- Department of Microbiology and Institute for Applied Life Sciences, University of Massachusetts, Amherst, MA, United States
Introduction
There is a debate whether Geobacter sulfurreducens produces electrically conductive pili (e-pili) from its pilin monomer, PilA, a protein encoded by gene GSU 1496. G. sulfurreducens assembly of the PilA into e-pili was proposed over a decade ago (Reguera et al., 2005). As detailed below, many subsequent studies have provided additional data consistent with this concept (Figure 1). However, Gu et al. have recently concluded that G. sulfurreducens does not express e-pili from PilA (Gu et al., 2021).
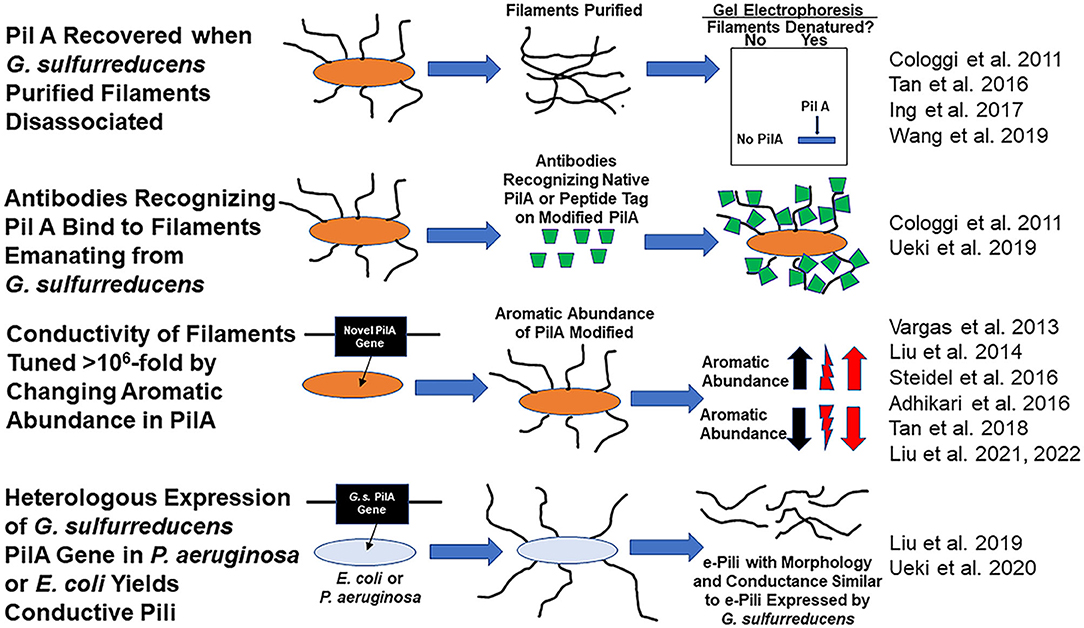
Figure 1. Evidence consistent with the hypothesis that Geobacter sulfurreducens expresses e-pili comprised of the pilin monomer, PilA and that PilA can be assembled into conductive filaments.
This is not a controversy over small details of the physiology of one microbe. Geobacter species play an important role in natural environments and biotechnologies. For example, Geobacter species are typically abundant in soils and sediments in which Fe(III) oxide reduction has a significant impact on the biogeochemical cycling of carbon, nutrients, and trace metals as well as in bioremediation (Lovley et al., 2011; Reguera and Kashefi, 2019; Lovley and Holmes, 2022). Geobacter species are also often abundant in soils and anaerobic digesters in which direct interspecies electron transfer (DIET) appears to be an important mechanism for methane production (Zhao et al., 2020; Lovley and Holmes, 2022). Geobacter and closely related species are often enriched on the anodes of electrodes harvesting electricity from organic matter and G. sulfurreducens generates the highest current densities of all known electroactive isolates (Lovley et al., 2011; Logan et al., 2019). Although other microbes, most notably Shewanella species, have been helpful for developing an understanding of key extracellular electron transfer mechanisms (Shi et al., 2016; Lovley and Holmes, 2022), there are no pure cultures that are as effective in Fe(III) oxide reduction, DIET, and current production as G. sulfurreducens and its close relative G. metallireducens.
Furthermore, if it were true that PilA cannot be assembled into conductive filaments, this would mean that attempts to develop new protein-based electronic materials based on concepts for electron transport along e-pili (Creasey et al., 2018; Dorval Courchesne et al., 2018; Gutermann and Gazit, 2018; Cosert et al., 2019; Roy et al., 2020) may be misguided. The reported heterologous expression of e-pili from PilA in Pseudomonas aeruginosa (Liu et al., 2019) or Escherichia coli (Ueki et al., 2020) for mass production of e-pili for the fabrication of electronics would require new, non-obvious explanations to describe how introducing G. sulfurreducens PilA confers the capacity for conductive filament expression. Other apparent accomplishments for electronics applications, also achieved simply by modifying the structure of PilA, such as tuning of the conductivity of G. sulfurreducens filaments or the introduction of novel binding sites on filaments to enhance sensor selectivity (Lovley and Yao, 2021), would also need reevaluation. The function of electronic devices for electricity generation (Liu et al., 2020b), sensing (Liu et al., 2020a; Smith et al., 2020), and neuromorphic memory (Fu et al., 2020, 2021) would need to be reconsidered.
The Claim That Wild-Type G. sulfurreducens Does Not Express Filaments Comprised of PilA
Gu et al. (2021) conclude that G. sulfurreducens does not assemble PilA into pili because “Purified filament preparations from wild-type cells grown under these nanowire-producing conditions did not show either PilA-N or PilA-C using immunoblotting” (in Gu et al. the term PilA-N refers to the PilA protein encoded by gene GSU 1496). Yet just 2 years earlier the same lab reported that “we confirmed the presence of both PilA and OmcS with expected molecular weights of ~6.5 kDa and ~45 kDa, respectively, in our filament preparations using poly-acrylamide gel electrophoresis (SDS-PAGE), peptide mass spectrometry, and western immunoblotting” (Wang et al., 2019). Furthermore, the senior author of Gu et al. had also reported the recovery of PilA and OmcS from G. sulfurreducens filament preparations in another publication (Tan et al., 2016). It is important to recognize that these prior findings from some of the same investigators directly refute the Gu et al. hypothesis that wild-type G. sulfurreducens does not express filaments comprised of PilA. As detailed in the next section, there is also additional abundant evidence that wild-type G. sulfurreducens displays conductive filaments comprised of PilA.
Gu et al. did recover PilA-containing filaments from a mutant strain in which the gene for the outer-surface cytochrome OmcS was deleted (Gu et al., 2021). However, these filaments also contained another protein, and the filaments were poorly conductive. Gu et al. acknowledged that these hybrid filaments, which had a diameter of 6.5. nm, were an artifact produced only in the mutant strain; they were not expressed in wild-type G. sulfurreducens. As detailed below, no other study of G. sulfurreducens has observed 6.5 nm filaments emanating from G. sulfurreducens or in purified filament preparations. Such filaments were not even observed in other omcS-deletion mutants of G. sulfurreducens (Leang et al., 2010; Liu et al., 2022). Thus, the 6.5 nm PilA-containing filaments that Gu et al. report are an artifact, not replicated in other studies, and clearly have no relevance to the filament expression of wild-type G. sulfurreducens.
The Evidence for e-pili Comprised of PilA
Many studies have provided substantial evidence that wild-type G. sulfurreducens expresses filaments comprised of PilA (Figure 1). For example, the Reguera lab eloquently demonstrated that: (1) the PilA pilin monomer was the only protein recovered from purified G. sulfurreducens filaments sheared from cells and (2) that intact filaments harvested from the cells reacted with a PilA-specific antibody (Cologgi et al., 2011). Several other laboratories subsequently demonstrated that PilA was a major protein in filaments recovered from G. sulfurreducens (Tan et al., 2016; Ing et al., 2017).
G. sulfurreducens PilA is assembled into conductive filaments, not only in G. sulfurreducens, but also in other microbes. Expression of the G. sulfurreducens PilA pilin monomer gene in P. aeruginosa (Liu et al., 2019) or E. coli (Ueki et al., 2020) yielded filaments with the same morphology and conductance as G. sulfurreducens e-pili.
Another observation that only seems explicable if e-pili are comprised of PilA is the dynamic tuning of pili conductivity by more than one million-fold that is possible simply by modifying the abundance of aromatic amino acids in the pilin monomer protein. For example, replacing the G. sulfurreducens PilA gene with the G. metallireducens PilA gene yielded filaments with the same 3 nm diameter of the wild-type G. sulfurreducens pili, but with a conductivity that was 5,000-fold higher than wild-type (Tan et al., 2017). The higher conductivity was attributed to a higher abundance of aromatic amino acids in the G. metallireducens pilin. Conversely decreasing the abundance of aromatic amino acids in the pilin, still yielded 3 nm diameter filaments, but with a conductivity 1,000-fold lower than wild-type (Adhikari et al., 2016).
Not only is there substantial evidence that G. sulfurreducens expresses conductive filaments comprised of the PilA pilin monomer, but also direct examination of filaments emanating from cells revealed that e-pili are the primary filaments that G. sulfurreducens produces. In one approach, synthetic pilin monomer genes that yield pilin monomers with peptide tags were expressed in G. sulfurreducens (Ueki et al., 2019). All the pili that these strains of G. sulfurreducens displayed reacted with antibodies that specifically bind to the peptide tags that were incorporated in PilA. The stoichiometry of antibody binding to pili could be tuned by controlling the relative quantity of synthetic pilin with tags vs. wild-type pilin expressed in strains containing genes for both pilin types (Ueki et al., 2019).
In an alternative approach, atomic force microscopy revealed that 90% of the filaments that G. sulfurreducens displayed had the same 3 nm diameter, morphology, and conductance as the conductive filaments produced when E. coli heterologously expressed G. sulfurreducens PilA (Liu et al., 2021). The other 10% of the filaments had a morphology and diameter consistent with filaments comprised of the c-type cytochrome OmcS. Replacing the PilA gene in G. sulfurreducens with a gene for a pilin monomer with reduced aromatic amino acid content yielded a strain in which over 90 % of the filaments emanating from the cells were 3 nm diameter pili, morphologically similar to the pili of the strain expressing PilA, but with 1,000-fold less conductance. The abundance and conductance of the filaments comprised of OmcS was unchanged. A similar predominance of 3 nm diameter conductive pili and then decreased pili conductance when PilA was replaced with a gene for an aromatic-poor pilin was observed in studies conducted in a strain of G. sulfurreducens in which the gene for OmcS was deleted (Liu et al., 2022). The finding that changing the aromatic abundance of the pilin protein specifically and dramatically changed the conductance of the 3 nm diameter filaments indicated that these filaments were comprised of pilin (Liu et al., 2021, 2022). Thus, multiple lines of evidence suggest that G. sulfurreducens displays conductive pili comprised of PilA and that these are the most abundant filaments emanating from cells.
Importance of e-pili in Extracellular Electron Transfer
G. sulfurreducens requires its abundant e-pili for effective long-range extracellular electron transfer. The phenotypes of Geobacter strains that express poorly conductive pili provide the most direct evidence. Simply deleting the gene for PilA to prevent e-pili expression is not an appropriate approach because outer-surface c-type cytochromes that are also important for extracellular electron transfer are not properly localized to the outer surface in pilA-deletion mutants (Izallalen et al., 2008; Steidl et al., 2016; Liu et al., 2018). However, as noted above, G. sulfurreducens strains that express poorly conductive pili can be constructed by replacing the PilA gene with genes for pilins with a lower abundance of aromatic amino acids. These strains, which include G. sulfurreducens strains Aro-5, G. sulfurreducens strain Tyr3, G. sulfurreducens strain PA, and G. metallireducens strain Aro-5 express poorly conductive pili, while properly positioning outer-surface cytochromes on the outer cell surface (Vargas et al., 2013; Liu et al., 2014, 2021; Adhikari et al., 2016; Steidl et al., 2016; Ueki et al., 2018). None of these strains effectively reduce Fe(III) oxides or produce high current densities. G. metallireducens strain Aro-5 is an ineffective electron-donating partner for DIET (Ueki et al., 2018; Holmes et al., 2021).
The simplest explanation for these results is that the intrinsic conductivity of the wild-type e-pili is essential for effective extracellular electron transfer to Fe(III) oxides, other microbes, and through thick current-producing biofilms. G. sulfurreducens extracellular electron exchange is likely to rely on complex interactions between a suite of outer-surface c-type cytochromes, e-pili, and possibly other components (Lovley and Holmes, 2022). The phenotypes of strains expressing poorly conductive pili and cytochrome-deficient mutant strain phenotypes, as well as observations of cytochrome localization, demonstrate that cytochrome-based filaments alone cannot be the primary route for G. sulfurreducens long-range electron transfer (Lovley and Holmes, 2020, 2022).
Conclusions
In conclusion, many studies have provided evidence that G. sulfurreducens expresses e-pili comprised of the pilin monomer PilA. It remains a mystery as to why Gu et al. (2021) did not recover filaments comprised of PilA from their strain of ‘wild-type' G. sulfurreducens when so many other studies, including several by the senior author of Gu et al., had previously found PilA in filament preparations. Furthermore, e-pili comprised of PilA can clearly be seen emanating from cells of G. sulfurreducens. Other microbes can heterologously express the G. sulfurreducens PilA and assemble it into the same type of e-pili found in G. sulfurreducens. Consistent with these observations, G. sulfurreducens nanowire conductivity is readily tuned simply by changing the abundance of aromatic amino acids in the pilin expressed. Expression of poorly conductive pili has demonstrated the importance of e-pili in Fe(III) oxide reduction, electron transfer to other microbial species, and for generating high current densities in bioelectrochemical systems. Therefore, at present the preponderance of evidence is that e-pili, comprised of PilA, not only exist, but are an important feature in Geobacter extracellular electron exchange. The pilins and archaellins of phylogenetically distinct bacteria and archaea are assembled into conductive filaments and it seems likely that e-pili and e-archaella are spread throughout the microbial world (Walker et al., 2018, 2019, 2020; Bray et al., 2020; Lovley and Holmes, 2020).
Author Contributions
The author confirms being the sole contributor of this work and has approved it for publication.
Conflict of Interest
The author declares that the research was conducted in the absence of any commercial or financial relationships that could be construed as a potential conflict of interest.
Publisher's Note
All claims expressed in this article are solely those of the authors and do not necessarily represent those of their affiliated organizations, or those of the publisher, the editors and the reviewers. Any product that may be evaluated in this article, or claim that may be made by its manufacturer, is not guaranteed or endorsed by the publisher.
References
Adhikari, R. Y., Malvankar, N. S., Tuominen, M. T., and Lovley, D. R. (2016). Conductivity of individual Geobacter pili. RSC Advances. 6, 8354–8357. doi: 10.1039/C5RA28092C
Bray, M. S., Wu, J., Padilla, C. C., Stewart, F. J., Fowle, D. A., Henny, C., et al. (2020). Phylogenetic and structural diversity of aromatically dense pili from environmental metagenomes. Environ. Microbiol. Rep. 12, 49–57. doi: 10.1111/1758-2229.12809
Cologgi, D. L., Lampa-Pastirk, S., Speers, A. M., Kelly, S. D., and Reguera, G. (2011). Extracellular reduction of uranium via Geobacter conductive pili as a protective cellular mechanism. Proc. Natl. Acad. Sci. U S A. 108, 15248–15252. doi: 10.1073/pnas.1108616108
Cosert, K. M., Castro-Forero, A., Steidl, R. J., Worden, R. M., and Reguera, G. (2019). Bottom-up fabrication of protein nanowires via controlled self-assembly of recombinant Geobacter pilins. mBio. 10, e02721–19. doi: 10.1128/mBio.02721-19
Creasey, R. C. G., Mostert, A. B., Nguyen, T. A. H., Virdis, B., Freguia, S., et al. (2018). Microbial nanowires–electron transport and the role of synthetic analogues. Acta Biomater. 69, 1–30. doi: 10.1016/j.actbio.2018.01.007
Dorval Courchesne, N.-M., Debenedictis, E. P., Tresback, J., Kim, J. J., Duraj-Thatte, A., Zanuy, D., et al. (2018). Biomimetic engineering of conductive curli protein films. Nanotechnology. 29, 454002. doi: 10.1088/1361-6528/aadd3a
Fu, T., Liu, X., Fu, S., Woodard, T. L., Gao, H., Lovley, D. R., et al. (2021). Self-sustained green neuromorphic interfaces. Nat. Commun. 12, 3351. doi: 10.1038/s41467-021-23744-2
Fu, T., Liu, X., Gao, H., Ward, J. E., Liu, X., Yin, B., et al. (2020). Bioinspired bio-voltage memristors. Nat. Commun. 11, 1861. doi: 10.1038/s41467-020-15759-y
Gu, Y., Srikanth, V., Salazar-Morales, A. I., Jain, R., O'brien, J. P., Yi, S. M., et al. (2021). Structure of Geobacter pili reveals secretory rather than nanowire behaviour. Nature. 597, 430–434. doi: 10.1038/s41586-021-03857-w
Gutermann, T., and Gazit, E. (2018). Toward peptide-based bioelectronics: reductionist design of conductive pili mimetics. Bioelectron. Med. 1, 131–137. doi: 10.2217/bem-2018-0003
Holmes, D. E., Zhou, J., Ueki, T., Woodard, T. L., and Lovley, D. R. (2021). Mechanisms for electron uptake by Methanosarcina acetivorans during direct interspecies electron transfer. mBio. 12, e02344–21. doi: 10.1128/mBio.02344-21
Ing, N. L., Nusca, T. D., and Hochbaum, A. I. (2017). Geobacter sulfurreducens pili support ohmic electronic conduction in aqueous solution. PCCP. 19, 21791–21799. doi: 10.1039/C7CP03651E
Izallalen, M., Glaven, R. H., Mester, T., Nevin, K. P., Franks, A. E., and Lovley, D. R. (2008). Going wireless? Additional phenotypes of a pilin-deficient mutant weaken the genetic evidence for the role of microbial nanowires in extracellular electron transfer. In: Abstracts of the 108th Annual Meeting of the American Society for Microbiology (Boston, MA).
Leang, C., Qian, X., Mester, T., and Lovley, D. R. (2010). Alignment of the c-type cytochrome OmcS along pili of Geobacter sulfurreducens. Appl. Environ. Microbiol. 76, 4080–4084. doi: 10.1128/AEM.00023-10
Liu, X., Fu, T., Ward, J., Gao, H., Yin, B., Woodard, T. L., et al. (2020a). Multifunctional protein nanowire humidity sensors for green wearable electronics. Advanced Electronic Materials. 6, 2000721. doi: 10.1002/aelm.202000721
Liu, X., Gao, H., Ward, J., Liu, X., Yin, B., Fu, T., et al. (2020b). Power generation from ambient humidity using protein nanowires. Nature. 578, 550–554. doi: 10.1038/s41586-020-2010-9
Liu, X., Tremblay, P.-L., Malvankar, N. S., Nevin, K. P., Lovley, D. R., and Vargas, M. (2014). A Geobacter sulfurreducens strain expressing Pseudomonas aeruginosa type IV pili localizes OmcS on pili but Is deficient in Fe(III) oxide reduction and current production. Appl. Environ. Microbiol. 80, 1219–1224. doi: 10.1128/AEM.02938-13
Liu, X., Walker, D. J. F., Li, Y., Meier, D., Pinches, S., Holmes, D. E., et al. (2022). Cytochrome OmcS is not essential for extracellular electron transport via conductive pili in Geobacter sulfurreducens strain KN400. Appl. Environ. Microbiol. 88, e01622–21. doi: 10.1128/AEM.01622-21
Liu, X., Walker, D. J. F., Nonnenmann, S., Sun, D., and Lovley, D. R. (2021). Direct observation of electrically conductive pili emanating from Geobacter sulfurreducens. mBio. 12, e02209–21. doi: 10.1128/mBio.02209-21
Liu, X., Wang, S., Xu, A., Zhang, L., Liu, H., and Ma, L. Z. (2019). Biological synthesis of high-conductive pili in aerobic bacterium Pseudomonas aeruginosa. Appl. Microbiol. Biotechnol. 103, 1535–1544. doi: 10.1007/s00253-018-9484-5
Liu, X., Zhuo, S., Rensing, C., and Zhou, J. (2018). Syntrophic growth with direct interspecies electron transfer between pili-free Geobacter species. ISME J. 12, 2142–2151. doi: 10.1038/s41396-018-0193-y
Logan, B. E., Rossi, R., Ragab, A., and Saikaly, P. E. (2019). Electroactive microorganisms in bioelectrochemical systems. Nat. Rev. Microbiol. 17, 307–319. doi: 10.1038/s41579-019-0173-x
Lovley, D. R., and Holmes, D. E. (2020). Protein nanowires: the electrification of the microbial world and maybe our own. J. Bacteriol. 202, e00331–20. doi: 10.1128/JB.00331-20
Lovley, D. R., and Holmes, D. E. (2022). Electromicrobiology: the ecophysiology of phylogenetically diverse electroactive microorganisms. Nat. Rev. Microbiol. 20, 5–19. doi: 10.1038/s41579-021-00597-6
Lovley, D. R., Ueki, T., Zhang, T., Malvankar, N. S., Shrestha, P. M., Flanagan, K., et al. (2011). Geobacter: the microbe electric's physiology, ecology, and practical applications. Adv. Microb. Physiol. 59, 1–100. doi: 10.1016/B978-0-12-387661-4.00004-5
Lovley, D. R., and Yao, J. (2021). Intrinsically conductive microbial nanowires for “green” electronics with novel functions. Trends Biotechnol. 39, 940–952. doi: 10.1016/j.tibtech.2020.12.005
Reguera, G., and Kashefi, K. (2019). The electrifying physiology of Geobacter bacteria, 30 years on. Adv. Microb. Physiol. 74, 1–95. doi: 10.1016/bs.ampbs.2019.02.007
Reguera, G., Mccarthy, K. D., Mehta, T., Nicoll, J. S., Tuominen, M. T., and Lovley, D. R. (2005). Extracellular electron transfer via microbial nanowires. Nature. 435, 1098–1101. doi: 10.1038/nature03661
Roy, S., Xie, O., and Dorval Courchesne, N.-M. (2020). Challenges in engineering conductive protein fibres: Disentangling the knowledge. Canadian J. Chem. Engin. 98, 2081–2095. doi: 10.1002/cjce.23836
Shi, L., Dong, H., Reguera, G., Beyenal, H., Lu, A., Liu, J., et al. (2016). Extracellular electron transfer mechanisms between microorganisms and minerals. Nat. Rev. Microbiol. 14, 651–662. doi: 10.1038/nrmicro.2016.93
Smith, A. F., Liu, X., Woodard, T. L., Emrick, T., J.M., J., et al. (2020). Bioelectronic protein nanowire sensors for ammonia detection. Nano Res. 13, 1479–1484. doi: 10.1007/s12274-020-2825-6
Steidl, R. J., Lampa-Pastirk, S., and Reguera, G. (2016). Mechanistic stratification in electroactive biofilms of Geobacter sulfurreducens mediated by pilus nanowires. Nature Commun. 7, 12217. doi: 10.1038/ncomms12217
Tan, H.-Y., Adhikari, R. Y., Malvankar, N. S., Ward, J. E., Woodard, T. L., Nevin, K. P., et al. (2017). Expressing the Geobacter metallireducens PilA in Geobacter sulfurreducens yields pili with exceptional conductivity. mBio. 8, e02203–16. doi: 10.1128/mBio.02203-16
Tan, Y., Adhikari, R. Y., Malvankar, N. S., Ward, J. E., Nevin, K. P., Woodard, T. L., et al. (2016). The low conductivity of Geobacter uraniireducens pili suggests a diversity of extracellular electron transfer mechanisms in the genus Geobacter. Front. Microbiol. 7, 980. doi: 10.3389/fmicb.2016.00980
Ueki, T., Nevin, K. P., Rotaru, A.-E., Wang, L.-Y., Ward, J. E., Woodard, T. L., et al. (2018). Geobacter strains expressing poorly conductive pili reveal constraints on direct interspecies electron transfer mechanisms. mBio. 9, e01273–18. doi: 10.1128/mBio.01273-18
Ueki, T., Walker, D. J. F., Tremblay, P.-L., Nevin, K. P., Ward, J. E., Woodard, T. L., et al. (2019). Decorating the outer surface of microbially produced protein nanowires with peptides. ACS Synth. Biol. 8, 1809–1817. doi: 10.1021/acssynbio.9b00131
Ueki, T., Walker, D. J. F., Woodard, T. L., Nevin, K. P., Nonnenmann, S., and Lovley, D. R. (2020). An Escherichia coli chassis for production of electrically conductive protein nanowires. ACS Synth. Biol. 9, 647–654. doi: 10.1021/acssynbio.9b00506
Vargas, M., Malvankar, N. S., Tremblay, P.-L., Leang, C., Smith, J. A., Patel, P., et al. (2013). Aromatic amino acids required for pili conductivity and long-range extracellular electron transport in Geobacter sulfurreducens. mBio. 4:e00105–13. doi: 10.1128/mBio.00210-13
Walker, D. J. F., Adhikari, R. Y., Holmes, D. E., Ward, J. E., Woodard, T. L., Nevin, K. P., et al. (2018). Electrically conductive pili from genes of phylogenetically diverse microorganisms. ISME J. 12, 48–58. doi: 10.1038/ismej.2017.141
Walker, D. J. F., Martz, E., Holmes, D. E., Zhou, Z., Nonnenmann, S. S., and Lovley, D. R. (2019). The archaellum of Methanospirillum hungatei is electrically conductive. mBio. 10, e00579–19. doi: 10.1128/mBio.00579-19
Walker, D. J. F., Nevin, K. P., Nonnenmann, S. S., Holmes, D. E., Woodard, T. L., Ward, J. E., et al. (2020). Syntrophus conductive pili demonstrate that common hydrogen-donating syntrophs can have a direct electron transfer option. ISME J. 14, 837–846. doi: 10.1038/s41396-019-0575-9
Wang, F., Gu, Y., O'brien, J. P., Yi, S. M., Yalcin, S. E., Srikanth, V., et al. (2019). Structure of microbial nanowires reveals stacked hemes that transport electrons over micrometers. Cell. 177, 361–369. doi: 10.1016/j.cell.2019.03.029
Keywords: Geobacter, e-pili, extracellular electron transfer, electromicrobiology, protein, protein nanowires
Citation: Lovley DR (2022) On the Existence of Pilin-Based Microbial Nanowires. Front. Microbiol. 13:872610. doi: 10.3389/fmicb.2022.872610
Received: 09 February 2022; Accepted: 17 May 2022;
Published: 06 June 2022.
Edited by:
Nils Risgaard-Petersen, Aarhus University, DenmarkReviewed by:
Tom Clarke, University of East Anglia, United KingdomLi Zhuang, Jinan University, China
Copyright © 2022 Lovley. This is an open-access article distributed under the terms of the Creative Commons Attribution License (CC BY). The use, distribution or reproduction in other forums is permitted, provided the original author(s) and the copyright owner(s) are credited and that the original publication in this journal is cited, in accordance with accepted academic practice. No use, distribution or reproduction is permitted which does not comply with these terms.
*Correspondence: Derek R. Lovley, dlovley@umass.edu