- 1State Key Laboratory of Oral Diseases, National Clinical Research Center for Oral Diseases, West China Hospital of Stomatology, Sichuan University, Chengdu, China
- 2Department of Cariology and Endodontics, West China Hospital of Stomatology, Sichuan University, Chengdu, China
Corynebacterium matruchotii is a reported calcifying bacterium that can usually be isolated from dental calculus and induce mineralization in vitro. In recent years, based on in situ hybridization probe and sequencing technology, researchers have discovered the central “pillar” role of C. matruchotii in supragingival plaque, and many studies focused on bacterial interactions in the biofilm structure dominated by C. matruchotii have been conducted. Besides, C. matruchotii seems to be an indicator of “caries-free” oral status according to imaging and sequencing studies. Therefore, in this review, we summarize C. matruchotii ‘s role in supragingival plaque based on the structure, interactions, and potential connections with oral diseases.
Introduction
Corynebacterium matruchotii (previously known as Leptothrix buccalis and Bacterionema matruchotii) is a gram-positive aerobic bacterium that was first discovered in 1925 (Ennever and Creamer, 1967). Of all the anatomical sites in the oral cavity, it occurs most in dental plaque on the tooth surface and serves as one of the most predominant bacteria at the site (Mark Welch et al., 2016; Eriksson et al., 2017; Esberg et al., 2020). During the 1960's−2000's, the bacterium was verified to precipitate calcium phosphate on the intracytoplasmic membranes and share a similar calcified nucleator with dental calculus (Vogel and Smith, 1976; Boyan-Salyers et al., 1978; Ennever et al., 1978, 1979; Sidaway, 1978a). However, after these investigations reported this calcifying ability, studies on C. matruchotii in the oral ecosystem were strikingly absent from the literature for the next decade or so.
In the past few years, with the development of laboratory techniques such as fluorescence in situ hybridization with probes and high-throughput sequencing, the taxonomy of bacteria in highly structured dental plaque has been clearly depicted, and C. matruchotii has once again become a focus of research. As reported recently, C. matruchotii is in the center of supragingival plaque and interacts with a variety of bacteria around it via its special morphology, which may indicate a new sight for plaque development and maturation. In addition, several studies on oral diseases associated with supragingival plaque have pointed out C. matruchotii as a potential indicator of healthy status since there is an increase in the relative abundance of C. matruchotii compared with the morbid state, implying C. matruchotii might play more hidden roles in the development of different oral status.
This review is aimed at summarizing the latest findings of C. matruchotii in supragingival plaque, including its bio-geographic position in the organized oral biofilm, interactions with other bacteria, and possible effects in oral diseases, attempting to better understand the characteristics of dental plaque in various conditions to explore ways to maintain oral health from a microbial perspective.
C. matruchotii in the Structure of Supragingival Plaque
C. matruchotii was discovered in much higher abundance and prevalence in the supragingival plaque and subgingival plaque than in saliva or other oral surfaces such as the tongue, buccal mucosa, and keratinized gingivae (Mark Welch et al., 2016). Therefore, the potential big role for it in oral biofilms came to attention. In 2016, Borisy et al. used a fluorescence in situ hybridization probe to determine the bio-geographic location of each bacterium and first clearly showed the central position of C. matruchotii in the hedgehog structures in healthy people's supragingival plaque (Mark Welch et al., 2016; Borisy and Valm, 2021). The “hedgehog” structure is a special consortium of various bacteria named by its morphology seen under electron microscopy as spiny, radially oriented filaments, and within the structure, C. matruchotii stood in the center of the field of vision with a variety of bacteria surrounding it. Combined with a recent metaproteome study claiming that C. matruchotii represented a large proportion of the protein activity in supragingival dental plaque, which suggested its strong biological activity and metabolic capacity (Belda-Ferre et al., 2015), a hypothesis can be drawn that it undertakes numerous information exchanges among bacteria and performs important functions in healthy oral status. Additionally, based on the recent research conducted on the metabolic current production by C. matruchotii, the radial “hedgehog” structure may support electrically coupled organics oxidation and oxygen reduction in dental biofilm as in the cases of long-range extracellular electron transport which could facilitate colonization of various bacteria in the anaerobic environment (Naradasu et al., 2020). From the basal layer closest to the tooth surface outward, Corynebacterium traversed through the whole distance; from the staining, an ordered arrangement could be seen in the form of a small amount of Actinomyces located near the tooth base, Capnocytophaga/ Fusobacterium/ Leptotrichia forming a ring between the periphery and the base, Neisseriaceae clustering in and near the periphery, and Streptococcus/ Haemophilus/ Porphyromonas being located at the periphery encircling Corynebacterium as the “corncob structure.” The organized “hedgehog” structure was determined by the metabolism and biochemicals of different bacteria according to the group supposed, and Streptococcus seemed to be the major driver of this organized structure which used external oxygen to produce hydrogen peroxide, carbon dioxide, and a series of metabolites to impact growth and survival adaption of other bacteria.
The Interactions Between C. matruchotii and Other Commensal Bacteria Close in Bio-Geography to Supragingival Plaque
The development and maturation of oral biofilm were determined by the relationship between participating bacteria, which presented as bacterial co-aggregation and co-adhesion (Kolenbrander et al., 2010). Recently, to further explore a vital part of C. matruchotii in biofilm, Anders Esberg et al. systematically performed an in vivo and in vitro study to seek bacterial interactions in coaggregation and co-adhesion assays (Esberg et al., 2020). Consistent with the fact that C. matruchotii were in close proximity with Actinomyces spp reported by Borisy, Esberg et al. demonstrated an indispensable role of Actinomyces spp for C. matruchotii binding on the tooth surface. The group hypothesized that Actinomyces naeslundii could be an initial tooth colonizer binding to the saliva pellicle and providing attachment sites for C. matruchotii since in vivo the abundance of A. naeslundii was similar to C. matruchotii at all ages, and in vitro, A.naeslundii bound C.matruchotii both in the state of plankton and biofilm and had the ability to recruit C. matruchotii to the tooth surface.
In the “hedgehog” consortium of supragingival plaque, a unique structure named “corncob” could be seen at the periphery of supragingival plaque away from the enamel surface (Mark Welch et al., 2016), which was formed at the very beginning of the acquired pellicle and closest to the outside air, which determined a different metabolism to the inside bacteria (Rickard et al., 2003). This local, specific structure was made of the central filament C. matruchotii encased by cocci Streptococcus, which could not be formed around other filamentous bacteria nearby such as Fusobacterium, Leptotrichia, or Capnocytophaga, revealing a particularly close relationship between C. matruchotii and Streptococcus. Previous studies showed that the species belonging to Streptococcus involved in corncob structure included Streptococcus sanguis and Streptococcus cristatus (Listgarten et al., 1973; Takazoe et al., 1978). However, the results claimed by Anders Esberg et al. only verified the co-aggregation and co-adhesion between C. matruchotii and Streptococcus cristatus, which may imply a connection that did not result from directly binding specific components to the respective cell surfaces. Puthayalai Treerat et al. co-cultured Corynebacterium and S. sanguinis in transwell culture plates that prevented direct contact between bacteria and found out free fatty acid transported by membrane vesicles of Corynebacterium could elongate chains and promote fitness of S. sanguinis (Treerat et al., 2020). The free fatty acids mediated by membrane vesicles as potential signaling molecules to impact Streptococcus and participate in biological activity without direct contact were also reported in other Corynebacterium (Boyan et al., 1984; Bomar et al., 2016), which implied a specific role of membrane vesicles enveloping fatty acids.
Besides, around the “corncob” region, an interesting manifestation could be observed: only when Streptococcus bound to Corynebacterium did Haemophilus/Aggregatibacter appear next to Corynebacterium (Mark Welch et al., 2016). Recently, a group verified the co-occurrence between Haemophilus parainfluenzae and Streptococcus mitis and assumed their proximity may be attributed to H.parainfluenzae's dependence on NAD produced by S. mitis (Perera et al., 2022). Though many streptococci shared the common ability to generate hydrogen peroxide (H2O2), which inhibited other bacteria's growth, H.parainfluenzae seemed to evolve a hydrogen peroxide redundant system on account of frequent co-occurrence with Streptococcus (Kreth et al., 2009; Perera et al., 2022). Thus, from the local “corncob” region, it can be confirmed that behind the organized architecture of supragingival plaque, bacteria do have functional connections that match spatial structures, and there may be a strict temporal order for them to be involved. Additionally, C. matruchotii, as the predominant bacteria of Corynebacterium in the supragingival plaque, probably plays a big part in the beginning in shaping the biofilm structure.
C. matruchotii in Oral Diseases Associated With Supragingival Plaque
C. matruchotii and Caries
Dental caries remains one of the most prevalent chronic diseases in both children and adults, and it is shown to be a result of the demineralization of tooth enamel by acid corrosion (Heng, 2016). Streptococcus mutans is the primary pathogen of dental caries, and based on its strong ability of acid production and acid resistance, along with its possession of glycotransferase, it can use dietary sugar to produce insoluble glucans to form oral biofilm and generate acid to decalcify dental enamel and cause caries (Johansson et al., 2016). Recently, with the popularity of sequencing technology in clinical practice, the role of C. matruchotii seems to act as an opposite to S. mutans and tends to be considered as a marker of a caries-free state, which is depicted in Figure 1.
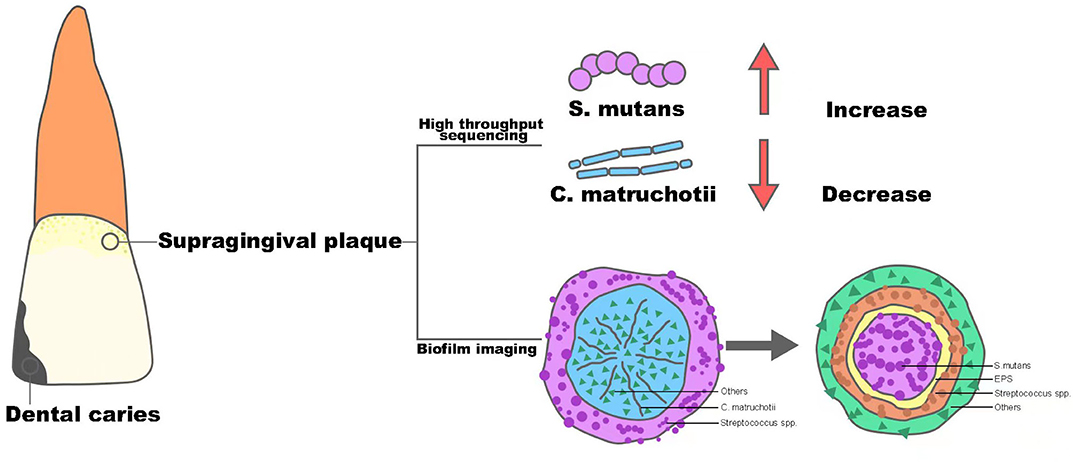
Figure 1. The structure alteration of supragingival plaque from healthy oral status to dental caries.
Muawia A Qudeimat et al. collected supragingival plaque samples from 64 caries-active and 64 caries-free middle eastern children and performed 16S rRNA sequencing (Qudeimat et al., 2021), and their results showed that C. matruchotii was relatively more abundant in the caries-free group. This result was consistent with the research conducted by Nezar Noor Al-Hebshi et al. using metagenome sequencing (Al-Hebshi et al., 2019). Based on the abundance alteration in different situations, these findings implied that C. matruchotii may own protective property and could be a representative species for caries-free status. At the same time, in all these studies, S. mutans showed a predominant abundance in the caries group and with the progression of caries, the abundance of S.mutans became higher, while the abundance of C. matruchotii decreased gradually (Gross et al., 2010). The consequence seemed to show a reciprocal relationship between S. mutans and C. matruchotii in microecology of supragingival plaque and dental caries was the outcome of transferred superiority from C. matruchotii to S.mutans.
In 2020, Dongyeop Kim et al. discovered a 3D corona-like structure with a high frequency of detection in people with caries by fluorescence in situ hybridization in intact biofilms formed on carious teeth of toddlers; this was constructed with an inner core of S. mutans surrounded by outer layers of other bacteria linked via extracellular polysaccharide (Kim et al., 2020). The highly organized rotund-shaped architecture, on the one hand, created localized regions of acidic pH and caused acute enamel demineralization, on the other hand, built a protective barrier against antimicrobials while increasing bacterial acid fitness. Compared with the in situ biofilm imaging study in healthy people by Gary G. Borisy et.al, the detection rate of “hedgehog” centered on C. matruchotii was cut in half, however, at the same time, the dominant “rotund-shaped” S. mutans became the most commonly detected architecture in people with caries (Mark Welch et al., 2016). Thus, combined with the “pillar” role of C. matruchotii in the structure of dental plaque in healthy people, it could be inferred that C. matruchotii might perform a key part in the formation and stabilization of healthy dental plaque. In contrast, the reduction of C. matruchotii may directly lead to the break of biofilm homostasis. Meanwhile, the decreased abundance of C. matruchotii, based on the close relationship shown in corncob structure of supragingival biofilm, might also be part of the reduction of commensal streptococci, such as Streptococcus gordonii and S. sanguinis (Agnello et al., 2017; Richards et al., 2017), reported before, which showed an antagonistic effect against S. mutans via their capability of producing hydrogen peroxide (Kreth et al., 2008). The alteration could finally contribute to a replacement of dominant species in the oral ecosystem and induce the formation of diseased-adapted supragingival plaque determined by S. mutans that lead to disorders in acid-base metabolism and eventually cause the demineralization of tooth enamel. In general, C. matruchotii is a potential indicator of a caries-free state, and the reduction of its abundance may indicate the evolution from healthy plaque to caries biofilm.
C. matruchotii and Supragingival Calculus Associated With Periodontal Diseases
Supragingival calculus is the plaque and sediment that has calcified or is calcifying on the tooth surface touching or above the gingival margin, which can cause gingival inflammation and contribute to periodontal diseases. As reported before, C. matruchotii was a calcified bacterium that had the strong ability to deposit calcium and induce mineralization in vitro and may participate in the formation of supragingival calculus (Sidaway, 1978a; Takazoe and Itoyama, 1980; van Dijk et al., 1998) (Figure 2). With the superior ability to accumulate and mineralize calcium (Sidaway, 1978b), together with the fact that salivary levels of calcium increased in higher calculus formers and in periodontal diseases (Mandel, 1972; Sutej et al., 2012), C. matruchotii seemed to be an “organ” responsible for calcium metabolism in oral ecosystems analogous to Entotheonella sp in marine ecosystems in that they may prompt a similar absorption, accumulation, and mineralization process of ions (Keren et al., 2017).
The reason for supragingival calculus to promote periodontal diseases, as past scholars have highlighted, is attributed to the porous structure derived from dental calculus, which accommodated a large number of periodontitis-related pathogenic bacteria such as Porphyromonas gingivalis and their virulence factors (Socransky, 1970; Velsko et al., 2019). Recently, in addition to bacterial factors, studies showed that crystal properties or small calcium phosphate particles contained in dental calculus could directly cause periodontal inflammation as well. Experiments by Jorge Luis Montenegro Raudales et al. demonstrated that after heating dental calculus at 250°C for 1 h to eliminate residual bacteria, the treated calculus still activated NLRP3 inflammasome and promoted IL-1β secretion, which was involved in bone resorption and could also be induced by P. gingivalis (Ziauddin et al., 2018). In another study, Yu Sakai et al. found that tiny calcium phosphate particles stimulated gingival epithelial cells through the NF-kB pathway leading to a significant increase in the expression of interleukin-8 at the gene and protein levels, which could attract more neutrophils to participate in the development of periodontitis (Sakai et al., 2014). Thus, whether supragingival calculus is a deposit of pathogenic bacteria or an independent physical stimulus factor, the potentially important calculus former, C. matruchotii, seems to indirectly influence the progression of periodontal diseases.
The formation of dental calculus can be separated into three steps, formation of the acquired pellicle, plaque maturation, and plaque mineralization, and the first two are similar to the formation of dental biofilm. Inspired by recent research displaying dental calculus as the revelator of information about plenty of biological information (Weyrich et al., 2017; Jersie-Christensen et al., 2018; Willmann et al., 2018), we speculate that calculus could also be able to mirror the characteristics of dental plaque that forms it. The ability of calcification, along with the relatively high abundance and biogeography in the center of supragingival plaque, may give C. matruchotii a necessary role in calculus formation, though the transformation from dental plaque to calculus indicates alteration of plaque composition and structure (Velsko et al., 2019). Therefore, to give a more convincing conclusion about the role of C. matruchotii in supragingival calculus, studies with more samples are needed.
Conclusion and Future Perspectives
After a long period of silence, C. matruchotii has once again appeared in research as a “pillar-like” structure in supragingival plaque, studies declaring its important role in oral biofilm and close interaction with numerous resident oral bacteria. Thus, it is promising to explore cooperative or competitive relationships between C. matruchotii and other commensals for a better understanding of the mechanisms behind the unique structure. Moreover, since interactions among bacteria determine the order for different bacteria joining in the formation of plaque, dynamics studies on a timeline can help us have a greater knowledge of the formation process of supragingival plaque and discover the “keystone” to stabilize or facilitate healthy plaque. The distinctive supragingival architecture along with microbial high-throughput sequencing results between caries-active and caries-free people shows the potential of C. matruchotii as an indicator of a healthy state, so it's worthy to investigate the function of the structure dominated by C. matruchotii. Additionally, it is also intriguing to see how carious “corona” replaces the healthy “hedgehog” to seek essential external or internal factors that cause a change in the nature of plaque; this can provide more insights into the development of strategies to prevent caries, precisely targeting on specific biofilm architecture.
Dental calculus in contact with gingival epithelial can induce inflammation to some extent and be an important contributing factor to periodontal diseases. The hidden reasons for its pathogenicity lie in the pathogens stuck in it as previously demonstrated and crystalline substances as recently claimed. C. matruchotii is confirmed to have a calcified ability, and it serves a central role in supragingival plaque establishing close associations with plenty of bacteria, which endow it with a non-negligible effect in the formation of biofilm and calculus. Moreover, in subgingival plaque of patients with periodontitis, C. matruchotii was reported to over-express a large number of putative virulence factors that could have importance in the evolution of the disease (Duran-Pinedo et al., 2014). Therefore, it is interesting to conduct studies on the relationship between C. matruchotii, calculus, and periodontal diseases, which may help us better understand the role C. matruchotii plays in plaque-related gingival diseases. Moreover, combined with the latest hot reports pointing out dental calculus as a potential repository of bio-information, we make an assumption that it can also reflect the bacterial composition of dental plaque in different calculus states, which may provide some clues on whether there are certain bacteria such as C. matruchotii that determine an individual's heterogeneity in the number and rate of calculus formation.
Apart from dental caries and periodontal diseases, there has been a study conducted on the bacteria isolated from patients with apical periodontitis demonstrating that C. matruchotii was present significantly more frequently in the root canal of patients with chronic periapical periodontitis compared with patients with endo-perio lesions or pulp necrosis without obvious changes in the periapical tissue X-ray image (Korona-Glowniak et al., 2021). Apical periodontitis is a result of pulpitis, and the bacteria probably contributing to apical inflammation came from the carious lesion, which went through the apical openings of the root canals, the lateral or chamber-periodontium canals, and the pathological gingival pocket. C. matruchotii has hardly been investigated in this apical niche, and the abnormal enrichment in this place may make its role distinct from that in dental biofilm. Besides, C. matruchotii was also found a higher prevalence and a dominant part in saliva and buccal mucosa of patients with oral lichen planus, a common chronic mucocutaneous inflammatory disease (Zhong et al., 2020). To sum up, C. matruchotii may perform multiple roles in different areas of the oral cavity and thus deserves more studies in the future.
Author Contributions
QL, FZ, YL, and JL: conceptualization, methodology, and writing-original draft preparation. QL, FZ, ZS, YL, and JL: writing-review and editing. YL and JL: supervision and project administration. All authors contributed to the article and approved the submitted version.
Funding
This study was supported by the National Natural Science Foundation of China (81991500, 81991501).
Conflict of Interest
The authors declare that the research was conducted in the absence of any commercial or financial relationships that could be construed as a potential conflict of interest.
Publisher's Note
All claims expressed in this article are solely those of the authors and do not necessarily represent those of their affiliated organizations, or those of the publisher, the editors and the reviewers. Any product that may be evaluated in this article, or claim that may be made by its manufacturer, is not guaranteed or endorsed by the publisher.
References
Agnello, M., Marques, J., Cen, L., Mittermuller, B., Huang, A., Chaichanasakul Tran, N., et al. (2017). Microbiome associated with severe caries in canadian first nations children. J. Dent. Res. 96, 1378–1385. doi: 10.1177/0022034517718819
Al-Hebshi, N. N., Baraniya, D., Chen, T., Hill, J., Puri, S., Tellez, M., et al. (2019). Metagenome sequencing-based strain-level and functional characterization of supragingival microbiome associated with dental caries in children. J. Oral Microbiol. 11, 1557986. doi: 10.1080/20002297.2018.1557986
Belda-Ferre, P., Williamson, J., Simón-Soro, Á., Artacho, A., Jensen, O. N., and Mira, A. (2015). The human oral metaproteome reveals potential biomarkers for caries disease. Proteomics 15, 3497–3507. doi: 10.1002/pmic.201400600
Bomar, L., Brugger, S. D., Yost, B. H., Davies, S. S., and Lemon, K. P. (2016). Corynebacterium accolens releases antipneumococcal free fatty acids from human nostril and skin surface triacylglycerols. mBio 7, e01725–e01715. doi: 10.1128/mBio.01725-15
Borisy, G. G., and Valm, A. M. (2021). Spatial scale in analysis of the dental plaque microbiome. Periodontol 2000. 86, 97–112. doi: 10.1111/prd.12364
Boyan, B. D., Landis, W. J., Knight, J., Dereszewski, G., and Zeagler, J. (1984). Microbial hydroxyapatite formation as a model of proteolipid-dependent membrane-mediated calcification. Scan Electron Microsc. 1793–800.
Boyan-Salyers, B. D., Vogel, J. J., and Ennever, J. (1978). Pre-apatitic mineral deposition in Bacterionema matruchotii. J. Dent. Res. 57, 291–295. doi: 10.1177/00220345780570022501
Duran-Pinedo, A. E., Chen, T., Teles, R., Starr, J. R., Wang, X., Krishnan, K., et al. (2014). Community-wide transcriptome of the oral microbiome in subjects with and without periodontitis. ISME J. 8, 1659–1672. doi: 10.1038/ismej.2014.23
Ennever, J., and Creamer, H. (1967). Microbiologic calcification: bone mineral and bacteria. Calcif Tissue Res. 1, 87–93. doi: 10.1007/BF02008078
Ennever, J., Riggan, L. J., Vogel, J. J., and Boyan-Salyers, B. (1978). Characterization of Bacterionema matruchotii calcification nucleator. J. Dent. Res. 57, 637–642. doi: 10.1177/00220345780570041901
Ennever, J., Vogel, J. J., Boyan-Salyers, B., and Riggan, L. J. (1979). Characterization of calculus matrix calcification nucleator. J. Dent. Res. 58, 619–623. doi: 10.1177/00220345790580021401
Eriksson, L., Lif Holgerson, P., and Johansson, I. (2017). Saliva and tooth biofilm bacterial microbiota in adolescents in a low caries community. Sci. Rep. 7, 5861. doi: 10.1038/s41598-017-06221-z
Esberg, A., Barone, A., Eriksson, L., Lif Holgerson, P., Teneberg, S., and Johansson, I. (2020). Corynebacterium matruchotii demography and adhesion determinants in the oral cavity of healthy individuals. Microorganisms 8, 1780. doi: 10.3390/microorganisms8111780
Gross, E. L., Leys, E. J., Gasparovich, S. R., Firestone, N. D., Schwartzbaum, J. A., Janies, D. A., et al. (2010). Bacterial 16S sequence analysis of severe caries in young permanent teeth. J. Clin. Microbiol. 48, 4121–4128. doi: 10.1128/JCM.01232-10
Jersie-Christensen, R. R., Lanigan, L. T., Lyon, D., Mackie, M., Belstrøm, D., Kelstrup, C. D., et al. (2018). Quantitative metaproteomics of medieval dental calculus reveals individual oral health status. Nat. Commun. 9, 4744. doi: 10.1038/s41467-018-07148-3
Johansson, I., Witkowska, E., Kaveh, B., Lif Holgerson, P., and Tanner, A. C. (2016). The Microbiome in Populations with a low and high prevalence of caries. J. Dent. Res. 95, 80–86. doi: 10.1177/0022034515609554
Keren, R., Mayzel, B., Lavy, A., Polishchuk, I., Levy, D., Fakra, S. C., et al. (2017). Sponge-associated bacteria mineralize arsenic and barium on intracellular vesicles. Nat. Commun. 8, 14393. doi: 10.1038/ncomms14393
Kim, D., Barraza, J. P., Arthur, R. A., Hara, A., Lewis, K., Liu, Y., et al. (2020). Spatial mapping of polymicrobial communities reveals a precise biogeography associated with human dental caries. Proc. Natl. Acad. Sci. U. S. A. 117, 12375–12386. doi: 10.1073/pnas.1919099117
Kolenbrander, P. E., Palmer, R. J. Jr., Periasamy, S., and Jakubovics, N. S. (2010). Oral multispecies biofilm development and the key role of cell-cell distance. Nat. Rev. Microbiol. 8, 471–480. doi: 10.1038/nrmicro2381
Korona-Glowniak, I., Piatek, D., Fornal, E., Lukowiak, A., Gerasymchuk, Y., Kedziora, A., et al. (2021). Patterns of oral microbiota in patients with apical periodontitis. J. Clin. Med. 10, 2707. doi: 10.3390/jcm10122707
Kreth, J., Merritt, J., and Qi, F. (2009). Bacterial and host interactions of oral streptococci. DNA Cell Biol. 28, 397–403. doi: 10.1089/dna.2009.0868
Kreth, J., Zhang, Y., and Herzberg, M. C. (2008). Streptococcal antagonism in oral biofilms: Streptococcus sanguinis and Streptococcus gordonii interference with Streptococcus mutans. J. Bacteriol. 190, 4632–4640. doi: 10.1128/JB.00276-08
Listgarten, M. A., Mayo, H., and Amsterdam, M. (1973). Ultrastructure of the attachment device between coccal and filamentous microorganisms in “corn cob” formations of dental plaque. Arch. Oral Biol. 18, 651–656. doi: 10.1016/0003-9969(73)90105-2
Mark Welch, J. L., Rossetti, B. J., Rieken, C. W., Dewhirst, F. E., and Borisy, G. G. (2016). Biogeography of a human oral microbiome at the micron scale. Proc. Natl. Acad. Sci. U. S. A. 113, E791–800. doi: 10.1073/pnas.1522149113
Naradasu, D., Miran, W., and Okamoto, A. (2020). Metabolic current production by an oral biofilm pathogen Corynebacterium matruchotii. Molecules. 25, 3141. doi: 10.3390/molecules25143141
Perera, D., McLean, A., Morillo-López, V., Cloutier-Leblanc, K., Almeida, E., Cabana, K., et al. (2022). Mechanisms underlying interactions between two abundant oral commensal bacteria. ISME J. 16, 948–957. doi: 10.1038/s41396-021-01141-3
Qudeimat, M. A., Alyahya, A., Karched, M., Behbehani, J., and Salako, N. O. (2021). Dental plaque microbiota profiles of children with caries-free and caries-active dentition. J. Dent. 104, 103539. doi: 10.1016/j.jdent.2020.103539
Richards, V. P., Alvarez, A. J., Luce, A. R., Bedenbaugh, M., Mitchell, M. L., Burne, R. A., et al. (2017). Microbiomes of site-specific dental plaques from children with different caries status. Infect. Immun. 85:e00106–17. doi: 10.1128/IAI.00106-17
Rickard, A. H., Gilbert, P., High, N. J., Kolenbrander, P. E., and Handley, P. S. (2003). Bacterial coaggregation: an integral process in the development of multi-species biofilms. Trends Microbiol. 11, 94–100. doi: 10.1016/S0966-842X(02)00034-3
Sakai, Y., Nemoto, E., Kanaya, S., Shimonishi, M., and Shimauchi, H. (2014). Calcium phosphate particles induce interleukin-8 expression in a human gingival epithelial cell line via the nuclear factor-κB signaling pathway. J. Periodontol. 85, 1464–1473. doi: 10.1902/jop.2014.130709
Sidaway, D. A.. (1978a). A microbiological study of dental calculus. I. The microbial flora of mature calculus. J. Periodontal Res. 13, 349–359. doi: 10.1111/j.1600-0765.1978.tb00189.x
Sidaway, D. A.. (1978b). A microbiological study of dental calculus. II. The in vitro calcification of microorganisms from dental calculus. J. Periodontal Res. 13, 360–366. doi: 10.1111/j.1600-0765.1978.tb00190.x
Socransky, S. S.. (1970). Relationship of bacteria to the etiology of periodontal disease. J. Dent. Res. 49, 203–222. doi: 10.1177/00220345700490020401
Sutej, I., Peros, K., Benutic, A., Capak, K., Basic, K., and Rosin-Grget, K. (2012). Salivary calcium concentration and periodontal health of young adults in relation to tobacco smoking. Oral Health Prev. Dent. 10, 397–403. doi: 10.3290/j.ohpd.a28911
Takazoe, I., and Itoyama, T. (1980). Analytical electron microscopy of Bacterionema matruchotii calcification. J. Dent. Res. 59, 1090–1094. doi: 10.1177/00220345800590062101
Takazoe, I., Matsukubo, T., and Katow, T. (1978). Experimental formation of “corn cob” in vitro. J. Dent. Res. 57, 384–387. doi: 10.1177/00220345780570024101
Treerat, P., Redanz, U., Redanz, S., Giacaman, R. A., Merritt, J., and Kreth, J. (2020). Synergism between Corynebacterium and Streptococcus sanguinis reveals new interactions between oral commensals. ISME J. 14, 1154–1169. doi: 10.1038/s41396-020-0598-2
van Dijk, S., Dean, D. D., Liu, Y., Zhao, Y., Chirgwin, J. M., Schwartz, Z., et al. (1998). Purification, amino acid sequence, and cDNA sequence of a novel calcium-precipitating proteolipid involved in calcification of corynebacterium matruchotii. Calcif. Tissue Int. 62, 350–358. doi: 10.1007/s002239900443
Velsko, I. M., Fellows Yates, J. A., Aron, F., Hagan, R. W., Frantz, L. A. F., Loe, L., et al. (2019). Microbial differences between dental plaque and historic dental calculus are related to oral biofilm maturation stage. Microbiome 7, 102. doi: 10.1186/s40168-019-0717-3
Vogel, J. J., and Smith, W. N. (1976). Calcification of membranes isolated from Bacterionema matruchotii. J. Dent. Res. 55, 1080–1083. doi: 10.1177/00220345760550061201
Weyrich, L. S., Duchene, S., Soubrier, J., Arriola, L., Llamas, B., Breen, J., et al. (2017). Neanderthal behaviour, diet, and disease inferred from ancient DNA in dental calculus. Nature 544, 357–361. doi: 10.1038/nature21674
Willmann, C., Mata, X., Hanghoej, K., Tonasso, L., Tisseyre, L., Jeziorski, C., et al. (2018). Oral health status in historic population: Macroscopic and metagenomic evidence. PLoS ONE. 13, e0196482. doi: 10.1371/journal.pone.0196482
Zhong, E. F., Chang, A., Stucky, A., Chen, X., Mundluru, T., Khalifeh, M., et al. (2020). Genomic analysis of oral lichen planus and related oral microbiome pathogens. Pathogens 9, 952. doi: 10.3390/pathogens9110952
Keywords: Corynebacterium matruchotii, supragingival plaque, biofilm structure, bacterial interaction, oral diseases
Citation: Li Q, Zhou F, Su Z, Li Y and Li J (2022) Corynebacterium matruchotii: A Confirmed Calcifying Bacterium With a Potentially Important Role in the Supragingival Plaque. Front. Microbiol. 13:940643. doi: 10.3389/fmicb.2022.940643
Received: 10 May 2022; Accepted: 30 May 2022;
Published: 06 July 2022.
Edited by:
Huancai Lin, Sun Yat-sen University, ChinaReviewed by:
Feng Chen, Peking University, ChinaCopyright © 2022 Li, Zhou, Su, Li and Li. This is an open-access article distributed under the terms of the Creative Commons Attribution License (CC BY). The use, distribution or reproduction in other forums is permitted, provided the original author(s) and the copyright owner(s) are credited and that the original publication in this journal is cited, in accordance with accepted academic practice. No use, distribution or reproduction is permitted which does not comply with these terms.
*Correspondence: Yuqing Li, bGl5dXFpbmcmI3gwMDA0MDtzY3UuZWR1LmNu; Jiyao Li, aml5YW9saXNjdSYjeDAwMDQwOzE2My5jb20=
†These authors have contributed equally to this work