- 1Guangdong Provincial Key Laboratory of Colorectal and Pelvic Floor Diseases, Department of Colorectal Surgery, The Sixth Affiliated Hospital, Guangdong Institute of Gastroenterology, Sun Yat-sen University, Guangzhou, China
- 2Guangdong Provincial Key Laboratory of Colorectal and Pelvic Floor Diseases, Department of Gastrointestinal Endoscopy, The Sixth Affiliated Hospital, Guangdong Institute of Gastroenterology, Sun Yat-sen University, Guangzhou, China
- 3School of Medicine, Sun Yat-sen University, Shenzhen, China
NLRP3 inflammasome is implicated in the pathogenesis of inflammatory bowel diseases (IBD). Since guanylate-binding protein 5 (GBP5) induces the NLRP3 inflammasome activity, we aim to investigate the potential role of GBP5 in IBD pathogenesis. The expression of GBP5, NLRP3 inflammasome, and related cytokines and chemokines was examined in two cohorts of IBD patients and healthy controls, by microarray transcriptome analysis and quantitative real-time PCR. Cellular localization of GBP5 in colonic biopsies was examined by immunohistochemistry and immunofluorescence with confocal microscopy. For functional studies, GBP5 was induced by interferon γ or silenced by siRNA or CRISPR/CAS9 technique, and inflammatory activities were evaluated at mRNA and protein levels. We found that the expression of GBP5 was elevated in colonic mucosa in two geographically and culturally distinct IBD cohorts. In colonic tissues of IBD patients, GBP5 expression was mainly confined to immune cells and the levels of GBP5 expression were correlated with those of the inflammatory cytokines and chemokines. In cultured T and macrophage cells, the expression of proinflammatory cytokines and chemokines was increased when GBP5 was induced, while GBP5 deficiency leads to decreased expression of proinflammatory mediators including gasdermin D, caspase 1, cytokines, and chemokines. We conclude that GBP5 is required in the expression of many proinflammatory cytokines and chemokines in intestinal immune cells. In addition, GBP5 may upregulate inflammatory reactions through an inflammasome-mediated mechanism. Since GBP5 plays a proinflammatory role at the early steps of the inflammatory cascades of IBD pathogenesis, and is implicated in IBD patients of distinct genetic and environmental backgrounds, targeting GBP5 could be an effective strategy for the management of IBD.
Introduction
Inflammatory bowel diseases (IBD), including Crohn’s disease (CD) and ulcerative colitis (UC), are chronic and relapsing inflammatory diseases mainly affecting the intestines. The pathogenesis of IBD is not known, but generally believed to be driven by abnormalities in genetics, environment, gut microbiota, and immunity (Seyed Tabib et al., 2020).
NLRP3 (NOD-like receptor family pyrin domain containing 3) inflammasome comprises the inflammasome sensor NLRP3, the adaptor protein ASC (apoptosis-associated speck-like protein containing a CARD domain), and the effector caspase 1. Activation of NLRP3 inflammasome leads to the production of active form of proinflammatory cytokines IL1β and IL-18. Different groups have reported that colonic mucosa of IBD patients exhibited higher levels of caspase 1 (McAlindon et al., 1998), IL1β (Mahida et al., 1989; Ligumsky et al., 1990; Reinecker et al., 1993; McAlindon et al., 1998), and IL-18 (Kanai et al., 2000), demonstrating an association of elevated NLRP3 inflammasome activity with IBD. Animal studies provided a chain of evidence in support of a key role for NLRP3 inflammasome in colitis. NLRP3 gene knockout mice are protected from dextran sodium sulfate (DSS) (Bauer et al., 2010) or 2,4,6-trinitrobenzene sulfonic acid (TNBS) (Bauer et al., 2012) induced colitis compared to wild-type animals. Similar protection was also observed with pharmacological inhibition of caspase 1 with pralnacasan (Bauer et al., 2010) or with caspase 1 knockout mice (Siegmund et al., 2001; Blazejewski et al., 2017). Along this line, inhibition of IL1β or its receptor suppressed experimental colitis (Seo et al., 2015; Neudecker et al., 2017). Importantly, IL-1 receptor blockade using anakinra resulted in a rapid and sustained improvement in patients with colitis (de Luca et al., 2014).
Further support for a causal role of NLRP3 inflammasome in IBD pathogenesis came from studies on CARD8, an inhibitor for NLRP3. Single-nucleotide polymorphism (SNP) studies found that a loss-of-function mutation in CARD8 is associated with IBD (Fisher et al., 2007; Yang et al., 2011). Without the inhibitory CARD8 activity, the unchecked NLRP3 inflammasome activity leads to elevated IL1β and consequently intestinal inflammation. Patients with CARD8 loss-of-function mutation specifically responded to IL1β blockers, but not to anti-TNFα (Mao et al., 2018), demonstrating the role of CARD8-NLRP3-IL1β axis in IBD.
Guanylate-binding protein 5 (GBP5), a member of the GBP family, is a GTPase highly inducible by interferon (Cheng et al., 1983). GBP5 has an emerging role in mediating cell autonomous defenses against intracellular pathogens including those of Francisella novicida (Meunier et al., 2015), Toxoplasma gondii (Matta et al., 2018), and HIV-1 (Krapp et al., 2016). Peripheral blood mononuclear cells (PBMCs) from children with decreased GBP5 expression were more susceptible to respiratory syncytial virus compared to adult (Li et al., 2020). Under pathological conditions, abnormal upregulated expression of GBP5 is caused by dysregulated immune responses, such as rheumatoid arthritis-affected synovial tissue (Haque et al., 2021) and some human malignancies including medullary carcinoma (Friedman et al., 2016), gastric adenocarcinomas (Patil et al., 2018), and glioblastoma (Yu et al., 2021). GBP5, but not other members of the GBP family, promotes the assembly of the NLRP3 inflammasome in response to live L. monocytogenes, S. Typhimurium, and soluble inflammasome priming agents, as demonstrated in GBP5 knockout mice and cell culture models (Shenoy et al., 2012; Santos et al., 2018). Consistent with its effect on inflammasome assembly, GBP5 was reported to stimulate NF-κB signaling, induce the expression of interferon (IFN) and other proinflammatory factors, and inhibit the replication of influenza A virus in a cell culture model (Feng et al., 2017). The dysregulation of the immune–microbiome axis is an important cause of IBD (Graham and Xavier, 2020). In the presence of microbial ligands, NLRP3 inflammasome is crucial for regulation of intestinal homeostasis (Tourkochristou et al., 2019). Therefore, we hypothesize that GBP5 plays a key role in IBD by regulating NLRP3 inflammasome activity. Here, we show that GBP5 is highly elevated in the colonic mucosa of IBD patients and that GBP5 is required in the stimulated secretion of proinflammatory mediators in cell culture models.
Results
Elevated Guanylate-Binding Protein 5 Expression in the Colonic Mucosa of Inflammatory Bowel Diseases Patients
We first examined GBP5 mRNA expression in patients with IBD using a published transcriptome dataset generated with colonic biopsies from European patients. The demographic and clinical characteristics of these patients were described previously (Arijs et al., 2009). Elevated GBP5 expression was observed in both CD and UC patients compared to healthy controls, with fold changes of 8.6 and 8.1, respectively (Figure 1A). To confirm these findings, a validation cohort including healthy controls, CD, and UC patients from south China was enrolled (Supplementary Table 1) and GBP5 mRNA levels in colonic mucosa were evaluated by quantitative real-time PCR (qRT-PCR). Similarly, elevated GBP5 expression was observed in CD and UC patients compared to healthy controls, with fold changes of 13.1 and 3.8, respectively (Figure 1B).
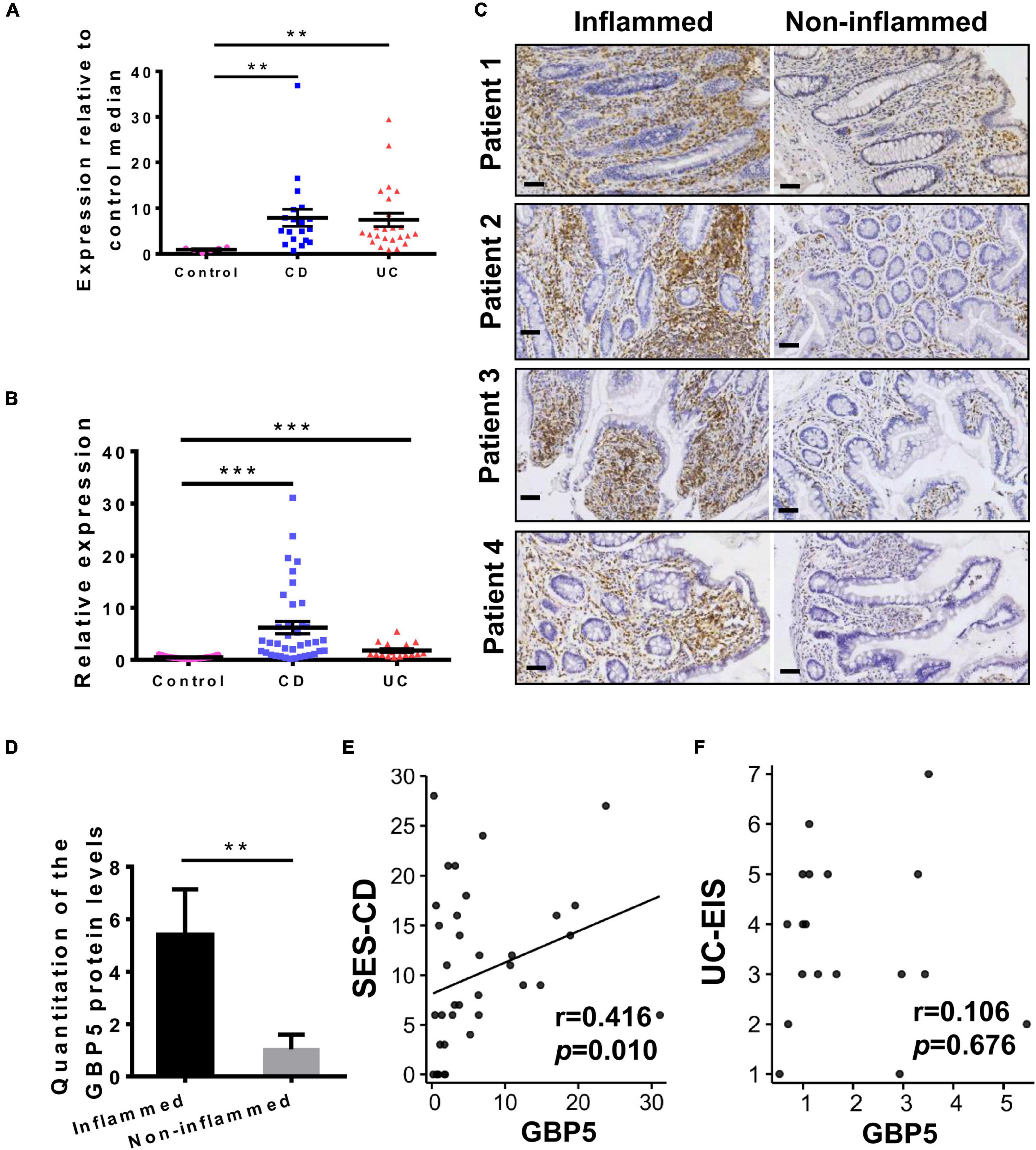
Figure 1. GBP5 is highly expressed in the inflamed intestinal tissue of IBD patients. (A) Messenger RNA expression of GBP5 in the colonic mucosa of healthy controls (n = 6), patients with Crohn’s disease (CD, n = 19), and ulcerative colitis (UC, n = 24). Data are from a microarray dataset generated from a European cohort. **P < 0.01, Dunn’s multiple comparison test. (B) Quantitative RT-PCR analysis of GBP5 mRNA in colonic mucosa from a Chinese cohort including healthy controls (n = 35), and patients with CD (n = 38) and UC (n = 17). ***P < 0.001, Dunn’s multiple comparison test. (C) Immunohistochemical staining of GBP5 in colonic mucosa from four representative patients with CD. Images of inflamed and non-inflamed sites from the same patient are compared side by side. Bar = 50 μm. (D) Quantitation of the GBP5 staining in the inflamed and non-inflamed sites in (C). **P < 0.01, paired Student’s t-test. (E,F) Spearman’s correlation analysis of the mucosal GBP5 expression levels and endoscopic severities. GBP5 expression levels are from quantitative RT-PCR results. SES-CD, simple endoscopic score for Crohn’s disease; UC-EIS, ulcerative colitis endoscopic index of severity.
To examine the GBP5 expression at protein level, inflamed and adjacent non-inflamed colonic tissues from the same CD patients who underwent colon resection were subjected to immunohistochemistry (IHC) staining. With all the samples examined, GBP5 staining was more intense in the inflamed tissue than in the non-inflamed tissue (Figures 1C,D). Interestingly, GBP5 positive cells were mostly found in the lamina propria, with a few exceptions located at the luminal or glandular epithelium (Figure 1C).
To understand the potential role of GBP5 in clinical outcome, correlation analysis between mucosal GBP5 expression and disease degree of IBD was performed. We used the simple endoscopic score for Crohn’s disease (SES-CD) and the ulcerative colitis endoscopic index of severity (UC-EIS) to evaluate the severity of CD and UC, respectively. The expression of GBP5 was positively correlated with SES-CD (Figure 1E, Spearman’s correlation coefficient = 0.416; P = 0.010), but not correlated with UC-EIS (Figure 1F, Spearman’s correlation coefficient = 0.106; P = 0.676).
Immune Cell-Specific Guanylate-Binding Protein 5 Expression in the Colon of Crohn’s Disease Patients
To better understand the tissue distribution of GBP5, IHC staining of GBP5 was examined at the entire depth of the inflamed and non-inflamed colonic tissues of CD patients who underwent colon resection. In the inflamed colon, GBP5 positive cells were densely populated in mucosa, mostly lamina propria (Figure 2AI). Besides, GBP5 positive cells were frequently observed in other layers of the colonic tissue including submucosa, circular muscle, longitudinal muscle, serosa, and mesentery (Figures 2AII–VI). Less GBP5 positive cells were observed in colonic layers of the non-inflamed tissue from the same patient (Figure 2B). It is noteworthy that GBP5 was not detected in muscle cell (Figures 2AIII,IV) or endothelial cell, but detected in the blood cells (Figure 2AVI). Another outstanding observation was the enrichment of GBP5 positive cells in Peyer’s patch (mucosa, Figure 2AVII) and lymph node (submucosa, Figure 2AVIII). The elevated expression of GBP5 across the colonic layers of CD patients is in line with the fact that CD is usually inflicted by transmural inflammation.
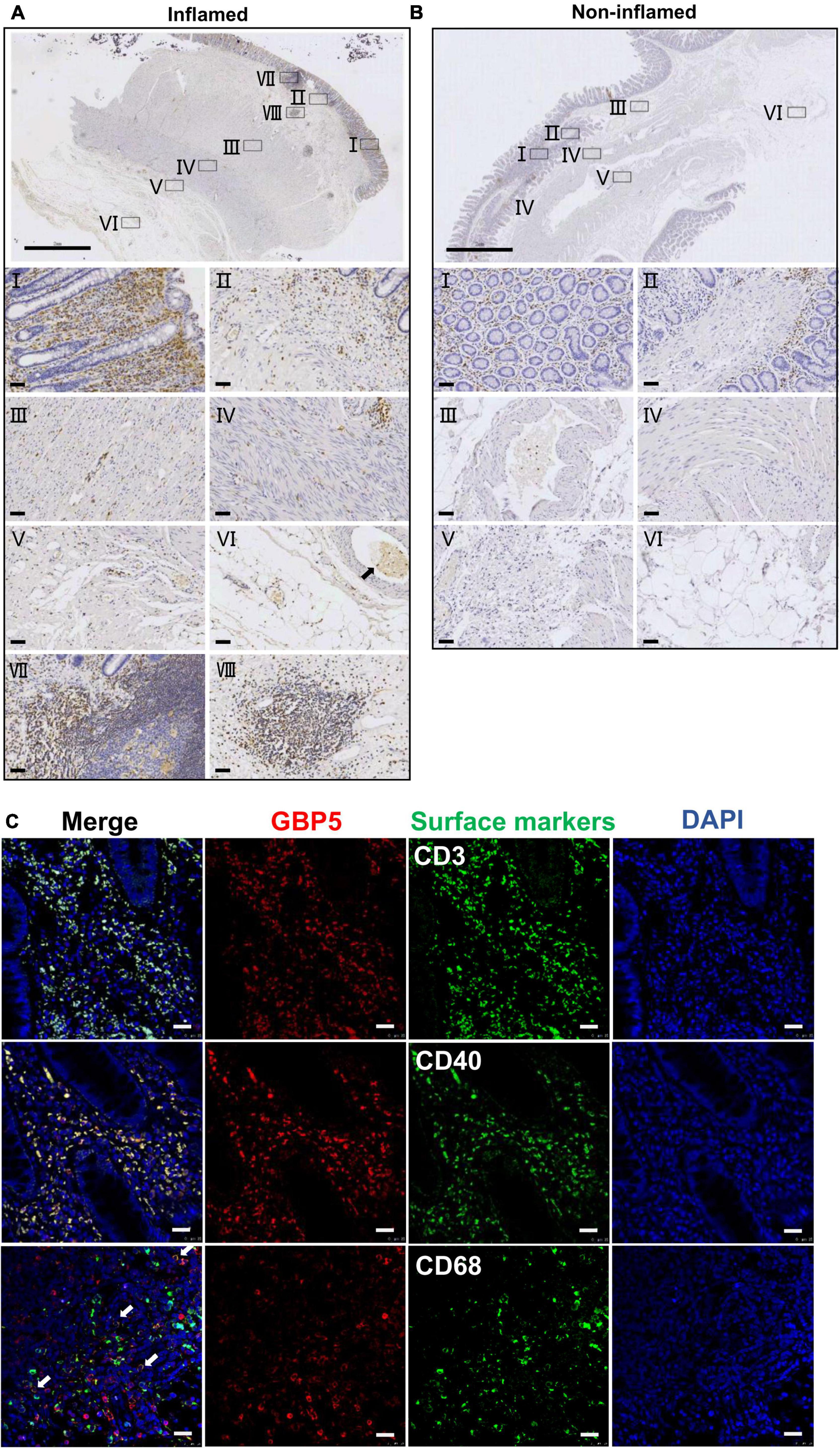
Figure 2. Immune cell-specific GBP5 expression in patients with CD. (A) Immunohistochemical staining of a colonic biopsy section from a representative CD patient with an anti-GBP5 antibody: an inflamed area. The top image shows a panoramic view of the section. Bar = 2 mm. Details are shown for boxed areas representing different anatomic structures. I, mucosa; II, muscularis mucosa and submucosa; III, circular muscle; IV, longitudinal muscle; V, serosa; VI, mesentery, black arrow indicates a blood vessel; VII, Peyer’s patch in mucosa; VIII, lymph node in submucosa. Bar = 50μm. (B) Immunohistochemical staining of a colonic biopsy section from a representative CD patient with an anti-GBP5 antibody: a non-inflamed area. The top image shows a panoramic view of the section. Bar = 2 mm. Details are shown for boxed areas representing different anatomic structures. I, mucosa; II, lamina propria, muscularis mucosa and submucosa; III, a blood vessel in submucosae layer; IV, circular muscle and longitudinal muscle; V, serosa; VI, mesentery. Bar = 50μm. (C) Immunofluorescence staining of inflamed colon tissue from a representative CD patient with antibodies against GBP5, CD3, CD40, and CD68. White arrow indicates the overlap of GBP5 and CD68. Bar = 50 μm.
To determine the cellular distribution of GBP5, inflamed colon tissues from CD patients were subjected to immunofluorescence staining for GBP5 and immune cell marker proteins. Confocal microscopy showed that most of the CD3 positive cells, most of the CD40 positive cells, and the majority of CD68 positive cells expressed GBP5 (Figure 2C). CD3, CD40, and CD68 are marker proteins for T lymphocytes, antigen-presenting cells (dendritic cells, macrophages, and B cells) (Croft and Siegel, 2017), and macrophages, respectively. Thus, the above results demonstrated immune cell-specific expression of GBP5.
Transcriptome Analysis Reveals Association Between Guanylate-Binding Protein 5 and Inflammatory Reaction Pathways
To identify potential links between GBP5 and IBD pathogenesis, we performed hierarchical clustering of GBP5 with 102 available cytokine and chemokine genes with the transcriptome dataset generated from colonic mucosa of IBD patients (GSE16879) (Arijs et al., 2009). The clustering result showed that GBP5 shared a similar expression pattern with gene coding for proinflammatory cytokines and chemokines including IL1B and IL-6, and they were highly elevated in most of the CD and UC patients compared to the healthy controls (Figure 3A). Interestingly, the anti-inflammatory cytokine IL-10 exhibited a similar expression pattern as GBP5. The elevated IL-10 expression in IBD was observed previously and was thought to reflect a futile effort of patient immune system to control the excessive inflammatory reaction (Autschbach et al., 1998). Next, we performed Pearson’s correlation analyses between every two genes based on the transcriptome data (Figure 3B). With a threshold of correlation coefficient greater than 0.6, 486 genes were correlated with GBP5. In comparison, 226, 123, and 112 genes were correlated with GBP1, GBP2, and GBP4, respectively. Apparently, at the transcription level, GBP5 had the largest impact on IBD among all GBP family genes. We then performed Gene Ontology (GO) enrichment analysis with all 486 genes correlated with GBP5. The top “biological process,” “cellular compartment,” and “molecular function” are listed in Figure 3C. The top “biological process,” including “leukocyte migration,” “response to molecule of bacterial origin,” “response to lipopolysaccharide,” and “cellular response to molecule of bacterial origin,” is related to inflammatory responses to bacterial infection. In addition, the top “cellular compartment” and the top “molecular function,” such as “collagen-containing extracellular matrix” and “extracellular matrix structural constituent,” are closely related to lymphocyte migration and infiltration. These results are consistent with previous reports that GBP5 plays a critical role in host defense against bacterial pathogens (Meunier et al., 2014; Pilla et al., 2014).
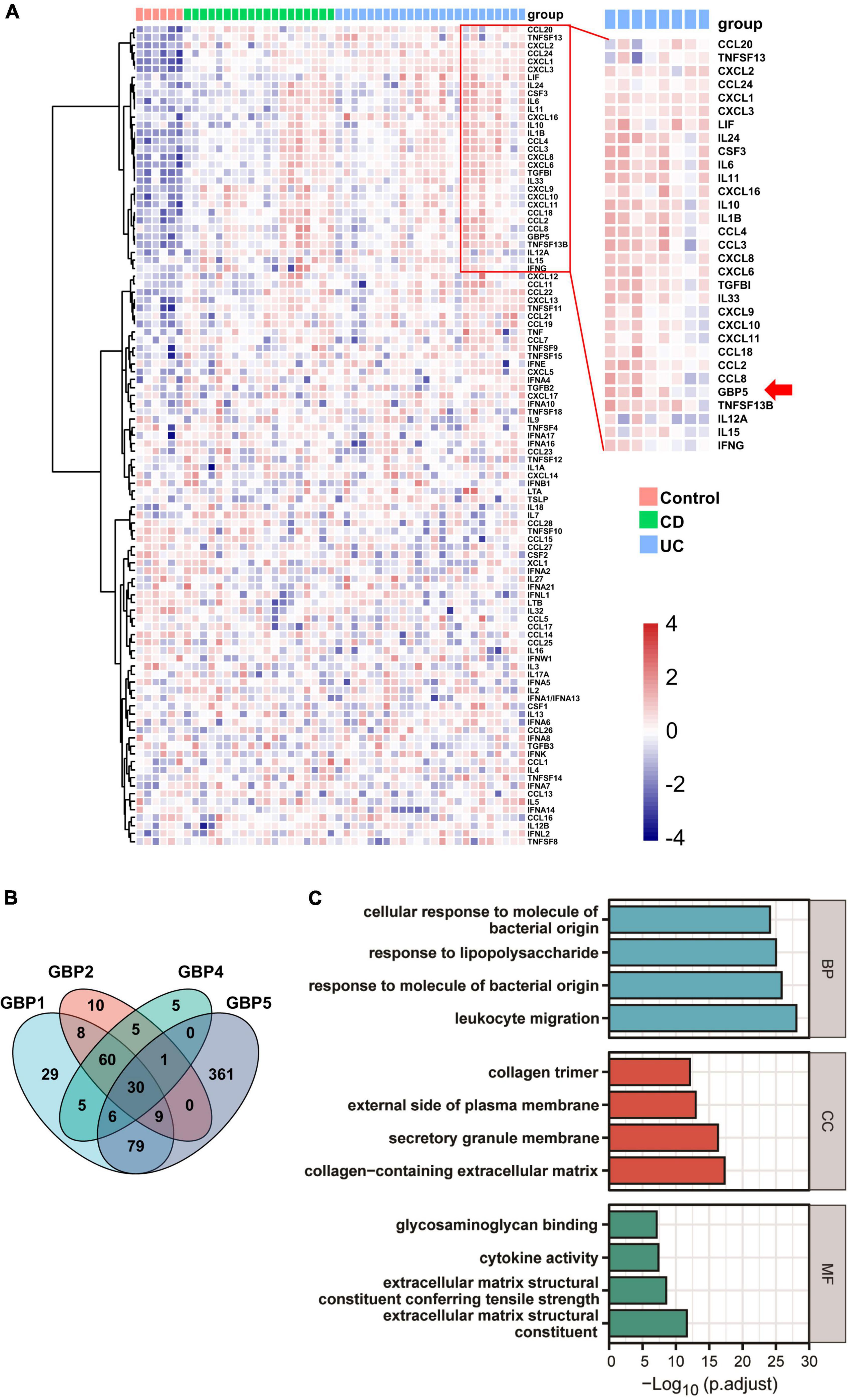
Figure 3. GBP5 is implicated in inflammatory processes in IBD according to transcriptome analysis. (A) Heatmap for mRNA expression levels of GBP5 (arrow), available cytokines, and chemokines based on transcriptome data generated from colonic mucosal biopsies of healthy controls (n = 6), patients with Crohn’s disease (CD, n = 19), and ulcerative colitis (UC, n = 24). Unsupervised hierarchical clustering of GBP5, cytokine, and chemokine genes was performed. Genes in the red box are more closely clustered with GBP5. (B) Venn plot of the number of genes associated with GBP1, GBP2, GBP4, and GBP5, based on their mRNA expression levels. Associations with Pearson’s correlation coefficient no less than 0.6 are counted. (C) Gene Ontology (GO) analysis of GBP5 associated genes. The cutoff value for the input gene list is Pearson’s correlation coefficient no less than 0.6. BP, biological process; CC, cellular compartment; MF, molecular function. All samples from GSE16879 are included (n = 90).
Guanylate-Binding Protein 5 Deficiency Downregulates Proinflammatory Chemokines and Cytokines in Cultured Cells
For further understanding of the association between GBP5 and inflammatory processes, we performed GBP5 siRNA knockdown in Jurkat cells to determine the impact of GBP5 on chemokine and cytokine secretion. Diminished GBP5 expression in Western blot analysis indicated efficient knockdown of GBP5 gene in Jurkat cells (Supplementary Figure 1A). The cell culture supernatants were then subjected to Luminex chemokine and cytokine assay. Compared to cells treated with control RNA, upon stimulation with IFNγ and lipopolysaccharide (LPS), GBP5 siRNA-treated cells exhibited decreased levels of CCL2, CCL8, CCL13, CCL25, CXCL10, CXCL11, CXCL12, CXCL16, CX3CL1, IL-1β, IL-10, and MIF in the cell culture supernatant (Supplementary Figure 1B). In addition, CCL19, CCL20, CCL22, CXCL2, and CXCL13 were not detected in the supernatant after GBP5 knockdown, but detected in the supernatant of the cells treated with control RNA (Supplementary Figure 1B). GBP5 knockdown also caused a trend of decreased levels in some proinflammatory chemokines and cytokines, including CCL1, CCL11, CCL15, CCL21, CCL26, CCL27, CXCL1, CXCL5, IL-16, and TNF-α, but statistical significance was not achieved (Supplementary Figure 1B). CCL7, CCL17, CCL23, CXCL6, CXCL9, GM-CSF, IL-2, IL-4, and IL-6 were not detected in any of the samples (Supplementary Figure 1B).
For a precise evaluation of GBP5 impact on chemokine and cytokine secretion, GBP5 gene was removed from THP-1 cell by CRISPR/CAS9 method and confirmed by Western blot showing no GBP5 expression in GBP5–/– THP-1 cells (Figure 4A). Global mRNA expression of the GBP5–/– THP-1 cells was assessed by RNA sequencing. As expected, GBP5 mRNA was reduced in GBP5–/– THP-1 cells compared to the wild-type controls, with or without induction by IFNγ plus LPS (Figures 4B,C). Surprisingly, GBP5 deficiency greatly reduced the mRNA expressions of many inflammation and immune related genes, including (1) IFNγ response genes such as AIM2, CASP1, GSDMD, and IL1B, and (2) IFNγ non-response genes such as NLRP3, CASP4, CASP5, and IL-18 (Figure 4B).
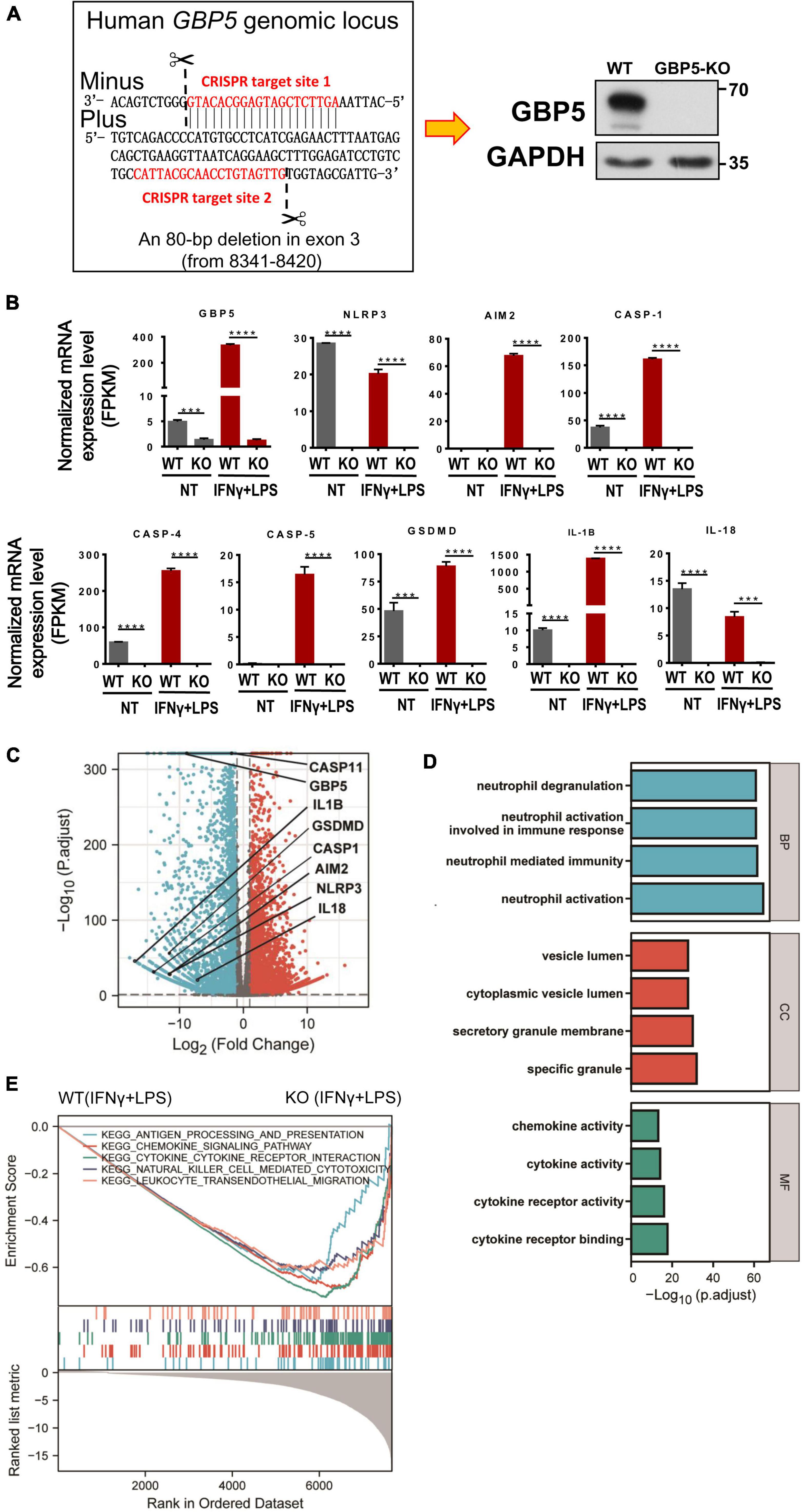
Figure 4. GBP5 knockout downregulated the expression of proinflammatory mediator genes in THP-1 cells. The transcriptomes of wild-type and GBP5–/– THP-1 cells, with or without stimulation [IFNγ and lipopolysaccharide (LPS)], respectively, were analyzed by RNAseq technique. N = 3 for each treatment group. (A) Generation of GBP5 knockout (GBP5–/–). THP-1 cell line by CRISPR/Cas9-mediated genome editing. The two CRISPR target sites are highlighted in red. Loss of GBP5 was confirmed by Western blot. WT, wild-type control. (B) Messenger RNA expression of GBP5 and related genes in wild-type and GBP5–/– THP-1 cells (clone B2), with or without stimulation (IFNγ and LPS), respectively. FPKM, fragments per kilobase million. ***P < 0.001; ****P < 0.0001; unpaired Student’s t-test. (C) Differential gene expression between wild-type and GBP5–/– THP-1 cells: volcano plot. Vertical and horizontal dashed lines indicate the cutoff values for differentially expressed genes:| Log2 (Fold Change)| > 1 and p.adjust < 0.05. (D) GO (Gene Ontology) analysis of downregulated genes in GBP5 knockout cells. The cutoff values for selecting differentially expressed genes for the input gene list: Log2(Fold Change) < -2, and p. adjust < 0.05. BP, biological process; CC, cellular compartment; MF, molecular function. (E) Gene set enrichment analysis (GSEA) of GBP5–/– THP-1 cells (clone B2) transcriptome compared to wild-type THP-1 cells. Upper panel: the enrichment score curves of the top KEGG (Kyoto Encyclopedia of Genes and Genomes) pathways exhibiting decreased expression in GBP5–/– THP-1 cells: immune related pathways. Middle panel: distribution of the genes related to the pathways indicated in the upper panel. The genes were ranked according to their differential expression between wild-type and GBP5–/– THP-1 cells. Genes of higher rank (left) exhibit relatively higher expression in wild-type THP-1 cells. Lower panel: Graphical representation of the correlations of the gene expression levels with the phenotypes: wild-type or GBP5 knockout. Genes on the right are more negatively correlated with GBP5 deficiency.
Comparing the GBP5–/– with wild-type THP-1 cells, many differentially expressed genes, including 2,298 genes upregulated and 2,813 genes downregulated in GBP5–/– cells, were identified (Figure 4C). The mRNA expression for most proinflammatory cytokines and chemokines was reduced or undetected in GBP5–/– cells (Supplementary Figure 2). We then performed GO enrichment analysis with the list of genes downregulated in GBP5–/– cells. The identified top “biological process,” “cellular compartment,” and “molecular function” are related to inflammatory signaling, immune cell migration, neutrophil activation, secretion of cytokines and chemokines, and other immune and inflammatory events (Figure 4D).
Using a different approach for functional analysis, gene set enrichment analysis (GSEA) identified a similarly broad suppression of immune and inflammatory functions in GBP5–/– THP-1 cells, including antigen processing, chemokine signaling, cytokine signaling, leukocyte migration, and NK cell cytotoxicity (Figure 4E). Compared to GO enrichment analysis that uses partial information of the biological pathways (in our case, the downregulated genes), GSEA considers all available information of relevant genes for functional analysis. Thus, both methods identified immune and inflammatory pathways as downregulated pathways in GBP5–/– THP-1 cells.
Next, we examined the altered immune and inflammatory pathways at protein level. Compared to the wild-type controls, THP-1 cells with GBP5 deficiency exhibited decreased levels of CCL2, CCL8, CCL13, CCL21, CCL25, CCL26, CXCL5, CXCL11, CXCL12, CX3CL1, IL1β, IL-10, and IL-16 in the cell culture supernatant (Figure 5). In addition, CCL1, CCL3, CCL7, CCL11, CCL15, CCL19, CCL20, CCL22, CCL23, CCL24, CCL27, CXCL1, CXCL2, CXCL6, CXCL9, CXCL10, CXCL13, CXCL16, IL-2, IL-4, IL-6, CXCL8, TNF-α, and GM-CSF were not detected in the supernatant of GBP5 knockout cells, but detected in the WT controls (Figure 5). CCL17 was again not detected in any of the samples (Figure 5). Therefore, GBP5 deficiency led to decreased secretion for most of the inflammatory mediators analyzed.
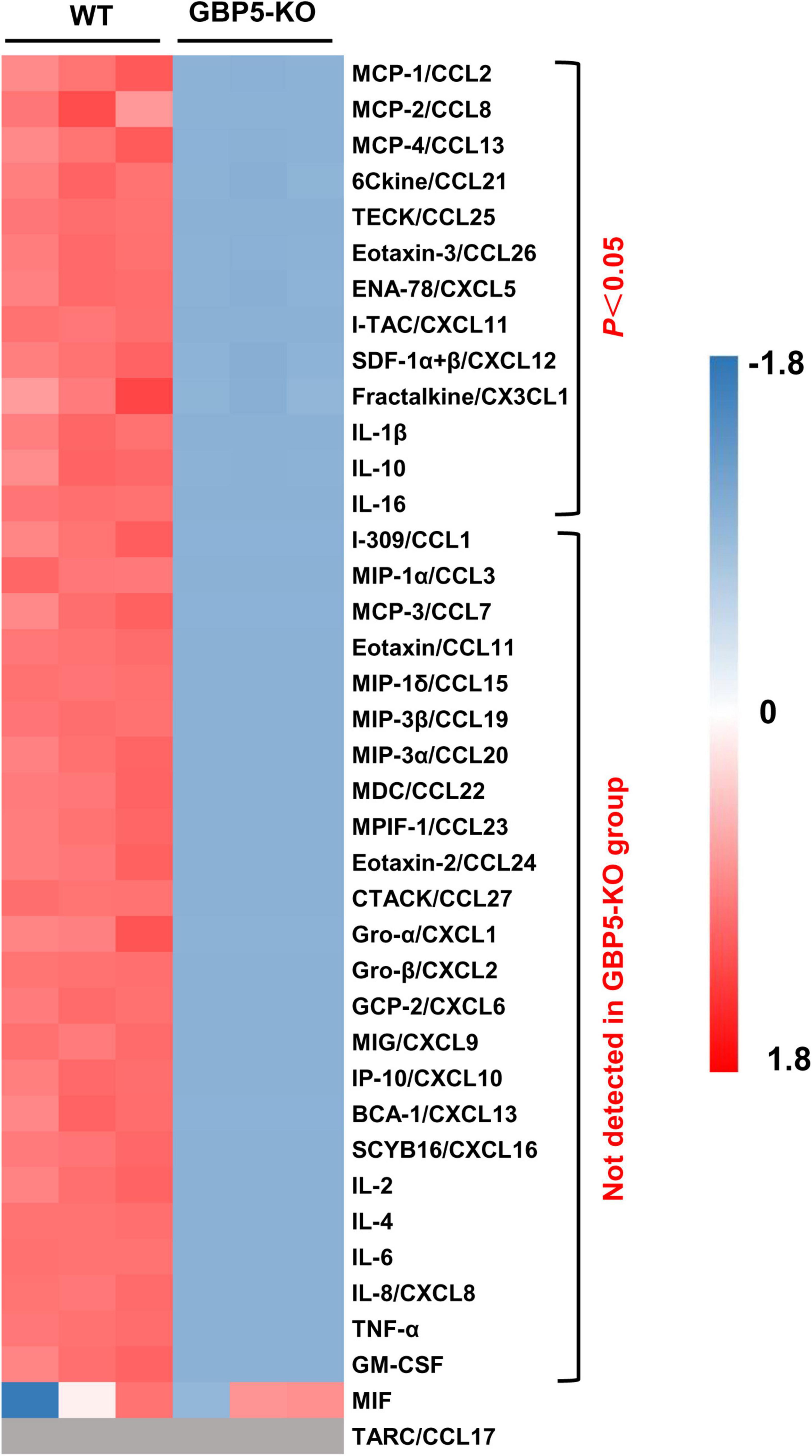
Figure 5. Decreased chemokine and cytokine secretion in GBP5 knockout THP-1 cells. The protein levels of chemokines and cytokines in the cell culture supernatant of wild-type (WT) and GBP5 knockout (KO) THP-1 cells are plotted. Cells were primed with IFNγ and LPS before sample collection. The concentrations of cytokines and chemokines were determined by Luminex liquid suspension chip. Data were normalized as (x-mean)/SD. Gray blocks indicate no detection. P-values were from Student’s t-tests.
Given the close relation among GBP5, inflammasomes, and IL1β, we examined the intracellular protein expression of some related molecules. Western blots showed that GBP5 deficiency greatly decreased the expression of gasdermin D, caspase 1, and pro-IL1β (Figure 6). Since the active form for gasdermin D or caspase 1 was not observed in any of the samples, the decreased production of IL1β is not likely due to impaired proteolytic activation by inflammasomes in GBP5 deficiency. Rather, the transcription and expression analyses indicate that the decreased IL1β production is part of the consequence of broad inhibition of inflammatory gene expression in GBP5 deficiency.
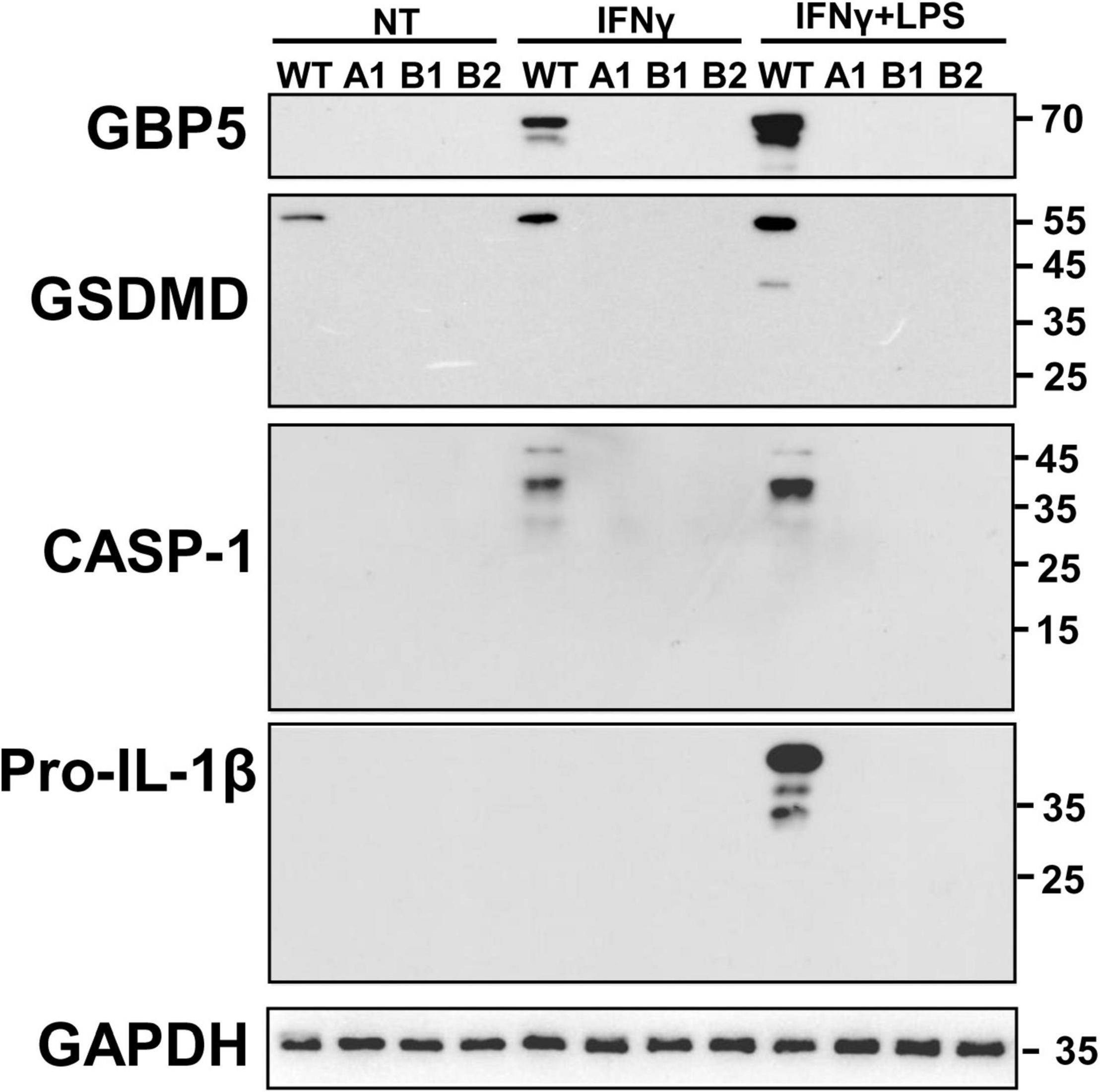
Figure 6. Diminished expression of inflammasome related proteins in GBP5 knockout THP-1 cells was subjected to wild-type THP-1 cells, and GBP5 knockout THP-1 cell clones (A1, B1, and B2) were left untreated (NT), treated with IFNγ (25 ng/ml) only, or treated with IFNγ (25 ng/ml) plus LPS (500 ng/ml) for 16 h, before Western blot analysis with antibodies against GBP5, gasdermin D (GSDMD), caspase 1 (CASP1), pro-IL1β, and GAPDH, respectively.
Discussion
Here, we show for the first time the elevated expression of GBP5 in colonic mucosa of patients with IBD and that GBP5 is required for the stimulated secretion of inflammatory cytokines and chemokines, including IL1β, from T lymphocytes and macrophages. In colonic tissues of IBD patients, the expression of GBP5 was mainly confined to immune cells and the levels of GBP5 expression were correlated with those of the inflammatory markers. Importantly, the expression of proinflammatory cytokines and chemokines was increased when GBP5 was induced, while GBP5 deficiency leads to decreased production of the proinflammatory mediators in T and macrophage cells. Thus, the specific role of GBP5 in IBD pathogenesis is to facilitate the stimulated production of inflammatory mediators in intestinal immune cells. Our observation of highly elevated GBP5 expression at the inflamed colonic mucosa in two geographically and culturally distinct IBD cohorts indicates that GBP5 plays a common and important role in the early steps of IBD pathogenesis and, therefore, is a potential therapeutic target for the management of IBD patients of various genetic and environmental backgrounds.
Our results suggest that GBP5 may promote inflammation through two mechanisms. First, gene knockout studies indicated that GBP5 is required for the stimulated expression of many inflammatory cytokines, such as IL1β, and is required for the expression of many IFNγ non-response cytokines, such as IL-18. Studies at both mRNA and protein levels indicated that GBP5 had a large impact on the expression of these proinflammatory cytokines and chemokines. Once IL1β is induced, in turn, IL1β may stimulate the production of other proinflammatory cytokines and chemokines including IL-6, IL8, CCL2, CCL5, CXCL1, CXCL2, CXCL3, CXCL6, and IFNγ (Cooper et al., 2001; Hengartner et al., 2015). The outstanding knowledge gap is how the cytoplasmic inflammasome interacting GBP5 regulates gene expression? Second, since GBP5 promotes NLRP3 inflammasome activity (Shenoy et al., 2012), and NLRP3 inflammasome is required for the excessive inflammatory reactions in IBD (reviewed in Yuan et al., 2018), it is expected that NLRP3 inflammasome may mediate the proinflammatory effect of GBP5. This is supported by our observation that the expression of caspase 1, gasdermin D, and IL1β was elevated when GBP5 is induced and was reduced when GBP5 was downregulated by siRNA or gene knockout. The puzzle here is the observation that the active forms of caspase 1 and gasdermin D were not observed in IFNγ primed THP-1 cells. For both mechanisms, future efforts are needed to unravel the puzzles and to join the knowledge gaps.
One major challenge for the interpretation of our data came from a few studies that reported protective role for NLRP3 inflammasome in mouse models of colitis, that is, mice lacking NLRP3, ASC, or caspase 1 were more susceptible for colitis in DSS and azoxymethane (AOM) models (Allen et al., 2010; Dupaul-Chicoine et al., 2010; Zaki et al., 2010a,b; Hirota et al., 2011). The discrepancies regarding the role of NLRP3 inflammasome in colitis may be explained by environmental factors including microbiota (Bauer et al., 2012) and diet (Youm et al., 2015). In addition, Zaki et al. (2010a) argued that the protective role of NLRP3 inflammasome is due to its beneficial effect on epithelial barrier function via induction of IL-18 in epithelial cells. However, this argument does not reconcile with the facts that little NLRP3 inflammasome activity presents in the intestinal epithelial cells and that IL-18 production by these cells is independent of NLRP3 inflammasome (Yao et al., 2017).
Besides the conventional NLRP3 inflammasome-mediated mechanism, a caspase-11-dependent pathway may relay the inflammatory signal from GBP5. Mice and cell culture studies suggested that, upon detection of cytoplasmic LPS, GBP proteins encoded on mouse chromosome 3 activate caspase-11-dependent cell autonomous immune responses (Pilla et al., 2014). This is consistent with current knowledge that structural and functional alterations in the gut microbiota, especially in gram-negative bacteria (Palmela et al., 2018), and their cell wall component LPS (Caradonna et al., 2000), are implicated in the pathogenesis of IBD. In support of a causal role for bacteria and LPS in IBD, we identified the following pathways as the top features of the GBP5 associated colonic mucosal transcriptome in IBD: “response to molecule of bacterial origin,” “response to lipopolysaccharide,” and “cellular response to molecule of bacterial origin.” On the contrary, it is noteworthy that virus infection is implicated in IBD pathogenesis (Yang et al., 2019), and GBP5 has been identified as an IFN-induced virus restriction factor that interferes with virus assembly (Krapp et al., 2016), or through induction of innate immune mediators (Feng et al., 2017). Further study is required to clarify potential collaborative roles of caspase-11 and GBP5 in IBD pathogenesis, and whether viral or bacterial infection triggers elevated GBP5 expression in the intestines of IBD.
The limitations of our study include lack of in vivo study and small sample size. Therefore, further experimentations have been planned to validate our findings. In addition, other interesting questions emerged from our study. For example, GBP5 seemed to play a more important role in CD than in UC. With the prospective cohort enrolled in our hospital, the expression level of GBP5 was more elevated in CD than in UC. Consistently, the expression levels of GBP5 were correlated with the disease severities of CD, but not that of UC. However, the differential expression of GBP5 was not observed with treatment-naive patients with IBD (Figure 1A). Therefore, the differential expression of GBP5 in CD and UC may be a consequence of different treatments.
In summary, GBP5 is highly expressed in the colonic immune cells of IBD patients. Induction of GBP5 is required for the stimulated production of proinflammatory cytokines and chemokines, while GBP5 deficiency decreases the expression of the proinflammatory mediators. Since GBP5 plays a proinflammatory role at the early steps of the inflammatory cascades of IBD pathogenesis, and is implicated in IBD patients of distinct genetic and environmental backgrounds, targeting GBP5 could be an effective strategy for the management of IBD.
Materials and Methods
Human Samples
Colonic pinch biopsies were obtained from patients with IBD at the Sixth Affiliated Hospital of Sun Yat-sen University, Guangzhou, China. Healthy control colonic biopsy samples were from patients suspected of intestinal diseases but diagnosed normal according to biopsy. The simple endoscopic score for Crohn’s disease (SES-CD) and the ulcerative colitis endoscopic index of severity (UC-EIS) were used to evaluate the endoscopic severity of CD and UC, respectively, by endoscopist as previously described (Daperno et al., 2004; Travis et al., 2013). Colon resections were obtained from patients with IBD who underwent colectomy. Non-inflamed control specimens were obtained 5 cm away from the inflamed lesion. Written informed consents were obtained from all donors. This study was approved by the Institutional Review Board of the Sixth Affiliated Hospital of Sun Yat-sen University.
RNA Extraction, Complementary DNA Synthesis, and Quantitative Real-Time PCR
Total RNA from cultured cells was isolated using TRIzol (Invitrogen, United States). For RNA isolation from clinical samples, colonic tissues were disrupted with lysis beads (Luka, China) before RNA isolation with AllPrep® DNA/RNA Micro Kits (Qiagen, United States). Using total RNA as template, Complementary DNA (cDNA) was synthesized with Fast Reverse Transcription kits (ES Science, China). For the gene expression analysis, qRT-PCR was performed with FastStart Essential DNA Green Master (Roche, United Kingdom) on LightCycler® 96 (Roche, United Kingdom). The gene expression levels were normalized with β-actin gene as the reference gene according to the 2–ΔΔCT method. The sequences of the qRT-PCR primers are as follows: GBP5, forward: 5′-CCTGATGATGAGCTAGAGCCTG-3′, and reverse: 5′-GCACCAGGTTCTTTAGACGAGA; β-actin, forward: 5′-TT GTTACAGGAAGTCCCTTGCC-3′, and reverse: 5′-ATGCT ATCACCTCCCCTGTGTG-3′.
Transcriptome Analysis
Global transcriptome analysis of the colonic tissue from IBD patients used the published microarray dataset generated from the colonic mucosa of IBD patients and healthy controls (GSE16879)1 (Arijs et al., 2009). Data were normalized by MAS5 method before further analysis. Unsupervised hierarchical clustering and Gene Ontology (GO) enrichment were performed with R (3.6.3).
For transcriptome analysis of the wild-type and GBP5 knockout THP-1 cells, total RNA from parental THP-1 cells and GBP5 knockout THP-1 clone B2, with and without stimulation (IFNγ and LPS) for 16 h, respectively, was isolated as described above. The RNA preparation was qualified with an Agilent 2100 Bioanalyzer (Thermo Fisher Scientific, United States). Oligo (dT)-attached magnetic beads were used to purify mRNA, which served as templates for cDNA synthesis. Libraries were constructed with the cDNA preparations at BGI-Shenzhen, China, amplified with phi29 to make DNA nanoball (DNB) which had more than 300 copies of one molecular, and sequenced on a BGIseq500 platform (BGI-Shenzhen, China). The sequencing data were filtered with SOAPnuke (v1.5.2) to remove sequences of adapters, to remove reads with low-quality base percentage (base quality less than or equal to 5) higher than 20%, and to remove reads whose unknown base (“N” base) percentage is higher than 5%. The clean reads were mapped to the reference genome using HISAT2 (v2.0.4). After that, EricScript (v0.5.5) and rMATS (V3.2.5) were used to identify fusion genes and differentially spliced genes (DSGs), respectively. Bowtie2 (v2.2.5) was applied to align the clean reads to a human mRNA database built by BGI (Shenzhen, China), and the expression level of gene was calculated by RSEM (v1.2.12). The heatmap was drawn by pheatmap (v1.0.8) according to the gene expression levels. Differential expression analysis was performed using the DESeq2 (v1.4.5) with Q-value ≤ 0.05. GO2 and KEGG3 enrichment analysis of differentially expressed genes was performed by phyper4 based on Hypergeometric test. The statistical significance for multiple tests was adjusted by Bonferroni method. Transcriptome data for wild-type and GBP5 knockout THP-1 cells are available at https://www.biosino.org/node/, accession ID: OEP002938.
Immunohistochemistry
Formalin-fixed paraffin-embedded intestine tissues from inflamed and non-inflamed sites of IBD patients were sectioned and collected onto glass slides. Antigen retrieval was performed in 10 mM sodium citrate (pH6.0) at 100°C for 15 min. GBP5 was stained with PV-6000 immunohistochemistry (IHC) kit (ZSGB-BIO, China) according to the protocol of the manufacturer, with the GBP5 antibody (Catalog #: 67798) from Cell Signaling (United States). The images were collected with Slide Scanning System SQS-1000 (TEKSQRAY, China). Quantitation of the GBP5 signals was performed with ImageJ with IHC-Toolbox plugins.
Immunofluorescence
The tissue section was prepared as in IHC method. Primary antibodies include anti-GBP5 (Cat. #: 67798, Cell Signaling, United States), anti-CD3 (Cat. #: 60181-1-Ig, Proteintech, China), anti-CD40 (Cat. #: ab280207, Abcam, United States), and anti-CD68 (Cat. #: ARG10514, Arigo, China). Secondary antibodies include Alexa Fluor647 or 488 conjugated goat anti-rabbit or goat anti-mouse antibodies (Invitrogen, United States). Cell nuclei were stained with 4′,6-diamidine-2′-phenylindole dihydrochloride (DAPI, Sigma-Aldrich, United States). Slides were visualized with a TCS-SP8 confocal microscope (Leica, Germany).
Cell Culture and Gene Knockdown With siRNA
Human cell lines Jurkat, THP-1, and 293T cells were purchased from American Type Culture Collection (ATCC, United States). Cells were cultured in RPMI 1640 medium (Gibco, United States) supplemented with 10% fetal bovine serum (Gibco, United States) maintained at 37°C and 5% CO2. To knockdown GBP5 in Jurkat cells, a pool of two different siRNAs (1, 5′-GCCATAATCTCTTCATTCA-3′; and 2, 5′-GCTCGGCTTTACTTAAGGA-3′) were used. Scrambled sequence of the siRNA was used as control. RNA was synthesized at Ribo-Bio (China) and provided lyophilized. RNA was reconstituted with nuclease-free water to reach a final concentration of 20μM, before transfection using Lipofectamine RNAiMAX (Life technologies, United States) following the manufacturer’s instructions. At 48 h post-transfection, cells were stimulated with human interferon γ (IFNγ, 25μg/ml, Novoprotein, China) and lipopolysaccharides from Escherichia coli O55:B5 (LPS, 500 ng/ml, Sigma-Aldrich, United States) for 16 h before harvest.
Western Blot
Cells were lysed in RIPA (Radio-Immunoprecipitation Assay) buffer (P0013B, Beyotime, China) supplemented with protease inhibitors (Cat. #: HY-K0011, MedChemExpress, China) and phosphatase inhibitors (Cat. #: HY-K0021 and HY-K0022, MedChemExpress, China). Lysates were mixed with loading buffer containing SDS and DTT and heated at 95°C for 5 min. Proteins of interest were probed with the following antibodies: anti-GBP5 antibody as described above, anti-GSDMD (Cat. #: 97558, Cell Signaling, United States), anti-caspase 1 (Cat. #: 3866, Cell Signaling, United States), anti-pro-IL1β (Cat. #: 12703, Cell Signaling, United States), and anti-GAPDH (Cat. #: 60004-1-Ig, Proteintech, China). HRP-conjugated secondary antibodies, anti-rabbit IgG and anti-mouse IgG, were from Proteintech, China.
Guanylate-Binding Protein 5 Knockout Cell Line
Two all-in-one sgRNA plasmids (HCP260159-CG04-3-10-a and HCP260159-CG04-3-10-b) for human GBP5 were purchased from GeneCopoeia (China). In addition to DNA elements required for the generation of recombinant lentivirus, these plasmids carry Cas9 sequence and one of the following sgRNAs targeting the exon 3 of GBP5: gRNA1, 5′-AGTTCTCGATGAGGCACATG-3′; gRNA2, 5′-CATTACGCAACCTGTAGTTG-3′. Recombinant lentivirus was produced by transfecting 293T cells with the two sgRNA plasmids together with lentiviral packaging plasmids psPAX2 and pMD2. GBP5 knockout THP-1 cells were generated by infecting THP-1 cells with the recombinant lentiviruses followed by G418 selection. After obtaining single-cell colonies from limiting dilution, three THP-1 clones (A1, B1, and B2) harboring all-allelic deletion of the 80-bp in exon 3 of GBP5 locus were identified by PCR and validated by DNA sequencing of the PCR products. The primers used for PCR screening are as follows: forward, 5′- AGTAGTATGTCCCCAGGTTC-3′, and reverse, 5′- AAGACCAGCTGTAGCCTAAA -3′.
Luminex-Based Assays
The cell culture supernatant was collected after IFNγ + LPS stimulation. Multiple cytokines and chemokines were examined with Luminex liquid suspension chip at Wayen Biotechnologies Shanghai, Inc. (China). The Bio-Plex Pro Human Chemokine Panel 40-Plex kit (Cat. #: 171AK99MR2, Bio-Rad, United States) was used following the manufacturer’s instructions.
Statistical Analysis
Data were analyzed by either one-way ANOVA and Dunn’s test or two-tailed Student’s t-test using GraphPad Prism (v7.0). Data are represented as the mean ± s.e.m (standard error of the mean). All in vitro experiments were performed in triplicate. Correlation analyses were evaluated by Spearman. The number of independent samples and statistical methods used in each experiment is reported in the figure legends. P < 0.05 was considered to be statistically significant.
Data Availability Statement
The datasets presented in this study can be found in online repositories. The names of the repository/repositories and accession number(s) can be found below: https://www.biosino.org/node/, OEP002938; https://www.ncbi.nlm.nih.gov/geo/, GSE16879.
Ethics Statement
The studies involving human participants were reviewed and approved by the Institutional Review Board of the Sixth Affiliated Hospital of Sun Yat-sen University. The patients/participants provided their written informed consent to participate in this study.
Author Contributions
LZ and JK conceived and designed the study. XL, YL, SC, YH, YZ, WXW, and JK collected patient samples and clinical data. YL, SC, and YH performed the experiments. YL, XL, LZ, and JK analyzed the data. YL and LZ prepared the manuscript. All authors critically revised the manuscript, had access to the study data, reviewed, and approved the final manuscript.
Funding
This work was supported by the Guangdong Province “Pearl River Talent Plan” Innovation and Entrepreneurship Team Project 2019ZT08Y464 (LZ) and the National Natural Science Foundation of China 81770571 (LZ).
Conflict of Interest
The authors declare that the research was conducted in the absence of any commercial or financial relationships that could be construed as a potential conflict of interest.
Publisher’s Note
All claims expressed in this article are solely those of the authors and do not necessarily represent those of their affiliated organizations, or those of the publisher, the editors and the reviewers. Any product that may be evaluated in this article, or claim that may be made by its manufacturer, is not guaranteed or endorsed by the publisher.
Supplementary Material
The Supplementary Material for this article can be found online at: https://www.frontiersin.org/articles/10.3389/fmicb.2022.926915/full#supplementary-material
Abbreviations
AOM, azoxymethane; ASC, apoptosis-associated speck-like protein containing a CARD domain; CD, Crohn’s disease; DSS, dextran sodium sulfate; GBP, guanylate-binding protein; GSEA, gene set enrichment analysis; IBD, inflammatory bowel diseases; IFN, interferon; IHC, immunohistochemistry; LPS, lipopolysaccharide; NLRP3, NOD-like receptor family pyrin domain containing 3; PBMCs, peripheral blood mononuclear cells; SES-CD, simple endoscopic score for Crohn’s disease; SNP, single-nucleotide polymorphism; TNBS, 2,4,6-trinitrobenzene sulfonic acid; UC, ulcerative colitis; UC-EIS, ulcerative colitis endoscopic index of severity.
Footnotes
- ^ http://www.ncbi.nlm.nih.gov/geo/
- ^ http://www.geneontology.org/
- ^ https://www.kegg.jp/
- ^ https://en.wikipedia.org/wiki/Hypergeometric_distribution
References
Allen, I. C., TeKippe, E. M., Woodford, R. M., Uronis, J. M., Holl, E. K., Rogers, A. B., et al. (2010). The NLRP3 inflammasome functions as a negative regulator of tumorigenesis during colitis-associated cancer. J. Exp. Med. 207, 1045–1056. doi: 10.1084/jem.20100050
Arijs, I., De Hertogh, G., Lemaire, K., Quintens, R., Van Lommel, L., Van Steen, K., et al. (2009). Mucosal gene expression of antimicrobial peptides in inflammatory bowel disease before and after first infliximab treatment. PLoS One 4:e7984. doi: 10.1371/journal.pone.0007984
Autschbach, F., Braunstein, J., Helmke, B., Zuna, I., Schurmann, G., Niemir, Z. I., et al. (1998). In situ expression of interleukin-10 in noninflamed human gut and in inflammatory bowel disease. Am. J. Pathol. 153, 121–130. doi: 10.1016/S0002-9440(10)65552-6
Bauer, C., Duewell, P., Lehr, H. A., Endres, S., and Schnurr, M. (2012). Protective and aggravating effects of Nlrp3 inflammasome activation in IBD models: influence of genetic and environmental factors. Dig. Dis. 30, (Suppl. 1), 82–90. doi: 10.1159/000341681
Bauer, C., Duewell, P., Mayer, C., Lehr, H. A., Fitzgerald, K. A., Dauer, M., et al. (2010). Colitis induced in mice with dextran sulfate sodium (DSS) is mediated by the NLRP3 inflammasome. Gut 59, 1192–1199. doi: 10.1136/gut.2009.197822
Blazejewski, A. J., Thiemann, S., Schenk, A., Pils, M. C., Galvez, E. J. C., Roy, U., et al. (2017). Microbiota normalization reveals that canonical caspase-1 activation exacerbates chemically induced intestinal inflammation. Cell Rep. 19, 2319–2330. doi: 10.1016/j.celrep.2017.05.058
Caradonna, L., Amati, L., Magrone, T., Pellegrino, N. M., Jirillo, E., and Caccavo, D. (2000). Enteric bacteria, lipopolysaccharides and related cytokines in inflammatory bowel disease: biological and clinical significance. J. Endotoxin Res. 6, 205–214. doi: 10.1179/096805100101532063
Cheng, Y. S., Colonno, R. J., and Yin, F. H. (1983). Interferon induction of fibroblast proteins with guanylate binding activity. J. Biol. Chem. 258, 7746–7750. doi: 10.1016/S0021-9258(18)32242-7
Cooper, M. A., Fehniger, T. A., Ponnappan, A., Mehta, V., Wewers, M. D., and Caligiuri, M. A. (2001). Interleukin-1beta costimulates interferon-gamma production by human natural killer cells. Eur. J. Immunol. 31, 792–801. doi: 10.1002/1521-4141(200103)31:3<792::AID-IMMU792>3.0.CO;2-U
Croft, M., and Siegel, R. M. (2017). Beyond TNF: TNF superfamily cytokines as targets for the treatment of rheumatic diseases. Nat. Rev. Rheumatol. 13, 217–233. doi: 10.1038/nrrheum.2017.22
Daperno, M., D’Haens, G., Van Assche, G., Baert, F., Bulois, P., Maunoury, V., et al. (2004). Development and validation of a new, simplified endoscopic activity score for Crohn’s disease: the SES-CD. Gastrointest. Endosc. 60, 505–512. doi: 10.1016/S0016-5107(04)01878-4
de Luca, A., Smeekens, S. P., Casagrande, A., Iannitti, R., Conway, K. L., Gresnigt, M. S., et al. (2014). IL-1 receptor blockade restores autophagy and reduces inflammation in chronic granulomatous disease in mice and in humans. Proc. Natl. Acad. Sci. U.S.A. 111, 3526–3531. doi: 10.1073/pnas.1322831111
Dupaul-Chicoine, J., Yeretssian, G., Doiron, K., Bergstrom, K. S., McIntire, C. R., LeBlanc, P. M., et al. (2010). Control of intestinal homeostasis, colitis, and colitis-associated colorectal cancer by the inflammatory caspases. Immunity 32, 367–378. doi: 10.1016/j.immuni.2010.02.012
Feng, J., Cao, Z., Wang, L., Wan, Y., Peng, N., Wang, Q., et al. (2017). Inducible GBP5 mediates the antiviral response via interferon-related pathways during influenza a virus infection. J. Innate Immun. 9, 419–435. doi: 10.1159/000460294
Fisher, S. A., Mirza, M. M., Onnie, C. M., Soars, D., Lewis, C. M., Prescott, N. J., et al. (2007). Combined evidence from three large British association studies rejects TUCAN/CARD8 as an IBD susceptibility gene. Gastroenterology 132, 2078–2080. doi: 10.1053/j.gastro.2007.03.086
Friedman, K., Brodsky, A. S., Lu, S., Wood, S., Gill, A. J., Lombardo, K., et al. (2016). Medullary carcinoma of the colon: a distinct morphology reveals a distinctive immunoregulatory microenvironment. Mod. Pathol. 29, 528–541. doi: 10.1038/modpathol.2016.54
Graham, D. B., and Xavier, R. J. (2020). Pathway paradigms revealed from the genetics of inflammatory bowel disease. Nature 578, 527–539. doi: 10.1038/s41586-020-2025-2
Haque, M., Singh, A. K., Ouseph, M. M., and Ahmed, S. (2021). Regulation of synovial inflammation and tissue destruction by guanylate binding protein 5 in synovial fibroblasts from patients with rheumatoid arthritis and rats with adjuvant-induced arthritis. Arthritis Rheumatol. 73, 943–954. doi: 10.1002/art.41611
Hengartner, N. E., Fiedler, J., Schrezenmeier, H., Huber-Lang, M., and Brenner, R. E. (2015). Crucial role of IL1beta and C3a in the in vitro-response of multipotent mesenchymal stromal cells to inflammatory mediators of polytrauma. PLoS One 10:e0116772. doi: 10.1371/journal.pone.0116772
Hirota, S. A., Ng, J., Lueng, A., Khajah, M., Parhar, K., Li, Y., et al. (2011). NLRP3 inflammasome plays a key role in the regulation of intestinal homeostasis. Inflamm. Bowel Dis. 17, 1359–1372. doi: 10.1002/ibd.21478
Kanai, T., Watanabe, M., Okazawa, A., Nakamaru, K., Okamoto, M., Naganuma, M., et al. (2000). Interleukin 18 is a potent proliferative factor for intestinal mucosal lymphocytes in Crohn’s disease. Gastroenterology 119, 1514–1523. doi: 10.1053/gast.2000.20260
Krapp, C., Hotter, D., Gawanbacht, A., McLaren, P. J., Kluge, S. F., Sturzel, C. M., et al. (2016). Guanylate Binding Protein (GBP) 5 is an interferon-inducible inhibitor of HIV-1 infectivity. Cell Host Microbe 19, 504–514. doi: 10.1016/j.chom.2016.02.019
Li, Z., Qu, X., Liu, X., Huan, C., Wang, H., Zhao, Z., et al. (2020). GBP5 is an interferon-induced inhibitor of respiratory syncytial virus. J. Virol. 94, e01407-20. doi: 10.1128/JVI.01407-20
Ligumsky, M., Simon, P. L., Karmeli, F., and Rachmilewitz, D. (1990). Role of interleukin 1 in inflammatory bowel disease–enhanced production during active disease. Gut 31, 686–689. doi: 10.1136/gut.31.6.686
Mahida, Y. R., Wu, K., and Jewell, D. P. (1989). Enhanced production of interleukin 1-beta by mononuclear cells isolated from mucosa with active ulcerative colitis of Crohn’s disease. Gut 30, 835–838. doi: 10.1136/gut.30.6.835
Mao, L., Kitani, A., Similuk, M., Oler, A. J., Albenberg, L., Kelsen, J., et al. (2018). Loss-of-function CARD8 mutation causes NLRP3 inflammasome activation and Crohn’s disease. J. Clin. Investig. 128, 1793–1806. doi: 10.1172/JCI98642
Matta, S. K., Patten, K., Wang, Q., Kim, B. H., MacMicking, J. D., and Sibley, L. D. (2018). NADPH oxidase and guanylate binding protein 5 restrict survival of avirulent type III strains of Toxoplasma gondii in naive macrophages. mBio 9, e01393-18. doi: 10.1128/mBio.01393-18
McAlindon, M. E., Hawkey, C. J., and Mahida, Y. R. (1998). Expression of interleukin 1 beta and interleukin 1 beta converting enzyme by intestinal macrophages in health and inflammatory bowel disease. Gut 42, 214–219. doi: 10.1136/gut.42.2.214
Meunier, E., Dick, M. S., Dreier, R. F., Schurmann, N., Kenzelmann Broz, D., Warming, S., et al. (2014). Caspase-11 activation requires lysis of pathogen-containing vacuoles by IFN-induced GTPases. Nature 509, 366–370. doi: 10.1038/nature13157
Meunier, E., Wallet, P., Dreier, R. F., Costanzo, S., Anton, L., Ruhl, S., et al. (2015). Guanylate-binding proteins promote activation of the AIM2 inflammasome during infection with Francisella novicida. Nat. Immunol. 16, 476–484. doi: 10.1038/ni.3119
Neudecker, V., Haneklaus, M., Jensen, O., Khailova, L., Masterson, J. C., Tye, H., et al. (2017). Myeloid-derived miR-223 regulates intestinal inflammation via repression of the NLRP3 inflammasome. J. Exp. Med. 214, 1737–1752. doi: 10.1084/jem.20160462
Palmela, C., Chevarin, C., Xu, Z., Torres, J., Sevrin, G., Hirten, R., et al. (2018). Adherent-invasive Escherichia coli in inflammatory bowel disease. Gut 67, 574–587. doi: 10.1136/gutjnl-2017-314903
Patil, P. A., Blakely, A. M., Lombardo, K. A., Machan, J. T., Miner, T. J., Wang, L. J., et al. (2018). Expression of PD-L1, indoleamine 2,3-dioxygenase and the immune microenvironment in gastric adenocarcinoma. Histopathology 73, 124–136. doi: 10.1111/his.13504
Pilla, D. M., Hagar, J. A., Haldar, A. K., Mason, A. K., Degrandi, D., Pfeffer, K., et al. (2014). Guanylate binding proteins promote caspase-11-dependent pyroptosis in response to cytoplasmic LPS. Proc. Natl. Acad. Sci. U.S.A. 111, 6046–6051. doi: 10.1073/pnas.1321700111
Reinecker, H. C., Steffen, M., Witthoeft, T., Pflueger, I., Schreiber, S., MacDermott, R. P., et al. (1993). Enhanced secretion of tumour necrosis factor-alpha, IL-6, and IL-1 beta by isolated lamina propria mononuclear cells from patients with ulcerative colitis and Crohn’s disease. Clin. Exp. Immunol. 94, 174–181. doi: 10.1111/j.1365-2249.1993.tb05997.x
Santos, J. C., Dick, M. S., Lagrange, B., Degrandi, D., Pfeffer, K., Yamamoto, M., et al. (2018). LPS targets host guanylate-binding proteins to the bacterial outer membrane for non-canonical inflammasome activation. EMBO J. 37:e98089. doi: 10.15252/embj.201798089
Seo, S. U., Kamada, N., Munoz-Planillo, R., Kim, Y. G., Kim, D., Koizumi, Y., et al. (2015). Distinct commensals induce interleukin-1beta via NLRP3 inflammasome in inflammatory monocytes to promote intestinal inflammation in response to injury. Immunity 42, 744–755. doi: 10.1016/j.immuni.2015.03.004
Seyed Tabib, N. S., Madgwick, M., Sudhakar, P., Verstockt, B., Korcsmaros, T., and Vermeire, S. (2020). Big data in IBD: big progress for clinical practice. Gut 69, 1520–1532. doi: 10.1136/gutjnl-2019-320065
Shenoy, A. R., Wellington, D. A., Kumar, P., Kassa, H., Booth, C. J., Cresswell, P., et al. (2012). GBP5 promotes NLRP3 inflammasome assembly and immunity in mammals. Science 336, 481–485. doi: 10.1126/science.1217141
Siegmund, B., Lehr, H. A., Fantuzzi, G., and Dinarello, C. A. (2001). IL-1 beta -converting enzyme (caspase-1) in intestinal inflammation. Proc. Natl. Acad. Sci. U.S.A. 98, 13249–13254. doi: 10.1073/pnas.231473998
Tourkochristou, E., Aggeletopoulou, I., Konstantakis, C., and Triantos, C. (2019). Role of NLRP3 inflammasome in inflammatory bowel diseases. World J. Gastroenterol. 25, 4796–4804. doi: 10.3748/wjg.v25.i33.4796
Travis, S. P., Schnell, D., Krzeski, P., Abreu, M. T., Altman, D. G., Colombel, J. F., et al. (2013). Reliability and initial validation of the ulcerative colitis endoscopic index of severity. Gastroenterology 145, 987–995. doi: 10.1053/j.gastro.2013.07.024
Yang, L., Tang, S., Baker, S. S., Arijs, I., Liu, W., Alkhouri, R., et al. (2019). Difference in pathomechanism between crohn’s disease and ulcerative colitis revealed by colon transcriptome. Inflamm. Bowel Dis. 25, 722–731. doi: 10.1093/ibd/izy359
Yang, S. K., Kim, H., Hong, M., Lim, J., Choi, E., Ye, B. D., et al. (2011). Association of CARD8 with inflammatory bowel disease in Koreans. J. Hum. Genet. 56, 217–223. doi: 10.1038/jhg.2010.170
Yao, X., Zhang, C., Xing, Y., Xue, G., Zhang, Q., Pan, F., et al. (2017). Remodelling of the gut microbiota by hyperactive NLRP3 induces regulatory T cells to maintain homeostasis. Nat. Commun. 8:1896. doi: 10.1038/s41467-017-01917-2
Youm, Y. H., Nguyen, K. Y., Grant, R. W., Goldberg, E. L., Bodogai, M., Kim, D., et al. (2015). The ketone metabolite beta-hydroxybutyrate blocks NLRP3 inflammasome-mediated inflammatory disease. Nat. Med. 21, 263–269. doi: 10.1038/nm.3804
Yu, X., Jin, J., Zheng, Y., Zhu, H., Xu, H., Ma, J., et al. (2021). GBP5 drives malignancy of glioblastoma via the Src/ERK1/2/MMP3 pathway. Cell Death Dis. 12:203. doi: 10.1038/s41419-021-03492-3
Yuan, Y. Y., Xie, K. X., Wang, S. L., and Yuan, L. W. (2018). Inflammatory caspase-related pyroptosis: mechanism, regulation and therapeutic potential for inflammatory bowel disease. Gastroenterol. Rep. 6, 167–176. doi: 10.1093/gastro/goy011
Zaki, M. H., Boyd, K. L., Vogel, P., Kastan, M. B., Lamkanfi, M., and Kanneganti, T. D. (2010a). The NLRP3 inflammasome protects against loss of epithelial integrity and mortality during experimental colitis. Immunity 32, 379–391. doi: 10.1016/j.immuni.2010.03.003
Keywords: Crohn’s disease, ulcerative colitis, inflammasome, pyroptosis, guanylate binding protein
Citation: Li Y, Lin X, Wang W, Wang W, Cheng S, Huang Y, Zou Y, Ke J and Zhu L (2022) The Proinflammatory Role of Guanylate-Binding Protein 5 in Inflammatory Bowel Diseases. Front. Microbiol. 13:926915. doi: 10.3389/fmicb.2022.926915
Received: 23 April 2022; Accepted: 09 May 2022;
Published: 02 June 2022.
Edited by:
Na Li, Hainan Medical University, ChinaReviewed by:
Haiyan Song, Shanghai University of Traditional Chinese Medicine, ChinaJian Lin, Affiliated Hospital of Putian University, China
Ioanna Aggeletopoulou, University of Patras, Greece
Copyright © 2022 Li, Lin, Wang, Wang, Cheng, Huang, Zou, Ke and Zhu. This is an open-access article distributed under the terms of the Creative Commons Attribution License (CC BY). The use, distribution or reproduction in other forums is permitted, provided the original author(s) and the copyright owner(s) are credited and that the original publication in this journal is cited, in accordance with accepted academic practice. No use, distribution or reproduction is permitted which does not comply with these terms.
*Correspondence: Jia Ke, a2ppYUBtYWlsLnN5c3UuZWR1LmNu; Lixin Zhu, emh1bHg2QG1haWwuc3lzdS5lZHUuY24=
†These authors have contributed equally to this work and share first authorship
‡These authors share last authorship