- 1School of Basic Medical Sciences and Forensic Medicine, Hangzhou Medical College, Hangzhou, China
- 2Hangzhou Chest Hospital, Zhejiang University School of Medicine, Hangzhou, China
- 3Department of Medical Microbiology and Parasitology, Zhejiang University School of Medicine, Hangzhou, China
Streptococcus pneumoniae is a common diplococcus pathogen found worldwide. The characterization of predominant serotypes, drug resistance, and virulence genes of S. pneumoniae isolates prevailing in different areas and countries is clinically important for choice of antibiotics and improvement of vaccines. In this study, pneumonia (78.7%) and meningitis (37.0%) were the predominant diseases observed in the 282 (children) and 27 (adults) S. pneumoniae-infected patients (p < 0.05) from seven hospitals in different areas of East China. Of the 309 pneumococcal isolates, 90.3% were classified by PCR into 15 serotypes, with serotypes 19F (27.2%) and the 6A/B (19.1%) being most predominant (p < 0.05). Importantly, serotypes 15A and 15B/C combined for a total of 10.4% of the isolates, but these serotypes are not included in the 13-valent pneumococcal capsule conjugate vaccine used in China. Antimicrobial susceptibility analysis by the E-test showed that >95% of the 309 pneumococcal isolates were susceptible to moxifloxacin and levofloxacin, as well as 18.4, 85.8, and 81.6% of the isolates displayed susceptibility to penicillin, cefotaxime, and imipenem, respectively. A significant correlation between the prevalence of predominant serotypes and their penicillin resistance was observed (p < 0.05). In particular, >95% of all the pneumococcal isolates showed resistance to erythromycin and azithromycin. Of the nine detected virulence genes, the lytA, ply, hysA, and nanA were the most common with 95–100% positive rates in the 309 pneumococcal isolates, while the pavA and psaA genes displayed a significant correlation with pneumococcal bacteremia and meningitis (p < 0.05). Overall, our data suggested that the predominant serotypes, drug resistance, and virulence genes of the S. pneumoniae isolates prevailing in East China are distinct from those observed in other areas of China and adjacent countries.
Introduction
Streptococcus pneumoniae is a common Gram-positive diplococcus pathogen that mainly causes pneumonia, upper respiratory tract infections (URTIs), and tympanitis in children (Dockrell et al., 2012; Korona-Glowniak et al., 2018; Oligbu et al., 2019). In the recent decade, S. pneumoniae has been frequently reported as the causative agent of community-acquired pneumonia, bacteremia, and meningitis in adults (Rozenbaum et al., 2013; Argemi et al., 2016; Oordt-Speets et al., 2018). However, the incidence of pneumococcal diseases in developing countries is significantly higher than in developed countries due to different economic and medical conditions (Amin et al., 2014; Droz et al., 2019).
Vaccination is the crucial measure to prevent from infections by microbial pathogens including S. pneumoniae. At present, the mainly used pneumococcal vaccine used in China is a 13-valent pneumococcal capsule conjugate containing the predominant or common serotypes of S. pneumoniae prevailing in China (Wang et al., 2021). However, the predominant serotypes of S. pneumoniae in different areas and countries are usually distinct and changed continually (Kim et al., 2020). Therefore, regular investigation of the predominant S. pneumoniae serotypes present in the different areas of China is necessary for control of pneumococcal diseases and improvement of pneumococcal vaccines.
β-Lactam antibiotics such as penicillin (PNC), cefotaxime (CTX), and imipenem (IPM), macrolide antibiotics such as erythromycin (ETM) and azithromycin (AZM), and quinolone antibiotics such as levofloxacin (LEV) and moxifloxacin (MXF) are generally used for treatment of pneumococcal diseases in clinics. Many epidemiological studies revealed that S. pneumoniae isolates from patients frequently display single or multiple drug resistance against different classes of antibiotics. S. pneumoniae drug resistance not only complicates antimicrobial therapy of pneumococcal diseases but also results in the specific prevalence of drug-resistant S. pneumoniae serotypes (Thummeepak et al., 2015; Nakano et al., 2016; Yahiaoui et al., 2016; Aliberti et al., 2019; Suaya et al., 2020). However, the drug resistance spectrum of S. pneumoniae isolates from different areas of China and the correlation between drug resistance and prevalence of different S. pneumoniae serotypes remain poorly characterized.
The S. pneumoniae capsule is its most important virulence factor because capsule-null strains are severely attenuated for their ability to cause any diseases in human and animals (van der Poll and Opal, 2009). Previous studies demonstrated that the capsule of S. pneumonias is able to resist the phagocytosis by phagocytes and promotes the pneumococcal colonization of host cells (Nelson et al., 2007; Brissac et al., 2021). Moreover, the capsule is the main surface antigen of S. pneumoniae and the basis for pneumococcal serotype classification (Weinberger et al., 2009). Except for capsule, S. pneumoniae can produce many other virulence factors for adherence, colonization, and invasion of hosts (Subramanian et al., 2019). For example, the products of the S. pneumoniae cbpA and 2A genes serve as an adherence factor and a component in the capsule, respectively (Zhao et al., 2019). The hyaluronidase expressed by the S. pneumoniae hysA gene is utilized for invasion into tissues of hosts (Marion et al., 2012). The autolysin, expressed by the S. pneumoniae lytA gene, is toxic due to the release of cell wall degradation products, which evokes inflammation reactions in hosts and Ply pneumolysin to induce DNA damage of cells (Mellro et al., 2014; Rai et al., 2016). The pneumococcal NanA neuraminidase promotes the formation of pneumococcal biofilms and facilitates the colonization by S. pneumoniae (Wren et al., 2017). PavA, a fibronectin-binding protein, mediates pneumococcal adherence to endothelial cells, while the PsaA, an adherence factor, is essential for the virulence of S. pneumoniae (Holmes et al., 2001; Romero-Steiner et al., 2003). Surface protein A, the product of the S. pneumoniae pspA gene, can inhibit complement-mediated phagocytosis after binding to lactoferrin (Croney et al., 2013). However, the correlation between these virulence factors and predominant serotypes of S. pneumoniae has not been well characterized.
In this study, 309 S. pneumoniae isolates collected from the seven hospitals in different areas in East China during 2021 were used for serotype classification, drug resistance detection, and virulence factor examination. The aim of this study was to understand the diversity of predominant pneumococcal diseases in children and adults, reveal the predominant pneumococcal serotypes prevailing in East China, and determine the correlations between predominant or common pneumococcal serotypes with their drug resistance and virulence factors. These results will be helpful for antibiotic choice during treatment of pneumococcal diseases and improvement of pneumococcal capsule conjugate vaccines by including capsular polysaccharide antigens from novel prevailing S. pneumoniae serotypes.
Materials and Methods
Separation and Identification of S. pneumoniae Isolates
Sputum, bronchoalveolar lavage fluid, peripheral blood, and cerebrospinal fluid samples of 4,665 patients suffering from bacterial URTI, pneumonia, tympanitis, bacteremia, or meningitis according to clinical signs and symptoms and laboratory examinations were collected in the year 2021 from the seven hospitals located in different areas of East China for bacterial isolated cultivation and identification. Briefly, each of the samples was inoculated on a Columbia blood agar plate (bioMérieux, France) and then incubated at 37°C with 5% CO2 for 24 h. Individual bacterial colonies grown on the agar plates were identified using the VITEK 2-Compact Auto-Bacterial Detector (bioMérieux). In this study, 309 cases of the patients’ samples (282 children and 27 adults) were isolated for S. pneumoniae, and all the 309 pneumococcal isolates were used. Besides, the S. pneumoniae isolates were confirmed again using the colony morphological examination, Gram staining, optochin test, and bile lysis test before use. In the examinations, staining, and tests, S. pneumoniae ATCC49619 was used as the control.
β-Lactamase Phenotype Confirmatory Test
β-Lactam antibiotics are the most commonly used antibacterial drugs in clinics. β-Lactamases play a critical role in bacterial resistance to β-lactam antibiotics. In this study, a β-lactamase phenotype confirmatory test was performed to detect the possible β-lactamases produced by the S. pneumoniae isolates using a Cephalothin Disk Kit (Oxoid, England) (Khan et al., 2014). The cephalothin disk became red rapidly within 5 min when a bacterial strain produced a β-lactamase. In the test, Staphylococcus aureus ATCC29213, S. aureus ATCC25923, and S. pneumoniae ATCC49619 were used as the positive and negative controls, respectively.
Serotyping of S. pneumoniae Isolates
The serotypes of 309 S. pneumoniae isolates were identified using PCR as previously reported (Pai et al., 2006; Dias et al., 2007; Da Gloria-Carvalho et al., 2010). Briefly, genomic DNA of the pneumococcal isolates was extracted using a Bacterial Genomic DNA MiniPrep Kit (Axygen, United States) and then quantified using ultraviolet spectrophotometry (Sun et al., 2010). Using 100 ng DNA of each of the genomic DNA samples as the template, separate PCRs were performed using a High-Fidelity Ex-Taq PCR Kit (TaKaRa, China) and deferent pairs of serotyping primers (Supplementary Table 1). The PCRs were initiated by incubation at 94°C for 5 min for DNA denaturation, followed by 35 cycles at 94°C for 30 s, 52–54°C for 30 s, and 72°C for 30–60 s to amplify the target gene fragments and finally an incubation step at 72°C for 7 min. The S. pneumoniae 16S rRNA gene and cpsA gene, a sequence-conserved capsule formation-determining gene, were used as the controls (El Aila et al., 2010; Coskun-Ari et al., 2012). For each of the genomic DNA samples, three independent PCR repeats were performed for serotype detection.
Drug Susceptibility Test
An E-test was used to detect the minimum inhibitory concentration (MIC) of PNC, CTX, IPM, ETM, AZM, LEV, and MXF for the 309 S. pneumoniae isolates. The obtained results were defined according to the 2021 Clinical and Laboratory Standards Institute (CLSI) Guideline M100-Ed31. Strains such as S. aureus ATCC25923, Escherichia coli ATCC25922, and S. pneumoniae ATCC49619 were used as the controls.
Detection of Virulence Genes in S. pneumoniae Isolates
Although S. pneumoniae does not produce endotoxin [i.e., lipopolysaccharide (LPS)] and typical exotoxins, this pathogenic diplococcus has many other virulence genes in its genome, such as the cbpA, cps2A, hysA, lytA, nanA, pavA, ply, psaA, and pspA genes, which encode products important for adherence, colonization, invasion, and survival (Holmes et al., 2001; Romero-Steiner et al., 2003; Marion et al., 2012; Croney et al., 2013; Mellro et al., 2014; Rai et al., 2016; Wren et al., 2017; Subramanian et al., 2019; Zhao et al., 2019). The presence of these nine virulence genes in each of the 309 S. pneumoniae isolates was detected by individual PCRs using a High-Fidelity Ex-Taq PCR Kit (TaKaRa) and deferent pairs of primers (Supplementary Table 2). The PCRs were initiated by incubation at 94°C for 5 min for DNA denaturation, followed by 35 cycles at 94°C for 30 s, 52–54°C for 30 s, and 72°C for 30–60 s to amplify the target gene fragments, and then incubation at 72°C for 7 min for extension. The 16S rRNA gene was used as the control (El Aila et al., 2010). For each of the virulence genes in the S. pneumoniae isolates, three independent PCR repeats were performed for detection.
Statistical Analysis
The obtained data were statistically analyzed using the SPSS 20.0 software. Chi-square test and Fisher’s exact probability examination were used to determine significant differences. The statistical significance was defined as p < 0.05.
Results
Predominant Diseases in S. pneumoniae-Infected Children and Adults
Of the samples collected from 4,665 patients suffering from bacterial URTI, pneumonia, tympanitis, bacteremia, or meningitis according to clinical signs and symptoms and laboratory examinations, a total of 309 cases were isolated for S. pneumoniae. Of the 309 patients infected with S. pneumoniae, 91.3% (282/309) were children (≤12 years old), while 8.7% (27/309) were adults (>49 years old) (Table 1). The children aged 1–5 years had the highest incidence of an S. pneumoniae infection compared with the younger and older children (≤1 and 6–12 years old) (p < 0.05), while the younger children displayed a higher incidence of infection than the older children (p < 0.05). Pneumonia was the most common disease in the children (78.7%, 222/282), while meningitis (37.0%, 10/27) was the most common disease in the adults (p < 0.05). These data suggest that children are the most susceptible population to a pneumococcal infection, while pneumonia and meningitis are the predominant types of pneumococcal diseases in children and adults, respectively.
Predominant Serotypes in the S. pneumoniae Isolates
All the 309 S. pneumoniae isolates were positive for the S. pneumoniae-specific 16S rRNA gene, and in 99.4% (307/309) of the isolates, the cpsA gene was detected. Of the 309 isolates, 90.3% (279/309) could be classified by PCR into fifteen serotypes (Table 2 and Supplementary Figure 1), while for 9.7% (30/309) of the isolates, a serotype could not be determined [non-typeable (NT)]. Among the fifteen identified serotypes, serotypes 19F (27.2%, 84/309) and 6A/B (19.1%, 59/309) were the most predominant (p < 0.05). Moreover, 23F, 14, 15A, and 4 were also as the common pneumococcal serotypes with a >5% incidence. More importantly, only 78.3% (242/309) of the 309 S. pneumoniae isolates belonged to thirteen serotypes covered by the 13-valent pneumococcal capsule conjugate vaccine (PCV13) mainly used in China for vaccination, while serotypes 15A (7.4%, 23/309) and 15B/C (2.9%, 9/309) were not included in this vaccine (Figure 1). These data show that 19F and 6A/B are the predominant pneumococcal serotypes prevailing in East China, while serotypes 15A and 15B/C should be added into the pneumococcal vaccine used in China.
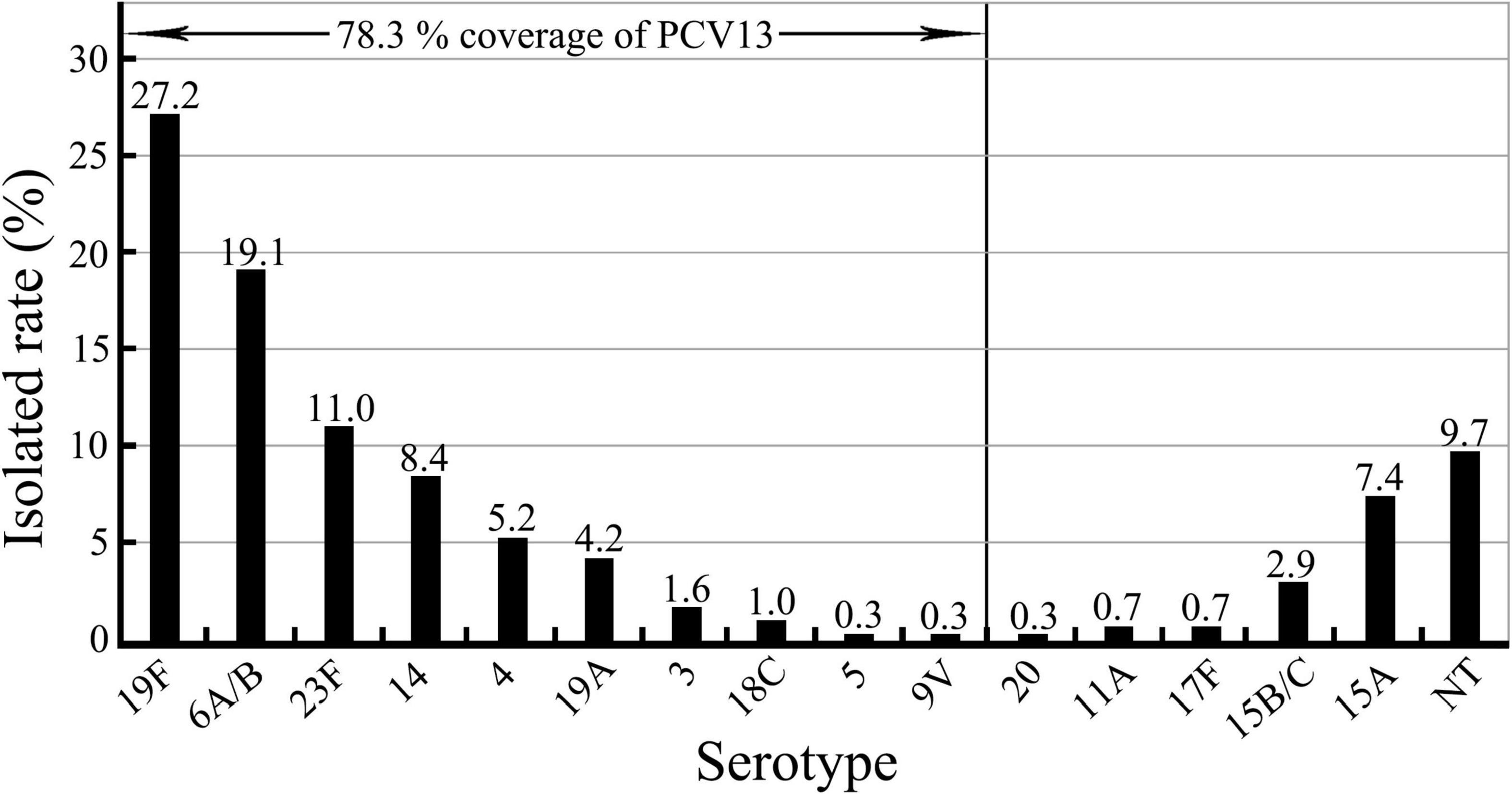
Figure 1. Serotypes in the 309 S. pneumoniae isolates and serotype coverage of pneumococcal vaccine PCV13.
Correlation Between the S. pneumoniae Serotypes and Patient Ages and Diseases
The chi-square test and Fisher’s exact probability examination showed that serotypes 19F, 6A/B, 23F, 14, 15A, and 4, the predominant or common identified S. pneumoniae serotypes, caused a higher total infection rate in the children (≤1–12 years old) than in the adults (49–>65 years old) (p < 0.05) (Table 3). Furthermore, the children aged 1–5 years old had significantly higher positive rates of each of the predominant/common pneumococcal serotypes than the younger and older children (≤1 and 6–12 years old) (Table 3). On the contrary, these predominant/common pneumococcal serotypes displayed the highest incidence of pneumonia compared with the other four pneumococcal diseases (p < 0.05) (Table 4). These data suggest that children aged between 1 and 5 years are the most susceptible population to an infection by S. pneumoniae, and pneumonia is the most common pneumococcal disease.
Drug Resistance of the S. pneumoniae Isolates
The β-lactamase phenotype confirmatory test confirmed that all the 309 S. pneumoniae isolates did not produce β-lactamases. Drug resistance of the isolates against seven antibiotics is shown in Figure 2. The isolates displayed higher incidences of susceptibility to IPM, CTX, LEV, and MXF (81.6–99.7%, 252–308/309) but a low incidence of susceptibility to PNC (18.4%, 57/309). However, 81.6% (252/309) of the isolates were non-susceptible to PNC, including 63.2% (195/309) intermediate and 18.4% (57/309) resistant. To our surprise, the incidence of resistance to ETM (95.8%) and AZM (96.1%) was high. Furthermore, the analysis of the antibiotic resistance patterns among the S. pneumoniae isolates showed that 96.1% (297/309) of the isolates were multiple resistant with resistance against ETM+AZM (239/300, 79.7%) and PNC+ETM+AZM (47/300, 15.7%) being the most common multidrug resistance patterns (Table 5). In particular, the chi-square test showed that serogroups 19F, 6A/B, and 23F serotypes were closely correlated with PNC resistance (p < 0.05) (Table 6). These data suggest that the S. pneumoniae isolates prevailing in East China are commonly resistant to ETM and AZM, with ETM+AZM being the most dominant multidrug resistance pattern, and the predominant pneumococcal serotypes (19F, 6A/B, and 23F) are significantly correlated with PNC resistance.
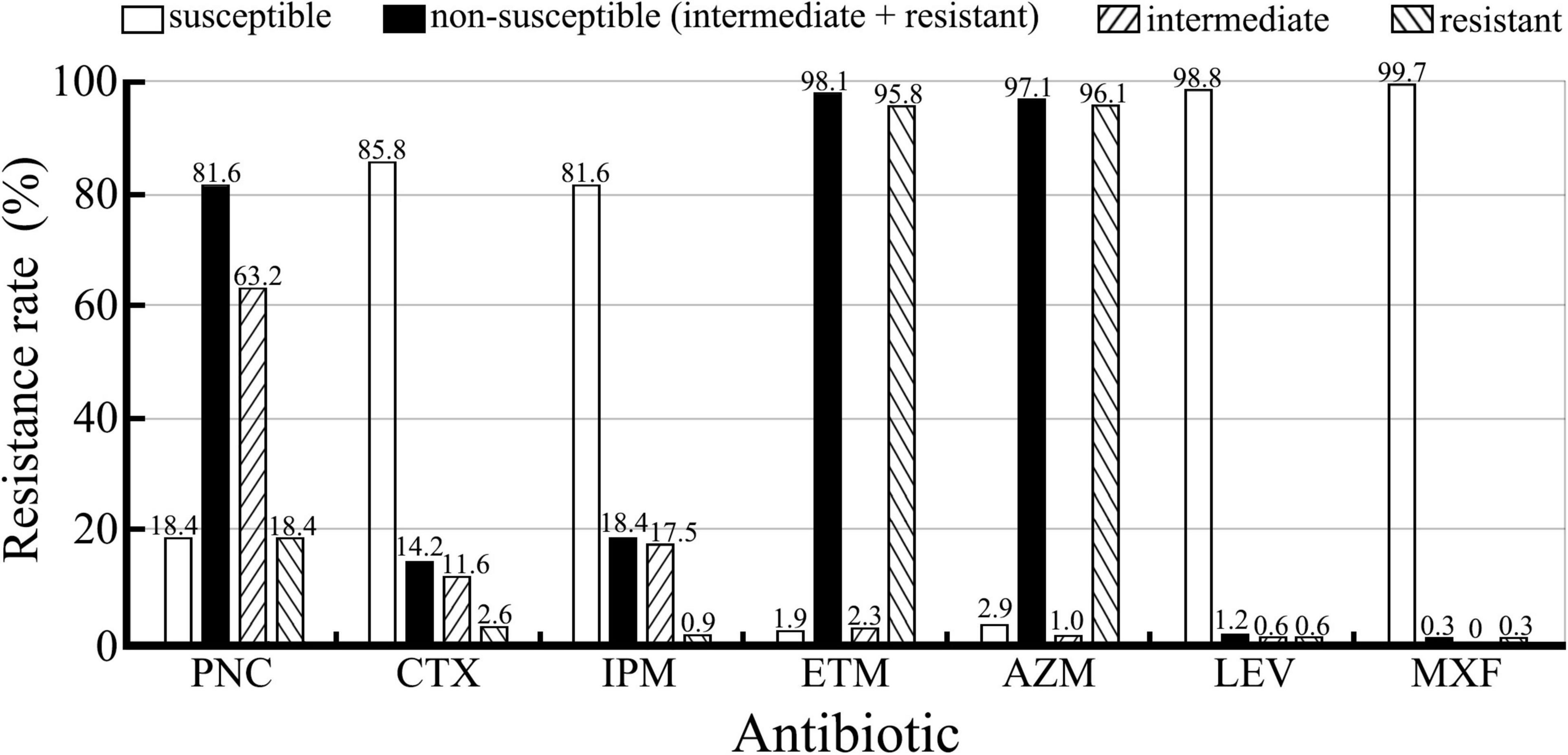
Figure 2. Resistance of the 309 S. pneumoniae isolates against seven antibiotics detected using the E-test. Non-susceptible are from the combination of intermediate and resistant.
Virulence Gene Distribution in the S. pneumoniae Isolates
Although all the nine virulence genes, namely, cbpA, cps2A, hysA, lytA, nanA, pavA, ply, psaA, and pspA, were detectable in this study (Supplementary Figure 2), there were large diversities in the positive rates for different virulence genes among the 309 S. pneumoniae isolates (Figure 3 and Tables 7, 8). All the isolates (309/309) were positive for the lytA gene, while the nanA, hysA, and ply genes were detected in 95.1% (294/309), 97.4% (301/309), and 99.7% (308/309) of the isolates, respectively. In contrast, the positive rates for the pavA and cps2A genes in the isolates were 84.1% (260/309) and 67.3% (208/309), respectively, while only 23.0% (71/309), 14.2% (44/309), and 4.2 (13/309) of the isolates contained the psaA, cbpA, and pspA genes, respectively. In particular, the chi-square test showed that the pavA and psaA genes were significantly correlated with pneumococcal bacteremia and meningitis, and serotype 23F displayed the highest positive rates of the cps2A, psaA, and cbpA genes (Tables 7, 8).
Discussion
Previous epidemiological investigation data consistently revealed that children are much more susceptible to S. pneumoniae than adults, and pneumonia is the most common pneumococcal disease in children (Erlichman et al., 2017; GBD 2016 Lower Respiratory Infections Collaborators, 2018; Wang et al., 2020). Our study also showed that 91.3% (282/309) of the 309 S. pneumoniae-infected patients were children, and 78.7% (222/282) of them suffered from pneumonia. Although our study contained much fewer S. pneumoniae-infected adult patients (8.7%, 27/309), with 37.0% (10/27), the incidence of meningitis in the adult patients was strikingly high. Since meningitis is usually considered as a highly lethal disease, doctors must pay more attention to the adult patients infected with S. pneumoniae.
Streptococcus pneumoniae strains can be classified into many different serotypes according to their antigenic diversity of their capsular polysaccharides (Jauneikaite et al., 2015; Zafar et al., 2017; Ganaie et al., 2020; Joshi et al., 2020). However, there are differences in the predominant and common serotypes of S. pneumoniae in different areas and counties, probably due to different vaccine types and vaccination rates (Klugman and Rodgers, 2021). Recent epidemiological studies reported the predominant or common serotypes of S. pneumoniae isolates prevailing in the different areas and adjacent countries of China. 19F, 6B, 23F, and 19A were the common serotypes observed among the S. pneumoniae isolates in Western China, while 19F, 19A, and 23F were the predominant serotypes among the S. pneumoniae isolates in North China (Zhao et al., 2020; Liang et al., 2021; Zhou et al., 2022). In the different areas of Southwest China, 19F and 19A were the predominant pneumococcal serotypes (Shen et al., 2017; Ma et al., 2021; Yan et al., 2021). In addition, serotypes 12F, 3, 23A, 19A, 10A, 6C, and 22F and serotypes 3, 19A, and 11A were the commonly found among the S. pneumoniae isolates from Japan and South Korea, respectively (Park et al., 2019; Heo et al., 2020; Yanagihara et al., 2021). Furthermore, 23F, 19F, 19A, and 14 were the predominant pneumococcal serotypes found in Thailand and Malaysia (Wangirapan et al., 2020; Dzaraly et al., 2021). In India, 14, 1, and 19F acted as the predominant serotypes of S. pneumoniae (Singh et al., 2017). However, in our study covering East China, 19F and 6A/B were the predominant serotypes, while 23F, 14, 15A, and 4 were the other common serotypes observed, which are distinct from the predominant and common pneumococcal serotypes found in the other areas of China and in the other counties in Asia. More importantly, PCV13, a 13-valent pneumococcal capsule conjugate vaccine mainly used in China (Wang et al., 2021), appears to only provide a 78.3% coverage of the S. pneumoniae isolates characterized in this study, while the commonly found serotypes 15A (7.4%, 23/309) and 15B/C (2.9%, 9/309) are not included in PCV13. These data suggest that the predominant and common S. pneumoniae serotypes from East China display a regional specificity, and that capsular polysaccharide antigens from pneumococcal 15A and 15B/C serotypes with a total positive rate of 10.4% should be included in the multivalent capsule conjugate vaccine.
β-Lactam antibiotics serve as the first-line therapy choice for the treatment of pneumococcal diseases. However, β-lactam antibiotic resistance rates of S. pneumoniae isolates from different areas and countries have a large diversity. For example, PNC and CTX resistance rates among the S. pneumoniae isolates from Western China, North China, and Southwest China were 1.2–79.9% and 0.5–28.6% (Shen et al., 2017; Zhao et al., 2020; Liang et al., 2021; Yan et al., 2021; Zhou et al., 2022), while the PNC resistance rates among the S. pneumoniae isolates from East Asia (Japan and South Korea), Southeast Asia (Thailand and Malaysia), India, United States, Europe, and Latin America were 1.7–22.8%, 4–83.1%, 10%, 10.8%, 19.4%, and 68.6%, respectively (Mendes et al., 2016; Yahiaoui et al., 2016; Singh et al., 2017; Park et al., 2019; Moreno et al., 2020; Wangirapan et al., 2020; Dzaraly et al., 2021; Yanagihara et al., 2021). In this study, the PNC non-susceptible rate among the 309 S. pneumoniae isolates was as high as 81.6% (63.2% intermediate and 18.4% resistant), but the CTX non-susceptible rate was only 14.2% (11.6% intermediate and 2.6% resistant). The ETM and AZM resistance rates among the 309 S. pneumoniae isolates were 95.8 and 96.1%, which are similar to those from Western China (94.3%), North China (95–98.2%), and Southwest China (99.1%) (Zhao et al., 2020; Liang et al., 2021; Yan et al., 2021; Zhou et al., 2022) but significantly higher than those from Southeast Asia (38.5–42.0%), India (37.0%), United States (37.1%), and Latin America (43.2%) (Mendes et al., 2016; Singh et al., 2017; Moreno et al., 2020; Wangirapan et al., 2020; Dzaraly et al., 2021). In addition, 96.1% of the 309 S. pneumoniae isolates in this study showed different multidrug resistance patterns, which is also similar to those from Western China (93.8%) (Liang et al., 2021), but significantly higher than those from South Korea (46.2%), Malaysia (18.0%), and Latin America (44.3%) (Park et al., 2019; Moreno et al., 2020; Dzaraly et al., 2021). More importantly, S. pneumoniae serotypes 19F, 6A/B, and 23F displayed a significant correlation with PNC resistance. These data suggest that the S. pneumoniae isolates from East China are commonly resistant to PNC, ETM, and AZM, and that the PNC resistance may contribute to the prevalence of pneumococcal serotypes 19F, 6A/B, and 23F.
Streptococcus pneumoniae has no endotoxin (i.e., LPS) and typical exotoxins, but its capsule plays a crucial role in pathogenesis through antiphagocytosis activity and benefiting colonization (Nelson et al., 2007; van der Poll and Opal, 2009; Brissac et al., 2021). In this study, only two of the 309 S. pneumoniae isolates did not contain the cpsA gene, a capsule formation-determining factor (Dias et al., 2007; Coskun-Ari et al., 2012), highlighting the importance of the capsule in pneumococcal pathogenesis. Of the nine pneumococcal virulence genes analyzed in this study, all the S. pneumoniae isolates were positive for the lytA gene, while the nanA, hysA, and ply genes were detectable in 95.1–99.7% of the isolates, which is similar to the positive detection rates (91.2 and 96.2%) of the ply and lytA genes in the 419 S. pneumoniae isolates from Western China and the positive rates (93.1, 97.1, and 100%) of the nanA, cbpA, and ply genes in the 102 S. pneumoniae isolates from Southwest China (Yan et al., 2019; Liang et al., 2021). Previous studies also confirmed that the lytA gene, encoding the S. pneumoniae autolysin, had high specificity and sensitivity for pneumococcal detection (Ramos-Sevillano et al., 2015; Afshar et al., 2020). Therefore, the product of the lytA gene can be used to identify S. pneumoniae and act as a candidate antigen of pneumococcal vaccines. Although the hysA and ply genes showed the 97.4 and 99.7% positive rates among the S. pneumoniae isolates, respectively, their products, a secretory hyaluronidase and an intracytoplasmic pneumolysin (Marion et al., 2012; Greene et al., 2015; Rai et al., 2016), are unsuitable for vaccine development. The product of the nanA gene (95.1% positive rates) is a surface neuraminidase of S. pneumoniae and can therefore also be used as a potential antigen for development of novel pneumococcal vaccines.
Data Availability Statement
The original contributions presented in the study are included in the article/Supplementary Material, further inquiries can be directed to the corresponding author.
Ethics Statement
Written informed consent was obtained from the individual(s), and minor(s)’ legal guardian/next of kin, for the publication of any potentially identifiable images or data included in this article.
Author Contributions
A-HS and Y-YH obtained the funds. A-HS and JY conceived and designed the experiments. L-DH and M-JY performed the experiments and analyzed the data. K-YJ performed the passage of S. pneumoniae isolates. L-DH, M-JY, JY, and A-HS wrote the manuscript. All authors reviewed the manuscript.
Funding
This study was supported by the National Natural Science Foundation of China (Grant No. 81772232) and Medical and Health Science and Technology Project of Zhejiang Province, China (Grant No. 2020KY737).
Conflict of Interest
The authors declare that the research was conducted in the absence of any commercial or financial relationships that could be construed as a potential conflict of interest.
Publisher’s Note
All claims expressed in this article are solely those of the authors and do not necessarily represent those of their affiliated organizations, or those of the publisher, the editors and the reviewers. Any product that may be evaluated in this article, or claim that may be made by its manufacturer, is not guaranteed or endorsed by the publisher.
Acknowledgments
We thank Du Peng for providing convenience and help in our experiments. We are grateful to Stijn van der Veen, a native English speaker working at Zhejiang University School of Medicine, for modification of English grammar of our manuscript.
Supplementary Material
The Supplementary Material for this article can be found online at: https://www.frontiersin.org/articles/10.3389/fmicb.2022.892364/full#supplementary-material
References
Afshar, D., Rafiee, F., Kheirandish, M., Ohadian-Moghadam, S., and Azarsa, M. (2020). Autolysin (lytA) recombinant protein: a potential target for developing vaccines against pneumococcal infections. Clin. Exp. Vaccine Res. 9, 76–80. doi: 10.7774/cevr.2020.9.2.76
Aliberti, S., Cook, G. S., Babu, B. L., Reyes, L. F., Rodriguez, A., Sanz, F., et al. (2019). International prevalence and risk factors evaluation for drug-resistant Streptococcus pneumoniae pneumonia. J. Infect. 79, 300–311. doi: 10.1016/j.jinf.2019.07.004
Amin, A. N., Cerceo, E. A., Deitelzweig, S. B., Pile, J. C., Rosenberg, D. J., and Sherman, B. M. (2014). The hospitalist perspective on treatment of community-acquired bacterial pneumonia. Postgrad. Med. 126, 18–29. doi: 10.3810/pgm.2014.03.2737
Argemi, X., Lefebvre, N., Baldeyrou, M., Douiri, N., and Hansmann, Y. (2016). Streptococcus pneumoniae appendicitis and bacteremia in an immunocompetent adult. Med. Mal. Infect. 46, 106–107. doi: 10.1016/j.medmal.2015.12.001
Brissac, T., Martínez, E., Kruckow, K. L., Riegler, A. N., Ganaie, F., Im, H., et al. (2021). Capsule promotes intracellular survival and vascular endothelial cell translocation during invasive pneumococcal disease. mBio 12:e02516-21. doi: 10.1128/mBio.02516-21
Coskun-Ari, F. F., Guldemir, D., and Durmaz, R. (2012). One-step multiplex PCR assay for detecting Streptococcus pneumoniae serogroups/types covered by 13-valent pneumococcal conjugate vaccine (PCV13). PLoS One 7:e50406. doi: 10.1371/journal.pone.0050406
Croney, C. M., Nahm, M. H., Juhn, S. K., Briles, D. E., and Crain, M. J. (2013). Invasive and noninvasive Streptococcus pneumoniae capsule and surface protein diversity following the use of a conjugate vaccine. Clin. Vaccine Immunol. 20, 1711–1718. doi: 10.1128/CVI.00381-13
Da Gloria-Carvalho, M., Pimenta, F. C., Jackson, D., Roundtree, A., Ahmad, Y., Millar, E. V., et al. (2010). Revisiting pneumococcal carriage by use of broth enrichment and PCR techniques for enhanced detection of carriage and serotypes. J. Clin. Microbiol. 48, 1611–1618. doi: 10.1128/JCM.02243-09
Dias, C. A., Teixeira, L. M., Carvalho, M. D. G., and Beall, B. (2007). Sequential multiplex PCR for determining capsular serotypes of pneumococci recovered from Brazilian children. J. Med. Microbiol. 56, 1185–1188. doi: 10.1099/jmm.0.47347-0
Dockrell, D. H., Whyte, M. K., and Mitchell, T. J. (2012). Pneumococcal pneumonia: mechanisms of infection and resolution. Chest 142, 482–491. doi: 10.1378/chest.12-0210
Droz, N., Hsia, Y., Ellis, S., Dramowski, A., Sharland, M., and Basmaci, R. (2019). Bacterial pathogens and resistance causing community acquired paediatric blood stream infections in low- and middle-income countries: a systematic review and meta-analysis. Antimicrob. Resist. Infect. Control 8, 207–218. doi: 10.1186/s13756-019-0673-5
Dzaraly, N. D., Mohd-Desa, M. N., Muthanna, A., Masri, S. N., Taib, N. M., Suhaili, Z., et al. (2021). Antimicrobial susceptibility, serotype distribution, virulence profile and molecular typing of piliated clinical isolates of pneumococci from east coast, Peninsular Malaysia. Sci. Rep. 11:8220. doi: 10.1038/s41598-021-87428-z
El Aila, N. A., Emler, S., Kaijalainen, T., De Baere, T., Saerens, B., Alkan, E., et al. (2010). The development of a 16S rRNA gene based PCR for the identification of Streptococcus pneumoniae and comparison with four other species specific PCR assays. BMC Infect. Dis. 10:104. doi: 10.1186/1471-2334-10-104
Erlichman, I., Breuer, O., Shoseyov, D., Cohen-Cymberknoh, M., Koplewitz, B., Averbuch, D., et al. (2017). Complicated community acquired pneumonia in childhood: different types, clinical course, and outcome. Pediatr. Pulmonol. 52, 247–254. doi: 10.1002/ppul.23523
Ganaie, F., Saad, J. S., McGee, L., van Tonder, A. J., Bentley, S. D., Lo, S. W., et al. (2020). A new pneumococcal capsule type, 10D, is the 100th serotype and has a large cps fragment from an oral Streptococcus. mBio 11:e00937-20. doi: 10.1128/mBio.00937-20
GBD 2016 Lower Respiratory Infections Collaborators (2018). Estimates of the global, regional, and national morbidity, mortality, and aetiologies of lower respiratory infections in 195 countries, 1990-2016: a systematic analysis for the Global Burden of Disease Study 2016. Lancet Infect. Dis. 18, 1191–1210. doi: 10.1016/S1473-3099(18)30310-4
Greene, N. G., Narciso, A. R., Filipe, S. R., and Camilli, A. (2015). Peptidoglycan branched stem peptides contribute to Streptococcus pneumoniae virulence by inhibiting pneumolysin release. PLoS Pathog. 11:e1004996. doi: 10.1371/journal.ppat.1004996
Heo, J. Y., Seo, Y. B., Jeong, H. W., Choi, M. J., Min, K. H., Choi, W. S., et al. (2020). Epidemiology of community-acquired pneumonia in the era of extended serotype-covering multivalent pneumococcal conjugate vaccines. Vaccine 38, 7747–7755. doi: 10.1016/j.vaccine.2020.10.046
Holmes, A. R., McNab, R., Millsap, K. W., Rohde, M., Hammerschmidt, S., Mawdsley, J. L., et al. (2001). The pavA gene of Streptococcus pneumoniae encodes a fibronectin-binding protein that is essential for virulence. Mol. Microbiol. 41, 1395–1408. doi: 10.1046/j.1365-2958.2001.02610.x
Jauneikaite, E., Tocheva, A. S., Jefferies, J. M., Gladstone, R. A., Faust, S. N., Christodoulides, M., et al. (2015). Current methods for capsular typing of Streptococcus pneumoniae. J. Microbiol. Methods 113, 41–49. doi: 10.1016/j.mimet.2015.03.006
Joshi, S. S., Al-Mamun, M. A., and Weinberger, D. M. (2020). Correlates of nonrandom patterns of serotype switching in pneumococcus. J. Infect. Dis. 221, 1669–1676. doi: 10.1093/infdis/jiz687
Khan, S., Sallum, U. W., Zheng, X., Nau, G. J., and Hasan, T. (2014). Rapid optical determination of β-lactamase and antibiotic activity. BMC Microbiol. 14:84. doi: 10.1186/1471-2180-14-84
Kim, S. H., Chung, D. R., Song, J. H., Baek, J. Y., Thamlikitkul, V., Wang, H., et al. (2020). Changes in serotype distribution and antimicrobial resistance of Streptococcus pneumoniae isolates from adult patients in Asia: emergence of drug-resistant non-vaccine serotypes. Vaccine 38, 6065–6073. doi: 10.1016/j.vaccine.2019.09.065
Klugman, K. P., and Rodgers, G. L. (2021). Impact of pneumococcal conjugate vaccine on vaccine serotype-specific pneumonia. Clin. Infect. Dis. 73, e1434–e1435. doi: 10.1093/cid/ciaa1867
Korona-Glowniak, I., Zychowski, P., Siwiec, R., Mazur, E., Niedzielska, G., and Malm, A. (2018). Resistant Streptococcus pneumoniae strains in children with acute otitis media- high risk of persistent colonization after treatment. BMC Infect. Dis. 18:478. doi: 10.1186/s12879-018-3398-9
Liang, Z., Fu, J., Li, L., Yi, R., Xu, S., Chen, J., et al. (2021). Molecular epidemiology of Streptococcus pneumoniae isolated from pediatric community-acquired pneumonia in pre-conjugate vaccine era in Western China. Ann. Clin. Microbiol. Antimicrob. 20, 4–12. doi: 10.1186/s12941-020-00410-x
Ma, M., Yuan, M., Li, M., Li, X., Huang, H., Wang, H., et al. (2021). Serotype distribution and characteristics of the minimum inhibitory concentrations of Streptococcus pneumoniae isolated from pediatric patients in Kunming, China. Curr. Microbiol. 78, 954–960. doi: 10.1007/s00284-021-02365-4
Marion, C., Stewart, J. M., Tazi, M. F., Burnaugh, A. M., Linke, C. M., Woodiga, S. A., et al. (2012). Streptococcus pneumoniae can utilize multiple sources of hyaluronic acid for growth. Infect. Immun. 80, 1390–1398. doi: 10.1128/IAI.05756-11
Mellro, P., Sandalova, T., Kikhney, A., Vilaplana, F., Hesek, D., Lee, M., et al. (2014). Structural and functional insights into peptidoglycan access for the lytic amidase LytA of Streptococcus pneumoniae. mBio 5:e01120-13. doi: 10.1128/mBio.01120-13
Mendes, R. E., Farrell, D. J., Flamm, R. K., Talbot, G. H., Ivezic-Schoenfeld, Z., Paukner, S., et al. (2016). In vitro activity of lefamulin tested against Streptococcus pneumoniae with defined serotypes, including multidrug-resistant isolates causing lower respiratory tract infections in the United States. Antimicrob. Agents Chemother. 60, 4407–4411. doi: 10.1128/AAC.00627-16
Moreno, J., Duarte, C., Cassiolato, A. P., Chacón, G. C., Alarcon, P., Sánchez, J., et al. (2020). Molecular characterization of Latin American invasive Streptococcus pneumoniae serotype 19A isolates. Vaccine 38, 3524–3530. doi: 10.1016/j.vaccine.2020.03.030
Nakano, S., Fujisawa, T., Ito, Y., Chang, B., Suga, S., Noguchi, T., et al. (2016). Serotypes, antimicrobial susceptibility, and molecular epidemiology of invasive and non-invasive Streptococcus pneumoniae isolates in paediatric patients after the introduction of 13-valent conjugate vaccine in a nationwide surveillance study conducted in Japan in 2012-2014. Vaccine 34, 67–76. doi: 10.1016/j.vaccine.2015.11.015
Nelson, A. L., Roche, A. M., Gould, J. M., Chim, K., Ratner, A. J., and Weiser, J. N. (2007). Capsule enhances pneumococcal colonization by limiting mucus mediated clearance. Infect. Immun. 75, 8–90. doi: 10.1128/IAI.01475-06
Oligbu, G., Fry, N. K., and Ladhani, S. N. (2019). The pneumococcus and its critical role in public health. Methods Mol. Biol. 1968, 205–213. doi: 10.1007/978-1-4939-9199-0_17
Oordt-Speets, A. M., Bolijn, R., van Hoorn, R. C., Bhavsar, A., and Kyaw, M. H. (2018). Global etiology of bacterial meningitis: a systematic review and meta-analysis. PLoS One 13:e0198772. doi: 10.1371/journal.pone.0198772
Pai, R., Gertz, R. E., and Beall, B. (2006). Sequential multiplex PCR approach for determining capsular serotypes of Streptococcus pneumoniae isolates. J. Clin. Microbiol. 44, 124–131. doi: 10.1128/JCM.44.1.124-131.2006
Park, D. C., Kim, S. H., Yong, D., Suh, I. B., Kim, Y. R., Yi, J., et al. (2019). Serotype distribution and antimicrobial resistance of invasive and noninvasive Streptococcus pneumoniae isolates in Korea between 2014 and 2016. Ann. Lab. Med. 39, 537–544. doi: 10.3343/alm.2019.39.6.537
Rai, P., He, F., Kwang, J., Engelward, B. P., and Chow, V. T. (2016). Pneumococcal pneumolysin induces DNA damage and cell cycle arrest. Sci. Rep. 6:22972. doi: 10.1038/srep22972
Ramos-Sevillano, E., Urzainqui, A., Campuzano, S., Moscoso, M., González-Camacho, F., Domenech, M., et al. (2015). Pleiotropic effects of cell wall amidase LytA on Streptococcus pneumoniae sensitivity to the host immune response. Infect. Immun. 83, 591–603. doi: 10.1128/IAI.02811-14
Romero-Steiner, S., Pilishvili, T., Sampson, J. S., Johnson, S. E., Stinson, A., Carlone, G. M., et al. (2003). Inhibition of pneumococcal adherence to human nasopharyngeal epithelial cells by anti-PsaA antibodies. Clin. Diagn. Lab. Immunol. 10, 246–251. doi: 10.1128/cdli.10.2.246-251.2003
Rozenbaum, M. H., Pechlivanoglou, P., van der Werf, T. S., Lo-Ten-Foe, J. R., Postma, M. J., and Hak, E. (2013). The role of Streptococcus pneumoniae in community-acquired pneumonia among adults in Europe: a meta-analysis. Eur. J. Clin. Microbiol. Infect. Dis. 32, 305–316. doi: 10.1007/s10096-012-1778-4
Shen, M., Yao, R., Yue, H., Zhang, J., Chen, M., Zhang, W., et al. (2017). Serotype prevalence and antibiotic susceptibility patterns of pneumococcal isolates in Zunyi city, China. Saudi Med. J. 38, 1243–1249. doi: 10.15537/smj.2017.12.21090
Singh, J., Sundaresan, S., Manoharan, A., and Shet, A. (2017). Serotype distribution and antimicrobial susceptibility pattern in children ≤5years with invasive pneumococcal disease in India – a systematic review. Vaccine 35, 4501–4509. doi: 10.1016/j.vaccine.2017.06.079
Suaya, J. A., Mendes, R. E., Sings, H. L., Arguedas, A., Reinert, R. R., Jodar, L., et al. (2020). Streptococcus pneumoniae serotype distribution and antimicrobial nonsusceptibility trends among adults with pneumonia in the United States, 2009-2017. J. Infect. 81, 557–566. doi: 10.1016/j.jinf.2020.07.035
Subramanian, K., Henriques-Normark, B., and Normark, S. (2019). Emerging concepts in the pathogenesis of the Streptococcus pneumoniae: from nasopharyngeal colonizer to intracellular pathogen. Cell. Microbiol. 21:e13077. doi: 10.1111/cmi.13077
Sun, A. H., Fan, X. L., Gu, Y., Du, P., Tang, R. X., Mao, Y. F., et al. (2010). Predominant porB1A and porB1B genotypes and correlation of gene mutations with drug resistance in Neisseria gonorrhoeae isolates in Eastern China. BMC Infect. Dis. 10:323. doi: 10.1186/1471-2334-10-323
Thummeepak, R., Leerach, N., Kunthalert, D., Tangchaisuriya, U., Thanwisai, A., and Sitthisak, S. (2015). High prevalence of multi-drug resistant Streptococcus pneumoniae among healthy children in Thailand. J. Infect. Public Health 8, 274–281. doi: 10.1016/j.jiph.2014.11.002
van der Poll, T., and Opal, S. M. (2009). Pathogenesis, treatment, and prevention of pneumococcal pneumonia. Lancet 374, 1543–1556. doi: 10.1016/S0140-6736(09)61114-4
Wang, C. X., Li, S., Mu, Q. L., Gu, X. Y., Guo, X. J., and Wang, X. X. (2021). Cost-effectiveness analysis of domestic 13-valent pneumococcal conjugate vaccine for children under 5 years of age in mainland China. Hum. Vaccin. Immunother. 17, 2241–2248. doi: 10.1080/21645515.2020.1870396
Wang, Y. X., Ma, L. J., Li, Y., Li, Y. Y., Zheng, Y. F., and Zhang, X. Y. (2020). Epidemiology and clinical characteristics of pathogens positive in hospitalized children with segmental/lobar pattern pneumonia. BMC Infect. Dis. 20:205. doi: 10.1186/s12879-020-4938-7
Wangirapan, A., Ayuthaya, S. I. N., Katip, W., Kasatpibal, N., Mektrirat, R., Anukool, U., et al. (2020). Serotypes and vaccine coverage of Streptococcus pneumoniae colonization in the nasopharynx of Thai children in congested areas in Chiang Mai. Pathogens 2020:988. doi: 10.3390/pathogens9120988
Weinberger, D. M., Trzciński, K., Lu, Y. J., Bogaert, D., Brandes, A., Galagan, J., et al. (2009). Pneumococcal capsular polysaccharide structure predicts serotype prevalence. PLoS Pathog. 5:e1000476. doi: 10.1371/journal.ppat.1000476
Wren, J. T., Blevins, L. K., Pang, B., Basu-Roy, A., Oliver, M. B., Reimche, J. L., et al. (2017). Pneumococcal neuraminidase a (NanA) promotes biofilm formation and synergizes with influenza a virus in nasal colonization and middle ear infection. Infect. Immun. 85:e01044-16. doi: 10.1128/IAI.01044-16
Yahiaoui, R. Y., den Heijer, C. D. J., van Bijnen, E. M., Paget, W. J., Pringle, M., Goossens, H., et al. (2016). Prevalence and antibiotic resistance of commensal Streptococcus pneumoniae in nine European countries. Future Microbiol. 11, 737–744. doi: 10.2217/fmb-2015-0011
Yan, Z., Cui, Y., Huang, X., Lei, S., Zhou, W., Tong, W., et al. (2021). Molecular characterization based on whole-genome sequencing of Streptococcus pneumoniae in children living in Southwest China during 2017-2019. Front. Cell. Infect. Microbiol. 11:726740. doi: 10.3389/fcimb.2021.726740
Yan, Z., Cui, Y., Zhou, W., Li, W., Tan, X., Chen, W., et al. (2019). Molecular characterization of Streptococcus pneumoniae in children living in southwest China and assessment of a potential protein vaccine, rPfbA. Vaccine 37, 721–731. doi: 10.1016/j.vaccine.2018.12.021
Yanagihara, K., Kosai, K., Mikamo, H., Mukae, H., Takesue, Y., Abe, M., et al. (2021). Serotype distribution and antimicrobial susceptibility of Streptococcus pneumoniae associated with invasive pneumococcal disease among adults in Japan. Int. J. Infect. Dis. 102, 260–268. doi: 10.1016/j.ijid.2020.10.017
Zafar, M. A., Hamaguchi, S., Zangari, T., Cammer, M., and Weiser, J. N. (2017). Capsule type and amount affect shedding and transmission of Streptococcus pneumoniae. mBio 8:e00989-17. doi: 10.1128/mBio.00989-17
Zhao, C., Xie, Y., Zhang, F., Wang, Z., Yang, S., Wang, Q., et al. (2020). Investigation of antibiotic resistance, serotype distribution, and genetic characteristics of 164 invasive Streptococcus pneumoniae from North China between April 2016 and October 2017. Infect. Drug Resist. 13, 2117–2128. doi: 10.2147/IDR.S256663
Zhao, W. T., Pan, F., Wang, B. J., Sun, Y., Zhang, T. D., Shi, Y. Y., et al. (2019). Epidemiology characteristics of Streptococcus pneumoniae from children with pneumonia in Shanghai: a retrospective study. Front. Cell. Infect. Microbiol. 9:258. doi: 10.3389/fcimb.2019.00258
Zhou, M., Wang, Z., Zhang, L., Kudinha, T., An, H., Qian, C., et al. (2022). Serotype distribution, antimicrobial susceptibility, multilocus sequencing type and virulence of invasive Streptococcus pneumoniae in China: a six-year multicenter study. Front. Microbiol. 12:798750. doi: 10.3389/fmicb.2021.798750
Keywords: East China, Streptococcus pneumoniae, predominant serotypes, drug resistance, virulence genes
Citation: Huang L-D, Yang M-J, Huang Y-Y, Jiang K-Y, Yan J and Sun A-H (2022) Molecular Characterization of Predominant Serotypes, Drug Resistance, and Virulence Genes of Streptococcus pneumoniae Isolates From East China. Front. Microbiol. 13:892364. doi: 10.3389/fmicb.2022.892364
Received: 09 March 2022; Accepted: 25 April 2022;
Published: 01 June 2022.
Edited by:
Longzhu Cui, Jichi Medical University, JapanReviewed by:
Haijian Zhou, National Institute for Communicable Disease Control and Prevention, China CDC, ChinaDalia Denapaite, University of Trento, Italy
Copyright © 2022 Huang, Yang, Huang, Jiang, Yan and Sun. This is an open-access article distributed under the terms of the Creative Commons Attribution License (CC BY). The use, distribution or reproduction in other forums is permitted, provided the original author(s) and the copyright owner(s) are credited and that the original publication in this journal is cited, in accordance with accepted academic practice. No use, distribution or reproduction is permitted which does not comply with these terms.
*Correspondence: Ai-Hua Sun, sunah123@126.com
†These authors have contributed equally to this work