- 1Guangxi Colleges and Universities Key Laboratory of Crop Cultivation and Tillage, Agricultural College, Guangxi University, Nanning, China
- 2Shaanxi Key Laboratory of Earth Surface System and Environmental Carrying Capacity, College of Urban and Environmental Science, Northwest University, Xi’an, China
- 3Maize Research Institute of Guangxi Academy of Agricultural Sciences, Nanning, China
- 4Department of Soil and Environment Science, University of Agriculture, Peshawar, Pakistan
Sustainable reduction of fertilization with technology acquisition for improving soil quality and realizing green food production is a major strategic demand for global agricultural production. Introducing legume (LCCs) and/or non-legume cover crops (NLCCs) during the fallow period before planting main crops such as wheat and corn increases surface coverage, retains soil moisture content, and absorbs excess mineral nutrients, thus reducing pollution. In addition, the cover crops (CCs) supplement the soil nutrients upon decomposition and have a green manure effect. Compared to the traditional bare land, the introduction of CCs systems has multiple ecological benefits, such as improving soil structure, promoting nutrient cycling, improving soil fertility and microbial activity, controlling soil erosion, and inhibiting weed growth, pests, and diseases. The residual decomposition process of cultivated crops after being pressed into the soil will directly change the soil carbon (C) and nitrogen (N) cycle and greenhouse gas emissions (GHGs), and thus affect the soil microbial activities. This key ecological process determines the realization of various ecological and environmental benefits of the cultivated system. Understanding the mechanism of these ecological environmental benefits provides a scientific basis for the restoration and promotion of cultivated crops in dry farming areas of the world. These findings provide an important contribution for understanding the mutual interrelationships and the research in this area, as well as increasing the use of CCs in the soil for better soil fertility, GHGs mitigation, and improving soil microbial community structure. This literature review studies the effects of crop biomass and quality on soil GHGs emissions, microbial biomass, and community structure of the crop cultivation system, aiming to clarify crop cultivation in theory.
Introduction
Cover crops (CCs) within agroecosystems impart ecological and environmental benefits, like enhancement of soil fertility, C sequestration, leaching reduction, erosion control, and pest and disease suppression (Alfonso Gomez et al., 2018; Berlanas et al., 2018). Cover crops also increase nutrient cycling and biological N fixation, soil organic matter (SOM), biological diversity (e.g., microbes, insects, and birds), weed control, and crop yields (Muhammad et al., 2019), decreasing drainage, increasing infiltration, and maintaining soil nutrients (Wawan, Dini and Hapsoh., 2019). In addition, CCs provide a friendly agronomic environment with suppression of weeds and thus decreasing the dependency for the herbicides uses (Gavazzi et al., 2010; Barros et al., 2013). Dhima et al. (2006) reported that winter CCs, such as cereal rye (Secale cereale L.) and barley (Hordeum vulgare L.), could release inhibitory substances known as allelochemicals that can affect the initial growth of grass weeds like barnyard grass.
Previous researchers studied the impact of CCs on SOM in temperate zones and some ephemeral and long-term pools in the Mediterranean and semi-arid annual agroecosystems (Zhou et al., 2016; Austin et al., 2017). Meisinger et al. (1991) reviewed past studies and demonstrated that CCs minimized 20 to 80% of nitrate losses through leaching, whereas NLCCs are more effective than leguminous CCs. They found that winter CCs (small grains) could reduce the nitrate load through leaching and nitrate concentrations by 64 and 50%, respectively. Potential nitrate N leaching in the drainage was minimized by proper crop rotation using CCs (Dinnes et al., 2002). Logsdon et al. (2002) demonstrated that oat and rye CCs significantly decreased nitrate N by 70% in maize-soybean rotation in three simulated years.
Cover crop residues mitigate the negative effects of soil disruption as a result of improving SOM, soil moisture, preventing the germination and emergence of weed seeds, and defending against erosion (Teasdale, 1996; Hall et al., 2010; Alfonso Gomez et al., 2018). Residues mulching maintain the soil moisture by reducing the soil temperature (Unger et al., 1997; Bagley et al., 2012; Li et al., 2013). Cover crops can also affect crop yields through changes in N dynamics in the soil. The addition of NLCCs such as oats tends to reduce mineralization and increases immobilization, lowering the inorganic N availability for the following crop (Haramoto and Brainard, 2012). However, farmers prefer cold-tolerant and productive cereal CCs in the Upper Midwest of the United States and seldom experiment with LCCs (Snapp and Borden, 2005). Leguminous CCs are a rich source of soil N and decompose faster than NLCCs, which results in higher nitrous oxide (N2O) emissions, however, NLCCs have a higher C:N ratio and are a rich source of soil organic carbon (SOC), which has higher carbon dioxide (CO2) emissions (Muhammad et al., 2019).
The introduction of CCs into agricultural soil is an important management practice (Figure 1). As shown in Figure 1, the CCs mulching and incorporation increase soil fertility, soil microbial growth, and hence SOM and residues decomposition. The schematic diagram shows that the incorporated residues decomposed faster than mulching, which released more GHGs and rapid availability of nutrients to plants. It has been used extensively to boost SOM and consequently increase cash crop productivity (Sainju and Singh, 2008). CCs cultivation not only provides physical protection to the soil by reducing the impact of rainfall but can also improve soil structure aggregation and microorganisms (Tang et al., 2017; Araujo et al., 2019). Soil organic carbon (SOC) is the critical component of SOM, soil functions, and agricultural ecosystems sustainability (Muhammad et al., 2018; Chalise et al., 2019). Labile organic C is the most active part of SOC and can be used in the short-term experiments as an indicator for assessing the soil quality (Ghimire et al., 2017; Sharma et al., 2018). Furthermore, soil microbes easily access labile organic C and serve as essential nutrients for crop growth (Haynes, 2005). The current review aims (1) to examine the effect of CC types and amount on soil microbial biomass, community abundance and structure, and soil GHGs emissions, (2) to understand the decomposition pattern of CCs residue based on the C:N ratio and its impact on microbial dynamics and GHGs emissions. Excessive fertilization of crops leads to watercourses pollution, thus adding interest to the area of research in green manure practices and its management. Even through a considerable amount of work has been done regarding mineralization, yet the whole concept of residues decomposition, residues quality (low and high C:N ratios), and different CCs types need further detailed exploration in future. Therefore, there is a need to identify the impact of residue management on soil property, soil microbes, and GHGs emission from various residue types and management.
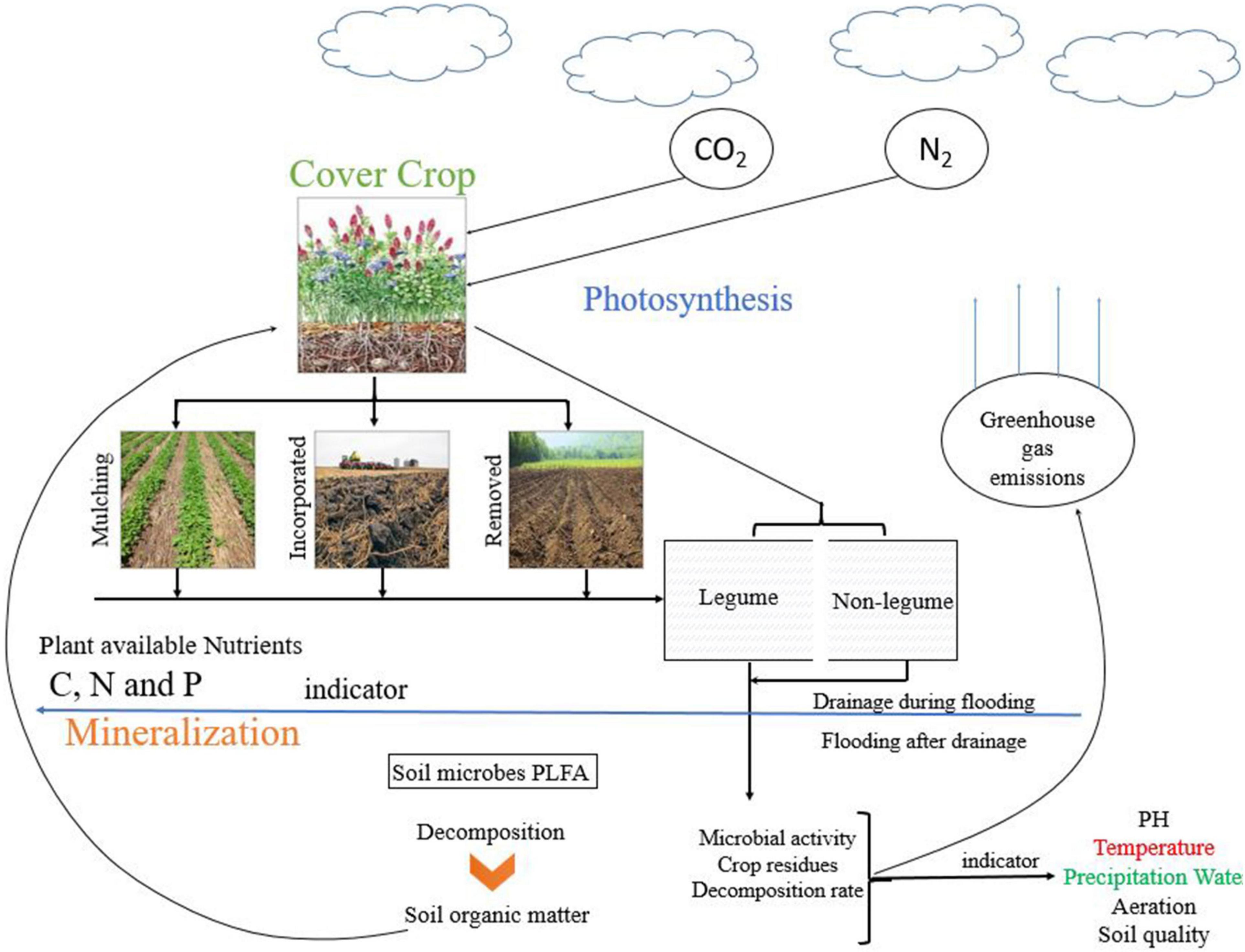
Figure 1. Schematic diagram of CCs growing, termination methods, and their relationship with soil microbes and GHG emissions.
Manipulating Cover Crop Types to Influence Soil Microbial Biomass, Community, and Greenhouse Gas Emissions
Cover crops have been increasingly grown to improve soil health and crop production and minimize the environmental impact compared to NCCs. It is making a tremendous contribution to the supply of food. CC growing and its residue management is an essential cultural practice for improving productivity on a sustainable basis. This study is truly relevant to the national development and socioeconomic stability of the world. This research will lead to utilizing the organic waste in a better and less harmful manner for crop improvement and soil fertility.
Legume Cover Crops
Legume CCs have been used to increase SOM and N concentration (Maltais-Landry and Crews, 2019). The amount of N fixed by legumes is dependent on legume species and environmental conditions (Liebman et al., 2018), and hence increases soil N2O emissions (Peyrard et al., 2016). It has been estimated that some LCCs can fix 115 kg of N ha–1 year–1 from atmospheric N (N2) (Peyrard et al., 2016). Kornecki et al. (2016) reported that crimson clover (Trifolium incarnatum L.) increased yield by 30% when compared to NCCs plots. However, Reddy (2001) reported that LCCs had reduced the soybean yield when compared to NCC plots. Reddy (2003) observed a 50% reduction in grass weeds, such as barnyard grass, broadleaf signal grass, brown top millet, and a 55% reduction in entire leaf morning-glory (Ipomoea purpurea) emergence when using crimson clover. The N taken up by CCs may be subsequently available through mineralization after incorporation (Figure 2), thereby reducing the commercial N fertilizer requirement of the subsequent crop (Ackroyd et al., 2019). The incorporation of LCCs had higher N2O emissions than NLCCs and mixed CCs (Peyrard et al., 2016; Kandel et al., 2018). In low input and organic farming systems, the N released after plants incorporation provides a valuable source of N for the following arable crop. In conventional farming systems, CCs have been found to retain up to 60 kg of N ha–1 during the growing seasons (De Almeida Acosta et al., 2014). The introduction of LCCs into the soil reduces the inputs of commercial N, thus limiting leaching of N and acts as green manuring (GM) for the succeeding main cash crop (Couedel et al., 2018a; Abdalla et al., 2019). Legumes such as vetch (Vicia sativa) and clover (Trifolium sp) CCs have higher N fixation capability than NLCCs (Sainju et al., 2007). Legume CCs decompose faster than NLCCs and mixed CCs, which results in higher N2O emissions and lower soil CO2 emissions (Gonsiorkiewicz Rigon et al., 2018), and thus decreases N leaching and emissions (Muhammad et al., 2019).
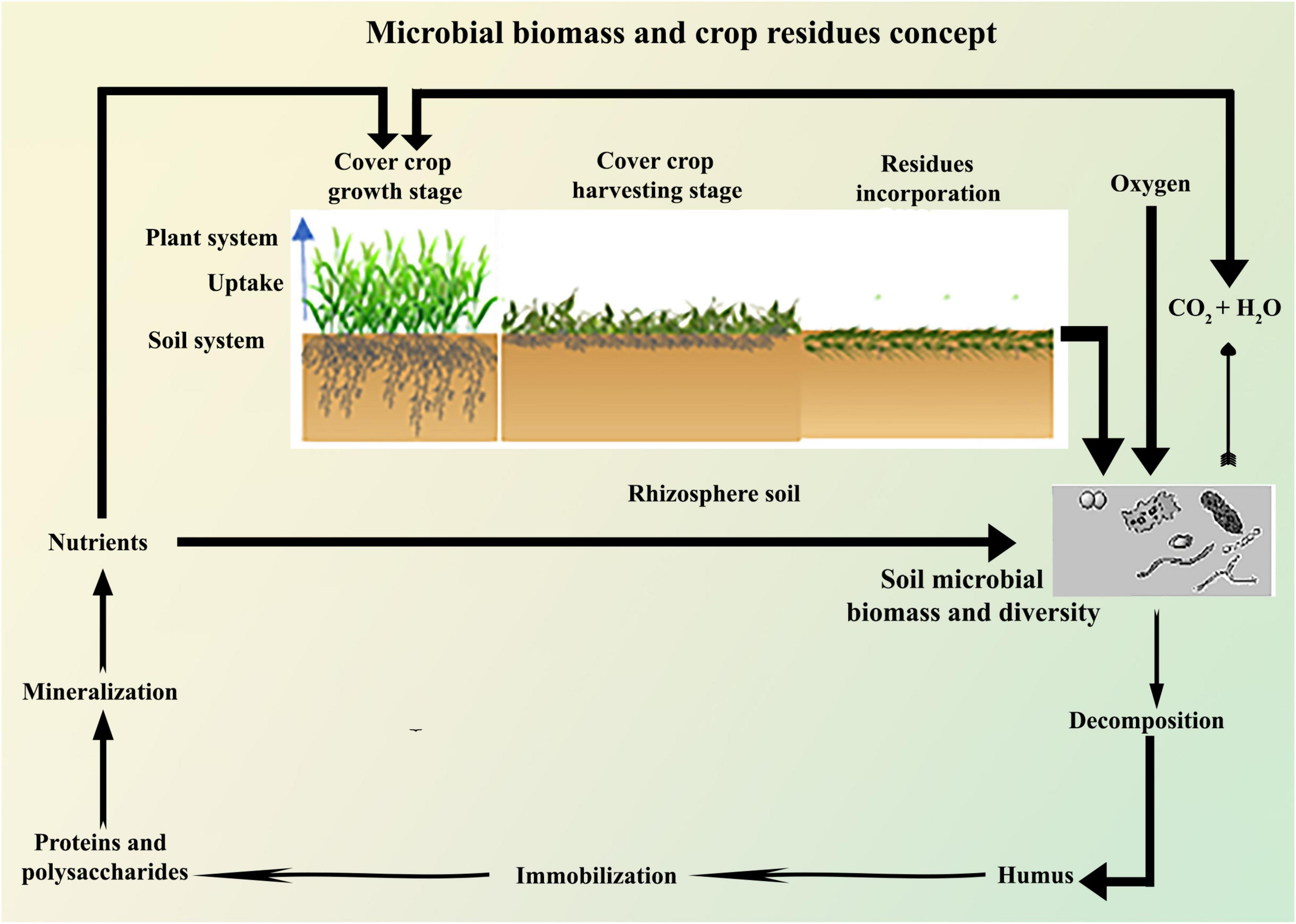
Figure 2. Schematic relationship of residues incorporation, decomposition, nutrient immobilization, and mineralization through soil microbes.
Green manuring crops are grown to increase soil fertility and provide a source of N for the subsequent crops. Since GM improves SOM content and especially nutrition value, thus GM is often incorporated in an early immature stage before the cash crop grows (Venkateswarlu et al., 2007; Hwang et al., 2015; Madsen et al., 2016). Legumes are commonly grown as GM due to their high-quality residues (lower C:N ratio) and fixed biological N2 from the atmosphere, which leads to decreased N2O emissions by 55% (Sanz-Cobena et al., 2014), and decreased N2O emissions by 86% in barley crop (Sanz-Cobena et al., 2017). However, CCs during the growth period may reduce gaseous losses by N uptake. Nevertheless, their incorporation may result in increased N2O production during nitrification and/or denitrification, released inorganic N in decomposition, and created anaerobic zones in the soil (Baggs et al., 2000; Couedel et al., 2018b). These emissions are generally higher where high N residues such as LCCs are incorporated (Peyrard et al., 2016). The importance of such gaseous losses, in relation to a crop recovery and leaching losses, needs to be quantified to improve N use efficiency in organic systems.
Non-legume Cover Crops
The capacity of non-legume CCs is to minimize soil erosion (wind and water) and water runoff, increase soil aeration, available water holding, SOM and nutrient cycling (Wawan, Dini and Hapsoh., 2019), reduce NO3–-N losses in fallow soils, and provide more N for subsequent cash crops (Sainju and Singh, 2008). The impact of NLCCs on N dynamics is not fully understood, particularly in vegetable fields (White et al., 2020). The mineralization of NLCCs by soil microbes can take place on a long fallow period, and readily available mineral N lost as leachate or emissions from fallow (Rodrigues Torres et al., 2008). It was found that CCs can provide 20 to 55% of the recovered N for succeeding crops (Malpassi et al., 2000) beside these losses. However, to reduce the dependency of nitrogenous fertilizers without compromising yield, the mineralization of crop residues N in soil should be synchronized with the N demand of the main crop (Weinert et al., 2002). Cover crop types, growth period, precipitation, and temperature affect N accumulation, N use efficiency, and soil fertility of subsequent cash crops (Toom et al., 2019). Non-legume CCs such as oats and rye produced greater biomass than LCCs (Whitehead and Singh, 2010). Furthermore, grasses use residual N more effectively than LCCs, hence preventing N losses through leaching (Campiglia et al., 2009). Winter CCs are more effective in reducing surface flow and increasing the evapotranspiration of water from field soil. Rotary tillage and rye CCs significantly boosted fungal substrate-induced respiration, SOC, and mean weight diameter (Nakamoto et al., 2012). Similarly, long-term rotations of maize, soybean, rye, and oat CCs decreased concentrations of NO3– in tile drainage by 48 and 26%, respectively (Kaspar et al., 2012). Winter CCs are effective, but in the fall, they must grow sufficiently to immobilize residual N in the soil, as shown by Mays et al. (2003), whereas delayed crop growth could be due to late seeding in the fall, which decreases NO3– immobilization and increases NO3– losses by leaching (Sanz-Cobena et al., 2012).
Mixed Cover Crops
To increase the utilization of CCs, growers need specific regional information to understand how the biomass and quality of CCs can affect crop yields and reduce emissions. Selection of CC types, tillage practices, termination date, residue decomposition, residue quality, and quantity is highly desirable in such a situation. Residue consistency is often distinguished by the content of C and N, lignification, C:N ratios, and the content of polyphenols (Muhammad et al., 2019; Liu et al., 2021; Wang et al., 2021). If the C:N of crop residues is low, it is generally considered that high-quality crops and nutrients will be released, which affects crop yield strongly and vice versa (Marahatta et al., 2012). A strategy for increasing the quality of crop residues (C:N ratio) and minimizing N immobilization is needed. It has been reported that a mixture of NLCCs and LCCs monocultures is the better choice to improve soil fertility, crop production, and minimize environmental contaminations (Odhiambo and Bomke, 2000). Mixed CCs provide another strategy to mitigate environmental problems because they have a relatively high C:N ratio compared with LCCs and consequently reduce N2O emissions (Aita and Giacomini, 2003; Schmeer et al., 2014).
Cover Crop Uses and Benefits for Soil Microbial Biomass and Community Improvement
Cover crop cultivation and its residues management practices are the main factors that improve soil water holding capacity, soil microbial abundance and structure, and weed suppression. CCs incorporation, mulching, and removal from the field after harvesting have a critical impact on soil microorganisms. The influence of Cover crop types, residues management, and restudies quality are the key strategies to improve soil microbial communities, soil bacteria, and soil fungi.
Soil Microbes
Decades of intensive farming have reduced SOM content, thus plummeting soil fertility and arable land biodiversity (Gardiano et al., 2013). Subsequently, important services for soil ecosystems like nutrient cycling, water management, C storage, and functional biodiversity have been impaired. Microbial communities are vital for improving soil structure conservation and act as main decomposers of fresh organic material and drive biogeochemical nutrients transformation (Pina, 2019). The impacts of management practices on microbial populations are well known, at least regarding the increase in bacterial abundance and enzymatic activity (Muhammad et al., 2021a). Soil with sweet corn residue removed, incorporated, or garland chrysanthemum had 5.0, 5.4, and 6.2% higher microbial populations and 22, 32, and 26% higher fruit yield, respectively, than control soil (Tian et al., 2011). Similarly, the perennial CCs increased the N mineralization rate and MBC by 37 and 41%, respectively, compared to the NCCs (Pandey and Begum, 2010). In organic farming, huge amounts of C are usually incorporated into the soil, replacing mineral fertilizers ultimately increases the SOM content (Lal, 2009). In a recent study, the SOM content was increased in organic farming as compared to non-organic farming (Cagnini et al., 2019). The introduction of CCs increased the quantity of SOC and improved SOM, microbial biomass carbon, and microbial community structure (Finney et al., 2017). These modifications are essentially based on the characteristics of CCs chemistry and the biotic interactions between plant and soil. Leguminous CCs can fix more atmospheric N due to rhizobia increasing the mineralization and N pool of soil (Schroth et al., 2001). Similarly, previous studies have shown that different CC species had strong correlations with soil microbial biomass, suggesting that milk vetch had the highest microbial biomass N (15.4 mg kg–1) followed by ryegrass (11.3 mg kg–1), while the lowest 6.1 mg kg–1 was observed for the NCCs (Zhu et al., 2012).
Growing CCs create a conducive environment for microbial growth and activities, and upon the decay of CCs, the fungi are attacking residues first followed by bacteria (Hodge et al., 2001). CCs had a positive impact on soil microbial abundance, microbial activity, plant metabolism affecting soil respiration, and plant mineral nutrition (Setyawan et al., 2011), depending on the weather, plant species, and the season of the year. Grasses as CCs played an important role in soil management in citrus orchards and showed that grass roots release stimulating compounds for arbuscular mycorrhizal fungi, which is beneficial for plant growth (Lekberg and Koide, 2005). Legume CCs are more effective at fixing N, which is necessary for protein synthesis and plant growth. According to our estimation, the LCCs increase AMF, fungi, bacteria, MBC, MBN, and total PLFA by 17.37, 19.74, 74.2847.19, 33.1, and 34.4% compared to NCCs, respectively (Figure 3), however, fungi to bacteria ratio (F:B) and MBC:MBN ratio decreased by 0.3 and 5.63%, respectively. These results are in line with the finding of Cavalca et al. (2013), who reported that the CCs increase SOM content and microbial activity. The cultivation of CCs adds both the above-ground and below-ground biomass to soil (Wang et al., 2010), which raised the soil C and N stocks (Sainju et al., 2006; Buechi et al., 2015). Growing CCs are encouraged to optimize the productive use of N for a subsequent cash crop and increase productivity due to decreased nutrient losses through leaching (Valkama et al., 2016). Furthermore, reducing dependency on mineral fertilizers improved water holding capacity, suppressing insect pests and weeds (Dorn et al., 2015; Brooks et al., 2018; Maltais-Landry and Crews, 2019).
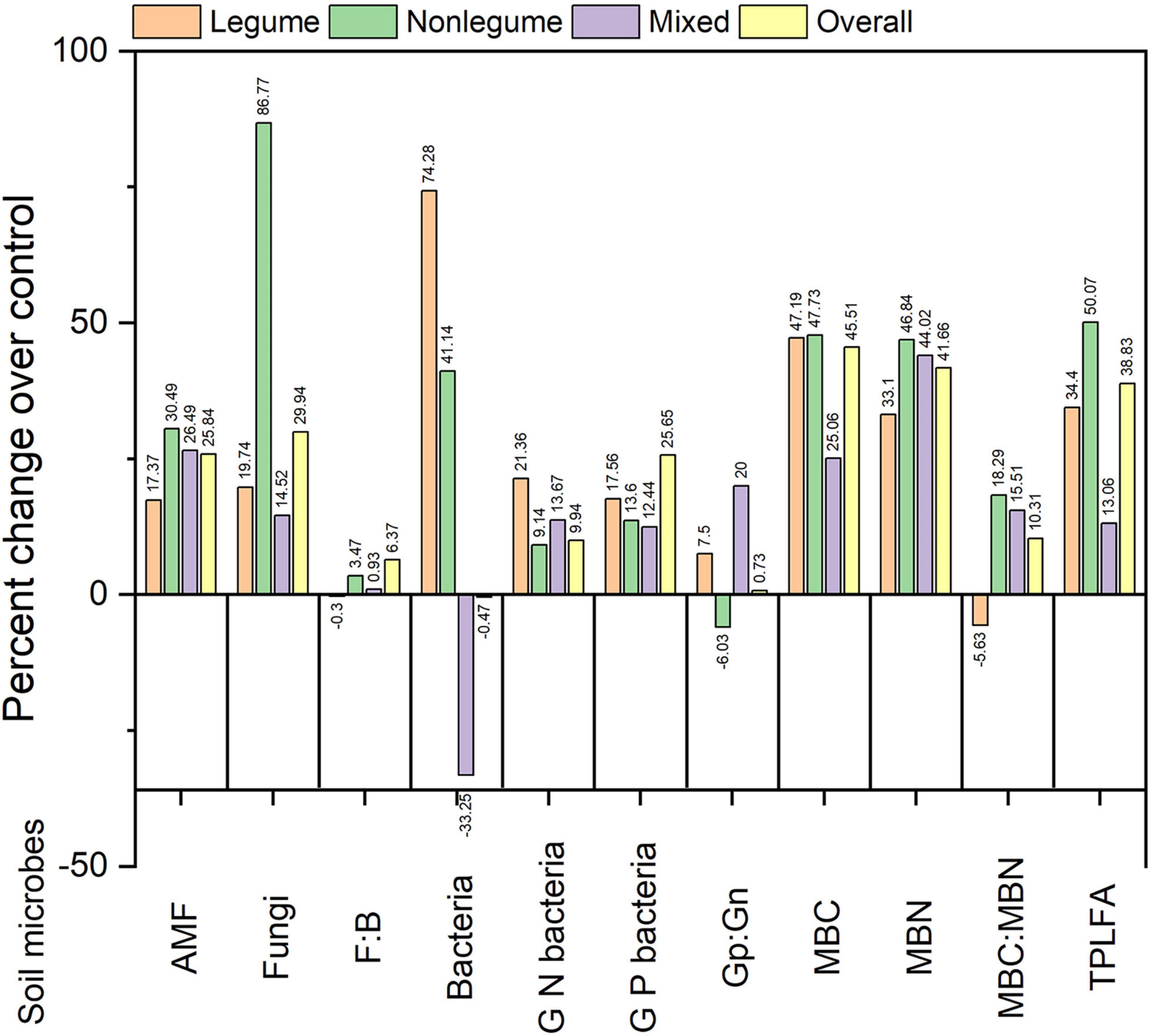
Figure 3. Percent changes of AMF, fungi, F:B, bacteria, Gram-positive bacteria (G P bacteria), Gram-negative bacteria (G N bacteria), Gram positive: Gram negative bacteria (Gp:Gn), microbial biomass carbon (MBC), microbial biomass nitrogen (MBN), MBC:MBN, and total phospholipid fatty acid analysis (PLFA) in cover crop (CC) treatments over control (Ncc).
Microbial Communities
The introduction of CCs into the agricultural system improves soil and environmental quality through increasing soil microbial population and reducing the application of chemical fertilization (Mitchell et al., 2017). Blanco-Canqui and Jasa (2019) testified that CCs enhanced the soil chemical, physical, and biological properties. CC utilization reduces soil erosion by covering the soil and improves soil quality by cycling nutrients, SOM, and MBC (Kerri and Belina, 2008). CCs significantly impact microbial communities’ size, operation, and structure by increasing soil C inputs (Xi et al., 2010). Soil microorganisms play a significant role in soil feeding, development, and restoration (Calderón et al., 2016; Zhou et al., 2017). According to Peregrina et al. (2014), soil microbial population characteristics are strongly linked to microbial biodiversity, soil and plant quality, and ecosystem sustainability. Researchers argued that nutrient cycling and C conservation are driven by soil microbial communities and vegetation species diversity (Carney and Matson, 2006). Soil microorganisms release enzymes that facilitate the breakdown of complex components in organic materials, and correlate enzymes with soil organic C and N substances, which is an indicators of microbial community due to changes in management systems (Tian et al., 2010).
Cover crops significantly improve SOM and boost soil microbial communities (Bacq-Labreuil et al., 2019). In addition, CCs used as GM could boost soil AMF, bacteria, microbial biomass, and total PLFA compared to NCCs (Figure 3). Higo et al. (2018) concluded that LCCs in rotation (corn-legume) form a symbiotic relationship with particular mycorrhizal fungi and bacterial groups. The continuous crop rotations are collectively referred to as legacy effects, such as beneficial legacy impacts enhancing competent AMF richness, AMF spore density, AMF root colonization, and microbial diversity, eventually improving soil health and agricultural production (Higo et al., 2019). Nonetheless, some of these legacy effects may not be necessary, such as replacing capable AMF with non-host crops and escalating the potential for nutrient immobilization and mineralization in subsurface soil in winter-wheat (Somenahally et al., 2018).
Soil Bacteria
The maintenance of biological health is important for restoring deteriorated soil because the living components of the soil are necessary for ecosystem functions and utilities (Lehman et al., 2015). CCs rotation and minimal tillage are approaches for enhancing the sequestration of organic compounds in agroecosystems that seem to be the most significant ecosystem services and significantly impact the soil biota. It was reported that the first principal component (PC1) distinguished the vetch treatments from the NCCs and wheat treatments, accounting for 23.4% of the total variability (Mbuthia et al., 2015). Gram-positive bacteria (i17:0, i16:0, a15:0, a17:0), Gram-negative bacteria (cy19:0ω8c,16.1ω7c), and actinomycetes (10Me16:0, 10Me17:0, 10Me18:0) were found in greater abundance in communities under the vetch CCs treatment. Communities with NCCs and wheat treatments, on the other hand, were linked to the mycorrhizae fungi fatty acid methyl ester biomarker (18:1ω9c) and the saprophytic fungi biomarker (18:2ω6c; Mbuthia et al., 2015). Cover crops and biological fertilizers are critical aspects of soil quality and fertility in organic management systems. Researchers discovered that CCs prevent soil C, boost SOM, reduce nutrient leaching, and LCCs fix N biologically (Snapp and Borden, 2005). CCs influence below ground soil functioning through soil microbial communities, such as nutrient cycling and availability, decomposition and transformation of crop residues, and disease suppression (Garbeva et al., 2004; Van Der Heijden et al., 2008). Cover crops generally increase overall microbial activity, nutrient cycling, and microbial diversity (Lori et al., 2017). Similarly, different compositions of CCs cause various changes in soil microbial populations, such as microbial infection, Gram-positive bacteria, Gram-negative bacteria, and bacterial/fungal ratios (Wortman et al., 2013; Buyer et al., 2017). It was reported that calopo (Calopogonium mucunoides) and callisia (Callisia repens) residues have 30 and 25% higher AMF than NCCs, respectively. However, mixed CCs significantly increased all the soil microbial biomass and community structure but decreased the soil bacteria by 33.25% (Figure 3). A previous study reported that root C content is more critical than residues C in maintaining stable C (Kong and Six, 2012) suggesting that the effect of litter quality on the sequestration of C is crucial (Mueller et al., 2017). Frasier et al. (2016) demonstrated that high quality litter and more effective soil biota would improve SOC stability and ultimately increase its storage.
Cover crops, the most commonly grown vegetation between cash crops, serve as organic matter modifications to the agroecosystems and increase SOC (Sharma et al., 2018). Studies have confirmed that CCs increased SOM and nutrient use efficiency in agroecosystems by reducing nitrate N losses through leaching and drainage, thereby increasing soil bacteria (Rice and Gowda, 2011; Alahmad et al., 2019). According to the microbial community structure estimated by PLFA profiles, the total bacterial and Gram-positive bacteria were significantly higher in CCs than in NCCs treatments. The PCoA analysis showed that the first two principal components, PC1 and PC2, accounted for 13.5 and 56.4% of the total bacterial variability. According to the PCoA, bacterial communities were clearly clustered according to their utilization of CCs (Figure 4), suggesting that the PC2 clearly separated the bacterial communities of CCs and NCCs plots. Furthermore, the bacterial community was not significantly affected by CCs with N fertilization in 10–30 cm soil depth (Alahmad et al., 2019); however, it reduced wind and water erosion (Moreira Rovedder and Foletto Eltz, 2008). The production of CCs improved soil quality and soil biota (Morales Salmeron et al., 2019). CC residues increased the amount of labile C in the agroecosystem, especially in the spring season after harvest, and the primary consumer of this labile C is the microbial community (Fernandez et al., 2016). These results show that glucose is a vital source of energy in microbial metabolism (Mukumbareza et al., 2016).
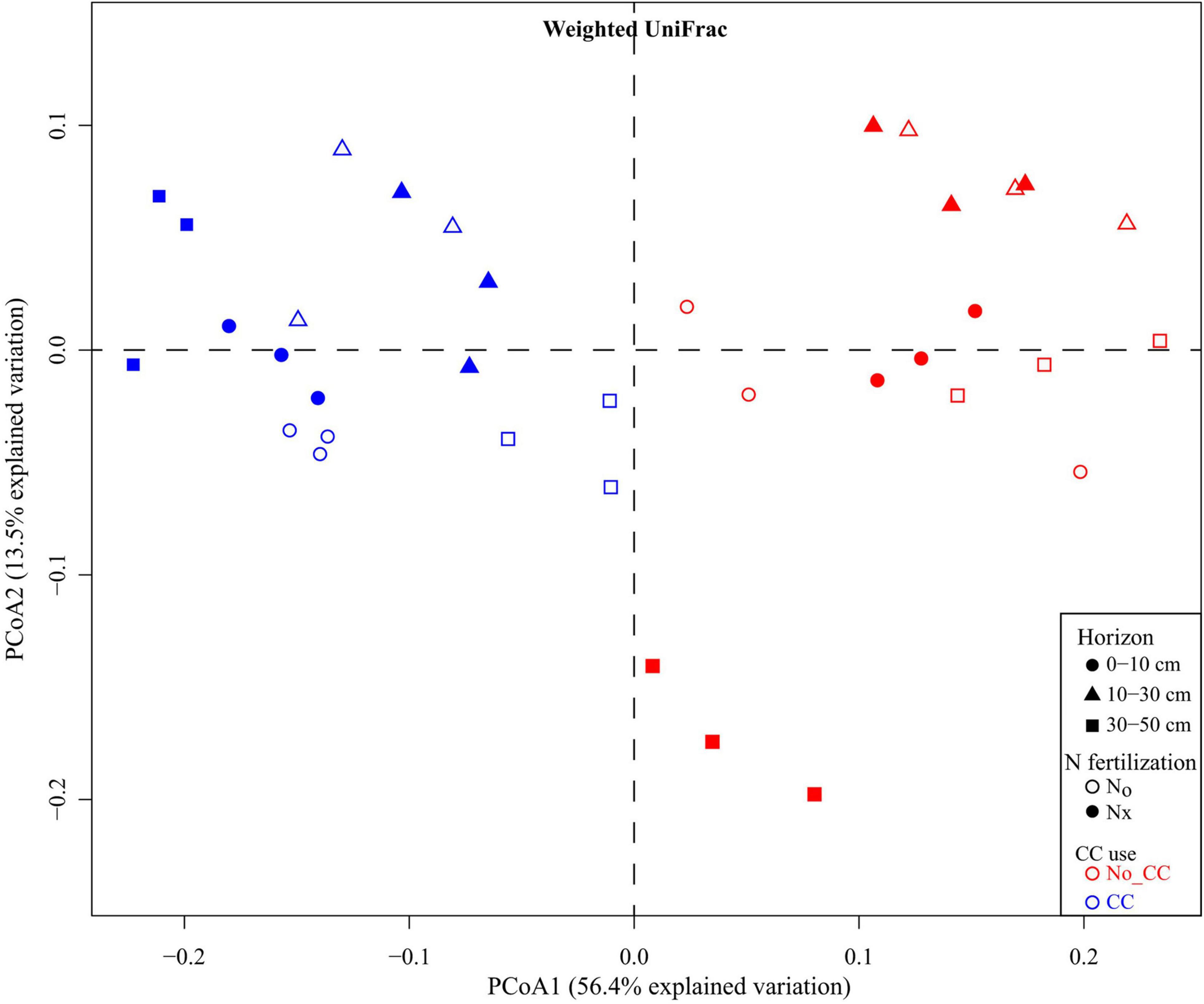
Figure 4. Principal coordinates analysis (PCoA) of microbial communities based on weighted UniFrac distances across soil horizons and among the different experimental treatments (Nx: conventional N fertilization; N0: no N fertilization; CC: presence of cover crops; No-CC: bare soil conditions). (Copied with permission from Alahmad et al., 2019, copyright (2018) John Wiley & Sons, Inc).
It was observed that cluster 5 (Figure 5) was associated with NCCs, dominated by Proteobacteria, and characterized by Blastocatella fastidiosa and Sphingomonas starnbergensis. The CCs were linked to Cluster 6, which included species from all major and minor phyla and was as described by Aciditerrimonas ferrireducens and Dehalogenimonas alkenigignensi (Figure 5). In CCs, the community was dominated by Actinobacteria, with Oscillochloris trichoides and Streptomyces griseus as characteristic species and containing many species from “other phyla” (Alahmad et al., 2019). The community in NCCs was characterized by species from Cluster 4, which included Proteobacteria, Actinobacteria, and Firmicutes, with Actinocatenispora rupis and Streptomyces chartreusis as representative species. Nitrogen fertilization resulted in functional divergence regardless of CCs, but this was supported by compositional divergence only in the presence of NCCs (Figure 5).
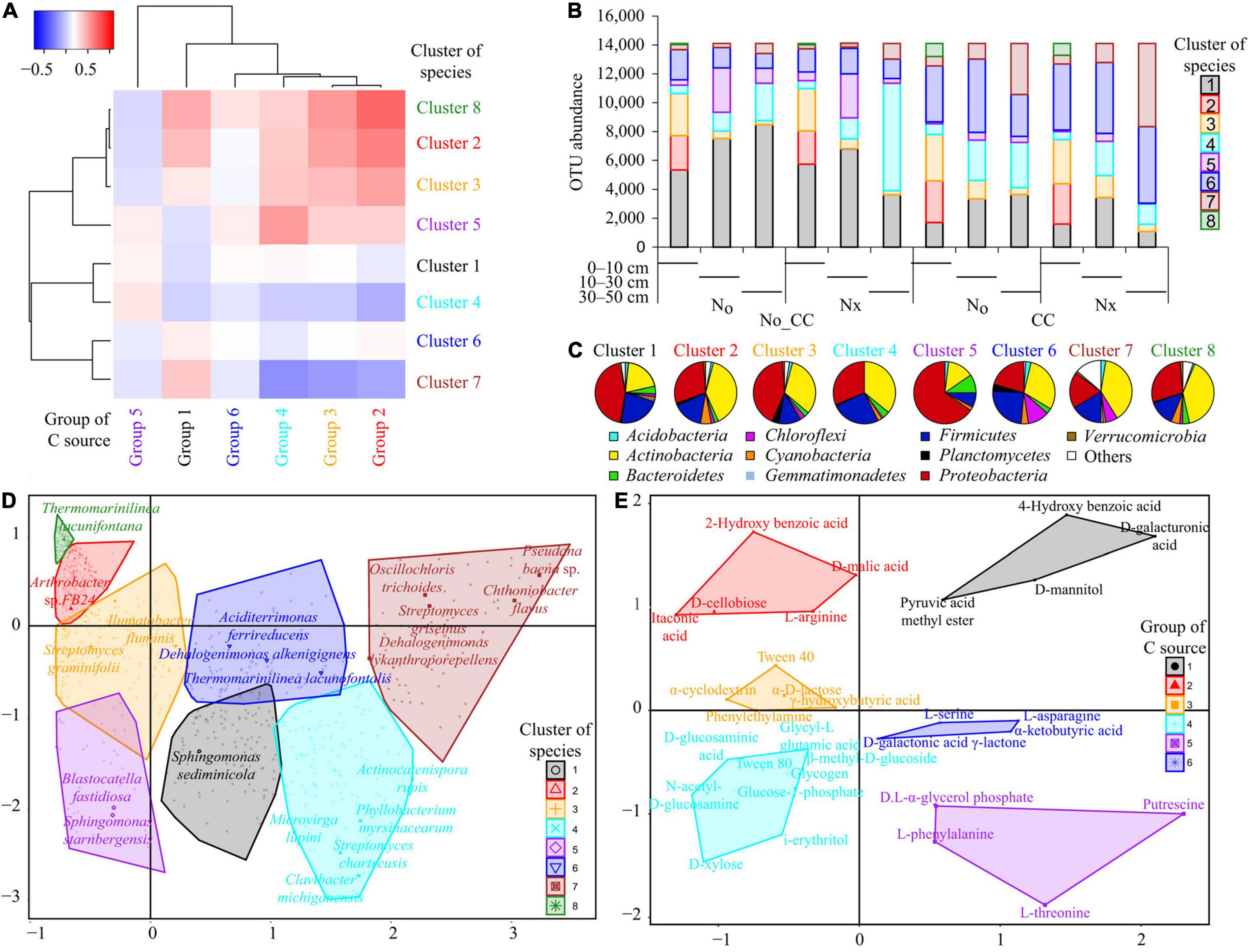
Figure 5. Results of the hierarchical clustering of bacterial species composition and used C-sources. (A) Heat-map of C-source groups and species clusters based on their values in the cross-table of the BGCoIA. (B) Relative importance of the clusters across soil depths and among treatments (Nx: conventional N fertilization; N0: no N fertilization; CC: presence of cover crops; No_CC: bare soil conditions). (C) Distribution of the main bacterial phyla among clusters. (D) Projection of the clusters and their constitutive species in the diagram defined by the first two BGCoIA axes. Only the name of the most characteristic species for each cluster is reported. (E) Projection of the groups and their constitutive C sources in the diagram defined by the first two BGCoIA axes. (Copied with permission from Alahmad et al., 2019, copyright (2018) John Wiley & Sons, Inc).
Soil Fungi
Kingdom fungi include a morphologically diverse group of species extending from single lad yeast to macro fungi, forming networks in soil over many meters. Fungi have attracted attention as major crop pathogens in cultivated agriculture. Nevertheless, they also play a key role in nutrient cycling via dead organic matter catabolism and mycorrhizal symbionts (Chavarria et al., 2016). In cultivated and grassy soils, AMF such as Glomeromycota is the primary mycorrhizal symbiont. Mycorrhizal colonization increased by 35, 29.4, and 20.9%, with hairy vetch, mixed CCs, and Indian mustard in maize crops, respectively. This suggests that releasing isothiocyanates in soil resulted in higher shoot biomass, N, and phosphorus content across all maize genotypes with mycorrhizal colonization (Njeru et al., 2013). However, recent progress in plant-soil interactions suggests that fungi have a broader range of effects that interact with higher grassland plants and thus play an important role in plant nutrition (Higo et al., 2017). CCs minimized nitrate leaching and plant disease and increased microbial populations and community structure (Singh and Kumar, 2021). White clover crop mulching increased AMF colonization and maize production (Deguchi et al., 2012). It has also been stated that the introduction of CCs during the bare fallow season increases the AMF inoculum capacity, AMF colonization, and production of subsequently cultivated major crops (Hontoria et al., 2019). Canonical correspondence analysis showed the relationships between AMF communities and winter CCs, revealing that winter CCs had a significant impact (F = 3.187, P = 0.001) on AMF communities (Higo et al., 2015).
Arbuscular mycorrhizal fungi provide many advantages in the symbiosis process for most plant species among various classes of microorganisms. Smith and Read (2008) suggested that AMF offered numerous benefits for host plants, including enhanced nutrition uptake, particularly in poor nutrient soils and plants tolerance to biotic and abiotic stresses. The AMF significantly improves agroecosystems sustainability and productibility while simultaneously decreasing the use of synthetic fertilizers (Lehman et al., 2015). Cover crops in agricultural practices permit native inoculum recovery and biodiversity, which are of particular interest to low-input systems (Martinez-Garcia et al., 2018). According to a structural equation model, root colonization (λ = 1.149) and maize phosphorus uptake (λ = 1.185) had immediate strong positive effects on crop performance, whereas AMF diversity (λ = 0.395) had intermediate positive effects (Higo et al., 2018). A recent study has shown that replacing fallow with CCs during intercropping time improves AMF root colonization in the succeeding cash crop (Cagnini et al., 2019).
Cover crop with negligible or zero tillage facilitate mycelium and make colonization faster (Brito et al., 2012). Likewise, CCs improve nutrient reusability, regulating weed growth, reducing erosion, subduing soil disease, and decreasing nutrient leakage (Briar et al., 2011). However, a limited number of literature studies are published to elaborate CCs effect on the AMF community (Bowles et al., 2017). Previous studies suggested that winter CCs influence and change the structure of the AMF population in the subsequent cash crops (Higo et al., 2018; Morimoto et al., 2018). However, instead of long-term experiments, researchers mostly studied short- to mid-term experiments in the past. Therefore, a literature review on how CC types and management practices influence the operation and microbial communities is required. Blanco-Canqui and Jasa (2019) reported that the AMF population could be significantly affected by various environmental factors, such as soil properties, nutrient availability, and air temperature. Arbuscular mycorrhizal fungi communities in legume CC plots are different from those in grass and herb plots (Figure 6A) and explain 16.1% of the variation in AMF communities. Likewise, archaeal communities in legume plots differed from grass and herb plots (Figure 6B), and the plant functional group accounted for 14.0% of the total variation. Regarding protists community composition, plant functional group had no significant explanatory power (Figure 6C). Abiotic properties appeared important for fungi and archaea, as shown in Figure 6D. The abiotic properties seemed important for fungal and archaea communities, which explained 9.0% of fungal community composition and 24.9% of archaeal community composition (Dassen et al., 2017).
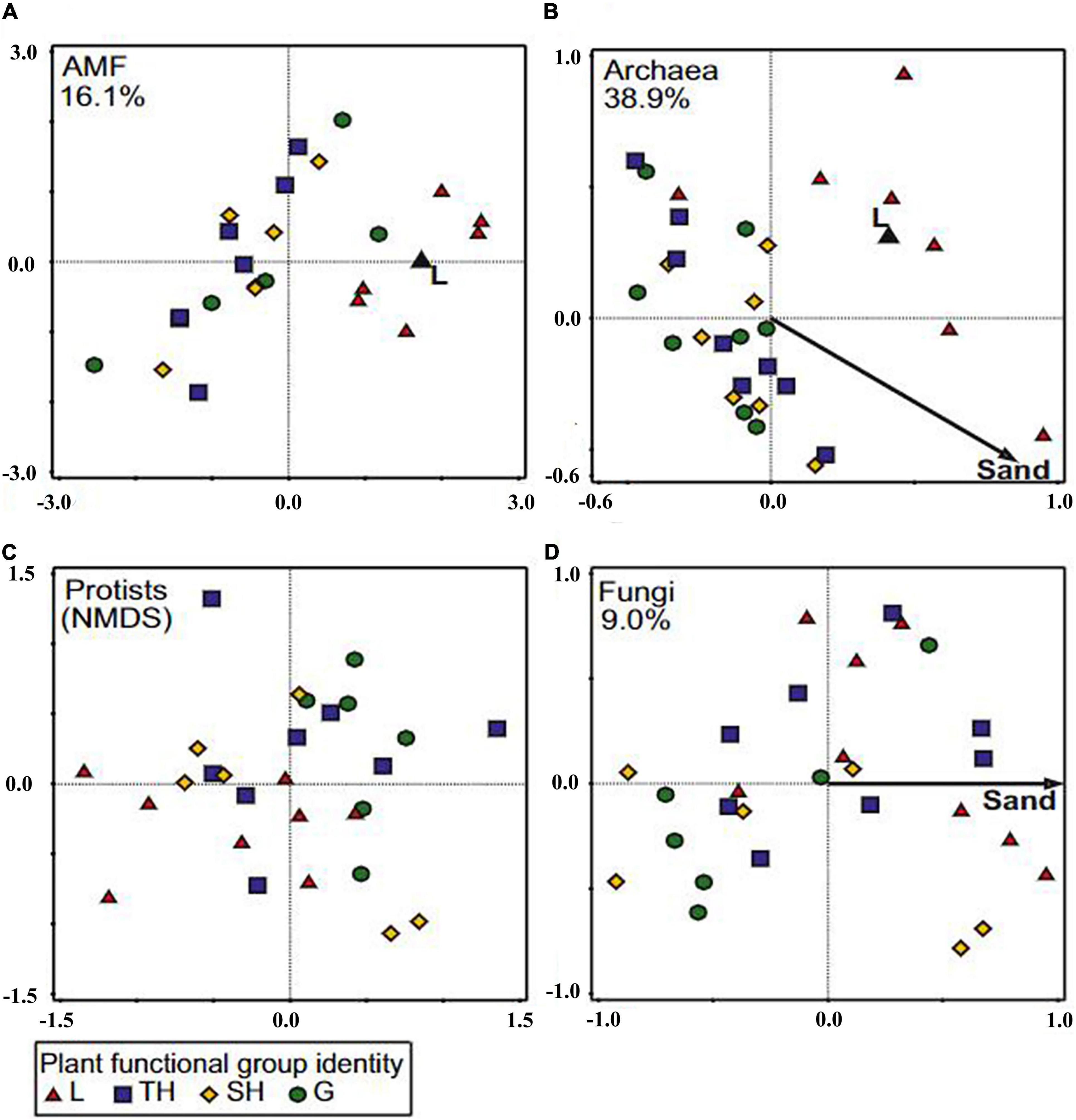
Figure 6. Distance-based redundancy analysis (db-RDA) plot showing the relationship of abiotic soil factors and plant functional group identities to community composition of AMF (A), archaea (B), and fungi (D). Community composition of protists (C) could not be explained by any of the factors measured; therefore, a non-metric multidimensional scaling (NMDS) of the community is shown instead. The plant functional groups tested were grasses (G; green), legumes (L; red), small herbs (SH; yellow), and tall herbs (TH; blue). The ordination is based on Bray–Curtis distance. With forward selection, factors were chosen that significantly (Padj < 0.05) contributed to the model. In each window, the percentage of explained variation is shown. (Adapted from Dassen et al., 2017 under the terms of the Creative Commons Attribution License 4.0).
Role of Soil Microbes in Greenhouse Gas Emissions
Crop production is the focusing center of agriculture in the world. It is making a tremendous contribution to the supply of food. CC growing and its residue management is an essential cultural practice for improving productivity and decreasing GHGs on a sustainable basis.
Greenhouse Gas Emissions
Agricultural soils are supposed to be a significant source of greenhouse gas (GHG) emissions, primarily nitrous oxide (N2O) and methane (CH4) as reported (IPCC, 2007). Over the past 100 years, the average global surface temperature has increased by 0.3–0.6°C due to the increase in GHG emissions (He et al., 2009). Carbon dioxide, N2O, and CH4 are the three main GHGs, and the increase in CO2 concentration contributes the most to the greenhouse effect (Ozturk and Acaravci, 2010). GHG emissions due to agricultural farming account for 10–12% of total GHG emissions. Agriculture is a large-scale human activity and has an important impact on global greenhouse emissions (Shelton et al., 2017). Numerous agricultural activities (e.g., irrigation, N fertilization, residues management) have been found to drive variables for these gas emissions during the crop cycle (IPCC, 2007; Muhammad et al., 2022). Some of these activities often impact gas emissions during the fallow period, particularly crops with low N use efficiency (NUE), while significant quantities of mineral N remain in the soil after the harvest (Sanchez et al., 2019). Intensively irrigated and fertilized agricultural systems generally have high levels of inorganic N that are lost in the ecosystem via leaching or denitrification during fallow or crop periods (Sanz-Cobena et al., 2012). Replacing bare fallow with CCs in the agricultural system is one of the most agronomic strategies for increasing the retention of inorganic surplus N and reducing nutrient losses (nitrate) through leaching (Dinnes et al., 2002).
In Mediterranean climate zones, winter CCs are used as catch crops (Gabriel and Quemada, 2011). However, due to CCs, the N and water requirements change and C pools may affect the processes leading to GHG emissions. CC root depth, crop N, water requirements, rhizosphere nutrient release, and climate change adaption are strategic factors for mitigating GHGs (Gabriel and Quemada, 2011). In this context, cereals crops generally reduce soil N content in the early growth stages due to higher N uptake and reduced losses through drainage water (Thorup-Kristensen et al., 2003; Moeller et al., 2008). Whereas cruciferous plants have a greater capacity to absorb N in deeper rooting systems at later growth stages and are more easily extracted from the deeper areas (Thorup-Kristensen et al., 2003). Legumes were assessed as catch crops, and it was shown that 50 to 60% of the N in legume tissue mainly comes from N absorption (Pappa et al., 2011). The soil alterations that affect GHGs during the CCs formation and its relation with GHGs are limited (Bayer et al., 2016).
After harvesting, CCs biomass is typically used as GM to reuse the N reserved in residues biomass and contribute to sustainable long-term soil fertility (Chirinda et al., 2010). The quality of CCs is mainly dependent on the C:N ratio, crop lignin content, and the N fixing capability, which affect the dynamics of C and N (Sofi et al., 2018). Incorporating crop residues into fertile soils will increase N2O emissions from cultivated soils (Bair et al., 2008), which depends largely on the residual biomass composition, suggesting that residues with low C:N ratios generally increase N2O emissions (Huang et al., 2016). Temporary N fixation often reduces N2O loss, and thus delays N availability for the crops as a result of CCs (Marahatta et al., 2012). In crop rotation, the N use efficiency of the main crops (cereals) is affected by GM of previous CCs and other N sources such as fertilizer. The joint effects of residues and synthetic N sources will also affect N2O emissions. Mineral N in a fertilized plot with greater C:N ratio residues promotes and increases N2O and CO2 emissions compared to sole N fertilizer because soluble residual organic C can be used as energy for denitrification (Ghimire et al., 2017; Ozturk, 2017). In terms of CH4 emissions, the amount of mineral N in the soil may influence the oxidation of this compound (Sanz-Cobena et al., 2014). The higher emissions might be due to the mineral N pool increasing through mineralization of N-rich plant material from legumes used as GM. Specific GHG emissions enable us to recommend specific crop management methods in irrigated corn systems to mitigate these losses (Rosolem et al., 2004; Sogbedji et al., 2006). At present, there are many studies on the effects of CC types and management practices on soil GHG emissions, but limited studies are available on CCs biomass rates and residue C:N ratios. The characteristics of GHGs emissions after CCs harvesting and whether the management of the residues (e.g., mulch, incorporation, and removal) are unclear. Thus, this review documented the effect of residue quality and quantity on different GHGs under different climatic reigns.
Carbon Dioxide Emissions
Greenhouse gas emissions from agricultural soils are affected by a variety of factors. After CCs introduction to the soil, the C and N cycle, pH, and biomass (microorganisms, roots, etc.) change, which affects GHG emissions in agriculture soil. Behnke and Villamil (2019) reported that CC introductions to the soil increased GHG emissions, which affected the initial C sequestration. In contrast, CCs mulching effectively reduced the GHG emissions (Tribouillois et al., 2018). CCs may accelerate soil CO2 efflux compared to conventional farming by increasing SOC and microbiological properties at the 0 to 2.5 cm soil surface (Peregrina, 2016). CC mulching increases the total porosity of the soil, and increases CO2 content of soil solution, which is conducive to the diffusion of CO2 (Mondal and Lenka, 2012). The range of CO2 emissions were 158 to 1884 and 154 to 1613 mg kg–1 for surface-applied and incorporated residues, respectively, and the highest emissions were found under the clover crop while the lowest were for the fallow (Linsler et al., 2016).
To use CCs, farmers select species that fulfill two functions: to keep soil protection and fertility in balance. Xiao et al. (2013) reported that CCs increased SOC content and changed it to a water-soluble form in the soil, thereby affecting the CO2 emission mode. Compared to NCC, the winter LCCs in the growing season had significantly higher CO2 emissions than in the rainy season. The range between NCCs (96.92 to 259.05 mg CO2 m–2 h–1) and winter LCCs (106.28 to 353.01 mg CO2 m–2 h–1) are shown in Table 1. Common vetch as a GM resulted in lowering soil C:N and higher CO2 emissions (range of CO2 8.09 to 10.58 g CO2 m–2 h–1), enzyme activity, and N release. Conversely, the GM of rye grass had a slightly lower CO2 emission than vetch CCs (range of CO2 emissions; 6.63 to 8.19 g CO2 m–2 h–1) and early soil N immobilization (Table 1). In contrast, NLCCs decreased CO2 emissions. Winter LCCs treatment had the greatest effect on soil CO2 and N2O emissions under furrow irrigation (Kallenbach et al., 2010). Zhou et al. (2019) suggested that CCs provide a C source for microorganisms, which promotes microbial growth and metabolism, thereby increasing CO2 emissions. A researcher demonstrated that CCs incorporation increases the content of water-stable aggregates in the surface soil, which positively correlated with CO2 emissions (Uliarte et al., 2013). In addition, CCs mulching can indirectly affect soil CO2 emissions by changing conditions such as preventing soil water losses and maintaining soil temperature. Kokalis-Burelle et al. (2017) reported that mulching of sun hemp as a CC improves the living environment of soil microorganisms increases microbial activity and may lead to increased CO2 emissions. Researchers found that CO2 emissions from bare land were much higher than those of CCs treatment, mainly because mulching reduced surface soil temperature and prevented the soil from emitting CO2 into the atmosphere (Li et al., 2013; Liang et al., 2017).
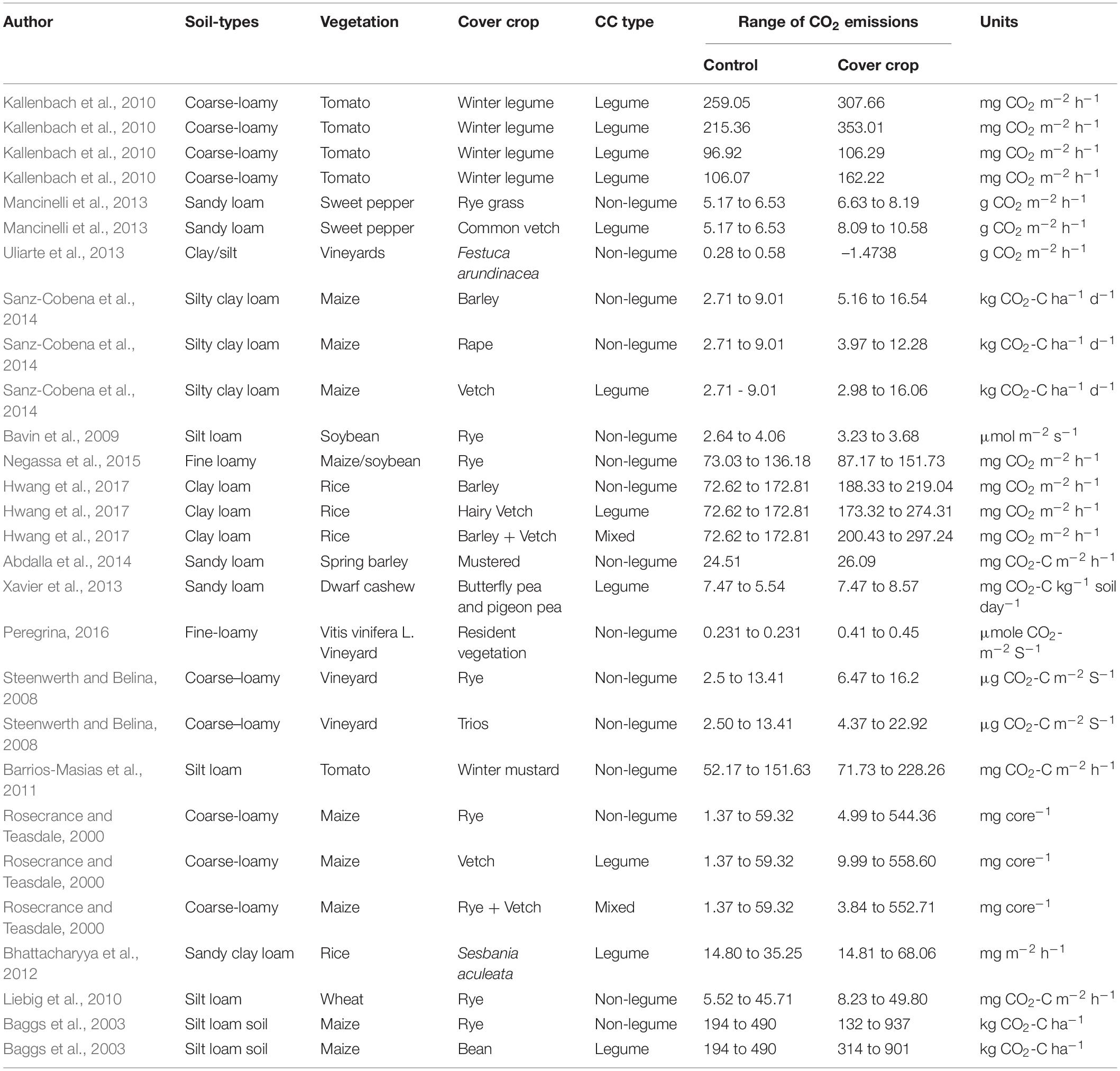
Table 1. Role of different cover crop types (legume, non-legume, and mixed) in soil carbon dioxide emissions (CO2).
Nitrous Oxide Emissions
Cover crop will minimize N losses from agricultural practices by minimizing both nitrate leaching and ammonia plus nitrous oxide transport to the atmosphere (Lacey and Armstrong, 2015). Nitrous oxide is the major contributor to global warming from the agricultural farming system (IPCC, 2007), which is released in soils primarily through two coupled microbial processes; nitrification in aerobic and denitrification in anaerobic environments (Bowen et al., 2018). The frequency and strength of these procedures are strongly influenced by the availability of soil mineral N, soluble C, water, oxygen, temperature, pH, and soil texture (Li et al., 2019). As changes in farming techniques directly affect substrate quality and the environmental conditions in the soil, they are expected to have an effect on N2O emissions (Abdalla et al., 2012). Steenwerth and Belina (2008) demonstrated that legume CCs might lead to greater N2O emissions, and much of this increase could be due to the decomposition of crop residues. The global impact of legume CCs during crop rotation on N2O emissions tends to be mostly neutral, although it may differ in relation to the quality (C:N ratios) of crop residues and management techniques (Muhammad et al., 2019). The seasonal N2O flux in barley cultivation did not differ from that in the NCCs (3.6 kg ha–1). However, LCCs (hairy vetch and/or barley + hairy vetch mixture) treatments increased seasonal N2O flux by 1.8 times than the barley treatment alone (Table 2).
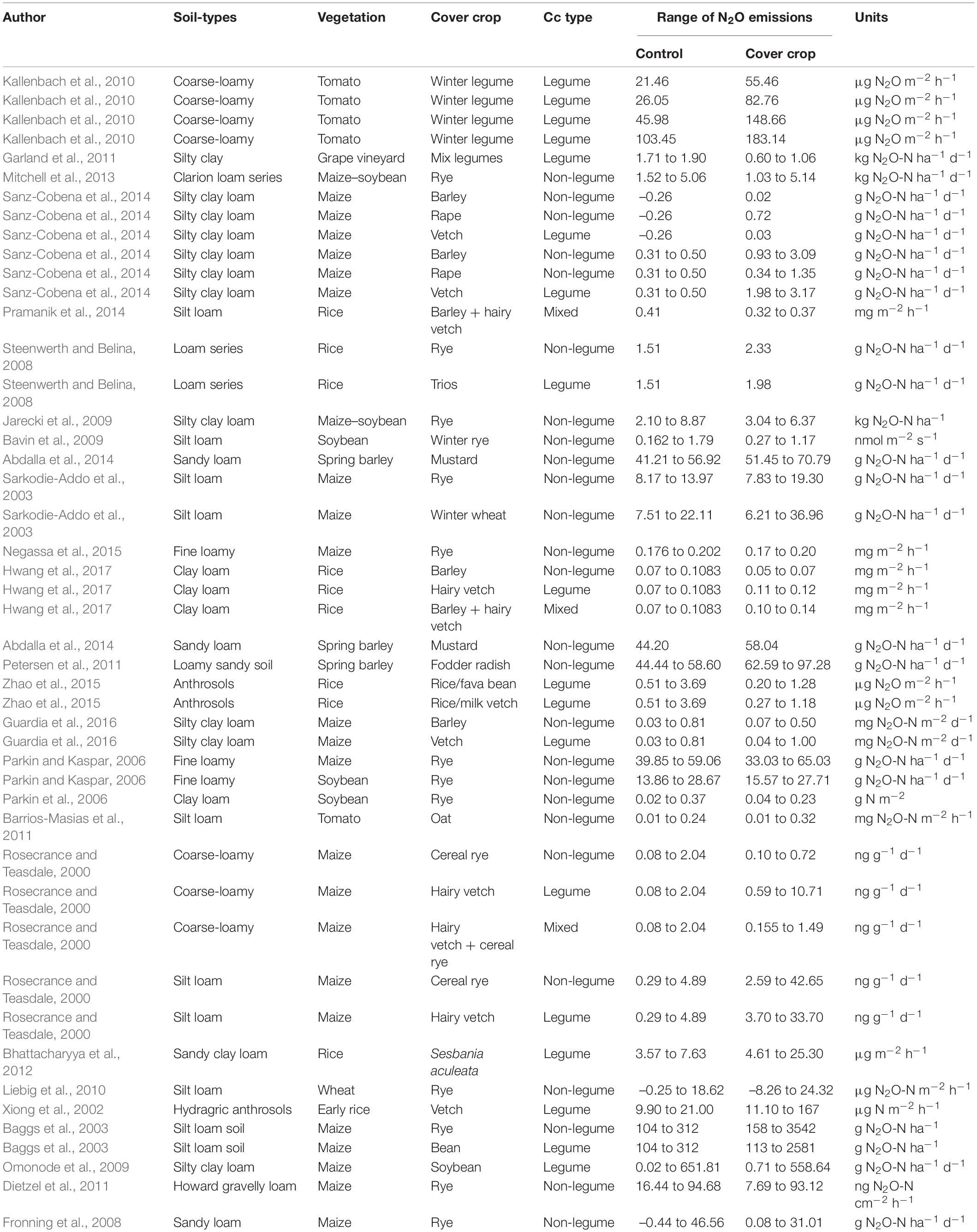
Table 2. Role of different cover crop types (legume, non-legume, and mixed) in nitrous oxide emissions (N2O).
Cover crops are most commonly used as catch crops to mitigate nitrates leaching during fall and winter periods (Plaza-Bonilla et al., 2015). Legume CCs alone or in combination with NLCCs as a GM provide additional N for the subsequent crop (Tribouillois et al., 2015). When compared to unfertilized plots, CCs had higher mean daily N2O emissions but lower yearly N2O emissions when compared to fertilized cropping systems. CC treatments had a two- to fourfold higher potential for nitrification, mineralization, and denitrification than conventional cultivation. Thus, CCs improved the soil capacity to support higher MBN, potential N mineralization, and microbiological functions of nitrification and denitrification. Kerri and Belina (2008) found that total soil C content was 40–50% higher in soils under five consecutive years of annual CCs than continuously cultivated soil. Cover crops also influence the quality of soil water by rising transpiration rate than bare soil. In general, existing studies show little effect of CCs (LCCs or NLCCs) on N2O emissions, particularly when results are integrated on the basis of residues quality and quantity (Muhammad et al., 2019). According to a meta-analysis, the effect of CCs on N2O emissions is mostly influenced by the residues C:N ratio, climatic condition, and residues management practices (Muhammad et al., 2019). Similarly, another meta-analysis demonstrated that the introduction of CCs residues into soil often leads to a short-term increase in N2O emissions, especially for LCCs (Basche et al., 2014).
Higher precipitations have a deleterious effect on N2O emissions from CCs fields. CC residue incorporation through tillage has a significant impact on soil structure, soil water dynamics, soil nutrients, and organic residues, which may have an impact on crop production (Gregorich et al., 2008; Negassa et al., 2015). Soil N2O is mainly produced by soil microorganisms through nitrification and denitrification. Similarly, farmland cultivation measures also affect the soil N2O emission process by affecting soil temperature, humidity, and nutrient status (Sanz-Cobena et al., 2012). A previous study reported that CCs mulching significantly reduces N2O emissions (Fiorini et al., 2020), whereas some researchers have found that soil N2O emissions significantly increased with CCs incorporation (Snapp and Borden, 2005; Tang et al., 2015). Compared to saturated moisture, higher soil moisture content (45–75%) had significantly higher N2O emissions. Nitrous oxide emissions increase with higher soil water content and gradually decrease after reaching saturated water content (Sheppard et al., 2013).
Methane Emissions
Cold-resistant legumes like Chinese milk vetch and hairy vetch and NLCCs like rye and barley could be the only option for winter CCs in temperate countries with cold and dry weather during winter seasons. Vetch is considered the most common GM in rice fields due to its high N-fixing capacity to adapt to harsh winter conditions and better growth in wet paddy soil. Hwang et al. (2017) demonstrated that hairy vetch and barley mixtures as GM are favored in rice paddy soil because of their higher biomass productivity than sole vetch or barley crops and stronger resistance to winter drought (Ahmad et al., 2022). The addition of NLCCs (barley or rye) would not efficiently increase rice production due to the high C:N ratio and slow mineralization process of crop residues. The combination of barley and hairy vetch therefore reduce the C:N ratio below 20, which support the mineralization of organic substrates in soil (Marton et al., 2014). During rice cultivation, CCs biomass application as GM increased soil C balance by 39–142% over NCCs and increased the seasonal net global warming potential by 3.2–5.7 times due to significantly higher CH4 emissions under flooded soil conditions.
As shown in Table 3, the CH4 emission ranges from 73.52–83.08 mg CH4 m–2 h–1 in milk vetch CCs, which is significantly higher than control (0.74–26 mg CH4 m–2 h–1), and rye CCs (1.11–80.14 mg CH4 m–2 h–1) in rice paddy soil (Sang et al., 2012). Most lowlands are under entirely irrigated or rain-fed conditions, and their cultivation serves as an important source of CH4 emissions (Zschornack et al., 2016). The average global CH4 emissions from rice paddy fields are reported to be around 11% of the overall anthropogenic CH4 emissions (Yan et al., 2009). It was stated previously that the production of rice, which was 473 million tons in 1990, needs to be increased by 39.43% in 2020 to meet the world population food demand and that anthropogenic CH4 emissions have been raised by 40–50% (Lee et al., 2010). Since CH4 is primarily produced under strictly anaerobic conditions, it might be because of the decay of organic matter through archaeal methanogens (Chandio et al., 2020). Similarly, the introduction of organic materials such as GM into flooded rice fields will promote CH4 emissions by methanogens with readily available C. Agricultural Mediterranean soils produce significant CH4 emissions through methanogens in flooded crops (e.g., rice), which represent 6% of the total agricultural production (Sanz-Cobena et al., 2017). Methane emissions mainly depend on residue incorporation, and the CH4 emissions increase from rice paddy soil by 100–500 kg CH4 ha–1 year–1 when the straw is added at the rate of 0–7 tha–1 (Sanchis et al., 2012). Improved crop production approaches are likely to clash with the extenuation of CH4 emissions (Yan et al., 2009).
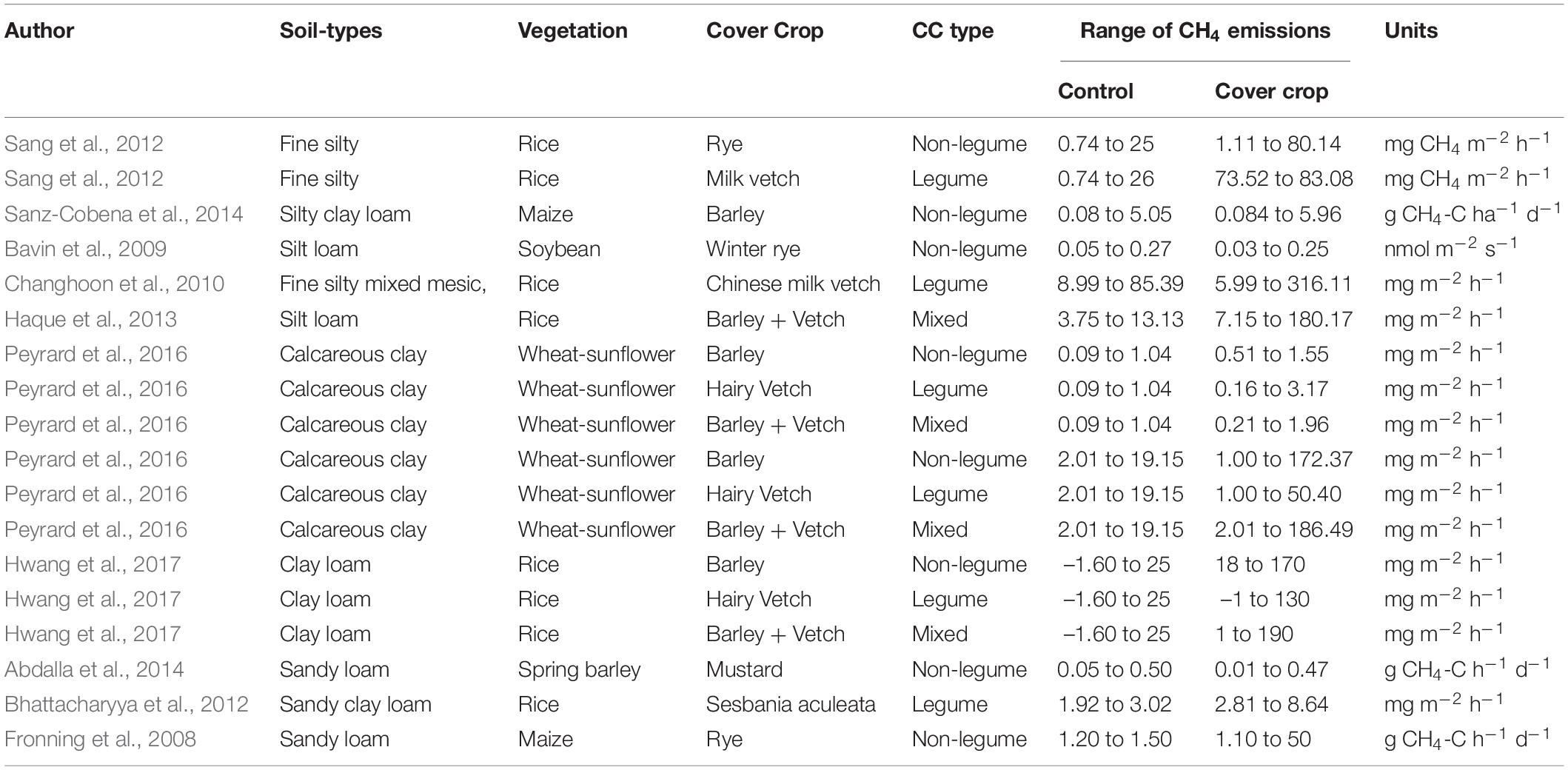
Table 3. Role of different cover crop types (legume, non-legume, and mixed) in soil methane emissions (CH4).
Residues C:N Ratios and Greenhouse Gas Emission
Cover crop residue C:N ratios have a direct impact on soil GHG (CO2, N2O, and CH4) emissions. Results showed that residues with a high C:N ratio significantly increase CO2 and CH4 fluxes while decreasing N2O flux. These results are in line with the finding of earlier researchers (Muhammad et al., 2019), who documented that CO2 emissions are positively and N2O emissions are negatively affected by increasing the residues C:N ratios. Huang et al. (2002) reported that seasonal N2O emission from wheat-cultivated soil was negatively correlated with increasing soil C:N ratio. The N2O/NO3– ratio and N2O emission rate increased with decreasing C:N ratio in organic amendments in a well-aerated soil (Melling et al., 2007). Reddy and Crohn (2014) demonstrated that the rate of N2O production is partially controlled by C susceptible through the mineralization process. Compared with wheat monoculture, wheat-chickpea crop rotation showed a C sequestration rate of 0.53 Mg C ha–1 y–1 during 20 years (López-Bellido et al., 2010), which could be related to the fluxes of CO2. Huang et al. (2004) found that the root decay content was strongly negatively correlated with increasing the root residue C:N ratio. Similarly, Khalil et al. (2002) reported that N2O production was increased by decreasing the C:N ratio of different organic materials. In addition, Kato et al. (2011) found a strongly negative correlation between the mean annual N2O emission and C:N ratio of tropical rain forest soils. Residues with lower C:N decomposed more rapidly, might provide a greater opportunity for producing more dissolved organic C, hence resulting in higher N2O emissions.
Low C:N Ratio Residues
Cover crops develop resistance against pests, weeds, and potential environmental degradation, improve soil quality, and thus increase the production of subsequent cash crops (Neely et al., 2018). Leguminous CCs release N into the soil and thus enhance crop yield (Holman et al., 2018). Lentil (Lens culinaris Medik), field pea (Pisum sativum L.), and faba bean (Vicia faba L.) were used as LCCs for potential N fixation in rotation with cash crops. The biennial clover (Melilotus officinalis L.) has advantages over annual legumes because of its low seed costs, a strong competitor to weeds, and high productivity in biomass production and N fixation in semi-arid environments (Ravenel et al., 2015). Intercropping LCCs with winter cereals may have fixed atmospheric N2, but after winter cereal harvest, they may show maximum growth and N fixation. Cover crops such as alfalfa (Medicago sativa L.) and red clover (Trifolium pratense L.) had higher production of biomass and added N to winter wheat crops due to their faster decomposition rate (Chen et al., 2006). The research revealed that alfalfa and red clover as relay crops and lentil and chickling vetch (Lathyrus sativus L.) as double crops were productive legumes in a cereal cropping system in winter (Martens and Entz, 2001). It has been shown that alfalfa and red clover can fix significant amounts of N without adversely affecting the winter wheat yield in moderate to high rainfall regions (Martens and Entz, 2001), while the incorporation of these crops into the soil increases soil health due to mineralization through soil microorganisms (Li et al., 2019). Similarly, alfalfa and red clover showed potential for N fixation in moderate to high rainfall areas without adversely affecting the yield of winter wheat (Martens et al., 2001).
High C:N Ratio Residue
The CCs are selected based on N-fixing capacity, biomass quality (C:N ratios), and quantities in many agricultural systems. However, there is insufficient evidence to suggest that crops increase soil fertility, crop yield, and soil microbial populations (Blesh, 2018). Non-legume CCs are also the best choice for spring forage because of their regrowth behavior during the growth stage as compared to small grain cereals (White and Weil, 2010). CCs during the following seasons decrease the soil water and wind erosion due to covering soil surface (Kaspar et al., 2001), and rye CCs help to sustain higher SOM (Kaspar et al., 2006). Researchers proposed that CCs decrease soil bulk density and increase water retention potential, soil microbial processes, and porosity of soils (Villamil et al., 2006). However, other researchers reported that CCs have either improved or have no effect on soil properties (Mupambwa, 2012; Mupambwa and Wakindiki, 2012). Soil response to agronomic methods is also influenced by soil and environmental conditions (Bescansa et al., 2006) in addition to CCs management. The introduction of rye CCs conserved soil resources and had environmental benefits immediately and long-term economic benefits during the cropping system (Igos et al., 2016).
Optimum C:N Ratio Residues or Mixed Residues
Mixed CCs are an important management method used extensively to increase SOM and subsequently increase cash crop productivity (Sainju and Singh, 2008). Growing CCs can provide physical protection to the soil by reducing the impact of rainfall and also improve soil structure and aggregation (Tang et al., 2017), and similarly improve soil microorganisms (Araujo et al., 2019). Morales Salmeron et al. (2019) found that soil structure and fertility are improved by the presence of legumes and legume-grain mixtures in row crop systems. In addition, the residues of the main crop rotated with a potato crop are supposed to affect the accumulation of soil N and C over time. Planting CCs mixtures (multi-species) could be a viable solution to enhance the ecological stability, microbial diversity, and resilience of CCs communities, which contribute to higher and consistent productivity. The production benefits of multi-species plant communities include the potential for increased efficiency in resource use and crop yields (Tosti et al., 2012).
Termination Method to Mitigate Greenhouse Gas Emissions
The goal of cultivation and the availability of machinery are the two main factors that mitigate GHG emissions. The CCs are terminated through incorporation, surface mulch, and/or removal, as well as using herbicide for killing or improving the productivity of the following cash crop. The influence of CCs variety, termination timing, and termination method on mulch, weed cover, and soil nitrate in organic systems plays a vital role in GHG emissions.
Residues Incorporation
A traditional termination method involves using a fixed machine-driven disk to NCCs and CCs plot (Figure 7A) to incorporate residues into the soil (Jani et al., 2016; Rojas et al., 2018). Termination of CCs through disking results in rapid residue decomposition and release of nutrients due to close contact with soil microorganisms. As a result, microbial decomposition has been facilitated by better residue soil interaction and higher levels of soil oxygen. Cover crop incorporating through disking improved the total bacteria population while decreasing or stabilizing the populations of fungi and actinomycetes (Elfstrand et al., 2007). The incorporation of barley and rape CCs residue increased the soil respiration by 21 and 28%, respectively. The CH4 emissions were decreased with CCs incorporation with mean values of –0.12 and –0.10 kg CH4–C ha–1 for plots with and without CCs, respectively (Table 3; Sanz-Cobena et al., 2014). Fungi abundance and reduction in the disked soils could be due to high lignin materials, increased soil disturbance, and reduced soil moisture (Elfstrand et al., 2007). Incorporating CCs residue into the soil increased CO2 and N2O emissions compared to residue placed on the soil surface because of increased contact with soil microorganisms.
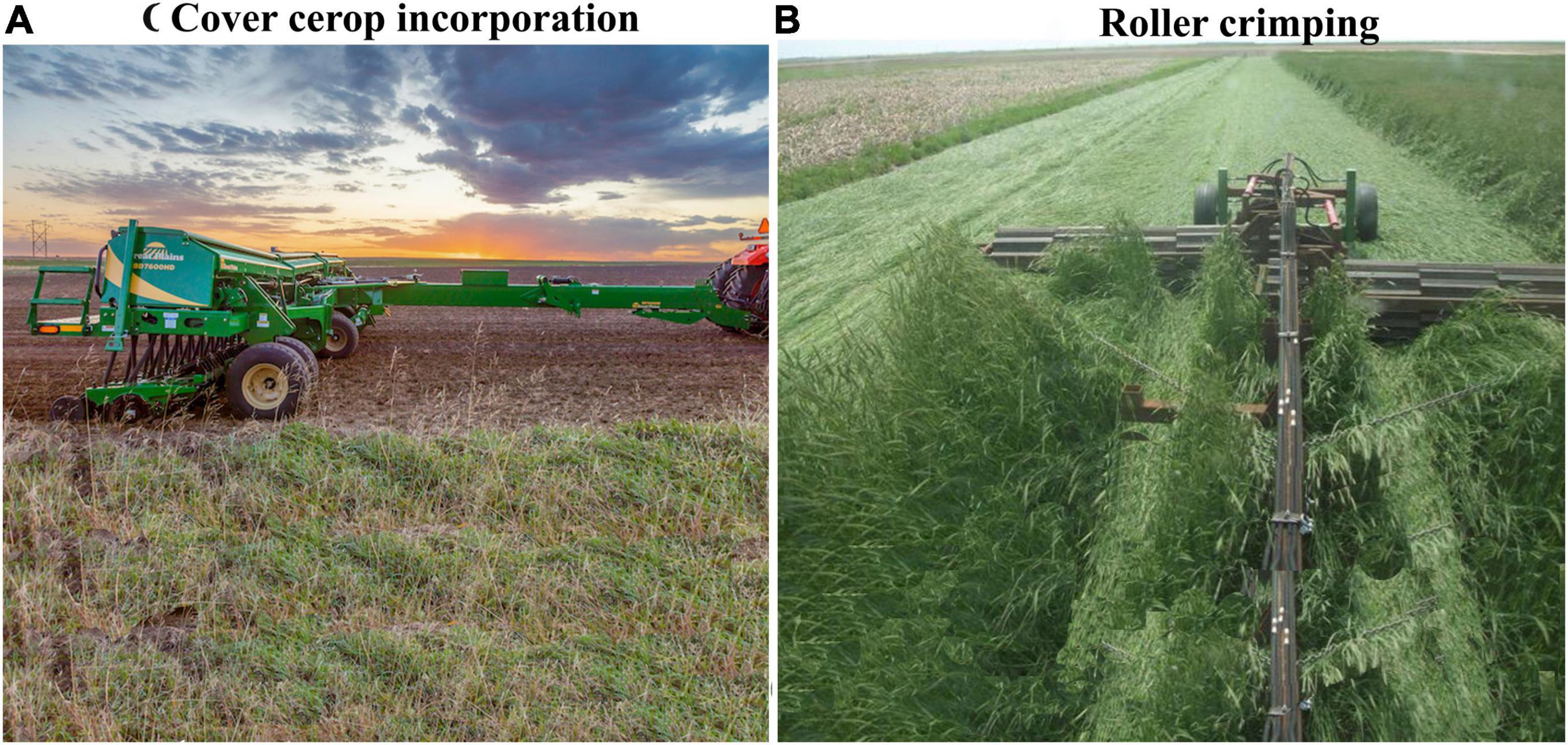
Figure 7. (A,B) Examples of cover cropping termination methods (incorporation, mulching, and removing), and the concept of cover crop residues biomass and soil microbes interaction.
Tillage practices expose more residue to microorganisms, which enhances aeration and microbial activity, resulting in higher CO2 and N2O emissions (Alluvione et al., 2010). Several studies have found that incorporating CC residues into the soil increases N2O emissions when compared to surface placement (Xiong et al., 2002; Basche et al., 2014; Muhammad et al., 2021b). Further research is needed to examine the impact of disking on CC residues breakdown, retention of soil water content, OM, dynamics of N releases, and subsequent cash crops. CCs with a high C:N ratio, such as rye, stimulated more CH4 emissions than CCs with a low C:N ratio per grain yield, but LCCs vetch was more effective in improving the rice growth and yield (Table 3). To improve soil properties and rice productivity while limiting CH4 release, it may be more beneficial to use a low C:N ratio, such as milk vetch, as a CC than a high C:N ratio, such as rye (Sang et al., 2012).
Residues Mulching
Using a rotary or flail mower to terminate CCs cuts the residue into slices and shreds the residue on the soil surface (Rose et al., 2016). In early fall, the soil microbial biomass was 150 mg C kg–1 soil, which is 50% higher than in late spring (100 mg C kg–1 soil; Zibilske and Makus, 2009). Mowing rye at the vegetative growth stage resulted in regrowth, which eventually resulted in water and nutrient competition with subsequent cash crops (Kornecki et al., 2009). The termination of CCs with rotating mowers can be difficult due to the irregular size and dispersal of plant remains. Compared to the arable weed community present in NCCs plots, LCCs increased total PLFA concentration by 5.37 nmol g–1 and 10.20 nmol g–1 in the fall and spring (Finney et al., 2017). Hairy vetch was positively associated with non-AMF (Vicia villosa L.). The associations between CCs and microbial groups were found in monocultures and in multispecies CC mixtures (Finney et al., 2017). In comparison to CCs incorporation, CCs termination with a flail mower may be important in controlling soil nutrient transformations and SOM maintenance in subtropical climates, which regulate soil temperature and moisture at more suitable levels for microbial activity and residue decomposition (Zibilske and Makus, 2009; Khan et al., 2021). Moreover, another study found that flail-mowing is difficult to achieve uniform mulching, allowing weeds to emerge via thin openings (Teasdale et al., 2007; Wegner et al., 2018).
Mechanical mowing of rye, mustard, and hairy vetch mixture improved N mineralization. Compared to the NCCs treatment, white mustard (Sinapis alba L.), Lacy phacelia (Phacelia tanacetifolia Benth. LP), and hairy vetch (Vicia villosa Roth) mulching increased microbial biomass C by 38, 80, and 44%, respectively. These increases could be due to the increase in soil C and N by 19 and 44% in white mustard, 6 and 2% in Lacy phacelia, and 10 and 13% in hairy vetch, respectively, when compared to NCCs (Marinari et al., 2015). Several studies have found that incorporating CC residues increases N2O emissions when compared to surface placement (Basche et al., 2014; Muhammad et al., 2019). The CCs residue removal had no effect on N2O emissions when compared to NCCs but resulted in higher CO2 emissions because of CCs roots in the soil that were not removed. Only the above-ground biomass of CCs was removed from the soil for animal forage in the residue removal treatment.
Timing is the most crucial factor when operating a roller-crimper for CCs termination. Termination of grass CCs should occur once flowering has begun (Figure 7B), while LCCs should be terminated after pods formation. The CCs will not be terminated successfully if it is performed too late (McMechan et al., 2021; Werle et al., 2021). The use of a roller-crimper is one of the best ways to suppress weeds with CCs residue (Mirsky et al., 2009). To avoid competing with cash crops for water and nutrients, effective termination of CCs with a roller-crimper is critical (Bavougian et al., 2019). It has been demonstrated that crimping LCCs with roller crimped produce 10 to 217 kg N ha–1 reliant on CCs species and killing times (Parr et al., 2014), and soil inorganic N peaks after 4 to 6 weeks of roller crimping (Parr et al., 2014). Further study is required to discover how mulching and removing the CCs termination method affects soil C, N release, and soil microbial diversity in agricultural ecosystems.
Residue Removal With Herbicide Application
Non-selective herbicides are commonly used for terminating the CCs because they are effective at all growth phases (Clark et al., 2007). These have low application costs and the ability to terminate CCs on a large scale within a short period of time (Hay et al., 2019; Farooq et al., 2022). As the role of herbicides in non-target species has increased, along with their negative impact on these species, there is an increased desire to learn about their impact on plant nutrition and nutrient cycling (Cornelius and Bradley, 2017). Previous studies have demonstrated that glyphosate has no impact on soil microorganisms diversity and activity (Weaver et al., 2007; Lane et al., 2012), although, after one week of glyphosate treatment, a significant drop in total microbial biomass in soybean rhizosphere soil was detected (Lane et al., 2012). Selection of herbicide with residual soil activity when planning to use CCs again in the fall must be done carefully to avoid impacts on CCs species establishment. Glyphosate should be applied when temperatures reach 55°F during the day and 40°F during the night. Applying glyphosate before the boot stage will help to improve effective cereal rye. Improved spray coverage will increase the efficacy of contact herbicides such as paraquat (Gramoxone) and glufosinate (Liberty, Cheetah, and Scout; Werle et al., 2021). During the spring thaw until the end of rye, CO2 emissions were higher in green fallow than in chemical fallow (P = 0.0071). The atmospheric CH4 uptake was the dominant exchange process, and it was significantly (P = 0.0124) higher under chemical fallow (2.7 g CH4-C ha–1 d–1) than under green fallow (1.5 g CH4-C ha–1 d–1). Cumulative CO2, CH4, and N2O emissions did not differ between the chemical and green-fallow phases (P = 0.1293, 0.2629, and 0.9979, respectively) during the 19-month period (Liebig et al., 2010).
Conclusion
The effect of CC types, biomass, and residue C:N ratios on soil GHGs emissions, soil microbial biomass, and community was investigated in this study. Compared to NCCs, the CCs increased microbial biomass and community abundance due to additional organic matter input. A higher fungi/bacteria ratio with CCs suggests that CCs have a greater impact on fungi than bacteria. The LCCs had lower actinomycete levels but a higher MBC/MBN ratio than the NLCCs. The benefits of CCs on soil microbial biomass were reduced when mixed LCCs and NLCCs were used instead of LCCs or NLCCs alone. Carbon dioxide and N2O emissions vary according to CCs species, biomass residue quality and quantity, and method of residue placement in the soil. When compared to NCCs, CCs increased CO2 emissions. Legume CCs emitted more N2O than NLCCs or mixed CCs. Increased CCs biomass resulted in higher CO2 emissions but lower N2O emissions. The increases in N2O emissions can cause changes in global warming potential, thus affecting the C sinks (soil organic C input via plants) and losses (CO2 emissions/mineralization). The LCC and NLCCs combined application as CCs reduced the GHG emissions and improved soil health and crop yields. Although CCs increase CO2 emissions compared to NCCs, they have positive effects on soil and C sequestration and other known soil health and environmental quality parameters. Further studies are needed to clarify the effect of CCs biomass rates and residues quality (C:N ratios) on soil microbial community structure and abundance and their influence on soil GHG emissions.
Author Contributions
IM, XZ, and JW did the conceptualization. IM and SAh performed the methodology. IM and SF investigated the data. XZ carried out the resources. IM carried out the data curation and wrote the original draft of the manuscript. SAl, XZ, and JW wrote, reviewed, and edited the manuscript. XZ supervised the data. All authors have read and agreed to the published version of the manuscript.
Funding
This study was supported by the National Natural Science Foundation of China (31760354), the Natural Science Foundation of Guangxi (2019GXNSFAA185028), Special Fund for Guangxi Innovation Team Construction of National Modern Agricultural Industrial Technology System (nycytxgxcxtd-2021-04-04), CAS: “Light of West China” Program for introducing talent in the west, the National Natural Science Foundation of China (Grant Nos. 31570440 and 31270484), and the Key International Scientific and Technological Cooperation and Exchange Project of Shaanxi Province China (2020KWZ-010).
Conflict of Interest
The authors declare that the research was conducted in the absence of any commercial or financial relationships that could be construed as a potential conflict of interest.
Publisher’s Note
All claims expressed in this article are solely those of the authors and do not necessarily represent those of their affiliated organizations, or those of the publisher, the editors and the reviewers. Any product that may be evaluated in this article, or claim that may be made by its manufacturer, is not guaranteed or endorsed by the publisher.
References
Abdalla, M., Hastings, A., Cheng, K., Yue, Q., Chadwick, D., Espenberg, M., et al. (2019). A critical review of the impacts of cover crops on nitrogen leaching, net greenhouse gas balance and crop productivity. Glob. Change Biol. 25, 2530–2543. doi: 10.1111/gcb.14644
Abdalla, M., Hastings, A., Helmy, M., Prescher, A., Osborne, B., Lanigan, G., et al. (2014). Assessing the combined use of reduced tillage and cover crops for mitigating greenhouse gas emissions from arable ecosystem. Geoderma 223–225, 9–20.
Abdalla, M., Rueangritsarakul, K., Jones, M., Osborne, B., Helmy, M., Roth, B., et al. (2012). How effective is reduced tillage-cover crop management in reducing N2O fluxes from arable crop soils? Water Air Soil Pollut. 223, 5155–5174. doi: 10.1007/s11270-012-1268-4
Ackroyd, V. J., Cavigelli, M. A., Spargo, J. T., Davis, B., Garst, G., and Mirsky, S. B. (2019). Legume cover crops reduce poultry litter application requirements in organic systems. Agron. J. 111, 2361–2369. doi: 10.2134/agronj2018.09.0622
Ahmad, S., Wang, G. Y., Muhammad, I., Chi, Y. X., Zeeshan, M., Nasar, J., et al. (2022). Interactive effects of melatonin and nitrogen improve drought tolerance of maize seedlings by regulating growth and physiochemical attributes. Antioxidants 11:359. doi: 10.3390/antiox11020359
Aita, C., and Giacomini, S. J. (2003). Crop residue decomposition and nitrogen release in single and mixed cover crops. Rev. Bras. Cienc. Solo 27, 601–612.
Alahmad, A., Decocq, G., Spicher, F., Kheirbeik, L., Kobaissi, A., Tetu, T., et al. (2019). Cover crops in arable lands increase functional complementarity and redundancy of bacterial communities. J. Appl. Ecol. 56, 651–664. doi: 10.1111/1365-2664.13307
Alfonso Gomez, J., Campos, M., Guzman, G., Castillo-Llanque, F., Vanwalleghem, T., Lora, A., et al. (2018). Soil erosion control, plant diversity, and arthropod communities under heterogeneous cover crops in an olive orchard. Environ. Sci. Pollut. Res. 25, 977–989. doi: 10.1007/s11356-016-8339-9
Alluvione, F., Bertora, C., Zavattaro, L., and Grignani, C. (2010). Nitrous oxide and carbon dioxide emissions following green manure and compost fertilization in corn. Soil Sci. Soc. Am. J. 74, 384–395. doi: 10.2136/sssaj2009.0092
Araujo, F. S., Barroso, J. R., Freitas, L. D. O., Teodoro, M. S., De Souza, Z. M., and Torres, J. L. R. (2019). Chemical attributes and microbial activity of soil cultivated with cassava under different cover crops. Rev. Bras. Engenharia Agricola E Ambient. 23, 614–619. doi: 10.1590/1807-1929/agriambi.v23n8p614-619
Austin, E. E., Wickings, K., Mcdaniel, M. D., Robertson, G. P., and Grandy, A. S. (2017). Cover crop root contributions to soil carbon in a no-till corn bioenergy cropping system. Glob. Change Biol. Bioenergy 9, 1252–1263.
Bacq-Labreuil, A., Crawford, J., Mooney, S. J., Neal, A. L., and Ritz, K. (2019). Cover crop species have contrasting influence upon soil structural genesis and microbial community phenotype. Sci. Rep. 9:7473. doi: 10.1038/s41598-019-43937-6
Baggs, E., Stevenson, M., Pihlatie, M., Regar, A., Cook, H., and Cadisch, G. (2003). Nitrous oxide emissions following application of residues and fertiliser under zero and conventional tillage. Plant Soil 254, 361–370.
Baggs, E. M., Watson, C. A., and Rees, R. M. (2000). The fate of nitrogen from incorporated cover crop and green manure residues. Nutr. Cycl. Agroecosyst. 56, 153–163.
Bagley, J. E., Desai, A. R., Dirmeyer, P. A., and Foley, J. A. (2012). Effects of land cover change on moisture availability and potential crop yield in the world’s breadbaskets. Environ. Res. Lett. 7:014009. doi: 10.1088/1748-9326/7/1/014009
Bair, K. E., Davenport, J. R., and Stevens, R. G. (2008). Release of available nitrogen after incorporation of a legume cover crop in concord grape. Hortscience 43, 875–880. doi: 10.21273/hortsci.43.3.875
Barrios-Masias, F. H., Cantwell, M. I., and Jackson, L. E. (2011). Cultivar mixtures of processing tomato in an organic agroecosystem. Organ. Agric. 1, 17–30.
Barros, D. L., Oliveira Gomide, P. H., and De Carvalho, G. J. (2013). Cover crops and their effects on succession culture. Biosci. J. 29, 308–318.
Basche, A. D., Miguez, F. E., Kaspar, T. C., and Castellano, M. J. (2014). Do cover crops increase or decrease nitrous oxide emissions? A meta-analysis. J. Soil Water Conserv. 69, 471–482. doi: 10.2489/jswc.69.6.471
Bavin, T. K., Griffis, T. J., Baker, J. M., and Venterea, R. T. (2009). Impact of reduced tillage and cover cropping on the greenhouse gas budget of a maize/soybean rotation ecosystem. Agric. Ecosyst. Environ. 134, 234–242.
Bavougian, C. M., Sarno, E., Knezevic, S., and Shapiro, C. A. (2019). Cover crop species and termination method effects on organic maize and soybean. Biol. Agric. Hortic. 35, 1–20. doi: 10.1093/ee/nvx149
Bayer, C., Gomes, J., Zanatta, J. A., Beber Vieira, F. C., and Dieckow, J. (2016). Mitigating greenhouse gas emissions from a subtropical Ultisol by using long-term no-tillage in combination with legume cover crops. Soil Tillage Res. 161, 86–94. doi: 10.1016/j.still.2016.03.011
Behnke, G. D., and Villamil, M. B. (2019). Cover crop rotations affect greenhouse gas emissions and crop production in Illinois, USA. Field Crops Res. 241:107580. doi: 10.1016/j.fcr.2019.107580
Berlanas, C., Andres-Sodupe, M., Lopez-Manzanares, B., Mercedes Maldonado-Gonzalez, M., and Gramaje, D. (2018). Effect of white mustard cover crop residue, soil chemical fumigation and Trichoderma spp. root treatment on black-foot disease control in grapevine. Pest Manage. Sci. 74, 2864–2873. doi: 10.1002/ps.5078
Bescansa, P., Imaz, M., Virto, I., Enrique, A., and Hoogmoed, W. (2006). Soil water retention as affected by tillage and residue management in semiarid Spain. Soil Tillage Res. 87, 19–27. doi: 10.1016/j.still.2005.02.028
Bhattacharyya, P., Neogi, S., Chakravorti, S. P., Behera, K. S., Das, K. M., Bardhan, S., et al. (2012). Effect of long-term application of organic amendment on C storage in relation to global warming potential and biological activities in tropical flooded soil planted to rice. Nutr. Cycl. Agroecosyst. 94, 273–285.
Blanco-Canqui, H., and Jasa, P. J. (2019). Do grass and legume cover crops improve soil properties in the long term? Soil Sci. Soc. Am. J. 83, 1181–1187. doi: 10.2136/sssaj2019.02.0055
Blesh, J. (2018). Functional traits in cover crop mixtures: biological nitrogen fixation and multifunctionality. J. Appl. Ecol. 55, 38–48. doi: 10.1111/1365-2664.13011
Bowen, H., Maul, J. E., Poffenbarger, H., Mirsky, S., Cavigelli, M., and Yarwood, S. (2018). Spatial patterns of microbial denitrification genes change in response to poultry litter placement and cover crop species in an agricultural soil. Biol. Fertil. Soils 54, 769–781. doi: 10.1007/s00374-018-1301-x
Bowles, T. M., Jackson, L. E., Loeher, M., and Cavagnaro, T. R. (2017). Ecological intensification and arbuscular mycorrhizas: a meta-analysis of tillage and cover crop effects. J. Appl. Ecol. 54, 1785–1793. doi: 10.1111/1365-2664.12815
Briar, S. S., Fonte, S. J., Park, I., Six, J., Scow, K., and Ferris, H. (2011). The distribution of nematodes and soil microbial communities across soil aggregate fractions and farm management systems. Soil Biol. Biochem. 43, 905–914. doi: 10.1016/j.soilbio.2010.12.017
Brito, I., Goss, M., and De Carvalho, M. (2012). Effect of tillage and crop on arbuscular mycorrhiza colonization of winter wheat and triticale under Mediterranean conditions. Soil Use Manage. 28, 202–208. doi: 10.1111/j.1475-2743.2012.00404.x
Brooks, J. P., Tewolde, H., Adeli, A., Shankle, M. W., Way, T. R., Smith, R. K., et al. (2018). Effects of subsurface banding and broadcast of poultry litter and cover crop on soil microbial populations. J. Environ. Qual. 47, 427–435. doi: 10.2134/jeq2017.09.0382
Buechi, L., Gebhard, C.-A., Liebisch, F., Sinaj, S., Ramseier, H., and Charles, R. (2015). Accumulation of biologically fixed nitrogen by legumes cultivated as cover crops in Switzerland. Plant Soil 393, 163–175. doi: 10.1007/s11104-015-2476-7
Buyer, J. S., Baligar, V. C., He, Z., and Arevalo-Gardini, E. (2017). Soil microbial communities under cacao agroforestry and cover crop systems in Peru. Appl. Soil Ecol. 120, 273–280. doi: 10.1016/j.apsoil.2017.09.009
Cagnini, C. Z., Garcia, D. M., Silva, N. D. S., Macedo, E. C., Huelse, De Souza, S. G., et al. (2019). Cover crop and deep tillage on sandstone soil structure and microbial biomass. Arch. Agron. Soil Sci. 65, 980–993. doi: 10.1080/03650340.2018.1542684
Calderón, F. J., Nielsen, D., Acosta-Martínez, V., Vigil, M. F., and Lyon, D. (2016). Cover crop and irrigation effects on soil microbial communities and enzymes in semiarid agroecosystems of the central great plains of North America. Pedosphere 26, 192–205. doi: 10.1016/s1002-0160(15)60034-0
Campiglia, E., Paolini, R., Colla, G., and Mancinelli, R. (2009). The effects of cover cropping on yield and weed control of potato in a transitional system. Field Crops Res. 112, 16–23. doi: 10.1016/j.fcr.2009.01.010
Carney, K. M., and Matson, P. A. (2006). The influence of tropical plant diversity and composition on soil microbial communities. Microb. Ecol. 52, 226–238. doi: 10.1007/s00248-006-9115-z
Cavalca, L., Corsini, A., Zaccheo, P., Andreoni, V., and Muyzer, G. (2013). Microbial transformations of arsenic: perspectives for biological removal of arsenic from water. Future Microbiol. 8, 753–768. doi: 10.2217/fmb.13.38
Chalise, K. S., Singh, S., Wegner, B. R., Kumar, S., Perez-Gutierrez, J. D., Osborne, S. L., et al. (2019). Cover crops and returning residue impact on soil organic carbon, bulk density, penetration resistance, water retention, infiltration, and soybean yield. Agron. J.111, 99–108. doi: 10.2134/agronj2018.03.0213
Chandio, A. A., Magsi, H., and Ozturk, I. (2020). Examining the effects of climate change on rice production: case study of Pakistan. Environ. Sci. Pollut. Res. 27, 7812–7822. doi: 10.1007/s11356-019-07486-9
Changhoon, L., Kido, P., Kiyuol, J., Muhammadaslam, A., Dokyoung, L., Jessie, G., et al. (2010). Effect of Chinese milk vetch (Astragalus sinicus L.) as a green manure on rice productivity and methane emission in paddy soil. Agric. Ecosyst. Environ. 138, 343–347.
Chavarria, D. N., Verdenelli, R. A., Serri, D. L., Restovich, S. B., Andriulo, A. E., Meriles, J. M., et al. (2016). Effect of cover crops on microbial community structure and related enzyme activities and macronutrient availability. Eur. J. Soil Biol. 76, 74–82. doi: 10.1016/j.ejsobi.2016.07.002
Chen, S., Wyse, D. L., Johnson, G. A., Porter, P. M., Haar, M. J., Stetina, S. R., et al. (2006). Effect of cover crops alfalfa, red clover, perennial ryegrass, and rye on soybean cyst nematode population and soybean and corn yields in Minnesota. J. Nematol. 38, 267–267.
Chirinda, N., Olesen, J. E., Porter, J. R., and Schjønning, P. (2010). Soil properties, crop production and greenhouse gas emissions from organic and inorganic fertilizer-based arable cropping systems. Agric. Ecosyst. Environ. 139, 584–594. doi: 10.1016/j.agee.2010.10.001
Clark, A. J., Meisinger, J. J., Decker, A. M., and Mulford, F. R. (2007). Effects of a grass-selective herbicide in a vetch-rye cover crop system on corn grain yield and soil moisture. Agron. J.99, 43–48. doi: 10.2134/agronj2005.0362
Cornelius, C. D., and Bradley, K. W. (2017). Herbicide programs for the termination of various cover crop Species. Weed Technol. 31, 514–522. doi: 10.1017/wet.2017.20
Couedel, A., Alletto, L., Kirkegaard, J., and Justes, E. (2018a). Crucifer glucosinolate production in legume-crucifer cover crop mixtures. Eur. J. Agron. 96, 22–33. doi: 10.1016/j.eja.2018.02.007
Couedel, A., Alletto, L., Tribouillois, H., and Justes, E. (2018b). Cover crop crucifer-legume mixtures provide effective nitrate catch crop and nitrogen green manure ecosystem services. Agric. Ecosyst. Environ. 254, 50–59. doi: 10.1016/j.agee.2017.11.017
Dassen, S., Cortois, R., Martens, H., De Hollander, M., Kowalchuk, G. A., Van Der Putten, W. H., et al. (2017). Differential responses of soil bacteria, fungi, archaea and protists to plant species richness and plant functional group identity. Mol. Ecol. 26, 4085–4098. doi: 10.1111/mec.14175
De Almeida Acosta, J. A., Carneiro Amado, T. J., Da Silva, L. S., Santi, A., and Weber, M. A. (2014). Fitomass decomposition and nitrogen release of cover crops in function of the level of residue input to soil under no-tillage system. Cienc. Rural 44, 801–809.
Deguchi, S., Uozumi, S., Touno, E., Kaneko, M., and Tawaraya, K. (2012). Arbuscular mycorrhizal colonization increases phosphorus uptake and growth of corn in a white clover living mulch system. Soil Sci. Plant Nutr. 58, 169–172. doi: 10.1080/00380768.2012.662697
Dhima, K. V., Vasilakoglou, I. B., Eleftherohorinos, I. G., and Lithourgidis, A. S. (2006). Allelopathic potential of winter cereal cover crop mulches on grass weed suppression and sugarbeet development. Crop Sci. 46, 1682–1691. doi: 10.2135/cropsci2005.09-0311
Dietzel, R., Wolfe, D., and Thies, J. E. (2011). The influence of winter soil cover on spring nitrous oxide emissions from an agricultural soil. Soil Biol. Biochem. 43, 1989–1991.
Dinnes, D. L., Karlen, D. L., Jaynes, D. B., Kaspar, T. C., Hatfield, J. L., Colvin, T. S., et al. (2002). Nitrogen management strategies to reduce nitrate leaching in tile-drained Midwestern soils. Agron. J. 94, 153–171. doi: 10.2134/agronj2002.0153
Dorn, B., Jossi, W., and Van Der Heijden, M. G. A. (2015). Weed suppression by cover crops: comparative on-farm experiments under integrated and organic conservation tillage. Weed Res. 55, 586–597. doi: 10.1111/wre.12175
Elfstrand, S., BåTh, B., and MåRtensson, A. (2007). Influence of various forms of green manure amendment on soil microbial community composition, enzyme activity and nutrient levels in leek. Appl. Soil Ecol. 36, 70–82. doi: 10.1016/j.apsoil.2006.11.001
Farooq, S., Wu, H., Nie, J., Ahmad, S., Muhammad, I., Zeeshan, M., et al. (2022). Application, advancement and green aspects of magnetic molecularly imprinted polymers in pesticide residue detection. Sci. Total Environ. 804:150293. doi: 10.1016/j.scitotenv.2021.150293
Fernandez, A. L., Sheaffer, C. C., Wyse, D. L., Staley, C., Gould, T. J., and Sadowsky, M. J. (2016). Structure of bacterial communities in soil following cover crop and organic fertilizer incorporation. Appl. Microbiol. Biotechnol. 100, 9331–9341. doi: 10.1007/s00253-016-7736-9
Finney, D. M., Buyer, J. S., and Kaye, J. P. (2017). Living cover crops have immediate impacts on soil microbial community structure and function. J. Soil Water Conserv. 72, 361–373. doi: 10.2489/jswc.72.4.361
Fiorini, A., Maris, S. C., Abalos, D., Amaducci, S., and Tabaglio, V. (2020). Combining no-till with rye (Secale cereale L.) cover crop mitigates nitrous oxide emissions without decreasing yield. Soil Tillage Res. 196:104442. doi: 10.1016/j.still.2019.104442
Frasier, I., Noellemeyer, E., Figuerola, E., Erijman, L., Permingeat, H., and Quiroga, A. (2016). High quality residues from cover crops favor changes in microbial community and enhance C and N sequestration. Glob. Ecol. Conserv. 6, 242–256. doi: 10.1016/j.gecco.2016.03.009
Fronning, B. E., Thelen, K. D., and Min, D. H. (2008). Use of manure, compost, and cover crops to supplant crop residue carbon in corn stover removed cropping systems. Agron. J. 100, 1703–1710.
Gabriel, J. L., and Quemada, M. (2011). Replacing bare fallow with cover crops in a maize cropping system: yield, N uptake and fertiliser fate. Eur. J. Agron. 34, 133–143. doi: 10.1016/j.eja.2010.11.006
Garbeva, P. V., Van Veen, J., and Van Elsas, J. (2004). Microbial diversity in soil: selection of microbial populations by plant and soil type and implications for disease suppressiveness. Annu. Rev. Phytopathol. 42, 243–270. doi: 10.1146/annurev.phyto.42.012604.135455
Gardiano, C. G., Krzyzanowski, A. A., Abib Saab, O. J., Dallemole-Giaretta, R., and Lopes, E. A. (2013). Population reduction of the reniform nematode with the incorporation of soil cover crops in greenhouse. Nematropica 43, 138–142.
Garland, G. M., Suddick, E., Burger, M., Horwath, W. R., and Six, J. (2011). Direct N2O emissions following transition from conventional till to no-till in a cover cropped Mediterranean vineyard (Vitis vinifera). Agric. Ecosyst. Environ. 144, 423–428.
Gavazzi, C., Schulz, M., Marocco, A., and Tabaglio, V. (2010). Sustainable weed control by allelochemicals from rye cover crops: from the greenhouse to field evidence. Allelopathy J. 25, 259–273.
Ghimire, B., Ghimire, R., Vanleeuwen, D., and Mesbah, A. (2017). Cover crop residue amount and quality effects on soil organic carbon mineralization. Sustainability 9:2316. doi: 10.3390/su9122316
Gonsiorkiewicz Rigon, J. P., Calonego, J. C., Rosolem, C. A., and La Scala, N. Jr. (2018). Cover crop rotations in no-till system: short-term CO2 emissions and soybean yield. Sci. Agr. 75, 18–26. doi: 10.1590/1678-992x-2016-0286
Gregorich, E., Rochette, P., St-Georges, P., Mckim, U., and Chan, C. (2008). Tillage effects on N2O emission from soils under corn and soybeans in Eastern Canada. Can. J. Soil Sci. 88, 153–161. doi: 10.4141/cjss06041
Guardia, G., Abalos, D., García-Marco, S., Quemada, M., Alonso-Ayuso, M., Cárdenas, L. M., et al. (2016). Effect of cover crops on greenhouse gas emissions in an irrigated field under integrated soil fertility management. Biogeosci. Discuss. 13, 1–36.
Hall, H., Li, Y., Comerford, N., Gardini, E. A., Cernades, L. Z., Baligar, V., et al. (2010). Cover crops alter phosphorus soil fractions and organic matter accumulation in a Peruvian cacao agroforestry system. Agrofor. Syst. 80, 447–455. doi: 10.1007/s10457-010-9333-8
Haque, M. M., Kim, S. Y., Pramanik, P., Kim, G. Y., and Kim, P. J. (2013). Optimum application level of winter cover crop biomass as green manure under considering methane emission and rice productivity in paddy soil. Biol. Fertil. Soils 49, 487–493.
Haramoto, E. R., and Brainard, D. C. (2012). Strip tillage and oat cover crops increase soil moisture and influence N mineralization patterns in cabbage. Hortscience 47, 1596–1602. doi: 10.21273/hortsci.47.11.1596
Hay, M. M., Dille, J. A., and Peterson, D. E. (2019). Integrated pigweed (Amaranthus spp.) management in glufosinate-resistant soybean with a cover crop, narrow row widths, row-crop cultivation, and herbicide program. Weed Technol. 33, 710–719. doi: 10.1017/wet.2019.62
Haynes, R. (2005). Labile organic matter fractions as central components of the quality of agricultural soils: an overview. Adv. Agron. 85, 221–268. doi: 10.1016/s0065-2113(04)85005-3
He, J., Wang, Q., Li, H., Liu, L., and Gao, H. (2009). Effect of alternative tillage and residue cover on yield and water use efficiency in annual double cropping system in North China Plain. Soil Tillage Res. 104, 198–205. doi: 10.1016/j.still.2008.08.015
Higo, M., Isobe, K., Yamaguchi, M., and Torigoe, Y. (2015). Impact of a soil sampling strategy on the spatial distribution and diversity of arbuscular mycorrhizal communities at a small scale in two winter cover crop rotational systems. Ann. Microbiol. 65, 985–993. doi: 10.1007/s13213-014-0942-8
Higo, M., Sasaki, R., Unoki, T., Gunji, K., Suzuki, D., and Isobe, K. (2017). Influence of cover crop residue management on the indigenous arbuscular mycorrhizal fungi, corn growth and yield. Can. J. Plant Pathol. 39, 560–560.
Higo, M., Takahashi, Y., Gunji, K., and Isobe, K. (2018). How are arbuscular mycorrhizal associations related to maize growth performance during short-term cover crop rotation? J. Sci. Food Agric. 98, 1388–1396. doi: 10.1002/jsfa.8606
Higo, M., Tatewaki, Y., Gunji, K., Kaseda, A., and Isobe, K. (2019). Cover cropping can be a stronger determinant than host crop identity for arbuscular mycorrhizal fungal communities colonizing maize and soybean. PeerJ 7:e6403. doi: 10.7717/peerj.6403
Hodge, A., Campbell, C. D., and Fitter, A. H. (2001). An arbuscular mycorrhizal fungus accelerates decomposition and acquires nitrogen directly from organic material. Nature 413, 297–299. doi: 10.1038/35095041
Holman, J. D., Arnet, K., Dille, J., Maxwell, S., Obour, A., Roberts, T., et al. (2018). Can cover or forage crops replace fallow in the semiarid central great plains? Crop Sci. 58, 932–944. doi: 10.2135/cropsci2017.05.0324
Hontoria, C., Garcia-Gonzalez, I., Quemada, M., Roldan, A., and Alguacil, M. M. (2019). The cover crop determines the AMF community composition in soil and in roots of maize after a ten-year continuous crop rotation. Sci. Total Environ. 660, 913–922. doi: 10.1016/j.scitotenv.2019.01.095
Huang, S., Tang, J., Liao, P., Zeng, Y., Shi, Q., and Pan, X. (2016). Interaction of winter legume manure covering(Astragalus sinicus L.) and atraw retention on yield and soil properties in a double rice cropping system. Acta Agric. Univ. Jiangxiensis 38, 215–222.
Huang, Y., Jiao, Y., Zong, L., Zhou, Q., Zheng, X., and Sass, R. (2002). N2O emission from wheat cultivated soils as influenced by soil physicochemical properties. Acta Scientiae Circumstantiae 22, 598–602.
Huang, Y., Zou, J., Zheng, X., Wang, Y., and Xu, X. (2004). Nitrous oxide emissions as influenced by amendment of plant residues with different C: N ratios. Soil Biol. Biochem. 36, 973–981. doi: 10.1016/j.soilbio.2004.02.009
Hwang, H. Y., Kim, G. W., Kim, S. Y., Haque, M. M., Khan, M. I., and Kim, P. J. (2017). Effect of cover cropping on the net global warming potential of rice paddy soil. Geoderma 292, 49–58. doi: 10.1016/j.scitotenv.2020.143193
Hwang, H. Y., Kim, G. W., Lee, Y. B., Kim, P. J., and Kim, S. Y. (2015). Improvement of the value of green manure via mixed hairy vetch and barley cultivation in temperate paddy soil. Field Crops Res. 183, 138–146. doi: 10.1016/j.fcr.2015.08.001
Igos, E., Golkowska, K., Koster, D., Vervisch, B., and Benetto, E. (2016). Using rye as cover crop for bioenergy production: an environmental and economic assessment. Biomass Bioenergy 95, 116–123.
IPCC (2007). The Physical Science Basis. Contribution of Working Group I to the Fourth Assessment Report of the Intergovernmental Panel on Climate Change. Cambridge: Cambridge University Press.
Jani, A. D., Grossman, J., Smyth, T. J., and Hu, S. (2016). Winter legume cover-crop root decomposition and N release dynamics under disking and roller-crimping termination approaches. Renew. Agric. Food Syst. 31, 214–229. doi: 10.1017/s1742170515000113
Jarecki, M. K., Parkin, T. B., Chan, A. S. K., Kaspar, T. C., Moorman, T. B., Singer, J. W., et al. (2009). Cover crop effects on nitrous oxide emission from a manure-treated Mollisol. Agr. Ecosyst. Environ. 134, 29–35.
Kallenbach, C. M., Rolston, D. E., and Horwath, W. R. (2010). Cover cropping affects soil N2O and CO2 emissions differently depending on type of irrigation. Agric. Ecosyst. Environ. 137, 251–260. doi: 10.1016/j.agee.2010.02.010
Kandel, T. P., Gowda, P. H., Somenahally, A., Northup, B. K., Dupont, J., and Rocateli, A. C. (2018). Nitrous oxide emissions as influenced by legume cover crops and nitrogen fertilization. Nutr. Cycl. Agroecosyst. 112, 119–131. doi: 10.1016/j.scitotenv.2022.153352
Kaspar, T. C., Jaynes, D. B., Parkin, T. B., Moorman, T. B., and Singer, J. W. (2012). Effectiveness of oat and rye cover crops in reducing nitrate losses in drainage water. Agric. Water Manage. 110, 25–33. doi: 10.1016/j.agwat.2012.03.010
Kaspar, T. C., Parkin, T. B., Jaynes, D. B., Cambardella, C. A., Meek, D. W., and Jung, Y. S. (2006). Examining changes in soil organic carbon with oat and rye cover crops using terrain covariates. Soil Sci. Soc. Am. J. 70, 1168–1177. doi: 10.2136/sssaj2005.0095
Kaspar, T. C., Radke, J. K., and Laflen, J. M. (2001). Small grain cover crops and wheel traffic effects on infiltration, runoff, and erosion. J. Soil Water Conserv. 56, 160–164.
Kato, T., Hirota, M., Tang, Y., and Wada, E. (2011). Spatial variability of CH4 and N2O fluxes in alpine ecosystems on the Qinghai–Tibetan Plateau. Atmos. Environ. 45, 5632–5639. doi: 10.1016/j.atmosenv.2011.03.010
Kerri, S., and Belina, K. M. (2008). Cover crops and cultivation: impacts on soil N dynamics and microbiological function in a Mediterranean vineyard agroecosystem. Appl. Soil Ecol. 40, 370–380. doi: 10.1016/j.apsoil.2008.06.004
Khalil, M., Rosenani, A., Van Cleemput, O., Boeckx, P., Shamshuddin, J., and Fauziah, C. (2002). Nitrous oxide production from an ultisol of the humid tropics treated with different nitrogen sources and moisture regimes. Biol. Fertil. Soils 36, 59–65.
Khan, I., Lei, H., Shah, A. A., Khan, I., and Muhammad, I. (2021). Climate change impact assessment, flood management, and mitigation strategies in Pakistan for sustainable future. Environ. Sci. Pollut. Res. 28, 29720–29731. doi: 10.1007/s11356-021-12801-4
Kokalis-Burelle, N., Mcsorley, R., Wang, K.-H., Saha, S. K., and Mcgovern, R. J. (2017). Rhizosphere microorganisms affected by soil solarization and cover cropping in Capsicum annuum and Phaseolus lunatus agroecosystems. Appl. Soil Ecol. 119, 64–71. doi: 10.1016/j.apsoil.2017.06.001
Kong, A. Y. Y., and Six, J. (2012). Microbial community assimilation of cover crop rhizodeposition within soil microenvironments in alternative and conventional cropping systems. Plant Soil 356, 315–330. doi: 10.1007/s11104-011-1120-4
Kornecki, T. S., Price, A. J., Raper, R. L., and Arriaga, F. J. (2009). New roller crimper concepts for mechanical termination of cover crops in conservation agriculture. Renew. Agric. Food Syst. 24, 165–173. doi: 10.1017/s1742170509002580
Kornecki, T. S., Prior, S. A., and Torbert, H. A. (2016). Effects of a custom cover crop residue manager in a no-till cotton system. Appl. Eng. Agric. 32, 333–340. doi: 10.13031/aea.32.11567
Lacey, C., and Armstrong, S. (2015). The efficacy of winter cover crops to stabilize soil inorganic nitrogen after fall-applied anhydrous ammonia. J. Environ. Qual. 44, 442–448. doi: 10.2134/jeq2013.12.0529
Lal, R. (2009). Soil quality impacts of residue removal for bioethanol production. Soil Tillage Res. 102, 233–241. doi: 10.1016/j.still.2008.07.003
Lane, M., Lorenz, N., Saxena, J., Ramsier, C., and Dick, R. P. (2012). Microbial activity, community structure and potassium dynamics in rhizosphere soil of soybean plants treated with glyphosate. Pedobiologia 55, 153–159. doi: 10.1016/j.pedobi.2011.12.005
Lee, C. H., Do Park, K., Jung, K. Y., Ali, M. A., Lee, D., Gutierrez, J., et al. (2010). Effect of Chinese milk vetch (Astragalus sinicus L.) as a green manure on rice productivity and methane emission in paddy soil. Agric. Ecosyst. Environ. 138, 343–347. doi: 10.1016/j.agee.2010.05.011
Lehman, R. M., Cambardella, C. A., Stott, D. E., Acosta-Martinez, V., Manter, D. K., Buyer, J. S., et al. (2015). Understanding and enhancing soil biological health: the solution for reversing soil degradation. Sustainability 7, 988–1027. doi: 10.3390/su7010988
Lekberg, Y., and Koide, R. (2005). Is plant performance limited by abundance of arbuscular mycorrhizal fungi? A meta-analysis of studies published between 1988 and 2003. New Phytol. 168, 189–204. doi: 10.1111/j.1469-8137.2005.01490.x
Li, F., Sorensen, P., Li, X., and Olesen, J. E. (2019). Carbon and nitrogen mineralization differ between incorporated shoots and roots of legume versus non-legume based cover crops. Plant Soil 446, 243–257. doi: 10.1007/s11104-019-04358-6
Li, R., Hou, X., Jia, Z., Han, Q., Ren, X., and Yang, B. (2013). Effects on soil temperature, moisture, and maize yield of cultivation with ridge and furrow mulching in the rainfed area of the Loess Plateau, China. Agric. Water Manage. 116, 101–109. doi: 10.1016/j.agwat.2012.10.001
Liang, H., Hu, K., Qin, W., Zuo, Q., and Zhang, Y. (2017). Modelling the effect of mulching on soil heat transfer, water movement and crop growth for ground cover rice production system. Field Crops Res. 201, 97–107. doi: 10.1016/j.fcr.2016.11.003
Liebig, M., Tanaka, D., and Gross, J. (2010). Fallow effects on soil carbon and greenhouse gas flux in central North Dakota. Soil Sci. Soc. Am. J. 74, 358–365. doi: 10.2136/sssaj2008.0368
Liebman, A. M., Grossman, J., Brown, M., Wells, M. S., Reberg-Horton, S. C., and Shi, W. (2018). Legume cover crops and tillage impact nitrogen dynamics in organic corn production. Agron. J.110, 1046–1057. doi: 10.2134/agronj2017.08.0474
Linsler, D., Kaiser, M., Andruschkewitsch, R., Piegholdt, C., and Ludwig, B. (2016). Effects of cover crop growth and decomposition on the distribution of aggregate size fractions and soil microbial carbon dynamics. Soil Use Manage. 32, 192–199. doi: 10.1111/sum.12267
Liu, Y., Pan, Y., Yang, L., Ahmad, S., and Zhou, X. B. (2021). Stover return and nitrogen application affect soil organic carbon and nitrogen in a double-season maize field. Plant Biol. 24, 387–395. doi: 10.1111/plb.13370
Logsdon, S. D., Kaspar, T. C., Meek, D. W., and Prueger, J. H. (2002). Nitrate leaching as influenced by cover crops in large soil monoliths. Agron. J. 94, 807–814. doi: 10.2134/agronj2002.8070
López-Bellido, R. J., Fontán, J. M., López-Bellido, F. J., and López-Bellido, L. (2010). Carbon sequestration by tillage, rotation, and nitrogen fertilization in a Mediterranean Vertisol. Agron. J.102, 310–318. doi: 10.2134/agronj2009.0165
Lori, M., Symnaczik, S., Mäder, P., De Deyn, G., and Gattinger, A. (2017). Organic farming enhances soil microbial abundance and activity—A meta-analysis and meta-regression. PLoS One 12:e0180442. doi: 10.1371/journal.pone.0180442
Madsen, H., Talgre, L., Eremeev, V., Alaru, M., Kauer, K., and Luik, A. (2016). Do green manures as winter cover crops impact the weediness and crop yield in an organic crop rotation? Biol. Agric. Hortic. 32, 182–191. doi: 10.1080/01448765.2016.1138141
Malpassi, R., Kaspar, T., Parkin, T., Cambardella, C., and Nubel, N. (2000). Oat and rye root decomposition effects on nitrogen mineralization. Soil Sci. Soc. Am. J. 64, 208–215. doi: 10.2136/sssaj2000.641208x
Maltais-Landry, G., and Crews, T. E. (2019). Hybrid systems combining manures and cover crops can substantially reduce nitrogen fertilizer use. Agroecol. Sustain. Food Syst.
Mancinelli, R., Marinari, S., Di Felice, V., Savin, M. C., and Campiglia, E. (2013). Soil property, CO2 emission and aridity index as agroecological indicators to assess the mineralization of cover crop green manure in a Mediterranean environment. Ecol. Indic. 34, 31–40.
Marahatta, S. P., Wang, K. H., and Sipes, B. S. (2012). Does integration of high and low C:N ratio cover crops benefit soil health management J. Nematol. 44, 476–477.
Marinari, S., Mancinelli, R., Brunetti, P., and Campiglia, E. (2015). Soil quality, microbial functions and tomato yield under cover crop mulching in the Mediterranean environment. Soil Tillage Res. 145, 20–28. doi: 10.1016/j.still.2014.08.002
Martens, J. R. T., and Entz, M. H. (2001). Availability of late-season heat and water resources for relay and double cropping with winter wheat in prairie Canada. Can. J. Plant Sci. 81, 273–276. doi: 10.4141/p00-105
Martens, J. R. T., Hoeppner, J. W., and Entz, M. H. (2001). Legume cover crops with winter cereals in southern Manitoba: establishment, productivity, and microclimate effects. Agron. J.93, 1086–1096. doi: 10.2134/agronj2001.9351086x
Martinez-Garcia, L. B., Korthals, G., Brussaard, L., Jorgensen, H. B., and De Deyn, G. B. (2018). Organic management and cover crop species steer soil microbial community structure and functionality along with soil organic matter properties. Agric. Ecosyst. Environ. 263, 7–17. doi: 10.1016/j.agee.2018.04.018
Marton, J. M., Fennessy, M. S., and Craft, C. B. (2014). USDA conservation practices increase carbon storage and water quality improvement functions: an example from Ohio. Restor. Ecol. 22, 117–124. doi: 10.1111/rec.12033
Mays, D. A., Sistani, K. R., and Malik, R. K. (2003). Use of winter annual cover crops to reduce soil nitrate levels. J. Sustain. Agric. 21, 5–19. doi: 10.2134/jeq2006.0468
Mbuthia, L. W., Acosta-Martínez, V., Debruyn, J., Schaeffer, S., Tyler, D., Odoi, E., et al. (2015). Long term tillage, cover crop, and fertilization effects on microbial community structure, activity: implications for soil quality. Soil Biol. Biochem. 89, 24–34. doi: 10.1016/j.soilbio.2015.06.016
McMechan, A. J., Hodgson, E. W., Varenhorst, A. J., Hunt, T., Wright, R., and Potter, B. (2021). Soybean gall midge (diptera: cecidomyiidae), a new species causing injury to soybean in the United States. J. Integr. Pest Manage. 12:8.
Meisinger, J., Hargrove, W., Mikkelsen, R., Williams, J., and Benson, V. (1991). Effects of cover crops on groundwater quality. Cover Crops Clean Water 57–68.
Melling, L., Hatano, R., and Goh, K. J. (2007). Nitrous oxide emissions from three ecosystems in tropical peatland of Sarawak, Malaysia. Soil Sci. Plant Nutr. 53, 792–805. doi: 10.1111/j.1747-0765.2007.00196.x
Mirsky, S. B., Curran, W. S., Mortensen, D. A., Ryan, M. R., and Shumway, D. L. (2009). Control of cereal rye with a roller/crimper as influenced by cover crop phenology. Agron. J.101, 1589–1596. doi: 10.2134/agronj2009.0130
Mitchell, D. C., Castellano, M. J., Sawyer, J. E., and Pantoja, J. (2013). Cover crop effects on nitrous oxide emissions: role of mineralizable carbon. Soil Sci. Soc. Am. J. 77, 1765–1773.
Mitchell, J. P., Shrestha, A., Mathesius, K., Scow, K. M., Southard, R. J., Haney, R. L., et al. (2017). Cover cropping and no-tillage improve soil health in an arid irrigated cropping system in California’s San Joaquin Valley, USA. Soil Tillage Res. 165, 325–335. doi: 10.1016/j.still.2016.09.001
Moeller, K., Stinner, W., and Leithold, G. (2008). Growth, composition, biological N2 fixation and nutrient uptake of a leguminous cover crop mixture and the effect of their removal on field nitrogen balances and nitrate leaching risk. Nutr. Cycl. Agroecosyst. 82, 233–249. doi: 10.1007/s10705-008-9182-2
Mondal, M. K., and Lenka, M. (2012). Solubility of CO2 in aqueous strontium hydroxide. Fluid Phase Equilib. 336, 59–62. doi: 10.1016/j.fluid.2012.08.032
Morales Salmeron, L., Martin-Lammerding, D., Tenorio Pasamon, J. L., and Sanchez-Moreno, S. (2019). Effects of cover crops on soil biota, soil fertility and weeds, and Pratylenchus suppression in experimental conditions. Nematology 21, 227–241. doi: 10.1163/15685411-00003208
Moreira Rovedder, A. P., and Foletto Eltz, F. L. (2008). Revegetation with cover crops for soils under arenization and wind erosion in Rio Grande do Sul state, Brazil. Rev. Bras. Cienc. Solo 32, 315–321.
Morimoto, S., Uchida, T., Matsunami, H., and Kobayashi, H. (2018). Effect of winter wheat cover cropping with no-till cultivation on the community structure of arbuscular mycorrhizal fungi colonizing the subsequent soybean. Soil Sci. Plant Nutr. 64, 545–553. doi: 10.1080/00380768.2018.1486171
Mueller, P., Granse, D., Nolte, S., Do, H. T., Weingartner, M., Hoth, S., et al. (2017). Top-down control of carbon sequestration: grazing affects microbial structure and function in salt marsh soils. Ecol. Appl. 27, 1435–1450. doi: 10.1002/eap.1534
Muhammad, I., Khan, F., Khan, A., and Wang, J. (2018). Soil fertility in response to urea and farmyard manure incorporation under different tillage systems in Peshawar, Pakistan. Int. J. Agric. Biol. 20, 1539–1547.
Muhammad, I., Sainju, U. M., Zhao, F., Khan, A., Ghimire, R., Fu, X., et al. (2019). Regulation of soil CO2 and N2O emissions by cover crops: a meta-analysis. Soil Tillage Res. 192, 103–112. doi: 10.1016/j.still.2019.04.020
Muhammad, I., Wang, J., Khan, A., Ahmad, S., Yang, L., Ali, I., et al. (2021a). Impact of the mixture verses solo residue management and climatic conditions on soil microbial biomass carbon to nitrogen ratio: a systematic review. Environ. Sci. Pollut. Res. 28, 64241–64252. doi: 10.1007/s11356-021-15579-7
Muhammad, I., Wang, J., Sainju, U. M., Zhang, S., Zhao, F., and Khan, A. (2021b). Cover cropping enhances soil microbial biomass and affects microbial community structure: a meta-analysis. Geoderma 381:114696. doi: 10.1016/j.geoderma.2020.114696
Muhammad, I., Yang, L., Ahmad, S., Zeeshan, M., Farooq, S., Ali, I., et al. (2022). Irrigation and nitrogen fertilization alter soil bacterial communities, soil enzyme activities, and nutrient availability in maize crop. Front. Microbiol. 105:833758. doi: 10.3389/fmicb.2022.833758
Mukumbareza, C., Muchaonyerwa, P., and Chiduza, C. (2016). Bicultures of oat (Avena sativa L.) and grazing vetch (Vicia dasycarpa L.) cover crops increase contents of carbon pools and activities of selected enzymes in a loam soil under warm temperate conditions. Soil Sci. Plant Nutr. 62, 447–455. doi: 10.1080/00380768.2016.1206833
Mupambwa, H., and Wakindiki, I. (2012). Winter cover crops effects on soil strength, infiltration and water retention in a sandy loam Oakleaf soil in Eastern Cape, South Africa. S. Afr. J. Plant Soil 29, 121–126. doi: 10.1080/02571862.2012.722697
Mupambwa, H. A. (2012). Winter Rotational Cover Crops Effects on Soil Strength, Aggregate Stability and Water Conservation of a Hardsetting Cambisol in Eastern Cape Province, South Africa/dcHupenyu Allan Mupambwa. Alice: University of Fort Hare.
Nakamoto, T., Komatsuzaki, M., Hirata, T., and Araki, H. (2012). Effects of tillage and winter cover cropping on microbial substrate-induced respiration and soil aggregation in two Japanese fields. Soil Sci. Plant Nutr. 58, 70–82. doi: 10.1080/00380768.2011.650134
Neely, C. B., Rouquette, F. M. Jr., Morgan, C. L., Smith, G. R., Hons, F. M., and Rooney, W. L. (2018). Integrating legumes as cover crops and intercrops into grain sorghum production systems. Agron. J. 110, 1363–1378. doi: 10.2134/agronj2017.05.0293
Negassa, W., Price, R. F., Basir, A., Snapp, S. S., and Kravchenko, A. (2015). Cover crop and tillage systems effect on soil CO2 and N2O fluxes in contrasting topographic positions. Soil Tillage Res. 154, 64–74. doi: 10.1016/j.still.2015.06.015
Njeru, E. M., Avio, L., Sbrana, C., Turrini, A., Bocci, G., Barberi, P., et al. (2013). First evidence for a major cover crop effect on arbuscular mycorrhizal fungi and organic maize growth. Agron. Sustain. Dev. 34, 841–848. doi: 10.1007/s13593-013-0197-y
Odhiambo, J. J. O., and Bomke, A. A. (2000). Short term nitrogen availability following overwinter cereal/grass and legume cover crop monocultures and mixtures in south coastal British Columbia. J. Soil Water Conserv. 55, 347–354.
Omonode, R. A., Smith, D. R., Gál, A., and Vyn, T. J. (2009). Soil nitrous oxide emissions in corn following three decades of tillage and rotation treatments. Soil Sci. Soc. Am. J. 75, 152–163.
Ozturk, I. (2017). Measuring the impact of alternative and nuclear energy consumption, carbon dioxide emissions and oil rents on specific growth factors in the panel of Latin American countries. Prog. Nucl. Energy 100, 71–81. doi: 10.1016/j.pnucene.2017.05.030
Ozturk, I., and Acaravci, A. (2010). CO2 emissions, energy consumption and economic growth in Turkey. Renew. Sustain. Energy Rev. 14, 3220–3225. doi: 10.1016/j.rser.2010.07.005
Pandey, C., and Begum, M. (2010). The effect of a perennial cover crop on net soil N mineralization and microbial biomass carbon in coconut plantations in the humid tropics. Soil Use Manage. 26, 158–166. doi: 10.1111/j.1475-2743.2010.00272.x
Pappa, V. A., Rees, R. M., Walker, R. L., Baddeley, J. A., and Watson, C. A. (2011). Nitrous oxide emissions and nitrate leaching in an arable rotation resulting from the presence of an intercrop. Agric. Ecosyst. Environ. 141, 153–161. doi: 10.1016/j.agee.2011.02.025
Parkin, T. B., and Kaspar, T. C. (2006). Nitrous oxide emissions from corn-soybean systems in the Midwest. J. Environ. Q. 35, 1496.
Parkin, T. B., Kaspar, T. C., and Singer, J. W. (2006). Cover crop effects on the fate of N following soil application of swine manure. Plant Soil 289, 141–152.
Parr, M., Grossman, J. M., Reberg-Horton, S. C., Brinton, C., and Crozier, C. (2014). Roller-crimper termination for legume cover crops in north carolina: impacts on nutrient availability to a succeeding corn crop. Commun. Soil Sci. Plant Anal. 45, 1106–1119. doi: 10.1080/00103624.2013.867061
Peregrina, F. (2016). Surface soil properties influence carbon oxide pulses after precipitation events in a semiarid vineyard under conventional tillage and cover crops. Pedosphere 26, 499–509. doi: 10.1016/s1002-0160(15)60060-1
Peregrina, F., Pilar Perez-Alvarez, E., and Garcia-Escudero, E. (2014). Soil microbiological properties and its stratification ratios for soil quality assessment under different cover crop management systems in a semiarid vineyard. J. Plant Nutr. Soil Sci. 177, 548–559. doi: 10.1002/jpln.201300371
Petersen, S. O., Mutegi, J. K., Hansen, E. M., and Munkholm, L. J. (2011). Tillage effects on N2O emissions as influenced by a winter cover crop. Soil Biol. Biochem. 43, 1509–1517. doi: 10.1016/j.soilbio.2011.03.028
Peyrard, C., Mary, B., Perrin, P., Véricel, G., Gréhan, E., Justes, E., et al. (2016). N2O emissions of low input cropping systems as affected by legume and cover crops use. Agric. Ecosyst. Environ. 224, 145–156. doi: 10.1016/j.agee.2016.03.028
Pina, M. (2019). Cover crops and biochar soil amendments shift microbial communities to improve soil fertility and fruit yield in a southern california organic mango orchard. Hortscience 54, S18–S18.
Plaza-Bonilla, D., Nolot, J.-M., Raffaillac, D., and Justes, E. (2015). Cover crops mitigate nitrate leaching in cropping systems including grain legumes: Field evidence and model simulations. Agric. Ecosyst. Environ. 212, 1–12. doi: 10.1016/j.agee.2015.06.014
Pramanik, P., Haque, M. M., Sang, Y. K., and Kim, P. J. (2014). C and N accumulations in soil aggregates determine nitrous oxide emissions from cover crop treated rice paddy soils during fallow season. Sci. Total Environ. 490, 622–628. doi: 10.1016/j.scitotenv.2014.05.046
Ravenel, C., Deneufbourg, F., Pateau, Y., Casals, M. L., Coste, F., and Hellou, G. (2015). Tall fescue seed crops grown with legume cover crops: impact on nitrogen requirements. Fourrages 224, 287–291.
Reddy, K. N. (2001). Effects of cereal and legume cover crop residues on weeds, yield, and net return in soybean (Glycine max). Weed Technol. 15, 660–668. doi: 10.1614/0890-037x(2001)015[0660:eocalc]2.0.co;2
Reddy, K. N. (2003). Impact of rye cover crop and herbicides on weeds, yield, and net return in narrow-row transgenic and conventional soybean (Glycine max). Weed Technol. 17, 28–35. doi: 10.1614/0890-037x(2003)017[0028:iorcca]2.0.co;2
Reddy, N., and Crohn, D. M. (2014). Effects of soil salinity and carbon availability from organic amendments on nitrous oxide emissions. Geoderma 235, 363–371. doi: 10.1016/j.geoderma.2014.07.022
Rice, W. C., and Gowda, P. H. (2011). Influence of geographical location, crop type and crop residue cover on bacterial and fungal community structures. Geoderma 160, 271–280. doi: 10.1016/j.geoderma.2010.09.003
Rodrigues Torres, J. L., Pereira, M. G., and Fabian, A. J. (2008). Cover crops biomass production and its residues mineralization in a Brazilian no-till Oxisol. Pesqui. Agropecu. Bras. 43, 421–428.
Rojas, M. A., Van Eerd, L. L., O’halloran, I. P., Sikkema, P. H., and Robinson, D. E. (2018). Responses of spring-seeded cover crop roots by herbicide residues and short-term influence in soil aggregate stability and N cycling. Can. J. Plant Sci. 98, 990–1004. doi: 10.1139/cjps-2017-0213
Rose, T. J., Wood, R. H., Gleeson, D. B., Rose, M. T., and Van Zwieten, L. (2016). Removal of phosphorus in residues of legume or cereal plants determines growth of subsequently planted wheat in a high phosphorus fixing soil. Biol. Fertil. Soils 52, 1085–1092. doi: 10.1007/s00374-016-1143-3
Rosecrance, R. C., and Teasdale, J. R. (2000). Denitrification and N mineralization from hairy vetch (Vicia villosa Roth) and rye (Secale cereale L.) cover crop monocultures and bicultures. Plant Soil 227, 283–290.
Rosolem, C. A., Pace, L., and Crusciol, C. A. C. (2004). Nitrogen management in maize cover crop rotations. Plant Soil 264, 261–271. doi: 10.1023/b:plso.0000047761.50641.a3
Sainju, U. M., and Singh, B. P. (2008). Nitrogen storage with cover crops and nitrogen fertilization in tilled and nontilled soils. Agron. J. 100, 619–627. doi: 10.2134/agronj2007.0236
Sainju, U. M., Singh, B. P., Whitehead, W. F., and Wang, S. (2007). Accumulation and crop uptake of soil mineral nitrogen as influenced by tillage, cover crops, and nitrogen fertilization. Agron. J. 99, 682–691. doi: 10.2134/agronj2006.0177
Sainju, U. M., Whitehead, W. F., Singh, B. P., and Wang, S. (2006). Tillage, cover crops, and nitrogen fertilization effects on soil nitrogen and cotton and sorghum yields. Eur. J. Agron. 25, 372–382. doi: 10.1016/j.eja.2006.07.005
Sanchez, I. I., Fultz, L. M., Lofton, J., and Haggard, B. (2019). Soil biological response to integration of cover crops and nitrogen rates in a conservation tillage corn production system. Soil Sci. Soc. Am. J. 83, 1356–1367. doi: 10.2136/sssaj2019.02.0051
Sanchis, E., Ferrer, M., Torres, A. G., Cambra-López, M., and Calvet, S. (2012). Effect of water and straw management practices on methane emissions from rice fields: a review through a meta-analysis. Environ. Eng. Sci. 29, 1053–1062. doi: 10.1089/ees.2012.0006
Sang, Y. K., Gutierrez, J., and Kim, P. J. (2012). Considering winter cover crop selection as green manure to control methane emission during rice cultivation in paddy soil. Agric. Ecosyst. Environ. 161, 130–136. doi: 10.1016/j.agee.2012.07.026
Sanz-Cobena, A., Garcia-Marco, S., Quemada, M., Gabriel, J. L., Almendros, P., and Vallejo, A. (2014). Do cover crops enhance N2O, CO2 or CH4 emissions from soil in Mediterranean arable systems? Sci. Total Environ. 466, 164–174. doi: 10.1016/j.scitotenv.2013.07.023
Sanz-Cobena, A., Lassaletta, L., Aguilera, E., Del Prado, A., Garnier, J., Billen, G., et al. (2017). Strategies for greenhouse gas emissions mitigation in Mediterranean agriculture: A review. Agric. Ecosyst. Environ. 238, 5–24.
Sanz-Cobena, A., Sánchez-Martín, L., García-Torres, L., and Vallejo, A. (2012). Gaseous emissions of N2O and NO and NO3– leaching from urea applied with urease and nitrification inhibitors to a maize (Zea mays) crop. Agric. Ecosyst. Environ. 149, 64–73. doi: 10.1016/j.agee.2011.12.016
Sarkodie-Addo, J., Lee, H., and Baggs, E. (2003). Nitrous oxide emissions after application of inorganic fertilizer and incorporation of green manure residues. Soil Use Manage. 19, 331–339.
Schmeer, M., Loges, R., Dittert, K., Senbayram, M., Horn, R., and Taube, F. (2014). Legume-based forage production systems reduce nitrous oxide emissions. Soil Tillage Res. 143, 17–25. doi: 10.1016/j.still.2014.05.001
Schroth, G., Salazar, E., and Da Silva, J. P. (2001). Soil nitrogen mineralization under tree crops and a legume cover crop in multi-strata agroforestry in central Amazonia: Spatial and temporal patterns. Exp. Agric. 37, 253–267. doi: 10.1017/s0014479701002058
Setyawan, D., Gilkes, R., and Tongway, D. (2011). Nutrient cycling index in relation to organic matter and soil respiration of rehabilitated mine sites in Kelian, East Kalimantan. J. Trop. Soils 16, 219–223. doi: 10.5400/jts.2011.16.3.219
Sharma, V., Irmak, S., and Padhi, J. (2018). Effects of cover crops on soil quality: Part I. Soil chemical properties-organic carbon, total nitrogen, pH, electrical conductivity, organic matter content, nitrate-nitrogen, and phosphorus. J. Soil Water Conserv. 73, 637–651. doi: 10.2489/jswc.73.6.637
Shelton, R. E., Jacobsen, K. L., and Mcculley, R. L. (2017). Cover crops and fertilization alter nitrogen loss in organic and conventional conservation agriculture systems. Front. Plant Sci. 8:2260–2260. doi: 10.3389/fpls.2017.02260
Sheppard, L., Leith, I., Leeson, S., Dijk, N. V., Field, C., and Levy, P. (2013). Fate of N in a peatland, Whim bog: immobilisation in the vegetation and peat, leakage into pore water and losses as N2O depend on the form of N. Biogeosciences 10, 149–160. doi: 10.5194/bg-10-149-2013
Singh, J., and Kumar, S. (2021). Responses of soil microbial community structure and greenhouse gas fluxes to crop rotations that include winter cover crops. Geoderma 385:114843. doi: 10.1016/j.geoderma.2020.114843
Smith, S., and Read, D. (2008). “Colonization of roots and anatomy of arbuscular mycorrhiza,” in Mycorrhizal Symbiosis, eds S. E. Smith and D. Read (London: Academic Press), 42–90. doi: 10.1016/b978-012370526-6.50004-0
Snapp, S. S., and Borden, H. (2005). Enhanced nitrogen mineralization in mowed or glyphosate treated cover crops compared to direct incorporation. Plant Soil 270, 101–112. doi: 10.13287/j.1001-9332.201906.035
Sofi, J. A., Dar, I. H., Chesti, M. H., Bisati, I. A., Mir, S. A., and Sofi, K. A. (2018). Effect of nitrogen fixing cover crops on fertility of apple (Malus domestica Borkh) orchard soils assessed in a chronosequence in North-West Himalaya of Kashmir valley, India. Legume Res. 41, 87–94.
Sogbedji, J. M., Van Es, H. M., and Agbeko, K. L. (2006). Cover cropping and nutrient management strategies for maize production in western Africa. Agron. J. 98, 883–889. doi: 10.2134/agronj2005.0025
Somenahally, A., Dupont, J. I., Brady, J., Mclawrence, J., Northup, B., and Gowda, P. (2018). Microbial communities in soil profile are more responsive to legacy effects of wheat-cover crop rotations than tillage systems. Soil Biol. Biochem. 123, 126–135. doi: 10.1016/j.soilbio.2018.04.025
Steenwerth, K., and Belina, K. M. (2008). Cover crops and cultivation: Impacts on soil N dynamics and microbiological function in a Mediterranean vineyard agroecosystem. Appl. Soil Ecol. 40, 370–380. doi: 10.1016/j.apsoil.2008.06.004
Tang, H., Xiao, X., Tang, W., Wang, K., Li, C., and Cheng, K. (2017). Returning winter cover crop residue influences soil aggregation and humic substances under double-cropped rice fields. Rev. Bras. Cienc. Solo 41:e0160488. doi: 10.1590/18069657rbcs20160488
Tang, H., Xiao, X., Tang, W., Wang, K., Sun, J., Li, W., et al. (2015). Effects of winter covering crop residue incorporation on CH4 and N2O emission from double-cropped paddy fields in southern China. Environ. Sci. Pollut. Res. 22, 12689–12698. doi: 10.1007/s11356-015-4557-9
Teasdale, J. R. (1996). Contribution of cover crops to weed management in sustainable agricultural systems. J. Prod. Agric. 9, 475–479. doi: 10.1021/jf201731u
Teasdale, J. R., Coffman, C. B., and Mangum, R. W. (2007). Potential long-term benefits of no-tillage and organic cropping systems for grain production and soil improvement. Agron. J. 99, 1297–1305. doi: 10.2134/agronj2006.0362
Thorup-Kristensen, K., Magid, J., and Jensen, L. S. (2003). Catch crops and green manures as biological tools in nitrogen management in temperate zones. Adv. Agron. 79, 227–302. doi: 10.1016/s0065-2113(02)79005-6
Tian, L., Dell, E., and Shi, W. (2010). Chemical composition of dissolved organic matter in agroecosystems: correlations with soil enzyme activity and carbon and nitrogen mineralization. Appl. Soil Ecol. 46, 426–435. doi: 10.1016/j.apsoil.2010.09.007
Tian, Y., Zhang, X., Liu, J., and Gao, L. (2011). Effects of summer cover crop and residue management on cucumber growth in intensive Chinese production systems: soil nutrients, microbial properties and nematodes. Plant Soil 339, 299–315. doi: 10.1007/s11104-010-0579-8
Toom, M., Talgre, L., Maee, A., Tamm, S., Narits, L., Edesi, L., et al. (2019). Selecting winter cover crop species for northern climatic conditions. Biol. Agric. Hortic. 35, 263–274. doi: 10.1080/01448765.2019.1627908
Tosti, G., Benincasa, P., Farneselli, M., Pace, R., Tei, F., Guiducci, M., et al. (2012). Green manuring effect of pure and mixed barley-hairy vetch winter cover crops on maize and processing tomato N nutrition. Eur. J. Agron. 43, 136–146. doi: 10.1016/j.eja.2012.06.004
Tribouillois, H., Constantin, J., and Justes, E. (2018). Cover crops mitigate direct greenhouse gases balance but reduce drainage under climate change scenarios in temperate climate with dry summers. Glob. Change Biol. 24, 2513–2529. doi: 10.1111/gcb.14091
Tribouillois, H., Cruz, P., Cohan, J.-P., and Justes, E. (2015). Modelling agroecosystem nitrogen functions provided by cover crop species in bispecific mixtures using functional traits and environmental factors. Agric. Ecosyst. Environ. 207, 218–228. doi: 10.1016/j.agee.2015.04.016
Uliarte, E. M., Schultz, H. R., Frings, C., Pfister, M., Parera, C. A., and Del Monte, R. F. (2013). Seasonal dynamics of CO2 balance and water consumption of C-3 and C-4-type cover crops compared to bare soil in a suitability study for their use in vineyards in Germany and Argentina. Agric. For. Meteorol. 181, 1–16. doi: 10.1016/j.agrformet.2013.06.019
Unger, P. W., Schomberg, H. H., Dao, T. H., and Jones, O. R. (1997). Tillage and crop residue management practices for sustainable dryland farming systems. Ann. Arid Zone 36, 209–232.
Valkama, E., Rankinen, K., Virkajärvi, P., Salo, T., Kapuinen, P., and Turtola, E. (2016). Nitrogen fertilization of grass leys: yield production and risk of N leaching. Agric. Ecosyst. Environ. 230, 341–352. doi: 10.1016/j.agee.2016.05.022
Van Der Heijden, M. G., Bardgett, R. D., and Van Straalen, N. M. (2008). The unseen majority: soil microbes as drivers of plant diversity and productivity in terrestrial ecosystems. Ecol. Lett. 11, 296–310. doi: 10.1111/j.1461-0248.2007.01139.x
Venkateswarlu, B., Srinivasarao, C., Ramesh, G., Venkateswarlu, S., and Katyal, J. C. (2007). Effects of long-term legume cover crop incorporation on soil organic carbon, microbial biomass, nutrient build-up and grain yields of sorghum/sunflower under rain-fed conditions. Soil Use Manage. 23, 100–107. doi: 10.1111/j.1475-2743.2006.00068.x
Villamil, M. B., Bollero, G. A., Darmody, R. G., Simmons, F. W., and Bullock, D. G. (2006). No-till corn/soybean systems including winter cover crops: Effects on soil properties. Soil Sci. Soc. Am. J. 70, 1936–1944. doi: 10.2136/sssaj2005.0350
Wang, G. Y., Hu, Y. X., Liu, Y. X., Ahmad, S., and Zhou, X. B. (2021). Effects of supplement irrigation and nitrogen application levels on soil carbon–nitrogen content and yield of one-year double cropping maize in subtropical region. Water 13:1180. doi: 10.3390/w13091180
Wang, K., Hooks, C. R., and Marahatta, S. P. (2010). Use of a strip-till cover crop system to manipulate above and below ground organisms in cucurbit plantings. Phytopathology 100, S133–S133.
Wawan, Dini, I. R., and Hapsoh. (2019). “The effect of legume cover crop Mucuna bracteata on soil physical properties, runoff and erosion in three slopes of immature oil palm plantation,” in Proceedings of the 2018 International Conference on Sustainable Agriculture for Rural Development, Purwokerto.
Weaver, M. A., Krutz, L. J., Zablotowicz, R. M., and Reddy, K. N. (2007). Effects of glyphosate on soil microbial communities and its mineralization in a Mississippi soil. Pest Manage. Sci. 63, 388–393. doi: 10.1002/ps.1351
Wegner, B. R., Chalise, K. S., Singh, S., Lai, L., Abagandura, G. O., Kumar, S., et al. (2018). Response of soil surface greenhouse gas fluxes to crop residue removal and cover crops under a corn-soybean rotation. J. Environ. Qual. 47, 1146–1154. doi: 10.2134/jeq2018.03.0093
Weinert, T. L., Pan, W. L., Moneymaker, M. R., Santo, G. S., and Stevens, R. G. (2002). Nitrogen recycling by nonleguminous winter cover crops to reduce leaching in potato rotations. Agron. J. 94, 365–372. doi: 10.2134/agronj2002.0365
Werle, R., Mobli, A., Striegel, S., Arneson, N., Dewerff, R., Brown, A., et al. (2021). Large Scale Evaluation of 2, 4-D Choline Off-Target Movement and Injury in 2, 4-D-susceptible Soybean. Available online at: https://www.wiscweeds.info/img/Update%20Series%20Articles/WWRUS%20Article%206_Large%20Scale%2024D%20Choline%20Drift.pdf (accessed January 3, 2022).
White, C. M., and Weil, R. R. (2010). Forage radish and cereal rye cover crop effects on mycorrhizal fungus colonization of maize roots. Plant Soil 328, 507–521. doi: 10.1007/s11104-009-0131-x
White, K. E., Brennan, E. B., and Cavigelli, M. A. (2020). Soil carbon and nitrogen data during eight years of cover crop and compost treatments in organic vegetable production. Data Brief 33:106481. doi: 10.1016/j.dib.2020.106481
Whitehead, W., and Singh, B. P. (2010). Impact of inorganic nitrogen and legume-non legume cover crops on aboveground biomass yields and leaf area index of two sweet corn cultivars. Hortscience 45, S172–S172.
Wortman, S. E., Drijber, R. A., Francis, C. A., and Lindquist, J. L. (2013). Arable weeds, cover crops, and tillage drive soil microbial community composition in organic cropping systems. Appl. Soil Ecol. 72, 232–241. doi: 10.1016/j.apsoil.2013.07.014
Xavier, F. A. D. S., Maia, S. M. F., Ribeiro, K. A., De Sá Mendonça, E., and De Oliveira, T. S. (2013). Effect of cover plants on soil C and N dynamics in different soil management systems in dwarf cashew culture. Agric. Ecosyst. Environ. 165, 173–183.
Xi, Z., Li, H., Long, Y., Zhang, J., and Pang, X. (2010). Variation of soil microbial populations and relationships between microbial factors and soil nutrients in cover cropping system of vineyard. Acta Hortic. Sin. 37, 1395– 1402.
Xiao, X., Tang, H., Nie, Z., Guo, L., Liu, Z., Tang, W., et al. (2013). Effects of winter cover crop straw recycling on soil organic carbon and soil carbon pool management index in paddy fields. Chin. J. Eco Agric. 21, 1202–1208. doi: 10.13287/j.1001-9332.201702.009
Xiong, Z., Xing, G., Tsuruta, H., Shen, G., Shi, S., and Du, L. (2002). Measurement of nitrous oxide emissions from two rice-based cropping systems in China. Nutr. Cycl. Agroecosyst. 64, 125–133. doi: 10.1038/srep17774
Yan, X., Akiyama, H., Yagi, K., and Akimoto, H. (2009). Global estimations of the inventory and mitigation potential of methane emissions from rice cultivation conducted using the 2006 Intergovernmental Panel on Climate Change Guidelines. Global Biogeochem. Cycles 23:GB2002.
Zhao, X., Wang, S., and Xing, G. (2015). Maintaining rice yield and reducing N pollution by substituting winter legume for wheat in a heavily-fertilized rice-based cropping system of southeast China. Agric. Ecosyst. Environ. 202, 79–89.
Zhou, L., Wei, J., Tang, X., Cheng, X., Xiao, Z., Xu, H., et al. (2016). Effects of winter green manure crops with and without chicken rearing on microbial biomass and effective carbon and nitrogen pools in a double-crop rice paddy soil. Acta Prataculturae Sin. 25, 103–114.
Zhou, T., Jiao, K., Qin, S., and Lyu, D. (2019). The impact of cover crop shoot decomposition on soil microorganisms in an apple orchard in northeast China. Saudi J. Biol. Sci. 26, 1936–1942. doi: 10.1016/j.sjbs.2019.07.004
Zhou, Y., Zhu, H., and Yao, Q. (2017). “Improving soil fertility and soil functioning in cover cropped agroecosystems with symbiotic microbes,” in Agro-Environmental Sustainability, eds J. Singh and G. Seneviratne (Cham: Springer), 149–171. doi: 10.1007/978-3-319-49724-2_8
Zhu, B., Yi, L., Guo, L., Chen, G., Hu, Y., Tang, H., et al. (2012). Performance of two winter cover crops and their impacts on soil properties and two subsequent rice crops in Dongting Lake Plain, Hunan, China. Soil Tillage Res. 124, 95–101. doi: 10.1016/j.still.2012.05.007
Zibilske, L. M., and Makus, D. J. (2009). Black oat cover crop management effects on soil temperature and biological properties on a Mollisol in Texas, USA. Geoderma 149, 379–385. doi: 10.1016/j.geoderma.2009.01.001
Keywords: cover crops, soil microbial community structure, greenhouse gas emission, decomposition, cover crop management practices
Citation: Muhammad I, Lv JZ, Wang J, Ahmad S, Farooq S, Ali S and Zhou XB (2022) Regulation of Soil Microbial Community Structure and Biomass to Mitigate Soil Greenhouse Gas Emission. Front. Microbiol. 13:868862. doi: 10.3389/fmicb.2022.868862
Received: 03 February 2022; Accepted: 28 February 2022;
Published: 25 April 2022.
Edited by:
Hafiz M. N. Iqbal, Monterrey Institute of Technology and Higher Education (ITESM), MexicoReviewed by:
Babar Usman, Kyung Hee University, South KoreaMujeeb Ur Rahman, Poznań University of Medical Sciences, Poland
Copyright © 2022 Muhammad, Lv, Wang, Ahmad, Farooq, Ali and Zhou. This is an open-access article distributed under the terms of the Creative Commons Attribution License (CC BY). The use, distribution or reproduction in other forums is permitted, provided the original author(s) and the copyright owner(s) are credited and that the original publication in this journal is cited, in accordance with accepted academic practice. No use, distribution or reproduction is permitted which does not comply with these terms.
*Correspondence: Jun Wang, wangj@nwu.edu.cn; Xun Bo Zhou, xunbozhou@gmail.com
†These authors have contributed equally to this work