- 1State Key Laboratory of Animal Nutrition, Institute of Animal Science, Chinese Academy of Agricultural Sciences, Beijing, China
- 2Yunnan Provincial Key Laboratory of Animal Nutrition and Feed Science, College of Animal Science and Technology, Yunnan Agricultural University, Kunming, China
- 3Precision Livestock and Nutrition Unit, Gembloux Agro-Bio Tech, Liège University, Gembloux, Belgium
The effects of multi-enzymes mixture supplementation or combination with inactivated Lactobacillus on growth performance, intestinal barrier, and cecal microbiota were investigated in broilers at the age of 15–42 days fed a wheat-based diet. A total of 576 broilers (12 broilers/cage; n = 12) were used and divided into four groups and randomly allotted to four experimental diets throughout grower (15–28 days of age) and finisher (29–42 days of age) phases. Diets consisted of a corn-soybean meal-based diet (BD), a wheat-soybean meal-based diet (WD), and WD supplemented multi-enzymes (WED) or combined with inactivated Lactobacillus (WEPD). The results showed that the average daily gain (ADG) and body weight (BW) were reduced in broilers fed WD diet compared with those fed BD diet during the grower period (P < 0.05). Broilers in the WED or WEPD group had higher ADG and BW during the grower period (P < 0.05) and had a lower feed-to-gain ratio (F/G) compared to broilers in the WD group during the grower and overall periods (P < 0.05). Improved expression of intestinal barrier genes (claudin-1, ZO-1, and mucin-2) was observed in WEPD compared to the BD or WD group (P < 0.05). Compared to the BD group, the WD group decreased the abundance of Oscillospira, norank_f__Erysipelotrichaceae, and Peptococcus, which are related to anti-inflammatory function and BW gain. The WD also increased Bifidobacterium and some short-chain fatty acid (SCFA)-producing bacteria (Anaerotruncus, Blautia, and Oscillibacter), and Barnesiella, which were presumed as “harmful microbes” [false discovery rate (FDR) < 0.05]. WED and WEPD groups, respectively, improved Bilophila and Eubacterium_hallii_group compared with those in the WD group (FDR < 0.05). In addition, the Enterococcus abundance was reduced in the WEPD group compared to the WD group (FDR < 0.05). Higher acetate and total SCFA concentrations were observed (P < 0.05) among broilers who received a WD diet. Compared with the WD group, the WED or WEPD group further increased cecal propionate content (P < 0.05) and tended to improve butyrate concentration. These results suggested that supplemental multi-enzymes alone and combined with inactivated Lactobacillus could improve the growth performance based on the wheat-based diet and offer additional protective effects on the intestinal barrier function of broilers.
Introduction
Wheat is widely used in poultry diets across Europe, consisting of up to 70% by weight of the diet (Ball et al., 2013). However, non-starch polysaccharides (NSPs) present in wheat could reduce feed nutrient digestion and growth performance of broiler (Kiarie et al., 2014; Romero et al., 2014). The NSP, a kind of complex high molecular weight carbohydrate, can be categorized into water-soluble NSP or insoluble NSP (Musigwa et al., 2021). The NSP disturbs digestion and absorption by resisting the digestion of endogenous enzymes (Nguyen et al., 2021), increasing the viscosity of the intestinal contents (Knudsen, 2014; Munyaka et al., 2016), or blocking the nutrients’ access to endogenous digestive enzymes in poultry (Choct et al., 2004; Musigwa et al., 2021). Moreover, intestinal microbiota composition is impacted by NSP in wheat-based diets (Munyaka et al., 2016; Wang et al., 2021b), which further leads to growth performance reduction (Crisol-Martínez et al., 2017) and intestinal physiological disfunction (Raza et al., 2019). Crisol-Martínez et al. (2017) reported that several operational taxonomic units (OTUs) belonging to Clostridiales are prevalent in wheat-fed chickens and associated with low FCR values. Also, other studies found correlations between the cecal microbiota composition and growth performance in broilers fed wheat-based diets (Torok et al., 2011; Stanley et al., 2012).
Supplementation of NSP-degrading enzymes or probiotics has been commonly accepted to eliminate the possible antinutritional effects of wheat-based diets and improve production performance and intestinal health. Studies showed that an enzyme mixture addition in wheat diets improved nutrient digestibility and broiler performance, especially for high NSP concentration wheat (Adeola and Cowieson, 2011; Smeets et al., 2018). The beneficial mechanisms of NSPases include the release of nutrients and the reducing chyme viscosity through hydrolysis. However, McCafferty et al. (2019b) reported that no effects of supplementation of NSPases on growth performance and cecal short-chain fatty acids (SCFAs) concentrations were observed. Rebolé et al. (2010) also found that enzymes added to the diet have no effects on specific selected bacterial populations. These inconsistent observations could be ascribed to the specificity of NSPases for specific NSP or feed ingredients (Smeets et al., 2014) and the various quality of wheat (Choct et al., 1995). On the other hand, supplementation of probiotics also can improve the growth performance and regulate the balance of bacteria in broilers (Alagawany et al., 2018; Rehman et al., 2020). A previous study showed that diet addition inactivated Lactobacillus to broilers diet increased body weight (BW) gain and FCR, and hypertrophied intestinal absorptive epithelial cells are found at the villus apical surface (Khonyoung and Yamauchi, 2019). In agreement with that finding, Incharoen et al. (2019) observed that adding inactivated Lactobacillus improved the growth performance and immune function in broilers. Besides, in yellow-feathered broilers, diet supplementation with heat-inactivated compound probiotics (Bacillus subtilis and Lactobacillus acidophilus BFI) modulated the community composition of cecal microbiota, including the high abundance of Barnesiellaceae, Barnesiella, and Lactobacillus aviaries, whereas the low abundance of Lachnoclostridium and Peptococcus, consequently enhanced feed efficiency and decreased plasma cholesterol and creatinine contents (Zhu et al., 2020). At present, most studies focused on the effects of a single NSP enzyme or probiotics. However, there is a limited understanding of the combined effects of multi-enzymes and probiotics on growth performance, intestinal barrier function, and intestinal microbiota in broiler fed a wheat diet.
Therefore, this study investigated the effects of NSP enzyme mixture and combined with probiotics on growth performance, intestinal barrier, gut microbiota, and microbial metabolites in broilers fed wheat diet from 15 to 42 days of age.
Materials and methods
Enzyme mixture and probiotics
The kinds and amounts of multi-enzymes used in this study were screened and optimized by our previous study (Zhang et al., 2017). The multi-enzymes included cellulase, xylanase, β-mannanase, α-galactosidase, β-glucanase, and pectinase. The addition amount was 1,117.9, 35,087.7, 1,917.1, 305.0, 806.7, and 133.7 U/kg of diet, respectively. The probiotic was inactivated Lactobacillus, containing more than 1 × 1010 inactivated bacterial cells/g, and was supplemented to diet at a level of 800 mg/kg recommended by the company (Shandong Biocom Biotech Co., Ltd., Linyi, China), which has been investigated and obtained a good input-output ratio in our previous experiments (data unpublished).
Birds, housing, and experimental diets
One-day-age Arbor Acres broilers were randomly assigned into 48 cages containing 12 broilers each. All broilers were fed a corn-based diet for the first 14 days. On day 15, a total of 576 chicks were used and individually weighed (406.2 ± 9.8 g), then divided into 4 groups with 12 replicates per group and 12 broilers per replicate, to evaluate the effects of the 4 different diets on broilers in a completely randomized design. Broilers were offered a grower diet from day 15 to 28, and a finisher ration from day 15 to 28. Birds were kept in three-layer pens (150 cm × 70 cm × 60 cm for one cell). Feed and water were supplied ad libitum with a light schedule of 23-h light and 1-h darkness per day throughout the entire experiment. The room temperature gradually decreased from 35 (d1–d3) to 24°C (d28). After 28 days of age, the temperature was kept at 22∼24°C until the end of the experiment.
The diets contained a corn-based diet (BD), wheat replacement diet (WD), and wheat replacement diet with an enzyme mixture alone or combined probiotics (WED or WEPD), resulting in four diet treatments for grower and finisher stages, respectively. All diets met or exceeded the nutrient requirements of broilers according to the National Research Council (1994) (Table 1). All diets were fed in mash form. No antibiotics were offered to broilers throughout the trial.
Chemical analysis
All experimental diets were ground to pass through a 0.43-mm screen before analyses. Dry matter (method 934.01) and crude protein (nitrogen × 6.25, method 990.03) contents of diets were determined according to the AOAC (2012). Gross energy contents in the diet samples were analyzed using an adiabatic oxygen bomb calorimeter (model 6400, Parr Instruments, Moline, IL, United States).
Growth performance and sample collection
Birds and their feed in each replicate cage were weighted and recorded at the beginning and end of each period (d15, d28, and d42), respectively, to calculate average BW, average daily gain (ADG), and average daily feed intake (ADFI) of each treatment during the grower (15–28 days), finisher (29–42 days), and overall (15-42 days) periods. The ratio between ADFI and ADG was also calculated (F/G).
At the end of the experiment, chickens were selected for intestinal sample collection. One bird from each cage was chosen according to the BW which was closed to the average, with a total of eight individuals from each treatment. The jejunal mucosa and cecal digesta were collected and frozen at –80°C for further analysis.
Inflammatory cytokines and tight junction gene expression
Total RNA of the jejunal mucosa was isolated using the RNeasy Kit (Qiagen, Hilden, Germany) according to the manufacturer’s directions. The reverse transcription was completed using a Prime Script RT Kit (Takara, Kusatsu, Shiga, Japan). The quantitative real-time-PCR was conducted on a CFX96 real-time PCR detection system (Bio-Rad, CA, United States) according to the SYBR Premix Ex Taq II recommendations (Takara, Kusatsu, Shiga, Japan). The reaction protocol was 30 s for predenaturation at 95°C, 5 s for 40 cycles of denaturation at 95°C, 30 s for annealing at 60?, and 10 s for an extension at 95?, then melt curve analysis was done. All primer sequences for genes associated with immune function and intestinal barrier function are provided in Supplementary Table 1. β-Actin was used as an internal reference gene, and the relative gene expression was calculated by the 2–ΔΔCt Ct method (Livak and Schmittgen, 2001).
Intestinal microbiota
The extraction of DNA of cecal digesta samples was performed using the Qiagen DNA isolation kit (Qiagen, Hilden, Germany), following the instructions of the manufacturers. The V3–V4 region of the bacterial 16S rRNA gene was amplified with primers 338F (5′-barcodeACTCCTACGGGAGGCAGCAG-3′) and 806R (5′-GGACTACHVGGGTWTCTAAT-3′). The purified amplicons were paired-end sequenced (2 × 300) on the Illumina HiSeq sequencing platform (Illumina, San Diego, CA, United States) according to the standard protocols by Majorbio Bio-Pharm Technology Co. Ltd. (Shanghai, China), as previously described (Tang et al., 2021a).
Raw fastq files were demultiplexed and quality-filtered by QIIME (version 1.70). OTUs were clustered using a 97% similarity cut-off with UPARSE (version 7.1), and chimeric sequences were removed using UCHIME (version 7.1). OUT representative sequence was obtained based on the RDP classifier with a confidence threshold of 0.7 (Wang et al., 2007).
Short-chain fatty acid concentration
The short-chain fatty acid determination in the caecum digesta was performed according to the procedure described previously (Tang et al., 2021b). In brief, the digesta (about 0.5 g) was extracted with ultrapure water, and centrifugation at 10,000 × g to obtain the extracts. Metaphosphoric acid (25%, w/v) was mixed with the extracts at a ratio of 1:9. After centrifugation at 12,000 × g, the supernatant was passed through the 0.45-μm Milled-LG filter (Millipore, Billerica, MA, United States) and subjected to SCFA analysis with the Agilent 7890 N gas chromatograph (Agilent, Santa Clara, CA, United States).
Statistical analysis
Data on growth performance, carcass traits, and intestinal morphology were analyzed by one-way ANOVA with Duncan’s multiple comparison test to determine differences among treatments using SAS 8.0 (SAS Institute, Inc., Cary, NC, United States). The data from a replicate cage were calculated as the experimental unit for the growth performance evaluation, involving BW and feed intake. The bird individual from each cage was regarded as the basic unit for physiological and microbial analysis.
Microbial analysis was performed using the online platform of Majorbio I-Sanger Cloud Platform.1 Bacterial alpha-diversity indices (Sobs, Shannon, ACE, and Chao) were analyzed using Wilcoxon rank-sum test, and the principal coordinate (PCoA, beta-diversity) analysis based on the unweighted unifrac and ANOSIM test was employed. Also, a partial least squares discriminant analysis was performed to predict the group to which the samples belonged, according to the diet. After the OUT data were employed in the CSS normalization, the analysis of differential genera between groups was calculated by the DESeq2 method (MicrobiomeAnalyst2) with corrected p-value [false discovery rate (FDR)] < 0.05.
Results
Growth performance
In Table 2, the initial BW at 14 days was not different among the four groups (P = 0.92). Birds fed the WD diet showed lower ADG and BW by 28 days compared with those fed the BD diet (P < 0.05). Whereas, the WED group or the WEPD group improved the ADG and BW (P < 0.05) compared to the WD group (P < 0.05), which was similar to the BD group. The WED group decreased ADFI (P < 0.05) but did not affect BW at 42 days (P > 0.05). In addition, compared to the WD group, WED and WEPD groups decreased the F/G during the grower and overall periods (P < 0.05).
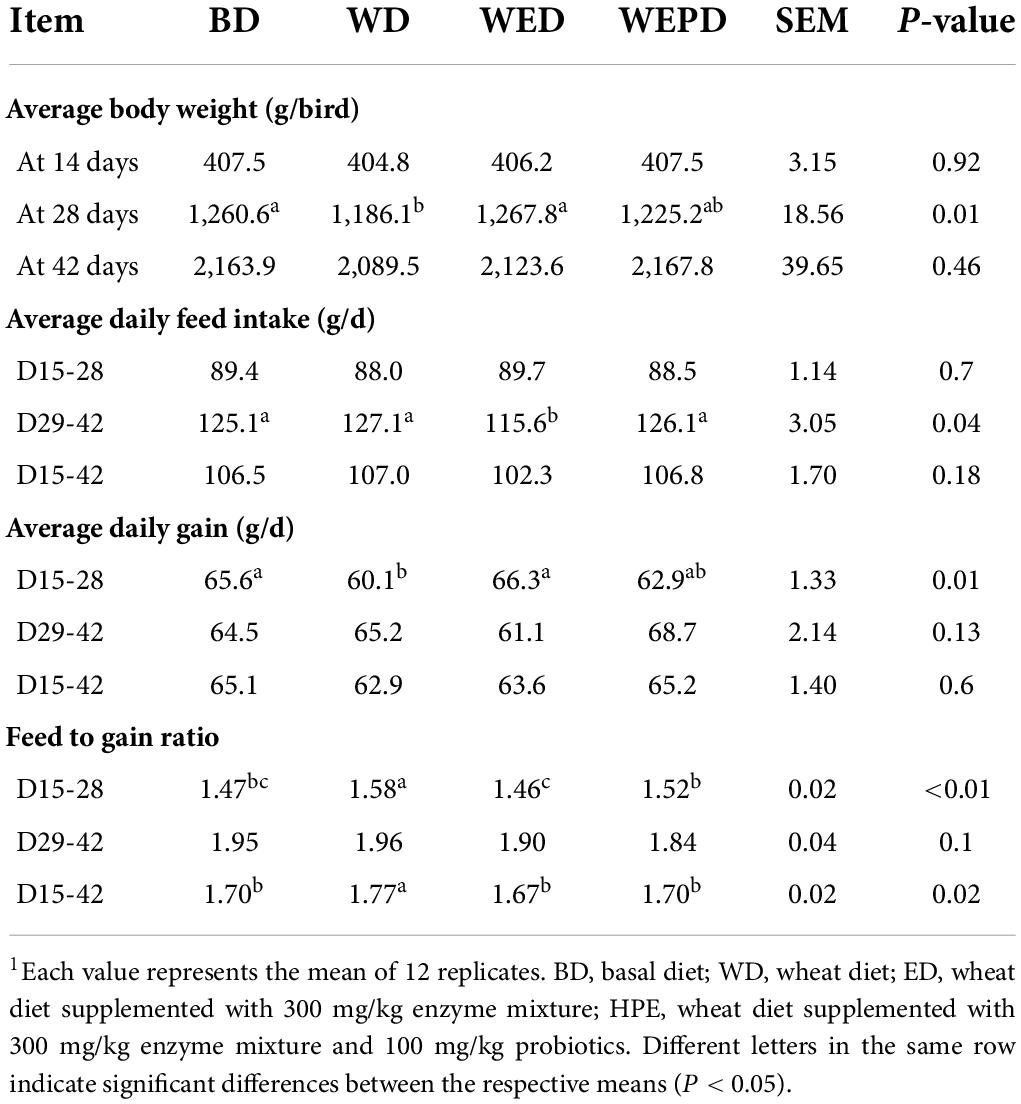
Table 2. Effects of wheat diet supplement with enzyme mixture alone or combined with probiotics on broiler growth performance.1
Inflammatory cytokines and tight junction gene expression
The wheat-soybean meal-based diet group increased mRNA expression of IL-6 and decreased major tight junction protein expression (claudin, occludin, and ZO-1) in the jejunum of broilers compared to the BD group, even though these did not reach the significant level (P > 0.05, Figures 1A–C,F). The WED group improved the expression level of TNF-α (P < 0.05, Figure 1G). In addition, the WEPD group showed higher mRNA expressions of jejunal mucosal claudin-1, ZO-1, and mucin-2 (P < 0.05, Figures 1A–D), and tended to increase the occludin expression (P = 0.07, Figure 1B). However, the WEP group also increased the expression level of IL-6 (P < 0.05, Figure 1F). There was no significant difference in expression of IL-1β and IL-10 among groups (P > 0.05, Figures 1E,H).
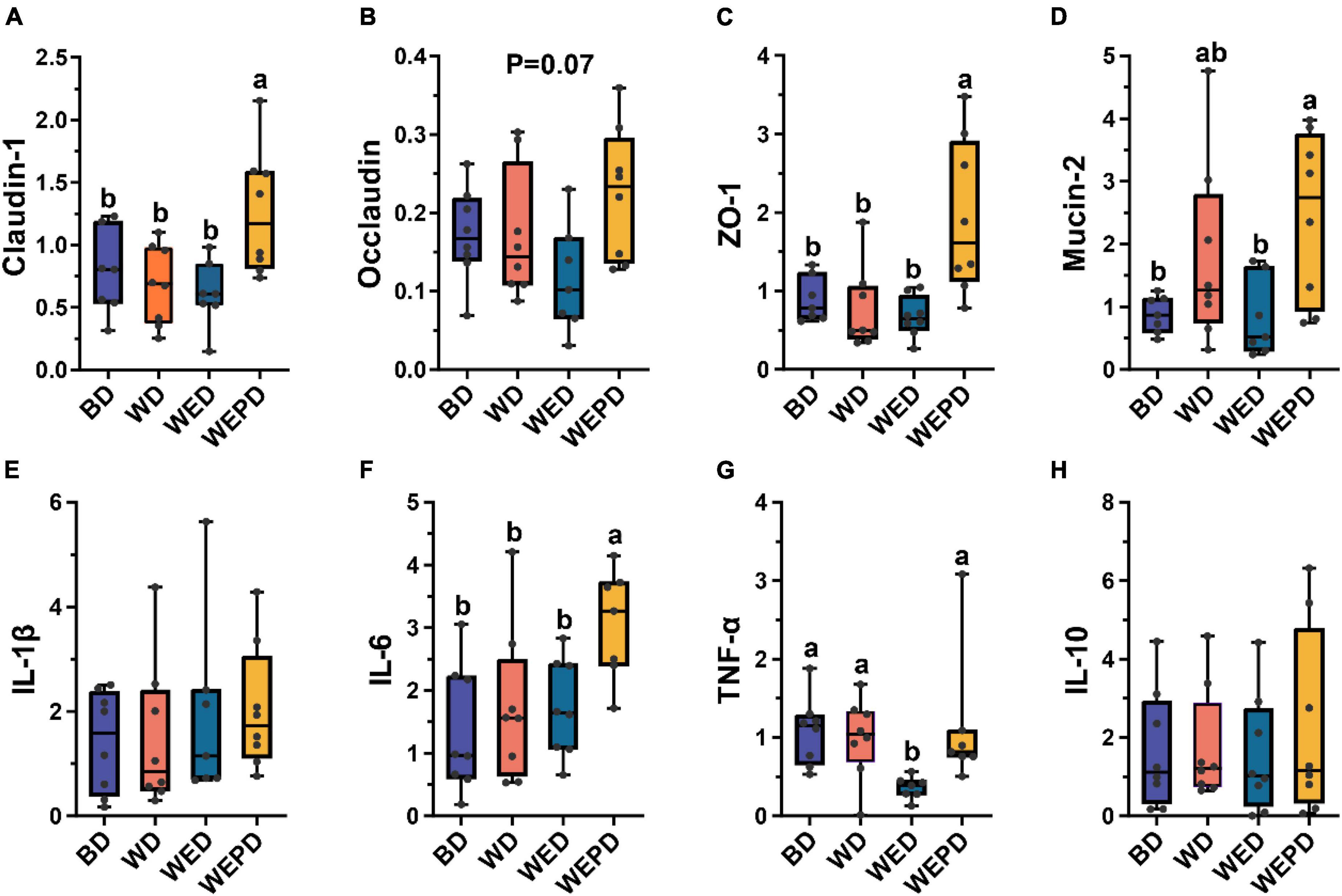
Figure 1. Expression of inflammatory cytokines and tight junction protein in the jejunal mucosa among different treatments. (A–D) mRNA expression of claudin, occludin, ZO-1, and mucin-2. (E–H) mRNA expression of IL-1β, IL-6, TNF-α, and IL-10. Data are expressed as min to max showing all points (n = 7∼8 broilers/group). Different letters (a, b) means significant differences (P < 0.05).
Evaluation of sequencing data
A total of 1,536,879 valid sequences from 32 caecum samples were obtained (with sequences ranging from 34,325 to 61,141 per sample) after denoising and cleaning. The reads of all the samples were normalized based on the minimum number of sample sequences. A total of 34,325 reads in each sample was clustered into 645 OTUs, 163 genera, 72 families, 46 orders, 20 classes, and 11 phyla. Good coverage (>0.998) and rarefaction curves indicated that the sampling of each group provided sufficient sequences to reflect the microbial diversity and bacterial communities in cecum digesta samples (Supplementary Figure 1).
Diversity and structure of intestinal microbiota
In the BD group, Sobs, Shannon, Ace, and Chao indexes were significantly higher than those in the WD and WEPD groups (Figures 2A–D; P < 0.05). The WED group tended to increase the α-diversity compared to the WD group and up to the level of those in the BD group (P > 0.05). The result of PCoA indicated that the microbial composition of broilers in the BD group separated from those in the other three diet groups (Figure 2E; R = 0.1693, P = 0.0020). No distinct clustering was observed among WD, WED, and WEPD groups. The PLS-DA showed distinct clusters among the four groups (Figure 2F).
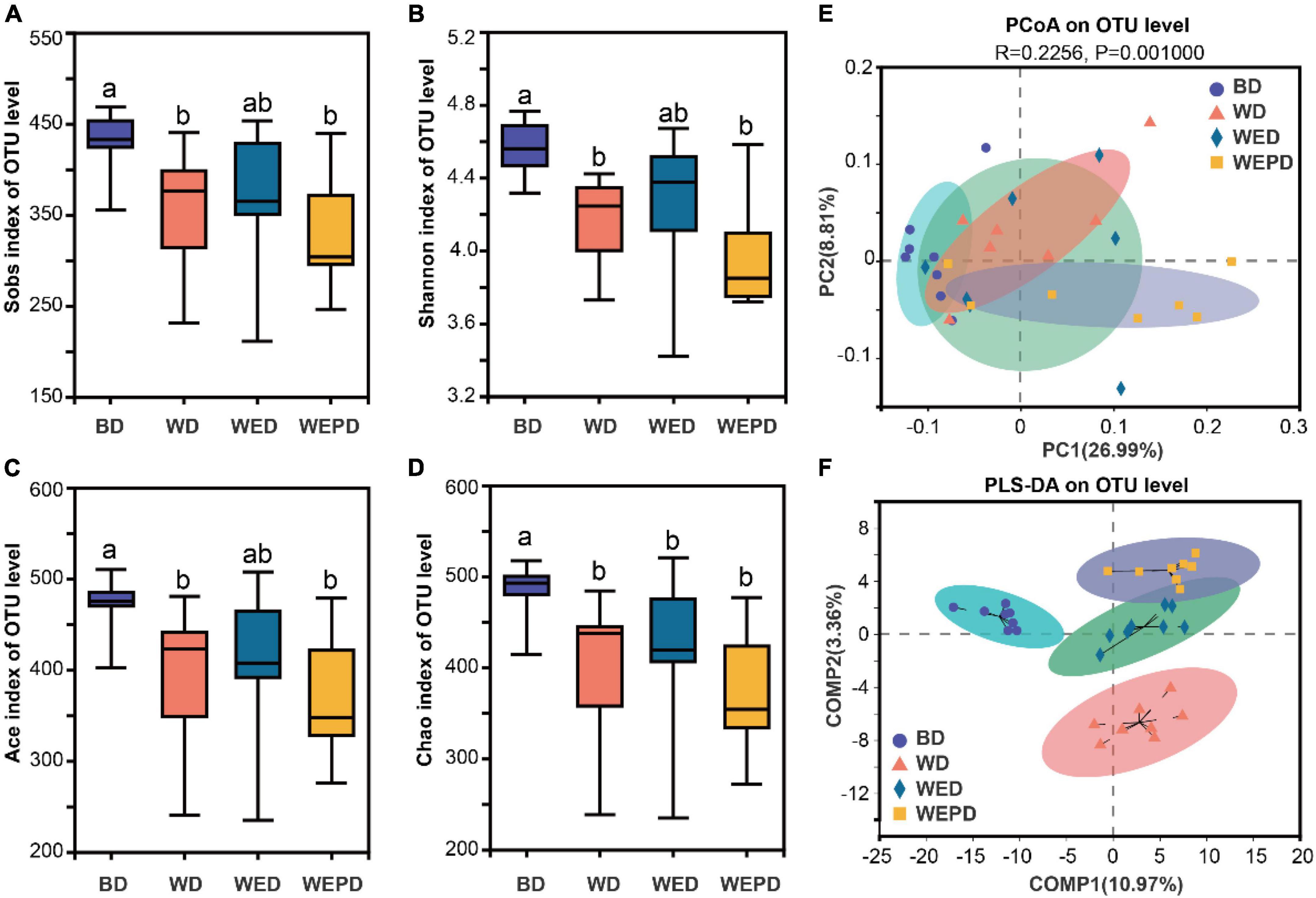
Figure 2. Alpha and beta diversities of the microbial community in the cecum digesta among different treatments. (A) The Sobs, (B) Shannon, (C) ACE, and (D) Chao indexes in the cecum among four groups; PCoA (E) and PLS-DA (F) of microbial community based on unweighted UniFrac and ANOSIM test in cecum digesta. Different letters (a, b) means significant differences (P < 0.05).
Composition and alteration of specific microbiota
At the phylum level (Figure 3A), the most abundant phyla were Firmicutes and Bacteroidetes, accounting for 97.07% on average in the BD group, 95.76% in the WED group, and 97.34% in the WEPD group (n = 8), respectively. The WD group, the WED group, and the WEPD group decreased the Firmicutes abundance and increased the Bacteroidota abundance, but none of them reached a significant level (P > 0.05).
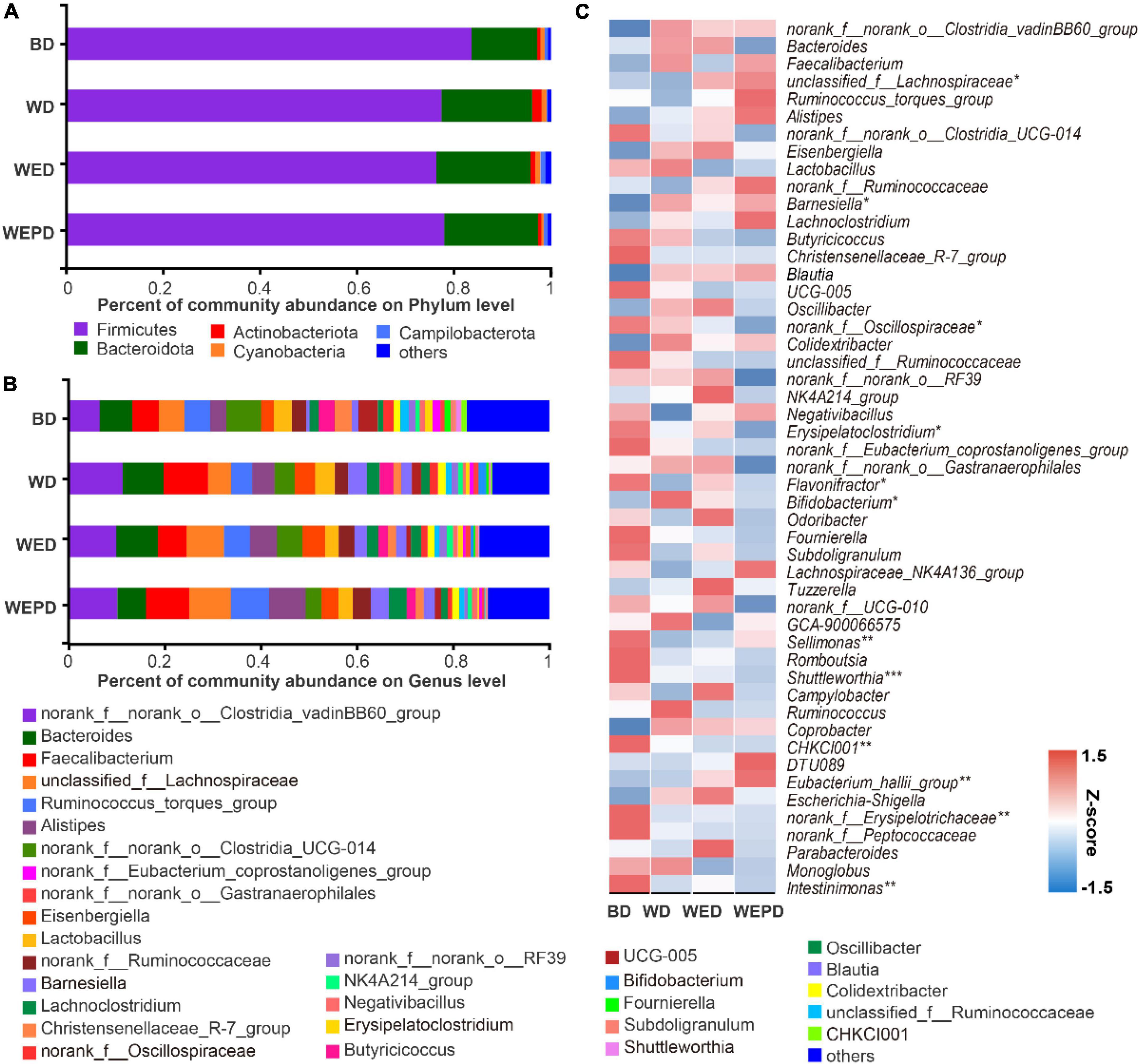
Figure 3. Community composition analysis. Phylum (A) and genus (B) level relative abundance, and trends change (C) of microbiota (phylum level) from the cecal digesta of broilers fed different diets.
At the genus level (Figure 3B), the top 10 dominant genera were norank_f__norank_o__Clostridia_vadinBB60_group, Bacteroides, Faecalibacterium, unclassified_f__Lachnospiraceae, Ruminococcus_torques_group, Alistipes, norank_f__norank_o__Clostridia_UCG-014, Eisenbergiella, Lactobacillus, norank_f__Ruminococcaceae, Barnesiella, and Lachnoclostridium, which consist of 52∼70% of total genera. The trends change in the top 50 genera of the cecal digesta attracted more attention and were presented in a heatmap (Figure 3C). The relative abundance of some genera notably decreased in broilers fed WD group compared to the BD group (e.g., Flavonifractor, Subdoligranulum, Lachnospiraceae_NK4A136_group, Sellimonas, and Intestinimonas; Figure 3C), but restored in the WED group. Other genera had no difference between BD and WD groups, but showed a remarkable variation in WEDP (e.g., unclassified_f__Lachnospiraceae, norank_f__Oscillospiraceae, Erysipelatoclostridium, Eubacterium_hallii_group, and norank_f__Peptococcaceae; Figure 3C).
Compared with the BD group, seven genera (Bifidobacterium, Barnesiella, Catenibacillus, Anaerotruncus, Blautia, norank_f__norank_o__Clostridia_vadinBB60_group, and Oscillibacter) were increased, while four genera (Eubacterium_ventriosum_group, Oscillospira, norank_f__Erysipelotrichaceae, and Peptococcus) were decreased in the WD group (FDR < 0.05, Figure 4A). The relative abundance of Bilophila in the WED group was increased compared with that in the WD group (FDR < 0.05, Figure 4D). The relative abundance of Eubacterium_hallii_group was improved, while the Enterococcus abundance was reduced in the WEPD group compared to the WD group (FDR < 0.05, Figure 4E). Beyond that, more differential genera were observed in WED and WEPD groups compared the BD group. The genera of Eubacterium_hallii_group and unclassified_f__Lachnospiraceae were enriched in WED and WEPD groups, whereas the genera of Shuttleworthia, norank_f__Erysipelotrichaceae, CHKCI001, and Intestinimonas were enriched in the BD group (FDR < 0.05, Figures 4B,C).
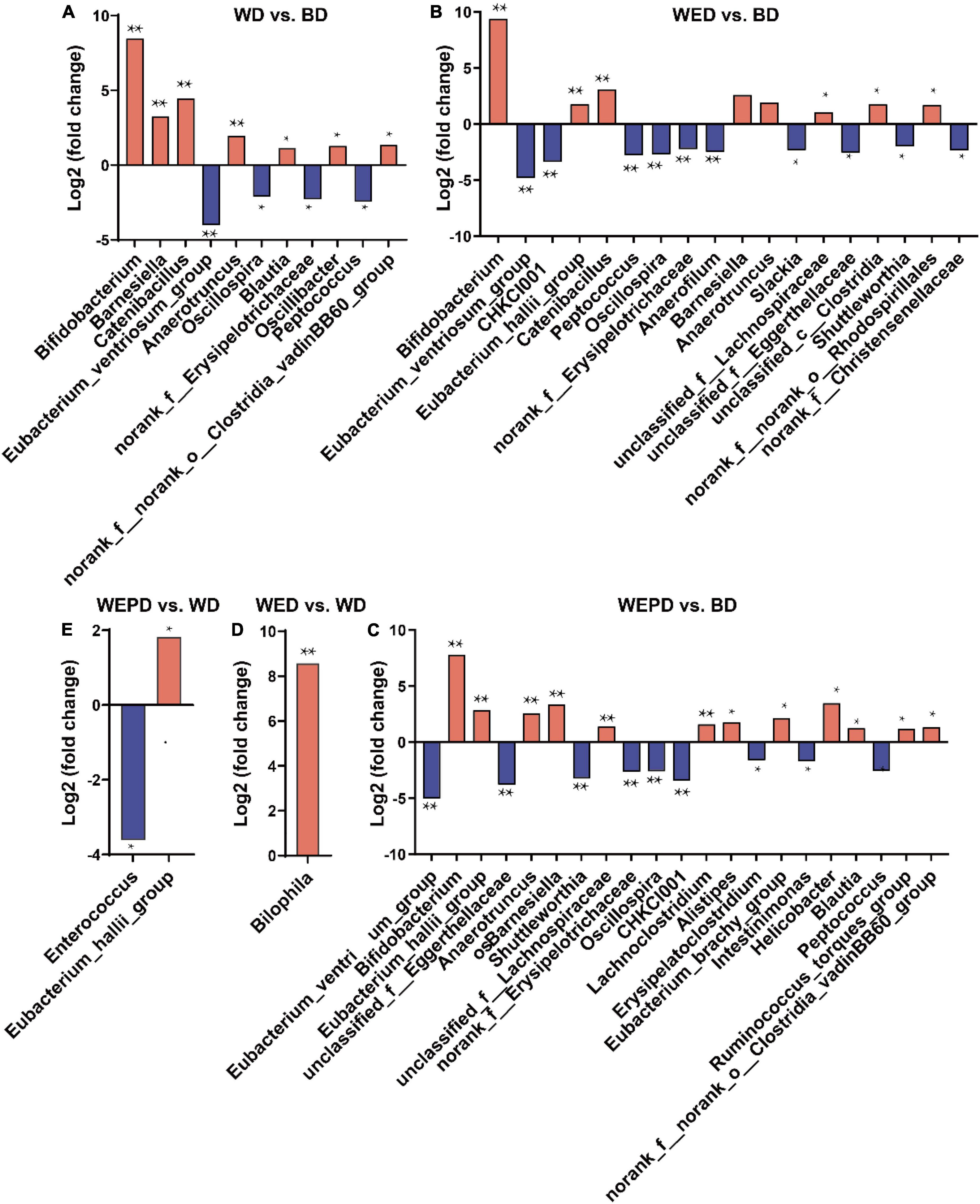
Figure 4. Differential genera analysis of cecum microbiota. (A) Pairwise comparisons between WD and BD groups using the DESeq2 method. Positive fold changes mean the genus is enriched in the WD group, while negative fold changes mean those enriched in the BD group. (B) Pairwise comparisons between WED and BD groups. (C) Pairwise comparisons between WEPD and BD groups. (D) Pairwise comparisons between WED and WD groups. (E) Pairwise comparisons between WEPD and WD groups. *P-value (FDR) < 0.05; **P-value (FDR) < 0.01.
Short-chain fatty acid concentration
Short-chain fatty acids concentrations among different diet groups were shown in Figure 5. There was no difference in concentrations of propionate, isobutyrate, butyrate, isovalerate, and valerate in the WD and BD groups. Broilers receiving the WED diet improved (P < 0.05) propionate production, compared with the WD diet. Besides, WED and WEPD groups improved (P < 0.05) butyrate yield, compared with the BD group. The WEPD group had the lowest (P < 0.05) valerate concentration among all diet groups.
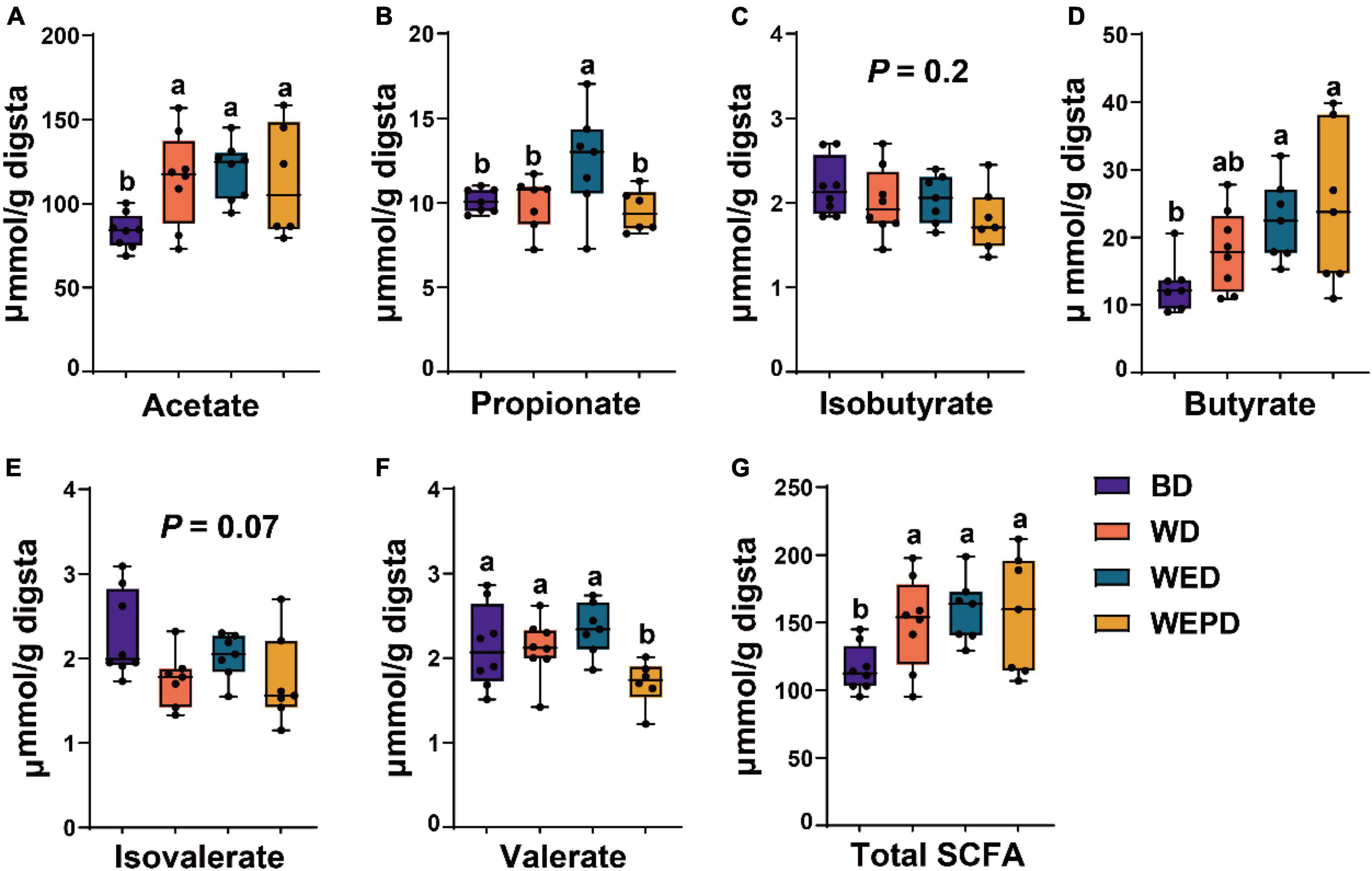
Figure 5. Concentrations of short-chain fatty acids. Difference of acetate (A), propionate (B), isobutyrate (C), butyrate (D), isovalerate (E), valerate (F), and total SCFA (G) among four diet treatments. Different letters (a, b) means significant differences (P < 0.05).
Discussion
Supplementation of exogenous NSP enzyme or probiotics has been reported to improve growth performance and intestinal health of broilers fed wheat-based diets (De Maesschalck et al., 2015; Bhogoju et al., 2021; Wang et al., 2021a,b). The chemical structure and composition of NSP are varied and complex (Ward, 2021). Therefore, combining multiple NSPases that target different NSPs may result in greater benefits than using individual NSP-degrading enzymes (Munyaka et al., 2016). The use of probiotics improved balanced microbiota and good intestinal health, leading to better nutrient digestion and absorption (Alagawany et al., 2018), whereas, the effects of probiotic supplementation might not be consistent (Rehman et al., 2020). Moreover, the increasing use of probiotics may spread live bacteria to the environment (Kareem et al., 2017). Heat-inactivated Lactobacillus has been reported to stimulate the immune system and growth performance (Incharoen et al., 2019). However, there is a lack of information regarding the effects of multi-enzymes and combined with inactivated Lactobacillus on growth performance, intestinal barrier, and intestinal microbiota in broilers fed a wheat diet. The present results underscore the potential of the multi-enzymes mixture and combined with inactivated Lactobacillus to improve growth performance might be related to the microbial composition, metabolites, and intestinal barrier function in broilers.
In the current study, broilers fed a wheat-based diet showed lower ADG and higher F/G than broilers received a corn-based diet, which was consistent with published reports that feeding broilers with a wheat-based diet decreased BW gain from day 1 to 42 (Wang et al., 2021a) and BW at day 28 (McCafferty et al., 2019a), compared with that broilers fed a corn-based diet. Masey-O’Neill et al. (2014) also observed broilers fed a corn-based diet increased BW gain and feed intake and decreased F/G of broilers from day 1 to 28 and day 1 to 40 when compared with those fed a wheat-based diet. This might be related to the high concentration of soluble NSP in wheat, leading to the increase of intestinal digesta viscosity, affecting gut transit time, and thus reducing absorption and utilization of nutrients in broilers (Knudsen, 2014; Munyaka et al., 2016). However, McCafferty et al. (2019b) reported no effects of supplementation of NSPases on growth performance. These inconsistent observations could be ascribed to the specificity of NSPases for specific NSP or feed ingredients (Smeets et al., 2014) and the various quality of wheat (Choct et al., 1995). In the current study, supplementation of multi-enzymes alone or combined with inactivated Lactobacillus improved the growth performance of broilers, indicated by high ADG from day 14 to 28, high BW at day 28, and low F/G for the whole trial period. Similar to our results, it has been reported that broilers fed a wheat-based diet supplemented with enzymes or probiotics could improve growth performance (Zhao et al., 2016; Wang et al., 2021b). Khonyoung and Yamauchi (2019) also found that dietary supplementation with inactivated Lactobacillus increased body weight gain and FCR of broilers, which might be related to the reduction of digesta viscosity and protection of intestinal health, including intestinal immunity and intestinal barrier function improvement (Gonzalez-Ortiz et al., 2017; Bhogoju et al., 2021).
A healthy gut is extremely important, as the gut is related to the absorption and metabolism of nutrients and barrier function (Taciak et al., 2015; Xiong et al., 2019). The intestinal epithelial barrier function comprises physical, ecological, and immunological barriers, which positively affect nutrient digestion and utilization. In this study, the WD group tended to increase mRNA expression of IL-6 and decrease expression of occludin in the jejunum of broilers compared to the BD group. The IL-6 is an important proinflammatory cytokine that is considered to regulate pathological responses (Umare et al., 2014). The occludin is one of the tight junction proteins that is associated with modulating intestinal barrier function (Wang et al., 2020). The results suggested wheat diet impacted intestinal barrier function, which may lead to the decrease of ADG and FCR in broilers. Wheat diet supplemented enzymes inhibited the expression level of tumor necrosis factor (TNF)-α. In addition, supplementation of wheat diet with enzymes and probiotics improved mRNA expressions of jejunal mucosal claudin-1, ZO-1, and tended to improve mucin-2 and occludin expression. Similar to IL-6, TNF-α is another important proinflammatory cytokine. Claudin-1, ZO-1, and mucin-2 are also essential components of barrier function (Tornavaca et al., 2015). In previous studies, Wang et al. (2021b) found that supplementing xylanase in the wheat diet reduced IL-1β and TNF-α concentrations of ileal tissues in broilers, but no significant differences were observed in occluding and ZO-1 mRNA expression. However, some researchers focused on the exogenous enzyme complex reported a significant mRNA expression increase in claudin, occludin, and ZO-1, and also a decrease of IL-1β and TNF-α levels in the jejunum of nursery pigs and broiler, respectively (Tiwari et al., 2018; Wang et al., 2021c). Our results indicate that NSP enzymes or enzymes combined with probiotic supplementation could enhance intestinal barrier function. The healthy intestinal barrier function is beneficial to the effective digestion and absorption of nutrients in broilers, thus improving the growth performance of broilers. A large number of microorganisms inhabit the gastrointestinal tract, which is generally considered a “microbial organ.” The stabilization of the “microbial organ” is critical to gut health, barrier function, and nutrient uptake (Fraumene et al., 2018), contributing to better growth performance. Therefore, further analysis of the community structure of microbiota was performed. We found that the WD group had a lower alpha-diversity and separated from the BD group. It was consistent with a previous study that the corn-based diet increased the alpha-diversity of the cecal bacterial community and separated from the wheat-based diet (Wang et al., 2021a). A previous study reported that an increased α-diversity may promote the stability of microbiota, which is beneficial to health (Díaz Carrasco et al., 2018). The α-diversity was not affected by either NSP enzyme supplementing or combined with inactivated Lactobacillus in a wheat-based diet, which was also revealed by xylanase supplementation of wheat-based and corn-based diets in broilers (Wang et al., 2021a). Zhang et al. (2021) also found that Bacillus subtilis addition in the corn-based diet has no effects on the alpha diversity of microbiota in the cecum of broilers. Moreover, there was no distinct cluster among the WD group, the WED group, and the WEPD group, which was also similar to the results that no distinct clustering between the wheat diet group and the wheat diet added with the xylanase group was observed (Wang et al., 2021a). Those results suggested that the microbial community structure of broilers was mainly affected by types of diet rather than enzymes or probiotic supplementation.
The variations in the relative abundance of microbiota were further analyzed to illustrate the modulation of microbiota by supplemental enzymes and probiotics. We found that enzymes and probiotics modulated the abundance of specific bacteria without changing the overall microbial structure. In the current study, the abundance of Bifidobacterium, classified as Actinobacteria, was higher in the WD group compared with those in the BD group regardless of whether enzymes and probiotics were added. Consistent with the present study, Wang et al. (2021a) also reported that broilers fed a wheat-based diet has a high abundance of Bifidobacterium in the cecum. A previous study indicates that Bifidobacterium could produce the SCFA acetate (Engevik et al., 2021) by synthesizing exopolysaccharides as substrate (Salazar et al., 2008). This also explains the higher concentrations of acetate and total SCFA in the cecum of broilers fed wheat diet than those fed the BD diet. However, Barnesiella, presumed as “harmful microbes” (Han et al., 2021), was higher in the WD group compared to the BD group. The abundance of Barnesiella in the multi-enzyme supplementation group (WED) was reduced to the same levels as that in the BD group. Moreover, the genera Blautia, belonging to Firmicutes, was negatively correlated with the BW of the host (Ozato et al., 2019). Our current results demonstrated that broilers fed the WD diet had higher Blautia abundance in the cecum, which may be ascribed to the poor growth performance of broilers. Supplementation of NSP enzymes has improved growth performance and may be related to the decrement of Blautia abundance. Blautia is also well-known as the butyric-acid-producing and acetic-acid-producing bacteria in the gut microbiota (Kim et al., 2021). In addition, genera Anaerotruncus and Oscillibacter are also butyrate-producing bacteria, which have been reported to have a positive correlation with butyric acid production (Wang et al., 2018). Our results showed genera Blautia, Anaerotruncus, and Oscillibacter were all enriched in the WD group, which may all contribute to the higher acetate, butyrate, and total SCFA concentration in the cecum of broilers. On the other hand, broilers fed the WB diet decreased the abundance of Oscillospira, which of the genera have been reported to have a potential anti-inflammatory effect (Santoru et al., 2017). The anti-inflammatory effect of the WB diet has also been further confirmed by our experimental results of IL-6 mRNA level enhancing and jejunum occludin attenuating, which indicate an inflammatory response and concomitant intestinal barrier dysfunction. A previous study has reported that genera Peptococcus does not have carbohydrate fermentation functions (Liang et al., 2019). Wheat-containing diets have higher NSP content than corn-containing diets. Our results showed that genera Peptococcus was lower in the WD group than that in the BD group, which may reduce the degradation of NSP, thus decreasing the nutritional value and increasing the detrimental effects of NSP. Those negative effects may subsequently result in the poor growth performance of broilers fed WD diet. The Bilophila abundance is higher in the WED group than in the BD group, which may contribute to the BW of broilers as Bilophila was positively correlated with obesity in humans (Turnbaugh et al., 2009). However, other studies have reported that Bilophila is a pathobiont, which is related to the bile acid dysregulation in mice (Banerjee et al., 2016). Previous studies have reported that genera Eubacterium_hallii_group was butyrate producers (Bunesova et al., 2018), whereas, Enterococcus was opportunistic pathogenic bacteria (Huang et al., 2018). In the current study, supplementation of enzyme combined with probiotics improved Eubacterium_hallii_group abundance and reduced Enterococcus abundance, indicating an additional protective effect of inactivated Lactobacillus on broiler intestinal health. Diet supplemented with NSP enzymes alone or combined with inactivated Lactobacillus improved the intestinal bacterial communities, thus improving gut health, which may further contribute to the improvement of growth performance of broilers. In SCFA production, broilers fed wheat diet had higher concentrations of acetate and total SCFA compared to those provided the BD diet. A wheat diet supplemented with NSP enzyme or combined with probiotics improved propionate or butyrate production compared to the BD diet. McCafferty et al. (2019b) reported that broilers fed a wheat diet produced more butyrate compared to a corn diet, but no significant difference in acetate and total SCFA was observed. This is probably related to the higher abundance of Eubacterium_hallii_group and unclassified_f__Lachnospiraceae, the two SCFAs-producing genera (Bunesova et al., 2018; Hu et al., 2019) in WED and WEPD groups in our study. Increment of SCFA production, which can be used as an energy source for the improved growth performance of broilers.
Conclusion
Wheat-based diet has negative effects on growth performance, indicated by lower ADG, BW, and higher F/G compared with a corn-based diet, whereas supplementing multi-enzymes alone or combined with inactivated Lactobacillus improved the growth performance of broilers. The added multi-enzymes inhibited the expression of TNF-α and combined with inactivated Lactobacillus supplementation further improved the mRNA expression of claudin-1, ZO-1, and mucin-2, indicating that the beneficial effect of inactivated Lactobacillus focus more on protecting gut health. Moreover, dietary enzyme supplementation alone or with probiotics could improve SCFA-producing bacteria (Eubacterium_hallii_group and unclassified_f__Lachnospiraceae) and further improve propionate and butyrate concentration. Collectively, our results suggested that the better growth performance might be related to an improvement in intestinal barrier function.
Data availability statement
The data presented in this study are deposited in the NCBI Sequence Read Archive database, accession number: PRJNA825386.
Ethics statement
The animal study was reviewed and approved by The Animal Ethics Committee of the Institute of Animal Sciences, Chinese Academy of Agricultural Sciences reviewed and approved the experimental protocol (Ethics Approval Code: IAS2021-233).
Author contributions
QG, YW, and TM conceived and designed the experiments and wrote—review and editing. JL, GB, QG, and RZ performed the experiments. QG and YW analyzed the data. HZ, HP, and LL contributed to the reagents and materials. QG wrote—original draft. All authors read and approved the final manuscript.
Funding
This work was supported by the China Agriculture Research System of MOF and MARA (CARS-41) and the State Key Laboratory of Animal Nutrition (2004DA125184G2102).
Conflict of interest
The authors declare that the research was conducted in the absence of any commercial or financial relationships that could be construed as a potential conflict of interest.
Publisher’s note
All claims expressed in this article are solely those of the authors and do not necessarily represent those of their affiliated organizations, or those of the publisher, the editors and the reviewers. Any product that may be evaluated in this article, or claim that may be made by its manufacturer, is not guaranteed or endorsed by the publisher.
Supplementary material
The Supplementary Material for this article can be found online at: https://www.frontiersin.org/articles/10.3389/fmicb.2022.927932/full#supplementary-material
Footnotes
References
Adeola, O., and Cowieson, A. J. (2011). Board-invited review: opportunities and challenges in using exogenous enzymes to improve nonruminant animal production. J. Anim. Sci. 89, 3189–3218. doi: 10.2527/jas.2010-3715
Alagawany, M., Abd El-Hack, M. E., Farag, M. R., Sachan, S., Karthik, K., and Dhama, K. (2018). The use of probiotics as eco-friendly alternatives for antibiotics in poultry nutrition. Environ. Sci. Pollut. Res. Int. 25, 10611–10618. doi: 10.1007/s11356-018-1687-x
AOAC (2012). Official methods of analysis, 19th ed. Arlington, VA: Association of Official Agricultural Chemists.
Ball, M. E., Owens, B., and McCracken, K. J. (2013). The effect of variety and growing conditions on the chemical composition and nutritive value of wheat for broilers. Asian-Australas. J. Anim. Sci. 26, 378–385. doi: 10.5713/ajas.2012.12180
Banerjee, S., Sindberg, G., Wang, F., Meng, J., Sharma, U., Zhang, L., et al. (2016). Opioid-induced gut microbial disruption and bile dysregulation leads to gut barrier compromise and sustained systemic inflammation. Mucosal. Immunol. 9, 1418–1428. doi: 10.1038/mi.2016.9
Bhogoju, S., Khwatenge, C. N., Taylor-Bowden, T., Akerele, G., Kimathi, B. M., Donkor, J., et al. (2021). Effects of Lactobacillus reuteri and Streptomyces coelicolor on growth performance of broiler chickens. Microorganisms 9, 1314–1325. doi: 10.3390/microorganisms9061341
Bunesova, V., Lacroix, C., and Schwab, C. (2018). Mucin cross-feeding of infant Bifidobacteria and Eubacterium hallii. Microb. Ecol. 75, 228–238. doi: 10.1007/s00248-017-1037-4
Choct, M., Hughes, R. J., Trimble, R. P., Angkanaporn, K., and Annison, G. (1995). Non-starch polysaccharide-degrading enzymes increase the performance of broiler chickens fed wheat of low apparent metabolizable energy. J. Nutr. 125, 485–492. doi: 10.1093/jn/125.3.485
Choct, M., Kocher, A., Waters, D. L. E., Petterson, D., and Ross, G. (2004). A comparison of three xylanases on the nutritive value of two wheats for broiler chickens. Br. J. Nutr. 92, 53–61. doi: 10.1079/BJN20041166
Crisol-Martínez, E., Stanley, D., Geier, M. S., Hughes, R. J., and Moore, R. J. (2017). Sorghum and wheat differentially affect caecal microbiota and associated performance characteristics of meat chickens. PeerJ. 5:e3071. doi: 10.7717/peerj.3071
De Maesschalck, C., Eeckhaut, V., Maertens, L., De Lange, L., Marchal, L., Nezer, C., et al. (2015). Effects of xylo-oligosaccharides on broiler chicken performance and microbiota. Appl. Environ. Microbiol. 81, 5880–5888. doi: 10.1128/AEM.01616-15
Díaz Carrasco, J. M., Redondo, E. A., Pin Viso, N. D., Redondo, L. M., Farber, M. D., and Fernández Miyakawa, M. E. (2018). Tannins and bacitracin differentially modulate gut microbiota of broiler chickens. Biomed. Res. Int. 2018:1879168. doi: 10.1155/2018/1879168
Engevik, M. A., Luck, B., Visuthranukul, C., Ihekweazu, F. D., Engevik, A. C., Shi, Z., et al. (2021). Human-derived Bifidobacterium dentium modulates the mammalian serotonergic system and gut-brain axis. Cell Mol. Gastroenterol. Hepatol. 11, 221–248. doi: 10.1016/j.jcmgh.2020.08.002
Fraumene, C., Manghina, V., Cadoni, E., Marongiu, F., Abbondio, M., Serra, M., et al. (2018). Caloric restriction promotes rapid expansion and long-lasting increase of Lactobacillus in the rat fecal microbiota. Gut Microbes 9, 104–114. doi: 10.1080/19490976.2017.1371894
Gonzalez-Ortiz, G., Sola-Oriol, D., Martinez-Mora, M., Perez, J. F., and Bedford, M. R. (2017). Response of broiler chickens fed wheat-based diets to xylanase supplementation. Poult. Sci. 96, 2776–2785. doi: 10.3382/ps/pex092
Han, H., Zhong, R., Zhou, Y., Xiong, B., Chen, L., Jiang, Y., et al. (2021). Hydroxytyrosol benefits boar semen quality via improving gut microbiota and blood metabolome. Front. Nutr. 8:815922. doi: 10.3389/fnut.2021.815922
Hu, S., Xu, Y., Gao, X., Li, S., Jiang, W., Liu, Y., et al. (2019). Long-chain bases from sea cucumber alleviate obesity by modulating gut microbiota. Mar. Drugs. 17:455. doi: 10.3390/md17080455
Huang, P., Zhang, Y., Xiao, K., Jiang, F., Wang, H., Tang, D., et al. (2018). The chicken gut metagenome and the modulatory effects of plant-derived benzylisoquinoline alkaloids. Microbiome 6:211. doi: 10.1186/s40168-018-0590-5
Incharoen, T., Charoensook, R., Onoda, S., Tatrakoon, W., Numthuam, S., and Pechkong, T. (2019). The effects of heat-killed Lactobacillus plantarum L-137 supplementation on growth performance, intestinal morphology, and immune-related gene expression in broiler chickens. 257:114272. doi: 10.1016/j.anifeedsci.2019.114272
Kareem, K. Y., Loh, T. C., Foo, H. L., Asmara, S. A., and Akit, H. (2017). Influence of postbiotic RG14 and inulin combination on cecal microbiota, organic acid concentration, and cytokine expression in broiler chickens. Poult. Sci. 96, 966–975. doi: 10.3382/ps/pew362
Khonyoung, D., and Yamauchi, K.-E. (2019). Improved growth performance due to hypertrophied intestinal absorptive epithelial cells by heat-killed Lactobacillus sakei HS-1 in broiler chickens1. J. Anim. Sci. 97, 2066–2075. doi: 10.1093/jas/skz075
Kiarie, E., Romero, L. F., and Ravindran, V. (2014). Growth performance, nutrient utilization, and digesta characteristics in broiler chickens fed corn or wheat diets without or with supplemental xylanase. Poult. Sci. 93, 1186–1196. doi: 10.3382/ps.2013-03715
Kim, H. J., Kim, D., Kim, K. W., Lee, S. H., and Jang, A. (2021). Comparative analysis of the gut microbiota of mice fed a diet supplemented with raw and cooked beef loin powder. Sci. Rep. 11, 11489–11489. doi: 10.1038/s41598-021-90461-7
Knudsen, K. E. B. (2014). Fiber and nonstarch polysaccharide content and variation in common crops used in broiler diets. Poult. Sci. 93, 2380–2393. doi: 10.3382/ps.2014-03902
Liang, Y. N., Yu, J. G., Zhang, D. B., Zhang, Z., Ren, L. L., Li, L. H., et al. (2019). Indigo naturalis ameliorates dextran sulfate sodium-induced colitis in mice by modulating the intestinal microbiota community. Molecules 24:4086. doi: 10.3390/molecules24224086
Livak, K. J., and Schmittgen, T. D. (2001). Analysis of relative gene expression data using real-time quantitative PCR and the 2(-Delta Delta C(T)) Method. Methods 25, 402–408. doi: 10.1006/meth.2001.1262
Masey-O’Neill, H. V., Singh, M., and Cowieson, A. J. (2014). Effects of exogenous xylanase on performance, nutrient digestibility, volatile fatty acid production and digestive tract thermal profiles of broilers fed on wheat- or maize-based diet. Br. Poult. Sci. 55, 351–359. doi: 10.1080/00071668.2014.898836
McCafferty, K. W., Bedford, M. R., Kerr, B. J., and Dozier, W. A. (2019b). Effects of cereal grain source and supplemental xylanase concentrations on broiler growth performance and cecal volatile fatty acid concentrations from 1 to 40 d of age. Poult. Sci. 98, 2866–2879. doi: 10.3382/ps/pez032
McCafferty, K. W., Bedford, M. R., Kerr, B. J., and Dozier, W. A. (2019a). Effects of age and supplemental xylanase in corn- and wheat-based diets on cecal volatile fatty acid concentrations of broilers1. Poult. Sci. 98, 4787–4800. doi: 10.3382/ps/pez194
Munyaka, P. M., Nandha, N. K., Kiarie, E., Nyachoti, C. M., and Khafipour, E. (2016). Impact of combined β-glucanase and xylanase enzymes on growth performance, nutrients utilization and gut microbiota in broiler chickens fed corn or wheat-based diets. Poult. Sci. 95, 528–540. doi: 10.3382/ps/pev333
Musigwa, S., Cozannet, P., Morgan, N., Swick, R. A., and Wu, S. B. (2021). Multi-carbohydrase effects on energy utilization depend on soluble non-starch polysaccharides-to-total non-starch polysaccharides in broiler diets. Poult. Sci. 100, 788–796. doi: 10.1016/j.psj.2020.10.038
National Research Council (1994). Nutrient Requirements of Poultry. 9th Edn. Washington, DC: The National Academies Press.
Nguyen, H. T., Bedford, M. R., Wu, S. B., and Morgan, N. K. (2021). Soluble non-starch polysaccharide modulates broiler gastrointestinal tract environment. Poult. Sci. 100:101183. doi: 10.1016/j.psj.2021.101183
Ozato, N., Saito, S., Yamaguchi, T., Katashima, M., Tokuda, I., Sawada, K., et al. (2019). Blautia genus associated with visceral fat accumulation in adults 20-76 years of age. NPJ Biofilms Microb. 5:28. doi: 10.1038/s41522-019-0101-x
Raza, A., Bashir, S., and Tabassum, R. (2019). An update on carbohydrases: growth performance and intestinal health of poultry. Heliyon 5:e01437. doi: 10.1016/j.heliyon.2019.e01437
Rebolé, A., Ortiz, L. T., Rodríguez, M. L., Alzueta, C., Treviño, J., and Velasco, S. (2010). Effects of inulin and enzyme complex, individually or in combination, on growth performance, intestinal microflora, cecal fermentation characteristics, and jejunal histomorphology in broiler chickens fed a wheat- and barley-based diet. Poult. Sci. 89, 276–286. doi: 10.3382/ps.2009-00336
Rehman, A., Arif, M., Sajjad, N., Al-Ghadi, M. Q., Alagawany, M., Abd El-Hack, M. E., et al. (2020). Dietary effect of probiotics and prebiotics on broiler performance, carcass, and immunity. Poult. Sci. 99, 6946–6953. doi: 10.1016/j.psj.2020.09.043
Romero, L. F., Sands, J. S., Indrakumar, S. E., Plumstead, P. W., Dalsgaard, S., and Ravindran, V. (2014). Contribution of protein, starch, and fat to the apparent ileal digestible energy of corn- and wheat-based broiler diets in response to exogenous xylanase and amylase without or with protease. Poult. Sci. 93, 2501–2513. doi: 10.3382/ps.2013-03789
Salazar, N., Gueimonde, M., Hernández-Barranco, A. M., Ruas-Madiedo, P., and de los Reyes-Gavilán, C. G. (2008). Exopolysaccharides produced by intestinal Bifidobacterium strains act as fermentable substrates for human intestinal bacteria. Appl. Environ. Microbiol. 74, 4737–4745. doi: 10.1128/AEM.00325-08
Santoru, M. L., Piras, C., Murgia, A., Palmas, V., Camboni, T., Liggi, S., et al. (2017). Cross sectional evaluation of the gut-microbiome metabolome axis in an Italian cohort of IBD patients. Sci. Rep. 7, 9523–9523. doi: 10.1038/s41598-017-10034-5
Smeets, N., Nuyens, F., Van Campenhout, L., Delezie, E., and Niewold, T. A. (2018). Interactions between the concentration of non-starch polysaccharides in wheat and the addition of an enzyme mixture in a broiler digestibility and performance trial. Poult. Sci. 97, 2064–2070. doi: 10.3382/ps/pey038
Smeets, N., Nuyens, F., Van Campenhout, L., and Niewold, T. (2014). Variability in the in vitro degradation of non-starch polysaccharides from wheat by feed enzymes. Anim. Feed Sci. Technol. 187, 110–114. doi: 10.1016/j.anifeedsci.2013.10.020
Stanley, D., Denman, S. E., Hughes, R. J., Geier, M. S., Crowley, T. M., Chen, H., et al. (2012). Intestinal microbiota associated with differential feed conversion efficiency in chickens. Appl. Microbiol. Biotechnol. 96, 1361–1369. doi: 10.1007/s00253-011-3847-5
Taciak, M., Barszcz, M., Tuśnio, A., and Pastuszewska, B. (2015). Interactive effects of indigestible carbohydrates, protein type, and protein level on biomarkers of large intestine health in rats. PLoS One 10:e0142176. doi: 10.1371/journal.pone.0142176
Tang, S., Zhang, S., Zhong, R., Su, D., Xia, B., Liu, L., et al. (2021a). Time-course alterations of gut microbiota and short-chain fatty acids after short-term lincomycin exposure in young swine. Appl. Microbiol. Biotechnol. 105, 8441–8456. doi: 10.1007/s00253-021-11627-x
Tang, S., Zhong, R., Yin, C., Su, D., Xie, J., Chen, L., et al. (2021b). Exposure to high aerial ammonia causes hindgut dysbiotic microbiota and alterations of microbiota-derived metabolites in growing pigs. Front. Nutr. 8:689818. doi: 10.3389/fnut.2021.689818
Tiwari, U. P., Chen, H., Kim, S. W., and Jha, R. (2018). Supplemental effect of xylanase and mannanase on nutrient digestibility and gut health of nursery pigs studied using both in vivo and in vitro models. Anim. Feed Sci. Technol. 245, 77–90. doi: 10.1016/j.anifeedsci.2018.07.002
Tornavaca, O., Chia, M., Dufton, N., Almagro, L. O., Conway, D. E., Randi, A. M., et al. (2015). ZO-1 controls endothelial adherens junctions, cell-cell tension, angiogenesis, and barrier formation. J. Cell Biol. 208, 821–838. doi: 10.1083/jcb.201404140
Torok, V. A., Hughes, R. J., Mikkelsen, L. L., Perez-Maldonado, R., Balding, K., MacAlpine, R., et al. (2011). Identification and characterization of potential performance-related gut microbiotas in broiler chickens across various feeding trials. Appl. Environ. Microbiol. 77, 5868–5878. doi: 10.1128/AEM.00165-11
Turnbaugh, P. J., Ridaura, V. K., Faith, J. J., Rey, F. E., Knight, R., and Gordon, J. I. (2009). The effect of diet on the human gut microbiome: a metagenomic analysis in humanized gnotobiotic mice. Sci. Transl. Med. 1:6ra14. doi: 10.1126/scitranslmed.3000322
Umare, V., Pradhan, V., Nadkar, M., Rajadhyaksha, A., Patwardhan, M., Ghosh, K. K., et al. (2014). Effect of proinflammatory cytokines (IL-6, TNF-α, and IL-1β) on clinical manifestations in Indian SLE patients. Mediators Inflamm. 2014:385297. doi: 10.1155/2014/385297
Wang, J., Ji, H., Wang, S., Liu, H., Zhang, W., Zhang, D., et al. (2018). Probiotic promotes intestinal barrier function by strengthening the epithelium and modulating gut microbiota. Front. Microbiol. 9:1953. doi: 10.3389/fmicb.2018.01953
Wang, Q., Garrity, G. M., Tiedje, J. M., and Cole, J. R. (2007). Naive Bayesian classifier for rapid assignment of rRNA sequences into the new bacterial taxonomy. Appl. Environ. Microbiol. 73, 5261–5267. doi: 10.1128/AEM.00062-07
Wang, T., Yao, W., Li, J., Shao, Y., He, Q., Xia, J., et al. (2020). Dietary garcinol supplementation improves diarrhea and intestinal barrier function associated with its modulation of gut microbiota in weaned piglets. J. Anim. Sci. Biotechnol. 11, 12–24. doi: 10.1186/s40104-020-0426-6
Wang, J., Liu, S., Ma, J., and Piao, X. (2021b). Changes in growth performance and ileal microbiota composition by xylanase supplementation in broilers fed wheat-based diets. Front. Microbiol. 12:706396. doi: 10.3389/fmicb.2021.706396
Wang, J., Cao, H., Bao, C., Liu, Y., Dong, B., Wang, C., et al. (2021a). Effects of xylanase in corn- or wheat-based diets on cecal microbiota of broilers. Front. Microbiol. 12:757066. doi: 10.3389/fmicb.2021.757066
Wang, Y., Heng, C., Zhou, X., Cao, G., Jiang, L., Wang, J., et al. (2021c). Supplemental DSM 29784 and enzymes, alone or in combination, as alternatives for antibiotics to improve growth performance, digestive enzyme activity, anti-oxidative status, immune response and the intestinal barrier of broiler chickens. Br. J. Nutr. 125, 494–507. doi: 10.1017/S0007114520002755
Ward, N. E. (2021). Debranching enzymes in corn-soybean meal-based poultry feeds: a review. Poult. Sci. 100, 765–775. doi: 10.1016/j.psj.2020.10.074
Xiong, X., Tan, B., Song, M., Ji, P., Kim, K., Yin, Y., et al. (2019). Nutritional intervention for the intestinal development and health of weaned pigs. Front. Vet. Sci. 6:46–59. doi: 10.3389/fvets.2019.00046
Zhang, L., Gao, L., Chen, L., Zhong, R., and Zhang, H. (2017). Optimization of non-starch polysaccharide enzymes of corn-soybean-diets and wheat-soybean diets for growing pig using in vitro method. Chin. J. Anim. Vet. Sci. 48, 1468–1480.
Zhang, S., Zhong, G., Shao, D., Wang, Q., Hu, Y., Wu, T., et al. (2021). Dietary supplementation with Bacillus subtilis promotes growth performance of broilers by altering the dominant microbial community. Poult. Sci. 100, 100935–100947. doi: 10.1016/j.psj.2020.12.032
Zhao, W., Sun, Z., Wang, X., Fu, L., Liang, H., and Zhen, Y. (2016). Effect of inactivated Lactobacillus cultures on growth performance, cecal microflora and serum biochemical indexes of broilers. Chin. J. Vet. Sci. 36, 1440–1445. doi: 10.16303/j.cnki.1005-4545.2016.08.33
Keywords: multi-enzymes, inactivated Lactobacillus, growth performance, microbiota, broilers
Citation: Gao Q, Wang Y, Li J, Bai G, Liu L, Zhong R, Ma T, Pan H and Zhang H (2022) Supplementation of multi-enzymes alone or combined with inactivated Lactobacillus benefits growth performance and gut microbiota in broilers fed wheat diets. Front. Microbiol. 13:927932. doi: 10.3389/fmicb.2022.927932
Received: 25 April 2022; Accepted: 30 June 2022;
Published: 01 August 2022.
Edited by:
Shyam Sundar Paul, Directorate of Poultry Research (DPR) (ICAR), IndiaReviewed by:
Shiyu Tao, Huazhong Agricultural University, ChinaShimeng Huang, Jiangsu Academy of Agricultural Sciences, China
Copyright © 2022 Gao, Wang, Li, Bai, Liu, Zhong, Ma, Pan and Zhang. This is an open-access article distributed under the terms of the Creative Commons Attribution License (CC BY). The use, distribution or reproduction in other forums is permitted, provided the original author(s) and the copyright owner(s) are credited and that the original publication in this journal is cited, in accordance with accepted academic practice. No use, distribution or reproduction is permitted which does not comply with these terms.
*Correspondence: Teng Ma, mateng@caas.cn
†These authors have contributed equally to this work