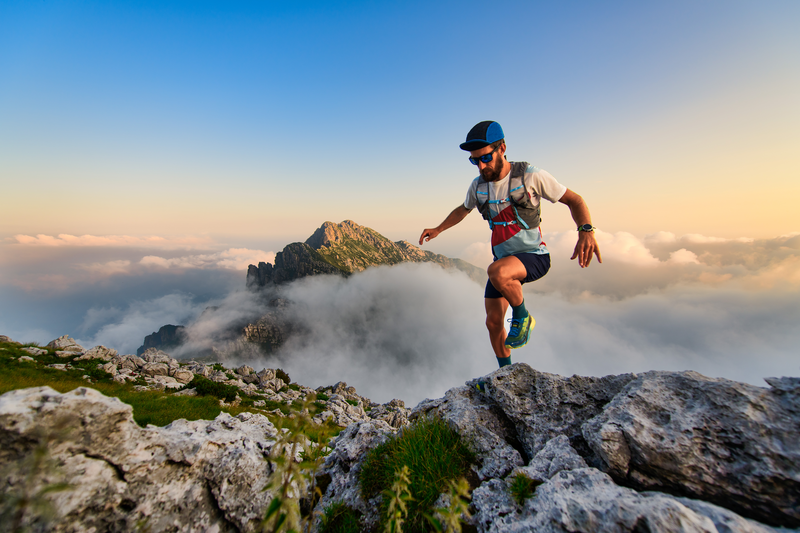
94% of researchers rate our articles as excellent or good
Learn more about the work of our research integrity team to safeguard the quality of each article we publish.
Find out more
ORIGINAL RESEARCH article
Front. Microbiol. , 13 May 2022
Sec. Evolutionary and Genomic Microbiology
Volume 13 - 2022 | https://doi.org/10.3389/fmicb.2022.864053
Nitrogen cycle is an essential process for environmental health. Dirammox (direct ammonia oxidation), encoded by the dnfT1RT2ABCD cluster, was a novel pathway for microbial N2 production defined in Alcaligenes ammonioxydans HO-1. Here, a copy of the cluster dnfT1RT2ABCD as a whole was proved to have existed and very conserved in all Alcaligenes genomes. Phylogenetic analyses based on 16S rRNA gene sequences and amino acid sequences of DnfAs, together with G + C content data, revealed that dnf cluster was evolved associated with the members of the genus Alcaligenes. Under 20% O2 conditions, 14 of 16 Alcaligenes strains showed Dirammox activity, which seemed likely taxon-related. However, the in vitro activities of DnfAs catalyzing the direct oxidation of hydroxylamine to N2 were not taxon-related but depended on the contents of Fe and Mn ions. The results indicated that DnfA is necessary but not sufficient for Dirammox activity. The fact that members of the genus Alcaligenes are widely distributed in various environments, including soil, water bodies (both freshwater and seawater), sediments, activated sludge, and animal–plant-associated environments, strongly suggests that Dirammox is important to the nitrogen cycle. In addition, Alcaligenes species are also commonly found in wastewater treatment plants, suggesting that they might be valuable resources for wastewater treatment.
The nitrogen cycle is important for nitrogen balance in the biosphere and global environmental health (Gruber and Galloway, 2008). Biological nitrogen removal of fixed nitrogen is not only an essential part of the natural nitrogen cycle (Gruber and Galloway, 2008), but also the basic principle in the development of nitrogen removal technologies in wastewater treatment (Kuypers et al., 2018). Up to date, only two pathways have been identified and recognized as biological N2 generation pathways, namely, nitrification/denitrification (Van Cleemput and Samater, 1995; Sigman and Fripiat, 2019) and anaerobic ammonia oxidation (anammox) (Mulder et al., 1995; Strous et al., 1999). In nitrification/denitrification pathway, ammonia oxidation (NH4+ → NH2OH → NO2– → NO3–) is the energy source of autotrophic nitrifers, ammonia can be oxidized aerobically via hydroxylamine to nitrite, nitrate, or nitrogenous gas by a single-strain Nitrospira species (comammox, complete ammonia oxidation), or by a combination of ammonia-oxidizing bacteria or ammonia-oxidizing archaea, and nitrite-oxidizing bacteria (Abell et al., 2010; Pester et al., 2012; Baptista et al., 2014). The enzyme ammonia monooxygenase catalyzes the oxidation of ammonia to hydroxylamine (Ensign et al., 1993; Gilch et al., 2009), which is then converted to NO by hydroxylamine oxidoreductase (Maalcke et al., 2014; Caranto and Lancaster, 2017). In denitrification pathway, nitrate or nitrite is sequentially reduced to N2 (NO3– → NO2– → NO → N2O → N2) when O2 is limited (Skiba, 2008). In anammox pathway, N2 is produced from oxidation of ammonia, with nitrite produced by nitrifiers as the electron acceptor (NH4+ + NO2– → N2 or NH4 + + 1.32 NO2– + 0.066 HCO3– + 0.13 H+ → 1.02 N2 + 0.26 NO3– + 0.066 CH2O0.5N0.15 + 2.03 H2O) under anaerobic conditions by anammox bacteria (phylum Planctomycetes) (Mulder et al., 1995; van de Graaf et al., 1996; Strous et al., 1999).
We previously isolated and characterized Alcaligenes ammonioxydans strain HO-1 (Wu et al., 2021) from a SHARON bioreactor treating ammonium-rich piggery wastewater (Du et al., 2016). Strain HO-1 could be tolerant to high ammonia load up to 3,400 mg/L and directly convert ammonia via hydroxylamine to N2 under aerobic conditions (Wu et al., 2021). The high ammonia tolerance property makes it a potential strain in wastewater treatment plants (WWTPs). Furthermore, it also demonstrated its ability to directly convert ammonia to N2 under aerobic conditions as a result of a novel pathway, termed Dirammox (direct ammonia oxidation) (Wu et al., 2021). It was also demonstrated that the production of N2 from ammonia in HO-1 was the activity of Dirammox, but not that of nitrite denitrification (Wu et al., 2021). This result was further confirmed in strain JQ135 (Xu et al., 2022), another Alcaligenes strain with the same ability of Dirammox as HO-1. The knockout of denitrification pathway in strain JQ135 did not change its N2 yield under aerobic conditions (Xu et al., 2022). This novel pathway is conducted by enzymes encoded by the gene cluster dnfT1RT2ABCD defined in the genome of HO-1; and just dnfABC could enable recombinant Escherichia coli strain to generate hydroxylamine and N2 from ammonia (Wu et al., 2021). These seven genes were annotated as 3-phosphoserine/phosphohydroxythreonine transaminase, PLP-dependent aminotransferase family protein, serine hydroxymethyltransferase, diiron N-oxygenase, 2Fe–2S iron–sulfur cluster binding domain-containing protein, glutamine amidotransferase, and pyridoxine/pyridoxal/pyridoxamine kinase, respectively (Wu et al., 2021). Among the seven genes, only dnfA encoded an N-oxygenase catalyzing the oxidation of hydroxylamine to N2, and its deletion resulted in the total loss of Dirammox activity in strain JQ135 (Xu et al., 2022). Therefore, dnfA was selected as a marker gene of Dirammox pathway for the following investigations.
Dirammox is quite different from the two known N2 production pathways mentioned above, namely, (1) N2 generation in Dirammox is quite efficient under physiological O2 levels by a single heterotrophic bacterium, while denitrification and anammox occur anaerobically; and (2) Dirammox is the simplest N loss process, of which N2 is directly generated from ammonia NH3+ (-NH2) via hydroxylamine by a single bacterium such as A. ammonioxydans HO-1. However, N2 produced by nitrification/denitrification is the result of the subsequent cooperation of multiple strains as mentioned above. Although an anammox bacterium could oxidize ammonia directly to N2 with nitrite as the final electron acceptor, it needs the cooperation of nitrifiers for providing nitrite (Kuypers et al., 2018).
Currently, there are five species in the genus, namely, Alcaligenes faecalis, A. aquatilis (Van Trappen et al., 2005), A. pakistanensis (Abbas et al., 2015), A. endophyticus (Lu et al., 2017), and A. ammonioxydans HO-1 (Wu et al., 2021). Members of the genus Alcaligenes are widely distributed in natural environments such as soil (Liu X. et al., 2016), water (Regar et al., 2016), as well as human and other vertebrates (Simmons et al., 1981; Kaliaperumal et al., 2006; Laham et al., 2017). With the disclosed Dirammox in Alcaligenes strain HO-1 (Wu et al., 2021), for which the N2 produced aerobically is not related to aerobic denitrification, whether the nitrogen removal of Alcaligenes strains comes from heterotrophic nitrification and aerobic denitrification (HNAD, the process: NH4+ → NH2OH → NO2– → NO3– → NO2– → NO → N2O → N2) (Wehrfritz et al., 1993; Richardson et al., 1998, Stein, 2011) or the novel pathway remained to be clarified.
However, it is still not clear (1) whether Dirammox is widely distributed in Alcaligenes or not and (2) whether N2 generation is exclusively from Dirammox or the combination of Dirammox and HNAD under physiological conditions in Alcaligenes strains? In this study, the distribution of dnfT1RT2ABCD in Alcaligenes genomes was analyzed widely by GenBank. Meanwhile, the sequences of this gene cluster defined in the genomes were further compared and phylogenetically studied. Furthermore, 16 Alcaligenes strains were obtained and investigated for their Dirammox and denitrification activities using stable isotope incubations. In addition, the representatives of each phylogenetic type of DnfA were investigated for their enzymatic activity catalyzing oxidation of hydroxylamine to N2. The results provided insights into the distribution and evolution of Dirammox in the genus Alcaligenes, activity of Dirammox among Alcaligenes strains, as well as its mechanism, and thereby the possible importance of Dirammox to nature.
To investigate the distribution of gene cluster dnfT1RT2ABCD in Alcaligenes genomes, DnfT1, DnfR, DnfT2, DnfA, DnfB, DnfC, and DnfD of strain HO-1 were used as a query individually against the non-redundant protein sequences database (NR) restricted to Alcaligenes genomes to perform BlastP program in NCBI blast web (Johnson et al., 2008), respectively. The algorithm parameter “Max target sequences” was adjusted to 5,000. The posted back results were filtered with conditions, namely, expect threshold ≤0.001, query coverage ≥70%, identity ≥30%, and extract the accession numbers of proteins. Get identical protein groups by the above accession numbers using the NCBI Batch Entrez tool. Then, detailed information about each homologous protein was obtained, including nucleotide accession number, start and stop site in nucleotide, strand, accession number of protein, protein name, organism, strain, and assembly. Finally, only the genomes containing all the seven genes of the dnf cluster arranged in one DNA fragment of the strains of a bacterial species were screened as the genomes containing gene cluster dnfT1RT2ABCD.
Phylogenetic trees based on 16S rRNA gene sequences or amino acid sequences of DnfAs were reconstructed using the neighbor-joining method (Saitou and Nei, 1987) performed in MEGA X software (Kumar et al., 2018) with 1,000 bootstrap resamplings. Clustal Omega (Sievers and Higgins, 2013) or Clustal W (Thompson et al., 1994) was used to align multiple sequences. ESPript 3.01 (Robert and Gouet, 2014) with default setting was used to render sequence similarities.
Bacterial strains and plasmids used in this study are listed in Table 1. Luria–Bertani medium (LB) was prepared according to Liu Y. et al. (2016). The basal medium was prepared by dissolving KH2PO4 0.50 g, Na2HPO4⋅12H2O 1.25 g, MgSO4⋅7H2O 0.2 g, NaCl 10 g, and trace element solution 2 ml in 1 L of distilled water, pH 7.0–7.5. The trace element solution was prepared according to Joo et al. (2005). A total of 4.72 g of succinate was added as carbon source in the basal medium per liter. Ammonia or nitrite was added as nitrogen source as needed listed in Table 2. Alcaligenes strains were cultivated at 30°C, 160 rpm in synthetic media (Table 2). E. coli strains were cultivated at 37°C in LB media. Ampicillin (100 mg/L) was supplemented with media as necessary. For agar plates, 1.5% (w/v) of agar was added to the media.
Primers used in this study are listed in Table 3. Primer pair dnfA-upF/dnfA-downR targeting complete dnfA were designed based on upstream and downstream sequences of dnfAs of Alcaligenes fetched from GenBank. High-fidelity DNA polymerase TransStart® FastPfu (Transgen, China) was used. The PCR products were sequenced by a commercial company. Finally, the start and end codons were determined by aligning with dnfA sequence of strain HO-1. EMBOSS Transeq2 was applied to translate nucleotides into proteins. These sequence data are available in the GenBank3 under accession number OM293488-511.
Growth (OD600) and hydroxylamine were monitored over time when ammonia (medium A, Table 2) was employed as the sole nitrogen source in Erlenmeyer flasks. The ability to grow on nitrite as the sole nitrogen source (medium D, Table 2) was determined over time in sealed bottles as previously described (Wu et al., 2021) but without displacement of the gas of the headspace, in which the seeds were prepared in agar plates, resuspended, and injected into the bottles.
The ability to convert ammonia to N2 was tested with 15N2 production from (15NH4)2SO4 (medium B, Table 2) or in the presence of nitrite (medium C, Table 2). For detection of gaseous nitrogen products (N2 and N2O) of Dirammox and aerobic denitrification, strains were cultivated in media B or E (Table 2) in air-tight bottles prepared according to the previous description (Wu et al., 2021). All samples were assayed when ammonia and formed hydoxylamine were completely consumed.
15N-labeled hydroxylamine (15NH2OH), Na15NO2, and (15NH4)2SO4 were all 99 atom% 15N and got from Cambridge Isotope Laboratories Inc., United States.
In this study, the gene sequences of WP_009459326.1, WP_137431195.1, and HCA15598.1 were obtained from GenBank and synthesized by a commercial company, then the obtained genes were cloned into pET-21a(+) (Table 1). For construction of other plasmids listed in Table 1, PCR products amplified by TransStart® FastPfu were purified and digested with BamHI (NEB) and HindIII (NEB). The digested amplicons and BamHI/HindIII digested pET-21a(+) plasmid were ligated by T4 ligase (Takara) at 4°C overnight. For expression of DnfA and its s, E. coli Transetta (DE3) containing pET-21a ligating dnfA or dnfAs from other Alcaligenes strains was cultured in LB supplemented with 100 μg/ml ampicillin at 37°C on a rotary shaker (160 rpm) to OD600 = 0.3–0.6, then induced with 0.5 mM isopropyl-β-D -thiogalactopyranoside on a rotary shaker (160 rpm) for another 20 h at 16°C. DnfA or its s was purified individually using Ni-NTA resin (QIAGEN) with the following procedures, namely, cells were harvested by centrifugation (5,000 × g, 30 min, 4°C), resuspended in buffer A (100 mM Tris-HCl, 100 mM NaCl, 10 mM imidazole, pH 8.0), lysed by ultrasonication, and centrifuged (14,000 × g, 30 min, 4°C). Supernatant was applied to Ni-NTA resin (QIAGEN) column pre-equilibrated with buffer A. Ni-NTA matrix was washed with buffer A and added with 10–50 mM imidazole to remove impurities. 6xHis-tagged proteins were eluted with buffer B (100 mM Tris-HCl, 100 mM NaCl, 250 mM imidazole, pH 8.0), desalted with centrifugal filter devices (MilliporeSigma), and excess imidazole was removed with PD-10 desalting column (GE Healthcare).
To analyze the distribution of the genus Alcaligenes in nature, BlastN was performed using the 16S rRNA gene sequence (1542 nt) of strain HO-1 as a query against the nucleotide database (NT). Only the sequences with coverage >70% and similarity >95% were included. The isolation source or sample source was extracted and used to analyze the environmental distribution of the genus Alcaligenes.
In vitro enzymatic assays, DnfA, and its homologs catalyzing the conversion of hydroxylamine to N2 were carried out in 300 μl reaction mixtures in 20 mM buffer Tris-HCl (pH 8.5) containing 330 μM DnfA or its homologs, 10 mM 15NH2OH, 10 mM NADH, and 20 μM FAD, as described in our preprint4 (Wu et al., 2020). Mixtures lacking DnfA were used as the control. The reaction was started with the addition of 15NH2OH, and the mixture was directly injected into 10 ml gastight tubes whose top air had been completely replaced by 20% O2 and 80% He mixed gas. The reactions were incubated at 30°C without agitation in the dark for 100 min. Enzymatic assays were performed in triplicate.
Bacterial growth and concentrations of ammonium (NH4+) of nitrite (NO2–), nitrate (NO3–), hydroxylamine, 15N2, and 15N2O were determined as described previously (Wu et al., 2021), except nitrite determine in enzymatic activity system, which was monitored by ion chromatography. For determination of gas products, strain cultivation and DnfA enzymatic reaction were performed in 250 ml sealed bottles containing 20 ml media or 10 ml gastight tube with 300 μl reaction system, respectively. The air was replaced completely by 80% He and 20% O2 mixed gas. PerkinElmer Optima 5300EV ICP-OES was used for the determination of atoms, including Fe, Mn, Ca, Cu, K, Mg, Ni, P, Zn, Si, and S. Results are shown as mean ± SD. The correlation relationship was demonstrated by Pearson’s correlation coefficient.
All the available Alcaligenes genomes in GenBank (updated to May 2015) were analyzed for the presence of the gene cluster dnfT1RT2ABCD. As shown in Figure 1, a copy (and only one copy) of the gene cluster dnfT1RT2ABCD as a whole was defined in the 36 well-assembled Alcaligenes genomes (totally 49 genomes of unique Alcaligenes strain), including all 19 complete genomes, with the same arrangement and position as those in HO-1 (Figure 1). Among them, A. faecalis was the most dominant, with 29 genomes containing dnfT1RT2ABCD, followed by unnamed Alcaligenes strains (four genomes) and A. aquatilis (three genomes) (Figure 1B). In addition, parts of the dnf cluster were also defined in the rest of the 15 low-level assembled genomes of the total 49 Alcaligenes genomes of unique strain deposited in GenBank. Furthermore, the dnf clusters from different Alcaligenes genomes exhibited very high sequence similarity (>97% for dnfABC), suggesting that the dnf cluster was very conserved in the genus Alcaligenes. Based on these results, it was deduced that the conserved dnf cluster and so the Dirammox were commonly distributed in the genus Alcaligenes.
Figure 1. Arrangement (A) and statistics on distribution (B) of dnfT1RT2ABCD among the genomes of the genus Alcaligenes. Here, A. faecalis includes all three subspecies, namely, A. faecalis subsp. faecalis, A. faecalis subsp. Parafaecalis, and A. faecalis subsp. phenolicus. There are 49 genomes of unique Alcaligenes strains in GenBank updated to May 2021, and strain HO-1 was recognized as one of Alcaligenes sp.
A total of 16 Alcaligenes strains (Table 1) were obtained for verifying the existence of Dirammox pathway and nitrogen removal ability. As shown in Figure 2A, ammonia could be used as the sole nitrogen source for aerobic growth of all Alcaligenes strains. In contrast, no growth for all tested strains was observed on nitrite as the sole nitrogen source (Figure 2A), suggesting that Alcaligenes spp. have no the ability to assimilate nitrite. Analysis of the gaseous nitrogen products indicated that most of the tested Alcaligenes strains (except of strains RL12 and CGMCC 1.1799) could aerobically convert 15NH4+ to 15N2 when 15NH4+ was used as the sole nitrogen source, in spite of their difference in N2-producing ability (Figure 2B). The results in Figure 2B also indicate that 15N2 amount released was not affected by the addition of nitrite. Figure 2C displays the gaseous nitrogen products released from aerobic denitrification of nitrite by the strains tested. The results showed that no tested strain could produce 15N2 from nitrite denitrification, and most of them could release 15N2O from 15NO2– (medium E, in Table 2) under aerobic conditions. All these results were in good agreement with those of HO-1 (Wu et al., 2021). These results strongly suggested that the production of N2 resulted from the direct conversion of ammonia in Alcaligenes spp. Therefore, it was concluded that the activity of Dirammox was the only way to produce N2 in Alcaligenes spp. under aerobic conditions.
Figure 2. Growth and Dirammox (direct ammonia oxidation) activity of Alcaligenes strains in different medium. (A) Aerobic growth in medium A and medium D, and OD600 shown here were data of stationary growth phase. (B) 15N2 release from (15NH4)2SO4 (initial amount 100 μmol) in the absence or presence of NaNO2 (medium B and medium C, respectively). (C) Gaseous products of aerobic denitrification of Alcaligenes strain in medium E (60 μmol of initial amount of Na15NO2).
Figure 3 depicts the results of phylogenetic analyses of the genus Alcaligenes. It is noticed that the topology of the tree based on 16S rRNA gene sequences (Figure 3A, Table 4) was almost the same as that of the tree based on the amino acid sequences of DnfAs (Figure 3B), and both trees can be divided into two clades, namely, clade I and clade II (Figures 3A,B). Dirammox activities of Alcaligenes spp. seemed likely taxon-related, as indicated by the right column of (A) showing the heatmap of conversion (%) of consumed 15NH4+ to 15N2 in medium B, the members in clade I exhibited lower Dirammox activities than those in clade II with the exception of strains CGMCC 1.10269 and CGMCC 1.1799 (Figure 3), both strains had very low or no Dirammox activities. Furthermore, the G + C contents of dnf clusters of Alcaligenes spp. were all around 56.3%, a value very close to that (∼57.2%) of the genomes of Alcaligenes spp. These results, together with the very high sequence similarity of the dnf clusters among Alcaligenes species, significantly referred that the dnf cluster was evolved associated with the members of the genus Alcaligenes. This means that Alcaligenes species obtain dnf cluster and so the Dirammox genetic potential ability from heredity, but not from horizontal gene transfer from other microbes.
Figure 3. Phylogenetic analyses of the genus Alcaligenes based on 16S rRNA gene sequences (A) and amino acid sequences of DnfAs (B). The protein accession numbers shown in parentheses represent the best hit of DnfA against NR database (the same in Table 4). The columns on the right of (A) represent the heatmap showing the conversion (%) of consumed 15NH4+ to 15N2 in medium B.
Results of multiple sequence alignment of DnfA and its homologs (DnfAs) from Alcaligenes species showed only a few amino acid differences (Figure 4A), suggesting that the Alcaligenes DnfAs share the same function. In addition, Figure 4A also shows that Alcaligenes DnfAs can be divided into 14 subtypes. Among them, DnfA with accession number WP_042487153.1 was the most abundant one that existed in 18 genomes, followed by DnfA with accession number WP_035272004.1, which existed in five genomes (Figure 4B). To verify the functions of DnfAs, some representatives were selected and their genes were obtained by PCR amplification or gene synthesis for their enzymatic activity assays.
Figure 4. Multiple-sequence alignment (A) and statistics (B) of Alcaligenes DnfAs. Accession numbers of the proteins (DnfAs, or DnfA homologs) are shown. All genomes (49) in Figure 1 were analyzed, and five of them with incomplete DnfA sequence were excluded.
It was found that DnfAs could catalyze the direct oxidation of hydroxylamine to N2 with a molecular ratio of 2:1 in the presence of molecular O2, FAD, and NADH. Therefore, in vitro reconstitution enzymatic activity assays using FAD as the chemical electron mediator were performed for DnfAs as shown in Figure 5, according to previous method (Choi et al., 2008). The results (Figure 5B) indicated that all the enzyme reaction samples could release 15N2 from 15NH2OH, in spite of the different amounts of 15N2 released, suggesting that all tested DnfAs could oxidize hydroxylamine directly to N2. These results also showed that the different original DnfAs exhibited different enzyme activity. DnfAs with accession numbers WP_042487153.1 and WP_045930341.1 released 0.63 ± 0.01 and 0.43 ± 0.04 μmol 15N2-N, respectively, showing the lowest activity (Figure 5B). The results also suggested that the in vitro enzymatic activities of the tested DnfAs seemed likely none phylogenetically related, a phenomenon quite different from the Dirammox activities of Alcaligenes strains (Figure 3). Furthermore, when combined with the results presented in Figure 3, an interesting fact was noticed. The Alcaligenes strains with low in vitro DnfA activity also exhibited low Dirammox activity, such as strains RL12, CGMCC 1.2006T, PC01, and DSM 16503T. In contrast, the strains with low Dirammox activity may harbor DnfA of high in vitro activity, such as strain CGMCC 1.3937.
Figure 5. Hydroxylamine oxidation activities of DnfAs from Alcaligenes strains. Phylogenetic tree of the representatives of all DnfA homologs defined in the genomes of all Alcaligenes strains deposited in GenBank (A); 15N2 release of the representatives of DnfA homologs from 15NH2OH under 20% O2 and 80% He in vitro (B). The content of Fe and Mn atoms of DnfAs (C). The data are shown as the mole content of metal atoms per mole of DnfA.
Based on homology alignment against the public database, DnfA was annotated as a diiron oxygenase and related to the AurF N-oxygenase (Choi et al., 2008; Leipoldt et al., 2017) and CmlIs (Knoot et al., 2016; Komor et al., 2018). Similarly, DnfAs are predicted to contain a diiron motif. In this case, atoms of DnfAs were determined. Only Fe and Mn existed in DnfA, and the results (Figure 5C) showed that all the tested DnfAs contained both Fe and Mn atoms with contents ranging from 0.11 ± 0.00 to 1.60 ± 0.01 and from 0.03 ± 0.00 to 0.48 ± 0.00 mmol per mmol of protein, respectively (Figure 5C). Notably, a positive correlationship was defined between the in vitro activities (release amount of 15N2) of DnfAs and the contents of Fe, Mn, or Fe + Mn with correlation coefficient r = 0.92, 0.86, and 0.93, respectively.
The above results indicated that Dirammox pathway was commonly distributed in the genus Alcaligenes, in spite of a few Alcaligenes species exhibiting low or even no ability of transforming ammonia to N2 under 20% oxygen. In this case, the distribution of the genus Alcaligenes in environments was investigated first based on 16S rRNA gene sequences.
For concise purpose, samples were briefly divided into five categories, namely, soil, water, sediment, sludge, and animal–plant-associated, according to their sources. Each category includes samples from both natural environments and human-made surroundings. Clinical samples and those directly derived from animals or plants were classified as animal/plant-associated samples. A total of 824 different Alcaligenes 16S rRNA gene sequences with definite environment/source information were defined in NT database of the GenBank. The results (Figure 6) showed that there were more Alcaligenes 16S rRNA gene sequences (∼35%, 288/824) from animal/plant-associated samples than other samples, followed by soil samples (27.3%, 225/824). Also, there were 11.7, 6.90, and 4.85% (96, 57, and 40 of 824, respectively) of the Alcaligenes 16S RNA gene sequences from samples of water, sludge, and sediment, respectively. These results indicated that members of the genus Alcaligenes are widely distributed in various environments with quite high biodiversity, in good agreement with the results described in the literature as mentioned in the “Introduction” section 1. Therefore, the genus Alcaligenes is an important conductor of elemental biogeochemical cycles, including the nitrogen cycle, and so the Dirammox pathway is probably important to the nitrogen cycle since most members of the genus Alcaligenes could exhibit Dirammox activity (Figure 2B). In addition, approximately 31% (256/824) Alcaligenes strains were derived from WWTPs or various industrial effluents and agricultural wastewater. This means that Alcaligenes might also be efficient candidates for wastewater treatment.
Figure 6. Distribution of the genus Alcaligenes in nature. Categories of samples and numbers of Alcaligenes 16S rRNA gene sequences are indicated. The results were based on the analysis of 16S rRNA gene sequences defined in GenBank of NCBI database.
In our previous work, a novel microbial N2 production pathway, termed Dirammox, was defined in Alcaligenes strain HO-1 and encoded by the gene cluster dnfT1RT2ABCD (Wu et al., 2021). Recently, dnfABC was further proved to be the genetic basis for Dirammox and regulated by dnfR in another Alcaligenes strain, JQ135 (Xu et al., 2022). Here, it was found that high homogeneity of Dirammox was among the members of the genus Alcaligenes. Our results showed that the production of N2 from ammonia by an Alcaligenes strain must be the results of Dirammox pathway, but not the results of aerobic denitrification that could only emit N2O (Figure 2) under aerobic conditions. The results are quite different from previous studies on nitrogen removal of Alcaligenes strains (van Niel et al., 1992; Joo et al., 2006; Liu et al., 2015; Shoda and Ishikawa, 2016; Chen et al., 2021), which all attributed to N2 producing or nitrogen removal to HNAD. Although it was also speculated that N2 generation in Alcaligenes strains was through hydroxylamine (NH4+ → NH2OH → N2O → N2) (Joo et al., 2006; Zhao et al., 2012), the pathway and molecular basis remained unclear. Here, we proved the use of Dirammox, a process of direct oxidization of ammonia to N2 via hydroxylamine in Alcaligenes strains.
In this study, this gene cluster dnfT1RT2ABCD as a whole was investigated for its distribution in members of the genus Alcaligenes, and it was found that dnf cluster not only existed in all members of the genus Alcaligenes (Figure 1), but also was highly conserved among Alcaligenes species. Phylogenetic data revealed that DnfA was taxon-related (Figure 3), referring to the Alcaligenes species acquiring Dirammox pathway by heredity but not horizontal gene transfer from other microbes, which in turn determines different Dirammox activities among Alcaligenes members in different clades. The function and activity of DnfA are of course important, but other conditions, such as low or even no transcription or expression of dnf genes and the activity of DnfB or DnfC, will also influence the Dirammox activity. So, we can see strains CGMCC 1.10269 and CGMCC 1.1799 breaking the rule, whose Dirammox activities differ from their very closely related neighbors HO-1 (Figure 3).
DnfA, annotated as a diiron oxygenase, contained both Fe and Mn atoms whose content greatly affected the in vitro activities of DnfA catalyzing the direct oxidation of hydroxylamine to N2. It was reported that Mn and Fe have similar atomic numbers and FeII/FeIII and MnII/MnIII are common active states in many proteins. Although DnfAs were predicted to contain a diiron site, Mn atoms might take up the site in place of Fe atoms. This means that the contents of Fe or Mn or Fe + Mn were a very important factor determining the in vitro activities or even physiological activities of DnfAs, and this needs further investigation in the future. The differences of Fe and Mn atoms among Alcaligenes spp. might result from the sequences of amino acid of DnfA, and the change of some amino acid residues might decrease the combination of protein and Fe and Mn. The reasons for this might be quite complex and need to be further revealed in the future.
With general N2-producing ability of Alcaligenes members and the wide distribution of the genus Alcaligenes in nature as shown above, it was speculated that Dirammox was also important to the nitrogen cycle. Certainly, the Dirammox activity in situ and the assessment of N-fluxes contributed by Dirammox conducted by Alcaligenes or even other bacteria should be investigated urgently in the near future.
In this study, the cluster dnfT1RT2ABCD encoding Dirammox proved to be universally distributed, conserved, and dependently evolved in the genus Alcaligenes. Most of the Alcaligenes strains exhibited Dirammox activities, which seemed likely taxon-related, in spite of their different performance. In contrast, the in vitro activities of DnfAs catalyzing the direct oxidation of hydroxylamine to N2 were not taxon-related but depended on the contents of Fe and Mn ions. Dirammox might be important to the nitrogen cycle, as well as the environment, since the genus Alcaligenes was widely distributed in environment.
The datasets presented in this study can be found in online repositories. The names of the repository/repositories and accession number(s) can be found in the article/supplementary material.
Z-PL and S-JL contributed to conception. L-LM and T-TH designed the study, analyzed data, and wrote the first draft of the manuscript. T-TH and LM contributed to the data collection. Z-PL reviewed and edited the manuscript. J-SP, QH, YL, and M-RW provided resources for the study. G-MA built the N2 and N2O detection methods. All authors contributed to manuscript revision, read and approved the submitted version.
This study was supported by programs from the National Natural Science Foundation of China (Grant Nos. 91951101 and 31870103) and National Key R&D Program of China (Grant No. 2019YFA0905500).
The authors declare that the research was conducted in the absence of any commercial or financial relationships that could be construed as a potential conflict of interest.
All claims expressed in this article are solely those of the authors and do not necessarily represent those of their affiliated organizations, or those of the publisher, the editors and the reviewers. Any product that may be evaluated in this article, or claim that may be made by its manufacturer, is not guaranteed or endorsed by the publisher.
The authors acknowledge the important support given by Lin-Jun Wu, who wrote all codes in this study.
Abbas, S., Ahmed, I., Iida, T., Lee, Y.-J., Busse, H.-J., Fujiwara, T., et al. (2015). A heavy-metal tolerant novel bacterium, Alcaligenes pakistanensis sp. nov., isolated from industrial effluent in Pakistan. Antonie Van Leeuwenhoek 108, 859–870. doi: 10.1007/s10482-015-0540-1
Abell, G. C. J., Revill, A. T., Smith, C., Bissett, A. P., Volkman, J. K., and Robert, S. S. (2010). Archaeal ammonia oxidizers and nirS-type denitrifiers dominate sediment nitrifying and denitrifying populations in a subtropical macrotidal estuary. ISME J. 4, 286–300. doi: 10.1038/ismej.2009.105
Baptista, J. D., Lunn, M., Davenport, R. J., Swan, D. L., Read, L. F., Brown, M. R., et al. (2014). Agreement between amoA gene-specific quantitative PCR and fluorescence in situ hybridization in the measurement of ammonia-oxidizing bacteria in activated sludge. Appl. Environ. Microbiol. 80, 5901–5910. doi: 10.1128/AEM.01383-14
Caranto, J. D., and Lancaster, K. M. (2017). Nitric oxide is an obligate bacterial nitrification intermediate produced by hydroxylamine oxidoreductase. Proc. Natl. Acad. Sci. U.S.A. 114, 8217–8222.
Chen, J., Xu, J., Zhang, S., Liu, F., Peng, J., Peng, Y., et al. (2021). Nitrogen removal characteristics of a novel heterotrophic nitrification and aerobic denitrification bacteria, Alcaligenes faecalis strain WT14. J. Environ. Manage. 282:111961. doi: 10.1016/j.jenvman.2021.111961
Choi, Y. S., Zhang, H., Brunzelle, J. S., Nair, S. K., and Zhao, H. (2008). In vitro reconstitution and crystal structure of p-aminobenzoate N-oxygenase (AurF) involved in aureothin biosynthesis. Proc. Natl. Acad. Sci. U.S.A. 105, 6858–6863. doi: 10.1073/pnas.0712073105
Du, W.-L., Huang, Q., Miao, L.-L., Liu, Y., and Liu, Z.-P. (2016). Association of running manner with bacterial community dynamics in a partial short-term nitrifying bioreactor for treatment of piggery wastewater with high ammonia content. AMB Express 6:76. doi: 10.1186/s13568-016-0245-5
Ensign, S. A., Hyman, M. R., and Arp, D. J. (1993). In vitro activation of ammonia monooxygenase from Nitrosomonas europaea by copper. J. Bacteriol. 175, 1971–1980. doi: 10.1128/jb.175.7.1971-1980.1993
Gilch, S., Meyer, O., and Schmidt, I. (2009). A soluble form of ammonia monooxygenase in Nitrosomonas europaea. Biol. Chem. 390, 863–873. doi: 10.1515/BC.2009.085
Gruber, N., and Galloway, J. N. (2008). An Earth-system perspective of the global nitrogen cycle. Nature 451, 293–296. doi: 10.1038/nature06592
Johnson, M., Zaretskaya, I., Raytselis, Y., Merezhuk, Y., McGinnis, S., and Madden, T. L. (2008). NCBI BLAST: a better web interface. Nucleic Acids Res. 36, W5–W9. doi: 10.1093/nar/gkn201
Joo, H. S., Hirai, M., and Shoda, M. (2005). Nitrification and denitrification in high-strength ammonium by Alcaligenes faecalis. Biotechnol. Lett. 27, 773–778. doi: 10.1007/s10529-005-5634-9
Joo, H.-S., Hirai, M., and Shoda, M. (2006). Piggery wastewater treatment using Alcaligenes faecalis strain No. 4 with heterotrophic nitrification and aerobic denitrification. Water Res. 40, 3029–3036. doi: 10.1016/j.watres.2006.06.021
Kaliaperumal, S., Srinivasan, R., Gupta, A., and Parija, S. (2006). Postoperative endophthalmitis due to an unusual pathogen: Alcaligenes faecalis. Eye 20, 968–969. doi: 10.1038/sj.eye.6702080
Knoot, C. J., Kovaleva, E. G., and Lipscomb, J. D. (2016). Crystal structure of CmlI, the arylamine oxygenase from the chloramphenicol biosynthetic pathway. J. Biol. Inorg. Chem. 21, 589–603. doi: 10.1007/s00775-016-1363-x
Komor, A. J., Jasniewski, A. J., Que, L., and Lipscomb, J. D. (2018). Diiron monooxygenases in natural product biosynthesis. Nat. Prod. Rep. 35, 646–659. doi: 10.1039/C7NP00061H
Kumar, S., Stecher, G., Li, M., Knyaz, C., and Tamura, K. (2018). MEGA X: molecular evolutionary genetics analysis across computing platforms. Mol. Biol. Evol. 35, 1547–1549. doi: 10.1093/molbev/msy096
Kuypers, M. M. M., Marchant, H. K., and Kartal, B. (2018). The microbial nitrogen-cycling network. Nat. Rev. Microbiol. 16, 263–276.
Laham, N. A., Chavda, K. D., Cienfuegos-Gallet, A. V., Kreiswirth, B. N., and Chen, L. (2017). Genomic characterization of VIM metallo-β-lactamase-producing Alcaligenes faecalis from Gaza, Palestine. Antimicrob. Agents Chemother. 61:e01499-17. doi: 10.1128/AAC.01499-17
Leipoldt, F., Santos-Aberturas, J., Stegmann, D. P., Wolf, F., Kulik, A., Lacret, R., et al. (2017). Warhead biosynthesis and the origin of structural diversity in hydroxamate metalloproteinase inhibitors. Nat. Commun. 8:1965. doi: 10.1038/s41467-017-01975-6
Liu, X., Huang, D., Wu, J., Yu, C., Zhou, R., Liu, C., et al. (2016). The genome sequence of Alcaligenes faecalis NBIB-017 contains genes with potentially high activities against Erwinia carotovora. Genome Announc. 4:e00222-16. doi: 10.1128/genomeA.00222-16
Liu, Y., Ai, G. M., Miao, L. L., and Liu, Z. P. (2016). Marinobacter strain NNA5, a newly isolated and highly efficient aerobic denitrifier with zero N2O emission. Bioresour. Technol. 206, 9–15. doi: 10.1016/j.biortech.2016.01.066
Liu, Y., Wang, Y., Li, Y., An, H., and Lv, Y. (2015). Nitrogen removal characteristics of heterotrophic nitrification-aerobic denitrification by Alcaligenes faecalis C16. Chin. J. Chem. Eng. 23, 827–834.
Lu, C. Y., Li, Y. Q., Tian, Y., Han, M. X., Rao, M. P. N., Li, Y. R., et al. (2017). Alcaligenes endophyticus sp. nov., isolated from roots of Ammodendron bifolium. Int. J. Syst. Evol. 67, 939–943. doi: 10.1099/ijsem.0.001719
Maalcke, W. J., Dietl, A., Marritt, S. J., Butt, J. N., Jetten, M., Keltjens, J. T., et al. (2014). Structural basis of biological NO generation by octaheme oxidoreductases. J. Biol. Chem. 289, 1228–1242. doi: 10.1074/jbc.M113.525147
Málek, I., Radochová, M., and Lysenko, O. (1963). Taxonomy of the species Pseudomonas odorans. Microbiology 33, 349–355. doi: 10.1099/00221287-33-3-349
Mulder, A., van de Graaf, A. A., Robertson, L. A., and Kuenen, J. G. (1995). Anaerobic ammonium oxidation discovered in a denitrifying fluidized bed reactor. FEMS Microbiol. Ecol. 16, 177–183.
Papen, H., von Berg, R., Hinkel, I., Thoene, B., and Rennenberg, H. (1989). Heterotrophic nitrification by Alcaligenes faecalis: NO2–, NO3–, N2O, and NO production in exponentially growing cultures. Appl. Environ. Microbiol. 55, 2068–2072. doi: 10.1128/aem.55.8.2068-2072.1989
Pester, M., Rattei, T., Flechl, S., Grongroft, A., Richter, A., Overmann, J., et al. (2012). amoA-based consensus phylogeny of ammonia-oxidizing archaea and deep sequencing of amoA genes from soils of four different geographic regions. Environ. Microbiol. 14, 525–539. doi: 10.1111/j.1462-2920.2011.02666.x
Regar, R. K., Gaur, V. K., Mishra, G., Jadhao, S., Kamthan, M., and Manickam, N. (2016). Draft genome sequence of Alcaligenes faecalis strain IITR89, an indole-oxidizing bacterium. Genome Announc. 4:e00067-16. doi: 10.1128/genomeA.00067-16
Rehfuss, M., and Urban, J. (2005). Alcaligenes faecalis subsp. phenolicus subsp. nov. a phenol-degrading, denitrifying bacterium isolated from a graywater bioprocessor. Syst. Appl. Microbiol. 28, 421–429. doi: 10.1016/j.syapm.2005.03.003
Richardson, D. J., Wehrfritz, J. M., Keech, A., Crossman, L. C., and Spiro, S. (1998). The diversity of redox proteins involved in bacterial heterotrophic nitrification and aerobic denitrification. Biochem. Soc. Trans. 26, 401–408. doi: 10.1042/bst0260401
Robert, X., and Gouet, P. (2014). Deciphering key features in protein structures with the new ENDscript server. Nucleic Acids Res. 42, W320–W324. doi: 10.1093/nar/gku316
Saitou, N., and Nei, M. (1987). The Neighbor-joining method: a new method for reconstructing phylogenetic trees. Mol. Biol. Evol. 4, 406–425.
Schroll, G., Busse, H.-J., Busse, H.-J., Parrer, G., Rölleke, S., Lubitz, W., et al. (2001). Alcaligenes faecalis subsp. parafaecalis subsp. nov., a bacterium accumulating poly-β-hydroxybutyrate from acetone-butanol bioprocess residues. Syst. Appl. Microbiol. 24, 37–43. doi: 10.1078/0723-2020-00001
Shoda, M., and Ishikawa, Y. (2016). Removal of high-strength of ammonium and phenol from coking wastewater by Alcaligenes faecalis No. 4. J. Appl. Biotechnol. Bioeng. 1:00014.
Sievers, F., and Higgins, D. G. (2013). Clustal Omega, accurate alignment of very large numbers of sequences. Methods Mol. Biol. 1079, 105–116. doi: 10.1007/978-1-62703-646-7_6
Sigman, D. M., and Fripiat, F. (2019). “Nitrogen isotopes in the ocean,” in Encyclopedia of Ocean Sciences, 3rd Edn, eds J. K. Cochran, H. J. Bokuniewicz, and P. L. Yager (Oxford: Academic Press).
Simmons, D. G., Davis, D. E., Rose, L. P., Gray, J. G., and Luginbuhl, G. H. (1981). Alcaligenes faecalis-associated respiratory disease of chickens. Avian Dis. 25, 610–613. doi: 10.2307/1589991
Skiba, U. (2008). “Denitrification,” in Encyclopedia of Ecology, eds S. E. Jørgensen and B. D. Fath (Oxford: Academic Press).
Stein, L. Y. (2011). Heterotrophic nitrification and nitrifier denitrification. Nitrification 25, 95–114.
Strous, M., Fuerst, J. A., Kramer, E. H., Logemann, S., Muyzer, G., van de Pas-Schoonen, K. T., et al. (1999). Missing lithotroph identified as new Planctomycete. Nature 400, 446–449. doi: 10.1038/22749
Thompson, J. D., Higgins, D. G., and Gibson, T. J. (1994). CLUSTAL W: improving the sensitivity of progressive multiple sequence alignment through sequence weighting, position-specific gap penalties and weight matrix choice. Nucleic Acids Res. 22, 4673–4680. doi: 10.1093/nar/22.22.4673
Van Cleemput, O., and Samater, A. H. (1995). Nitrite in soils: accumulation and role in the formation of gaseous N compounds. Fertilizer Res. 45, 81–89. doi: 10.1007/bf00749884
van de Graaf, A. A., de Bruijn, P., Robertson, L. A., Jetten, M. S. M., and Kuenen, J. G. (1996). Autotrophic growth of anaerobic ammonium-oxidizing micro-organisms in a fluidized bed reactor. Microbiology 142, 2187–2196. doi: 10.1099/13500872-142-8-2187
van Niel, E. W. J., Braber, K. J., Robertson, L. A., and Kuenen, J. G. (1992). Heterotrophic nitrification and aerobic denitrification in Alcaligenes faecalis strain TUD. Antonie Van Leeuwenhoek 62, 231–237. doi: 10.1007/BF00582584
Van Trappen, S., Tan, T.-L., Samyn, E., and Vandamme, P. (2005). Alcaligenes aquatilis sp. nov., a novel bacterium from sediments of the Weser Estuary, Germany, and a salt marsh on Shem Creek in Charleston Harbor, USA. Int. J. Syst. Evol. 55, 2571–2575. doi: 10.1099/ijs.0.63849-0
Wehrfritz, J.-M., Reilly, A., Spiro, S., and Richardson, D. J. (1993). Purification of hydroxylamine oxidase from Thiosphaera pantotropha: identification of electron acceptors that couple heterotrophic nitrification to aerobic denitrification. FEBS Lett. 335, 246–250. doi: 10.1016/0014-5793(93)80739-h
Wilson, K. H., Blitchington, R. B., and Greene, R. C. (1990). Amplification of bacterial 16S ribosomal DNA with polymerase chain reaction. J. Clin. Microbiol. 28, 1942–1946. doi: 10.1128/jcm.28.9.1942-1946.1990
Wu, M.-R., Hou, T.-T., Liu, Y., Miao, L.-L., Ai, G.-M., Ma, L., et al. (2021). Novel Alcaligenes ammonioxydans sp. nov. from wastewater treatment sludge oxidizes ammonia to N2 with a previously unknown pathway. Environ. Microbiol. 23, 6965–6980. doi: 10.1111/1462-2920.15751
Wu, M.-R., Miao, L.-L., Liu, Y., Hou, T.-T., Ai, G.-M., Ma, L., et al. (2020). A novel oxidase from Alcaligenes sp. HO-1 oxidizes hydroxylamine to N2. bioRxiv [Preprint] doi: 10.1101/2020.08.20.256677
Xu, S.-Q., Qian, X.-X., Jiang, Y.-H., Qin, Y.-L., Zhang, F.-Y., Zhang, K.-Y., et al. (2022). Genetic foundations of direct ammonia oxidation (Dirammox) to N2 and MocR-like transcriptional regulator DnfR in Alcaligenes faecalis JQ135. Appl. Environ. Microbiol. 88:e0226121. doi: 10.1128/aem.02261-21
Zhang, Y., Chen, Q., Ji, J., Zhao, L., Zhang, L., Qiu, J., et al. (2018). Complete genome sequence of Alcaligenes faecalis strain JQ135, a bacterium capable of efficiently degrading nicotinic acid. Curr. Microbiol. 75, 1551–1554. doi: 10.1007/s00284-018-1486-0
Keywords: nitrogen cycle, Dirammox, Alcaligenes, DnfA, environmental distribution
Citation: Hou T-T, Miao L-L, Peng J-S, Ma L, Huang Q, Liu Y, Wu M-R, Ai G-M, Liu S-J and Liu Z-P (2022) Dirammox Is Widely Distributed and Dependently Evolved in Alcaligenes and Is Important to Nitrogen Cycle. Front. Microbiol. 13:864053. doi: 10.3389/fmicb.2022.864053
Received: 28 January 2022; Accepted: 19 April 2022;
Published: 13 May 2022.
Edited by:
Catarina Magalhães, University of Porto, PortugalReviewed by:
Ping Penny Han, East China Normal University, ChinaCopyright © 2022 Hou, Miao, Peng, Ma, Huang, Liu, Wu, Ai, Liu and Liu. This is an open-access article distributed under the terms of the Creative Commons Attribution License (CC BY). The use, distribution or reproduction in other forums is permitted, provided the original author(s) and the copyright owner(s) are credited and that the original publication in this journal is cited, in accordance with accepted academic practice. No use, distribution or reproduction is permitted which does not comply with these terms.
*Correspondence: Zhi-Pei Liu, bGl1emhwQGltLmFjLmNu
†These authors have contributed equally to this work
Disclaimer: All claims expressed in this article are solely those of the authors and do not necessarily represent those of their affiliated organizations, or those of the publisher, the editors and the reviewers. Any product that may be evaluated in this article or claim that may be made by its manufacturer is not guaranteed or endorsed by the publisher.
Research integrity at Frontiers
Learn more about the work of our research integrity team to safeguard the quality of each article we publish.