- 1Beijing Key Lab for Source Control Technology of Water Pollution, College of Environmental Science and Engineering, Beijing Forestry University, Beijing, China
- 2Engineering Research Center for Water Pollution Source Control and Eco-Remediation, College of Environmental Science and Engineering, Beijing Forestry University, Beijing, China
- 3School of Energy and Environmental Engineering, Hebei University of Technology, Tianjin, China
Anaerobic digestion is an effective and sustainable technology for resource utilization of organic wastes. Recently, adding conductive materials in anaerobic digestion to promote direct interspecies electron transfer (DIET) has become a hot topic, which enhances the syntrophic conversion of various organics to methane. This review comprehensively summarizes the recent findings of DIET mechanisms with different mediating ways. Meanwhile, the influence of DIET on anaerobic digestion performance and the underlying mechanisms of how DIET mediated by conductive materials influences the lag phase, methane production, and system stability are systematically explored. Furthermore, current challenges such as the unclear biological mechanisms, influences of non-DIET mechanisms, limitations of organic matters syntrophically oxidized by way of DIET, and problems in practical application of DIET mediated by conductive materials are discussed in detail. Finally, the future research directions for practical application of DIET are outlined.
Introduction
Methane production from anaerobic digestion can reduce the dependence on fossil energy, which is critical to realize the current global sustainable development goals of climate change, waste recycling, and clean energy production (Wang P. et al., 2018; Li et al., 2019; Akor et al., 2021; Wang et al., 2021). Generally, four groups of microorganisms (fermentative bacteria, acidogens, acetogenes, and methanogens) with specific metabolic functions participate in anaerobic digestion process (Alavi-Borazjani et al., 2020; Abbas et al., 2021). These microorganisms have distinct characteristics on physiology, nutrient metabolism, growth kinetics, and environmental sensitivity (Luo et al., 2016). Thus, the interactions between microorganisms are complex during the entire anaerobic digestion process. Compared with methanogens, bacteria have a shorter generation time, faster growth rate, and more extensive adaptability to pH variations. The imbalance between methanogens and bacteria usually impairs anaerobic digestion performance (Feng et al., 2019). Hence, the efficient syntrophic associations between methanogens and bacteria play an important role in anaerobic digestion process.
For many years, interspecies hydrogen transfer (IHT) and interspecies formate transfer (IFT) are believed to be two common modes for interspecies electron transfer in syntrophic oxidation of organic matters (Dong and Stams, 1995; Zhao et al., 2016b). However, H2 production in syntrophic oxidation with energy consuming is an unfavorable thermodynamic reaction (Li Y. et al., 2018; Zhao J. et al., 2018). Recently, direct interspecies electron transfer (DIET) has been proved to be a new syntrophic association mode, in which electrons transfer directly from electron donors to electron acceptors with fewer biological enzymatic reactions (Summers et al., 2010; Gahlot et al., 2020). DIET can replace IHT in anaerobic digestion systems because it does not require H2 as an electron carrier, overcoming the thermodynamic limitation under high hydrogen partial pressure (Baek et al., 2018; Zhao and Zhang, 2019). A mathematical model shows that the electron transfer rate based on DIET was 8.57 times higher than that of IHT (Storck et al., 2015). Therefore, the electron transfer capacity of DIET is more efficient than that of IHT (Zhao et al., 2016a; Wang et al., 2020c; Zhang et al., 2020).
Currently, Geobacter species has been proven to be able to establish DIET-based syntrophic associations with methanogens via conductive pili (e-pili) and c-type cytochrome (OmcS), in which methanogens accept electrons and reduce CO2 to methane (Rotaru et al., 2014a,b). Studies have shown that various bacteria that cannot produce e-pili or OmcS can also build DIET-based syntrophic partnerships through adding conductive materials, such as granular activated carbon (GAC) (Zhang et al., 2020), biochar (Qi et al., 2021), carbon cloth (Jia et al., 2020), carbon nanotube (Shen et al., 2020), graphene (Igarashi et al., 2019), and magnetite (Cheng et al., 2020). It is generally agreed that non-biological conductive materials act as an electrical conduit to replace the function of e-pili or OmcS (Chen et al., 2014a; Xu et al., 2020).
The discovery of DIET provides a new way to improve anaerobic digestion performance. DIET mediated by conductive materials has been successfully applied to anaerobic digestion systems with different substrates over the years. As of late, several corresponding reviews have been published, elaborating the importance and advantages of DIET mediated by conductive materials (Barua and Dhar, 2017; Baek et al., 2018; Martins et al., 2018; Park et al., 2018a; Xu et al., 2019; Gahlot et al., 2020; Nguyen et al., 2021). However, there are few reviews on comprehensively summarizing the mechanisms of DIET and the effects of DIET mediated by conductive materials on anaerobic digestion performances. Furthermore, some significant questions need to be answered. For example, the biological path of electron transfer remains unclear (Yin and Wu, 2019). In addition to DIET, other stimulatory mechanisms of conductive materials also influence anaerobic digestion performances, such as microbial immobilization, buffering effect, and adsorption effect, which are related to the surface physicochemical characteristics of conductive materials, such as large surface area, rich redox groups and surface pH (Martins et al., 2018; Park et al., 2018a). These elements of knowledge should be considered because it is difficult to determine the contribution of non-DIET function to methane production. Meanwhile, limited substrates and microorganisms have been proved to be involved in DIET, and some substrates and microorganisms are only speculated to be involved in DIET according to anaerobic digestion performance improvement and microbial characteristics. Furthermore, the negative impacts of conductive materials on downstream processing, ecological environment, and anaerobic digestion performance restrict the practical application of DIET (Nguyen et al., 2021). Therefore, a review of literatures on mechanisms, performances, and challenges of DIET improvement via adding conductive materials in anaerobic digestion are imperative for a better vision in the practical application.
This review comprehensively summarizes the deep-rooted mechanisms of DIET and systematically explores the advantages and effects of DIET mediated by conductive materials in anaerobic digestion systems. Furthermore, this review focuses on existing challenges and critically discusses the unclear biological mechanisms of DIET, influences of non-DIET mechanisms, limitations of organic matters syntrophically oxidized by way of DIET, and problems in practical application of DIET mediated by conductive materials. Finally, the future research directions of DIET proposed herein are expected to provide a theoretical basis and reference for practical application.
Establishment of Direct Interspecies Electron Transfer in Anaerobic Digestion
Recently, the establishment of DIET by adding conductive materials to promote anaerobic digestion performance has become a hot topic. The realization of DIET methanogenic pathway no longer entirely rely on biological tools such as e-pili or OmcS because of the presence of conductive materials (Chen et al., 2014b; Xu et al., 2019). Further, the addition of conductive materials enriches microorganisms with an extracellular electron transfer ability (Nguyen et al., 2021). It can be speculated that these microorganisms may participate in different stages of anaerobic digestion (Figure 1), making it possible to develop the DIET pathway in methanogenic systems with different substrates (Yin and Wu, 2019; Li et al., 2021). Therefore, many studies have focused on the establishment of DIET in anaerobic digestion reactors by adding different types of conductive materials.
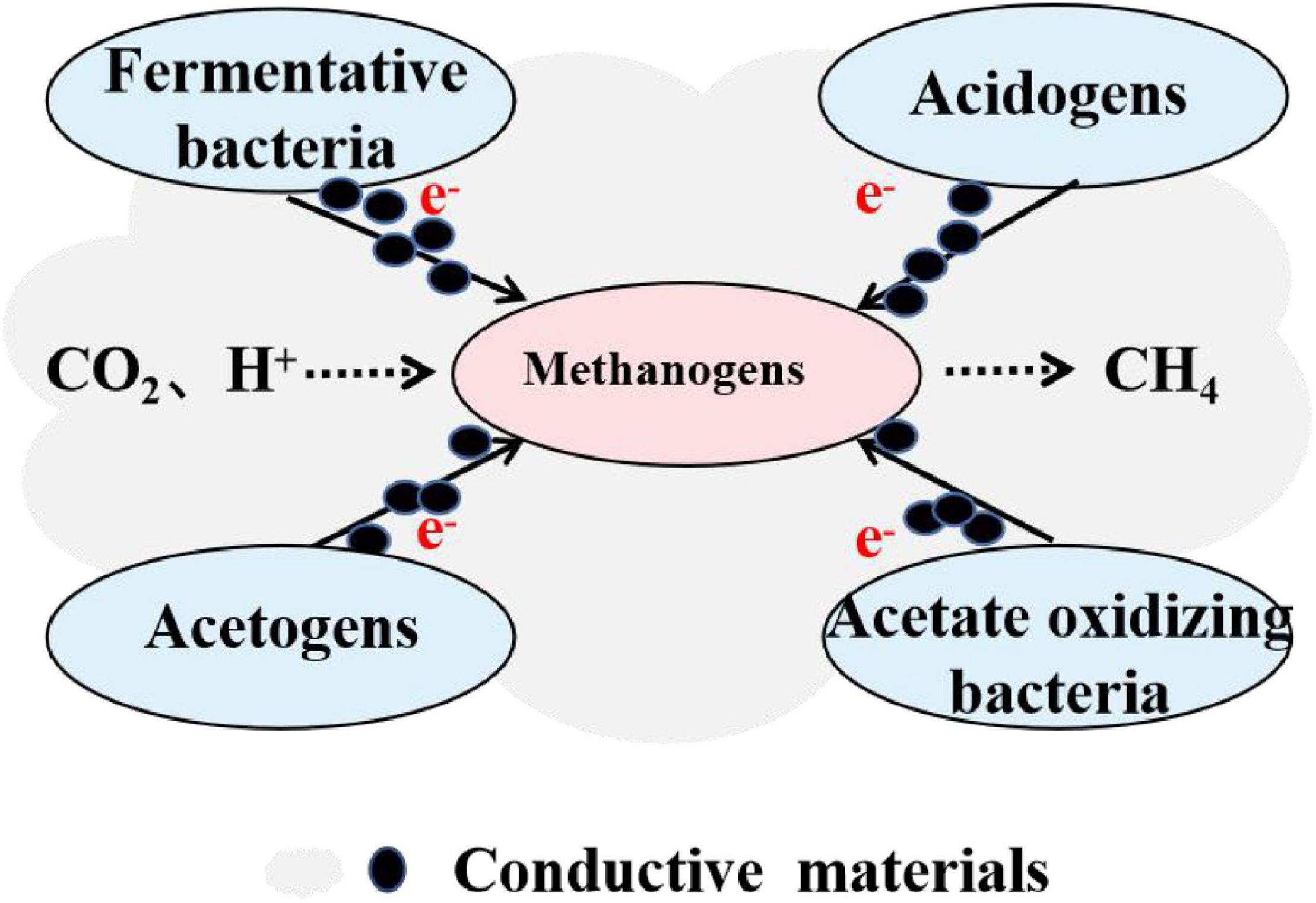
Figure 1. Possible mechanisms on establishing direct interspecies electron transfer (DIET) via addition of conductive materials.
Another promising strategy is to initially provide ethanol stimulation to anaerobic digestion (Zhao et al., 2015, 2017a; Li et al., 2020b). This method achieves the purpose of enriching Geobacter and overcomes the lack of Geobacter in most traditional methanogenic communities (Zhao et al., 2016b). Its possible mechanism may be that the energy produced by ethanol metabolism can promote DIET to overcome the thermodynamic limitation of syntrophic oxidation (Zhao and Zhang, 2019). Based on these advantages, the ethanol-type fermentation product, which is used as a substrate, will continuously provoke the methanogenic communities to perform DIET, avoiding the economic problems related to the extra supply of ethanol (Zhao et al., 2017a). However, this coupling process is complex and increases the operation cost. Besides, not all organic wastes are suitable substrates for ethanol fermentation.
Studies have found that even if the substrates contain ethanol, the addition of conductive materials can further stimulate the DIET and promote anaerobic digestion performance (Yang et al., 2015a; Zhao et al., 2016b; Li et al., 2020a). This may be because that the DIET mediated by conductive materials can realize long-distance electron transfer (Martins et al., 2018), thereby making the electron transfer more efficient compared to the ethanol stimulation. Furthermore, conductive materials have the advantages of being readily available and relatively inexpensive. Therefore, establishing DIET by adding conductive materials remains an attractive option.
Direct Interspecies Electron Transfer Mechanisms
Biological Structure Mediating Direct Interspecies Electron Transfer
Generally, researchers conduct in-depth research on the mechanisms of DIET in defined co-culture systems using ethanol as a substrate (Table 1). Compared with indirect electron transfer, which relies on H2 and formate as electron carriers (Figure 2A), biological structure mediating DIET achieves electrical connection via e-pili and OmcS of electron-donating microorganisms (Summers et al., 2010). E-pili, also known as nanowires, are protein filaments produced by microorganisms during electron transfer under appropriate conditions (Malvankar and Lovley, 2012). Malvankar et al. (2011) found that the conductivity of pili protein extracted from Geobacter sulfurreducens was approximately 5 μS/cm, and exhibited temperature dependence similar to that of disordered metals. OmcS is a type of Fe(III) redox protein that is related to the process of electron transfer from cell surface to extracellular electron acceptor. Leang et al. (2010) observed that the OmcS was mainly distributed along nanowires or exists on the outer surface of cells using scanning electron microscopy. Shrestha et al. (2013) found that the key genes encoding e-pili and OmcS were highly expressed in the co-culture system of G eobacter metallireducens and G. sulfurreducens. Rotaru et al. (2014b) confirmed that co-culture of microorganisms cannot reproduce without the key genes encoding e-pili and OmcS. Moreover, e-pili- or OmcS-deficient strains cannot metabolize ethanol to produce methane in the defined co-cultures (Rotaru et al., 2014a,b). These results indicate that e-pili and OmcS play an important role in establishing DIET. The possible related mechanisms are summarized in Figure 2B.
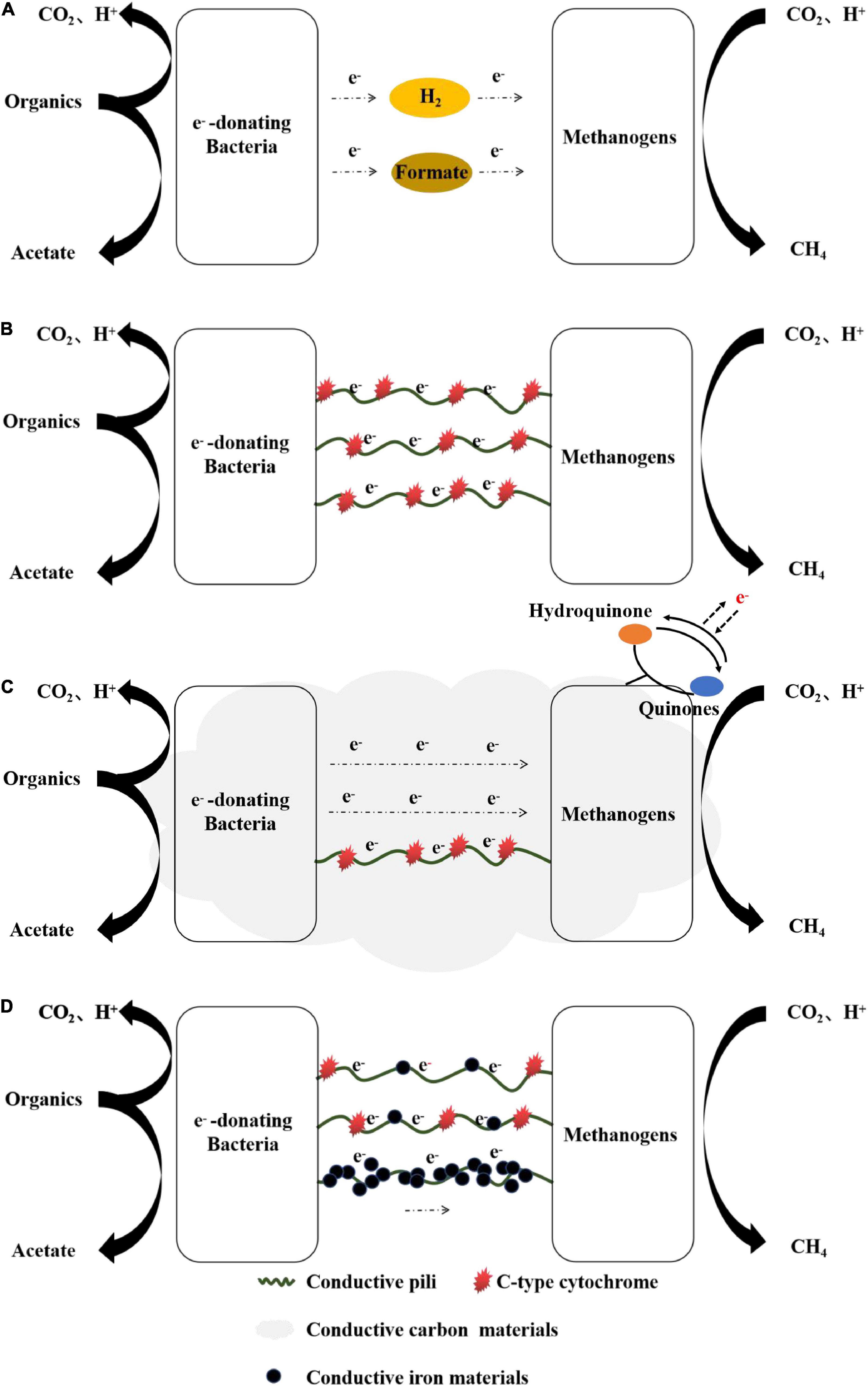
Figure 2. Electron transfer mechanisms (A) IHT and IFT, (B) biological structure mediating DIET, (C) conductive carbon material mediating DIET, and (D) conductive iron material mediating DIET.
The mechanism of methanogens to accept electrons may be similar to that of electron donation microorganisms (Li et al., 2021). However, methanogens, including Methanosarcina barkeri, and Methanosarcina harundinaceae have been confirmed to participate in DIET without OmcS on their outer surface (Holmes et al., 2018; Yee and Rotaru, 2020). In addition, Walker et al. (2019) reported Methanospirillum hungatei could secrete e-pili. However, M. hungatei could not conduct the DIET with G. metallireducens in the co-culture system (Rotaru et al., 2014b). The study on extracellular electron transfer mechanism of methanogens has just begun, which is far less than the understanding with Geobacter species. Therefore, it is necessary to deeply study the molecular targets related to biological pathway of methanogens to accept electrons.
Conductive Carbon Material Mediating Direct Interspecies Electron Transfer
Conductive carbon materials have been proven to significantly improve anaerobic digestion performance (Lee et al., 2016; Lin et al., 2017; Li J. et al., 2018). Liu et al. (2012) thought that the reason why active carbon promoted DIET was that the conductivity of active carbon was higher than that of methanogenic aggregates. However, the promotion effect of biochar is almost the same as that of active carbon even though the conductivity of biochar is one-thousandth that of activated carbon (Chen et al., 2014b). Therefore, it can be inferred that any conductivity greater than a certain threshold is sufficient to trigger the DIET methanogenic pathway (Barua and Dhar, 2017).
Direct interspecies electron transfer promoted by conductive carbon materials is not completely dependent on e-pili or OmcS as the media for electron transfer (Zhao and Zhang, 2019). Rather, the electrical connection was realized through carbon materials, not intercellular contact, because there were no aggregates of G. metallireducens and M. harundinaceae on the surface of carbon materials (Chen et al., 2014b). Liu et al. (2012) confirmed that e-pili- and OmcS-deficient strains could metabolize ethanol and reduce fumarate to succinate with addition of conductive carbon materials. Park et al. (2018b) found that conductive carbon materials made the abundance of key genes encoding e-pili and OmcS in wild-type strains decrease compared with the control, indicating that conductive carbon materials replaced the functions of e-pili and OmcS. Therefore, establishing DIET by adding conductive carbon materials might consume less energy to satisfy the growth of syntrophic partners and metabolize more substrates as the microorganisms did not need to highly express the key genes encoding e-pili and OmcS (Zhao et al., 2015).
Furthermore, conductive carbon materials have large specific surface areas and porosities (Figueiredo et al., 1999; Chen et al., 2014b; Zhao et al., 2016a), which play a vital role in the adsorption, fixation, and enrichment of microorganisms involved in DIET (Qi et al., 2021). Meanwhile, the redox groups on the surface of conductive carbon materials, such as quinone or quinone hydrogen, may be closely related to DIET (Wang et al., 2020b). The possible related mechanisms are shown in Figure 2C.
Conductive Iron Material Mediating Direct Interspecies Electron Transfer
Conductive iron materials also promote DIET formation (Kato et al., 2012; Zhu et al., 2017). Currently, researches are mainly focused on the promotion of DIET by magnetite. Ueki et al. (2018) found that magnetite could not replace conductive carbon materials as adding magnetite did not promote DIET in a co-culture system of e-pili-deficient strains. However, Liu et al. (2015) found that magnetite enabled OmcS-deficient strains to have the ability to realize extracellular electron transfer. They found that the expression of wild-type OmcS gene was inhibited because of the presence of magnetite. Moreover, transmission electron microscopy showed that magnetite, similar to OmcS, was dispersed between cells and distributed along the e-pili (Liu et al., 2015). These results indicated that magnetite replaced the role of OmcS rather than that of e-pili (Lovley, 2017). The stimulating effect of magnetite is different from that of conductive carbon materials probably because of their size and structural differences. Magnetite particles are generally 20–50 nm in size, which is smaller than microbial cells, while conductive carbon materials have a significantly bigger size and provide a large surface area for microbial attachment (Lovley, 2017; Yin and Wu, 2019; Zhang et al., 2020).
In addition, many studies have shown that conductive iron materials can enrich microorganisms with extracellular electron transfer abilities, which may be involved in DIET (Yamada et al., 2015; Yang et al., 2015b). The possible related mechanisms are shown in Figure 2D.
Improvements of Anaerobic Digestion Performances
The establishment of DIET-based syntrophic relationships via adding conductive materials improve anaerobic digestion performance in several aspects under different working conditions (Table 2).
Shortening Lag Period and Start-Up Time
Lag period is the time required for inoculum to adapt to the new environment. A long lag period means that a longer start-up time is needed for anaerobic digestion. In a practical start-up process, microorganisms must firstly recover from dormancy. The growth rate of methanogens in the original anaerobic sludge is much slower than that of bacteria. Usually, anaerobic digestion reactors start with short hydraulic retention time, low organic load rate (OLR), and low feeding concentration. These factors lead to a longer start-up cycle, which affects anaerobic digestion efficiency and causes an increase in operating cost. Studies have shown that DIET mediated by conductive materials can significantly shorten lag time of anaerobic digestion reactor with different types of substrates, such as glucose (Luo et al., 2015; Yan et al., 2017), phenol (Wang et al., 2020a), benzoate (Zhuang et al., 2015), and mixed organic carbon (Yin et al., 2017b; Wang G. et al., 2018). Moreover, establishing DIET by adding conductive materials significantly shortened the lag period of anaerobic digestion system even under conditions of high hydrogen partial pressure, high concentration of ammonia nitrogen, and sulfur inhibition. Wang et al. (2020b) found that DIET stimulation shortened the lag time of acetate, propionate, butyrate, and valerate by 29.2, 20.5, 9.1, and 10.5%, respectively. Therefore, the promotion on syntrophic metabolism of volatile fatty acids (VFAs) may be the main reason why DIET shortens the lag period.
Improving Methane Yield
As DIET is more efficient than IHT, the establishment of DIET mediated by conductive materials has been widely recognized to promote methane yield. Park et al. (2018b) found that methane yield with GAC addition increased by 31% compared to control without GAC addition. Similarly, Zhang et al. (2020) observed that the methane yield in GAC-amended systems increased by 3.9 times. Li et al. (2020a) reported that the methane yield with biochar increased by about 44% and the effluent concentration of total organic substrate decreased compared with control. Besides, improvement in the methane yield has been also repeatedly reported and verified with other conductive materials, such as graphite (Tan et al., 2015), magnetite (Ye et al., 2018), and carbon cloth (Zhao et al., 2017c). Studies showed that the increase in methane yield is mainly because that DITE mediated by conductive materials facilitated the conversion of interspecies metabolites to methane (Tian et al., 2017; Jiang et al., 2020; Sun et al., 2020).
Enhancing System Stability
Direct interspecies electron transfer mediated by conductive materials plays an important role in maintaining the stability of anaerobic digestion system. To achieve efficient energy production, anaerobic digestion systems are generally maintained at high OLRs (Appels et al., 2011). However, high OLRs often cause the acidogenesis rate to be faster than methanogenesis rate, resulting in a sharp drop in pH and VFA accumulation (Babel et al., 2004). Nevertheless, DIET can still improve anaerobic digestion performances under high OLR conditions (Dang et al., 2016, 2017). Moreover, a high hydrogen partial pressure is another factor that leads to VFA accumulation. However, DIET-based syntrophic relationships can enable anaerobic digestion systems to overcome the limitation of hydrogen partial pressure (Nagarajan et al., 2013; Zhao et al., 2016b; Zhao Z. et al., 2018). Organic matters containing nitrogen (protein, amino acids, and urea) can release high concentration of ammonia in an anaerobic digestion system. The ammonia can exist in the form of ammonium ion or free ammonia, which inhibit microbial activity and cell growth (Kayhanian, 1999). However, Lu et al. (2016) found that the methane yield increased with the addition of biochar under an ammonia inhibition environment. This may be because that the establishment of DIET resisted the toxic inhibition of ammonia and maintained the stability of syntrophic metabolism. In addition, supplemental magnetite significantly increased the conversion rate of acetate to methane under high ammonium concentrations (Zhuang et al., 2018). Sulfate in the anaerobic digestion system can stimulate the growth of sulfate-reducing bacteria which compete with methanogens for acetate, H2, and intermediates, such as propionate, butyrate, and ethanol (Li Q. et al., 2015; Qian et al., 2019). Hydrogen sulfide, the product of sulfate reduction, can significantly inhibit the activities of methanogens, which decreases the anaerobic digestion performances (Chen et al., 2008). However, some studies have demonstrated that DIET mediated by conductive materials was expected to promote methane production in anaerobic digestion system treating organic wastewater with a high concentrations of sulfate (Li et al., 2017; Jin et al., 2019). The potential mechanism may be that methanogens through DIET metabolic pathway are more competitive to substrates than sulfate-reducing bacteria (Liu et al., 2019).
Challenges of Direct Interspecies Electron Transfer in Anaerobic Digestion
Although the establishment of DIET via addition of conductive materials shows a significant promotion effect in anaerobic digestion system, the current research is only in its primary stage. Therefore, this section discusses the existing challenges.
Unclear Biological Mechanism of Direct Interspecies Electron Transfer
Study on the biological pathway of DIET is helpful for understanding microbial syntrophic metabolism. Figure 3 shows the model of DIET methanogenic pathways between G. metallireducens and M. barkeri. However, the electron transfer biological pathway in DIET between microorganisms remains unclear.
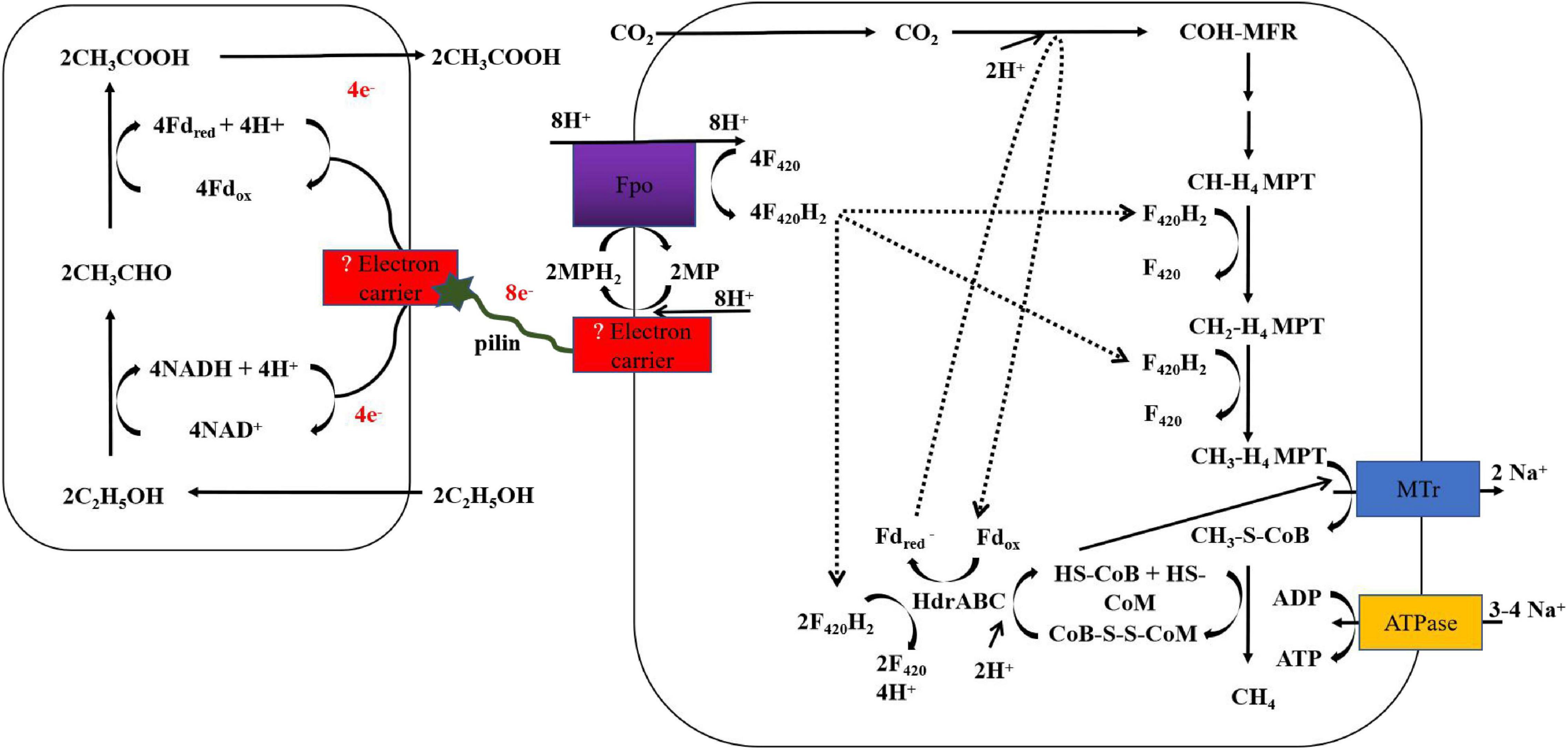
Figure 3. DIET model in co-culture of G. metallireducens and M. barkeri. This figure is adapted from previous paper (Rotaru et al., 2014a; Holmes et al., 2018).
To date, it is unclear how electron donating bacteria transfer electrons outside of the cell. Geobacter is often used as a model microorganism to study the mechanism of extracellular electron transfer because of its completely available genome sequence and skilled gene engineering methods (Coppi et al., 2001). In general, it is believed that the e-pili of Geobacter transfer electrons to OmcS and then to extracellular insoluble electron acceptors such as iron oxides and electrodes. However, the mechanism of electron transfer to extracellular e-pili is unclear. Currently, cytochromes (OmaB, OmaC, OmcB, and OmcC) and porins (OmbB and OmbC) are considered to play essential roles in the transfer of electrons to e-pili (Costa et al., 2018). Besides, it remains unclear how electron-accepting microorganisms directly accept extracellular electrons from their syntrophic partners. Studies on extracellular electron transfer showed that OmcS and pili nanowires were indispensable during the process of extracellular electron transfer. However, unlike Geobacter species, M. barkeri, and M. harundinaceae without outer surface e-pili and OmcS have also been found to be involved in DIET (Holmes et al., 2018). Therefore, it is necessary to further find the differential expression of genes in electroactive methanogens under different methanogenic conditions, and then verify the functions of related genes through gene mutations, and finally reveal the extracellular electron transfer mechanism of electron-donating microorganisms. Finally, it is unclear how methanogens utilize extracellular electrons and reduce CO2 through DIET. Thauer et al. (2008) proposed that the CO2 reduction pathways of methanogens with and without cytochromes might vary. Recent research showed that DIET promoted transcripts of F420H2 dehydrogenase (Fpo) and heterodisulfide reductase (HdrABC) (Holmes et al., 2018). Electrons transferred via the DIET pathway were first employed to reduce methanophenazine (MP), and then the generated MPH2 could be used as the electron donor for Fpo to reduce coenzyme F420. Half of the produced F420H2 in this model was regarded as the electron donor for HdrABC bifurcation reaction to further yield reduced ferredoxin for CO2 reduction (Holmes et al., 2018). Simultaneously, a bifurcation reaction facilitated the reduction of coenzyme B-coenzyme M heterodisulfide (CoB-S-S-CoM), which is necessary for CH4 production. However, how electrons are transferred to MP should be further studied (Holmes et al., 2018).
Recently, Holmes et al. (2021) proposed a new model of DIET methanogenic pathways, which was confirmed in the defined co-culture of G. metallireducens and Methanosarcina acetivorans. Multiheme membrane-bound c-type cytochrome A (MmcA) in M. acetivorans played an important role in electron transfer. GAC did not make the co-culture system with MmcA-deficient strains produce methane rapidly, which was mainly because that MmcA was embedded in the cell membrane of M. acetivorans (Ferry, 2020). Researches show that MmcA may exchange electrons with MP or rhodobacter nitrogen (Rnf) complex (Suharti et al., 2014; Ferry, 2020; Holmes et al., 2021). The function of the Rnf is to accept electrons to produce the reduced ferredoxin, which is required for the first step in the reduction of CO2 (Holmes et al., 2021). The model in M. acetivorans is significantly different from that in M. barkeri, because M. Barkeri lack genes for MmcA and Rnf (Zhou et al., 2021). Therefore, Methanogen growth via DIET may adopt different electron-acceptance strategies to accommodate these physiological differences (Holmes et al., 2021).
Influence of Non-direct Interspecies Electron Transfer Mechanisms
The positive effect of conductive materials on anaerobic digestion performances has been confirmed. Therefore, most studies speculated that performance improvement might be attributed to DIET existing in complex anaerobic digestion systems based on microorganisms already known to take part in DIET. However, the relative abundance of Geobacter in anaerobic sludge is low (Dang et al., 2016; Peng et al., 2018; Li et al., 2020b) or even undetectable (Wang et al., 2020b). In addition, several studies have demonstrated that conductive materials would enrich microorganisms with extracellular electron transfer ability, such as Desulfuromonas (Barua et al., 2018), Pseudomonas (Barua and Dhar, 2017), Azovibrio (Ruiz et al., 2014), and Syntrophomonas (Chen Y. et al., 2020), which may participate in DIET. However, it has not been proven that these microorganisms can conduct DIET in defined co-cultures with methanogens. Therefore, it is insufficient to infer that DIET occurred in anaerobic digestion systems based on the anaerobic digestion performance improvement and microbial community structure.
In addition to DIET, other stimulatory mechanisms of conductive materials in anaerobic digestion cannot be ignored. The related mechanisms are illustrated in Figure 4. Conductive carbon materials not only promote metabolism of inhibitory intermediates by fixing and enriching specific microorganisms but also alleviate inhibition of toxic substances via adsorption effect (Martins et al., 2018; Jung et al., 2020). The pH buffering capacity of conductive carbon materials also creates a favorable environment for methanogenesis (Wang et al., 2017, 2020b). Simultaneously, conductive carbon materials can improve anaerobic digestion performances through other mechanisms, such as function of released ions and surface redox groups, which may affect the redox potential of anaerobic digestion systems. In general, it is believed that a redox potential between −200 and −400 mV is beneficial for methane production (Hirano et al., 2013). Salvador et al. (2017) confirmed that a more negative redox potential occurred with an increase in the carbon nanotube dosage in co-culture systems. Similarly, the effects of non-DIET mechanisms cannot be neglected with addition of conductive iron materials. The iron ions released from conductive iron materials can decrease the negative charge on the surface of extracellular polymeric substances, thereby reducing the repulsive force between cells and increasing the stability of microbial aggregates. Furthermore, the release of iron ions might be regarded as a trace element that promotes microorganism activity.
Therefore, whether the improvement of anaerobic digestion performances is caused by DIET mediated by conductive materials and the DIET is the dominant mechanism in a complex anaerobic digestion system requires in-depth exploration and analysis.
Limitations of Organic Matters Syntrophically Oxidized by Way of Direct Interspecies Electron Transfer
Ethanol has been proven to participate in DIET methanogenesis in a defined co-culture (Rotaru et al., 2014a,b). Although acetate has not been proven to be a substrate that participates in DIET, Zhuang et al. (2018) found that the degradation of acetate in bioreactor containing magnetite was much less affected by hydrogen partial pressure, which may be because that the acetate oxidation metabolism was not completely dependent on IHT. Propionate and butyrate are important intermediate metabolites in anaerobic digestion. Thus, limiting propionate and butyrate accumulation is crucial for improving anaerobic digestion performances. Studies showed that magnetite significantly increased propionate and butyrate degradation, suggesting that propionate and butyrate may be converted to methane via DIET metabolic pathway (Cruz Viggi et al., 2014; Li H. et al., 2015; Jing et al., 2017). Similarly, Barua et al. (2018) confirmed that carbon fibers promoted the conversion of propionate and butyrate to methane. In a syntrophic propionate metabolism, Cruz Viggi et al. (2014) confirmed that conductive materials provided 106 times higher electron carrier flux than that of IHT. In a syntrophic butyrate metabolism, Xu et al. (2018) found that the conversion rate of butyrate into acetate increased by 2.5–7.0 times when conductive material dosage increased from 0.5 to 25 g/L, which may be because that the increase in conductive material dosage provided more carriers for syntrophic partners to exchange electron (Yin and Wu, 2019). Zhuang et al. (2015) found that the addition of magnetite and hematite promoted the anaerobic digestion performances of benzoate. Similar studies demonstrated that adding magnetite or carbon nanotubes to an anaerobic digestion system accelerated the degradation of phenol and reduced its inhibitory effect on biological activity of methanogens (Yan et al., 2018). In addition, glucose and sucrose have been widely reported as substrates of DIET methanogenesis. However, in addition to ethanol as a substrate that participates in DIET, the establishment of DIET metabolic pathway in an anaerobic digestion system with other organics as substrates lacks direct biological evidence.
Compared with simple organics, complex organics must undergo hydrolysis and acidification before methanogenesis. Therefore, the DIET mechanisms of complex organics may be more complicated than that of simple organics. Yang et al. (2017) and Sun et al. (2020) confirmed that addition of conductive materials improved the conversion rate of sludge to methane. Similarly, although the anaerobic digestion of leachate from municipal solid waste is limited by high OLRs and toxic substances, some studies found that establishing DIET could increase the digestibility of leachate and methane production (Lei et al., 2016, 2018). However, Zhao et al. (2017b) added magnetite and GAC to hydrolysis-acidogenesis and methanogenesis reactors, respectively, and found that the supplemental GAC had little effect on syntrophic metabolism of alcohols and VFAs when hydrolysis-acidogenesis was ineffective or did not work. After magnetite promoted acidogenesis efficiency, the syntrophic metabolism of alcohols and VFAs in methanogenesis phase was markedly enhanced by GAC. Anaerobic digestion modified by magnetite can enrich iron-reducing bacteria, which play an important role in the hydrolysis-acidogenesis of complex organics (Peng et al., 2018). The increase in methane production in anaerobic digestion with GAC is probably caused by DIET between syntrophic bacteria and methanogens (Peng et al., 2018). Therefore, establishing DIET may not change the rate-limiting step of anaerobic digestion with complex organic matters because the confirmed DIET mainly occurs in the methanogenic stage. Thus, further researches are necessary to promote the hydrolysis and acidogenesis of complex organics via DIET metabolic pathway.
Problems in Practical Application
There are few successful practical application of DIET in anaerobic digestion via adding conductive materials. This is mainly because that the establishment of DIET does not achieve satisfactory results in amplification experiments and demonstration projects. The complexity of reactor operation control makes it difficult to ensure that conductive materials take effect in the engineered anaerobic digestion system. In addition to considering the positive effect of DIET mediated by conductive materials, it is also important to consider the economic cost and benefit. Although researchers usually add conductive materials to anaerobic digestion, this method is not economically scalable (Zhao et al., 2020). There is loss of conductive materials in the digester discharge (Yin et al., 2017a; Zhang et al., 2018). For example, Zhang et al. (2018) found that the cost required to maintain the level of GAC in the anaerobic digestion accounted for 38.3–81.8% of the revenue from methane production each day. The labor for supplement of conductive materials also increases the economic cost on a daily basis (Zhao et al., 2020). Furthermore, the impacts of conductive material discharge on ecological environment and downstream processing should be valued. Currently, there are few studies on the influence of adding conductive materials to anaerobic digestion system on sludge dewatering (Nguyen et al., 2021). Obviously, addition of conductive materials significantly increases the amount of biosolids (Chen S. et al., 2020; Yuan et al., 2021), which increases the cost of solid waste transportation and treatment in the later stage. Recycling and reuse of conductive materials may be an effective strategy, but the complex technical operation and high investment cannot be ignored. Some studies have suggested that conductive materials designed as packed beds inside the reactor should be considered to continually promote DIET. However, this approach usually incurs considerable cost for the maintenance of equipment and construction of reactor (Tatara et al., 2008; Zhao and Zhang, 2019).
Some researchers have also found that conductive materials may have an inhibitory effect on anaerobic digestion performances. For example, Tian et al. (2017) found that the methane yield at a graphene dosage of 30 mg/L was 14.3% higher than that of the control after long-term operation at room temperature, while the methane yield at a graphene dosage of 120 mg/L exhibited a slight inhibitory effect. Salvador et al. (2017) found that carbon nanotubes of 5 g/L inhibited methane production compared with dosages of 0.5 and 1 g/L. These results indicated that excessive dosage of conductive materials could inhibit the microbial metabolism. The potential inhibitory effect may be attributed to the increased resistance to mass transfer between substrates and microorganisms. In addition, studies showed that the supplementation of carbon black nanoparticles resulted in a significant inhibitory effect on an anaerobic digestion system treating glucose, which might be because that carbon black nanoparticles possessed antibacterial properties (Martins et al., 2018; Fujinawa et al., 2019). Similar to conductive carbon materials, conductive iron materials, such as ferrihydrite, also exhibit an inhibitory effect on methane production (Zhou et al., 2014; Martins et al., 2018). Therefore, the dosage and type of conductive material may have unpredictable effect on the anaerobic digestion system. Further, the surface structure of conductive materials, such as the specific surface area, pore size, and roughness, affects the adhesion and colonization of relevant microorganisms in anaerobic digestion process. These factors limit the practical application of DIET mediated by conductive materials in anaerobic digestion.
Conclusion and Perspectives
The DIET has been proven to be more efficient than IHT in terms of electron transfer efficiency and energy conservation. Therefore, the establishment of DIET by adding conductive materials can significantly enhance anaerobic digestion performances, and have attracted increasing attention in recent years. This review summarizes the DIET mechanisms and promotion effect of DIET mediated by conductive materials on anaerobic digestion system. Based on our understanding, this review presents current challenges including unclear biological mechanism of DIET, influences of non-DIET mechanisms of conductive materials, limitations of organic matters syntrophically oxidized by way of DIET, and problems in practical application of DIET mediated by conductive materials. Therefore, more detailed exploration researches are necessary to fill up the gaps of relevant knowledge.
Limited by biological means and technical level, it is difficult to track electron transfer paths throughout the DIET process and determine all proteins and structures involved. Therefore, further researches are necessary to analyze the biological mechanisms of DIET in order to provide a theoretical reference for the application of DIET mediated by conductive materials in anaerobic digestion. As the knowledge regarding DIET enhancement by conductive materials is limited, the current researches only speculated that DIET occurred in the anaerobic digestion based on the following phenomena: (1) the microbial aggregates exhibited metal-like electrical conductivity; (2) Geobacter, Methanosaeta, and Methanosarcina were dominant in the microbial community structure; and (3) the anaerobic digestion system could overcome the limitation of hydrogen partial pressure. However, these studies did not provide direct evidence for these conclusions. Therefore, the interaction mechanisms between conductive materials and microorganisms require further exploration. The metabolic network of complex organic matters in anaerobic digestion is intricate. Therefore, future researches are also necessary to discover more microorganisms capable of DIET, which provides the possibility of complex organic matters that participate DIET metabolic pathway. Moreover, interdisciplinary development may be beneficial for the application of DIET in anaerobic digestion as the establishment of DIET mediated by conductive materials involves the integration of microbiology, biochemistry, material science, and other subjects.
Author Contributions
LC conceptualized and drafted the manuscript. PZ revised the manuscript and supervised the study. WF and GZ improved the manuscript. JC and JL revised the manuscript. All authors approved the submitted version.
Funding
This research work was funded by the National Natural Science Foundation of China (51578068).
Conflict of Interest
The authors declare that the research was conducted in the absence of any commercial or financial relationships that could be construed as a potential conflict of interest.
Publisher’s Note
All claims expressed in this article are solely those of the authors and do not necessarily represent those of their affiliated organizations, or those of the publisher, the editors and the reviewers. Any product that may be evaluated in this article, or claim that may be made by its manufacturer, is not guaranteed or endorsed by the publisher.
References
Abbas, Y., Yun, S., Wang, Z., Zhang, Y., Zhang, X., and Wang, K. (2021). Recent advances in bio-based carbon materials for anaerobic digestion: a review. Renew. Sustain. Energy Rev. 135:110378. doi: 10.1016/j.rser.2020.110378
Akor, C. I., Osman, A. I., Farrell, C., McCallum, C. S., John Doran, W., Morgan, K., et al. (2021). Thermokinetic study of residual solid digestate from anaerobic digestion. Chem. Eng. J. 406:127039. doi: 10.1016/j.cej.2020.127039
Alavi-Borazjani, S. A., Capela, I., and Tarelho, L. A. C. (2020). Over-acidification control strategies for enhanced biogas production from anaerobic digestion: a review. Biomass Bioenergy 143:105833. doi: 10.1016/j.biombioe.2020.105833
Appels, L., Lauwers, J., Degrève, J., Helsen, L., Lievens, B., Willems, K., et al. (2011). Anaerobic digestion in global bio-energy production: potential and research challenges. Renew. Sustain. Energy Rev. 15, 4295–4301. doi: 10.1016/j.rser.2011.07.121
Babel, S., Fukushi, K., and Sitanrassamee, B. (2004). Effect of acid speciation on solid waste liquefaction in an anaerobic acid digester. Water Res. 38, 2417–2423. doi: 10.1016/j.watres.2004.02.005
Baek, G., Kim, J., Kim, J., and Lee, C. (2018). Role and potential of direct interspecies electron transfer in anaerobic digestion. Energies 11:107. doi: 10.3390/en11010107
Barua, S., and Dhar, B. R. (2017). Advances towards understanding and engineering direct interspecies electron transfer in anaerobic digestion. Bioresour. Technol. 244, 698–707. doi: 10.1016/j.biortech.2017.08.023
Barua, S., Zakaria, B. S., and Dhar, B. R. (2018). Enhanced methanogenic co-degradation of propionate and butyrate by anaerobic microbiome enriched on conductive carbon fibers. Bioresour. Technol. 266, 259–266. doi: 10.1016/j.biortech.2018.06.053
Chen, S., Rotaru, A. E., Liu, F., Philips, J., Woodard, T. L., Nevin, K. P., et al. (2014a). Carbon cloth stimulates direct interspecies electron transfer in syntrophic co-cultures. Bioresour. Technol. 173, 82–86. doi: 10.1016/j.biortech.2014.09.009
Chen, S., Rotaru, A. E., Shrestha, P. M., Malvankar, N. S., Liu, F., Fan, W., et al. (2014b). Promoting interspecies electron transfer with biochar. Sci. Rep. 4:5019. doi: 10.1038/srep05019
Chen, S., Tao, Z., Yao, F., Wu, B., He, L., Hou, K., et al. (2020). Enhanced anaerobic co-digestion of waste activated sludge and food waste by sulfidated microscale zerovalent iron: insights in direct interspecies electron transfer mechanism. Bioresour. Technol. 316:123901. doi: 10.1016/j.biortech.2020.123901
Chen, Y., Yang, Z., Zhang, Y., Xiang, Y., Xu, R., Jia, M., et al. (2020). Effects of different conductive nanomaterials on anaerobic digestion process and microbial community of sludge. Bioresour. Technol. 304:123016. doi: 10.1016/j.biortech.2020.123016
Chen, Y., Cheng, J., and Creamer, K. S. (2008). Inhibition of anaerobic digestion process: a review. Bioresour. Technol. 99, 4044–4064. doi: 10.1016/j.biortech.2007.01.057
Cheng, J., Li, H., Ding, L., Zhou, J., Song, W., Li, Y. Y., et al. (2020). Improving hydrogen and methane co-generation in cascading dark fermentation and anaerobic digestion: the effect of magnetite nanoparticles on microbial electron transfer and syntrophism. Chem. Eng. J. 397:125394. doi: 10.1016/j.cej.2020.125394
Coppi, M. V., Leang, C., Sandler, S. J., and Lovley, D. R. (2001). Development of a genetic system for Geobacter sulfurreducens. Appl. Environ. Microbiol. 67, 3180–3187. doi: 10.1128/AEM.67.7.3180-3187.2001
Costa, N. L., Clarke, T. A., Philipp, L. A., Gescher, J., Louro, R. O., and Paquete, C. M. (2018). Electron transfer process in microbial electrochemical technologies: the role of cell-surface exposed conductive proteins. Bioresour. Technol. 255, 308–317. doi: 10.1016/j.biortech.2018.01.133
Cruz Viggi, C., Rossetti, S., Fazi, S., Paiano, P., Majone, M., and Aulenta, F. (2014). Magnetite particles triggering a faster and more robust syntrophic pathway of methanogenic propionate degradation. Environ. Sci. Technol. 48, 7536–7543. doi: 10.1021/es5016789
Dang, Y., Holmes, D. E., Zhao, Z., Woodard, T. L., Zhang, Y., Sun, D., et al. (2016). Enhancing anaerobic digestion of complex organic waste with carbon-based conductive materials. Bioresour. Technol. 220, 516–522. doi: 10.1016/j.biortech.2016.08.114
Dang, Y., Sun, D., Woodard, T. L., Wang, L. Y., Nevin, K. P., and Holmes, D. E. (2017). Stimulation of the anaerobic digestion of the dry organic fraction of municipal solid waste (OFMSW) with carbon-based conductive materials. Bioresour. Technol. 238, 30–38. doi: 10.1016/j.biortech.2017.04.021
Dong, X., and Stams, A. J. M. (1995). Evidence for H2 and formate formation during syntrophic butyrate and propionate degradation. Anaerobe 1, 35–39. doi: 10.1016/S1075-9964(95)80405-6
Feng, S., Hou, S., Huang, X., Fang, Z., Tong, Y., and Yang, H. (2019). Insights into the microbial community structure of anaerobic digestion of municipal solid waste landfill leachate for methane production by adaptive thermophilic granular sludge. Electron. J. Biotechnol. 39, 98–106. doi: 10.1016/j.ejbt.2019.04.001
Ferry, J. G. (2020). Methanosarcina acetivorans: a model for mechanistic understanding of aceticlastic and reverse methanogenesis. Front. Microbiol. 11:1806. doi: 10.3389/fmicb.2020.01806
Figueiredo, J. L., Pereira, M. F. R., Freitas, M. M. A., and Órfão, J. J. M. (1999). Modification of the surface chemistry of activated carbons. Carbon 37, 1379–1389. doi: 10.1016/S0008-6223(98)00333-9
Fujinawa, K., Nagoya, M., Kouzuma, A., and Watanabe, K. (2019). Conductive carbon nanoparticles inhibit methanogens and stabilize hydrogen production in microbial electrolysis cells. Appl. Microbiol. Biotechnol. 103, 6385–6392. doi: 10.1007/s00253-019-09946-1
Gahlot, P., Ahmed, B., Tiwari, S. B., Aryal, N., Khursheed, A., Kazmi, A. A., et al. (2020). Conductive material engineered direct interspecies electron transfer (DIET) in anaerobic digestion: mechanism and application. Environ. Technol. Innov. 20:101056. doi: 10.1016/j.eti.2020.101056
Hirano, S., Matsumoto, N., Morita, M., Sasaki, K., and Ohmura, N. (2013). Electrochemical control of redox potential affects methanogenesis of the hydrogenotrophic methanogen Methanothermobacter thermautotrophicus. Lett. Appl. Microbiol. 56, 315–321. doi: 10.1111/lam.12059
Holmes, D. E., Rotaru, A. E., Ueki, T., Shrestha, P. M., Ferry, J. G., and Lovley, D. R. (2018). Electron and proton flux for carbon dioxide reduction in methanosarcina barkeri during direct interspecies electron transfer. Front. Microbiol. 9:3109. doi: 10.3389/fmicb.2018.03109
Holmes, D. E., Zhou, Z., Ueki, T., Woodard, T., and Lovley, D. R. (2021). Mechanisms for electron uptake by Methanosarcina acetivorans during direct interspecies electron transfer. mBio 12:e02344-21. doi: 10.1128/mBio.02344-21
Igarashi, K., Miyako, E., and Kato, S. (2019). Direct interspecies electron transfer mediated by graphene oxide-based materials. Front. Microbiol. 10:3068. doi: 10.3389/fmicb.2019.03068
Jia, R., Sun, D., Dang, Y., Meier, D., Holmes, D. E., and Smith, J. A. (2020). Carbon cloth enhances treatment of high-strength brewery wastewater in anaerobic dynamic membrane bioreactors. Bioresour. Technol. 298:122547. doi: 10.1016/j.biortech.2019.122547
Jiang, Q., Chen, Y., Yu, S., Zhu, R., Zhong, C., Zou, H., et al. (2020). Effects of citrus peel biochar on anaerobic co-digestion of food waste and sewage sludge and its direct interspecies electron transfer pathway study. Chem. Eng. J. 398:125643. doi: 10.1016/j.cej.2020.125643
Jin, Z., Zhao, Z., and Zhang, Y. (2019). Potential of direct interspecies electron transfer in synergetic enhancement of methanogenesis and sulfate removal in an up-flow anaerobic sludge blanket reactor with magnetite. Sci. Total Environ. 677, 299–306. doi: 10.1016/j.scitotenv.2019.04.372
Jing, Y., Wan, J., Angelidaki, I., Zhang, S., and Luo, G. (2017). iTRAQ quantitative proteomic analysis reveals the pathways for methanation of propionate facilitated by magnetite. Water Res. 108, 212–221. doi: 10.1016/j.watres.2016.10.077
Jung, H., Baek, G., and Lee, C. (2020). Magnetite-assisted in situ microbial oxidation of H2S to S0 during anaerobic digestion: a new potential for sulfide control. Chem. Eng. J. 397:124982. doi: 10.1016/j.cej.2020.124982
Kato, S., Hashimoto, K., and Watanabe, K. (2012). Methanogenesis facilitated by electric syntrophy via (semi)conductive iron-oxide minerals. Environ. Microbiol. 14, 1646–1654. doi: 10.1111/j.1462-2920.2011.02611.x
Kayhanian, M. (1999). Ammonia inhibition in high-solids biogasification: an overview and practical solutions. Environ. Technol. 20, 355–365. doi: 10.1080/09593332008616828
Leang, C., Qian, X., Mester, T., and Lovley, D. R. (2010). Alignment of the c-type cytochrome OmcS along pili of Geobacter sulfurreducens. Appl. Environ. Microbiol. 76, 4080–4084. doi: 10.1128/AEM.00023-10
Lee, J. Y., Lee, S. H., and Park, H. D. (2016). Enrichment of specific electro-active microorganisms and enhancement of methane production by adding granular activated carbon in anaerobic reactors. Bioresour. Technol. 205, 205–212. doi: 10.1016/j.biortech.2016.01.054
Lei, Y., Sun, D., Dang, Y., Chen, H., Zhao, Z., Zhang, Y., et al. (2016). Stimulation of methanogenesis in anaerobic digesters treating leachate from a municipal solid waste incineration plant with carbon cloth. Bioresour. Technol. 222, 270–276. doi: 10.1016/j.biortech.2016.10.007
Lei, Y., Wei, L., Liu, T., Xiao, Y., Dang, Y., Sun, D., et al. (2018). Magnetite enhances anaerobic digestion and methanogenesis of fresh leachate from a municipal solid waste incineration plant. Chem. Eng. J. 348, 992–999. doi: 10.1016/j.cej.2018.05.060
Li, H., Chang, J., Liu, P., Fu, L., Ding, D., and Lu, Y. (2015). Direct interspecies electron transfer accelerates syntrophic oxidation of butyrate in paddy soil enrichments. Environ. Microbiol. 17, 1533–1547. doi: 10.1111/1462-2920.12576
Li, Q., Li, Y. Y., Qiao, W., Wang, X., and Takayanagi, K. (2015). Sulfate addition as an effective method to improve methane fermentation performance and propionate degradation in thermophilic anaerobic co-digestion of coffee grounds, milk and waste activated sludge with AnMBR. Bioresour. Technol. 185, 308–315. doi: 10.1016/j.biortech.2015.03.019
Li, J., Xiao, L., Zheng, S., Zhang, Y., Luo, M., Tong, C., et al. (2018). A new insight into the strategy for methane production affected by conductive carbon cloth in wetland soil: beneficial to acetoclastic methanogenesis instead of CO2 reduction. Sci. Total Environ. 643, 1024–1030. doi: 10.1016/j.scitotenv.2018.06.271
Li, Y., Sun, Y., Li, L., and Yuan, Z. (2018). Acclimation of acid-tolerant methanogenic propionate-utilizing culture and microbial community dissecting. Bioresour. Technol. 250, 117–123. doi: 10.1016/j.biortech.2017.11.034
Li, L., Xu, Y., Dai, X., and Dai, L. (2021). Principles and advancements in improving anaerobic digestion of organic waste via direct interspecies electron transfer. Renew. Sustain. Energy Rev. 148:111367. doi: 10.1016/j.rser.2021.111367
Li, Y., Chen, Y., and Wu, J. (2019). Enhancement of methane production in anaerobic digestion process: a review. Appl. Energ. 240, 120–137. doi: 10.1016/j.apenergy.2019.01.243
Li, Y., Liu, M., Che, X., Li, C., Liang, D., Zhou, H., et al. (2020a). Biochar stimulates growth of novel species capable of direct interspecies electron transfer in anaerobic digestion via ethanol-type fermentation. Environ. Res. 189:109983. doi: 10.1016/j.envres.2020.109983
Li, Y., Tang, Y., Xiong, P., Zhang, M., Deng, Q., Liang, D., et al. (2020b). High-efficiency methanogenesis via kitchen wastes served as ethanol source to establish direct interspecies electron transfer during anaerobic co-digestion with waste activated sludge. Water Res. 176:115763. doi: 10.1016/j.watres.2020.115763
Li, Y., Zhang, Y., Yang, Y., Quan, X., and Zhao, Z. (2017). Potentially direct interspecies electron transfer of methanogenesis for syntrophic metabolism under sulfate reducing conditions with stainless steel. Bioresour. Technol. 234, 303–309. doi: 10.1016/j.biortech.2017.03.054
Lin, R., Cheng, J., Zhang, J., Zhou, J., Cen, K., and Murphy, J. D. (2017). Boosting biomethane yield and production rate with graphene: the potential of direct interspecies electron transfer in anaerobic digestion. Bioresour. Technol. 239, 345–352. doi: 10.1016/j.biortech.2017.05.017
Liu, F., Rotaru, A. E., Shrestha, P. M., Malvankar, N. S., Nevin, K. P., and Lovley, D. R. (2012). Promoting direct interspecies electron transfer with activated carbon. Energy Environ. Sci. 5, 8982–8989. doi: 10.1039/C2EE22459C
Liu, F., Rotaru, A. E., Shrestha, P. M., Malvankar, N. S., Nevin, K. P., and Lovley, D. R. (2015). Magnetite compensates for the lack of a pilin-associated c-type cytochrome in extracellular electron exchange. Environ. Microbiol. 17, 648–655. doi: 10.1111/1462-2920.12485
Liu, X., Zhan, J., Liu, L., Gan, F., Ye, J., Nealson, H., et al. (2021). In situ spectroelectrochemical characterization reveals cytochrome-mediated electric syntrophy in Geobacter coculture. Environ. Sci. Technol. 55, 10142–10151. doi: 10.1021/acs.est.1c00356
Liu, Y., Gu, M., Yin, Q., and Wu, G. (2019). Inhibition mitigation and ecological mechanism of mesophilic methanogenesis triggered by supplement of ferroferric oxide in sulfate-containing systems. Bioresour. Technol. 288:121546. doi: 10.1016/j.biortech.2019.121546
Lovley, D. R. (2017). Syntrophy goes electric: direct interspecies electron transfer. Annu. Rev. Microbiol. 71, 643–664. doi: 10.1146/annurev-micro-030117-020420
Lu, F., Luo, C., Shao, L., and He, P. (2016). Biochar alleviates combined stress of ammonium and acids by firstly enriching Methanosaeta and then Methanosarcina. Water Res. 90, 34–43. doi: 10.1016/j.watres.2015.12.029
Luo, C., Lu, F., Shao, L., and He, P. (2015). Application of eco-compatible biochar in anaerobic digestion to relieve acid stress and promote the selective colonization of functional microbes. Water Res. 68, 710–718. doi: 10.1016/j.watres.2014.10.052
Luo, G., Li, J., Li, Y., Wang, Z., Li, W. T., and Li, A. M. (2016). Performance, kinetics behaviors and microbial community of internal circulation anaerobic reactor treating wastewater with high organic loading rate: role of external hydraulic circulation. Bioresour. Technol. 222, 470–477. doi: 10.1016/j.biortech.2016.10.023
Malvankar, N. S., and Lovley, D. R. (2012). Microbial nanowires: a new paradigm for biological electron transfer and bioelectronics. ChemSusChem 5, 1039–1046. doi: 10.1002/cssc.201100733
Malvankar, N. S., Vargas, M., Nevin, K. P., Franks, A. E., Leang, C., Kim, B. C., et al. (2011). Tunable metallic-like conductivity in microbial nanowire networks. Nat. Nanotechnol. 6, 6573–6579. doi: 10.1038/nnano.2011.119
Martins, G., Salvador, A. F., Pereira, L., and Alves, M. M. (2018). Methane production and conductive materials: a critical review. Environ. Sci. Technol. 52, 10241–10253. doi: 10.1021/acs.est.8b01913
Nagarajan, H., Embree, M., Rotaru, A. E., Shrestha, P. M., Feist, A. M., Palsson, B. O., et al. (2013). Characterization and modelling of interspecies electron transfer mechanisms and microbial community dynamics of a syntrophic association. Nat. Commun. 4:2809. doi: 10.1038/ncomms3809
Nguyen, L. N., Vu, M. T., Johir, M., Pernice, M., Hao, H. N., Zdarta, J., et al. (2021). Promotion of direct interspecies electron transfer and potential impact of conductive materials in anaerobic digestion and its downstream processing. Bioresour. Technol. 341:125847. doi: 10.1016/j.biortech.2021.125847
Park, J. H., Kang, H. J., Park, K. H., and Park, H. D. (2018a). Direct interspecies electron transfer via conductive materials: a perspective for anaerobic digestion applications. Bioresour. Technol. 254, 300–311. doi: 10.1016/j.biortech.2018.01.095
Park, J. H., Park, J. H., Je Seong, H., Sul, W. J., Jin, K. H., and Park, H. D. (2018b). Metagenomic insight into methanogenic reactors promoting direct interspecies electron transfer via granular activated carbon. Bioresour. Technol. 259, 414–422. doi: 10.1016/j.biortech.2018.03.050
Peng, H., Zhang, Y., Tan, D., Zhao, Z., Zhao, H., and Quan, X. (2018). Roles of magnetite and granular activated carbon in improvement of anaerobic sludge digestion. Bioresour. Technol. 249, 666–672. doi: 10.1016/j.biortech.2017.10.047
Qi, Q., Sun, C., Zhang, J., He, Y., and Wah Tong, Y. (2021). Internal enhancement mechanism of biochar with graphene structure in anaerobic digestion: the bioavailability of trace elements and potential direct interspecies electron transfer. Chem. Eng. J. 406:126833. doi: 10.1016/j.cej.2020.126833
Qian, Z., Tianwei, H., Mackey, H. R., van Loosdrecht, M. C. M., and Guanghao, C. (2019). Recent advances in dissimilatory sulfate reduction: from metabolic study to application. Water Res. 150, 162–181. doi: 10.1016/j.watres.2018.11.018
Rotaru, A. E., Shrestha, P. M., Liu, F., Markovaite, B., Chen, S., Nevin, K. P., et al. (2014a). Direct interspecies electron transfer between Geobacter metallireducens and Methanosarcina barkeri. Appl. Environ. Microbiol. 80, 4599–4605. doi: 10.1128/AEM.00895-14
Rotaru, A. E., Shrestha, P. M., Liu, F., Shrestha, M., Shrestha, D., Embree, M., et al. (2014b). A new model for electron flow during anaerobic digestion: direct interspecies electron transfer to Methanosaeta for the reduction of carbon dioxide to methane. Energy Environ. Sci. 7, 408–415. doi: 10.1039/C3EE42189A
Ruiz, V., Ilhan, Z. E., Kang, D. W., Brown, R. K., and Buitrón, G. (2014). The source of inoculum plays a defining role in the development of MEC microbial consortia fed with acetic and propionic acid mixtures. J. Biotechnol. 182-183, 11–18. doi: 10.1016/j.jbiotec.2014.04.016
Salvador, A. F., Martins, G., Melle-Franco, M., Serpa, R., Stams, A. J. M., Cavaleiro, A. J., et al. (2017). Carbon nanotubes accelerate methane production in pure cultures of methanogens and in a syntrophic coculture. Environ. Microbiol. 19, 2727–2739. doi: 10.1111/1462-2920.13774
Shen, N., Liang, Z., Chen, Y., Song, H., and Wan, J. (2020). Enhancement of syntrophic acetate oxidation pathway via single walled carbon nanotubes addition under high acetate concentration and thermophilic condition. Bioresour. Technol. 306:123182. doi: 10.1016/j.biortech.2020.123182
Shrestha, P. M., Rotaru, A. E., Summers, Z. M., Shrestha, M., Liu, F., and Lovley, D. R. (2013). Transcriptomic and genetic analysis of direct interspecies electron transfer. Appl. Environ. Microbiol. 79, 2397–2404. doi: 10.1128/AEM.03837-12
Storck, T., Virdis, B., and Batstone, D. J. (2015). Modelling extracellular limitations for mediated versus direct interspecies electron transfer. ISME J. 10, 621–631. doi: 10.1038/ismej.2015.139
Suharti, S., Wang, M., de Vries, S., and Ferry, J. G. (2014). Characterization of the RnfB and RnfG subunits of the Rnf complex from the archaeon Methanosarcina acetivorans. PLoS One 9:e97966. doi: 10.1371/journal.pone.0097966
Summers, Z. M., Fogarty, H. E., Leang, C., Franks, A. E., Malvankar, N. S., and Lovley, D. R. (2010). Direct exchange of electrons within aggregates of an evolved syntrophic coculture of anaerobic bacteria. Science 330, 1413–1415. doi: 10.1126/science.1196526
Sun, W. X., Fu, S. F., Zhu, R., Wang, Z. Y., Zou, H., and Zheng, Y. (2020). Improved anaerobic digestion efficiency of high-solid sewage sludge by enhanced direct interspecies electron transfer with activated carbon mediator. Bioresour. Technol. 313:123648. doi: 10.1016/j.biortech.2020.123648
Tan, J., Wang, J., Xue, J., Liu, S. Y., Peng, S. C., Ma, D., et al. (2015). Methane production and microbial community analysis in the goethite facilitated anaerobic reactors using algal biomass. Fuel 145, 196–201. doi: 10.1016/j.fuel.2014.12.087
Tatara, M., Makiuchi, T., Ueno, Y., Goto, M., and Sode, K. (2008). Methanogenesis from acetate and propionate by thermophilic down-flow anaerobic packed-bed reactor. Bioresour. Technol. 99, 4786–4795. doi: 10.1016/j.biortech.2007.09.069
Thauer, R. K., Kaster, A. K., Seedorf, H., Buckel, W., and Hedderich, R. (2008). Methanogenic archaea: ecologically relevant differences in energy conservation. Nat. Rev. Microbiol. 6, 579–591. doi: 10.1038/nrmicro1931
Tian, T., Qiao, S., Li, X., Zhang, M., and Zhou, J. (2017). Nano-graphene induced positive effects on methanogenesis in anaerobic digestion. Bioresour. Technol. 224, 41–47. doi: 10.1016/j.biortech.2016.10.058
Ueki, T., Nevin, K. P., Rotaru, A. E., Wang, L. Y., Ward, J. E., Woodard, T. L., et al. (2018). Geobacter strains expressing poorly conductive pili reveal constraints on direct interspecies electron transfer mechanisms. mBio 9:e-1273-18. doi: 10.1128/mBio.01273-18
Walker, D., Martz, E., Holmes, D. E., Zhou, Z., and Lovley, D. R. (2019). The archaellum of methanospirillum hungatei is electrically conductive. mBio 10:e00579-19. doi: 10.1128/mBio.00579-19
Wang, D., Ai, J., Shen, F., Yang, G., Zhang, Y., Deng, S., et al. (2017). Improving anaerobic digestion of easy-acidification substrates by promoting buffering capacity using biochar derived from vermicompost. Bioresour. Technol. 227, 286–296. doi: 10.1016/j.biortech.2016.12.060
Wang, G., Gao, X., Li, Q., Zhao, H., Liu, Y., Wang, X. C., et al. (2020a). Redox-based electron exchange capacity of biowaste-derived biochar accelerates syntrophic phenol oxidation for methanogenesis via direct interspecies electron transfer. J. Hazard. Mater. 390:121726. doi: 10.1016/j.jhazmat.2019.121726
Wang, G., Li, Q., Yuwen, C., Gong, K., Sheng, L., Li, Y., et al. (2020b). Biochar triggers methanogenesis recovery of a severely acidified anaerobic digestion system via hydrogen-based syntrophic pathway inhibition. Int. J. Hydrogen Energy 46, 9666–9677. doi: 10.1016/j.ijhydene.2020.03.115
Wang, Z., Yun, S., Shi, J., Han, F., Liu, B., Wang, R., et al. (2020c). Critical evidence for direct interspecies electron transfer with tungsten-based accelerants: an experimental and theoretical investigation. Bioresour. Technol. 311:123519. doi: 10.1016/j.biortech.2020.123519
Wang, G., Li, Q., Gao, X., and Wang, X. C. (2018). Synergetic promotion of syntrophic methane production from anaerobic digestion of complex organic wastes by biochar: performance and associated mechanisms. Bioresour. Technol. 250, 812–820. doi: 10.1016/j.biortech.2017.12.004
Wang, P., Wang, H., Qiu, Y., Ren, L., and Jiang, B. (2018). Microbial characteristics in anaerobic digestion process of food waste for methane production–A review. Bioresour. Technol. 248, 29–36. doi: 10.1016/j.biortech.2017.06.152
Wang, L., Zhang, H., Dai, Z., Liu, Y., Chen, C., and Liu, G. (2021). Effect of nicotine inhibition on anaerobic digestion and the co-digestion performance of tobacco stalks with different animal manures. Process Saf. Environ. Prot. 146, 377–382. doi: 10.1016/j.psep.2020.09.005
Xu, H., Chang, J., Wang, H., Liu, Y., Zhang, X., Liang, P., et al. (2019). Enhancing direct interspecies electron transfer in syntrophic-methanogenic associations with (semi)conductive iron oxides: effects and mechanisms. Sci. Total Environ. 695:133876. doi: 10.1016/j.scitotenv.2019.133876
Xu, S., Han, R., Zhang, Y., He, C., and Liu, H. (2018). Differentiated stimulating effects of activated carbon on methanogenic degradation of acetate, propionate and butyrate. Waste Manag. 76, 394–403. doi: 10.1016/j.wasman.2018.03.037
Xu, Y., Wang, M., Yu, Q., and Zhang, Y. (2020). Enhancing methanogenesis from anaerobic digestion of propionate with addition of Fe oxides supported on conductive carbon cloth. Bioresour. Technol. 302:122796. doi: 10.1016/j.biortech.2020.122796
Yamada, C., Kato, S., Ueno, Y., Ishii, M., and Igarashi, Y. (2015). Conductive iron oxides accelerate thermophilic methanogenesis from acetate and propionate. J. Biosci. Bioeng. 119, 678–682. doi: 10.1016/j.jbiosc.2014.11.001
Yan, W., Shen, N., Xiao, Y., Chen, Y., Sun, F., Kumar Tyagi, V., et al. (2017). The role of conductive materials in the start-up period of thermophilic anaerobic system. Bioresour. Technol. 239, 336–344. doi: 10.1016/j.biortech.2017.05.046
Yan, W., Sun, F., Liu, J., and Zhou, Y. (2018). Enhanced anaerobic phenol degradation by conductive materials via EPS and microbial community alteration. Chem. Eng. J. 352, 1–9. doi: 10.1016/j.cej.2018.06.187
Yang, Y., Zhang, Y., Li, Z., Zhao, Z., Quan, X., and Zhao, Z. (2017). Adding granular activated carbon into anaerobic sludge digestion to promote methane production and sludge decomposition. J. Clean. Prod. 149, 1101–1108. doi: 10.1016/j.jclepro.2017.02.156
Yang, Z., Shi, X., Wang, C., Wang, L., and Guo, R. (2015a). Magnetite nanoparticles facilitate methane production from ethanol via acting as electron acceptors. Sci. Rep. 5:16118. doi: 10.1038/srep16118
Yang, Z., Xu, X., Guo, R., Fan, X., and Zhao, X. (2015b). Accelerated methanogenesis from effluents of hydrogen-producing stage in anaerobic digestion by mixed cultures enriched with acetate and nano-sized magnetite particles. Bioresour. Technol. 190, 132–139. doi: 10.1016/j.biortech.2015.04.057
Ye, Q., Zhang, Z., Huang, Y., Fang, T., Cui, Q., He, C., et al. (2018). Enhancing electron transfer by magnetite during phenanthrene anaerobic methanogenic degradation. Int. Biodeterior. Biodegrad. 129, 109–116. doi: 10.1016/j.ibiod.2018.01.012
Yee, M. O., and Rotaru, A. E. (2020). Extracellular electron uptake in Methanosarcinales is independent of multiheme c-type cytochromes. Sci. Rep. 10:372. doi: 10.1038/s41598-019-57206-z
Yin, Q., He, K., Liu, A., and Wu, G. (2017a). Enhanced system performance by dosing ferroferric oxide during the anaerobic treatment of tryptone-based high-strength wastewater. Appl. Microbiol. Biotechnol. 101, 3929–3939. doi: 10.1007/s00253-017-8194-8
Yin, Q., Miao, J., Li, B., and Wu, G. (2017b). Enhancing electron transfer by ferroferric oxide during the anaerobic treatment of synthetic wastewater with mixed organic carbon. Int. Biodeterior. Biodegrad. 119, 104–110. doi: 10.1016/j.ibiod.2016.09.023
Yin, Q., and Wu, G. (2019). Advances in direct interspecies electron transfer and conductive materials: electron flux, organic degradation and microbial interaction. Biotechnol. Adv. 37:107443. doi: 10.1016/j.biotechadv.2019.107443
Yuan, T., Shi, X., Sun, R., Ko, J. H., and Xu, Q. (2021). Simultaneous addition of biochar and zero-valent iron to improve food waste anaerobic digestion. J. Cleaner Prod. 278:123627. doi: 10.1016/j.jclepro.2020.123627
Zhang, J., Zhang, R., Wang, H., and Yang, K. (2020). Direct interspecies electron transfer stimulated by granular activated carbon enhances anaerobic methanation efficiency from typical kitchen waste lipid-rapeseed oil. Sci. Total Environ. 704:135282. doi: 10.1016/j.scitotenv.2019.135282
Zhang, L., Zhang, J., and Loh, K. (2018). Activated carbon enhanced anaerobic digestion of food waste-laboratory-scale and pilot-scale operation. Waste Manage. 75, 270–279. doi: 10.1016/j.wasman.2018.02.020
Zhao, J., Westerholm, M., Qiao, W., Yin, D., Bi, S., Jiang, M., et al. (2018). Impact of temperature and substrate concentration on degradation rates of acetate, propionate and hydrogen and their links to microbial community structure. Bioresour. Technol. 256, 44–52. doi: 10.1016/j.biortech.2018.01.150
Zhao, Z., Li, Y., He, J., and Zhang, Y. (2018). Establishing direct interspecies electron transfer during laboratory-scale anaerobic digestion of waste activated sludge via biological ethanol-type fermentation pretreatment. ACS Sustain. Chem. Eng. 6, 13066–13077. doi: 10.1021/acssuschemeng.8b02618
Zhao, Z., Li, Y., Quan, X., and Zhang, Y. (2017a). New application of ethanol-type fermentation: stimulating methanogenic communities with ethanol to perform direct interspecies electron transfer. ACS Sustain. Chem. Eng. 5, 9441–9453. doi: 10.1021/acssuschemeng.7b02581
Zhao, Z., Li, Y., Quan, X., and Zhang, Y. (2017b). Towards engineering application: potential mechanism for enhancing anaerobic digestion of complex organic waste with different types of conductive materials. Water Res. 115, 266–277. doi: 10.1016/j.watres.2017.02.067
Zhao, Z., Zhang, Y., Li, Y., Dang, Y., Zhu, T., and Quan, X. (2017c). Potentially shifting from interspecies hydrogen transfer to direct interspecies electron transfer for syntrophic metabolism to resist acidic impact with conductive carbon cloth. Chem. Eng. J. 333, 10–18. doi: 10.1016/j.cej.2016.11.149
Zhao, Z., Li, Y., Zhang, Y., and Lovley, D. R. (2020). Sparking anaerobic digestion: promoting direct interspecies electron transfer to enhance methane production. iScience 23:10179. doi: 10.1016/j.isci.2020.101794
Zhao, Z., and Zhang, Y. (2019). Application of ethanol-type fermentation in establishment of direct interspecies electron transfer: a practical engineering case study. Renew. Energy 136, 846–855. doi: 10.1016/j.renene.2019.01.055
Zhao, Z., Zhang, Y., Holmes, D. E., Dang, Y., Woodard, T. L., Nevin, K. P., et al. (2016a). Potential enhancement of direct interspecies electron transfer for syntrophic metabolism of propionate and butyrate with biochar in up-flow anaerobic sludge blanket reactors. Bioresour. Technol. 209, 148–156. doi: 10.1016/j.biortech.2016.03.005
Zhao, Z., Zhang, Y., Yu, Q., Dang, Y., Li, Y., and Quan, X. (2016b). Communities stimulated with ethanol to perform direct interspecies electron transfer for syntrophic metabolism of propionate and butyrate. Water Res. 102, 475–484. doi: 10.1016/j.watres.2016.07.005
Zhao, Z., Zhang, Y., Woodard, T. L., Nevin, K. P., and Lovley, D. R. (2015). Enhancing syntrophic metabolism in up-flow anaerobic sludge blanket reactors with conductive carbon materials. Bioresour. Technol. 191, 140–145. doi: 10.1016/j.biortech.2015.05.007
Zhou, S., Xu, J., Yang, G., and Zhuang, L. (2014). Methanogenesis affected by the co-occurrence of iron (III) oxides and humic substances. FEMS Microbiol. Ecol. 88, 107–120. doi: 10.1111/1574-6941.12274
Zhou, Z., Holmes, D. E., Tang, H. Y., and Lovley, D. R. (2021). Correlation of key physiological properties of methanosarcina isolates with environment of origin. Appl. Environ. Microbiol. 87:e0073121. doi: 10.1128/AEM.00731-21
Zhu, M. Y., Peng, S. C., Tao, W., Wang, J., Tang, T., Chen, T.-H., et al. (2017). Response of methane production and microbial community to the enrichment of soluble microbial products in goethite-dosed anaerobic reactors. Fuel 191, 495–499. doi: 10.1016/j.fuel.2016.11.108
Zhuang, L., Ma, J., Yu, Z., Wang, Y., and Tang, J. (2018). Magnetite accelerates syntrophic acetate oxidation in methanogenic systems with high ammonia concentrations. Microb. Biotechnol. 11, 710–720. doi: 10.1111/1751-7915.13286
Keywords: anaerobic digestion, direct interspecies electron transfer, conductive materials, methanogenesis, syntrophic metabolism
Citation: Chen L, Fang W, Chang J, Liang J, Zhang P and Zhang G (2022) Improvement of Direct Interspecies Electron Transfer via Adding Conductive Materials in Anaerobic Digestion: Mechanisms, Performances, and Challenges. Front. Microbiol. 13:860749. doi: 10.3389/fmicb.2022.860749
Received: 23 January 2022; Accepted: 09 March 2022;
Published: 30 March 2022.
Edited by:
Yong Yuan, Guangdong University of Technology, ChinaReviewed by:
Li Zhuang, Jinan University, ChinaJia Tang, Guangdong Institute of Eco-environmental and Soil Sciences (CAS), China
Copyright © 2022 Chen, Fang, Chang, Liang, Zhang and Zhang. This is an open-access article distributed under the terms of the Creative Commons Attribution License (CC BY). The use, distribution or reproduction in other forums is permitted, provided the original author(s) and the copyright owner(s) are credited and that the original publication in this journal is cited, in accordance with accepted academic practice. No use, distribution or reproduction is permitted which does not comply with these terms.
*Correspondence: Panyue Zhang, cGFueXVlX3poYW5nQGJqZnUuZWR1LmNu; Guangming Zhang, MjAyMDAxN0BoZWJ1dC5lZHUuY24=