- Department of Pharmaceutical and Health Sciences, School of Pharmacy, CEU Universities, Universidad CEU San Pablo, Madrid, Spain
Heavy metal contamination of soils is a large-scale environmental problem. It leads to significant disqualification of the territory, in addition to being a source of the potential risk to human health. The exposure of plants to mercury (Hg) generates responses in its growth and their oxidative metabolism. The impact of increasing concentrations of Hg on the development of Lupinus albus var. Orden Dorado seedlings has been studied, as well as the plant’s response to the maximum concentration of Hg that allows its development (16 μg ml–1). The result shows that only the inoculum with plant growth promoting bacteria (PGPB) allows the biometric development of the seedling (root length, weight, and number of secondary roots) and prevents the toxic effects of the heavy metal from aborting the seedlings. Specifically, treatments with strains 11, 20 (Bacillus toyonensis), 48 (not determined), and 76 (Pseudomonas syringae) are interesting candidates for further PGPB-assisted phytoremediation trials as they promote root biomass development, through their PGPB activities. The plant antioxidant response has been analyzed by quantifying the catalase (CAT), superoxide dismutase (SOD), ascorbate peroxidase (APX), and glutathione reductase (GR) enzyme activity in the root, under 16 μg ml–1 of HgCl2 and different PGPB treatments. Results show that, although Hg stress generally induces enzyme activity, strains 31 and 69I (Pseudomonas corrugata) and 18 and 43 (Bacillus toyonensis) can keep SOD and APX levels close to those found in control without Hg (p < 0.01). Strain 18 also shows a significant reduction of GR to control levels without Hg. The present work demonstrates the benefit of PGPB treatments in situations of high Hg stress. These findings may be a good starting point to justify the role of PGPB naturally isolated from bulk soil and the rhizosphere of plants subjected to high Hg pressure in plant tolerance to such abiotic stress conditions. More studies will be needed to discover the molecular mechanisms behind the phytoprotective role of the strains with the best results, to understand the complex plant-microorganism relationships and to find effective and lasting symbioses useful in bioremediation processes.
Introduction
The World Report on Soil Resources (FAO, 2015) identified soil contamination as one of the main threats worldwide. In this same sense, the United Nations Environmental Assembly (UNEA, 2017) adopted a resolution calling for urgent decisions to address and mitigate soil pollution. According to the World Health Organization (World Health Organization [WHO], 2007), Hg is one of the ten chemicals that pose special problems for public health. Poisoning by this heavy metal can cause death (European Commission, 2011). The organic and more labile forms of Hg, mainly methylmercury, are the most toxic for human, animal, and plant health (Peralta-Videa et al., 2009). It accumulates in biological membranes due to its high lipid solubility, which facilitates its biomagnification in the food chain (Paisio et al., 2012). In the mining district of Almadén (Spain, Ciudad Real), mining activity has led to the mobilization of mercury (Hg) into more labile forms. This mobilization has promoted an increase in the concentration of Hg in certain locations (Díaz Puente, 2013).
For Hg to be incorporated into plants with storage capacity, it must be in its bioavailable form and close to the root. Given the similarity of the Hg (II) ion with essential molecules for plants, such as zinc (Zn), copper (Cu), or iron (Fe) (Patra and Sharma, 2000), it is incorporated through the pathways by which essential micronutrients are incorporated. Once incorporated, the Hg can be retained in the roots or translocated to the aerial part of the plant (Tiodar et al., 2021) and can be recovered through harvesting. Consequently, several visible symptoms start in the root cells (Tiwari and Lata, 2018). Of all of them, the inhibition of the longitudinal growth of the root is one of the first external symptoms of the toxic effect. Measurement of the relative rate of root elongation is often used as an early indicator to differentiate between sensitive and tolerant genotypes (Sabreen and Sugiyama, 2008).
Plants are also affected by Hg by altering antioxidant enzyme systems, hindering photosynthesis, interfering with nutrient uptake, unbalancing homeostasis in general, and slowing growth (Wang et al., 2012). Lupinus genus (lupine) is a plant with heavy metal mobilizing capacity when grown in contaminated soils (Tounsi-Hammami et al., 2020). The choice of this species is based on its ability to adapt to various ecophysiological characteristics. This adaptability allows Lupinus to develop in the conditions that occur in the mines, such as high salinity, excess nitrates, and low amounts of nutrients (González et al., 2021b). The ability to solubilize and absorb soil elements thanks to powerful root development makes it behave as a candidate plant for the plant growth promoting bacteria (PGPB)-assisted phytoremediation of these ecosystems. Plant tolerance to abiotic stress depends, to a large extent, on the bacteria present in its rhizosphere to mitigate the harmful effects of pollutants (Gontia-Mishra et al., 2016; Mariano et al., 2020; González et al., 2021a). Additionally, these bacteria have been used to improve plant resistance to different abiotic stress situations, such as salinity or desiccation (Ali et al., 2022), as well as the oxidative stress produced by Hg (Ajitha et al., 2021; Çavuşoğlu et al., 2022). However, no references have been found verifying the potential of PGPB in reversing lethality resulting from exposure to high Hg concentrations.
The objective of this work is the study of the effects of PGPB inoculants of Pseudomonas sp. and Bacillus sp. genera, isolated from the rhizosphere of plants naturally grown in chronic Hg polluted soils, on the germination and development of Lupinus albus var. Orden Dorado, under high Hg concentrations. Once the maximum concentration of Hg tolerable by the seeds in the absence of inoculum had been determined, it was sought to demonstrate whether the incorporation of PGPB reversed necrosis and cell death, allowing plant development under conditions of high Hg stress. To this end, the study of biometric parameters (weight, length of radicles, and number of secondary roots), as well as enzymes directly related to oxidative stress in plants [superoxide dismutase (SOD), catalase (CAT), ascorbate peroxidase (APX), and glutathione reductase (GR)] were quantified as measures of the plant fitness, compared with the absence of inoculum.
Materials and Methods
Tested Strains
In total, twenty-four strains from bulk soil and rhizospheres of plants naturally grown in the mining district of Almadén, Ciudad Real (Spain) were used (Table 1). The experimental plot M (38°46′24.8″N, 4°51′04.7″W), is classified as an area of high contamination by Hg with concentrations of 1,710 mg kg–1 total Hg (Millán et al., 2011), was sampled. The plants sampled for the extraction of bacteria from the rhizosphere were Rumex induratus Boiss. & Reut., Rumex bucephalophorus L., Avena sativa L., Medicago sativa L., and Vicia benghalensis L., as well as bulk soil.
The strains were selected based on their biomercury remediation suitability index (BMRSI) (Robas et al., 2021). This index is calculated from the variables: (i) tolerance to abiotic pressure by Hg, quantified from the calculation of the minimum bactericidal concentration (MBC), and (ii) its PGPB activities: auxin production [indole-3-acetic acid (IAA)], the presence of the enzyme 1-aminocyclopropane-1-carboxylate decarboxylase (ACCd), production of siderophores (SID), and the phosphate solubilization capacity. The BMRSI was calculated using the following formula, where the values 1 and 0 for ACCd and PO43– indicate presence and absence, respectively:
Candidates have a BMRSI > 5.5, a reference value established by the authors as a good indicator of the bioremedial capacity of the strains.
Seed Preparation
The seeds of L. albus var. Orden Dorado (Seed Bank of the Center for Scientific and Technological Research of Extremadura, Spain) were sterilized following the modified protocol of Abdel Latef et al. (2017). First, a 70% (v/v) ethanol bath for 30 s, followed by two consecutive washes with sterile distilled water for 1 min each. They were imbibed in sterile water and kept refrigerated (4°C) for 24 h. Pre-germination was carried out in trays with autoclaved vermiculite and irrigated with sterile water up to field capacity. The incubation of the trays was carried out in the dark, at room temperature (20 ± 2°C), and with aluminum foil wrapping the tray superficially to avoid environmental contamination and fungal growth. The pre-germinated seeds with a visible 1 ± 0.2 cm emerged radicle, were transferred in blocks of nine seeds, to Ø140 mm Petri dishes prepared with sterile vermiculite (121°C, 1 atm, and 20 min steam heat sterilization).
Determination of the Maximum Tolerance of Hg by the Seeds
Dilutions of Hg (using HgCl2) were prepared in sterile distilled water at 0.0625, 0.25, 0.5, 1.0, 2.0, 4.0, 8.0, and 16 μg.mL–1. Each plate, containing nine L. albus var. Orden Dorado seedlings, was irrigated with 50 ml of each HgCl2 dilution (experimental volume up to previously assayed water holding capacity, the WHO). The plates were incubated in darkness, humidity (daily hydration status check and maintenance at the WHO with distilled water), and room temperature (20 ± 2°C) for 120 h.
Inoculation of the Seeds With the Plant Growth Promoting Bacteria Strains and Growth Conditions
A bacterial suspension was made in a sterile 0.45% saline solution (to ensure osmolarity) with a final microbial density of 0.5 McFarland. This process was repeated for each of the 24 bacterial strains tested. For each PGPB treatment, five pre-germinated L. albus var. Order Dorado seeds were inoculated with 1 ml of the corresponding bacterial suspension. Sterile vermiculite was brought to field capacity with 16 μg ml–1 HgCl2 dilution. The Hg-free control consisted of nine pregerminated seeds of L. albus var. Golden Orden watered with 50 ml of distilled water. Control with Hg was treated with 50 ml of a 16 μg ml–1 HgCl2 dilution. Both controls were without PGPB inoculum. The plates were incubated in darkness, humidity (daily hydration status check and maintenance at field capacity with distilled water), and room temperature (20 ± 2°C) for 120 h.
Harvest
Seedlings were subjected to different PGPB treatments after 5 days of sowing (120 h), and their controls were harvested. All plants were completely removed from the substrate and washed in a 0.45% saline solution to maintain osmolarity. The root part was then excised to proceed with measurements. For each biometric and enzymatic measurement, five treatment replicates were used (n = 5).
Biometry
For each treatment and control, biometric measurements of root length (cm), root weight (g), and number of secondary roots (No) were made. For root length measurement, a caliper (precision ± 0.5 mm) was used. For root weight, a precision balance (Gram RYI, FinTech, Cardiff, United Kingdom) was used (linearity and reproducibility ± 0.0002). All samples were handled minimally and under aseptic conditions. Groups of treatments with a minimum mass of 1 g were kept at −80°C for enzymatic measurements.
Enzymatic Measurements
Catalase, SOD, APX, and GR enzyme activities were assayed.
Enzymes were extracted at 4°C from 1 g of fresh radicle sample, with a mortar and using 50 mg of polyvinylpolypyrrolidone (PVPP) and 10 ml of the following medium: 50 mM K-phosphate buffer (pH 7.8) with 0.1 mM EDTA (for SOD, CAT, and APX). The same medium, supplemented with 10 Mm of β-mercaptoethanol, was used for the GR extraction.
Superoxide Dismutase Activity
This activity was measured based on the ability of SOD to inhibit the reduction of nitro blue tetrazolium (NBT) by photochemically generated superoxide radicals. One unit of SOD is defined as the amount of enzyme needed to inhibit the reduction rate of NBT by 50% at 25°C (Burd et al., 2000).
Catalase Activity
This activity was measured following the Aebi (1984) method. H2O2 consumption was monitored for 1 min at 240 nm (Spectrophotometer visible GENESYS™ 30, Thermo Fisher Scientific™, Hampton, NH, United States). This was done by mixing 50 mM potassium phosphate buffer with 10 mM H2O2 and 100 μl of the extract.
Ascorbate Peroxidase Activity
This activity was measured in a 1 ml reaction containing 80 nM potassium phosphate buffer, 2.5 mM H2O2, and 1 M sodium ascorbate. H2O2 was added to start the reaction and the decrease in absorbance was measured for 1 min at 290 nm, (Spectrophotometer visible GENESYS™ 30, Thermo Fisher Scientific™, Hampton, NH, United States) to determine the oxidation rate of ascorbate (Amako et al., 1994).
Glutathione Reductase Activity
This activity was estimated spectrophotometrically at 25°C, according to the Carlberg and Mannervik (1985) method. The method is based on the measurement at 340 nm of the reduction of NADPH oxidation (Spectrophotometer visible GENESYS™ 30, Thermo Fisher Scientific™, Hampton, NH, United States). The reaction mixture contained 50 mM Tris-MgCl2 buffer, 3 mM, 1 mM GSSG, 50 μl enzyme, and 0.3 mM NADPH, added to start the reaction. The activity was calculated with the initial rate of the reaction and the molar extinction coefficient of NADPH (ε340 = 6.22 mM–1 cm–1).
Statistical Analysis
The Kruskal–Wallis statistical analysis was carried out based on non-parametric tests (N < 30). The variables analyzed were root length and weight, number of secondary roots, and enzymatic activities (SOD, CAT, APX, and GR). For those cases in which the value of p < 0.01, a post hoc pairwise comparison test was carried out for all treatments against controls, using the non-parametric Mann–Whitney U-test, applied to two independent samples. Statistically different results were those for which the value of p was <0.01. Duncan’s test was used to group the treatments, based on the difference in their means. A principal component analysis (PCA) was carried out for the reduction of the model. The projection of the two variables that best explain the model on the two-dimensional plane was carried out to study possible groupings between treatments. All analyses were carried out using SPSS Statistics AMOS™ v. 27.0 software (IBM® Company, Armonk, NY, United States).
Results
The results on seed germination and biometry under increasing concentrations of HgCl2 are shown in Figure 1.
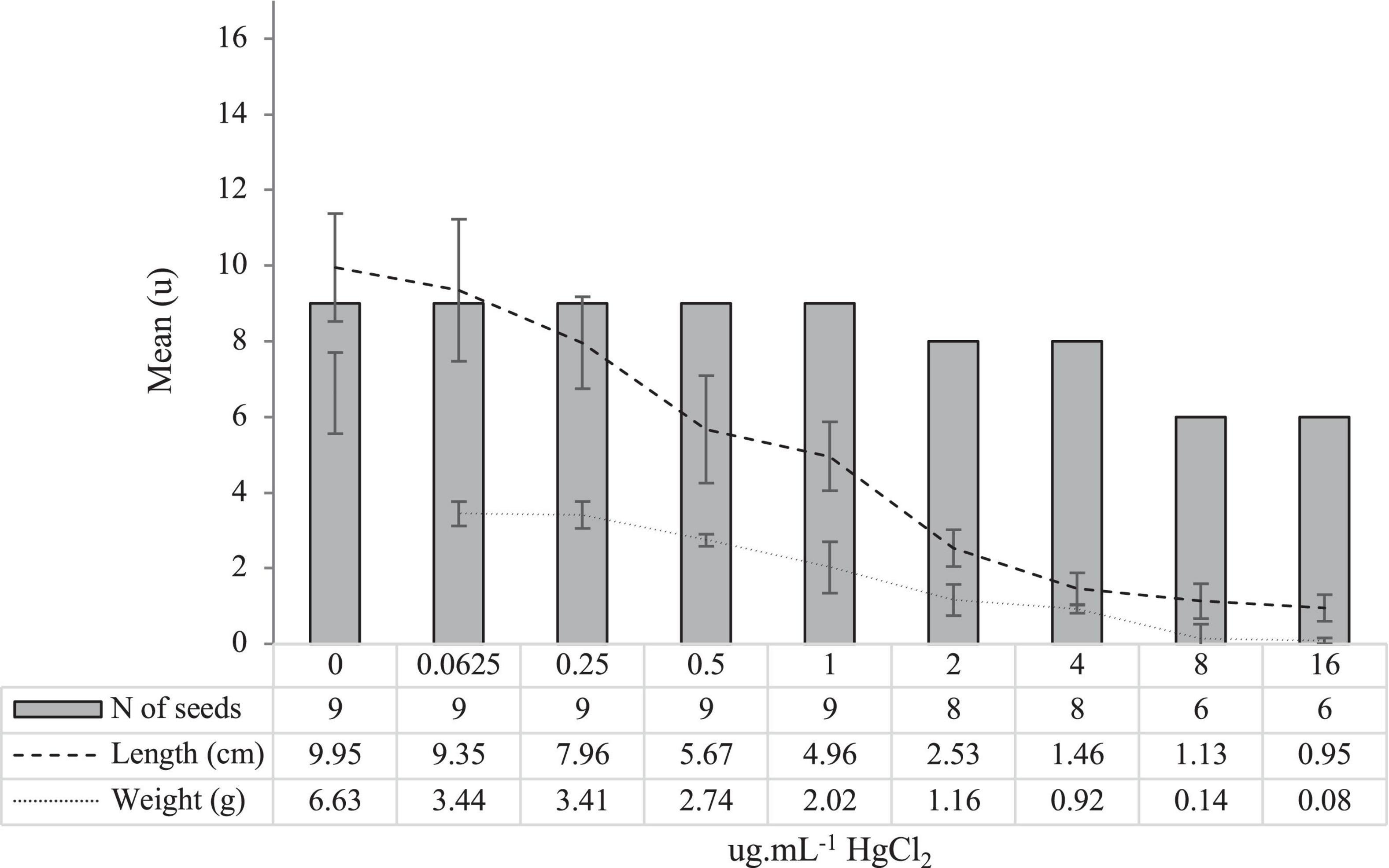
Figure 1. The mean (n = 5 of experimental units with 9 seeds) germination number of seeds (No), root length (cm), and root weight (g) for each concentration of HgCl2 tested (μg ml–1), after 120 h of development.
Results show the ability of L. albus var. Orden Dorado seeds to tolerate high concentrations of Hg, to the detriment of root elongation and weight. The highest concentration at which the seeds were able to germinate was selected (16 μg mL–1).
The biometry results in the presence of the PGPB strains and 16 μg mL–1 of HgCl2 are shown in Table 2.
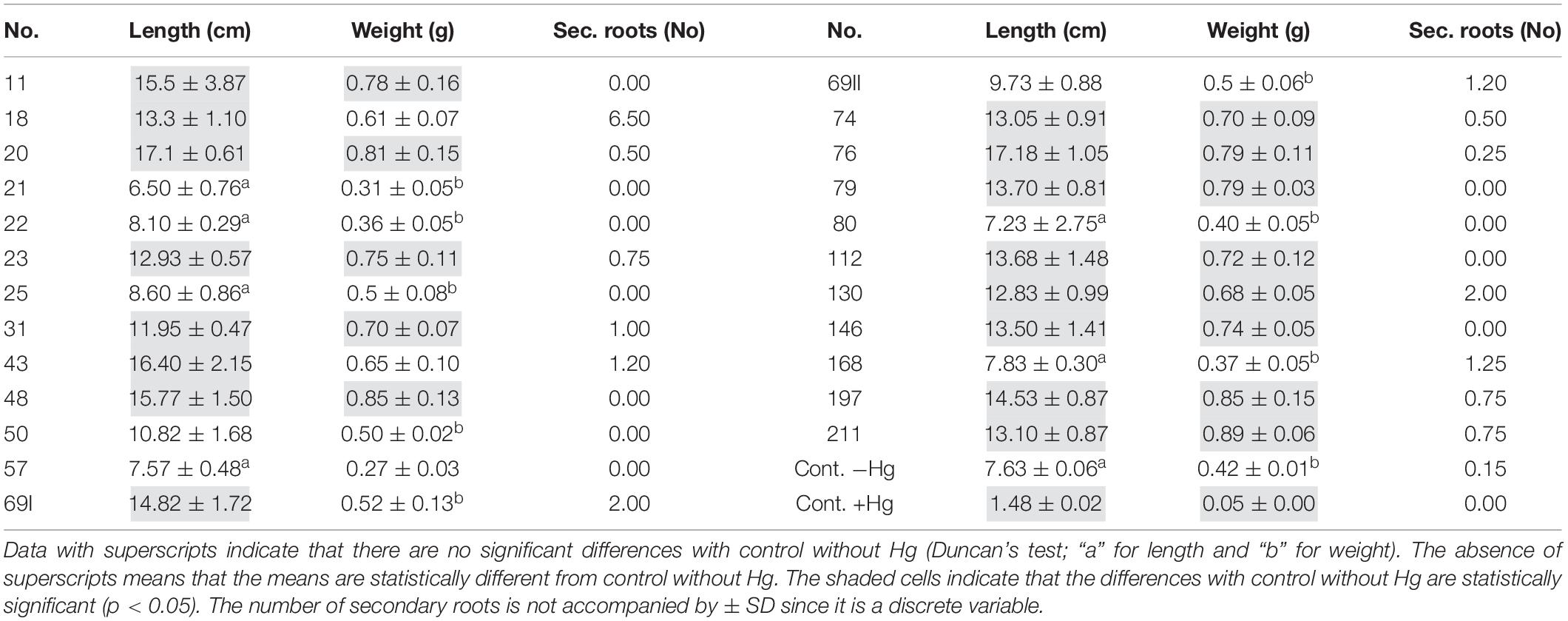
Table 2. The mean values of replicates (n = 5) ± SD for root length (cm), root weight (g) of the different treatments, and controls (absence of Hg and presence of Hg).
Seeds treated with strains 18, 31, 43, 69I, 69II, and 130 develop secondary roots in at least three of their replicates. Specifically, the treatment with strain 20, makes the five replicates develop secondary roots in values that range between 1 and 6 secondary roots per seedling.
All biometric parameters in control without PGPB inoculum, both in the presence of Hg and in its absence, were significantly lower. The observation of the seeds in control with Hg shows that the root elongation was minimal and did not undergo variations concerning the moment of sowing (emerged radicle in pre-germination). The accumulation of Hg in embryonic tissue was evident to the naked eye.
The results of the antioxidant response in the presence of the PGPB strains and 16 μg ml–1 of HgCl2 are shown in Table 3. Treatments with strains 18, 31, 43, and 69I maintain the levels of antioxidant enzymatic activity of control without Hg (p < 0.01), for the enzymes SOD and APX. Strain 18, on the other hand, maintains GR levels statistically analogous to those of control without Hg (p < 0.01).
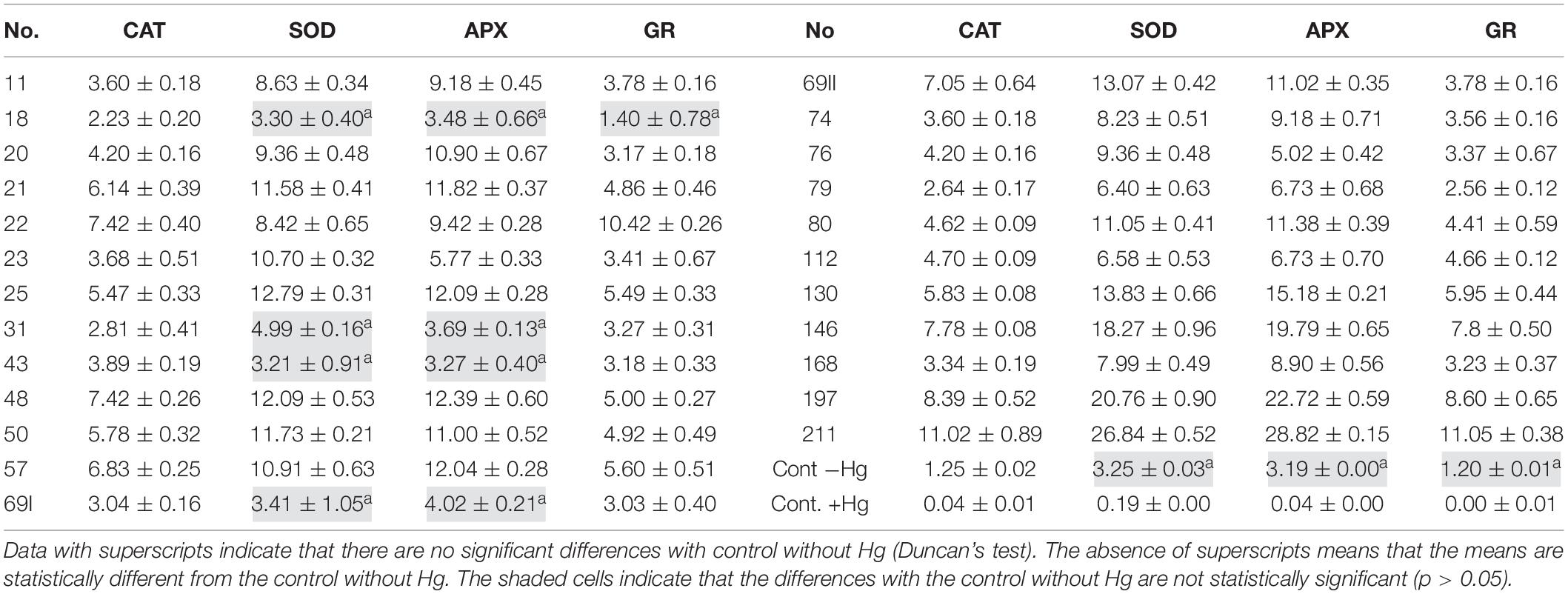
Table 3. The mean values of replicates (n = 5) ± SD for the antioxidant enzymatic activity (enzyme mg Prot–1 min–1) in the presence of PGPB treatments and controls (absence of Hg and presence of Hg).
Principal component analysis allows ordering the variables according to their variance. The variable that most contributes to the explanation of the model is the root length (63.95% of the variance), followed by the root weight (28.17% of the variance), explaining between them 92.12% of the model. The correlation matrix (Table 4), furthermore, establishes a strong positive correlation between them (R = 0.750).
In Figure 2, the two main components (PC1 and PC2) have been projected. The analysis allows the segregation of the strains into two large groups: (i) those that promote biometric improvements in the plant under Hg stress conditions (strains 11, 18, 20, 23, 31, 43, 48, 69I, 74, 76, 79, and 112). Of these, strains 11, 20, 48, and 76 are especially noteworthy. (ii) Those that buffer the antioxidant enzymatic response under Hg stress conditions to levels not significantly different from control in the absence of Hg (strains 18, 31, 43, and 69I).
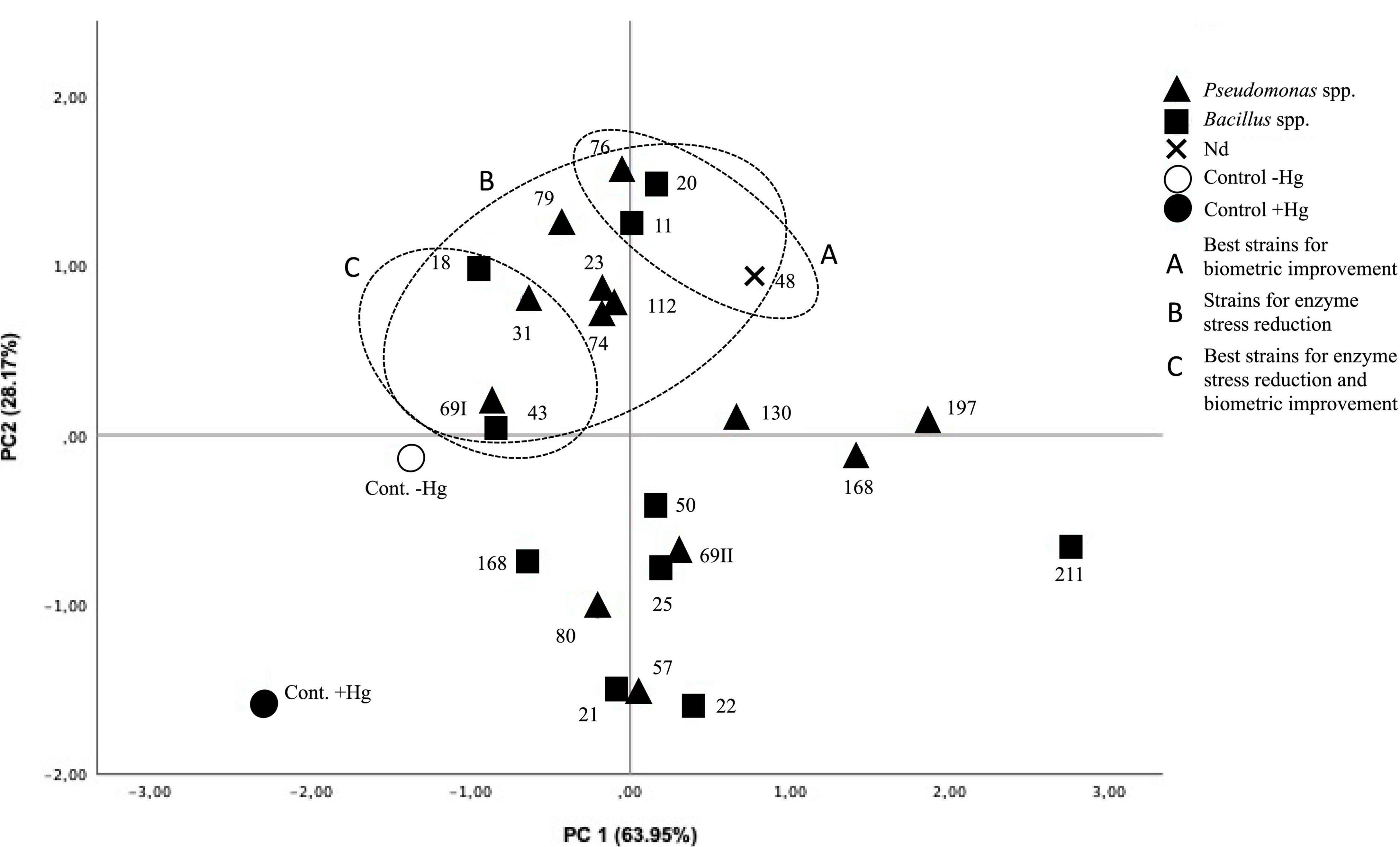
Figure 2. Projection of the two main components (PC1 63.95% and PC2 28.17%). Treatments with higher elongations and root weights segregate toward the quadrant (x, y). Treatments with a high antioxidant response, which distances them from control in the absence of Hg, segregate toward the (x, –y) quadrant. Treatments close to control without Hg are grouped in the (–x, –y) quadrant.
Discussion
Exposure of L. albus seedlings to increasing concentrations of Hg in the absence of PGPB inocula caused physically detectable phytotoxic effects from 24 h after exposure. Since Hg is a non-essential element in biological systems, there are no specific pathways for its metabolism and/or excretion (Kumari et al., 2020). As Hg accumulates and as observed in our results, the toxic effects become more evident as its concentration increases (Figure 1). In the present study, the phytotoxic effects were evaluated at 120 h of growth. The biometric damages (weight and root length) became significantly notable (p < 0.05) from a concentration of 0.5 μg ml–1 of HgCl2, compared with control without Hg. From this concentration, the radicles appeared thickened and necrotic, something that coincides with what was observed by other authors, working with several herbaceous species, such as Medicago sativa (Ortega-Villasante et al., 2007; Zhou et al., 2007) or Allium sativum (Zhao et al., 2013). At the highest concentrations (8 and 16 μg ml–1), the inhibition of root elongation was total, resulting in weights and lengths close to zero (Figure 1). Given that the objective of the present study was to verify whether PGPB inocula allowed to mitigate, or even reverse, the phytotoxic effects of high Hg pressure exposure, the maximum concentration at which the seeds survived (16 μg ml–1) was selected for all further trials. At this concentration, growth inhibition of 33.34% was recorded, results analogous to those observed by Pokorska-Niewiada et al. (2018) in Secale cereale (34.6%) at a concentration somewhat higher than that tested in the present work (20 μg ml–1).
Recent research focuses on the role that microorganisms that inhabit the rhizosphere can play to help the plant in its development in soils contaminated with Hg (Tiodar et al., 2021). Specifically, there is consensus on the importance of understanding the complex plant-microorganism interactions under stress conditions. In the present study, we proceeded with PGPB inocula isolated from the rhizosphere of plants that naturally grow in the mining district of Almadén (Spain) (Table 1). We agree with other authors that, in a situation of Hg stress, plants are more dependent on microbial activity, and are capable of reducing the harmful effect of metals (Amari et al., 2017). This is what we observed after inoculation with the selected PGPB strains. In the presence of Hg, they reversed the process and produced improvements in root length and weight parameters, as well as in the number of secondary roots, in some cases, even significantly compared with control in the absence of Hg. Such is the case of seeds treated with PGPB 18, 31, 43, 69I, 130, and 168, to a greater extent, and strains 20, 23, 74, 76, 197, and 211, to a lesser degree. Although we agree with the observations of Zafar et al. (2015) and Mukhraiya and Bhat (2017), according to which exposure to Hg produces a significant reduction in seed weight, compared with control in the absence of Hg, it is considered that this parameter is not a good indicator of biometric enhancement. The generalized increase in weight in the presence of Hg and PGPB treatment may be due to abnormal thickening due to Hg pressure rather than adequate root development or an increase in the number of secondary roots (Sabreen and Sugiyama, 2008).
One of the mechanisms by which bacteria can facilitate seed development under abiotic stress conditions is through the synthesis of phytohormones. In the present study, those auxin-producing PGPB (5.5–6.0 μg ml–1), such as IAA, have been used as a selection criterion. These hormones are produced in the plant stems and transported to the root tips (Tiodar et al., 2021) where, at moderate concentrations, they favor elongation and promote the development of secondary roots, as occurs in plants inoculated with strains 18, 31, 43, 69I, 130, and 168. On the contrary, IAA concentrations ≥6.0 μg ml–has an inhibitory effect on root growth and development. In part, this could be related to ethylene synthesis (Glick, 2003), which translates into an almost null secondary roots development when seeds are inoculated with strains 57, 74, 80, and 211. The regulation of ACC concentrations in response to stress conditions is another mechanism by which bacteria exert a beneficial effect on plants subjected to abiotic stress (Saleem et al., 2007). The hydrolysis of ACC by the bacterial enzyme ACC deaminase (ACCd) produces a decrease in ethylene levels, which increases root development (Glick, 2003; Belimov et al., 2009). Strains 11, 20, 48, and 76 produce significantly higher root elongation than the rest of the traits and controls, possibly due to the combined action of blocking the effect of ethylene produced by the plant under Hg stress conditions via ACCd and the activation of cell elongation by the moderate production of IAA. In addition, bacterial siderophores counteract the plant’s inability to accumulate enough Fe from the soil, favoring its bioavailability and avoiding a stressful situation that can promote an increase in ethylene concentration. Unlike what happens with other heavy metals, the role of siderophores in relation to Hg chelation is not known, although it is believed that they could improve Hg absorption in the root (Tiodar et al., 2021). Strains 11, 21, 23, 25, 43, 80, 130, and 146 are good producers of siderophores and could be interesting for further field use in bioremediation processes, where Fe is a limiting element. Strains 11 and 20 (Bacillus toyonensis), 48 (not described), and 76 (Pseudomonas corrugata), capable of favoring plant development by promoting their growth in the presence of high heavy metals pressure, are interesting candidates for further biotechnological uses, in which an increase in root biomass or plant cover is sought.
Another consequence of Hg exposure is the appearance of oxidative stress with the accumulation of reactive oxygen species (ROS) (Çavuşoğlu et al., 2022) and an increase in the antioxidant enzymatic response (Kim et al., 2017). When plants exceed the threshold of tolerance to heavy metals, their metabolism can be altered, cellular homeostasis is compromised, electron transport chains and the functioning of lipids and proteins are altered, DNA damage is produced and activates the antioxidant defense system (Yang et al., 2018). In the present work, we observe how the exposure of L. albus to Hg in the absence of PGPB causes the necrosis of the seeds at 24 h of exposure, which translates into an antioxidant activity tending to zero for the four quantified enzymes. This should not be interpreted as a lack of activity per se, but rather the death of the seeds in the absence of PGPB treatment that neutralizes Hg toxicity, as occurs in treatments with PGPB 18, 23, 41, and 69I. The molecular mechanisms by which heavy metals induce ROS formation are not well described, although they have been shown to induce oxidative stress (Cargnelutti et al., 2006; Ajitha et al., 2021; Çavuşoğlu et al., 2022). Traditionally, the accumulation of ROS has been considered a negative effect on plants, the prelude to the cell degradation process. However, there is evidence that it can be a nuclear part of the cellular response system (Flores-Cáceres, 2013), being able to act as secondary messengers that regulate growth and development functions (Foyer and Noctor, 2005). Consequently, it is important for the plant to control the concentration of ROS, but not to eliminate them completely (Çavuşoğlu et al., 2022). In this sense, Kim et al. (2017) studied the role of GR in mitigating the toxicity of Hg (and other heavy metals) on the development of Arabidopsis thaliana. They concluded that GR has a much stronger binding affinity for Hg than for Cd, Cu, or Zn, suggesting that tight binding of GR to Hg prevents its uptake, leading to a low accumulation of Hg in plant cells, additionally improving their physiology. This fact is observed in the treatment with strain 18.
In accordance with other authors (Sapre et al., 2019), in the present study, it has been shown that seeds are viable at high Hg concentrations only when treated with PGPB. In the assays carried out in this work, the seeds were developed under the same conditions (environmental, model plant species, and Hg concentration), so the unequal enzymatic responses could be explained by the PGPB effect. For further biotechnological uses of PGPB strains in biotechnological processes, those treatments that manage to mitigate the accumulation of ROS in the plant will be interesting. This effect has been observed by other authors after the use of PGPB from the Bacillus sp. (Vardharajula et al., 2011; Moreno-Galván et al., 2020) and Pseudomonas sp. genera (Dos Santos et al., 2021). In the present work, strains 31 and 69I (P. corrugata) and 18 and 43 (Bacillus toyonensis) can maintain the SOD and APX enzymatic responses at low-stress levels, without significant differences compared with control in the absence of Hg (p < 0.01). Additionally, strain 18 shows a significant reduction to control levels in the absence of Hg in the GR enzyme. This minimizing effect of enzymatic activity in the presence of abiotic stress has been documented by other authors when working with PGPB inocula and other heavy metals, such as Pb (Abdelkrim et al., 2018), Cu (Fatnassi et al., 2015), Zn (Islam et al., 2014) and Cd (Azimychetabi et al., 2021). According to Kasim et al. (2013), a decrease in antioxidant activity in plants subjected to PGPB treatment can be interpreted as a better adaptation to stress conditions, as it plays a phytoprotective role, which protects plants from abiotic stress. Treatment with strains 18, 31, 43, and 69I could be indicated to improve plant physiology in biotechnological processes involved in the recovery of environments contaminated with heavy metals.
Conclusion
The recovery of Hg-contaminated sites is a topic of current interest. Phytoremediation of Hg-contaminated soils is an emerging strategy. Species, such as L. albus have improved tolerance to inhibitory concentrations of Hg when inoculated with PGPB. The symbiotic relationship of some of these PGPB with plants exerts a phytoprotective role that improves their fitness, from biometrics to oxidative stress alleviation. In particular, we find that treating L. albus var. Orden Dorado seeds with strains 31 and 69I (P. corrugata), 18 and 43 (Bacillus toyonensis) in the presence of high Hg concentrations, minimize the antioxidant response of the seed at these levels of treatment in the absence of Hg. This reflects the phytoprotective nature of these PGPB traits, and as a result, they are postulated as good candidates for further bioremediation processes, in which plant physiology improvement is sought. Similarly, treatment of L. albus var. Orden Dorado seedlings with PGPB strains 11 and 20 (B. toyonensis), 48 (Not described), and 76 (P. corrugata) improves plant biometry (root length and weight) due to the unique combination of their PGPB activities. This makes them good candidates for further bioremediation trials where there is a special interest in achieving notable increases in biomass and plant cover. A detailed investigation is needed to understand the molecular mechanisms underlying Hg uptake, accumulation, and sequestration in plants, and the interactions between plants and their associated microorganisms. In this sense, it is also necessary to unravel the molecular mechanisms by which certain PGPB indirectly prevent Hg from exerting a phytoprotective role as described in this work.
Data Availability Statement
The original contributions presented in this study are included in the article/supplementary material, further inquiries can be directed to the corresponding author/s.
Author Contributions
MR and PJ: conceptualization. MR, DG, AP, and PJ: methodology. MR: software, writing—original draft preparation, and visualization. PJ: validation and data curation. MR, AP, and PJ: formal analysis and writing—review and editing. MR and DG: investigation. AP and PJ: resources, supervision, and funding acquisition. AP: project administration. All authors read and agreed to the published version of the manuscript.
Funding
This research has been funded by the Fundacióìn Universitaria San Pablo CEU and Banco Santander, grant number FUSP-BS-PPC01/2014. Grants to recognized research groups 2021/22 (Vice President for Research and Teaching Staff, CEU San Pablo University).
Conflict of Interest
The authors declare that the research was conducted in the absence of any commercial or financial relationships that could be construed as a potential conflict of interest.
Publisher’s Note
All claims expressed in this article are solely those of the authors and do not necessarily represent those of their affiliated organizations, or those of the publisher, the editors and the reviewers. Any product that may be evaluated in this article, or claim that may be made by its manufacturer, is not guaranteed or endorsed by the publisher.
Acknowledgments
We are grateful to the Seed Bank of the Center for Scientific and Technological Research of Extremadura, for its permanent availability in the supply of seeds for trials.
References
Abdel Latef, A. A. H., Abu Alhmad, M. F., and Abdelfattah, K. E. (2017). The possible roles of priming with ZnO nanoparticles in mitigation of salinity stress in Lupine (Lupinus termis) Plants. J. Plant Growth Regul. 36, 60–70.
Abdelkrim, S., Jebara, S. H., and Jebara, M. (2018). Antioxidant systems responses and the compatible solutes as contributing factors to lead accumulation and tolerance in Lathyrus sativus inoculated by plant growth promoting rhizobacteria. Ecotoxicol. Environ. Saf. 166, 427–436. doi: 10.1016/j.ecoenv.2018.09.115
Ajitha, V., Sreevidya, C. P., Sarasan, M., Park, J. C., Mohandas, A., Singh, I. S. B., et al. (2021). Effects of zinc and mercury on ROS-mediated oxidative stress-induced physiological impairments and antioxidant responses in the microalga Chlorella vulgaris. Environ. Sci. Pollut. Res. 28, 32475–32492. doi: 10.1007/s11356-021-12950-6
Ali, B., Wang, X., Saleem, M. H., Hafeez, A., Afridi, M. S., Khan, S., et al. (2022). PGPR-Mediated salt tolerance in maize by modulating plant physiology, antioxidant defense, compatible solutes accumulation and bio-surfactant producing genes. Plants 11:345. doi: 10.3390/plants11030345
Amako, K., Chen, G.-X., and Asada, K. (1994). Separate assays specific for ascorbate peroxidase and guaiacol peroxidase and for the chloroplastic and cytosolic isozymes of ascorbate peroxidase in plants. Plant Cell Physiol. 35, 497–504. doi: 10.1093/oxfordjournals.pcp.a078621
Amari, T., Ghnaya, T., and Abdelly, C. (2017). Nickel, cadmium, and lead phytotoxicity and potential of halophytic plants in heavy metal extraction. S. Afr. J. Bot. 111, 99–110. doi: 10.1016/j.sajb.2017.03.011
Azimychetabi, Z., Sabokdast Nodehi, M., Karami Moghadam, T., and Motesharezadeh, B. (2021). Cadmium stress alters the essential oil composition and the expression of genes involved in their synthesis in peppermint (Mentha piperita L.). Ind. Crops Prod. 168:113602. doi: 10.1016/j.indcrop.2021.113602
Belimov, A. A., Dodd, I. C., Hontzeas, N., Theobald, J. C., Safronova, V. I., and Davies, W. J. (2009). Rhizosphere bacteria containing 1-aminocyclopropane-1-carboxylate deaminase increase yield of plants grown in drying soil via both local and systemic hormone signalling. New Phytol. 181, 413–423. doi: 10.1111/j.1469-8137.2008.02657.x
Burd, G. I., Dixon, D. G., and Glick, B. R. (2000). Plant growth-promoting bacteria that decrease heavy metal toxicity in plants. Can. J. Microbiol. 46, 237–245. doi: 10.1139/w99-143
Cargnelutti, D., Tabaldi, L. A., Spanevello, R. M., de Oliveira Jucoski, G., Battisti, V., Redin, M., et al. (2006). Mercury toxicity induces oxidative stress in growing cucumber seedlings. Chemosphere 65, 999–1006. doi: 10.1016/j.chemosphere.2006.03.037
Çavuşoğlu, D., Macar, O., Kalefetoğlu Macar, T., Çavuşoğlu, K., and Yalçın, E. (2022). Mitigative effect of green tea extract against mercury (II) chloride toxicity in Allium cepa L. model. Environ. Sci. Pollut. Res. Int. 4, 27862–27874. doi: 10.1007/s11356-021-17781-z
Díaz Puente, F. J. (2013). Variación de la Actividad Fisiológica de la Microflora del Suelo en Terrenos Afectados por Actividades Mineras. Ph.D. tesis. Madrid: Universidad Complutense.
Dos Santos, M., Dias-Filho, M. B., and Cajueiro, E. S. (2021). Successful plant growth-promoting microbes: inoculation methods and abiotic factors. Front. Sustain. Food Syst. 5:606454. doi: 10.3389/fsufs.2021.606454
European Commission (2011). Council Conclusions, 2011. Review of the Community Strategy Concerning Mercury. Available online at: http://ec.europa.eu/environment/chemicals/mercury/ (accessed March 14, 2011).
Fatnassi, I. C., Chiboub, M., Saadani, O., Jebara, M., and Jebara, S. H. (2015). Impact of dual inoculation with Rhizobium and PGPR on growth and antioxidant status of Vicia faba L. under copper stress. C. R. Biol. 338, 241–254. doi: 10.1016/j.crvi.2015.02.001
Flores-Cáceres, M. L. (2013). Caracterización de los Mecanismos de Respuesta Antioxidantede Medicago sativa a Metales Pesados. Ph.D. tesis. Madrid: Universidad Autónoma de Madrid.
Foyer, C. H., and Noctor, G. (2005). Oxidant and antioxidant signalling in plants: a re-evaluation of the concept of oxidative stress in a physiological context. Plant Cell Environ. 28, 1056–1071. doi: 10.1111/j.1365-3040.2005.01327.x
Glick, B. R. (2003). Phytoremediation: synergistic use of plants and bacteria to clean up the environment. Biotechnol. Adv. 21, 383–393. doi: 10.1016/s0734-9750(03)00055-7
Gontia-Mishra, I., Sapre, S., Sharma, A., and Tiwari, S. (2016). Alleviation of mercury toxicity in wheat by the interaction of mercury-tolerant plant growth-promoting rhizobacteria. J. Plant Growth Regul. 35, 1000–1012.
González, D., Robas, M., Probanza, A., and Jiménez, P. A. (2021b). Selection of mercury-resistant PGPR strains using the BMRSI for bioremediation purposes. Int. J. Environ. Res. Public Health 18:9867. doi: 10.3390/ijerph18189867
González, D., Blanco, C., Probanza, A., Jiménez, P. A., and Robas, M. (2021a). Evaluation of the PGPR capacity of four bacterial strains and their mixtures, tested on Lupinus albus var. dorado seedlings, for the bioremediation of mercury-polluted soils. Processes 9:1293.
Islam, F., Yasmeen, T., Ali, Q., Ali, S., Arif, M. S., Hussain, S., et al. (2014). Influence of Pseudomonas aeruginosa as PGPR on oxidative stress tolerance in wheat under Zn stress. Ecotoxicol. Environ. Saf. 104, 285–293. doi: 10.1016/j.ecoenv.2014.03.008
Kasim, W. A., Osman, M. E., Omar, M. N., and Abd El-Daim, I. A. (2013). Control of drought stress in wheat using plant-growth-promoting bacteria. J. Plant Growth Regul. 32, 122–130. doi: 10.1007/s00344-012-9283-7
Kim, Y. O., Bae, H. J., Cho, E., and Kang, H. (2017). Exogenous glutathione enhances mercury tolerance by inhibiting mercury entry into plant cells. Front. Plant Sci. 8:683. doi: 10.3389/fpls.2017.00683
Kumari, S., Jamwal, R., Mishra, N., and Singh, D. K. (2020). Recent developments in environmental mercury bioremediation and its toxicity: a review. Environ. Nanotechnol. Monit. Manage. 13:100283.
Mariano, C., Mello, I. S., Barros, B. M., da Silva, G. F., Terezo, A. J., and Soares, M. A. (2020). Mercury alters the rhizobacterial community in Brazilian wetlands and it can be bioremediated by the plant-bacteria association. Environ. Sci. Pollut. Res. 27, 13550–13564.
Millán, R., Schmid, M. J., Carrasco-Gil, S., Villadóniga, M., Rico, C., Sánchez Ledesma, D. M., et al. (2011). Spatial variation of biological and pedological properties in an area affected by a metallurgical Mercury plant: Almadenejos (Spain). Appl. Geochem. 26, 174–181. doi: 10.1016/j.apgeochem.2010.11.016
Moreno-Galván, A., Romero-Perdomo, F. A., Estrada-Bonilla, G., Meneses, C. H., and Bonilla, R. R. (2020). Dry-Caribbean Bacillus spp. strains ameliorate drought stress in maize by a strain-specific antioxidant response modulation. Microorganisms 8:823. doi: 10.3390/microorganisms8060823
Mukhraiya, D., and Bhat, J. L. (2017). Assessment of mercury toxicity on seed germination, growth and antioxidant enzyme expression of Sorghum vulgare var. SG- 1000 seedlings. IJTAS 9, 45–50.
Ortega-Villasante, C., Hernández, L. E., Rellán-Alvarez, R., Del Campo, F. F., and Carpena-Ruiz, R. O. (2007). Rapid alteration of cellular redox homeostasis upon exposure to cadmium and mercury in alfalfa seedlings. New phytol. 176, 96–107. doi: 10.1111/j.1469-8137.2007.02162.x
Paisio, C. E., González, P. S., Talano, M. A., and Agostini, E. (2012). Remediación biológica de Mercurio: Recientes avances. Rev. Latinoam. Biotecnol. Amb. Algal. 3, 119–146.
Peralta-Videa, J. R., Lopez, M. L., Narayan, M., Saupe, G., and Gardea-Torresdey, J. (2009). The biochemistry of environmental heavy metal uptake by plants: Implications for the food chain. Int. J. Biochem. Cell Biol. 41, 1665–1677. doi: 10.1016/j.biocel.2009.03.005
Pokorska-Niewiada, K., Rajkowska-Myśliwiec, M., and Protasowicki, M. (2018). Acute lethal toxicity of heavy metals to the seeds of plants of high importance to humans. Bull. Environ. Contam. Toxicol. 101, 222–228. doi: 10.1007/s00128-018-2382-9
Robas, M., Jiménez, P. A., González, D., and Probanza, A. (2021). Bio-mercury remediation suitability index: a novel proposal that compiles the PGPR features of bacterial strains and its potential use in phytoremediation. Int. J. Environ. Res. Public Health 18:4213. doi: 10.3390/ijerph18084213
Sabreen, S., and Sugiyama, S. (2008). Trade-off between cadmium tolerance and relative growth rate in 10 grass species. Environ. Exp. Bot. 63, 327–332. doi: 10.1016/j.envexpbot.2007.10.019
Saleem, M., Arshad, M., Hussain, S., and Bhatti, A. S. (2007). Perspective of plant growth promoting rhizobacteria (PGPR) containing ACC deaminase in stress agriculture. J. Ind. Microbiol. Biotechnol. 34, 635–648. doi: 10.1007/s10295-007-0240-6
Sapre, S., Deshmukh, R., Gontia-Mishra, I., and Tiwari, S. (2019). “Problem of Mercury Toxicity in Crop Plants: Can Plant Growth Promoting Microbes (PGPM) Be an Effective Solution?,” in Field Crops: Sustainable Management by PGPR. Sustainable Development and Biodiversity, eds D. Maheshwari and S. Dheeman (Cham: Springer).
Tiodar, E. D., Vǎcar, C. L., and Podar, D. (2021). Phytoremediation and Microorganisms-Assisted Phytoremediation of Mercury-Contaminated Soils: Challenges and Perspectives. Int. J. Environ. Res. Public Health 18:2435. doi: 10.3390/ijerph18052435
Tiwari, S., and Lata, C. (2018). Heavy metal stress, signaling, and tolerance due to plant-associated microbes: an overview. Front. Plant Sci. 9:452. doi: 10.3389/fpls.2018.00452
Tounsi-Hammami, S., Dhane-Fitouri, S., Le Roux, C., Hammami, Z., and Ben Jeddi, F. (2020). Potential of native inoculum to improve the nodulation and growth of white lupin in Tunisia. Ann. Inst. Nat. Rech. Agron. Tunis. 93, 102–114.
Vardharajula, S., Zulfikar Ali, S., Grover, M., Reddy, G., and Bandi, V. (2011). Drought-tolerant plant growth promoting Bacillus spp.: effect on growth, osmolytes, and antioxidant status of maize under drought stress. J. Plant Interact. 6, 1–14. doi: 10.1080/17429145.2010.535178
Wang, J., Feng, X., Anderson, C. W., Xing, Y., and Shang, L. (2012). Remediation of mercury contaminated sites - A review. J. Hazard Mater. 22, 1–18. doi: 10.1016/j.jhazmat.2012.04.035
World Health Organization [WHO] (2007). Exposure to Mercury: a Major Public Health Concern. Geneva: World Health Organization.
Yang, J., Li, G., Bishopp, A., Heenatigala, P. P. M., Hu, S., Chen, Y., et al. (2018). Comparison of growth on mercuric chloride for three lemnaceae species reveals differences in growth dynamics that effect their suitability for use in either monitoring or remediating ecosystems contaminated with mercury. Front. Chem. 16:112. doi: 10.3389/fchem.2018.00112
Zafar, M., Khan, M., Athar, M., Shafiq, M., Farooqi, Z. U., and Kabir, M. (2015). Effect of Mercury on Seed Germination and Seedling Growth of Mungbean (Vigna radiata (L.) Wilczek). J. Appl. Sci. Environ. Manage. 19, 191–199. doi: 10.4314/jasem.v19i2.4
Zhao, J., Gao, Y., Li, Y. F., Hu, Y., Peng, X., Dong, Y., et al. (2013). Selenium inhibits the phytotoxicity of mercury in garlic (Allium sativum). Environ. Res. 125, 75–81. doi: 10.1016/j.envres.2013.01.010
Keywords: oxidative stress, bioremediation, heavy metals – contamination, plant biometry, soil bacteria
Citation: Robas Mora M, Jiménez Gómez PA, González Reguero D and Probanza Lobo A (2022) Effect of Plant Growth-Promoting Bacteria on Biometrical Parameters and Antioxidant Enzymatic Activities of Lupinus albus var. Orden Dorado Under Mercury Stress. Front. Microbiol. 13:891882. doi: 10.3389/fmicb.2022.891882
Received: 08 March 2022; Accepted: 02 May 2022;
Published: 22 June 2022.
Edited by:
Krishnendu Pramanik, Visva-Bharati University, IndiaReviewed by:
Hayssam M. Ali, King Saud University, Saudi ArabiaHimani Singh, Shri Ramswaroop Memorial University, India
Copyright © 2022 Robas Mora, Jiménez Gómez, González Reguero and Probanza Lobo. This is an open-access article distributed under the terms of the Creative Commons Attribution License (CC BY). The use, distribution or reproduction in other forums is permitted, provided the original author(s) and the copyright owner(s) are credited and that the original publication in this journal is cited, in accordance with accepted academic practice. No use, distribution or reproduction is permitted which does not comply with these terms.
*Correspondence: Marina Robas Mora, bWFyaW5hLnJvYmFzbW9yYUBjZXUuZXM=; Pedro Antonio Jiménez Gómez, UGVkcm8uamltZW5lemdvbWV6QGNldS5lcw==