- 1Agroécologie, INRAE, Institut Agro Dijon, Université Bourgogne, Université Bourgogne Franche-Comté, Dijon, France
- 2CIRAD, UPR Recyclage et Risque, Montpellier, France
- 3Recyclage et risque, Univ Montpellier, CIRAD, Montpellier, France
The soil microbial community plays important roles in nutrient cycling, plant pathogen suppression, decomposition of residues and degradation of pollutants; as such, it is often regarded as a good indicator of soil quality. Repeated applications of mixed organic and inorganic materials in agriculture improve the soil microbial quality and in turn crop productivity. The soil microbial quality following several years of repeated fertilizer inputs has received considerable attention, but the dynamic of this community over time has never been assessed. We used high-throughput sequencing targeting 16S ribosomal RNA genes to investigate the evolution of the bacterial and archaeal community throughout 6 years of repeated organic and inorganic fertilizer applications. Soils were sampled from a field experiment in La Mare (Reunion Island, France), where different mixed organic-inorganic fertilizer inputs characterized by more or less stable organic matter were applied regularly for 6 years. Soil samples were taken each year, more than 6 months after the latest fertilizer application. The soil molecular biomass significantly increased in some organically fertilized plots (by 35–45% on average), 3–5 years after the first fertilizers application. The significant variations in soil molecular microbial biomass were explained by the fertilization practices (cumulated organic carbon inputs) and sometimes by the soil parameters (sand and soil carbon contents). The structure of the bacterial and archaeal community was more influenced by time than by the fertilization type. However, repeated fertilizer applications over time tended to modify the abundance of the bacterial phyla Acidobacteria, Actinobacteria, Bacteroidetes, Firmicutes, and Proteobacteria. To conclude, the present study highlights that the soil bacterial and archaeal community is lastingly modified after 6 years of repeated fertilizer inputs. These changes depend on the nature of the organic input and on the fertilization practice (frequency and applied quantity).
Introduction
To meet the global demand for food production, fertilization is a common/basic farming practice usually repeated every year to maintain soil fertility and crop yields. In parallel, intensive agricultural practices contribute to decrease the soil organic matter content, with negative consequences on soil fertility (Clapp et al., 1986; Tate, 1987). One way of reversing this degradation is to increase the soil organic matter content by applying organic fertilizers such as manures, composts, or crop residues (Hutchinson et al., 2007; Peltre et al., 2012). In the field, organic fertilizers are often used in association with inorganic fertilizers to improve crop productivity. However, assessing the effect of these practices on soil quality is important to reach a sustainable management of agriculture. Soil quality was defined as “the capacity of a soil to function within ecosystem and land-use boundaries to sustain biological productivity, maintain environmental quality, and promote plant and animal health” by Doran and Parkin (1994, 1997). As underlined by Bünemann et al. (2018), biochemical and biological indicators are potentially highly valuable tools for assessing soil quality. The most frequently used biochemical indicators are soil organic matter/carbon and the pH (Bünemann et al., 2018). As for soil biological indicators, microorganisms are essential for soil quality because they play a key role in soil functioning (Vivant et al., 2013; Tardy et al., 2014; Maron et al., 2018; Abis et al., 2020). The most frequently used microbiological indicator is microbial biomass (Bünemann et al., 2018). However, recent advances in soil biology—more precisely molecular methods based on DNA—provide access to and describe a larger part of the soil microbiota. Thus, other indicators focused on the soil microbial diversity—e.g., taxonomic richness or community composition—are now used to assess the effect of agricultural practices on soil quality (Tardy et al., 2015; Chemidlin Prévost-Bouré et al., 2021; Dunn et al., 2021).
Several studies have compared the impact of repeated organic or inorganic fertilization on soil biochemical and biological indicators. There is no consensus in the literature about soil biochemical indicators. Some studies highlight no difference in the pH of unfertilized and organically/inorganically fertilized soils (Gu et al., 2009; Ho et al., 2015; Chen et al., 2016; Li et al., 2017; van der Bom et al., 2018; Guo et al., 2019; Diallo et al., 2021). Other works show an impact of fertilization practices: sometimes the soil pH decreases whatever the fertilization type (Li et al., 2015; Sun et al., 2015; Francioli et al., 2016), and sometimes it increases under organic or combined inorganic-organic fertilization (Zhong et al., 2010; Dong et al., 2014; Hartmann et al., 2015; Ding et al., 2016; Zhao et al., 2016; Wang et al., 2017; Feder, 2021a,b). As regards the soil organic carbon content, an increase is overall observed whatever the fertilization type considered (organic or inorganic, or a combination of both) (Hao et al., 2008; Zhong et al., 2010; Chakraborty et al., 2011; Dong et al., 2014; Zhang et al., 2014; Hartmann et al., 2015; Li et al., 2015, 2017; Sun et al., 2015; Francioli et al., 2016; Kumar et al., 2017; Wang et al., 2017; Guo et al., 2019). However, some of these studies also highlight a greatest increase of the soil organic carbon content when organic material is used (alone or combined with an inorganic material) compared with inorganic fertilization (Hao et al., 2008; Zhang et al., 2014; Hartmann et al., 2015; Li et al., 2015; Sun et al., 2015; Francioli et al., 2016; Wang et al., 2017; Guo et al., 2019). This suggests that fertilization practices including organic material are a better way to improve soil fertility, organic matter content (Mäder et al., 2002; Liang et al., 2012), and consequently to enhance soil microbial community (Esperschütz et al., 2007; Gomiero et al., 2011).
The literature is more consensual about soil biological indicators. Most studies show an increase of the soil microbial biomass after repeated organic or inorganic fertilization, or a combination of the two fertilization types (Stark et al., 2007; Hao et al., 2008; Gu et al., 2009; Liu et al., 2009; Chakraborty et al., 2011; Zhang et al., 2012; Li et al., 2015; Francioli et al., 2016; Kumar et al., 2017; Guo et al., 2019). The soil microbial biomass increases more when organic material is used in addition to inorganic fertilizer (Hao et al., 2008; Chakraborty et al., 2011; Zhang et al., 2012; Francioli et al., 2016; Kumar et al., 2017; Guo et al., 2019). Most studies also highlight that organic inputs (alone or combined with mineral fertilizers) increase the soil microbial diversity compared with sole mineral fertilizer applications (Zhong et al., 2010; Kamaa et al., 2011; Zhang et al., 2012; Chen et al., 2016; Francioli et al., 2016). Organic fertilization also modifies the soil microbial community composition by stimulating microbial groups (Firmicutes and Proteobacteria) known to prefer nutrient-rich environments and involved in the degradation of complex organic compounds (Hartmann et al., 2015; Francioli et al., 2016; Wang et al., 2017; van der Bom et al., 2018). Interestingly, among the soil microbes involved in soil functioning, bacteria appear more sensitive than fungi to fertilization practices (Ai et al., 2018). This suggests that the bacterial community is a good indicator to assess the effect of these practices on the soil (Kamaa et al., 2011; Chen et al., 2016; Francioli et al., 2016).
The different literature results about soil biochemical indicators could be explained by different experimental conditions, i.e., the duration of repeated fertilization, the rate of fertilizer applications, the time lapse between fertilizer application and soil sampling, the quality of the different organic inputs, and the soil type. Nevertheless, despite these differences, one consensual observation is that repeated application of organic material mixed with inorganic material improves the soil microbial quality by increasing soil microbial biomass and diversity. To our knowledge, the majority of studies assessed soil quality following several years of repeated fertilizer inputs, but did not address the dynamic of the soil community over the years. The dynamic of the soil microbial community has been assessed over several months after a single organic fertilizer application (Calbrix et al., 2007; Bastida et al., 2008). These works reveal that organic fertilization initially induced strong changes in bacterial functional and genetic structures during the first 3 months after organic fertilization, followed by a period of resilience leading to similar communities in both the fertilized and control plots after 6 months (Calbrix et al., 2007). However, this transient effect of a single dose of organic fertilizer (3–6 Mg ha–1; Calbrix et al. (2007)) on the soil microbial community seems dependent on the applied quantity; since a persistent effect has been observed with 195 Mg ha–1 of organic material (Bastida et al., 2013). Therefore, determining when the soil microbial communities are persistently modified in response to repeated fertilizer inputs (type and quantity) is important to improve fertilization practices.
The present study takes advantage of a mid-term field experiment in La Mare, Reunion Island (France). The site was laid out for the experiment in 2013, and different mixed organic/inorganic fertilizer inputs were applied from March 2014. Soil samples were generally collected 1 year after the organic-inorganic material application every year for 6 years to monitor microbial community changes. The experiment involved different types of organic inputs and fertilization practices. The responses of the bacterial and archaeal community to organic-inorganic inputs were assessed by high-throughput sequencing targeting 16S ribosomal genes. Based on previous works, we hypothesized that the soil microbial community indicators (microbial biomass, diversity and composition) would not increase or change in the first years of fertilizer inputs, and that lasting modifications would occur several years after repeated fertilizer inputs. The time needed for this modification to occur is not documented in currently available literature. We also hypothesized that this interval would be dependent on the fertilization practice (frequency of application, quality and quantity of organic material inputs).
Materials and methods
Experimental sites, soil sampling strategy, and soil chemical analysis
The experiment was conducted at the SOERE PRO1 experimental station of La Mare (Sainte-Marie), Reunion Island (20°54’12.2”S 55°31’46.6”E). The soil is a Nitisol (Feder, 2013; WRB, 2015) containing 42% clay, 47% silt, and 11% sand on average in its tilled layer. The climate is tropical with a mean rainfall of 2,000 mm year–1 and a mean annual temperature of 25°C. The trial was planted in March 2014 from viable buds of the R579 sugarcane variety with 1.5 m spacing between rows. Four different organic materials representing the typical organic fertilizers found in Reunion Island were applied: (1) liquid pig manure (LP); (2) poultry litter (PL); and (3) two similar sewage sludge materials (SS1 and SS2). These organic treatments were compared with a control treatment that did not receive any organic input (CON). Each treatment was replicated three times within a randomized complete block design. Each plot was composed of 6 sugarcane rows 28 m in length, making up a total plot area of 250 m2. Additionally, all plots received mineral fertilization adjusted to annual sugarcane needs (Supplementary Table 1). SS1 and LP were applied every year, in February and November 2014, November 2015 and 2016, October 2017, 2018, and 2019 (Supplementary Table 1). SS2 and PL were applied every 3 years, in February 2014 and October 2017 (Supplementary Table 1). The amounts of organic inputs are given in Supplementary Table 1. The nature and doses of organic fertilizers and the associated mineral supplementation are representative of the local context in Reunion Island. The requirements for sugar cane to reach a yield of ca. 120 T/ha are 180, 60, and 230 kg/ha of N, P, and K, respectively; these values are similar to recommendations in other situations (Leal et al., 2009; Blum et al., 2013; Ghube et al., 2017). The sugarcane yields are given in Supplementary Table 2. Each plot was equipped with a TDR CS 650 probe to measure the moisture level every hour at the following depths: 10, 30, 50, 70, 90, and 110 cm. Irrigation was adjusted according to rainfall so that the soil never underwent an intense or long dry period. A description of the pesticides treatments is given in Supplementary Table 3. We conducted the initial soil characterization of the site in November 2013, after plowing the plot. In March 2014, sugarcane cuttings were buried and there was no further tillage thereafter. The following years, soils samples were collected just after the sugar cane harvest and before the organic fertilizer applications in November 2013, 2014, 2015, and 2016, and in October 2017, 2018, and 2019 on bulk soils. In each plot, 5 soil cores (Ø 7 cm) were randomly taken at 0–25 cm depth, mixed and homogenized by 4 mm mesh sieving, so that above-ground plant debris, roots and stones were also removed. This led to a total of 105 soil samples (5 treatments × 3 replicates × 7 years). A portion of each soil was air-dried for physico-chemical analysis: particle size distribution, pH, soil organic carbon, soil total nitrogen (N), soil C/N ratio, total phosphorus (P), and Cation Exchange Capacity (CEC). These analyses were performed at the COFRAC-accredited INRAE Soil Analysis Laboratory (ARRAS, France).2 The mean soil physico-chemical data measured between 2013 and 2018 are given in Table 1. The rest of the sieved soil was lyophilized and stored at −40°C prior to DNA extraction and molecular analyses.
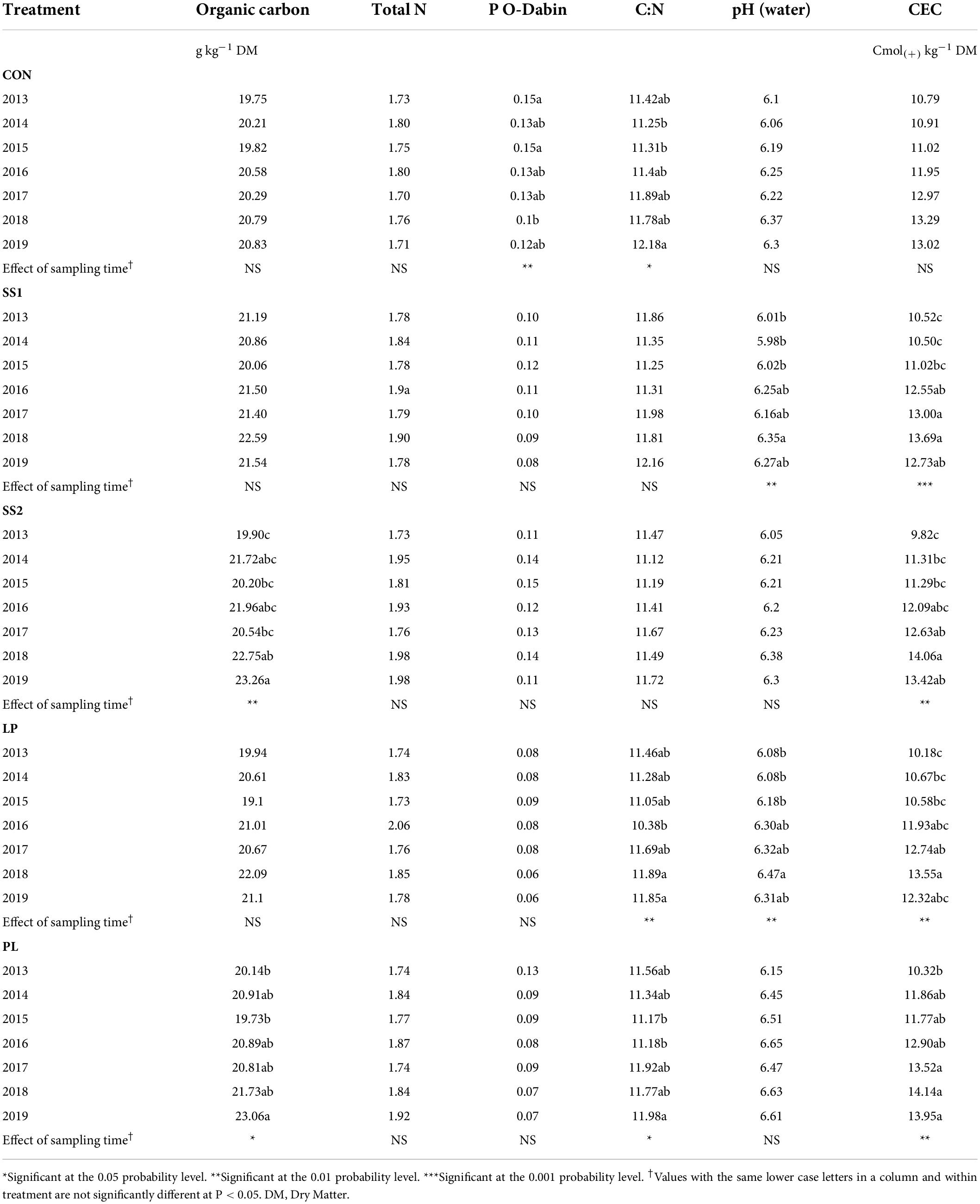
Table 1. Physico-chemical parameters of soils from La Mare (Reunion Island) according to the fertilizer input (CON, inorganic fertilized control; SS1 and SS2, sewage sludge inputs; LP, liquid pig manure; PL, poultry litter) and the sampling time.
Chemical characteristics of organic fertilizers
Each organic material was dried and ground to 1 mm to measure the pH, organic C, total N, and P. The total N and organic C contents were measured by elemental analysis (NA 1500, Fison Instrument, San Carlos, CA, United States) after additional grinding to 200 μm (Retsch SM 2000, Haan, Germany). The mean characteristics of the organic fertilizers measured between 2014 and 2019 are given in Supplementary Table 1. The organic fertilizers were representative of the most common recycled waste in agriculture, particularly in Reunion Island. They were analyzed before each application. Considering all sampling times, both sewage sludge materials (SS1 and SS2) were characterized by a high dry matter content (91.6 and 79.1% on average, respectively), a high pH (11.8 and 9.4) and a low K concentration (1.7 and 3.9 g kg–1 DM). Liquid pig manure (LP) had a low dry matter content (3.7% of DM on average), a low pH (4.1) and a high K concentration (99.7 g kg–1 DM). Poultry litter (PL) had a low P concentration (14.6 g kg–1 DM on average) and a high organic carbon content (410.2 g kg–1 DM).
Total DNA was extracted from 1 g (dry weight) of soil using a single procedure standardized by the GenoSol platform (INRAE, Dijon, France)3 (Terrat et al., 2012). A highly positive linear relationship exists between soil DNA recovery and C biomass measurements (Ranjard et al., 2003), so the DNA concentrations of the crude extracts were determined by electrophoresis in 1% agarose gel stained with ethidium bromide using a calf thymus DNA standard curve, and used as estimates of microbial biomass (Dequiedt et al., 2011). After quantification, 100 μL of crude DNA extract were separated from the residual impurities, particularly humic substances, using the purification steps of the Nucleospin Soil kit (Macherey-Nagel GmbH & Co., KG, Düren, Germany). Purified DNA concentrations were finally measured using the Quantifluor (Promega, Lyon, France) staining kit, according to the manufacturer’s instructions.
High throughput sequencing of 16S rRNA gene sequences
For bacterial and archaeal diversity, a 440-base 16S rRNA fragment was amplified from each DNA sample (5 ng) with the corresponding primers: F479 (5′-CAG CMG CYG CNG TAA NAC-3′) and R888 (5′-CCG YCA ATT CMT TTR AGT-3′) as previously described (Tardy et al., 2014). For each sample, 5 ng of DNA were used for a 25 μL PCR conducted under the following conditions: 94°C for 2 min, 35 cycles of 30 s at 94°C, 52°C for 30 s, and 72°C for 1 min, followed by 7 min at 72°C.
All PCR products were purified using the Agencourt® AMPure® XP kit (Beckman Coulter, Italy, Milano) and quantified with the Quantifluor (Promega, Lyon, France) staining kit according to the manufacturer’s instructions.
A second PCR was performed with the purified PCR products (7.5 ng of DNA for bacteria and archaea), with 10-bp multiplex identifiers added to the 5’ end of the primers for the specific identification of each sample and the prevention of PCR biases. The second PCR conditions were the same than previously described but with only seven cycles. PCR products were purified with the MinElute PCR purification kit (Qiagen NV) and quantified with the Quantifluor (Promega, Lyon, France) staining kit according to the manufacturer’s instructions. Equal amounts of each sample were pooled and then cleaned with the SPRI (Solid Phase Reverse Immobilization Method) using the Agencourt® AMPure® XP kit (Beckman Coulter, Italy, Milano). The pool was finally sequenced with a MiSeq Illumina instrument (Illumina Inc., San Diego, CA) operating with V3 chemistry and producing 250 bp paired-reads (GenoScreen).
Bioinformatic analysis of 16S rRNA gene sequences
Bioinformatic analyses were performed using BIOCOM-PIPE v.20 (Djemiel et al., 2020). First, all the 16S rRNA raw reads were sorted according to the multiplex identifier sequences. Next, all raw sequences were checked and discarded if (i) they contained an ambiguous base (Ns), (ii) their length was less than 350 nucleotides, and (iii) the exact primer sequences were not found. Then, we applied a rigorous dereplication step (i.e., a clustering of strictly identical sequences). The dereplicated reads were aligned using Infernal tool (Cole et al., 2009), and clustered into operational taxonomic units (OTUs) using a similarity threshold of 95%. The threshold at 95% is more adapted for the targeted amplicon (Djemiel et al., 2020; Terrat et al., 2020). A filtering step was carried out to remove chimeras based on the quality of their taxonomic assignments. Finally, the retained reads were homogenized by random selection (10,000 reads for 16S rRNA gene sequences) to compare the datasets efficiently and avoid biased community comparisons. More detailed information about sequencing data quality is given in Supplementary Figure 1.
The retained high-quality reads were used for taxonomy-independent analyses, to determine alpha-diversity metrics (e.g., richness), and taxonomy-based analysis is performed using USEARCH (Edgar, 2010) against the SILVA 16S rRNA reference database (r132) (Quast et al., 2012). The raw datasets are available in the EBI database system under project accession number PRJEB52689.
Statistical analyses
The soil physico-chemical characteristics and the fertilization practices, a.k.a. explanatory variables potentially involved in the variations of soil microbial indices were first tested for autocorrelation. Explanatory variables with | r| > 0.6 were excluded from further analyses, and the following soil variables were retained: organic carbon content, C/N ratio, pH, P, clay, and sand, together with the following fertilization variables: organic carbon inputs, mineral nitrogen inputs, mineral phosphorous inputs, organic potassium inputs, and cumulated organic carbon inputs (Cumul_Corg). These variables were used to evaluate whether the soil characteristics and the fertilization practices significantly discriminated the different treatments over time. For this purpose, a principal component analysis was performed using the dudi.pca function of the ade4 package in R (Chessel et al., 2004). Discrimination between treatments, years or treatments x years was tested using a Monte Carlo permutation test (1,000 permutations, bca and randtest function, ade4 package). The significance threshold was set at P < 0.001.
Alpha diversity was analyzed using Hill numbers generated by vegan package (Chao et al., 2010; Oksanen et al., 2013). The Hill numbers make it possible to interpret alpha diversity in a linear way by progressively taking into account the abundance of OTUs (q-value), between rare and dominant OTUs. Thus, q = 0 represents richness, q = 1 the exponential of Shannon entropy and q = 2 the inverse Simpson index.
The variations of the soil microbial biomass and diversity indices (Hill indices q0, q1, and q2) over time were tested for each organic material treatment by means of a Kruskal-Wallis test with Bonferroni correction. The significance threshold was set at P < 0.05. For each soil microbial index exhibiting significant differences over time within an organic material treatment, a variance partitioning approach was used to decipher which soil physico-chemical characteristics and fertilization practices were involved. For this purpose, the most parsimonious additive model was selected from null to full model according to a forward selection procedure by minimizing the Akaike information criterion (rda and ordistep functions, vegan package). Once the model was selected, the variance inflation factor was checked (vif.cca function, vegan package) (Oksanen et al., 2013). Variables with vif > 4 were excluded, and the selection procedure was run a second time.
The composition of the bacterial and archaeal communities associated with each treatment and sampling time were compared using Non-Metric multiDimensional Scaling approach (NMDS). The relative abundances of bacterial and archaeal phyla were determined, and then a Bray-Curtis dissimilarity matrix was computed. Then, NMDS was applied using the metaMDS function in the vegan package (Oksanen et al., 2013; 1,000 permutations). Individuals were plotted in the NMDS space according to NMDS1 and NMDS2 axes, which depicted the differences in the bacterial and archaeal community compositions. The significance of the differences between treatments and sampling times were assessed using a PERMANOVA approach was used to test for the relative effect of organic material treatment, time and organic material treatment x time (adonis2 function, vegan package; 1,000 permutations, significance threshold: P < 0.001). To better understand the sources of variations of the composition of bacterial and archaeal communities, phylum, soil physico-chemical properties and fertilization practices data were fitted in the NMDS space using the envfit function (vegan package; 1,000 permutations). Only the variables with P < 0.001 were retained and plotted in the NMDS space. To better understand these variations, a linear discriminant analysis effect size (LEfSe) was used to detect the taxa with significant differential abundances among the soil fertilization types for all years (Segata et al., 2011). Briefly, the LEfSe method is based on a first step using the non-parametric Kruskal-Wallis rank sum test to identify the statistically different bacterial groups, followed by a linear discriminant analysis step (LDA scores > 3) to evaluate the effect size of each differentially abundant taxon. The cladogram represented the taxa with different abundance among the different groups. To better characterize these biomarkers, major phyla and genera were selected with LDA scores higher than 4 and their relative abundance was used to evaluate the responses of the bacterial and archaeal community to organic-inorganic inputs over time.
All statistical analyses were carried out with R studio (RStudio, Version 1.4.1717, RStudio Inc., Boston, Massachusetts, United States) using R software (R version 4.1.1).
Results
Soil physico-chemical characteristics
The Principal Component Analysis (PCA) of the soil physico-chemical characteristics and fertilization practices highlighted that the soil samples were discriminated according to treatments and years (Figure 1; Monte Carlo permutation test: P < 0.001). Discrimination between treatments was mainly explained by fertilization practices: organic inputs (C and K) vs. mineral input (N), whereas inter-annual variations were mainly explained by the soil physico-chemical characteristics and the cumulated organic C inputs (Figure 1). More precisely, as described in Table 1, the soil organic carbon significantly increased in SS2 and PL plots (by 15% on average), 5 and 6 years after the start of the experiment, respectively (P < 0.05, Table 1). The total N content remained stable over time, irrespective of the treatment (P > 0.05; Table 1). The soil pH increased significantly (by 6% on average) only in SS1 and LP plots, 5 years after the start of the experiment (P < 0.05, Table 1). CEC significantly increased in all organically fertilized plots (by 37% on average) 3–4 years after the start of the experiment (P < 0.01; Table 1).
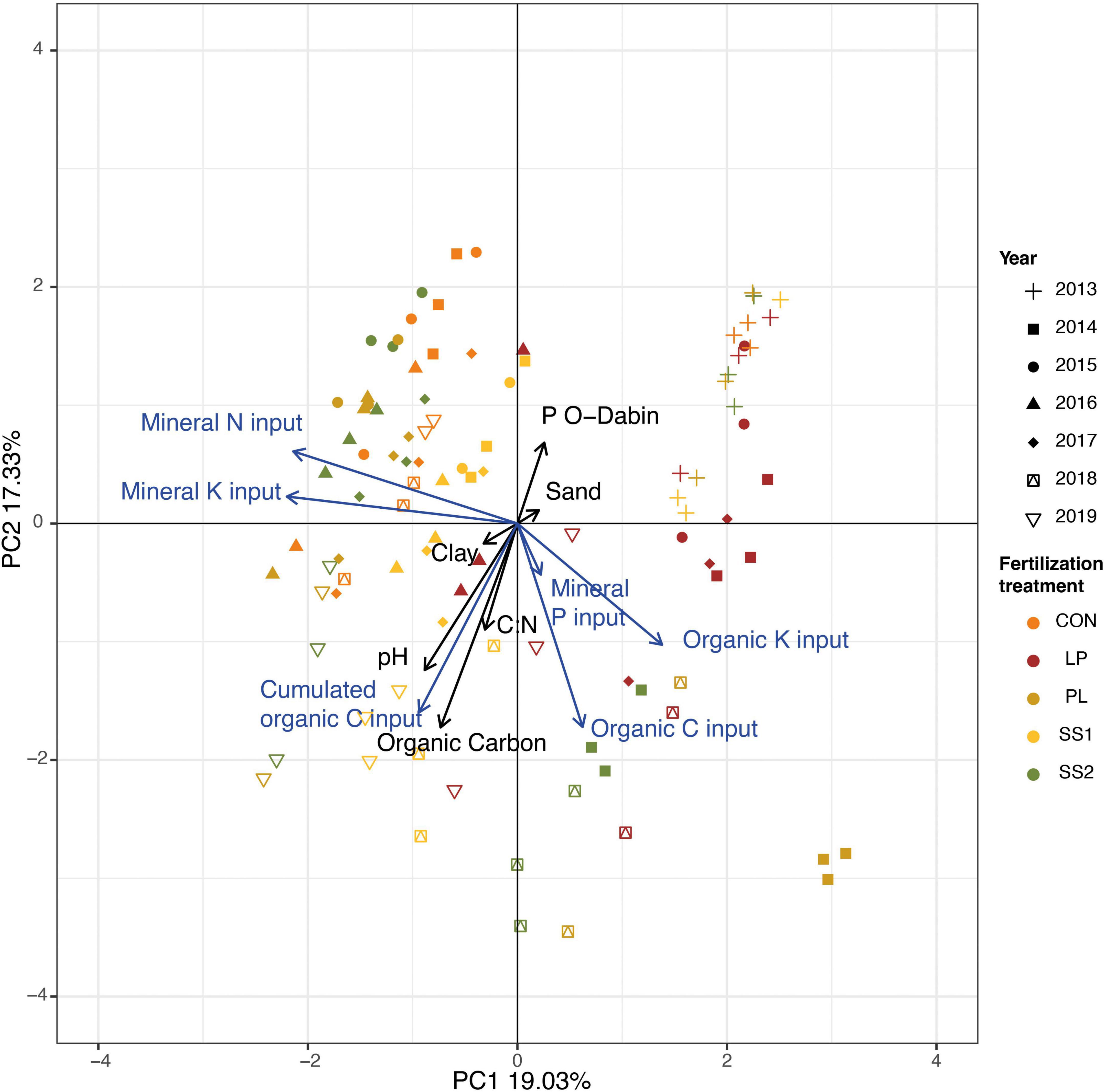
Figure 1. Principal component analysis of the soil physico-chemical characteristics and fertilization practices in the experimental site of La Mare (Reunion Island) fertilized solely with inorganic fertilizer (control, CON), or fertilized with four different organic materials (SS1 and SS2, sewage sludge inputs; LP, liquid pig manure; PL, poultry litter). Discrimination between treatments, years or treatments x years was tested using a Monte Carlo permutation test (1,000 permutations). The black arrows are the soil physico-chemical characteristics, and the blue are the fertilizers physico-chemical characteristics.
Effects of organic fertilization on the microbial community
The estimated soil molecular biomass did not change over time in the control and SS2 plots (P > 0.05, Figure 2), but significantly increased in the other organically fertilized plots (SS1, LP, and PL) (by 35–45% on average) 3, 4, and 5 years after the first fertilizer application, respectively (P < 0.01; Figure 2). In the SS1 and LP treatments, soil microbial biomass variations were mainly related to fertilization practices. Cumul_Corg represented 55 and 59% of the explained variance of the soil microbial biomass in the SS1 and LP plots, respectively (Table 2). Soil parameters were also important: the sand and soil carbon contents represented 23 and 41% of the explained variance in the SS1 and PL plots, respectively (Table 2).
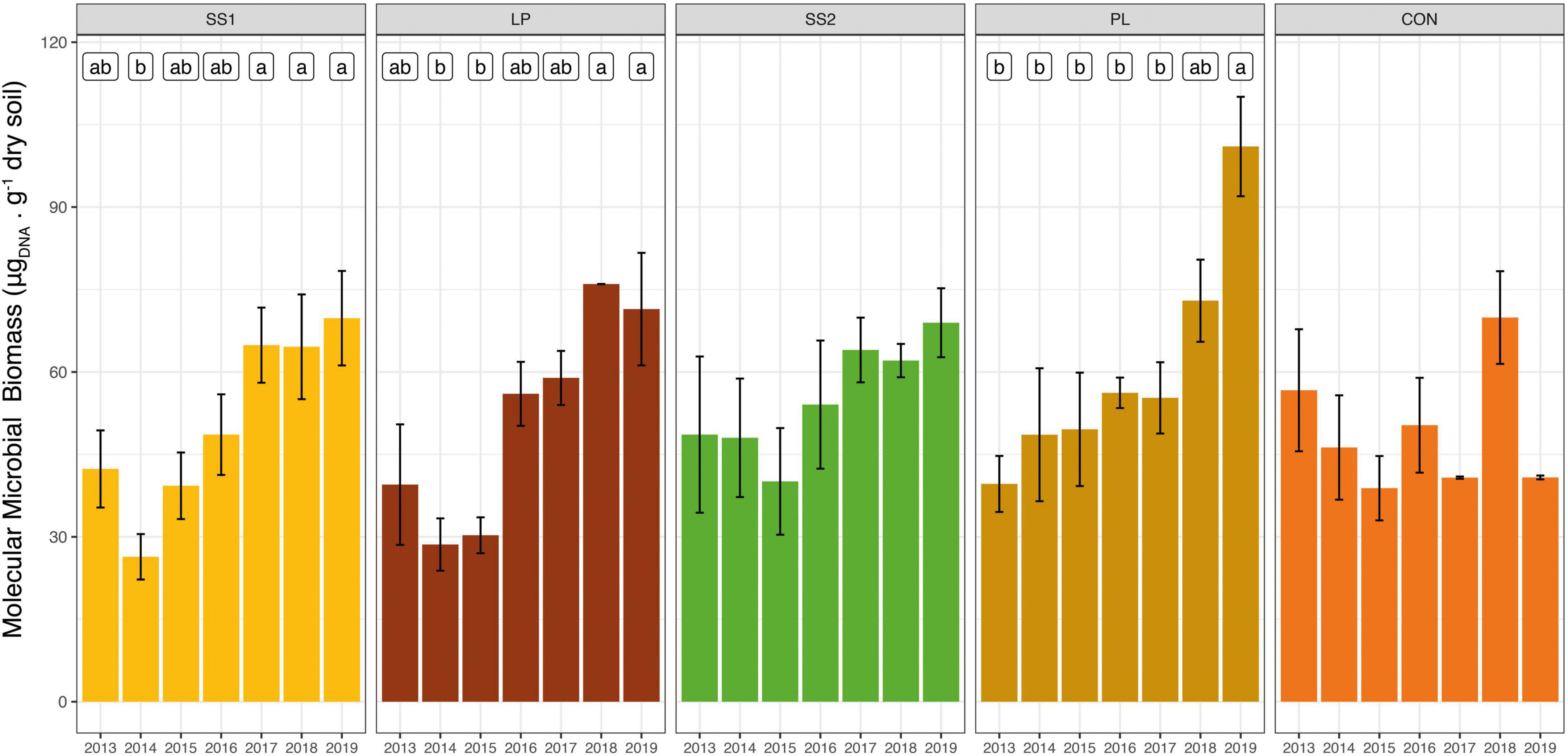
Figure 2. Dynamic of molecular microbial biomass in soil from La Mare (Reunion Island) fertilized or not (inorganic fertilizer control, CON) with four different organic materials (SS1 and SS2, sewage sludge inputs; LP, liquid pig manure; PL, poultry litter). Different letters indicate significant differences based on Kruskal-Wallis test with Bonferroni correction (P < 0.05).
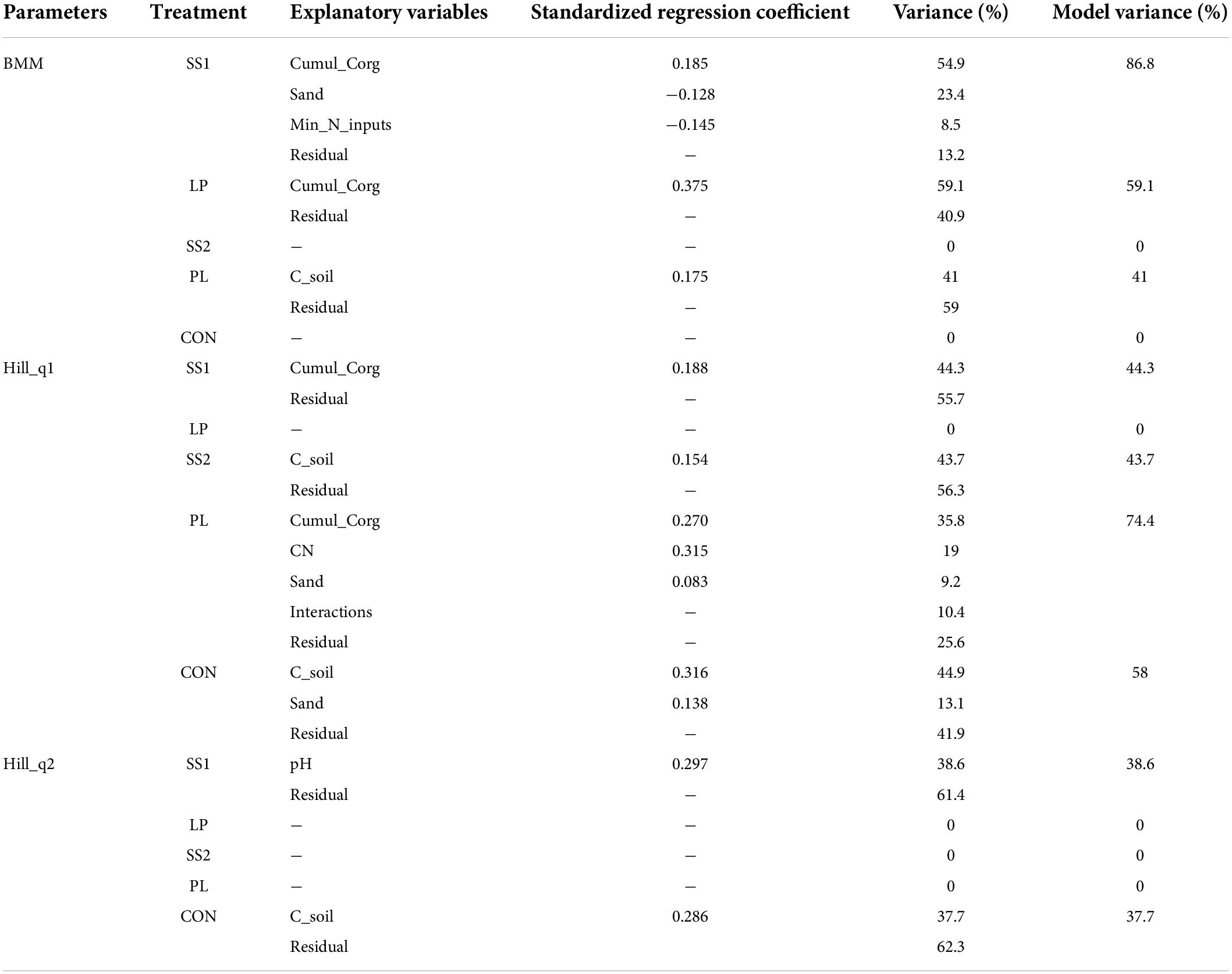
Table 2. Effects of environmental conditions on the soil molecular microbial biomass (BMM) and the bacterial—archaeal diversity indices (Hill number with an order 1 and 2) of soils from La Mare (Reunion Island) according to the fertilizer input (CON, inorganic fertilized control; SS1 and SS2, sewage sludge inputs; LP, liquid pig manure; PL, poultry litter).
The dynamic of the bacterial and archaeal diversity indices throughout the experiment is showed in Figure 3. Irrespective of the treatment, the soil bacterial and archaeal Hill number with an order 0 (q = 0, aka H0) diversity index did not change over time (P > 0.05, Figure 3). The Hill number with an order 1 (q = 1, aka H1) and 2 (q = 2, aka H2) indices in general increased in the SS1 and control plots 4 years after the first fertilizer application (P < 0.001; Figure 3). In plots SS1, H1 index variations were mainly related to fertilization practices. Cumul_Corg represented 44% of the explained variance of the H1 index variations in plots SS1 (Table 2). In the control plots, H1 index variations were mainly related to soil parameters: soil carbon content and sand represented 45 and 13% of the explained variance, respectively (Table 2). For the H2 index, variations were exclusively related to soil parameters: soil pH and soil carbon content represented 39 and 38% of the explained variance in the SS1 and control plots, respectively (Table 2).
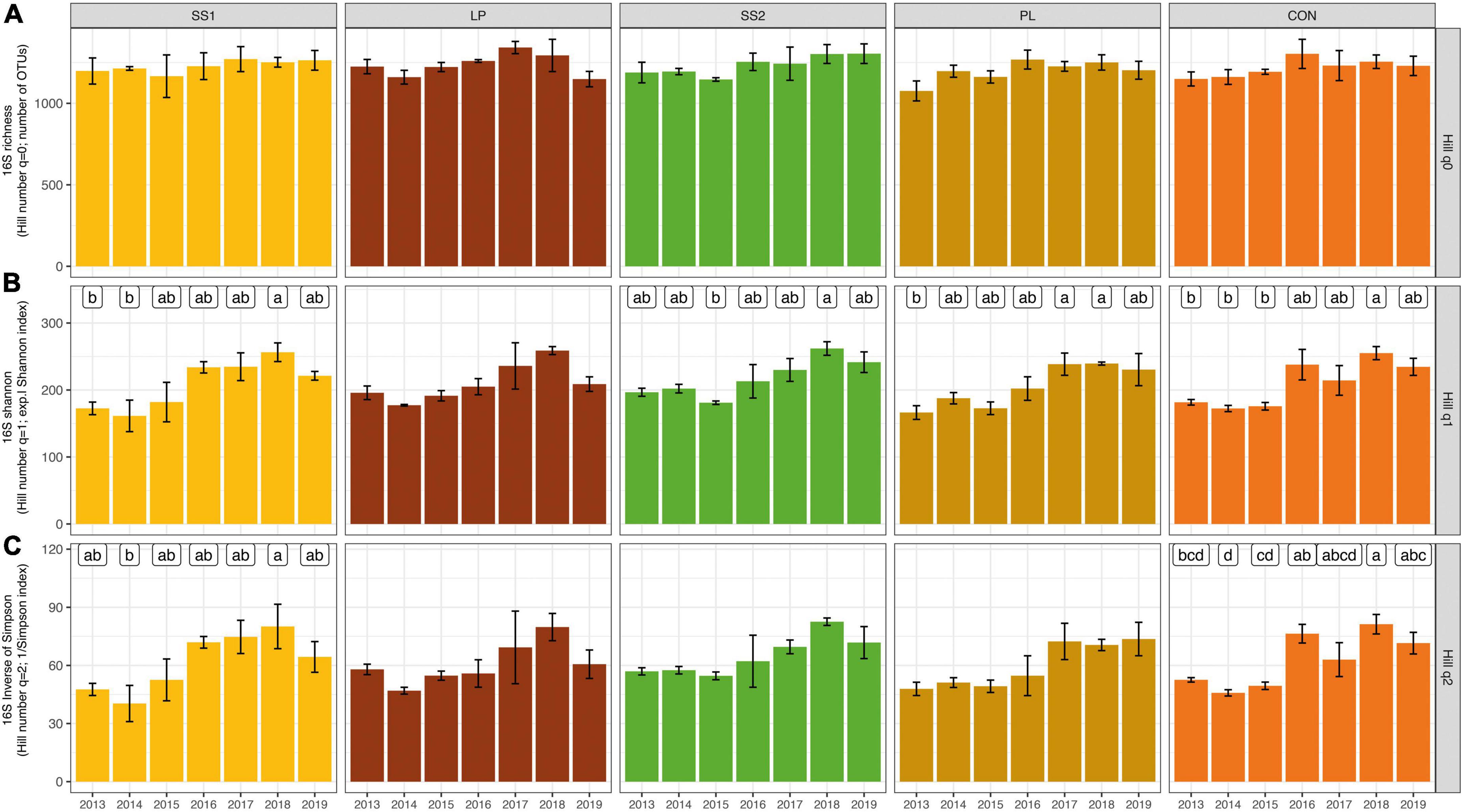
Figure 3. Dynamic of the bacterial and archaeal community based on Hill numbers [Hill number with an order 0 (A), 1 (B), and 2 (C)] in soil from La Mare (Reunion Island) fertilized or not (CON, inorganic fertilizer control) with four different organic materials (SS1 and SS2, sewage sludge inputs; LP, liquid pig manure; PL, poultry litter). For each treatment, different letters indicate significant differences between years based on Kruskal-Wallis test with Bonferroni correction (P < 0.05).
The NMDS ordination highlighted that the structure of the bacterial and archaeal community was more influenced by time (P < 0.001; PERMANOVA; Table 3 and Figure 4) than by the fertilization type (P > 0.05; PERMANOVA, Table 3 and Figure 4). Thus, repeated fertilizer applications over time tended to modify the abundance of the bacterial and archaeal phyla and genera (Figures 4, 5). The LEfSe analysis allowed identifying major bacterial and archaeal phyla and genera presenting the strongest response to fertilization practices over time (Figure 6). Five phyla were significantly impacted: Acidobacteria, Actinobacteria, Bacteroidetes, Firmicutes, and Proteobacteria (Figure 6 and Supplementary Figures 2–6). In general, 1 year after the start of the experiment (2014), the relative abundance of most bacterial and archaeal phyla shifted whatever the fertilization type, with a return to the initial state 1 year later (2015). At the phylum level, this shift was characterized by a decrease of Acidobacteria and Bacteroidetes and an increase of Actinobacteria and Firmicutes (Figures 5, 6). Whatever the treatment, Proteobacteria was a major phylum with high relative abundance over time. This phyla recorded, however, smallest variations over time compared to other phyla (Figures 5, 6). In 2016, a second shift was observed. From this period, Bacteroidetes and Proteobacteria increase lastingly in all plots, whereas a decrease of Acidobacteria and Actinobacteria was observed (Figures 5, 6). Three major bacterial genera presented the strongest response to fertilization practices over time, following various patterns. Gaiella (Actinobacteria) increased 1 year after the start of the experiment, and then decreased gradually over time and in all fertilization types (Figure 6). Rhodoplanes (Proteobacteria) were steadily found highly abundant from 2013 to 2015 whatever the fertilization type, and then declined slightly until the end of the study (Figure 6). Finally, Flavobacterium (Bacteroidetes) was mostly absent whatever the fertilization type during the first 3 years and then increased significantly, in particular the SS1 and LP plots (Figure 6).
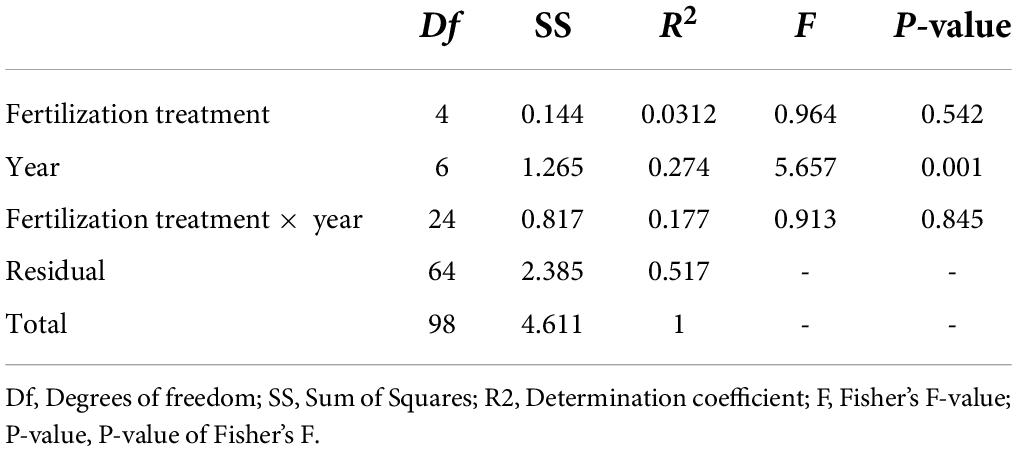
Table 3. PERMANOVA analysis results to depicted the differences in the bacterial and archaeal community compositions of soils from La Mare (Reunion Island) according to the organic material treatment, time, and organic material treatment × time (1,000 permutations, significance threshold: P < 0.001).
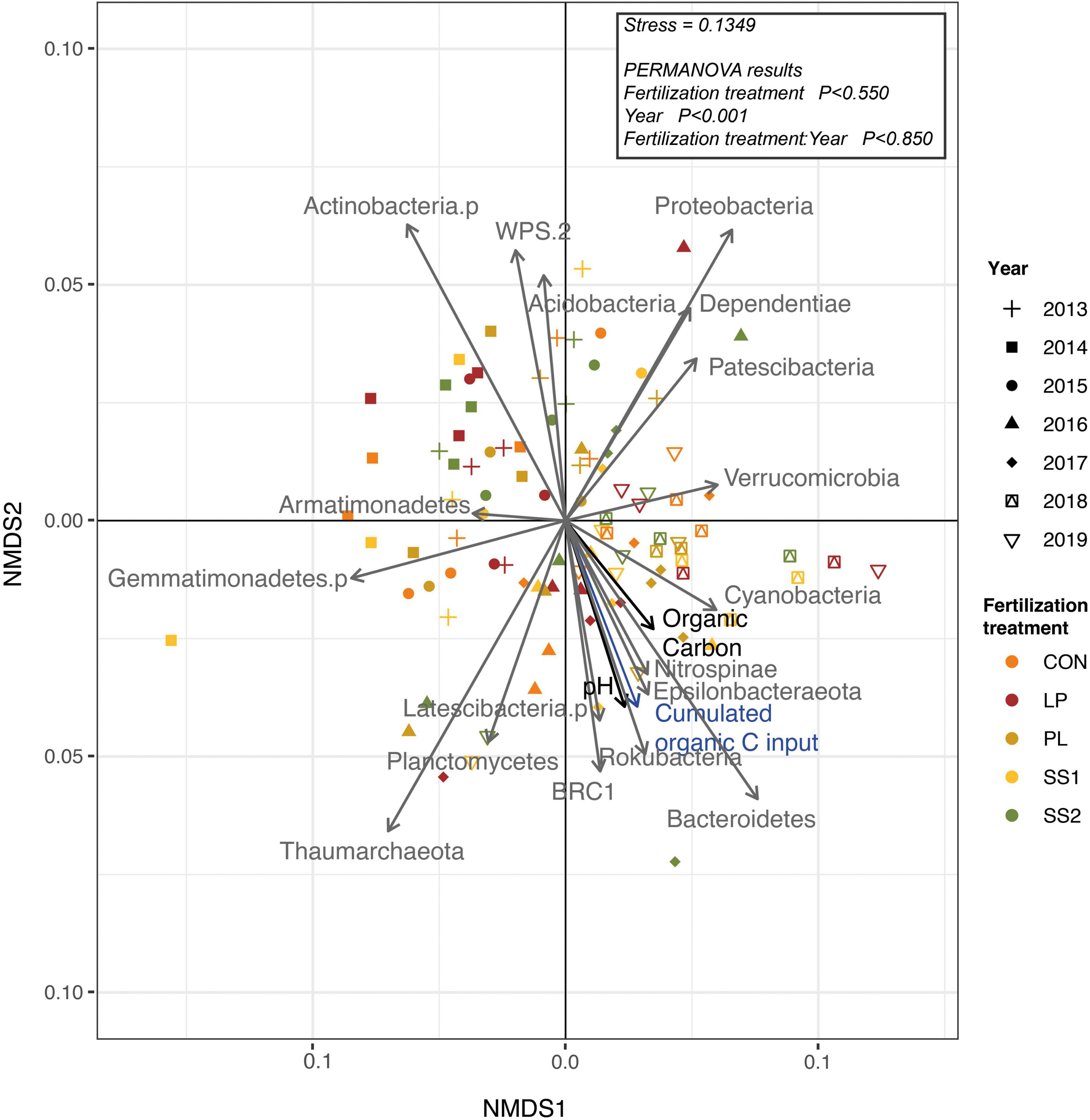
Figure 4. Non-metric Multi-Dimensional Scaling (NMDS) ordination plot derived from Bray Curtis dissimilarity distances for the bacterial and archaeal community in soil from La Mare (Reunion Island) fertilized or not (CON, inorganic fertilizer control) with four different organic materials (SS1 and SS2, sewage sludge inputs; LP, liquid pig manure; PL, poultry litter).
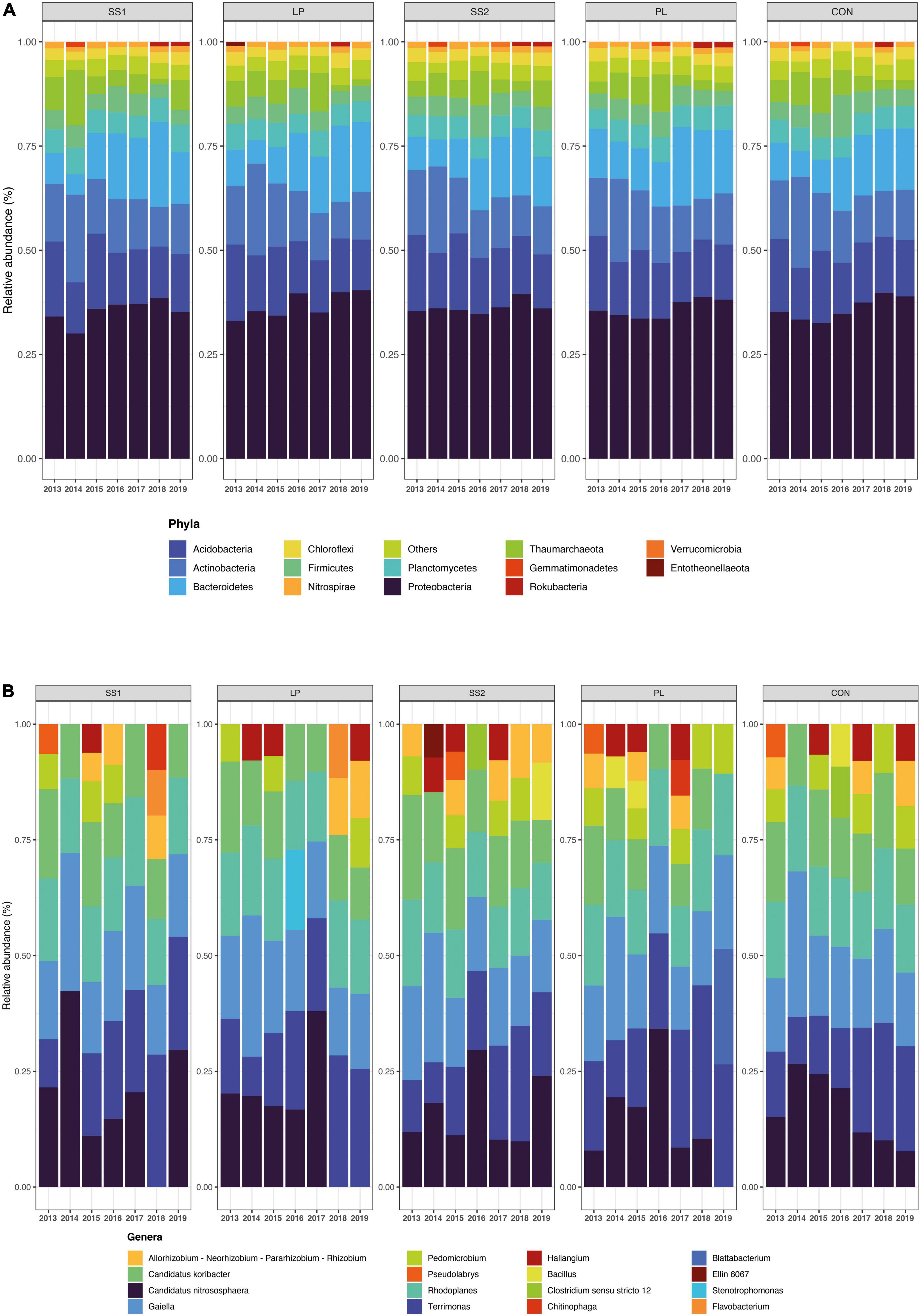
Figure 5. Relative abundance of archaea and bacteria at the phylum (A) and genus (B) level across years for the different fertilization treatments (CON, inorganic fertilizer control) with four different organic materials (SS1 and SS2, sewage sludge inputs; LP, liquid pig manure; PL, poultry litter). Relative taxonomic abundances below 1 or 2% were grouped in “Others” for the phylum and genus levels, respectively. Unknown taxa were excluded from genus plotting.
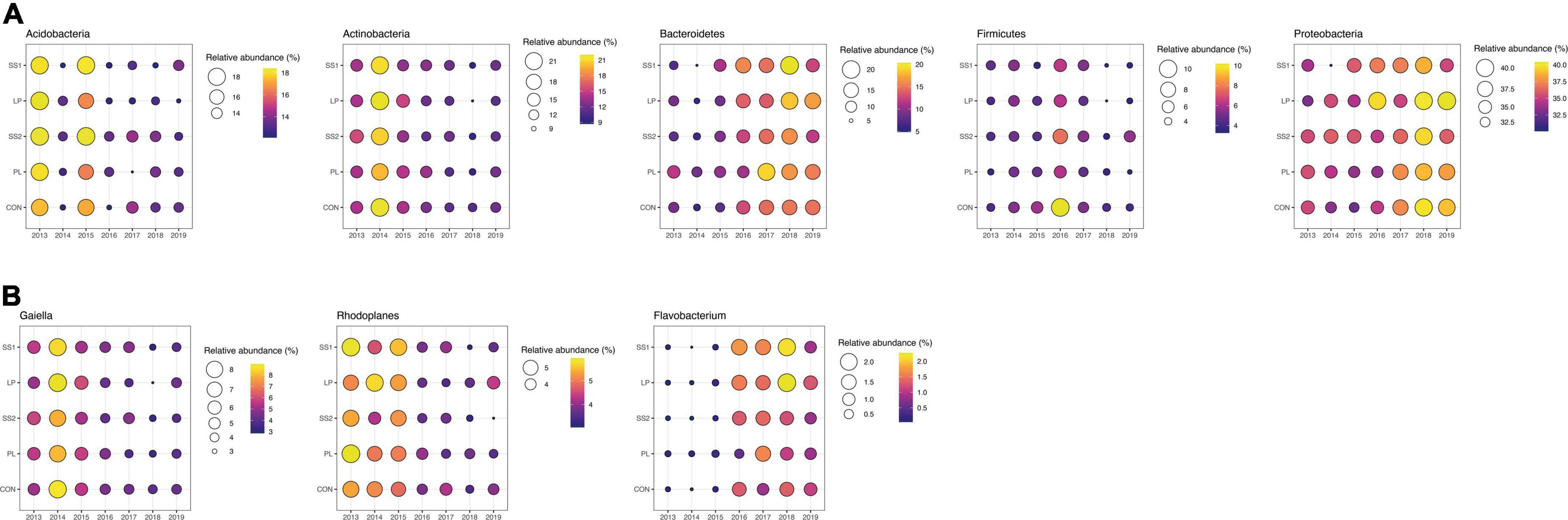
Figure 6. Bubble plots showing the relative abundance of the major bacterial phyla (A) and genera (B) significantly different depending on fertilization practices (CON, inorganic fertilizer control; SS1 and SS2, sewage sludge inputs; LP, liquid pig manure; PL, poultry litter) over time after a LEfSe analysis. Presented phyla and genera were selected based on LEfSe analysis allowing to detect the taxa with significant differential abundances among the soil fertilization types for all years.
Discussion
Repeated use of organic and inorganic fertilizers over several years improves crop productivity. To better understand the impact of these fertilization practices on soil fertility, we assessed the dynamic of the soil bacterial and archaeal communities in response to different repeated mixed organic-inorganic fertilizer inputs in a field experiment. To our knowledge, this has never been assessed before.
Repeated applications of mixed organic and inorganic materials induced lasting modifications of the soil chemical properties under some fertilization conditions, with increases of the soil organic carbon content and the pH. Along with the control plots that only received inorganic fertilization, the SS2 and PL plots received the greatest quantity of inorganic inputs. As mentioned by Giacometti et al. (2013), the increase of the soil organic carbon content under mid-term mineral fertilizer application may be an indirect effect of higher plant biomass production and C return to soils. However, the soil organic carbon content did not increase in the control plots. This suggests that the increase of the soil organic carbon content was not only due to the sole indirect effect of mineral fertilization. In parallel, several studies performed following several years of repeated fertilizer inputs have shown that the combination of organic and inorganic inputs increases the soil organic carbon content compared with inorganic fertilization (Hao et al., 2008; Zhong et al., 2010; Chakraborty et al., 2011; Li et al., 2015, 2017; Sun et al., 2015; Francioli et al., 2016; Kumar et al., 2017; Guo et al., 2019; Diallo et al., 2021; Feder, 2021a). Therefore, we suppose that the increased soil organic carbon content observed in SS2 and PL resulted from mixed organic-inorganic fertilizer inputs. The quality of organic material applied could also explain the lasting increase of the soil organic carbon content for these two treatments. The C:N ratio is good proxy to assess the quality of organic materials applied (labile or recalcitrant) (Vallejo, 1993). Thus, SS2 and PL could have more recalcitrant organic carbon (C:N = 7.43 and 9.68, respectively, Supplementary Table 1) which could explain increase of soil organic carbon contain.
Five and 6 years after the start of the experiment (2018), a significant increase of the pH value was observed only in the SS1 and LP, respectively. Plots SS1 and LP were the only two plots that received organic fertilization yearly. Our findings are consistent with previous studies highlighting that repeated applications of mixed organic and inorganic materials increase the soil pH (Zhong et al., 2010; Dong et al., 2014; Hartmann et al., 2015; Zhao et al., 2016; Wang et al., 2017).
As observed in previous studies, our results highlight that some repeated mixed organic-inorganic fertilizer inputs (SS1, LP and PL) induce a lasting increase of the soil microbial biomass (Hao et al., 2008; Gu et al., 2009; Chakraborty et al., 2011; Li et al., 2015; Francioli et al., 2016; Kumar et al., 2017; Guo et al., 2019) occurring 4–6 years after the start of the experiment. Compared to a low frequency of organic material application (every 3 years; SS2 and PL), a yearly application (SS1 and LP) lastingly increased the soil microbial biomass. Interestingly, a positive correlation between the soil carbon content and the soil microbial biomass was observed in the PL plots (Table 2). PL was applied every 3 years, and had a high C:N ratio (9.68 on average). This suggests that poultry litter would be more resistant to decomposition and would have a higher C sequestration potential. Thus, low-frequency application of an organic material with a high C:N ratio (e.g., PL) also led to a lasting increase of the soil carbon content that allowed for optimal growth of soil microbes, and in turn an increase of the soil microbial biomass.
The bacterial and archaeal richness index (i.e., H0) of the organically inorganically fertilized plots and control plots that only received inorganic fertilization did not evolve over time. Literature results are not congruent about the effect of fertilization practices on soil alpha-diversity. Some works report a higher bacterial richness in soil fertilized with a mixed organic-inorganic fertilization input compared with a soil solely fertilized with inorganic material (Hartmann et al., 2015; Sun et al., 2015), whereas others show opposite results (Gu et al., 2009). The quantities of organic and inorganic materials were similar across all studies. However, as pointed out by Hartmann et al. (2015), these different results could be due to the use of different methods (primer sequences, bioinformatics analysis), the metric itself, and most importantly the experimental design. Regarding H1 and H2 indices, both increased significantly in SS1 and control plots 4 years after the first fertilizer input; and H1 similarly tended to increase in SS2 and PL plots. Altogether, this suggested that these fertilization practices stimulate abundant OTUs more than LP treatment, and that control and SS1 treatments stimulated dominant OTUs. Except for H2 in SS1 plot, variations of H1 or H2 were mainly related either to soil organic carbon content or cumulated carbon inputs. The increase of H1 and H2 indices may be related to organic fertilizer application with high C:N ratio in SS1, SS2, and PL plots but also by returning straw to soil in all plots. This would suggest that increasing soil carbon resources may increase some abundant and dominant phyla as supported by Francioli et al. (2016); leading to an increase in H1 and H2. In SS1 plots, changes in H2 were not related to carbon resources but to soil pH variations. This may be explained a confounding effect of soil pH and organic fertilizer annual inputs or cumulated organic carbon inputs since slight but not significant correlations between these variables were observed.
As observed by Durrer et al. (2017), the dominant bacterial and archaeal phyla in tropical soils under sugarcane cultivation were Proteobacteria, Acidobacteria, Actinobacteria, Bacteroidetes, Planctomycetes, Firmicutes, Thaumarchaeota, and Chloroflexi. In the present study, the structure of the bacterial and archaeal community evolved over time whatever fertilizer input: Acidobacteria, and Actinobacteria decreased, while Bacteroidetes and Proteobacteria increased. A strong modification of bacterial and archaeal community composition occurred in 2014 but this returned to its initial state in 2015. This suggests that the experimental set up impacted strongly the community composition likely because of soil plowing (Lienhard et al., 2014). On the other hand, since 2016, bacterial and archaeal community composition was lastingly modified regarding to 2015 whatever the fertilization practice, with slight variations between 2016 and 2019. During this period, there were no major physical perturbations but sugarcane straw were systematically reallocated. This supplemental organic material input may have reduced the relative effect of each organic treatments on bacterial and archaeal community composition. This is not in agreement with other studies reporting significant differences between the composition of soil bacterial communities associated to different fertilizer inputs (Hartmann et al., 2015; Francioli et al., 2016; Wang et al., 2017; van der Bom et al., 2018). Indeed, these authors highlight that organic fertilization stimulates copiotrophic phyla such as Firmicutes and Proteobacteria, known to prefer nutrient-rich environments and involved in the degradation of complex organic compounds. In contrast, the soils that did not receive manure harbored distinct microbial communities enriched in oligotrophic organisms adapted to nutrient-limited environments, such as Acidobacteria and Bacteroidetes. Actinobacteria have been described abundant both in organically (Poulsen et al., 2013; Riber et al., 2014) and inorganically fertilized soils (Francioli et al., 2016). This phylum is often described as having a copiotrophic lifestyle (Fierer et al., 2007; Ramirez et al., 2012). At the end point of our study (2019), our results are not in accordance with these previous works that as altogether, our results show no differences in bacterial community composition between treatments (Figure 4, PERMANOVA, P < 0.0850), despite several years of fertilizer application. The similar soil bacterial and archaeal community dynamics of inorganic and mixed organic-inorganic fertilizer inputs found in the present study are surprising compared to the literature. A stimulation of oligotrophic phyla such as Acidobacteria and Bacteroidetes in the control plots was expected. However, as sugarcane residues were returned to the soil, they also contributed to organic fertilization in the plots that only received inorganic fertilization. This could explain the similar dynamic of bacterial and archaeal community structures in plots receiving different fertilizer inputs.
The lasting modification over time of the bacterial and archaeal community composition was also observed at the genus level. Three genera were implied: Gaiella (Actinobacteria), Rhodoplanes (Proteobacteria), and Flavobacterium (Bacteroidetes). Except for Flavobacterium, the relative abundance of all of these genera decreased over time. Little information is available in the literature on these genera. However, Gaiella and Flavobacterium would have a higher relative abundance in soil fertilized with inorganic material (van der Bom et al., 2018; Cai et al., 2020), whereas Rhodoplanes would tend to increase in soil fertilized with organic material. The difference with our results could be explained by the mixed fertilization practices in our trial: although all plots received inorganic fertilization, all of them also received organic material inputs through sugarcane residue restitution, which added to organic fertilizer when applied.
The present study indicates that the bacterial and archaeal community of a tropical soil evolves over time, with few changes in the first years following the start of the experiment, and a lasting modification 3–6 years after it (depending on the soil microbial indicator considered). The structure of the bacterial and archaeal community was more influenced by time than by the nature of organic input or the fertilization practice (frequency and applied quantity).
Data availability statement
The datasets presented in this study can be found in online repositories. The names of the repository/repositories and accession number(s) can be found below: https://www.ebi.ac.uk/ena/browser/view/PRJEB52689.
Author contributions
SS-B and FF planned the study and performed the lab work. FF coordinated the sampling. SS-B, FF, CD, and NC analyzed the data and provided general guidance. SS-B wrote the manuscript. FF, CD, and NC contributed to reviewing the manuscript. All authors contributed to the article and approved the submitted version.
Funding
This study was co-financed by special funds provided by ADEME-GRAINE and the University of Bourgogne Franche-Comté—France.
Acknowledgments
This work, through the involvement of technical facilities of the GenoSol platform of the infrastructure ANAEE-Services, received a grant from the French state through the National Agency for Research under the program “Investments for the Future” (reference ANR-11-INBS-0001), as well as a grant from the Regional Council of Burgundy. The La Mare field experiment is conducted within a collaboration between INRAE and CIRAD and is part of the INRAE SOERE PRO (network of mid-term experiments dedicated to the study of impacts of organic waste product recycling), certified and funded in 2013 by ALLENVI (Alliance Nationale de Recherche pour l’Environnement) and integrated as a service of the infrastructure “Investment for the future” AnaEE-France, overseen by the French National Research Agency (ANR 11-INBS-0001). We thank all those who participated in the field experiments and management, as well as the partners. The Reunion field experiment is also a partnership of CIRAD, RUNEO and the European Agricultural Guidance and Guarantee Fund (EAGGF, Fonds Européen d’Orientation et de Garantie Agricole, French acronym FEOGA). We are grateful to two journal reviewers, and the editor valuable suggestions for improving this manuscript.
Conflict of interest
The authors declare that the research was conducted in the absence of any commercial or financial relationships that could be construed as a potential conflict of interest.
Publisher’s note
All claims expressed in this article are solely those of the authors and do not necessarily represent those of their affiliated organizations, or those of the publisher, the editors and the reviewers. Any product that may be evaluated in this article, or claim that may be made by its manufacturer, is not guaranteed or endorsed by the publisher.
Supplementary material
The Supplementary Material for this article can be found online at: https://www.frontiersin.org/articles/10.3389/fmicb.2022.943314/full#supplementary-material
Footnotes
- ^ https://www6.inrae.fr/valor-pro/Les-sites-experimentaux/SOERE-PRO-Reunion
- ^ https://www6.hautsdefrance.inrae.fr/las
- ^ https://www2.dijon.inrae.fr/plateforme_genosol/
References
Abis, L., Loubet, B., Ciuraru, R., Lafouge, F., Houot, S., Nowak, V., et al. (2020). Reduced microbial diversity induces larger volatile organic compound emissions from soils. Sci. Rep. 10:6104. doi: 10.1038/s41598-020-63091-8
Ai, C., Zhang, S., Zhang, X., Guo, D., Zhou, W., and Huang, S. (2018). Distinct responses of soil bacterial and fungal communities to changes in fertilization regime and crop rotation. Geoderma 319, 156–166. doi: 10.1016/j.geoderma.2018.01.010
Bastida, F., Hernández, T., Albaladejo, J., and García, C. (2013). Phylogenetic and functional changes in the microbial community of long-term restored soils under semiarid climate. Soil Biol. Biochem. 65, 12–21. doi: 10.1016/j.soilbio.2013.04.022
Bastida, F., Kandeler, E., Moreno, J. L., Ros, M., García, C., and Hernández, T. (2008). Application of fresh and composted organic wastes modifies structure, size and activity of soil microbial community under semiarid climate. Appl. Soil Ecol. 40, 318–329. doi: 10.1016/j.apsoil.2008.05.007
Blum, J., Melfi, A. J., Montes, C. R., and Gomes, T. M. (2013). Nitrogen and phosphorus leaching in a tropical Brazilian soil cropped with sugarcane and irrigated with treated sewage effluent. Agric. Water Manage. 117, 115–122. doi: 10.1016/j.agwat.2012.11.010
Bünemann, E. K., Bongiorno, G., Bai, Z., Creamer, R. E., De Deyn, G., De Goede, R., et al. (2018). Soil quality–A critical review. Soil Biol. Biochem. 120, 105–125. doi: 10.1016/j.soilbio.2018.01.030
Cai, S., Wang, J., Lv, W., Xu, S., and Zhu, H. (2020). Nitrogen fertilization alters the effects of earthworms on soil physicochemical properties and bacterial community structure. Appl. Soil Ecol. 150:103478. doi: 10.1016/j.apsoil.2019.103478
Calbrix, R., Barray, S., Chabrerie, O., Fourrie, L., and Laval, K. (2007). Impact of organic amendments on the dynamics of soil microbial biomass and bacterial communities in cultivated land. Appl. Soil Ecol. 35, 511–522. doi: 10.1016/j.apsoil.2006.10.007
Chakraborty, A., Chakrabarti, K., Chakraborty, A., and Ghosh, S. (2011). Effect of long-term fertilizers and manure application on microbial biomass and microbial activity of a tropical agricultural soil. Biol. Fertil. Soils 47, 227–233. doi: 10.1007/s00374-010-0509-1
Chao, A., Chiu, C.-H., and Jost, L. (2010). Phylogenetic diversity measures based on Hill numbers. Philos. Trans. R. Soc. B Biol. Sci. 365, 3599–3609. doi: 10.1098/rstb.2010.0272
Chemidlin Prévost-Bouré, N., Karimi, B., Sadet-Bourgeteau, S., Djemiel, C., Brie, M., Dumont, J., et al. (2021). Microbial transfers from permanent grassland ecosystems to milk in dairy farms in the Comté cheese area. Sci. Rep. 11:18144. doi: 10.1038/s41598-021-97373-6
Chen, C., Zhang, J., Lu, M., Qin, C., Chen, Y., Yang, L., et al. (2016). Microbial communities of an arable soil treated for 8 years with organic and inorganic fertilizers. Biol. Fertil. Soils 52, 455–467. doi: 10.1007/s00374-016-1089-5
Chessel, D., Dufour, A. B., and Thioulouse, J. (2004). The ade4 package-I-One-table methods. R News 4, 5–10.
Clapp, C. E., Stark, S. A., Clay, D. E., and Larson, W. E. (1986). “Sewage sludge organic matter and soil properties,” in The Role of Organic Matter in Modern Agriculture, eds Y. Chen and Y. Avnimelech (Berlin: Springer). doi: 10.1007/978-94-009-4426-8_10
Cole, J. R., Wang, Q., Cardenas, E., Fish, J., Chai, B., Farris, R. J., et al. (2009). The Ribosomal Database Project: improved alignments and new tools for rRNA analysis. Nucleic Acids Res. 37, D141–D145.
Dequiedt, S., Saby, N. P. A., Lelievre, M., Jolivet, C., Thioulouse, J., Toutain, B., et al. (2011). Biogeographical patterns of soil molecular microbial biomass as influenced by soil characteristics and management. Glob. Ecol. Biogeogr. 20, 641–652. doi: 10.1111/j.1466-8238.2010.00628.x
Diallo, F., Legros, S., Diarra, K., and Feder, F. (2021). Varying effects of organic waste products on yields of market garden crops in a 4-year field experiment under tropical conditions. Agronomy 12:32. doi: 10.3390/agronomy12010032
Ding, J., Jiang, X., Ma, M., Zhou, B., Guan, D., Zhao, B., et al. (2016). Effect of 35 years inorganic fertilizer and manure amendment on structure of bacterial and archaeal communities in black soil of northeast China. Appl. Soil Ecol. 105, 187–195. doi: 10.1016/j.apsoil.2016.04.010
Djemiel, C., Dequiedt, S., Karimi, B., Cottin, A., Girier, T., El Djoudi, Y., et al. (2020). BIOCOM-PIPE: a new user-friendly metabarcoding pipeline for the characterization of microbial diversity from 16S, 18S and 23S rRNA gene amplicons. BMC Bioinformatics 21:492. doi: 10.1186/s12859-020-03829-3
Dong, W.-Y., Zhang, X.-Y., Dai, X.-Q., Fu, X.-L., Yang, F.-T., Liu, X.-Y., et al. (2014). Changes in soil microbial community composition in response to fertilization of paddy soils in subtropical China. Appl. Soil Ecol. 84, 140–147. doi: 10.1016/j.apsoil.2014.06.007
Doran, J., and Parkin, T. (1994). “Defining soil quality for a sustainable environment,” in Defining and Assessing Soil Quzality, eds W. Doran, D. C. Coleman, D. F. Bezdicek, and B. A. Stewart (Madison, WI: SSSA Special Publications). doi: 10.2136/sssaspecpub35
Doran, J. W., and Parkin, T. B. (1997). “Methods for assessing soil quality,” in Quantitative Indicators of Soil Quality: A Minimum Data Set, eds J. W. Doran and A. J. Jones (Madison, WI: SSSA Special Publications). doi: 10.2136/sssaspecpub49
Dunn, L., Lang, C., Marilleau, N., Terrat, S., Biju-Duval, L., Lelièvre, M., et al. (2021). Soil microbial communities in the face of changing farming practices: a case study in an agricultural landscape in France. PLoS One 16:e0252216. doi: 10.1371/journal.pone.0252216
Durrer, A., Gumiere, T., Taketani, R. G., Da Costa, D. P. E., Silva, M. D. C. P., and Andreote, F. D. (2017). The drivers underlying biogeographical patterns of bacterial communities in soils under sugarcane cultivation. Appl. Soil Ecol. 110, 12–20.
Edgar, R. C. (2010). Search and clustering orders of magnitude faster than BLAST. Bioinformatics 26, 2460–2461.
Esperschütz, J., Gattinger, A., Mäder, P., Schloter, M., and Fließbach, A. (2007). Response of soil microbial biomass and community structures to conventional and organic farming systems under identical crop rotations. FEMS Microbiol. Ecol. 61, 26–37. doi: 10.1111/j.1574-6941.2007.00318.x
Feder, F. (2013). Soil map update: Procedure and problems encountered for the island of Réunion. Catena 110, 215–224.
Feder, F. (2021b). Irrigation with treated wastewater in humid regions: effects on Nitisols, sugarcane yield and quality. Agric. Water Manage. 247:106733.
Feder, F. (2021a). Effects of fertilisation using organic waste products with mineral complementation on sugarcane yields and soil properties in a 4 year field experiment. Agriculture 11:985.
Fierer, N., Bradford, M. A., and Jackson, R. B. (2007). Toward and ecological classification of soil bacteria. Ecology 88, 1354–1364.
Francioli, D., Schulz, E., Lentendu, G., Wubet, T., Buscot, F., and Reitz, T. (2016). Mineral vs. organic amendments: microbial community structure, activity and abundance of agriculturally relevant microbes are driven by long-term fertilization strategies. Front. Microbiol. 7:1446. doi: 10.3389/fmicb.2016.01446
Ghube, N., Kadlag, A., and Kamble, B. (2017). Impact of different levels of organic and inorganic fertilizers on growth, yield and quality of preseasonal sugarcane ratoon in Inceptisols. J. Appl. Nat. Sci. 9, 812–820.
Giacometti, C., Demyan, M. S., Cavani, L., Marzadori, C., Ciavatta, C., and Kandeler, E. (2013). Chemical and microbiological soil quality indicators and their potential to differentiate fertilization regimes in temperate agroecosystems. Appl. Soil Ecol. 64, 32–48.
Gomiero, T., Pimentel, D., and Paoletti, M. G. (2011). Environmental impact of different agricultural management practices: conventional vs. organic agriculture. Crit. Rev. Plant Sci. 30, 95–124.
Gu, Y., Zhang, X., Tu, S., and Lindström, K. (2009). Soil microbial biomass, crop yields, and bacterial community structure as affected by long-term fertilizer treatments under wheat-rice cropping. Eur. J. Soil Biol. 45, 239–246.
Guo, Z., Han, J., Li, J., Xu, Y., and Wang, X. (2019). Effects of long-term fertilization on soil organic carbon mineralization and microbial community structure. PLoS One 14:e0211163. doi: 10.1371/journal.pone.0211163
Hao, X., Liu, S., Wu, J., Hu, R., Tong, C., and Su, Y. (2008). Effect of long-term application of inorganic fertilizer and organic amendments on soil organic matter and microbial biomass in three subtropical paddy soils. Nutr. Cycl. Agroecosyst. 81, 17–24.
Hartmann, M., Frey, B., Mayer, J., Mäder, P., and Widmer, F. (2015). Distinct soil microbial diversity under long-term organic and conventional farming. ISME J. 9, 1177–1194.
Ho, A., El-Hawwary, A., Kim, S. Y., Meima-Franke, M., and Bodelier, P. (2015). Manure-associated stimulation of soil-borne methanogenic activity in agricultural soils. Biol. Fertil. Soils 51, 511–516.
Hutchinson, J., Campbell, C., and Desjardins, R. (2007). Some perspectives on carbon sequestration in agriculture. Agric. For. Meteorol. 142, 288–302.
Kamaa, M., Mburu, H., Blanchart, E., Chibole, L., Chotte, J.-L., Kibunja, C., et al. (2011). Effects of organic and inorganic fertilization on soil bacterial and fungal microbial diversity in the Kabete long-term trial, Kenya. Biol. Fertil. Soils 47, 315–321.
Kumar, U., Shahid, M., Tripathi, R., Mohanty, S., Kumar, A., Bhattacharyya, P., et al. (2017). Variation of functional diversity of soil microbial community in sub-humid tropical rice-rice cropping system under long-term organic and inorganic fertilization. Ecol. Indic. 73, 536–543.
Leal, R. M. P., Herpin, U., Da Fonseca, A. F., Firme, L. P., Montes, C. R., and Melfi, A. J. (2009). Sodicity and salinity in a Brazilian Oxisol cultivated with sugarcane irrigated with wastewater. Agric. Water Manage. 96, 307–316.
Li, F., Chen, L., Zhang, J., Yin, J., and Huang, S. (2017). Bacterial community structure after long-term organic and inorganic fertilization reveals important associations between soil nutrients and specific taxa involved in nutrient transformations. Front. Microbiol. 8:187. doi: 10.3389/fmicb.2017.00187
Li, J., Cooper, J. M., Li, Y., Yang, X., and Zhao, B. (2015). Soil microbial community structure and function are significantly affected by long-term organic and mineral fertilization regimes in the North China Plain. Appl. Soil Ecol. 96, 75–87.
Liang, Q., Chen, H., Gong, Y., Fan, M., Yang, H., Lal, R., et al. (2012). Effects of 15 years of manure and inorganic fertilizers on soil organic carbon fractions in a wheat-maize system in the North China Plain. Nutr. Cycl. Agroecosyst. 92, 21–33.
Lienhard, P., Terrat, S., Prévost-Bouré, N., Nowak, V., Régnier, T., Sayphoummie, S., et al. (2014). Pyrosequencing evidences the impact of cropping on soil bacterial and fungal diversity in Laos tropical grassland. Agron. Sustain. Dev. 34, 525–533.
Liu, M., Hu, F., Chen, X., Huang, Q., Jiao, J., Zhang, B., et al. (2009). Organic amendments with reduced chemical fertilizer promote soil microbial development and nutrient availability in a subtropical paddy field: the influence of quantity, type and application time of organic amendments. Appl. Soil Ecol. 42, 166–175.
Mäder, P., Fliessbach, A., Dubois, D., Gunst, L., Fried, P., and Niggli, U. (2002). Soil fertility and biodiversity in organic farming. Science 296, 1694–1697.
Maron, P.-A., Sarr, A., Kaisermann, A., Lévêque, J., Mathieu, O., Guigue, J., et al. (2018). High microbial diversity promotes soil ecosystem functioning. Appl. Environ. Microbiol. 84:e2738-17.
Oksanen, J., Blanchet, F. G., Kindt, R., Legendre, P., Minchin, P. R., O’hara, R., et al. (2013). Package ‘vegan’. Commun. Ecol. Package 2, 1–295.
Peltre, C., Christensen, B. T., Dragon, S., Icard, C., Kätterer, T., and Houot, S. (2012). RothC simulation of carbon accumulation in soil after repeated application of widely different organic amendments. Soil Biol. Biochem. 52, 49–60.
Poulsen, P. H. B., Al-Soud, W. A., Bergmark, L., Magid, J., Hansen, L. H., and Sørensen, S. J. (2013). Effects of fertilization with urban and agricultural organic wastes in a field trial – Prokaryotic diversity investigated by pyrosequencing. Soil Biol. Biochem. 57, 784–793.
Quast, C., Pruesse, E., Yilmaz, P., Gerken, J., Schweer, T., Yarza, P., et al. (2012). The SILVA ribosomal RNA gene database project: improved data processing and web-based tools. Nucleic Acids Res. 41, D590–D596. doi: 10.1093/nar/gks1219
Ramirez, K. S., Craine, J. M., and Fierer, N. (2012). Consistent effects of nitrogen amendments on soil microbial communities and processes across biomes. Global Change Biol. 18, 1918–1927.
Ranjard, L., Lejon, D. P., Mougel, C., Schehrer, L., Merdinoglu, D., and Chaussod, R. (2003). Sampling strategy in molecular microbial ecology: influence of soil sample size on DNA fingerprinting analysis of fungal and bacterial communities. Environ. Microbiol. 5, 1111–1120. doi: 10.1046/j.1462-2920.2003.00521.x
Riber, L., Poulsen, P. H. B., Al-Soud, W. A., Skov Hansen, L. B., Bergmark, L., Brejnrod, A., et al. (2014). Exploring the immediate and long-term impact on bacterial communities in soil amended with animal and urban organic waste fertilizers using pyrosequencing and screening for horizontal transfer of antibiotic resistance. FEMS Microbiol. Ecol. 90, 206–224. doi: 10.1111/1574-6941.12403
Segata, N., Izard, J., Waldron, L., Gevers, D., Miropolsky, L., Garrett, W. S., et al. (2011). Metagenomic biomarker discovery and explanation. Genome biology 12:R60.
Stark, C., Condron, L. M., Stewart, A., Di, H. J., and O’callaghan, M. (2007). Influence of organic and mineral amendments on microbial soil properties and processes. Appl. Soil Ecol. 35, 79–93.
Sun, R., Zhang, X.-X., Guo, X., Wang, D., and Chu, H. (2015). Bacterial diversity in soils subjected to long-term chemical fertilization can be more stably maintained with the addition of livestock manure than wheat straw. Soil Biol. Biochem. 88, 9–18.
Tardy, V., Chabbi, A., Charrier, X., De Berranger, C., Reignier, T., Dequiedt, S., et al. (2015). Land use history shifts in situ fungal and bacterial successions following wheat straw input into the soil. PLoS One 10:e0130672. doi: 10.1371/journal.pone.0130672
Tardy, V., Mathieu, O., Lévêque, J., Terrat, S., Chabbi, A., Lemanceau, P., et al. (2014). Stability of soil microbial structure and activity depends on microbial diversity. Environ. Microbiol. Rep. 6, 173–183.
Terrat, S., Christen, R., Dequiedt, S., Lelièvre, M., Nowak, V., Regnier, T., et al. (2012). Molecular biomass and MetaTaxogenomic assessment of soil microbial communities as influenced by soil DNA extraction procedure: soil DNA extraction impact on bacterial diversity. Microb. Biotechnol. 5, 135–141. doi: 10.1111/j.1751-7915.2011.00307.x
Terrat, S., Djemiel, C., Journay, C., Karimi, B., Dequiedt, S., Horrigue, W., et al. (2020). ReClustOR: a re-clustering tool using an open-reference method that improves operational taxonomic unit definition. Methods Ecol. Evol. 11, 168–180.
Vallejo, V. (1993). Evaluation of C: N ratio as a parameter of N mineralization. Mitt. Österreich Bodenkundlichen Ges. 47, 71–78.
van der Bom, F., Nunes, I., Raymond, N. S., Hansen, V., Bonnichsen, L., Magid, J., et al. (2018). Long-term fertilisation form, level and duration affect the diversity, structure and functioning of soil microbial communities in the field. Soil Biol. Biochem. 122, 91–103.
Vivant, A.-L., Garmyn, D., Maron, P.-A., Nowak, V., and Piveteau, P. (2013). Microbial diversity and structure are drivers of the biological barrier effect against Listeria monocytogenes in soil. PLoS One 8:e76991. doi: 10.1371/journal.pone.0076991
Wang, J., Song, Y., Ma, T., Raza, W., Li, J., Howland, J. G., et al. (2017). Impacts of inorganic and organic fertilization treatments on bacterial and fungal communities in a paddy soil. Appl. Soil Ecol. 112, 42–50.
WRB, I. W. G. (2015). World Reference Base for Soil Resources 2014, update 2015. International Soil Classification System for Naming Soils and Creating Legends for Soil Maps. Roma: FAO.
Zhang, H., Ding, W., He, X., Yu, H., Fan, J., and Liu, D. (2014). Influence of 20–Year Organic and Inorganic Fertilization on Organic Carbon Accumulation and Microbial Community Structure of Aggregates in an Intensively Cultivated Sandy Loam Soil. PLoS One 9:e92733. doi: 10.1371/journal.pone.0092733
Zhang, Q.-C., Shamsi, I. H., Xu, D.-T., Wang, G.-H., Lin, X.-Y., Jilani, G., et al. (2012). Chemical fertilizer and organic manure inputs in soil exhibit a vice versa pattern of microbial community structure. Appl. Soil Ecol. 57, 1–8.
Zhao, J., Ni, T., Li, J., Lu, Q., Fang, Z., Huang, Q., et al. (2016). Effects of organic–inorganic compound fertilizer with reduced chemical fertilizer application on crop yields, soil biological activity and bacterial community structure in a rice–wheat cropping system. Appl. Soil Ecol. 99, 1–12.
Keywords: organic and inorganic fertilization, high-throughput sequencing, tropical, soil, microbial communities
Citation: Sadet-Bourgeteau S, Djemiel C, Chemidlin Prévost-Bouré N and Feder F (2022) Dynamic of bacterial and archaeal diversity in a tropical soil over 6 years of repeated organic and inorganic fertilization. Front. Microbiol. 13:943314. doi: 10.3389/fmicb.2022.943314
Received: 13 May 2022; Accepted: 08 July 2022;
Published: 16 August 2022.
Edited by:
Martin Hartmann, ETH Zürich, SwitzerlandReviewed by:
Martina Lori, Research Institute of Organic Agriculture (FiBL), SwitzerlandAdriana Giongo, Julius Kühn-Institut, Germany
Copyright © 2022 Sadet-Bourgeteau, Djemiel, Chemidlin Prévost-Bouré and Feder. This is an open-access article distributed under the terms of the Creative Commons Attribution License (CC BY). The use, distribution or reproduction in other forums is permitted, provided the original author(s) and the copyright owner(s) are credited and that the original publication in this journal is cited, in accordance with accepted academic practice. No use, distribution or reproduction is permitted which does not comply with these terms.
*Correspondence: Sophie Sadet-Bourgeteau, sophie.bourgeteau-sadet@agrosupdijon.fr
†ORCID: Sophie Sadet-Bourgeteau, orcid.org/0000-0002-7533-4289; Christophe Djemiel, orcid.org/0000-0002-5659-7876; Nicolas Chemidlin Prévost-Bouré, orcid.org/0000-0002-8575-4295; Frederic Feder, orcid.org/0000-0001-8434-5193