- College of Life Science, Hunan Normal University, Changsha, China
Russula subnigricans is the only deadly species in the genus Russula with a mortality rate of more than 50%, and Russula japonica is the most common poisonous species, making rapid species identification in mushroom poisoning incidents extremely important. The main objective of this study was to develop a rapid, specific, sensitive, and simple loop-mediated isothermal amplification (LAMP) assay for the detection of R. subnigricans and R. japonica. Two sets of species-specific LAMP primers targeting internal transcribed spacer (ITS) regions were designed to identify R. subnigricans and R. japonica. The results demonstrated that while LAMP could specifically detect R. subnigricans and R. japonica, the polymerase chain reaction (PCR) could not distinguish R. subnigricans from Russula nigricans. In addition, the results demonstrated that, compared to electrophoresis-LAMP and real-time quantitative LAMP (RT-qLAMP), the detection sensitivity of HNB-LAMP (a mixture of LAMP with hydroxy naphthol blue (HNB) dye) for R. subnigricans could reach 0.5 pg/μl and was 100-fold higher than that of PCR. The LAMP reaction could be completed in 45 min, which is much faster than the conventional PCR. In the future, LAMP can be used a quick, specific, and sensitive detection tool in various fields.
Introduction
Mushroom poisoning is a public health emergency in China, with the highest mortality rate and the highest number of food poisoning deaths. To date, 1,662 taxa of fungi have been checked and corrected in China, including 1,020 edible fungal taxa, 692 medicinal fungal taxa, and 480 toxic fungal taxa (Wu et al., 2019). Between 2004 and 2014, there were 576 mushroom poisoning cases in mainland China, with 3,701 patients and 786 deaths (Zhou et al., 2016). Between 2019 and 2021, the Chinese Center for Disease Control and Prevention reported 1,279 cases of mushroom poisoning, with 3,411 patients and 67 deaths (Li et al., 2020, 2021, 2022).
Russula Pers., one of the largest genera of ectomycorrhizal fungi, contains both edible and poisonous species (Buyck et al., 2018). According to records, the edible taxa of the Russula genus are typically Russula virescens, Russula griseocarnosa, Russula delica, and others, and 18 poisonous taxa have been reported (Wu et al., 2019; Wang, 2020). In China, the most common cause of mushroom poisoning is rhabdomyolysis caused by accidental eating of Russula subnigricans as well as the fatal deaths caused by the lethal amanitas, which causes acute liver damage, or species in the genera Lepiota and Galerina. Between 1994 and 2012, Chen et al. (2014) investigated 102 cases of mushroom poisoning in southern China and found that R. subnigricans is one of the three mushrooms responsible for the majority of deaths, with a mortality rate of more than 50%. R. subnigricans is the only lethal mushroom of the Russula genus that causes rhabdomyolysis, and it has been linked to numerous poisoning deaths in the last 20 years (Lee et al., 2001; Matsuura et al., 2009; Cho and Han, 2016; Li et al., 2020, 2021, 2022). The most common species in China is Russula japonica, which causes gastroenteritis when consumed. According to the reports published by the China CDC between 2019 and 2021, the top three species that cause clinical syndromes associated with gastroenteritis are Chlorophyllum molybdites, R. japonica, and Entoloma omiense, with R. japonica ranking second for three consecutive years (Li et al., 2020, 2021, 2022).
Rapid and accurate identification of poisonous mushroom species is of vital importance for the prevention, source investigation, and accurate and effective treatment of mushroom poisoning. At present, poisonous mushroom species are identified mainly through morphological evidence, molecular biology, and toxin analysis. Morphological identification requires professional knowledge of fungal taxonomy. Due to the diversity of species in the Russula genus, it is difficult for the public to distinguish poisonous species and edible species because of their similar morphology. The specimens examined for this study indicate that Russula nigricans and Russula densifolia closely resembled R. subnigricans, and R. japonica resembles other edible Russula species, making species identification difficult (Figure 1). With the development of molecular biology and analytical chemistry, DNA barcoding techniques and high-performance liquid chromatography (HPLC) have been used for species identification and toxin analysis (White, 1990; Enjalbert et al., 1992; Parnmen et al., 2016). However, the limitations of expensive equipment and professional operations are not suitable for primary institutions and rural areas.
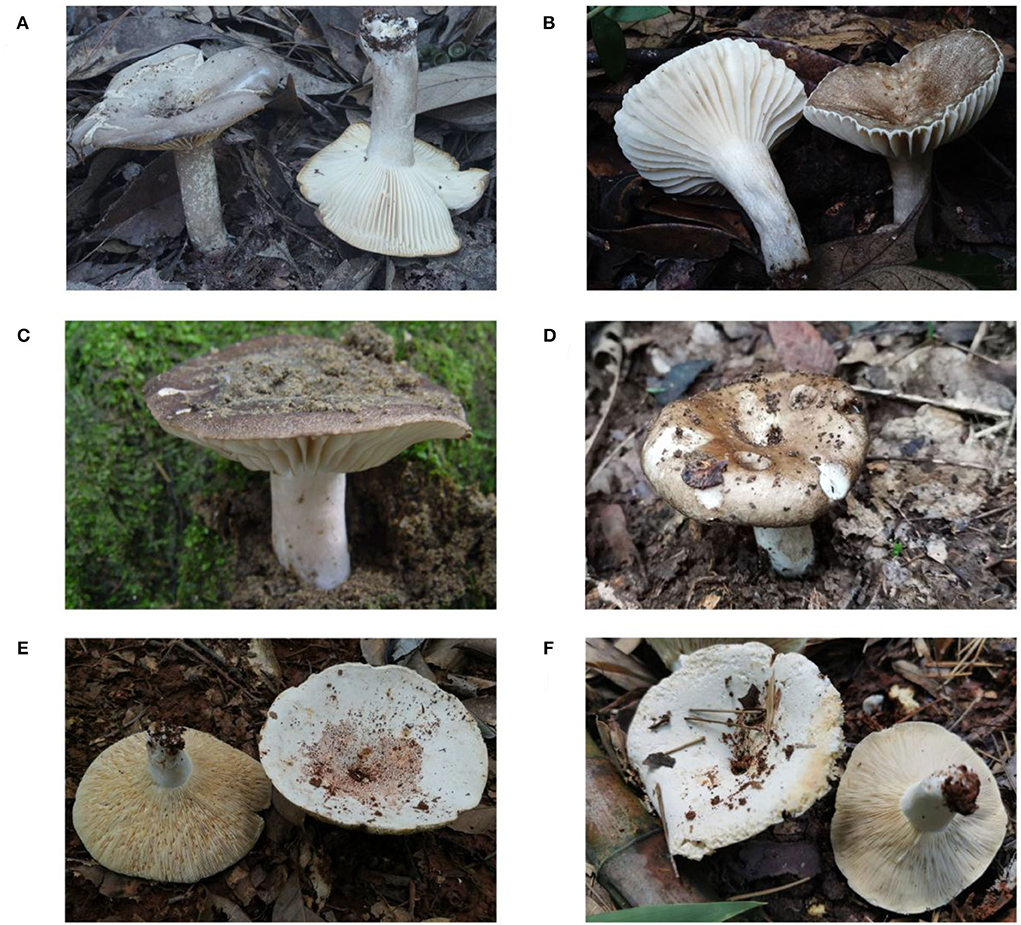
Figure 1. Basidiomata of Russula species. (A) and (B): Russula subnigricans; (C): R. nigricans; (D): R. densifolia; and (E) and (F): R. japonica.
In recent years, loop-mediated isothermal amplification (LAMP) has been used in environmental monitoring and detection of pathogenic microorganisms because of its simplicity, rapidity, high specificity, and sensitivity (Wang et al., 2008; Chen et al., 2013; Yang et al., 2018; Baek et al., 2020; Waliullah et al., 2020). More importantly, the results of LAMP reactions with hydroxy naphthol blue (HNB) allow for visual discrimination without the use of costly specialized equipment (Goto et al., 2009; Naraporn and Choopara, 2019). Notomi first developed LAMP as a potential method for nucleic acid amplification, and the reaction is carried out at a constant temperature ranging from 60 to 65°C using a set of four primers catalyzed by a DNA polymerase with strand displacement activity called Bst DNA polymerase (Notomi et al., 2000). A set of primers reflects specificity, and high sensitivity is due to the fact that it generates a vast quantity of amplification products (109-1010 times) in a short period of time (Nagamine et al., 2002; Notomi et al., 2015). The reaction time is ~1 h, but the addition of loop primers reduces the consumption time (Nagamine et al., 2002).
LAMP has been used in the detection of lethal mushrooms belonging to Amanita, R. senecis, and C. molybdites in the field of mushroom identification to date (Vaagt et al., 2013; He et al., 2019a; Wang et al., 2021, 2022). In this study, LAMP, as a rapid and effective method, is applied to detect the deadly poisonous species R. subnigricans and the widely distributed toxic species R. japonica.
Materials and methods
Mushroom samples
Table 1 lists 32 mushroom samples from 18 species that were used in this study. R. subnigricans samples were collected from nine different poisoning incidents, and mushrooms from other Russulaceae species were collected in the wild or from the CDC in China. All specimen vouchers, including samples S-81 and S-114, have been deposited in the Mycological Herbarium of Hunan Normal University (MHHNU).
DNA extraction, polymerase chain reaction amplification, and internal transcribed spacer sequencing
Approximately 10 mg of each dried sample was weighed. Total genomic DNA was extracted with the Fungal DNA Mini kit (Omega, USA). Internal transcribed spacers (ITSs) 4 and 5 were used to amplify ITS sequences. Each polymerase chain reaction (PCR) mixture contained 1 × PCR buffer, 1.5 mM MgCl2, 0.2 mM dNTPs, 0.4 μM of each primer, 1.25 U of Taq polymerase, and 1 μl DNA template in a total volume of 25 μl. PCRs were run on an Eppendorf Mastercycler thermal cycler (Eppendorf, Inc., Germany) as follows: initial denaturation at 94°C for 4 min; followed by 30 cycles of 94°C for 30 s, 54°C for 30 s, and 72°C for 30 s; and a final extension at 72°C for 8 min. Amplified PCR products were detected using gel electrophoresis on 1% agarose gels and then sequenced at Tsingke Biological Technology (China).
Design of primers for LAMP and PCR
A set of six specific primers based on the published ITS sequences of R. subnigricans and a set of five specific primers (GenBank accession Nos. MK430039 and OM760677) based on the sequences of R. japonica were designed using the professional online software PrimerExplorer V5 (http://primerexplorer.jp/lampv5e/index.html). In the case of R. subnigricans, a forward inner primer (FIP) consisting of the complementary sequences of F1c and F2, a backward inner primer (BIP) consisting of B1c and B2, and two outer primers (F3 and B3), and two loop primers (LF and LB) were used for LAMP. For R. japonica, only one loop primer was suitable for R. japonica. Multiple-sequence alignment of ITS of Russula samples and the target regions used to design LAMP primers are labeled and seen in Figure 2. Species-specific PCR primers for R. subnigricans were designed using Primer 5.0 software, including Rs-f (forward primer of R. subnigricans) and Rs-r (reverse primer of R. subnigricans). The primers (PAGE) were synthesized by Tsingke Biological Technology (China), and their sequences and lengths are presented in Table 2.
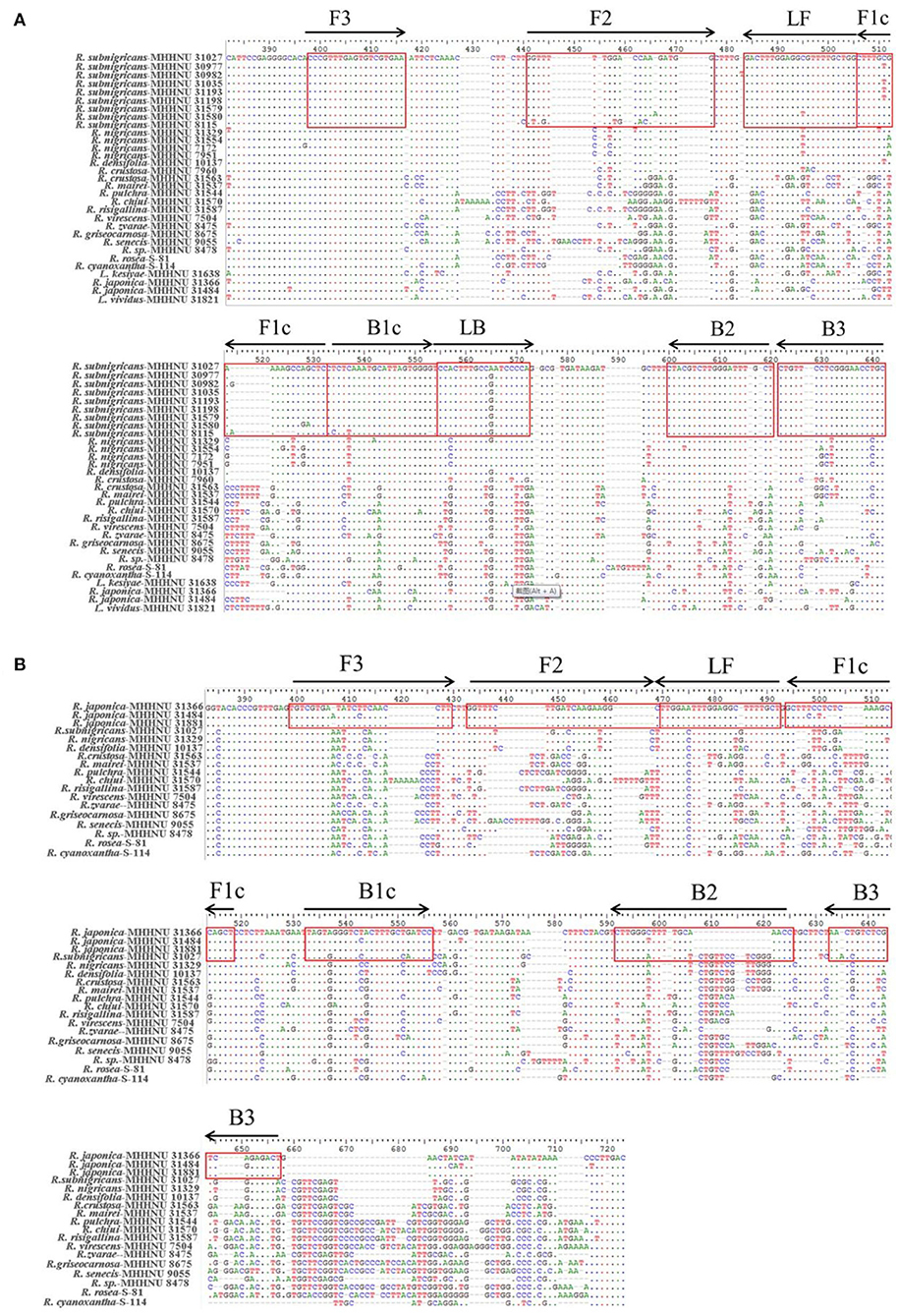
Figure 2. Multiple-sequence alignment of internal transcribed spacer (ITS) of Russula samples. The target regions used for designing loop-mediated isothermal amplification (LAMP) primers were labeled. LAMP primers for R. subnigricans (A) and LAMP primers for R. japonica (B).
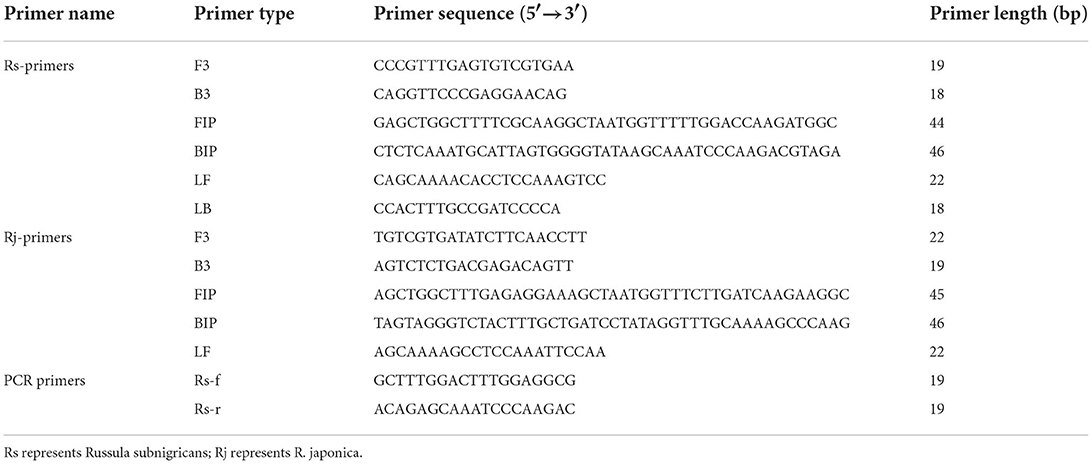
Table 2. Information on the species-specific primers used for loop-mediated isothermal amplification (LAMP).
Optimization of LAMP reaction temperatures
Real-time quantitative LAMP (RT-qLAMP) was carried out in a 10-μl reaction mixture: 1× ThermoPol buffer (20 mM Tris-HCl, 10 mM (NH4)2SO4, 10 mM KCl, 2 mM MgSO4, and 0.1% Triton X-100, pH 8.8), 4 mM MgSO4, 1.4 mM dNTP mix, 1.28 μM FIP, 1.28 μM BIP, 0.12 μM F3, 0.12 μM B3, 0.64 μM LF, 0.64 μM LB (absent in the LAMP reaction of R. japonica), 3.2 U of Bst DNA polymerase (NEB, USA), 5 ng DNA template, 150 μM 0.5 × SYBR Green I, and ddH2O to a total volume of 10 μl. These reactions were performed using the QuantStudio 5 Real-Time PCR System (ABI, USA). The reaction mixtures were incubated at different temperatures ranging from 60 to 65°C for 40 min. A real-time fluorescence detection system was used for monitoring the LAMP reactions. As each cycle was set to 1 min, the threshold time (Tt) value was equivalent to the cycle time (Ct). The SYBR Green I data were plotted as the relative fluorescence signal vs. time, with each cycle set to 1 min at a constant temperature of 62°C.
LAMP reaction and product detection
As previously described, the LAMP reaction was carried out in a 10-μl reaction mixture with HNB replacing 0.5 × SYBR Green I. Each reaction was performed in a 0.2-ml tube with a water bath incubated at 62°C for 45 min and finally at 80°C for 10 min to terminate the reaction (Figure 3). Conventional agarose gel electrophoresis was used to analyze DNA amplification products. In addition, in our study, another low-pollution approach,direct visual inspection of LAMP mixtures with HNB by the naked eye, was also used to detect amplification products of LAMP. Real-time measurement of SYBR Green I-based fluorescence was used to verify the HNB staining method (Figure 4).
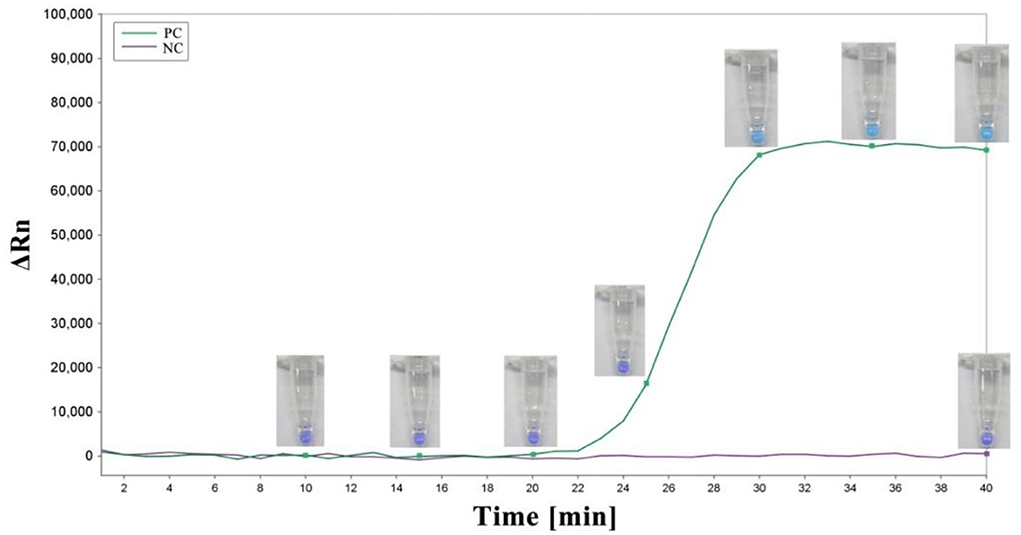
Figure 4. The amplification plot and corresponding colors of the LAMP mixture over time. A sky blue color was observed in the case of amplification, whereas the negative control remained violet after the reaction. PC, positive control; NC, negative control.
Tests of specificity and sensitivity of the LAMP assay and PCR
To test the specificity of the LAMP primers for R. subnigricans, genomic DNA from 31 wild samples, including R. subnigricans collected from nine different poisoning incidents, was used in 45-min LAMP reactions. To test the specificity of LAMP and PCR, specific PCR primers were designed to amplify the genomic DNA of R. subnigricans. For the specificity of the LAMP primers of R. japonica, genomic DNA of 19 wild samples, including R. japonica collected from three wild points, was used for 45-min LAMP reactions. To determine the detection limit, LAMP and PCR assays were performed using a series of 10-fold dilutions of genomic DNA from R. subnigricans (5 ng to 50 fg) at 45 min intervals.
Results
Optimized temperature of the LAMP reaction
To optimize the temperature, LAMP reactions were carried out at different temperatures from 60 to 65°C at 1°C intervals (each Tt value displayed is the mean of three replications). The threshold time (Tt) values of different temperatures obtained from the RT-qPCR system were analyzed, and the temperature of 62°C was found to be optimal in terms of the lowest Tt (Table 3).
Specificity of LAMP and PCR
To confirm the specificity of the LAMP and PCR primers for R. subnigricans, a total of 31 samples of mushroom species in Russulaceae, including nine R. subnigricans specimens from different poisoning incidents, 15 other Russula species, and two Lactarius species, were used to test the specificity of the LAMP assay. As seen in Figure 5, the amplification products of the PCR reactions were analyzed using agarose gel electrophoresis, while the LAMP reactions were analyzed using agarose gel electrophoresis and HNB dye staining (Figure 6). Not only R. subnigricans but also R. nigricans were successfully amplified by PCR, and bright bands were observed. The two LAMP detection methods produced consistent results. Positive reactions were observed in all R. subnigricans samples from different poisoning incidents, with typical ladder-like bands and sky blue mixtures, whereas no bands were observed for other Russula and Lactarius samples, and the color in the tubes remained violet after the reaction.
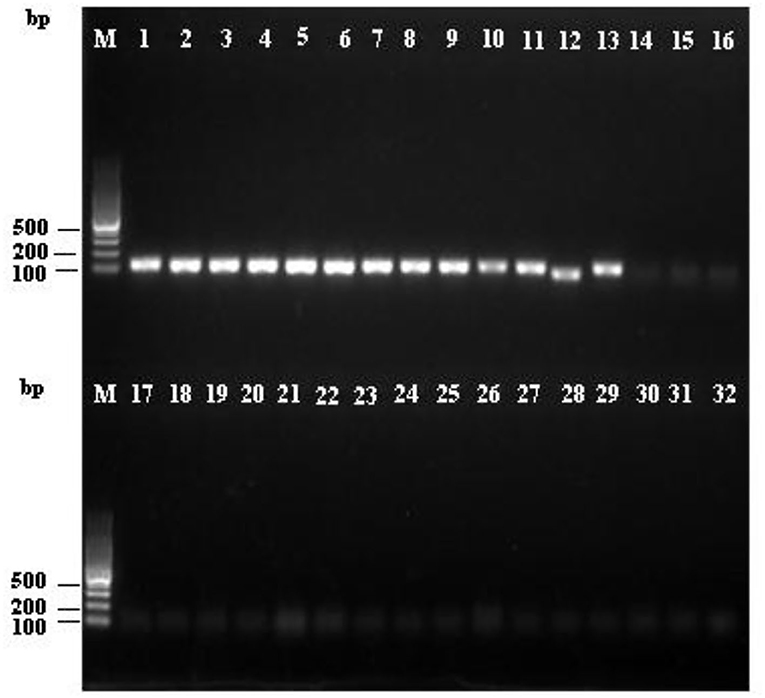
Figure 5. Specificity of the polymerase chain reaction (PCR) assay for R. subnigricans. Amplification was detected by agarose gel electrophoresis of PCR amplification products. M: 100 bp ladder; lanes 1–9: R. subnigricans; lanes 10–13: R. nigricans; lane 14: R. densifolia; lanes 15–16: R. crustosa; lane 17: R. mairei; lane 18: R. pulchra; lane 19: R. chiui; lane 20: R. risigallina; lane 21: R. virescens; lane 22: R. zvarae; lane 23: R. griseocarnosa; lane 24: R. senecis; lane 25: R. sp.; lane 26: R. rosea; lane 27: Lactarius kesiyae; lane 28: R. japonica; lane 29: R. japonica; lane 30: Lactarius vividus; lane 31: R. cyanoxantha; and lane 32: ddH2O (blank control).
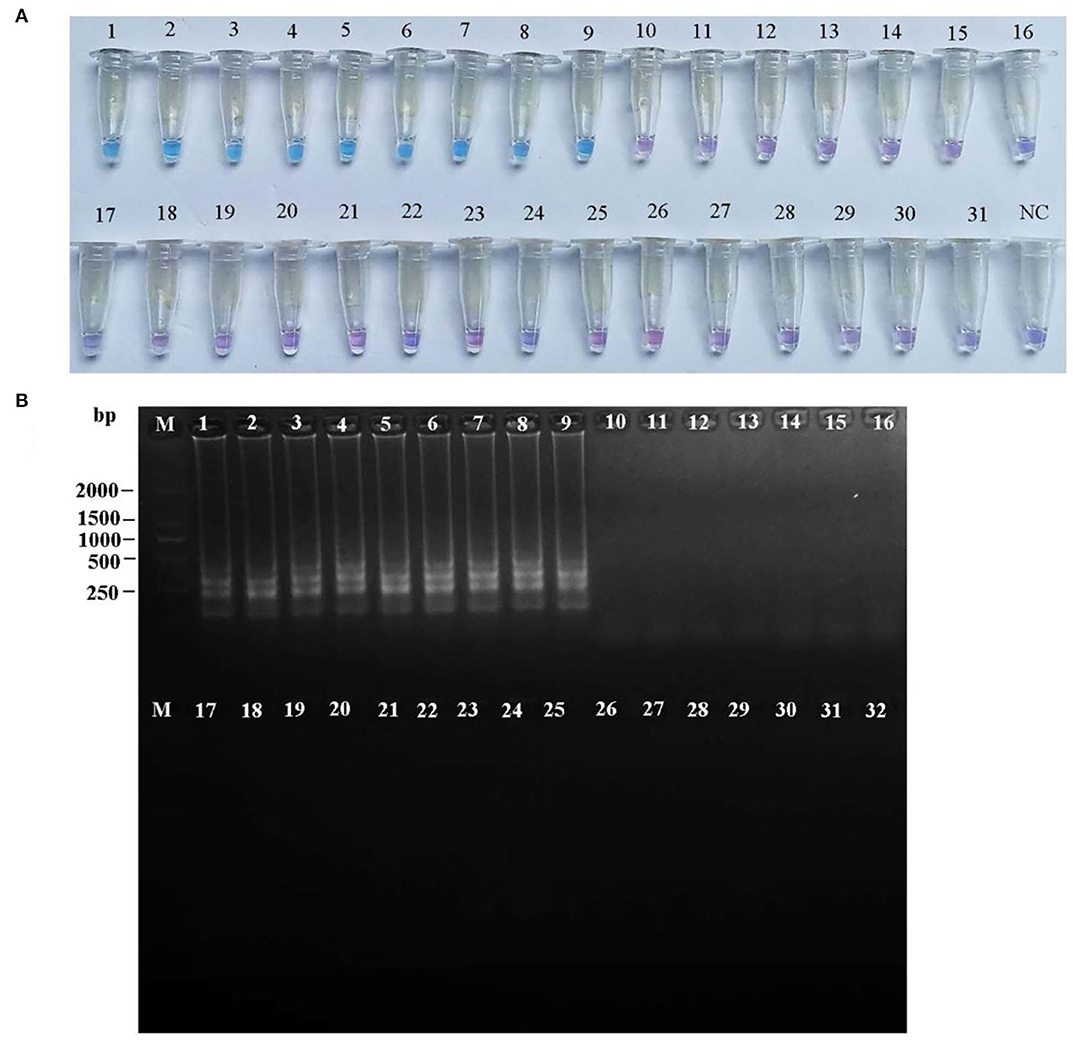
Figure 6. Specificity of the LAMP assay for R. subnigricans. Hydroxy naphthol blue (HNB) dye staining of LAMP products (A) and agarose gel electrophoresis of LAMP products (B). M: 100 bp ladder; lanes 1–9: R. subnigricans; lanes 10–13: R. nigricans; lane 14: R. densifolia; lanes 15–16: R. crustosa; lane 17: R. mairei; lane 18: R. pulchra; lane 19: R. chiui; lane 20: R. risigallina; lane 21: R. virescens; lane 22: R. zvarae; lane 23: R. griseocarnosa; lane 24: R. senecis; lane 25: R. sp.; lane 26: R. rosea; lane 27: L. kesiyae; lane 28: R. japonica; lane 29: R. japonica; lane 30: L. vividus; lane 31: R. cyanoxantha; and lane 32: ddH2O (blank control).
To verify the specificity of the LAMP primers for R. japonica, a total of 19 samples of mushroom species Russulaceae, including three R. japonica specimens from different locations in the wild and 15 other Russula species, were used to test the specificity of the LAMP assay. As seen in Figure 7, the results of the two methods of LAMP-positive detection of R. japonica were consistent with those of R. subnigricans. Three R. japonica were found with typical ladder-like bands and sky blue mixtures from all R. japonica samples, contrary to the patterns from other Russula samples with no bands, and with violet mixtures in the tubes after amplification.
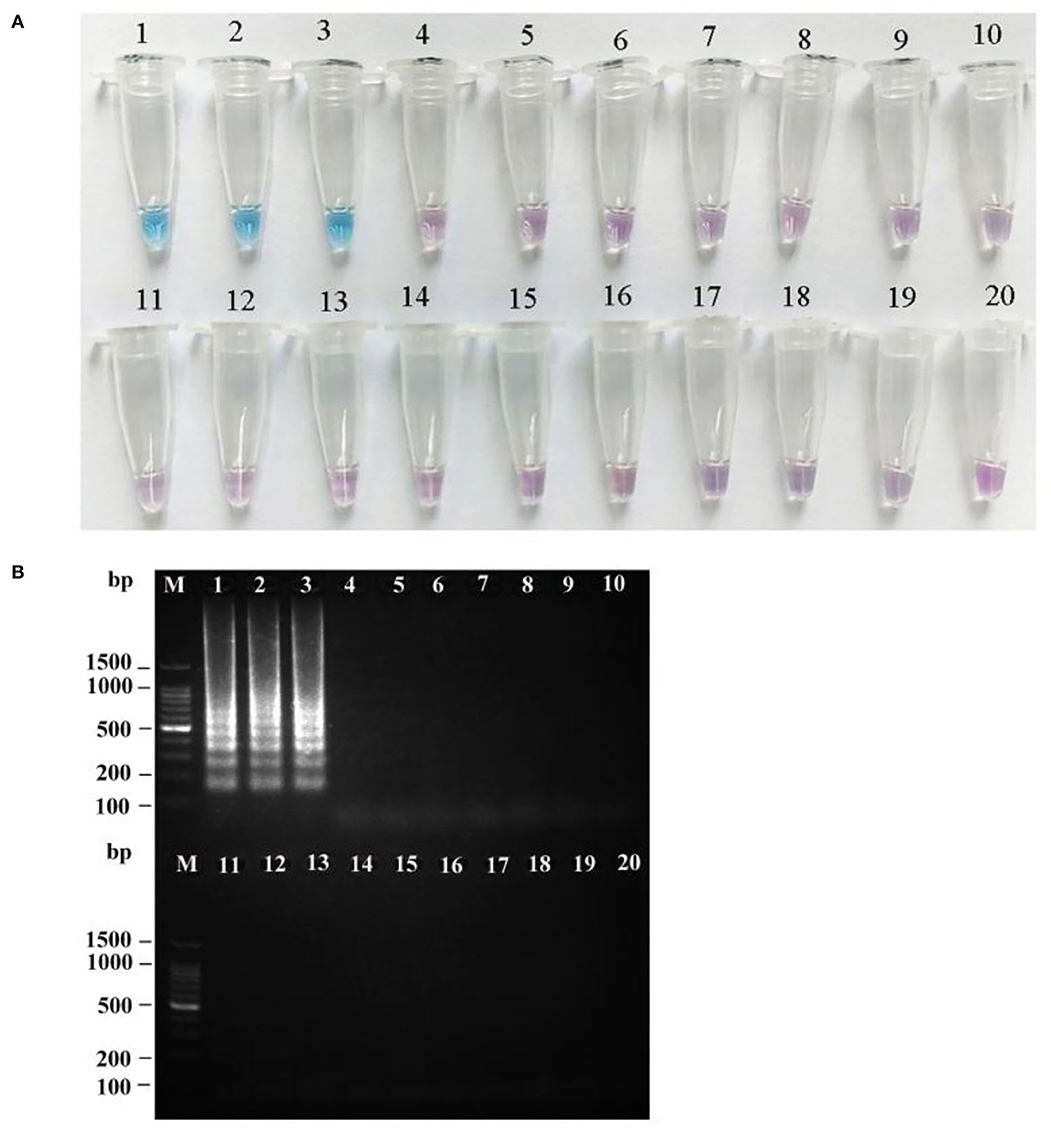
Figure 7. Specificity of the LAMP assay for R. japonica. HNB dye staining of LAMP products (A) and agarose gel electrophoresis of LAMP products (B). M: 100 bp ladder; lanes 1–3: R. japonica; lane 4: R. subnigricans; lane 5: R. nigricans; lane 6: R. densifolia; lane 7: R. crustosa; lane 8: R. crustosa; lane 9: R. mairei; lane 10: R. pulchra; lane 11: R. chiui; lane 12: R. risigallina; lane 13: R. virescens; lane 14: R. zvarae; lane 15: R. griseocarnosa; lane 16: R. senecis; lane 17: R. sp.; lane 18: R. rosea; lane 19: R. cyanoxantha; and lane 20: ddH2O (blank control).
Sensitivity of LAMP and PCR
To determine the detection limit, LAMP and PCR reactions were performed on serial 5 ng to 50 fg 10-fold diluted DNA templates of R. subnigricans. The detection of PCR is 500 pg/reaction (Figure 8). A real-time fluorescent quantitative system, gel electrophoresis, and HNB dye were used to detect the amplification products of LAMP. LAMP detection limits of the three methods for R. subnigricans analysis were identical and 100-fold higher than PCR: 5 pg per reaction (Figure 8). These results suggest that the detection sensitivity of the LAMP mixture with HNB dye (HNB-LAMP) for R. subnigricans is comparable to that of electrophoresis-LAMP and RT-qLAMP (Figure 9).
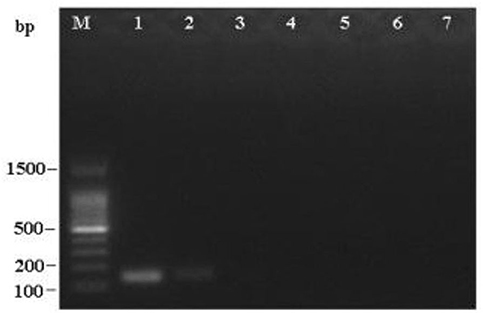
Figure 8. Sensitivity of the PCR assay for R. subnigricans. A dilution series of R. subnigricans DNA was prepared as follows: (1) 5 ng; (2) 500 pg; (3) 50 pg; (4) 5 pg; (5) 500 fg; (6) 50 fg; and (7) no template for negative control.
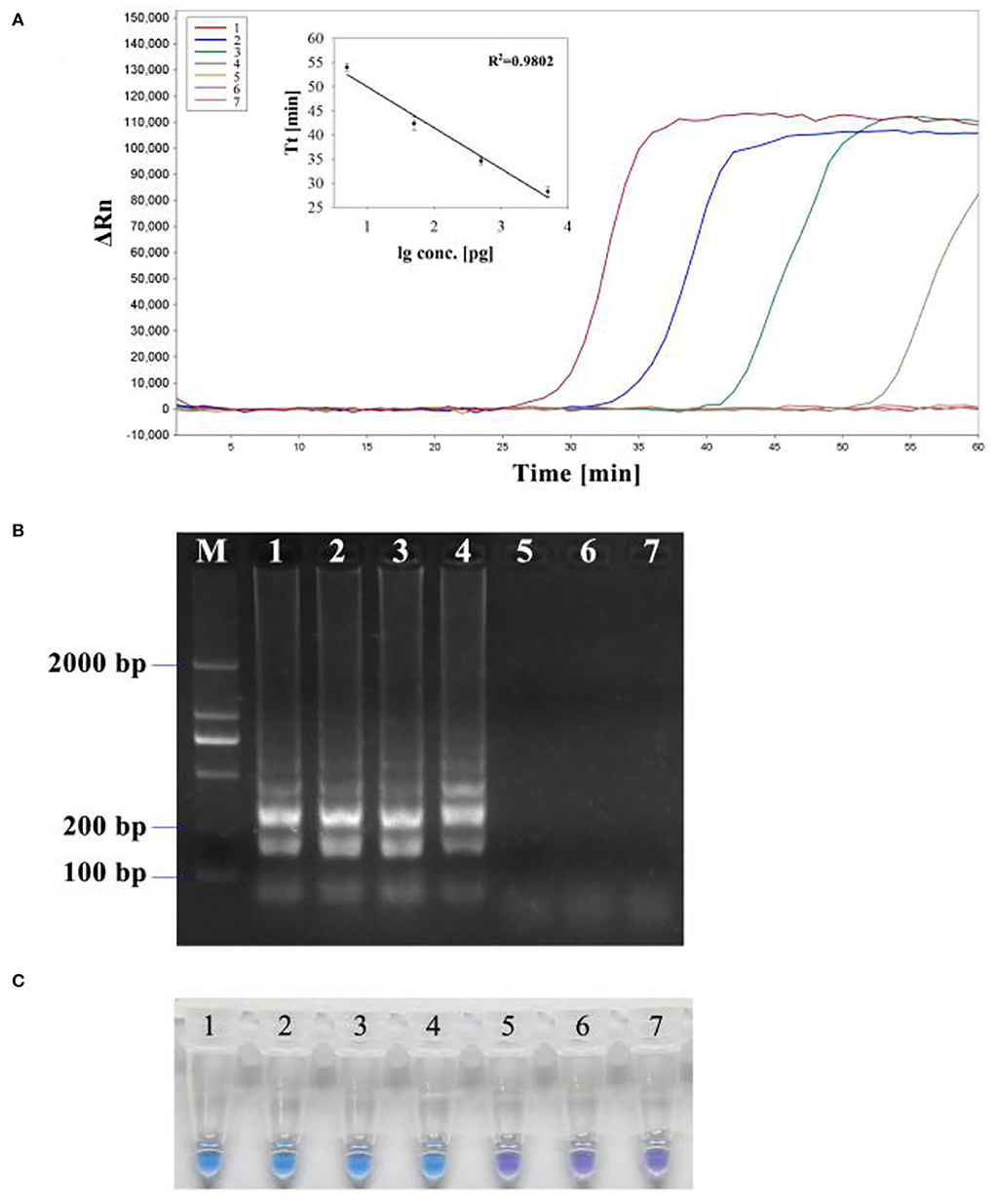
Figure 9. Sensitivity of the LAMP assay for R. subnigricans. A dilution series of R. subnigricans DNA was prepared as follows: (1) 5 ng; (2), 500 pg; (3) 50 pg; (4) 5 pg; (5) 500 fg; (6) 50 fg; and (7) no template for negative control. (A) Real-time quantitative LAMP (RT-qLAMP) analysis with different DNA concentrations. The standard curve based on this dilution series showed a linear relationship between the log of the quantity of initial template DNA (lg conc.) and threshold time (Tt). The coefficient of determination (R2) of the linear regression was 0.9802. (B) Electrophoresis-LAMP with different DNA concentrations. (C) HNB-LAMP with different DNA concentrations.
Discussion
Fungi of the genus Russula, widely distributed worldwide, play an important role in all ecosystems and the natural kingdom (Buyck et al., 2018; Adamčík et al., 2019). R. subnigricans is easily confused morphologically with edible wild mushrooms such as R. nigricans and R. densifolia, which is consistent with their phylogenetic relationships, since all are members of the Russula subsection Nigricantinae (Wang et al., 2020). R. japonica is a poisonous mushroom most difficult to differentiate from edible species because the subgenus to which R. japonica belongs also includes many famous edible species, such as R. delica and Russula brevipes (Wang et al., 2020). The lethality of R. subnigricans and the universality of R. japonica cause poisoning events to occur frequently from May to September of every year, especially in southern China (Matsuura et al., 2009; Lin et al., 2015; Cho and Han, 2016; Li et al., 2020, 2021, 2022).
Loop-mediated isothermal amplification, with the qualities of high sensitivity and specificity, offers a novel, simple, and rapid poisonous mushroom detection approach based on DNA amplification, which eliminates the stages of DNA denaturation and thermal cycling. Vaagt et al. (2013) first used LAMP in the detection of the poisonous mushroom Amanita phalloides. He et al. (2019a) achieved the detection of lethal Amanita species and introclade discrimination of lethal Amanita species with LAMP, while hyperbranched rolling circle amplification (HRCA) could discriminate it as introclade or intraclade species. However, HRCA requires an extra ligation of the padlock probe (1 h) before isothermal amplification, which is more time-consuming than LAMP though it has high specificity (He et al., 2019a,b). In the detection of Mycoplasma ovipneumoniae, the reaction times of real-time recombinase polymerase amplification (RT-RPA) and lateral flow strip recombinase polymerase amplification (LFS-RPA) are shorter than LAMP via a lateral flow dipstick (LAMP-LFD) assay, but the effectiveness of RT-RPA and LFS-RPA in the detection needs to be further validated (Zhang et al., 2019; Wang et al., 2020). Furthermore, LAMP primers for detecting poisonous mushrooms all are based on ITS regions, and according to various families and genera, we can design specific primers based on other gene fragments, such as nrLSU, rpb1, and rpb2 (Vaagt et al., 2013; He et al., 2019a; Wang et al., 2021, 2022).
In this study, two sets of species-specific LAMP primers based on ITS and to specifically detect R. subnigricans and R. japonica successfully were matched as expected. Although R. subnigricans, R. nigricans, and R. densifolia have close phylogenetic relationships, LAMP could specifically discriminate R. subnigricans from other Russula species, while species-specific PCR primers failed to differentiate R. subnigricans from R. nigricans. R. japonica was also specifically detected using LAMP (Table 4). The results indicated that the LAMP assay could reach the target detection compared with traditional PCR though LAMP is less specific than HRCA (He et al., 2019a). Furthermore, in the sensitivity test, the results demonstrated that the detection limits of visual HNB-LAMP, electrophoresis-LAMP, and RT-qLAMP assays could reach 0.5 pg/μl, indicating that the detection sensitivity was 100-fold higher than that of PCR (Table 4). Compared with the detection of other species using LAMP, the detection limit was half of that in Amanita and was higher than that in R. senecis and C. molybdites (He et al., 2019a; Wang et al., 2021, 2022). In addition, LAMP showed excellent advantages of strong specificity and high sensitivity, which have also been observed in other fields, such as Cucurbit Leaf Crumple Virus, Avian infectious laryngotracheitis (AILT), and SARS-CoV-2 (Ghodrati et al., 2017; Waliullah et al., 2020; Yan et al., 2020; Yu et al., 2021). More importantly, in terms of simplicity, the HNB-LAMP visual system was validated using agarose gel electrophoresis, and the results of HNB-LAMP assays were consistent with those of agarose gel electrophoresis. With the addition of HNB as a visual indication, the result is easy to observe with the naked eye without opening the tube and causing aerosol pollution (Goto et al., 2009; Naraporn and Choopara, 2019). Owing to the strand displacement activity of DNA polymerase superseding thermal denaturation steps and the addition of loop primers, the result can be obtained in 45 min, much less than the reaction time of other isothermal amplification technologies like helicase-dependent amplification (HAD), strand displacement amplification (SDA), and HRCA (Nagamine et al., 2002; Barreda-García et al., 2018; He et al., 2019a,b; Kolm et al., 2019; Hu et al., 2020; Chi et al., 2022). Moreover, the temperature of LAMP assays ranging from 60 to 65°C confirmed their feasibility for field applications. By eliminating the need for expensive specific facilities and reagents, LAMP-based assays coupled with HNB dye will be more suitable for primary institutions and remote areas (Tomita et al., 2008; Pandey et al., 2018).
In conclusion, LAMP-based assays examined in this study proved to be a promising, well-performing, rapid, highly specific, and sensitive detection method and to be suitable for on-site applications because of their feasibility and cost-effectiveness in terms of money and time. Furthermore, LAMP-based assays are expected to have broad application prospects in other potential fields.
Data availability statement
The datasets presented in this study can be found in online repositories. The names of the repository/repositories and accession number(s) can be found in the article/supplementary material.
Author contributions
ZC and PL conceived and designed the experiments, and wrote this manuscript. PL, ZH, and ZJ carried out the LAMP assay. All authors contributed to the article and approved the submitted version.
Funding
This study was supported by the Key Research and Development Program of Hunan province (Grant No. 2020SK2103) and the Biodiversity Survey and Assessment Project of the Ministry of Ecology and Environment, China (Grant No. 2019HJ2096001006).
Conflict of interest
The authors declare that the research was conducted in the absence of any commercial or financial relationships that could be construed as a potential conflict of interest.
Publisher's note
All claims expressed in this article are solely those of the authors and do not necessarily represent those of their affiliated organizations, or those of the publisher, the editors and the reviewers. Any product that may be evaluated in this article, or claim that may be made by its manufacturer, is not guaranteed or endorsed by the publisher.
References
Adamčík, S., Looney, B., Cabon, M., Jančovičová, S., Adamčíková, K., Avis, P. G., et al. (2019). The quest for a globally comprehensible Russula language. Fungal Divers. 99, 369–449. doi: 10.1007/s13225-019-00437-2
Baek, Y. H., Um, J., Antigua, K. J. C., Parket, J., Kim, Y., Oh, S., et al. (2020). Development of a reverse transcription-loop-mediated isothermal amplification as a rapid early-detection method for novel SARS-CoV-2. Emerg. Microbes. Infect. 9, 998–1007. doi: 10.1080/22221751.2020.1756698
Barreda-García, S., Miranda-Castro, R., de-Los-Santos-Álvarez, N., Miranda-Ordieres, A. J., and Lobo-Castañón, M. J. (2018). Helicase-dependent isothermal amplification: a novel tool in the development of molecular-based analytical systems for rapid pathogen detection. Anal. Bioanal. Chem. 410, 679–693. doi: 10.1007/s00216-017-0620-3
Buyck, B., Zoller, S., and Hofstetter, V. (2018). Walking the thin line… ten years later: the dilemma of above- versus below-ground features to support phylogenies in the Russulaceae (Basidiomycota). Fungal Divers. 89, 267–292. doi: 10.1007/s13225-018-0397-5
Chen, G. F., Ma, C. S., Zhang, C. Y., Zhou, J., Wang, Y. Y., Wang, G. C., et al. (2013). A rapid and sensitive method for field detection of Prorocentrum donghaiense using reverse transcription-coupled loop-mediated isothermal amplification. Harmful Algae 29, 31–39. doi: 10.1016/j.hal.2013.08.001
Chen, Z. H., Zhang, P., and Zhang, Z. G. (2014). Investigation and analysis of 102 mushroom poisoning cases in Southern China from 1994 to 2012. Fungal Divers. 64, 123–131. doi: 10.1007/s13225-013-0260-7
Chi, Z., Wu, Y. H., Chen, L. H., Yang, H., Khan, M. R., Busquets, R., et al. (2022). CRISPR-Cas14a-integrated strand displacement amplification for rapid and isothermal detection of cholangiocarcinoma associated circulating microRNAs. Anal. Chim. Acta 1205, 339763. doi: 10.1016/j.aca.2022.339763
Cho, J. T., and Han, J. H. (2016). A case of mushroom poisoning with Russula subnigricans: development of rhabdomyolysis, acute kidney injury, cardiogenic shock, and death. J. Korean Med. Sci. 31, 1164–1167. doi: 10.3346/jkms.2016.31.7.1164
Enjalbert, F., Gallion, C., Jehl, F., Monteil, H., and Faulstich, H. (1992). Simultaneous assay for amatoxins and phallotoxins in Amanita phalloides Fr. by high-performance liquid chromatography. J. Chromatogr. 598, 227–236. doi: 10.1016/0021-9673(92)85052-U
Ghodrati, M., Spotin, A., Hazratian, T., Mahami-Oskouei, M., Bordbar, A., Ebrahimi, S., et al. (2017). Diagnostic accuracy of loop-mediated isothermal amplification assay as a field molecular tool for rapid mass screening of old world Leishmania infections in sand flies and in vitro culture. Iran J. Parasitol. 12, 506–515.
Goto, M., Honda, E., Oqura, A., Nomoto, A., and Hanaki, K. (2009). Clorimetric detection of loop-mediated isothermal amplification reaction by using hydroxyl naphthol blue. Biotechniques 46, 167–172. doi: 10.2144/000113072
He, Z. M., Lou, T., Fan, F. X., Zhang, P., and Chen, Z. H. (2019b). Universal identification of lethal amanitas by using Hyperbranched rolling circle amplification based on α-amanitin gene sequences. Food Chem. 15, 298. doi: 10.1016/j.foodchem.2019.125031
He, Z. M., Su, Y. T., Li, S. N., Long, P., Zhang, P., and Chen, Z. H. (2019a). Development and evaluation of isothermal amplification methods for rapid detection of lethal amanita species. Front. Microbiol. 10, 1523. doi: 10.3389/fmicb.2019.01523
Hu, X. J., Deng, Q. Y., Li, J. M., Chen, J. R., Wang, Z. X., Zhang, X. Q., et al. (2020). Development and clinical application of a rapid and sensitive loop-mediated isothermal amplification test for SARS-CoV-2 infection. mSphere. 5, e00808–e00820. doi: 10.1128/mSphere.00808-20
Kolm, C., Martzy, R., Führer, M., Mach, R. L., Krska, R., Baumgartner, S., et al. (2019). Detection of a microbial source tracking marker by isothermal helicase-dependent amplification and a nucleic acid lateral-flow strip test. Sci. Rep. 9, 393. doi: 10.1038/s41598-018-36749-7
Lee, P. T., Wu, M. L., Tsai, W. J., Ger, J., Deng, J. F., and Chung, H. M. (2001). Rhabdomyolysis: an unusual feature with mushroom poisoning. Am. J. Kidney Dis. 38, e17.1–e17.5. doi: 10.1053/ajkd.2001.27725
Li, H. J., Zhang, H. S., Zhang, Y. Z., Zhang, K. P., Zhou, J., Yin, Y., et al. (2020). Mushroom poisoning outbreaks: China, 2019. China CDC Weekly 2, 19–24. doi: 10.46234/ccdcw2020.005
Li, H. J., Zhang, H. S., Zhang, Y. Z., Zhou, J., Yin, Y., He, Q., et al. (2021). Mushroom poisoning outbreaks: China, 2020. China CDC Weekly 3, 41–45. doi: 10.46234/ccdcw2021.014
Li, H. J., Zhang, H. S., Zhang, Y. Z., Zhou, J., Yin, Y., He, Q., et al. (2022). Mushroom poisoning outbreaks: China, 2021. China CDC Weekly 4, 35–40. doi: 10.46234/ccdcw2022.010
Lin, S. D., Mu, M. Y., Yang, F. W., and Yang, C. F. (2015). Russula subnigricans poisoning: from gastrointestinal symptoms to rhabdomyolysis. Wilderness Environ. Med. 26, 380–383. doi: 10.1016/j.wem.2015.03.027
Matsuura, M., Saikawa, Y., Inui, K., Nakae, K., Igarashi, M., Hashimoto, K., et al. (2009). Identification of the toxic trigger, in mushroom poisoning. Nat. Chem. Biol. 5, 465. doi: 10.1038/nchembio.179
Nagamine, K., Hase, T., and Notomi, T. (2002). Accelerated reaction by loop-mediated isothermal amplification using loop primers. Mol. Cell. Probe. 16, 223–229. doi: 10.1006/mcpr.2002.0415
Naraporn, S., and Choopara, I. (2019). Point-of-care chlamydia trachomatis detection using loop-mediated isothermal amplification and hydroxynaphthol blue. Methods Mol. Biol. 2042, 11–17. doi: 10.1007/978-1-4939-9694-0_2
Notomi, T., Mori, Y., Tomita, N., and Kanda, H. (2015). Loop-mediated isothermal amplification (LAMP): principle, features, and future prospects. J. Microbiol. 53, 1–5. doi: 10.1007/s12275-015-4656-9
Notomi, T., Okayama, H., Masubuchi, H., Yonekawa, T., Watanabe, K., Amino, N., et al. (2000). Loop-mediated isothermal amplification of DNA. Nucl. Acids Res. 28, E63. doi: 10.1093/nar/28.12.e63
Pandey, M., Singh, D., and Onteru, S. K. (2018). Reverse transcription loop-mediated isothermal amplification (RT-LAMP), a light for mammalian transcript analysis in low-input laboratories. J. Cell Biochem. 119, 4334–4338. doi: 10.1002/jcb.26624
Parnmen, S., Sikaphan, S., Leudang, S., Boonpratuang, T., Rangsiruji, A., and Naksuwankul, K. (2016). Molecular identification of poisonous mushrooms using nuclear ITS region and peptide toxins: a retrospective study on fatal cases in Thailand. J. Toxicol. Sci. 41, 65–76. doi: 10.2131/jts.41.65
Tomita, N., Mori, Y., Kanda, H., and Notomi, T. (2008). Loop-mediated isothermal amplification (LAMP) of gene sequences and simple visual detection of products. Nat. Protoc. 3, 877–882. doi: 10.1038/nprot.2008.57
Vaagt, F., Haase, I., and Fischer, M. (2013). Loop-mediated isothermal amplificationLAMP-basedmethodforrapidmushroomspeciesidentification. J. Agr. Food Chem. 61, 1833–1840. doi: 10.1021/jf304824b
Waliullah, S., Ling, K. S., Cieniewicz, E. J., Oliver, J. E., Ji, P., and Ali, M. E. (2020). Development of loop-mediated isothermal amplification assay for rapid detection of Cucurbit Leaf Crumple Virus. Int. J. Mol. Sci. 21, 1756. doi: 10.3390/ijms21051756
Wang, J. F., Li, R. W., Sun, X. X., Liu, L. B., Hao, X. P., Wang, J. C., et al. (2020). Development and validation of the isothermal recombinase polymerase amplification assays for rapid detection of Mycoplasma ovipneumoniae in sheep. BMC Vet Res. 16, 172. doi: 10.1186/s12917-020-02387-3
Wang, L., Li, L., Alam, M. J., Geng, Y. H., Li, Z. Y., Yamasaki, S., et al. (2008). Loop-mediated isothermal amplification method for rapid detection of the toxic dinoflagellate Alexandrium, which causes algal blooms and poisoning of shellfish. FEMS Microbiol. Lett. 282, 15–21. doi: 10.1111/j.1574-6968.2008.01074.x
Wang, N., Gao, J., Tian, E. J., Yu, W. J., Li, H., Zhang, J., et al. (2022). Rapid Russula senecis identification assays using loop-mediated isothermal amplification based on real-time fluorescence and visualization. Appl. Microbiol. Biotechnol. 106, 1227–1239. doi: 10.1007/s00253-022-11774-9
Wang, N., Zhao, Z. Y., Gao, J., Tian, E. J., Yu, W. J., Li, H., et al. (2021). Rapid and visual identification of Chlorophyllum molybdites with loop-mediated isothermal amplification method. Front. Microbiol. 12, 638315. doi: 10.3389/fmicb.2021.638315
Wang, X. H. (2020). Taxonomic comments on edible species of Russulaceae. Mycosystema. 39, 1617–1639. doi: 10.13346/j.mycosystema.200209
White, T. J. (1990). Amplification and Direct Sequencing of Fungal Ribosomal RNA Genes for Phylogenetics. San Diego, CA: Academic Press. doi: 10.1016/B978-0-12-372180-8.50042-1
Wu, F., Zhou, L. W., Yang, Z. L., Bau, T., and Dai, Y. C. (2019). Resource diversity of Chinese macrofungi: edible, medicinal and poisonous species. Fungal Divers. 98, 1–76. doi: 10.1007/s13225-019-00432-7
Yan, C., Cui, J. H., Huang, L., Du, B., Chen, L., Xue, G. H., et al. (2020). Rapid and visual detection of 2019 novel coronavirus (SARS-CoV-2) by a reverse transcription loop-mediated isothermal amplification assay. Clin. Microbiol. Infect. 26, 773–779. doi: 10.1016/j.cmi.2020.04.001
Yang, Q., Domesle, K. J., and Ge, B. L. (2018). Loop-mediated isothermal amplification for salmonella detection in food and feed: current applications and future directions. Foodborne Pathog. Dis. 15, 309–331. doi: 10.1089/fpd.2018.2445
Yu, J. F., Xie, J., Cao, Y., Kang, R. M., Lin, Y., Wei, Y., et al. (2021). A modified loop-mediated isothermal amplification method for detecting avian infectious laryngotracheitis virus. Anim. Biotechnol. 32, 766–773. doi: 10.1080/10495398.2020.1755676
Zhang, J., Cao, J. J., Zhu, M. S., Xu, M. G., and Shi, F. (2019). Loop-mediated isothermal amplification-lateral-flow dipstick (LAMP-LFD) to detect Mycoplasma ovipneumoniae. World J. Microbiol. Biotechnol. 35, 31. doi: 10.1007/s11274-019-2601-5
Keywords: loop-mediated isothermal amplification, Russula subnigricans, Russula japonica, ITS, mushroom poisoning
Citation: Long P, Jiang Z, He Z and Chen Z (2022) Development of a loop-mediated isothermal amplification assay for the rapid detection of Russula subnigricans and Russula japonica. Front. Microbiol. 13:918651. doi: 10.3389/fmicb.2022.918651
Received: 12 April 2022; Accepted: 20 June 2022;
Published: 23 August 2022.
Edited by:
Giancarlo Perrone, Italian National Research Council, ItalyReviewed by:
Massimo Ferrara, Italian National Research Council, ItalyBiswajit Maiti, Nitte University, India
Copyright © 2022 Long, Jiang, He and Chen. This is an open-access article distributed under the terms of the Creative Commons Attribution License (CC BY). The use, distribution or reproduction in other forums is permitted, provided the original author(s) and the copyright owner(s) are credited and that the original publication in this journal is cited, in accordance with accepted academic practice. No use, distribution or reproduction is permitted which does not comply with these terms.
*Correspondence: Zuohong Chen, Y2hlbnp1b2hvbmcmI3gwMDA0MDsyNjMubmV0