- 1Laboratory of Bacterial Evolution and Molecular Epidemiology, Instituto de Tecnologia Química e Biológica, Universidade Nova de Lisboa (ITQB-NOVA), Oeiras, Portugal
- 2Unit for Healthcare-Associated Infections, Surveillance of Antimicrobial Resistance and Consumption, Robert Koch Institute, Berlin, Germany
- 3Department of Microbiology and Immunology, Faculty of Pharmacy, Alexandria University, Alexandria, Egypt
- 4Department of Microbiology and Immunology, Faculty of Pharmacy, AlAlamein International University, Alalamein, Egypt
- 5Biotechnology, Environmental Technology and Valorisation of Bio-Resources Team, Department of Biology, Faculty of Sciences and Techniques of Al Hoceima, Abdelmalek Essaadi University, Tetouan, Morocco
- 6Division of Medical Microbiology, Department of Clinical Laboratory Sciences, Faculty of Health Sciences, University of Cape Town, Cape Town, South Africa
- 7Department of Bacteriology, Noguchi Memorial Institute for Medical Research, College of Health Sciences, University of Ghana, Accra, Ghana
- 8Division of Molecular Biology and Human Genetics, Faculty of Medicine and Health Sciences, DSI-NRF Centre of Excellence for Biomedical Tuberculosis Research, South African Medical Research Council Centre for Tuberculosis Research, Stellenbosch University, Cape Town, South Africa
- 9Department of Microbiology, Obafemi Awolowo University, Ile-Ife, Nigeria
- 10Institute of Medical Microbiology, University Hospital Münster, Münster, Germany
Background: Methicillin-resistant Staphylococcus aureus (MRSA) is a leading cause of hospital-associated (HA) and community-associated (CA) infections globally. The multi-drug resistant nature of this pathogen and its capacity to cause outbreaks in hospital and community settings highlight the need for effective interventions, including its surveillance for prevention and control. This study provides an update on the clonal distribution of MRSA in Africa.
Methods: A systematic review was conducted by screening for eligible English, French, and Arabic articles from November 2014 to December 2020, using six electronic databases (PubMed, EBSCOhost, Web of Science, Scopus, African Journals Online, and Google Scholar). Data were retrieved and analyzed according to the Preferred Reporting Items for Systematic Review and Meta-Analysis guidelines (registered at PROSPERO: CRD42021277238). Genotyping data was based primarily on multilocus sequence types (STs) and Staphylococcal Cassette Chromosome mec (SCCmec) types. We utilized the Phyloviz algorithm in the cluster analysis and categorization of the MRSA STs into various clonal complexes (CCs).
Results: We identified 65 studies and 26 publications from 16 of 54 (30%) African countries that provided sufficient genotyping data. MRSA with diverse staphylococcal protein A (spa) and SCCmec types in CC5 and CC8 were reported across the continent. The ST5-IV [2B] and ST8-IV [2B] were dominant clones in Angola and the Democratic Republic of Congo (DRC), respectively. Also, ST88-IV [2B] was widely distributed across the continent, particularly in three Portuguese-speaking countries (Angola, Cape Verde, and São Tomé and Príncipe). The ST80-IV [2B] was described in Algeria and Egypt, while the HA-ST239/ST241-III [3A] was only identified in Egypt, Ghana, Kenya, and South Africa. ST152-MRSA was documented in the DRC, Kenya, Nigeria, and South Africa. Panton–Valentine leukocidin (PVL)-positive MRSA was observed in several CCs across the continent. The median prevalence of PVL-positive MRSA was 33% (ranged from 0 to 77%; n = 15).
Conclusion: We observed an increase in the distribution of ST1, ST22, and ST152, but a decline of ST239/241 in Africa. Data on MRSA clones in Africa is still limited. There is a need to strengthen genomic surveillance capacity based on a “One-Health” strategy to prevent and control MRSA in Africa.
Background
Methicillin-resistant Staphylococcus aureus (MRSA) is one of the important antibiotic-resistant pathogens and a leading cause of hospital-associated (HA) and community-associated (CA) infections worldwide (Lee et al., 2018). Recently, the World Health Organization (WHO) included MRSA as one of the indicators for antimicrobial resistance in the Sustainable Development Goals connected to the health target 3.d (WHO, 2021). MRSA is a major burden in hospital-acquired neonatal infections in sub-Saharan Africa (Okomo et al., 2019). Vancomycin, a glycopeptide, is considered one of the last therapeutic agents for MRSA infections (McGuinness et al., 2017). However, MRSA isolates from clinical samples exhibiting reduced susceptibility to vancomycin have been documented in Africa (Fortuin-de Smidt et al., 2015; Zorgani et al., 2015; Eshetie et al., 2016; Bamigboye et al., 2018; ElSayed et al., 2018). In addition, mecA-positive (Lozano et al., 2016), mecC-positive MRSA (Dweba and Zishiri, 2019), and vancomycin-resistant (vanA, vanB-positive) MRSA (Al-Amery et al., 2019) have been identified in food animals on the African continent.
There are varying prevalence rates of MRSA reported in Africa (Wangai et al., 2019), and the epidemiological picture depicts diverse clonal types within regions and countries. We published a systematic review on the molecular epidemiology of MRSA in Africa (Abdulgader et al., 2015). It revealed that the pandemic MRSA clones: sequence type (ST) 5 and ST239/241 were dominant on the continent. However, some clones were limited to specific countries (e.g., ST612 in South Africa) or regions (ST80 in North Africa). Moreover, CA-MRSA (ST8 and ST88) were identified in clinical and non-clinical settings (Abdulgader et al., 2015). Africa is described as a Panton–Valentine leukocidin (PVL) endemic region (Schaumburg et al., 2014). Also, the 2015 review observed a PVL prevalence of 0.3–100% among MRSA identified from humans (carriage and infection) in Africa. Despite these findings, data is still limited, and there are knowledge gaps on the clonal nature of MRSA in Africa.
The epidemiology of MRSA is characterized by the occurrence and dissemination of new and emerging clones leading to constant changes globally (Turner et al., 2019). For instance, a steady increase of ST5 and ST93 as the predominant CA-MRSA clones have been described in Australia (Bloomfield et al., 2020), and ST59 has been replaced by ST239 in China (Li et al., 2018). Furthermore, a decline of ST5 and an increase in ST8 cases have been observed in the United States of America (See et al., 2020) and Canada (Guthrie et al., 2020). Since MRSA is a significant public health problem, understanding the changes in epidemiology through regular monitoring and surveillance is essential to minimize its healthcare and economic burden. Therefore, this review aimed to provide an update describing the clonal characteristics of MRSA in Africa.
Methods
This systematic review is a 6-year update on the MRSA clonal diversity in Africa. We performed the systematic literature search and analysis according to the Preferred Reporting Items for Systematic Reviews and Meta-Analyses (PRISMA) (Page et al., 2021). The study was registered in the PROSPERO database (CRD42021277238). Since this review focused on a narrative description of the eligible studies instead of effect sizes and other related quantitative outcomes, methodological features like sample size, study population, use of appropriate study design were not assessed. Therefore, we did not do a formal risk of bias scoring system.
Literature Search Approach
We used six electronic databases to identify and retrieve relevant information (PubMed, EBSCOhost, Web of Science, Scopus, African Journals Online, and Google Scholar). The search included articles published in English, French, and Arabic from November 01, 2014, to December 31, 2020. The literature search date was selected to complement the data previously described (Abdulgader et al., 2015). The literature search was also complemented with Publish or perish literature and citation mining algorithm (Harzing, 2007).
Predefined search terms were used (Supplementary Table 1), first on a continent-wide basis and then for the 54 African countries. Article titles and abstracts were screened and reviewed independently by two authors (OL, AS), including full-text reviews on all eligible studies.
Identification of Eligible Studies
Studies were eligible on the condition that identification of MRSA was based primarily on the molecular detection of the methicillin resistance (mecA) gene (including mecC), and the investigations used at least one molecular tool to characterize the isolates. We also included global surveys that involved African countries. All duplicate articles were removed, and data only on phenotypic antibiotic susceptibility testing to identify methicillin-susceptible Staphylococcus aureus (MSSA) and mecA were excluded. Moreover, African studies that described isolates recovered from humans or animals not resident on the continent were excluded. Sufficient genotyping data was based primarily on multilocus sequence type (MLST) and the Staphylococcal Cassette Chromosome mec (SCCmec) typing nomenclature as previously reported (Abdulgader et al., 2015). Also, we included additional data, e.g., staphylococcal protein A (spa) types and PVL status. The MRSA STs cluster analysis was performed and categorized into various clonal complexes (CCs) using Phyloviz version 2.0.1
Data Extraction and Analyses
We extracted the epidemiological and genotypic data of MRSA from the eligible articles using standardized forms. Publications that described a previously analyzed collection within the period under review were considered as a single study. We determined the PVL rate from eligible studies with a sample size of ≥30 MRSA isolates.
Cluster Analysis and Minimum Spanning Tree
The relationship between the MRSA STs described in this review with other common lineages reported worldwide was analyzed as previously described (Abdulgader et al., 2015). Briefly, we downloaded the allelic profiles of the African MRSA STs from the MLST website.2 Furthermore, 236 randomly selected STs representing the diversity in the database and based on the differences in their allelic profiles were included (Supplementary Table 2). The minimum spanning tree was constructed with the goeBURST algorithm using the Phyloviz version 2.0 (see text footnote 1).
Results
Literature Search and Description of the Articles Included in the Review
The systematic search yielded 3367 articles (Figure 1). We screened 314 full-text articles after removing duplicate studies and assessing titles and abstracts. Overall, 65 studies were considered eligible for the qualitative analysis. The data from these studies were obtained from investigations conducted in 22 countries. Most of the single-center studies were from Egypt (n = 9), Nigeria (n = 9), South Africa (n = 8), Algeria (n = 6), and Ghana (n = 5) (Table 1). Multicentre studies were from six reports. They included four investigations in Portuguese-speaking African countries: Angola, Cape Verde, and São Tomé and Príncipe (Conceição et al., 2015a,b; Aires-de-Sousa et al., 2018; Rodrigues et al., 2018). Others were one study each from Cameroon and South Africa (Founou et al., 2019), Cote d’Ivoire and the Democratic Republic of Congo (DRC) (Schaumburg et al., 2015).
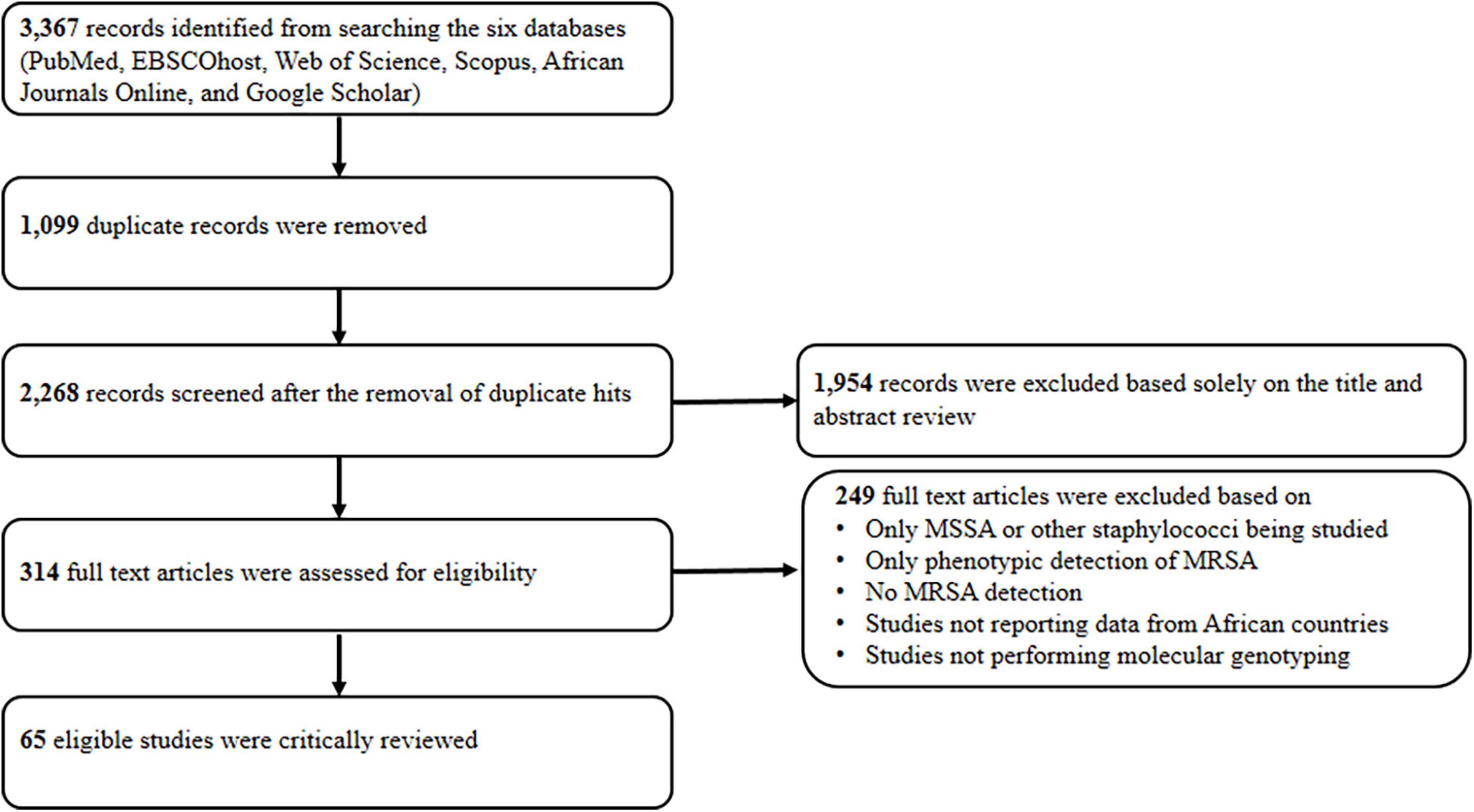
Figure 1. Standard preferred reporting item for systematic reviews. MSSA, methicillin susceptible Staphylococcus aureus; MRSA, methicillin-resistant Staphylococcus aureus.
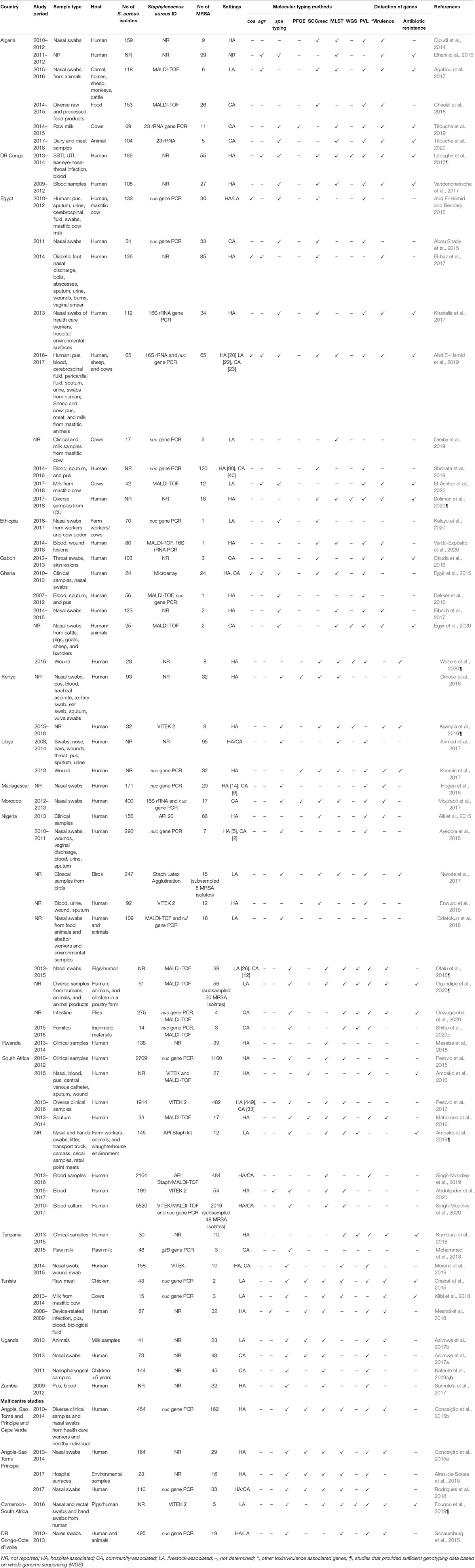
Table 1. Summary of the characteristics of eligible articles on the molecular epidemiology of methicillin-resistant Staphylococcus aureus (MRSA) in Africa.
Identification of S. aureus in more than 50% (36/65) of the eligible studies was based on protein profiling (MALDI-TOF) or methods established on PCR detection of conserved (16S rRNA, nuc, tuf, gltB) genes, or the combination of both. The detection of antibiotic resistance and toxin/virulence genes were described only in 37% (24/65) and 83% (54/65) of the studies, respectively (Table 1). One study reported mecC-positive MRSA from animals (Dweba and Zishiri, 2019). While all the eligible studies characterized MRSA using at least one molecular typing technique, only 40% (26/65) from 16 African countries provided sufficient genotyping data (Supplementary Table 3). Furthermore, 12 studies performed whole-genome sequencing (WGS), of which eight carried out adequate analyses to infer MRSA clones (Table 1).
Source of Methicillin-Resistant Staphylococcus aureus
Methicillin-resistant Staphylococcus aureus from the eligible studies was classified as either HA, CA, or livestock-associated (LA) based on their source of isolation as provided in the articles. Overall, 40% (26/65) of the studies were on HA-MRSA, while 18% (12/65) each were from the community and animal/livestock settings (Table 1). Additionally, 22% (14/65) of studies characterized MRSA from either two (HA-CA: n = 10; HA-LA: n = 2; CA-LA: n = 1) or all the study settings (HA-CA-LA: n = 1). We could not infer the source of isolates in one study.
High Clonal Diversity Among Methicillin-Resistant Staphylococcus aureus Isolates Reported in Africa
We observed a high genetic heterogeneity among MRSA in the 26 eligible studies that provided sufficient genotyping data. Based on MLST, they were classified into 39 STs, four of which were unassigned types (Supplementary Table 3). The MLST cluster analysis using Phyloviz based on the geoBURST algorithm revealed 15 CCs. They comprised mainly CC1, CC5, CC8, CC22, CC30, and CC88. Others were CC7, CC15, CC20, CC45, CC80, CC97, CC121, CC152, and CC398 (Figures 2, 3).
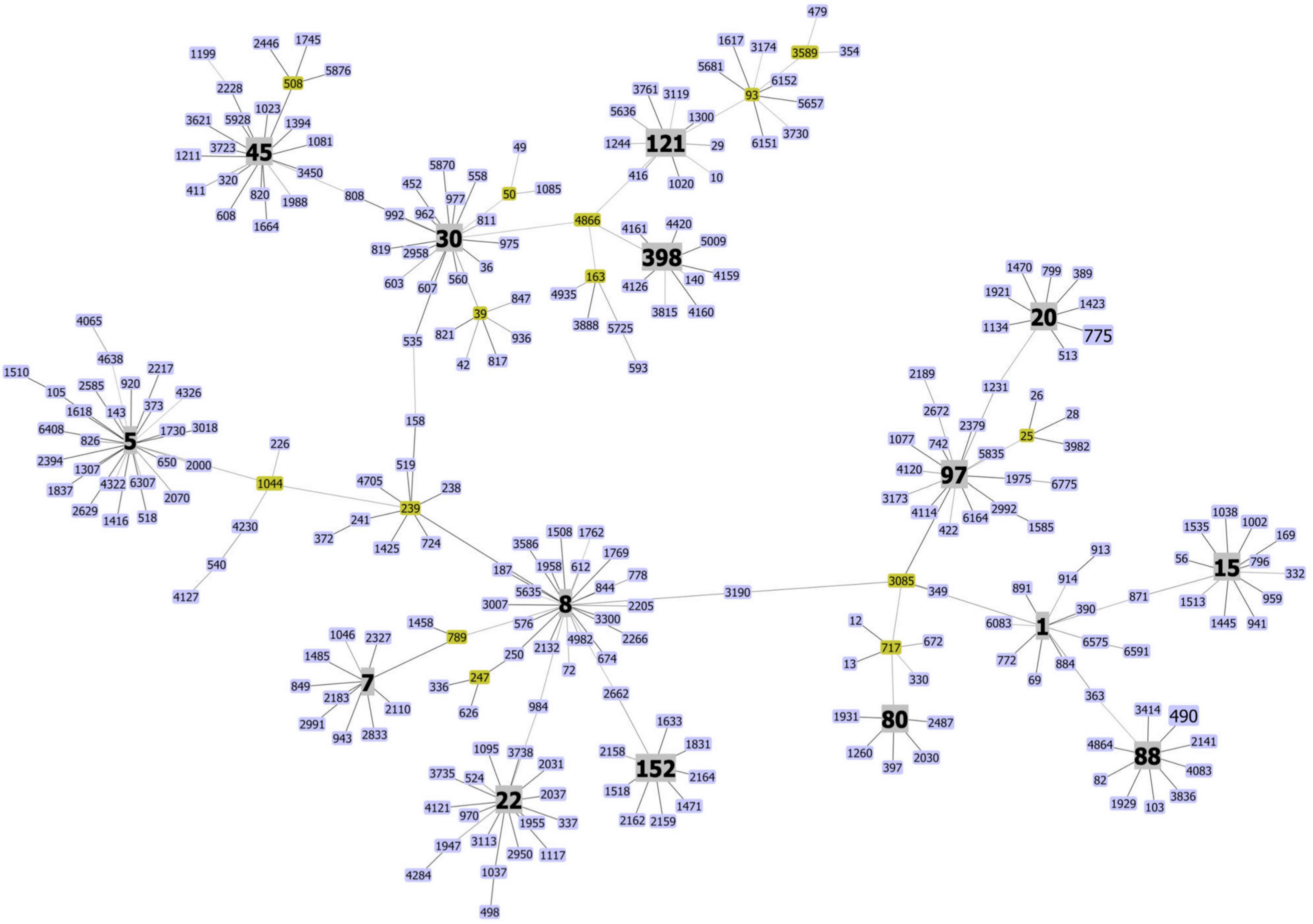
Figure 2. Clonal diversity of methicillin-resistant Staphylococcus aureus in Africa. The minimum spanning tree was constructed with Phyloviz software version 2.0 hosted on http://www.phyloviz.net. The allelic profiles were obtained from the MLST database hosted on (https://pubmlst.org/organisms/staphylococcus-aureus) that included the sequence types of the MRSA described in this review, and 236 randomly selected STs based on the differences in their allelic profiles and representative of the MRSA diversity worldwide. Each node depicts an ST, and nodes centrally located and bearing different colors correspond to a group founder or sub-founder. Clonal complexes (CCs) reported in this study are colored in gray.
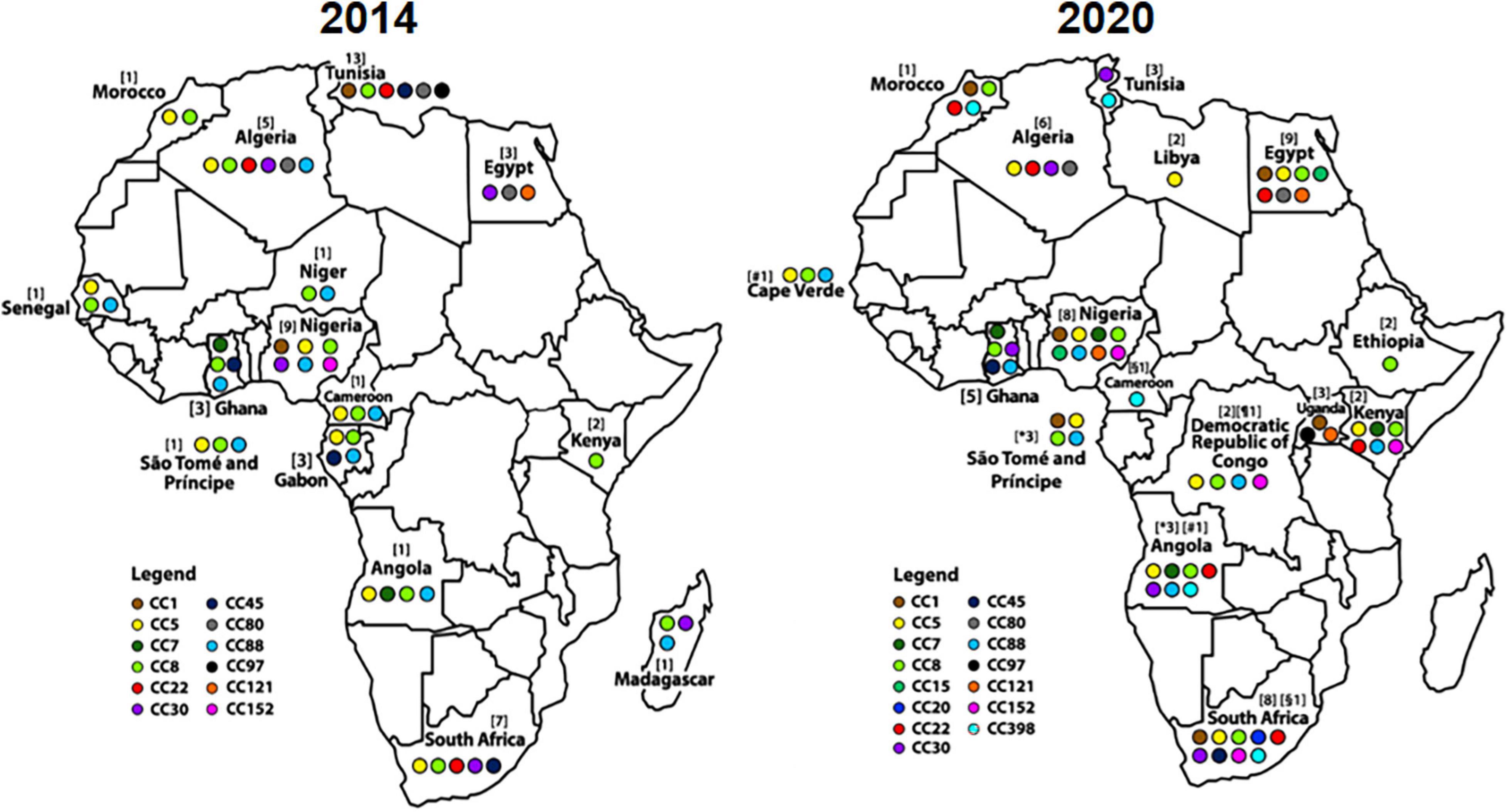
Figure 3. Distribution of methicillin-resistant Staphylococcus aureus (MRSA) clones in Africa as reported (Abdulgader et al., 2015) and this current study (2020). Each clonal complex (CC) is represented with colored oval shape and eligible studies carried out in each country is indicated in parenthesis. The symbols (#, *, §, or ¶) on the right side (2020) depict data that were extracted from the same multicentre study.
Clonal Complex 1
This clone was identified in six countries (Figure 3). PVL-positive t590-ST1-V [5C2] was documented from nasal samples both in hospitalized patients and health care workers (HCWs) in São Tomé and Príncipe (Conceição et al., 2015a,b). Another PVL-positive lineage: t657-ST772-V [5C2] (Bengal Bay Clone), was detected from human nasal samples in the community setting in Nigeria (Ogundipe et al., 2020). Moreover, PVL-negative t127-ST1-IV [2B] was described in a nasal sample of a non-hospitalized individual in Morocco (Mourabit et al., 2017), while it’s variant (t127-ST1-V [5C2]) was identified from non-human specimens (milk products) in Uganda (Asiimwe et al., 2017b). ST1-V [5C2] and ST913-V [5C2] were recovered from clinical samples in Egypt (Soliman et al., 2020). In South Africa, t465-ST1-I [1B]/IV [2B] was isolated from patients with cystic fibrosis (CF) (Mahomed et al., 2018).
Clonal Complex 5
This lineage was reported in 10 countries (Figure 3). The PVL-negative t105-ST5-IV [2B] was the dominant lineage colonizing patients and HCWs (Conceição et al., 2015b; Rodrigues et al., 2018), as well as inanimate surfaces in Angola (Aires-de-Sousa et al., 2018). Also, it was detected in nasal samples of patients and HCWs in São Tomé and Príncipe (Conceição et al., 2015b), and a community patient admitted to a hospital in Algeria (Djoudi et al., 2014). In the DRC, three ST5-IV [2B] variants (t002-ST5-IV [2B], t105-ST5-IV [2B], and PVL-positive t311-ST5-IV [2B]) were described (Lebughe et al., 2017; Vandendriessche et al., 2017). In Kenya, t13150-ST5-II [2A] and t007-ST39-II [2A] were identified from clinical samples (Omuse et al., 2016; Kyany’a et al., 2019). ST5-VI [4B] was reported in a tertiary care hospital in Egypt (Soliman et al., 2020) and Cape Verde (Conceição et al., 2015b). ST5-VII [5C1] was recovered from a patient in the nephrology ward in Algeria (Djoudi et al., 2014). Other reports include ST5-III/V/non-typeable (NT) in South Africa (Abdulgader et al., 2020; Singh-Moodley et al., 2020). The related genotypes such as t6065-ST5/ST2629-V [5C2] in Angola (Conceição et al., 2015a,b), t6065-ST69-V [5C2] in Libya (Khemiri et al., 2017), and t002-ST105-II [2A] in São Tomé and Príncipe (Conceição et al., 2015b) were also noted. One study reported t002/t11469-ST5-V [5C2] in poultry birds (Nworie et al., 2017) in Nigeria (Supplementary Table 3).
Clonal Complex 8
ST8-IV [2B] (with diverse spa types) was documented in hospitalized patients and HCWs in Angola, Cape Verde, and São Tomé and Príncipe (Conceição et al., 2015a; Rodrigues et al., 2018), and clinical samples in Ghana (Egyir et al., 2015) and Kenya (Omuse et al., 2016). PVL-positive t121-ST8-IV [2B] was identified in Cape Verde (Conceição et al., 2015b), Ghana (Egyir et al., 2015), and São Tomé and Príncipe (Rodrigues et al., 2018). The t451-ST8-V [5C2] was one of the dominant clones among hospitalized patients and HCWs in São Tomé and Príncipe (Conceição et al., 2015a,b; Rodrigues et al., 2018). Also, ST8-V [5C2] was described in hospital settings in Angola (Conceição et al., 2015b; Aires-de-Sousa et al., 2018), Egypt (Soliman et al., 2020), Ghana (Egyir et al., 2015), and Kenya (Omuse et al., 2016). The PVL-negative ST8-V/VII (largely t1476) was the major clone in the DRC (Lebughe et al., 2017; Vandendriessche et al., 2017), and Angola (Aires-de-Sousa et al., 2018). Two countries, i.e., Morocco (Mourabit et al., 2017) and Nigeria (Ogundipe et al., 2020), described ST8-V [5C2] with different spa types (t2231, t2658, and t12236) in non-clinical settings. The t456-ST8-I [1B] was only identified in South Africa (Mahomed et al., 2018). Furthermore, ST239/ST241-III [3A] was noted in hospital settings in Egypt (Soliman et al., 2020), Ghana (Egyir et al., 2015), Kenya (Omuse et al., 2016; Kyany’a et al., 2019), and South Africa (Abdulgader et al., 2020; Singh-Moodley et al., 2020). ST612-IV [2B], which comprised mainly spa type t1257, was a major clone in clinical (Singh-Moodley et al., 2020) and non-clinical settings (Amoako et al., 2019) in South Africa. Other related STs include ST72-V [5C2] in Angola (Conceição et al., 2015b; Aires-de-Sousa et al., 2018; Rodrigues et al., 2018) and ST4705-III [3A] in Kenya (Kyany’a et al., 2019).
Clonal Complex 22
ST22-MRSA was identified in six African countries. They include Angola (Conceição et al., 2015b), Algeria (Djoudi et al., 2014), Egypt (Soliman et al., 2020), Kenya (Omuse et al., 2016), and South Africa (Abdulgader et al., 2020; Singh-Moodley et al., 2020). Various spa types (t005, t012, t022, t032, t223, t6397, t11293, and t13149) were associated with this lineage that harbored the SCCmec IV element (Supplementary Table 3). Moreover, it was the major clone recovered from nasal samples of volunteers and outpatients in Tangier, Morocco (Mourabit et al., 2017). Most MRSA isolates from Algeria and Morocco possessed the gene encoding for toxic shock syndrome (tst).
Clonal Complex 30
This clone was observed in both human and animal samples. We identified seven spa types (t012, t018, t030, t037, t045, t064, and t6278; Supplementary Table 3). In South Africa, ST30-II [2A], ST36-II [2A], and ST36-III [3A] were identified from bacteremic patients (Abdulgader et al., 2020; Singh-Moodley et al., 2020), including ST30-I/IV [2B] from CF patients (Mahomed et al., 2018). ST30-V [5C2] was reported in different settings in Angola (Conceição et al., 2015a,b; Rodrigues et al., 2018), and from a chicken meat sample in Tunisia (Chairat et al., 2015). One isolate characterized as t018-ST36-II [2A] was described in Ghana (Egyir et al., 2015) and from the rinsate of processed animals in an abattoir in South Africa (Amoako et al., 2019). Furthermore, the genetically related ST535-IV [2B] was described in a patient in a nephrology ward in Algeria (Djoudi et al., 2014).
Clonal Complex 88
ST88-IV [2B] with diverse spa types (t186, t325, t335, t786, t1451, t1603, t1814, t3869, and t12827) was documented in eight studies from seven African countries (Supplementary Table 3 and Figure 3), particularly in Portuguese-speaking nations. It was widely distributed in Angola (Conceição et al., 2015a,b; Aires-de-Sousa et al., 2018; Rodrigues et al., 2018), Cape Verde (Conceição et al., 2015b), and São Tomé and Príncipe (Conceição et al., 2015a,b; Aires-de-Sousa et al., 2018; Rodrigues et al., 2018). Other reports include the DRC (Lebughe et al., 2017; Vandendriessche et al., 2017), and Ghana (Egyir et al., 2015; Wolters et al., 2020). PVL-negative ST88-IV [2B] was recovered from nasal samples of both humans and pigs in Nigeria (Otalu et al., 2018), and ST88-V [5C2] was detected in a blood culture sample in the DRC (Vandendriessche et al., 2017). ST88-MRSA with a NT SCCmec was identified in Kenya (Omuse et al., 2016).
Other Clonal Complexes
These include eight clones that belonged to smaller (in number or limited spread across countries) groups (Supplementary Table 3 and Figure 3). They consist of CC7 (ST789-IV [2B]/V [5C2]) (Egyir et al., 2015; Omuse et al., 2016; Ogundipe et al., 2020), CC15 (ST15-V [5C2], and ST1535-V [5C2]) (Nworie et al., 2017; Soliman et al., 2020), and CC20 (ST20-IV [2B]) (Mahomed et al., 2018). CC45 comprising ST45-I [1B], ST45-IV [2B], and ST508-I [1B] was detected in CF patients in South Africa (Mahomed et al., 2018). Also, ST508-V [5C2] associated with CC45 was described in Ghana (Egyir et al., 2015). PVL-positive CC80 (ST80-IV [2B]) was only described in Algeria (Djoudi et al., 2014; Agabou et al., 2017) and Egypt (Soliman et al., 2020). CC152 (mostly PVL-positive) with various spa types (t355, t715, t4960, t5691, and t15644) and SCCmec types (I, II, IV, V, and VII) were identified in four countries. They include the DRC (Lebughe et al., 2017; Vandendriessche et al., 2017), Kenya (Kyany’a et al., 2019), Nigeria (Ogundipe et al., 2020), and South Africa (Mahomed et al., 2018). ST121-V [5C2] was documented in Egypt (Soliman et al., 2020) and Uganda (Asiimwe et al., 2017b), in addition to PVL-positive isolates in Nigeria (Ogundipe et al., 2020). The LA ST398-IV [2B]/V [5C2] was recovered from the rectal and nasal samples of pigs in Cameroon, South Africa (Founou et al., 2019), and in the nasal sample of a healthy individual in Morocco (Mourabit et al., 2017). Also, ST398-IV [2B] was detected in raw meat samples in Tunisia (Chairat et al., 2015). MRSA with the genotype ST140-IV [2B] (associated with CC398) was recovered from inanimate surfaces in a health care institution in Angola (Aires-de-Sousa et al., 2018).
The Dynamics of Methicillin-Resistant Staphylococcus aureus Clones (2014–2020)
We compared MRSA clones reported from a previous study (Abdulgader et al., 2015) and the period under review. New genotyping data were available from Cape Verde, Ethiopia, the DRC, Libya, and Uganda. However, reports on MRSA clones from Senegal, Gabon, and Madagascar in the previous study were absent in the current period under review. Overall, genotyping data from 11 African countries (Angola, Algeria, Cameroon, Egypt, Ghana, Kenya, Tunisia, Morocco, Nigeria, São Tomé and Príncipe, and South Africa) in the two study periods were identified and compared (Figure 3). We observed an increase in the number of MRSA clones reported in seven (Angola, Egypt, Kenya, Morocco, Nigeria, São Tomé and Príncipe, and South Africa) of the 11 countries. Specifically, CC1, previously described only in Nigeria and Tunisia (Abdulgader et al., 2015), was identified in clinical and non-clinical settings in six countries (Egypt, Morocco, Nigeria, São Tomé and Príncipe, South Africa, and Uganda). ST22-IV [2B] (CC22), previously documented only in South Africa (Abdulgader et al., 2015), was described in Angola, Algeria, Egypt, Kenya, Morocco, and South Africa. Also, CC152-MRSA identified only in Nigeria (Abdulgader et al., 2015) was reported in the DRC, Kenya, Nigeria, and South Africa. In contrast, the HA Brazilian/Hungarian clone (ST239/241-III [3A]), which was previously described as a major clone on the continent, was noted only in four countries (Egypt, Ghana, Kenya, and South Africa). Some MRSA clones were still limited to specific countries and regions. ST80-IV [2B] (CC80) and ST612-IV [2B] (CC8) were identified only in North African countries and South Africa, respectively. Overall, CC5-MRSA, CC8-MRSA, and CC88-MRSA remained widely distributed across the continent (Figure 3).
Panton–Valentine Leukocidin-Positive Methicillin-Resistant Staphylococcus aureus and Clonal Population in Africa
Methicillin-resistant Staphylococcus aureus carriage of the PVL gene was investigated in 50 of the 65 eligible studies. PVL-positive isolates were reported in 26 studies in 11 countries (Table 1 and Supplementary Table 3). The lineages and countries were: CC1 (Egypt, São Tomé and Príncipe, and Nigeria), CC5 (Algeria, Angola, and DRC), CC8 (Cape Verde, DRC, Ghana, São Tomé and Príncipe, and South Africa), CC22 (Angola), and CC30 (Angola and South Africa). Others are CC80 (Algeria and Egypt), CC121 (Nigeria, Egypt, and Uganda), CC152 (DRC and Nigeria), and CC398 (Cameroon). The prevalence of PVL-positive MRSA ranged from 0% (Otalu et al., 2018) to 77% (23/30) (Ogundipe et al., 2020), with a median of 33% (Table 2).
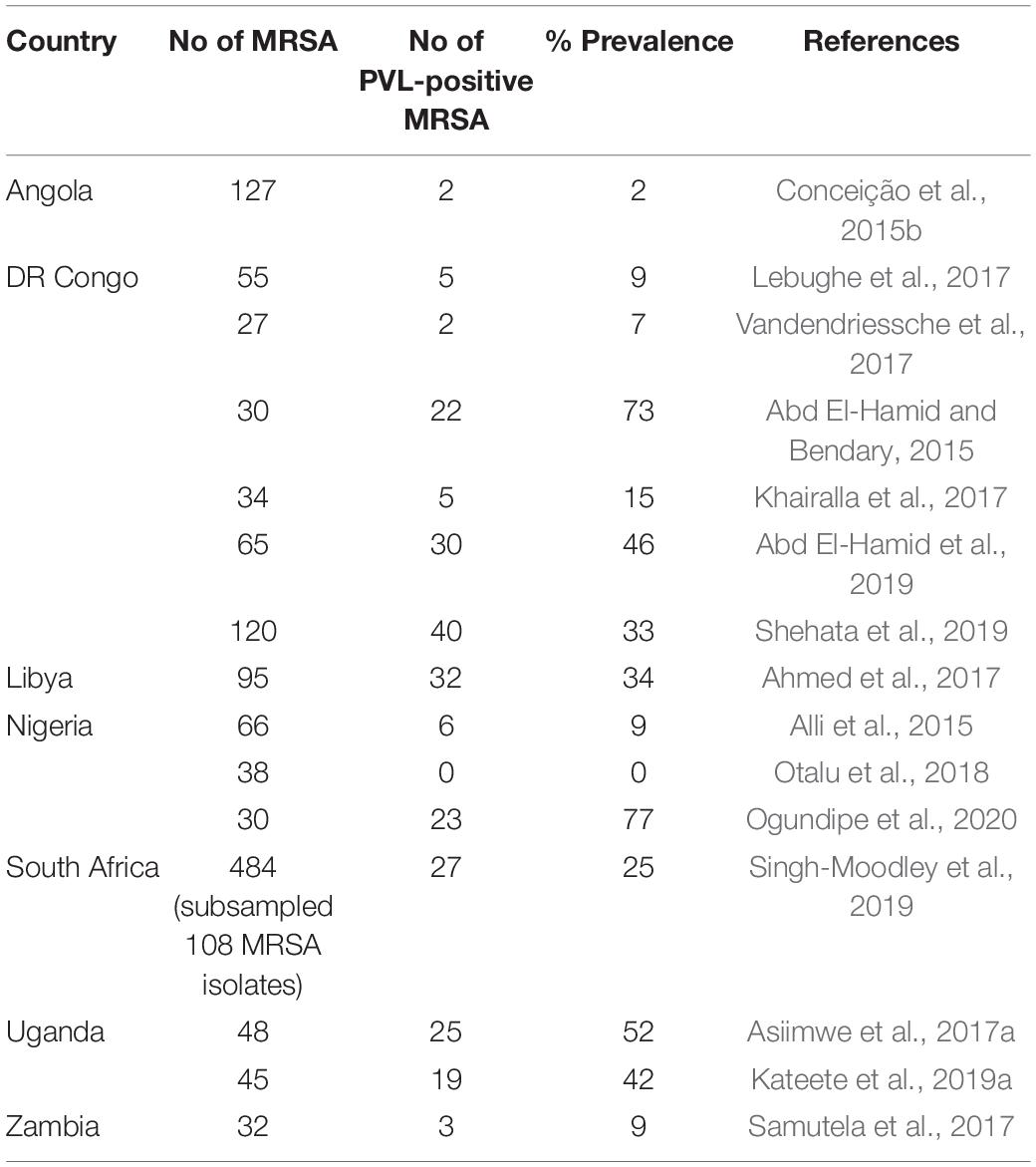
Table 2. Prevalence of Panton–Valentine leukocidin (PVL) gene reported in eligible studies with ≥30 methicillin-resistant Staphylococcus aureus (MRSA) isolates.
Discussion
This systematic review provided an update on the diversity of MRSA clones in Africa for the past 6 years (2014–2020). We observed a slight increase in the number of studies and countries that provided sufficient genotyping data. Diverse MRSA clones were distributed across human, environmental, and animal settings. CC5, CC8, and CC88 were the major clones identified in Africa. Various spa types and SCCmec elements characterized CC5-MRSA. It was postulated that the African ST5-MRSA evolved from ST5-MSSA through acquiring the SCCmec element (Schaumburg et al., 2014). Its capacity and higher propensity to acquire various SCCmec elements could play a significant role in its increased dissemination and adaptation to different environments in Africa. However, the phylogeny, origin, and features for the spread of CC5-MRSA remain unclear in Africa.
Five SCCmec types and 11 spa types were associated with CC8-MRSA suggesting its high diversity in Africa. The CC8 is comprised of the hospital (Archaic [ST250], Iberian [ST247], and Brazilian/Hungarian/EMRSA-1 [ST239]) and CA (USA300 [t008-ST8], USA500 [t064-ST8]) clones (Bowers et al., 2018). ST239 was described as a major clone on the continent (Abdulgader et al., 2015) but has declined in the current period under review. It was identified only in four countries. ST239-MRSA evolved from recombination events between ST8 and ST30, in addition to the acquisition of antibiotic resistance and virulence determinants that contribute to its pathogenic capabilities (Robinson and Enright, 2004; Gill et al., 2021). However, this clone’s low competitive potential relative to ST8 and ST30 could contribute to its gradual decline in different continents (Dai et al., 2019; Gill et al., 2021).
USA300 isolates harbor the SCCmec type IVa element, PVL-positive, with the arginine catabolic mobile element (ACME). These factors are lacking in USA500 isolates. A phylogenomic study provided some insights on the origin and the features for the spread of ST8-MRSA in Africa (Strauß et al., 2017). First, the heterogeneity of SCCmec types suggests the different introduction of these genetic elements to the ST8 genetic background. Secondly, African USA300 isolates formed a monophyletic group within the North American Epidemic (NAE) USA300 clade. This observation suggests a single introduction episode of this clone to the African continent followed by an extensive spread in the population (Strauß et al., 2017). However, it should be noted that the African USA300 isolates analyzed in the investigation were PVL-positive, unlike most of the MRSA (PVL-negative) identified in our study (Supplementary Table 3). Also, a phylogenetic analysis of t1476-ST8-IV-MRSA isolates (PVL, ACME-negative) from HIV-infected patients in Tanzania (Manyahi et al., 2021) revealed that they were unrelated to NAE USA300 and the African USA300 previously described in Gabon and East Africa. We hypothesize that t1476-ST8-MRSA from Tanzania, Angola, DRC, and Kenya (Supplementary Table 3) may have acquired different SCCmec elements despite sharing common genetic characteristics. Further studies are needed to unravel the origin and nature of CC8-MRSA in Africa.
CC88-MRSA is regarded as an “African” clone due to its wide distribution in West, Central, and East Africa (Schaumburg et al., 2014). It is noteworthy that CC88-MRSA was widely distributed in Portuguese-speaking African countries (Angola, Cape Verde, and São Tomé and Príncipe). The reasons for this observation are unclear. However, we postulate that demographic and cultural relationships could play a significant role in establishing this clone in these African countries. We observed an expansion of CC1-MRSA, CC22-MRSA, and CC152-MRSA in Africa. Unlike the European CC1-MRSA, which is mainly t127-ST1-IV [2A], the African CC1-MRSA (identified in six countries) comprised spa types t127, t465, and t590, and most of them harbored the SCCmec V element. ST22-IV [2B] (CC22), which is tagged epidemic MRSA-15 (EMRSA-15), was previously documented only in South Africa (Abdulgader et al., 2015), but now in six African countries. The CC152 lineage is a successful MSSA clone in Africa that is mainly PVL-positive. CC152-MRSA was previously noted in Nigeria (Abdulgader et al., 2015), but it is now described in four countries. The increasing trend of CC152-MRSA with diverse spa types and SCCmec elements in Africa is also noteworthy. This observation supports the evidence of multiple introductions among MSSA isolates in sub-Saharan Africa as the basis for the evolution of this clone (Baig et al., 2020). Recent studies have also reported CC152-MRSA from humans (Egyir et al., 2020, 2021) and animals (Shittu et al., 2021), including fomites (Shittu et al., 2020b) in Africa. The emergence of PVL-positive CC152-MRSA is of public health concern. Hence, there is a need to understand the dynamics for introducing and acquiring the mecA gene by CC152-MSSA isolates in Africa.
ST80-IV [2B] (CC80) was limited to North African countries and ST612-IV [2B] (CC8) in South Africa, as described previously (Abdulgader et al., 2015). However, MRSA in various STs (ST80, ST728, ST1931, ST2030, ST3247, and ST5440) assigned to CC80 was recently described in environmental samples associated with livestock in South Africa (Ramaite et al., 2021). ST612-IV [2B] has been detected in wound patients in Tanzania (Moremi et al., 2019). Also, it has been described in a poultry farm and workers in South Africa, raising concerns about its spread across the poultry food chain (Amoako et al., 2019). There is still a paucity of data on the molecular epidemiology of MRSA in animals in Africa. Hence, their role in the dissemination of MRSA remains unclear. Nonetheless, we observed diverse clones (ST1, ST5, ST8, ST36, and ST88) with various SCCmec types associated with the hospital and community settings recovered from livestock and their surroundings. Our findings suggest human to animal transmission and adaptation in poultry and food animals, which warrants further investigations. These observations somewhat indicate the changing epidemiological landscape and highlight the need for a “One-Health” approach to understanding MRSA epidemiology in Africa.
Panton–Valentine leukocidin is a pore-forming protein consisting of two sub-units (lukF-PV, lukS-PV) that target human granulocytes, monocytes, and macrophages (Holzinger et al., 2012). It is mainly associated with skin and soft tissue infection (SSTI) (Friesen et al., 2020), and in particular, pyomyositis in developing countries (Shittu et al., 2020a). This study identified PVL-positive MRSA from nine CCs in 10 countries. Africa is regarded as a PVL-endemic region (Schaumburg et al., 2015). The high prevalence (median: 33%) of PVL-positive MRSA, particularly among nasal samples of hospitalized patients and non-hospitalized individuals in Africa (Supplementary Table 3), is of public health concern. Recurrent SSTIs are associated with S. aureus carriers colonized with PVL-positive S. aureus (Rentinck et al., 2021). So far, the burden of PVL-positive S. aureus is not well known despite its high prevalence in Africa. Knowledge on factors that contribute to the high prevalence of PVL in Africa could help unravel the pathogenic role of PVL and develop strategies against PVL-related diseases.
Genomic epidemiology is a powerful tool to provide valuable information on the emergence of high-risk pandemic clones, antibiotic resistance mechanisms, and virulence determinants (Baker et al., 2018). The characterization of MRSA using conventional molecular typing techniques (e.g., spa typing, MLST) describes only a fraction of the entire S. aureus genome (Price et al., 2013). WGS offers a better opportunity to expand our knowledge about clinical and epidemiologic aspects of MRSA infection and colonization, including transmission patterns, evolution, and guide on appropriate interventions (Humphreys and Coleman, 2019). Our data showed that 12 of the 26 studies utilized WGS. However, eight provided sufficient genotyping data. Understanding the epidemiology of MRSA based on WGS is still in its infancy in Africa. Nonetheless, international scientific cooperation efforts support genomic sequencing capacity building on the continent, e.g., the Fleming Fund, SEQAFRICA. It is expected that these initiatives will provide quality genotyping data that will assist in MRSA surveillance in Africa. However, these efforts will require complementary local investment to ensure quality and representative genotyping data and sustainability.
In August 2017, two independent consortia converged to form the StaphNet Africa. This consortium was co-convened by the corresponding author and Dr. Beverly Egyir (Ghana). The first kick-off meeting took place at the Noguchi Memorial Institute for Medical Research, University of Ghana. The conference, sponsored by the Wellcome Trust-Cambridge Centre for Global Health Research, brought together biomedical scientists and physicians with a research focus on S. aureus from 10 African countries (Nigeria, Ghana, Egypt, Gabon, Kenya, Mozambique, South Africa, Uganda, Kenya, and the Gambia), and the United Kingdom. Although the network’s activities have been hampered by funding, one of its resolutions was to provide regular updates on the epidemiology of S. aureus in Africa. This systematic review is an affirmation of this resolution. Also, an African version of the biennial International Symposium on Staphylococci and Staphylococcal Infections (ISSSI), known as the African Symposium on Staphylococci and Staphylococcal Infections (ASSSI), was adopted for implementation. The symposium is to provide a platform for researchers to network and share current research work on S. aureus in Africa. We anticipate that this initiative, with others, will provide periodic data on MRSA surveillance in Africa.
Conclusion
We have provided an update on the clonal diversity of MRSA in Africa in the past 6 years. Nonetheless, there is still a paucity of data as sufficient genotyping data were available in only 16 of 54 (30%) countries. This systematic review did not investigate antibiotic resistance and virulence gene repertoire of the various African MRSA clones and their level of transmissibility. The origin and features underlying the spread of MRSA clones in Africa are not clear. Identifying human-associated lineages in food animals and products provides evidence to adopt a “One-Health” approach to understand the epidemiology of MRSA in Africa. There is a need to develop robust local capacity in genotyping, including WGS technologies, to determine the genetic factors that contribute to the evolution and adaptation of various African MRSA clones. Lastly, an active continent-wide antimicrobial resistance surveillance program and data exchange across One-Health sectors and professionals are required to monitor the clonal dissemination and emergence of new MRSA clones in Africa.
Author Contributions
AS, BE, SA, and AA initiated the update on the diversity of MRSA in Africa. OL retrieved the data from the various databases and was jointly reviewed by AS. AS and OL wrote the initial manuscript. All authors reviewed and agreed on the final version of the manuscript before submission for peer review.
Funding
This study received support from the Deutsche Forschungsgemeinschaft (SCHA 1994/5-1), the Alexander von Humboldt Foundation (“Georg Forster-Forschungsstipendium”), and the Westfälische Wilhelms-Universität Münster Fellowship granted to AS.
Conflict of Interest
The authors declare that the research was conducted in the absence of any commercial or financial relationships that could be construed as a potential conflict of interest.
Publisher’s Note
All claims expressed in this article are solely those of the authors and do not necessarily represent those of their affiliated organizations, or those of the publisher, the editors and the reviewers. Any product that may be evaluated in this article, or claim that may be made by its manufacturer, is not guaranteed or endorsed by the publisher.
Acknowledgments
We appreciate the kind assistance of Akintunde Sallam.
Supplementary Material
The Supplementary Material for this article can be found online at: https://www.frontiersin.org/articles/10.3389/fmicb.2022.860436/full#supplementary-material
Supplementary Table 1 | Search strings used to identify eligible studies available in six electronic databases.
Supplementary Table 2 | Sequence types and corresponding allele profiles used for clustering analysis.
Supplementary Table 3 | Summary of the methicillin-resistant Staphylococcus aureus (MRSA) clones reported in 26 eligible studies.
Footnotes
References
Abd El-Hamid, M., and Bendary, M. (2015). Comparative phenotypic and genotypic discrimination of methicillin resistant and susceptible Staphylococcus aureus in Egypt. Cell. Mol. Biol. 61, 101–112. doi: 10.14715/cmb/2015.61.4.17
Abd El-Hamid, M. I. A., Bendary, M. M., Merwad, A. M. A., Elsohaby, I., Ghaith, D. M., and Alshareef, W. A. (2019). What is behind phylogenetic analysis of hospital-, community- and livestock-associated methicillin-resistant Staphylococcus aureus? Transbound. Emerg. Dis. 66, 1506–1517. doi: 10.1111/tbed.13170
Abdulgader, S., van Rijswijk, A., Whitelaw, A., and Newton-Foot, M. (2020). The association between pathogen factors and clinical outcomes in patients with Staphylococcus aureus bacteraemia in a tertiary hospital, Cape Town. Int. J. Infect. Dis. 91, 111–118. doi: 10.1016/j.ijid.2019.11.032
Abdulgader, S. M., Shittu, A. O., Nicol, M. P., and Kaba, M. (2015). Molecular epidemiology of methicillin-resistant Staphylococcus aureus in Africa: a systematic review. Front. Microbiol. 6:348. doi: 10.3389/fmicb.2015.00348
Abou Shady, H. M. A., Bakr, A. E. A., Hashad, M. E., Alzohairy, M. A., Shady, H. M. A., Bakr, A. E. A., et al. (2015). Staphylococcus aureus nasal carriage among outpatients attending primary health care centers: a comparative study of two cities in Saudi Arabia and Egypt. Braz. J. Infect. Dis. 19, 68–76. doi: 10.1016/j.bjid.2014.09.005
Agabou, A., Ouchenane, Z., Ngba Essebe, C., Khemissi, S., Chehboub, M. T. E., Chehboub, I. B., et al. (2017). Emergence of nasal carriage of ST80 and ST152 PVL+ Staphylococcus aureus isolates from livestock in Algeria. Toxins 9:303. doi: 10.3390/toxins9100303
Ahmed, M. O., Baptiste, K. E., Daw, M. A., Elramalli, A. K., Abouzeed, Y. M., and Petersen, A. (2017). Spa typing and identification of pvl genes of meticillin-resistant Staphylococcus aureus isolated from a Libyan hospital in Tripoli. J. Glob. Antimicrob. Resist. 10, 179–181. doi: 10.1016/j.jgar.2017.06.004
Aires-de-Sousa, M., Rodrigues, S., Conceição, T., and de Lencastre, H. (2018). Evaluation of different screening methodologies for the detection of methicillin-resistant Staphylococcus aureus from environmental surfaces: swabs, gauzes, and polywipes. Microb. Drug Resist. 24, 585–589. doi: 10.1089/mdr.2017.0349
Al-Amery, K., Elhariri, M., Elsayed, A., El-Moghazy, G., Elhelw, R., El-Mahallawy, H., et al. (2019). Vancomycin-resistant Staphylococcus aureus isolated from camel meat and slaughterhouse workers in Egypt. Antimicrob. Resist. Infect. Control 8, 1–8. doi: 10.1186/s13756-019-0585-4
Alli, O. A. T., Ogbolu, D. O., Shittu, A. O., Okorie, A. N., Akinola, J. O., and Daniel, J. B. (2015). Association of virulence genes with mecA gene in Staphylococcus aureus isolates from Tertiary Hospitals in Nigeria. Indian J. Pathol. Microbiol. 58:464. doi: 10.4103/0377-4929.168875
Amoako, D. G., Bester, L. A., Somboro, A. M., Baijnath, S., Govind, C. N., and Essack, S. Y. (2016). Plasmid-mediated resistance and virulence mechanisms in the private health sector in KwaZulu-Natal, South Africa: an investigation of methicillin resistant Staphylococcus aureus (MRSA) clinical isolates collected during a three month period. Int. J. Infect. Dis. 46, 38–41. doi: 10.1016/j.ijid.2016.03.019
Amoako, D. G., Somboro, A. M., Abia, A. L. K., Allam, M., Ismail, A., Bester, L., et al. (2019). Genomic analysis of methicillin-resistant Staphylococcus aureus isolated from poultry and occupational farm workers in umgungundlovu District, South Africa. Sci. Total Environ. 670, 704–716. doi: 10.1016/j.scitotenv.2019.03.110
Asiimwe, B. B., Baldan, R., Trovato, A., and Cirillo, D. M. (2017b). Prevalence and molecular characteristics of Staphylococcus aureus, including methicillin resistant strains, isolated from bulk can milk and raw milk products in pastoral communities of South-West Uganda. BMC Infect. Dis. 17:422. doi: 10.1186/s12879-017-2524-4
Asiimwe, B. B., Baldan, R., Trovato, A., and Cirillo, D. M. (2017a). Molecular epidemiology of panton-valentine leukocidin-positive community-acquired methicillin resistant Staphylococcus aureus isolates in pastoral communities of rural south western Uganda. BMC Infect. Dis. 17:24. doi: 10.1186/s12879-016-2124-8
Ayepola, O. O., Olasupo, N. A., Egwari, L. O., Becker, K., and Schaumburg, F. (2015). Molecular characterization and antimicrobial susceptibility of Staphylococcus aureus isolates from clinical infection and asymptomatic carriers in southwest Nigeria. PLoS One 10:e0137531. doi: 10.1371/journal.pone.0137531
Baig, S., Rhod Larsen, A., Martins Simões, P., Laurent, F., Johannesen, T. B., Lilje, B., et al. (2020). Evolution and population dynamics of clonal complex 152 community-associated methicillin-resistant Staphylococcus aureus. mSphere 5, e226–e220. doi: 10.1128/mSphere.00226-20
Baker, S., Thomson, N., Weill, F.-X., and Holt, K. E. (2018). Genomic insights into the emergence and spread of antimicrobial-resistant bacterial pathogens. Science 360, 733–738. doi: 10.1126/science.aar3777
Bamigboye, B. T., Olowe, O. A., and Taiwo, S. S. (2018). Phenotypic and molecular identification of vancomycin resistance in clinical Staphylococcus aureus Isolates in Osogbo, Nigeria. Eur. J. Microbiol. Immunol. 8:25. doi: 10.1556/1886.2018.00003
Bloomfield, L. E., Coombs, G. W., Tempone, S., and Armstrong, P. K. (2020). Marked increase in community-associated methicillin-resistant Staphylococcus aureus infections, Western Australia, 2004–2018. Epidemiol. Infect. 148:e153. doi: 10.1017/S0950268820000849
Bowers, J. R., Driebe, E. M., Albrecht, V., Mcdougal, L. K., Granade, M., Roe, C. C., et al. (2018). Improved subtyping of Staphylococcus aureus clonal complex 8 strains based on whole-genome phylogenetic analysis. mSphere 3, e00464–17. doi: 10.1128/mSphere.00464-17
Chaalal, W., Chaalal, N., Bourafa, N., Kihal, M., Diene, S. M., and Rolain, J.-M. (2018). Characterization of Staphylococcus aureus isolated from food products in western Algeria. Foodborne Pathog. Dis. 15, 353–360. doi: 10.1089/fpd.2017.2339
Chairat, S., Gharsa, H., Lozano, C., Gómez-Sanz, E., Gómez, P., Zarazaga, M., et al. (2015). Characterization of Staphylococcus aureus from raw meat samples in tunisia: detection of clonal lineage ST398 from the African continent. Foodborne Pathog. Dis. 12, 686–692. doi: 10.1089/fpd.2015.1958
Conceição, T., Coelho, C., de Lencastre, H., and Aires-de-Sousa, M. (2015a). Frequent occurrence of oxacillin-susceptible mecA-positive Staphylococcus aureus (OS-MRSA) strains in two African countries. J. Antimicrob. Chemother. 70, 3200–3204. doi: 10.1093/jac/dkv261
Conceição, T., Coelho, C., Silva, I. S., de Lencastre, H., and Aires-de-Sousa, M. (2015b). Staphylococcus aureus in former Portuguese colonies from Africa and the far east: missing data to help fill the world map. Clin. Microbiol. Infect. 21, 842.e1–842.e10. doi: 10.1016/j.cmi.2015.05.010
Dai, Y., Liu, J., Guo, W., Meng, H., Huang, Q., He, L., et al. (2019). Decreasing methicillin-resistant Staphylococcus aureus (MRSA) infections is attributable to the disappearance of predominant MRSA ST239 clones, Shanghai, 2008–2017. Emerg. Microbes Infect. 8, 471–478. doi: 10.1080/22221751.2019.1595161
Dekker, D., Wolters, M., Mertens, E., Boahen, K. G., Krumkamp, R., Eibach, D., et al. (2016). Antibiotic resistance and clonal diversity of invasive Staphylococcus aureus in the rural Ashanti region. Ghana. BMC Infect. Dis. 16:720. doi: 10.1186/s12879-016-2048-3
Djoudi, F., Benallaoua, S., Aleo, A., Touati, A., Challal, M., Bonura, C., et al. (2014). Descriptive epidemiology of nasal carriage of Staphylococcus aureus and Methicillin-resistant Staphylococcus aureus among patients admitted to two healthcare facilities in Algeria. Microb. Drug Resist. 21, 218–223. doi: 10.1089/mdr.2014.0156
Dweba, C. C., and Zishiri, O. T. (2019). Isolation and molecular identification of virulence, antimicrobial and heavy metal resistance genes in Staphylococcus aureus. Pathogens 8, 1–21.
Egyir, B., Bentum, J., Attram, N., Fox, A., Obeng-Nkrumah, N., Appiah-Korang, L., et al. (2021). Whole genome sequencing and antimicrobial resistance of Staphylococcus aureus from surgical site infections in Ghana. Pathogens 10:196. doi: 10.3390/pathogens10020196
Egyir, B., Guardabassi, L., Monecke, S., Addo, K. K., Newman, M. J., and Larsen, A. R. (2015). Methicillin-resistant Staphylococcus aureus strains from Ghana include USA300. J. Glob. Antimicrob. Resist. 3, 26–30. doi: 10.1016/j.jgar.2014.11.006
Egyir, B., Hadjirin, N. F., Gupta, S., Owusu, F., Agbodzi, B., Adogla-Bessa, T., et al. (2020). Whole-genome sequence profiling of antibiotic-resistant Staphylococcus aureus isolates from livestock and farm attendants in Ghana. J. Glob. Antimicrob. Resist. 22, 527–532. doi: 10.1016/j.jgar.2020.03.029
Eibach, D., Nagel, M., Hogan, B., Azuure, C., Krumkamp, R., Dekker, D., et al. (2017). Nasal Carriage of Staphylococcus aureus among children in the Ashanti region of Ghana. PLoS One 12:e0170320. doi: 10.1371/journal.pone.0170320
El-Ashker, M., Gwida, M., Monecke, S., El-Gohary, F., Ehricht, R., Elsayed, M., et al. (2020). Antimicrobial resistance pattern and virulence profile of S. aureus isolated from household cattle and buffalo with mastitis in Egypt. Vet. Microbiol. 240:108535. doi: 10.1016/j.vetmic.2019.108535
El-baz, R., Rizk, D. E., Barwa, R., and Hassan, R. (2017). Virulence characteristics and molecular relatedness of methicillin resistant Staphylococcus aureus harboring different staphylococcal cassette chromosome mec. Microb. Pathog. 113, 385–395. doi: 10.1016/j.micpath.2017.11.021
Elhani, D., Gharsa, H., Kalai, D., Lozano, C., Gómez, P., Boutheina, J., et al. (2015). Clonal lineages detected amongst tetracycline-resistant meticillin-resistant Staphylococcus aureus isolates of a Tunisian hospital, with detection of lineage ST398. J. Med. Microbiol. 64, 623–629. doi: 10.1099/jmm.0.000066
ElSayed, N., Ashour, M., and Amine, A. E. K. (2018). Vancomycin resistance among Staphylococcus aureus isolates in a rural setting, Egypt. Germs 8:134. doi: 10.18683/germs.2018.1140
Enwuru, N. V., Adesida, S. A., Enwuru, C. A., Ghebremedhin, B., Mendie, U. E., and Coker, A. O. (2018). Genetics of bi-component leukocidin and drug resistance in nasal and clinical Staphylococcus aureus in Lagos, Nigeria. Microb. Pathog. 115, 1–7. doi: 10.1016/j.micpath.2017.12.030
Eshetie, S., Tarekegn, F., Moges, F., Amsalu, A., Birhan, W., and Huruy, K. (2016). Methicillin resistant Staphylococcus aureus in Ethiopia: a meta-analysis. BMC Infect. Dis. 16:689. doi: 10.1186/s12879-016-2014-0
Fortuin-de Smidt, M. C., Singh-Moodley, A., Badat, R., Quan, V., Kularatne, R., Nana, T., et al. (2015). Staphylococcus aureus bacteraemia in Gauteng academic hospitals, South Africa. Int. J. Infect. Dis. 30, 41–48. doi: 10.1016/j.ijid.2014.10.011
Founou, L. L., Founou, R. C., Allam, M., Ismail, A., Djoko, C. F., and Essack, S. Y. (2019). Genome analysis of methicillin-resistant Staphylococcus aureus isolated from pigs: detection of the clonal lineage ST398 in Cameroon and South Africa. Zoonoses Public Health 66, 512–525. doi: 10.1111/zph.12586
Friesen, J., Neuber, R., Fuhrmann, J., Kietzmann, H., Wenzel, T., Schaumburg, F., et al. (2020). Panton-valentine leukocidin–positive Staphylococcus aureus in skin and soft tissue infections from primary care patients. Clin. Microbiol. Infect. 26, 1416.e1–1416.e4. doi: 10.1016/j.cmi.2020.06.029
Gill, J. L., Hedge, J., Wilson, D. J., and MacLean, R. C. (2021). Evolutionary processes driving the rise and fall of Staphylococcus aureus ST239, a dominant hybrid Pathogen. mBio 12:e0216821. doi: 10.1128/mBio.02168-21
Guthrie, J. L., Teatero, S., Hirai, S., Fortuna, A., Rosen, D., Mallo, G. V., et al. (2020). Genomic epidemiology of invasive methicillin-resistant Staphylococcus aureus infections among hospitalized individuals in Ontario, Canada. J. Infect. Dis. 222, 2071–2081. doi: 10.1093/infdis/jiaa147
Harzing, A. (2007). Publish or Perish; Harzing Resources. Available online at: https://harzing.com/resources/publish-or-perish (accessed May 4, 2021).
Hogan, B., Rakotozandrindrainy, R., Al-Emran, H., Dekker, D., Hahn, A., Jaeger, A., et al. (2016). Prevalence of nasal colonisation by methicillin-sensitive and methicillin-resistant Staphylococcus aureus among healthcare workers and students in Madagascar. BMC Infect. Dis. 16:420. doi: 10.1186/s12879-016-1733-6
Holzinger, D., Gieldon, L., Mysore, V., Nippe, N., Taxman, D. J., Duncan, J. A., et al. (2012). Staphylococcus aureus Panton-valentine leukocidin induces an inflammatory response in human phagocytes via the NLRP3 inflammasome. J. Leukoc. Biol. 92, 1069–1081. doi: 10.1189/jlb.0112014
Humphreys, H., and Coleman, D. C. (2019). Contribution of whole-genome sequencing to understanding of the epidemiology and control of meticillin-resistant Staphylococcus aureus. J. Hosp. Infect. 102, 189–199. doi: 10.1016/j.jhin.2019.01.025
Kalayu, A. A., Woldetsadik, D. A., Woldeamanuel, Y., Wang, S.-H., Gebreyes, W. A., and Teferi, T. (2020). Burden and antimicrobial resistance of S. aureus in dairy farms in Mekelle, Northern Ethiopia. BMC Vet. Res. 16:20. doi: 10.1186/s12917-020-2235-8
Kateete, D. P., Asiimwe, B. B., Mayanja, R., Mujuni, B., Bwanga, F., Najjuka, C. F., et al. (2019a). Nasopharyngeal carriage, spa types and antibiotic susceptibility profiles of Staphylococcus aureus from healthy children less than 5 years in Eastern Uganda. BMC Infect. Dis. 19:1023. doi: 10.1186/s12879-019-4652-5
Kateete, D. P., Bwanga, F., Seni, J., Mayanja, R., Kigozi, E., Mujuni, B., et al. (2019b). CA-MRSA and HA-MRSA coexist in community and hospital settings in Uganda. Antimicrob. Resist. Infect. Control 8:94. doi: 10.1186/s13756-019-0551-1
Khairalla, A. S., Wasfi, R., and Ashour, H. M. (2017). Carriage frequency, phenotypic, and genotypic characteristics of methicillin-resistant Staphylococcus aureus isolated from dental health-care personnel, patients, and environment. Sci. Rep. 7:7390. doi: 10.1038/s41598-017-07713-8
Khemiri, M., Akrout Alhusain, A., Abbassi, M. S., El Ghaieb, H., Santos Costa, S., Belas, A., et al. (2017). Clonal spread of methicillin-resistant Staphylococcus aureus-t6065-CC5-SCCmecV-agrII in a Libyan hospital. J. Glob. Antimicrob. Resist. 10, 101–105. doi: 10.1016/j.jgar.2017.04.014
Klibi, A., Jouini, A., Gómez, P., Slimene, K., Ceballos, S., Torres, C., et al. (2018). Molecular characterization and clonal diversity of methicillin-resistant and -susceptible Staphylococcus aureus isolates of milk of cows with clinical mastitis in Tunisia. Microb. Drug Resist. 24, 1210–1216. doi: 10.1089/mdr.2017.0278
Kumburu, H. H., Sonda, T., Leekitcharoenphon, P., van Zwetselaar, M., Lukjancenko, O., Alifrangis, M., et al. (2018). Hospital epidemiology of methicillin-resistant Staphylococcus aureus in a tertiary care hospital in Moshi, Tanzania, as determined by whole genome sequencing. Biomed Res. Int. 2018:2087693. doi: 10.1155/2018/2087693
Kyany’a, C., Nyasinga, J., Matano, D., Oundo, V., Wacira, S., Sang, W., et al. (2019). Phenotypic and genotypic characterization of clinical Staphylococcus aureus isolates from Kenya. BMC Microbiol. 19:245. doi: 10.1186/s12866-019-1597-1
Lebughe, M., Phaku, P., Niemann, S., Mumba, D., Peters, G., Muyembe-Tamfum, J.-J., et al. (2017). The Impact of the Staphylococcus aureus virulome on infection in a developing country: a cohort study. Front. Microbiol. 8:1662. doi: 10.3389/fmicb.2017.01662
Lee, A. S., de Lencastre, H., Garau, J., Kluytmans, J., Malhotra-Kumar, S., Peschel, A., et al. (2018). Methicillin-resistant Staphylococcus aureus. Nat. Rev. Dis. Primer 4:18033. doi: 10.1038/nrdp.2018.33
Li, S., Sun, S., Yang, C., Chen, H., Yin, Y., Li, H., et al. (2018). The changing pattern of population structure of Staphylococcus aureus from bacteremia in China from 2013 to 2016: ST239-030-MRSA replaced by ST59-t437. Front. Microbiol. 9:332. doi: 10.3389/fmicb.2018.00332
Lozano, C., Gharsa, H., Slama, K. B., Zarazaga, M., and Torres, C. (2016). Staphylococcus aureus in animals and food: methicillin resistance, prevalence and population structure. a review in the African continent. Microorganisms 4:12. doi: 10.3390/microorganisms4010012
Mahomed, G. T., Kock, M. M., Masekela, R., Hoosien, E., and Ehlers, M. M. (2018). Genetic relatedness of Staphylococcus aureus isolates obtained from cystic fibrosis patients at a tertiary academic hospital in Pretoria, South Africa. Sci. Rep. 8:12222. doi: 10.1038/s41598-018-30725-x
Manyahi, J., Moyo, S. J., Aboud, S., Langeland, N., and Blomberg, B. (2021). Predominance of PVL-negative community-associated methicillin-resistant Staphylococcus aureus sequence type 8 in newly diagnosed HIV-infected adults, Tanzania. Eur. J. Clin. Microbiol. Infect. Dis. 40, 1477–1485. doi: 10.1007/s10096-021-04160-2
Masaisa, F., Kayigi, E., Seni, J., Bwanga, F., and Muvunyi, C. M. (2018). Antibiotic resistance patterns and molecular characterization of methicillin-resistant Staphylococcus aureus in clinical settings in Rwanda. Am. J. Trop. Med. Hyg. 99, 1239–1245. doi: 10.4269/ajtmh.17-0554
McGuinness, W. A., Malachowa, N., and DeLeo, F. R. (2017). Vancomycin resistance in Staphylococcus aureus. Yale J. Biol. Med. 90, 269–281.
Mesrati, I., Saidani, M., Jemili, M., Ferjeni, S., Slim, A., and Boubaker, I. B.-B. (2018). Virulence determinants, biofilm production and antimicrobial susceptibility in Staphylococcus aureus causing device-associated infections in a Tunisian hospital. Int. J. Antimicrob. Agents 52, 922–929. doi: 10.1016/j.ijantimicag.2018.05.004
Mohammed, J., Ziwa, M. H., Hounmanou, Y. M. G., Kisanga, A., and Tuntufye, H. N. (2018). Molecular typing and antimicrobial susceptibility of methicillin-resistant Staphylococcus aureus isolated from bovine milk in Tanzania. Int. J. Microbiol. 2018:e4287431. doi: 10.1155/2018/4287431
Moremi, N., Claus, H., Vogel, U., and Mshana, S. E. (2019). The role of patients and healthcare workers Staphylococcus aureus nasal colonization in occurrence of surgical site infection among patients admitted in two centers in Tanzania. Antimicrob. Resist. Infect. Control 8:102. doi: 10.1186/s13756-019-0554-y
Mourabit, N., Arakrak, A., Bakkali, M., and Laglaoui, A. (2017). Nasal carriage of sequence type 22 MRSA and livestock-associated ST398 clones in Tangier, Morocco. J. Infect. Dev. Ctries. 11, 536–542. doi: 10.3855/jidc.9235
Nworie, A., Onyema, A. S., Okekpa, S. I., Elom, M. O., Umoh, N. O., Usanga, V. U., et al. (2017). A novel methicillin-resistant Staphylococcus aureus t11469 and a poultry endemic strain t002 (ST5) are present in chicken in Ebonyi State, Nigeria. Biomed Res. Int. 2017:2936461. doi: 10.1155/2017/2936461
Odetokun, I. A., Ballhausen, B., Adetunji, V. O., Ghali-Mohammed, I., Adelowo, M. T., Adetunji, S. A., et al. (2018). Staphylococcus aureus in two municipal abattoirs in Nigeria: risk perception, spread and public health implications. Vet. Microbiol. 216, 52–59. doi: 10.1016/j.vetmic.2018.01.022
Ogundipe, F. O., Ojo, O. E., Feßler, A. T., Hanke, D., Awoyomi, O. J., Ojo, D. A., et al. (2020). Antimicrobial resistance and virulence of methicillin-resistant Staphylococcus aureus from human, chicken and environmental samples within live bird markets in three Nigerian cities. Antibiotics 9:588. doi: 10.3390/antibiotics9090588
Okomo, U., Akpalu, E. N. K., Doare, K. L., Roca, A., Cousens, S., Jarde, A., et al. (2019). Aetiology of invasive bacterial infection and antimicrobial resistance in neonates in sub-Saharan Africa: a systematic review and meta-analysis in line with the STROBE-NI reporting guidelines. Lancet Infect. Dis. 19, 1219–1234. doi: 10.1016/S1473-3099(19)30414-1
Okuda, K. V., Toepfner, N., Alabi, A. S., Arnold, B., Bélard, S., Falke, U., et al. (2016). Molecular epidemiology of Staphylococcus aureus from Lambaréné, Gabon. Eur. J. Clin. Microbiol. Infect. Dis. 35, 1963–1973. doi: 10.1007/s10096-016-2748-z
Omuse, G., Van Zyl, K. N., Hoek, K., Abdulgader, S., Kariuki, S., Whitelaw, A., et al. (2016). Molecular characterization of Staphylococcus aureus isolates from various healthcare institutions in Nairobi, Kenya: a cross sectional study. Ann. Clin. Microbiol. Antimicrob. 15:51. doi: 10.1186/s12941-016-0171-z
Onwugamba, F. C., Mellmann, A., Nwaugo, V. O., Süselbeck, B., and Schaumburg, F. (2020). Antimicrobial resistant and enteropathogenic bacteria in ‘filth flies’: a cross-sectional study from Nigeria. Sci. Rep. 10:16990. doi: 10.1038/s41598-020-74112-x
Oreiby, A, Khalifa, H., Eid, A., Ahmed, A., Shimamoto, T., and Shimamoto, T. (2019). Staphylococcus aureus and bovine mastitis: molecular typing of methicillin resistance and clinical description of infected quarters. J. Hell. Vet. Med. Soc. 70, 1511–1516. doi: 10.12681/jhvms.20956
Otalu, O. J., Kwaga, J. K. P., Okolocha, E. C., Islam, M. Z., and Moodley, A. (2018). High Genetic Similarity of MRSA ST88 isolated from pigs and humans in Kogi State, Nigeria. Front. Microbiol. 9:3098. doi: 10.3389/fmicb.2018.03098
Page, M. J., McKenzie, J. E., Bossuyt, P. M., Boutron, I., Hoffmann, T. C., Mulrow, C. D., et al. (2021). The PRISMA 2020 statement: an updated guideline for reporting systematic reviews. BMJ 372:n71. doi: 10.1136/bmj.n71
Perovic, O., Iyaloo, S., Kularatne, R., Lowman, W., Bosman, N., Wadula, J., et al. (2015). Prevalence and trends of Staphylococcus aureus bacteraemia in hospitalized patients in South Africa, 2010 to 2012: laboratory-based surveillance mapping of antimicrobial resistance and molecular epidemiology. PLoS One 10:e0145429. doi: 10.1371/journal.pone.0145429
Perovic, O., Singh-Moodley, A., Govender, N. P., Kularatne, R., Whitelaw, A., Chibabhai, V., et al. (2017). A small proportion of community-associated methicillin-resistant Staphylococcus aureus bacteraemia, compared to healthcare-associated cases, in two South African provinces. Eur. J. Clin. Microbiol. Infect. Dis. 36, 2519–2532. doi: 10.1007/s10096-017-3096-3
Price, J. R., Didelot, X., Crook, D. W., Llewelyn, M. J., and Paul, J. (2013). Whole genome sequencing in the prevention and control of Staphylococcus aureus infection. J. Hosp. Infect. 83, 14–21. doi: 10.1016/j.jhin.2012.10.003
Ramaite, K., Ekwanzala, M. D., Dewar, J. B., and Momba, M. N. B. (2021). Human-associated methicillin-resistant Staphylococcus aureus clonal complex 80 isolated from cattle and aquatic environments. Antibiotics 10:1038. doi: 10.3390/antibiotics10091038
Rentinck, M.-N., Krüger, R., Hoppe, P.-A., Humme, D., Niebank, M., Pokrywka, A., et al. (2021). Skin infections due to Panton-Valentine leukocidin (PVL)-producing S. aureus—cost effectiveness of outpatient treatment. PLoS One 16:e0253633. doi: 10.1371/journal.pone.0253633
Robinson, D. A., and Enright, M. C. (2004). Evolution of Staphylococcus aureus by large chromosomal replacements. J. Bacteriol. 186, 1060–1064. doi: 10.1128/JB.186.4.1060-1064.2004
Rodrigues, S., Conceição, T., Silva, I. S., de Lencastre, H., and Aires-de-Sousa, M. (2018). Frequent MRSA nasal colonization among hospitalized children and their parents in Angola and São Tomé and Príncipe. J. Hosp. Infect. 100, 344–349. doi: 10.1016/j.jhin.2018.05.015
Samutela, M. T., Kalonda, A., Mwansa, J., Lukwesa-Musyani, C., Mwaba, J., Mumbula, E. M., et al. (2017). Molecular characterisation of methicillin-resistant Staphylococcus aureus (MRSA) isolated at a large referral hospital in Zambia. Pan Afr. Med. J. 26:108. doi: 10.11604/pamj.2017.26.108.10982
Schaumburg, F., Alabi, A. S., Peters, G., and Becker, K. (2014). New epidemiology of Staphylococcus aureus infection in Africa. Clin. Microbiol. Infect. 20, 589–596. doi: 10.1111/1469-0691.12690
Schaumburg, F., Pauly, M., Anoh, E., Mossoun, A., Wiersma, L., Schubert, G., et al. (2015). Staphylococcus aureus complex from animals and humans in three remote African regions. Clin. Microbiol. Infect 21, 345.e1–8. doi: 10.1016/j.cmi.2014.12.001
See, I., Mu, Y., Albrecht, V., Karlsson, M., Dumyati, G., Hardy, D. J., et al. (2020). Trends in incidence of methicillin-resistant Staphylococcus aureus bloodstream infections differ by strain type and healthcare exposure, united states, 2005–2013. Clin. Infect. Dis. 70, 19–25. doi: 10.1093/cid/ciz158
Shehata, M. M. K., Radwan, S. M., and Ali, S. A. M. (2019). Effects of gamma-irradiation on antibiotic resistance and diagnostic molecular markers of methicillin-resistant Staphylococcus aureus in Egyptian cancer patients. Int. J. Radiat. Biol. 95, 1728–1743. doi: 10.1080/09553002.2019.1664785
Shittu, A. O., Mellmann, A., and Schaumburg, F. (2020b). Molecular characterization of Staphylococcus aureus complex from fomites in Nigeria. Infect. Genet. Evol. 85:104504. doi: 10.1016/j.meegid.2020.104504
Shittu, A. O., Deinhardt-Emmer, S., Vas Nunes, J., Niemann, S., Grobusch, M. P., and Schaumburg, F. (2020a). Tropical pyomyositis: an update. Trop. Med. Int. Health 25, 660–665. doi: 10.1111/tmi.13395
Shittu, A. O., Taiwo, F. F., Froböse, N. J., Schwartbeck, B., Niemann, S., Mellmann, A., et al. (2021). Genomic analysis of Staphylococcus aureus from the West African Dwarf (WAD) goat in Nigeria. Antimicrob. Resist. Infect. Control 10:122. doi: 10.1186/s13756-021-00987-8
Singh-Moodley, A., Lowe, M., Mogokotleng, R., and Perovic, O. (2020). Diversity of SCCmec elements and spa types in South African Staphylococcus aureus mecA-positive blood culture isolates. BMC Infect. Dis. 20:816. doi: 10.1186/s12879-020-05547-w
Singh-Moodley, A., Strasheim, W., Mogokotleng, R., Ismail, H., and Perovic, O. (2019). Unconventional SCCmec types and low prevalence of the Panton-valentine leukocidin exotoxin in South African blood culture Staphylococcus aureus surveillance isolates, 2013-2016. PLoS One 14:e0225726. doi: 10.1371/journal.pone.0225726
Soliman, M. S., Soliman, N. S., El-Manakhly, A. R., ElBanna, S. A., Aziz, R. K., and El-Kholy, A. A. (2020). Genomic characterization of methicillin-resistant Staphylococcus aureus (MRSA) by high-throughput sequencing in a tertiary care Hospital. Genes 11:1219. doi: 10.3390/genes11101219
Strauß, L., Stegger, M., Akpaka, P. E., Alabi, A., Breurec, S., Coombs, G., et al. (2017). Origin, evolution, and global transmission of community-acquired Staphylococcus aureus ST8. PNAS 114, 10596–10604. doi: 10.1073/pnas.1702472114
Titouche, Y., Hakem, A., Houali, K., Meheut, T., Vingadassalon, N., Ruiz-Ripa, L., et al. (2019). Emergence of methicillin-resistant Staphylococcus aureus (MRSA) ST8 in raw milk and traditional dairy products in the Tizi Ouzou area of Algeria. J. Dairy Sci. 102, 6876–6884. doi: 10.3168/jds.2018-16208
Titouche, Y., Houali, K., Ruiz-Ripa, L., Vingadassalon, N., Nia, Y., Fatihi, A., et al. (2020). Enterotoxin genes and antimicrobial resistance in Staphylococcus aureus isolated from food products in Algeria. J. Appl. Microbiol. 129, 1043–1052. doi: 10.1111/jam.14665
Turner, N. A., Sharma-Kuinkel, B. K., Maskarinec, S. A., Eichenberger, E. M., Shah, P. P., Carugati, M., et al. (2019). Methicillin-resistant Staphylococcus aureus: an overview of basic and clinical research. Nat. Rev. Microbiol. 17, 203–218. doi: 10.1038/s41579-018-0147-4
Vandendriessche, S., De Boeck, H., Deplano, A., Phoba, M.-F., Lunguya, O., Falay, D., et al. (2017). Characterisation of Staphylococcus aureus isolates from bloodstream infections, Democratic Republic of the Congo. Eur. J. Clin. Microbiol. Infect. Dis. 36, 1163–1171. doi: 10.1007/s10096-017-2904-0
Verdú-Expósito, C., Romanyk, J., Cuadros-González, J., TesfaMariam, A., Copa-Patiño, J. L., Pérez-Serrano, J., et al. (2020). Study of susceptibility to antibiotics and molecular characterization of high virulence Staphylococcus aureus strains isolated from a rural hospital in Ethiopia. PLoS One 15:e0230031. doi: 10.1371/journal.pone.0230031
Wangai, F. K., Masika, M. M., Maritim, M. C., and Seaton, R. A. (2019). Methicillin-resistant Staphylococcus aureus (MRSA) in East Africa: red alert or red herring? BMC Infect. Dis. 19:596. doi: 10.1186/s12879-019-4245-3
WHO (2021). Global Antimicrobial Resistance And Use Surveillance System (GLASS) Report: 2021. Geneva: World Health Organization.
Wolters, M., Frickmann, H., Christner, M., Both, A., Rohde, H., Oppong, K., et al. (2020). Molecular characterization of Staphylococcus aureus isolated from chronic infected wounds in rural Ghana. Microorganisms 8:2052. doi: 10.3390/microorganisms8122052
Keywords: MRSA – methicillin-resistant Staphylococcus aureus, clonal complex (CC), Panton–Valentine leukocidin (PVL), molecular typing, Africa
Citation: Lawal OU, Ayobami O, Abouelfetouh A, Mourabit N, Kaba M, Egyir B, Abdulgader SM and Shittu AO (2022) A 6-Year Update on the Diversity of Methicillin-Resistant Staphylococcus aureus Clones in Africa: A Systematic Review. Front. Microbiol. 13:860436. doi: 10.3389/fmicb.2022.860436
Received: 22 January 2022; Accepted: 15 March 2022;
Published: 03 May 2022.
Edited by:
Jean-Marc Rolain, URMITE CNRS-IRD UMR 6236, FranceReviewed by:
Grainne Brennan, National MRSA Reference Laboratory, St. James’s Hospital, IrelandBen Pascoe, University of Bath, United Kingdom
Copyright © 2022 Lawal, Ayobami, Abouelfetouh, Mourabit, Kaba, Egyir, Abdulgader and Shittu. This is an open-access article distributed under the terms of the Creative Commons Attribution License (CC BY). The use, distribution or reproduction in other forums is permitted, provided the original author(s) and the copyright owner(s) are credited and that the original publication in this journal is cited, in accordance with accepted academic practice. No use, distribution or reproduction is permitted which does not comply with these terms.
*Correspondence: Adebayo Osagie Shittu, YmF5b19zaGl0dHVAeWFob28uY29t
†Present address: Opeyemi Uwangbaoje Lawal, Department of Food Science, Canadian Research Institute for Food Safety (CRIFS), University of Guelph, Guelph, ON, Canada