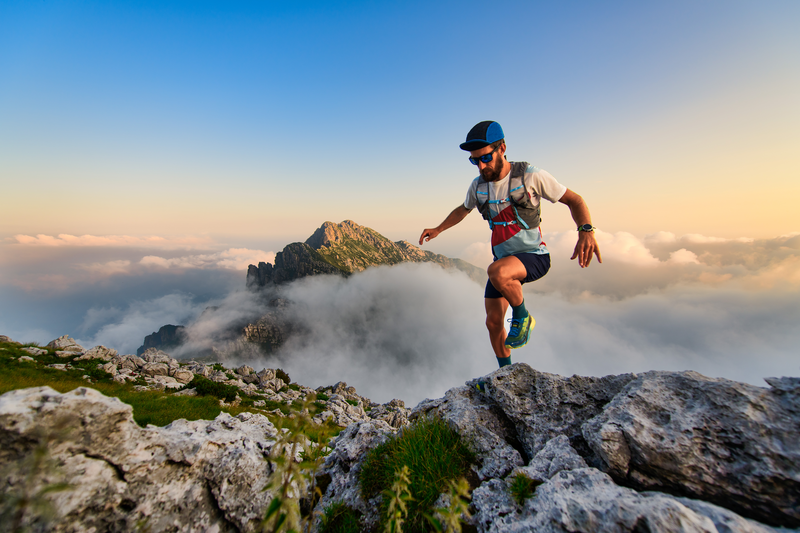
95% of researchers rate our articles as excellent or good
Learn more about the work of our research integrity team to safeguard the quality of each article we publish.
Find out more
REVIEW article
Front. Microbiol. , 09 June 2022
Sec. Infectious Agents and Disease
Volume 13 - 2022 | https://doi.org/10.3389/fmicb.2022.919501
Secondary fungal infections may complicate the clinical course of patients affected by viral respiratory diseases, especially those admitted to intensive care unit. Hospitalized COVID-19 patients are at increased risk of fungal co-infections exacerbating the prognosis of disease due to misdiagnosis that often result in treatment failure and high mortality rate. COVID-19-associated fungal infections caused by predominantly Aspergillus and Candida species, and fungi of the order Mucorales have been reported from several countries to become significant challenge for healthcare system. Early diagnosis and adequate antifungal therapy is essential to improve clinical outcomes, however, drug resistance shows a rising trend highlighting the need for alternative therapeutic agents. The purpose of this review is to summarize the current knowledge on COVID-19-associated mycoses, treatment strategies and the most recent advancements in antifungal drug development focusing on peptides with antifungal activity.
Fungal diseases remain a significant medical issue considering as a worldwide threat to human health affecting close to one billion individuals. There are several reasons for ongoing increase of invasive fungal infections, including the use of immunosuppressive therapies in context of cancer treatment or transplantation, the increased use of modern medical devices, such as catheters and implants, and the use of broad-spectrum antibiotics. The coronavirus disease 2019 (COVID-19) pandemic further worsened the current situation since this viral respiratory disease predispose the patients to secondary life-threatening fungal infections in the intensive care units (ICUs) making adequate diagnosis more difficult. The overlapping respiratory manifestations complicate the treatment of COVID-19. Prolonged hospitalization period and potentially required mechanical ventilation along with lymphopenia, leukopenia, and systemic hyperinflammatory reaction facilitates the growth of fungi in COVID-19 patients (Arastehfar et al., 2020a; Amin et al., 2021; Baddley et al., 2021; Gregoire et al., 2021). In addition, World Health Organization (WHO) COVID-19 treatment guideline recommend empirically prescribed broad-spectrum antibiotics to treat possible bacterial co-infections, but only in severe COVID-19 patients (World Health Organization, 2021). The main causative organisms responsible for the majority of serious fungal diseases are Candida, Aspergillus, Mucorales, and Cryptococcus. Despite of fungal healthcare-associated invasive infections have unacceptably high mortality rate, the number of deaths is most likely an underestimation due to poor epidemiological data and misdiagnosis (Bongomin et al., 2017). The high clinical mortality and economic burden posed by invasive fungal infections has resulted in the widespread use of antifungal agents. Antifungal treatments and/or prophylaxis are essential to reduce comorbidities and mortalities caused by fungal infections. However, due to selective drug pressure, the efficacy of the limited systemic antifungal drugs has been changed yielding species with less predictable antifungal susceptibility (Friedman and Schwartz, 2019). Microbial resistance is common in certain fungal species and involves both intrinsic resistance (strains are inherently less susceptible to a given antifungal agent), and secondary resistance (acquired resistance in an otherwise susceptible strain following drug exposure). The most notable species emerging worldwide and regarded as major concern for public health are triazole-resistant Aspergillus fumigatus (Verweij et al., 2016; Romero et al., 2019; Yang et al., 2021), C. tropicalis, C. parapsilosis (Pfaller et al., 2019), multidrug-resistant (MDR) Candida auris (Chowdhary et al., 2018; Chaabane et al., 2019; Chow et al., 2020) and MDR C. glabrata showing increasing prevalence globally (Haeley and Perlin, 2018). The narrow spectrum activity and cross-resistance due to similar mechanisms of action across drugs has triggered the search for safer alternatives with reduced toxicity, improved pharmacodynamics and pharmacokinetics, and increased specificity (Parente-Rocha et al., 2017; Gintjee et al., 2020; Duncan et al., 2021). In this review we provide an overview of emerging fungal diseases in COVID-19 patients highlighting the current antifungal treatments. We also highlight some aspects of new antifungal drug development with a great promise to overcome resistance issues. In particular, we focus on novel antifungal peptides as other emerging alternatives, such as agents with new structure for a known target or entirely novel targets, combination therapy of antifungals with non-antifungal drugs and quorum-sensing molecules have been recently reviewed by other authors (Kovács and Majoros, 2020; Bouz and Doležal, 2021; Rossato et al., 2021).
In the last decade, a multidrug-resistant nosocomial pathogen, C. auris, has emerged and spread worldwide. Since its discovery in 2009 from the ear discharge of a Japanese patient (Satoh et al., 2009), C. auris has been reported from 46 countries causing outbreaks in healthcare institutions (Allaw et al., 2021; Chakrabarti and Sood, 2021; de Almeida et al., 2021; Kurt et al., 2021; Pandya et al., 2021; Shaukat et al., 2021). Phylogenetic analysis using whole genome sequencing has revealed deep divergence within the C. auris species. High inter-clade genetic diversity has been found suggesting that distinct clades emerged independently at different geographic regions (Lockhart et al., 2017). Four major clades have been described so far: South Asian (clade I), South African (clade III), South American (clade IV) and East Asian (clade II). A potential fifth clade has also been identified in Iran (Chow et al., 2019; Rhodes and Fisher, 2019). The majority of strains in the East Asian clade was isolated from ear infection only, whereas other clades are known to cause nosocomial invasive infections and outbreaks in healthcare settings (Welsh et al., 2019).
Invasive microbial co-infections during hospitalization may lead to more severe outcomes. The crude in-hospital mortality rate of invasive infections caused by C. auris is larger than that of caused by other Candida species, ranging from 30 to 72% (Taori et al., 2019; Arensman et al., 2020; Chakrabarti and Singh, 2020; de Almeida et al., 2021; Garcia-Bustos et al., 2021). C. auris superinfections in critically ill COVID-19 patients have been associated with 30-day mortality rates usually above 50%, although the high case-fatality rate is multifactorial and not exclusively attributed to C. auris infection (Table 1; Chowdhary et al., 2020; Magnasco et al., 2021; Villanueva-Lozano et al., 2021).
The pandemics of COVID-19 has overwhelmed healthcare facilities promoting the nosocomial transmission of Candida species especially in ICUs. Both prospective and retrospective data of COVID-19 patients admitted to ICU suggested that risk factors driving the high incidence of candidaemia include prolonged hospital stay, mechanical ventilation, central venous catheters, surgical procedure, and use of broad-spectrum antibiotics, steroids and immunosuppressant drugs (Ezeokoli et al., 2021; Nucci et al., 2021). The hospital environment also plays a crucial role in the local spread of C. auris as it is able to efficiently survive for 7 days on steel and porous surfaces and for 14 days on plastics. C. auris survives desiccation and resists quaternary ammonium compound disinfectants, peracetic acid, standard ultraviolet-C cycle times and standard concentration of sodium hypochlorite as well (Welsh et al., 2017; Cadnum et al., 2018; Rutala et al., 2019; Chakrabarti and Sood, 2021). Healthcare workers and physicians transiently colonized on their hands, nares and groin, and contaminated medical devices may facilitate the dissemination of C. auris. Nobrega de Almeida et al. (2021) investigated C. auris colonization of patients, healthcare workers, and inanimate sites of a Brazilian hospital. Among the samples collected from inanimate surfaces, the digital thermometers had the highest rate of positive cultures (17%), followed by bed rails (14.9%), vital signs monitors/intravenous infusion pumps (10.6%), and tray tables (10.6%). In addition, the COVID-19 pandemic may provide ideal conditions for prolonged outbreaks of C. auris in hospital ICUs due to over-occupancy and limited resources for infection control practices (e.g., prolonged usage of personnel protective equipment through shortages) (Chowdhary et al., 2020; Allaw et al., 2021; Prestel et al., 2021; Villanueva-Lozano et al., 2021). However, observational studies showed that C. albicans and C. glabrata and not C. auris were the causative agent in the majority of COVID-19-associated candidaemia significantly increasing the mortality rate of patients as well (Casalini et al., 2021). Machado et al. reported that the incidence of candidaemia caused by common species in ICU patients with COVID-19 was higher than patients without COVID-19. Interestingly, the higher incidence was not driven by patient-to-patient Candida spp. transmission suggesting that individual risk factors might also contribute to the rising number of Candida infections (Machado et al., 2022).
The management of C. auris-infected patients is cumbersome since conventional biochemical microbiological techniques are unable to properly identify C. auris and isolates are often associated with multi- or pandrug resistance yielding high therapeutic failure rate with all types of antifungal treatments (Chaabane et al., 2019; Fasciana et al., 2020). The relationship between minimal inhibitory concentration (MIC) values and clinical outcomes is still not fully understood, resulting in a lack of established susceptibility breakpoints for C. auris. As C. auris pose substantial risks for infection control and prevention, the CDC has defined tentative antifungal breakpoints using susceptibility data from hundreds of clinical C. auris isolates. Based on tentative breakpoints, echinocandins are recommended as initial treatment for C. auris infections and, if no clinical improvement is observed, amphotericin B can be prescribed, or amphotericin B should be added in combination with other antifungal drugs (Table 2; Centers for Disease Control and Prevention, 2020, 2021). Nevertheless, C. auris has the capacity to rapidly acquire resistance to antifungals in vivo. A report from India showed that multidrug-resistant C. auris was responsible for two third of candidaemia cases among COVID-19 patients in New Delhi with a case-fatality rate of 60%. Applying antifungal susceptibility testing, all isolates were resistant to fluconazole (MIC > 32 mg/L) and 30% were non-susceptible to voriconazole (VOR) (MIC > 2 mg/L). Moreover, 40% showed resistance to amphotericin B (MIC > 2 mg/L) and 60% were resistant to 5-flucytosine (MIC > 32 mg/L). Overall, 30% of C. auris isolates were multiazole (fluconazole + VOR) resistant, whilst 70% were multidrug resistant (Chowdhary et al., 2020). Another study reported large number of fluconazole resistant clinical isolates (99.8%) in New York. Fifty percent of the isolates were amphotericin B resistant, whereas echinocandin resistance increased from 0 to 4% and pan-resistance increased from 0 to <1% for C. auris clinical isolates in a 5-year period (Kilburn et al., 2022). C. auris is able to form biofilms, which is also contributed to the increased virulence, antifungal resistance and poor clinical outcomes. Biofilms are structured microbial communities that form on abiotic and biotic surfaces and are embedded in an extracellular matrix influencing drug resistance by hindering drug penetration into dense biofilms. A large number of C. auris infections has been connected to the use of health devices that can serve as a source of infection and can spread to other parts of the body (Eyre et al., 2018; Ruiz-Gaitán et al., 2018; Castro et al., 2019; Jabeen et al., 2020). According to these characteristics, C. auris is an emerging invasive pathogen in critically ill COVID-19 patients that requires the implementation of strict infectious control measures such as contact precautions, screening, and diligent decolonization of the patients to prevent the potential nosocomial spread.
Aspergillus spp. are ubiquitous, environmental molds, forming spores that enter the body via inhalation. A. fumigatus is the most common etiological agent worldwide, probably due to the relatively small size of conidia, which allows for its deep penetration into the alveolar space. Aspergillus spores that reach the lungs of immunocompetent humans are generally eliminated by cellular components of the innate immune system, such as neutrophils and macrophages (Hohl and Feldmesser, 2007). However, Aspergillus spp. can cause a variety of clinical manifestations in immunocompromised individuals. Following inhalation or inoculation with spores that circulate in the environment, infection may develop leading to allergic reactions or to infectious diseases, which may progress from the respiratory system to a disseminated or invasive infection (Latgé and Chamilos, 2019). Invasive pulmonary aspergillosis (IPA) was reported to be common in critically ill patients that cause high morbidity and mortality (Barnes and Marr, 2006). Several factors predispose hospitalized patients to IPA including corticosteroid therapy, antibiotics, and hematologic malignancy. Respiratory viral infections such as influenza have also been associated with IPA (Schauwvlieghe et al., 2018). Due to immunopathological similarities between severe influenza and severe acute respiratory syndrome coronavirus 2 (SARS-CoV-2) pneumonia (e.g., cytokine storm syndrome, epithelial damage within the airways, lymphopenia) critically ill patients are at risk of secondary infections with Aspergillus spp. (Khorramdelazad et al., 2021). The diagnosis of COVID-19-associated pulmonary aspergillosis (CAPA) is difficult as COVID-19 patients in ICUs generally have less-specific radiological signs of infection in the presence of acute respiratory distress syndrome (Koehler et al., 2019; Bartoletti et al., 2021). The recently proposed consensus criteria for definition of CAPA facilitate the uniform CAPA classification across medical practices enabling more accurate estimation of aspergillosis cases in COVID-19 patients. Diagnosis of CAPA relies especially on direct microscopic indication of fungal characteristics that are specific of Aspergillus spp., culture-based methods and indirect fungal biomarkers (galactomannan and 1,3-β-D-glucan). In accordance with sample validity and diagnostic evidence, proven, probable and possible CAPA categories have been established. Although upper respiratory samples often cannot distinguish between Aspergillus colonization and invasive disease, and serum galactomannan as well as serum β-D-glucan exhibit suboptimal sensitivity and specificity, the detection of galactomannan in lower respiratory samples (i.e., bronchoalveolar lavage) is highly suggestive for CAPA (Koehler et al., 2021). Even though the consensus regarded bronchoscopy with bronchoalveolar lavage as an efficient technique in the detection of CAPA, bronchoscopy is not routinely performed in many institutions to reduce COVID-19 transmission risk related to aerosolisation generated during this sampling method (Tio et al., 2021). Therefore, the majority of reported cases were classified as probable or possible CAPA as it has been commonly difficult to prove the correlation between IPA and COVID-19 (Lamoth et al., 2021). A systematic review of autopsy series with histopathological investigations of COVID-19 decedents have also demonstrated the problems with CAPA diagnosis since post-mortem incidence of CAPA was somewhat lower than expected based on clinical findings (Kula et al., 2021).
There are additional risk factors typically connected with the management of severe COVID-19 patients. Prospective and retrospective cohort studies with COVID-19 patients admitted to ICUs showed association between the use of high-dose corticosteroid administration and Aspergillus co-infection (Bartoletti et al., 2021; Dellière et al., 2021). Moreover, anti-interleukin-6 (IL-6) receptor treatment, such as tocilizumab therapy that is widely used to treat COVID-19, seems to potentially confer higher risk for developing CAPA as the significantly elevated level of IL-6 in severe COVID-19 patients has also been found as a contributing factor in protection against Aspergillus (Table 1; Guaraldi et al., 2020; Bartoletti et al., 2021; Feys et al., 2021). Assessing the worldwide burden of CAPA is complicated due to differences in diagnostic criteria and the wide range of respiratory specimen types obtained for mycological diagnostics. Additionally, observational studies have been performed mainly in European countries and only few data from other continents are available (Feys et al., 2021). Based on analysis of clinical data from several countries, regional variability in CAPA incidence have been observed ranging from 0.54 to 42.1% considering overall CAPA incidence (Feys et al., 2021; Takazono et al., 2021; Jiang et al., 2022). Lahmer et al. (2021) investigated 32 patients with severe COVID-19 associated pneumonia and reported a high incidence of CAPA among COVID-19 patient (11/32; 34%) (Lahmer et al., 2021). Similar incidence rate (8/19; 42.1%) was observed by Jiang et al.; however, both studies were conducted with limited number of COVID-19 patients (Jiang et al., 2022). Despite the differences in reported incidences of CAPA, COVID-19 increased the risk of developing an IPA and CAPA was significantly associated with higher mortality rate (up to 50%) underscoring the importance of global awareness and early diagnosis (Ezeokoli et al., 2021; Lahmer et al., 2021; Meijer et al., 2021; Jiang et al., 2022).
The first-line antifungal agents recommended by the European Confederation of Medical Mycology (ECMM) and International Society for Human and Animal Mycology (ISHAM) for CAPA are VOR or isavuconazole (Koehler et al., 2021). However, several adverse effects related to VOR treatment are known (e.g., liver abnormalities, gastrointestinal disturbance). VOR also has multiple drug-drug interactions including remdesivir, which is generally used in COVID-19 treatment, as both drugs are metabolized by cytochrome P450 enzyme CYP3A4 (McCreary and Pogue, 2020). Plasma concentration monitoring is required due to the unpredictable metabolism of VOR as both subtherapeutic and toxic levels have been detected in critically ill patients. Its narrow therapeutic window and toxicity along with its interactions with other drugs yielded its limited use in ICU patients (Cadena et al., 2021; Tio et al., 2021). Compared to VOR, isavuconazole has better pharmacokinetic profile and is less toxic, but also serves as a substrate for CYP3A4 reducing its efficacy. Liposomal amphotericin B is the alternative option except for patients with COVID-19 related renal insufficiency. Alternative second-line agents are posaconazole or echinocandins. Echinocandins should be used for salvage therapy or in combination with other drugs (Table 2; Koehler et al., 2021).
Another growing concern about the management of CAPA is the case reports of triazole-resistant A. fumigatus infections in COVID-19 patients. Triazole-resistance of Aspergillus species often varies substantially among geographic regions (from less than 1% in France to an estimated ∼11% prevalence in the Netherlands) worsening the prognosis of patients with invasive aspergillosis (Alanio et al., 2011; Lestrade et al., 2020). Isolates can acquire azole resistance either following prolonged azole treatment of patients in clinical settings by single point mutations in the lanosterol 14-α-demethylase gene (cyp51A), encoding a key protein in the ergosterol biosynthesis pathway or by tandem repeat integrations of different sizes in the cyp51A promoter inducing point mutations in the gene (TR34/L98H, TR46/Y121F/T289A, and TR53) due to the selective pressure caused by the extended use of demethylation inhibitors in agriculture (Arastehfar et al., 2020b; Garcia-Rubio et al., 2017). Considering CAPA, five cases of triazole-resistant A. fumigatus have been reported so far and four out of five isolates had TR34/L98H mutation in cyp51A gene, which is associated with acquired environmental resistance, commonly resulting in pan-azole resistance (Borman et al., 2020; Ghelfenstein-Ferreira et al., 2021; Meijer et al., 2020, 2021; Mohamed et al., 2021). This environmentally acquired resistance in cyp51A gene is in line with clinical data where most of the patients with azole-resistant infections had no history of azole prophylaxis or treatment (Meis et al., 2016; Ghelfenstein-Ferreira et al., 2021). The occurrence of triazole and multi-triazole resistance underscores the importance of early diagnosis and the urgent need for antifungal drug susceptibility testing of Aspergillus isolates on a routine basis using a rapid and simple phenotypic method and/or by detection of cyp51A gene associated triazole resistance mutations directly on respiratory samples.
Mucormycosis is an angioinvasive fungal infection caused by filamentous fungi of the order of Mucorales. It is the third most common fungal infection after aspergillosis and candidiasis accounting for 9% of all invasive mycosis in immunocompromised patients (Pasternak and Olszanecki, 2021). The disease is characterized by rapidly progressive nature and high mortality rate (reaching 40-80%) even despite adequate treatment. The most frequently identified pathogens responsible for mucormycosis are Rhizopus spp., Mucor spp., Lichteimia spp., Rhizomucor spp., Cunninghamella spp., Apophysomyces spp., and Saksenaea spp. (Cornely et al., 2019). The incidence of mucormycosis in recent years and during COVID-19 pandemic has increased gradually, especially in India (Hussain et al., 2021). During the pre-pandemic era, the prevalence of mucormycosis varied from 0.005 to 1.7 per million population worldwide, whereas in India it was 80 times more common with approximately 0.14 cases per 1,000 individuals (Skiada et al., 2020). India have seen an enormous increase in COVID-19-associated mucormycosis (CAM) cases in the second wave of COVID-19 that was declared as an outbreak in May 2021 leading to a collapse of the healthcare system in the middle of the pandemic (Hussain et al., 2021). Nevertheless, the incidence of mucormycosis in patients having or recovering from COVID-19 has been escalating throughout the world. Multiple CAM case reports from United States, Pakistan, Iran, Mexico and single case reports from Brazil and Chile have been published (Khan et al., 2020; Monte Junior et al., 2020; Rabagliati et al., 2021; Shakir et al., 2021; Tabarsi et al., 2021; Guzmán-Castro et al., 2022). In Europe, CAM cases have been reported from Austria, Germany, Spain, Czech Republic, France, Italy, the Netherlands and the United Kingdom (Hanley et al., 2020; Arana et al., 2021; Bellanger et al., 2021; Buil et al., 2021; Pasero et al., 2021; Zurl et al., 2021; Danion et al., 2022; Hoenigl et al., 2022; Seidel et al., 2022).
Mucorales are abundant in nature and can be found on decaying organic matter and in the soil. Infection may develop by inhaled fungal spores that are ubiquitously present in the air or by inoculation of the spores into disrupted mucosa or wounds. The hyphal growth cause invasion of blood vessels, resulting in thrombosis and progressive necrosis that leads to soft tissue and bone destruction irrespective of the route of invasion (Pasternak and Olszanecki, 2021; Kamath et al., 2022). In susceptible hosts, six distinctive clinical manifestation could be observed including rhino-orbital-cerebral, pulmonary, gastrointestinal, cutaneous, disseminated, and uncommon infection. Rhino-orbital-cerebral is the most common form of mucormycosis with Rhizopus spp. being the most prevalent causative pathogen (Pasternak and Olszanecki, 2021; Hoenigl et al., 2022). The major predisposing factors for the development of mucormycosis are uncontrolled diabetes mellitus with ketoacidosis, neutropenia, hematological malignancy, stem cell and solid organ transplantations, iron chelation therapy with deferoxamine, and corticosteroid usage (Hussain et al., 2021; Hoenigl et al., 2022; Kamath et al., 2022). Hyperglycemic state, low oxygen level, high iron levels, an acidic medium, and a decreased phagocytic activity occurred in most COVID-19 patients favor the growth of fungi, particularly Mucorales that use free iron levels in the serum for their pathogenesis (Morales-Franco et al., 2021; Darwish et al., 2022).
The clinical and radiological features of pulmonary and disseminated mucormycosis are non-specific and could overlap with signs associated with COVID-19 making the diagnosis problematic. Additionally, the result of imaging techniques or serological tests from sputum and BAL samples are inconclusive, hence CAM can also be misdiagnosed as CAPA, which is the predominant mould infection in patients with COVID-19-associated acute respiratory distress syndrome (Fathima et al., 2021; Pasternak and Olszanecki, 2021). It is considered that histopathological examination of paraffin-embedded tissue samples is a gold standard for the diagnosis of disease. Species belong to Mucorales have broad, ribbon-like, aseptate hyphae branching at right angles. New molecular diagnostic techniques such as polymerase chain reaction (PCR) may offer an alternative approach allowing fast diagnosis which can lead to an early initiation of therapy (Hammond et al., 2011; Darwish et al., 2022). The risk of all-cause mortality of mucormycosis is high (54%) depending on body site infected, fungus type, and the patient’s overall condition. The lack of routinely available fungal biomarker for mucormycosis results in delayed diagnosis; thus, CAM in patients with known co-morbidities (diabetes, transplantation, malignancies) and medications (steroids) has often fatal outcome (Table 1; Monte Junior et al., 2020; Kanwar et al., 2021; Waizel-Haiat et al., 2021). Co-infections with multiple fungal species also worsen the survival rate of COVID-19 patients that was shown in a case report of combined aspergillosis and mucormycosis (Benhadid-Brahmi et al., 2022).
Essential treatment principles of mucormycosis are control of the underlying disease or risk factor, surgical debridement of necrotic infected tissue, and the implementation of adequate antifungal pharmacotherapy. Surgical intervention prior to the spread of infection to different organs and tissues is crucial in the successful management of mucormycosis as it is associated with significantly better clinical outcomes when combined with early, adequate systemic antifungal therapy (Brunet and Rammaert, 2020). In general, patients will be treated empirically for mucormycosis due to the difficulty of correct diagnosis. However, effective treatment options are limited because Mucorales are naturally resistant to the majority of antifungal agents causing therapeutic failure (Drogari-Apiranthitou et al., 2012; Darwish et al., 2022). First-line treatment with high-dose liposomal amphotericin B is strongly recommended for serious life-threatening mucormycosis since lipid formulations of amphotericin B have less nephrotoxicity than other formulations that particularly advantageous when given in high daily doses in case of orbital-cerebral involvement. Posaconazole and isavuconazole have emerged as second-line or salvage therapy for individuals with impaired renal function. VOR and echinocandins are ineffective. Other alternative strategies against mucormycosis (e.g., combination therapy) should be considered in severe cases (Table 2; Chao et al., 2022; Hoenigl et al., 2022).
The narrow spectrum of activity, adverse effects, drug-drug interactions of currently used antifungals and the high emergence of resistance has triggered the search for new agents with improved safety profile and broad-spectrum antimicrobial activity. Novel antifungals are being currently developed to overcome these limitations especially reducing their toxicity, optimizing pharmacodynamics and pharmacokinetics, improving their formulations, and increasing specificity. Some developments have been focused on agents with new structure for a known target (rezafungin, tetrazoles, ibrexafungerp) or establish entirely novel targets (fosmanogepix, olorofim) (Figure 1; Gintjee et al., 2020; Bouz and Doležal, 2021). In spite of current efforts, antifungal drug resistance remained a major concern thus introducing alternative therapeutic approaches are irrefutable. One of the alternative treatment strategies might be the use of quorum-sensing molecules (e.g., farnesol, tyrosol) or non-antifungal agents in combination with traditional antifungals (Kovács and Majoros, 2020; Rossato et al., 2021). Other potential antimicrobial candidates attracting the attention of researchers are antimicrobial peptides (AMPs). AMPs are host defense peptides playing essential role in innate immune response. AMPs are composed of 15–50 amino acids that are mainly cationic at physiological pH and their amphipathic conformation facilitates the interaction with the negatively charged membrane of microorganisms leading to membrane insertion, destabilization and disruption of the cell (Figure 1). Resistance to AMPs is less likely to emerge because AMPs demonstrate rapid and drastic effect on fungal cell membrane that otherwise evolves slowly (Fernández de Ullivarri et al., 2020; Mookherjee et al., 2020).
Figure 1. Mechanism of action of conventional antifungal drugs, novel agents under development and potential antimicrobial candidates on cellular targets.
The knowledge of antifungal effect of modified AMPs falls away that for the antimicrobial activity of AMPs against pathogenic bacteria, however, some new antifungal peptides offer possible therapeutic alternatives. Duncan et al. evaluated the antifungal activity of a 2-kDa polyarginine peptide (NP339) obtained by a solid-phase synthesis, which was inspired by host defense peptides (Duncan et al., 2021). NP339 showed pronounced antifungal activity against C. albicans as rapid cell destruction was seen after 4 mg/L NP339 (1xMIC) exposure with electron microscopy analysis within 30 min. In contrast, caspofungin and human β-defensin 2 did not demonstrate similar effect after exposure of 1xMIC concentration. NP339 was also active against Aspergillus species including A. fumigatus, A. flavus, and A. niger by lysing biofilm forming cells. Time-kill experiments with C. auris revealed same effectiveness of NP339 than amphotericin B and it was more effective than fluconazole and caspofungin. Further advantage of this molecule is that no cytotoxic effect was observed on human peripheral blood mononuclear cells and the A549 epithelial lung cell line even at the concentration of hundreds of folds higher than the proposed therapeutic dose. Additionally, exogenous peptides are not metabolized in the liver reducing the risk of drug-drug interactions. Although authors did not notice significant effect of NP339 administration in murine model of disseminated candidiasis, nebulized NP339 reduced the fungal burden of the lungs in rodents with invasive pulmonary aspergillosis. Of note, the method of kidney fungal burden evaluation is not optimized for membrane-acting agents since during tissue homogenization the peptide-fungal cell interaction will be disbanded consequently yielding underestimation of peptide activity.
LL-37 is a human cathelicidin-related peptide produced by macrophages, neutrophils, various epithelial cells and natural killer cells. It interacts with cell wall carbohydrates and destabilizes membrane permeability with elevating reactive oxygen species (ROS) causing oxidative stress within the cell. LL-37 proved to be fungicidal against C. auris including resistant or multidrug resistant strains and found synergy when combined with fluconazole in most of the C. auris strains (80%), whereas, synergism was also observed in the presence of LL-37 with amphotericin B and caspofungin in all C. auris strains. Cell count and viability assay was performed as well in order to confirm the fungicidal potential of LL-37. Based on results, LL-37 inhibited the growth and survival of C. auris cells. Nevertheless, further in vivo investigations are needed regarding LL-37 cytotoxicity on mammalian cells to be a potential drug candidate for combating C. auris infections (Rather et al., 2022). Unfortunately, controversial results have been reported for LL-37 activity in case of Aspergillus species (Luo et al., 2019; Ballard et al., 2020), however, cathelicin-based synthesized peptides seem to be promising antifungals against A. fumigatus (van Eijk et al., 2020).
Myxidin, originated from the epidermal mucus of hagfish (Myxine glutinosa L.), was modified (WMR) by Maione et al. (2022) to increase the number of positively charged amino acids in the original sequence. The peptide previously represented antimicrobial activity against Gram-positive and Gram-negative bacteria. According to this activity, in vitro and in vivo studies were carried out to determine the antifungal effect of WMR. Studies tended to investigate WMR mechanism of action revealed that it induces ROS generation resulting cell death via oxidative damage. Although WMR exhibited low antifungal activity for C. albicans, C. auris, and C. glabrata planktonic cells, it had significant anti-biofilm effect by eradication of mature biofilms formed by C. tropicalis, C. parapsilosis, and C. auris. The synergism in combination with fluconazole also promotes the consideration of possible therapeutic application of WMR. Bugli et al. designed lipopeptides starting from the sequence of the amphipathic α-helix of chionodracine by fatty acid acylation with myristic acid (Olivieri et al., 2018; Bugli et al., 2022). Myristoylated peptides showed lower MIC90 values against C. albicans, C. glabrata, C. parapsilosis, C. tropicalis, and C. auris than the native peptides with outstanding antifungal activity of Myr-B against C. auris. Myr-B seemed to be effective 24 h post-infection against multidrug-resistant C. auris in Galleria mellonella model as well at a concentration of 640 μg/mL and was not toxic when testing on primary human fibroblast cell line (FB789).
Secondary fungal infections associated with COVID-19 are an emerging major concern as delayed diagnosis due to similar symptoms of infections and difficulty of identification methods and treatment increase the mortality rate in ICUs especially in patients with underlying diseases. Besides candidiasis, aspergillosis and mucormycosis; fungaemia caused by Cryptococcus and Trichosporon species are also increasingly reported in COVID-19 patients (Ali et al., 2021; Gil et al., 2021). Rapid and reliable identification of fungal pathogens and implementation of strict infection control strategies challenges the medical practices resulting underestimation of fungal co-infection in COVID-19 patients. The dosage and duration of treatment with corticosteroids, immunosuppressants and broad-spectrum antibiotics should be considered carefully as well. Prompt diagnosis along with adequate antifungal management may improve the survival rate of hospitalized COVID-19 patients with fungal co-infections, however, the available therapeutic options are limited and the emergence of resistant or MDR fungal species impels us to search for new alternatives to overcome nosocomial drug-resistant infections in hospital settings. The diversity and broad-spectrum activity of AMPs qualify them as promising candidates for the development of novel antimycotics. The poor pharmacological properties, toxicity and high cost in large-scale production hampers the commercial applications of AMPs. In spite of these disadvantages, there are some promising AMPs currently in pre-clinical or clinical trials including NP339 (Novamycin) and LL-37. Of note, LL-37 involved in the treatment of leg ulcers and it has been not tested against fungal infections (Koo and Seo, 2019). Although further investigations on recently published AMPs are required regarding their distinct mechanism of action and toxicity, modified natural AMPs will be involved in clinical setting in the future facilitated by the rapid development of in silico analyses that help the optimization of peptides.
MD: conceptualization, data collection, and writing the draft manuscript. KB: data collection and revising and editing the manuscript. Both authors have agreed to the published version of the manuscript.
The authors declare that the research was conducted in the absence of any commercial or financial relationships that could be construed as a potential conflict of interest.
All claims expressed in this article are solely those of the authors and do not necessarily represent those of their affiliated organizations, or those of the publisher, the editors and the reviewers. Any product that may be evaluated in this article, or claim that may be made by its manufacturer, is not guaranteed or endorsed by the publisher.
Alanio, A., Dellière, S., Fodil, S., Bretagne, S., and Mégarbane, B. (2020). Prevalence of putative invasive pulmonary aspergillosis in critically ill patients with COVID-19. Lancet Respir. Med. 8, e48–e49. doi: 10.1016/S2213-2600(20)30237-X
Alanio, A., Sitterle, E., Liance, M., Farrugia, C., Foulet, F., Botterel, F., et al. (2011). Low prevalence of resistance to azoles in Aspergillus fumigatus in a French cohort of patients treated for haematological malignancies. J. Antimicrob. Chemother. 66, 371–374. doi: 10.1093/jac/dkq450
Ali, G. A., Husain, A., Salah, H., and Goravey, W. (2021). Trichosporon asahii fungemia and COVID-19 co-infection: an emerging fungal pathogen; case report and review of the literature. IDCases 25:e01244. doi: 10.1016/j.idcr.2021.e01244
Allaw, F., Kara Zahreddine, N., Ibrahim, A., Tannous, J., Taleb, H., Bizri, A. R., et al. (2021). First Candida auris outbreak during a COVID-19 pandemic in a tertiary-care center in Lebanon. Pathogens 10:157. doi: 10.3390/pathogens10020157
Amin, A., Vartanian, A., Poladian, N., Voloshko, A., Yegiazaryan, A., Al-Kassir, A. L., et al. (2021). Root causes of fungal coinfections in COVID-19 infected patients. Infect. Dis. Rep. 13, 1018–1035. doi: 10.3390/idr13040093
Arana, C., Cuevas Ramírez, R. E., Xipell, M., Casals, J., Moreno, A., Herrera, S., et al. (2021). Mucormycosis associated with COVID-19 in two kidney transplant patients. Transpl. Infect. Dis. 23:e13652. doi: 10.1111/tid.13652
Arastehfar, A., Carvalho, A., van de Veerdonk, F. L., Jenks, J. D., Koehler, P., Krause, R., et al. (2020a). COVID-19 associated pulmonary aspergillosis (CAPA)—from immunology to treatment. J. Fungi (Basel) 6:91. doi: 10.3390/jof6020091
Arastehfar, A., Lass-Flörl, C., Garcia-Rubio, R., Daneshnia, F., Ilkit, M., Boekhout, T., et al. (2020b). The quiet and underappreciated rise of drug-resistant invasive fungal pathogens. J. Fungi (Basel) 6:138. doi: 10.3390/jof6030138
Arensman, K., Miller, J. L., Chiang, A., Mai, N., Levato, J., LaChance, E., et al. (2020). Clinical outcomes of patients treated for Candida auris infections in a multisite health system, Illinois, USA. Emerg. Infect. Dis. 26, 876–880. doi: 10.3201/eid2605.191588
Baddley, J. W., Thompson, G. R., Chen, S. C.-A., White, P. L., Johnson, M. D., Nguyen, M. H., et al. (2021). Coronavirus disease 2019–associated invasive fungal infection. Open Forum Infect. Dis. 8:ofab510. doi: 10.1093/ofid/ofab510
Ballard, E., Yucel, R., Melchers, W. J. G., Brown, A. J. P., Verweij, P. E., and Warris, A. (2020). Antifungal activity of antimicrobial peptides and proteins against Aspergillus fumigatus. J. Fungi (Basel) 6:65. doi: 10.3390/jof6020065
Barnes, P. D., and Marr, K. A. (2006). Aspergillosis: spectrum of disease, diagnosis, and treatment. Infect. Dis. Clin. N. Am. 20, 545–561. doi: 10.1016/j.idc.2006.06.001
Bartoletti, M., Pascale, R., Cricca, M., Rinaldi, M., Maccaro, A., Bussini, L., et al. (2021). Epidemiology of invasive pulmonary aspergillosis among intubated patients with COVID-19: a prospective study. Clin. Infect. Dis. 73, e3606–e3614. doi: 10.1093/cid/ciaa1065
Bellanger, A.-P., Navellou, J.-C., Lepiller, Q., Brion, A., Brunel, A.-S., Millon, L., et al. (2021). Mixed mold infection with Aspergillus fumigatus and Rhizopus microsporus in a severe acute respiratory syndrome Coronavirus 2 (SARS-CoV-2) patient. Infect. Dis. Now 51, 633–635. doi: 10.1016/j.idnow.2021.01.010
Benhadid-Brahmi, Y., Hamane, S., Soyer, B., Mebazaa, A., Alanio, A., Chousterman, B., et al. (2022). COVID-19-associated mixed mold infection: a case report of aspergillosis and mucormycosis and a literature review. J. Med. Mycol. 32:101231. doi: 10.1016/j.mycmed.2021.101231
Bongomin, F., Gago, S., Oladele, R., and Denning, D. (2017). Global and multi-national prevalence of fungal diseases—estimate precision. J. Fungi (Basel) 3:57. doi: 10.3390/jof3040057
Borman, A. M., Palmer, M. D., Fraser, M., Patterson, Z., Mann, C., Oliver, D., et al. (2020). COVID-19-associated invasive aspergillosis: data from the UK national mycology reference laboratory. J. Clin. Microbiol. 59:e02136-20. doi: 10.1128/JCM.02136-20
Bouz, G., and Doležal, M. (2021). Advances in antifungal drug development: an up-to-date mini review. Pharmaceuticals 14:1312. doi: 10.3390/ph14121312
Brunet, K., and Rammaert, B. (2020). Mucormycosis treatment: recommendations, latest advances, and perspectives. J. Mycol. Méd. 30:101007. doi: 10.1016/j.mycmed.2020.101007
Bugli, F., Massaro, F., Buonocore, F., Saraceni, P. R., Borocci, S., Ceccacci, F., et al. (2022). Design and characterization of myristoylated and non-myristoylated peptides effective against Candida spp. clinical isolates. Int. J. Mol. Sci. 23:2164. doi: 10.3390/ijms23042164
Buil, J. B., van Zanten, A. R. H., Bentvelsen, R. G., Rijpstra, T. A., Goorhuis, B., van der Voort, S., et al. (2021). Case series of four secondary mucormycosis infections in COVID-19 patients, the Netherlands, December 2020 to May 2021. Euro Surveill. 26:2100510. doi: 10.2807/1560-7917.ES.2021.26.23.2100510
Cadena, J., Thompson, G. R., and Patterson, T. F. (2021). Aspergillosis. Infect. Dis. Clin. N. Am. 35, 415–434. doi: 10.1016/j.idc.2021.03.008
Cadnum, J. L., Shaikh, A. A., Piedrahita, C. T., Jencson, A. L., Larkin, E. L., Ghannoum, M. A., et al. (2018). Relative resistance of the emerging fungal pathogen Candida auris and other Candida species to killing by ultraviolet light. Infect. Control Hosp. Epidemiol. 39, 94–96. doi: 10.1017/ice.2017.239
Casalini, G., Giacomelli, A., Ridolfo, A., Gervasoni, C., and Antinori, S. (2021). Invasive fungal infections complicating COVID-19: a narrative review. JoF 7:921. doi: 10.3390/jof7110921
Castro, L. A., Álvarez, M. I., Giusiano, G., and Martínez, E. (2019). Candida auris infection in the central catheter of a patient without sepsis symptoms. Colomb. Med. 50, 293–298. doi: 10.25100/cm.v50i4.4248
Centers for Disease Control and Prevention (2020). Antifungal Susceptibility Testing and Interpretation. Atlanta, GA: Centers for Disease Control and Prevention.
Centers for Disease Control and Prevention (2021). Recommendations for Treatment of Candida auris Infections. Atlanta, GA: Centers for Disease Control and Prevention.
Chaabane, F., Graf, A., Jequier, L., and Coste, A. T. (2019). Review on antifungal resistance mechanisms in the emerging pathogen Candida auris. Front. Microbiol. 10:2788. doi: 10.3389/fmicb.2019.02788
Chakrabarti, A., and Singh, S. (2020). Multidrug-resistant Candida auris: an epidemiological review. Expert Rev Anti Infect. Ther. 18, 551–562. doi: 10.1080/14787210.2020.1750368
Chakrabarti, A., and Sood, P. (2021). On the emergence, spread and resistance of Candida auris: host, pathogen and environmental tipping points. J. Med. Microbiol. 70:001318. doi: 10.1099/jmm.0.001318
Chao, C.-M., Lai, C.-C., and Yu, W.-L. (2022). COVID-19 associated mucormycosis – An emerging threat. J. Microbiol. Immunol. Infect. 55, 183–190. doi: 10.1016/j.jmii.2021.12.007
Chow, N. A., de Groot, T., Badali, H., Abastabar, M., Chiller, T. M., and Meis, J. F. (2019). Potential fifth clade of Candida auris, Iran, 2018. Emerg. Infect. Dis. 25, 1780–1781. doi: 10.3201/eid2509.190686
Chow, N. A., Muñoz, J. F., Gade, L., Berkow, E. L., Li, X., Welsh, R. M., et al. (2020). Tracing the evolutionary history and global expansion of Candida auris using population genomic analyses. mBio 11:e03364-19. doi: 10.1128/mBio.03364-19
Chowdhary, A., Prakash, A., Sharma, C., Kordalewska, M., Kumar, A., Sarma, S., et al. (2018). A multicentre study of antifungal susceptibility patterns among 350 Candida auris isolates (2009–17) in India: role of the ERG11 and FKS1 genes in azole and echinocandin resistance. J. Antimicrob. Chemother. 73, 891–899. doi: 10.1093/jac/dkx480
Chowdhary, A., Tarai, B., Singh, A., and Sharma, A. (2020). Multidrug-resistant Candida auris infections in critically Ill coronavirus disease patients, India, April–July 2020. Emerg. Infect. Dis. 26, 2694–2696. doi: 10.3201/eid2611.203504
Cornely, O. A., Alastruey-Izquierdo, A., Arenz, D., Chen, S. C. A., Dannaoui, E., Hochhegger, B., et al. (2019). Global guideline for the diagnosis and management of mucormycosis: an initiative of the European Confederation of Medical Mycology in cooperation with the Mycoses Study Group Education and Research Consortium. Lancet Infect. Dis. 19, e405–e421. doi: 10.1016/S1473-3099(19)30312-3
Danion, F., Letscher-Bru, V., Guitard, J., Sitbon, K., Dellière, S., Angoulvant, A., et al. (2022). Coronavirus disease 2019-associated mucormycosis in France: a rare but deadly complication. Open Forum Infect. Dis. 9:ofab566. doi: 10.1093/ofid/ofab566
Darwish, R. M., AlMasri, M., and Al-Masri, M. M. (2022). Mucormycosis: the hidden and forgotten disease. J. Appl. Microbiol. 132, 4042–4057. doi: 10.1111/jam.15487
de Almeida, J. N., Francisco, E. C., Hagen, F., Brandão, I. B., Pereira, F. M., Presta Dias, P. H., et al. (2021). Emergence of Candida auris in Brazil in a COVID-19 intensive care unit. J. Fungi (Basel) 7:220. doi: 10.3390/jof7030220
Dellière, S., Dudoignon, E., Fodil, S., Voicu, S., Collet, M., Oillic, P.-A., et al. (2021). Risk factors associated with COVID-19-associated pulmonary aspergillosis in ICU patients: a French multicentric retrospective cohort. Clin. Microbiol. Infect. 27, 790.e1–790.e5.
Drogari-Apiranthitou, M., Mantopoulou, F.-D., Skiada, A., Kanioura, L., Grammatikou, M., Vrioni, G., et al. (2012). In vitro antifungal susceptibility of filamentous fungi causing rare infections: synergy testing of amphotericin B, posaconazole and anidulafungin in pairs. J. Antimicrob. Chemother. 67, 1937–1940. doi: 10.1093/jac/dks137
Duncan, V., Smith, D., Simpson, L., Lovie, E., Katvars, L., Berge, L., et al. (2021). Preliminary characterization of NP339, a novel polyarginine peptide with broad antifungal activity. Antimicrob. Agents Chemother. 65:e02345-20. doi: 10.1128/AAC.02345-20
Eyre, D. W., Sheppard, A. E., Madder, H., Moir, I., Moroney, R., Quan, T. P., et al. (2018). A Candida auris outbreak and its control in an intensive care setting. N. Engl. J. Med. 379, 1322–1331. doi: 10.1056/NEJMoa1714373
Ezeokoli, O. T., Gcilitshana, O., and Pohl, C. H. (2021). Risk factors for fungal co-infections in critically Ill COVID-19 patients, with a focus on immunosuppressants. J. Fungi (Basel) 7:545. doi: 10.3390/jof7070545
Fasciana, T., Cortegiani, A., Ippolito, M., Giarratano, A., Di Quattro, O., Lipari, D., et al. (2020). Candida auris: an overview of how to screen, detect, test and control this emerging pathogen. Antibiotics 9:778. doi: 10.3390/antibiotics9110778
Fathima, A. S., Mounika, V. L., Kumar, V. U., Gupta, A. K., Garapati, P., Ravichandiran, V., et al. (2021). Mucormycosis: a triple burden in patients with diabetes during COVID-19 Pandemic. Health Sci. Rev. 1:100005. doi: 10.1016/j.hsr.2021.100005
Fernández de Ullivarri, M., Arbulu, S., Garcia-Gutierrez, E., and Cotter, P. D. (2020). Antifungal peptides as therapeutic agents. Front. Cell. Infect. Microbiol. 10:105. doi: 10.3389/fcimb.2020.00105
Feys, S., Almyroudi, M. P., Braspenning, R., Lagrou, K., Spriet, I., Dimopoulos, G., et al. (2021). A visual and comprehensive review on COVID-19-associated pulmonary aspergillosis (CAPA). J. Fungi (Basel) 7:1067. doi: 10.3390/jof7121067
Flikweert, A. W., Grootenboers, M. J. J. H., Yick, D. C. Y., du Mée, A. W. F., van der Meer, N. J. M., Rettig, T. C. D., et al. (2020). Late histopathologic characteristics of critically ill COVID-19 patients: different phenotypes without evidence of invasive aspergillosis, a case series. J. Crit. Care 59, 149–155. doi: 10.1016/j.jcrc.2020.07.002
Friedman, D. Z. P., and Schwartz, I. S. (2019). Emerging fungal infections: new patients, new patterns, and new pathogens. J. Fungi (Basel) 5:67. doi: 10.3390/jof5030067
Garcia-Bustos, V., Cabanero-Navalon, M. D., Ruiz-Saurí, A., Ruiz-Gaitán, A. C., Salavert, M., Tormo, M. Á, et al. (2021). What do we know about Candida auris? State of the art, knowledge gaps, and future directions. Microorganisms 9:2177. doi: 10.3390/microorganisms9102177
Garcia-Rubio, R., Cuenca-Estrella, M., and Mellado, E. (2017). Triazole resistance in Aspergillus species: an emerging problem. Drugs 77, 599–613. doi: 10.1007/s40265-017-0714-4
Ghelfenstein-Ferreira, T., Saade, A., Alanio, A., Bretagne, S., Araujo de Castro, R., Hamane, S., et al. (2021). Recovery of a triazole-resistant Aspergillus fumigatus in respiratory specimen of COVID-19 patient in ICU – A case report. Med. Mycol. Case Rep. 31, 15–18. doi: 10.1016/j.mmcr.2020.06.006
Gil, Y., Gil, Y. D., and Markou, T. (2021). The emergence of cryptococcemia in COVID-19 infection: a case report. Cureus 13:e19761. doi: 10.7759/cureus.19761
Gintjee, T. J., Donnelley, M. A., and Thompson, G. R. (2020). Aspiring antifungals: review of current antifungal pipeline developments. J. Fungi (Basel) 6:28. doi: 10.3390/jof6010028
Gregoire, E., Pirotte, B. F., Moerman, F., Altdorfer, A., Gaspard, L., Firre, E., et al. (2021). Incidence and risk factors of COVID-19-associated pulmonary aspergillosis in intensive care unit—a monocentric retrospective observational study. Pathogens 10:1370. doi: 10.3390/pathogens10111370
Guaraldi, G., Meschiari, M., Cozzi-Lepri, A., Milic, J., Tonelli, R., Menozzi, M., et al. (2020). Tocilizumab in patients with severe COVID-19: a retrospective cohort study. Lancet Rheumatol. 2, e474–e484. doi: 10.1016/S2665-9913(20)30173-9
Guzmán-Castro, S., Chora-Hernandez, L. D., Trujillo-Alonso, G., Calvo-Villalobos, I., Sanchez-Rangel, A., Ferrer-Alpuin, E., et al. (2022). COVID-19–associated mucormycosis, diabetes and steroid therapy: experience in a single centre in Western Mexico. Mycoses 65, 65–70. doi: 10.1111/myc.13383
Haeley, K. R., and Perlin, D. S. (2018). Fungal resistance to echinocandins and the MDR phenomenon in Candida glabrata. J. Fungi 4:105. doi: 10.3390/jof4030105
Hammond, S. P., Bialek, R., Milner, D. A., Petschnigg, E. M., Baden, L. R., and Marty, F. M. (2011). Molecular methods to improve diagnosis and identification of mucormycosis. J. Clin. Microbiol. 49, 2151–2153. doi: 10.1128/JCM.00256-11
Hanley, B., Naresh, K. N., Roufosse, C., Nicholson, A. G., Weir, J., Cooke, G. S., et al. (2020). Histopathological findings and viral tropism in UK patients with severe fatal COVID-19: a post-mortem study. Lancet Microbe 1, e245–e253. doi: 10.1016/S2666-5247(20)30115-4
Hoenigl, M., Seidel, D., Carvalho, A., Rudramurthy, S. M., Arastehfar, A., Gangneux, J.-P., et al. (2022). The emergence of COVID-19 associated mucormycosis: a review of cases from 18 countries. Lancet Microbe S2666524721002378. doi: 10.1016/S2666-5247(21)00237-8 [Epub ahead of print].
Hohl, T. M., and Feldmesser, M. (2007). Aspergillus fumigatus: principles of pathogenesis and host defense. Eukaryot. Cell 6, 1953–1963. doi: 10.1128/EC.00274-07
Hussain, S., Baxi, H., Riad, A., Klugarová, J., Pokorná, A., Slezáková, S., et al. (2021). COVID-19-associated mucormycosis (CAM): an updated evidence mapping. Int. J. Environ. Res. Public Health 18:10340. doi: 10.3390/ijerph181910340
Jabeen, K., Mal, P. B., Tharwani, A., Hashmi, M., and Farooqi, J. (2020). Persistence of Candida auris on latex and nitrile gloves with transmission to sterile urinary catheters‡. Med. Mycol. 58, 128–132. doi: 10.1093/mmy/myz033
Jiang, Z., Chen, S., Zhu, Q., Xiao, Y., and Qu, J. (2022). COVID-19-associated pulmonary aspergillosis in a tertiary care center in Shenzhen City. J. Infect. Public Health 15, 222–227. doi: 10.1016/j.jiph.2021.12.015
Kamath, S., Kumar, M., Sarkar, N., Ahmed, T., and Sunder, A. (2022). Study of profile of mucormycosis during the second wave of COVID-19 in a tertiary care hospital. Cureus 14:e21054. doi: 10.7759/cureus.21054
Kanwar, A., Jordan, A., Olewiler, S., Wehberg, K., Cortes, M., and Jackson, B. R. (2021). A fatal case of Rhizopus azygosporus pneumonia following COVID-19. J. Fungi (Basel) 7:174. doi: 10.3390/jof7030174
Khan, N., Gutierrez, C. G., Martinez, D. V., and Proud, K. C. (2020). A case report of COVID-19 associated pulmonary mucormycosis. Arch. Clin. Cases 07, 46–51. doi: 10.22551/2020.28.0703.10172
Khatri, A., Chang, K.-M., Berlinrut, I., and Wallach, F. (2021). Mucormycosis after Coronavirus disease 2019 infection in a heart transplant recipient – Case report and review of literature. J. Med. Mycol. 31:101125. doi: 10.1016/j.mycmed.2021.101125
Khorramdelazad, H., Kazemi, M. H., Najafi, A., Keykhaee, M., Zolfaghari Emameh, R., and Falak, R. (2021). Immunopathological similarities between COVID-19 and influenza: investigating the consequences of Co-infection. Microb. Pathog. 152:104554. doi: 10.1016/j.micpath.2020.104554
Kilburn, S., Innes, G., Quinn, M., Southwick, K., Ostrowsky, B., Greenko, J. A., et al. (2022). Antifungal resistance trends of Candida auris clinical isolates in New York and New Jersey from 2016 to 2020. Antimicrob. Agents Chemother. 66:e02242-21. doi: 10.1128/aac.02242-21
Koehler, P., Bassetti, M., Chakrabarti, A., Chen, S. C. A., Colombo, A. L., Hoenigl, M., et al. (2021). Defining and managing COVID-19-associated pulmonary aspergillosis: the 2020 ECMM/ISHAM consensus criteria for research and clinical guidance. Lancet Infect. Dis. 21, e149–e162. doi: 10.1016/S1473-3099(20)30847-1
Koehler, P., Bassetti, M., Kochanek, M., Shimabukuro-Vornhagen, A., and Cornely, O. A. (2019). Intensive care management of influenza-associated pulmonary aspergillosis. Clin. Microbiol. Infect. 25, 1501–1509. doi: 10.1016/j.cmi.2019.04.031
Koehler, P., Cornely, O. A., Böttiger, B. W., Dusse, F., Eichenauer, D. A., Fuchs, F., et al. (2020). COVID-19 associated pulmonary aspergillosis. Mycoses 63, 528–534. doi: 10.1111/myc.13096
Koo, H. B., and Seo, J. (2019). Antimicrobial peptides under clinical investigation. Pept. Sci. 111:e24122. doi: 10.1002/pep2.24122
Kovács, R., and Majoros, L. (2020). Fungal quorum-sensing molecules: a review of their antifungal effect against Candida biofilms. J. Fungi (Basel) 6:99. doi: 10.3390/jof6030099
Kula, B. E., Clancy, C. J., Hong Nguyen, M., and Schwartz, I. S. (2021). Invasive mould disease in fatal COVID-19: a systematic review of autopsies. Lancet. Microbe. 2, e405–e414. doi: 10.1016/S2666-5247(21)00091-4
Kurt, A. F., Kuskucu, M. A., Balkan, I. I., Baris, A., Yazgan, Z., Serife, O. A., et al. (2021). Candida auris Fungemia and a local spread taken under control with infection control measures: first report from Turkey. Indian J. Med. Microbiol. 39, 228–230. doi: 10.1016/j.ijmmb.2021.03.007
Lahmer, T., Kriescher, S., Herner, A., Rothe, K., Spinner, C. D., Schneider, J., et al. (2021). Invasive pulmonary aspergillosis in critically ill patients with severe COVID-19 pneumonia: results from the prospective AspCOVID-19 study. PLoS One 16:e0238825. doi: 10.1371/journal.pone.0238825
Lamoth, F., Glampedakis, E., Boillat-Blanco, N., Oddo, M., and Pagani, J.-L. (2020). Incidence of invasive pulmonary aspergillosis among critically ill COVID-19 patients. Clin. Microbiol. Infect. 26, 1706–1708. doi: 10.1016/j.cmi.2020.07.010
Lamoth, F., Lewis, R. E., Walsh, T. J., and Kontoyiannis, D. P. (2021). Navigating the uncertainties of COVID-19–associated aspergillosis: a comparison with influenza-associated aspergillosis. J. Infect. Dis. 224, 1631–1640. doi: 10.1093/infdis/jiab163
Latgé, J.-P., and Chamilos, G. (2019). Aspergillus fumigatus and Aspergillosis in 2019. Clin. Microbiol. Rev. 33:e00140-18. doi: 10.1128/CMR.00140-18
Lestrade, P. P. A., Buil, J. B., van der Beek, M. T., Kuijper, E. J., van Dijk, K., Kampinga, G. A., et al. (2020). Paradoxal trends in azole-resistant Aspergillus fumigatus in a national multicenter surveillance program, the Netherlands, 2013–2018. Emerg. Infect. Dis. 26, 1447–1455. doi: 10.3201/eid2607.200088
Lockhart, S. R., Etienne, K. A., Vallabhaneni, S., Farooqi, J., Chowdhary, A., Govender, N. P., et al. (2017). Simultaneous emergence of multidrug-resistant Candida auris on 3 continents confirmed by whole-genome sequencing and epidemiological analyses. Clin. Infect. Dis. 64, 134–140. doi: 10.1093/cid/ciw691
Luo, X.-L., Li, J.-X., Huang, H.-R., Duan, J.-L., Dai, R.-X., Tao, R.-J., et al. (2019). LL37 inhibits Aspergillus fumigatus infection via directly binding to the fungus and preventing excessive inflammation. Front. Immunol. 10:283. doi: 10.3389/fimmu.2019.00283
Machado, M., Estévez, A., Sánchez-Carrillo, C., Guinea, J., Escribano, P., Alonso, R., et al. (2022). Incidence of candidemia is higher in COVID-19 versus Non-COVID-19 patients, but not driven by intrahospital transmission. JoF 8:305. doi: 10.3390/jof8030305
Magnasco, L., Mikulska, M., Giacobbe, D. R., Taramasso, L., Vena, A., Dentone, C., et al. (2021). Spread of carbapenem-resistant gram-negatives and Candida auris during the COVID-19 pandemic in critically Ill patients: one step back in antimicrobial stewardship? Microorganisms 9:95. doi: 10.3390/microorganisms9010095
Maione, A., Bellavita, R., de Alteriis, E., Galdiero, S., Albarano, L., La Pietra, A. L., et al. (2022). WMR peptide as antifungal and antibiofilm against Albicans and non-albicans Candida species: shreds of evidence on the mechanism of action. Int. J. Mol. Sci. 23:2151. doi: 10.3390/ijms23042151
McCreary, E. K., and Pogue, J. M. (2020). Coronavirus disease 2019 treatment: a review of early and emerging options. Open Forum Infect. Dis. 7:ofaa105. doi: 10.1093/ofid/ofaa105
Meijer, E. F. J., Dofferhoff, A. S. M., Hoiting, O., and Meis, J. F. (2021). COVID-19–associated pulmonary aspergillosis: a prospective single-center dual case series. Mycoses 64, 457–464. doi: 10.1111/myc.13254
Meijer, E. F. J., Dofferhoff, A. S. M., Hoiting, O., Buil, J. B., and Meis, J. F. (2020). Azole-resistant COVID-19-associated pulmonary aspergillosis in an immunocompetent host: a case report. J. Fungi (Basel) 6:79. doi: 10.3390/jof6020079
Meis, J. F., Chowdhary, A., Rhodes, J. L., Fisher, M. C., and Verweij, P. E. (2016). Clinical implications of globally emerging azole resistance in Aspergillus fumigatus. Philos. Trans. R. Soc. B 371:20150460. doi: 10.1098/rstb.2015.0460
Mohamed, A., Hassan, T., Trzos-Grzybowska, M., Thomas, J., Quinn, A., O’Sullivan, M., et al. (2021). Multi-triazole-resistant Aspergillus fumigatus and SARS-CoV-2 co-infection: a lethal combination. Med. Mycol. Case Rep. 31, 11–14. doi: 10.1016/j.mmcr.2020.06.005
Monte Junior, E. S. D., Santos, M. E. L. D., Ribeiro, I. B., Luz, G. O., Baba, E. R., Hirsch, B. S., et al. (2020). Rare and fatal gastrointestinal Mucormycosis (Zygomycosis) in a COVID-19 patient: a case report. Clin. Endosc. 53, 746–749. doi: 10.5946/ce.2020.180
Mookherjee, N., Anderson, M. A., Haagsman, H. P., and Davidson, D. J. (2020). Antimicrobial host defence peptides: functions and clinical potential. Nat. Rev. Drug Discov. 19, 311–332. doi: 10.1038/s41573-019-0058-8
Morales-Franco, B., Nava-Villalba, M., Medina-Guerrero, E. O., Sánchez-Nuño, Y. A., Davila-Villa, P., Anaya-Ambriz, E. J., et al. (2021). Host-pathogen molecular factors contribute to the pathogenesis of Rhizopus spp. in diabetes mellitus. Curr. Trop. Med. Rep. 8, 6–17. doi: 10.1007/s40475-020-00222-1
Nasir, N., Farooqi, J., Mahmood, S. F., and Jabeen, K. (2020). COVID-19-associated pulmonary aspergillosis (CAPA) in patients admitted with severe COVID-19 pneumonia: an observational study from Pakistan. Mycoses 63, 766–770. doi: 10.1111/myc.13135
Nobrega de Almeida, J., Brandão, I. B., Francisco, E. C., Almeida, S. L. R., Oliveira Dias, P., Pereira, F. M., et al. (2021). Axillary digital thermometers uplifted a multidrug-susceptible Candida auris outbreak among COVID-19 patients in Brazil. Mycoses 64, 1062–1072. doi: 10.1111/myc.13320
Nucci, M., Barreiros, G., Guimarães, L. F., Deriquehem, V. A. S., Castiñeiras, A. C., and Nouér, S. A. (2021). Increased incidence of candidemia in a tertiary care hospital with the COVID-19 pandemic. Mycoses 64, 152–156. doi: 10.1111/myc.13225
Olivieri, C., Bugli, F., Menchinelli, G., Veglia, G., Buonocore, F., Scapigliati, G., et al. (2018). Design and characterization of chionodracine-derived antimicrobial peptides with enhanced activity against drug-resistant human pathogens. RSC Adv. 8, 41331–41346. doi: 10.1039/C8RA08065H
Pandya, N., Cag, Y., Pandak, N., Pekok, A. U., Poojary, A., Ayoade, F., et al. (2021). International multicentre study of Candida auris infections. J. Fungi (Basel) 7:878. doi: 10.3390/jof7100878
Parente-Rocha, J. A., Bailão, A. M., Amaral, A. C., Taborda, C. P., Paccez, J. D., Borges, C. L., et al. (2017). Antifungal resistance, metabolic routes as drug targets, and new antifungal agents: an overview about endemic dimorphic fungi. Mediators Inflamm. 2017:9870679. doi: 10.1155/2017/9870679
Pasero, D., Sanna, S., Liperi, C., Piredda, D., Branca, G. P., Casadio, L., et al. (2021). A challenging complication following SARS-CoV-2 infection: a case of pulmonary mucormycosis. Infection 49, 1055–1060. doi: 10.1007/s15010-020-01561-x
Pasternak, M., and Olszanecki, R. (2021). Mucormycosis in head and neck area — the emerging health problem in COVID-19 pandemic. The perspective of a dental practitioner. Folia Med. Cracov. 61, 117–127. doi: 10.24425/FMC.2021.137228
Pfaller, M. A., Diekema, D. J., Turnidge, J. D., Castanheira, M., and Jones, R. N. (2019). Twenty years of the SENTRY antifungal surveillance program: results for Candida species from 1997–2016. Open Forum Infect. Dis. 6, S79–S94. doi: 10.1093/ofid/ofy358
Prestel, C., Anderson, E., Forsberg, K., Lyman, M., de Perio, M. A., Kuhar, D., et al. (2021). Candida auris outbreak in a COVID-19 specialty care unit — Florida, July–August 2020. MMWR Morb. Mortal. Wkly. Rep. 70, 56–57. doi: 10.15585/mmwr.mm7002e3
Rabagliati, R., Rodríguez, N., Núñez, C., Huete, A., Bravo, S., and Garcia, P. (2021). COVID-19–associated mold infection in critically Ill Patients, Chile. Emerg. Infect. Dis. 27, 1454–1456. doi: 10.3201/eid2705.204412
Rather, I. A., Sabir, J. S. M., Asseri, A. H., and Ali, S. (2022). Antifungal activity of human cathelicidin LL-37, a membrane disrupting peptide, by triggering oxidative stress and cell cycle arrest in Candida auris. J. Fungi (Basel) 8:204. doi: 10.3390/jof8020204
Rhodes, J., and Fisher, M. C. (2019). Global epidemiology of emerging Candida auris. Curr. Opin. Microbiol. 52, 84–89. doi: 10.1016/j.mib.2019.05.008
Romero, M., Messina, F., Marin, E., Arechavala, A., Depardo, R., Walker, L., et al. (2019). Antifungal resistance in clinical isolates of Aspergillus spp.: when local epidemiology breaks the norm. J. Fungi (Basel) 5:41. doi: 10.3390/jof5020041
Rossato, L., Camargo dos Santos, M., Vitale, R. G., Hoog, S., and Ishida, K. (2021). Alternative treatment of fungal infections: synergy with non-antifungal agents. Mycoses 64, 232–244. doi: 10.1111/myc.13203
Ruiz-Gaitán, A., Moret, A. M., Tasias-Pitarch, M., Aleixandre-López, A. I., Martínez-Morel, H., Calabuig, E., et al. (2018). An outbreak due to Candida auris with prolonged colonisation and candidaemia in a tertiary care European hospital. Mycoses 61, 498–505. doi: 10.1111/myc.12781
Rutala, W. A., Kanamori, H., Gergen, M. F., Sickbert-Bennett, E. E., and Weber, D. J. (2019). Susceptibility of Candida auris and Candida albicans to 21 germicides used in healthcare facilities. Infect. Control Hosp. Epidemiol. 40, 380–382. doi: 10.1017/ice.2019.1
Rutsaert, L., Steinfort, N., Van Hunsel, T., Bomans, P., Naesens, R., Mertes, H., et al. (2020). COVID-19-associated invasive pulmonary aspergillosis. Ann. Intensive Care 10:71. doi: 10.1186/s13613-020-00686-4
Satoh, K., Makimura, K., Hasumi, Y., Nishiyama, Y., Uchida, K., and Yamaguchi, H. (2009). Candida auris sp. nov., a novel ascomycetous yeast isolated from the external ear canal of an inpatient in a Japanese hospital. Microbiol. Immunol. 53, 41–44. doi: 10.1111/j.1348-0421.2008.00083.x
Schauwvlieghe, A. F. A. D., Rijnders, B. J. A., Philips, N., Verwijs, R., Vanderbeke, L., Van Tienen, C., et al. (2018). Invasive aspergillosis in patients admitted to the intensive care unit with severe influenza: a retrospective cohort study. Lancet Respir. Med. 6, 782–792. doi: 10.1016/S2213-2600(18)30274-1
Seidel, D., Simon, M., Sprute, R., Lubnow, M., Evert, K., Speer, C., et al. (2022). Results from a national survey on COVID-19-associated mucormycosis in Germany: 13 patients from six tertiary hospitals. Mycoses 65, 103–109. doi: 10.1111/myc.13379
Shakir, M., Maan, M. H. A., and Waheed, S. (2021). Mucormycosis in a patient with COVID-19 with uncontrolled diabetes. BMJ Case Rep. 14:e245343. doi: 10.1136/bcr-2021-245343
Shaukat, A., Al Ansari, N., Al Wali, W., Karic, E., El Madhoun, I., Mitwally, H., et al. (2021). Experience of treating Candida auris cases at a general hospital in the state of Qatar. IDCases 23:e01007. doi: 10.1016/j.idcr.2020.e01007
Skiada, A., Pavleas, I., and Drogari-Apiranthitou, M. (2020). Epidemiology and diagnosis of mucormycosis: an update. J. Fungi (Basel) 6:265. doi: 10.3390/jof6040265
Tabarsi, P., Khalili, N., Pourabdollah, M., Sharifynia, S., Safavi Naeini, A., Ghorbani, J., et al. (2021). Case report: COVID-19-associated Rhinosinusitis Mucormycosis caused by Rhizopus arrhizus: a rare but potentially fatal infection occurring after treatment with corticosteroids. Am. J. Trop. Med. Hyg. 105, 449–453. doi: 10.4269/ajtmh.21-0359
Takazono, T., Mukae, H., Izumikawa, K., Hasegawa, N., and Yokoyama, A. (2021). COVID-19 associated pulmonary aspergillosis: a nationwide survey by the Japanese Respiratory Society. ERJ Open Res. 7:00402-2021. doi: 10.1183/23120541.00402-2021
Taori, S. K., Khonyongwa, K., Hayden, I., Athukorala, G. D. A., Letters, A., Fife, A., et al. (2019). Candida auris outbreak: mortality, interventions and cost of sustaining control. J. Infect. 79, 601–611. doi: 10.1016/j.jinf.2019.09.007
Tio, S. Y., Williams, E., Worth, L. J., Deane, A. M., Bond, K., Slavin, M. A., et al. (2021). Invasive pulmonary aspergillosis in critically ill patients with COVID-19 in Australia: implications for screening and treatment. Intern. Med. J. 51, 2129–2132. doi: 10.1111/imj.15602
van Arkel, A. L. E., Rijpstra, T. A., Belderbos, H. N. A., van Wijngaarden, P., Verweij, P. E., and Bentvelsen, R. G. (2020). COVID-19–associated pulmonary aspergillosis. Am. J. Respir. Crit. Care Med. 202, 132–135. doi: 10.1164/rccm.202004-1038LE
van Eijk, M., Boerefijn, S., Cen, L., Rosa, M., Morren, M. J. H., van der Ent, C. K., et al. (2020). Cathelicidin-inspired antimicrobial peptides as novel antifungal compounds. Med. Mycol. 58, 1073–1084. doi: 10.1093/mmy/myaa014
Verweij, P. E., Zhang, J., Debets, A. J. M., Meis, J. F., van de Veerdonk, F. L., Schoustra, S. E., et al. (2016). In-host adaptation and acquired triazole resistance in Aspergillus fumigatus: a dilemma for clinical management. Lancet Infect. Dis. 16, e251–e260. doi: 10.1016/S1473-3099(16)30138-4
Villanueva-Lozano, H., Treviño-Rangel, R. J., González, G. M., Ramírez-Elizondo, M. T., Lara-Medrano, R., Aleman-Bocanegra, M. C., et al. (2021). Outbreak of Candida auris infection in a COVID-19 hospital in Mexico. Clin. Microbiol. Infect. 27, 813–816. doi: 10.1016/j.cmi.2020.12.030
Waizel-Haiat, S., Guerrero-Paz, J. A., Sanchez-Hurtado, L., Calleja-Alarcon, S., and Romero-Gutierrez, L. (2021). A case of fatal rhino-orbital mucormycosis associated with new onset diabetic ketoacidosis and COVID-19. Cureus 13:e13163. doi: 10.7759/cureus.13163
Welsh, R. M., Bentz, M. L., Shams, A., Houston, H., Lyons, A., Rose, L. J., et al. (2017). Survival, persistence, and isolation of the emerging multidrug-resistant pathogenic yeast Candida auris on a plastic health care surface. J. Clin. Microbiol. 55, 2996–3005. doi: 10.1128/JCM.00921-17
Welsh, R. M., Sexton, D. J., Forsberg, K., Vallabhaneni, S., and Litvintseva, A. (2019). Insights into the unique nature of the East Asian clade of the emerging pathogenic yeast Candida auris. J. Clin. Microbiol. 57:e00007-19. doi: 10.1128/JCM.00007-19
World Health Organization (2021). Living Guidance for Clinical Management of COVID-19. Geneva: World Health Organization.
Yang, X., Chen, W., Liang, T., Tan, J., Liu, W., Sun, Y., et al. (2021). A 20-year antifungal susceptibility surveillance (From 1999 to 2019) for Aspergillus spp. and proposed epidemiological cutoff values for Aspergillus fumigatus and Aspergillus flavus: a study in a tertiary hospital in China. Front. Microbiol. 12:680884. doi: 10.3389/fmicb.2021.680884
Keywords: fungal co-infections, Candida auris, COVID-19-associated aspergillosis, antimicrobial peptide, COVID-19-associated mucormycosis
Citation: Domán M and Bányai K (2022) COVID-19-Associated Fungal Infections: An Urgent Need for Alternative Therapeutic Approach? Front. Microbiol. 13:919501. doi: 10.3389/fmicb.2022.919501
Received: 13 April 2022; Accepted: 19 May 2022;
Published: 09 June 2022.
Edited by:
Uwe Groß, University Medical Center Göttingen, GermanyReviewed by:
Carolina Henritta Pohl, University of the Free State, South AfricaCopyright © 2022 Domán and Bányai. This is an open-access article distributed under the terms of the Creative Commons Attribution License (CC BY). The use, distribution or reproduction in other forums is permitted, provided the original author(s) and the copyright owner(s) are credited and that the original publication in this journal is cited, in accordance with accepted academic practice. No use, distribution or reproduction is permitted which does not comply with these terms.
*Correspondence: Marianna Domán, ZG9tYW4ubWFyaWFubmFAdm1yaS5odQ==
Disclaimer: All claims expressed in this article are solely those of the authors and do not necessarily represent those of their affiliated organizations, or those of the publisher, the editors and the reviewers. Any product that may be evaluated in this article or claim that may be made by its manufacturer is not guaranteed or endorsed by the publisher.
Research integrity at Frontiers
Learn more about the work of our research integrity team to safeguard the quality of each article we publish.