- 1Department of Special Medicine, School of Basic Medicine, Qingdao Medical College, Qingdao University, Qingdao, China
- 2Oral Research Center, Qingdao Municipal Hospital, Qingdao, China
- 3Department of Pathogenic Biology, School of Basic Medicine, Qingdao Medical College, Qingdao University, Qingdao, China
Human cytomegalovirus (HCMV) is a β-herpesvirus whose genome consists of double stranded linear DNA. HCMV genome can generate non-coding RNAs (ncRNAs) through transcription in its host cells. Besides that, HCMV infection also changes the ncRNAs expression profile of the host cells. ncRNAs play a key role in maintaining the normal physiological activity of cells, and the disorder of ncRNAs expression has numerous adverse effects on cells. However, until now, the relationship between ncRNAs and HCMV-induced adverse effects are not summarized in detail. This review aims to give a systematic summary of the role of HCMV infection in ncRNAs expression while providing insights into the molecular mechanism of unnormal cellular events caused by ncRNAs disorder. ncRNAs disorder induced by HCMV infection is highly associated with cell proliferation, apoptosis, tumorigenesis, and immune regulation, as well as the development of cardiovascular diseases, and the potential role of biomarker. We summarize the studies on HCMV associated ncRNAs disorder and suggest innovative strategies for eliminating the adverse effects caused by HCMV infection.
Background
Non-coding RNAs (ncRNAs) are transcribed but not translated, thousands of ncRNAs are produced from gene transcription. ncRNAs can be reclassified as microRNA (miRNA), long non-coding RNA (lncRNA), and circular RNA (circRNA) (Hombach and Kretz, 2016; Liu et al., 2020). Most ncRNAs lose the function of encoding proteins (Zhu et al., 2021) but reportedly have other essential biological functions in all biological processes, including regulation of the profiles of mRNA (Zhou B. et al., 2021) and protein (Yu et al., 2021). ncRNAs play key role in cell growth (Chen et al., 2021), differentiation (Fatica and Bozzoni, 2014), polarization (Mohapatra et al., 2021), apoptosis (Zhao et al., 2020), and cellular defense (Tan Gana et al., 2012). Increasing evidence indicates that aberrant expression of ncRNAs results in critical pathogenesis during viral infection, such as HCMV (Shi L. et al., 2020).
HCMV is a kind of DNA virus that consists of double-stranded linear DNA. The genome of HCMV is 236 kb, which encodes 167 genes and translates more than 750 open reading frames (Stern-Ginossar et al., 2012). HCMV can generate its ncRNAs through transcription in the infected cells (Babu et al., 2014), meanwhile changing the expression profile of ncRNAs derived from its host cells (Yan et al., 2019). Most ncRNAs modulated by HCMV benefit viral replication but are harmful to the host. The process of ncRNAs expression regulated by HCMV infection is shown in Figure 1.
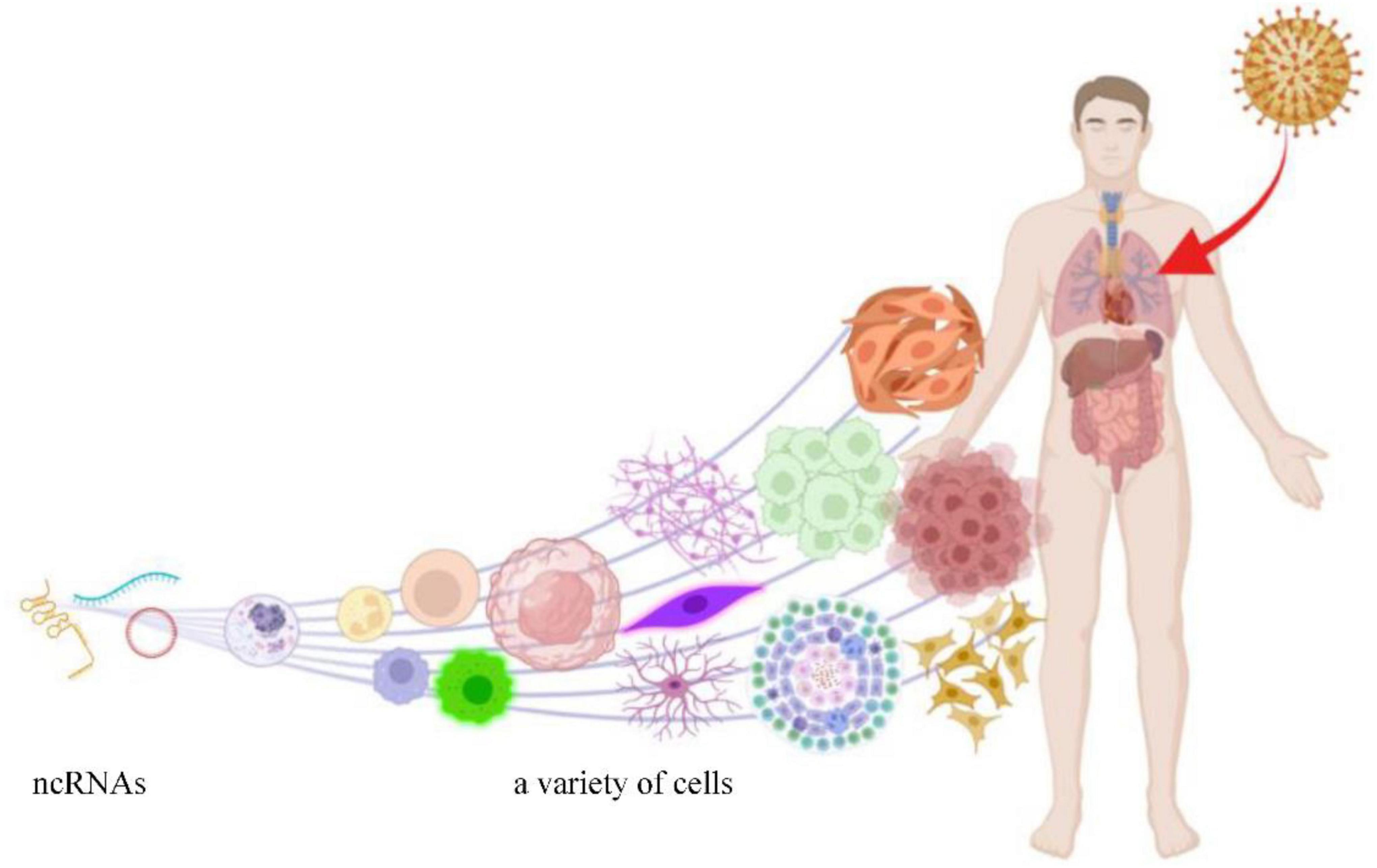
Figure 1. HCMV can infect a variety of cells and induce aberrant expression of ncRNAs (this figure was created in Biorender.com).
The main concerns of most HCMV related reviews focus on the change of immune response or mRNA/proteins. However, the important role of HCMV in the regulation of ncRNAs is neglected habitually. ncRNAs are the key node among HCMV and the altered mRNA. This review summarizes and points out the detailed regulatory role of HCMV in ncRNAs expression and adverse effects on its host cells. Our review’s novel insights will deepen our understanding of the HCMV pathogenic mechanism.
The Roles of Aberrant Expression of Human Cytomegalovirus-Induced Ncrna in Viral Infection and Cellular Disorder
ncRNAs exert multiple biological properties by regulating the expression level of mRNAs. The homeostasis of ncRNAs is essential to maintain the normal physiological activities of cells, and ncRNAs disorder also results in disastrous consequences for cells, such as the abnormal control of cell cycle and cell death (Liang et al., 2021; Zhou et al., 2022). HCMV is a kind of DNA virus that can produce ncRNAs by transcribing from its genome and interfering with the expression profile of host ncRNAs (Babu et al., 2014; Zhang et al., 2017). These dual changes in the ncRNAs landscape alter the pathophysiology of HCMV infections and accelerate the progression of HCMV related disease. Most aberrant ncRNAs induced by HCMV downregulate the host immune response, apoptosis, and autophagy (Babu et al., 2014). The effects of ncRNAs regulated by HCMV are detailed in Figure 2 and Table 1.
The Altered ncRNAs Benefit Human Cytomegalovirus Replication, Latency, and Reactivation
Many ncRNAs are preferentially used in the infected cells to create a cellular viral-friendly environment to ensure the replication, latency, and reactivation of HCMV.
Firstly, raw materials from the host were utilized by HCMV to complete self-replication and product infectious progeny. ncRNAs play a key regulatory role in this process. It was reported that miR-UL36 contributed to HCMV replication by down-regulating UL138 expression (Huang et al., 2013a). Hook et al. (2014) pointed out that HCMV miRs-UL112-1, US5-1, and US5-2 coordinately regulated reorganization of the secretory pathway to control cytokine secretion and facilitate the formation of the VAC for efficient infectious virus production. HCMV-encoded lncRNA4.9 formed an RNA-DNA hybrid (R-loop) through its G + C-rich 5’ end, which played an important role in initiating viral DNA replication (Tai-Schmiedel et al., 2020).
lncRNA beta2.7 is the most highly transcribed viral gene during lytic and latent infection (Gatherer et al., 2011; Stern-Ginossar et al., 2012; Shnayder et al., 2018). This RNA plays an anti-apoptotic role during infection by directly binding with complex I and also through mitigation of reactivate oxygen species (ROS) production (Reeves et al., 2007; Perera et al., 2022). The anti-apoptotic property of lncRNA beta2.7 is essential for successful completion the life cycle of HCMV.
In contrast, some cellular ncRNAs displayed an anti-virus effect by inhibiting HCMV replication. miR-100 and miR-101 modestly suppressed the production of infectious progeny by depressing mTOR expression (Wang et al., 2008). miR-182 suppressed HCMV replication by inducing type I interferon (IFN-I) through the FOXO3/interferon regulatory factor 7 (IRF7) pathway (He et al., 2018). Besides that, miR-200b-3p, miR-200c-3p and miR-21 also could inhibit HCMV replication (Fu et al., 2015; Han et al., 2017; Lee et al., 2018). However, HCMV selectively downregulated the anti-virus cellular miRNAs to help its replication.
Next, ncRNAs exert multiple functions to maintain latent infections. During the late stages of latent HCMV infection, miR-UL148D inhibited immediate early response gene 5 (IER5) expression, thereby rescuing the expression and activity of CDC25B to promote HCMV latency (Pan C. et al., 2016). miR-200 targeted the immediate-early protein 2 (IE2) 3’ untranslated region, resulting in repression of this viral protein to maintain latent infections (O’Connor et al., 2014). Decreased miR-92a contributed to the maintenance of latent viral genomes by increasing the expression of GATA-2 and cellular IL-10 (Poole et al., 2011). In addition, miR-US25-1, miR-UL112-3p, miR-US29, and miR-92a, etc. could play a critical role in maintenance of latency (Mohammad et al., 2014; Poole et al., 2014; Jiang et al., 2015; Meshesha et al., 2016; Diggins et al., 2021).
Furthermore, ncRNAs participated in the regulation of HCMV reactivation. Hancock et al. (2020b) reported that miR-US5-2 directly downregulated epidermal growth factor receptor (EGFR) adaptor protein GAB1, which regulated EGR1 and UL138 expression by affecting downstream MEK/ERK signaling, and then played a key role during reactivation from latency. miR-US25-1-3p significantly upregulated in the reactivation autoimmune patients than others and exhibited an obvious shift-switch from latency to reactivation (Zhou et al., 2020).
Together, these findings provided specific targets for anti-HCMV treatment by regulating replication, latency, and reactivation of HCMV.
The Altered ncRNAs Interfere Proliferation, Apoptosis and Cell Cycle of Host Cells
Besides the expression of viral ncRNAs in host cells, HCMV infection also changed the profiles of ncRNAs encoded by the host genome. These two sources of ncRNAs are involved in pathways of proliferation, apoptosis, and cell cycle progression, all of which may be implicated in viral pathogenesis.
During HCMV infection, the proliferation of host cells was affected by ncRNAs. It’s reported that viral miR-US22 downregulated EGR-1 and inhibited CD34+ HPCs self-renewal and proliferation (Mikell et al., 2019). Conversely, Shen et al. (2018) demonstrated that ectopically expressed miR-UL112 in HUVECs significantly increased proliferation.
ncRNAs also played a key role in regulating cell apoptosis. For example, miR-UL70-3p and UL148D countered cellular apoptosis and autophagy by regulating proapoptotic genes MOAP1, PHAP, and ERN1 (Babu et al., 2014). mir-UL148D exerted anti-apoptotic property by downregulating immediate early gene X-1 (IEX-1) (Wang et al., 2013). miR-UL36-5p inhibited apoptosis by directly downregulating adenine nucleotide translocator 3 (ANT3) (Guo et al., 2015). miR-US5-1 and miR-UL112-3p protected CD34+ HPCs from virus-induced apoptosis by mediating FOXO3a/BCL2L11 pathway (Hancock et al., 2021). However, to benefit the discharge of infectious virus particles, miR-US4-1 promotes the apoptosis of the infected cell by silencing the expression of glutaminyl-tRNA Synthetase (QARS) (Shao et al., 2016).
Moreover, ncRNAs encoded by HCMV also affected cell cycle progression. Grey et al. (2010) reported that miR-US25-1 disordered the cell cycle by regulating the expression of cyclin E2, BRCC3, EID1, MAPRE2, and CD147. Ectopically expressed miR-UL112 in HUVECs significantly raised the S-phase fraction in the cell cycle (Shen et al., 2018).
Collectively, these reports demonstrated that ncRNAs mediated signaling pathways, including proliferation, apoptosis, and cell cycle of host cells, played critical roles in the physiological effects of HCMV induced diseases.
The Effects of ncRNAs in Oncomodulatory
Cancer is the second leading cause of mortality globally, accounting for about 10 million deaths in 2020 (Jameus et al., 2021). Increasing evidence indicates that the products of the HCMV genome are involved in oncomodulatory (Dziurzynski et al., 2012), particularly ncRNAs.
During HCMV latent infection, miR-US25-1, miR-US25-2-5p, miR-UL112, and miR-124-3p were upregulated, and the target genes of those miRNAs were involved in melanogenesis and pathways in cancer (Fu et al., 2014). miR-UL112-3p promoted glioblastoma cell proliferation, clone formation, migration, and invasion by directly regulating tumor suppressor candidate 3 (TUSC3), and the miR-UL112-3p expression was positively associated with glioma size, differentiation, WHO stage and the overall and disease-free survival of patients (Liang et al., 2017). In addition, HCMV lncRNA4.9 gave rise to fast-growing triple-negative tumors in NSG mice (Kumar et al., 2018).
Besides the ncRNAs encoded by its genome, HCMV also employed the ncRNAs encoded by the host genome to promote cancer development. HCMV infection affected the progression of GC by regulating the miR-34c/IL6/STAT3 pathway (Shi L. et al., 2020). miR-27b played a key role in developing HCMV induced neurological disorders by affecting the growth of glioma cells (Wang L. et al., 2017). CMV70-3P miRNA increases glioblastoma multiforme (GBM) cancer stem cells (CSC) stemness (Ulasov et al., 2017). Moreover, HCMV infection contributed to migration and tube formation of endothelial cells through downregulation of SIRT1/eNOS by miR-199a-5p, which contributed to the progress of cancer (Zhang et al., 2013b).
Even worse, HCMV can depress the expression of ncRNAs, which have an anti-cancer function. HCMV upregulated the expression of Sox2 via inhibiting miR-145 and subsequently enhanced the stemness and proliferation of GBM cells (Soroceanu et al., 2015). HCMV reduced the level of miR-613, and the reduction of miR-613 expression also correlated to the unfavorable variables of cancer patients (Wang Y. et al., 2017). The expression of miR-144-3p was suppressed by HCMV and then decreased its anti-cancer property (Song et al., 2018).
ncRNAs disorder induced by HCMV infection is highly linked with cancer patients’ poor outcomes. Targeting the changed ncRNAs represented a promising therapeutic strategy for HCMV-related cancers.
The Effects of ncRNAs in Immune Regulation
During HCMV infection, the host immune system exerts a key antiviral property; however, ncRNAs are employed by HCMV to change the immune microenvironment, escape immunological surveillance and benefit virus survival.
ncRNAs encoded by HCMV contributed to immune evasion by reducing the production of cytokines. It was reported that miR-UL112-1 could functionally down-regulate the level of IL-32 (Huang et al., 2013b), and miR-UL112-3p reduced the expression of multiple cytokines (IL-1β, IL-6, and IL-8) by directly down-regulating TLR2 (Landais et al., 2015). miR-US5-2 suppressed the transcriptional repressor NGFI-A binding protein (NAB1) to induce myelosuppression of uninfected CD34+ HPCs by increasing TGF-β secretion (Hancock et al., 2020a). Moreover, HCMV lncRNA1.2 mediated downstream immune responses through manipulating intrinsic NF-κB-dependent cytokine and chemokine release (Lau et al., 2020). miR-US5-1 and miR-UL112-3p limited the production of pro-inflammatory factors (Hancock et al., 2017).
ncRNAs also contributed to the virus-infected cells evading immune cells’ killing. miR-UL59 inhibited the function of natural killer cells by downregulating cytomegalovirus UL16-binding protein 1 (Ding et al., 2017). miR-UL112 blocked NK cell cytotoxicity by suppressing the expression of IFN-1 (Huang et al., 2015). miR-UL148D decreased the aggregation of immune cells by downregulating the chemokine RANTES (Kim et al., 2012). miR-US4-1 and miR-UL112-5p reduced the level of ERAP1, thereby inhibiting the presentation of the HCMV-derived peptides to specific CTLs (Kim et al., 2011; Romania et al., 2017), thus leading to less susceptibility of infected cells to HCMV-specific CTLs. Inversely, edited-miR-376a contributed NK cells to eliminate HCMV infected cells by downregulating the immune-modulating molecule HLA-E (Nachmani et al., 2014). Those results identify a novel immune evasion mechanism mediated by ncRNAs derived from HCMV.
Besides that, miR-US25-1-5p evaded innate antiviral immunity by regulating Cyclophilin A-CD147-ERK/NF-κB pathway targeting CD147 (Chen et al., 2017). miR-US33as-5p downregulated the expression of IFN-stimulated genes (ISGs), inhibited STAT1 translocation into the nucleus, and subsequently evaded the immune system’s killing (Zhang et al., 2021). Moreover, in clinical research, miR-US5-2-3p increased T-cell responses and HCMV reactivation in renal transplant recipients (Waters et al., 2020). In HCMV positive patients, antiviral miRNA, miR-214-3p, was remarkedly decreased with astrocytoma progressing. miR-UL-112-3p was significantly upregulated in glioblastoma and contributed to immune escape of glioblastoma (Deshpande et al., 2018).
miR-221 positively regulates the phosphorylation and activation of NF-κB by directly suppressing the suppressor of cytokine signaling 1 (SOCS1) expression. Moreover, miR-221 alleviates CMV-induced tissue injury by promoting the production of IFN- I and ISGs. Thus, miR-221 can be served as an intrinsic antiviral factor and developed as a treatment target for anti-HCMV treatment (Yan et al., 2019).
Collectively, these findings demonstrated that ncRNAs mediated immune escape by regulating multiple pathways. Targeted these ncRNAs may provide an effective strategy for HCMV treatment.
ncRNAs Contribute to the Development of Cardiovascular Diseases
Cardiovascular diseases are one of the main causes of morbidity and mortality in developed and developing countries, affecting millions of people yearly (Zuraini et al., 2021). Recently, studies found that HCMV infection is involved in the development of cardiovascular diseases, one of the risk factors is ncRNAs disorder.
These studies revealed that miR-US25-1 accelerated the development and severity of HCMV-induced atherosclerosis by aggravating ox-LDL-promoted apoptosis (Fan et al., 2014). miR-217 and miR-138 promoted HCMV-induced angiogenesis by depressing the expression of SIRT1 (Zhang et al., 2013a,2017). In addition, Zhou W. et al. (2021) reported that miR-1929-3p could improve MCMV-induced vascular remodeling and endothelial cell injury, possibly through the deactivation of the NLRP3 inflammasome by ET-1/endothelin A receptor (Ednra). MCMV infection reduced mmu-miR-1929-3p expression, subsequently increased Ednra expression level, and raised the blood pressure (Shi Y. et al., 2020). These findings provide novel insights into HCMV-related cardiovascular diseases.
Ncrnas Serve as Potential Biomarkers of Human Cytomegalovirus Infection
Accumulating evidence indicated that HCMV is involved in the occurrence and development of numerous diseases. Thus, it’s essential to screen out biomarkers of HCMV infection. With the development of the sequencing technique, a growing number of specific ncRNAs were found in HCMV infections. These ncRNAs not only mediated cellular events induced by HCMV and also could be emerged as potent biomarkers.
It was reported that the serum level of miR-US4-1 could be used to classify chronic hepatitis B (CHB) patients who were and were not responsive to IFN-α treatment with a correct rate of 84.00 and 71.74%, respectively (Pan Y. et al., 2016). Thus, miR-US4-1 could serve as a novel biomarker for predicting the outcome of a CHB patient’s treatment with IFNα. The plasma level of miR-US25-1-5p was significantly increased in pregnant women with adverse pregnancy outcomes (APOs), and the sensitivity and specificity were 68 and 71%, respectively (Gao et al., 2021). This miRNA could be a potential non-invasive biomarker for monitoring APOs during HCMV infection. miR-UL22A-5p is strongly linked with solid organ transplant patients’ specific virologic and clinical outcomes (Lisboa et al., 2015). Levels of miR-US25-1-5p and miR-UL112-3p in serum from infants with HCMV active infection were significantly correlated with liver damage (Zhang et al., 2020). Thus, these ncRNAs could further serve as biomarkers to monitor HCMV related diseases. Moreover, miR-US4-5p and miR-UL112-3p could be selected for cytomegalovirus diagnosis and follow-up (Mohammad et al., 2014; Caputo et al., 2019).
Like the ncRNAs encoded by the HCMV genome, the ncRNAs derived from the host genome could also be denoted as biomarkers. Kawano et al. (2016) revealed that plasma levels of miR-183-5p and miR-210-3p were significantly higher in patients with congenital CMV infection. miR-155 showed a significant difference between kidney transplant patients negative for HCMV infection and positive patients (Bergallo et al., 2018). In addition, the levels of circular RNAs hsa_circ_0001445 and hsa_circ_0001206 were significantly different in HCMV-infected patients vs. normal controls (Lou et al., 2019). These findings suggested that these ncRNAs could potentially serve as biomarkers of HCMV related disease.
Conclusion and Future Perspectives
ncRNAs exert multiple functions by regulating the expression level of cellular proteins (Deng et al., 2021). HCMV infection changed the expression profiles of ncRNAs, which was the leading cause of HCMV related diseases (Zhang et al., 2016; Mohammad et al., 2017; Lou et al., 2019). The previous studies revealed the key regulatory effect of ncRNAs in HCMV infections. Meanwhile, these findings also provided novel targets for HCMV treatment. For example, miR-221 alleviated CMV-induced tissue injury by promoting IFN- I and ISGs production (Yan et al., 2019). Furthermore, an inhibitor for miR-UL36-5p remarkedly reduced apoptosis mediated by miR-UL36-5p (Guo et al., 2015). Thus, ncRNAs could be developed as targets for anti-HCMV treatment. It has been proved that targeted ncRNA can effectively treat HCMV related disease in cell and animal models, but none clinical trials are reported so far. We sees a promising future for treatment HCMV related diseases by targeting ncRNAs in facilitating the translation of basic science to the clinical setting. However, how to specifically and efficiently regulate the targeted ncRNAs for HCMV therapy still need further studies.
Author Contributions
ZY and BW: project administration and funding acquisition. ZY, JW, XZ, SJ, and WS: writing—original draft preparation. ZY, FN, and BW: writing—review and editing. All authors have read and agreed to the published version of the manuscript.
Funding
This review was supported by BW. This research was funded by the Qingdao Postdoctoral Application Research Project (Grant No. RZ2100001326), Shandong Provincial SCIENCE and Technology Foundation (Grant No. 2019JZZY011009), Qingdao Municipal Science and Technology Foundation (Grant No. 20-2-3-4-nsh), and the National Key Research and Development Program of China (Grant No. 2018YFA0900802).
Conflict of Interest
The authors declare that the research was conducted in the absence of any commercial or financial relationships that could be construed as a potential conflict of interest.
Publisher’s Note
All claims expressed in this article are solely those of the authors and do not necessarily represent those of their affiliated organizations, or those of the publisher, the editors and the reviewers. Any product that may be evaluated in this article, or claim that may be made by its manufacturer, is not guaranteed or endorsed by the publisher.
Abbreviations
HCMV, human cytomegalovirus; ncRNAs, non-coding RNAs; lncRNA, long non-coding RNA; circRNA, circular RNA; FOXO3, fork head box O3; IRF7, interferon regulatory factor 7; IER5, immediate early response gene 5; IE2, immediate early protein 2; VAC, virion assembly compartment; GATA-2, GATA binding protein 2; EGFR, epidermal growth factor receptor; GAB1, GRB2 associated binding protein 1; MEK, MAP kinse-ERK kinase; ERK, extracellular regulated MAP kinase; EGR1, early growth response 1; HUVECs, human umbilical vein endothelial cells; MOAP1, modulator of apoptosis 1; PHAP, putative HLA-DR-associated proteins; ERN1, endoplasmic reticulum to nucleus signaling 1; IEX-1, immediate early gene X-1; ANT3, adenine nucleotide translocator 3; HPCs, hematopoietic progenitor cells; QARS, glutaminyl-tRNA Synthetase; BCL2L11, BCL2 like 11; BRCC3, BRCA1/BRCA2-containing complex subunit 3; EID1, EP300 interacting inhibitor of differentiation 1; MAPRE2, microtubule associated protein RP/EB family member 2; TUSC3, tumor suppressor candidate 3; NSG, NOD scid gamma; GBM, glioblastoma multiforme; CSC, cancer stem cells; SIRT1, sirtuin 1; Enos, nitric oxide synthase; Sox2, SRY-box transcription factor 2; TLR2, toll like receptor 2; NAB1, NGFI-A binding protein; TGF- β, transforming growth factor beta; RANTES, Regulated on activation, normal T-cell expressed and secreted; CTLs, cytotoxic T lymphocytes; HLA-E, major histocompatibility complexclass IE; NF- κ B, nuclear factor kappa B; ISGs, IFN-stimulated genes; STAT1, signal transducer and activator of transcription 1; SOCS1, suppressor of cytokine signaling 1; IFN, type I interferon; NLRP3, NLR family pyrin domain containing 3; MCMV, murine cytomegalovirus; ET-1, endothelin 1; Ednra, endothelin A receptor; CHB, chronic hepatitis B; APOs, adverse pregnancy outcomes.
References
Babu, S. G., Pandeya, A., Verma, N., Shukla, N., Kumar, R. V., and Saxena, S. (2014). Role of HCMV miR-UL70-3p and miR-UL148D in overcoming the cellular apoptosis. Mol. Cell Biochem. 393, 89–98. doi: 10.1007/s11010-014-2049-8
Bergallo, M., Daprà, V., Calvi, C., Montanari, P., Galliano, I., and Ravanini, P. (2018). Is HERV-K and HERV-W Expression Regulated by miR-155 in Kidney Transplant Patients with Human Cytomegalovirus Infection? Intervirology 61, 23–29. doi: 10.1159/000490057
Caputo, T. M., Cummaro, A., Lettera, V., Mazzarotta, A., Battista, E., Netti, P. A., et al. (2019). One-step scalable fluorescent microgel bioassay for the ultrasensitive detection of endogenous viral miR-US4-5p. Analyst 144, 1369–1378. doi: 10.1039/c8an02166j
Chen, J., Xia, S., Yang, X., Chen, H., Li, F., Liu, F., et al. (2017). Human Cytomegalovirus Encoded miR-US25-1-5p Attenuates CD147/EMMPRIN-Mediated Early Antiviral Response. Viruses 9:7
Chen, Q., Li, Y., Liu, Y., Xu, W., and Zhu, X. (2021). Exosomal Non-coding RNAs-Mediated Crosstalk in the Tumor Microenvironment. Front. Cell Dev. Biol. 9:646864. doi: 10.3389/fcell.2021.646864
Deng, J., Huang, Y., Wang, Q., Li, J., Ma, Y., Qi, Y., et al. (2021). Human Cytomegalovirus Influences Host circRNA Transcriptions during Productive Infection. Virol Sin. 36, 241–253.
Deshpande, R. P., Panigrahi, M. Y. B. V. K. C., and Babu, P. P. (2018). Profiling of microRNAs modulating cytomegalovirus infection in astrocytoma patients. Neurol. Sci. 39, 1895–1902. doi: 10.1007/s10072-018-3518-8
Diggins, N. L., Crawford, L. B., Hancock, M. H., Mitchell, J., and Nelson, J. A. (2021). Human Cytomegalovirus miR-US25-1 Targets the GTPase RhoA To Inhibit CD34(+) Hematopoietic Progenitor Cell Proliferation To Maintain the Latent Viral Genome. mBio 12, e00621–21. doi: 10.1128/mBio.00621-21
Ding, M., Wang, X., Wang, C., Liu, X., Zen, K., Wang, W., et al. (2017). Distinct expression profile of HCMV encoded miRNAs in plasma from oral lichen planus patients. J. Transl. Med. 15:133. doi: 10.1186/s12967-017-1222-8
Dziurzynski, K., Chang, S. M., Heimberger, A. B., Kalejta, R. F., Mcgregor Dallas, S. R., Smit, M., et al. (2012). Consensus on the role of human cytomegalovirus in glioblastoma. Neuro Oncol. 14, 246–255. doi: 10.1093/neuonc/nor227
Fan, J., Zhang, W., and Liu, Q. (2014). Human cytomegalovirus-encoded miR-US25-1 aggravates the oxidised low density lipoprotein-induced apoptosis of endothelial cells. Biomed. Res. Int. 2014:531979. doi: 10.1155/2014/531979
Fatica, A., and Bozzoni, I. (2014). Long non-coding RNAs: new players in cell differentiation and development. Nat. Rev. Genet. 15, 7–21. doi: 10.1038/nrg3606
Fu, M., Gao, Y., Zhou, Q., Zhang, Q., Peng, Y., Tian, K., et al. (2014). Human cytomegalovirus latent infection alters the expression of cellular and viral microRNA. Gene 536, 272–278. doi: 10.1016/j.gene.2013.12.012
Fu, Y. R., Liu, X. J., Li, X. J., Shen, Z. Z., Yang, B., Wu, C. C., et al. (2015). MicroRNA miR-21 attenuates human cytomegalovirus replication in neural cells by targeting Cdc25a. J. Virol. 89, 1070–1082. doi: 10.1128/JVI.01740-14
Gao, Z., Zhou, L., Bai, J., Ding, M., Liu, D., Zheng, S., et al. (2021). Assessment of HCMV-encoded microRNAs in plasma as potential biomarkers in pregnant women with adverse pregnancy outcomes. Ann. Transl. Med. 9:638. doi: 10.21037/atm-20-7354
Gatherer, D., Seirafian, S., Cunningham, C., Holton, M., Dargan, D. J., Baluchova, K., et al. (2011). High-resolution human cytomegalovirus transcriptome. Proc. Natl. Acad. Sci. U. S. A. 108, 19755–19760. doi: 10.1073/pnas.1115861108
Grey, F., Meyers, H., White, E. A., Spector, D. H., and Nelson, J. (2007). A human cytomegalovirus-encoded microRNA regulates expression of multiple viral genes involved in replication. PLoS Pathog. 3:e163. doi: 10.1371/journal.ppat.0030163
Grey, F., Tirabassi, R., Meyers, H., Wu, G., Mcweeney, S., Hook, L., et al. (2010). A viral microRNA down-regulates multiple cell cycle genes through mRNA 5’UTRs. PLoS Pathog. 6:e1000967. doi: 10.1371/journal.ppat.1000967
Guo, X., Huang, Y., Qi, Y., Liu, Z., Ma, Y., Shao, Y., et al. (2015). Human cytomegalovirus miR-UL36-5p inhibits apoptosis via downregulation of adenine nucleotide translocator 3 in cultured cells. Arch. Virol. 160, 2483–2490. doi: 10.1007/s00705-015-2498-8
Han, S. H., Kumar, D., Ferreira, V. H., Egli, A., Hirsch, H. H., Weisser, M., et al. (2017). Human MicroRNA Responses Predict Cytomegalovirus Replication Following Solid Organ Transplantation. J. Infect. Dis. 215, 537–546. doi: 10.1093/infdis/jiw596
Hancock, M. H., Crawford, L. B., Perez, W., Struthers, H. M., Mitchell, J., and Caposio, P. (2021). Human Cytomegalovirus UL7, miR-US5-1, and miR-UL112-3p Inactivation of FOXO3a Protects CD34(+) Hematopoietic Progenitor Cells from Apoptosis. mSphere 6, e986–e920. doi: 10.1128/mSphere.00986-20
Hancock, M. H., Crawford, L. B., Pham, A. H., Mitchell, J., Struthers, H. M., Yurochko, A. D., et al. (2020a). Human Cytomegalovirus miRNAs Regulate TGF-ß to Mediate Myelosuppression while Maintaining Viral Latency in CD34(+) Hematopoietic Progenitor Cells. Cell Host Microbe 27, 104–114.e4. doi: 10.1016/j.chom.2019.11.013
Hancock, M. H., Mitchell, J., Goodrum, F. D., and Nelson, J. A. (2020b). Human Cytomegalovirus miR-US5-2 Downregulation of GAB1 Regulates Cellular Proliferation and UL138 Expression through Modulation of Epidermal Growth Factor Receptor Signaling Pathways. mSphere 5, e582–e520. doi: 10.1128/mSphere.00582-20
Hancock, M. H., Hook, L. M., Mitchell, J., and Nelson, J. A. (2017). Human Cytomegalovirus MicroRNAs miR-US5-1 and miR-UL112-3p Block Proinflammatory Cytokine Production in Response to NF-κB-Activating Factors through Direct Downregulation of IKKa and IKKß. mBio 8, e109–e117. doi: 10.1128/mBio.00109-17
He, X., Teng, J., Cui, C., Li, D., and Wen, L. (2018). MicroRNA-182 inhibits HCMV replication through activation of type I IFN response by targeting FOXO3 in neural cells. Exp. Cell Res. 369, 197–207. doi: 10.1016/j.yexcr.2018.05.019
Hombach, S., and Kretz, M. (2016). Non-coding RNAs: classification, Biology and Functioning. Adv. Exp. Med. Biol. 937, 3–17. doi: 10.1007/978-3-319-42059-2_1
Hook, L. M., Grey, F., Grabski, R., Tirabassi, R., Doyle, T., Hancock, M., et al. (2014). Cytomegalovirus miRNAs target secretory pathway genes to facilitate formation of the virion assembly compartment and reduce cytokine secretion. Cell Host Microbe 15, 363–373. doi: 10.1016/j.chom.2014.02.004
Huang, Y., Chen, D., He, J., Cai, J., Shen, K., Liu, X., et al. (2015). Hcmv-miR-UL112 attenuates NK cell activity by inhibition type I interferon secretion. Immunol. Lett. 163, 151–156. doi: 10.1016/j.imlet.2014.12.003
Huang, Y., Qi, Y., Ma, Y., He, R., Ji, Y., Sun, Z., et al. (2013a). Down-regulation of human cytomegalovirus UL138, a novel latency-associated determinant, by hcmv-miR-UL36. J. Biosci. 38, 479–485. doi: 10.1007/s12038-013-9353-4
Huang, Y., Qi, Y., Ma, Y., He, R., Ji, Y., Sun, Z., et al. (2013b). The expression of interleukin-32 is activated by human cytomegalovirus infection and down regulated by hcmv-miR-UL112-1. Virol J. 10:51. doi: 10.1186/1743-422X-10-51
Jameus, A., Kennedy, A. E., and Thome, C. (2021). Hematological Changes Following Low Dose Radiation Therapy and Comparison to Current Standard of Care Cancer Treatments. Dose Response 19:15593258211056196. doi: 10.1177/15593258211056196
Jiang, S., Qi, Y., He, R., Huang, Y., Liu, Z., Ma, Y., et al. (2015). Human cytomegalovirus microRNA miR-US25-1-5p inhibits viral replication by targeting multiple cellular genes during infection. Gene 570, 108–114. doi: 10.1016/j.gene.2015.06.009
Jiang, S., Huang, Y., Qi, Y., He, R., Liu, Z., Ma, Y., et al. (2017). Human cytomegalovirus miR-US5-1 inhibits viral replication by targeting Geminin mRNA. Virol. Sin. 32, 431–439. doi: 10.1007/s12250-017-4064-x
Kawano, Y., Kawada, J., Kamiya, Y., Suzuki, M., Torii, Y., Kimura, H., et al. (2016). Analysis of circulating human and viral microRNAs in patients with congenital cytomegalovirus infection. J. Perinatol. 36, 1101–1105. doi: 10.1038/jp.2016.157
Kim, S., Lee, S., Shin, J., Kim, Y., Evnouchidou, I., Kim, D., et al. (2011). Human cytomegalovirus microRNA miR-US4-1 inhibits CD8(+) T cell responses by targeting the aminopeptidase ERAP1. Nat. Immunol. 12, 984–991. doi: 10.1038/ni.2097
Kim, Y., Lee, S., Kim, S., Kim, D., Ahn, J. H., and Ahn, K. (2012). Human cytomegalovirus clinical strain-specific microRNA miR-UL148D targets the human chemokine RANTES during infection. PLoS Pathog 8:e1002577. doi: 10.1371/journal.ppat.1002577
Kumar, A., Tripathy, M. K., Pasquereau, S., Al Moussawi, F., Abbas, W., Coquard, L., et al. (2018). The Human Cytomegalovirus Strain DB Activates Oncogenic Pathways in Mammary Epithelial Cells. EBioMedicine 30, 167–183. doi: 10.1016/j.ebiom.2018.03.015
Landais, I., Pelton, C., Streblow, D., Defilippis, V., Mcweeney, S., and Nelson, J. A. (2015). Human Cytomegalovirus miR-UL112-3p Targets TLR2 and Modulates the TLR2/IRAK1/NFκB Signaling Pathway. PLoS Pathog 11:e1004881. doi: 10.1371/journal.ppat.1004881
Lau, B., Kerr, K., Gu, Q., Nightingale, K., Antrobus, R., Suárez, N. M., et al. (2020). Human Cytomegalovirus Long Non-coding RNA1.2 Suppresses Extracellular Release of the Pro-inflammatory Cytokine IL-6 by Blocking NF-κB Activation. Front. Cell Infect. Microbiol. 10:361. doi: 10.3389/fcimb.2020.00361
Lee, K. H., Lim, B. J., Ferreira, V. H., Min, S. Y., Hong, Y. M., Jo, J. H., et al. (2018). Expression of human miR-200b-3p and -200c-3p in cytomegalovirus-infected tissues. Biosci. Rep. 38:7 doi: 10.1042/BSR20180961
Liang, J., Zhang, L., and Cheng, W. (2021). Non-coding RNA-mediated autophagy in cancer: a protumor or antitumor factor? Biochim. Biophys Acta Rev. Cancer 1876:188642. doi: 10.1016/j.bbcan.2021.188642
Liang, Q., Wang, K., Wang, B., and Cai, Q. (2017). HCMV-encoded miR-UL112-3p promotes glioblastoma progression via tumour suppressor candidate 3. Sci. Rep. 7:44705. doi: 10.1038/srep44705
Lisboa, L. F., Egli, A., O’shea, D., Åsberg, A., Hartmann, A., Rollag, H., et al. (2015). Hcmv-miR-UL22A-5p: a Biomarker in Transplantation With Broad Impact on Host Gene Expression and Potential Immunological Implications. Am. J. Transplant 15, 1893–1902. doi: 10.1111/ajt.13222
Liu, N., Wang, Z. Z., Zhao, M., Zhang, Y., and Chen, N. H. (2020). Role of non-coding RNA in the pathogenesis of depression. Gene 735:144276. doi: 10.1016/j.gene.2019.144276
Lou, Y. Y., Wang, Q. D., Lu, Y. T., Tu, M. Y., Xu, X., Xia, Y., et al. (2019). Differential circRNA expression profiles in latent human cytomegalovirus infection and validation using clinical samples. Physiol. Genomics. 51, 51–58. doi: 10.1152/physiolgenomics.00096.2018
Meshesha, M. K., Bentwich, Z., Solomon, S. A., and Avni, Y. S. (2016). In vivo expression of human cytomegalovirus (HCMV) microRNAs during latency. Gene 575, 101–107. doi: 10.1016/j.gene.2015.08.040
Mikell, I., Crawford, L. B., Hancock, M. H., Mitchell, J., Buehler, J., Goodrum, F., et al. (2019). HCMV miR-US22 down-regulation of EGR-1 regulates CD34+ hematopoietic progenitor cell proliferation and viral reactivation. PLoS Pathog. 15:e1007854. doi: 10.1371/journal.ppat.1007854
Mohammad, A. A., Costa, H., Landázuri, N., Lui, W. O., Hultenby, K., Rahbar, A., et al. (2017). Human cytomegalovirus microRNAs are carried by virions and dense bodies and are delivered to target cells. J. Gen. Virol. 98, 1058–1072. doi: 10.1099/jgv.0.000736
Mohammad, A. A., Rahbar, A., Lui, W. O., Davoudi, B., Catrina, A., Stragliotto, G., et al. (2014). Detection of circulating hcmv-miR-UL112-3p in patients with glioblastoma, rheumatoid arthritis, diabetes mellitus and healthy controls. PLoS One 9:e113740. doi: 10.1371/journal.pone.0113740
Mohapatra, S., Pioppini, C., Ozpolat, B., and Calin, G. A. (2021). Non-coding RNAs regulation of macrophage polarization in cancer. Mol. Cancer 20:24. doi: 10.1186/s12943-021-01313-x
Nachmani, D., Zimmermann, A., Oiknine Djian, E., Weisblum, Y., Livneh, Y., Khanh Le, V. T., et al. (2014). MicroRNA editing facilitates immune elimination of HCMV infected cells. PLoS Pathog 10:e1003963. doi: 10.1371/journal.ppat.1003963
Naqvi, A. R., Shango, J., Seal, A., Shukla, D., and Nares, S. (2018). Viral miRNAs alter host cell miRNA profiles and modulate innate immune responses. Front. Immunol. 9:433. doi: 10.3389/fimmu.2018.00433
O’Connor, C. M., Vanicek, J., and Murphy, E. A. (2014). Host microRNA regulation of human cytomegalovirus immediate early protein translation promotes viral latency. J. Virol. 88, 5524–5532. doi: 10.1128/JVI.00481-14
Pan, C., Zhu, D., Wang, Y., Li, L., Li, D., Liu, F., et al. (2016). Human Cytomegalovirus miR-UL148D Facilitates Latent Viral Infection by Targeting Host Cell Immediate Early Response Gene 5. PLoS Pathog 12:e1006007. doi: 10.1371/journal.ppat.1006007
Pan, Y., Wang, N., Zhou, Z., Liang, H., Pan, C., Zhu, D., et al. (2016). Circulating human cytomegalovirus-encoded HCMV-miR-US4-1 as an indicator for predicting the efficacy of IFNa treatment in chronic hepatitis B patients. Sci. Rep. 6:23007. doi: 10.1038/srep23007
Perera, M. R., Roche, K. L., Murphy, E. A., and Sinclair, J. H. (2022). A Viral Long Non-Coding RNA Protects against Cell Death during Human Cytomegalovirus Infection of CD14+ Monocytes. Viruses 14:246. doi: 10.3390/v14020246
Poole, E., Avdic, S., Hodkinson, J., Jackson, S., Wills, M., Slobedman, B., et al. (2014). Latency-associated viral interleukin-10 (IL-10) encoded by human cytomegalovirus modulates cellular IL-10 and CCL8 Secretion during latent infection through changes in the cellular microRNA hsa-miR-92a. J. Virol. 88, 13947–13955. doi: 10.1128/JVI.02424-14
Poole, E., Mcgregor Dallas, S. R., Colston, J., Joseph, R. S. V., and Sinclair, J. (2011). Virally induced changes in cellular microRNAs maintain latency of human cytomegalovirus in CD34+ progenitors. J. Gen. Virol. 92, 1539–1549. doi: 10.1099/vir.0.031377-0
Qi, M., Qi, Y., Ma, Y., He, R., Ji, Y., Sun, Z., et al. (2013). Over-expression of human cytomegalovirus miR-US25-2-3p downregulates eIF4A1 and inhibits HCMV replication. FEBS Lett. 587, 2266–2271.
Reeves, M. B., Davies, A. A., Mcsharry, B. P., Wilkinson, G. W., and Sinclair, J. H. (2007). Complex I binding by a virally encoded RNA regulates mitochondria-induced cell death. Science 316, 1345–1348. doi: 10.1126/science.1142984
Romania, P., Cifaldi, L., Pignoloni, B., Starc, N., D’alicandro, V., Melaiu, O., et al. (2017). Identification of a Genetic Variation in ERAP1 Aminopeptidase that Prevents Human Cytomegalovirus miR-UL112-5p-Mediated Immunoevasion. Cell Rep. 20, 846–853. doi: 10.1016/j.celrep.2017.06.084
Rossetto, C. C., Tarrant-Elorza, M., and Pari, G. S. (2013). Cis and trans acting factors involved in human cytomegalovirus experimental and natural latent infection of CD14 (+) monocytes and CD34 (+) cells. PLoS Pathog. 9:e1003366. doi: 10.1371/journal.ppat.1003366
Shao, Y., Qi, Y., Huang, Y., Liu, Z., Ma, Y., Guo, X., et al. (2016). Human cytomegalovirus-encoded miR-US4-1 promotes cell apoptosis and benefits discharge of infectious virus particles by targeting QARS. J. Biosci. 41, 183–192. doi: 10.1007/s12038-016-9605-1
Shen, K., Xu, L., Chen, D., Tang, W., and Huang, Y. (2018). Human cytomegalovirus-encoded miR-UL112 contributes to HCMV-mediated vascular diseases by inducing vascular endothelial cell dysfunction. Virus Genes 54, 172–181. doi: 10.1007/s11262-018-1532-9
Shi, L., Fan, B., Chen, D., Guo, C., Xiang, H., Nie, Y., et al. (2020). Human cytomegalovirus protein UL136 activates the IL-6/STAT3 signal through MiR-138 and MiR-34c in gastric cancer cells. Int. J. Clin. Oncol. 25, 1936–1944. doi: 10.1007/s10147-020-01749-z
Shi, Y., Xi, D., Zhang, X., Huang, Z., Tang, N., Liu, Y., et al. (2020). Screening and validation of differentially expressed microRNAs and target genes in hypertensive mice induced by cytomegalovirus infection. Biosci. Rep. 40:BSR20202387. doi: 10.1042/BSR20202387
Shnayder, M., Nachshon, A., Krishna, B., Poole, E., Boshkov, A., Binyamin, A., et al. (2018). Defining the Transcriptional Landscape during Cytomegalovirus Latency with Single-Cell RNA Sequencing. mBio 9, e00013–e18. doi: 10.1128/mBio.00013-18
Song, J., Ma, Q., Hu, M., Qian, D., Wang, B., and He, N. (2018). The Inhibition of miR-144-3p on Cell Proliferation and Metastasis by Targeting TOP2A in HCMV-Positive Glioblastoma Cells. Molecules 23:3259. doi: 10.3390/molecules23123259
Soroceanu, L., Matlaf, L., Khan, S., Akhavan, A., Singer, E., Bezrookove, V., et al. (2015). Cytomegalovirus Immediate-Early Proteins Promote Stemness Properties in Glioblastoma. Cancer Res. 75, 3065–3076. doi: 10.1158/0008-5472.CAN-14-3307
Stern-Ginossar, N., Saleh, N., Goldberg, M. D., Prichard, M., Wolf, D. G., and Mandelboim, O. (2009). Analysis of human cytomegalovirus-encoded microRNA activity during infection. J. Virol. 83, 10684–10693.
Stern-Ginossar, N., Weisburd, B., Michalski, A., Le, V. T., Hein, M. Y., Huang, S. X., et al. (2012). Decoding human cytomegalovirus. Science 338, 1088–1093. doi: 10.1126/science.1227919
Tai-Schmiedel, J., Karniely, S., Lau, B., Ezra, A., Eliyahu, E., Nachshon, A., et al. (2020). Human cytomegalovirus long noncoding RNA4.9 regulates viral DNA replication. PLoS Pathog. 16:e1008390. doi: 10.1371/journal.ppat.1008390
Tan Gana, N. H., Onuki, T., Victoriano, A. F., and Okamoto, T. (2012). MicroRNAs in HIV-1 infection: an integration of viral and cellular interaction at the genomic level. Front. Microbiol. 3:306. doi: 10.3389/fmicb.2012.00306
Ulasov, I. V., Kaverina, N. V., Ghosh, D., Baryshnikova, M. A., Kadagidze, Z. G., Karseladze, A. I., et al. (2017). CMV70-3P miRNA contributes to the CMV mediated glioma stemness and represents a target for glioma experimental therapy. Oncotarget 8, 25989–25999. doi: 10.18632/oncotarget.11175
Wang, F. Z., Weber, F., Croce, C., Liu, C. G., Liao, X., and Pellett, P. E. (2008). Human cytomegalovirus infection alters the expression of cellular microRNA species that affect its replication. J. Virol. 82, 9065–9074. doi: 10.1128/JVI.00961-08
Wang, L., Yang, M., Liao, S., Liu, W., Dai, G., Wu, G., et al. (2017). Hsa-miR-27b is up-regulated in cytomegalovirus-infected human glioma cells, targets engrailed-2 and inhibits its expression. Exp. Biol. Med. 242, 1227–1233. doi: 10.1177/1535370217699535
Wang, Y., Zhao, P., Qian, D., Hu, M., Zhang, L., Shi, H., et al. (2017). MicroRNA-613 is downregulated in HCMV-positive glioblastoma and inhibits tumour progression by targeting arginase-2. Tumour Biol. 39:1010428317712512. doi: 10.1177/1010428317712512
Wang, Y. P., Qi, Y., Huang, Y. J., Qi, M. L., Ma, Y. P., He, R., et al. (2013). Identification of immediate early gene X-1 as a cellular target gene of hcmv-mir-UL148D. Int. J. Mol. Med. 31, 959–966. doi: 10.3892/ijmm.2013.1271
Waters, S., Lee, S., Munyard, K., Irish, A., Price, P., and Wang, B. H. (2020). Human Cytomegalovirus-Encoded microRNAs Can Be Found in Saliva Samples from Renal Transplant Recipients. Noncoding RNA 6:50. doi: 10.3390/ncrna6040050
Yan, B., Ma, H., Jiang, S., Shi, J., Yang, Z., Zhu, W., et al. (2019). microRNA-221 restricts human cytomegalovirus replication via promoting type I IFN production by targeting SOCS1/NF-κB pathway. Cell Cycle 18, 3072–3084. doi: 10.1080/15384101.2019.1667706
Yu, Z., Wang, Y., Liu, L., Zhang, X., Jiang, S., and Wang, B. (2021). Apoptosis Disorder, a Key Pathogenesis of HCMV-Related Diseases. Int. J. Mol. Sci. 22:4106. doi: 10.3390/ijms22084106
Zhang, J., Huang, Y., Wang, Q., Ma, Y., Qi, Y., Liu, Z., et al. (2020). Levels of human cytomegalovirus miR-US25-1-5p and miR-UL112-3p in serum extracellular vesicles from infants with HCMV active infection are significantly correlated with liver damage. Eur. J. Clin. Microbiol. Infect Dis. 39, 471–481. doi: 10.1007/s10096-019-03747-0
Zhang, Q., Lai, M. M., Lou, Y. Y., Guo, B. H., Wang, H. Y., and Zheng, X. Q. (2016). Transcriptome altered by latent human cytomegalovirus infection on THP-1 cells using RNA-seq. Gene 594, 144–150. doi: 10.1016/j.gene.2016.09.014
Zhang, Q., Song, X., Ma, P., Lv, L., Zhang, Y., Deng, J., et al. (2021). Human Cytomegalovirus miR-US33as-5p Targets IFNAR1 to Achieve Immune Evasion During Both Lytic and Latent Infection. Front. Immunol. 12:628364. doi: 10.3389/fimmu.2021.628364
Zhang, S., Liu, L., Wang, R., Tuo, H., Guo, Y., Yi, L., et al. (2013a). MicroRNA-217 promotes angiogenesis of human cytomegalovirus-infected endothelial cells through downregulation of SIRT1 and FOXO3A. PLoS One 8:e83620. doi: 10.1371/journal.pone.0083620
Zhang, S., Liu, L., Wang, R., Tuo, H., Guo, Y., Yi, L., et al. (2013b). MiR-199a-5p promotes migration and tube formation of human cytomegalovirus-infected endothelial cells through downregulation of SIRT1 and eNOS. Arch. Virol. 158, 2443–2452. doi: 10.1007/s00705-013-1744-1
Zhang, S., Liu, L., Wang, R., Tuo, H., Guo, Y., Yi, L., et al. (2017). miR-138 promotes migration and tube formation of human cytomegalovirus-infected endothelial cells through the SIRT1/p-STAT3 pathway. Arch. Virol. 162, 2695–2704. doi: 10.1007/s00705-017-3423-0
Zhao, T., Du, J., and Zeng, H. (2020). Interplay between endoplasmic reticulum stress and non-coding RNAs in cancer. J. Hematol. Oncol. 13:163. doi: 10.1186/s13045-020-01002-0
Zhou, B., Yang, H., Yang, C., Bao, Y. L., Yang, S. M., Liu, J., et al. (2021). Translation of noncoding RNAs and cancer. Cancer Lett. 497, 89–99.
Zhou, W., Xi, D., Shi, Y., Wang, L., Zhong, H., Huang, Z., et al. (2021). MicroRNA-1929-3p participates in murine cytomegalovirus-induced hypertensive vascular remodeling through Ednra/NLRP3 inflammasome activation. Int. J. Mol. Med. 47, 719–731.
Zhou, W., Wang, C., Ding, M., Bian, Y., Zhong, Y., Shen, H., et al. (2020). Different expression pattern of human cytomegalovirus-encoded microRNAs in circulation from virus latency to reactivation. J. Transl. Med. 18:469. doi: 10.1186/s12967-020-02653-w
Zhou, Y., Yong, H., Cui, W., Chu, S., Li, M., Li, Z., et al. (2022). Long noncoding RNA SH3PXD2A-AS1 promotes NSCLC proliferation and accelerates cell cycle progression by interacting with DHX9. Cell Death Discov. 8:192. doi: 10.1038/s41420-022-01004-6
Zhu, L., Li, N., Sun, L., Zheng, D., and Shao, G. (2021). Non-coding RNAs: The key detectors and regulators in cardiovascular disease. Genomics 113, 1233–1246. doi: 10.1016/j.ygeno.2020.10.024
Zuraini, N. Z. A., Sekar, M., Wu, Y. S., Gan, S. H., Bonam, S. R., Mat Rani, N. N. I., et al. (2021). Promising Nutritional Fruits Against Cardiovascular Diseases: an Overview of Experimental Evidence and Understanding Their Mechanisms of Action. Vasc. Health Risk Manag. 17, 739–769. doi: 10.2147/VHRM.S328096
Keywords: HCMV, ncRNAs, cellular events, target therapy, aberrant expression
Citation: Yu Z, Wang J, Nan F, Shi W, Zhang X, Jiang S and Wang B (2022) Human Cytomegalovirus Induced Aberrant Expression of Non-coding RNAs. Front. Microbiol. 13:918213. doi: 10.3389/fmicb.2022.918213
Received: 12 April 2022; Accepted: 26 May 2022;
Published: 13 June 2022.
Edited by:
Patricia Fitzgerald-Bocarsly, The State University of New Jersey, United StatesReviewed by:
Qiliang Cai, Fudan University, ChinaBenjamin Anthony Krishna, Lerner Research Institute, Cleveland Clinic, United States
Copyright © 2022 Yu, Wang, Nan, Shi, Zhang, Jiang and Wang. This is an open-access article distributed under the terms of the Creative Commons Attribution License (CC BY). The use, distribution or reproduction in other forums is permitted, provided the original author(s) and the copyright owner(s) are credited and that the original publication in this journal is cited, in accordance with accepted academic practice. No use, distribution or reproduction is permitted which does not comply with these terms.
*Correspondence: Bin Wang, d2FuZ2JpbjUzMkAxMjYuY29t