegene Biotech Co., Ltd., Tianjin, China). Raw sequences of 16S rRNA and ITS gene have been submitted to NCBI Sequence Read Archive (Nos. PRJNA846123 and PRJNA846147, respectively).
The raw fastq files were demultiplexed and quality filtered using QIIME version 1.9.1 according to Caporaso et al. (2011). In subsequent analyses, reads were truncated at any site with an average quality score < 20 over a 50-bp sliding window and without ambiguous bases. Sequences with overlap longer than 10 bp were merged by overlapping sequences. UPARSE software (version 7.1) was used to assemble the operational taxonomic units (OTUs) at 3% evolutionary sites, and UCHIME software was used to identify and remove chimeric sequences. The taxonomic identity of each 16S rRNA and each ITS gene sequence was determined using the Ribosomal Database Project classifier with 70% confidence intervals for the Silva (Release 128) 16S rRNA and Unite (Release 7.0) databases, respectively. The OTUs were then analyzed. Alpha diversity was calculated using Mothur (version 1.30.1), while the beta diversity metrics were calculated using QIIME and the R base package (Oksanen et al., 2013). We also estimated alpha diversity using the Chao1 and Shannon indexes. The co-occurrence networks were constructed in this study. Networks were constructed based on Spearman correlation (r > 0.7 and p < 0.05) using the R package igraph (1.2.5). The division of network modules was based on the greedy optimization of the modularity algorithm. All networks were visualized using Gephi software (0.9.2).
Statistical analysis
Each data point represents the average value ± standard error for three replicates. The effects of different N and S fertilizer treatments were evaluated by two-way analysis of variance (ANOVA). The Shapiro–Wilk test was used to test the normality of the analytical features. According to the distributions of the estimated parameters, the least significant difference method was used for parametric tests, while the Kruskal–Wallis method was used for nonparametric tests. SPSS 19.0 (IBM, America) was used for all statistical analyses, with p < 0.05 indicating significant difference. Redundancy analysis (RDA) was performed using CANOCO software (Windows version 4.5) to determine the relationship between soil properties and microbial communities. The differences in community composition were visualized by the non-metric multidimensional scaling (NMDS) method using the vegan package meta-MDS function in R. Variance partial analysis (VPA) with RDA (X, Y, Z) was carried out in the vegan package to analyze the effects of main environmental factors and co-environmental factors on species distribution and determine the contributions of various environmental factors to species distribution. Structural equation modeling (SEM) performed using the robust maximum likelihood estimation in AMOS 24.0 (Amos, Development Corporation, Meadville, PA, United States).
Results
Total absorption of Pb and Cd
The simultaneous application of N and S fertilizers significantly enhanced the absorption of Pb and Cd by S. integra, whereas the application of N or S fertilizer alone did not have this effect (Figure 1). The N and S fertilizers had an interactive effect on the absorption of Pb and Cd. Among the treatments, the total absorptions of Pb (0.58 mg·plant−1) and Cd (1.46 mg·plant−1) by S. integra were highest under the N100S200 treatment (71.11 and 114.17% compared with the control, respectively). In contrast, the total absorptions of Pb (0.08 mg·plant−1) and Cd (0.40 mg·plant−1) were lowest under the N200S200 treatment (not significantly different compared to the control).
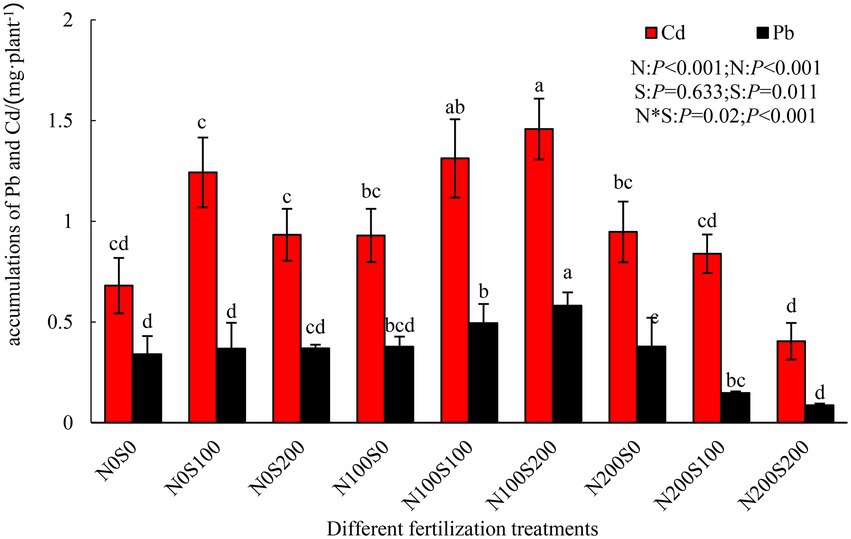
Figure 1. Effect of different fertilization treatments on the total accumulation of Pb and Cd of Salix integra Thunb. Bars with different letters represent statistically significant differences.
Soil properties
The single or simultaneous application of N and S fertilizers significantly reduced the pH (Table 1), with the lowest pH (7.84) observed under the N200S100 treatment. Interactive effects between N and S fertilization were observed on pH, CEC, and the AP, AK, nitrate, and AS contents. The single application of N fertilizer and the simultaneous application of N and S fertilizers significantly reduced the content of SOM, with the lowest value (10.11 g·kg−1) observed under the N200S100 treatment. The simultaneous application of N and S fertilizers significantly increased the CEC and AP content, with the largest values (13.24 cmol·kg−1 and 43.36 mg·kg−1, respectively) observed under the N200S200 treatment. However, the simultaneous application of N and S fertilizers significantly reduced the content of AK, with lowest value (110.95 mg·kg−1) observed under the N200S200 treatment. The application of N and S fertilizers significantly increased the content of nitrate, which was highest (105.24 mg·kg−1) under the N200S200 treatment. However, the single application of N fertilizer or the simultaneous application of N and S fertilizers significantly reduced the content of ammonium, which was lowest (23.71 mg·kg−1) under the N200S0 treatment. The application of N and S fertilizers significantly increased the content of AS, which was highest (40.81 mg·kg−1) under the N200S0 treatment.
Partitioning of Pb and Cd in soil
The relative contents of the four different forms of Pb and Cd were similar under different treatments, but the main forms of the metals were different (Figure 2). The contents of reducible Pb and Cd were relatively high, whereas the contents of acid-soluble, oxidizable, and residual Pb and Cd were relatively low. The application of N and S fertilizers increased the proportion of acid-soluble Pb in rhizosphere soil and reduced the proportion of residual Pb, but this effect was not obvious in bulk soil. Apart from the N100S0 treatment, the application of N and S fertilizers increased the relative content of acid-soluble Cd in bulk soil.
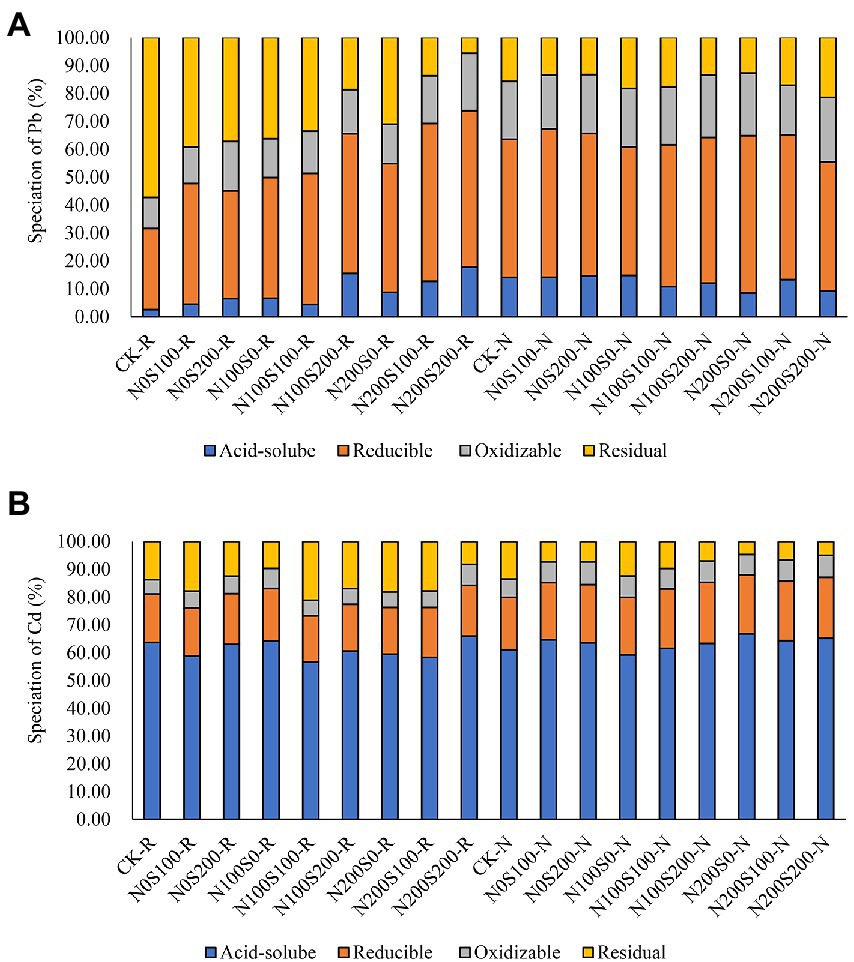
Figure 2. The content of different speciation Pb (A) and Cd (B) in rhizosphere and bulk soil under different treatments. CK rhizosphere soil (C-R), CK bulk soil (C-N), the same below.
Microbial metabolism
Metabolic activity of the microbial community
Microbial metabolic activity is reflected by average well color development (AWCD). The change in AWCD during the initial 24 h was relatively small; subsequently, AWCD gradually increased with incubation time (Figure 3). The trend of AWCD value was similar among the different treatments. The application of N and S fertilizers significantly enhanced the metabolic activity of the microbial community and increased the AWCD in the bulk soil. The rate of carbon source utilization was basically stable at 240 h; thus, the AWCD values at 240 h were used for further analysis. In the rhizosphere and bulk soils, the AWCD values were significantly higher under the N100S100 and N100S200 treatments compared to the control. The AWCD values under the other treatments did not differ significantly from that of the control.
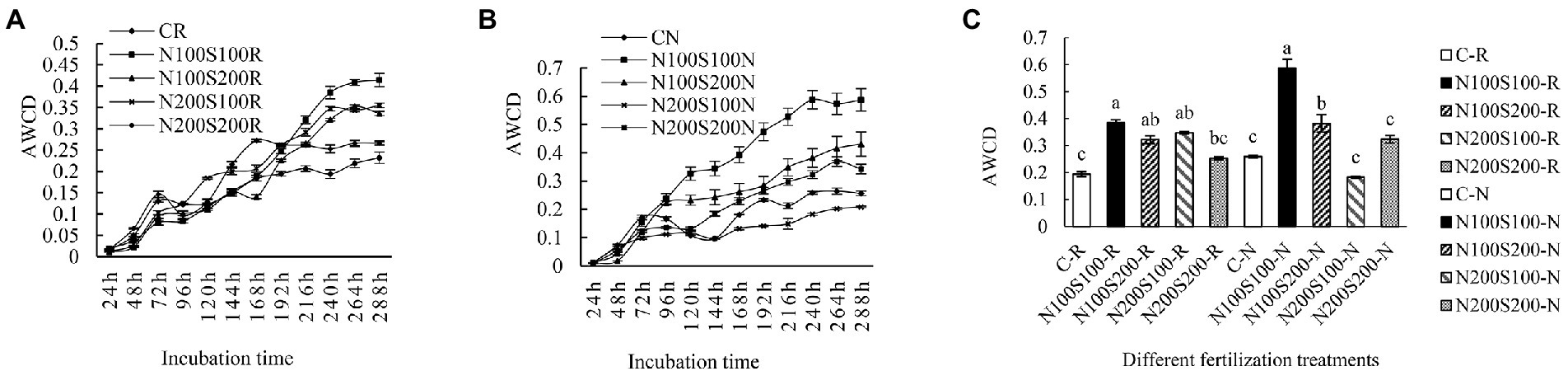
Figure 3. Effect of different fertilization treatments on microbial community metabolic activity. AWCD changes of microbial community in rhizosphere (A), AWCD changes of microbial community in bulk soil (B), microbial 240 h AWCD value (C), CK rhizosphere soil (C-R), CK bulk soil (C-N), the same below. Different letters indicate that the values differ significantly at p < 0.05.
Utilization of specific carbon sources
We classified the 31 carbon sources into four categories based on the microbial metabolic pathways of the three major nutrients: carbohydrates and their derivatives (CG, 12 kinds); amino acids and their derivatives (AG, 6 kinds); fatty acids and lipids (FG, 5 kinds); and metabolic regulation and secondary metabolites (MG, 8 kinds; Xun et al., 2018). The utilization rates of the specific carbon sources varied among the different treatments. In rhizosphere soil, the simultaneous application of N and S fertilizers significantly increased the utilization of CG, AG, FG, and MG compared with the control (Figure 4A). In bulk soil, the N100S100 and N100S200 treatments increased the utilization rates of CG, AG, and FG compared to the control (Figure 4B), while the N200S100 treatment significantly decreased the utilization of CG and AG, and the N200S200 treatment significantly increased the utilization of FG.
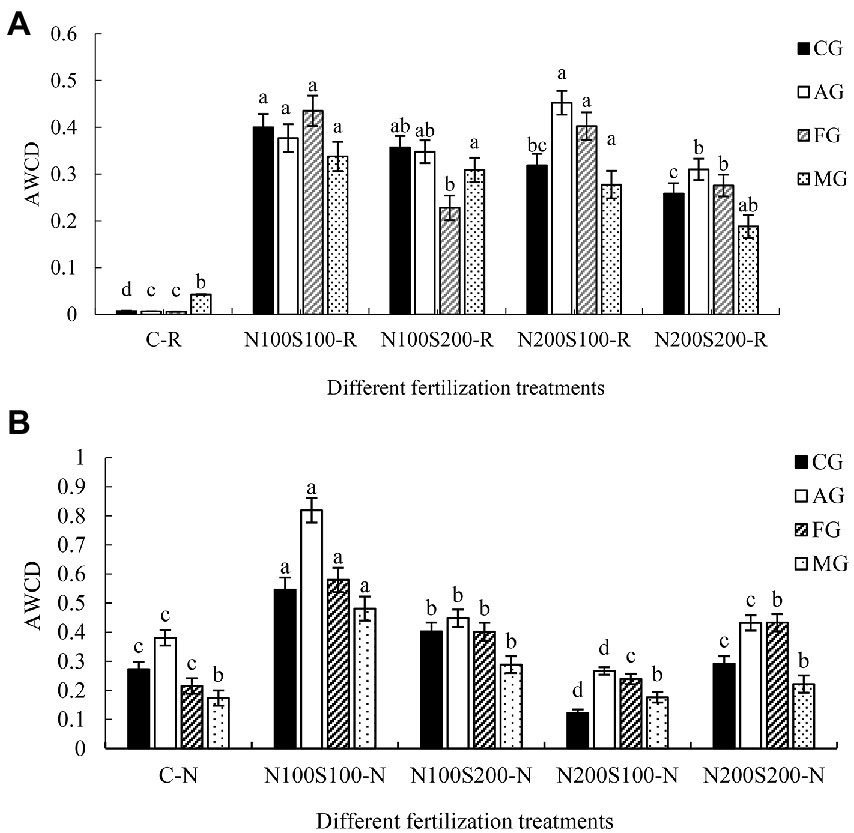
Figure 4. Utilization of the carbon source groups of microbial community. Utilization of the carbon source groups of microbial community in rhizosphere (A), utilization of the carbon source groups of microbial community in bulk soil (B), carbohydrates and their derivatives (CG), amino acids and their derivatives (AG), fatty acids and lipids (FG), metabolic regulation and secondary metabolites (MG). Different letters indicate that the values differ significantly at p < 0.05.
Metabolic profile of the microbial community
Principal component analysis was used to visualize the CSUPs of the microbial communities (Figure 5). The contribution of the first principal component (PC1) to the variance in the data was 93.1%, while the second principal component (PC2) accounted for 3.6% of the variance. All samples were distributed along the positive direction of the PC1 axis. The carbon sources utilized by the microorganisms under different treatments could be divided into three groups according to the N concentration. The first group contained the CK rhizosphere soil. The bulk CK soil and N200S100 N200S200 treatments were close to the second group, which included the rhizosphere and bulk soils. The third group contained the rhizosphere and bulk soils under the N100S100 and N100S200 treatments. The microbial metabolism also showed significant differences between the rhizosphere and bulk soils for the same treatment. Fertilization and root activity have positive effects on microbial metabolism.
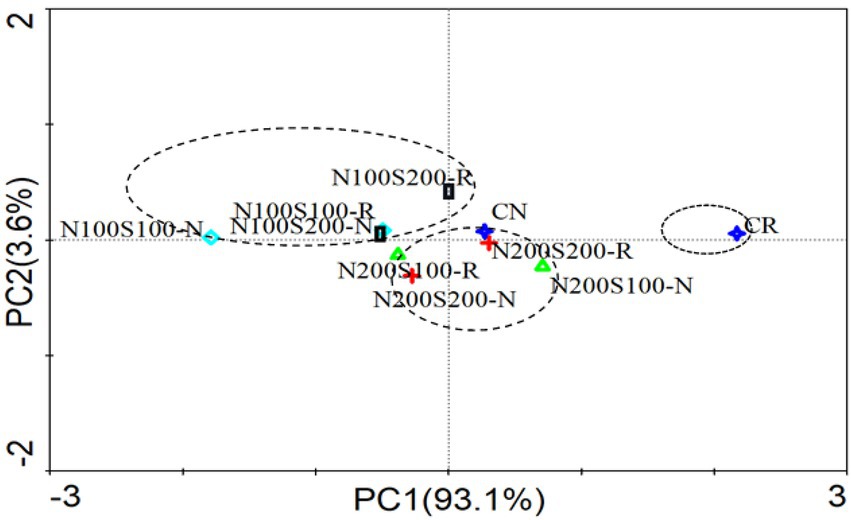
Figure 5. Principal component analysis basing carbon source utilization patterns of microbial community.
Microbial community
Richness and diversity of the microbial community
The results indicated a good coverage of the bacterial and fungal communities (between 0.98 and 0.998), suggesting that most of the fungal and bacterial taxa were detected in all soil samples (Tables 2, 3). Compared with the control, the diversity (characterized by the Shannon index) and richness (characterized by the Chao 1 index) of the fungal and bacterial communities were significantly reduced by the simultaneous application of N and S fertilizers. Among all treatments, the fungal community diversity and richness were lowest under the N100S100 treatment (Shannon index = 2.66, Chao1 index = 479.09), while the bacterial community diversity and richness were lowest under the N100S200 treatment (Shannon index = 9.40, Chao1 index = 3831.89).
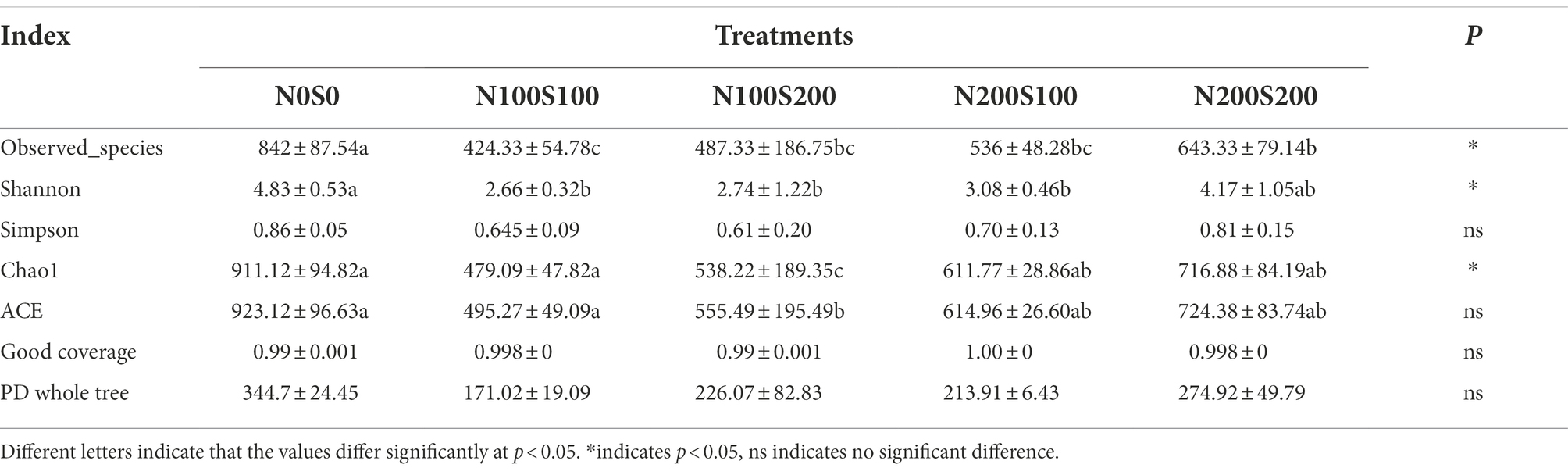
Table 2. Effect of different fertilization treatments on alpha diversity indices of fungi community.
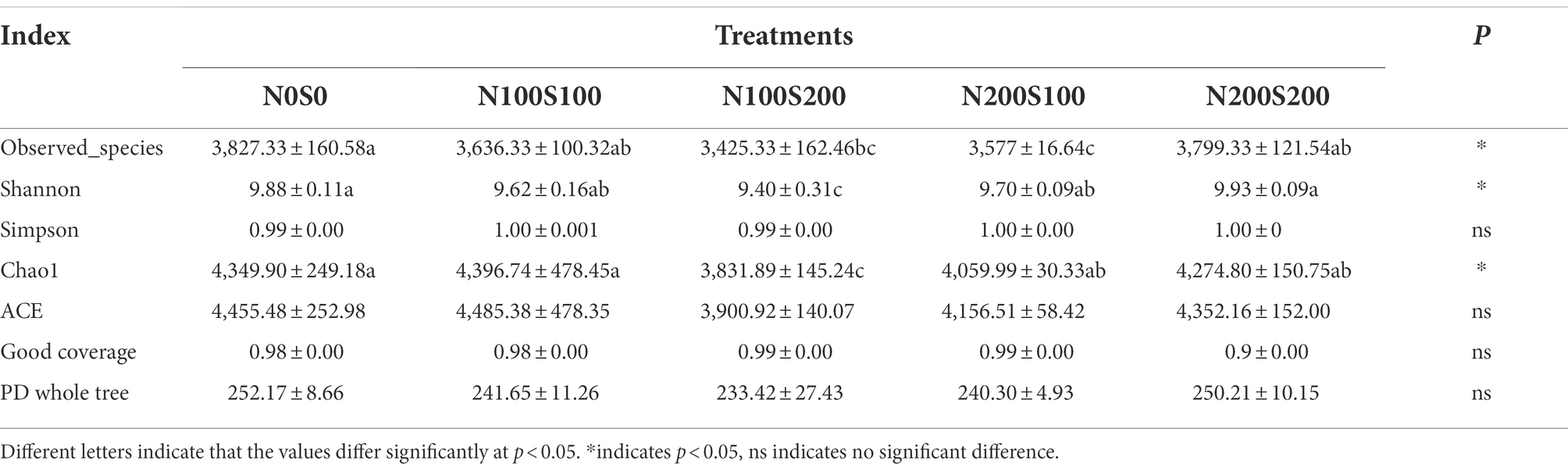
Table 3. Effect of different fertilization treatments on alpha diversity indices of bacteria community.
Composition and co-occurrence network of bacterial and fungal communities
The fungi belonged to 13 phyla (Figure 6A). While the dominant phyla were similar among the different treatments, their relative abundances differed (Table 4). Among the phyla, the relative abundance of Ascomycota was the highest, exceeding 65% in each treatment. The applied N and S fertilizers had significant interactive effects on the relative abundances of Ascomycota and Mucoromycota; their application significantly increased the relative abundance of Ascomycota and significantly decreased the relative abundance of Mucoromycota. Compared with the control, the relative abundance of Mortierellomycota increased significantly under N200S200 treatment. The bacteria belonged to 30 phyla (Figure 6B). As for the fungi, the dominant phyla (relative abundance >1%) were similar under the different treatments, while their relative abundances differed (Table 5). Among all phyla, Proteobacteria had the highest abundance in all samples followed by Acidobacteria. The applied N and S fertilizers had significant interactive effects on the abundances of Proteobacteria, Acidobacteria, Actinobacteria, Bacteroidetes and Chloroflexi; the simultaneous application of N and S fertilizers significantly increased the relative abundances of Proteobacteria, Firmicutes, Bacteroidetes and Verrucomicrobia but suppressed the relative abundances of Acidobacteria, Actinobacteria, Gemmatimonadetes, Chloroflexi and Rokubacteria. Furthermore, the relative abundances of bacteria were affected by the concentrations of N and S. For example, the relative abundances of Firmicutes, Gemmatimonadetes, Chloroflexi, and Rokubacteria were significantly reduced under N100S100 treatment compared the control, whereas no significant differences were observed under N200S200 treatment. Compared with the control, the relative abundance of Verrucomicrobia was significantly increased under N100S100 treatment, but the difference was not significant under N200S200 treatment.
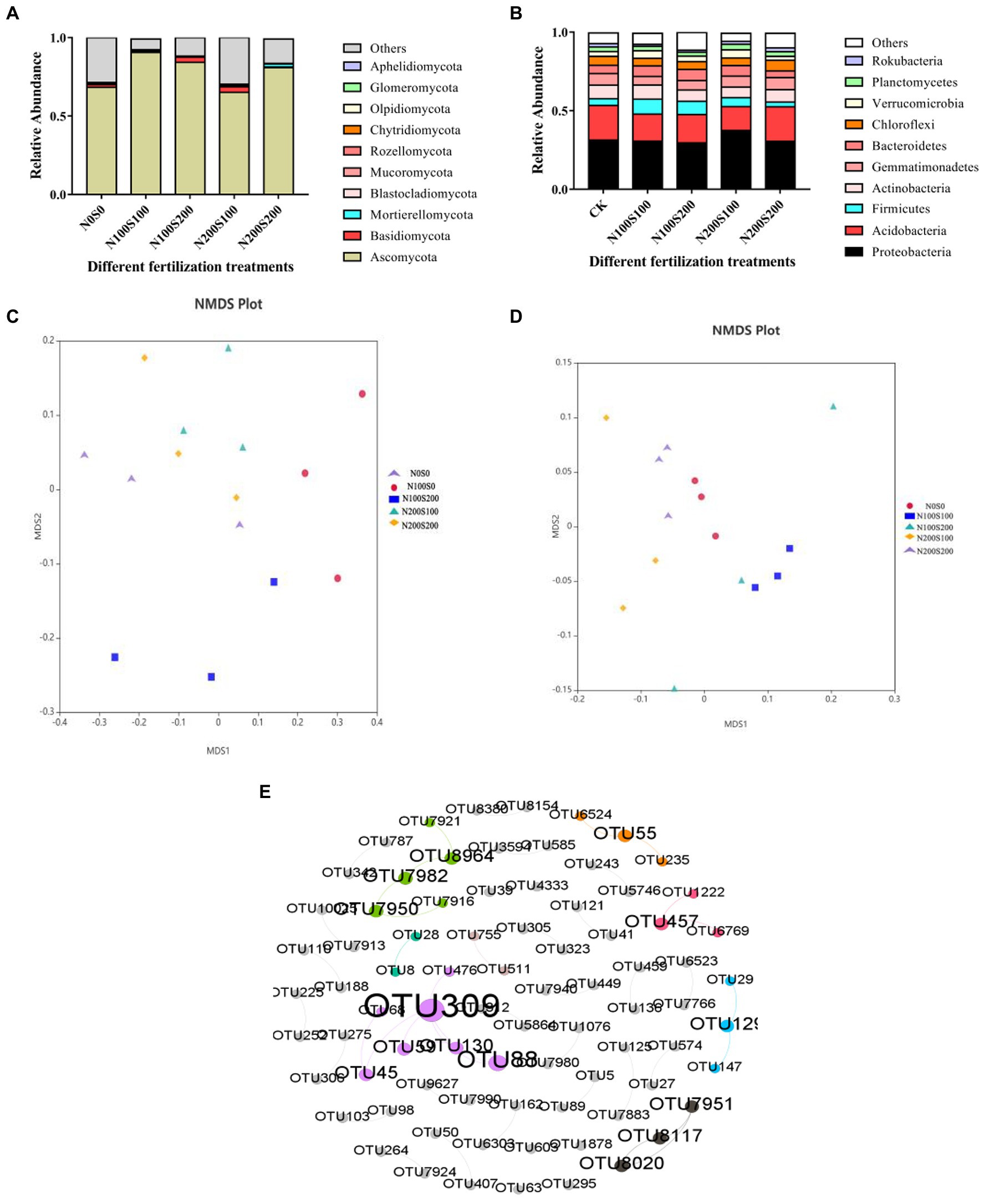
Figure 6. Effect of different fertilization treatments on fungi and bacterial community structure. The relative abundance of fungi and bacteria in rhizosphere soil with different treatments at phylum level [fungi (A), bacteria (B)]; NMDS ordination plots derived from Unifrac distance matrix [fungi (C), bacteria (D)], Co-Occurrence Patterns of bacteria and fungi (E).
NMDS analysis (stress = 0.113; stress = 0.102) revealed obvious differences in microbial community structure among the treatments (Figures 6C,D). The microorganisms formed different clusters under the different fertilization treatments, indicating that N and S fertilizers had interactive effects on the fungal and bacterial community structures. The fungal communities formed similar clusters under the N100S100 and N100S200 treatments, indicating that the N fertilizer had a stronger effect on the fungal community than the S fertilizer.
Based on an examination of the co-occurrence patterns of OTUs in the meta-network of bacteria and fungi (Figure 6E), we found a certain correlation between bacteria and fungi (Supplementary Table S2), The degree of connection was low, which may be due to the increased activity of heavy metals after fertilization, thereby weakening the connection between microorganisms. We identified two keystone taxa (nodes with a high value of both betweenness centrality and closeness centrality were defined as keystone taxa):TUO309 (g__Marinobacter) and OTU45(g__Bacillus).
Specific microbial species under different treatments
At the genus level, metastasis was used to analyze special microbial species with significant changes in relative abundance between treatments. Two-way ANOVA was used to analyze the effects of N and S fertilizers on these special species with the relative abundance of the bacteria and top 12 fungi in each sample. A total of 13 fungi genera were included (Table 6). The abundances of Gaeumannomyces, Pseudallescheria, Russula and Fusarium were lower under the N100S100 and N100S200 treatments than under the N200S100 and N200S200 treatments. The simultaneous application of N and S fertilizers reduced the relative abundances of Geopora, Dactylonectria, Sirastachys, Neocosmospora, Beauveria, Aspergillus and Aureobasidium, which were lower under the N100S100 and N100S200 treatments compared to under the N200S100 and N200S200 treatments. A total of 16 genera of bacteria were included. As shown in Table 7, the simultaneous application of N and S fertilizers increased the relative abundances of Ensifer, Iamia, Lachnoclostridium, Lacibacter, Niastella, Streptomyces and unidentified_Nitrospiraceae, which were more enriched in the promotive treatments (N100S100 and N100S200) compared with the suppressive treatment. The simultaneous application of N and S fertilizers reduced the relative abundances of Acidibacter, Faecalibaculum, Gemmatimonas and Haliangium, which were greater under the N100S100 and N100S200 treatments than under the N200S100 and N200S200 treatments.
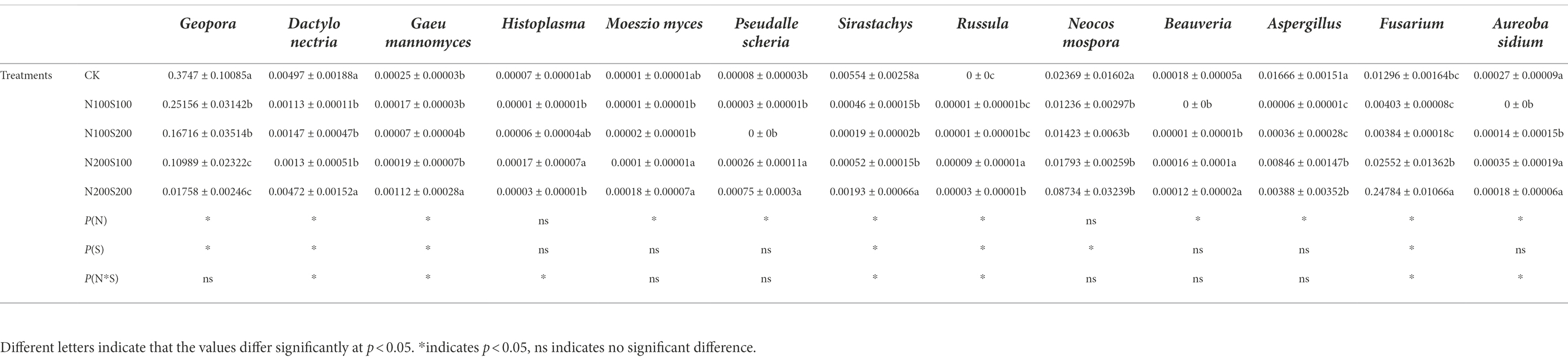
Table 6. Effect of different fertilization treatments on the relative abundance of specific fungi assemblages.
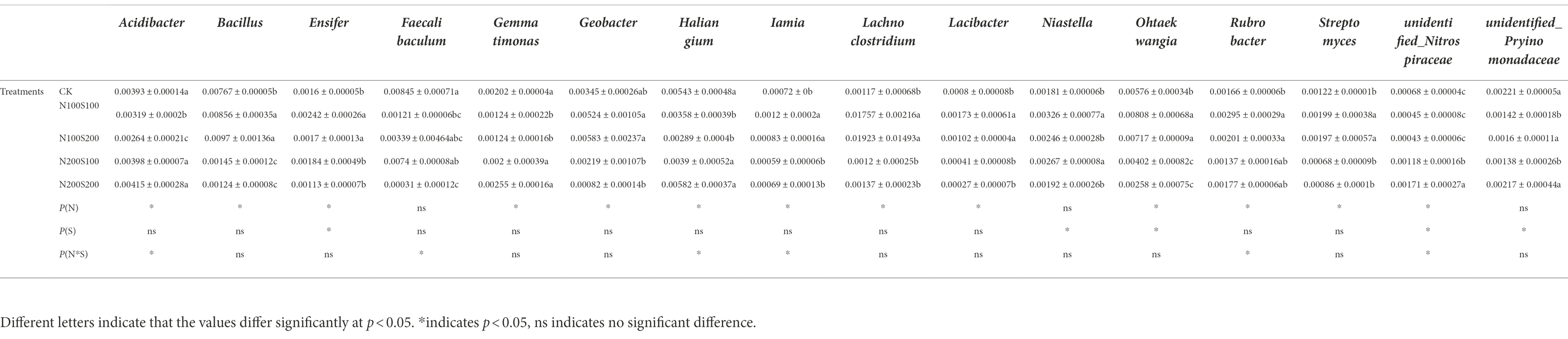
Table 7. Effect of different fertilization treatments on the relative abundance of specific bacteria assemblages.
Relationships between microbial community composition and environmental factors
RDA and VPA were used to analyze the comprehensive contribution of soil chemistry and heavy metals to bacteria and fungi (Figures 7, 8). The first and second RDA axes explained 11.5 and 38.9% of the fungal community variables, respectively. Based on RDA, the pH (R2 = 0.49), nitrate content (R2 = 0.48), and Cd content (R2 = 0.43) were the key factors affecting the fungal community in rhizosphere soil (p < 0.05; Figure 7A). VPA indicated that the Pb and Cd contents explained 21.7 and 44.3% of the total variation of the fungal community, respectively, and the interaction between Pb and Cd contents accounted for 4.23% of the total variation (Figure 8A). The first and second RDA axes explained 11.8 and 44% of the variance in the bacterial community, respectively. RDA indicated that pH (R2 = 0.61), nitrate content (R2 = 0.5), and Pb content (R2 = 0.38) were the key factors affecting the bacterial community in rhizosphere soil (p < 0.05; Figure 7B). VPA showed that the Pb and Cd contents, respectively, explained 55.78 and 26.34% of the total variation in the bacterial community (Figure 8B).
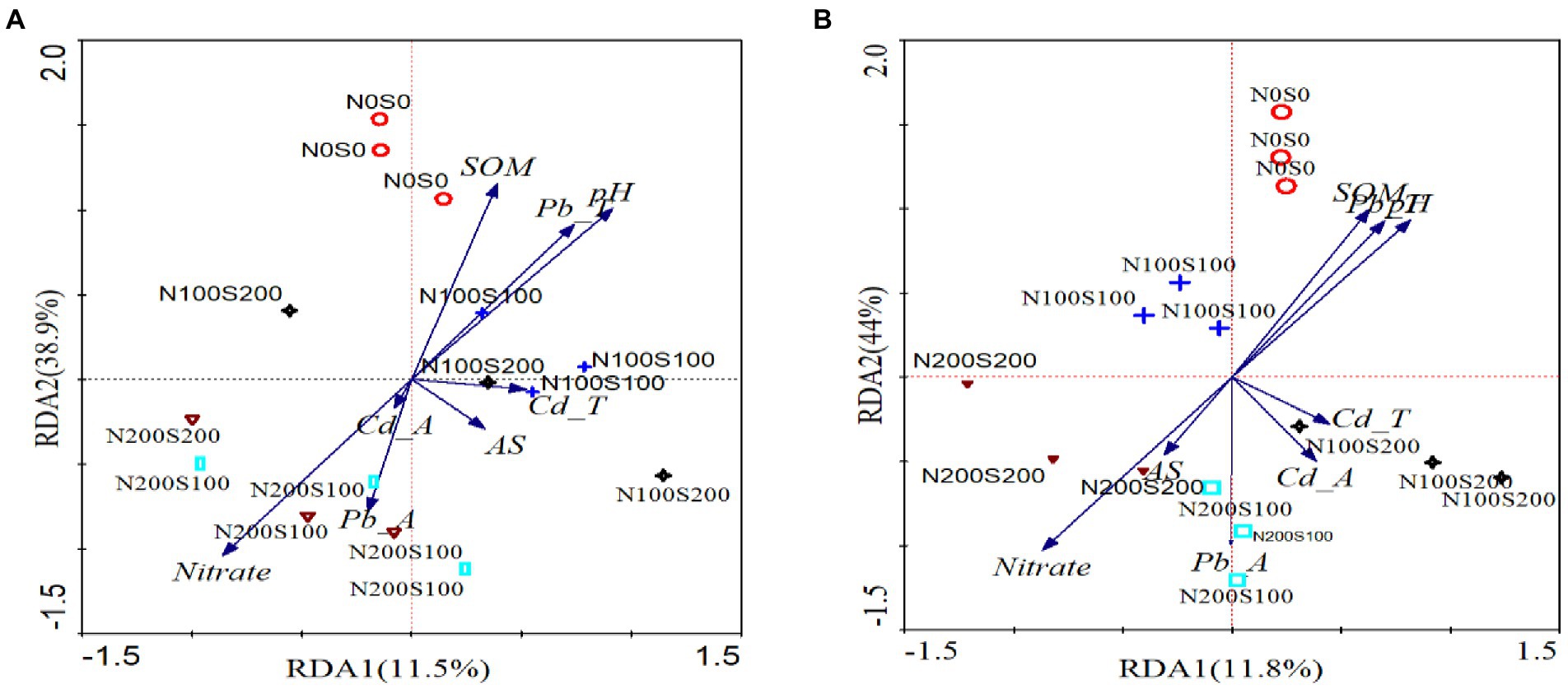
Figure 7. The RDA analysis of fungal (A), Bacterrial (B) community and soil properties. pH (pH), soil organic matter (SOM), nitrate nitrogen (Nitrate), ammonium nitrogen (Animonium), soil total Pb (Pb-T), the content of acid-solube Pb (Pb-A), soil total Cd (Cd-T), and the content of acid-solube Cd (Cd-A).
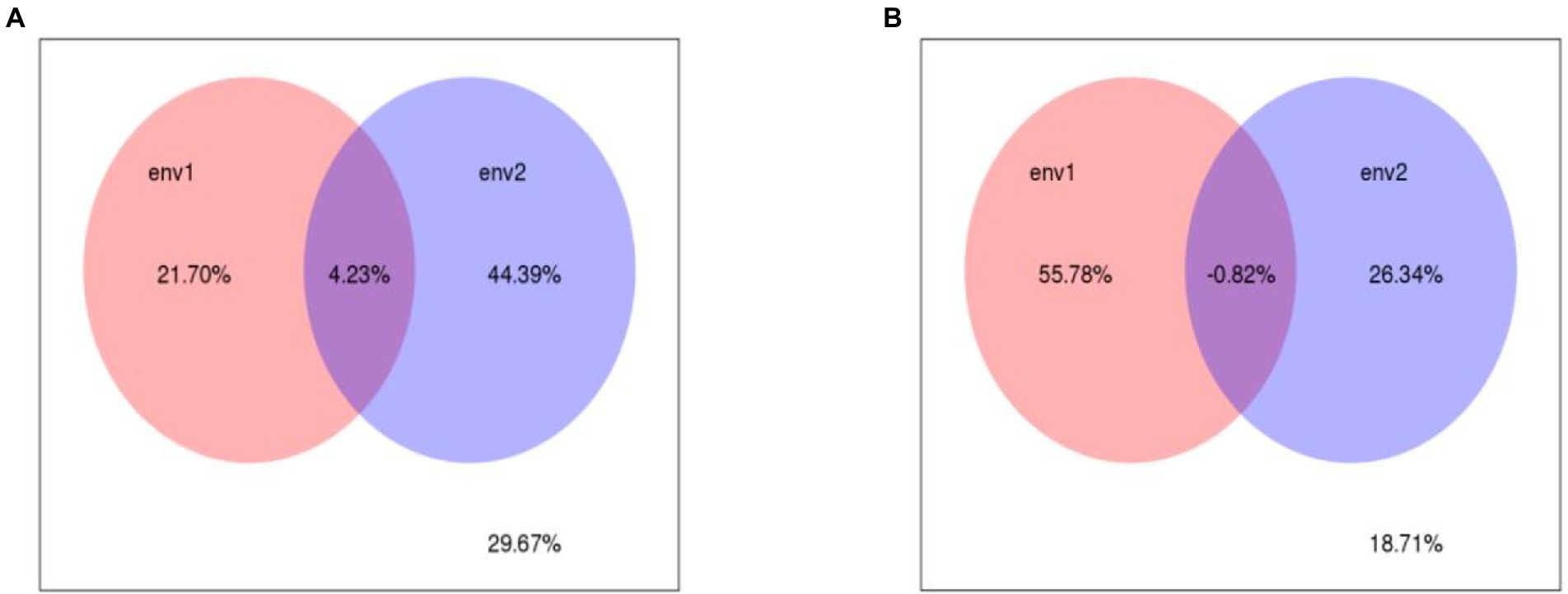
Figure 8. The VPA analysis of fungal (A), Bacterrial (B) community and soil properties. env1 (The content of different speciation Pb) and env2 (The content of different speciation Cd).
The SEM model showed that pH had significant positive effects on the cotent of acid-soluble and composition of bacteria community (Figure 9). Bacterial communities alter fungal diversity and thereby affect plant biomass and heavy metal uptake. The content of acid-soluble heavy metals was positively correlated with the total absorption of heavy metals.
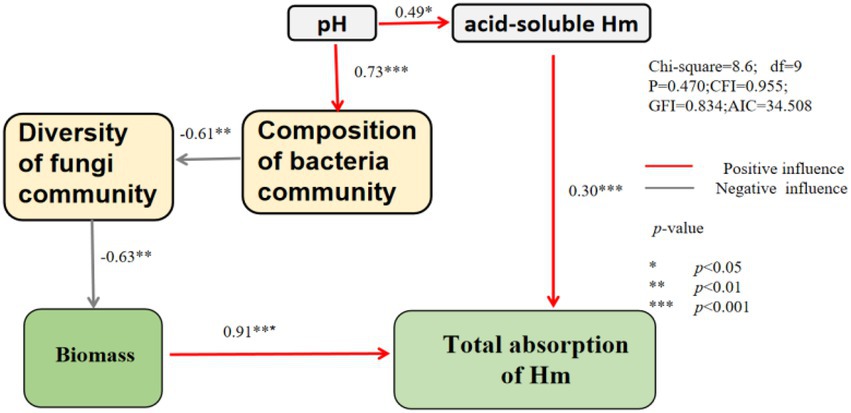
Figure 9. Structural equational model on the relationship between soil properties ˎmicrobial community and the total absorption of heavy metal.
Discussion
Effects of N and S fertilizers on soil parameters and the total absorption of soil heavy metals
In this study, the decrease in pH was likely due to the application of N fertilizer, which stimulated nitrification and caused soil acidification (Zhou et al., 2015). Additionally, the contents of nitrate, AP and AS also increased after the application of N and S fertilizers, whereas the effects on soil chemical properties became more significant as the concentrations of N and S increased. These results might be attributed to changes in nutrients that improved microbial activity, stimulated nitrification, and increased the activity of soil phosphatase (Marklein et al., 2012; Tafazoli et al., 2019). Furthermore, the availability of N and S stimulate each other, the addition of S fertilizer reduced the leaching of soil nitrate, thereby improving the availability of N and increasing the content of AS in soil (Wang et al., 2016).
The phytoextraction of Pb and Cd by S. integra was enhanced under the N100S100 and N100S200 treatments, which can be attributed to the increased biomass and activity of heavy metals. Chai et al. (2018) reported that fertilizers enhanced soil fertility and prometed plant growth, which increased the accumulation of heavy metals. Fertilizers can also regulate microbial community to affect heavy metal absorption (Wang et al., 2020). The addition of N and S fertilizers can further increase the activity of heavy metal ions, and promote the accumulation of heavy metals in plants, however, excessive fertilizer can increase the activity of heavy metal ions to a level that causes toxic effects in plants themselves and inhibits plant growth (Wang et al., 2019).
Bioavailability of soil heavy metals
In this study, the application of N and S fertilizers increased the bioavailability of soil heavy metal ions by reducing the soil pH, in agreement with previous studies thatis associated with enhanced heavy metal ion mobility and bioavailability (Gabarrón et al., 2018; Novak et al., 2018). Decreasing the SOM content also increased the solubility and activity of heavy metals, which might be due to a reduction in the number of metal adsorption sites and metal chelating agents (Venegas et al., 2015, 2016). Moreover, the application of N and S fertilizers increased the contents of acid-extracted Pb and Cd in rhizosphere soil but had no effect in non-rhizosphere soil, indicating that the heavy metal form was also affected by the root activity, particularly root exudates (Montiel-Rozas et al., 2016). Other studies have demonstrated that root exudates such as organic acids and amino acids can stimulate the conversion of insoluble heavy metal forms into soluble forms, thereby increasing the bioavailability and improving the remediation efficiency of plants (Luo et al., 2017; Liu et al., 2018b; Moshiri et al., 2019).
Microbial community and metabolism
The application of N and S fertilizers reduced the diversity and richness of rhizosphere microorganisms. The structure of the rhizosphere microbial community varied significantly between different treatments, suggesting that soil microorganisms may adapt to different levels of nutrients in the environment by changing their community structure and composition (Zhou et al., 2017; Wang et al., 2018b; Li et al., 2019). The application of N and S fertilizers caused the Chao1 and Shannon indexes of the fungal community to decrease more than those of the bacterial community, consistent with Fierer et al. (2012), who found N application had little effect on bacterial community diversity. This may be because Pb and Cd are less toxic to the bacterial community than to the fungal community. Bacteria are more adaptable to abiotic stresses (e.g., heavy metals) than fungi, possibly due to the faster metabolism and higher substrate utilization of bacteria compared to fungi (David and Erland, 2016). Additionally, bacterial communities are dominant in soil and compete with fungi for nutrients (Deng et al., 2015). Changes in the microbial community structure can lead to changes in microbial metabolic functions (Tipayno et al., 2018). In this study, N and S fertilization increased the utilization rate of carbon sources. The utilization rate of CG in rhizosphere soil was greater than that in bulk soil, while the utilization rates of AG and CG in rhizosphere soil were lower than those in bulk soil. These results suggest that different microbial communities formed in the rhizosphere and bulk soils as a result of plant root activity. Compared with the bulk soil, the metabolic activity of rhizosphere microorganisms was significantly improved after fertilization, indicating that the establishment of beneficial interactions of microorganisms with plants, which was beneficial to improve phytoremediation efficiency. Microbial variation is closely related to the rhizosphere environment, leading to differences in microbial metabolism (Sasse et al., 2018). These changes have significant effects on the activation of heavy metals and the promotion of phytoremediation. In this study, RDA analysis indicated that the pH and nitrate content had significant effects on the structure of the rhizosphere microbial community. The composition of the microbial community changes in long-term contaminated soil, and the changes depend on the heavy metals and chemical properties of the soil (Zhang et al., 2016; Fajardo et al., 2018). Therefore, the application N and S fertilizers influences the absorption of heavy metals by affecting the metabolic activity and metabolic mode of microorganisms. The rhizosphere pH and the content of nitrate might play key roles in phytoremediation by S. integra.
Changes in dominant and special microbes
The dominant bacterial species in this study were the same under different fertilization treatments; however, the relative abundances of different species were different. This may be due to the selection of microorganisms in response to changes in the rhizosphere environment. Increasing the bioavailability of heavy metals may affect the diversity of microorganisms by suppressing sensitive species that lack sufficient tolerance to heavy metal stress while also stimulating heavy metal-tolerant species (Xie et al., 2016). Under all treatments, the dominant fungal phyla were Ascomycota and Basidiomycota, with the relative abundance of Ascomycota exceeding 65%. These species play an important role in improving the tolerance of the host to heavy metals (Wang et al., 2021). In this study, N and S fertilizers increased the relative abundance of Ascomycota in rhizosphere soil. We speculate that the application of N and S fertilizers might affect mycorrhizal symbiosis, thereby influencing the resistance of S. integra to heavy metals. The relative abundance of Proteobacteria exceeded 29.5% under the different treatments. Guo et al. (2019) reported that Proteobacteria may be the most metal-resistant bacteria found in sites contaminated with heavy metals. Therefore, we speculate that Proteobacteria plays an important role in the restoration of heavy metal-contaminated soils by S. integra. However, the relative abundance of Acidobacteria, which was the second most abundant phylum, was suppressed by N and S fertilization, and its function cannot be ignored. Xu et al. (2021) suggested that Acidobacteria may resist or transform metal(loid)s in metal-contaminated sites. In this study, the relative abundance of Bacteroidetes was also increased by the application of N and S fertilizers, and Lin et al. (2019) reported that Bacteroidetes are the most dominant microbial group in heavy metal-contaminated soil. Actinobacteria are considered to be the key taxon in contaminated soil (Chao et al., 2016; Jiao et al., 2016; Hou et al., 2018; Samiran et al., 2018). These detected bacteria can reduce metal toxicity, prevent and control soil diseases, and adapt to extreme environments and environmental pressures (Li et al., 2015; Guo et al., 2019). Therefore, to improve the phytoremediation efficiency, rhizosphere microorganisms can be added to the rhizosphere of fast-growing woody plants.
At the genus level, some special fungi are pathogenic fungi such as Fusarium (Wen et al., 2020). They were less enriched in treatments (N100S100, N100S200), which promote phytoremediation. Thus, we speculate that the simultaneous application of N and S fertilizers improve the rhizosphere health of S. integra and promote its resistance to pathogens. Other special bacterial species that are enriched by fertilization may promote phytoremediation and play an important role in the remediation of contaminated soil. For example, Streptomyces produces siderophores to improve the absorption of certain nutrients by plants, and the secreted IAA can also stimulate plant growth (Huang et al., 2014), Bacillus and Ensifer have been reported to promote rhizosphere growth (Mesa et al., 2017; Sajid et al., 2020). Inoculating rhizosphere growth-promoting bacteria improve the repair efficiency of plants (Ke et al., 2021) and interact beneficially with AMF to enhance the extraction of heavy metals by plants (Mahohi and Raiesi, 2019). Some beneficial rhizosphere genera such as Acidibacter and Streptomyces significantly increased Cd uptake by S. alfredii (Yang et al., 2019). Other indigenous microbial communities also play important roles in the absorption of heavy metals by S. integra. (Niu et al., 2020). The mutually beneficial relationships between bacteria and fungi can be taken advantage of to improve the heavy metal tolerance of plants and assist in the phytoremediation of heavy metal-contaminated soil. We can infer that the application of N and S fertilizers affects the absorption of Pb and Cd by regulating the rhizosphere microbial composition.
Conclusion
The application of N and S fertilizers affected the pH and nitrate content in the rhizosphere environment, increased the bioavailability of heavy metals, changed the utilization patterns of carbon sources by microbes, and altered the structure of the microbial community to affect the absorption of Pb and Cd by S. integra. Moreover, the application of N and S fertilizers can limit soil-born fungal diseases and enrich rhizosphere growth-promoting bacteria to improve the phytoremediation efficiency. Our findings provide a possible strategy for the plant-microbial remediation of polluted soil using fast-growing trees.
Data availability statement
The datasets generated for this study can be found in https://www.ncbi.nlm.nih.gov/sra/PRJNA846123 and PRJNA846147, respectively.
Author contributions
SW: methodology, visualization, investigation, formal analysis, and writing–original draft. XN: software, writing– review and editing, and supervision. DD: formal analysis and methodology. DH: resources, writing–review and editing, and supervision. All authors contributed to the article and approved the submitted version.
Funding
This research was funded by Natural Science Foundation of Hebei province (No. C2022204161), Basic Scientific Research Funds of Hebei Provincial colleges and universities (No. KY2021053), Youth Fund Project of Hebei Provincial Education Department (No. QN2022120), and Subjects Group of Modern Forestry (No. XK1008601519). This research was also partly supported by the National Natural Science Foundation of China (Nos. 31971651 and 31600486).
Conflict of interest
The authors declare that the research was conducted in the absence of any commercial or financial relationships that could be construed as a potential conflict of interest.
Publisher’s note
All claims expressed in this article are solely those of the authors and do not necessarily represent those of their affiliated organizations, or those of the publisher, the editors and the reviewers. Any product that may be evaluated in this article, or claim that may be made by its manufacturer, is not guaranteed or endorsed by the publisher.
Supplementary material
The Supplementary Material for this article can be found online at: https://www.frontiersin.org/articles/10.3389/fmicb.2022.945847/full#supplementary-material
References
Buée, M., De, B. W., Martin, F., Van Overbeek, L., and Jurkevitch, E. (2009). The rhizosphere zoo: an overview of plant-associated communities of microorganisms, including phages, bacteria, archaea, and fungi, and of some of their structuring factors. Plant Soil 321, 189–212. doi: 10.1007/s11104-009-9991-3
Cao, Y. N., Ma, C. X., Chen, H. J., Chen, G. C., White, J. C., and Xing, B. S. (2020). Copper stress in flooded soil: impact on enzyme activities microbial community composition and diversity in the rhizosphere of Salix integra. Sci. Total Environ. 704:135350. doi: 10.1016/j.scitotenv.2019.135350
Caporaso, J. G., Lauber, C. L., Walters, W. A., Berg-Lyons, D., Lozupone, C. A., Turnbaugh, P. J., et al. (2011). Global patterns of 16S rRNA diversity at a depth of millions of sequences per sample. Proc. Natl. Acad. Sci. U. S. A. 108, 4516–4522. doi: 10.1073/pnas.1000080107
Chai, M. W., Li, R. Y., Shen, X. X., Tam, N. F. Y., Zan, Q. J., and Li, R. L. (2018). Does ammonium nitrogen affect accumulation subcellular distribution and chemical forms of cadmium in Kandelia obovata? Ecotox. Environ. Safe. 162, 430–437. doi: 10.1016/j.ecoenv.2018.07.031
Chao, Y. Q., Liu, W. S., Chen, Y. M., Chen, W. H., Zhao, L. H., Ding, Q. B., et al. (2016). Structure, variation, and co-occurrence of soil microbial communities in abandoned sites of a rare earth elements mine. Environ. Sci. Technol. 50, 11481–11490. doi: 10.1021/acs.est.6b02284
David, F. C., and Erland, B. (2016). Interaction between pH and cu toxicity on fungal and bacterial performance in soil. Soil Biol. Biochem. 96, 20–29. doi: 10.1016/j.soilbio.2016.01.010
Dede, G., and Ozdemir, S. (2016). Effects of elemental sulfur on heavy metal uptake by plants growing on municipal sewage sludge. J. Environ. Manag. 166, 103–108. doi: 10.1016/j.jenvman
Deng, L. J., Zeng, G. M., Fan, C. Z., Lu, L. H., Chen, X. F., Chen, M., et al. (2015). Response of rhizosphere microbial community structure and diversity to heavy metal co-pollution in arable soil. Appl. Microbiol. Biot. 99, 8259–8269. doi: 10.1007/s00253-015-6662-6
Fajardo, C., Costa, G., Nande, M., Botías, P., García-Cantalejo, J., and Martín, M. (2018). Pb, Cd, and Zn soil contamination: monitoring functional and structural impacts on the microbiome. Appl. Soil Ecol. 135, 56–64. doi: 10.1016/j.apsoil.2018.10.022
Fierer, N., Lauber, C. L., Ramirez, K. S., Zaneveld, J., Bradford, M. A., and Knight, R. (2012). Comparative metagenomic, phylogenetic and physiological analyses of soil microbial communities across nitrogen gradients. ISME J. 6, 1007–1017. doi: 10.1038/ismej.2011.159
Gabarrón, M., Afaz, S., Martínez, M., and Acosta, J. A. (2018). Change in metals and arsenic distribution in soil and their bioavailability beside old tailing ponds. J. Environ. Manag. 212, 292–300. doi: 10.1016/j.jenvman.2018.02.010
Gong, X. M., Huang, D. L., Liu, Y. G., Zeng, G. M., Wang, R. Z., Wei, J. J., et al. (2018). Pyrolysis and reutilization of plant residues after phytoremediation of heavy metals contaminated sediments: for heavy metals stabilization and dye adsorption. Bioresour. Technol. 253, 64–71. doi: 10.1016/j.biortech.2018.01.018
Guo, D. C., Fan, Z. Z., Lu, S. Y., Ma, Y. J., Nie, X. H., Tong, F. P., et al. (2019). Changes in rhizosphere bacterial communities during remediation of heavy metal accumulating plants around the Xikuangshan mine in southern China. Sci. Rep. 9:1947. doi: 10.1038/s41598-018-38360-2
Hou, D. D., Lin, Z., Wang, R. Z., Ge, J., We, I. S., Xie, R. H., et al. (2018). Cadmium exposure Sedum alfredii planting interactions shape the bacterial community in the hyperaccumulator plant rhizosphere. Appl. Environ. Microb. 84:e02797-17. doi: 10.1128/AEM.02797-17
Hou, D. D., Wang, K., Liu, T., Wang, H. X., Lin, Z., Qian, J., et al. (2017). Unique rhizosphere micro-characteristics facilitate phytoextraction of multiple metals in soil by the hyperaccumulating plant Sedum alfredii, environ. Sci. Technol. 51, 5675–5684. doi: 10.1021/acs.est.6b06531
Hu, H., Jin, Q., and Kavan, P. (2014). A study of heavy metal pollution in China: current status, pollution control policies and countermeasures. Sustainability 6, 5820–5838. doi: 10.3390/su6095820
Huang, X. F., Chaparro, J. M., Reardon, K. F., Zhang, R. F., Shen, Q. R., and VivancoJorge, M. (2014). Rhizosphere interactions: root exudates, microbes, and microbial communities. Botany 92, 267–275. doi: 10.1139/CJB-2013-0225
Iqbal, M., Puschenreiter, M., Oburger, E., Santner, J., and Wenzel, W. W. (2012). Sulfur-aided phytoextraction of Cd and Zn by Salix smithiana combined with in situ metal immobilization by gravel sludge and red mud. Environ. Pollut. 70, 222–231. doi: 10.1016/j.envpol.2012.07.008
Jiao, S., Liu, Z. S., Lin, Y. B., Yang, J., Chen, W. M., and Wei, G. H. (2016). Bacterial communities in oil contaminated soils: biogeography and co-occurrence patterns. Soil Biol. Biochem. 98, 64–73. doi: 10.1016/j.soilbio.2016.04.005
Ke, T., Zhang, J., Tao, Y., Zhang, C., Zhang, Y. R., Xu, Y. H., et al. (2021). Individual and combined application of Cu tolerant Bacillus spp. enhance the Cu phytoextraction efficiency of perennial ryegrass. Chemosphere 263:127952. doi: 10.1016/j.chemosphere.2020.127952
Khan, K. S., Mack, R., Castillo, X., Kaiser, M., and Joergensen, R. G. (2016). Microbial biomass, fungal and bacterial residues, and their relationships to the soil organic matter C/N/P/S ratios. Geoderma 271, 115–123. doi: 10.1016/j.geoderma.2016.02.019
Konkolewska, A., Piechalak, A., Ciszewska, L., Antos-Krzemińska, N., Skrzypczak, T., Hanć, A., et al. (2020). Combined use of companion planting and PGPR for the assisted phytoextraction of trace metals (Zn, Pb, Cd). Environ. Sci. Pollut. R. 27, 13809–13825. doi: 10.1007/s11356-020-07885-3
Li, Y. Y., Chen, L. Q., and Wen, H. Y. (2015). Changes in the composition and diversity of bacterial communities 13 years after soil reclamation of abandoned mine land in eastern China. Ecol. Res. 30, 357–366. doi: 10.1007/s11284-014-1230-6
Li, X. Z., Yu, H., Sun, X. W., Yang, J. T., Wang, D. C., Shen, L. F., et al. (2019). Effects of sulfur application on cadmium bioaccumulation in tobacco and its possible mechanisms of rhizospheric microorganisms. J. Hazard. Mater. 368, 308–315. doi: 10.1016/j.jhazmat.2018.12.099
Lin, Z. W., Dou, C. Y., Li, Y. F., Wang, H. L., Niazi, N. K., Zhang, S. B., et al. (2020). Nitrogen fertilizer enhances zinc and cadmium uptake by hyperaccumulator Sedum alfredii. J. Soils Sediments 20, 320–329. doi: 10.1007/s11368-019-02405-4
Lin, Y. B., Ye, Y. M., Hu, Y. M., and Shi, H. K. (2019). The variation in microbial community structure under different heavy metal contamination levels in paddy soils. Ecotox. Environ. Safe. 180, 557–564. doi: 10.1016/j.ecoenv.2019.05.057
Liu, C. J., Lin, H., Dong, Y. B., Li, B., and Liu, Y. (2018b). Investigation on microbial community in remediation of lead contaminated soil by Trifolium repens L. Ecotox. Environ. Safe. 165, 52–60. doi: 10.1016/j.ecoenv.2018.08.054
Liu, J. S., Zhang, X., Wang, H., Hui, X. L., Wang, Z. H., and Qiu, W. H. (2018a). Long-term nitrogen fertilization impacts soil fungal and bacterial community structures in a dryland soil of Loess Plateau in China. J. Soils Sediments 18, 1632–1640. doi: 10.1007/s11368-017-1862-6
Lu, R. K., (2000). Methods of Soil and Agrochemical Analysis. Beijing: China Agricultural Science and Technology Press, 127–332.
Luo, Q., Wang, S. Y., Sun, L. N., and Wang, H. (2017). Metabolic profiling of root exudates from two ecotypes of Sedum alfredii treated with Pb based on GC-MS. Sci. Rep. 7:39878. doi: 10.1038/srep39878
Ma, Y. T., Li, J. Q., Wu, J., Kong, Z. Y., Feinstein, L. M., Ding, X., et al. (2018). Bacterial and fungal community composition and functional activity associated with Lake wetland water level gradients. Sci. Rep. 8:760. doi: 10.1038/s41598-018-19153-z
Ma, Y., Rajkumar, M., Zhang, C., and Freitas, H. (2016). Beneficial role of bacterial endophytes in heavy metal phytoremediation. J. Environ. Manag. 174, 14–25. doi: 10.1016/j.jenvman.2016.02.047
Mahohi, A., and Raiesi, F. (2019). Functionally dissimilar soil organisms improve growth and Pb/Zn uptake by Stachys inflata grown in a calcareous soil highly polluted with mining activities. J. Environ. Manag. 247, 780–789. doi: 10.1016/j.jenvman.2019.06.130
Manta, D. S., Angelone, M., Bellanca, A., Neri, R., and Sprovieri, M. (2002). Heavy metals in urban soils: a case study from the city of Palermo (Sicily), Italy. Sci. Total Environ. 300, 229–223. doi: 10.1016/s0048-9697(02)00273-5
Maqbool, A., Ali, S., Rizwan, M., Arif, M. S., Yasmeen, T., Riaz, M., et al. (2020). N-fertilizer (urea) enhances the phytoextraction of cadmium through Solanum nigrum L. Int. J. Environ. Res. Public Health 17:3850. doi: 10.3390/ijerph17113850
Marklein, H., Marklein, A. R., and Houlton, B. Z. (2012). Nitrogen inputs accelerate phosphorus cycling rates across a wide variety of terrestrial ecosystems. New Phytol. 193, 696–704. doi: 10.1111/j.1469-8137.2011.03967.x
Masuda, S., Bao, Z. H., Okubo, T., Sasaki, K., Ikeda, S., Shinoda, R., et al. (2016). Sulfur fertilization changes the community structure of rice root-, and soil-associated bacteria. Microbes Environ. 31, 70–75. doi: 10.1264/jsme2.ME15170
Mesa, V., Navazas, A., González-Gil, R., González, A., Weyens, N., Lauga, B., et al. (2017). Use of endophytic and rhizosphere bacteria to improve phytoremediation of arsenic-contaminated industrial soils by autochthonous Betula celtiberica. Appl. Environ. Microb. 83, e03411–e03416. doi: 10.1128/AEM.03411-16
Mitra, S., Pramanik, K., Sarkar, A., Ghosh, P. K., Soren, T., and Maiti, T. K. (2018). Bioaccumulation of cadmium by Enterobacter sp. and enhancement of rice seedling growth under cadmium stress. Ecotox. Environ. Safe. 156, 183–196. doi: 10.1016/j.ecoenv.2018.03.001
Moharami, S., and Jalali, M. (2015). Effect of acid rain on the fractionation of heavy metals and major elements in contaminated soils. Chem. Ecol. 31, 160–172. doi: 10.1080/02757540.2014.917173
Montiel-Rozas, M. M., Madejón, E., and Madejón, P. (2016). Effect of heavy metals and organic matter on root exudates (low molecular weight organic acids) of herbaceous species: An assessment in sand and soil conditions under different levels of contamination. Environ. Pollut. 216, 273–281. doi: 10.1016/j.envpol.2016.05.080
Moshiri, F., Ebrahimi, H., Ardakani, M. R., Rejali, F., and Mousavi, S. M. (2019). Biogeochemical distribution of Pb and Zn forms in two calcareous soils affected by mycorrhizal symbiosis and alfalfa rhizosphere. Ecotox. Environ. Safe. 179, 241–248. doi: 10.1016/j.ecoenv.2019.04.055
Niu, X. Y., Wang, S. K., Zhou, J., Di, D. I., Sun, P., and Huang, D. Z. (2021). Inoculation with indigenous rhizosphere microbes enhances aboveground accumulation of lead in Salix integra Thunb. by improving transport coefficients. Front. Microbiol. 12:686812. doi: 10.3389/fmicb.2021.686812
Niu, X. Y., Zhou, J., Wang, X. N., Su, X. Y., Du, S. H., Zhu, Y. F., et al. (2020). Indigenous bacteria have high potential for promoting Salix integra Thunb. remediation of lead contaminated soil by adjusting soil properties. Front. Microbiol. 11:194. doi: 10.3389/fmicb.2020.00924
Niu, X. Y., Zhou, Y., Zhou, J., Wang, X. N., Gao, Z. T., and Huang, D. Z. (2019). The effects of different lead pollution levels on soil microbial quantities and metabolic function with/without Salix integra Thunb. planting. Forests 10:77. doi: 10.3390/f10020077
Novak, J. M., Ippolito, J. A., Ducey, T. F., Wattsa, D. W., Spokase, K. A., Tripped, K. M., et al. (2018). Remediation of an acidic mine spoil: miscanthus biochar and lime amendment affects metal availability, plant growth, and soil enzyme activity. Chemosphere 205, 709–718. doi: 10.1016/j.chemosphere.2018.04.107
Oksanen, J., Blanchet, F. G., and Kindt, R., (2013). Vegan: community ecology package. R package version 2.0-10 (online).
Philippot, L., Raaijmakers, J. M., Lemanceau, P., and Putten, W. H. (2013). Going back to the roots: the microbial ecology of the rhizosphere. Nat. Rev. Microbiol. 11, 789–799. doi: 10.1038/nrmicro3109
Pramanik, K., Mitra, S., Sarkar, A., and Maiti, T. K. (2018). Alleviation of phytotoxic effects of cadmium on rice seedlings by cadmium resistant PGPR strain, Enterobacter aerogenes MCC 3092. J. Hazard. Mater. 351, 317–329. doi: 10.1016/j.jhazmat.2018.03.009
Rauret, G., Lopez-Sanchez, J. F., Sahuquillo, A., Rubio, R., Davidson, C., Ure, A., et al. (1999). Improvement of the BCR three step sequential extraction procedure prior to the certification of new sediment and soil reference materials. Environ. Monit. Assess. 1, 57–61. doi: 10.1039/A807854H
Sajid, M., Zhao, X. Q., and Shen, R. F. (2020). Bacillus pumilus promotes the growth and nitrogen uptake of tomato plants under nitrogen fertilization. Sci. Hortic. 272:109581. doi: 10.1016/j.scienta.2020.109581
Samiran, B., Klaus, S., and Marcel, G. A. (2018). Keystone taxa as drivers of microbiome structure and functioning. Nat. Rev. Microbiol. 16, 567–576. doi: 10.1038/s41579-018-0024-1
Sasse, J., Martinoia, E., and Northen, T. (2018). Feed your friends: do plant exudates shape the root microbiome? Trends Plant Sci. 23, 25–41. doi: 10.1016/j.tplants.2017.09.003
Sessitsch, A., Kuffner, M., Kidd, P., Vangronsveld, J., Wenzel, W. W., Fallmann, K., et al. (2013). The role of plant-associated bacteria in the mobilization and phytoextraction of trace elements in contaminated soils. Soil Biol. Biochem. 60, 182–194. doi: 10.1016/j.soilbio.2013.01.012
Shi, X., Wang, S. F., Sun, H. J., Chen, Y. T., Wang, D. X., Pan, H. W., et al. (2017). Comparative of Quercus spp. and Salix spp. for phytoremediation of Pb/Zn mine tailings. Environ. Sci. Pollut. R. 24, 3400–3411. doi: 10.1007/s11356-016-7979-0
Sofo, A., Elshafifie, H. S., Scopa, A., Mang, S. M., and Camele, I. (2018). Impact of airborne zinc pollution on the antimicrobial activity of olive oil and the microbial metabolic profiles of Zn-contaminated soils in an Italian olive orchard. J. Trace Elem. Med. Biol. 49, 276–284. doi: 10.1016/j.jtemb.2018.02.017
Tafazoli, M., Hojjati, S. M., Jalilvand, H., and Lamersdorf, N. (2019). Simulated nitrogen deposition reduces the concentration of soil base cations in Acer velutinum Bioss. plantation, North of Iran. J. Soil Sci. Plant Nut. 19, 440–449. doi: 10.1007/s42729-019-00048-5
Tipayno, S. C., Truu, J., Samaddar, S., Truu, M., Preem, J., Oopkaup, K., et al. (2018). The bacterial community structure and functional profile in the heavy metal contaminated paddy soils, surrounding a nonferrous smelter in South Korea. Isr. J. Ecol. Evol. 8, 6157–6168. doi: 10.1002/ece3.4170
Venegas, A., Rigol, A., and Vidal, M. (2015). Viability of organic wastes and biochars as amendments for the remediation of heavy metal contaminated soils. Chemosphere 119, 190–198. doi: 10.1016/j.chemosphere.2014.06.009
Venegas, A., Rigol, A., and Vidal, M. (2016). Changes in heavy metal extractability from contaminated soils remediated with organic waste or biochar. Geoderma 279, 132–140. doi: 10.1016/j.geoderma.2016.06.010
Wan, Y., Huang, Q., Wang, Q., Yao, Y., Su, D. C., Qiao, Y. H., et al. (2020). Accumulation and bioavailability of heavy metals in an acid soil and their uptake by paddy rice under continuous application of chicken and swine manure. J. Hazard. Mater. 384:121293. doi: 10.1016/j.jhazmat.2019.121293
Wang, R. L., Chen, S., Qin, Z., Feng, Z. L., Xia, W. N., Su, Y. J., et al. (2016). Effects of simulated nitrogen deposition on the seeding growth, photosynthetic characteristics and allelopathic potential of invasive plant Ipomoea cairica sweet. Allelopathy J. 38, 183–192.
Wang, M., Chen, S. B., Zheng, H., Li, S. S., Chen, L., and Wang, D. (2020). The responses of cadmium phytotoxicity in rice and the microbial community in contaminated paddy soils for the application of different long-term N fertilizers. Chemosphere 238:124700. doi: 10.1016/j.chemosphere.2019.124700
Wang, H. H., Chu, H. L., Dou, Q., Feng, H., Tang, M., Zhang, S. X., et al. (2021). Seasonal changes in Pinus tabuliformis root-associated fungal microbiota drive N and P cycling in terrestrial ecosystem. Front. Microbiol. 11:526898. doi: 10.3389/fmicb.2020.526898
Wang, Y. P., Li, Q. B., Wang, H., Shi, J. Y., Lin, Q., Chen, X. C., et al. (2008). Effect of sulfur on soil Cu/Zn availability and microbial community composition. J. Hazard. Mater. 159, 385–389. doi: 10.1016/j.jhazmat.2008.02.029
Wang, C., Liu, D. W., and Bai, E. (2018a). Decreasing soil microbial diversity is associated with decreasing microbial biomass under nitrogen addition. Soil Biol. Biochem., 120, 126–133. doi: 10.1016/j.soilbio.02.003
Wang, R. Q., Xiao, Y. P., Lv, F. J., Hu, L. Y., Wei, L. G., Yuan, Z. Q., et al. (2018b). Bacterial community structure and functional potential of rhizosphere soils as influenced by nitrogen addition and bacterial wilt disease under continuous sesame cropping. Appl. Soil Ecol. 125, 117–127. doi: 10.1016/j.apsoil.2017.12.014
Wang, J. J., Yi, X., Cui, J., Chang, Y. J., Yao, D. R., Zhou, D. M., et al. (2019). Onlinear effects of increasing nitrogen deposition on rice growth and heavy metal uptake in a red soil ecosystem of southeastern China. Sci. Total Environ. 670, 1060–1067. doi: 10.1016/j.scitotenv.2019.03.245
Wen, Y. C., Li, H. Y., Lin, Z. A., Zhao, B. Q., Sun, Z. B., Yuan, L., et al. (2020). Long-term fertilization alters soil properties and fungal community composition in fluvo-aquic soil of the North China Plain. Sci. Rep. 10:7198. doi: 10.1038/s41598-020-64227-6
Xie, Y., Fan, J. B., Zhu, W. X., Amombo, E., Lou, Y. H., Chen, L., et al. (2016). Effect of heavy metals pollution on soil microbial diversity and bermudagrass genetic variation. Front. Plant Sci. 7:755. doi: 10.3389/fpls.2016.00755
Xu, J., Liu, S. J., Song, S. R., Guo, H. L., Tang, J. J., Yong, J. W. H., et al. (2018). Arbuscular mycorrhizal fungi influence decomposition and the associated soil microbial community under different soil phosphorus availability. Soil Biol. Biochem. 120, 181–190. doi: 10.1016/j.soilbio.2018.02.010
Xu, R., Sun, X. X., Häggblom, M. M., Dong, Y. R., Zhang, M. M., Yang, Z. H., et al. (2021). Metabolic potentials of members of the class Acidobacteriia in metal-contaminated soils revealed by metagenomic analysis. Environ. Microbiol. 24, 803–818. doi: 10.1111/1462-2920.15612
Xun, Y., Zhang, X. J., Chaoliang, C., Luo, X. G., and Zhang, Y. (2018). Comprehensive evaluation of soil near uranium tailings, Beishan City. China. Bull. Environ. Contam. Toxicol. 100, 843–848. doi: 10.1007/s00128-018-2330-8
Yang, W. H., Wang, S. S., Ni, W. Z., Rensing, C., and Xing, S. H. (2019). Enhanced cd-Zn-Pb-contaminated soil phytoextraction by Sedum alfredii and the rhizosphere bacterial community structure and function by applying organic amendments. Plant Soil 444, 101–118. doi: 10.1007/s11104-019-04256-x
Yang, W. D., Wang, Y. Y., Zhao, F. L., Ding, Z. L., Zhang, X. C., Zhu, Z. Q., et al. (2014). Variation in copper and zinc tolerance and accumulation in 12 willow clones: implications for phytoextraction. J. Zhejiang Univ. Sci. 15, 788–800. doi: 10.1631/jzus.B1400029
Yang, W. D., Zhao, F. L., Ding, Z. L., Wang, Y. Y., Zhang, X. C., Zhu, Z. Q., et al. (2018). Variation of tolerance and accumulation to excess iron in 24 willow clones: implications for phytoextraction. Int. J. Phytoremediation 20, 1284–1291. doi: 10.1080/15226514.2014.922927
Zhang, C., Nie, S., Liang, J., Zeng, G. M., Wu, H. P., Hua, S. S., et al. (2016). Effects of heavy metals and soil physical chemical properties on wetland soil microbial biomass and bacterial community structure. Sci. Total Environ. 557–558, 785–790. doi: 10.1016/j.scitotenv.2016.01.170
Zhang, Z. H., Zhou, T., Tang, T. J., Song, H. X., Guan, C. Y., Huang, J. Y., et al. (2019). A multiomics approach reveals the pivotal role of subcellular reallocation in determining rapeseed resistance to cadmium toxicity. J. Exp. Bot. 70, 5437–5455. doi: 10.1093/jxb/erz295
Zhao, Z., Shi, H. J., Liu, C. Q., Kang, X. J., Chen, L. C., Liang, X. F., et al. (2018). Duckweed diversity decreases heavy metal toxicity by altering the metabolic function of associated microbial communities. Chemosphere 203, 76–82. doi: 10.1016/j.chemosphere.2018.03.175
Zheng, Q., Hu, Y. T., Zhang, S. S., Noll, L., Böckle, T., Dietrich, M., et al. (2019). Soil multifunctionality is affected by the soil environment and by microbial community composition and diversity. Soil Biol. Biochem. 136:107521. doi: 10.1016/j.soilbio.2019.107521
Zhou, S. W., Liu, J., Xu, M. G., Lv, J. L., and Sun, N. (2015). Accumulation, availability, and uptake of heavy metals in a red soil after 22-year fertilization and cropping. Environ. Sci. Pollut. R. 22, 15154–15163. doi: 10.1007/s11356-015-4745-7
Keywords: phytoremediation, fertilization, rhizosphere, microbial metabolic activity, microbial community structure
Citation: Wang S, Niu X, Di D and Huang D (2022) Nitrogen and sulfur fertilizers promote the absorption of lead and cadmium with Salix integra Thunb. by increasing the bioavailability of heavy metals and regulating rhizosphere microbes. Front. Microbiol. 13:945847. doi: 10.3389/fmicb.2022.945847
Edited by:
Ajar Nath Yadav, Eternal University, IndiaReviewed by:
Xiangwei Gong, Shenyang Agricultural University, ChinaAke Liu, Changzhi University, China
My Dung Jusselme, Université Paris-Est Créteil Val de Marne, France
Copyright © 2022 Wang, Niu, Di and Huang. This is an open-access article distributed under the terms of the Creative Commons Attribution License (CC BY). The use, distribution or reproduction in other forums is permitted, provided the original author(s) and the copyright owner(s) are credited and that the original publication in this journal is cited, in accordance with accepted academic practice. No use, distribution or reproduction is permitted which does not comply with these terms.
*Correspondence: Xiaoyun Niu, bnh5ODUwMTAxQDE2My5jb20=; Dazhuang Huang, aHVhbmdkYXpodWFuZ0AxMjYuY29t
†These authors have contributed equally to this work