- College of Pharmacy, Yonsei Institute of Pharmaceutical Sciences, Yonsei University, Incheon, South Korea
In this study, we focused on endophytes of Maesa japonica (Thunb.) Moritzi & Zoll. and the plant-microbe interaction at metabolite levels. We isolated seven endophytes associated with M. japonica (JB1−7), and focused on Streptomyces olivaceus JB1 because of antibacterial activities of its secondary metabolites. We confirmed lobophorin analogs production from the bacterial strain JB1 by using spectroscopic techniques such as NMR, UV, and LC/Q-TOF-MS. In the LC/MS system, thirteen reported lobophorin analogs and twelve unreported analogs were detected. Among metabolites, lobophorin A was clearly detected in the dried foliar residues of M. japonica which implies that JB1 resides in the host and accumulates its secondary metabolites likely interacting with the plant. Antimicrobial activity tests of the secondary metabolites against undesirable contaminants isolated from the external surface of M. japonica supported the host and microbe mutualistic relationship. In the meantime, lobophorin producing Streptomyces spp. were isolated from marine environments such as marine sediments, algae, corals, and sponges. As lobophorin producing Streptomyces is isolated commonly from marine environments, we conducted a saline water stress tolerance test with JB1 showing saline medium does not accelerate the growth of the bacterium.
Introduction
Maesa japonica (Thunb.) Moritzi & Zoll. of the monotypic family Maesaceae is a perennial evergreen shrub growing up to 1–1.5 m that is geographically distributed throughout humid subtropical climate regions such as South-Central China, Southeast China, Japan, Taiwan, and Vietnam. In South Korea, the previously unrecorded M. japonica was first discovered in 2006 and thrives within a specific wet shrub forest area across southwestern Jeju (Supplementary Figure 12). M. japonica has been used as a medicinal plant in certain parts of China and has high potential pharmaceutical value because of its rich secondary metabolites, including maesaquinone, embelin, rapanone, and 1,1-diphenyl-2-picrylhydrazyl (DPPH), which act as antioxidants (Moon et al., 2006; Kim, 2014; Kwon et al., 2020). Recently, M. japonica has also been recognized as an indoor air purifying plant that removes particulate matter (PM) and reduces fine dust including PM1, PM2.5, and PM10 (Kwon et al., 2021). Despite these unique advantages of M. japonica, its phyllosphere endophytes have remained unstudied due to its low accessibility and non-agricultural uses.
In comparison to deciduous plants whose leaves basically have an annual lifespan, the longer leaf lifespan of perennial evergreen shrubs could allow the establishment of a relatively more stable year-round microbial community within the phyllosphere. For example, a broad-leaf evergreen plant such as southern magnolia, Magnolia grandiflora, combining a typical leaf surface with the presence of year-round leaves proves to be an effective plant-microbe ecosystem, little influenced by environmental change and seasonal variation in its phyllosphere communities and displaying no major changes in bacterial lineages (Jackson and Denney, 2011). Additionally, Müller et al. (2015) reported that the endophytic bacterial diversity of olive trees, a representative evergreen species, may be stable over long periods of time because of the longevity and high genetic variability of cultivars; and these organisms may have antagonistic potential against the fungus Verticillium dahliae (Aranda-Ocampo et al., 2011). Human et al. (2016) detected antifungal secondary metabolites such as antifungal fungichromin, and antiviral actiphenol from endophytic Streptomyces spp. of Protea infructescences, an evergreen perennial plant distributed throughout sub-Saharan Africa and identified Streptomyces spp. as the most likely organism contributing diverse advantages to Protea infructescences by maintaining the plant-microbe symbiosis.
This study reports bacterial and fungal endophytes from M. japonica and antibiotic metabolites produced by Streptomyces olivaceus as endophytes in nature. The S. olivaceus sp. is known as a marine-derived bacterial strain isolated from diverse marine environments. The strain of S. olivaceus SCSIO T05 was isolated from an Indian Ocean deep-sea sediment producing rishirilides, galvaquinones, and lupinacidin D (Zhang et al., 2018) and the S. olivaceus OUCLQ19-3 was isolated from a mud sample collected from the cold-seep area of the South China Sea producing antibacterial dixiamycins (Jin et al., 2021). The S. olivaceus FXJ8.012 producing tetroazolemycins and spoxazomicins was isolated from an Indian Ocean deep-sea water sample (Liu et al., 2013). In recent years, the S. olivaceus sp. as producers of lobophorins were isolated from marine sediment (Chen et al., 2013; Nguyen et al., 2020) and the S. olivaceus sp. associated with the cold-water coral Lophelia pertusa produces our target metabolites, lobophorin analogs (Braña et al., 2017).
We focused on a specific hypothesis that unrevealed beneficial endophytes of the host, M. japonica, a perennial evergreen shrub, produce antibiotic metabolites that are accumulated in planta at detectable levels. The antimicrobial activities of secondary metabolites of the S. olivaceus JB1 (GenBank accession no. OM845781, 99%) against external strains of known phytopathogen strains, including four bacterial strains, Bacillus megaterium, Bacillus thuringiensis, Leclercia adecarboxylata, and Pseudomonas punonensis, and one fungal strain, Mucor circinelloides were also demonstrated.
Materials and Methods
Plant Collection, Bacterial Isolation, and Cultivation Procedures
A vegetative shoot of the M. japonica was initially collected as a plant material from an unmanned forest (Hwasun Gotjawal, N33.2636198 E126.3346747) in Jeju Island, South Korea in April 2021. The foliage of M. japonica was soaked in 5% sodium hypochlorite for 5 min and the surface was disinfected with 80% aqueous ethanol to remove external contaminants from the leaf blade. The sterilized leaves and leaf residues of M. japonica were cut into pieces and placed onto chitin medium (6 g of chitin, 0.75 g of K2HPO4, 0.5 g of MgSO4⋅7H2O, and 3.5 g of K2HPO4, 10 mg of FeSO4⋅7H2O, 10 mg of MnCl2⋅4H2O, 10 mg of ZnSO4⋅7H2O, 100 mg of cycloheximide, and 36 g of agar per 1 L of sterilized water), Czapek Dox medium (30 g of sucrose, 2 g of NaNO3, 1 g of K2HPO4, 0.5 g of MgCl, 0.5 g of KCl, 0.01 g of FeCl2, 100 mg of cycloheximide, and 18 g of agar per 1 L of sterilized water), and A1 medium (10 g of starch, 4 g of yeast extract, 2 g of peptone, 100 mg of cycloheximide, and 18 g of agar per 1 L of sterilized water) for 20 days to isolate endophytes associated with M. japonica. Identical bacterial isolation media without cycloheximide were used for isolating external microbes from M. japonica. Each isolate was cultivated in modified K media (12 g of LB, 12 g of PDB, 1 g of TSB, and 18 g of agar per 1 L of sterilized water, 28°C, 6 days).
DNA was extracted from the isolates by using a QIAamp DNA Mini Kit (Qiagen) according to the manufacturer’s instructions. The DNA extract was amplified with 10 pmole/μL primers, 2.5 mM dNTP mixture, 10x Taq PCR buffer 2 μL, template 20 ng/μL, KOMA-Taq 2.5 U/μL and distilled water. The amplification conditions were as follows: initial denaturation at 95°C for 5 min, followed by 30 cycles of denaturation at 95°C for 30 s, annealing at 55°C, and extension at 68°C for 1.5 min, and a final extension at 68°C for 10 min. The purified PCR products were sequenced in the forward and reverse directions in separate reactions and in duplicate. The PCR amplification primer sequences were as follows: 27F 5′ (AGA GTT TGA TCM TGG CTC AG) 3′ and 1492R 5′ (TAC GGY TAC CTT GTT ACG ACT T) 3′ for 16S rRNA. The primer sequences were as follows: NS1 5′ (GTA GTC ATA TGC TTG TCT C) 3′ and NS24 5′ (AAA CCT TGT TAC GAC TTT TA) 3′ for 18S rRNA. The purified PCR products were sequenced by using two primer pairs, 785F 5′ (GGA TTA GAT ACC CTG GTA) 3′ and 907R 5′ (CCG TCA ATT CMT TTR AGT TT) 3′ for 16S rRNA and NS1 5′ (GTA GTC ATA TGC TTG TCT C) 3′ and NS24 5′ (AAA CCT TGT TAC GAC TTT TA) 3′ for 18S rRNA. Sequencing was performed by using a Big Dye terminator cycle sequencing kit v.3.1 (Applied Biosystems, United States). Sequencing products were resolved on an Applied Biosystems model 3730XL automated DNA sequencing system (Applied Biosystems, United States).
Bacterial Metabolomic Studies
After cultivating the isolates JB1−JB7 in modified K liquid media for 6 days, the cultures were subjected to extraction with ethyl acetate/water layer separation by a separating funnel, and the organic phase was concentrated in vacuo. The crude extract was dissolved in methanol at a concentration of 250 μg/mL and analyzed with HPLC (high-performance liquid chromatography) − MS. HPLC measurements were carried out on an Agilent 1290 UHPLC system (Agilent Technologies, Santa Clara, CA, United States) equipped with a 1290 Infinity binary pump and YMC-triart C18 column (150 × 2.0 mm, 1.9 μm; YMC Korea Co., Seongnam, South Korea). Each sample was analyzed by using the following conditions: gradient: 0-20 min, 10-100% B, 20-23 min, 100% B, 23-24 min, 100-10% B, 24-30 min, 10% B, with an injection volume of 10 μL and a flow rate of 0.4 mL/min. All eluents of the HPLC system were acidified with 0.1% formic acid. H2O was used as eluent A, while acetonitrile served as eluent B. Mass spectrometry was performed on an Agilent 6530 quadrupole-time of flight mass spectrometer (Q-TOF-MS, Agilent Technologies, Santa Clara, CA, United States) equipped with an electrospray ion (ESI) source. The MS experiment was performed under the following conditions: drying gas temperature 300°C, drying gas flow rate 8 L/min, sheath gas temperature 350°C, sheath gas flow rate 11 L/min, and capillary voltage +3.5 kV with positive mode (pressure of nebulizer 35 psig, capillary voltage 3500 V, fragmentor 175 V, skimmer 65 V, and OCT 1 RF Vpp 750 V).
Impact of Saline Water Stress Tolerance on S. olivaceus JB1
A 10 mL preculture of the bacterial strain JB1 was transferred into non-saline and saline K media (salinity; 33 per mille of sodium chloride) separately. Both cultures were cultivated under the same conditions at 28°C with shaking at 180 rpm for 4 days. Bacterial cell growth rates were compared based on the measurement of optical density (OD) and weighing of the centrifuged bacterial cells (4000 rpm, 10 min, Centrifuge 5810 R, Eppendorf, Hamburg, Germany) after removing the supernatant of the bacterial cultures of S. olivaceus. Additionally, a metabolomic comparison of S. olivaceus JB1 from saline and non-saline media was performed after analyzing secondary metabolites of the bacterial strain JB1 with LC/Q-TOF-MS. The analyses were performed with a 1290 Infinity binary pump and YMC-triart C18 column (150 × 2.0 mm, 1.9 μm; YMC Korea Co., Seongnam, South Korea). The LC/MS conditions were as follows: 0-20 min, 10-100% B, 20-23 min, 100% B, 23-24 min, 100-10% B, 24-30 min, 10% B, 30-35 min with an injection volume of 20 μL and a flow rate of 0.4 mL/min. Eluent A of the HPLC system was acetonitrile acidified with 0.1% formic acid, and eluent B was H2O acidified with 0.1% formic acid. Mass spectrometry was performed on an Agilent 6530 Q-TOF-MS (Agilent Technologies, Santa Clara, CA, United States) with an electrospray ion (ESI) source.
Analyses of Secondary Metabolites From S. olivaceus JB1
The modified K Medium was used for HRMS2-based GNPS (Global Natural Product Social Molecular Networking) analysis of ethyl acetate crude extracts obtained from 1-week-old cultures of S. olivaceus JB1. Based on the analyzed tandem mass (MS2) data from an Agilent 6530 Q-TOF-MS, the molecular networks of the secondary metabolites were created by using the GNPS platform1. The data was converted to the .mzML format with MS-Convert. The converted files were used to generate an MS/MS molecular network using the GNPS web-server. The precursor ion mass tolerance was set to 2.0 Da and the product ion tolerance was set to 0.05 Da. The molecular networks were generated using a minimum of 6 matched peaks, a cosine score of 0.7 and a minimum cluster size of 2-3. After analysis, data were visualized using Cytoscape 3.8.2 software (Shannon et al., 2003). A literature investigation on chemical structures of lobophorin analogs and their biological function was conducted based on SciFinder-n and AntiBase comparing with the acquired combinational data of MS, UV, and elution times on LC/MS of individual lobophorin analogs.
Identification of Lobophorins A and G From S. olivaceus JB1
A 10 mL aliquot of Streptomyces olivaceus JB1 preculture was transferred to a 250 mL Erlenmeyer flask containing 100 mL of modified K medium. With continuous shaking (28°C, 180 rpm, 7 days), 10 mL of the liquid culture was inoculated in a 2 L Erlenmeyer flask containing 1.2 L of the medium (28°C, 180 rpm, 6 days, 48 L in total). Then, the culture was extracted by using ethyl acetate/water layer separation, and the ethyl acetate layer was concentrated in vacuo. The dried extract was loaded onto a prepacked SPE-C18 column (S*Pure, Singapore) and stepwise-eluted (20, 40, 60, 80, and 100% aqueous methanol). The lobophorin analogs were eluted with 80% aqueous methanol, and the fraction was further purified with semipreparative HPLC equipped with a 1260 Infinity binary pump and YMC-triart C18 column (150 × 10.0 mm, 5 μm; YMC Korea Co., Seongnam, South Korea) using the following conditions: gradient: 0–60 min, 10–90% B, 61–80 min, 90% B with an injection volume of 200 μL and a flow rate of 3 mL/min, UV detection at 204 and 268 nm. All eluents of the HPLC system were acidified with 0.1% formic acid. H2O was used as eluent A, while acetonitrile served as eluent B. NMR data of the separated single compounds, lobophorin A and G, were recorded on Bruker 700 and 800 MHz NMR spectrometers equipped with a Bruker CryoPlatform at the Korea Basic Science Institute in Ochang, South Korea. 1H NMR chemical shifts at δH 7.24, 3.31, and 2.50 (CDCl3, CD3OD, in a ratio of 8:3 and DMSO-d6) and 13C NMR chemical shifts at δC 77.2, 49.1, and 49.0 (CDCl3, CD3OD in a ratio of 8:3 and DMSO-d6) were set as reference peaks, and the chemical structures of lobophorins A and G were assigned by interpreting combinational data from correlation spectroscopy (COSY), heteronuclear single quantum coherence (HSQC), and heteronuclear multiple bond correlation (HMBC) experiments.
Lobophorin Detection in a Botanical Specimen
The collected fresh foliage of M. japonica was dried at 50°C for 24 h with a dehydrator (Shinil, Cheonan, South Korea). The dried leaves and leaf residues (6.0 g in total) were ground into a powder, and then the powder was extracted with 60 mL methanol at 40°C for 60 min with an ultrasound-assisted extraction method. The methanol extract was concentrated in vacuo and dissolved in methanol at a concentration of 250 μg/mL. Metabolite analysis was conducted with MS/Q-TOF-MS with the following conditions: gradient: 0–20 min, 10–100% B, 20–23 min, 100% B, 23–24 min, 100–10% B, 24–30 min, 10% B, with an injection volume of 10 μL and a flow rate of 0.4 mL/min. All eluents of the HPLC system were acidified with 0.1% formic acid. H2O was used as eluent A, while acetonitrile served as eluent B. Mass spectrometry was performed with an electrospray ion (ESI) source in positive mode. Extracted ion current (EIC) chromatograms (m/z [M + H]+ and m/z [M + Na]+) and fragmentation patterns were analyzed to compare and detect lobophorin analogs in both the methanol extract of M. japonica and the liquid culture broth of S. olivaceus JB1.
Paper Disk Diffusion Assay
To isolate the external contaminants of M. japonica, the fresh foliage was placed onto K solid medium in the absence of cycloheximide for 20 days, and then four gram-negative bacterial and one fungus strains were isolated. For the paper disk diffusion assay on agar plates against contaminants, K agar medium was prepared in 90-mm-diameter Petri dishes. A total of 500 μL of each bacterial culture [Bacillus megaterium (JB8), B. thuringiensis (JB9), L. adecarboxylata (JB10), and P. punonensis (JB11)] was inoculated onto a K agar plate, evenly spread with cell spreaders and then incubated at 28°C for 3 days. The colonies of the fungus strain [M. circinelloides (JB12)] were inoculated in the center of the K agar medium plate and incubated at 28°C for 7 days. Three 6-mm-diameter sterile paper disks were placed onto the surface of each Petri dish and then imbued with the fractions of cultures of the gram-positive bacterial strain S. olivaceus (JB1) (1 mg/mL, 20 μL). Ciprofloxacin (1 mg/mL, 20 μL) and amphotericin B (1 mg/mL, 20 μL) were used as positive controls for the antibacterial and antifungal assays, respectively. A total of 20 μL of DMSO was added to every Petri dish as a negative control.
Results
Isolation of Microbes Associated With M. japonica and DNA Sequencing
From the well-disinfected foliar residues of M. japonica, a total of seven putative endophytes were obtained, including one gram-positive bacterium (JB1), four gram-negative bacteria including Enterobacter ludwigii (JB2) (GenBank accession no. OM845779, 99%), Pseudomonas koreensis (JB3) (GenBank accession no. OM845778, 99%), Pseudomonas aeruginosa (JB4) (GenBank accession no. OM845780, 99%), and Enterobacter cloacae (JB5) (GenBank accession no. OM838295, 99%), and two fungi [JB6 (GenBank accession no. OM845784, 99%) and JB7 (GenBank accession no. OM845783, 99%)]. Only the bacterial strain JB1 showed a typical Streptomyces morphological feature, namely, the formation of mycelium covered with white spores (Figure 1). The bacterial strain JB2-5 displayed typical gram-negative bacterial characteristics. The aligned 16 rRNA dataset and phylogenetic analyses identified one Actinobacterium Streptomyces sp. (Figure 2). Four gram-negative bacteria were identified as common bacterial endophytes, including Enterobacter ludwigii (JB2) known as tomato growth-promoting endophyte (Bendaha and Belaouni, 2020), and Pseudomonas koreensis (JB3) studied as tobacco plants endophyte reducing bacterial wilt disease (Shang et al., 2021). Also, Pseudomonas aeruginosa (JB4) and Enterobacter cloacae (JB5) were known as Achyranthes aspera growth-stimulating endophyte (Devi et al., 2017), and banana plant growth supporting endophyte (Macedo-Raygoza et al., 2019), respectively. Two fungi, JB6-7 (identical species), were identified Colletotrichum musae, endophytic fungi from wild banana (Nuangmek et al., 2001; Bendaha and Belaouni, 2020; Shang et al., 2021).
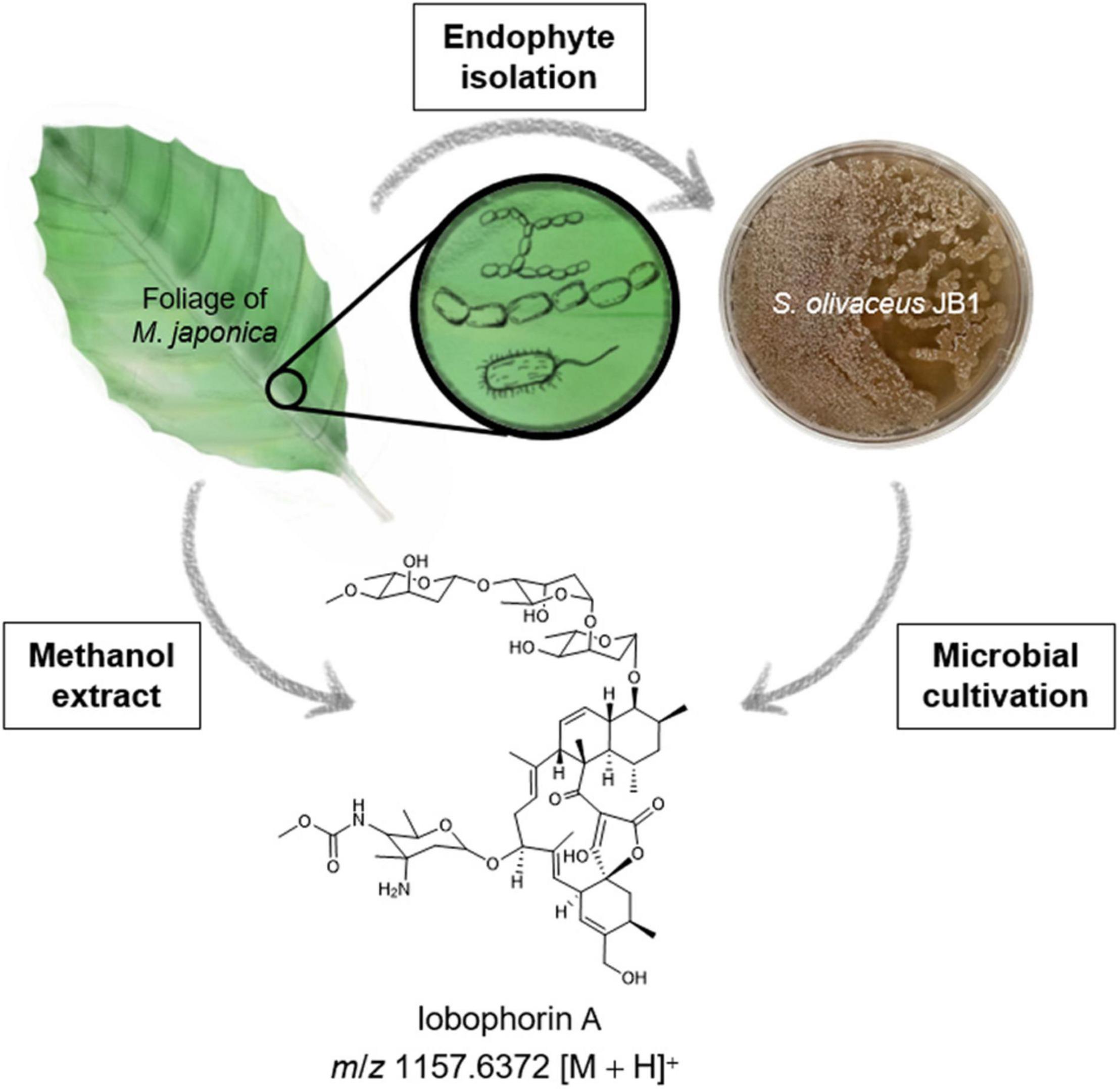
Figure 1. Schematic overview of isolation of endophytes associated with M. japonica and lobophorin detection from the plant material and the endophytic bacterium S. olivaceus JB1. The bacterial strain S. olivaceus JB1 was isolated from the sterilized lateral vein of M. japonica, S. olivaceus JB1 was cultured in modified K liquid medium for 6 days, and liquid culture broth was extracted by using ethyl acetate/water layer separation. The organic phase was concentrated in vacuo. The foliage and petioles of M. japonica were subjected to extraction with methanol. The extracts were analyzed using Q-TOF-MS, and notably, lobophorin A (m/z 1157.6372 [M + H]+) was detected from both the S. olivaceus JB1 extract and M. japonica.
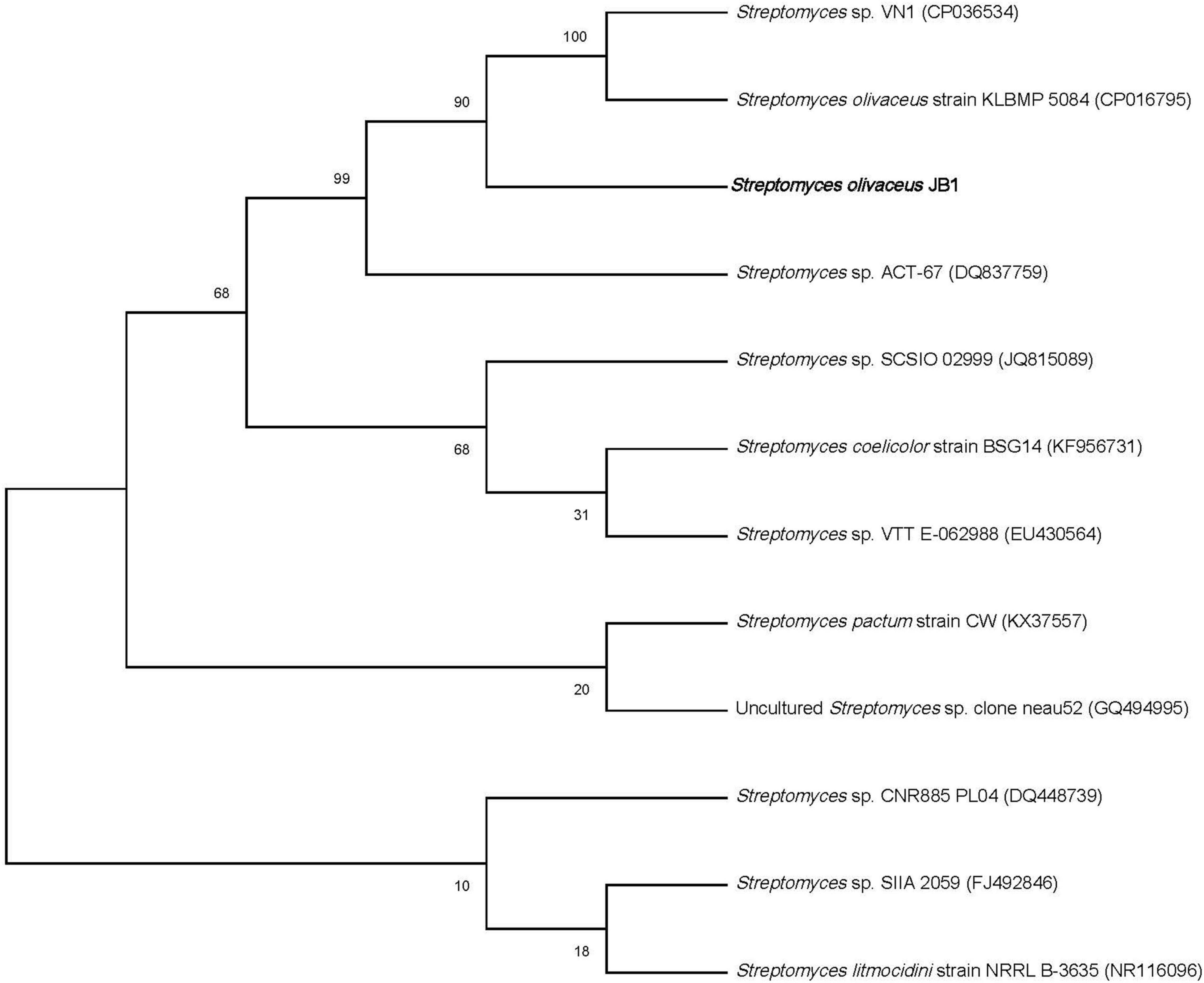
Figure 2. Maximum-likelihood phylogenetic tree showing the phylogenetic relationship of S. olivaceus JB1 and other closely related species based on 16S rRNA gene sequences.
Additionally, the external microbes from the surface of M. japonica were isolated without the addition of antibiotics. The isolates were four gram-negative bacterial strains, Bacillus megaterium (JB8) (GenBank accession no. OM838304, 99%), Bacillus thuringiensis (JB9) (GenBank accession no. OM838312, 99%), Leclercia adecarboxylata (JB10) (GenBank accession no. OM838305, 99%), and Pseudomonas punonensis (JB11) (GenBank accession no. OM838294, 99%), and one fungus strain Mucor circinelloides (JB12) (GenBank accession no. OM845785, 99%).
Metabolomics Study of S. olivaceus JB1
After cultivating the endophytes (JB1–7) associated with M. japonica in the modified K liquid and solid media to maximize the diversity of metabolites, the organic phase of the ethyl acetate/water layer separation was analyzed with the LC/MS system. The metabolite production from the isolates did not demonstrate prominent differentiation between either solid or liquid cultures. The bacterial strain JB1 showed broad mass peaks between m/z 883.5−1219.6 (Figure 3A and Supplementary Figure 13), displaying identical UV spectra (λmax at 204, 268). A further literature study for the metabolites was performed with SciFinder (Schwall and Zielenbach, 2000) and AntiBase (Laatsch, 2017) and revealed that the major metabolites were lobophorin analogs belonging to a group of spirotetronate antibiotics. In accordance with the LC/MS chemical profiling, we annotated thirteen lobophorin analogs (1−13, Table 1 and Supplementary Figure 13). As we initially intended to investigate the antimicrobial secondary metabolites produced by the endophytes of M. japonica against external strains of known phytopathogen strains to verify this defensive symbiotic system, the predominant metabolites from S. olivaceus JB1, lobophorin A and G (Figure 3C, Table 1 and Supplementary Figure 13), were representatively isolated as single compounds with HPLC, and the chemical structures of 2 and 6 were confirmed based on NMR analysis (Supplementary Figures 2−11). HRMS2-based analysis of the ethyl acetate extract of S. olivaceus JB1 clustered structurally related molecules with GNPS, and the results were visualized by Cytoscape 3.9.0 (Figure 3B). Eight lobophorin analogs were clustered (2, 3, 5, 16, 18, 19, 20, and 24), and the cluster represents these analogs have similar MS2 fragments. Among them, three nodes were reported for the lobophorin analogs lobophorin L (2), M (3), and E (5). Five nodes were unreported for the lobophorin analogs 16 (m/z 937.6 [M + H]+), 18 (m/z 997.6 [M + H]+), 19 (m/z 1011.6 [M + H]+), 20 (m/z 1141.7 [M + H]+), and 24 (m/z 951.6 [M + H]+).
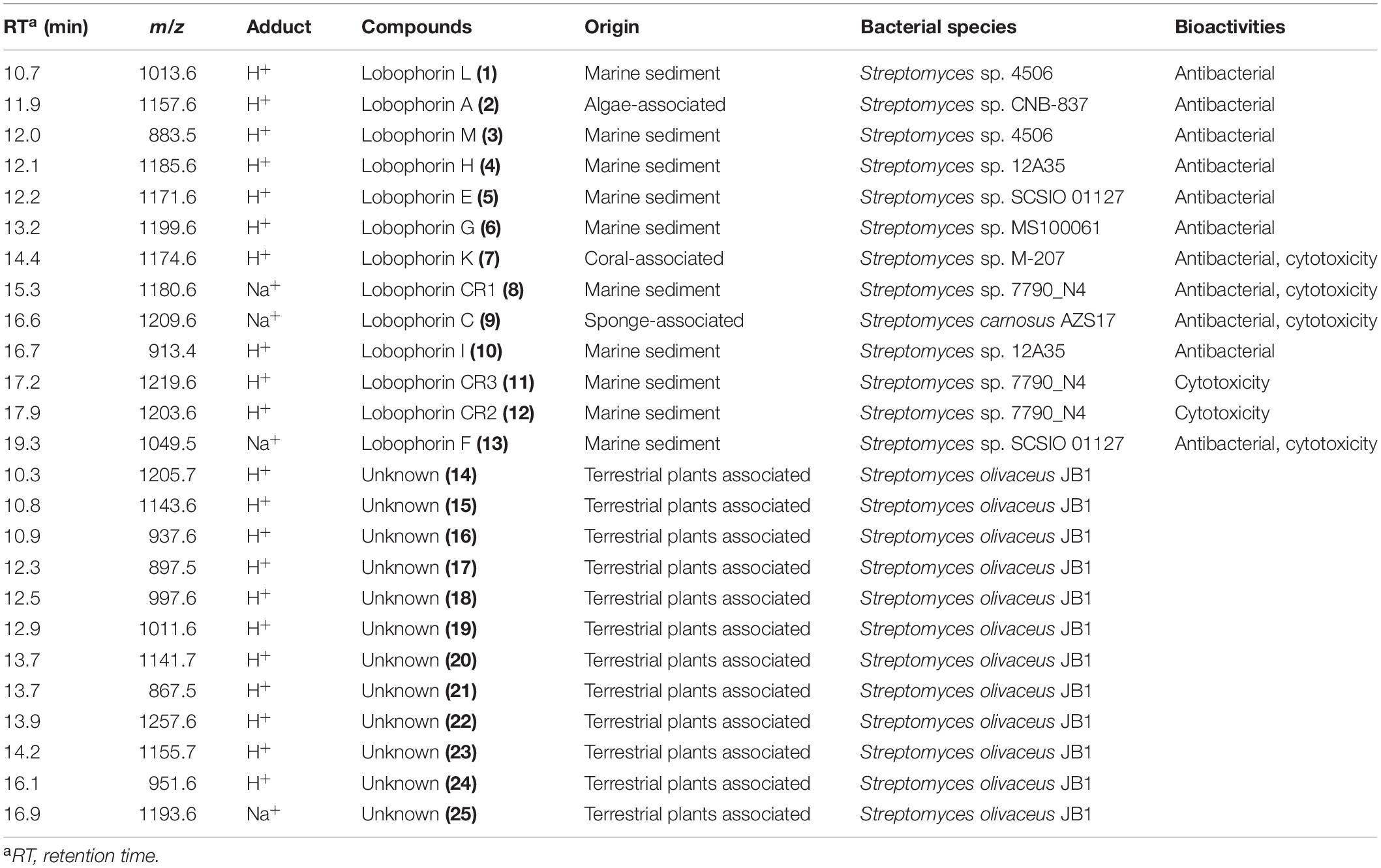
Table 1. List of previously isolated lobophorins that detected from S. olivaceus JB1 and unknown compounds of S. olivaceus JB1.
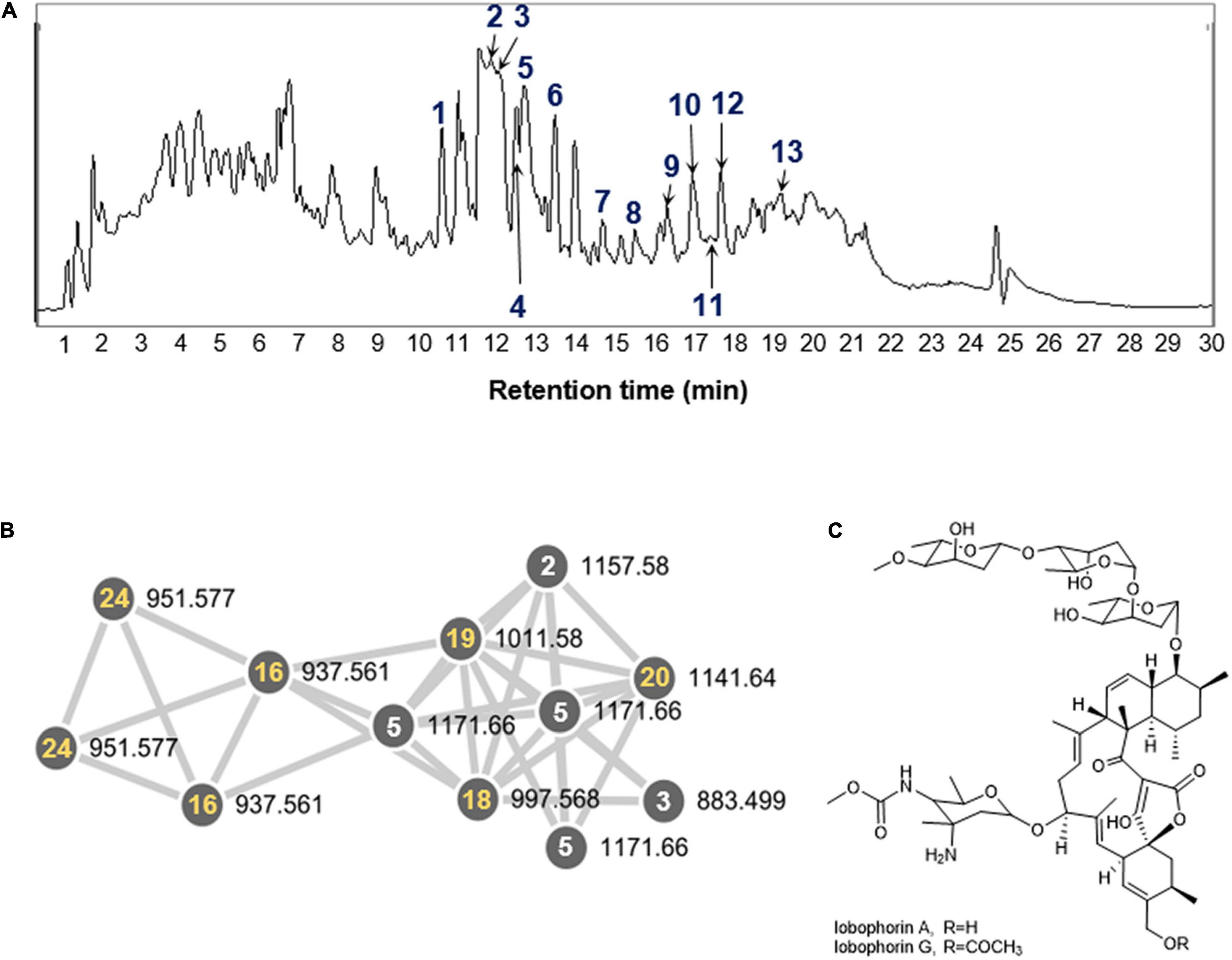
Figure 3. (A) LC-MS chemical analysis of lobophorin analogs in the extract of S. olivaceus JB1 and 25 annotated compounds. Thirteen compounds (1–13, blue) indicate previously reported lobophorin analogs. (B) A GNPS cluster assigned to lobophorin analogs from the organic phase of the S. olivaceus JB1 liquid culture. Five specific compounds (16, 18, 19, 20, and 24, yellow) correspond to unreported lobophorin analogs that clustered with reported lobophorin analogs in GNPS (selected). (C) The chemical structures of lobophorin A and G (2 and 6).
Impact of Saline Water Stress Tolerance on S. olivaceus JB1
Interestingly, lobophorin-producing Streptomyces spp. have been isolated from marine environments such as marine sediment, algae−associated, coral−associated, and sponge−associated environments, whereas S. olivaceus JB1 was obtained from a terrestrial environment associated with M. japonica (Table 1). The growth and metabolite production of S. olivaceus JB1 were investigated by cultivating the strain in saline bacterial growth medium with 33 per mille of sodium chloride as a stressor to reveal whether S. olivaceus JB1 originated from a marine environment. JB1 was cultivated in saline media and non-saline media separately. Except for the differences in the salinity of the growth media, the cultivation conditions, such as the inoculum concentration and incubation temperature, were identical. JB1 grew more in the non-saline medium than in the saline medium (cell wet biomass in a ratio of 2.7:1.6 from 50 mL of the liquid cultures, 4 days, 180 rpm, centrifuged at 4,000 rpm, 10 min). Additionally, a comparison of the lobophorin A production rate in non-saline and saline media revealed that S. olivaceus JB1 produces 3.5 times more lobophorin A in non-saline medium. Unlike other lobophorin-producing Streptomyces spp., S. olivaceus JB1 showed differentiation in saline media, not accelerating its growth and secondary metabolite production (Figure 4 and Supplementary Figure 1).
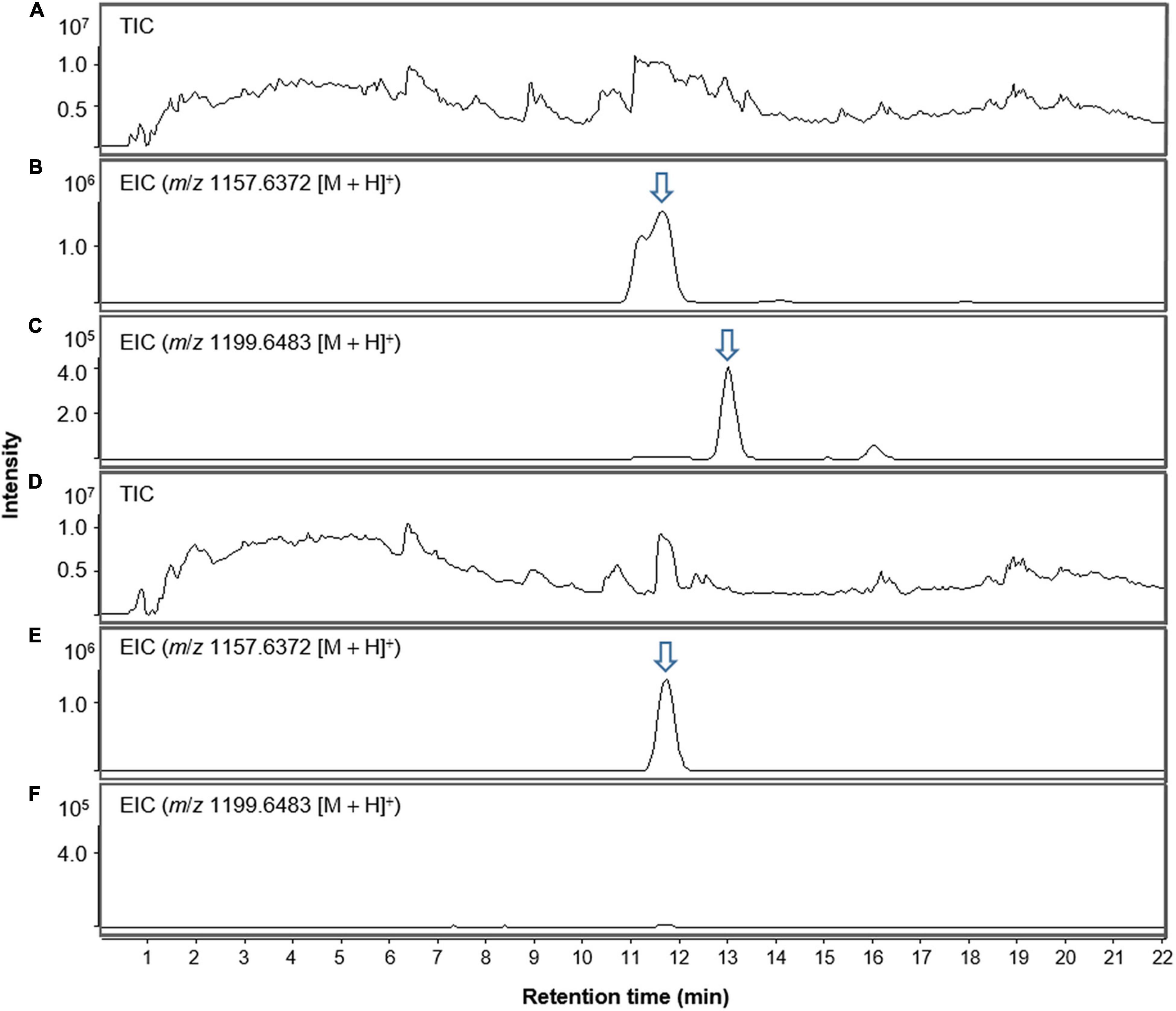
Figure 4. LC-MS chromatogram comparison of S. olivaceus JB1 culture extract in non-saline and saline media (salinity; 33 per mille of sodium chloride) in positive ion mode. (A) Total ion current (TIC) chromatogram of non-saline liquid culture broth of S. olivaceus JB1. (B) Extracted-ion chromatogram (EIC) of the non-saline liquid broth of S. olivaceus JB1 (lobophorin A, m/z 1157.6372 [M + H]+). (C) Extracted-ion chromatogram (EIC) of the non-saline liquid broth of S. olivaceus JB1 (lobophorin G, m/z 1199.6438 [M + H]+). (D) Total ion current (TIC) chromatogram of saline liquid culture broth of S. olivaceus JB1. (E) Extracted-ion chromatogram (EIC) of the saline liquid broth of S. olivaceus JB1 (lobophorin A, m/z 1157.6372 [M + H]+). (F) Extracted-ion chromatogram (EIC) of the saline liquid broth of S. olivaceus JB1 (lobophorin G, m/z 1199.6438 [M + H]+, not detected). The arrows indicate exact elution times of the extracted ions.
Streptomyces olivaceus resides in certain plants as an endophytic bacterium in territorial environments. However, production of lobophorin analogs by endophytic S. olivaceus in a territorial environment has not been previously reported. Thus, the bacterial strain JB1 is the first example of a bacterium producing lobophorin analogs in a non-saline habitat.
Lobophorin Detection in a Botanical Specimen
The methanol extract of the dried foliar residues of M. japonica was analyzed with LC/MS chemical profiling. The results clearly showed that lobophorin A (m/z 1157.6372 [M + H]+) was detected at the identical retention time observed in the S. olivaceus JB1 culture broth extract (Figures 5A–D). Additionally, the MS fragment patterns of the metabolite (m/z 590.3 [M + H]+, 753.4 [M + H]+, and 883.5 [M + H]+) from two different extracts support the existence of lobophorin A in leaves of M. japonica (Figures 5E,F). However, the mass signal intensities were too low to detect the other lobophorin analogs except lobophorin A and G unreported analogs m/z 997.6 [M + H]+ (18), and 1141.7 [M + H]+ (20). In practice, the bacterial cultivation experiment demonstrated a predominant production of lobophorin A from S. olivaceus JB1 compared to the lobophorin analogs. We also assume that the actual production and accumulation of lobophorin analogs from the endophytic S. olivaceus JB1 in M. japonica is limited in planta.
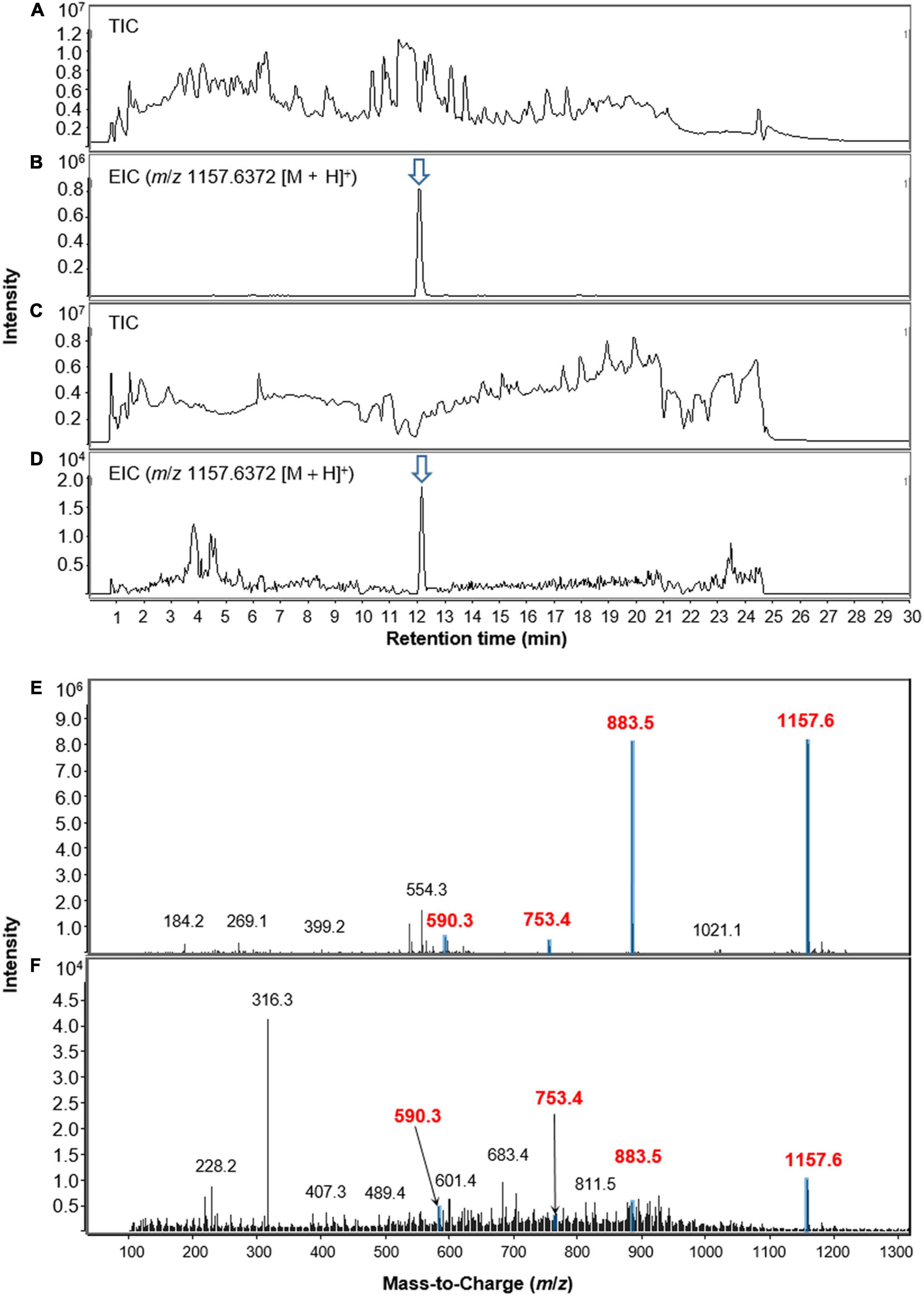
Figure 5. LC-MS chromatograms of lobophorin analogs obtained from extracts of S. olivaceus JB1 in broth medium and the foliage and petioles of M. japonica in positive ion mode. (A) Total ion current (TIC) chromatogram of liquid culture broth of S. olivaceus JB1. (B) Extracted-ion chromatogram (EIC) of the liquid broth of S. olivaceus JB1 (lobophorin A, m/z 1157.6372 [M + H]+). (C) Total ion current (TIC) chromatogram of the methanol extract of the dried leaf material of M. japonica. (D) Extracted-ion chromatogram (EIC) of methanol extract of M. japonica leaves (lobophorin A, m/z 1157.6372 [M + H]+). (E) MS fragmentation patterns of lobophorin A detected from the liquid culture of S. olivaceus JB1 and (F) methanol extract from the dried leaf material of M. japonica. The arrows indicate exact elution times of the extracted ions.
Paper Disk Diffusion Assay
The antibacterial activities of lobophorin analogs were previously reported (Niu et al., 2011; Wei et al., 2011; Pan et al., 2013), whereas the analogs did not show antifungal activity. In this study, in the LC/MS system, lobophorin A and G (2 and 6) were detected from the botanical sample of M. japonica, and the compounds were reported to show antibacterial activities in previous studies. We conducted antimicrobial tests with the 80% aqueous methanol fraction containing 2 and 6 from the S. olivaceus JB1 liquid culture against the four external strains of known phytopathogen strains of M. japonica (Figure 6). The antibacterial activities of each methanol fraction of the liquid culture were evaluated with diametrical measurement of the inhibition zone. The assay demonstrated that the 80% fraction showed moderate antibacterial activities by suppressing the proliferation of two bacterial contaminants (Bacillus megaterium JB8 and Bacillus thuringiensis JB9). The diameters of the growth inhibition zones of JB8 and JB9 affected by the 80% methanol fraction were 0.9 and 1.5 cm, respectively (1.8 and 1.9 cm for ciprofloxacin, a positive control), while the fraction did not show antibacterial activity against JB10 (Leclercia adecarboxylata) and JB11 (Pseudomonas punonensis). An antifungal activity test was performed on agar media containing the fungal inoculum of JB12 (Mucor circinelloides), the fungus isolated from the external surface of M. japonica, with an 80% fraction; however, no antifungal activity was observed. The results demonstrated that the 80% fraction containing lobophorin A and G only showed antibacterial activities against gram-positive Bacillus strains.
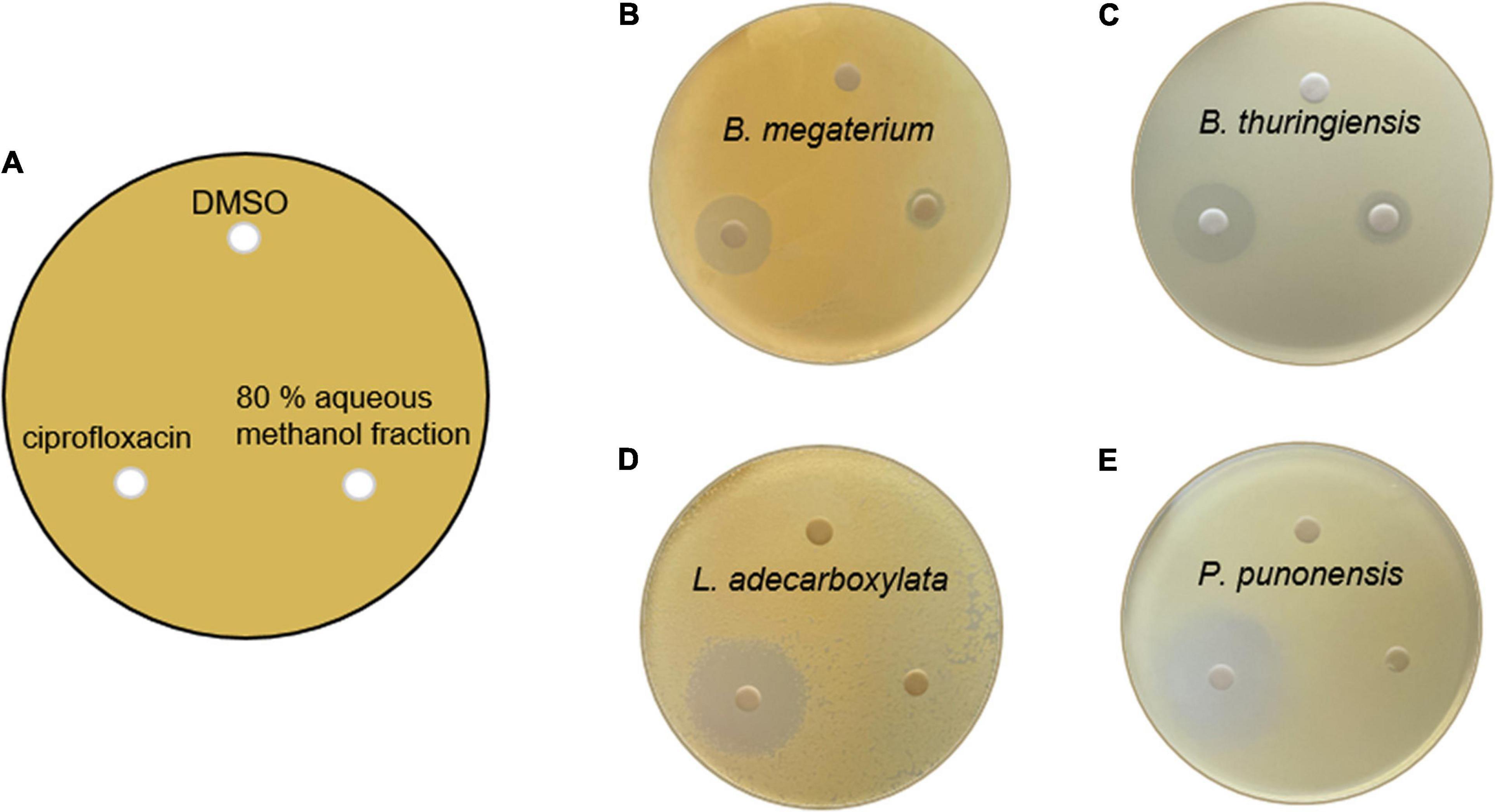
Figure 6. (A) Petri plate antibacterial activity assay setup, showing that 500 μL of the four external bacterial contaminants from the leaves of M. japonica was inoculated and spread on a K agar plate. The placement of lobophorin A and G-containing 80% methanol fraction of S. olivaceus JB1 extract dissolved in DMSO (1 mg/mL, 20 μL), the negative control (DMSO only, 20 μL), and the positive control ciprofloxacin dissolved in DMSO (1 mg/mL, 20 μL) 3 days after inoculation. (B) Antimicrobial activity against Bacillus megaterium JB8; the diameter of growth inhibition of ciprofloxacin was 1.8 cm, and the fraction was 0.9 cm. (C) Bacillus thuringiensis JB9; the diameter of growth inhibition of ciprofloxacin was 1.9 cm, and the fraction was 1.5 cm. (D) Leclercia adecarboxylata JB10; the diameter of growth inhibition of ciprofloxacin was 2.5 cm, and the fraction did not show antibacterial activity against L. adecarboxylata. (E) Pseudomonas punonensis JB11; the diameter of growth inhibition of ciprofloxacin was 3.0 cm, and the fraction did not show antibacterial activity against P. punonensis.
Discussion
Endophytes utilize the plant’s endosphere as a unique niche to protect themselves from drastic changes in the external environment (Schindler et al., 2021). Recently, medicinal plants have been reported to be influenced by their interaction with beneficial endophytes (Lindow and Brandl, 2003; Chaudhry et al., 2021). Leaf nodules harboring microbial endophytes in special glandular structures have only recently gained increased interest as examples of plant-microbe interactions in the phyllosphere (Schindler et al., 2021). Detection of the secondary metabolites of symbiotic microorganisms or endophytes have often been reported. For example, the antifungal Streptomyces spp. associated with Protea infructescences produce fungichromin and actiphenol, and the compounds were detected in planta with LC/MS (Human et al., 2016). Antitumor astins were found, with molecular techniques, to originate from the fungal endophyte Cyanodermella asteris living within the medicinal plant Aster tataricus (Schafhauser et al., 2019).
In this study, we demonstrate the first discovery of seven endophytes of Maesa japonica (Thunb.) Moritzi & Zoll. and the presence of lobophorin analogs in the leaf and leaf residues produced by the endophytic bacterium Streptomyces olivaceus JB1. At the outset, we postulated that certain isolates may play a pivotal role in defending their host, M. japonica, against foreign phytopathogens in planta, with minimal risk of the plant succumbing to disease. We then isolated seven putative endophytes from M. japonica – one gram-positive bacterium (S. olivaceus JB1), four gram-negative bacteria (E. ludwigii JB2, P. koreensis JB3, P. aeruginosa JB3, and E. cloacae JB5) and two fungi (C. musae JB6 and JB7) – and five external strains of known phytopathogen strains, including B. megaterium, B. thuringiensis, L. adecarboxylata, P. punonensis, and M. circinelloides, from the phylloplane to verify the defensive biological system.
Large-scale cultivation of S. olivaceus JB1 and diverse analyses with LC/MS and NMR data led us to identify the predominant secondary metabolites in the fraction as lobophorin analogs. To track the possible accumulated secondary metabolites produced by the endophytes in M. japonica, the foliar residues were extracted with methanol, and the extract was analyzed with LC/MS. Interestingly, the lobophorin analogs, mainly lobophorin A and G (2 and 6), were detected in the plant material extracts from which the bacterial strain S. olivaceus JB1 was originally isolated. The detection was confirmed and supported by performing M. japonica extraction in duplicate, demonstrating the existence of the bacterial strain S. olivaceus JB1 residing in a leaf microhabitat of M. japonica as its endophyte and verifying lobophorin analogs accumulation in situ.
Among the fractions of the isolate cultures, the 80% aqueous methanol fraction of the bacterial strain JB1 culture displayed moderate antimicrobial activities against two gram-positive bacteria, JB8 and JB9 (B. megaterium and B. thuringiensis). Lobophorin analogs exhibited strong to moderate antibacterial activities against M. luteus, M. tuberculosis and Bacillus sp. and no activity against Pseudomonas sp. which matched antibacterial activity test results (Niu et al., 2011; Luo et al., 2021).
Lobophorins A and B were initially isolated from Actinomyces sp. CNB-837 associated with the Caribbean brown alga Lobophora variegata (Jiang et al., 1999). Lobophorin analogs belonging to a group of spirotetronate are marine natural products with diverse biological activities. Lobophorin analogs such as L (1), A (2), M (3), H (4), E (5), G (6), K (7), CR1 (8), C (9), I (10), and F (13) showed antimicrobial activities. For example, lobophorins A, B, E, and F displayed antimicrobial activity against Bacillus thuringiensis SCSIO BT01 (Niu et al., 2011), Lobophorin H showed antibacterial activity against Bacillus subtilis (Pan et al., 2013). Lobophorin F showed antibacterial activity against Staphylococcus aureus ATCC 29213, Enterococcus faecalis ATCC 29212 and Bacillus thuringiensis SCSIO BT01. Lobophorin K promoted bacteriostatic effects against Staphylococcus aureus EPI1167 MSSA. Lobophorins B and F exhibited cytotoxic activity against MCF-7 cells (human breast adenocarcinoma cell line), NCI-H460 (human non-small cell lung cancer cell line), and SF-268 (human glioma cell line) (Niu et al., 2011). Also, lobophorin C represented potent cytotoxic activity against human liver cancer cells, and lobophorin D displayed a significant inhibitory effect on human breast cancer cells (Wei et al., 2011). The case of lobophorin K, the compound represented cytotoxic activity against tumor cell lines such as MIA Paca-2 (pancreatic carcinoma) and MCF-7 (breast adenocarcinoma) (Braña et al., 2017). Lobophorin CR1, CR2, and CR3 showed human oral cancer cell growth inhibition (Cruz et al., 2015). Additionally, potent anti-inflammatory activities of lobophorins A and B were reported (Jiang et al., 1999).
It is currently widely known that lobophorin analogs, spirotetronate polyketides, are produced by marine-associated Streptomyces spp. Recently, Rodríguez-Hernández et al. (2019) isolated lobophorin-producing Streptomyces sp. from nurse and foraging bees. Here we demonstrate that Streptomyces olivaceus JB1 isolated from a terrestrial environment associated with M. japonica produces lobophorin analogs in planta. Environmental transitions of bacteria across the freshwater–marine boundary with a dramatic shift in salinity rarely occur during bacterial evolution (Gophna, 2013). Even though the evolutionary history of the bacterial strain JB1 is currently unknown, we assume that the role of ecological interactions between M. japonica and S. olivaceus JB1 in the emergence of the marine and territorial boundary likely diversifies among taxa because of bacterial lifestyle strategies. The results of our salinity manipulation experiment with S. olivaceus JB1 suggested that the bacterial strain JB1 grows faster and relatively produces more lobophorin analogs in non-saline water. Our LC/MS study indicates that the S. olivaceus JB1 produces not only known lobophorin analogs produced by marine bacteria but also unreported lobophorin analogs (14–25) (Supplementary Figure 13). Further lobophorin analog studies are needed with mass bacterial cultivation and chemical diversity analysis based on the backbone of the lobophorin spirotetronate. Furthermore, the biological activity of 12 unreported lobophorin analogs detected only by LC/MS and the ecological functions of the compounds associated with M. japonica will be examined in the future.
Data Availability Statement
The datasets presented in this study can be found in online repositories. The names of the repository/repositories and accession number(s) can be found below: NCBI – OM845781, OM845779, OM845778, OM845780, OM838295, OM845784, OM845783, OM838304, OM838312, OM838305, OM838294, and OM845785.
Author Contributions
SU and SK conceptualized the study. SU collected the plant material, designed the chemical and biological analyses, and identified lobophorin analogs by NMR spectroscopic data. JL did bacterial isolations and fermentations, purified compounds, and performed the biological activity tests. SU, JL, and SK wrote and edited the manuscript. All authors contributed to the article and approved the submitted version.
Funding
We acknowledge financial support from the National Research Foundation of Korea (NRF-2018R1A6A1A03023718 and NRF-2020R1A2C1012632), funded by the Ministry of Education, Science and Technology, South Korea.
Conflict of Interest
The authors declare that the research was conducted in the absence of any commercial or financial relationships that could be construed as a potential conflict of interest.
Publisher’s Note
All claims expressed in this article are solely those of the authors and do not necessarily represent those of their affiliated organizations, or those of the publisher, the editors and the reviewers. Any product that may be evaluated in this article, or claim that may be made by its manufacturer, is not guaranteed or endorsed by the publisher.
Acknowledgments
We would like to thank Dae Yang Park of Korea Medicinal Plant Farming Corporation for collecting plant samples in Jeju.
Supplementary Material
The Supplementary Material for this article can be found online at: https://www.frontiersin.org/articles/10.3389/fmicb.2022.881253/full#supplementary-material
Abbreviations
LC/Q-TOF-MS, liquid chromatography-quadrupole-time of flight mass spectrometer; HPLC, high-performance liquid chromatography; GNPS, Global Natural Product Social Molecular Networking; TIC, total ion chromatogram; EIC, extracted ion current chromatogram; NMR, nuclear magnetic resonance; COSY, correlation spectroscopy; HSQC, heteronuclear single quantum coherence; HMBC, heteronuclear multiple bond correlation.
Footnotes
References
Aranda-Ocampo, S., Montes-Borrego, M., Jiménez-Dıìaz, R., and Landa, B. (2011). Microbial communities associated with the root system of wild olives (Olea europaea L. subsp. europaea var. sylvestris) are good reservoirs of bacteria with antagonistic potential against Verticillium dahliae. Plant Soil 343, 329–345. doi: 10.1007/s11104-011-0721-2
Bendaha, M. E. A., and Belaouni, H. A. (2020). Effect of the endophytic plant growth promoting EB4B on tomato growth. Hell. Plant Prot. J. 13, 54–65. doi: 10.2478/hppj-2020-0006
Braña, A. F., Sarmiento-Vizcaíno, A., Osset, M., Pérez-Victoria, I., Martín, J., de Pedro, N., et al. (2017). Lobophorin K, a new natural product with cytotoxic activity produced by Streptomyces sp. M-207 associated with the deep-sea coral Lophelia pertusa. Mar. Drugs 15:144. doi: 10.3390/md15050144
Chaudhry, V., Runge, P., Sengupta, P., Doehlemann, G., Parker, J. E., and Kemen, E. (2021). Shaping the leaf microbiota: plant-microbe-microbe interactions. J. Exp. Bot. 72, 36–56. doi: 10.1093/jxb/eraa417
Chen, C., Wang, J., Guo, H., Hou, W., Yang, N., Ren, B., et al. (2013). Three antimycobacterial metabolites identified from a marine-derived Streptomyces sp MS100061. Appl. Microbiol. Biotechnol. 97, 3885–3892. doi: 10.1007/s00253-012-4681-0
Cruz, P. G., Fribley, A. M., Miller, J. R., Larsen, M. J., Schultz, P. J., Jacob, R. T., et al. (2015). Novel lobophorins inhibit oral cancer cell growth and induce Atf4- and chop-dependent cell death in murine fibroblasts. ACS Med. Chem. Lett. 6, 877–881. doi: 10.1021/acsmedchemlett.5b00127
Devi, K. A., Pandey, G., Rawat, A., Sharma, G. D., and Pandey, P. (2017). The endophytic symbiont—Pseudomonas aeruginosa stimulates the antioxidant activity and growth of Achyranthes aspera L. Front. Microbiol. 8:1897. doi: 10.3389/fmicb.2017.01897
Human, Z. R., Moon, K., Bae, M., de Beer, Z. W., Cha, S., Wingfield, M. J., et al. (2016). Antifungal Streptomyces spp. associated with the infructescences of Protea spp. in South Africa. Front. Microbiol. 7:1657. doi: 10.3389/fmicb.2016.01657
Jackson, C. R., and Denney, W. C. (2011). Annual and seasonal variation in the phyllosphere bacterial community associated with leaves of the southern Magnolia (Magnolia grandiflora). Microb. Ecol. 61, 113–122. doi: 10.1007/s00248-010-9742-2
Jiang, Z. D., Jensen, P. R., and Fenical, W. (1999). Lobophorins A and B, new antiinflammatory macrolides produced by a tropical marine bacterium. Bioorg. Med. Chem. Lett. 9, 2003–2006. doi: 10.1016/s0960-894x(99)00337-6
Jin, E., Li, H., Liu, Z., Xiao, F., and Li, W. (2021). Antibiotic dixiamycins from a cold-seep-derived Streptomyces olivaceus. J. Nat. Prod. 84, 2606–2611. doi: 10.1021/acs.jnatprod.1c00411
Kim, J. S. (2014). Antioxidant, α-glucosidase inhibitory and antimicrobial activities of extracts from Maesa japonica (Thunb.). Korean J. Med. Crop Sci. 22, 289–294.
Kwon, H., Shin, S.-L., Kim, Y.-R., and Kim, S.-Y. (2020). Effects of temperature, gibberellic acid, and KNO 3 treatments on seed germination of the endangered wild plant Maesa japonica. Seed Sci. Technol. 48, 65–72. doi: 10.15258/sst.2020.48.1.09
Kwon, K.-J., Kwon, H.-J., Oh, Y.-A., Kim, S.-Y., and Park, B.-J. (2021). Particulate matter removal of three woody plant species, Ardisia crenata, Ardisia japonica, and Maesa japonica. Sustainability 13:11017.
Lindow, S. E., and Brandl, M. T. (2003). Microbiology of the phyllosphere. Appl. Environ. Microbiol. 69, 1875–1883. doi: 10.1128/aem.69.4.1875-1883.2003
Liu, N., Shang, F., Xi, L., and Huang, Y. (2013). Tetroazolemycins A and B, two new oxazole-thiazole siderophores from deep-sea Streptomyces olivaceus FXJ8.012. Mar. Drugs 11, 1524–1533. doi: 10.3390/md11051524
Luo, M., Tang, L., Dong, Y., Huang, H., Deng, Z., and Sun, Y. (2021). Antibacterial natural products lobophorin L and M from the marine-derived Streptomyces sp. 4506. Nat. Prod. Res. 35, 5581–5587. doi: 10.1080/14786419.2020.1797730
Macedo-Raygoza, G. M., Valdez-Salas, B., Prado, F. M., Prieto, K. R., Yamaguchi, L. F., Kato, M. J., et al. (2019). Enterobacter cloacae, an endophyte that establishes a nutrient-transfer symbiosis with banana plants and protects against the black Sigatoka pathogen. Front. Microbiol. 10:804. doi: 10.3389/fmicb.2019.00804
Moon, M. O., Tho, J. H., Kim, C. H., Kim, C. S., and Kim, M. H. (2006). Unrecorded species from Korean flora: Maesa japonica (Thunb.) Moritzi & Zoll. [Maesaceae] and Cardamine tanakae Franch. & Sav. [Brassicaceae]. Korean J. Pl. Taxon 36, 153–161. doi: 10.11110/kjpt.2006.36.2.153
Müller, H., Berg, C., Landa, B. B., Auerbach, A., Moissl-Eichinger, C., and Berg, G. (2015). Plant genotype-specific archaeal and bacterial endophytes but similar Bacillus antagonists colonize Mediterranean olive trees. Front. Microbiol. 6:138. doi: 10.3389/fmicb.2015.00138
Nguyen, H. T., Pokhrel, A. R., Nguyen, C. T., Pham, V. T. T., Dhakal, D., Lim, H. N., et al. (2020). Streptomyces sp. VN1, a producer of diverse metabolites including non-natural furan-type anticancer compound. Sci. Rep. 10:1756. doi: 10.1038/s41598-020-58623-1
Niu, S., Li, S., Chen, Y., Tian, X., Zhang, H., Zhang, G., et al. (2011). Lobophorins E and F, new spirotetronate antibiotics from a South China Sea-derived Streptomyces sp. SCSIO 01127. J. Antibiot (Tokyo). 64, 711–716. doi: 10.1038/ja.2011.78
Nuangmek, W., Lumyong, S., Lumyong, P., and Hyde, K. (2001). Endophytic fungi of wild banana (Musa acuminata) at Doi Suthep Pui National Park, Thailand * Paper presented at the Asian Mycological Congress 2000 (AMC 2000), incorporating the 2nd Asia-Pacific Mycological Congress on Biodiversity and Biotechnology, and held at the University of Hong Kong on 9-13 July 2000. Mycol. Res. 105, 1508–1513. doi: 10.1017/S0953756201004968
Pan, H. Q., Zhang, S. Y., Wang, N., Li, Z. L., Hua, H. M., Hu, J. C., et al. (2013). New spirotetronate antibiotics, lobophorins H and I, from a South China Sea-derived Streptomyces sp. 12A35. Mar. Drugs 11, 3891–3901. doi: 10.3390/md11103891
Rodríguez-Hernández, D., Melo, W. G., Menegatti, C., Lourenzon, V. B., do Nascimento, F. S., and Pupo, M. T. (2019). Actinobacteria associated with stingless bees biosynthesize bioactive polyketides against bacterial pathogens. New J. Chem. 43, 10109–10117.
Schafhauser, T., Jahn, L., Kirchner, N., Kulik, A., Flor, L., Lang, A., et al. (2019). Antitumor astins originate from the fungal endophyte Cyanodermella asteris living within the medicinal plant Aster tataricus. Proc. Natl. Acad. Sci. U.S.A. 116, 26909–26917. doi: 10.1073/pnas.1910527116
Schindler, F., Fragner, L., Herpell, J. B., Berger, A., Brenner, M., Tischler, S., et al. (2021). Dissecting metabolism of leaf nodules in Ardisia crenata and Psychotria punctata. Front. Mol. Biosci. 8:683671. doi: 10.3389/fmolb.2021.683671
Schwall, K., and Zielenbach, K. (2000). SciFinder: a new generation of research tool. Chem. Innov. 30, 38–52.
Shang, X.-C., Cai, X., Zhou, Y., Zhang, C., Ilyas, N., Li, Y., et al. (2021). Pseudomonas inoculation Stimulates Endophytic Azospira population and induces systemic resistance to bacterial wilt. Front. Plant Sci. 12:738611. doi: 10.3389/fpls.2021.738611
Shannon, P., Markiel, A., Ozier, O., Baliga, N. S., Wang, J. T., Ramage, D., et al. (2003). Cytoscape: a software environment for integrated models of biomolecular interaction networks. Genome Res. 13, 2498–2504. doi: 10.1101/gr.1239303
Wei, R. B., Xi, T., Li, J., Wang, P., Li, F. C., Lin, Y. C., et al. (2011). Lobophorin C and D, new kijanimicin derivatives from a marine sponge-associated actinomycetal strain AZS17. Mar. Drugs 9, 359–368. doi: 10.3390/md9030359
Keywords: actinobacteria, Streptomyces, endophyte, symbiosis, lobophorin, antimicrobial
Citation: Um S, Lee J and Kim SH (2022) Lobophorin Producing Endophytic Streptomyces olivaceus JB1 Associated With Maesa japonica (Thunb.) Moritzi & Zoll.. Front. Microbiol. 13:881253. doi: 10.3389/fmicb.2022.881253
Received: 22 February 2022; Accepted: 11 April 2022;
Published: 29 April 2022.
Edited by:
Tofazzal Islam, Bangabandhu Sheikh Mujibur Rahman Agricultural University, BangladeshReviewed by:
Sahil Mehta, International Centre for Genetic Engineering and Biotechnology, IndiaBijayalaxmi Mohanty, National University of Singapore, Singapore
Eduardo L. Almeida, University College Cork, Ireland
Copyright © 2022 Um, Lee and Kim. This is an open-access article distributed under the terms of the Creative Commons Attribution License (CC BY). The use, distribution or reproduction in other forums is permitted, provided the original author(s) and the copyright owner(s) are credited and that the original publication in this journal is cited, in accordance with accepted academic practice. No use, distribution or reproduction is permitted which does not comply with these terms.
*Correspondence: Seung Hyun Kim, a2ltc2gxMUB5b25zZWkuYWMua3I=
†These authors have contributed equally to this work