- Instituto de Investigaciones Biológicas (IIB-CONICET-UNMDP), Universidad Nacional de Mar del Plata, Mar del Plata, Argentina
Proteolysis plays a fundamental role in many processes that occur within the cellular membrane including protein quality control, protein export, cell signaling, biogenesis of the cell envelope among others. Archaea are a distinct and physiologically diverse group of prokaryotes found in all kinds of habitats, from the human and plant microbiomes to those with extreme salt concentration, pH and/or temperatures. Thus, these organisms provide an excellent opportunity to extend our current understanding on the biological functions that proteases exert in cell physiology including the adaptation to hostile environments. This revision describes the advances that were made on archaeal membrane proteases with regard to their biological function and potential natural targets focusing on the model haloarchaeon Haloferax volcanii.
Introduction
Proteolysis occurring at the cell surface is necessary to maintain membrane protein homeostasis, protein export, cell signaling and biogenesis of the cell envelope among other membrane-related processes. The targets of these enzymes are mainly membrane-anchored and secretory proteins including receptors, transporters and a variety of enzymes such as transferases, oxidoreductases, and hydrolases. Archaeal genomes encode many of the membrane protease families found in bacteria and eukaryotes, however, only a limited number of these enzymes have been studied and their biological function and natural targets in most cases remain unknown. The repertory of membrane-localized proteases that occur in archaeal model organisms as well as their biochemical features were previously reviewed (Maupin-Furlow et al., 2005; De Castro et al., 2006; Rotanova et al., 2006; Ng et al., 2007; Gimenez et al., 2015; Maupin-Furlow, 2018; Pohlschroder et al., 2018).
This article describes advances on the biological role and natural targets of membrane-localized proteases of archaea, taking as case study the model euryarchaeon Haloferax volcanii. In recent years, the impact of LonB and Rhomboid RhoII proteases on H. volcanii physiology was explored by genetic, physiology as well as different proteomics strategies (Cerletti et al., 2014, 2015, 2018; Costa et al., 2018). Several potential natural targets were identified which allowed to begin unraveling the cellular processes regulated by these proteases. A novel mechanism was addressed in H. volcanii which anchors cell envelope proteins (S-layer glycoprotein, SLG) by means of lipid attachment on the C-terminus after processing by the membrane protease archaeosortase A (ArtA) (Abdul Halim et al., 2013). Recently, additional components of the ArtA-dependent cell wall maturation mechanism were discovered, advancing the understanding on the cell envelope biogenesis in archaea.
Table 1 shows a list of membrane-localized proteases of H. volcanii, including potential target proteins and the associated biological processes.
Cellular Processes Regulated by Membrane Proteases in Haloferax volcanii
Carotenoid Biosynthesis
The carotenoid biosynthesis pathway is conserved between photosynthetic organisms and haloarchaea (Rodrigo-Baños et al., 2015). The key step of the pathway is the conversion of two molecules of geranylgeranyl diphosphate (GGPP) into phytoene and is catalyzed by the enzyme phytoene synthase (PSY). In haloarchaea this route leads to the formation of two main products: retinal (C20) and the bacterioruberin-type carotenoids (C50). Bacterioruberin inserts into the membrane and is responsible for the reddish pigmentation of haloarchaeal cells. It prevents oxidative damage, increases membrane rigidity in hyposaline conditions and provides structural support to archaerhodopsin, a retinal-containing protein complex that is found in some haloarchaea including Halorubrum sp. and Halobacterium sp. (Giani et al., 2019). The carotenoid biosynthesis pathway and its regulation has been studied in detail at the transcriptional level in photosynthetic organisms (Toledo-Ortiz et al., 2010; Stanley and Yuan, 2019) and to a very limited extent in archaea (Tarasov et al., 2008; Peck et al., 2017, 2019). In the last years post-translational control of PSY by proteolysis emerged as a novel strategy to modulate carotenogenesis in plants (Welsch et al., 2018; Wang et al., 2020). Interestingly, LonB was found to be a negative regulator of carotenoids biosynthesis in the archaeon H. volcanii by controlling the turnover (degradation) of PSY (Cerletti et al., 2018).
In H. volcanii the membrane-associated LonB is an essential protease meaning that it has a central role in its physiology (Cerletti et al., 2014). H. volcanii mutant cells producing suboptimal (reduced) levels of LonB (strain HVLON3) incremented their pigmentation remarkably (Cerletti et al., 2014) and in contrast, when overexpressing LonB, the cells became completely colorless (Sastre et al., 2010; Cerletti et al., 2014). A shotgun proteomics analysis showed that the abundance of PSY was incremented by about 50-fold in HVLON3 compared to the parental strain H26 (Cerletti et al., 2015). Further studies aiming to explore H. volcanii proteome turnover under varying LonB concentrations demonstrated that the degradation of PSY was strongly LonB-dependent (Cerletti et al., 2018). Altogether these results indicate that PSY is a (direct or indirect) target of this protease. These studies also show that proteolysis of PSY is a posttranslational mechanism for the control of carotenogenesis that is conserved in distantly related organisms such as plants and archaea.
Cell Shape and Cell Division
Haloferax volcanii cells grown in liquid cultures show an irregular plate-shaped morphology but they differentiate into rods to attain efficient swimming motility. This change in morphology is directed by the tubulin-like protein CetZ1 (HVO_2204) (Duggin et al., 2015). Interestingly, H. volcanii mutant cells containing reduced LonB amounts growing in liquid cultures showed unusually irregular-elongated cells compared to the parental strain H26 (Cerletti et al., 2014) while swarmer cells induced to overexpress LonB evidenced a rounded-shaped morphology (Ferrari et al., 2020). Consistent with these phenotypes, high resolution proteomics showed that the abundance and/or turnover of several tubulin homologues were modified depending on LonB expression (Cerletti et al., 2015, 2018). Specifically, the archaeal rod-shape determinant CetZ1 was degraded faster when LonB expression was incremented, which was substantiated by an in vivo degradation assay (Ferrari et al., 2020). Under reduced LonB levels CetZ1 was still degraded suggesting that the turnover of this protein may rely on the activity of other protease/s in addition to LonB. In vivo crosslinking assays coupled to immunoprecipitation with anti-LonB antibodies identified CetZ1 (and CetZ5) among the co-precipitated LonB partners (Ferrari et al., 2020). Altogether, these results provide evidence that LonB is likely involved in the modulation of H. volcanii cell shape by controlling the stability of CetZ1.
As in bacteria, many archaea (including haloarchaea) use the tubulin family proteins FtsZ1 and FtsZ2 to build up the septum during cell division (Liao et al., 2021). However, the regulatory factors affecting this process are largely unknown in these organisms. The Lon protease is a regulator of cell division in bacteria. Upon DNA damage, the inhibitor protein SulA prevents FtsZ polymerization arresting cell division. Once DNA has been repaired, SulA is degraded by the Lon protease allowing FtsZ polymerization. Escherichia coli Δlon cells form filaments after irradiation with UV-B light as they cannot complete cell division. A recent work showed that E. coli Δlon cells complemented with the recombinant LonB protease from the haloarchaeon Natrialba magadii produced shorter filaments after irradiation with UV-B light compared to the control (non-induced cells), and FtsZ1 and FtsZ2 were identified as potential interacting partners with LonB in a pull-down assay (Ferrari et al., 2020). Although SulA homologs have not been identified in archaeal genomes, this preliminary evidence may guide future investigations aiming to explore the implication of LonB in (halo)archaeal cell division.
Biogenesis of Cell Envelope and Surface Structures
Protein Translocation and Secretion
In archaea proteins are transported into or across membranes by means of the General Secretion (Sec) pathway (unfolded substrates), or through the Twin Arginine Translocation (Tat) system (folded proteins) (Pohlschröder et al., 2005; Calo and Eichler, 2011). Even though in most organisms the Tat pathway is used to transport a minor set of proteins, in haloarchaea it is used for the majority of the secretome (Bolhuis, 2002; Rose et al., 2002). Some known Tat substrates are halolysins Nep from N. magadii (Ruiz et al., 2012) and SptA from Natrinema sp. (Du et al., 2015). Signal Peptidase I (SPI) cleaves the type I signal peptide of the majority of preproteins transported through these pathways (Paetzel et al., 2002; Pohlschröder et al., 2005; Sargent et al., 2006). Haloferax volcanii has two SPIs with catalytic activity, Sec11a (HVO_2603) and Sec11b (HVO_0002) (Fine et al., 2006), from which only Sec11b is essential for H. volcanii viability.
In Archaea, the assembly and structure of the archaellum are similar to that of bacterial type IV pilins (Chaudhury et al., 2018). The preproteins composing these appendages are processed by a specific SP, the type IV prepilin peptidase (TFPP). Haloarchaeal genomes encode a single TFPP and contain several pilin-like genes (Szabó et al., 2007). In H. volcanii the TFPP homolog PibD (HVO_2993) is responsible for processing two archaellins and six pilins (Tripepi et al., 2010; Esquivel et al., 2013), prior to assembly of the corresponding cell surface structures which are required for a set of physiologically relevant processes such as motility, adhesion, and biofilm generation (Tripepi et al., 2010; Pohlschroder and Esquivel, 2015). To the best of our knowledge, studies on the regulation of PibD expression have not been performed, however, in a quantitative proteomics study it was observed that the concentration of this protease was decreased and its electrophoretic mobility was affected in the H. volcanii rhoII KO mutant (MIG) in comparison to the parental strain (Costa et al., 2018) (see below). In addition, PibD substrates PilA1 and FlgA1 were also affected in the rhomboid mutant, as they evidenced altered electrophoretic mobility along with accumulation at the membrane (PilA1) and a decrease in concentration in the extracellular fraction, indicative of impaired archaellum assembly (Flga1). Whether these effects result from a direct action of RhoII on PibD and/or its substrates or, alternatively, are an indirect consequence remains to be clarified.
Processing and Glycosylation of the Cell Wall (S-Layer Glycoprotein)
The cell surface of haloarchaea is surrounded by a proteinaceous 2D structure, the S-layer, which in the case of H. volcanii is composed by a sole protein, the S-layer glycoprotein (SLG). Recent studies confirmed that the SLG is arranged in a hexagonal structure tightly associated with the cell membrane and stabilized by positively charged ions (von Kügelgen et al., 2021). The SLG not only provides protection to the cell but is important for its interaction with the environment and other cells (Albers and Meyer, 2011; Klingl, 2014; Sivabalasarma et al., 2021). In H. volcanii the SLG is matured through extensive post-translational modifications (PTMs) including proteolytic processing (Abdul Halim et al., 2013), lipidation (Konrad and Eichler, 2002a) and glycosylation (Konrad and Eichler, 2002b; Eichler et al., 2013; Kaminski et al., 2013; Kandiba and Eichler, 2014; Parente et al., 2014; Kandiba et al., 2016). This glycoprotein has been used as a reporter protein to study the N-glycosylation process in archaea. In H. volcanii two glycosylation pathways have been identified having two distinct sets of enzymes denoted as Agls (archaeal glycosylation) enzymes. The AglB-dependent pathway is responsible for the biosynthesis of a pentasaccharide (glucose–glucuronic acid–galacturonic acid–methyl-glucuronic acid–manose) at positions N13, N83, N274, and N279 of the SLG (Kandiba et al., 2016) also found decorating other glycoproteins (Esquivel et al., 2016; Schulze et al., 2021). The Agl15-dependent pathway mediates the assembly of a tetrasaccharide (sulfated hexose–hexose–hexose–rhamnose) originally identified at position N498 under at low salt conditions (Kaminski et al., 2013). A recent in-depth glycoproteomics analysis identified an additional N-glycosite at N370 harboring AglB-dependent glycans. Also, positions N274 and N279 were found to be modified with AglB and Agl15-dependent glycans in control salt conditions (18% NaCl), while AglB-dependent glycosylation was also observed at N498, suggesting that Agl15-dependent N-glycosylation is not dependent on low salt conditions and that both pathways can modify the same N-glycosites (Schulze et al., 2021). A PTM at position N732 with a sulfoquinovose-containing oligosaccharide was reported even though the pathway responsible for its assembly has not been addressed (Parente et al., 2014).
Haloferax volcanii encodes two rhomboid homologs, RhoI (HVO_1474) and RhoII (HVO_0727) (Table 1). KO mutants in the gene encoding rhoII have been constructed and characterized phenotypically and at the proteome level. Haloferax volcanii cells with a deletion in rhoII produced a truncated oligosaccharide bound at N732 in the SLG, evidencing the participation of rhomboids in the regulation of protein glycosylation in this haloarchaeon (Parente et al., 2014). This hypothesis was supported by the observation that several proteins of the N-glycosylation pathway were affected in abundance and/or electrophoretic mobility in the H. volcanii mutant compared to the parental strain (Costa et al., 2018). Among these, GalE epimerase/dehydratase (HVO_1576), Agl12 (HVO_2059), Agl18 (HVO_2060), an undecaprenyl pyrophosphate synthetase (HVO_2318), and phosphohexomutase (HVO_2989) are soluble proteins, meaning that the influence of RhoII on their differential processing/stability is most likely indirect. Conversely, the membrane-associated predicted flippase Agl15 (HVO_2055), which displayed differential electrophoretic mobility, is an integral membrane protein and could be a potential endogenous substrate of RhoII. Recently, it was reported that the rhomboid protease RHBDL4 controls protein glycosylation in the endoplasmic reticulum in humans by processing the oligosaccharyl-transferase subunits releasing the products to the cytoplasm for proteasome degradation (Knopf et al., 2020). Altogether, this evidence suggests that the participation of Rhomboids as regulators of the N-glycosylation process is a conserved trait.
The proteomic analysis of the LonB mutant HVLON3 (Cerletti et al., 2015, 2018) revealed that a number of glycosyltransferases (GTs) were affected in their abundance and/or turnover depending on the cellular concentration of LonB. Specifically, HVO_0704, HVO_1529 (AglG), and HVO_2049 (Agl10) were selected as potential LonB targets as their pattern of degradation was notoriously enhanced when the cellular LonB concentration was increased (Cerletti et al., 2018). A comparative analysis of the SLG glycosylation pattern in wt vs the LonB mutant will help to clarify the participation of LonB in the regulation of this process. Based on the available evidence, it can be hypothesized that the membrane proteases RhoII and LonB are likely regulatory factors of the (SLG) N-glycosylation process in H. volcanii.
In H. volcanii insertion of the SLG into the cell surface is achieved by a novel protein anchoring mechanism that involves the proteolytic processing of a C-terminal sorting signal by the peptidase archaeosortase A (ArtA) followed by lipid attachment (Abdul Halim et al., 2013). The sorting signal recognized by ArtA is a tripartite structure containing PGF, a hydrophobic fragment (TM) and a stretch of basic residues localized at the C-terminus or in other regions of the substrate protein (Abdul Halim et al., 2015, 2017). Site directed mutagenesis studies of H. volcanii ArtA showed that the highly conserved Cys173, Arg214, and Arg253 of ArtA are critical for its catalytic activity (Abdul Halim et al., 2018). Mutants lacking the artA gene (HVO_0915) evidence poor growth, differences in cell shape and were impaired in motility and conjugation (Abdul Halim et al., 2013, 2015, 2017). Recently, it was shown that HvPssA and HvPssD, homologs of the bacterial phosphatidyl serine synthase and phosphatidyl serine decarboxylase, respectively, are involved in the maturation of ArtA substrates. This conclusion was supported by the observation that mutants lacking these genes were impaired in the proteolytic processing and/or lipid attachment and showed similar phenotypes as those displayed by ΔartA mutants. Based on co-localization experiments, it was suggested that the interaction between HvPssA, HvPss, ArtA, and SLG occurs at the midcell during growth and cytokinesis and a model was proposed in which recruitment of ArtA, HvPssA, and HvPssD to the mid cell may promote the insertion of newly matured SLG to the cell wall at midcell contributing to cell division and elongation (Abdul-Halim et al., 2020).
Expression and Potential Functions of Putative Membrane Protease Homologs
Based on proteomics studies performed in H. volcanii, various proteins related to membrane protease families were detected, including proteins related to CAAX prenyl proteases, Site 2 Proteases (S2P) and HtpX. Some of these showed differential expression depending on the environmental condition and/or in strains lacking other proteases (Rho II). This preliminary evidence suggests that these proteins (or at least some of them) may be functional enzymes in this organism.
Increased HtpX transcript levels under heat shock were detected in Pyrococcus furiosus (Shockley et al., 2003) as well as increased protein abundance of HtpX during oxidative stress in H. volcanii (McMillan et al., 2018) suggesting that, as in bacteria, HtpX protease likely participates in membrane protein quality control in archaea. Three HtpX homologs (HVO_0102, HVO_2904, and HVO_A0045) were detected in the H. volcanii proteome (Archaeal Proteome Project data base)1 (Schulze et al., 2020). Of these, HVO_A0045 showed differential expression (increased abundance) in a strain lacking the rhomboid homolog RhoII (Costa et al., 2018), suggesting some sort of stress in the membrane that may trigger the regulation of this putative HtpX protease.
Four S2P protease homologs with different structure and putative regulatory domains were detected in the H. volcanii proteome, HVO_0285 with a CBS domain (binds ATP, AMP or S-adenosyl derivatives), HVO_1870 with PDZ domain (recognizes the C-terminus of target protein) and two, HVO_1555 and HVO_1862, without an additional domain (Schulze et al., 2020). Elimination of the rhomboid protease gene encoding RhoII increased the protein abundance of HVO_1870 as well as that of the PrsW-like homolog (HVO_0408) (Costa et al., 2018). In Bacillus subtilis PrsW acts as S1P in the signaling cascade leading to degradation of an anti-sigma factor under stress conditions (Heinrich et al., 2009). Whether there is a functional connection between these proteins (PrsW/HVO_1870) in H. volcanii is an issue to be investigated in the future.
Homologs of the CAAX prenyl-protease family are present in all domains of life. In eukaryotes they participate in signaling pathways, however, their function is scarcely known in prokaryotes some members proposed in bacteriocin maturation (Pei and Grishin, 2001). The gene encoding a hypothetical protein related to the family of CAAX prenyl proteases (HVO_0784) is expressed as a transcription unit together with lonB mRNA in the haloarchaea Natrialba magadii and H. volcanii (Sastre et al., 2010; Cerletti et al., 2014). Deletion of this gene did not affect the viability and growth performance in H. volcanii cells but produced an impact on its proteome (Cerletti et al., 2015).
The current knowledge on the proposed biological functions and targets of membrane proteases in H. volcanii is depicted in Figure 1, which can be used as a working model for future research.
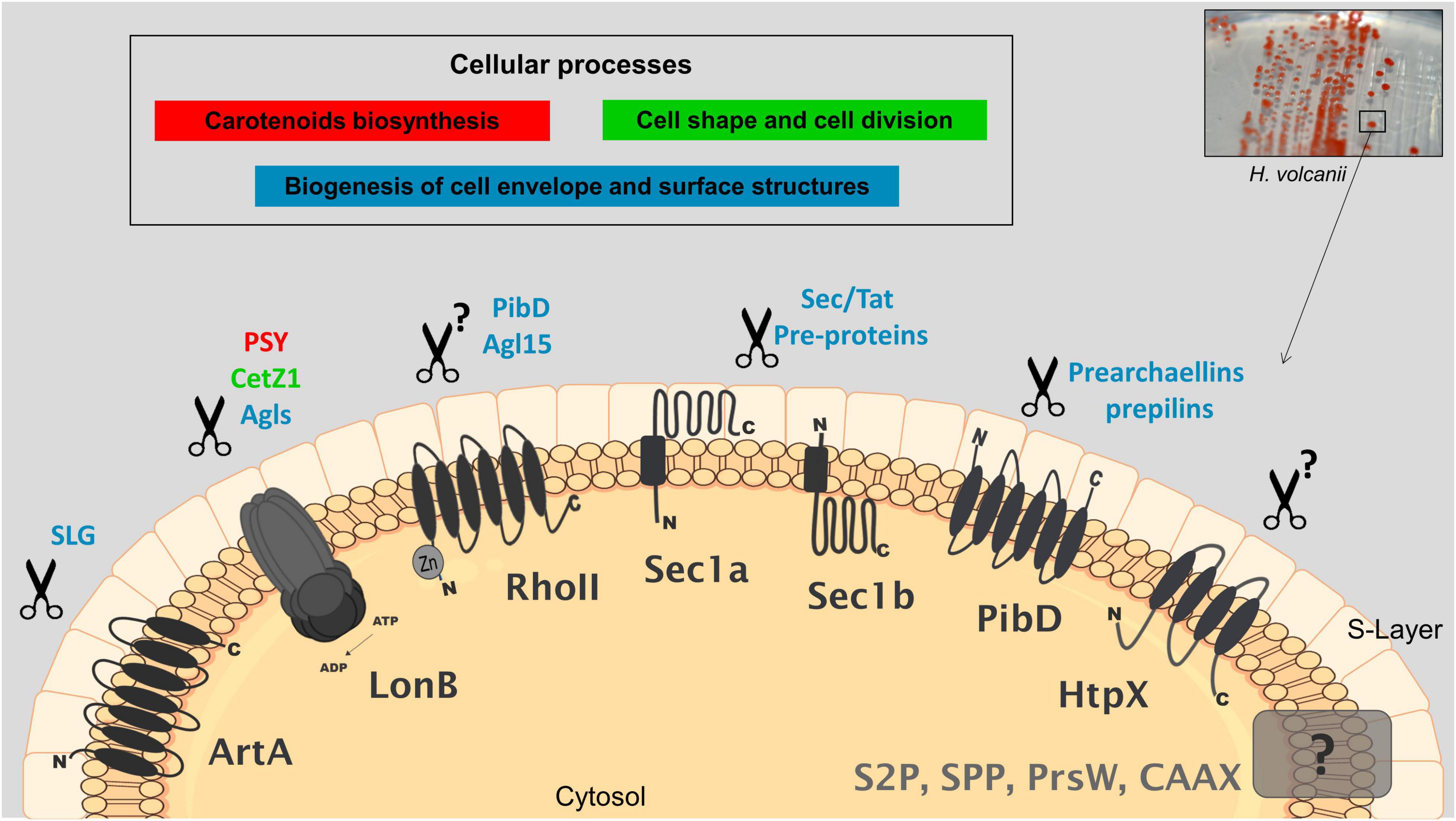
Figure 1. Cellular processes and potential targets of membrane-localized proteases in Haloferax volcanii. Proteases that have been characterized by genetic, biochemical and/or proteomics studies are shaded in black; those uncharacterized are shaded in gray; proposed biological functions and associated potential targets are indicated with the same color. Genetics combined with proteomics have shown that LonB affects the degradation of key proteins involved in carotenogenesis (PSY), cell shape determination (CetZ1) and likely the glycosylation of SLG (several GTs). Rhomboid RhoII is implicated in the biogenesis of a sulfoquinovose-containing oligosaccharide as a mutant deleted in the rhoII produces a truncated version of this oligosaccharide. PibD and Agl15 are suggested as potential targets of RhoII based on differential proteomics on wt and a mutant lacking rhoII. Anchoring of the SLG into the cell surface involves the proteolytic processing of a C-terminal sorting signal by the peptidase archaeosortase A (ArtA) followed by lipid attachment, this mechanism has been substantiated by various types of evidence. SPIs Sec11a/b and TFPP-like PibD process pre-proteins for membrane insertion, secretion and for cell surface structures. Protease homologs of HtpX, S2P, SPP, CAAX prenyl-proteases, and PrsW have been detected by differential proteomics. HtpX was suggested to participate in stress response. Additional details and the corresponding references are described in the text.
Concluding Remarks
In spite of the fact that proteolysis plays a central role in many processes that occur within the context of the membrane and cell envelope of prokaryotes, the physiological relevance, endogenous targets and processes regulated by membrane-localized proteases are scarcely known in archaea. In the last years first insights on the processes controlled by the regulatory proteases LonB and Rhomboids and some potential physiological targets have begun to emerge as well as a better understanding of the mechanism of SLG maturation mediated by archaeosortase ArtA. The participation of membrane proteases in the control of key cellular processes such as carotenogenesis may guide biotechnological developments including overproducing strains as a source of bioactive compounds with novel properties.
Still, this is an area open to research and studies are encouraged to contribute to extend our fundamental knowledge on proteases, archaeal physiology as well as aid in the design of biotechnological applications of these “unusual” organisms and their biomolecules.
Author Contributions
All authors made substantial contributions to the acquisition, analysis and interpretation of data for this review, critically reviewed and edited the manuscript, approved the final version before submission to publication, and agreed to be accountable for all aspects of the work in ensuring that questions related to the accuracy or integrity of any part of the work are appropriately investigated and resolved.
Funding
This work was supported by grants from the National University of Mar del Plata (EXA934/19, UNMdP), the National Council of Scientific and Technological Research (PIP2053-21, CONICET) and the National Agency of Promotion of Science and Technology (PICT2018-2228, ANPCyT), Argentina.
Conflict of Interest
The authors declare that the research was conducted in the absence of any commercial or financial relationships that could be construed as a potential conflict of interest.
Publisher’s Note
All claims expressed in this article are solely those of the authors and do not necessarily represent those of their affiliated organizations, or those of the publisher, the editors and the reviewers. Any product that may be evaluated in this article, or claim that may be made by its manufacturer, is not guaranteed or endorsed by the publisher.
Footnotes
References
Abdul Halim, M. F., Karch, K. R., Zhou, Y., Haft, D. H., Garcia, B. A., and Pohlschroder, M. (2015). Permuting the PGF signature motif blocks both archaeosortase-dependent C-terminal cleavage and prenyl lipid attachment for the Haloferax volcanii S-layer glycoprotein. J. Bacteriol. 198, 808–815. doi: 10.1128/JB.00849-15
Abdul Halim, M. F., Pfeiffer, F., Zou, J., Frisch, A., Haft, D., Wu, S., et al. (2013). Haloferax volcanii archaeosortase is required for motility, mating, and C-terminal processing of the S-layer glycoprotein. Mol. Microbiol. 88, 1164–1175. doi: 10.1111/mmi.12248
Abdul Halim, M. F., Rodriguez, R., Stoltzfus, J. D., Duggin, I. G., and Pohlschroder, M. (2018). Conserved residues are critical for Haloferax volcanii archaeosortase catalytic activity: implications for convergent evolution of the catalytic mechanisms of non-homologous sortases from archaea and bacteria. Mol. Microbiol. 108, 276–287. doi: 10.1111/mmi.13935
Abdul Halim, M. F., Stoltzfus, J. D., Schulze, S., Hippler, M., and Pohlschroder, M. (2017). ArtA-dependent processing of a Tat substrate containing a conserved tripartite structure that is not localized at the C terminus. J. Bacteriol. 199, e00802–16. doi: 10.1128/JB.00802-16
Abdul-Halim, M. F., Schulze, S., DiLucido, A., Pfeiffer, F., Bisson Filho, A. W., and Pohlschroder, M. (2020). Lipid anchoring of archaeosortase substrates and midcell growth in haloarchaea. MBio 11, e00349–20. doi: 10.1128/mBio.00349-20
Albers, S. V., and Meyer, B. H. (2011). The archaeal cell envelope. Nat. Rev. Microbiol. 9, 414–426. doi: 10.1038/nrmicro2576
Began, J., Cordier, B., Březinová, J., Delisle, J., Hexnerová, R., Srb, P., et al. (2020). Rhomboid intramembrane protease YqgP licenses bacterial membrane protein quality control as adaptor of FtsH AAA protease. EMBO J. 39:e102935. doi: 10.15252/embj.2019102935
Bolhuis, A. (2002). Protein transport in the halophilic archaeon Halobacterium sp. NRC-1: a major role for the twin-35 arginine translocation pathway? Microbiol 148(11), 3335–3346. doi: 10.1099/00221287-148-11-3335
Calo, D., and Eichler, J. (2011). Crossing the membrane in Archaea, the third domain of life. Biochimica. Et. Biophysica. Acta Biomembr. 1808, 885–891. doi: 10.1016/j.bbamem.2010.03.020
Cerletti, M., Martinez, M. J., Gimenez, M. I., Sastre, D. E., Paggi, R. A., and De Castro, R. E. (2014). The LonB protease controls membrane lipids composition and is essential for viability in the extremophilic haloarchaeon Haloferax volcanii. Environ. Microbiol. 16, 1779–1792. doi: 10.1111/1462-2920.12385
Cerletti, M., Paggi, R., Troetschel, C., Ferrari, M., Guevara, C., Albaum, S., et al. (2018). LonB protease is a novel regulator of carotenogenesis controlling degradation of phytoene synthase in Haloferax volcanii. J. Proteome. Res. 17, 1158–1171. doi: 10.1021/acs.jproteome.7b00809
Cerletti, M., Paggi, R. A., Guevara, C. R., Poetsch, A., and De Castro, R. E. (2015). Global role of the membrane protease LonB in Archaea: potential protease targets revealed by quantitative proteome analysis of a lonB mutant in Haloferax volcanii. J. Proteomics 121, 1–14. doi: 10.1016/j.jprot.2015.03.016
Chaudhury, P., Quax, T. E., and Albers, S. V. (2018). Versatile cell surface structures of archaea. Mol. Microbiol. 107, 298–311. doi: 10.1111/mmi.13889
Costa, M. I., Cerletti, M., Paggi, R. A., Trötschel, C., De Castro, R., Poetsch, A., et al. (2018). Haloferax volcanii proteome response to deletion of a rhomboid protease gene. J Proteome Res. 17, 961–977 doi: 10.1021/acs.jproteome.7b00530
Costa, M. I., Álvarez Cerimedo, M. S., Urquiza, D., Ayude, M. A., Hoppe, C. E., Fasce, D. P., et al. (2020). Synthesis, characterization and kinetic study of silver and gold nanoparticles produced by the archaeon Haloferax volcanii. J. Appl. Microbiol. 129, 1297–1308. doi: 10.1111/jam.14726
De Castro, R. E., Maupin-Furlow, J. A., Gimenez, M. I., Herrera Seitz, M. K., and Sanchez, J. J. (2006). Haloarchaeal proteases and proteolytic systems. FEMS Microbiol. Rev. 30, 17–35. doi: 10.1111/j.1574-6976.2005.00003.x
Du, X., Li, M., Tang, W., Zhang, Y., Zhang, L., Wang, J., et al. (2015). Secretion of Tat-dependent halolysin SptA capable of autocatalytic activation and its relation to haloarchaeal growth. Mol. Microbiol. 96, 548–565. doi: 10.1111/mmi.12955
Duggin, I. G., Aylett, C. H., Walsh, J. C., Michie, K. A., Wang, Q., Turnbull, L., et al. (2015). CetZ tubulin-like proteins control archaeal cell shape. Nature 519, 362–365. doi: 10.1038/nature13983
Eichler, J., Arbiv, A., Cohen-Rosenzweig, C., Kaminski, L., Kandiba, L., and Konrad, Z. (2013). N-glycosylation in Haloferax volcanii: adjusting the sweetness. Front. Microbiol. 4:403. doi: 10.3389/fmicb.2013.00403
Esquivel, R. N., Schulze, S., Xu, R., Hippler, M., and Pohlschroder, M. (2016). Identification of Haloferax volcanii pilin N-glycans with diverse roles in pilus biosynthesis, adhesion, and microcolony formation. J. Biol. Chem. 291, 10602–10614. doi: 10.1074/jbc.M115.693556
Esquivel, R. N., Xu, R., and Pohlschroder, M. (2013). Novel archaeal adhesion pilins with a conserved N terminus. J. Bacteriol. 195, 3808–3818. doi: 10.1128/JB.00572-13
Feng, L., Yan, H., Wu, Z., Yan, N., Wang, Z., Jeffrey, P. D., et al. (2007). Structure of a site-2 protease family intramembrane metalloprotease. Science 318, 1608–1612. doi: 10.1126/science.1150755
Ferrari, M. C., Cerletti, M., Paggi, R. A., Troetschel, C., Poetsch, A., and De Castro, R. E. (2020). The LonB protease modulates the degradation of CetZ1 involved in rod-shape determination in Haloferax volcanii. J. Proteom. 211:103546. doi: 10.1016/j.jprot.2019.103546
Fine, A., Irihimovitch, V., Dahan, I., Konrad, Z., and Eichler, J. (2006). Cloning, expression, and purification of functional Sec11a and Sec11b, type I signal peptidases of the archaeon Haloferax volcanii. J. Bacteriol. 188, 1911–1919. doi: 10.1128/JB.188.5.1911-1919.2006
Fink-Lavi, E., and Eichler, J. (2008). Identification of residues essential for the catalytic activity of Sec11b, one of the two type I signal peptidases of Haloferax volcanii. FEMS Microbiol. Lett. 278, 257–260. doi: 10.1111/j.1574-6968.2007.01000.x
Giani, M., Garbayo, I., Vílchez, C., and Martínez-Espinosa, R. M. (2019). Haloarchaeal carotenoids: healthy novel compounds from extreme environments. Mar. drugs 17:524. doi: 10.3390/md17090524
Gimenez, M. I., Cerletti, M., and De Castro, R. E. (2015). Archaeal membrane-associated proteases: insights on Haloferax volcanii and other haloarchaea. Front. Microbiol. 6:39. doi: 10.3389/fmicb.2015.00039
Haft, D. H., Payne, S. H., and Selengut, J. D. (2012). Archaeosortases and exosortases are widely distributed systems linking membrane transit with posttranslational modification. J. Bacteriol. 194, 36–48. doi: 10.1128/JB.06026-11
Heinrich, J., Hein, K., and Wiegert, T. (2009). Two proteolytic modules are involved in regulated intramembrane proteolysis of Bacillus subtilis RsiW. Mol. Microbiol. 74, 1412–1426. doi: 10.1111/j.1365-2958.2009.06940.x
Kaminski, L., Guan, Z., Yurist-Doutsch, S., and Eichler, J. (2013). Two distinct N-glycosylation pathways process the Haloferax volcanii S-layer glycoprotein upon changes in environmental salinity. MBio 4, e00716–13. doi: 10.1128/mBio.00716-13
Kandiba, L., and Eichler, J. (2014). Archaeal S-layer glycoproteins: post-translational modification in the face of extremes. Front. Microbiol. 5:661. doi: 10.3389/fmicb.2014.00661
Kandiba, L., Lin, C.-W., Aebi, M., Eichler, J., and Guerardel, Y. (2016). Structural characterization of the N-linked pentasaccharide decorating glycoproteins of the halophilic archaeon Haloferax volcanii. Glycobiology 26, 745–756. doi: 10.1093/glycob/cww014
Klingl, A. (2014). S-layer and cytoplasmic membrane–exceptions from the typical archaeal cell wall with a focus on double membranes. Front. Microbiol. 5:624. doi: 10.3389/fmicb.2014.00624
Knopf, J. D., Landscheidt, N., Pegg, C. L., Schulz, B. L., Kühnle, N., Chao, C.-W., et al. (2020). Intramembrane protease RHBDL4 cleaves oligosaccharyltransferase subunits to target them for ER-associated degradation. J. Cell Sci. 133:jcs243790. doi: 10.1242/jcs.243790
Konrad, Z., and Eichler, J. (2002a). Lipid modification of proteins in Archaea: attachment of a mevalonic acid-based lipid moiety to the surface-layer glycoprotein of Haloferax volcanii follows protein translocation. Biochem. J. 366, 959–964. doi: 10.1042/BJ20020757
Konrad, Z., and Eichler, J. (2002b). Protein glycosylation in Haloferax volcanii: partial characterization of a 98-kDa glycoprotein. FEMS Microbiol. Lett. 209, 197–202. doi: 10.1111/j.1574-6968.2002.tb11131.x
Li, X., Dang, S., Yan, C., Gong, X., Wang, J., and Shi, Y. (2013). Structure of a presenilin family intramembrane aspartate protease. Nature 493, 56–61. doi: 10.1038/nature11801
Liao, Y., Ithurbide, S., Evenhuis, C., Löwe, J., and Duggin, I. G. (2021). Cell division in the archaeon Haloferax volcanii relies on two FtsZ proteins with distinct functions in division ring assembly and constriction. Nat. Microbiol. 6, 594–605. doi: 10.1038/s41564-021-00894-z
Liu, G., Beaton, S. E., Grieve, A. G., Evans, R., Rogers, M., Strisovsky, K., et al. (2020). Bacterial rhomboid proteases mediate quality control of orphan membrane proteins. EMBO J. 39:e102922. doi: 10.15252/embj.2019102922
Manolaridis, I., Kulkarni, K., Dodd, R. B., Ogasawara, S., Zhang, Z., Bineva, G., et al. (2013). Mechanism of farnesylated CAAX protein processing by the intramembrane protease Rce1. Nature 504, 301–305. doi: 10.1038/nature12754
Maupin-Furlow, J. A. (2018). Proteolytic systems of archaea: slicing, dicing, and mincing in the extreme. Emerg. Top. Life Sci. 2, 561–580. doi: 10.1042/ETLS20180025
Maupin-Furlow, J. A., Gil, M. A., Humbard, M. A., Kirkland, P. A., Li, W., Reuter, C. J., et al. (2005). Archaeal proteasomes and other regulatory proteases. Curr. Opin. Microbiol. 8, 720–728. doi: 10.1016/j.mib.2005.10.005
McMillan, L. J., Hwang, S., Farah, R. E., Koh, J., Chen, S., and Maupin-Furlow, J. A. (2018). Multiplex quantitative SILAC for analysis of archaeal proteomes: a case study of oxidative stress responses. Environ. Microbiol. 20, 385–401. doi: 10.1111/1462-2920.14014
Mentrup, T., Cabrera-Cabrera, F., Fluhrer, R., and Schröder, B. (2020). Physiological functions of SPP/SPPL intramembrane proteases. Cell Mol. Life Sci. 77, 2959–2979. doi: 10.1007/s00018-020-03470-6
Ng, S. Y., Chaban, B., VanDyke, D. J., and Jarrell, K. F. (2007). Archaeal signal peptidases. Microbiology 153, 305–314. doi: 10.1099/mic.0.2006/003087-0
Paetzel, M., Karla, A., Strynadka, N. C., and Dalbey, R. E. (2002). Signal peptidases. Chem. Rev. 102, 4549–4580. doi: 10.1021/cr010166y
Parente, J., Casabuono, A., Ferrari, M. C., Paggi, R. A., De Castro, R. E., Couto, A. S., et al. (2014). A rhomboid protease gene deletion affects a novel oligosaccharide N-linked to the S-layer glycoprotein of Haloferax volcanii. J. Biol. Chem. 289, 11304–11317. doi: 10.1074/jbc.M113.546531
Peck, R. F., Graham, S. M., and Gregory, A. M. (2019). Species widely distributed in halophilic archaea exhibit opsin-mediated inhibition of bacterioruberin biosynthesis. J. Bacteriol. 201, e00576–18. doi: 10.1128/JB.00576-18
Peck, R. F., Pleşa, A. M., Graham, S. M., Angelini, D. R., and Shaw, E. L. (2017). Opsin-mediated inhibition of bacterioruberin synthesis in halophilic archaea. J. Bacteriol. 199, e00303–17. doi: 10.1128/JB.00303-17
Pei, J., and Grishin, N. V. (2001). Type II CAAX prenyl endopeptidases belong to a novel superfamily of putative membrane-bound metalloproteases. Trends Biochem. Sci. 26, 275–277. doi: 10.1016/S0968-0004(01)01813-8
Pohlschroder, M., and Esquivel, R. N. (2015). Archaeal type IV pili and their involvement in biofilm formation. Front. Microbiol. 6:190. doi: 10.3389/fmicb.2015.00190
Pohlschröder, M., Giménez, M. I., and Jarrell, K. F. (2005). Protein transport in Archaea: sec and twin arginine translocation pathways. Curr. Opin. Microbiol. 8, 713–719. doi: 10.1016/j.mib.2005.10.006
Pohlschroder, M., Pfeiffer, F., Schulze, S., and Halim, M. F. A. (2018). Archaeal cell surface biogenesis. FEMS Microbiol. Rev. 42, 694–717. doi: 10.1093/femsre/fuy027
Raut, P., Glass, J. B., and Lieberman, R. L. (2021). Archaeal roots of intramembrane aspartyl protease siblings signal peptide peptidase and presenilin. Proteins 89, 232–241. doi: 10.1002/prot.26009
Rawson, R. B. (2013). The site-2 protease. Biochim. Biophys. Acta Biomembr. 1828, 2801–2807. doi: 10.1016/j.bbamem.2013.03.031
Rodrigo-Baños, M., Garbayo, I., Vilchez, C., Bonete, M. J., and Martinez-Espinosa, R. M. (2015). Carotenoids from Haloarchaea and Their Potential in Biotechnology. Mar. Drugs 13, 5508–5532. doi: 10.3390/md13095508
Rotanova, T. V., Botos, I., Melnikov, E. E., Rasulova, F., Gustchina, A., Maurizi, M. R., et al. (2006). Slicing a protease: structural features of the ATP-dependent Lon proteases gleaned from investigations of isolated domains. Protein. Sci. 15, 1815–1828. doi: 10.1110/ps.052069306
Rose, R. W., Brüser, T., Kissinger, J. C., and Pohlschröder, M. (2002). Adaptation of protein secretion to extremely high-salt conditions by extensive use of the twin-arginine translocation pathway. Mol. Microbiol. 45, 943–950. doi: 10.1046/j.1365-2958.2002.03090.x
Ruiz, D. M., Paggi, R. A., Gimenez, M. I., and De Castro, R. E. (2012). Autocatalytic maturation of the Tat-dependent halophilic subtilase Nep produced by the archaeon Natrialba magadii. J. Bacteriol. 194, 3700–3707. doi: 10.1128/JB.06792-11
Sargent, F., Berks, B. C., and Palmer, T. (2006). Pathfinders and trailblazers: a prokaryotic targeting system for transport of folded proteins. FEMS Microbiol. Lett. 254, 198–207. doi: 10.1111/j.1574-6968.2005.00049.x
Sastre, D. E., Paggi, R. A., and De Castro, R. E. (2010). The Lon protease from the haloalkaliphilic archaeon Natrialba magadii is transcriptionally linked to a cluster of putative membrane proteases and displays DNA-binding activity. Microbiol. Res. 166, 304–313. doi: 10.1016/j.micres.2010.07.002
Schacherl, M., Gompert, M., Pardon, E., Lamkemeyer, T., Steyaert, J., and Baumann, U. (2017). Crystallographic and biochemical characterization of the dimeric architecture of site-2 protease. Biochimica. Et. Biophysica. Acta Biomembr. 1859, 1859–1871. doi: 10.1016/j.bbamem.2017.05.006
Schulze, S., Adams, Z., Cerletti, M., De Castro, R., Ferreira-Cerca, S., Fufezan, C., et al. (2020). The Archaeal Proteome Project advances knowledge about archaeal cell biology through comprehensive proteomics. Nat. Comm. 11:3145. doi: 10.1038/s41467-020-16784-7
Schulze, S., Pfeiffer, F., Garcia, B. A., and Pohlschroder, M. (2021). Comprehensive glycoproteomics shines new light on the complexity and extent of glycosylation in archaea. PLoS Biol. 19:e3001277. doi: 10.1371/journal.pbio.3001277
Shockley, K. R., Ward, D. E., Chhabra, S. R., Conners, S. B., Montero, C. I., and Kelly, R. M. (2003). Heat shock response by the hyperthermophilic archaeon Pyrococcus furiosus. Appl. Environ. Microbiol. 69, 2365–2371. doi: 10.1128/AEM.69.4.2365-2371.2003
Sivabalasarma, S., Wetzel, H., Nußbaum, P., Van der Does, C., Beeby, M., and Albers, S.-V. (2021). Analysis of Cell–Cell Bridges in Haloferax volcanii Using Electron Cryo-Tomography Reveal a Continuous Cytoplasm and S-Layer. Front Microbiol. 11:612239. doi: 10.3389/fmicb.2020.612239
Stanley, L., and Yuan, Y.-W. (2019). Transcriptional Regulation of Carotenoid Biosynthesis in Plants: so Many Regulators, So Little Consensus. Front. Plant Sci. 10:1017. doi: 10.3389/fpls.2019.01017
Stevenson, L. G., Strisovsky, K., Clemmer, K. M., Bhatt, S., Freeman, M., and Rather, P. N. (2007). Rhomboid protease AarA mediates quorum-sensing in Providencia stuartii by activating TatA of the twin-arginine translocase. Proc. Natl. Acad. Sci. U.S.A. 104, 1003–1008. doi: 10.1073/pnas.0608140104
Surmann, K., Ćudić, E., Hammer, E., and Hunke, S. (2016). Molecular and proteome analyses highlight the importance of the Cpx envelope stress system for acid stress and cell wall stability in Escherichia coli. MicrobiologyOpen 5, 582–596. doi: 10.1002/mbo3.353
Szabó, Z., Stahl, A. O., Albers, S.-V., Kissinger, J. C., Driessen, A. J., and Pohlschroder, M. (2007). Identification of diverse archaeal proteins with class III signal peptides cleaved by distinct archaeal prepilin peptidases. J. Bacteriol. 189, 772–778. doi: 10.1128/JB.01547-06
Tarasov, V. Y., Besir, H., Schwaiger, R., Klee, K., Furtwangler, K., Pfeiffer, F., et al. (2008). A small protein from the bop-brp intergenic region of Halobacterium salinarum contains a zinc finger motif and regulates bop and crtB1 transcription. Mol. Microbiol. 67, 772–780. doi: 10.1111/j.1365-2958.2007.06081.x
Toledo-Ortiz, G., Huq, E., and Rodriguez-Concepcion, M. (2010). Direct regulation of phytoene synthase gene expression and carotenoid biosynthesis by phytochrome-interacting factors. Proc. Natl. Acad. Sci. U.S.A. 107, 11626–11631. doi: 10.1073/pnas.0914428107
Tripepi, M., Imam, S., and Pohlschröder, M. (2010). Haloferax volcanii flagella are required for motility but are not involved in PibD-dependent surface adhesion. J. Bacteriol. 192, 3093–3102. doi: 10.1128/JB.00133-10
Vickerman, M., Mather, N., Minick, P., and Edwards, C. (2002). Initial characterization of the Streptococcus gordonii htpX gene. Oral Microbiol. Immunol. 17, 22–31. doi: 10.1046/j.0902-0055.2001.00000.x
von Kügelgen, A., Alva, V., and Bharat, T. A. (2021). Complete atomic structure of a native archaeal cell surface. Cell rep. 37:110052. doi: 10.1016/j.celrep.2021.110052
Wang, P., Wang, Y., Wang, W., Chen, T., Tian, S., and Qin, G. (2020). Ubiquitination of phytoene synthase 1 precursor modulates carotenoid biosynthesis in tomato. Comm. Biol. 3:730. doi: 10.1038/s42003-020-01474-3
Keywords: archaea, Haloferax volcanii, membrane proteases, protease targets, archaeal physiology, proteolysis
Citation: De Castro RE, Giménez MI, Cerletti M, Paggi RA and Costa MI (2022) Proteolysis at the Archaeal Membrane: Advances on the Biological Function and Natural Targets of Membrane-Localized Proteases in Haloferax volcanii. Front. Microbiol. 13:940865. doi: 10.3389/fmicb.2022.940865
Received: 10 May 2022; Accepted: 06 June 2022;
Published: 24 June 2022.
Edited by:
Nicole Buan, University of Nebraska-Lincoln, United StatesReviewed by:
Rosa María Martínez-Espinosa, University of Alicante, SpainXian Fu, Beijing Genomics Institute (BGI), China
Copyright © 2022 De Castro, Giménez, Cerletti, Paggi and Costa. This is an open-access article distributed under the terms of the Creative Commons Attribution License (CC BY). The use, distribution or reproduction in other forums is permitted, provided the original author(s) and the copyright owner(s) are credited and that the original publication in this journal is cited, in accordance with accepted academic practice. No use, distribution or reproduction is permitted which does not comply with these terms.
*Correspondence: Rosana E. De Castro, ZGVjYXN0cm9AbWRwLmVkdS5hcg==; María I. Giménez, bWlnaW1lbkBtZHAuZWR1LmFy
†These authors have contributed equally to this work