- 1Department of Pediatrics, Peking University Third Hospital, Beijing, China
- 2School of Public Health, Peking University Health Science Center, Beijing, China
- 3National Engineering Center of Dairy for Maternal and Child Health, Beijing Sanyuan Foods Co., Ltd., Beijing, China
The gut microbiota plays a key role in the pathogenesis of diseases affecting preterm infants and gestational age is one of the important factors which affect the gut microbiota of infants. To determine the characteristics of the gut microbiota in preterm infants of different gestational ages from birth to 1 year after birth, we collected 622 fecal samples from neonates of different gestational ages at different time points after birth. According to the gestational ages, the samples were divided into four groups, extremely preterm, very preterm, moderate to late preterm, and term group. Meconium and fecal samples at day 14, 28, 120, and 365 after birth were collected. 16S rRNA sequencing was performed and the composition and structure of the gut microbiota in preterm infants of different gestational age was compared with that of term infants. In our study, alpha diversity of meconium in extremely preterm group was higher than very preterm group, moderate to late preterm group and term group and alpha diversity of meconium in preterm group was decreased with increasing of gestational age. At day 14 to day 120 after birth, alpha diversity of term and moderate to late preterm group were significantly higher than other two preterm groups. However, moderate to late preterm group owned the highest alpha diversity which was higher than term group at day 365 after birth. Besides, the results shown the duration of opportunistic pathogen such as Klebsiella and Enterococcus which dominant colonization was different in different gestational age groups. As well as the probiotics, such as Bifidobacterium, which abundance enriched at different time point in different gestational age groups. We profiled the features of dynamic changes of gut microbiome from different gestational ages infants. The results of our research provide new insights for individualized interventions of specific microbes of preterm infants with different gestational ages at different time points after birth.
Introduction
Preterm births are a major public health issue. A study (Deng et al., 2021) used national monitoring data from China’s National Maternal Near Miss Surveillance System (NMNMSS) to estimate the preterm birth rate and trends between 2012 and 2018 in China. The results showed that among the babies of 9 million women with at least one live baby, 6.1% were preterm and the overall preterm birth rate increased from 5.9% in 2012 to 6.4% in 2018. Recently, with the development of medical technology the survival rate of some preterm infants has increased. From 1990 to 2018, the global annual neonatal mortality rate has decreased by 51% (Yu et al., 2021). Some extremely preterm infants [gestational age (GA) < 28 weeks] receive good medical treatment and grow up. However, preterm neonates still face more challenges than term infants because of their immaturity, which may confer an increased risk of morbidity and mortality and lead to diseases, such as necrotizing enterocolitis (NEC) with a mortality rate of 10–30% (Hui et al., 2021), sepsis (El Manouni El Hassani et al., 2021) etc. (Lee et al., 2020). Lots of studies have indicated that many preterm infant diseases are highly associated with the gut microbiota. Stewart et al. (2013) reported that babies who developed NEC owned lower diversity of gut microbiota. Olm et al. (2019) performed metagenomic analysis of fecal samples from premature infants and found replication of all bacteria were significantly higher 2 days before NEC diagnosis, meanwhile samples collected before NEC diagnosis harbored significantly more Klebsiella. For late-onset neonatal sepsis (LONS), pathogens isolated in cases of LONS are usually existed in the gut microbiota (Stewart et al., 2017). Beghetti et al. (2022) suggested that early Bifidobacterium deficiency seems to be a negative biomarker of adverse neurological outcomes in very low birth weight infants through a pilot observational study.
Gestational age is one of factors which influence the gut microbiota in infants (Aguilar-Lopez et al., 2021). Compared with term infants, preterm infants have altered gut microbiota. Studies have shown that the meconium of preterm infants has lower diversity, reduced number of Bifidobacteria, and increased number of Enterobacter, Enterococcus, and Staphylococcus, compared with that of term infants (Korpela et al., 2018). Although numerous studies indicate that intestinal bacteria play an essential role in the pathogenesis of NEC, early onset neonatal sepsis (EONS), and LONS, no single bacterial species has been consistently identified as the causative agent. Several microorganisms have been implicated in NEC, primarily from the phylum Firmicutes and Proteobacteria. Specifically, an increased abundance of Clostridium spp. and associated toxins have been observed in the stools of neonates with NEC (Lee et al., 2020). However, few studies have investigated the dynamic changes in the gut microbiota of preterm infants for over half a year.
In this study, we collected meconium and fecal samples from preterm infants with different GA and term infants from the neonatal period until 1 year after birth, and analyzed the microbiota composition using 16S rRNA sequencing. Subsequently, we compared the gut microbiota composition of different GA preterm infants with that of term infants at different time points after birth. Finally, we profiled the dynamic changes in the gut microbiota of preterm infants between different GA and different ages after birth.
Materials and Methods
Study Cohort
Informed consent was obtained from the parents of the enrolled newborns. The study was conducted in accordance with the Declaration of Helsinki, and the protocol was approved by the Ethics Committee of Peking University Third Hospital (No. M2017183).
Term infants were recruited at the Department of Obstetrics of Peking University Third Hospital as controls. Preterm infants (GA: 24–37 weeks) were recruited at the neonatal intensive care unit (NICU) of the Department of Pediatrics of Peking University Third Hospital.
Eligibility criteria included women aged 18 years and older with the intention to breastfeed for at least fourth months postpartum. Women who delivered infants with congenital malformations, genetic diseases, or those who required surgery were excluded. Participants were grouped into four groups according to their infants’ GA at birth: extremely preterm (< 28 weeks; EP), very preterm (28–32 weeks; VP), moderate to late preterm (32–37 weeks; MP), and full-term (37–42 weeks, T). The study flowchart is depicted in Figure 1.
Baseline information of both the mothers and infants, which included maternal factors (age, weight, height, and mode of delivery) and the infants’ gestational age, birth weight, length, head circumference, Apgar score at 1 min and 5 min after birth, and disease, was collected through the electronic medical record system.
Sample Collection
Meconium samples were collected the of every neonate during first 2–3 days, for some preterm babies, within 1 week after birth. And other fecal samples were collected at days 14, 28, 120, and 1 year after birth. Using a collection tube containing 8 mL of DNA stabilizer reagent (PSP Spin Stool DNA Plus Kit, STRATEC Biomedical AG, Birkenfeld, Germany). The fecal samples of preterm infants were obtained as part of routine care in the NICU by nurses and stored at −80°C. At home, fecal samples were collected by the parents of the infants. Home-collected samples were immediately stored at −20°C and delivered to the hospital on ice bags or dry ice and stored at −80°C.
16S rRNA Sequencing
DNA was extracted from each fecal sample using QIAamp Fast DNA Stool Mini Kit (Qiagen, Germany), following the manufacturer’s protocol, which included preceding bead beating. The V3–V4 hypervariable region of the 16S rDNA gene was amplified using the universal primer pair 341F (5′-CCTACGGGAGGCAGCAG-3′) and 806R (5′-GGACTACHVGGGTWTCTAAT-3′). The PCR amplification conditions were as follows: initial denaturation at 98°C for 1 min, followed by 30 cycles at 98°C for 10 s, 50°C for 30 s, and 72°C for 30 s, and a final extension step at 72°C for 5 min. The PCR products (approximately 200 bp) were separated by electrophoresis in agarose gels (2%, w/v), purified with Qiagen Gel Extraction Kit (Qiagen, Germany), and then pooled at equal concentrations. Pyrosequencing was conducted on an Illumina Hiseq 2500 (Illumina, United States).
Sequence Data Processing and Statistics
All paired-end reads were assigned to each sample based on their unique barcodes and then merged using Fast Length Adjustment of Short reads (FLASH) software. Merged sequences of poor quality were identified and removed by Quantitative Insights into Microbial Ecology (QIIME2) quality filters under specific filtering conditions (Q-score ≥ 25). Chimera removal and dereplication of the reads were performed using USEARCH. Unique sequences in the high-quality merged sequences of each sample were assigned to the same operational taxonomic units (OTUs) at an identity threshold of 97% similarity. A representative sequence was screened for each OTU and used to assign taxonomic composition by the classifier approach in the Ribosomal Database Project database (Wang et al., 2007; Cole et al., 2009).
All statistical analyses were performed using R version 4.0.3. Alpha-diversity (Shannon index) based on the genus level abundance profile was calculated using the Vegan package and compared by using Wilcoxon rank-sum test. Anosim analysis of beta-diversity was calculated based on unweighted unifrac distances using the Vegan package, and principal co-ordinates analysis (PCoA) plot were performed using the ggplot2 package. Linear discriminant analysis (LDA) was applied to determine the most discriminant taxa between the groups, and the size effect cutoff was 2.0. In order to compare the microbiota community structure across four groups, Kruskal–Wallis test was used, and p-values were corrected for multiple testing using the Benjamin and Hochberg method.
Clinical Data Analysis
EpiData 3.1 was used for parallel double entry of demographic and clinical data. Baseline characteristics of the mothers and infants are presented as the mean ± SD for continuous variables and frequencies for categorical variables. A p-value of < 0.05 was considered statistically significant.
Results
Demographic and Clinical Information
We included 116 preterm (27 EP, 48 VP, and 41 MP) and 50 term infants in this study. The study flowchart is depicted in Figure 1. We analyzed the basic characteristics of infants and their mothers (Table 1) and found that gestational weight gain, conception method (spontaneous), delivery mode (C-section), multiple births (singleton), mother’s high school education or less, hypertension, and pre-eclampsia during pregnancy were significantly different among the four groups (all P < 0.05). Because of the different GA at delivery, the birth weight, birth length, head circumference, and Apgar score of the neonates in the four groups at 1 min and 5 min after birth were markedly different (all P < 0.001).
Among preterm infants, the number of infants with neonatal respiratory distress syndrome (NRDS), pneumonia, periventricular leukomalacia (PVL), patent ductus arteriosus (PDA), and bronchopulmonary dysplasia (BPD) were remarkably different, and the shorter the GA, the higher the number of newborns with NRDS, pneumonia, and BPD. The number of infants with sepsis and NEC was not significantly different among preterm infants.
For preterm infants, especially preterm infants in NICU, we always use amoxicillin clavulanate potassium, cefoperazone sulbactam, ceftazidime, meropenem, vancomycin for treatment according to the clinical infect manifestations and biochemical tests. Because different infants used different antibiotics for different durations and one infant could use two antibiotics simultaneously, we did not perform the statistics on antibiotics using.
We collected the feeding pattern through medical records and questionnaires, furthermore analyzed the feeding pattern of each group (Table 2).
Structure of the Gut Microbiota in Preterm Infants With Different Gestational Age
We collected fecal samples at each time point from as many infants as possible, and obtained a total of 622 samples. Excluding samples with poor sequencing quality, 617 samples (Table 3) were available for sequencing and finally we got 616 sequencing data. A comparison of the results of the gut microbiota composition in preterm infants with different GA was conducted at different days after birth.
Meconium
The three most abundant bacteria at the phylum level in each group were Proteobacteria, Firmicutes, and Bacteroidetes (Figure 2A). Although Proteobacteria was both 54% and Firmicutes was nearly 28–30% in meconium of EP and T group, the abundance of the three phyla were significantly different among four groups (Supplementary Table 1). The genera varied greatly among the groups. The top three most abundant genera in the EP group were Klebsiella (14.5%), Staphylococcus (11.9%), and Sphingomonas (8.7%), those in the VP group were Klebsiella (37.8%), Sphingomonas (8.3%), and Enterococcus (6.0%), those in the MP group were Klebsiella (53.5%), Escherichia/Shigella (6.5%) and Clostridium (4.3%), and those in the T group were Escherichia/Shigella (15.5%), Klebsiella (12.1%), and Clostridium XI (9.4%) (Figure 2B). Except Enterococcus and Escherichia/Shigella, the left genera were significantly different (Supplementary Table 1). Compared with the EP group, the Shannon index of alpha diversity was completely different in the other preterm and term groups (Figure 2C). The Shannon index of EP was higher than other preterm infant groups and term group. The index of analysis of similarities (ANOSIM) that reflected the beta diversity (R = 0.072, P < 0.05; Figure 2D), indicated that the four groups exhibited significantly different structures of meconium microbiota. Klebsiella was nearly about 12–15% in EP and T group lower than VP (38%) group and MP group (53%). Klebsiella was the main genus in the meconium of preterm infants. The main genus contributing to the T group was completely different from that of the preterm infant groups. LDA showed that the communities that had a significant impact on the group division were Bacillus (order), Staphylococcaceae (family), and Staphylococcus in the EP group, Xanthomonadales (order), Xanthomonadaceae (family), and Stenotrophomonas in the VP group, Gammaproteobacteria (class), Klebsiella, and Enterobacteriaceae (family) in the MP group, and Bacteroidetes, Bacteroidia (class), and Bacteroidales (order) in the T group (Supplementary Figure 1).
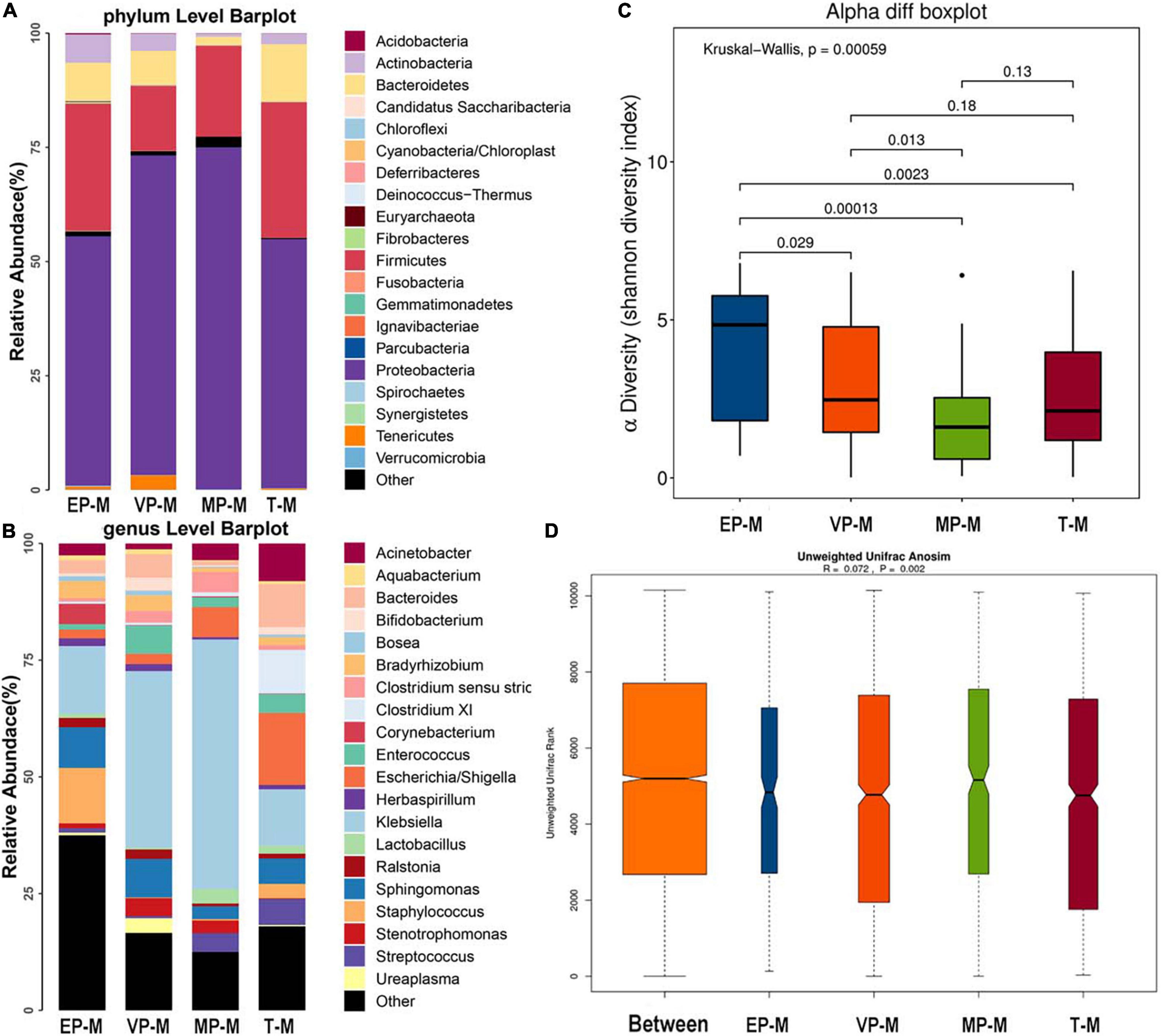
Figure 2. The composition of gut microbiota from neonates with different gestational ages at first day after birth. EP, extremely preterm (<28 weeks); VP, very preterm (28–32 weeks); MP, moderate to late preterm (32–37 weeks); T, full-term (37–42 weeks). (A) Comparison of abundance at phylum level. The abscissa is the group name, and the ordinate is the relative abundance of species. Different colors correspond to different species names, and the length of the color block indicates the relative abundance of the species represented by the color block. (B) Comparison of abundance at genus level. The abscissa is the group name, and the ordinate is the relative abundance of species. Different colors correspond to different species names, and the length of the color block indicates the relative abundance of the species represented by the color block. (C) Shannon diversity index of α diversity. The abscissa represents the groups, and the ordinate represents the Alpha diversity index value. (D) Anosim analysis of β diversity. The abscissa represents all samples (Between) and each group, and the ordinate represents the rank of the unifrac distance. When the rank of the Between group is high relative to each of the other groups, it indicates that the between-group difference is greater than the within-group difference. R is between (−1, 1), R > 0, indicating that the difference between groups is greater than the difference within the group; R < 0, indicating that the difference within the group is greater than the difference between the groups, the reliability of statistical analysis is represented by P, P < 0.05 indicates statistical significance.
Day 14 After Birth
The fecal microbiota composition of the preterm and term groups at the phylum and genus levels at day 14 after birth is shown in Figure 3. The gut microbiota composition at 14 days after birth was similar to that meconium in the EP group at the phylum level, because of the three most abundant phyla being Proteobacteria, Firmicutes, and Bacteroidetes. The third most abundant phylum in the VP and MP groups changed to Actinobacteria, while in the T group, the three most abundant bacteria at the phylum level were Firmicutes, Proteobacteria, and Actinobacteria (Figure 3A) and all above phyla were significantly different (Supplementary Table 2). At the genus level, the top three most abundant genera in the EP group were Klebsiella (62.5%), Enterococcus (7.2%), and Staphylococcus (5.9%), and those in the VP group were Klebsiella (70.8%), Enterococcus (8.1%), and Bifidobacterium (6.4%). Those in the MP group were Klebsiella (35.0%), Bifidobacterium (23.8%) and Escherichia/Shigella (8.6%), and those in the T group included Bifidobacterium (20.0%), Streptococcus (13.7%), and Klebsiella (12.5%). Also, except Enterococcus and Escherichia/Shigella, the left genera were significantly different (Supplementary Table 2). The abundance of Bifidobacterium in the VP, MP, and T groups gradually increased at 14 days after birth, while the abundance of Bifidobacterium in the EP group was still lower; however, the main genus in preterm groups was Klebsiella (Figure 3B). The Shannon index of alpha diversity (Figure 3C) showed no significant difference between the EP and VP groups, MP and T groups, the rest were significantly different. The ANOSIM index, reflecting beta diversity (showed R = 0.122 and P = 0.001) (Figure 3D), indicated that the four groups exhibited marked differences. The communities that mainly contributed to the T group were Clostridiales (order), Clostridia (class), and Streptococcaceae (family), while those in the MP group were Bifidobacterium, Bifidobacteriales (order), and Bifidobacteriaceae (family). Those in the VP group were Klebsiella, Gammaproteobacteria (class), and Enterobacteriaceae (family), and those in the EP group were Bacillales (order), Staphylococcus, and Staphylococcaceae (family) (Supplementary Figure 2).
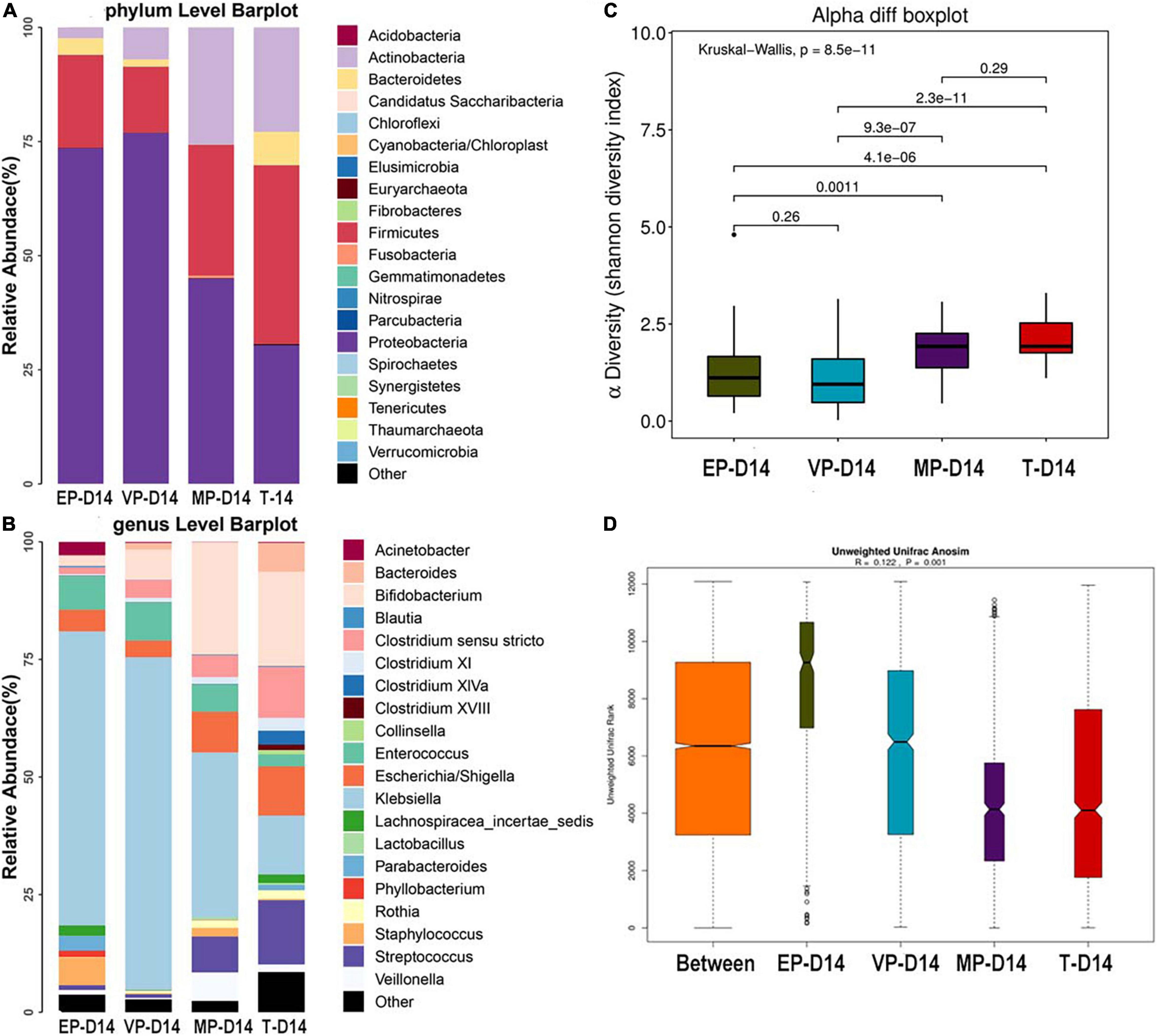
Figure 3. The composition of gut microbiota from neonates with different gestational ages at 14 days after birth. EP, extremely preterm; VP, very preterm; MP, moderate to late preterm; T, full-term. (A) Comparison of abundance at phylum level. (B) Comparison of abundance at genus level. (C) Shannon diversity index of α diversity. (D) Anosim analysis of β diversity.
Day 28 After Birth
At 28 days after birth, the top three most abundant phyla in the EP and VP groups were Proteobacteria, Firmicutes, and Actinobacteria, which the gut microbiota composition was similar to that at day 14 in the VP and MP group at the phylum level. Those in the MP group were Proteobacteria, Actinobacteria, and Firmicutes. Those in the T group were Firmicutes, followed by Proteobacteria and Actinobacteria (Figure 4A). However, the abundance of above phyla among four groups were totally different (Supplementary Table 3). At the genus level, the top three most abundant genera in the EP group were Klebsiella (64.0%), Enterococcus (11.9%), and Escherichia/Shigella (6.5%). Those in the VP group were Klebsiella (48.7%), Bifidobacterium (13.6%), and Veillonella (9.4%), and those in the MP group were Bifidobacterium (32.1%), Escherichia/Shigella (16.5%), and Klebsiella (15.9%). Those in the T group were Bifidobacterium (24.8%), Clostridium (15.1%), and Klebsiella (13.0%). Except Escherichia/Shigella the left genera were significantly different (Supplementary Table 3). Klebsiella was still the main genus of the gut microbiota in the preterm groups. While the abundance of Bifidobacterium in the EP group was still low at 28 days after birth (Figure 4B). However, Bifidobacterium gradually enriched in VP, MP, and T group at 28 days after birth. There was no significant difference in alpha diversity between the MP and T group (Figure 4C). Beta diversity (Figure 4D), with R = 0.13 and P = 0.001, significantly differed among the groups. Clostridiales (order), Clostridia (class), and Firmicutes were the main contributors in the T group. Those in the MP group were Actinobacteria (class), Actinobacteria and Bifidobacterium (order), and those in the EP group were Klebsiella, Proteobacteria, and Gammaproteobacteria (class) (Figure 4E).
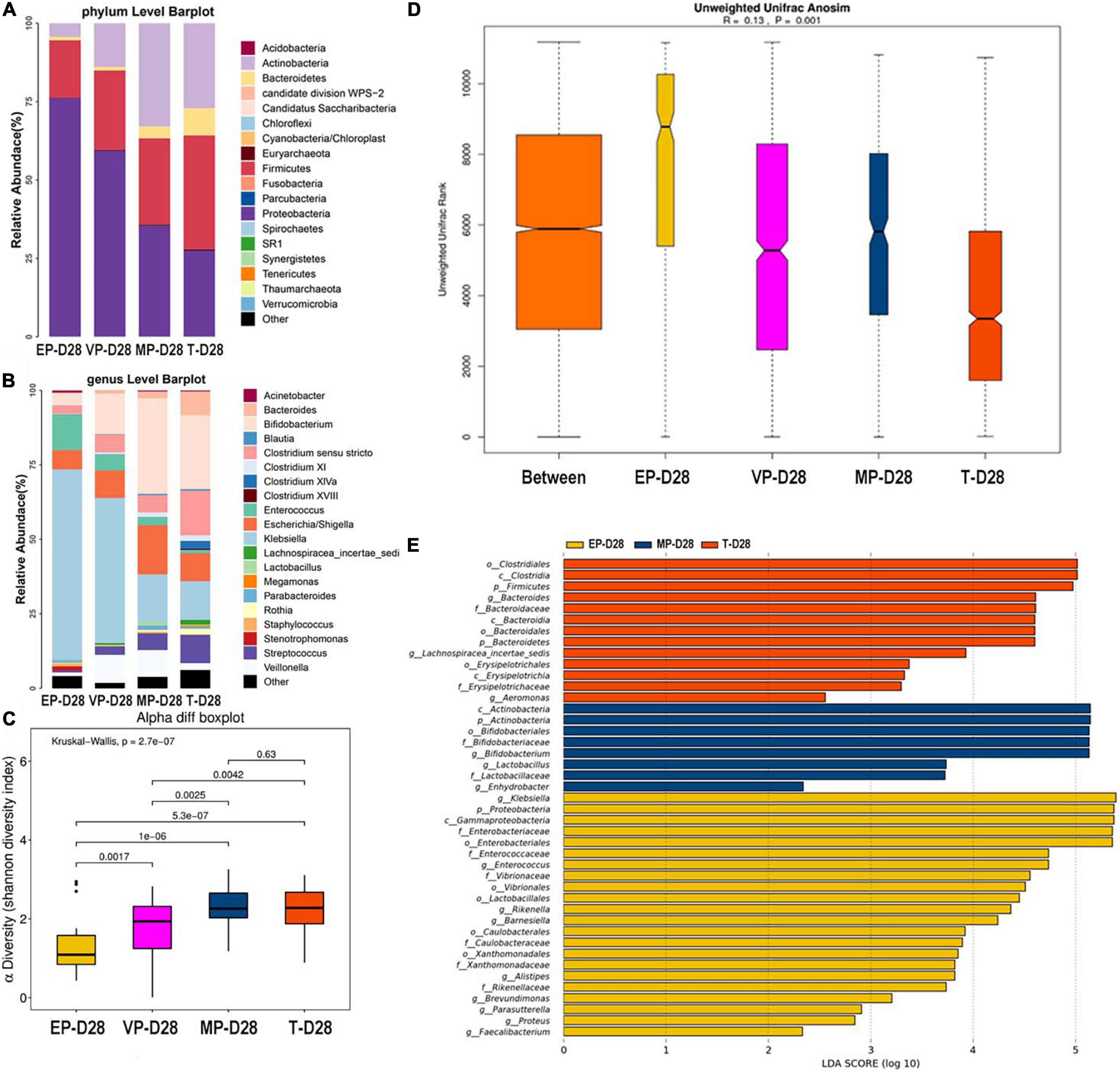
Figure 4. The composition of gut microbiota from neonates with different gestational ages at 28 days after birth. EP, extremely preterm; VP, very preterm; MP, moderate to late preterm; T, full-term. (A) Comparison of abundance at phylum level. (B) Comparison of abundance at genus level. (C) Shannon diversity index of α diversity. (D) Anosim analysis of β diversity. (E) Communities or species that have significantly different effects for each group. The abscissa represents LDA score. LDA score obtained by linear regression analysis of microbial groups with significant effects in different groups.
Day 120 After Birth
The top three most abundant phyla of the four groups were Actinobacteria, Firmicutes, and Proteobacteria at 120 days after birth (Figure 5A). The abundance of Actinobacteria of four groups were significantly different (Supplementary Table 4). At the genus level, the top three most abundant genera of the EP and MP groups were Bifidobacterium (43.8, 39.8%), Escherichia/Shigella (10.54, 11.9%), and Veillonella (10.45, 10.6%). Those of the VP group were Bifidobacterium (40.6%), Escherichia/Shigella (12.8%), and Enterococcus (6.3%) and those for the T group were Bifidobacterium (33.9%), Escherichia/Shigella (10.6%), and Bacteroides (8.5%) (Figure 5B). The abundance of Enterococcus of four groups were significantly different, the abundance of Bifidobacterium of four groups were shown no markedly different at day 120 (Supplementary Table 4). The abundance of Bifidobacterium in the EP group increased from 28 days after birth to 120 days after birth, and increased in other groups too. There was no significant difference among the four groups in alpha diversity as shown in Figure 5C. Beta diversity was R = 0.164, P = 0.001 (Figure 5D), and the difference among groups was also significant. The communities that mainly contributed to the T group were Clostridia (class), Clostridiales (order), and Lachnospiraceae (family), and those for the MP group were Lactobacillaceae (family), Lactobacillus, and Gemmiger and those for the VP group were Enterococcus, Enterococcaceae (family), and Megasphaera. Those for the EP group were Parabacteroides, Olsenella, and Clostridiales (family) (Supplementary Figure 3).
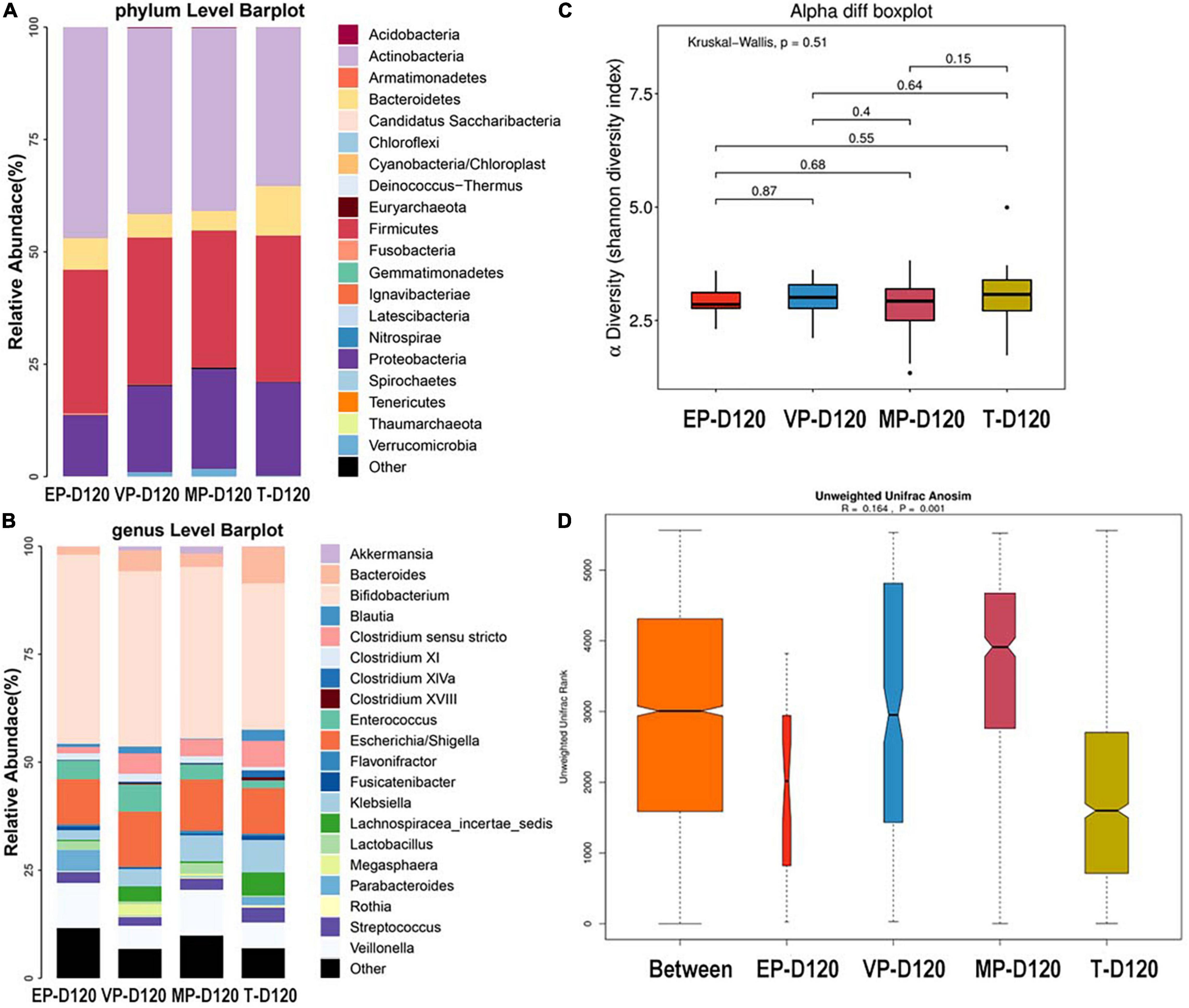
Figure 5. The composition of gut microbiota from neonates with different gestational ages at 120 days after birth. EP, extremely preterm; VP, very preterm; MP, moderate to late preterm; T, full-term. (A) Comparison of abundance at phylum level. (B) Comparison of abundance at genus level. (C) Shannon diversity index of α diversity. (D) Anosim analysis of β diversity.
Day 365 After Birth
At 365 days after birth, the top three most abundant phyla of the EP group were Actinobacteria, Firmicutes, and Bacteroidetes. Those for the VP and MP groups were Firmicutes, Actinobacteria, and Bacteroidetes, and those for the T group were Firmicutes, Bacteroidetes, and Actinobacteria (Figure 6A). Firmicutes and Actinobacteria shown significantly different (Supplementary Table 5). At the genus level, the top three most abundant genera of the EP group were Bifidobacterium (38.1%), Enterococcus (7.9%), and Bacteroides (7.8%), and those for the VP group were Bifidobacterium (19.7%), Bacteroides (18.2%), and Lachnospiracea (9.5%). Those for the MP group were Bifidobacterium (15.5%), Lachnospiracea (12.1%), and Faecalibacterium (10.3%), and those for the T group were Bacteroides (21.3%), Bifidobacterium (16.7%), and Lachnospiracea (13.4%) (Figure 6B). Except Lachnospiracea, the left genera above were significantly different (Supplementary Table 5). The alpha diversity is shown in Figure 6C, there was no significant difference between the EP and T groups, while the other groups showed significant differences and the Shannon index of MP was significantly higher than other three groups. Beta diversity with R = 0.223 and P = 0.001 (Figure 6D), indicated significant differences among groups. The communities that mainly contributed to the T group were Bacteroides, Bacteroidaceae (family), and Clostridium XIVa, while those in the MP group were Clostridia (class), Clostridiales (order), and Firmicutes. Those in the VP group were Fusobacterium, Fusobacteria, and Fusobacteriia (class), while those in the EP group were Actinobacteria (class), Actinobacteria, and Bifidobacteriales (order) (Figure 6E).
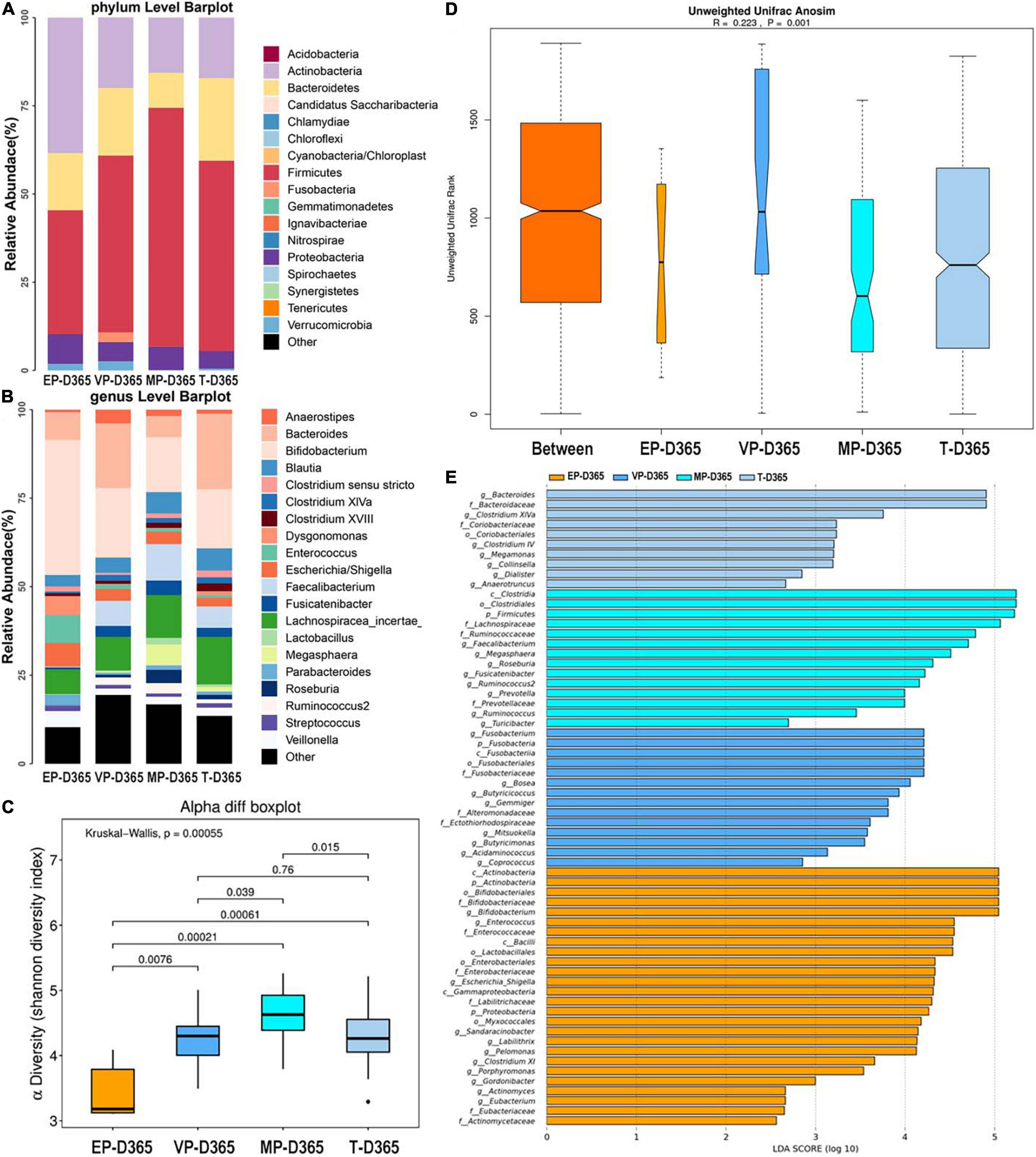
Figure 6. The composition of gut microbiota from neonates with different gestational ages at 365 days after birth. EP, extremely preterm; VP, very preterm; MP, moderate to late preterm; T, full-term. (A) Comparison of abundance at phylum level. (B) Comparison of abundance at genus level. (C) Shannon diversity index of α diversity. (D) Anosim analysis of β diversity. (E) Communities or species that have significantly different effects for infants with different gestational ages at 365 days after birth.
Principal Co-ordinates Analysis
All samples were combined, and PCoA was carried out. PCoA preliminarily reflects the distribution of different samples, which may show dispersion and aggregation. The results revealed that the dispersion of extremely preterm infants and term infants was similar in meconium, but at day 365 was completely different (Figure 7A). There was resemblance in the dispersion of groups VP and MP at days 14, 28, 120, and 365.
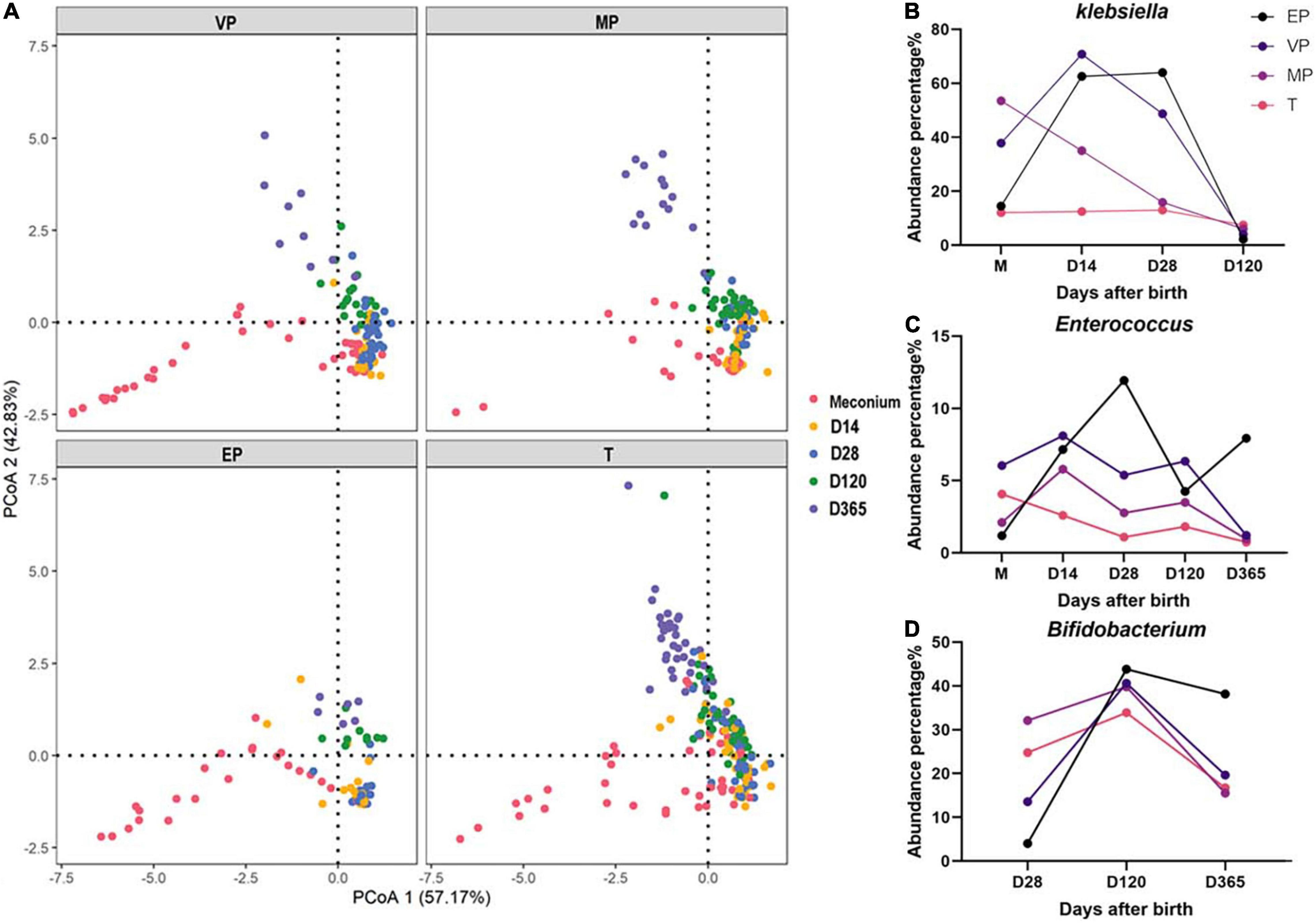
Figure 7. (A) PCoA of all samples in each group. (B–D) The dynamic changes of abundance for Klebsiella, Enterococcus, and Bifidobacterium.
Fecal Microbiome of Infants With Different Gestational Age at Different Days After Birth
The fecal microbiota structure of preterm infants in the same group at different days after birth was compared (Supplementary Figure 4). We found that at the phylum level, the abundance of Proteobacteria in the EP and VP groups first increased and then decreased, whereas it decreased continuously in the MP and T groups (Supplementary Figures 4A,C,E,G). The abundance of Actinobacteria increased from day 120 in the EP and VP groups. Klebsiella was highly abundant in the EP and VP groups until 28 days after birth (Figure 7B and Supplementary Figures 4B,D) and Enterococcus was enriched in the EP group at days 14–28 after birth (Figure 7C). Meanwhile, the abundance of Bifidobacterium increased until 120 days after birth in the EP group, while it remained stable in the MP and T groups from day 14 after birth (Figure 7D and Supplementary Figures 4F,H).
Discussion
Complications in preterm infants are the leading cause of death in the first month of life and continue to affect the outcome of preterm infants (He et al., 2017; Cao et al., 2021; Bell et al., 2022). The gut microbiota plays important roles in health and disease. There is a critical period in early life when the gut microbiota composition is particularly malleable and influenced by various factors. Disruption to the microbiome during this period can be beneficial or detrimental to health (Ahearn-Ford et al., 2022). In order to summary the features, we profiled the gut microbiota of infants with different GAs at different postnatal time points.
As we know, the establishment of gut microbiome from neonates effect by variable factors, mode of delivery, feeding pattern, use of antibiotics, environment and so on (Healy et al., 2022). It is reported that in term infants c-section associates with stunted microbiota and colonization of opportunistic pathogen, including Enterococcus, Enterobacter and Klebsiella species (Shao et al., 2019). C-section also can delay the establishment of gut Microbiota in term infants (Kim et al., 2020). In our study, Klebsiella was significantly different in meconium among four groups. Meanwhile, delivery mode of four groups were significantly different (P < 0.001). It indicated that besides GA, gut microbiome of preterm infants can be greatly influenced by delivery mode.
With the growth of infants after birth, the situation of every infant became different and complex, especially for preterm infants. In our cohort of this research, the number of preterm infants with respiratory-related diseases such as NRDS, pneumonia and BPD obviously increased with the GA decreasing, which was significantly different among four groups. The number of infants with PVL and PDA was markedly different. The number of preterm infants with sepsis and NEC was not statistical difference, but morbidity of sepsis and NEC of preterm groups was higher than term group. Almost all preterm infants used different kinds of antibiotics in NICU. At the same time, especially extremely preterm and very preterm infants, most of their birth was due to the diseases of mothers, they always accepted mixed feeding pattern after birth that first were fed with formula milk and changed when their mother offered breast milk. It is well known that the gut microbiota is also strongly affected by feeding patterns and antibiotics (Healy et al., 2022). Bozzi Cionci et al. (2022) reported longtime exposure to antibiotics in the first weeks of in very preterm neonates affected Clostridium and B. fragilis levels and these changes did not persist at 90 days of life. In addition, NICUs harbor highly diverse bacterial communities and it is thought that bidirectional colonization between infants and NICU environment (Brooks et al., 2018). All above influenced the composition of gut microbiota of preterm infants. The gut microbiota of four groups were completely different from 2 weeks until 28 days after birth as a result.
The gut microbiota of preterm infants were gradually catching up with that of term infants 120 days after birth, because it showed that top three phylum and top two genus were the same between the preterm groups and term group which meant the bacteria composition was similarly, although the abundance was different. However, at 1 year after birth, the gut microbiota of preterm and term infants remained differed, not only the diversity of each group was different, but also the top three abundance of phylum and genus was different among four groups, and abundance were different too. Dynamic changes for gut microbiota of MP and T infants, the abundance of Proteobacteria decreased with the growth of neonates, but for EP and VP infants, the abundance of Proteobacteria first increased and then decreased. These differences between four groups especially from days 120 to 1 year after birth might be caused by feeding patterns, nature environment of family and infection situations of each infant after they left hospital.
At the genus level, the Klebsiella abundance in preterm infants was significantly different among four groups at different time points after birth. Klebsiella is a gram-negative bacterium, and one of the most common species is Klebsiella pneumoniae. Seki et al. (2021) reported that Klebsiella overgrowth in the gut is highly associated with brain damage in extremely preterm infants. Klebsiella is also associated with NEC (Paveglio et al., 2020), nosocomial infections, and LONS (Chen et al., 2020; Ma et al., 2021). van Best et al. (2020) observed that preterm infants (GA < 32 weeks) who received probiotics had increased abundance of Bifidobacterium and reduced abundance of Klebsiella and reduced monthly incidence of NEC. Enterococcus was significantly different from 28 days after birth till 1 year. Enterococcus was thought to be one of four phases and was only observed among EP infants and appeared to disturb the normal microbiota succession (Korpela et al., 2018). Furthermore, Al-Judaibi et al. (2021) reported that three common species were found in 15 of 19 stool samples of the preterm infants in the NICU, which were affected by commonly used antibiotics, with significant resistance of Enterococcus and K. pneumoniae to some of the tested antibiotics. Enterococcus is also a common pathogen in the NICU, and Enterococcus infection is the major cause of neonatal prematurity, meningitis, and sepsis (Reuschel et al., 2020). In another study, Enterococcus and Streptococcus were enriched in the non-probiotic formula-feeding group of premature infants (Chi et al., 2021). Bifidobacterium is a probiotic that confers health benefits on the host and its administration is regarded as a promising strategy to prevent neonatal NEC and sepsis (Hui et al., 2021). The abundance of Bifidobacterium showed the opposite trend of that of Klebsiella in our study. Except at 120 days, Bifidobacterium appeared significantly different among four groups at other time points. One study showed that probiotics, including Bifidobacterium supplementation in preterm infants, may prevent serious morbidities (Plummer et al., 2021). Another study showed that routine administration of a multispecies probiotic containing Bifidobacterium and Lactobacillus to very low birth weight infants had no significant impact on the NEC incidence (Juber et al., 2021) which shown the different results compared with reference Reuschel et al. (2020).
Comparing with term infants, the gut microbiota of preterm infants is always characterized by decreased microbial diversity (Chi et al., 2019; Henderickx et al., 2019; Jia et al., 2020). In our study, Shannon index for alpha diversity of meconium in EP group was significantly higher than other preterm groups and term group and Shannon index of meconium in preterm group was decreased with increasing of GA. Besides GA, some demographic characteristics of mothers in each group were significantly different, which suggested different alpha diversity of meconium were strongly associated with other situations not only GA. At day 14 to day 28 after birth, alpha diversity of T and MP group were significantly higher than other two preterm groups. However, alpha diversity of preterm groups and term group was no significant different at day 120 after birth, and MP group owned the highest alpha diversity at day 365 after birth which was higher than term group.
We tracked the development of the gut microbiota in preterm infants with different GA from newborn to 365 days after birth, compared it with that of term infants, and profiled the features of dynamic changes of gut microbiome. The results of our research provide new insights that we can interfere with specific microbes of preterm infants with different GA and different time points after birth, such as opportunistic pathogen Klebsiella and Enterococcus, as well as the probiotics Bifidobacterium.
Our study has some certain limitations. The number of fecal samples from EP and VP infants at day 365 was smaller than that in the other groups because of the weak compliance of the parents. Because of the limitation of sample size, we did not further divide by delivery mode, feeding pattern and antibiotic use. However, we carefully draw conclusions from our existing data, we will enlarge the sample size for validation in out further studies.
Data Availability Statement
The data presented in the study are deposited in the NCBI repository with accession BioProject ID: PRJNA837453.
Ethics Statement
The studies involving human participants were reviewed and approved by the Ethics Committee of Peking University Third Hospital. Written informed consent to participate in this study was provided by the participants or their legal guardian/next of kin.
Author Contributions
XT: conceiving and design. XT and YX: supervising of study and critical revision of manuscript. YC, YY, and YW: subject recruitment and performance of clinical procedure. XY, ZC, BL, LC, and DM: bioinformatics analysis. QJ and XY: drafting of manuscript. All authors contributed to the article and approved the submitted version.
Funding
This work was financially supported by the Beijing Natural Science Foundation (Nos. S160004 and 7214270).
Conflict of Interest
BL and LC were employed by National Engineering Center of Dairy for Maternal and Child Health, Beijing Sanyuan Foods Co., Ltd.
The remaining authors declare that the research was conducted in the absence of any commercial or financial relationships that could be construed as a potential conflict of interest.
Publisher’s Note
All claims expressed in this article are solely those of the authors and do not necessarily represent those of their affiliated organizations, or those of the publisher, the editors and the reviewers. Any product that may be evaluated in this article, or claim that may be made by its manufacturer, is not guaranteed or endorsed by the publisher.
Acknowledgments
We are grateful to all subjects who participated in this study. We also thanks to the doctors and nurses in the NICU of Department of Pediatrics of Peking University Third Hospital for their kindly helping in this project.
Supplementary Material
The Supplementary Material for this article can be found online at: https://www.frontiersin.org/articles/10.3389/fmicb.2022.923273/full#supplementary-material
References
Aguilar-Lopez, M., Dinsmoor, A. M., Ho, T. T. B., and Donovan, S. M. (2021). A systematic review of the factors influencing microbial colonization of the preterm infant gut. Gut Microbes 13, 1–33. doi: 10.1080/19490976.2021.1884514
Ahearn-Ford, S., Berrington, J. E., and Stewart, C. J. (2022). Development of the gut microbiome in early life. Exp. Physiol. 107, 415–421.
Al-Judaibi, E. A., Altammar, K. A., Alshareef, S. A., Alrashedi, M. E., Al-Gamdee, Y. A., Al Gamdee, M. A., et al. (2021). Antibiotic sensitivity and resistance of gastrointestinal microflora isolated from preterm neonates. Future Microbiol. 16, 1029–1039. doi: 10.2217/fmb-2021-0057
Beghetti, I., Barone, M., Turroni, S., Biagi, E., Sansavini, A., Brigidi, P., et al. (2022). Early-life gut microbiota and neurodevelopment in preterm infants: any role for Bifidobacterium? Eur. J. Pediatr. 181, 1773–1777.
Bell, E. F., Hintz, S. R., Hansen, N. I., Bann, C. M., Wyckoff, M. H., DeMauro, S. B., et al. (2022). Mortality, In-Hospital Morbidity, Care Practices, and 2-Year Outcomes for Extremely Preterm Infants in the US, 2013-2018. JAMA 327, 248–263. doi: 10.1001/jama.2021.23580
Bozzi Cionci, N., Lucaccioni, L., Pietrella, E., Ficara, M., Spada, C., Torelli, P., et al. (2022). Antibiotic Exposure, Common Morbidities and Main Intestinal Microbial Groups in Very Preterm Neonates: A Pilot Study. Antibiotics 11:237. doi: 10.3390/antibiotics11020237
Brooks, B., Olm, M. R., Firek, B. A., Baker, R., Geller-McGrath, D., Reimer, S. R., et al. (2018). The developing premature infant gut microbiome is a major factor shaping the microbiome of neonatal intensive care unit rooms. Microbiome 6:112. doi: 10.1186/s40168-018-0493-5
Cao, Y., Jiang, S., Sun, J., Hei, M., Wang, L., Zhang, H., et al. (2021). Assessment of Neonatal Intensive Care Unit Practices, Morbidity, and Mortality Among Very Preterm Infants in China. JAMA Netw. Open 4:e2118904. doi: 10.1001/jamanetworkopen.2021.18904
Chen, Y., Brook, T. C., Soe, C. Z., O’Neill, I., Alcon-Giner, C., Leelastwattanagul, O., et al. (2020). Preterm infants harbour diverse Klebsiella populations, including atypical species that encode and produce an array of antimicrobial resistance- and virulence-associated factors. Microb. Genom. 6:e000377. doi: 10.1099/mgen.0.000377
Chi, C., Fan, Y., Li, C., Li, Y., Guo, S., Li, T., et al. (2021). Early Gut Microbiota Colonisation of Premature Infants Fed with Breastmilk or Formula with or without Probiotics: A Cohort Study. Nutrients 13:4068. doi: 10.3390/nu13114068
Chi, C., Xue, Y., Lv, N., Hao, Y., Liu, R., Wang, Y., et al. (2019). Longitudinal Gut Bacterial Colonization and Its Influencing Factors of Low Birth Weight Infants During the First 3 Months of Life. Front. Microbiol. 10:1105. doi: 10.3389/fmicb.2019.01105
Cole, J. R., Wang, Q., Cardenas, E., Fish, J., Chai, B., Farris, R. J., et al. (2009). The Ribosomal Database Project: improved alignments and new tools for rRNA analysis. Nucleic Acids Res. 37, D141–D145. doi: 10.1093/nar/gkn879
Deng, K., Liang, J., Mu, Y., Liu, Z., Wang, Y., Li, M., et al. (2021). Preterm births in China between 2012 and 2018: an observational study of more than 9 million women. Lancet Glob. Health 9, e1226–e1241. doi: 10.1016/S2214-109X(21)00298-9
El Manouni El Hassani, S., Niemarkt, H. J., Berkhout, D. J. C., Peeters, C. F. W., Hulzebos, C. V., et al. (2021). Profound Pathogen-Specific Alterations in Intestinal Microbiota Composition Precede Late-Onset Sepsis in Preterm Infants: A Longitudinal, Multicenter, Case-Control Study. Clin. Infect. Dis. 73, e224–e232. doi: 10.1093/cid/ciaa1635
He, C., Liu, L., Chu, Y., Perin, J., Dai, L., Li, X., et al. (2017). National and subnational all-cause and cause-specific child mortality in China, 1996-2015: a systematic analysis with implications for the Sustainable Development Goals. Lancet Glob. Health 5, e186–e197. doi: 10.1016/S2214-109X(16)30334-5
Healy, D. B., Ryan, C. A., Ross, R. P., Stanton, C., and Dempsey, E. M. (2022). Clinical implications of preterm infant gut microbiome development. Nat. Microbiol. 7, 22–33. doi: 10.1038/s41564-021-01025-4
Henderickx, J. G. E., Zwittink, R. D., van Lingen, R. A., Knol, J., and Belzer, C. (2019). The Preterm Gut Microbiota: An Inconspicuous Challenge in Nutritional Neonatal Care. Front. Cell. Infect. Microbiol. 9:85. doi: 10.3389/fcimb.2019.00085
Hui, Y., Smith, B., Mortensen, M. S., Krych, L., Sorensen, S. J., Greisen, G., et al. (2021). The effect of early probiotic exposure on the preterm infant gut microbiome development. Gut Microbes 13:1951113. doi: 10.1080/19490976.2021.1951113
Jia, J., Xun, P., Wang, X., He, K., Tang, Q., Zhang, T., et al. (2020). Impact of Postnatal Antibiotics and Parenteral Nutrition on the Gut Microbiota in Preterm Infants During Early Life. J. Parenter. Enter. Nutr. 44, 639–654. doi: 10.1002/jpen.1695
Juber, B. A., Boly, T. J., Pitcher, G. J., and McElroy, S. J. (2021). Routine Administration of a Multispecies Probiotic Containing Bifidobacterium and Lactobacillus to Very Low Birth Weight Infants Had No Significant Impact on the Incidence of Necrotizing Enterocolitis. Front. Pediatr. 9:757299. doi: 10.3389/fped.2021.757299
Kim, G., Bae, J., Kim, M. J., Kwon, H., Park, G., Kim, S. J., et al. (2020). Delayed Establishment of Gut Microbiota in Infants Delivered by Cesarean Section. Front. Microbiol. 11:2099. doi: 10.3389/fmicb.2020.02099
Korpela, K., Blakstad, E. W., Moltu, S. J., Strommen, K., Nakstad, B., Ronnestad, A. E., et al. (2018). Intestinal microbiota development and gestational age in preterm neonates. Sci. Rep. 8:2453. doi: 10.1038/s41598-018-20827-x
Lee, J. K., Hern Tan, L. T., Ramadas, A., Ab Mutalib, N. S., and Lee, L. H. (2020). Exploring the Role of Gut Bacteria in Health and Disease in Preterm Neonates. Int. J. Environ. Res. Public Health 17:6963. doi: 10.3390/ijerph17196963
Ma, L., Peng, Q., Wang, W., Yang, Y., Chen, Y., Wang, L., et al. (2021). Late-onset sepsis in very low birth weight preterm infants: 7 years’ experience at a tertiary hospital in China. Pediatr. Neonatol. 62, 529–535. doi: 10.1016/j.pedneo.2021.05.011
Olm, M. R., Bhattacharya, N., Crits-Christoph, A., Firek, B. A., Baker, R., Song, Y. S., et al. (2019). Necrotizing enterocolitis is preceded by increased gut bacterial replication, Klebsiella, and fimbriae-encoding bacteria. Sci. Adv. 5:eaax5727. doi: 10.1126/sciadv.aax5727
Paveglio, S., Ledala, N., Rezaul, K., Lin, Q., Zhou, Y., Provatas, A. A., et al. (2020). Cytotoxin-producing Klebsiella oxytoca in the preterm gut and its association with necrotizing enterocolitis. Emerg. Microbes Infect. 9, 1321–1329. doi: 10.1080/22221751.2020.1773743
Plummer, E. L., Danielewski, J. A., Garland, S. M., Su, J., Jacobs, S. E., and Murray, G. L. (2021). The effect of probiotic supplementation on the gut microbiota of preterm infants. J. Med. Microbiol. 70:001403. doi: 10.1099/jmm.0.001403
Reuschel, E., Toelge, M., Haeusler, S., Deml, L., Seelbach-Goebel, B., and Solano, M. E. (2020). Perinatal Gram-Positive Bacteria Exposure Elicits Distinct Cytokine Responses In Vitro. Int. J. Mol. Sci. 22:332. doi: 10.3390/ijms22010332
Seki, D., Mayer, M., Hausmann, B., Pjevac, P., Giordano, V., Goeral, K., et al. (2021). Aberrant gut-microbiota-immune-brain axis development in premature neonates with brain damage. Cell Host Microbe 29, 1558–1572.e6. doi: 10.1016/j.chom.2021.08.004
Shao, Y., Forster, S. C., Tsaliki, E., Vervier, K., Strang, A., Simpson, N., et al. (2019). Stunted microbiota and opportunistic pathogen colonization in caesarean-section birth. Nature 574, 117–121. doi: 10.1038/s41586-019-1560-1
Stewart, C. J., Embleton, N. D., Marrs, E. C. L., Smith, D. P., Fofanova, T., Nelson, A., et al. (2017). Longitudinal development of the gut microbiome and metabolome in preterm neonates with late onset sepsis and healthy controls. Microbiome 5:75. doi: 10.1186/s40168-017-0295-1
Stewart, C. J., Marrs, E. C., Nelson, A., Lanyon, C., Perry, J. D., Embleton, N. D., et al. (2013). Development of the preterm gut microbiome in twins at risk of necrotising enterocolitis and sepsis. PLoS One 8:e73465. doi: 10.1371/journal.pone.0073465
van Best, N., Trepels-Kottek, S., Savelkoul, P., Orlikowsky, T., Hornef, M. W., and Penders, J. (2020). Influence of probiotic supplementation on the developing microbiota in human preterm neonates. Gut Microbes 12, 1–16. doi: 10.1080/19490976.2020.1826747
Wang, Q., Garrity, G. M., Tiedje, J. M., and Cole, J. R. (2007). Naive Bayesian classifier for rapid assignment of rRNA sequences into the new bacterial taxonomy. Appl. Environ. Microbiol. 73, 5261–5267. doi: 10.1128/AEM.00062-07
Keywords: gut microbiota, gestational age, Klebsiella, Enterococcus, Bifidobacterium
Citation: Jia Q, Yu X, Chang Y, You Y, Chen Z, Wang Y, Liu B, Chen L, Ma D, Xing Y and Tong X (2022) Dynamic Changes of the Gut Microbiota in Preterm Infants With Different Gestational Age. Front. Microbiol. 13:923273. doi: 10.3389/fmicb.2022.923273
Received: 19 April 2022; Accepted: 30 May 2022;
Published: 30 June 2022.
Edited by:
George Grant, University of Aberdeen, United KingdomReviewed by:
Jennifer Carla Boer, RMIT University, AustraliaWanli Xu, University of Connecticut, United States
Copyright © 2022 Jia, Yu, Chang, You, Chen, Wang, Liu, Chen, Ma, Xing and Tong. This is an open-access article distributed under the terms of the Creative Commons Attribution License (CC BY). The use, distribution or reproduction in other forums is permitted, provided the original author(s) and the copyright owner(s) are credited and that the original publication in this journal is cited, in accordance with accepted academic practice. No use, distribution or reproduction is permitted which does not comply with these terms.
*Correspondence: Yan Xing, eXhzeHpAb3V0bG9vay5jb20=; Xiaomei Tong, dG9uZ3htMjAwN0AxMjYuY29t
†These authors share first authorship