- 1Department of Cell Biology, Zunyi Medical University, Zunyi, China
- 2Department of Immunology, Zunyi Medical University, Zunyi, China
- 3Engineering Research Center of Key Technology Development for Gui Zhou Provincial Dendrobium Nobile Industry, Zunyi Medical University, Zunyi, China
Endophytes is a kind of microorganism resource with great potential medicinal value. The interactions between endophytes and host not only promote the growth and development of each other but also drive the biosynthesis of many new medicinal active substances. In this review, we summarized recent reports related to the interactions between endophytes and hosts, mainly regarding the research progress of endophytes affecting the growth and development of host plants, physiological stress and the synthesis of new compounds. Then, we also discussed the positive effects of multiomics analysis on the interactions between endophytes and their hosts, as well as the application and development prospects of metabolites synthesized by symbiotic interactions. This review may provide a reference for the further development and utilization of endophytes and the study of their interactions with their hosts.
Introduction
Endophytes are special “microorganism” with much value. They can parasitize different parts of living plants but do not obviously cause symptoms of external infection in host plants or symbiosis and coevolution with their host (Chen et al., 2022). As an important cooperative “partner” of plant growth and development, endophytes greatly impact on host physiological metabolism, which helps or stimulates host plants against various stresses (Koskimäki et al., 2022). More importantly, during this long-term association, the endophytes and their host form an interactive relationship of coevolution and mutually beneficial symbiosis. The genetics and metabolism of endophytes ameliorate and supplement the material metabolic pathway and related gene expression of their hosts (Mei et al., 2019). Conversely, the host also creates a unique community structure and gene characteristics of endophytes (Oukala et al., 2021; Li et al., 2022).
Exploring the application of endophytes has become a hot topic with the gradual understanding of the interaction between endophytes and hosts. Plants were first thought of as individual organisms that produced metabolites by themselves to completely support their growth and development. This cannot explain the phenomenon in which plants growing in the wild are “better,” such as in medicinal characters, than those under artificial cultivation; artificial cultivation of medicinal plants loses the original effect, etc. It was not until the discovery of endophytes that their interactive relationship was gradually revealed, especially after the application of omics technology at the cellular and molecular levels in which the study of the interactive relationship between the two progressed rapidly. In recent years, researchers have been trying to explore the evolutionary relationship between host and endophytes, and trace the reciprocity mechanism of this specific evolutionary relationship, in order to provide theoretical basis for the commercial development of endophytes. At present, some endophytic bacteria and their active products have been successfully used in commercial production and obtained great benefits in the preparation of new drugs and agricultural protection (Singh and Gaur, 2017; Mishra et al., 2018b; Sahu et al., 2021). It is of great economic value to reveal the interactive mechanisms between plants and endophytes to explore and release the potential for the industrial development of endophytes.
To provide a reference for revealing the complex interaction between endophytes and their hosts, this review first discusses the interaction between endophytes and host plants and then the effects of endophytes and their secondary metabolites on plant growth and development. The application of endophyte resources instead of is also discussed.
Interaction Between Endophytes and Host Plants
Plant Microecology Under Endophyte Invasion
Many plant biological functions are dependent on endophytes, and each plant is actually a complex microecosm (Compant et al., 2021). A broad consensus has been reached that endophytes and their host plants have had a coevolutionary history for millions of years. The interaction between them not only maintains ecological stability but also promotes the growth and development of both partners (Pérez-Alonso et al., 2020). With the improvement and application of cell and molecular science, omics and even “macro-omics,” the study of the interactions between endophytes and hosts has made great progress, and related theories, such as “mosaic theory,” “acquired immune system,” “equilibrium confrontation,” and “exogenous chemical excitation reaction,” have been proposed (Cui et al., 2017). In recent years, many studies on the interactions between endophytes and hosts have shown that the “balanced antagonism” theory is more accurate in explaining the relationship between endophytes and hosts. The core of the theory is that the “confrontation” between microorganism and plants is different from that of general pathogenic bacteria, and its essence is the special balance between the endophytic virulence factor and the plant defense and immune system; when the virulence factor is too strong, the plant will become sick. When defense stress is too strong, microorganisms are killed (Schulz et al., 1998). Actually, the balance of interaction between endophytes and hosts is far from a simple “confrontation” between virulence factors and the defense system, and its regulatory mechanisms are far more complex and precise than maintaining the balance between virulence factors and the defense system (Khaiwa et al., 2021).
Further studies showed that when microbes invade the host plant tissue, the recognition of the plant self-defense system will begin the crosstalk of signal molecules to identify endophytes (Figure 1). For example, the medicinal plant Camptotheca acuminata kills invading microorganisms by producing camptothecin, which competitively inhibits the activity of the microbial topoisomerase I complex (Khaiwa et al., 2021). Fusarium solani, an endophytic fungus in Camptotheca acuminata, uses special amino acid residues to bind the relatively active domain of camptothecin to escape the attack of the host, while the other endophytes avoid the invasion risk by encoding a unique topoisomerase. Therefore, the colonization of endophytes is not easy (Sirikantaramas et al., 2009; Kusari et al., 2011, 2012a). Robinson’s results showed that the rhizosphere-dominant bacterium Bacillus mycoides could not colonize wheat in an aseptic system without competitive bacteria (Robinson et al., 2016). Further studies found that when competitive bacteria exist, wheat secretes special root exudates, which are rich in nutrients and can induce and promote Bacillus mycoides symbiosis (Robinson et al., 2016). In addition, Wang successfully colonized rhizobia in the roots of legumes by applying exogenous metabolites of flavonoids to realize the symbiosis of endophytes (Wang et al., 2021). Therefore, the formation of the interaction between endophytes and hosts is far from a simple combination of heredity and material between them but is accompanied by the overall cooperation of the “micro niche” formed by the internal and external environment of their hosts.
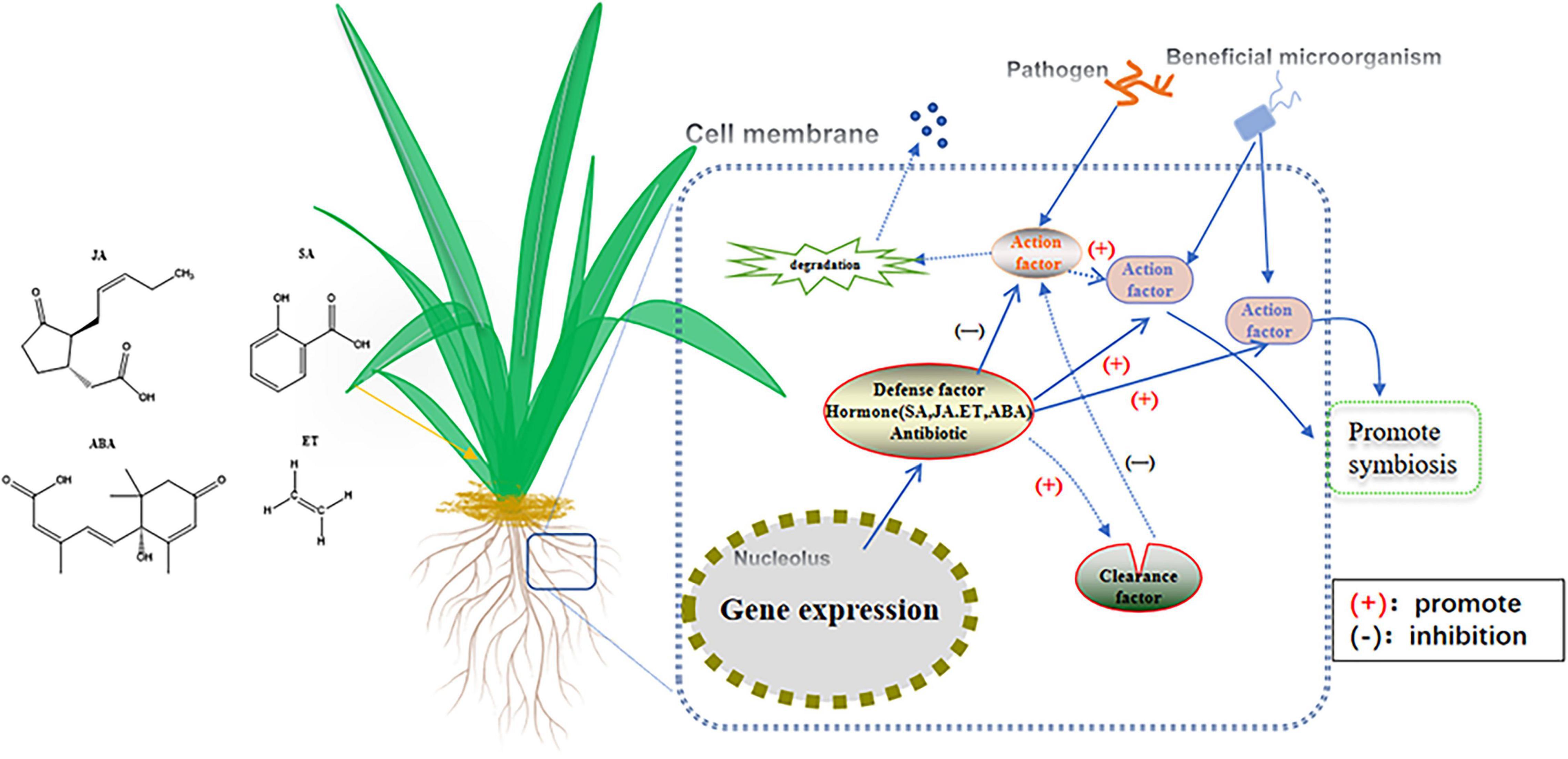
Figure 1. Signal response mechanisms for promoting (+) or inhibiting (–) plant – microbial interactions. Exogenous microorganisms release action factors to infest plants, which respond with gene expression and release metabolites such as antibiotics and hormones (SA, JA, GA, ET, and ABA) to interfere with the infestation to protect themself.
Dilemmas in Interaction Mechanisms Study
Interactive mechanisms between endophytes and hosts are the material basis for the formation of interactions. Previous work has mainly explored the interactions between endophytes and their hosts from superficial aspects, such as morphological observations and chemical composition analysis. However, this does not fully explain the pathways of host growth, development and metabolism under the action of endophytes. At present, although the action mechanisms of some endophytes have been described, it is still difficult to accurately reveal the interactive mechanisms. The dilemmas are mainly manifested in three aspects: a large number of microorganisms react with plants at the same time, endophytes have signal interference, and symbiosis is difficult to simulate.
At present, it is very important to find and understand the growth mechanism of host endophytes. Studies suggest that endophytes affect plants mainly in two aspects. On the one hand, endophytes induced systemic resistance (ISR) production in the host. ISR differs from traditional system acquired resistance (SAR) in that its phenotype is similar to that of pathogen induced SAR, both of which can induce broad-spectrum resistance to pathogens in plants (Pieterse et al., 2014; Pozo et al., 2015). In essence, ISR differs from other induced-resistance mechanisms in that the host usually has primic defense associated with the jasmonic acid (JA) pathway under symbiotic association, and can initiate faster, stronger, and more durable defense expression under adverse conditions (Martinez-Medina et al., 2016; Teixeira et al., 2019). However, the mechanism of “priming” state is still unclear, and it is speculated that transcription factors and related signals play an important role in it (Bentham et al., 2020; Mengistu, 2020). On the other hand, endophytes can effectively regulate defense-related signaling pathways in the host to achieve self-colonization and thus establish symbiotic links with host plants. After colonization, endophytes invasion plants, are bound to across the plant immune system highly complex in the first line of defense, also known as microbial associated molecular patterns (MAMP), a process that involves many molecules [such as elongation factors Tu (EF-Tu), peptidoglycan (PG), superoxide dismutase (SOD), β-chitosan bacteria cold shock protein (RNP1motif), chitin, etc.] and the specificity of the host cell surface receptor molecules PRR identification (Newman et al., 2013). Under current conditions, although it is known that symbiosis with endophytes endows hosts with additional defense mechanisms, little is known about this aspect (Eid et al., 2021; Roy et al., 2021). In fact, plant immune system activation and mechanical changes are the result of ongoing molecular dialogue with endophytes, a common example of which is the JA pathway discussed above (Gutjahr, 2014; Pozo et al., 2015; Bastias et al., 2017). After plant infection with endophytes, the salicylic acid (SA) pathway is usually inhibited, the JA precursor level is enhanced, and then the JA reaction gene is upregulated (Wasternack and Hause, 2013). However, different from the SA pathway, the JA pathway is not well understood (Zhang M. J. et al., 2021). Existing studies believe that the increase of JA pathway seems to be related to the development and function of mycorrhizal symbiosis, which may be understood and verified in future studies (Siciliano et al., 2007).
Application of Omics to Elucidate the Interactions Between Endophytes and Hosts
Endophytes are important biological resources. It is important to fully understand the interaction between endophytes and hosts to fully exploit the value of endophytes. Although research on endophytes has made great progress, they are still unknown in regard to many aspects of research value. At present, the rational use of scientific methods to fully understand the interaction between endophytes and hosts is helpful for providing more choices and possibilities in the study of the conversion value of endophytes. Modern high-throughput genomic technology provides a technical basis for exploring the potential value of endophytes. An in-depth analysis of endophytes from the aspects of sequencing, taxonomic classification, phylogeny and biological evolution has greatly promoted enthusiasm for endophyte research (Selosse et al., 2022). Genome-wide analysis of endophytes directly reflects the colonization preference and genetic characteristics of endophytes on different hosts. This greatly promotes the identification of related genes, such as host growth and development mechanisms, antibiotic production, insertion elements, endophytic secretory system, surface attachment and other metabolic processes (Subudhi et al., 2018). Moreover, genome-wide analysis has also been applied to explain the survival and evolution of endophytes in hosts (Singh et al., 2021). For example, whole genome sequencing analysis revealed the potential of endophytic fungus P. indica as a plant probiotic preparation. Genome-wide analysis of the endophytic bacterium Pantoea ananatis revealed the existence of genes encoding hydrolase, n-acyl high serine lactone synthase, superadhesion factor and fusylic acid resistance protein. This shows its great biological potential for commercial production (Wu et al., 2020). At present, the whole genomes of some endophytes have been sequenced, and the number is increasing (Table 1). This can not only intuitively reveal the changes in gene expression and genetic characteristics of plants under interactive relationships but can also serve as a systematic model for the study of interactions between endophytes and hosts.
In addition, based on the commonly used single omics technology, omics has been further developed into “macro omics,” which provides more powerful technical support for the study of the interactions between hosts and endophytes. However, the comprehensive application of polyrecombinationics in the field of endophytes research can often yield more comprehensive results. In addition, we should fully consider the complex “network” system of interactions between endophytes and hosts. Then, using omics technology to analyze the composition differences between individuals, and even the interactions between multiple individuals, in order to form a new model to reveal the nature of interactions between endophytes and hosts (Lin, 2015). In recent years, many researchers have carried out studies on the interaction between endophytes and hosts by using joint omics, but there is still a huge gap in the understanding of the interaction between endophytes and hosts. In the next stage, there is still a long way to go to realize the perfect combination of information technology and data and to study the interactions between endophytes and hosts in a real sense.
Studies on the Secondary Induction Role of Endophytes in Interactions With Hosts
Endophytes Promote Host Growth and Development
Endophytes can affect host growth and development by producing metabolites (Figure 2). Studies have shown that some endophytes can promote host growth by regulating hormones such as SA, JA, abscisic acid (ABA), endothelin (ET), and gibberellins (GA). For example, endophytes can reduce host ethylene levels by regulating 1-aminocyclopropane-1-carboxylate (ACC) deaminase activity. At the same time, the ability to synthesize plant hormone indole-3-acetic acid (IAA) is utilized to promote host growth and repair host activity during toxic damage (Glick and Stearns, 2011). Besides, some endophytes affect host growth through their own metabolism. For example, nitrogen-fixing endophytes in many hosts, such as soybeans and corn, can reduce free N2 from the atmosphere to NH4+ for host uptake and promote host growth (Jin, 2014; Guzmán et al., 2022). Some endophytes isolated from soybean root nodules promote the absorption of P by their hosts by dissolving P minerals (Varga et al., 2020). In addition, N, P, C and other elements were also stronger in soybean plants infected with endophytic arbuscular mycorrhizal fungi (AMF) than in uninfected soybean plants. This is mainly due to the rapid formation of special arbuscular mycorrhizal structures in soybean root AMF (Khaekhum et al., 2021).
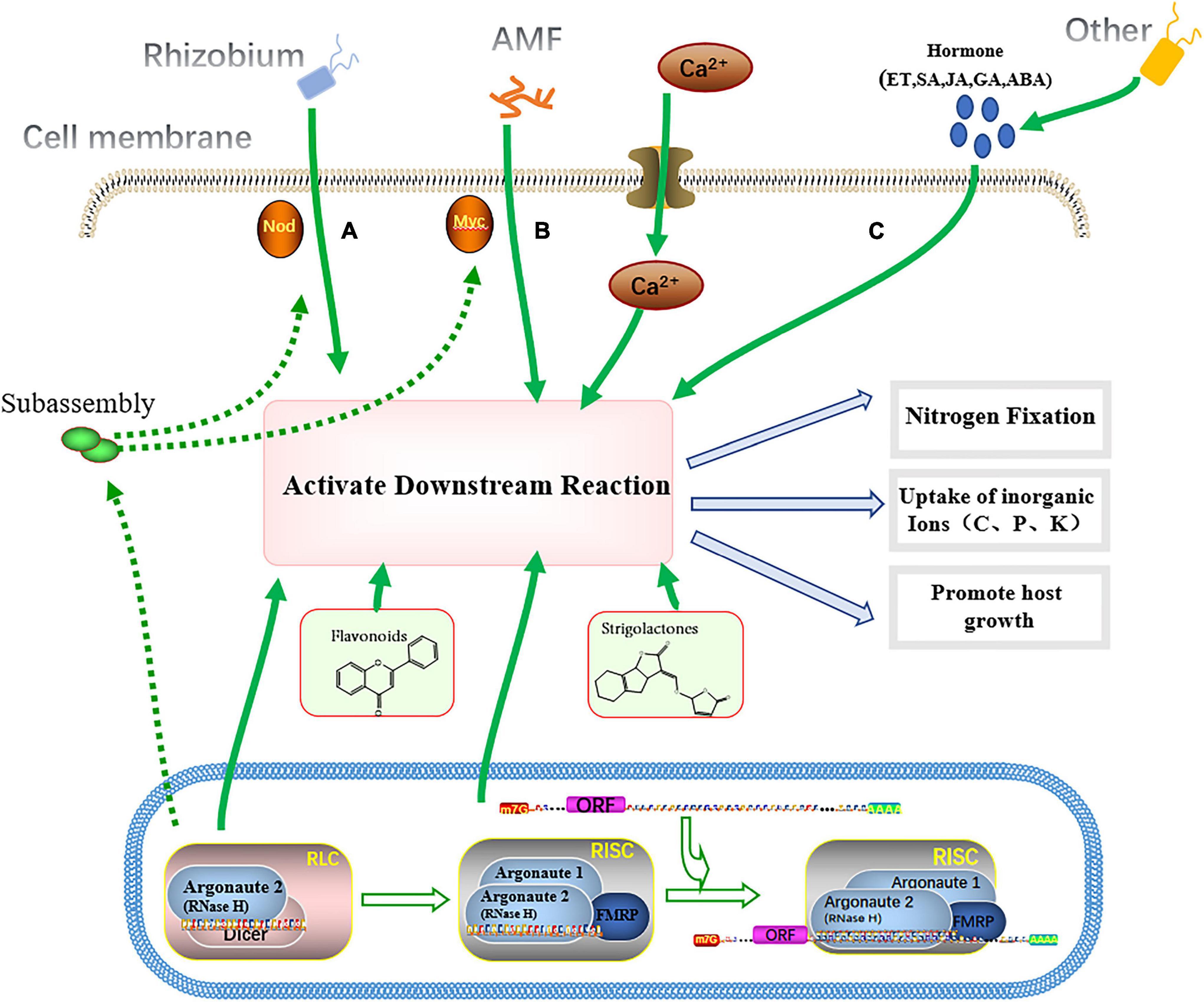
Figure 2. Endophytes affect host growth. Endophytes release factors that act on host cells, which express RNA-induced silencing complex (RISC) genes and silence specific genes, thereby regulating the invasion process of endophytes. (A) Under the action of Nod factor, rhizobia formed root nodules and fixed N2. (B) AMF form clumped mycorrhizae (AM) under the action of Myc factors, which promote host uptake of inorganic ions (C, P, N, etc.) and regulate the root environment. (C) Some endophytes are able to produce hormones (SA, JA, GA, ET, ABA, etc.) that promote host growth.
Endophytes Increase Host Stress Tolerance
Most endophytes enhance host tolerance to stress to some extent, while some plants may not survive due to lack of endophytes (Wu et al., 2021). The improvement effect of endophytes on their hosts is not only in the regulation of abiotic stress, such as tolerance to temperature, drought, salinity and metal ion stress, but also in the regulation of biological stress, such as resistance to diseases and insect pests and herbivorous organisms. Therefore, endophytes not only show a rich source of stress tolerance to their hosts but also show a wide range of effects.
Increase Stress Tolerance to Abiotic Stresses
Endophytes can effectively regulate the stress tolerance of hosts to abiotic stresses (Table 2). Studies have shown that the diversity of endophytic communities or changes in metabolism have a significant impact on host adaptability to the environment (Li et al., 2022). Some endophytes activate membrane receptor molecular mechanisms and signaling pathways, such as G-protein-coupled receptors (GPCRs), receptor-like kinases (RLKs), ion channels, and histidine kinases, when the host is exposed to salinity, temperature, drought, and heavy metal toxicity. Change the concentration of Ca2+ in the cytoplasm and produce corresponding signaling molecules such as reactive oxygen species (ROS), ABA and inositol phosphates (IPs). Further kinase responses activate various downstream transcription factors, such as MYC/MYB, WRKY, DREB/CBF, AREB/ABF, bHLH, NAC, and bZIP (Sirikantaramas et al., 2009). Activated transcription factors lead to the activation of different stress-responsive genes, including the expression of lipid-transfer proteins (LTPs), heat shock proteins (HSPs), late embryogenesis abundant protein (LEA), antioxidant response element (ARE) and osmotic proteins, through reciprocal transformation of protein phosphorylation and dephosphorylation. Some endophytes activate pattern recognition receptor (PRR) membrane receptors under the influence of exogenous signals, amplify secondary signals through cascade reactions, and then activate the downstream MAPK signaling pathway, resulting in NLRs, TFs, HSFA2, RLKs and other related gene expression. Through the above series of biochemical reactions, endophytes can regulate host permeability to reduce or even offset the effects of stress and can finally achieve the purpose of repairing damage (Wang et al., 2020; Figure 3). Baek et al. (2020) found that salt stress-related genes were expressed in the shoots and roots of rice seedlings after infecting them with endophytic bacterium Bacillus oryzicola YC7007. AMF, an endophytic fungus isolated from chickpeas, can help the host to relieve drought stress (Hashem et al., 2019). In addition, under salt stress, some endophytic fungi can secrete exopolysaccharide to change soil structure, regulate soil material composition and change host permeability, so as to relieve stress (Kidd et al., 2021).
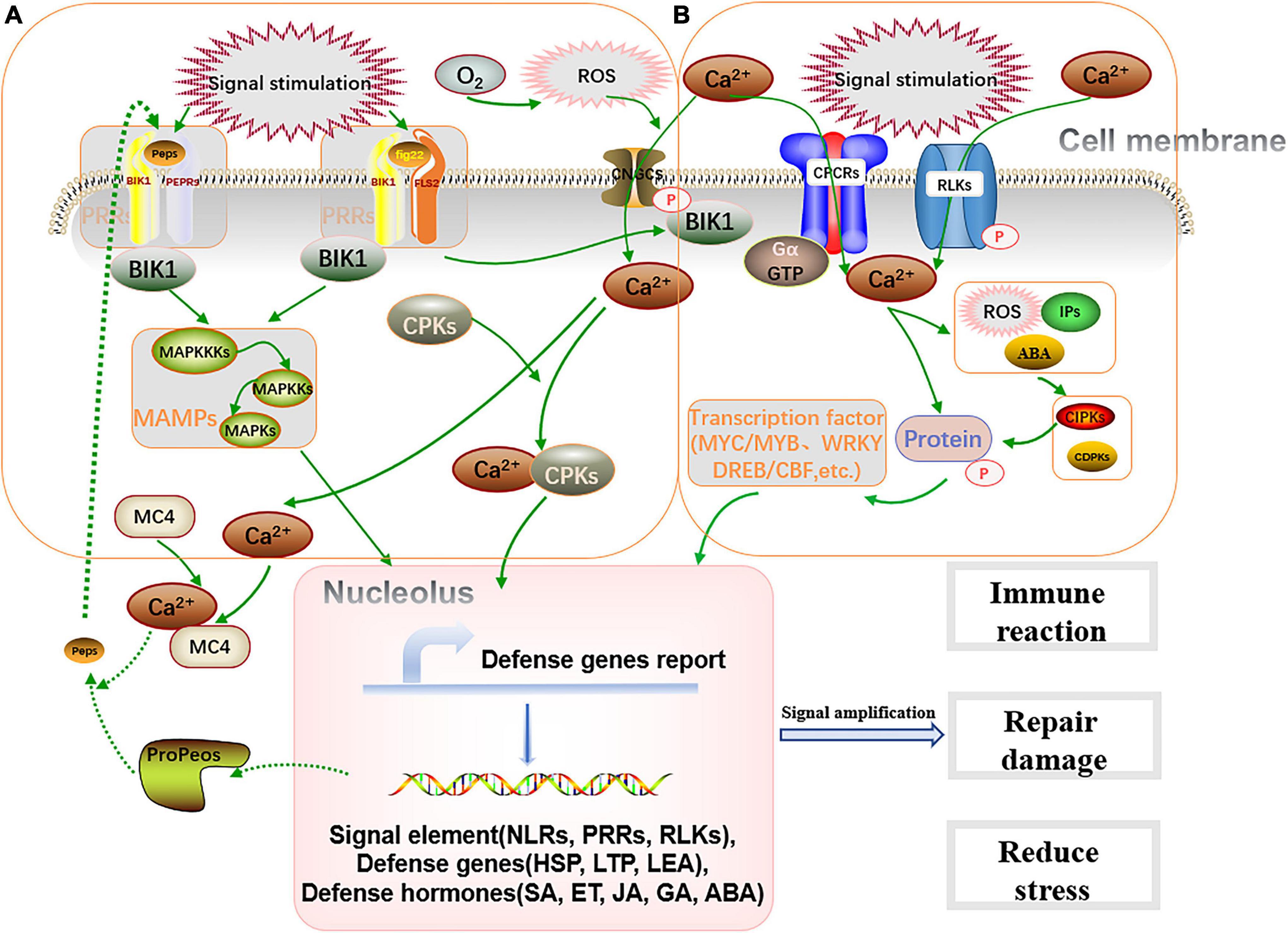
Figure 3. Endophytes regulate the tolerance of plants to stress. (A) Under stress environment, endophytes can produce exogenous signals and quickly activate plant PRRs membrane receptors. On the one hand, they activate the downstream MAPKs level connection On the other hand, they regulate the intracellular Ca2+ concentration. (B) Under the action of exogenous signal of endophytes, membrane receptors such as CPCRs and RLKs are activated to induce downstream reaction. Cascade signaling is transmitted in the nucleus to promote the expression of relevant response genes, so as to improve the ability of host in immune response, repair damage and reduce stress.
Increasing Stress Tolerance to Biotic Stress
Endophytes can enhance host resistance to biological stresses such as diseases and insect pests in a variety of ways (Table 3). On the one hand, some endophytes can avoid the competition of pathogenic microorganisms by producing lipopeptides, chitinases, pyrrolidine, glucanase, and other antibiotic metabolites (Mishra et al., 2018a). Baek et al. (2020) isolated the endophytic bacterium Bacillus oryzicola YC7007 from the rhizosphere of rice (Oryza sativa), which can induce systemic resistance and antibiotics, inhibit rice bacterial diseases and promote rice growth and development. The endophytic bacterium Bacillus subtilis EDR4 isolated from wheat competitively inhibited the growth of pathogens Fusarium oxysporum by producing the antifungal protein E2 (Liu et al., 2010). Bacillus triticum JC-K3, an endophytic bacterium isolated from wheat, can regulate local IAA concentration and then regulate the absorption of inorganic ions to promote the growth of host, thus alleviating the biological diseases under salt stress (Ji et al., 2021). On the other hand, endophytes can colonize hosts in a manner similar to the invasion of pathogens, which creates niche competition with pathogens (Ahmed et al., 2020). Therefore, plants can recruit endophytes and reduce the possibility of colonization of their own pathogens by giving priority to occupying the niche. Researchers isolated the endophytic bacterium Stenotrophomonas from Pistacia chinensis, which can preferentially occupy the niche by producing iron carriers, thus reducing the colonization of pathogenic bacteria and further relieving the iron stress environment of Pistacia chinensis (Etminani and Harighi, 2018). In addition, endophytes can also help hosts to cope with insect feeding. The endophytic alkaloid metabolites and neurotoxins may lead to disordered behavior, hindered growth and development, and even death of insects (Song et al., 2020; Tooker and Giron, 2020; Grabka et al., 2022). For example, Bacillus in sugarcane can induce the abnormal development of giant borers, thus reducing diseases and insect pests (Rocha et al., 2021).
Endophytes Promote the Production of Host Secondary Metabolites
The production of active substances in endophytes seems to be inseparable from the role of endophytes. Recent studies have shown that when endophytes promote the production of host secondary metabolites, their hosts do not simply increase substances but the endophytes trigger a series of biochemical processes in their hosts, such as host growth and stress resistance regulation. There are two main ways for endophytes to promote the host. One way is that endophytes generate the same signal pathway as their hosts by during gene mutation or information exchange and then produce secondary metabolites similar to their hosts. For example, endophytes isolated from marigold can produce the same hydrolytic enzymes and IAA as their host (Shurigin et al., 2021). Another way is that endophytes cooperate with their hosts to complete a process of the same signal pathway, which may produce key enzymes in the pathway, or change a reaction direction in the process of host metabolism to make their hosts produce specific metabolites (Khattab and Farag, 2021).
Applications and Research Prospects of Endophytes and Secondary Metabolites
As reliable companions of hosts, endophytes play an important role in the growth and development of hosts and the accumulation of secondary metabolites (Singh et al., 2022). In recent years, with the rapid increase in endophyte-related research, endophytes have gradually become the focus of attention. The study of abundant endophytic species and their response genes suggests their important role in symbiosis systems and mechanisms (Tariq et al., 2022). Studies have shown that under the interaction between hosts and endophytes, hosts can use their own defense system to “screen” specific microorganisms to form a symbiotic system. Conversely, successfully “recruited” endophytes can also make their hosts better adapted to the environment during growth (Wu et al., 2021). Therefore, endophytes are not only natural drug substitutes for promoting host growth and development but also seed banks of new active metabolites. However, due to the complexity of the interactions between species, there are still many unknown fields in research on the lifestyles and action mechanisms of endophytes.
Currently, Endophytic effects have been studied in only a small number of plant species, the vast majority of which come from land plants (Nasiruddin et al., 2020). Studies based on metabolomics have also shown that endophytes are repositories of bioactive metabolites that can produce many active products with pharmacological effects, such as antimicrobial, antitumor, antibiotic, antioxidant, and immune agents (Gupta et al., 2020; Table 4). Although endophytes can continuously and effectively produce several bioactive compounds, it is not realistic to obtain these active substances only from endophytes. Further studies have shown that the acquisition of beneficial active products from endophytes is affected by many internal and external factors, such as the living state of the host plants, species, geographic location, climatic conditions and even the season of sample collection (Li et al., 2020). In fact, the active products of endophytes can solve the shortage of natural resources and provide new age ideas for the development and preparation of new drugs, but current research on endophytes is far from reaching this goal (Yan et al., 2019). At present, although some endophytic strains that can produce host active metabolites have been isolated, almost none of them can be used in production due to the difficulties of in vitro culture. Therefore, the problem of in vitro propagation of endophytes from hosts and mass production of active ingredients is a key problem faced by current applied research, which is also the premise of endophytes replacing medicinal plants to achieve commercial production of good pharmacodynamically active ingredients (Shao et al., 2021).
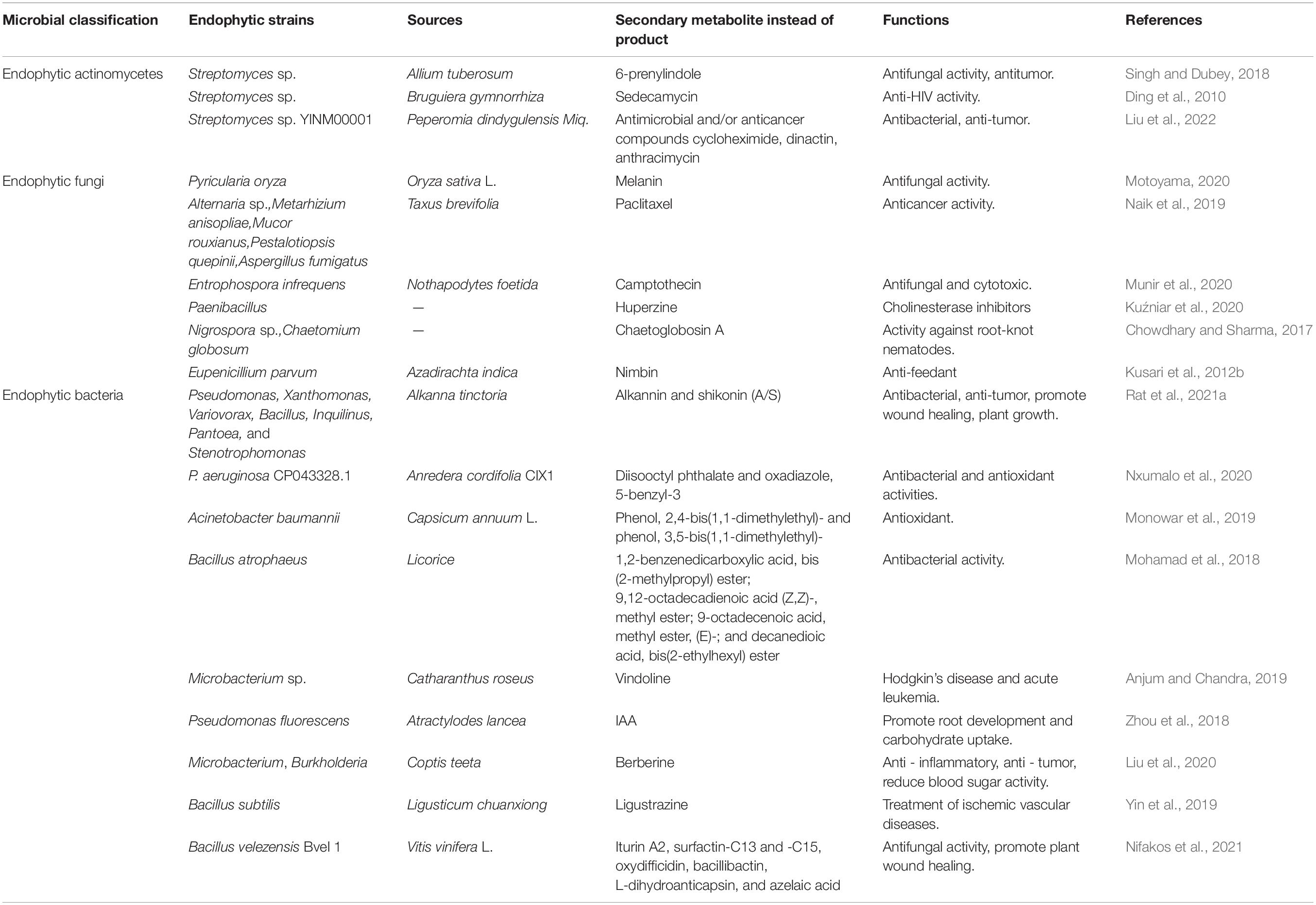
Table 4. Endophyte induced secondary metabolites and their biological activities during plant–endophyte interaction.
Overall, the active metabolites produced from the interactions between endophytes and host plants have great potential in future, and there is a large demand in the fields of medicine, agriculture, biodegradation and bioremediation. In recent years, with the improvement and application of HPCE, HPLC–MS and other technologies, the rapid identification of active metabolites of plant endophytes has become possible, and endophyte based nanoparticles are expected to play an important role in drug development in future (Pentimone et al., 2020). For instance, Zhang D. et al. (2021) isolated and identified a new endophytic bacterium, Bacillus altitudinis SB001, from wild sweet grass in China. Transcriptome sequencing showed that mature enzyme K, Tetratricopeptide repeats (TPR)-like superfamily proteins, Lateral organ boundaries (LOB) domain proteins and Broad-complex (BTB)/pox virus (POZ)/PDZ-binding motif (TAZ) domain proteins may play a role in the growth promotion of wild Chinese sweet grass (Zhang D. et al., 2021). In addition, the main promoters in the interactions with their host, the MFS transporter and DNA rotase subunit B, were also found in Bacillus altitudinis SB001. These findings suggest that endophytes may be useful candidates for host growth promotion.
Perspectives
Endophytes are a kind of microbial resource with abundant species and wide host. Most endophytes can regulate the growth, development and metabolism of their host. Therefore, a comprehensive and in-depth study of endophytes is of great significance. At present, research on endophytes is still in an early stage of relative development, and the embodiment of their application value still needs in-depth research and improvement. Although the whole genome of some endophytes has been deciphered, there are still many aspects to be clarified in endophyte research, especially the symbiotic mechanisms between endophytes and hosts, which remain to be further explored. Only by deeply understanding the interactive mechanisms between endophytes and their hosts can we further explore the potential value of endophytes in improving the growth and development of their hosts and the production of active metabolites. Currently, research on endophytes faces four major problems. In terms of endophyte invasion of their hosts, their mode of action still needs to be discussed, such as invasion site and invasion form (spores, hyphae, etc.). The study of the mechanisms of interactions between endophytes and their hosts, such as the specific ways of endophyte colonization, whether the endophyte can achieve proliferation after colonization and whether their host has antagonistic reactions, is still unclear. In terms of host growth and development, how endophytes regulate host metabolism, such as producing new metabolites and “reprocessing” host metabolites, still needs to be further revealed. In terms of production and application, problems such as difficulty in breeding on a large scale in vitro and the sharp decline of the effects in vitro are still serious.
Solving the above problems will not only help to tap into the ecological functions of endophytes but will also further improve the application potential of endophytes and will provide a base for the further development and utilization of endophytes. At present, although there have been an increasing number of studies on endophytes, there have been few reports on the large-scale application of endophytic preparations and their active metabolites in commercial production. The utilization of endophyte biological resources is still difficult, and there is no effective detection technology to directly identify endophytic bacteria in vivo from their host. In vitro endophyte isolation, culture and even fermentation cannot accurately obtain the corresponding strains or even metabolites. In addition, there are interactions between endophytes in the host, which undoubtedly adds difficulties in endophytes study. The mystery of endophytes is gradually being revealed. In future, on the basis of ensuring the biological activity of isolated and cultured endophytes, the improvement of their characteristics and application in basic research and commercial production will be of great significance. For example, new drugs can be created to treat diseases and for agricultural production. Regulating host gene expression and pathways improves the growth of valuable medicinal plants, realizing transgenic breeding and improving crop quality. Now is the time to elevate endophyte research from traditional physiological and biochemical research to higher cellular- and molecular-level research. Combined with omics technology, a database of endophytes and their active metabolites should be established. Then, the database should be used to understand the unknown field of endophytes and host interactions and to benefit from it.
Author Contributions
DX and LL conceived, supervised, and wrote and reviewed the manuscript. ZL, WW, YH, and MQ originally wrote and reviewed the draft. LL, DX, and YH co-founded and co-administrated the project. All authors read and approved the final version.
Funding
This research was financially supported by the National Natural Science Foundation of China (31560079 and 31960074), the Science and Technology Department Foundation of Guizhou Province of China (Nos. [2017]5733-050, [2019]-027, [2019]5657, and QKHJC-ZK[2022]YB610), the Special Joint Bidding Project of Zunyi Sci & Tech Bureau and Zunyi Medical University (ZSKHHZ-2020-91), and the Program for Excellent Young Talents of Zunyi Medical University (15zy-004).
Conflict of Interest
The authors declare that the research was conducted in the absence of any commercial or financial relationships that could be construed as a potential conflict of interest.
Publisher’s Note
All claims expressed in this article are solely those of the authors and do not necessarily represent those of their affiliated organizations, or those of the publisher, the editors and the reviewers. Any product that may be evaluated in this article, or claim that may be made by its manufacturer, is not guaranteed or endorsed by the publisher.
References
Abd-Allah, E. F., Alqarawi, A. A., and Hashem, A. (2018). Endophytic bacterium Bacillus subtilis (BERA 71) improves salt tolerance in chickpea plants by regulating the plant defense mechanisms. J. Plant. Interact. 13, 37–44. doi: 10.1080/17429145.2017.1414321
Agriculture, S. O., Food and Wine, W., Campus, U. O., Adelaide, S., and Australia, A. D. (2018). First detection of Russian wheat aphid Diuraphis noxia Kurdjumov (Hemiptera: Aphididae) in Australia: a major threat to cereal production. Austral. Entomol. 57, 410–417. doi: 10.1111/aen.12292
Aguilar, A., Mora, Y., Dávalos, A., Girard, L., Mora, J., and Peralta, H. (2018). Analysis of genome sequence and symbiotic ability of rhizobial strains isolated from seeds of common bean (Phaseolus vulgaris). BMC Genomics 19:645. doi: 10.1186/s12864-018-5023-0
Ahmed, A., Munir, S., He, P., Li, Y., He, P., Yixin, W., et al. (2020). Biocontrol arsenals of bacterial endophyte: An imminent triumph against clubroot disease. Microbiol. Res. 241:126565. doi: 10.1016/j.micres.2020.126565
Anjum, N., and Chandra, R. (2019). Endophytic bacteria of Catharanthus roseus as an alternative source of vindoline and application of response surface methodology to enhance its production. Archiv. Biolog. Sci. 71, 27–38. doi: 10.2298/ABS180802044A
Babalola, O. O., Adeleke, B. S., and Ayangbenro, A. S. (2021). Whole Genome Sequencing of Sunflower Root-Associated Bacillus cereus. Evol. Bioinform. Online 17:11769343211038948. doi: 10.1177/11769343211038948
Baek, D., Rokibuzzaman, M., Khan, A., Kim, M. C., Park, H. J., Yun, D. J., et al. (2020). Plant-Growth Promoting Bacillus oryzicola YC7007 Modulates Stress-Response Gene Expression and Provides Protection From Salt Stress. Front. Plant. Sci. 10:1646. doi: 10.3389/fpls.2019.01646
Baroncelli, R., Da, D., Lio, G., and Sarrocco, S. (2020). Genome Resources for the Endophytic Fungus Paraphaeosphaeria sporulosa. Mol. Plant. Microbe. Interact. 33, 1098–1099. doi: 10.1094/MPMI-04-20-0097-A
Bastias, D. A., Martínez-Ghersa, M. A., Ballaré, C. L., and Gundel, P. E. (2017). Epichloë Fungal Endophytes and Plant Defenses: not Just Alkaloids. Trends Plant. Sci. 22, 939–948. doi: 10.1016/j.tplants.2017.08.005
Bentham, A. R., De la Concepcion, J. C., Mukhi, N., Zdrzałek, R., Draeger, M., Gorenkin, D., et al. (2020). A molecular roadmap to the plant immune system. J. Biol. Chem. 295, 14916–14935. doi: 10.1074/jbc.REV120.010852
Bhattacharya, A., Giri, V. P., Singh, S. P., Pandey, S., Chauhan, P., Soni, S. K., et al. (2019). Intervention of bio-protective endophyte Bacillus tequilensis enhance physiological strength of tomato during Fusarium wilt infection. Biolog. Control. 139:104074. doi: 10.1016/j.biocontrol.2019.104074
Bziuk, N., Maccario, L., Straube, B., Wehner, G., Sørensen, S. J., Schikora, A., et al. (2021). The treasure inside barley seeds: microbial diversity and plant beneficial bacteria. Environ. Microb. 16:20. doi: 10.1186/s40793-021-00389-8
Challacombe, J. F., Hesse, C. N., Bramer, L. M., McCue, L. A., Lipton, M., Purvine, S., et al. (2019). Genomes and secretomes of Ascomycota fungi reveal diverse functions in plant biomass decomposition and pathogenesis. BMC. Genom. 20:976. doi: 10.1186/s12864-019-6358-x
Chauhan, P., Bhattacharya, A., Giri, V. P., Singh, S. P., Gupta, S. C., Verma, P., et al. (2022). Bacillus subtilis suppresses the charcoal rot disease by inducing defence responses and physiological attributes in soybean. Arch. Microbiol. 204:266. doi: 10.1007/s00203-022-02876-z
Chen, X. L., Sun, M. C., Chong, S. L., Si, J. P., and Wu, L. S. (2022). Transcriptomic and Metabolomic Approaches Deepen Our Knowledge of Plant-Endophyte Interactions. Front. Plant. Sci. 12:700200. doi: 10.3389/fpls.2021.700200
Cheng, C., Nie, Z. W., Wang, R., He, L. Y., and Sheng, X. F. (2020). Metal(loid)-resistant Bacillus megaterium H3 reduces arsenic uptake in rice(Oryza sativa Nanjing 5055)at different growth stages in arsenic-contaminated soil. Geoderma 375:114510. doi: 10.1016/j.geoderma.2020.114510
Chowdhary, K., and Sharma, S. (2017). Potential of fungal endophytes in plant growth and disease management. Plant-Microb. Interact. Agro-Ecol. Perspect. 6, 275–290. doi: 10.1007/978-981-10-5813-4-14
Compant, S., Cambon, M. C., Vacher, C., Mitter, B., Samad, A., and Sessitsch, A. (2021). The plant endosphere world - bacterial life within plants. Environ. Microbiol. 23, 1812–1829. doi: 10.1111/1462-2920.15240
Cui, J. L., Guo, S. X., and Xiao, P. G. (2017). Interaction between endophytes and host plant and the role of endophytes in genuineness analysis of medicinal plant. Acta. Pharmaceutica. Sin. 52, 214–221.
Diabankana, R. G. C., Afordoanyi, D. M., Safin, R. I., Nizamov, R. M., Karimova, L. Z., and Validov, S. Z. (2021). Antifungal Properties, Abiotic Stress Resistance, and Biocontrol Ability of Bacillus mojavensis PS17. Curr. Microbiol. 78, 3124–3132. doi: 10.1007/s00284-021-02578-7
Ding, L., Münch, J., Goerls, H., Maier, A., Fiebig, H. H., Lin, W. H., et al. (2010). Xiamycin, a pentacyclic indolosesquiterpene with selective anti-HIV activity from a bacterial mangrove endophyte. Bioorg. Med. Chem. Lett. 20, 6685–6687. doi: 10.1016/j.bmcl.2010.09.010
Ding, Z., Wang, X., Kong, F. D., Huang, H. M., Zhao, Y. N., Liu, M., et al. (2020). Overexpression of Global Regulator Talae1 Leads to the Discovery of New Antifungal Polyketides From Endophytic Fungus Trichoderma afroharzianum. Front. Microbiol. 11:622785. doi: 10.3389/fmicb.2020.622785
Eid, A. M., Fouda, A., Abdel-Rahman, M. A., Salem, S. S., Elsaied, A., Oelmüller, R., et al. (2021). Harnessing Bacterial Endophytes for Promotion of Plant Growth and Biotechnological Applications: an Overview. Plants 10:935. doi: 10.3390/plants10050935
Eida, A. A., Bougouffa, S., L’Haridon, F., Alam, I., Weisskopf, L., Bajic, V. B., et al. (2020). Genome Insights of the Plant-Growth Promoting Bacterium Cronobacter muytjensii JZ38 With Volatile-Mediated Antagonistic Activity Against Phytophthora infestans. Front. Microbiol. 11:369. doi: 10.3389/fmicb.2020.00369
Etminani, F., and Harighi, B. (2018). Isolation and Identification of Endophytic Bacteria with Plant Growth Promoting Activity and Biocontrol Potential from Wild Pistachio Trees. Plant. Pathol. J. 34, 208–217. doi: 10.5423/PPJ.OA.07.2017.0158
Fan, D., Subramanian, S., and Smith, D. L. (2020). Plant endophytes promote growth and alleviate salt stress in Arabidopsis thaliana. Sci. Rep. 10:12740. doi: 10.1038/s41598-020-69713-5
Feygenberg, O., Diskin, S., Maurer, D., and Alkan, N. (2021). Effect of Biological and Chemical Treatments During Flowering on Stem-End Rot Disease, and Mango Yield. Plant. Dis. 105, 1602–1609. doi: 10.1094/PDIS-03-19-0612-RE
Flores, F. R., Camacho, H. B., González, E. D., Trujillo, J. A. R., Beltrán, L. F. L. A., Medina, N. R., et al. (2018). Draft Genome Sequences of Endophytic Isolates of Klebsiella variicola and Klebsiella pneumoniae Obtained from the Same Sugarcane Plant. Genome Announc. 6, e147–e118. doi: 10.1128/genomeA.00147-18
Fn, A., He, B., Sn, C., Ef, D., Gsj, E., and Mam, F. (2021). Whole genome sequence of Pantoea agglomerans ANP8, a salinity and drought stress-resistant bacterium isolated from alfalfa (Medicago sativa L.) root nodules, Biotechnology. Rep 29:e00600. doi: 10.1016/j.btre.2021.e00600
Gao, Y., Ning, Q., Yang, Y., Liu, Y., Niu, S., Hu, X., et al. (2021). Endophytic Streptomyces hygroscopicus OsiSh-2-Mediated Balancing between Growth and Disease Resistance in Host Rice. mBio 12:e0156621. doi: 10.1128/mBio.01566-21
Glick, B. R., and Stearns, J. C. (2011). Making phytoremediation work better: maximizing a plant’s growth potential in the midst of adversity. Int. J. Phytoremed. 1, 4–16. doi: 10.1080/15226514.2011.568533
Grabka, R., d’Entremont, T. W., Adams, S. J., Walker, A. K., Tanney, J. B., Abbasi, P. A., et al. (2022). Fungal Endophytes and Their Role in Agricultural Plant Protection against Pests and Pathogens. Plants 11:384. doi: 10.3390/plants11030384
Guo, D. J., Singh, R. K., Singh, P., Li, D. P., Sharma, A., Xing, Y. X., et al. (2020). Complete Genome Sequence of Enterobacter roggenkampii ED5, a Nitrogen Fixing Plant Growth Promoting Endophytic Bacterium With Biocontrol and Stress Tolerance Properties, Isolated From Sugarcane Root. Front. Microbiol. 11:580081. doi: 10.3389/fmicb.2020.580081
Gupta, S., Chaturvedi, P., Kulkarni, M. G., and Van Staden, J. (2020). A critical review on exploiting the pharmaceutical potential of plant endophytic fungi. Biotechnol. Adv. 39:107462. doi: 10.1016/j.biotechadv.2019.107462
Gutjahr, C. (2014). Phytohormone signaling in arbuscular mycorhiza development. Curr. Opin. Plant. Biol. 20, 26–34. doi: 10.1016/j.pbi.2014.04.003
Guzmán, M. H., Hernández, V. P., Noya, Y. E. N., Guido, M. L. L., Verhulst, N., Govaerts, B., et al. (2022). Application of ammonium to a N limited arable soil enriches a succession of bacteria typically found in the rhizosphere. Sci. Rep. 12:4110. doi: 10.1038/s41598-022-07623-4
Hameed, A., Shahina, M., Lai, W. A., Stothard, P., Young, L. S., Lin, S. Y., et al. (2020). Draft genome sequence reveals co-occurrence of multiple antimicrobial resistance and plant probiotic traits in rice root endophytic strain Burkholderia sp. LS-044 affiliated to Burkholderia cepacia complex. J. Glob. Antimicrob. Resist. 20, 28–30. doi: 10.1016/j.jgar.2019.11.017
Hashem, A., Kumar, A., Al-Dbass, A. M., Alqarawi, A. A., Al-Arjani, A. F., Singh, G., et al. (2019). Arbuscular mycorrhizal fungi and biochar improves drought tolerance in chickpea. Saudi. J. Biol. Sci. 26, 614–624. doi: 10.1016/j.sjbs.2018.11.005
Jan, F. G., Hamayun, M., Hussain, A., Iqbal, A., Jan, G., Khan, S. A., et al. (2019). A promising growth promoting Meyerozyma caribbica from Solanum xanthocarpum alleviated stress in maize plants. Biosci. Rep. 39:BSR20190290. doi: 10.1042/BSR20190290
Ji, C., Wang, X., Song, X., Zhou, Q., Li, C., Chen, Z., et al. (2021). Effect of Bacillus velezensis JC-K3 on Endophytic Bacterial and Fungal Diversity in Wheat Under Salt Stress. Front. Microbiol. 12:802054. doi: 10.3389/fmicb.2021.802054
Jiang, W., Pan, R., Wu, C., Xu, L., Abdelaziz, M. E., Oelmüller, R., et al. (2020). Piriformospora indica enhances freezing tolerance and post-thaw recovery in Arabidopsis by stimulating the expression of CBF genes. Plant. Signal. Behav. 15:1745472. doi: 10.1080/15592324.2020.1745472
Jin, D. F. (2014). Expression and Regulation of Small RNAs in the Symbiosis between Leguminous Plants and Microorganisms. Zhejiang: Zhejiang. University.
Kamal, N., Liu, Z., Qian, C., Wu, J., and Zhong, X. (2021). Improving hybrid Pennisetum growth and cadmium phytoremediation potential by using Bacillus megaterium BM18-2 spores as biofertilizer. Microbiol. Res. 242:126594. doi: 10.1016/j.micres.2020.126594
Khaekhum, S., Ekprasert, J., Suebrasri, T., Seemakram, W., Mongkolthanaruk, W., Riddech, N., et al. (2021). Co-Inoculation of an Endophytic and Arbuscular Mycorrhizal Fungus Improve Growth and Yield of Helianthus tuberosus L. under Field Condition. J. Fungi. 7:976. doi: 10.3390/jof7110976
Khaiwa, N., Maarouf, N. R., Darwish, M. H., Alhamad, D. W. M., Sebastian, A., Hamad, M., et al. (2021). Camptothecin’s journey from discovery to WHO Essential Medicine: Fifty years of promise. Eur. J. Med. Chem. 223:113639. doi: 10.1016/j.ejmech.2021.113639
Khan, M. A., Asaf, S., Khan, A. L., Jan, R., Kang, S. M., Kim, K. M., et al. (2020). Thermotolerance effect of plant growth-promoting Bacillus cereus SA1 on soybean during heat stress. BMC Microbiol. 20:175. doi: 10.1186/s12866-020-01822-7
Khattab, A. R., and Farag, M. A. (2021). Marine and terrestrial endophytic fungi: a mine of bioactive xanthone compounds, recent progress, limitations, and novel applications. Crit. Rev. Biotechnol. 15, 1–28.
Kidd, P. S., Álvarez, A., Álvarez-López, V., Cerdeira-Pérez, A., Rodríguez-Garrido, B., Prieto-Fernández, A., et al. (2021). Beneficial traits of root endophytes and rhizobacteria associated with plants growing in phytomanaged soils with mixed trace metal-polycyclic aromatic hydrocarbon contamination. Chemosphere 277:130272. doi: 10.1016/j.chemosphere.2021.130272
Koskimäki, J. J., Pohjanen, J., Kvist, J., Fester, T., Härtig, C., Podolich, O., et al. (2022). The meristem-associated endosymbiont Methylorubrum extorquens DSM13060 reprograms development and stress responses of pine seedlings. Tree. Physiol. 42, 391–410. doi: 10.1093/treephys/tpab102
Kurokawa, M., Nakano, M., Kitahata, N., Kuchitsu, K., and Furuya, T. (2021). An efficient direct screening system for microorganisms that activate plant immune responses based on plant-microbe interactions using cultured plant cells. Sci. Rep. 11:7396. doi: 10.1038/s41598-021-86560-0
Kusari, S., Hertweck, C., and Spiteller, M. (2012a). Chemical ecology of endophytic fungi: origins of secondary metabolites. Chem. Biol. 19, 792–798. doi: 10.1016/j.chembiol.2012.06.004
Kusari, S., Verma, V. C., Lamshoeft, M., and Spiteller, M. (2012b). An endophytic fungus from Azadirachta indica. Juss. that produces azadirachtin. World. J. Microbiol. Biotechnol. 28, 1287–1294. doi: 10.1007/s11274-011-0876-2
Kusari, S., Zühlke, S., and Spiteller, M. (2011). Effect of artificial reconstitution of the interaction between the plant Camptotheca acuminata and the fungal endophyte Fusarium solani on camptothecin biosynthesis. J. Nat. Prod. 74, 764–775. doi: 10.1021/np1008398
Kuźniar, A., Włodarczyk, K., Grza̧dziel, J., Goraj, W., Gała̧zka, A., and Wolińska, A. (2020). Culture-independent analysis of an endophytic core microbiome in two species of wheat: Triticum aestivum L. (cv. ‘Hondia’) and the first report of microbiota in Triticum spelta L. (cv. ‘Rokosz’). Syst. Appl. Microbiol. 43:126025. doi: 10.1016/j.syapm.2019.126025
Lastochkina, O. (2019). “Bacillus subtilis-Mediated Abiotic Stress Tolerance in Plants,” in Bacilli and Agrobiotechnology: Phytostimulation and Biocontrol. Bacilli in Climate Resilient Agriculture and Bioprospecting, eds M. Islam, M. Rahman, P. Pandey, M. Boehme, and G. Haesaert (Cham: Springer), doi: 10.1007/978-3-030-15175-1_6
Lekota, K. E., Bezuidt, O. K. I., Mafofo, J., Rees, J., Muchadeyi, F. C., Madoroba, E., et al. (2018). Whole genome sequencing and identification of Bacillus endophyticus and B. anthracis isolated from anthrax outbreaks in South Africa. BMC Microbiol. 18:67. doi: 10.1186/s12866-018-1205-9
Li, D., Zheng, X., Lin, L., An, Q., Jiao, Y., Li, Q., et al. (2022). Remediation of soils co-contaminated with cadmium and dichlorodiphenyltrichloroethanes by king grass associated with Piriformospora indica: Insights into the regulation of root excretion and reshaping of rhizosphere microbial community structure. J. Hazard. Mater. 422:126936. doi: 10.1016/j.jhazmat.2021.126936
Li, Y., Kong, D., Fu, Y., Sussman, M. R., and Wu, H. (2020). The effect of developmental and environmental factors on secondary metabolites in medicinal plants. Plant. Physiol. Biochem. 148, 80–89. doi: 10.1016/j.plaphy.2020.01.006
Li, Z., Song, X., Wang, J., Bai, X., Gao, E., and Wei, G. (2018). Nickel and cobalt resistance properties of Sinorhizobium meliloti isolated from Medicago lupulina growing in gold mine tailing. PeerJ 6:e5202. doi: 10.7717/peerj.5202
Lin, L. (2015). The conditional metabolic models based on omics dataintegration. Shanghai: Jiao. Tong. University.
Lipps, S. M., and Samac, D. A. (2022). Pseudomonas viridiflava: An internal outsider of the Pseudomonas syringae species complex. Mol. Plant. Pathol. 23, 3–15. doi: 10.1111/mpp.13133
Liu, B., Huang, L., Buchenauer, H., and Kang, Z. (2010). Isolation and partial characterization of an antifungal protein from the endophytic Bacillus subtilis strain EDR4, Pesticide. Biochem. Physiol. 98, 305–311. doi: 10.1016/j.pestbp.2010.07.001
Liu, T. H., Zhang, X. M., Tian, S. Z., Chen, L. G., and Yuan, J. L. (2020). Bioinformatics analysis of endophytic bacteria related to berberine in the Chinese medicinal plant Coptis teeta Wall. 3. Biotech. 10:96. doi: 10.1007/s13205-020-2084-y
Liu, T., Ren, Z., Chunyu, W. X., Li, G. D., Chen, X., Zhang, Z. T., et al. (2022). Exploration of Diverse Secondary Metabolites From Streptomyces sp. YINM00001, Using Genome Mining and One Strain Many Compounds Approach. Front. Microbiol. 13:831174. doi: 10.3389/fmicb.2022.831174
Lubna, S., Asaf, M., Khan, A. L., Waqas, M., Khan, M. A., et al. (2018). Salt tolerance of Glycine max induced by endophytic fungus Aspergillus flavus CSH1, via regulating its endogenous hormones and antioxidative system. Plant. Physiol. Biochem. 128, 13–23. doi: 10.1016/j.plaphy.2018.05.007
Martinez-Medina, A., Flors, V., Heil, M., Mauch-Mani, B., and Corné, M. J. (2016). Recognizing Plant Defense Priming. Trends. Plant. Ence. 2016, 818–822. doi: 10.1016/j.tplants.2016.07.009
Masmoudi, F., Tounsi, S., Dunlap, C. A., and Trigui, M. (2021). Endophytic halotolerant Bacillus velezensis FMH2 alleviates salt stress on tomato plants by improving plant growth and altering physiological and antioxidant responses. Plant. Physiol. Biochem. 165, 217–227. doi: 10.1016/j.plaphy.2021.05.025
Mei, Y. Z., Zhu, Y. L., Huang, P. W., Yang, Q., and Dai, C. C. (2019). Strategies for gene disruption and expression in filamentous fungi. Appl. Microbiol. Biotechnol. 103, 6041–6059. doi: 10.1007/s00253-019-09953-2
Mengistu, A. A. (2020). Endophytes: Colonization, Behaviour, and Their Role in Defense Mechanism. Int. J. Microbiol. 2020:6927219. doi: 10.1155/2020/6927219
Mishra, A., Singh, S. P., Mahfooz, S., Bhattacharya, A., Mishra, N., Shirke, P.A., et al. (2018a). Bacterial endophytes modulates the withanolide biosynthetic pathway and physiological performance in Withania somnifera under biotic stress. Microbiol. Res. 212–213, 17–28. doi: 10.1016/j.micres.2018.04.006
Mishra, A., Singh, S. P., Mahfooz, S., Singh, S. P., Bhattacharya, A., Mishra, N., et al. (2018b). Endophyte-Mediated Modulation of Defense-Related Genes and Systemic Resistance in Withania somnifera (L.) Dunal under Alternaria alternata Stress. Appl. Environ. Microbiol. 84, e2845–e2817. doi: 10.1128/AEM.02845-17
Mohamad, O. A. A., Li, L., Ma, J. B., Hatab, S., Xu, L., Guo, J. W., et al. (2018). Evaluation of the Antimicrobial Activity of Endophytic Bacterial Populations From Chinese Traditional Medicinal Plant Licorice and Characterization of the Bioactive Secondary Metabolites Produced by Bacillus atrophaeus Against Verticillium dahlia. Front. Microbiol. 9:924. doi: 10.3389/fmicb.2018.00924
Monowar, T., Rahman, M. S., Bhore, S. J., Raju, G., and Sathasivam, K. V. (2019). Secondary Metabolites Profiling of Acinetobacter baumannii Associated with Chili (Capsicum annuum L.) Leaves and Concentration Dependent Antioxidant and Prooxidant Properties. Biomed. Res. Int. 2019:6951927. doi: 10.1155/2019/6951927
Motoyama, T. (2020). Secondary Metabolites of the Rice Blast Fungus Pyricularia oryzae: Biosynthesis and Biological Function. Int. J. Mol. Sci. 21:8698. doi: 10.3390/ijms21228698
Munir, S., Li, Y., He, P., Huang, M., He, P., He, P., et al. (2020). Core endophyte communities of different citrus varieties from citrus growing regions in China. Sci. Rep. 10:3648. doi: 10.1038/s41598-020-60350-6
Naik, S., Shaanker, R. U., Ravikanth, G., and Dayanandan, S. (2019). How and why do endophytes produce plant secondary metabolites? Symbiosis 78, 193–201. doi: 10.1007/s13199-019-00614-6
Nasiruddin, N., Chen, G. Y., Yu, Z. X., and Zhao, T. (2020). Endophytes of Terrestrial Plants: a Potential Source of Bioactive Secondary Metabolites. J. Nutr. Res. 7, 362–377. doi: 10.12691/jfnr-8-7-8
Newman, M. A., Sundelin, T., Nielsen, J. T., and Erbs, G. (2013). MAMP (microbe-associated molecular pattern) triggered immunity in plants. Front. Plant. Sci. 4:139. doi: 10.3389/fpls.2013.00139
Nifakos, K., Tsalgatidou, P. C., Thomloudi, E. E., Skagia, A., Kotopoulis, D., Baira, E., et al. (2021). Genomic Analysis and Secondary Metabolites Production of the Endophytic Bacillus velezensis Bvel1: A Biocontrol Agent against Botrytis cinerea Causing Bunch Rot in Post-Harvest Table Grapes. Plants 10:1716. doi: 10.3390/plants10081716
Nigris, S., Baldan, E., Tondello, A., Zanella, F., Vitulo, N., Favaro, G., et al. (2018). Biocontrol traits of Bacillus licheniformis GL174, a culturable endophyte of Vitis vinifera cv. Glera. BMC Microbiol. 18:133. doi: 10.1186/s12866-018-1306-5
Nouioui, I., Ha, S. M., Baek, I., Chun, J., and Goodfellow, M. (2022). Genome insights into the pharmaceutical and plant growth promoting features of the novel species Nocardia alni sp. BMC Genom. 23:70. doi: 10.1186/s12864-021-08257-y
Nxumalo, C. I., Ngidi, L. S., Shandu, J. S. E., and Maliehe, T. S. (2020). Isolation of endophytic bacteria from the leaves of Anredera cordifolia CIX1 for metabolites and their biological activities. BMC Compl. Med. Ther. 20:300. doi: 10.1186/s12906-020-03095-z
Oukala, N., Aissat, K., and Pastor, V. (2021). Bacterial Endophytes: the Hidden Actor in Plant Immune Responses against Biotic Stress. Plants 10:1012. doi: 10.3390/plants10051012
Pentimone, I., Colagiero, M., Rosso, L. C., and Ciancio, A. (2020). Omics applications: towards a sustainable protection of tomato. Appl. Microbiol. Biotechnol. 104, 4185–4195. doi: 10.1007/s00253-020-10500-7
Pérez-Alonso, M. M. P., Guerrero-Galán, C., Scholz, S. S., Kiba, T., Sakakibara, H., Ludwig-Müller, J., et al. (2020). Harnessing symbiotic plant-fungus interactions to unleash hidden forces from extreme plant ecosystems. J. Exp. Bot. 71, 3865–3877. doi: 10.1093/jxb/eraa040
Pieterse, C. M., Zamioudis, C., Berendsen, R. L., Weller, D. M., Van Wees, S. C., and Bakker, P. A. (2014). Induced systemic resistance by beneficial microbes. Annu. Rev. Phytopathol. 52, 347–375. doi: 10.1146/annurev-phyto-082712-102340
Pozo, M. J., ópez-Ráez, J. A. L., Azcón-Aguilar, C., and García-Garrido, J. M. (2015). Phytohormones as integrators of environmental signals in the regulation of mycorrhizal symbioses. New. Phytol. 205, 1431–1436. doi: 10.1111/nph.13252
Rahman, S. U., Khalid, M., Kayani, S. I., and Tang, K. (2020). The ameliorative effects of exogenous inoculation of Piriformospora indica on molecular, biochemical and physiological parameters of Artemisia annua L. under arsenic stress condition. Ecotoxicol. Environ. Saf. 206:111202. doi: 10.1016/j.ecoenv.2020.111202
Rat, A., Naranjo, H. D., Krigas, N., Grigoriadou, K., Maloupa, E., Alonso, A. V., et al. (2021a). Endophytic Bacteria From the Roots of the Medicinal Plant Alkanna tinctoria Tausch (Boraginaceae): Exploration of Plant Growth Promoting Properties and Potential Role in the Production of Plant Secondary Metabolites. Front. Microbiol. 12:633488. doi: 10.3389/fmicb.2021.633488
Rat, A., Naranjo, H. D., Lebbe, L., Cnockaert, M., Krigas, N., Grigoriadou, K., et al. (2021b). Roseomonas hellenica sp. nov., isolated from roots of wild-growing Alkanna tinctoria. Syst. Appl. Microbiol. 44:126206. doi: 10.1016/j.syapm.2021.126206
Ren, X. M., Guo, S. J., Tian, W., Chen, Y., Han, H., Chen, E., et al. (2019). Effects of Plant Growth-Promoting Bacteria (PGPB) Inoculation on the Growth, Antioxidant Activity, Cu Uptake, and Bacterial Community Structure of Rape (Brassica napus L.) Grown in Cu-Contaminated Agricultural Soil. Front. Microbiol. 10:1455. doi: 10.3389/fmicb.2019.01455
Robinson, R. J., Fraaije, B. A., Clark, I. M., Jackson, R. W., Hirsch, P. R., and Mauchline, T. H. (2016). Wheat seed embryo excision enables the creation of axenic seedlings and Koch’s postulates testing of putative bacterial endophytes. Sci. Rep. 6:25581. doi: 10.1038/srep25581
Rocha, F. Y. O., Negrisoli, A. S., Júnior, G. F., de Matos, P. M., Rossi, C. N., Vidal, M. S., et al. (2021). Endophytic Bacillus Bacteria Living in Sugarcane Plant Tissues and Telchin licus licus Larvae (Drury) (Lepidoptera: Castniidae): the Symbiosis That May Open New Paths in the Biological Control. Front. Microbiol. 12:659965. doi: 10.3389/fmicb.2021.659965
Roy, S., Chakraborty, A. P., and Chakraborty, R. (2021). Understanding the potential of root microbiome influencing salt-tolerance in plants and mechanisms involved at the transcriptional and translational level. Physiol. Plant. 173, 1657–1681. doi: 10.1111/ppl.13570
Sahu, P. K., Singh, S., Singh, U. B., Chakdar, H., Sharma, P. K., Sarma, B. K., et al. (2021). Inter-Genera Colonization of Ocimum tenuiflorum Endophytes in Tomato and Their Complementary Effects on Na+/K+ Balance, Oxidative Stress Regulation, and Root Architecture Under Elevated Soil Salinity. Front. Microbiol. 12:744733. doi: 10.3389/fmicb.2021.744733
Saikia, J., Sarma, R. K., Dhandia, R., Yadav, A., Bharali, R., Gupta, V. K., et al. (2018). Alleviation of drought stress in pulse crops with ACC deaminase producing rhizobacteria isolated from acidic soil of Northeast India. Sci. Rep. 8:3560. doi: 10.1038/s41598-018-21921-w
Schulz, B., Guske, S., Dammann, U., and Boyle, C. (1998). Endophyte-host interactions. II. Defining symbiosis of the endophyte-host interaction. Symbiosis 15, 213–227.
Selosse, M. A., Petrolli, R., Mujica, M. I., Laurent, L., Perez-Lamarque, B., Figura, T., et al. (2022). The Waiting Room Hypothesis revisited by orchids: were orchid mycorrhizal fungi recruited among root endophytes? Ann. Bot. 129, 259–270. doi: 10.1093/aob/mcab134
Shao, F., Wilson, I. W., and Qiu, D. (2021). The Research Progress of Taxol in Taxus. Curr. Pharm. Biotechnol. 22, 360–366. doi: 10.2174/1389201021666200621163333
Shu, X., Zhang, Y., Guan, L., Chen, Z., Huang, M., Chen, X., et al. (2020). Antibacterial secondary metabolites of Clonostachys rosea, an endophytic fungus from Blumea balsamifera (L.) DC. Chinese. J. Biotechnol. 36, 1650–1658. doi: 10.13345/j.cjb.190555
Shurigin, V., Alaylar, B., Davranov, K., Wirth, S., Bellingrath-Kimura, S. D., and Egamberdieva, D. (2021). Diversity and biological activity of culturable endophytic bacteria associated with marigold (Calendula officinalis L.), AIMS. Microbiol. 7, 336–353. doi: 10.3934/microbiol.2021021
Siciliano, V., Genre, A., Balestrini, R., Cappellazzo, G., deWit, P. J., and Bonfante, P. (2007). Transcriptome analysis of arbuscular mycorrhizal roots during development of the prepenetration apparatus. Plant. Physiol. 144, 1455–1466. doi: 10.1104/pp.107.097980
Singh, A., Singh, D. K., Kharwar, R. N., White, J. F., and Gond, S. K. (2021). Fungal Endophytes as Efficient Sources of Plant-Derived Bioactive Compounds and Their Prospective Applications in Natural Product Drug Discovery: insights, Avenues, and Challenges. Microorganisms 9:197. doi: 10.3390/microorganisms9010197
Singh, D., Thapa, S., Mahawar, H., Kumar, D., Geat, N., and Singh, S. K. (2022). Prospecting potential of endophytes for modulation of biosynthesis of therapeutic bioactive secondary metabolites and plant growth promotion of medicinal and aromatic plants. Antonie. Van. Leeuwenhoek. 2022:6. doi: 10.1007/s10482-022-01736-6
Singh, P., Singh, R. K., Guo, D. J., Sharma, A., Singh, R. N., Li, D. P., et al. (2021). Whole Genome Analysis of Sugarcane Root-Associated Endophyte Pseudomonas aeruginosa B18-A Plant Growth-Promoting Bacterium With Antagonistic Potential Against Sporisorium scitamineum. Front. Microbiol. 12:628376. doi: 10.3389/fmicb.2021.628376
Singh, R., and Dubey, A. K. (2018). Diversity and Applications of Endophytic Actinobacteria of Plants in Special and Other Ecological Niches. Front. Microbiol. 9:1767. doi: 10.3389/fmicb.2018.01767
Singh, S. P., and Gaur, R. (2016). Evaluation of antagonistic and plant growth promoting activities of chitinolytic endophytic actinomycetes associated with medicinal plants against Sclerotium rolfsii in chickpea. J. Appl. Microbiol. 121, 506–518. doi: 10.1111/jam.13176
Singh, S. P., and Gaur, R. (2017). Endophytic Streptomyces spp. underscore induction of defense regulatory genes and confers resistance against Sclerotium rolfsii in chickpea, Biological. Control 104, 44–56. doi: 10.1016/j.biocontrol.2016.10.011
Sirikantaramas, S., Yamazaki, M., and Saito, K. (2009). A survival strategy: the coevolution of the camptothecin biosynthetic pathway and self-resistance mechanism. Phytochemistry 70, 1894–1898. doi: 10.1016/j.phytochem.2009.07.034
Snak, A., Vendruscolo, E. C. G., Santos, M. F. D., Fiorini, A., and Mesa, D. (2021). Genome sequencing and analysis of plant growth-promoting attributes from Leclercia adecarboxylata. Genet. Mol. Biol. 44:e20O200130. doi: 10.1590/1678-4685-GMB-2020-0130
Song, Q. Y., Li, F., Nan, Z. B., Coulter, J. A., and Wei, W. J. (2020). Do Epichloë Endophytes and Their Grass Symbiosis Only Produce Toxic Alkaloids to Insects and Livestock? J. Agric. Food. Chem. 68, 1169–1185. doi: 10.1021/acs.jafc.9b06614
Su, Z., Zeng, Y., Li, X., Perumal, A. B., Zhu, J., Lu, X., et al. (2021). The Endophytic Fungus Piriformospora Indica-Assisted Alleviation of Cadmium in Tobacco. J. Fungi. 7:675. doi: 10.3390/jof7080675
Subudhi, E., Sahoo, D. R. K., Dey, S., Das, A., and Sahoo, K. (2018). Unraveling Plant-Endophyte Interactions: An Omics Insight. Switzerland: Springer Nature, 249–267. doi: 10.1007/978-3-319-76900-4_2-1
Tariq, M., Jameel, F., Ijaz, U., Abdullah, M., and Rashid, K. (2022). Biofertilizer microorganisms accompanying pathogenic attributes: a potential threat. Physiol. Mol. Biol. Plants 28, 77–90. doi: 10.1007/s12298-022-01138-y
Teixeira, P. J. P., Colaianni, N. R., Fitzpatrick, C. R., and Dangl, J. L. (2019). Beyond pathogens: microbiota interactions with the plant immune system. Curr. Opin. Microbiol. 49, 7–17. doi: 10.1016/j.mib.2019.08.003
Thomloudi, E. E., Tsalgatidou, P. C., Baira, E., Papadimitriou, K., Venieraki, A., and Katinakis, P. (2021). Genomic and Metabolomic Insights into Secondary Metabolites of the Novel Bacillus halotolerans Hil4, an Endophyte with Promising Antagonistic Activity against Gray Mold and Plant Growth Promoting Potential. Microorganisms 9:2508. doi: 10.3390/microorganisms9122508
Tooker, J. F., and Giron, D. (2020). The Evolution of Endophagy in Herbivorous Insects. Front. Plant. Sci. 11:581816. doi: 10.3389/fpls.2020.581816
Tsalgatidou, P. C., Thomloudi, E. E., Baira, E., Papadimitriou, K., Skagia, A., Venieraki, A., et al. (2022). Integrated Genomic and Metabolomic Analysis Illuminates Key Secreted Metabolites Produced by the Novel Endophyte Bacillus halotolerans Cal.l.30 Involved in Diverse Biological Control Activities. Microorganisms 10:399. doi: 10.3390/microorganisms10020399
Ulrich, K., Kube, M., Becker, R., Schneck, V., and Ulrich, A. (2021). Genomic Analysis of the Endophytic Stenotrophomonas Strain 169 Reveals Features Related to Plant-Growth Promotion and Stress Tolerance. Front. Microbiol. 12:687463. doi: 10.3389/fmicb.2021.687463
Varga, T., Hixson, K. K., Ahkami, A. H., Sher, A. W., Barnes, M. E., Chu, R. K., et al. (2020). Endophyte-Promoted Phosphorus Solubilization in Populus. Front. Plant. Sci. 11:567918. doi: 10.3389/fpls.2020.567918
Wang, H. W., Ma, C. Y., Xu, F. J., Lu, F., Zhang, W., and Dai, C. C. (2021). Root endophyte-enhanced peanut-rhizobia interaction is associated with regulation of root exudates. Microbiol. Res. 250:126765. doi: 10.1016/j.micres.2021.126765
Wang, Z., Zhu, J., Li, W., Li, R., Wang, X., Qiao, H., et al. (2020). Antibacterial mechanism of the polysaccharide produced by Chaetomium globosum CGMCC 6882 against Staphylococcus aureus. Int. J. Biol. Macromol. 159, 231–235. doi: 10.1016/j.ijbiomac.2020.04.269
Wasternack, C., and Hause, B. (2013). Jasmonates: biosynthesis, perception, signal transduction and action in plant stress response, growth and development. An update to the 2007 review in Annals of Botany. Ann. Bot. 111, 1021–1058. doi: 10.1093/aob/mct067
Worsley, S. F., Newitt, J., Rassbach, J., Batey, S. F. D., Holmes, N. A., Murrell, J. C., et al. (2020). Streptomyces Endophytes Promote Host Health and Enhance Growth across Plant Species. Appl. Environ. Microbiol. 86, e1053–e1020. doi: 10.1128/AEM.01053-20
Wu, L., Li, X., Ma, L., Blom, J., Wu, H., Gu, Q., et al. (2020). The “pseudo-pathogenic” effect of plant growth-promoting Bacilli on starchy plant storage organs is due to their α-amylase activity which is stimulating endogenous opportunistic pathogens. Appl. Microbiol. Biotechnol. 104, 2701–2714. doi: 10.1007/s00253-020-10367-8
Wu, W., Chen, W., Liu, S., Wu, J., Zhu, Y., Qin, L., et al. (2021). Beneficial Relationships Between Endophytic Bacteria and Medicinal Plants. Front. Plant. Sci. 12:646146. doi: 10.3389/fpls.2021.646146
Xiao, J. L., Sun, J. G., Pang, B., Zhou, X., Gong, Y., Jiang, L., et al. (2021). Isolation and screening of stress-resistant endophytic fungus strains from wild and cultivated soybeans in cold region of China. Appl. Microbiol. Biotechnol. 105, 755–768. doi: 10.1007/s00253-020-11048-2
Xu, J. X., Li, Z. Y., Lv, X., Yan, H., Zhou, G. Y., Cao, L. X., et al. (2020). Isolation and characterization of Bacillus subtilis strain 1-L-29, an endophytic bacteria from Camellia oleifera with antimicrobial activity and efficient plant-root colonization. PLoS One 15:e0232096. doi: 10.1371/journal.pone.0232096
Yan, L., Zhu, J., Zhao, X., Shi, J., Jiang, C., and Shao, D. (2019). Beneficial effects of endophytic fungi colonization on plants. Appl. Microbiol. Biotechnol. 103, 3327–3340. doi: 10.1007/s00253-019-09713-2
Yin, D. D., Wang, Y. L., Yang, M., Yin, D. K., Wang, G. K., and Xu, F. (2019). Analysis of Chuanxiong Rhizoma substrate on production of ligustrazine in endophytic Bacillus subtilis by ultra high performance liquid chromatography with quadrupole time-of-flight mass spectrometry. J. Sep. Sci. 42, 3067–3076. doi: 10.1002/jssc.201900030
Zeng, Q., Shi, G., Nong, Z., Ye, X., and Hu, C. (2020). Complete Genome Sequence of Pantoea ananatis Strain NN08200, an Endophytic Bacterium Isolated from Sugarcane. Curr. Microbiol. 77, 1864–1870. doi: 10.1007/s00284-020-01972-x
Zhang, D., Xu, H., Gao, J., Portieles, R., Du, L., Gao, X., et al. (2021). Endophytic Bacillus altitudinis Strain Uses Different Novelty Molecular Pathways to Enhance Plant Growth. Front. Microbiol. 12:692313. doi: 10.3389/fmicb.2021.692313
Zhang, M. J., Zhu, L., and Xia, Q. Z. (2021). Research progress on the regulation of plant hormones to stress responses. J. Hubei. Univ. 43, 242–253. doi: 10.3969/j.issn.1000-2375.2021.03.003
Zhou, J. Y., Sun, K., Chen, F., Yuan, J., Li, X., and Dai, C. C. (2018). Endophytic Pseudomonas induces metabolic flux changes that enhance medicinal sesquiterpenoid accumulation in Atractylodes lancea. Plant Physiol. Biochem. 130, 473–481. doi: 10.1016/j.plaphy.2018.07.016
Keywords: endophytes, interactive relationships, growth and development, secondary metabolites, biosynthesis mechanism
Citation: Li Z, Wen W, Qin M, He Y, Xu D and Li L (2022) Biosynthetic Mechanisms of Secondary Metabolites Promoted by the Interaction Between Endophytes and Plant Hosts. Front. Microbiol. 13:928967. doi: 10.3389/fmicb.2022.928967
Received: 26 April 2022; Accepted: 21 June 2022;
Published: 11 July 2022.
Edited by:
Tofazzal Islam, Bangabandhu Sheikh Mujibur Rahman Agricultural University, BangladeshReviewed by:
Satyendra Pratap Singh, National Botanical Research Institute (CSIR), IndiaMusrat Zahan Surovy, Bangabandhu Sheikh Mujibur Rahman Agricultural University, Bangladesh
Ahmed Eid, Al-Azhar University, Egypt
Sandeep Kumar Singh, Centre of Bio-Medical Research (CBMR), India
Copyright © 2022 Li, Wen, Qin, He, Xu and Li. This is an open-access article distributed under the terms of the Creative Commons Attribution License (CC BY). The use, distribution or reproduction in other forums is permitted, provided the original author(s) and the copyright owner(s) are credited and that the original publication in this journal is cited, in accordance with accepted academic practice. No use, distribution or reproduction is permitted which does not comply with these terms.
*Correspondence: Yuqi He, MTg0ODYwNDY2QHFxLmNvbQ==; Delin Xu, eHVkZWxpbjIwMDBAMTYzLmNvbQ==; Lin Li, bGlsaW56bWMyMDE1QDE2My5jb20=